- 1Departamento de Turismo y Organización Empresarial, Universidad de Ciego de Ávila “Máximo Gómez Báez”, Ciego de Ávila, Cuba
- 2Centro de Estudios Geomáticos, Ambientales y Marinos, Mexico City, Mexico
- 3Marine Chemistry and Geochemistry Department, Woods Hole Oceanographic Institution, Woods Hole, MA, United States
- 4Centro de Investigaciones Marinas, Universidad de La Habana, Habana, Cuba
- 5Instituto de Ciencias del Mar y Limnología, Universidad Nacional Autónoma de México, Mexico City, Mexico
Coral reefs are complex and biodiverse ecosystems that are undergoing significant change. Understanding reef composition and biodiversity at multiple spatial scales is necessary to track both large-scale and more subtle ecosystem changes. The Jardines de la Reina (JR) archipelago, located offshore of the island of Cuba, contains the largest marine protected area (MPA) in the Caribbean Sea but lacks multi-scale studies. In this contribution, we documented the diversity of scleractinian corals, octocorals, algae, and sponges across nested spatial scales spanning four orders of magnitude (101–105 m). In addition, we tested the hypothesis that species diversity followed a gradient along the ca. 200 km of reef tract. Across the archipelago, we examined benthic cover and species diversity within 255 photo-quadrats (25 × 25 cm) at 13 fore reef sites (two sampling locations per site, and 10 photo-quadrats per location). Small-scale (101 m) variability between photo-quadrats characterized the coral reef community structure in JR compared with local- (102 m) and mesoscale (104–105 m) variability. This finding suggests that biological processes (e.g., recruitment, competition) had primacy over hydrodynamics for driving the differences in reef community composition. However, the dominance of algae and low cover and diversity of scleractinian corals suggests the pervasive effects of global change on coral communities despite potential benefits provided by the MPA (e.g., oligotrophy and abundance of herbivores). There was no gradient of benthic community structure along the fore reef tract of JR; instead, a patchy distribution occurred in response to more subtle drivers acting at local scales. Overall, our multi-scale comparison was useful for differentiating the impacts of processes potentially impacting the JR reefs, thus providing important information to understand how reef communities are impacted by different environmental and anthropogenic stressors, and the potential benefits of MPAs.
Introduction
Coral reefs significantly contribute to the biodiversity and biomass of the global ocean (Côté and Knowlton, 2013) and provide vital goods and services to human populations (Hoegh-Guldberg et al., 2008). Nevertheless, coral reefs are exposed to multiple sources of environmental stress (Burke et al., 2004; Wilkinson, 2008) and are one of most vulnerable marine ecosystems on Earth (Roff and Mumby, 2012). The most featured property of coral reefs is their extensive diversity spanning most of the known phyla, although four groups provide most of the three-dimensional structure and play key roles in ecosystem functioning: scleractinian corals, macroalgae, sponges, and octocorals. These four groups also constitute important bioindicators of coral reef condition. There is a large body of literature devoted to the study of diversity patterns of these groups in coral reefs (reviewed by Côté and Knowlton, 2013). However, these patterns should be framed in appropriate temporal and spatial scales in order to be predictive and informative for understanding community ecology (Wiens, 1989).
Diversity may be analyzed using two components that are scale-dependent (Colwell and Coddington, 1994; Anderson et al., 2011): species richness and β-diversity (complementarity sensu Colwell and Coddington, 1994). Examining diversity patterns at multiple spatial scales is a fundamental approach that is used to disentangle the effects of ecological drivers on coral reef communities (Hatcher et al., 1987; Murdoch and Aronson, 1999). In the Caribbean, only one study (Williams et al., 2015a) has analyzed the magnitude of the change of coral community structure associated with different spatial scales, implying a gap in the knowledge. For instance, poorly studied aspects include the spatial scale that most contributes to the change in species richness and the relationship between β-diversity (i.e., the change in species composition) and the geographical distance. Quantifying the amount of variance associated with different spatial scales may also provide insights about the ecological drivers of communities. Hence, spatial scales of biological processes are usually defined by the reef zonation (Hatcher et al., 1987). For instance, large-scale variability may be attributed to oceanographic regime acting at hundreds of kilometers, while small-scale variability may be associated with biological interactions acting at tens of meters such as recruitment of larvae to the reef substrate. Supply side ecology (s.l. the arrival of new individuals to the community) is key for understanding the dynamics that occur over different scales (Lewin, 1986; Underwood and Fairweather, 1989). This is particularly true in coral reefs where the settlement of larvae, propagules, and sporangium plays fundamental roles in the community structure (Hughes et al., 1999).
The Jardines de la Reina (JR) archipelago contains the largest marine protected area (MPA) in the Caribbean Sea (Appeldoorn and Lindeman, 2003). This national park is remarkable at a global scale because there are large and relatively undisturbed populations of large vertebrates (e.g., sharks, grouper and sea turtles) and the reefs are relatively healthy (Perera-Valderrama et al., 2018). However, no explicit multi-scale study has been conducted there, although the information that such studies may provide are of particular concern for coral reef management in a possible scenario of further touristic development in the JR archipelago. Previous research has shown an ecological gradient of abundance and biomass of reef fishes from non-protected to protected zones in the JR archipelago (Pina-Amargós et al., 2014). However, such a gradient is not clear for other important reef groups including the Black sea urchin Diadema antillarum (Martín-Blanco et al., 2010, 2011), corals, and crustose coralline algae (Hernández-Fernández et al., 2018).
The objectives of this study were two fold. First, we sought to document the diversity (species richness and β-diversity) of benthic groups in a coral reef system within nested spatial scales. These scales were: (a) “site” separated from each other by 104–105 m; (b) “sampling location” separated from each other by ca. 102 m and nested within site; and (c) “quadrat” separated from each other by about 101 m. This nested hierarchical design spans four orders of magnitude in terms of spatial scale. Second, we tested the hypothesis of a gradient of diversity along the reef, which in the simplest pattern can take a northwest-southeast direction (i.e., along the reef tract).
Materials and Methods
Study Area
The Jardines de la Reina (JR) archipelago is located in the southeastern region of Cuba, bordering the southern limit of the Gulf of Ana María (Figure 1). The archipelago is formed by a long chain of cays bordered by mangrove forests in the shoreline and coral reef tracts facing the Caribbean Sea and runs from northwest to southeast. Fore reefs in the area are mainly structured in two zones: reef terrace and spur and groove. First, reef terrace zones (∼5–10 m depth) are followed by a gentle slope that ends in a sandy flat (Supplementary Material S1a). Second, the deeper spur and groove formation (15–25 m depth) ends in a reef drop-off. The JR archipelago contains the largest MPA in the Caribbean region (ca. 2000 km2) and has an effective level of enforcement evidenced by the highest fish biomass in the Caribbean region (Perera-Valderrama et al., 2018).
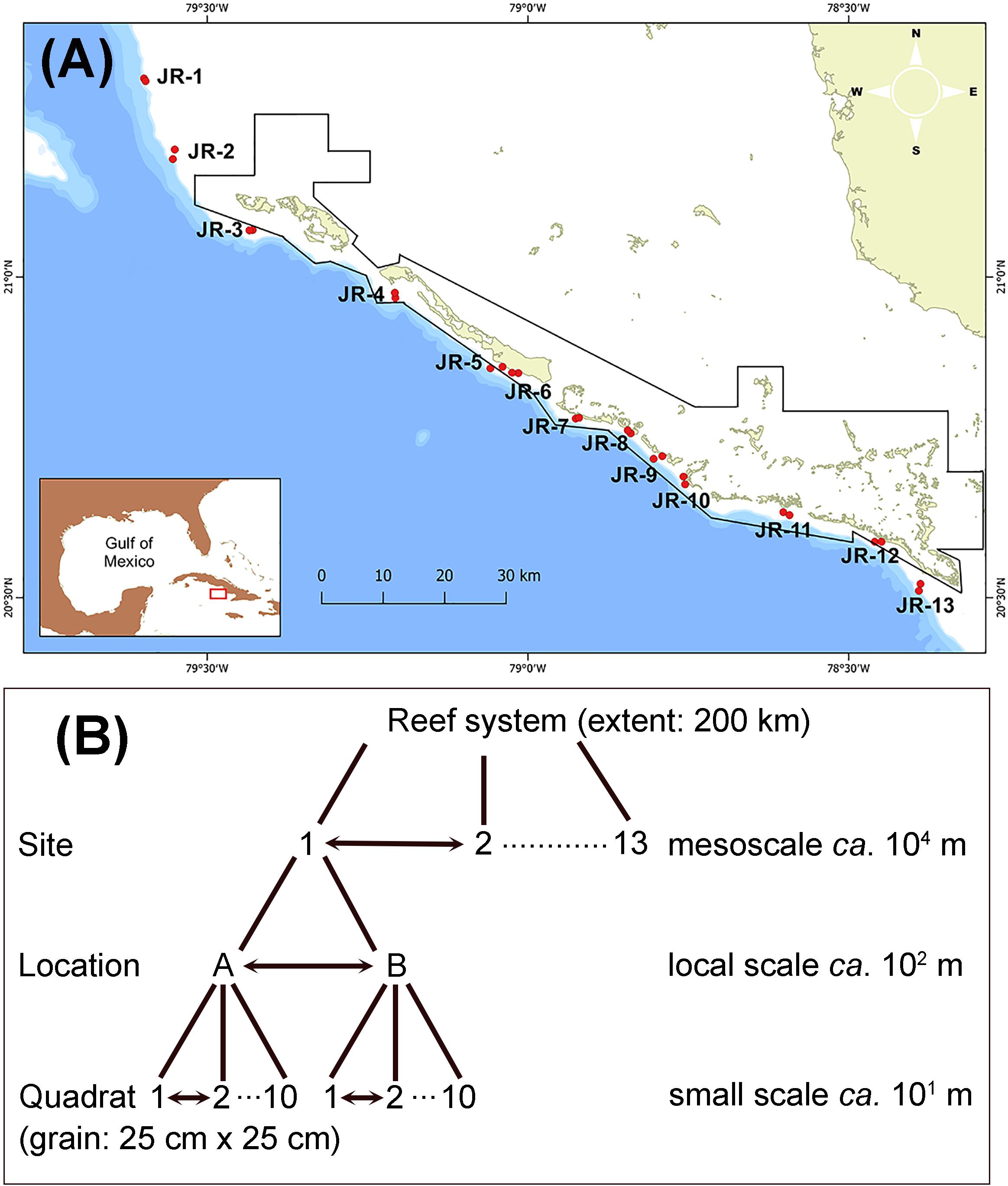
Figure 1. (A) Map of the study zone across the Jardines de la Reina archipelago. Red dots indicate the two sampling locations surveyed at each site (1–13). Black line polygon indicates the limits of the National Park Jardines de La Reina. (B) Scheme of the sampling design showing the hierarchical nested design and the associated spatial scales.
Sampling
A scientific expedition was conducted on the M/V Alucia from November 1–23, 2017 along the entire JR archipelago. Thirteen sampling sites were located approximately equidistant from each other in the terrace zone of the forereef system (Figure 1A and Supplementary Material S2); this Caribbean zone has been also termed as an Orbicella reef (Williams et al., 2015b). The two most distant sites (1 and 13) spanned a distance of ca. 200 km that corresponded to the extent of the study sensu Wiens (1989). The sampling design followed a hierarchical nested design with three levels: site, location, and quadrat (Figure 1B). At each site, two sampling locations (A and B) were located approximately 100–200 m from each other. At each sampling location, 10 replicates were made at random within a diameter of ca. 50 m from a central point; except in three locations (3A, 5B, and 8A) where the safe diving time limited the number of replicates to 7, 9, and 9, respectively. Thus, the total number of replicates was 255. A replicate consisted of a photograph at high resolution of a plastic quadrat of 25 cm × 25 cm laying on the bottom (Supplementary Material S1b), corresponding with the grain of the study sensu Wiens (1989). Usually, several photos were taken on the same quadrat to ensure a good record of the benthic organisms but only one photo was quantitatively analyzed. Photos were taken with an Olympus TG-5 digital camera within an underwater housing (Olympus PT-058).
Image Analysis
Images were computationally reframed to visualize only the area inside the frame. We identified to the lowest possible taxonomic level the following benthic sessile groups: scleractinian corals, macroalgae (hereafter algae), sponges, and octocorals. Scleractinian corals sensu lato included the orders Scleractinia (Class Anthozoa) and Capitata (Class Hydrozoa: genus Millepora) and were identified to species level following González-Ferrer (2004) and Zlatarski and Martínez-Estalella (2018). Macroalgae were identified to genus levels when possible following the taxonomy in Suárez et al. (2015). The identification of sponges to genus/species based on photographs is inaccurate and in consequence we worked with morphological groups (i.e., massive, tubular, branching, crusting, and other) after Alcolado (2002). Octocorals were identified to genus levels when possible following the criteria by Sánchez and Wirshing (2005). The coverage of each species/category was determined using the hierarchical segmentation-based software Seascape 3.2.4 (Teixidó et al., 2011). The software allows for semi-automatic calculation of area cover and the perimeter of each of the individual taxa/categories listed (Supplementary Material S1c).
Measuring of Abiotic Data
Reef-depth was measured with a diving computer and the temperature, dissolved oxygen, salinity, and pH were measured with an Exo1 multiparameter sonde at each sampling location. Measurements were made continuously along the water column and the values were averaged because of the relatively short depth and vertical homogeneity of the water column. Seawater samples were collected from reef-depth at each location using a groundwater pump for analyses of inorganic nutrients, total nitrogen, and chlorophyll a. Water samples (100 ml) collected for analysis of total nitrogen were acidified to a pH of 3 using phosphoric acid and stored at 4°C until they were analyzed using a Shimadzu TOC-VCSH total organic carbon analyzer (Hansell and Carlson, 2001) at the Woods Hole Oceanographic Institution (WHOI). Water (30 ml) samples collected for inorganic nutrient analyses were frozen until they were analyzed at Oregon State University using a continuous segmented flow system (as described in Apprill and Rappé, 2011). Inorganic nitrogen concentrations were obtained by adding the concentrations of nitrate + nitrate and ammonium together for each sample. For quantification of chlorophyll a concentrations across the reefs, four liters of reef-depth seawater were filtered using peristalsis onto a GF/F filter and filters were frozen at −80°C. Chlorophyll a was extracted from the filters using a 90% acetone solution and the concentration was determined by optical density using a calibrated spectrophotometer (Holm-Hansen et al., 1965).
Data Analyses
Data were structured into a matrix of cover of taxa/categories (%) × samples. The variability and asymmetry of the data suggested the use of median and interquartile range (IR) as measures of central position and dispersion, respectively. Two univariate types of community measures were calculated: (1) cover (as proxy of abundance) of the four categories (stony coral, algae, sponges, and octocorals); and (2) species richness (SR) of corals. Observed SR and associated 0.95 confidence intervals were estimated by permutations using the software EstimateS 9.0 (Colwell, 2013) for location and site. Accumulation curves of species richness vs. samples were built per site to estimate the accuracy of the used sample size. The software STATISTICA 7.0 (Statsoft) was used for making descriptive statistics and graphs. Bivariate correlations between biotic and abiotic variables were calculated using the Spearman rank coefficient (RS).
A permutational analysis of variance (PERMANOVA) was used to test for the statistical significance of the factors “site” (13 levels) and “location” (two levels: A and B) nested within site. The components of variance (ECV) were also computed to evaluate the explained variance associated with each factor, and hence the relative importance of the different spatial scales. These tasks were completed using the software PERMANOVA + 1.0.5 (Anderson et al., 2008). Euclidian distance was used as resemblance measure and 9999 permutations were used for the construction of the reference distribution. The multivariate structure of the coral communities was represented using non-metric multidimensional scaling (nMDS) with the software PRIMER 6.1.15 (Clarke and Gorley, 2006). The Bray-Curtis similarity index was used as resemblance measure with 500 starting configurations. Data were transformed as log(x + 1) in order to reduce the contribution of the dominant taxa/categories to the similarity pattern. A vector plot with those species/categories having Spearman rank correlation > 0.5 with the nMDS axes was represented in the ordination.
Results
Abiotic Data
The abiotic variables in the water column were generally homogeneous across the reef system with few exceptions (Supplementary Material S3). Therefore, we only presented the summary values (median ± interquartile range) across the 13 sites. Depth was 14 ± 5 m (range: 9–18 m), temperature was 28.6 ± 0.25°C (range: 28.2–28.8°C), dissolved oxygen was 6.47 ± 0.29 mg L–1 (range: 6.02–6.67 mg L1), salinity was 37.0 ± 0.1 (range: 36.9–37.1), and pH was 8.28 ± 0.038 (range: 8.26–8.33).
Nutrients in reef depth water showed more variability across the reef system (Supplementary Material S4). Phosphate was relatively stable across the reef with a median concentration of 0.2 ± 0.02 μM (range: 0.16–0.38 μM), but there were higher values at some sampling locations. Inorganic nitrogen (NO2– + NO3– + NH4+) steadily increased from site 1 to 13; the median value was 0.51 ± 0.43 μM (range: 0.15–1.98 μM). The specific nitrogen species did not show any trend across the reef system and their average values are given in parentheses: NO2– (0.04 ± 0.02 μM), NH4+ (0.28 ± 0.30 μM) and total N (5.08 ± 3.28 μM). Silicate had a median concentration of 1.42 ± 0.30 μM (range: 0.91–1.89 μM). Chlorophyll a was also relatively stable across the reef system with median concentration of 0.17 ± 0.05 μg l–1 (range: 0.08–1.09 μg l–1).
Some nutrients were significantly, but mildly, correlated with each other (Spearman rank coefficient (Rs), n = 26): Phosphate with nitrite (RS = 0.43) and with silicate (RS = 0.41). Chlorophyll a was correlated with total nitrogen (RS = 0.48) and inversely with coral cover (RS = −0.40).
Cover
The median cover across all sites was 1 ± 4% (range: 0–27%, n = 13) for corals, 30 ± 19% (0–70%) for algae, 1 ± 3% (0–47%) for sponges, and 0 ± 1% (0–22%) for octocorals. The cover of the four groups was highly variable across the sites and between locations at each site (Figures 2A–D). The only spatial trend observed was that octocoral cover was particularly lower at the central part of the reef system (sites 3–7) and higher at the extremes (sites 1, 2, 11–13) (Figure 2D). The results of the PERMANOVA were similar for the four groups (i.e., corals, algae, sponges, and octocorals), indicating that most of the variation occurred among quadrats (i.e., the smallest scale) (Figures 3A–D and Supplementary Material S5). The factor site had significant effect only for algae, octocoral, and multivariate community structure (all groups together).
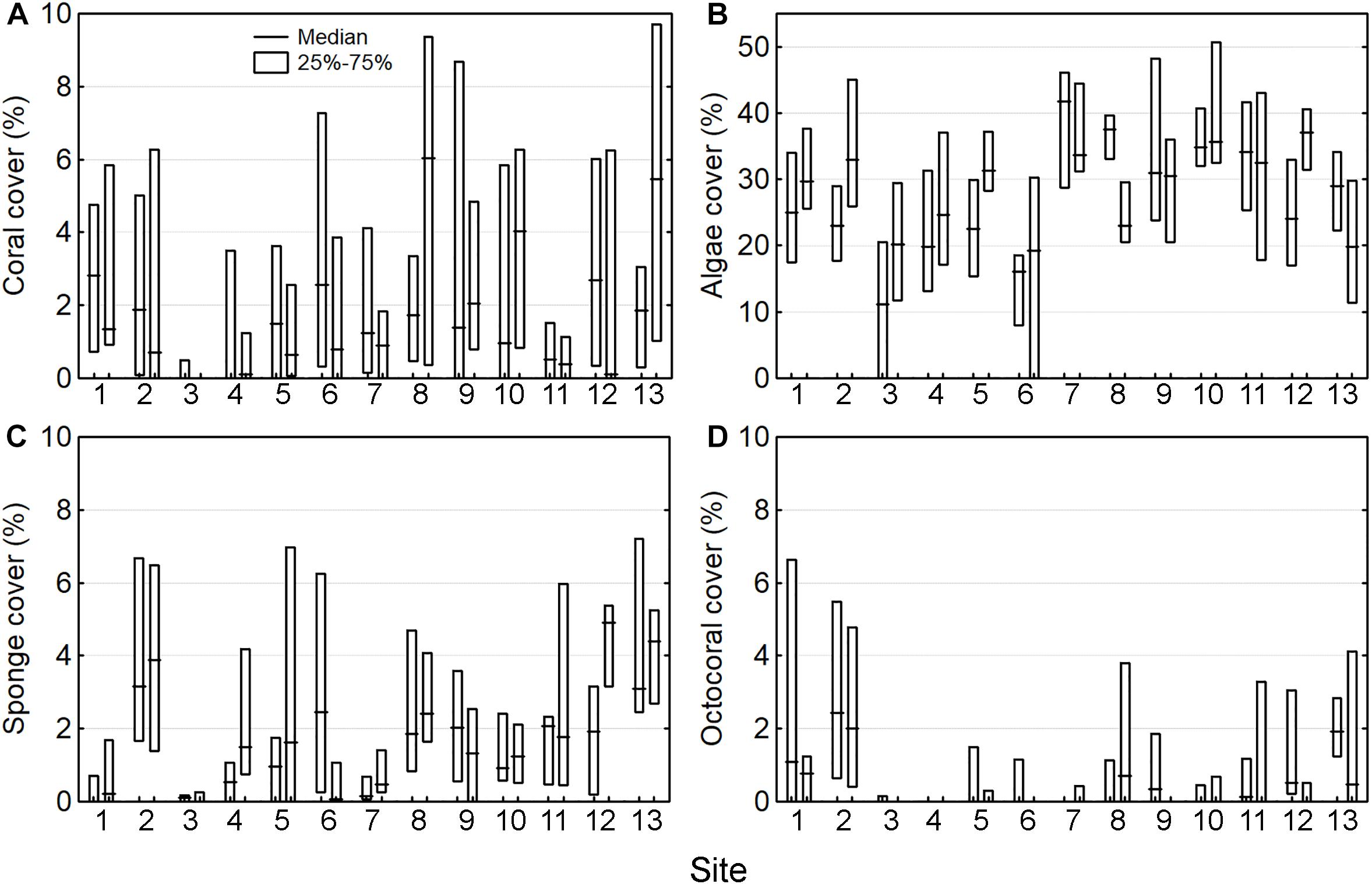
Figure 2. Cover of four benthic groups across 13 sites with two sampling locations per site, including (A) corals, (B) algae, (C) sponges, and (D) octocorals.
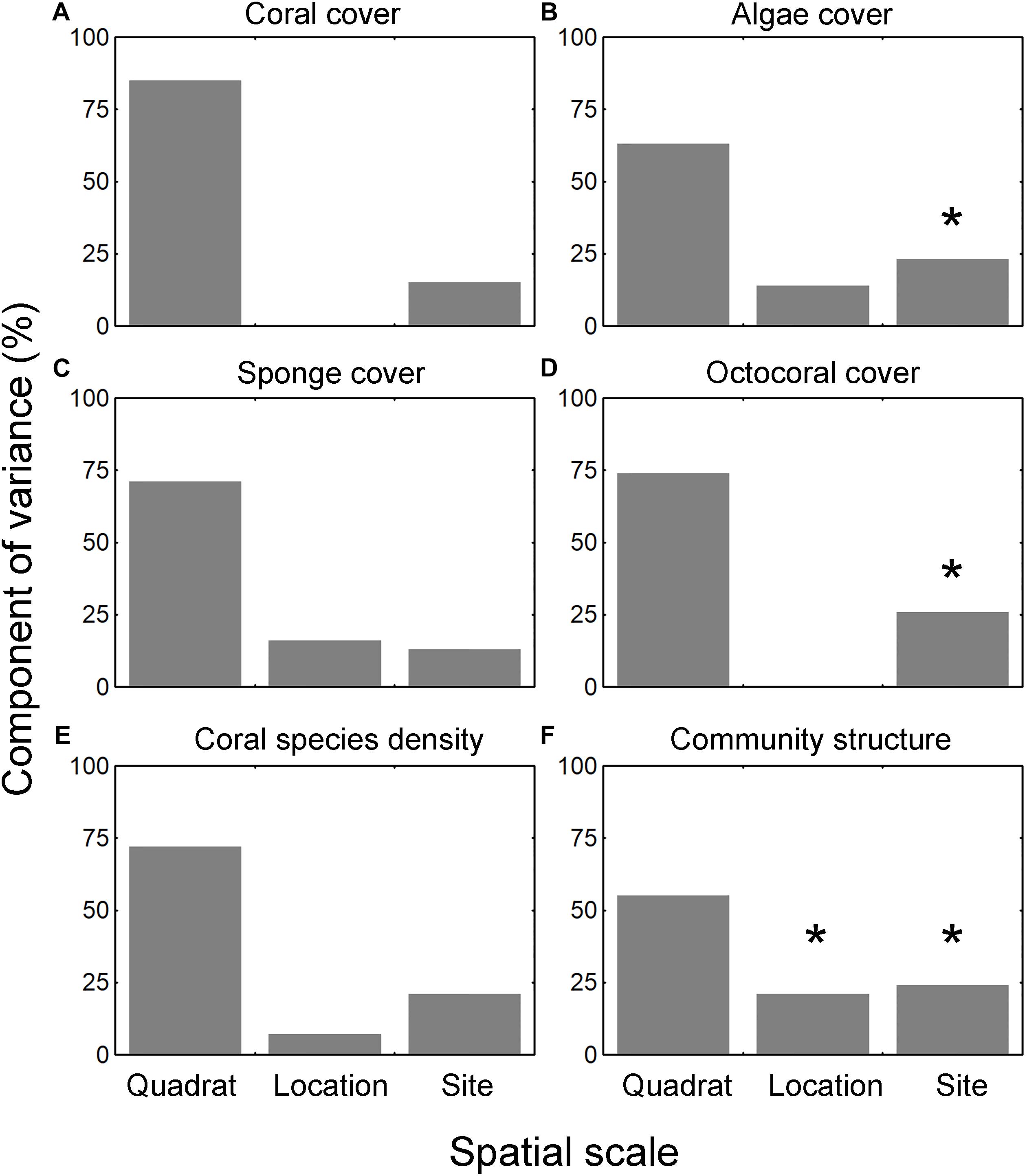
Figure 3. Component of variance (CV) of all the community metrics after spatial scales, including (A) coral cover, (B) algae cover, (C) sponge cover, (D) octocoral cover, (E) coral species richness, and (F) coral species composition. CVs are estimated from the PERMANOVA; the degrees of freedom in the design were 12 for site, 13 for location nested within site, 234 for residual, and 259 for total. ∗Indicates significant effect at p < 0.05.
The cover of the four groups were weakly correlated with each other across the sampling locations, according to Spearman rank correlation, with only sponge and octocoral covers mildly and significantly associated (RS = 0.45, n = 26).
Diversity
Overall, 20 scleractinian coral species were identified. Siderastrea siderea (Ellis and Solander, 1786), Agaricia spp., Porites astreoides Lamarck, 1816, Stephanocoenia intersepta (Esper, 1795), and Orbicella spp. were the most frequently observed. The most frequently observed macroalgae genera were Dictyota, Sargassum, and Halimeda (Supplementary Material S6). Four groups of sponges were recorded, in order of frequency: crusting (67%), massive (23%), tubular (<1%), and branching (<1%); some sponges could not be allocated with certainty to any group (9%). The following genera of octocorals were recorded: Antillogorgia, Plexaura, Pseudoplexaura, and Gorgonia as well as the species Briareum asbestinum (Pallas, 1766).
Scleractinian coral species richness (SR) at the scale of sampling location had a median of 7 ± 2 species (range: 3–10 species). SR was highly variable across the sampling locations (Figure 4A) and broad overlapping of the 0.95 confidence intervals indicated no clear differences among sampling locations. The calculation of the SR at the site scale showed a median of 9 ± 2 species (range: 6–13 species) and a similar pattern with broad overlapping and no differences among sites (Figure 4B).
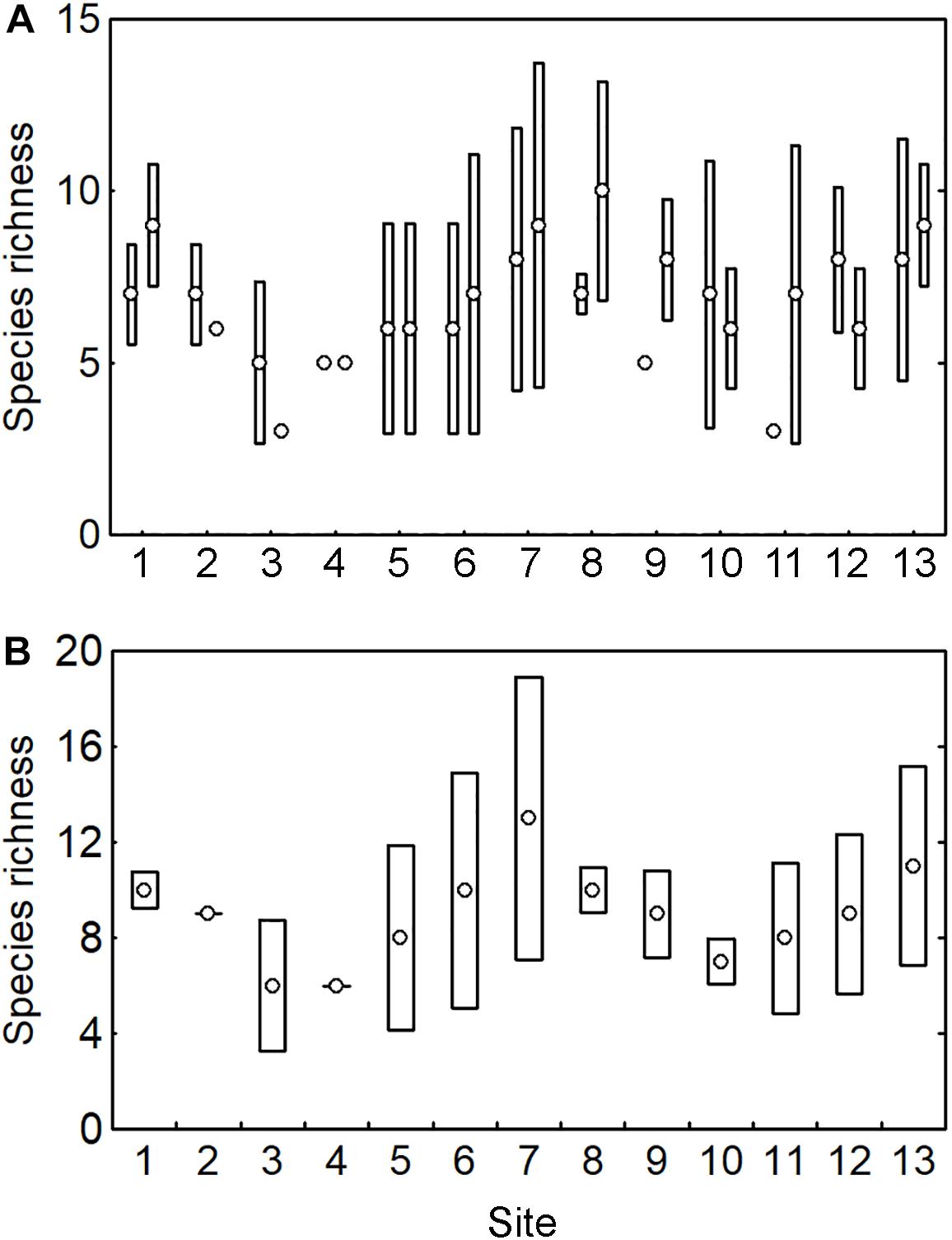
Figure 4. Coral species richness (SR) across 13 sites with two sampling locations per site, including (A) SR at sampling location, and (B) SR at site. Circles indicate the observed SR and bars the 0.95 confidence interval. Those circles without bars indicate that SR was the same in all the pooled samples.
The estimation of species richness implied the sum of all of the quadrats within each sampling location; therefore, it was not possible to assess the component of variance for this metric. Instead, we ran the PERMANOVA over the number of species within each quadrat (i.e., species density). No significant component of variance could be detected for site or location scales, but most of the variation was located at the quadrat scale (Figure 3E and Supplementary Material S5). SR of corals was not significantly correlated with the cover of the four benthic groups or with nutrients.
The multivariate structure of communities (i.e., all groups together) was highly variable across sites and sampling locations. The taxon/category composition varied significantly among sites and locations but most of the variation occurred among quadrats (Figure 3F and Supplementary Material S5). The ordination of the samples suggested a pattern of clustering based on groups of sites separated according to the position in the reef axis: western, central, and eastern sectors (Figure 5A). Sites in the western sector of the reef (1–3) were scattered in the left part of the plot. There was a cluster of sites located in the central sector of the reef (4–10). Two sites in the eastern sector (12–13) were located together, but site 11 was highly variable between locations A and B. There were seven species/categories correlated (RS > 0.5) with the ordination configuration as indicated by the vector plot; but, the different directions of the vectors suggest no clear gradient of cover across the reef system.
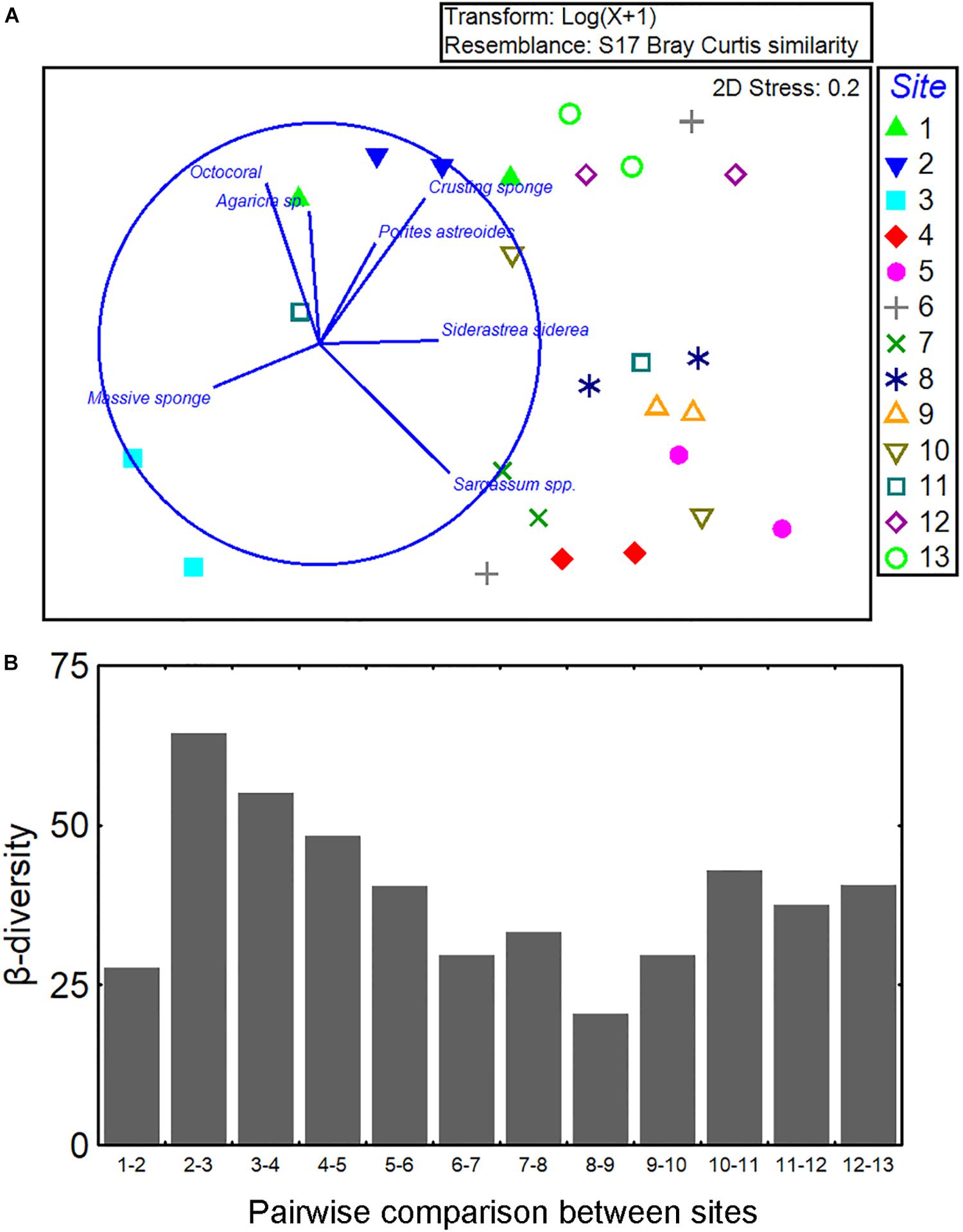
Figure 5. Description of coral species composition across the Jardines de la Reina archipelago. (A) Numerical ordination of the samples by non-metric multidimensional scaling based on transformed [log(x + 1)] data of cover per species/category. Data were averaged by location. Vector plot indicates those species/category having Spearman rank correlation > 0.5 with the ordination configuration, and (B) β-diversity along the reef tract; bars show the pairwise Bray–Curtis dissimilarity index between successive sites (e.g., 1 vs. 2, 2 vs. 3, etc.) in order to focus on the directional change from sites 1 to 13.
We assessed the β-diversity in community structure from sites 1 to 13 (Figure 5B). Calculations of dissimilarity were made between adjacent sites (e.g., 1 vs. 2, 2 vs. 3, and so on). No clear trend in species composition was observed in the graph suggesting the absence of a gradient in β-diversity (Figure 5B).
Discussion
This study constitutes the most extensive published sampling of the coral reef benthic communities coupled with abiotic data that has ever been accomplished in JR. Our extensive and comprehensive study allows us to address three lines of discussion: (i) the spatial scale where most of the variation occurred, (ii) the cover and diversity patterns of the four benthic groups, and (iii) the presence of a gradient in community structure along the reef tract.
The Spatial Scale of Variation
In our study, the spatial patterns of cover and diversity were characterized by greater small-scale variation (101 m) when compared with variation at intermediate (102 m) and meso (104–105 m) scales. It corroborates the results of Williams et al. (2015a), identifying wave exposure and substrate rugosity as the main drivers for coral community structure variability at the local scale in the Caribbean. Most of the processes shaping coral communities at the small scale (10s of meters) are likely related to biological interactions among individuals such as competition, predation, and symbiosis (Connell et al., 1997). Recruitment and settlement are key for determining the diversity of coral communities although they are influenced by larval behavior and chemical clues (Doropoulos et al., 2017) which are not possible to resolve with field-based surveys such as this study. The outcome of competition for resources (e.g., space and light) among corals, algae, and sponges might well be another driver of the community structure which can vary over small spatial scales depending on the heterogeneity of the reef substrate.
On the fore reefs of JR, hydrodynamic processes such as currents and waves likely have a small effect explaining the variability in the coral community (except for octocorals, see below). It does not mean that the hydrodynamic regime is of less importance for the reef community. Rather, the hydrodynamic regime likely impacts the reefs in a similar way throughout the sites because it operates at intermediate to large scales. The hydrodynamic regime in JR is complex due to the interaction of oceanic waters from the Caribbean Sea, the water exchange at the ends of the system (i.e., inflow in the southeast and outflow in the northwest; Arriaza et al., 2008), and tidal exchanges in the channels between cays. However, vigorous horizontal advection and vertical mixing in the water of fore reefs could explain the homogeneity of the water properties (i.e., oxygen, salinity, and nutrients) across the studied sites.
Lastly, it is worthy to devote an analysis of the benefits and consequences of the sampling method. The photo quadrat method introduces a few biases to the analysis of coral community structure (Leujak and Ormond, 2007; Lirman et al., 2007). The size of the quadrat (25 cm × 25 cm) fails to measure the large coral colonies that notably contribute to the living coral cover. Another source of bias is the small area sampled per location (10 quadrats × 0.0625 m2 = 0.6 m2). This area is smaller than the area covered by band transects (squared meters), a method that is used frequently to survey reef composition (Hill and Wilkinson, 2004). One of the solutions to this limitation is to conduct more quadrats (e.g., 20) and increase the size of the quadrat (e.g., 1 m × 1 m). However, time limitations using SCUBA at each site made this approach unfeasible. Despite these limitations, the method using photos bring a superior accuracy in the assessment of relative cover based on a supervised semi-automated method of numerical classification. The used method also provides enhanced reproducibility and taxonomic resolution, when compared with underwater visual census, since images are stored in hard drives and may be analyzed repeatedly (Ohlhorst et al., 1988; Mantelatto et al., 2013). No less important, the coral SR per site was reasonably well estimated as indicated by the asymptotic shape of most of the curves of species accumulation vs. samples (Supplementary Material S7). As our assessments for the variation in benthic reef communities at several scales, as well as the presence of a spatial gradient along the reef tract, were based on internal comparisons among sampling units, we believe the method used in this study is reliable and allows for valid inferences.
Cover and Diversity Patterns
The four most abundant coral species (S. siderea, Agaricia spp., P. astreoides, and S. intersepta) have been previously reported as dominant in JR (Hernández-Fernández et al., 2011, 2018; Ferrer-Rodríguez et al., 2016). S. siderea is a common species in Caribbean reefs and is tolerant to environmental stress caused by low salinity and/or high temperature (Muthiga and Szmant, 1987; Castillo et al., 2014; Banks and Foster, 2017). P. astreoides is also a tolerant species, especially to conditions of high sedimentation and eutrophication (Fabricius et al., 2005). The larger relative abundance of P. astreoides in the western sector of JR is likely because the species is selected due to the high levels of suspended particles transported from soft bottoms in Gulf of Ana Maria.
Agaricia spp., P. astreoides, and S. intersepta have substituted other massive and reef-building species in the Caribbean, such as Orbicella spp. (Green et al., 2008; Jackson et al., 2014; De Bakker et al., 2016) and dominate most of the Cuban coral reefs (González-Díaz et al., 2018; Caballero-Aragón et al., 2019). The dominance of these species likely reflects the response of coral assemblages to the global stressors affecting coral reefs (Bruno et al., 2019). In particular, Agaricia agaricites (Linnaeus, 1758) and Agaricia spp. were strongly affected by bleaching events in JR in 2005 (Hernández-Fernández et al., 2011). However, they were dominant and without symptoms of disease or bleaching (pers. observation) during these surveys, suggesting that JR was unaffected by bleaching in the summer of 2017.
The dominance of algae in JR was high and corroborates previous reports (Pina-Amargós et al., 2006; Martín-Blanco et al., 2011). High levels of algae are associated with degraded coral reefs (Aronson and Precht, 2006; Hughes et al., 2007). Local stressors such as eutrophication and decimation of herbivores have been argued to explain the dominance of algae (and depletion of coral cover) in the Caribbean (Gardner et al., 2003; Williams et al., 2017). The nutrient and chlorophyll data indicate that JR is an oligotrophic system. Even so, the relatively large differences in nutrient concentration between locations A and B (separate 100s m) in some sites of the reef system suggests complex dynamics with some sources of nutrient pulses. The nutrient dynamics in the JR reef system is likely influenced by the presence of several lagoons located in the adjacent cays. The three processes involved in these complex dynamics (González de Zayas et al., 2012) are the production of organic matter by the vegetation (fundamentally mangrove trees), the regeneration of nutrient in the muddy sediments, and tidal export of nutrients and organic matter to the open water though channels.
The genera Dictyota and Halimeda were the two most abundant macroalgae in agreement with other studies in JR (Martín-Blanco et al., 2011), Cuba (Chiappone et al., 2001; Trelles et al., 2001; Blanco-Ojeda et al., 2016) and the Caribbean (Williams and Polunin, 2001; Williams et al., 2001; Szmant, 2002). JR harbors a high biomass of herbivorous fishes such as surgeon and parrot fishes (Pina-Amargós et al., 2014). However, these macroalgae genera bear morphological adaptations to avoid herbivory (Hay, 1997) and herbivorous fishes may not be sufficient to control them.
The dominance of encrusting sponges, over other sponge morphotypes, has been also reported for other Caribbean reefs (e.g., López-Victoria and Zea, 2005) and may be a symptom of coral reef degradation (Aronson et al., 2002). This suggests that competition for resources is an important driver of benthic communities, since encrusting sponges are the most successful competitors of corals and octocorals in the Caribbean (Rutzler, 2002; Weil, 2002). Even more, encrusting clionid and other sponges may dramatically change the community structure and physical stability of shallow reefs that are readily compromised by natural or anthropogenic pressures (Rutzler, 2002). However, sponges also bring benefits to the reef communities by filtering water and thus increasing water transparency, adhering live coral to the reef framework, and facilitating reef regeneration (Wulff, 2006). The presence of diverse and abundant populations of sponges should also be an important component of JR reef communities.
Antillogorgia americana (Gmelin, 1791) was the most abundant octocoral across the reef system in agreement with Hernández-Fernández et al. (2011). Octocorals were more abundant in both extremes of the reef system likely due to a more intense hydrodynamic regime given by the inflow from the southeast into the Gulf of Ana María and the compensatory outflow in the northwest coming out to the Caribbean Sea. Octocorals are highly sensitive to the hydrodynamic regime with larger exposure and flow speed resulting in more abundant and diverse assemblages (Opresko, 1973; Alcolado, 1981; Moyer et al., 2003). This spatial trend in octocoral cover emerged despite the large variability in hydrodynamic forces at different temporal scales in coral reefs (Hatcher et al., 1987). The diversity of octocorals is generally an indicator of good water quality (Fabricius et al., 2005; Tsounis and Edmunds, 2017) and it was congruent with the oligotrophy of the waters in the JR archipelago.
The overall conditions of coral reef communities in JR pointed to an ongoing phase shift from coral- to algae-dominated reef (Hughes et al., 2007). This sort of phase shift has been reported for the Caribbean region (e.g., Hughes, 1994; Martínez-Rendis et al., 2015) as a result of the synergy of stressors such as eutrophication, overfishing, elevated sea temperatures, and hurricanes. Our data indicated that local stressors (i.e., eutrophication and lack of herbivorous fish due to overfishing), exerted a rather small influence on the JR coral reefs due to the effective enforcement in the MPA. Recreational diving takes place in several areas within JR but no evidence exist of deleterious effects on coral communities (pers. observation; Hernández-Fernández et al., 2016). The relatively long distance between the mainland and JR reefs also reduces the direct input of pollutants and nutrients to the system. Consistently, we detected some weak relationships among total nitrogen, chlorophyll a, and coral cover. The increase of nutrients can potentially fuel microbial and algal communities which may result in the increase of chlorophyll a in the water. As a consequence, these communities can cause deleterious effects on cover and growth rate of corals, but this usually happens when coral communities are subjected to sustained eutrophication further compromising reef resilience (Costa et al., 2002; Bell et al., 2007). In JR, we do not consider that elevated nutrients exert negative effects on coral communities. While there is some small-scale variations in nutrient concentrations, nutrients are relatively low across the JR system, and it is more likely that nutrient limitation may be a stressor for this system, rather than eutrophication.
Hurricanes affect negatively coral reefs (Mumby et al., 2014). JR has suffered the direct impact of two recent large hurricanes (Dennis on July 2005 and Paloma on November 2008). However, most of the effects of the former occurred on the crest reefs and caused the destruction of the Acropora palmata framework with minimal effects on the fore deeper reefs (Pina-Amargós et al., 2008b). No evidence of direct mechanical damage of hurricanes on fore reefs have been reported so far in JR.
Therefore, the most plausible explanation for the ongoing phase shift is the influence of global stressors (namely water warming) (Bruno et al., 2019) which is in line with the increasing sea surface temperature in the Caribbean Sea (Chollett et al., 2012; Chollett and Mumby, 2013). Diseases may have an important role as driver of coral reef communities (Lafferty and Harvell, 2014) and they have been reported as occasional stressors on Cuban reefs (Caballero-Aragón et al., 2019). However, no symptoms of disease were observed during the sampling, which is in contrast to other northern Caribbean reefs during this sampling timeframe (Walton et al., 2018), thus minimizing the potential role of this stressor on coral reef communities in JR.
Is There a Gradient Along the Reef Tract?
Jardines de la Reina is a heterogeneous system based on the number and size of cays, the distance from main land (source of freshwater and sediments), and the exposure to hydrodynamic forces. The evidence of an environmental gradient is conflictive so far and depends on the measured communities and sampled biotopes. For instance, Hernández-Fernández et al. (2019) found differences in the abundances of A. palmata populations between west and east sectors of the reef system. Pina-Amargós et al. (2008a) reported the homogeneity of community structure in fore and crest reefs using an analysis that split the system into zones according to the protection regime (i.e., non-reserve and reserve) and reef configuration (i.e., west-east). González de Zayas et al. (2006) reported homogeneity of major hydrochemical fields (e.g., salinity, temperature, nutrients) in the open waters of JR and largely agrees with our results about abiotic factors.
In our study, the existence of a gradient along the reef tract was elusive because the small-scale variability overcame the variation (e.g., β-diversity) between sites. We integrated three lines of evidence to disentangle the possible existence of a gradient: (i) the decomposition of total variance in sources according the spatial scale; (ii) patterns in species richness and β-diversity along the reef tract; and (iii) the multivariate analysis including the four benthic categories. Based on our evidence, we rejected the hypothesis of a gradient of community structure along the JR reef tract. However, we encourage more studies using a different sampling method that cover larger areas and other groups (e.g., fishes).
The homogenizing influence of oceanic waters on the studied fore reefs likely determined a community structure with lesser variation at site- and location-scales, and larger variation at the small scale between replicate photo-quadrats. Of course, environmental differences among sites occurred affecting community structure. For example, site 3 had different community structure compared to the other sites likely due to the influence of intense water exchange across Breton channel (located between sites 2 and 3) (Arriaza et al., 2008).
In summary, small-scale variability (101 m) characterized the coral community structure in the JR archipelago compared with larger spatial scales (102–105 m). This suggest that biological processes (e.g., recruitment, competition) had primacy over oceanographic processes for driving the differences in the coral reef communities. Dominance of algae and low cover and diversity of stony corals suggest an ongoing phase shift, despite locally favorable conditions (oligotrophy and abundance of herbivorous), likely due to pervasive effects of global change on coral communities. The dominance of opportunistic species (Agaricia spp., P. astreoides, and S. intersepta) over massive and reef-building species (e.g., Orbicella spp.) also points to ongoing negative changes in JR. There is not a gradient of benthic community structure along the fore reef tract of JR; instead, a patchy distribution occurs in response to more subtle drivers acting at the local scale.
Data Availability Statement
All datasets generated for this study are included in the article/Supplementary Material.
Author Contributions
MA and AA contributed to the conception and design of the study. MA, LW, and AA collected the data in the field. LH-F and RG analyzed the images, organized the database, and wrote the first draft of the manuscript. LW and AA performed the laboratory analyses. MA performed the statistical analysis. All authors contributed to the manuscript revision, and read and approved the submitted version. AA provided the funding for the field expedition.
Funding
This work was supported by the Dalio Foundation’s Dalio Ocean Initiative (now “OceanX”).
Conflict of Interest
The authors declare that the research was conducted in the absence of any commercial or financial relationships that could be construed as a potential conflict of interest.
The reviewer SE declared a shared affiliation, with no collaboration, with one of the authors, MA, to the handling Editor at the time of review.
Acknowledgments
We thank the captain and crew of M/V Alucia for their assistance with this project and Melissa Patrician and Rob Munier for logistics support. Thanks to Adrián Martínez for drawing the map, Cynthia Becker for chlorophyll analysis, Krista Longnecker for DON analysis, Joe Jennings for inorganic nutrient analysis, and Hansel Caballero for reviewing the draft of the manuscript. Sampling and collections were made under the permission AN-(90)-2017 granted by the Oficina de Regulación Ambiental y de Seguridad Nuclear, Ministerio de Ciencia, Tecnología y Medioambiente, Cuba. Research was conducted in the Jardines de la Reina in accordance with the requirements of the Republic of Cuba, conducted under permit NV2370 and NV2568 issued by the Ministerio de Relaciones Exteriores (MINREX). This research was also conducted in accordance with a memorandum of understanding between the National Center of Protected Areas (CNAP) of the Ministry of Science, Technology and Environment (CITMA) in Cuba and the Woods Hole Oceanographic Institution in the United States. We acknowledge the two reviewers that helped to improve early versions of the manuscript.
Supplementary Material
The Supplementary Material for this article can be found online at: https://www.frontiersin.org/articles/10.3389/fmars.2019.00747/full#supplementary-material
References
Alcolado, P. M. (1981). Zonación de los gorgonáceos someros de Cuba y su posible uso como indicadores comparativos de tensión hidrodinámica sobre los organismos del bentos. Inf. Cient. Téc. Inst. Oceanol. 187, 1–43.
Anderson, M. J., Crist, T. O., Chase, J. M., Vellend, M., Inouye, B. D., Freestone, A. L., et al. (2011). Navigating the multiple meanings of b diversity: a roadmap for the practicing ecologist. Ecol. Lett. 14, 19–28. doi: 10.1111/j.1461-0248.2010.01552.x
Anderson, M. J., Gorley, R. N., and Clarke, K. R. (2008). PERMANOVA+ for PRIMER: Guide to Software and Statistical Methods. Plymouth: PRIMER-E Ltd.
Appeldoorn, R. S., and Lindeman, K. C. (2003). A Caribbean-wide survey of marine reserves: spatial coverage and attributes of effectiveness. Gulf Caribb. Res. 14, 139–154. doi: 10.18785/gcr.1402.11
Apprill, A., and Rappé, M. S. (2011). Response of the microbial community to coral spawning in lagoon and reef flat environments of Hawaii, USA. Aquat. Microb. Ecol. 62, 251–266. doi: 10.3354/ame01471
Aronson, R. B., and Precht, W. F. (2006). Conservation, precaution, and Caribbean reefs. Coral Reefs 25, 441–450. doi: 10.1007/s00338-006-0122-9
Aronson, R. B., Precht, W. F., Toscano, M. A., and Koltes, K. H. (2002). The 1998 bleaching event and its aftermath on a coral reef in Belize. Mar. Biol. 141, 435–447. doi: 10.1007/s00227-002-0842-5
Arriaza, L., Simanca, J., Rodas, L., Lorenzo, S., Hernández, M., Linares, E. O., et al. (2008). Corrientes marinas estimadas en la plataforma suroriental cubana. Ser. Oceanol. 4, 1–10.
Banks, S., and Foster, K. (2017). Baseline levels of Siderastrea siderea bleaching under normal environmental conditions in Little Cayman. Open J. Mar. Sci. 7, 142–154. doi: 10.4236/ojms.2017.71011
Bell, P. R., Lapointe, B. E., and Elmetri, I. (2007). Reevaluation of ENCORE: support for the eutrophication threshold model for coral reefs. AMBIO 36, 416–424. doi: 10.1579/0044-7447(2007)36%5B416:roesft%5D2.0.co;2
Blanco-Ojeda, J., Campo-Castro, A., Tamayo-Fonseca, J. A., and Viña-Peláez, A. (2016). Macroalgas marinas de la provincia Santiago de Cuba, Cuba. Rev. Cub. Cienc. Biol. 5, 122–129.
Bruno, J. F., Coté, I. M., and Toth, L. T. (2019). Climate change, coral loss, and the curious case of the parrotfish paradigm: why don’t marine protected areas improve reef resilience? Annu. Rev. Mar. Sci. 11, 307–334. doi: 10.1146/annurev-marine-010318-095300
Burke, L., Maidens, J., Greenhalgh, S., Nobles, H., and Kool, J. (2004). Arrecifes en Peligro en el Caribe. Washington, DC: World Resources Institute.
Caballero-Aragón, H., Armenteros, M., Perera-Valderrama, S., Rey-Villiers, N., Cobián Rojas, D., Campos Verdecia, K., et al. (2019). Ecological condition of coral reef assemblages in the Cuban Archipelago. Mar. Biol. Res. 15, 61–73. doi: 10.1080/17451000.2019.1577557
Castillo, K. D., Ries, J. B., Bruno, J. F., and Westfield, I. T. (2014). The reef-building coral Siderastrea siderea exhibits parabolic responses to ocean acidification and warming. Proc. R. Soc. London B Biol. Sci. 281:20141856. doi: 10.1098/rspb.2014.1856
Chiappone, M., Sullivan-Sealey, K., Bustamante, G., and Tschirky, J. (2001). A rapid assessment of coral reef community structure and diversity patterns at naval station Guantánamo Bay, Cuba. Bull. Mar. Sci. 69, 373–394.
Chollett, I., Müller-Karger, F. E., Heron, S. F., Skirving, W., and Mumby, P. J. (2012). Seasonal and spatial heterogeneity of recent sea surface temperature trends in the Caribbean Sea and southeast Gulf of Mexico. Mar. Pollut. Bull. 64, 956–965. doi: 10.1016/j.marpolbul.2012.02.016
Chollett, I., and Mumby, P. J. (2013). Reefs of last resort: locating and assessing thermal refugia in the wider Caribbean. Biol. Conserv. 167, 179–186. doi: 10.1016/j.biocon.2013.08.010
Colwell, R. K. (2013). EstimateS: Statistical Estimation of Species Richness and Shared Species From Samples. Version 9.1.0. Available at: http://purl.oclc.org/estimates (accessed April 15, 2019).
Colwell, R. K., and Coddington, J. A. (1994). Estimating terrestrial biodiversity through extrapolation. Philos. Trans. R. Soc. Lond. B Biol. Sci. 345, 101–118. doi: 10.1098/rstb.1994.0091
Connell, J. H., Hughes, T. P., and Wallace, C. C. (1997). A 30-year study of coral abundance, recruitment, and disturbance at several scales in space and time. Ecol. Monogr. 67, 461–488. doi: 10.1890/0012-9615(1997)067%5B0461:aysoca%5D2.0.co;2
Costa, O. S. Jr., Attrill, M. J., Pedrini, A. G., and De-Paula, J. C. (2002). Spatial and seasonal distribution of seaweeds on coral reefs from Southern Bahia, Brazil. Bot. Mar. 45, 346–355. doi: 10.1515/BOT.2002.035
Côté, I. M., and Knowlton, N. (2013). “Coral reef ecosystems: a decade of discoveries,” in Marine Community Ecology and Conservation, eds M. D. Bertness, J. F. Bruno, B. R. Silliman, and J. J. Stachowicz, (Sunderland, MA: Sinauer Associates), 299–314.
De Bakker, D. M., Meesters, E. H., Bak, R. P. M., Nieuwland, G., and Van Duyl, F. C. (2016). Long term shift in coral communities on shallow to deep reef slopes of Curacao and Bonaire: are there any winners? Front. Mar. Sci. 3:247. doi: 10.3389/fmars.2017.00171
Doropoulos, C., Evensen, N. R., Gómez-Lemos, L. A., and Babcock, R. C. (2017). Density-dependent coral recruitment displays divergent responses during distinct early life-history stages. R. Soc. Open Sci. 4:170082. doi: 10.1098/rsos.170082
Fabricius, K., Death, G., McCook, L., Turak, E., and Williams, D. M. (2005). Changes in algal, coral and ?sh assemblages along water quality gradients on the inshore Great Barrier Reef. Mar. Pollut. Bull. 51, 384–398. doi: 10.1016/j.marpolbul.2004.10.041
Ferrer-Rodríguez, V. M., González-Díaz, S. P., Hernández-Fernández, L., Siciliano, D., Bretos, F., Apprill, A., et al. (2016). Salud de las comunidades de corales en arrecifes de Jardines de la Reina - Golfo de Ana María, región surcentral de Cuba. Rev. Invest. Mar. 36, 34–56.
Gardner, T. A., Cote, I. M., Gill, J. A., Grant, A., and Watkinson, A. R. (2003). Long-term region-wide declines in Caribbean corals. Science 301, 958–960. doi: 10.1126/science.1086050
González de Zayas, R., Lestayo, J. A. G., Merino-Ibarra, M., and Castillo, F. S. S. (2012). Condiciones hidroquímicas recientes de la zona central del golfo de Ana María, Cuba. Rev. Invest. Mar. 32, 9–14.
González de Zayas, R., Zúñiga-Ríos, A., Camejo-Cardoso, O., Batista-Tamayo, L. M., and Cárdenas-Murillo, R. (2006). “Atributos físicos del ecosistema Jardines de la Reina,” in Ecosistemas Costeros: Biodiversidad y Gestión de Recursos Naturales, ed. F. Pina-Amargós, (La Habana: CUJAE), 296–351.
González-Díaz, P., González-Sansón, G., Aguilar-Betancourt, C., Álvarez-Fernández, S., Perera-Pérez, O., Hernández-Fernández, L., et al. (2018). Status of Cuban coral reefs. Bull. Mar. Sci. 94, 229–247. doi: 10.5343/bms.2017.1035
González-Ferrer, S. (2004). Corales Pétreos. Jardines Sumergidos de Cuba. Habana: Editorial Academia.
Green, D. H., Edmunds, P. J., and Carpenter, R. C. (2008). Increasing relative abundance of porites astreoides on Caribbean reefs mediated by an overall decline in coral cover. Mar. Ecol. Progr. Ser. 359, 1–10. doi: 10.3354/meps07454
Hansell, D. A., and Carlson, C. A. (2001). Biogeochemistry of total organic carbon and nitrogen in the Sargasso Sea: control by convective overturn. Deep Sea Res. II 48, 1649–1667. doi: 10.1016/s0967-0645(00)00153-3
Hatcher, B. G., Imberger, J., and Smith, S. V. (1987). Scaling analysis of coral reef systems: an approach to problems of scale. Coral Reefs 5, 171–181. doi: 10.1007/bf00300960
Hay, M. E. (1997). The ecology and evolution of seaweed-herbivore interactions on coral reefs. Coral Reefs 16, S67–S76.
Hernández-Fernández, L., Bustamante, C., Dulce Sotolongo, L. B., Pina, F., and Figueredo, T. (2018). Influencia del gradiente de protección sobre el estado de las comunidades de corales y algas coralinas costrosas en el Parque Nacional Jardines de la Reina, Cuba. Rev. Invest. Mar. 38, 83–99.
Hernández-Fernández, L., González de Zayas, R., Olivera, Y. M., Pina Amargós, F., Bustamante López, C., Dulce Sotolongo, L. B., et al. (2019). Distribution and status of living colonies of Acropora spp. in the reef crests of a protected marine area of the Caribbean (Jardines de la Reina National Park, Cuba). PeerJ 7:e6470. doi: 10.7717/peerj.6470
Hernández-Fernández, L., Guimarais, M., Arias, R., and Clero, L. (2011). Composición de las comunidades de octocorales y corales pétreos y la incidencia del blanqueamiento del 2005 en Jardines de la Reina, Cuba. Rev. Cienc. Mar. Cost. 3, 77–90.
Hernández-Fernández, L., Olivera Espinosa, Y. M., Figueredo-Martín, T., Gómez Fernández, R., Brizuela-Pardo, L., and Pina-Amargós, F. (2016). Incidencia del buceo autónomo y capacidad de carga en sitios de buceo del Parque Nacional Jardines de la Reina, Cuba. Rev. Cienc. Mar. Cost. 8, 9–27.
Hill, J., and Wilkinson, C. (2004). Methods for Ecological Monitoring of Coral Reefs. Townsville: Australian Institute of Marine Science.
Hoegh-Guldberg, O., Mumby, P. J., Hooten, A. J., Steneck, R. S., Greenfield, P., Gomez, E., et al. (2008). Coral reefs under rapid climate change and ocean acidification. Science 318, 1737–1742.
Holm-Hansen, O., Lorenzen, C. J., Holmes, R. W., and Strickland, J. D. (1965). Fluorometric determination of chlorophyll. ICES J. Mar. Sci. 30, 3–15. doi: 10.1093/icesjms/30.1.3
Hughes, T. P. (1994). Catastrophes, phase shifts, and large-scale degradation of a Caribbean coral reef. Science 265, 1547–1551. doi: 10.1126/science.265.5178.1547
Hughes, T. P., Baird, A. H., Dinsdale, E. A., Moltschaniwskyj, N. A., Pratchett, M. S., Tanner, J. E., et al. (1999). Patterns of recruitment and abundance of corals along the Great Barrier Reef. Nature 397, 59–63. doi: 10.1038/16237
Hughes, T. P., Rodrigues, M. J., Bellwood, D. R., Ceccarelli, D., Hoegh-Guldberg, O., McCook, L., et al. (2007). Phase shifts, herbivory, and the resilience of coral reefs to climate change. Curr. Biol. 17, 360–365. doi: 10.1016/j.cub.2006.12.049
Jackson, J., Donovan, M., Cramer, K., and Lam, V. (2014). Status and Trends of Caribbean Coral Reefs: 1970-2012. Gland: Global Coral Reef Monitoring Network, IUCN.
Lafferty, K. D., and Harvell, C. D. (2014). “The role of infectious diseases in marine communities,” in Marine Community Ecology and Conservation, eds M. D. Bertness, J. F. Bruno, B. R. Silliman, and J. J. Stachowicz, (Sunderland, MA: Sinauer), 85–108.
Leujak, W., and Ormond, R. F. G. (2007). Comparative accuracy and efficiency of six coral community survey methods. J. Exp. Mar. Biol. Ecol. 351, 168–187. doi: 10.1016/j.jembe.2007.06.028
Lirman, D., Gracias, N. R., Gintert, B. E., Gleason, A. C. R., Reid, R. P., Negahdaripour, S., et al. (2007). Development and application of a video-mosaic survey technology to document the status of coral reef communities. Environ. Monit. Assess. 125, 59–73. doi: 10.1007/s10661-006-9239-0
López-Victoria, M., and Zea, S. (2005). Current trends of space occupation by encrusting excavation sponges on Colombian coral reefs. Mar. Ecol. 26, 33–41. doi: 10.1111/j.1439-0485.2005.00036.x
Mantelatto, M. C., Fleury, B. G., Menegola, C., and Creed, J. C. (2013). Cost-benefit of different methods for monitoring invasive corals on tropical rocky reefs in the southwest Atlantic. J. Exp. Mar. Biol. Ecol. 449, 129–134. doi: 10.1016/j.jembe.2013.09.009
Martín-Blanco, F., Clero-Alonso, L., González-Sansón, G., and Pina-Amargós, F. (2011). Influence of Diadema antillarum populations (Echinodermata: Diadematidae) on algal community structure in Jardines de la Reina, Cuba. Rev. Biol. Trop. 59, 1149–1163.
Martín-Blanco, F., González-Sansón, G., Pina-Amargós, F., and Clero-Alonso, L. (2010). Abundance, distribution and size structure of Diadema antillarum (Philippi 1845) in South Eastern Cuban coral reefs. Rev. Biol. Trop. 58, 663–676.
Martínez-Rendis, A., Acosta-González, G., Hernández-Stefanoni, J. L., and Arias-González, J. E. (2015). Quantifying the reefscape transformation of a coastal Caribbean coral reef during a phase shift and the associated coastal landscape change. Mar. Ecol. 37, 697–710. doi: 10.1111/maec.12334
Moyer, R. P., Riegl, B., Banks, K., and Dodge, R. E. (2003). Spatial patterns and ecology of benthic communities on a high-latitude South Florida (Broward County, USA) reef system. Coral Reefs 22, 447–464. doi: 10.1007/s00338-003-0334-1
Mumby, P. J., Flower, J., Chollett, I., Box, S. J., Bozec, Y. M., Fitzsimmons, C., et al. (2014). Towards Reefs Resilience and Sustainable Livelihoods: A Handbook for Caribbean Coral Reefs Managers. Exeter: University of Exeter, 172.
Murdoch, T., and Aronson, R. (1999). Scale-dependent spatial variability of coral assemblages along the Florida reef tract. Coral Reef 18, 341–351. doi: 10.1007/s003380050210
Muthiga, N. A., and Szmant, A. M. (1987). The effects of salinity stress on the rates of aerobic respiration and photosynthesis in the hermatypic coral Siderastrea siderea. Biol. Bull. 173, 539–551. doi: 10.2307/1541699
Ohlhorst, S. L., Liddell, W. D., Taylor, R. J., and Taylor, J. M. (1988). “Evaluation of reef census techniques,” in Proceedings of the 6th International Coral Reef Symposium, Vol. 2, (Townsville), 319–324.
Opresko, D. M. (1973). Abundance and distribution of shallow-water gorgonians in the area of Miami, Florida. Bull. Mar. Sci. 23, 535–558.
Perera-Valderrama, S., Hernández-Ávila, A., González-Méndez, J., Moreno-Martínez, O., Cobián-Rojas, D., Ferro-Azcona, H., et al. (2018). Marine protected areas in Cuba. Bull. Mar. Sci. 94, 423–442.
Pina-Amargós, F., Clero-Alonso, L., Martín-Blanco, F., Hernández-Fernández, L., Acosta de la Red, W., Cabreja-Ávila, L., et al. (2006). “Biota marina del ecosistema Jardines de la Reina,” in Ecosistemas Costeros: Biodiversidad y Gestión de Los Recursos Naturales, ed. F. Pina-Amargós, (Havana: CUJAE), 396–449.
Pina-Amargós, F., González-Sansón, G., Martín-Blanco, F., and Valdivia, A. (2014). Evidence for protection of targeted reef fish on the largest marine reserve in the Caribbean. PeerJ 2:e274. doi: 10.7717/peerj.274
Pina-Amargós, F., Hernández-Fernández, L., Clero-Alonso, L., and González-Sansón, G. (2008a). Características de los hábitats coralinos en Jardines de la Reina, Cuba. Rev. Invest. Mar. 29, 225–237.
Pina-Amargós, F., Jiménez, A., Martín, F., Zayas, A., and Acosta de la Red, W. (2008b). “Effects of hurricane Dennis on Jardines de la Reina. Coastal Ecosystems,” in Coastal Ecosystem: Hazards, Management and Rehabilitation, ed. En R.K. Datta, (New Delhi: Centre for Science & Technology of the Non-Aligned and Other Developing Countries), 207–214.
Roff, G., and Mumby, P. J. (2012). Global disparity in the resilience of coral reefs. Trends Ecol. Evol. 27, 404–413. doi: 10.1016/j.tree.2012.04.007
Rutzler, K. (2002). Impact of crustose clionid sponges on Caribbean reef corals. Acta Geol. Hisp. 37, 61–72.
Sánchez, J. A., and Wirshing, H. H. (2005). A field key to the identification of tropical Western Atlantic zooxanthellate Octocorales (Octocoralia: Cnidaria). Caribb. J. Sci. 41, 508–522.
Suárez, A. M., Martínez-Daranas, B., and Alfonso, Y. (2015). Macroalgas Marinas de Cuba. Habana: Editorial UH.
Szmant, A. M. (2002). Nutrient enrichment on coral reefs: is it a major cause of coral reef decline? Estuaries 25, 743–766. doi: 10.1007/bf02804903
Teixidó, N., Albajes-Eizagirre, A., Bolbo, D., Le Hir, E., Demestre, M., Garrabou, J., et al. (2011). Hierarchical segmentation-based software for cover classification analyses of seabed images (Seascape). Mar. Ecol. Prog. Ser. 431, 45–53. doi: 10.3354/meps09127
Trelles, J., Suárez, A. M., and de la Guardia, E. (2001). Macroalgas dominantes en playa herradura plataforma noroccidental de cuba: caulerpales y dictyotales. Rev. Invest. Mar. 22, 1–6.
Tsounis, G., and Edmunds, P. J. (2017). Three decades of coral reef community dynamics in St. John, USVI: a contrast of scleractinians and octocorals. Ecosphere 8:e01646. doi: 10.1002/ecs2.1646
Underwood, A. J., and Fairweather, P. G. (1989). Supply-side ecology and benthic marine assemblages. Trends Ecol. Evol. 4, 16–20. doi: 10.1016/0169-5347(89)90008-6
Walton, C., Hayes, N. K., and Gilliam, D. S. (2018). Impacts of a regional, multi-year, multi-species coral disease outbreak in Southeast Florida. Front. Mar. Sci. 5:323. doi: 10.3389/fmars.2018.00323
Weil, E. (2002). “Sponge-induced coral mortality in the Caribbean. A potential new threat to Caribbean coral reefs,” in Proceedings of the VI Internacional Sponge Conference, eds M. Sara, A. Arillo, and N. della Croce, (Genova), 211–212.
Wilkinson, C. (2008). Status of the Coral Reefs of the World. Townsville: Global Coral Reef Monitoring Network and Rainforest Research Centre.
Williams, I. D., and Polunin, N. V. C. (2001). Large-scale associations between macroalgal cover and grazer biomass on mid-depth reefs in the Caribbean. Coral Reefs 19, 358–366. doi: 10.1007/s003380000121
Williams, I. D., Polunin, N. V. C., and Hendrick, V. J. (2001). Limits to grazing by herbivorous fishes and the impact of low coral cover on macroalgal abundance on a coral reef in Belize. Mar. Ecol. Progr. Ser. 222, 187–196. doi: 10.3354/meps222187
Williams, S. M., Chollett, I., Roff, G., Cortés, J., Dryden, C. S., and Mumby, P. J. (2015a). Hierarchical spatial patterns in Caribbean reef benthic assemblages. J. Biogeogr. 42, 1327–1335. doi: 10.1111/jbi.12509
Williams, S. M., Mumby, P. J., Chollett, I., and Cortés, J. (2015b). Importance of differentiating Orbicella reefs from gorgonian plains for ecological assessments of Caribbean reefs. Mar. Ecol. Progr. Ser. 530, 93–101. doi: 10.3354/meps11333
Williams, S. M., Sánchez-Godínez, C., Newman, S. P., and Cortés, J. (2017). Ecological assessments of the coral reef communities in the Eastern Caribbean and the effects of herbivory in influencing coral juvenile density and algal cover. Mar. Ecol. 38, 1–10. doi: 10.1111/maec.12395
Wulff, J. L. (2006). Rapid diversity and abundance decline in a Caribbean coral reef sponge community. Biol. Conserv. 127, 167–176. doi: 10.1016/j.biocon.2005.08.007
Keywords: coral reef, Caribbean Sea, protected area, species richness, β-diversity, spatial scale
Citation: Hernández-Fernández L, González de Zayas R, Weber L, Apprill A and Armenteros M (2019) Small-Scale Variability Dominates Benthic Coverage and Diversity Across the Jardines de La Reina, Cuba Coral Reef System. Front. Mar. Sci. 6:747. doi: 10.3389/fmars.2019.00747
Received: 26 July 2019; Accepted: 15 November 2019;
Published: 29 November 2019.
Edited by:
Linda Wegley Kelly, San Diego State University, United StatesReviewed by:
Susana Enríquez, National Autonomous University of Mexico, MexicoCaroline Eve Dubé, Centre de Recherches Insulaires et Observatoire de l’Environnement CRIOBE (USR 3278), French Polynesia
Copyright © 2019 Hernández-Fernández, González de Zayas, Weber, Apprill and Armenteros. This is an open-access article distributed under the terms of the Creative Commons Attribution License (CC BY). The use, distribution or reproduction in other forums is permitted, provided the original author(s) and the copyright owner(s) are credited and that the original publication in this journal is cited, in accordance with accepted academic practice. No use, distribution or reproduction is permitted which does not comply with these terms.
*Correspondence: Maickel Armenteros, bWFpY2tlbC5hcm1lbnRlcm9zQGdtYWlsLmNvbQ==