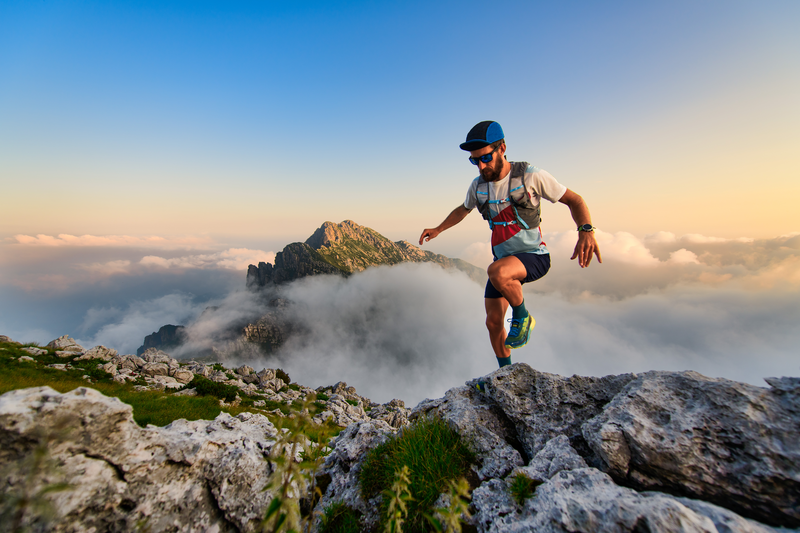
94% of researchers rate our articles as excellent or good
Learn more about the work of our research integrity team to safeguard the quality of each article we publish.
Find out more
PERSPECTIVE article
Front. Mar. Sci. , 10 December 2019
Sec. Ocean Observation
Volume 6 - 2019 | https://doi.org/10.3389/fmars.2019.00727
This article is part of the Research Topic Emerging Technologies with High Impact for Ocean Sciences, Ecosystem Management, and Environmental Conservation View all 17 articles
Coral reefs worldwide are declining at an accelerating rate due to multiple types of human impacts. Meanwhile, new technologies with applications in reef science and conservation are emerging at an ever faster rate and are simultaneously becoming cheaper and more accessible. Technology alone cannot save reefs, but it can potentially help scientists and conservation practitioners study, mitigate, and even solve key challenges facing coral reefs. We examine how new and emerging technologies are already being used for coral reef science and conservation. Examples include drones, autonomous underwater vehicles (AUVs), 3D mapping and modeling tools, high resolution and nano satellite imagery, and a suite of monitoring and surveillance tools that are revolutionizing enforcement of sustainable reef fisheries. We argue that emerging technologies can play a pivotal role in tackling many of the critical issues facing coral reef conservation science and practice, but maximizing the impact of these technologies requires addressing several significant barriers. These barriers include lack of awareness of technologies and tools, prohibitive cost, lack of transferability across systems and/or scales, lack of technical expertise, and lack of accessibility. We discuss where analogous challenges have been overcome in another system and identify insights that can provide guidance for wise application of emerging technologies to coral reef science and conservation. Thoughtful consideration of, and adaptation to, these challenges will help us best harness the potential of emerging and future technological innovations to help solve the global coral reef crisis.
Today, new technologies are emerging at an ever faster rate and are becoming cheaper and more accessible, due in large part to continued, rapid advances in computing power over the last half-century (as predicted by Moore, 1965). Increasingly, conservation biologists and managers seek to harness these new tools to better inform sustainable management of threatened ecosystems. This is especially true for coral reefs, which are historically difficult to study and govern due to their underwater, often-remote, locations and their extremely rich biodiversity. Yet, perhaps no ecosystem is more in need of support: global declines in coral reefs have accelerated in recent decades, with approximately one-third of the world’s largest coral reef system already lost (Hughes et al., 2018b) – a pattern which is likely reflective of many other reef systems globally. The loss of coral reefs puts at risk the many benefits corals provide to society, such as food security and coastal protection for tens of millions of people at least (Moberg and Folke, 1999), and medicinal resources (Bruckner, 2002) for fighting multiple common and life-threatening illnesses, including cancer, HIV, and heart disease.
The future of coral reefs depends upon swift and effective action at local, national, and international levels. Such action requires better science to inform better management–and better science depends on generating and analyzing more data, faster, and across larger scales than ever before. Technology is a critical part of the solution, but deployment of new technology alone is not enough.
Here, we outline some of the ways technological innovation is helping to generate more data and, importantly, note the barriers that are preventing conservation practitioners, scientists, and managers from maximizing application of this technology and implementing solutions at scale. By examining initiatives that currently utilize technological solutions for coral reefs, we also uncover common strategies for success and opportunities for future progress in this space. Our goal is to provide a framework for the coral reef conservation community to guide more effective engagement with technology in order to accelerate conservation and sustainable management of these threatened ecosystems.
Today, coral reef scientists, managers, and conservationists are developing or using a wide range of technologies and associated data analytics to address three pressing problems for coral reef conservation:
1. A dearth of data: over time, space, and at the appropriate resolution to understand threats and evaluate potential mitigation plans;
2. A lack of analytical power: faster and more complex analyses are necessary to handle growing volumes of data generated by new technologies; additional analyses are then needed in order to translate that data to more sophisticated and effective decision-making;
3. Appropriate governance: applying science- and technology-based management in culturally appropriate and effective ways.
To meet these challenges, practitioners are experimenting with a proliferation of both hardware and software innovations, many of which have been topics at key academic and conservation conferences. For example, at the Emerging Technology for Coral Reef Science and Conservation session at the most recent International Coral Reef Symposium (2016), researchers gave talks about applying a diversity of new technologies, such as drones, underwater remotely operated vehicles (ROVs), autonomous underwater vehicles (AUVs), and satellites to gather data on coral reefs. Each of these technologies generate information about reef ecology and health across a diverse range of depths, conditions, and spatial and temporal scales. For example, drones, ROVs, and AUVs can provide very high spatial resolution imagery of localized areas of reef, while satellites can provide a coarser-scale view of far larger areas of reef. Similarly, 3D maps of reefs are allowing new methods of assessing the functional importance of corals (Fontoura et al., in press; Zawada et al., 2019). Collectively, these tools can helps scientists and managers quantify aspects of reef ecosystem health across a wide range of spatial resolutions, depths, and locations. Despite these advantages, each of these tools has limitations, many of which involve trade-offs between spatial and temporal resolution and geographic coverage.
Likewise, advances in sensors and tracking systems are providing more accurate information about the movement of species and dynamic environmental conditions–in some cases, even in real- or near-real-time (Maxwell et al., 2015; Kroodsma et al., 2018). The application of such technology is wide: citizen-scientist surfers are piloting Smartfins to share data on ocean properties via sensors in their surfboard fins; smaller vessel monitoring systems (VMS) units by Pelagic Data Systems are providing new ways to track fishing vessels and, potentially, fishing gear; and new types of acoustic tags and receivers continue to lend insight into fish behavior, habitat use, and effectiveness of different kinds of fisheries management.
Another intersection between technology and marine science is in automation of image capture and processing, as well as development of platforms and algorithms to better analyze the enormous data sets generated by these new technologies. Some examples include underwater camera company View Into the Blue’s self-cleaning, time lapse camera systems to record coral bleaching events over multiple months, developed for the film Chasing Coral; Bayesian clustering models for labeling seafloor imagery from the Australian Centre for Field Robotics (Steinberg et al., 2015); application of facial recognition software to identify fishers’ catch, such as the FishFace program at The Nature Conservancy; algorithms to detect illegal fishing practices based on vessel tracks, such as those developed by Eyes on the Seas and Global Fishing Watch (Figure 1.2; McCauley et al., 2016); and new software by DeepLabCuts that digitally identifies limbs and tracks body movements of animals from within any video.
Figure 1. Matrix of illustrative coral reef science and conservation technology solutions that match innovative strategies to known barriers. From top left to bottom, (1) Google Earth Outreach offers free training and access to their remote sensing platforms to assist with geospatial data analysis; (2) Global Fishing Watch allows managers and scientists to freely monitor illegal fishing activity worldwide; (3) the Wendy Schmidt Ocean Health XPRIZE incentivizes ocean technology solutions from teams of engineers, scientists and innovators in a global competition; (4) represents open space where technology tools should strive to meet this gap; (5) the Coral Trait Database is a growing compilation of freely available coral species data to centralize and standardize previously fragmented scientific information; (6) MERMAID is an open source web application developed by coral reef field biologists and web designers to manage underwater transect data; (7) organizations like Conservation Drones and She Flies provide training in using unmanned aerial vehicles (drones) for ocean monitoring and biodiversity conservation; (8) Open ROV seeks to make telerobotic submarines available at low-cost for affordable underwater exploration; similarly, GoPro cameras and the Liquid Robotics Wave Glider (not shown) are examples of affordable cameras and ocean robots, respectively, to allow scientists to access ocean health information; (9) Planet Labs is a private Earth imaging company that manufactures nano satellites for commercial uses but provides free high resolution imaging data to scientists. All photographs provided by named organizations, with the exceptions of (5) Emily Darling; (6) James Morgan; (7) www.amazon.com.
These developments have helped to advance knowledge of oceans and human impacts to marine ecosystems. However, in the context of globally threatened coral reefs, we argue that the full potential of technology to positively advance conservation has not yet been tapped. To better understand how we can maximize the potential of emerging technologies to benefit coral reef science and conservation, we use qualitative analysis of a suite of existing initiatives that utilize technology to advance science or conservation of coral reefs. First, we looked for common “sticking points,” where practitioners from different projects have encountered similar challenges. Such reoccuring or common problems often point to underlying, systemic barriers that, if resolved, can help open opportunities across a sector. Secondly, we examined aspects of programs and projects that facilitated progress within a project and looked for similarities in these success factors across multiple initiatives. Identifying patterns in successful approaches to problem solving can point to strategies for scaling success more widely.
Figure 1 provides an overview of the three key barriers, as well as three potential strategies for success, that we identified, and notes examples of specific projects or initiatives that are tackling a given barrier with a specific strategy. While not comprehensive, these examples highlight several key barriers preventing more effective use of technology in coral reef science and conservation and provide insight into how we may begin to scale solutions to overcome these core sticking points in the system.
1. Data Without Insight: Several initiatives had the similar challenge of turning newly generated data into insights that could inform understanding or management of coral reefs. These problems include generation of too much data to process in a timely or accurate manner; the challenge of developing algorithms that work at both small and large scales; and a lack of cross-disciplinary training of individuals in both science and technology to accelerate and improve interpretation of data. The result is a condition where more and more data are generated, but without capacity to realize the full potential impact of these data on the management or understanding of the ecosystem.
2. Efforts and Data are Fragmented: A lack of coordination permeates the system. The majority of existing projects are one-off and isolated from one another, and larger platforms for shared learning remain scarce, especially given the rapid pace of technology development and usage. In addition, scientists and conservation practitioners have different and potentially competing priorities for what they need from technology, which stifles progress–particularly as it prevents a coordinated funding strategy to support technology development and data collection across the field.
3. Limited Accessibility to Technology: There are several different, but related, factors that prevent more widespread access to novel tools for coral reef science and conservation. First, although costs have largely come down, innovations remain expensive, especially for developing world practitioners and scientists, preventing many from using existing tools. Second, the rapidly evolving field makes communication about new tools or processes difficult and means many users remain unaware of potentially valuable and useful resources–this limits progress and can lead to wasted resources as individuals work to design a solution that already exists. Even once a tool is widely known, the technological expertise to apply it is often lacking. Finally, infrastructure limitations continue to hamstring use of tools and platforms where reliable electricity, high-speed internet connections, or other key elements to effective deployment of a technological solution may be missing.
1. Build technology expertise: Several technology-based conservation efforts have found success by providing focused training, often for free, to field practitioners who can then apply new technologies directly to the problem at hand (Figures 1.1,1.7). Rather than relying on technology specialists, these programs often work to make their technology accessible to core users through online and in-person courses, online tutorials and other materials, and follow up help when necessary.
2. Democratize data and tools: Like the push for Open Science, this strategy reflects an ethos of more data/tools, in more hands, makes for greater progress. A core tenet is reduction of redundancy, allowing for scientists and managers to capitalize on data and methods generated by one another. Doing so allows them to direct limited resources toward novel analyses or technology design, rather than re-generating data or re-developing methods that have already been created. This principle for success often manifests as open platforms for data sharing, open-source software and hardware, and other models that seek to reduce costs for technology innovation and access (Figures 1.2,1.5,1.8).
3. Develop new models for innovation: New approaches to adapting or harnessing the tech development pipeline, spanning the conception to implementation phases, is key to effectively designing and applying technologies to meet marine science and conservation needs. Currently, several initiatives are experimenting effectively in this space, including as a means to: (a) drive marine science or conservation-focused tech development (Figure 1.3); (b) generate methods to collectively handle existing, disparate scientific data from across the world (Figure 1.6); and (c) re-purpose tech developed for other uses to further conservation science (Figure 1.9). Each is an example of how novel business models or design platforms can accelerate effective application of new technologies for advancing coral reef science and conservation. Importantly, collaboration within this process between tech developers and the scientific and management communities is key (e.g., Box 1).
In many cases, a single project may tackle multiple barriers with one or more strategies. Figure 2 describes one ongoing project’s journey integrating emerging technologies into reef conservation science by highlighting the barriers encountered and the strategies used to overcome these barriers to achieve its goals.
Figure 2. Diagram of one project’s journey integrating emerging technologies into coral reef conservation science encompassing barriers (squares) and strategies (circles) to achieve its goals. The field component of this project took place at Heron Island on Australia’s southern Great Barrier Reef. The aim of the project was to determine the ecological mechanisms responsible for the formation of landscape-scale vegetation patterns surrounding coral reefs known as reef “halos” (Madin et al., 2019). In order to achieve this aim, it was necessary to obtain and process satellite imagery with specific characteristics. Arrows indicate the non-linear progression of the project through various barriers and strategies implemented for overcoming these barriers. Image credits: Barbara Breen/NZ Herald; Tim Calver/TNC; www.reseiwe.com; DigitalGlobe; SpotImage.
In addition to existing initiatives focused on coral reefs, other sectors working to integrate technological innovation with science and conservation can provide critical insights. See Box 1 for a specific example from the forestry realm. Similarly, cross-system, global scale examples of observation and monitoring initiatives that rely on emerging technologies include iNaturalist (Van Horn et al., 2018) and GEO BON (Scholes et al., 2012), which rely upon citizen and professional scientists, respectively. These and other related efforts, while diverse in nature, share the common aim of improving monitoring of the natural world through technology.
BOX 1. Learning from other systems.
What can be learned by coral reef scientists and conservation practitioners from other systems? The Global Forest Watch (GFW) project (Popkin, 2016) provides a recent and ongoing example of how many of these common challenges can be overcome by using the strategies identified in Figure 1. The GFW partnership, originally conceived by the World Resources Institute and partners and made possible by the open source Google Earth Engine platform (Figure 1.1), is “an interactive online forest monitoring and alert system designed to empower people everywhere with the information they need to better manage and conserve forest landscapes” (www.globalforestwatch.org). GFW focuses on the global challenge of deforestation, which–similar to many of the world’s coral reefs–occurs largely in remote, hard-to-access locations around the globe.
Through its online platform, GFW uses cloud based computing to make satellite data of forest cover freely accessible in near-real-time (overcoming lack of accessibility to the tools behind the data) within a single platform (overcoming fragmented data). In the process, it provides instantaneous two way feedback with users, such as sending alerts to changes in users’ local forests and conversely receiving uploaded alerts to the system where users observe changes on the ground (overcoming lack of insights generated from data). Aspects of this system tap into key strategies for overcoming these barriers: GFW is built on new analytical tools and software to generate these data and is (1) designed to allow users to rapidly gain the technical expertise needed to use the system, (2) democratizes global forest imagery and the data it produces (i.e., is open source and free for all users), and (3) is constantly improved by data input from users via local or regional observations. In addition, secondary analytical tools to generate further insight have been built on the base platform, such as GFW Commodities, a tool to address deforestation in commodity supply chains, and GFW Fires, which helps monitor emerging fires, identify potential causes, and analyze impacts of fires on forests and people.
Global Forest Watch offers guidance for coral reef science and conservation in that it provides a real-world example of how the technology community, scientists, conservation practitioners, and local citizens can collectively overcome barriers to applying emerging technology to an insidious conservation challenge. GFW also exemplifies focusing cooperative funding from diverse sources–including governmental, non-governmental, scientific, private philanthropic, and private sector organizations–on a common environmental challenge. Envisioning how the coral reef conservation community might adopt similar approaches could provide a valuable “guiding light” toward embracing emerging technologies and gaining new insights from them.
Effectively engaging with technology can accelerate the conservation and sustainable management of coral reefs. Drones (Chirayath and Earle, 2016; Kiszka et al., 2016), microsatellites (Asner et al., 2017), cloud databases (McCauley et al., 2016), UAV mapping (Williams et al., 2010; Burns et al., 2015), and other remote sensing approaches (Thompson et al., 2017) are just a few examples of the kinds of new tools that can help track ongoing changes in coral reef ecosystems or improve enforcement and management of coral reef areas. The question is: how might we strategically develop and deploy these and other technologies so they can be applied at larger scales and inform greater understanding, monitoring, and management? The barriers and strategies identified here provide insights into how to answer this challenge.
A critical first step is standardization and coordination of data workflows and reporting in order to curate global datasets of coral reef information (sensu historical, ongoing Global Coral Reef Monitoring Network and NOAA Coral Reef Watch Program). These datasets then need to be housed within platforms that allow for free or low cost, equitable, and easy access to diverse users across disciplines and geographies. One example for how to effectively do this exists within the Coral Trait Database (Figure 1.5; Madin et al., 2016), which collates existing information to help identify gaps in knowledge and provide more efficient and effective analyses based on existing information. For example, the Coral Trait Database allows any user with an internet connection to access its global quality-controlled database of peer-reviewed data on coral trait data at a species level (e.g., demographic parameters, geographic ranges, etc.). Access to the database eliminates the need for 1) access to potentially costly peer reviewed literature and b) extremely time-consuming extraction of data from that literature, in the process dramatically speeding up the pace at which insights can be generated from this vast body of knowledge.
Strategies to achieve up-scaling of data collection also include leveraging citizen science projects, such as OpenROV (Figure 1.8)1, where the lay public can engage in data collection in a robust and systematic manner– but success relies on creating the repositories where this information can be organized and accessed. Cloud based data storage and open source tools can help create accessible data infrastructure, but they require trainings and a community of coding scientists. The Coral Reef Science and Cyberinfrastructure Network2 is one model for how to build this capacity. Such efforts reflect a need for continued work to identify and build platforms that can funnel ever-growing information streams into useable, digestible formats. As technology continues to provide an increasing influx of data—from remote sensing, machine learning, and unmanned robotics systems, to name just a few—new platforms will be needed to collate, analyze, and distribute findings in an equitable and timely manner.
Adopting new technologies is risky, and requires time, effort, and training. Nations with greater capacity have more resources to trial and buy-in to new technologies, but amplifying support for developing world practitioners will optimize solution-building in the regions where coral reefs primarily exist. Strategic partnerships between nations with capacity and those with fewer resources can help offset the risk and costs of new technologies, while at the same time creating opportunity for mutually sharing lessons learned. Organizations such as Conservation Drones (Figure 1.7), a worldwide initiative to make and promote the use of low-cost drones, particularly in developing nations, is helping to build capacity and democratize technology use (Koh and Wich, 2012). Design challenge platforms, such as the Ocean Health XPrize (Figure 1.3), could be designed to specifically solicit ideas from more geographically diverse countries. Such injection of resources at early pilot stages would go a long way toward helping resource-strapped regions overcome initial barriers to entry into technological use and development.
New models for collaboration can lead to engagement among multiple stakeholders, such as technology companies, programmers, developers, and conservation scientists. An example of this is the nano satellite company Planet’s (Figure 1.9) staff scientist whose job is to liaise with the scientific and other communities to increase the company’s environmental and humanitarian engagement and impact. Planet’s model has resulted in numerous nascent projects with environmental and societal benefit potential, at least one of which has already resulted in assessments of difficult-to-access coral reef regions undergoing rapid human-induced change (Asner et al., 2017).
Ultimately, innovative funding models are needed to plant the seeds for scalable technologies and collaborations. The Moore Foundation’s Data-Driven Discovery Initiative, the Paul G. Allen Foundation’s investments in technology for conservation, and Google Earth Outreach (Figure 1.1) provide a few examples of existing direct or in-kind funding opportunities that support adoption of technology by conservation and scientific organizations. However, other types of funding support are needed. Accelerators such as Ocean X Labs and Ocean Solutions Incubator, traditionally focused on supporting early stage technology entrepreneurs to tackle general ocean challenges, could be harnessed or adapted to focus on coral reef-specific issues. Likewise, with so many livelihoods and food security dependent on coral health, more creative use of economic development funds is warranted.
With shrinking recovery windows between global bleaching events (Hughes et al., 2018a) and the increasing human footprint of consumption, carbon, and globalization, coral reefs face a grim future. Emerging technologies can help take the pulse of remote, underwater ecosystems to identify and protect the last remaining reefs as well as identify promising outliers, which are withstanding the onslaught and may hold clues for understanding resiliency (Guest et al., 2018). While technologies can collect more and more data, these workflows must be developed collaboratively with stakeholders and a with an explicit vision to strengthen scientific and technological capacities in the world’s coral reef regions. With robust data, meaningful collaborations between scientists, stakeholders and technology developers, and targeted funding to support the scaling up of coordinating technologies, coral reef science and conservation may just be able to keep up in a changing world.
EM led the study. All authors contributed to design of the study and writing of the manuscript.
EM was supported by an Australian Research Council DECRA Fellowship (DE120102614).
The authors declare that the research was conducted in the absence of any commercial or financial relationships that could be construed as a potential conflict of interest.
The reviewer IW declared a past co-authorship with one of the authors, MH, to the handling Editor.
The authors would like to thank Macquarie University’s Genes to Geoscience Research Centre for supporting the workshop that led to the development of the manuscript. In particular, we would like to thank Mark Westoby, Mike Gillings, Mariella Herberstein, and Wade Tozer.
Asner, G. P., Martin, R. E., and Mascaro, J. (2017). Coral reef atoll assessment in the South China Sea using Planet Dove satellites. Remote Sens. Ecol. Conserv. 3, 57–65. doi: 10.1002/rse2.42
Burns, J., Delparte, D., Gates, R., and Takabayashi, M. (2015). Integrating structure-from-motion photogrammetry with geospatial software as a novel technique for quantifying 3D ecological characteristics of coral reefs. PeerJ 3:e1077. doi: 10.7717/peerj.1077
Chirayath, V., and Earle, S. A. (2016). Drones that see through waves – preliminary results from airborne fluid lensing for centimetre-scale aquatic conservation. Aquat. Conserv. Mar. Freshw. Ecosyst. 26, 237–250. doi: 10.1002/aqc.2654
Fontoura, L., Zawada, K., D’agata, S., Baird, A., Álvarez-Noriega, M., Woods, R., et al. (in press). Climate-driven shift in coral morphological structure predicts decline of juvenile reef fishes. Glob. Chang. Biol. doi: 10.1111/gcb.14911
Guest, J. R., Edmunds, P. J., Gates, R. D., Kuffner, I. B., Andersson, A. J., Barnes, B. B., et al. (2018). A framework for identifying and characterising coral reef “oases” against a backdrop of degradation. J. Appl. Ecol. 55, 2865–2875. doi: 10.1111/1365-2664.13179
Hughes, T. P., Anderson, K. D., Connolly, S. R., Heron, S. F., Kerry, J. T., Lough, J. M., et al. (2018a). Spatial and temporal patterns of mass bleaching of corals in the Anthropocene. Science 359, 80–83. doi: 10.1126/science.aan8048
Hughes, T. P., Kerry, J. T., Baird, A. H., Connolly, S. R., Dietzel, A., Eakin, C. M., et al. (2018b). Global warming transforms coral reef assemblages. Nature 556, 492–496. doi: 10.1038/s41586-018-0041-2
Kiszka, J. J., Mourier, J., Gastrich, K., and Heithaus, M. R. (2016). Using unmanned aerial vehicles (UAVs) to investigate shark and ray densities in a shallow coral lagoon. Mar. Ecol. Prog. Ser. 560, 237–242. doi: 10.3354/meps11945
Koh, L. P., and Wich, S. A. (2012). Dawn of drone ecology: low-cost autonomous aerial vehicles for conservation. Trop. Conserv. Sci. 5, 121–132. doi: 10.1177/194008291200500202
Kroodsma, D. A., Mayorga, J., Hochberg, T., Miller, N. A., Boerder, K., Ferretti, F., et al. (2018). Tracking the global footprint of fisheries. Science 908, 904–908. doi: 10.1126/science.aao5646
Madin, E. M. P., Precoda, K., Harborne, A. R., Atwood, T. B., Roelfsema, C., and Luiz, O. J. (2019). Multi-trophic species interactions shape seascape-scale coral reef vegetation patterns. Front. Ecol. Evol. 7:102. doi: 10.3389/fevo.2019.00102
Madin, J. S., Hoogenboom, M. O., Connolly, S. R., Darling, E. S., Falster, D. S., Huang, D., et al. (2016). A trait-based approach to advance coral reef science. Trends Ecol. Evol. 31, 419–428. doi: 10.1016/j.tree.2016.02.012
Maxwell, S. M., Hazen, E. L., Lewison, R. L., Dunn, D. C., Bailey, H., Bograd, S. J., et al. (2015). Dynamic ocean management: defining and conceptualizing real-time management of the ocean. Mar. Policy 58, 42–50. doi: 10.1016/j.marpol.2015.03.014
McCauley, D. J., Woods, P., Sullivan, B., Bergman, B., Jablonicky, C., Roan, A., et al. (2016). Ending hide and seek at sea. Science 351, 1148–1150. doi: 10.1126/science.aad5686
Moberg, F., and Folke, C. (1999). Ecological goods and services of coral reef ecosystems. Ecol. Econ. 29, 215–233. doi: 10.1016/s0921-8009(99)00009-9
Popkin, G. (2016). Satellite alerts track deforestation in real time. Nature 530, 392–393. doi: 10.1038/530392a
Scholes, R. J., Walters, M., Turak, E., Saarenmaa, H., Heip, C. H. R., and Tuama, ÉÓ, et al. (2012). Building a global observing system for biodiversity. Curr. Opin. Environ. Sustainab. 4, 139–146.
Steinberg, D. M., Pizarro, O., and Williams, S. B. (2015). Hierarchical Bayesian models for unsupervised scene understanding. Comput. Vis. Image Understand. 131, 128–144. doi: 10.1109/TPAMI.2009.22
Thompson, D. R., Hochberg, E. J., Asner, G. P., Green, R. O., Knapp, D. E., Gao, B. C., et al. (2017). Airborne mapping of benthic reflectance spectra with Bayesian linear mixtures. Remote Sens. Environ. 200, 18–30. doi: 10.1016/j.rse.2017.07.030
Van Horn, G., Mac Aodha, O., Song, Y., Cui, Y., Sun, C., Shepard, A., et al. (2018). “The iNaturalist species classification and detection dataset,” in Proceedings of the IEEE Conference on Computer Vision and Pattern Recognition, (Salt Lake City, UT: IEEE), 8769–8778.
Williams, S. B., Pizarro, O., Webster, J. M., Beaman, R. J., Mahon, I., Johnson-Roberson, M., et al. (2010). Autonomous underwater vehicle-assisted surveying of drowned reefs on the shelf edge of the Great Barrier Reef. Australia. J. Field Robot. 27, 675–697. doi: 10.1002/rob.20356
Keywords: technology, coral reef, remote sensing, satellites, drones, sensors, citizen science, open source
Citation: Madin EMP, Darling ES and Hardt MJ (2019) Emerging Technologies and Coral Reef Conservation: Opportunities, Challenges, and Moving Forward. Front. Mar. Sci. 6:727. doi: 10.3389/fmars.2019.00727
Received: 14 November 2018; Accepted: 08 November 2019;
Published: 10 December 2019.
Edited by:
Eric Delory, Oceanic Platform of the Canary Islands, SpainReviewed by:
Ivor Douglas Williams, Pacific Islands Fisheries Science Center (NOAA), United StatesCopyright © 2019 Madin, Darling and Hardt. This is an open-access article distributed under the terms of the Creative Commons Attribution License (CC BY). The use, distribution or reproduction in other forums is permitted, provided the original author(s) and the copyright owner(s) are credited and that the original publication in this journal is cited, in accordance with accepted academic practice. No use, distribution or reproduction is permitted which does not comply with these terms.
*Correspondence: Elizabeth M. P. Madin, ZW1hZGluQGhhd2FpaS5lZHU=
†Present address: Elizabeth M. P. Madin, Hawaii Institute of Marine Biology, University of Hawaii, Manoa, HI, United States
Disclaimer: All claims expressed in this article are solely those of the authors and do not necessarily represent those of their affiliated organizations, or those of the publisher, the editors and the reviewers. Any product that may be evaluated in this article or claim that may be made by its manufacturer is not guaranteed or endorsed by the publisher.
Research integrity at Frontiers
Learn more about the work of our research integrity team to safeguard the quality of each article we publish.