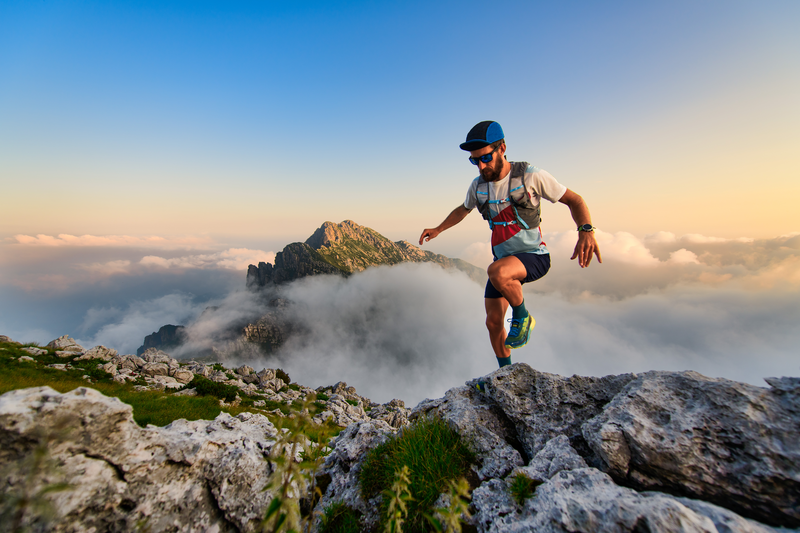
95% of researchers rate our articles as excellent or good
Learn more about the work of our research integrity team to safeguard the quality of each article we publish.
Find out more
ORIGINAL RESEARCH article
Front. Mar. Sci. , 26 November 2019
Sec. Marine Fisheries, Aquaculture and Living Resources
Volume 6 - 2019 | https://doi.org/10.3389/fmars.2019.00724
This article is part of the Research Topic The Status of Marine Fisheries in East Asia View all 16 articles
Based on growth and related fishery parameters, three approaches, yield-per-recruit (Y/R), utility-per-recruit (U/R) analyses, and relative biomass (B/B0) analyses were applied to 21 economically important, trawl-caught species in China's coastal seas to estimate their relative yield, economic value and biomass under different schedules of fishing mortality and mean length at first capture. The results show that all species suffer from overfishing, given the high average fishing mortality (F ~ 1 year−1) and small mesh size (~1 cm) used by trawlers. Long-term Y/R would double and U/R (expressed as price per landed weight) would increase 5-fold if mesh size were increased to about 10 cm. Comparing Y/R and U/R showed that the benefits of higher prices for larger individuals were detectable only if larger mesh sizes are used, so that individuals are caught only after they have been able to grow. The Y/R analyses also allowed estimating the biomass of the 21 assessed populations relative to their unexploited biomass, i.e., B/B0. Species-specific B/B0 values ranged from 0.01 to 0.58, with a mean of 0.16 (±0.03), i.e., much lower than the 50% reduction corresponding to Maximum Sustainable Yield (i.e., B/BMSY = 1, or B/B0 = 0.5). This confirms the many authors who reported systematic overfishing along China's coastlines, and suggests that rebuilding stocks should be the foremost goal of fisheries management in China.
According to statistics of the Food and Agriculture Organization of the United Nations (FAO, 2016), the People's Republic of China (hereafter referred to as “China”), was the top-ranking fishing country in the world with domestic marine catches of about 10 million t in the 2010s (www.fao.org and www.seaaroundus.org).
As part of its Thirteenth Five-Year Plan (2016–2020), China listed the need for improvement of its fishery management systems. Several policies aiming at stabilizing fisheries catches have been proposed, but their implementation has not necessarily been successful. Notably, many of the economic benefits that the policies we supposed to generate have failed to materialize.
One of the most important management measures, the “Double Control” system, was proposed in the early 1990s to regulate fisheries by controlling the number of engine-powered fishing vessels and the cumulative power of the fleet (Shen and Heino, 2014). However, despite a decrease in the number of fishing vessels since 2004, cumulative fleet engine power and tonnages have increased (Anonymous, 1979–2019; Figure 1A), and CPUE and total catch continued decreases that began in 1998 (Shen and Heino, 2014; Figure 1B).
Figure 1. Basic statistics on China's coastal fisheries (1990–2018). (A) Three proxies of fishing effort; (B) Two measures of catch per unit of effort (CPUE).
Mesh size studies in China's coastal have been conducted since 1980s (Ye et al., 1980; Li, 1990); however, the implementation of mesh regulation was initiated only in 2013 (Anonymous, 2013). The regulations allow mesh size ranging from 2.5 to 11 cm for different gear types and species. However, the average mesh size of commercial fishing in practice of China is 1 cm, far less than it legally allowed (Liang and Pauly, 2017a).
As a result, fish are caught that are extremely small and thus are considered “trash fish” and end up as fish feed, either directly, or after reduction to low-value fish meal (Cao et al., 2015). Moreover, the proportion of “trash fish” in the total catches appears to be steadily increasing (Lin et al., 2007), and currently contributes near 4 × 106 t annually (Greenpeace, 2017).
Historically, larger species were dominant in China's coastal seas, and were economically important. This applies Larimichtyys polyactis and Trichiurus lepturus, whose annual yield was more than 100,000 t, and for Scomberomorus niphonius and Scomber japonicus, which contributed over 10,000 t annually (Zhang and Liu, 1959). However, under intensive, decade-long fishing pressure, these dominant stocks were replaced by small, low-trophic level species, such as Engraulis japonicus, Setipinna tenuifilis, Pholis fangi, and Chaeturichthys stigmatias (Wang et al., 2011; Zhai et al., 2015), inducing a fishing down effect that is now well-documented (Liang and Pauly, 2017b). The degree of overfishing and the economic waste that this implies are key problems for China's fisheries.
Therefore, yield- and utility-per-recruit approaches were applied to 21 species commercially exploited along China's coast, which allowed a combination of fisheries biology and bio-economics to assess the extent of the reduction if their biomass by fishing, and their optimum exploitation levels terms of both yield and value. The ultimate goal of this contribution was to produce evidence required for a review of present policies for fisheries management.
We performed utility-per-recruit assessments, which allows consideration of different values per length or age group to be used in a yield-per-recruit context (Die et al., 1988), and hence allows the introduction of simple bio-economics into stock assessments. Here, the values considered were simply the market price per kilogram of the fish in question, which tended to sharply increase with size.
Also, relative biomass (B/B0) was estimated using a new set of equations, based on Beverton and Holt (1966), and derived by Froese et al. (2018). This method allows estimating B/B0 under different levels of fishing mortality and Lc values, using the same parameters as for yield-per-recruit analyses, i.e., von Bertalanffy growth parameters (Linf, K), natural mortality (M) and a and b from length-weight relationships (Froese et al., 2018).
Growth parameters can change over time, both because of fishing itself, which removes large individuals and gradually reduce the alleles associated with large sizes in an exploited population (Dieckmann et al., 2005; Enberg et al., 2012), and via ocean warming which will tend to modify growth parameter in the same direction as fishing itself (Cheung et al., 2013). However, these changes are much smaller than the rapid population truncation and size reduction that are due to removal of large individuals by intense fishing, and which are reflected in Y/R and related analyses.
A total of 21 species were analyzed in this paper. The growth parameters (a, b, K, Linf, and t0) of 14 fish species were assembled (Table 1) from the scientific literature and from FishBase (www.fishbase.org) to serve as basis for the 3 approaches mentioned above. To facilitate computations and between-method comparisons, the multiplicative term in all length-weight relationship (“a”) where recalculated such that the exponent (“b”) could be set at a value = 3. Given the cube law (Froese, 2006), b = 3 is a good approximation, and deviation from this will have only a negligible impact on the results. For the other 7 species (Table 2), Y/R analyses had already been performed (by Liang and Pauly, 2017a); thus, Equations (12)–(15) were used to convert the results of their Y/R estimates into estimates of B/BMSY and B/B0, so that they could also be included in overall evaluation of the status of Chinese fisheries (in Table 7).
Table 1. Parameters used for assessing 14 species in China's coastal seas (a refers to weight in g; K in year−1; Linf and TLinf in cm; T in °C).
Table 2. Parameters used to estimate relative biomass (B/B0) for seven fish species in China's coastal seasa.
Fish growth parameters commonly estimated by the von Bertalanffy Growth Function (VBGF; von Bertalanffy, 1934, 1938), as presented by Beverton and Holt (1957), i.e.,
where Lt is the mean length at age t of the fish in question, Linf is their asymptotic length, i.e., the mean length attained after an infinitely long time, K is a growth coefficient (here in year−1) and to is the (usually negative) age the fish in question would have had at a length of zero if they had always grown in the manner predicted by the equation (which they have not; see e.g., Pauly, 1998).
Following Geng et al. (2018) who recommended its use for assessments in China, the empirical formula of Pauly (1980) was used to estimate natural mortality (M), i.e.,
where Linf (in cm) and K are as defined for Equation (1) and T is the annual average water temperature (in °C) of the habitat for each species analyzed here (Table 1).
As Equation (2) requires Linf values as total length (TL), conversion from standard length (SL), fork length (FL), and vent length (VT, for T. lepturus) were performed as required based on drawings or photos of the species in question in FishBase (www.fishbase.org).
The mean length at first capture (Lc in cm), i.e., the length at which 50% of fish will be retained in the gear, was estimated for all species from
wherein S.F. is the selection factor of the gear, largely determined by the shape of the fish body.
S.F. estimates were derived from a simplified version of the nomogram constructed by Pauly (1983) on the basis of a large number of mesh selection experiments (Figure 2). Here, we applied the depth ratios from images in FishBase (www.fishbase.org).
Figure 2. Nomogram for the estimation of selection factors of fishes from their body proportion (modified from Pauly, 1983).
As about 50% of all catches in the Chinese coastal fisheries are actually made by trawlers, and the rest is taken by nets also designed to retain large fish when they are caught (see China's successive Fishery Statistical Yearbooks, 1979–2019), it is assumed that all nets in question have trawl-like selection curves. Thus, Equation (3), Pauly's (1983) nomogram were applied here to infer mean length at first capture for the 14 species in Table 1.
As we could not find estimates from China, the growth parameters for red tonguesole (Cynoglossus joyneri) are from South Korean waters (Baech and Huh, 2004), i.e., from the same latitude as China's Yellow Sea, to which South Korean waters are adjacent. As temperature is the major factor behind differences in the growth parameters of wild fish (Pauly, 2010), and temperature varies mainly with latitude, it is considered that the effect of this substitution is negligible.
The original equations derived by Beverton and Holt (1957) allowed the computation of absolute yield-per-recruit (Y/R, typically in g·year−1). However, subsequent consideration by Beverton and Holt (1966) allow a for a simplified approach, based on relative yield-per-recruit (Y′/R), i.e.,
where E = F/Z, Z = F + M;
where E is the exploitation rate, F is the fishing mortality, Z is the total mortality and the other parameters are defined as same as above (Equations 2, 3).
The relationship between Y/R and Y′/R, is
where M and t0 is the same definition with Equations (2) and (1), respectively, Winf is the asymptotic fish weight (corresponding to Linf), and tr is age at recruitment to the stock in question.
The utility of length class i for each species was computed from the Equations (6)–(11) by (Thompson and Bell, 1934):
where Yi is the yield for class i, vi is the unit value (or “price”) for class i, and Yi was obtained from
where the mean body weight in a class, computed by
and where the parameter of a and b are the coefficients of the length-weight relationship and Li and Li+1 are the lower limit and the upper limit of the length class i, respectively (Beyer, 1987).
Ci was obtained from:
where Ni is the cohort strength, as predicted by:
and
where Δti is the elapsed time from Li to Li+1.
Herein, the length class are 0.01·Linf, i.e., the computations involved 100 classes, and the market prices for the different length class of fish are given in Table 3.
Relative yield-per-recruit (Y′/R), as estimated by Equation (4), also can be expressed by (Froese et al., 2018)
Given that CPUE can be seen as proportional to biomass, dividing Equation (12) by F/M gives
The relative biomass of fish with length >Lc when no fishing occurs is expressed by
where B0 is the unexploited biomass. From this, the relative biomass of exploited fishery can be obtained by
(Froese et al., 2018). The limitations of this approach lie in its assumptions, i.e., that growth follow the von Bertalanffy model, that fishing and natural mortality rates behave as expressed in the above equations, that gear selection is of the trawl type and, most importantly, that the parameters of these various relationships are not density-dependent. These assumptions are generally accepted in fisheries science and we lack the data from Chinese fisheries that would allow us to replace these assumptions by locally-derived empirical relationship.
The growth parameters and hence the M values for these species, combined with F = 1 year−1 for Chinese waters (Liang and Pauly, 2017a), generates exploitation rates well over 50%, for example in P. fangi, P. haematocheila, C. herzensteini, P. olivaceus, and S. japonicus. The average exploitation rate of our 14 species was 66% (Table 4).
Table 4. Estimates of mortality, mean length at first capture and derived parameters in 14 species of fish exploited along Chinese coasts (M and Z in year−1; Lc in cm)a.
The estimated mean size at first capture (Lc) of 11 of 14 species were smaller than their predicted length at age zero, i.e., with the current mesh size, most of the fish are predicted to be caught as soon as they are hatched, i.e., as larvae. Therefore, considering that the von Bertalanffy equations does not represent well the growth of very young fish (Pauly, 1998), the Lc/Linf were slightly increased, such that Lc matched, in these cases, length at age zero. The exceptions were T. lepturus, P. olivaceous, and S. japonicus (Table 4).
The Y′/R and U/R values for 14 species were reported (Tables 5A,B). L. polyactis, T. lepturus, and S. niphonius are provided as illustrated examples (Figure 3); figures for the 11 other species are provided in the Supplementary Material. Overall, these results suggest that the fisheries in China's coastal seas have neither optimized yield, nor utility as expressed in fish prices.
Table 5A. Current and optimum yield-per-recruit and mean first capture length of 14 species in China's coastal seas.
Table 5B. Current and optimum utility-per-recruit and mean length at first capture of 14 species in China's coastal seas (Lc and mesh size in cm; U/R in Yuan).
Figure 3. Assessments of 3 fish species from Chinese coastal waters: Y′/R (left) and U/R (right) isopleth diagrams vs. fishing mortality and Lc/Linf. The solid curves connect optimum sizes for different every level of fishing mortality and the black dots and dotted lines show the current status of the fishery status in level. U/R for L. polyactis is in Yuan, and in 1,000 Yuan for T. lepturus and S. niphonius. (A) L. polyactis Y′/R vs. fishing mortality and Lc/Linf. (B) L. polyactis U/R vs. fishing mortality and Lc/Linf. (C) T. lepturus Y′/R vs. fishing mortality and Lc/Linf. (D) T. lepturus U/R vs. fishing mortality and Lc/Linf. (E) S. niphonius Y′/R vs. fishing mortality and Lc/Linf. (F) S. niphonius U/R vs. fishing mortality and Lc/Linf.
Indeed, the data suggest that Y/R would increase by over 80% on average if average Lc/Linf was increased to 0.53, which would correspond to a mesh size of about 10 cm (Table 5A). In general, the predicted increase was bigger in species that could potentially grow to larger sizes, for example in P. haematocheila, P. olivaceus, S. niphonius, and L. litulon.
The average U/R for 14 species was predicted to increase by five times under the present fishing mortality (F = 1 year−1) if mesh sizes were increased to 12 cm, i.e., if Lc/Linf were increased from 0.18 to 0.62.
The ratios of U/R against Y/R (U/Y) correlate with Lc/Linf, i.e., large fish are more sensitive to change of Lc than smaller species (Table 6). Thus, T. lepturus, C. joyneri, and P. argenteus increased more than P. fangi and L. polyactis. Perhaps more importantly, the values of U/Y appear to be sensitive to Lc only when Lc/Linf = 0.3–0.4 (Figure 4), i.e., utility-per-recruit differs from yield-per-recruit substantially only if fish are allowed to grow before they are caught. Indeed, peak U/Y appeared at Lc/Linf values of 0.79 on average. With current U/R at 58.5 and maximum U/R at 133 Yuan/kg, current practices cause an average loss of 75 Yuan/kg per recruit.
Table 6. Ratio of U/R vs. Y/R (i.e., U/Y, in Yuan) for 14 species in China's coastal seas, with the corresponding Lc (in cm).
Figure 4. Ratio of utility-per-recruit (U/R) to yield-per-recruit (Y/R) for different Lc/Linf for 6 of the species caught in China's coastal seas. Note that this ratio is near 1 when Lc/Linf is low.
If F was kept constant while Lc was increased to Lc_MSY, relative biomasses would increase by 77% on average (Table 7); the average mesh size generating BMSYwas about 9 cm.
Relationships between different levels of F or Lc and B/B0 were illustrated for P. haematocheila, P. olivaceus, and E. japonicus (Figure 5). Relative biomass increased almost linearly with increasing Lc (Figures 5A,C,E). For large and medium species, such as P. haematocheila and P. olivaceus, relative biomasses (for F < 0.8 year−1) was rather insensitive to increase in fishing mortality (Figures 5B,D). However, for small species, such as E. japonicus, relative biomass, i.e., B/B0 was impacted by a wide range of fishing mortality (Figure 5F).
Figure 5. Relative biomass (B/B0) under different Lc and F in China's coastal seas; respectively; the black dots represent current relative biomass levels. (A) P. haematocheila B/B0 vs. Lc/Linf. (B) P. haematocheila B/B0 vs. Fishing mortality. (C) P. olivaceus B/B0 vs. Lc/Linf. (D) P. olivaceus B/B0 vs. Fishing mortality. (E) E. japonicus B/B0 vs. Lc/Linf. (F) E. japonicus B/B0 vs. Fishing mortality.
It has been often assumed that fisheries produce the maximum sustainable yield (MSY) when E = M/Z = 0.5, i.e., F = M (Alverson and Pereyra, 1969), while other authors have suggested that Fopt < M (Die and Caddy, 1997; Zhou et al., 2012). This issue is moot, however, as the E values estimated here were much higher than 0.5. Indeed, the extremely small mesh size (~1cm) used along the coast of China leads to the bulk of the catch consisting of fish at the fingerling stage, too small for human consumption, leading to the “trash fish” and end use problems mentioned earlier.
The analyses in this contribution allowed to address these problems by considering both catch and value, since both the catch and its value were much lower than optimum levels, China's fisheries would substantially benefit from increased mesh size. Indeed, in view of difficulties in reducing fishing mortality, China's fisheries managers have attempted to increase the mesh sizes used by the commercial fisheries. Thus, mesh regulation for important species have been published (Anonymous, 2013), covering L. polyactis, T. lepturus, P. haematocheila, S. niphonius, P. argenteus, C. herzensteini, S. japonicus, and many other fish and invertebrates. While some of these new legal mesh sizes are still below the size shown here to be optimal, we hope that these new regulations will be respected.
The comparison of the yield- with utility-per-recruit for our species showed, unsurprisingly, that the benefit from large mesh sizes were more pronounced in the utility-per-recruit than in the yield-per-recruit analyses. Thus, Y′/R and U/R are essentially the same for P. fangi, because this fish remains small and its market price does not change much with size, whereas the opposite is true for species, such as L. polyactis, T. lepturus, or C. myriaster. As an aside, we also note that T. lepturus, which is most popular and high-value fish in China, is one of the few species that cannot be farmed; thus, its price remains high, especially when large, because there is no substitute to wild-caught fish. Therefore, T. lepturus is assumed to be the species from which most economic benefits would be derived if lengths at first capture were increased.
The relative biomass (B/B0) for 21 species in China's coastal seas assessed here was 0.16 on average, which implied a depletion rate of 84%. The result was similar to the 80% average depletion obtained by applying the CMSY method of Froese et al. (2016) to catch time series of 15 species exploited by Chinese fisheries (Zhai et al., submitted).
Overall, this contribution provided evidence that support efforts to increase the mean length at first capture (Lc) of fish exploited along the Chinese coasts, both in terms of yield- and utility-per-recruit, because higher Lc will produce benefits even if fishing mortality is not reduced (Teh et al., 2019). However, it must be realized that the results of yield-per-recruit and utility-per-recruit analyses, as presented here, are longer-term average. In the short term, yields and catch values would decrease upon introduction of the larger mesh sizes. Therefore, supportive policies would be appropriate, which could be running parallel to existing programs to support workers transiting from fisheries to land-based occupations (Song, 2007).
All datasets generated for this study are included in the article/Supplementary Material.
LZ was responsible for the data collecting, formal analysis, and writing the original draft. DP was responsible for the conceptualization, methodology, and supervision.
LZ research was funded by China Scholarship Council (CSC). DP research was supported by the Sea Around Us, which receives funding from the Oak Foundation, the Marisla Foundation, the Paul M. Angell Family Foundation, the David and Lucile Packard Foundation, the Minderoo Foundation, and the Bloomberg Philanthropies through RARE.
The authors declare that the research was conducted in the absence of any commercial or financial relationships that could be construed as a potential conflict of interest.
LZ would like to thank Mr. Y. Li from Dalian Modern Agricultural Production Development Service Center, China for contributing the fish price data used here. We also thank Ms. Evelyn Liu for drafting our figures.
The Supplementary Material for this article can be found online at: https://www.frontiersin.org/articles/10.3389/fmars.2019.00724/full#supplementary-material
Alverson, D. L., and Pereyra, W. T. (1969). Demersal fish explorations in the northeastern pacific ocean-an evaluation of exploratory fishing methods and analytical approaches to stock size and yield forecasts. J. Fish. Board Can. 26, 1985–2001. doi: 10.1139/f69-188
Anonymous (2013). Notice of the Ministry of Agriculture on the Implementation of the Minimum Mesh Size System for Marine Fishing and Fishing Gears. Available online at http://jiuban.moa.gov.cn/zwllm/tzgg/tz/201312/t20131205_3699050.htm (accessed May 12, 2013).
Baech, G. W., and Huh, S. H. (2004). Age and growth of red tongue sole (Cynoglossus joyneri) in the Southern Sea of Korea. Korean J. Fish. and Aquat. Sci. 37, 307–311. doi: 10.5657/kfas.2004.37.4.307
Beverton, R. J. H., and Holt, S. J. (1957). On the Dynamics of Exploited Fish Populations (Fishery Investigations, Series II, Vol. 19). London: Her Majesty's Stationery Office.
Beverton, R. J. H., and Holt, S. J. (1966). Manual of Methods for Fish Stock Assessment. Part II. Tables of Yield Function. FAO Fishery Biology Technical Paper, No. 38, Version 1. Rome: Food and Agriculture Organization of the United Nations.
Beyer, J. E. (1987). On length-weigh relationships: part I: computing the mean weight of the fish in a given length class. ICLARM Fishbyte 5, 11–13.
Cao, L., Naylor, R., Henriksson, P., Leadbitter, D., Metian, M., Troell, M., et al. (2015). China's aquaculture and the world's wild fisheries. Science 347, 133–135. doi: 10.1126/science.1260149
Chen, D. G., Liu, C. G., and Dou, S. Z. (1992). The biology of flatfish (Pleuronectinae) in the coastal waters of China. Netherlands J. Sea Res. 29, 25–33. doi: 10.1016/0077-7579(92)90005-Y
Cheung, W. W. L., Sarmiento, J. L., Dunne, J., Frölicher, T. L., Lam, V. W. L., Palomares, M. L. D., et al. (2013). Shrinking of fishes exacerbates impacts of global ocean changes on marine ecosystems. Nat. Clim. Change 3, 254–258. doi: 10.1038/nclimate1691
Chu, W. S., Wang, J. P., Hou, Y. Y., Ueng, Y. T., and Chu, P. H. (2011). Length-weight relationships for fishes off the southwestern coast of Taiwan. Afr. J. Biotechnol. 10, 3945–3950. doi: 10.5897/AJB10.2074
Cui, Q. M., Yuan, C. Y., Dong, J. G., and Zhang, Q. T. (2008). Age and growth of the silver pomfret Pampus argenteus in the Bohai Bay. J. Tianjin Univer. Sci. Technol. 23, 30–23.
Die, D. J., and Caddy, J. F. (1997). Sustainable yield indicators from biomass: are there appropriate reference points for use in tropical fisheries? Fish. Res. 32, 69–79. doi: 10.1016/S0165-7836(97)00029-5
Die, D. J., Restrepo, V. R., and Hoenig, J. M. (1988). Utility-per-recruit modeling: a neglected concept. Trans. Am. Fish. Soc. 117, 274–281. doi: 10.1577/1548-8659(1988)117<0274:UMANC>2.3.CO;2
Dieckmann, U., Heino, M., and Jin, X. (2005). Shrinking fish: fisheries-induced evolution in the yellow sea. IIASA Magazine Options, Autumn 2005:8.
Enberg, K., Jørgensen, C., Dunlop, E. S., Varpe, Ø., Boukal, D. S., Baulier, L., et al. (2012). Fishing-induced evolution of growth: concepts, mechanisms and the empirical evidence. Mar. Ecol. 33, 1–25. doi: 10.1111/j.1439-0485.2011.00460.x
FAO (2016). Fishery and Aquaculture Statistics. Rome: Food and Agriculture Organization of the United Nation.
Froese, R. (2006). Cube law, condition factor and weight–length relationships: history, meta-analysis and recommendations. J. Appl. Ichthyol. 22, 241–253. doi: 10.1111/j.1439-0426.2006.00805.x
Froese, R., Demirel, N., Coro, G., Kleisner, K. M., and Winker, H. (2016). Estimating fisheries reference points from catch and resilience. Fish Fish. 18, 506–526. doi: 10.1111/faf.12190
Froese, R., Winker, H., Coro, G., Demirel, N., Tsikliras, A. C., Dimarchopoulou, D., et al. (2018). A new approach for estimating stock status from length frequency data. ICES J. Mar Sci. 75, 2004–2015. doi: 10.1093/icesjms/fsy078
Geng, P., Zhang, K., Xu, S. S., and Chen, Z. Z. (2018). Assessment of natural mortality coefficients in fish stocks: a review. J. Fish. Sci. China 25, 694–704. doi: 10.3724/SP.J.1118.2018.17399
Geng, X. Y., Li, X. P., Ma, W. L., Liu, M. L., Fang, E. J., Wang, Z. Q., et al. (2001). The relationship of age and growth for Planiliza haematocheila in Bohai Sea. Tianjin Fish. 4, 30–32.
Greenpeace (2017). The Current Status of Marine Fishing for “Trash Fish” in China and its Implications for China's Sustainable Fisheries Development. Available online at: http://www.greenpeace.org.cn/ (accessed July 31, 2017).
Hong, X. Y. (1980). A study on the age and growth of the hairtail in the Bo Hai and Huang Hai. J. Fish. China 4, 361–372.
Huang, X. X. (2010). Study on the fishery biology of Enedrias fangi Wang and Wang in the inshore waters of Qingdao (Master's thesis), Ocean University of China, Qingdao, China.
Law, C. S., and Sadovy de Mitcheson, Y. (2018). Age and growth of black seabream Acanthopagrus schlegelii (Sparidae) in Hong Kong and adjacent waters of the northern South China Sea. J. Fish Biol. 93, 382–390. doi: 10.1111/jfb.13774
Li, H. Q. (1990). Estimation of the best mesh size for many fish species in the pearl river estuary. Fish. Sci. 9, 4–7.
Liang, C., and Pauly, D. (2017a). Growth and mortality of exploited fishes in China's coastal seas and their uses for yield-per-recruit analyses. J. Appl. Ichthyol. 33, 746–756. doi: 10.1111/jai.13379
Liang, C., and Pauly, D. (2017b). Fisheries impacts on China's coastal ecosystems: unmasking a pervasive ‘fishing down' effect. PLoS ONE 12:e0173296. doi: 10.1371/journal.pone.0173296
Lin, C. L., Su, J. L., Xu, B. R., and Tang, Q. S. (2001). Long-term variations of temperature and salinity of the Bohai Sea and their influence on its ecosystem. Prog. Oceanogr. 49, 7–19. doi: 10.1016/S0079-6611(01)00013-1
Lin, L. S., Cheng, J. Y., and Ling, J. Z. (2007). Analysis on recent status of the bottom trawl fishery resources in the East China sea region. Mar. Fish. 29, 371–374. doi: 10.13233/j.cnki.mar.fish.2007.04.016
Liu, X. Z., Guo, D., Wang, A. Y., Dong, J., Wang, X. L., Duan, Y., et al. (2018). Growth characteristics of small yellow croaker Larimichthys polyactis in the Liaodong Bay. Mar. Fish. 40, 139–146.
Pauly, D. (1980). On the interrelationships between natural mortality, growth parameters, and mean environmental temperature in 175 fish stocks. ICES J. Mar. Sci. 39, 175–192. doi: 10.1093/icesjms/39.2.175
Pauly, D. (1983). Some Simple Methods for the Assessment of Tropical Fish Stocks. Rome: Food and Agriculture Organization of the United Nation, FAO Fishery Biology Technical Paper, No. 234, 52.
Pauly, D. (1998). Beyond our original horizons: the tropicalization of Beverton and Holt. Rev. Fish. Biol. Fish. 8, 307–334. doi: 10.1023/A:1008863215253
Pauly, D. (2010). Gasping Fish and Panting Squids: Oxygen, Temperature and the Growth of Water-Breathing Animals. Preußisch Oldendorf: International Ecology Institute.
Shen, G. M., and Heino, M. (2014). An overview of marine fisheries management in China. Mar. Policy 44, 265–272. doi: 10.1016/j.marpol.2013.09.012
Song, L. Q. (2007). Study on Dual-Transform of Marine Fishermen in China. Qingdao: China Ocean University Press.
Teh, L. S. L., Cashion, T., Alava Saltos, J. J., Cheung, W. W. L., and Sumaila, U. R. (2019). Status, Trends, and the Future of Fisheries in the East and South China Seas. Vancouver: Institute for the Oceans and Fisheries, The University of British Columbia, Fisheries Centre Research Reports 27, 101.
Thompson, W. F., and Bell, F. H. (1934). Effect of changes in intensity upon total yield and yield per unit of gear. Rep. Int. Fish. Comm. 8, 7–49.
von Bertalanffy, L. (1934). Investigations on the rules of growth. Part I. General principles of the theory; mathematical and physiological laws of growth in aquatic animals. Wilhelm Roux Archiv. Entwicklungsmech. Organ. 131, 613–652. doi: 10.1007/BF00650112
von Bertalanffy, L. (1938). A quantitative theory of organic growth (inquiries on growth laws. II). Hum. Biol. 10, 181–213.
Wang, X. H., Qiu, Y. S., Du, F. Y., Lin, Z. J., Sun, D. R., and Huang, S. L. (2011). Spatio-temporal variability of fish diversity and dominant species in the Beibu Gulf. J. Fish. Sci. China 18, 427–436. doi: 10.3724/SP.J.1118.2011.00427
Ye, C. C., Tang, Q. S., and Qin, Y. J. (1980). The Huanghai herring and their fisheries. J. Fish. China 4, 339–352.
Yin, Z. Q., Lu, W. Q., Chen, Y., Liu, Y. H., Zhou, S. L., Meng, W. D., et al. (2016). Growth characteristics and resource evaluation of Sebastes schlegelii in Zhangzidao artificial reef area. Anim. Husbandry Feed Sci. 8, 186–188.
Yin, Z. Q., Xu, C. C., and Chen, Y. (2015). Growth and rational utilization of Lophius litulon in Dalian Sea. J. Anhui Agri. Sci. 43, 125–127. doi: 10.13989/j.cnki.0517-6611.2015.28.049
Zhai, L., Xu, B. D., Ji, Y. P., and Ren, Y. P. (2015). Spatial pattern of fish assemblage and the relationship with environmental factors in yellow river estuary and its adjacent waters in summer. Chin. J. Appl. Ecol. 26, 2852–2858. doi: 10.13287/j.1001-9332.20150630.030
Zhang, X. W., and Liu, X. S. (1959). Investigation and study on four main economic fish ecology in China in the past ten years. Oceanol. Limnol. Sinica 11, 233–240.
Zhang, Y. Q. (2018). The analysis on the current situation of the main fishery resources in the coastal waters of Shandong during 2015–2016 (Master's thesis), Yantai University, Yantai, China.
Zhou, S. J., Y, S.W., Thorson, J. T., Smith, A. D. M., and Fuller, M. (2012). Linking fishing mortality reference points to life history traits: an empirical study. Can. J. Fish. Aquat. Sci. 69, 1292–1301. doi: 10.1139/f2012-060
Keywords: data-poor fisheries, Chinese coastal fisheries, yield per recruit, utility per recruit, biomass estimation, stock assessments
Citation: Zhai L and Pauly D (2019) Yield-per-Recruit, Utility-per-Recruit, and Relative Biomass of 21 Exploited Fish Species in China's Coastal Seas. Front. Mar. Sci. 6:724. doi: 10.3389/fmars.2019.00724
Received: 09 August 2019; Accepted: 08 November 2019;
Published: 26 November 2019.
Edited by:
Simone Libralato, Istituto Nazionale di Oceanografia e di Geofisica Sperimentale (OGS), ItalyReviewed by:
Claudio Vasapollo, Italian National Research Council (CNR), ItalyCopyright © 2019 Zhai and Pauly. This is an open-access article distributed under the terms of the Creative Commons Attribution License (CC BY). The use, distribution or reproduction in other forums is permitted, provided the original author(s) and the copyright owner(s) are credited and that the original publication in this journal is cited, in accordance with accepted academic practice. No use, distribution or reproduction is permitted which does not comply with these terms.
*Correspondence: Lu Zhai, emhhaWx1MDcwOEBob3RtYWlsLmNvbQ==
Disclaimer: All claims expressed in this article are solely those of the authors and do not necessarily represent those of their affiliated organizations, or those of the publisher, the editors and the reviewers. Any product that may be evaluated in this article or claim that may be made by its manufacturer is not guaranteed or endorsed by the publisher.
Research integrity at Frontiers
Learn more about the work of our research integrity team to safeguard the quality of each article we publish.