- Red Sea Research Center and Computational BioScience Research Center, King Abdullah University of Science and Technology, Thuwal, Saudi Arabia
Characterizations of gelatinous zooplankton communities are necessary for an improved understanding of the ecological and temporal dynamics of such communities and their composing taxa. Yet, studies on gelatinous zooplankton communities are scarce in the Red Sea, which is characterized by extreme temperature, high salinity and oligotrophic conditions. Here, we analyzed the occurrence of gelatinous zooplankton taxa in a time-series of epipelagic samples taken from September 2016 to May 2018 in the central Red Sea to deliver the first complete characterization of gelatinous zooplankton in the Red Sea. General seasonal dynamics were found over the year, where higher gelatinous zooplankton abundances were relate to mostly with lower temperatures, lower salinity and to a lesser extent, with chlorophyll a, cross-shelf and along-shelf Ekman transport. Tunicates and siphonophores presented seasonal patterns, whereby total biovolume values were 103 – 105 higher in winter – early spring than in summer, and numbers > 100 higher in the bloom event of 2017/2018 than in 2016/2017. Ulmaridae (Aurelia sp.) peaked after the main bloom event of siphonophores and tunicates, and dominated total biovolume when present. Porpitidae was consistently present and showed no clear seasonality. Our results suggest that there is a noticeable seasonal trend in gelatinous zooplankton, marked by high occurrences in winter-early spring, very low occurrences over summer, and mostly dominated by Salpidae and Dyphidae. Porpitidae was a dominating group with non-seasonal occurrence, and Ulmaridae was also dominating but with very short and few occurrences. In addition, low abundance and biovolume (max. 8 ind m–3 and max. 103 – 106 mm3 m–3) suggest that oligotrophic conditions may be limiting the productivity of gelatinous zooplankton communities in the Red Sea.
Introduction
Gelatinous zooplankton communities include a diverse range of pelagic cnidarians, ctenophores, and tunicates (Haddock, 2004; Lucas et al., 2014). Populations of these gelatinous taxa are known to bloom, but often oscillate over seasonal and interannual time-scales (Boero et al., 2008; Purcell, 2011). Despite decades of observations on these gelatinous zooplankton populations, only recently has the fundamental role of these communities in pelagic food webs been highlighted (Madin and Harbison, 1977; Hoeksema and Waheed, 2012; Diaz Briz et al., 2017; Hays et al., 2018; Macali et al., 2018). Their importance in the pelagic food web is likely to vary according to their bloom dynamics and seasonal variability in occurrence and community structure (Boero et al., 2008; Fuentes et al., 2018).
Characterizations of gelatinous zooplankton communities are especially scarce in the Red Sea. Reports of gelatinous zooplankton are available from the 1960’s (e.g., Ponomareva, 1966; Halim, 1969), but most observations are focused on offshore waters in the Southern and Northern Red Sea (e.g., Godeaux, 1986; Aberle et al., 2010). Research on Red Sea gelatinous zooplankton communities has been included within broad studies of zooplanktonic communities (e.g., Cornils et al., 2007; Aberle et al., 2010; Zakaria, 2015; Abu El-Regal et al., 2018) or focused on conspicuous gelatinous species and single blooming events (El-Serehy and Al-Rasheid, 2011; Cruz-Rivera and Abu El-Regal, 2016). These studies are often limited to single time scales (e.g., daily or monthly differences) and thus may be limited in their capacity to resolve seasonal dynamics (Godeaux, 1987; Abu El-Regal et al., 2018). Collectively, these studies documented gelatinous zooplankton in the Red Sea as including at least 26 species of Siphonophora, 29 species of Hydrozoa, 2 Scyphozoa species, and 24 species of Tunicata (Godeaux, 1987; Dowidar, 2003a, b; Zakaria, 2015). However, any argument supporting differences in abundance between studies is presumably limited due to insufficient understanding of temporal dynamics in these gelatinous zooplankton communities (Kheireddine et al., 2017; Karati et al., 2019).
The Red Sea is oligotrophic, has limited external water inputs, consistently high temperatures throughout the year (Chaidez et al., 2017), and presents variations in temperature, nutrients and salinity across latitude and time (Raitsos et al., 2013). Northern regions of the Red Sea tend to present lower nutrient concentrations, higher salinities and temperatures, and low phytoplankton productivity, particularly in the summer period (Raitsos et al., 2013; Chaidez et al., 2017; Kheireddine et al., 2017). The Southern Red Sea is dominated by monsoon-driven, nutrient-rich Indian Ocean water inputs from the Gulf of Aden, which positively correlate with phytoplankton occurrence (Sofianos and Johns, 2007; Raitsos et al., 2015; Kürten et al., 2016). In the Southern Red Sea, phytoplankton productivity values were found to be two to three- fold higher than in the Northern Red Sea (0.41 vs. 0.16 g m–2 d–1; Ismael, 2015). Whereas, in the North Red Sea average annual productivity values were found to be 1.5 – 3 times higher than in the Central Red Sea (0.25–0.50 vs. 0.17 g m–2 d–1; Yentsch and Wood, 1961; Koblentz-Mishke et al., 1970; Dowidar, 1983; Ismael, 2015). Even so, mesoscale processes (e.g., cyclonic and anticyclonic eddies) and proximity to coral reefs have been shown to add variability to plankton dynamics within the Red Sea (Echelman and Fishelson, 1990; Pearman et al., 2014; Kürten et al., 2016; Amer, 2019).
Here, we developed a 21-month time series of epipelagic gelatinous zooplankton communities in the coastal Red Sea in parallel to a time series of environmental variability (Prabowo and Agusti, 2019). We conducted the first Red Sea characterization of occurrence and community structure of gelatinous planktonic communities over seasonal time scales. We subsequently explored, within the limitations of the data set, how environmental parameters may affect the seasonal dynamics of Red Sea gelatinous zooplankton communities.
Materials and Methods
Sample Design and Collection
Sampling was conducted approximately every 2 weeks between September 2016 and May 2018 as part of a larger time series sampling scheme (e.g., Martin et al., 2019; Prabowo and Agusti, 2019). Samples were collected at around midday (10–12 a.m.) to standardize the time of day for sampling across time points and to specifically target the daytime community. The sampling station was located in a shallow pelagic area (i.e., <50 m depth) of the Central Red Sea (22.31N, 39.0E: Supplementary Figure S1 in Supplementary Materials), which was <10 Km offshore and ∼3 Km from inshore coral reefs systems (Prabowo and Agusti, 2019). A conductivity-temperature-depth (CTD) probe (Ocean Seven 310; Idronaut) was deployed during sampling to measure sea surface temperature and salinity (Prabowo and Agusti, 2019). Surface seawater was sampled (i.e., 300 ml at 1 m depth), filtered with Wathmann GF/F filters and chlorophyll a (chl a) was extracted in 90% acetone for 24 h. Chl a concentration was obtained using fluorometric determination (Trilogy; Turner Design) as described in Prabowo and Agusti (2019). Cross- and along-shelf Ekman transport was calculated using methods in Resgalla et al. (2001) and wind data for Thuwal retrospectively retrieved from www.wunderground.com at the time of sampling.
A modified epipelagic neuston net1 (60 × 20 cm, 200 μm mesh; Martin et al., 2019) was trawled for 30 min at 2–3 knots. Mesh size and duration of the trawl were set to capture the small fraction of gelatinous zooplankton (e.g., small Salpidae and Dyphidae individuals), account for the small densities of gelatinous zooplankton individuals, and to collect samples for other studies (Martin et al., 2019). During each haul, the net was towed alongside a small boat (<10 m length), as opposed to deployment from the back of the boat, to prevent turbulence that could have induced sinking of planktonic organisms and resulted in underestimated abundances. One third of the net was kept above the water’s surface to avoid excessive resistance. A Flowmeter (General Oceanics 2030R) was used to measure flow velocity at the beginning and end of each tow. After each tow, the net was washed carefully with seawater to retrieve the neuston samples. The entire samples were transferred into 0.5 L plastic bottles and kept at low temperatures until samples were returned to the laboratory and fixed in 95% ethanol. Samples were inspected under a dissecting microscope (LEICA IC80 HD) to quantify and identify gelatinous zooplankton. Morphological characteristics of specimens were examined to identify taxa to the lowest taxonomic level up to family level; following taxon-specific ID diagnoses (see Supplementary Table S1 in Supplementary Materials). Each specimen was rehydrated in filtered sea water until no differences in biovolume were found over time (see Supplementary Figures S2, S3 in Supplementary Materials). Organisms were photographed and pictures were used to measure individual dimensions using Image J2, and later to calculate biovolume following the equations for planktonic organisms from Hillebrand et al. (1999). Abundance and biovolume data were standardized to sampling effort (i.e., m3 sampled) and corrected by a factor based on the effect of wind mixing on the vertical distribution of buoyant bodies (cf. Kukulka et al., 2012).
Statistical Analyses
Gelatinous zooplankton community biovolume data, and environmental data were used for statistical analyses. Biovolume data were log (x + 1) transformed and the potential influence of environmental drivers were assessed using multivariate redundancy analyses (RDA, Oksanen et al., 2018). Univariate relationships between gelatinous zooplankton taxa and hypothetical environmental drivers were further assessed using non-parametric Spearman’s rank correlation test (R Core Team, 2018). These correlations were also tested for 1 and 2 lags, thereby assessing the relationship between abundance and environmental properties recorded 2 and 4 weeks prior to sampling. All community variables used in correlation tests were examined for autocorrelation using autocorrelation and partial autocorrelation plots, and stationarity was tested using the augmented Dickey–Fuller test (sensu Qiu, 2015).
Results
Gelatinous zooplankton followed a seasonal pattern, with consistently higher biovolume during winter/spring and lower abundances during summer (Figure 1). Higher values of biovolume (i.e., 100 106 mm3 m–3) were found from December/January to April/May and lower biovolume values were 103- 105 times lower (i.e., 0.1 – 10 mm3 m–3) from July to October. However, this pattern was not consistent among all gelatinous taxa since Porpitidae occurrence presented rapid oscillations (i.e., up to 14.63 mm3 m–3) independent of season. Other hydrozoans, Ulmaridae and other rarer taxa were observed sporadically throughout the year.
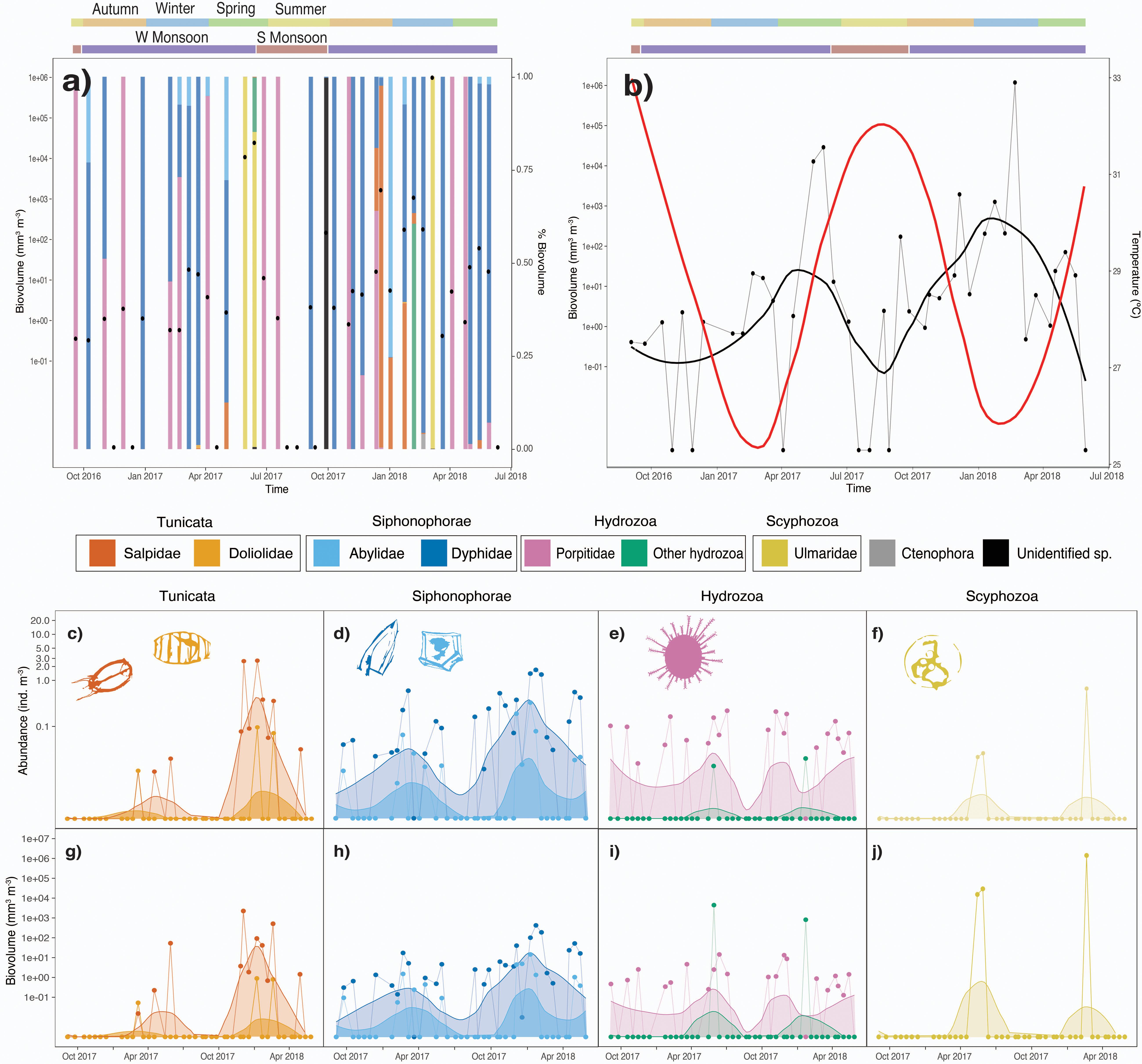
Figure 1. Temporal variation in (a) biovolume of gelatinous zooplankton and% biovolume, (b) biovolume of gelatinous zooplankton and temperature, (c–f) abundance of the most important taxa, (g–j) biovolume of the most important taxa. Lines connecting points represent the real variation, while loess interpolation is presented to aid interpretation. Temporal interpolation was performed with loess (span = 0.45). Monsoon seasonality is shown accordingly to Patzert (1974) and Murray and Johns (1997). Temperate seasonality is shown for comparison.
Seasonal fluctuations in community biovolume were one to two orders of magnitude larger in 2017/2018 (i.e., from 0.1 to >100 mm3 m–3) than those observed in 2016/2017 (i.e., from 1-10 to <100 mm3 m–3). This difference was also found for Tunicata, which presented 5 to >100 times lower values in abundance and biovolume during the blooming event in 2016/2017 than in 2017/2018. Taxa within Siphonophora also presented these interannual differences but the blooming event in 2016/2017 was only 3–25 times lower than that in 2017/2018. Observations of Porpitidae did not reveal a difference between years, and tended to be present throughout 2016/2017 and 2017/2018. Larger taxa of hydrozoans and Ulmaridae (Scyphozoa, i.e., Aurelia sp.) presented low abundances. Maximum abundances of these taxa typically occurred around April and dominated > 80% biovolume when present (i.e., Larger hydrozoans: 831 – 4555 mm3 m–3; Ulmaridae: 13871-1250975 mm3 m–3) except in one instance (i.e., larger hydrozoans in January 2018).
Environmental parameters had a significant but limited capacity (RDA; F = 2.53; P = 0.002; Figure 2) to explain variability in community structure (i.e.,% variance explained by the constrained variance: 28.97%). Temperature (RDA1: −0.43; RDA2: −0.70) and salinity (RDA1: −0.50; RDA2: −0.55) were the factors that explained a high proportion of the variance in both axes. Chl a (RDA1: 0.50; RDA2: −0.09) and cross-shelf Ekman transport (RDA1: −0.45; RDA2: 0.05) only explained variance above 0.4 in one of the axes, while along-shelf Ekman transport (RDA1: −0.27; RDA2: 0.29) explained a low portion of variance of biovolume in both axes (λRDA1 = 12.73; λRDA2 = 3.56). The variance in Tunicata, Siphonophora, hydrozoans and Ulmaridae were better explained by environmental drivers (RDA1: 2.04, 1.84, 0.58, 0.53; RDA2: −0.15, 0.42, −0.33, 0.06) than Porpitidae (RDA1: 0.20; RDA2: −0.70) and other less abundant taxa.
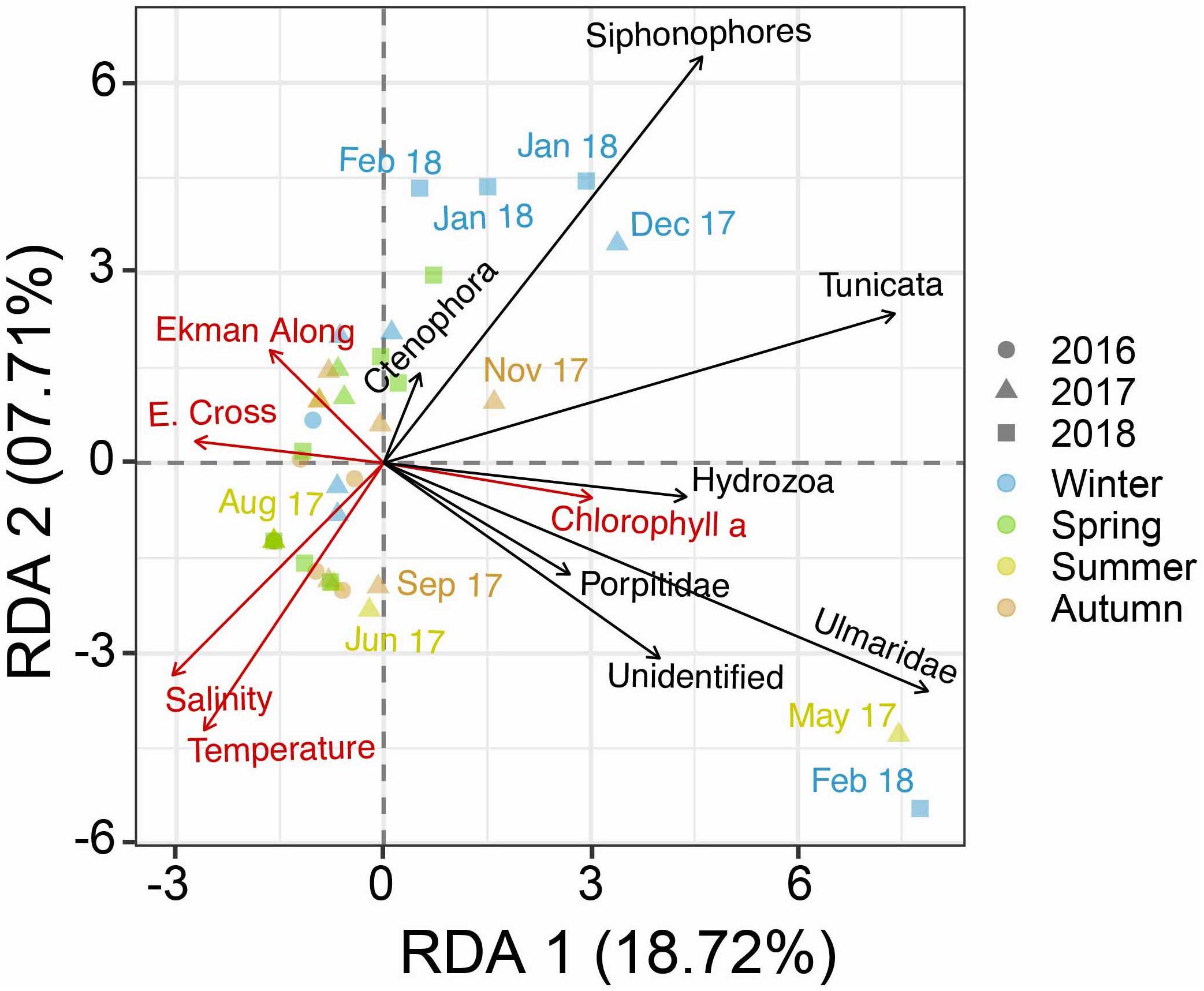
Figure 2. Redundancy Analysis (RDA) triplot showing the differences between the biovolume of the gelatinous zooplankton communities sampled, the directionality and relative strength of the relationship between environmental drivers, taxa occurrence, and the differences between sampling events for the RDA axes 1 and 2. Arrow scaling is proportional to eigenvalues.
Closer inspection revealed some generally weak but significant relationships between gelatinous abundance and environmental variables. These relationships were better explained by response lags of 1 (i.e., temperature and salinity) and 2 (i.e., chlorophyll a) in Tunicata (Figure 3). Temperature and salinity were the drivers presenting the highest number of significant correlations with composing gelatinous taxa. Tunicates and siphonophores were negatively correlated with both temperatures and salinity. Tunicates disappeared from the epipelagic layer when temperatures and salinity were high, and siphonophores decreased down to less than a third of their average biovolume when temperature and salinity were high. Tunicates also presented a positive correlation with chl a concentration, being absent or present at biovolumes 1/4th of those presented at higher chl a concentration when chl a levels were low. Porpitidae did not present any significant relationship with environmental drivers. Cross-shelf and along-shelf Ekman transport did not reveal any significant relationship with any taxon. Larger hydrozoans, Ulmaridae and other rare taxa were too sparse to present any clear pattern with the measured environmental drivers.
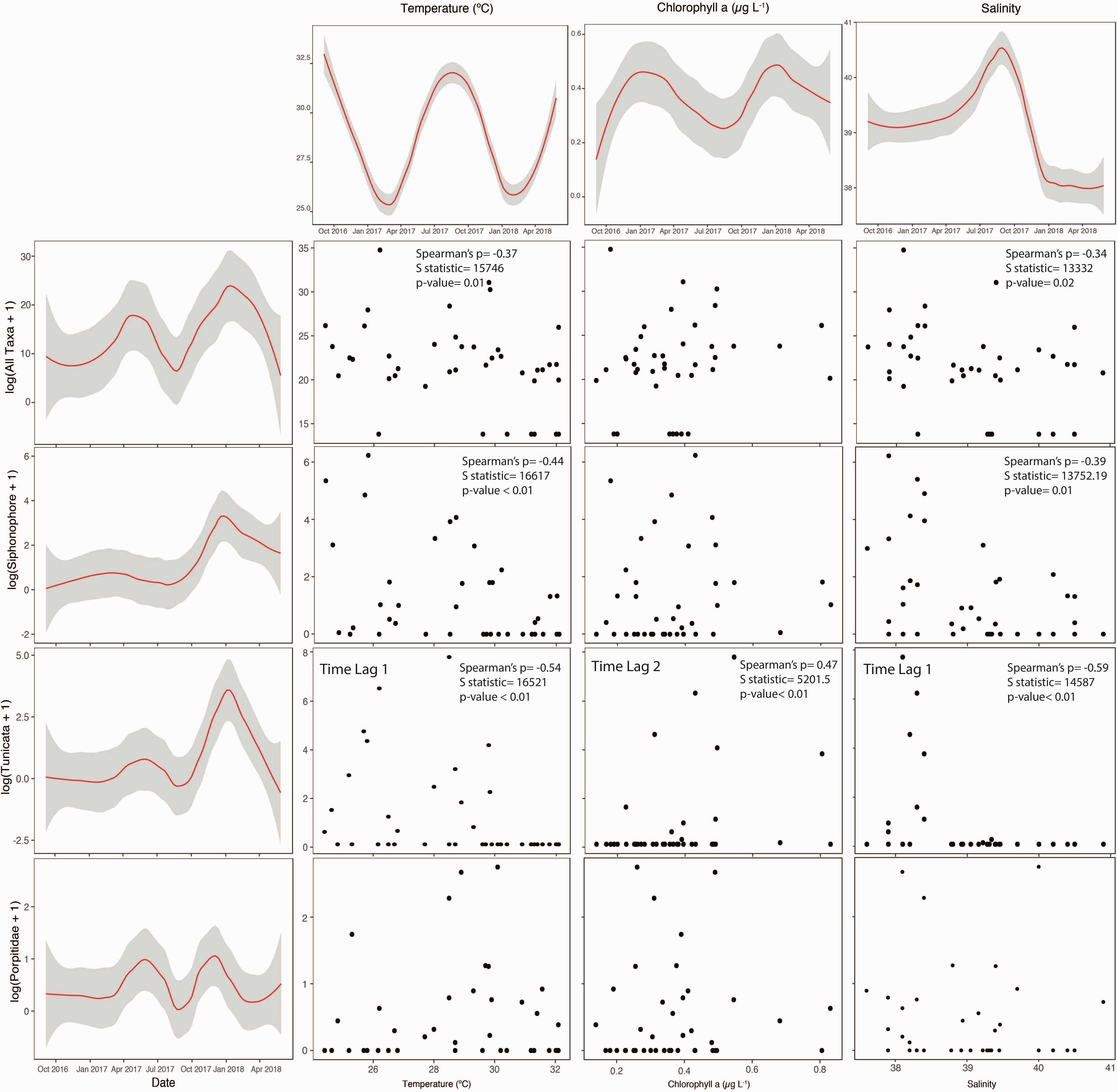
Figure 3. Correlation plots between environmental drivers over time (first row) and taxa occurrence over time (first column; biovolume). Spearman’s rank test results are shown where test resulted significant. Temporal interpolation was performed with loess (span = 0.45) and 95% confidence intervals.
Discussion
This study represents the first complete characterization of gelatinous zooplankton in the central Red Sea and describes community temporal patterns characterized by the seasonality of tunicates, siphonophores, and other larger medusae (i.e., Hydrozoa and Ulmaridae). The correspondence of these seasonal patterns with temperature, salinity and chl a fluctuations align with classic seasonal bottom-up succession dynamics (Figure 4A; Lewis and Boers, 1991; Hoover et al., 2006; Kenitz et al., 2017). Spring phytoplankton blooms specifically are often triggered by favorable conditions, including warmer temperatures, increased light levels, and the presence of nutrients (Al-Najjar et al., 2007). Such conditions often lead to the proliferation of small, fast-growing gelatinous taxa (i.e., siphonophores and tunicates), followed by larger taxa (i.e., Ulmaridae). This seasonal pattern, however, was inconsistent among gelatinous taxa, where, Porpitidae did not display clear seasonal dynamics. When combined, these data suggest that high temperature and oligotrophic conditions limit gelatinous zooplankton abundance in the Red Sea in summer. This may indicate a bottom–up control mechanism, in which gelatinous zooplankton occurrence depends on the occurrence of prey organisms (Cornils et al., 2007; Karati et al., 2019), and oligotrophic conditions limits gelatinous zooplankton abundance and biovolume to <8 ind m–3 and <103 – 106 mm3 m–3, respectively.
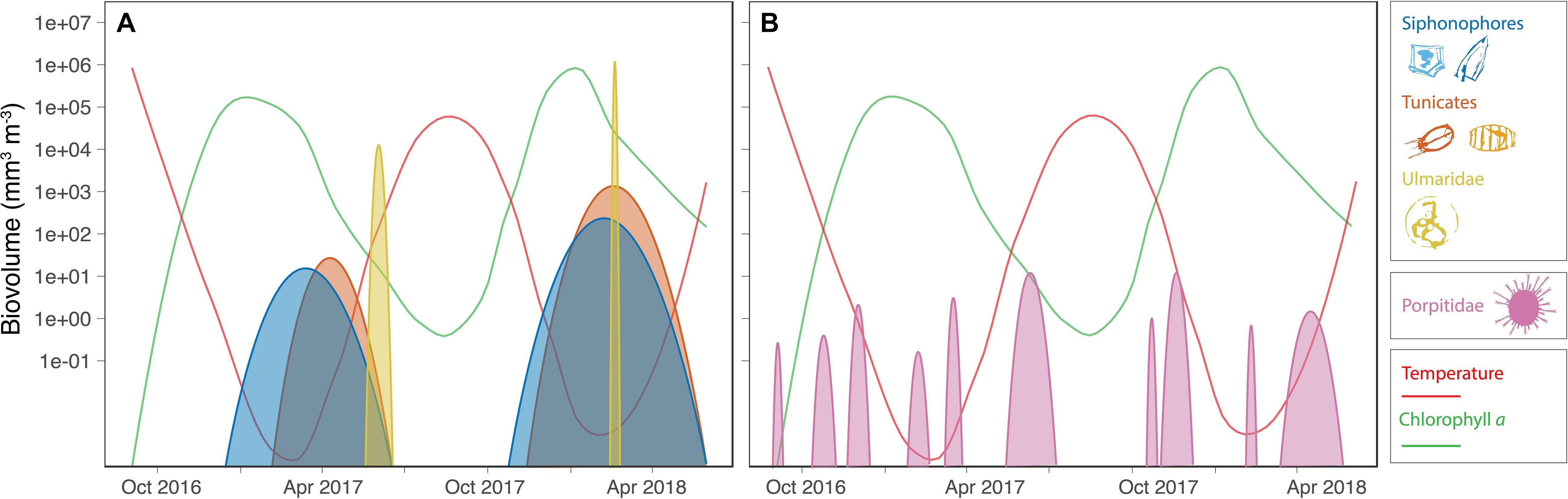
Figure 4. A summary of (A) seasonal blooming dynamics of main groups gelatinous zooplankton taxa (i.e., tunicates, siphonophores, Ulmaridae) and relative dynamics of temperature (°C) and chlorophyll a (μg L–1) found during the study period, and (B) rapid short-term fluctuations showed by Porpitidae and relative dynamics of temperature (°C) over the study period. Maximums are taken from maximum observations from the data, centered at the day at which the maximums where found, and limits represent the first time point at which individuals were observed and stopped being observed.
Seasonal successional dynamics reflected a previously observed time-lagged correlated response of zooplanktonic organisms to environmental fluctuations but tended to be less clear at higher trophic levels. In this study, tunicates tended to correlate with temperature and salinity observed in the preceding sampling event (i.e., 14 days before), and chlorophyll a observed two sampling events earlier, which suggests a closer coupling to temperature and salinity, and a slower response to phytoplankton availability (Frederiksen et al., 2006). In contrast, siphonophores showed no lagged correlation with environmental variables, which were generally weak. The weak relationship between siphonophore abundance and environmental properties is consistent with previous reports in the Red Sea for Chaetognatha (Cornils et al., 2007; Karati et al., 2019), and may result from their trophic dependence on copepods and other small heterotrophic organisms. The abundance of siphonophores may have also been affected by the input of allochthonous organic matter with dust deposition events (Jish Prakash et al., 2015; Rushdi et al., 2019), which can stimulate the growth of small zooplanktonic prey (Schmidt et al., 2016; Herrera et al., 2017). However, the presence of dust or smaller planktonic organisms such as Synechococcus, Prochlorococcus, Eukariots and Bacteria, did not show any relationship in our multivariate or univariate exploratory analysis. Even though not captured in our study, these trophic dependences could have contributed to the uncoupling of this zooplanktonic component to environmental factors (e.g., temperature, salinity; Boero et al., 2008; Al-Aidaroos et al., 2016; Karati et al., 2019). Lastly, the occurrence of larger taxa (i.e., particularly Ulmaridae) appears difficult to explain with local factors, and may be dependent on processes occurring at larger spatial and temporal scales (Bastian et al., 2011; Canepa et al., 2013). Seasonal occurrences of Ulmaridae in coastal areas have been reported in the Black Sea, where Aurelia sp. spatial distribution changed from spring to summer; increasing up to four times their abundance in southern coastal areas during summer but being present throughout the year in other areas (Mutlu, 2001). In relation to these dynamics, Ulmaridae (i.e., Aurelia sp.) occur more consistently throughout the year in the Northern Red Sea (El-Serehy and Al-Rasheid, 2011).
Weak to absent seasonal dynamics were found for Porpitidae, which depicted more stable numbers throughout the year and between years, but with markedly rapid fluctuations (Figure 4B). Taxa within the family Porpitidae contain symbiotic cells (Bouillon and Boero, 2000; Chowdhury et al., 2016), which can provide a food source (e.g., Pitt et al., 2005; Leal et al., 2017) and thus may make them less responsive to seasonal prey availability. Consequently, Porpitidae occurrence patterns in this study were better described by rapid fluctuations and the lack of a seasonal pattern, which corresponds to previous findings of occurrence dynamics of the symbiotic Cassiopea sp. in the Northern Red Sea (Niggl and Wild, 2010) as well as Cassiopea sp. elsewhere (Fitt and Costley, 1998). Porpitidae seasonal occurrence has not been studied before, but closely related species have been observed to be affected by temperature and display very patchy distributions (Evans, 1986; Parker et al., 2005; Gili et al., 2010). In this study, extreme temperatures over summer, water currents, or other environmental (i.e., wind; Kirkendale and Calder, 2003) and biotic drivers (e.g., trophic relationships; Arai, 2005) may have affected the occurrence of Porpitidae, but were not reflected in our data.
In this study, the seasonal dynamics of the gelatinous community appear to be driven mostly by temperature, reflecting the seasonality described in the Northern Red Sea (Raitsos et al., 2013), but interannual differences reveal uncoupled dynamics between chlorophyll a and temperature that could be related to variable nutrient inputs. Nutrient-driven seasonal dynamics may be aligned with the seasonality described for the Southern Red Sea (Raitsos et al., 2015), or result from mesoscale oceanic processes and proximity to reefs at the sampling site (Pearman et al., 2014; Kürten et al., 2016; Amer, 2019). Other observed seasonal patterns, showing higher zooplanktonic diversity in summer (Casas et al., 2017), were not observed in our gelatinous plankton community. Observations of individual taxa in the Northern Red Sea indicate important diversity of gelatinous zooplankton below the family level during winter and report other families of siphonophores that were not identified in this study (Godeaux, 1987; Mańko et al., 2017). In addition, our study reports the presence of a Porpitidae sp. in the Red Sea for the first time, which is consistent with other reports of Porpitidae spp. occurring in other regions close to coral reefs (Chowdhury et al., 2016) such as coral atolls in the Arabian Sea (Nagabhushanam and Rao, 1972), and in the eastern Mediterranean (Cinar et al., 2014).
Our sampling was limited to the uppermost epipelagic layer and therefore precluded the characterization of taxa occupying deeper layers, which may explain differences between our results and other observations or studies in the Red Sea (e.g., Godeaux, 1987; Dowidar, 2003a, b; Zakaria, 2015). Horizontal trawling focused on the surface layers, as opposed to vertical or oblique trawling, dictated the underestimation of number of taxa and the description of a gelatinous zooplankton community specific to the particular conditions occurring at the surface (i.e., high temperature, high irradiance, higher influence of wind). Capturing more taxa and targeting other gelatinous zooplankton communities across various depths in more than one geographical point, would have allowed for a better understanding of ecological dynamics and the ability to capture likely more diverse gelatinous zooplankton communities. However, we were able to track the dynamics of a specific community over time, which allowed us to disentangle the complexity that may have been more difficult to track across depths. Given the short timeframe of the study, the larger variability found across multiple depths could have hindered the ability to capture variability over time.
Comparisons with studies in other temperate and tropical latitudes are in consonance with the effects of oligotrophic conditions observed in the Red Sea (Lucas et al., 2014; Raitsos et al., 2015; Almahasheer et al., 2016), as confirmed in this study (Table 1). The oligotrophic conditions are exacerbated during the summer time (Prabowo and Agusti, 2019) where gelatinous zooplankton showed the annual minima. Indeed, Lucas et al. (2014) reported that oligotrophic conditions in the Red Sea limit the growth and presence of gelatinous zooplankton. In our study, seasonal peaks (i.e., 8 individuals m–3 and from 103 mm3 m–3 -when Ulmaridae was not present- to 106 mm3 m–3 -when Ulmaridae was present) were several times lower than abundance reported in other tropical, subtropical, temperate and polar regions, indicating a likely effect of the oligotrophic nature of the Red Sea. Seasonal succession dynamics described in other tropical and temperate regions may not be as defined and blooms can last longer (Racault et al., 2012). Non-seasonal dynamics are rarely reported in the literature, and it is still not clear from our data whether summer temperatures, seasonal winds or biotic interactions drive the abundance of Porpitidae, and other, less abundant taxa. Our data, however, also shows a large difference in the peak abundance among the 2 years studied. In other studies, interannual variations in gelatinous abundance are commonly explained by broad disparate drivers such as mild winter temperatures, global-scale climate fluctuations (i.e., NAO, El Niño) and changes in ocean eddies and currents (e.g., Ortega and Martínez, 2007; Rigual-Hernández et al., 2010; Sommer and Lewandowska, 2011; Racault et al., 2012; Kozak et al., 2014). The abundance and composition of gelatinous zooplankton in the central Red Sea may also depend on climate fluctuations and seasonal events such as of Monsoon winds and incoming currents from the Gulf of Aden, which have been previously linked to plankton blooming events in the southern Red Sea (i.e., in years of strong Monsoon winds; Raitsos et al., 2015; Kürten et al., 2016). However, gelatinous plankton has been shown to exhibit 18–20 years oscillations, thereby requiring very long (>40 years) datasets to resolve these dynamics (Condon et al., 2013). Interannual differences in temperature may also affect seasonality, and the temporal variability in the strength of small-scale drivers, such as those derived from the proximity to coral reefs and water currents must be also considered (Raitsos et al., 2013; Pearman et al., 2014, 2017; Kheireddine et al., 2017).
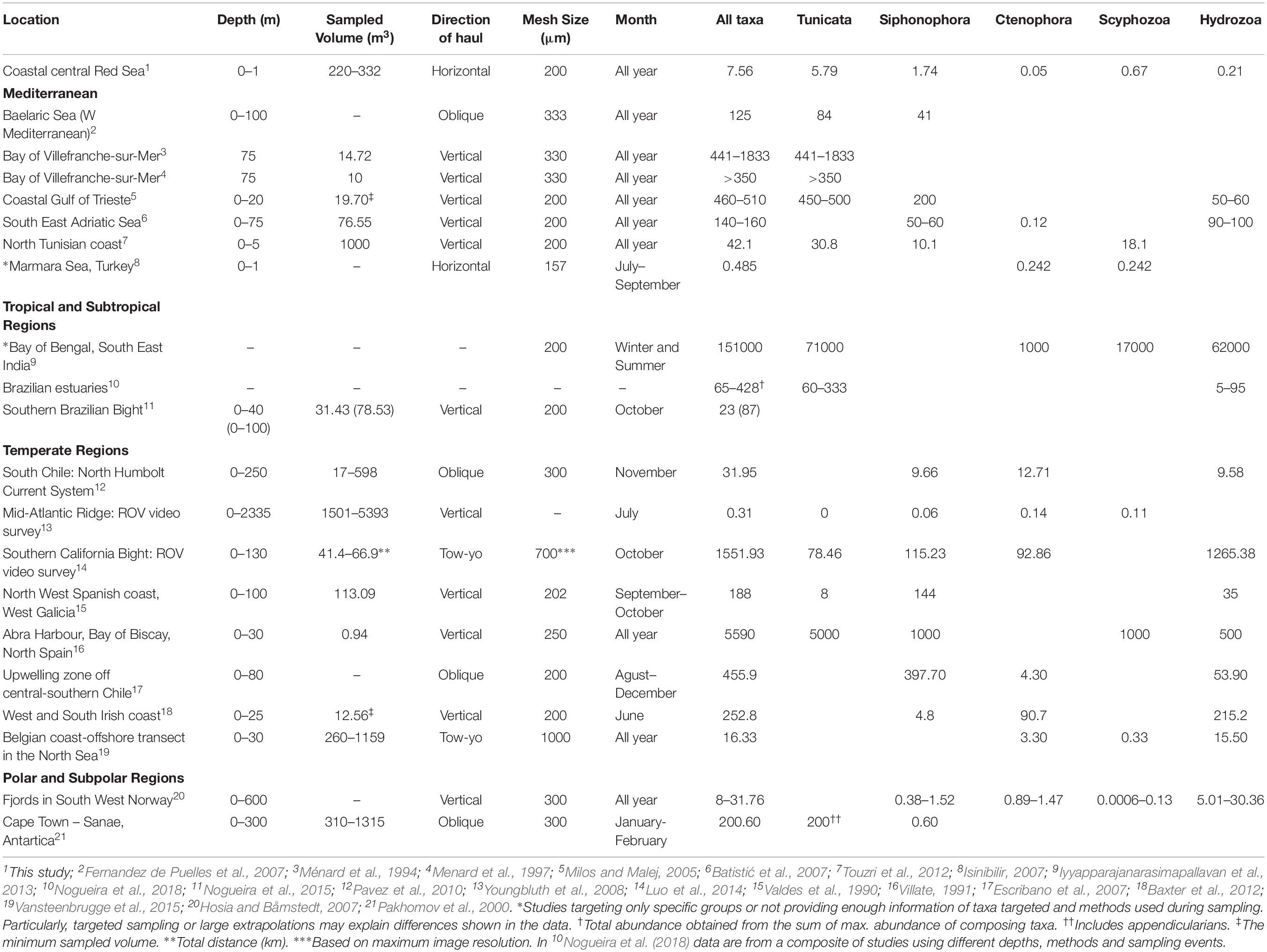
Table 1. Summary of studies on gelatinous zooplankton communities comparing maximum abundance (ind. m–3) of the all taxa sampled, and the maximum abundance found for each of the groups considered in this study.
This study shows the importance of Salpidae, Dyphidae, Porpitidae and larger taxa (e.g., Ulmaridae) as key groups shaping the seasonal dynamics of gelatinous zooplankton communities in the surface layers of the coastal central Red Sea. We have examined the ecological dynamics that may allow expanding the knowledge from global biological patterns described in Lucas et al. (2014) to local ecological dynamics. We have characterized the effects of temperature and associated planktonic blooms on temporal dynamics of gelatinous zooplankton in an oligotrophic area with no previous detailed temporal characterizations of gelatinous zooplankton communities. We also describe local putative non-seasonal dynamics for Porpitidae in these communities. Further research should include other gelatinous zooplankton communities covering a range of depths, other relevant taxonomic groups (i.e., Appendicularians, Chaetognatha), consider additional ecological drivers (i.e., presence of copepodites) and account for larger timeframes to better understand the temporal dynamics of gelatinous zooplankton communities in the Red Sea.
Data Availability Statement
The datasets generated for this study can be found as Supplementary Material and in the open repository Pangea.de.
Author Contributions
SA designed the study and provided the environmental data. CD, JS, and SK contributed to the definition of aims and hypotheses. CM contributed to sample collection and data collation. JS analyzed the samples and conducted the data analyses with advice from SK. JS, SK, and CD wrote the manuscript. All authors contributed to the improvement of the manuscript before approving the submission.
Funding
This research was funded by King Abdullah University of Science and Technology (KAUST) through baseline funding to SA and CD. JS was supported by KAUST through the VSRP Program.
Conflict of Interest
The authors declare that the research was conducted in the absence of any commercial or financial relationships that could be construed as a potential conflict of interest.
Acknowledgments
We thank S. Haddock, G. Paulay, W. Patry, and C. E. Mills for identification advice, J. de la Cruz Martinez for sampling, and CMOR for facilities and assistance throughout the sampling.
Supplementary Material
The Supplementary Material for this article can be found online at: https://www.frontiersin.org/articles/10.3389/fmars.2019.00726/full#supplementary-material
Footnotes
- ^ Design details in aquaticbiotechnology.com/en/plankton-nets/manta-net.
- ^ https://imagej.nih.gov/
References
Aberle, N., Hansen, T., Boettger-Schnack, R., Burmeister, A., Post, A. F., and Sommer, U. (2010). Differential routing of ‘new’ nitrogen toward higher trophic levels within the marine food web of the Gulf of Aqaba. Northern Red Sea. Mar. Biol. 157, 157–169. doi: 10.1007/s00227-009-1306-y
Abu El-Regal, M. A., El-Wazeer, A., Abou Elnaga, Z. S., and Amr, A. A. (2018). Composition and spatio-temporal distribution of zooplankton community in the Egyptian red sea coast at Hurghada. Egypt. J. Aqua. Biol. Fish. 22, 1–12. doi: 10.21608/ejabf.2018.8761
Al-Aidaroos, A. M., El-Sherbiny, M. M., and Mantha, G. (2016). Spatial heterogeneity of zooplankton abundance and diversity in the Saudi coastal waters of the Southern Red Sea. Indian J. Geo Mar. Sci. 45, 70–85.
Almahasheer, H., Duarte, C. M., and Irigoien, X. (2016). Nutrient limitation in central Red Sea mangroves. Front. Mar. Sci. 3:271. doi: 10.1038/s41598-017-10424-9
Al-Najjar, T., Badran, M. I., Richter, C., Meyerhoefer, M., and Sommer, U. (2007). Seasonal dynamics of phytoplankton in the Gulf of Aqaba. Red Sea. Hydrobiologia 579, 69–83. doi: 10.1016/j.marpolbul.2014.06.026
Amer, M. A. A. (2019). “Zooplankton of the Red Sea,” in Oceanographic and Biological Aspects of the Red Sea, eds N. M. A. Rasul and I C. F. Stewart (Cham: Springer), 477–490. doi: 10.1007/978-3-319-99417-8_26
Arai, M. N. (2005). Predation on pelagic coelenterates: a review. J. Mar. Biol. Assoc. U.K. 85, 523–536. doi: 10.1017/s0025315405011458
Bastian, T., Haberlin, D., Purcell, J. E., Hays, G. C., Davenport, J., McAllen, R., et al. (2011). Large-scale sampling reveals the spatio-temporal distributions of the jellyfish Aurelia aurita and Cyanea capillata in the Irish Sea. Mar. Biol. 158, 2639–2652. doi: 10.1007/s00227-011-1762-z
Batistić, M., Jasprica, N., Carić, M., and Lučić, D. (2007). Annual cycle of the gelatinous invertebrate zooplankton of the eastern South Adriatic coast (NE Mediterranean). J. Plankton Res. 29, 671–686. doi: 10.1093/plankt/fbm048
Baxter, E. J., McAllen, R., Allcock, A. L., and Doyle, T. K. (2012). “Abundance, distribution and community composition of small gelatinous zooplankton in southern irish coastal waters,” in Biology and Environment: Proceedings of the Royal Irish Academy, (Dublin: Royal Irish Academy), 91–103.
Boero, F., Bouillon, J., Gravili, C., Miglietta, M. P., Parsons, T., and Piraino, S. (2008). Gelatinous plankton: irregularities rule the world (sometimes). Mar. Ecol. Progr. Ser. 356, 299–310. doi: 10.3354/meps07368
Bouillon, J., and Boero, F. (2000). Synopsis of the families and genera of the Hydromedusae of the world, with a list of the worldwide species. Thalassia Salentina 24, 47–296.
Canepa, A., Fuentes, V., and Gili, J. M. (2013). “Spatiotemporal dynamics and environmental association of stranded Jellyfish along the Catalan coast,” in proceedings of the Conference: Fourth International Jellyfish Bloom Symposium, (NW Mediterranean).
Casas, L., Pearman, J. K., and Irigoien, X. (2017). Metabarcoding reveals seasonal and temperature-dependent succession of zooplankton communities in the Red Sea. Front. Mar. Sci. 4:241. doi: 10.3389/fmars.2017.00241
Chaidez, V., Dreano, D., Agusti, S., Duarte, C. M., and Hoteit, I. (2017). Decadal trends in Red Sea maximum surface temperature. Sci. Rep. 7:8144. doi: 10.1038/s41598-017-08146-z
Chowdhury, M. S. N., Sharifuzzaman, S. M., Chowdhury, S. R., Rashed-Un-Nabi, M., and Hossain, M. S. (2016). First Record of Porpita porpita (Cnidaria: Hydrozoa) from the coral reef ecosystem. Bangladesh. Ocean Sci. J. 51, 293–297. doi: 10.1007/s12601-016-0025-9
Cinar, M. E., Yokeş, M. B., Açik, ş, and Bakir, A. K. (2014). Checklist of cnidaria and ctenophora from the coasts of Turkey. Turk. J. Zool. 38, 677–697. doi: 10.3906/zoo-1405-68
Condon, R. H., Duarte, C. M., Pitt, K. A., Robinson, K. L., Lucas, C. H., Sutherland, K. R., et al. (2013). Recurrent jellyfish blooms are a consequence of global oscillations. Proc. Natl. Acad. Sci. U.S.A. 110, 1000–1005. doi: 10.1073/pnas.1210920110
Cornils, A., Schnack-Schiel, S. B., Al-Najjar, T., Badran, M. I., Rasheed, M., Manasreh, R., et al. (2007). The seasonal cycle of the epipelagic mesozooplankton in the northern Gulf of Aqaba (Red Sea). J. Mar. Syst. 68, 278–292. doi: 10.1016/j.jmarsys.2007.01.001
Cruz-Rivera, E., and Abu El-Regal, M. (2016). A bloom of an edible scyphozoan jellyfish in the Red Sea. Mar. Biodivers. 46, 515–519. doi: 10.1007/s12526-015-0381-1
Diaz Briz, L., Sánchez, F., Marí, N., Mianzan, H., and Genzano, G. (2017). Gelatinous zooplankton (ctenophores, salps and medusae): an important food resource of fishes in the temperate SW Atlantic Ocean. Mar. Biol. Res. 13, 630–644. doi: 10.1080/17451000.2016.1274403
Dowidar, M. M. (2003a). Distribution and abundance of Cnidaria community in the Gulf of Aqaba and Nortern Red Sea. Egypt. J. Soc. Environ. Dev. 4, 119–136.
Dowidar, M. M. (2003b). Mesozooplankton communities in the Gulf of Aqaba and northern Red Sea. Egypt. J. Aqua. Biol. Fish. 7, 1–21.
Dowidar, N. M. (1983). “Primary production in the central Red Sea off Jiddah,” in Proceedings of the International Conference on Marine Science in the Red Sea, Vol. 9, (Egypt: Bulletin of Institute of Oceanography and Fisheries), 160–170.
Echelman, T., and Fishelson, L. (1990). Surface zooplankton dynamics and community structure in the Gulf of Aqaba (Eilat). Red Sea. Mar. Biol. 107, 179–190. doi: 10.1007/bf01313255
El-Serehy, H. A., and Al-Rasheid, K. A. (2011). Reproductive strategy of the jellyfish Aurelia aurita (Cnidaria Scyphomedusae) in the Suez Canal and its migration between the Red Sea and Mediterranean. Aqua. Ecosyst. Health Manag. 14, 269–275. doi: 10.1080/14634988.2010.527231
Escribano, R., Hidalgo, P., González, H., Giesecke, R., Riquelme-Bugueño, R., and Manríquez, K. (2007). Seasonal and inter-annual variation of mesozooplankton in the coastal upwelling zone off central-southern Chile. Progr. Oceanogr. 75, 470–485. doi: 10.1016/j.pocean.2007.08.027
Evans, F. (1986). Velella velella (L.), the ‘by-the-wind-sailor’, in the North Pacific Ocean in 1985. Mar. Observ. 56, 196–200.
Fernandez de Puelles, M. L., Alemany, F., and Jansá, J. (2007). Zooplankton time-series in the Balearic Sea (Western Mediterranean): variability during the decade 1994–2003. Progr. Oceanogr. 74, 329–354. doi: 10.1016/j.pocean.2007.04.009
Fitt, W. K., and Costley, K. (1998). The role of temperature in survival of the polyp stage of the tropical rhizostome jellyfish Cassiopea xamachana. J. Exp. Mar. Biol. Ecol. 222, 79–91. doi: 10.1016/s0022-0981(97)00139-1
Frederiksen, M., Edwards, M., Richardson, A. J., Halliday, N. C., and Wanless, S. (2006). From plankton to top predators: bottom-up control of a marine food web across four trophic levels. J. Anim. Ecol. 75, 1259–1268. doi: 10.1111/j.1365-2656.2006.01148.x
Fuentes, V. L., Purcell, J. E., Condon, R. H., Lombard, F., and Lucas, C. H. (2018). Jellyfish blooms: advances and challenges. Mar. Ecol. Progr. Ser. 591, 3–5. doi: 10.3354/meps12536
Gili, J. M., Fuentes, V., Atienza, D., and Lewinsky, I. (2010). Report of the Medusa Project. Technical Report No. 8. Agencia Catalana de l’Aïgua, Generalitat de Catalunya, Barcelona.
Godeaux, J. (1986). The Gulf of Aqaba, a zone of great biological interest. UNESCO Tech. Papers Mar. Sci. 49, 104–106.
Godeaux, J. (1987). Distribution of Thalicea on a transect from the Gulf of Aden to the central Red Sea during the winter monsoon (March 1979). Oceanol. Acta 10, 197–204.
Haddock, S. H. (2004). A golden age of gelata: past and future research on planktonic ctenophores and cnidarians. Hydrobiologia 530, 549–556.
Hays, G. C., Doyle, T. K., and Houghton, J. D. (2018). A paradigm shift in the trophic importance of jellyfish? Trends Ecol. Evol. 33, 874–884. doi: 10.1016/j.tree.2018.09.001
Herrera, I., López-Cancio, J., Yebra, L., and Hernández-Léon, S. (2017). The effect of a strong warm winter on subtropical zooplankton biomass and metabolism. J. Mar. Res. 75, 557–577. doi: 10.1357/002224017822109523
Hillebrand, H., Dürselen, C. D., Kirschtel, D., Pollingher, U., and Zohary, T. (1999). Biovolume calculation for pelagic and benthic microalgae. J. Phycol. 35, 403–424. doi: 10.1046/j.1529-8817.1999.3520403.x
Hoeksema, B. W., and Waheed, Z. (2012). It pays to have a big mouth: mushroom corals ingesting salps at northwest Borneo. Mar. Biodivers. 42, 297–302. doi: 10.1007/s12526-012-0110-y
Hoover, R. S., Hoover, D., Miller, M., Landry, M. R., DeCarlo, E. H., and Mackenzie, F. T. (2006). Zooplankton response to storm runoff in a tropical estuary: bottom-up and top-down controls. Mar. Ecol. Progr. Ser. 318, 187–201. doi: 10.3354/meps318187
Hosia, A., and Båmstedt, U. (2007). Seasonal changes in the gelatinous zooplankton community and hydromedusa abundances in Korsfjord and Fanafjord, western Norway. Mar. Ecol. Progr. Ser. 351, 113–127. doi: 10.3354/meps07148
Isinibilir, M. (2007). Abundance of gelatinous zooplankton in Izmit Bay, the Sea of Marmara. Rapp. Com. Int. Mer. Medit. 38, 505.
Ismael, A. A. (2015). “Phytoplankton of the Red Sea,” in The Red Sea, eds N. M. A. Rasul and I. C. F. Stewart (Heidelberg: Springer), 567–583. doi: 10.1007/978-3-662-45201-1_32
Iyyapparajanarasimapallavan, G., Kumar, P. S., Kumar, C. P., Jalal, K. C. A., Kamaruzzaman, B. Y., and John, B. A. (2013). Distribution and abundance of gelatinous zooplankton along Tamil Nadu coastal waters. J. Biol. Sci. 13, 18. doi: 10.3923/jbs.2013.18.25
Jish Prakash, P., Stenchikov, G. L., Kalenderski, S., Osipov, S., and Bangalath, H. K. (2015). The impact of dust storms on the Arabian Peninsula and the Red Sea. Atmos. Chem. Phys. 15, 199–222. doi: 10.5194/acp-15-199-2015
Karati, K. K., Al-Aidaroos, A. M., Devassy, R. P., El-Sherbiny, M. M., Jones, B. H., Sommer, U., et al. (2019). Ecohydrographic control on the community structure and vertical distribution of pelagic Chaetognatha in the Red Sea. Mar. Biol. 166:30.
Kenitz, K. M., Visser, A. W., Mariani, P., and Andersen, K. H. (2017). Seasonal succession in zooplankton feeding traits reveals trophic trait coupling. Limnol. Oceanogr. 62, 1184–1197. doi: 10.1002/lno.10494
Kheireddine, M., Ouhssain, M., Claustre, H., Uitz, J., Gentili, B., and Jones, B. H. (2017). Assessing pigment-based phytoplankton community distributions in the Red Sea. Front. Mar. Sci. 4:132. doi: 10.3389/fmars.2017.00132
Kirkendale, L., and Calder, D. R. (2003). Hydroids (Cnidaria: Hydrozoa) from Guam and the Commonwealth of the Northern Marianas Islands (CNMI). Micronesica 35, 159–188.
Koblentz-Mishke, O. J., Volkovinsky, V. V., Kabanova, J. G., and Wooster, W. S. (1970). “Plankton primary production of the world ocean,” in Scientific Exploration of the South Pacific, (Washington, D.C: National Academy of Sciences), 183–193.
Kozak, E. R., Franco-Gordo, C., Suárez-Morales, E., and Palomares-García, R. (2014). Seasonal and interannual variability of the calanoid copepod community structure in shelf waters of the Eastern Tropical Pacific. Mar. Ecol. Progr. Ser. 507, 95–110. doi: 10.3354/meps10811
Kukulka, T., Proskurowski, G., Moret-Ferguson, S. E., Meyer, D. W., and Law, K. L. (2012). The effect of wind mixing on the vertical distribution of buoyant plastic debris. Geophys. Res. Lett. 39:L07601.
Kürten, B., Al-Aidaroos, A. M., Kürten, S., El-Sherbiny, M. M., Devassy, R. P., Struck, U., et al. (2016). Carbon and nitrogen stable isotope ratios of pelagic zooplankton elucidate ecohydrographic features in the oligotrophic Red Sea. Progr. Oceanogr. 140, 69–90. doi: 10.1016/j.pocean.2015.11.003
Leal, M. C., Rocha, R. J., Anaya-Rojas, J. M., Cruz, I. C., and Ferrier-Pagès, C. (2017). Trophic and stoichiometric consequences of nutrification for the intertidal tropical zoanthid Zoanthus sociatus. Mar. Pollut. Bull. 119, 169–175. doi: 10.1016/j.marpolbul.2017.03.054
Lewis, J. B., and Boers, J. J. (1991). Patchiness and composition of coral reef demersal zooplankton. J. Plankton Res. 13, 1273–1289. doi: 10.1093/plankt/13.6.1273
Lucas, C. H., Jones, D. O., Hollyhead, C. J., Condon, R. H., Duarte, C. M., Graham, W. M., et al. (2014). Gelatinous zooplankton biomass in the global oceans: geographic variation and environmental drivers. Glob. Ecol. Biogeogr. 23, 701–714. doi: 10.1111/geb.12169
Luo, J. Y., Grassian, B., Tang, D., Irisson, J. O., Greer, A. T., Guigand, C. M., et al. (2014). Environmental drivers of the fine-scale distribution of a gelatinous zooplankton community across a mesoscale front. Mar. Ecol. Progr. Ser. 510, 129–149. doi: 10.3354/meps10908
Macali, A., Semenov, A., Venuti, V., Crupi, V., D’Amico, F., Rossi, B., et al. (2018). Episodic records of jellyfish ingestion of plastic items reveal a novel pathway for trophic transference of marine litter. Sci. Rep. 8:6105. doi: 10.1038/s41598-018-24427-7
Madin, L. P., and Harbison, G. R. (1977). The associations of Amphipoda Hyperiidea with gelatinous zooplankton—I. Associations with Salpidae. Deep Sea Res. 24, 449–463. doi: 10.11646/zootaxa.4192.1.1
Mańko, M. K., Słomska, A. W., and Jażdżewski, K. (2017). Siphonophora of the Gulf of Aqaba (Red Sea) and their associations with crustaceans. Mar. Biol. Res. 13, 480–485. doi: 10.1080/17451000.2017.1296577
Martin, C., Agustí, S., and Duarte, C. M. (2019). Seasonality of marine plastic concentration in central Red Sea pelagic waters. Sci. Total Environ. 688, 536–541. doi: 10.1016/j.scitotenv.2019.06.240
Ménard, F., Dallot, S., Thomas, G., and Braconnot, J. C. (1994). Temporal fluctuations of two Mediterranean salp populations from 1967 to 1990. Analysis of the influence of environmental variables using a Markov chain model. Mar. Ecol. Progr. Ser. 104, 139–152. doi: 10.3354/meps104139
Menard, F., Fromentin, J. M., Goy, J., and Dallot, S. (1997). Temporal fluctuations of doliolid abundance in the bay of Villefranche-sur-Mer (Northwestern Mediterranean Sea). Oceanol. Acta 20, 733–742.
Milos, C., and Malej, A. (2005). Gelatinous zooplankton assemblages in temperate coastal waters-Seasonal variations (Gulf of Trieste. Adriatic Sea). Annales 15, 11–20.
Murray, S. P., and Johns, W. (1997). ‘Direct observations of seasonal exchange through the Bab el Mandab Strait’. Geophys. Res. Lett. 24, 2557–2560. doi: 10.1029/97GL02741
Mutlu, E. (2001). Distribution and abundance of moon jellyfish (Aurelia aurita) and its zooplankton food in the Black Sea. Mar. Biol. 138, 329–339. doi: 10.1007/s002270000459
Nagabhushanam, A. K., and Rao, G. C. (1972). An ecological survey of the marine fauna of Minicoy Atoll (Laccadive Archipelago, Arabian Sea). Mitteilungen aus dem Museum für Naturkunde in Berlin. Zoologisches Museum und Institut für Spezielle Zoologie 48, 265–324. doi: 10.1002/mmnz.4830480203
Niggl, W., and Wild, C. (2010). Spatial distribution of the upside-down jellyfish Cassiopea sp. within fringing coral reef environments of the Northern Red Sea: implications for its life cycle. Helgoland Mar. Res. 64:281. doi: 10.1007/s10152-009-0181-8
Nogueira, M. Jr., Brandini, F. P., and Codina, J. C. U. (2015). Diel vertical dynamics of gelatinous zooplankton (Cnidaria, Ctenophora and Thaliacea) in a subtropical stratified ecosystem (South Brazilian Bight). PLoS One 10:e0144161. doi: 10.1371/journal.pone.0144161
Nogueira, M., do Nascimento, L. S., Maciel, P. V., Tilbert, S., and Oliveira, L. D. (2018). “Diversity, species composition and assemblage dynamics of estuarine gelatinous and semi-gelatinous zooplankton from Brazil,” in Plankton Ecology of the Southwestern Atlantic, eds M. S. Hoffmeyer, M. E. Sabatini, F. P. Brandini, D. L. Calliari, and N. H. Santinelli (Cham: Springer), 375–412. doi: 10.1007/978-3-319-77869-3_18
Oksanen, J., Blanchet, F. G., Friendly, M., Kindt, R., Legendre, P., McGlinn, D., et al. (2018). vegan: Community Ecology Package. R package version 2.4-6. Available at: https://CRAN.R-project.org/package=vegan (accessed September 01, 2019).
Ortega, L., and Martínez, A. (2007). Multiannual and seasonal variability of water masses and fronts over the Uruguayan shelf. J. Coast. Res. 23, 618–629. doi: 10.2112/04-0221.1
Pakhomov, E. A., Perissinotto, R., McQuaid, C. D., and Froneman, P. W. (2000). Zooplankton structure and grazing in the Atlantic sector of the Southern Ocean in late austral summer 1993: Part 1. Ecological zonation. Deep Sea Res. Part I 47, 1663–1686. doi: 10.1016/s0967-0637(99)00122-3
Parker, D. M., Cooke, W. J., and Balazs, G. H. (2005). Diet of oceanic loggerhead sea turtles (Caretta caretta) in the central North Pacific. Fish. Bull. 103, 142–152.
Patzert, W. C. (1974). Wind-induced reversal in Red Sea circulation. Deep-Sea Res. Oceanogr. Abstr. 21, 109–121. doi: 10.1016/0011-7471(74)90068-90060
Pavez, M. A., Landaeta, M. F., Castro, L. R., and Schneider, W. (2010). Distribution of carnivorous gelatinous zooplankton in the upwelling zone off central Chile (austral spring 2001). J. Plankton Res. 32, 1051–1065. doi: 10.1093/plankt/fbq029
Pearman, J. K., Ellis, J., Irigoien, X., Sarma, Y. V. B., Jones, B. H., and Carvalho, S. (2017). Microbial planktonic communities in the Red Sea: high levels of spatial and temporal variability shaped by nutrient availability and turbulence. Sci. Rep. 7:6611. doi: 10.1038/s41598-017-06928-z
Pearman, J. K., El-Sherbiny, M. M., Lanzén, A., Al-Aidaroos, A. M., and Irigoien, X. (2014). Zooplankton diversity across three Red Sea reefs using pyrosequencing. Front. Mar. Sci. 1:27. doi: 10.3389/fmars.2014.00027
Pitt, K. A., Koop, K., and Rissik, D. (2005). Contrasting contributions to inorganic nutrient recycling by the co-occurring jellyfishes, Catostylus mosaicus and Phyllorhiza punctata (Scyphozoa, Rhizostomeae). J. Exp. Mar. Biol. Ecol. 315, 71–86. doi: 10.1016/j.jembe.2004.09.007
Ponomareva, L. A. (1966). Quantitative distribution of zooplankton in the Red Sea as observed in the period May-June, 1966. Inst. Oceanogr. USSR Acad. Sci. USSR Oceanol. 8, 240–242.
Prabowo, D. A., and Agusti, S. (2019). Free-living dinoflagellates of the central Red Sea, Saudi Arabia: Variability, new records and potentially harmful species. Mar. Pollut. Bull. 141, 629–648. doi: 10.1016/j.marpolbul.2019.03.012
Purcell, J. E. (2011). Jellyfish and ctenophore blooms coincide with human proliferations and environmental perturbations. Annu. Rev. 4, 209–235. doi: 10.1146/annurev-marine-120709-142751
Qiu, D. (2015). aTSA: Alternative Time Series Analysis. R Package Version 3.1.2. Available at: https://CRAN.R-project.org/package=aTSA (accessed September 10, 2019).
R Core Team (2018). R: A language and environment for statistical computing. Vienna: R Foundation for Statistical Computing.
Racault, M. F., Le Quéré, C., Buitenhuis, E., Sathyendranath, S., and Platt, T. (2012). Phytoplankton phenology in the global ocean. Ecol. Indicat. 14, 152–163. doi: 10.1016/j.ecolind.2011.07.010
Raitsos, D. E., Pradhan, Y., Brewin, R. J., Stenchikov, G., and Hoteit, I. (2013). Remote sensing the phytoplankton seasonal succession of the Red Sea. PLoS One 8:e64909. doi: 10.1371/journal.pone.0064909
Raitsos, D. E., Yi, X., Platt, T., Racault, M. F., Brewin, R. J., Pradhan, Y., et al. (2015). Monsoon oscillations regulate fertility of the Red Sea. Geophys. Res. Lett. 42, 855–862. doi: 10.1002/2014gl062882
Resgalla, C. Jr., De La Rocha, C., and Montú, M. (2001). The influence of Ekman transport on zooplankton biomass variability off southern Brazil. J. Plankton Res. 23, 641–650. doi: 10.1093/plankt/23.6.641
Rigual-Hernández, A. S., Bárcena, M. A., Sierro, F. J., Flores, J. A., Hernández-Almeida, I., Sanchez-Vidal, A., et al. (2010). Seasonal to interannual variability and geographic distribution of the silicoflagellate fluxes in the Western Mediterranean. Mar. Micropaleontol. 77, 46–57. doi: 10.1016/j.marmicro.2010.07.003
Rushdi, A. I., Chase, Z., Simoneit, B. R., and Paytan, A. (2019). “Sources of organic tracers in atmospheric dust, surface seawater particulate matter and sediment of the Red Sea,” in Oceanographic and Biological Aspects of the Red Sea, ed. I. C. F. Stewart (Cham: Springer), 75–88. doi: 10.1007/978-3-319-99417-8_4
Schmidt, K., Schlosser, C., Atkinson, A., Fielding, S., Venables, H. J., Waluda, C. M., et al. (2016). Zooplankton gut passage mobilizes lithogenic iron for ocean productivity. Curr. Biol. 26, 2667–2673. doi: 10.1016/j.cub.2016.07.058
Sofianos, S. S., and Johns, W. E. (2007). Observations of the summer Red Sea circulation. J. Geophys. Res. 112:C06025.
Sommer, U., and Lewandowska, A. (2011). Climate change and the phytoplankton spring bloom: warming and overwintering zooplankton have similar effects on phytoplankton. Glob. Change Biol. 17, 154–162. doi: 10.1111/j.1365-2486.2010.02182.x
Touzri, C., Hamdi, H., Goy, J., and Daly Yahia, M. N. (2012). Diversity and distribution of gelatinous zooplankton in the Southwestern Mediterranean Sea. Mar. Ecol. 33, 393–406. doi: 10.1111/j.1439-0485.2012.00510.x
Valdes, J. L., Roman, M. R., Alvarez-Ossorio, M. T., Gauzens, A. L., and Miranda, A. (1990). Zooplankton composition and distribution off the coast of Galicia, Spain. J. Plankton Res. 12, 629–643. doi: 10.1093/plankt/12.3.629
Vansteenbrugge, L., Van Regenmortel, T., De Troch, M., Vincx, M., and Hostens, K. (2015). Gelatinous zooplankton in the Belgian part of the North Sea and the adjacent Schelde estuary: spatio-temporal distribution patterns and population dynamics. J. Sea Res. 97, 28–39. doi: 10.1016/j.seares.2014.12.008
Villate, F. (1991). Annual cycle of zooplankton community in the Abra Harbour (Bay of Biscay): abundance, composition and size spectra. J. Plankton Res. 13, 691–706. doi: 10.1093/plankt/13.4.691
Yentsch, C. S., and Wood, L. (1961). Measurements of primary productivity in the Red Sea, Gulf of Aden and Indian Ocean. Woods Hole, MA: Woods Hole Oceanographic Institution, 61–66.
Youngbluth, M., Sørnes, T., Hosia, A., and Stemmann, L. (2008). Vertical distribution and relative abundance of gelatinous zooplankton, in situ observations near the Mid-Atlantic Ridge. Deep Sea Res. Part II 55, 119–125. doi: 10.1016/j.dsr2.2007.10.002
Keywords: jellyfish communities, cnidarians, siphonophores, tunicates, scyphozoans, hydrozoans, seasonality, oligotrophy
Citation: Sola J, Klein SG, Martin C, Agustí S and Duarte CM (2019) Gelatinous Zooplankton in the Surface Layers of the Coastal Central Red Sea. Front. Mar. Sci. 6:726. doi: 10.3389/fmars.2019.00726
Received: 01 July 2019; Accepted: 08 November 2019;
Published: 29 November 2019.
Edited by:
Michelle Jillian Devlin, Centre for Environment, Fisheries and Aquaculture Science (CEFAS), United KingdomReviewed by:
Ulisses Miranda Azeiteiro, University of Aveiro, PortugalLeo Berline, Aix-Marseille Université, France
Copyright © 2019 Sola, Klein, Martin, Agustí and Duarte. This is an open-access article distributed under the terms of the Creative Commons Attribution License (CC BY). The use, distribution or reproduction in other forums is permitted, provided the original author(s) and the copyright owner(s) are credited and that the original publication in this journal is cited, in accordance with accepted academic practice. No use, distribution or reproduction is permitted which does not comply with these terms.
*Correspondence: Jordi Sola, anNvbGFjb2RAZ21haWwuY29t