- 1College of Fisheries and Ocean Sciences, University of Alaska Fairbanks, Juneau, AK, United States
- 2Department of Marine Biology, Texas A&M University at Galveston, Galveston, TX, United States
- 3Texas Marine Mammal Stranding Network, Galveston, TX, United States
- 4Accademia del Leviatano, V.le dell’Astronomia 19, Rome, Italy
- 5Mysticetus LLC, Preston, WA, United States
- 6Department of Natural Sciences, University of Alaska Southeast, Juneau, AK, United States
The whale-watching industry in Juneau, Alaska relies primarily on the presence of North Pacific humpback whales (Megaptera novaeangliae). To meet demands from the rapidly growing tourism industry, the number of whale-watching vessels in this region has tripled over the last 18 years. As a result, increased vessel presence could have negative effects on humpback whales, ranging from short-term behavioral disturbance to long-term impacts. The current humpback whale viewing regulations are outdated and may not be as effective as they were 18 years ago, when both the whale-watching industry and humpback whale population were smaller. The present study assessed how humpback whale movement and behavioral patterns were affected by (1) vessel presence and number of vessels present, and (2) time spent in the presence of vessels. The study also determined how humpback whale behavioral state transitions were affected by vessel presence. A total of 201 humpback whale focal follows were conducted during summer 2016 and 2017. Based on linear mixed effects models, whales in the presence (vs. absence) of vessels exhibited 38.9% higher deviation in linear movement (p = 0.001), 6.2% increase in swimming speed (p = 0.047) and a 6.7% decrease in inter-breath intervals (IBI) (p = 0.025). For each additional vessel present, deviation increased by 6.2% (p = 0.022) and IBI decreased by 3.4% (p = 0.001). As time spent in the presence of vessels increased, respiration rate increased (p = 0.011). Feeding and traveling humpback whales were likely to maintain their behavioral state regardless of vessel presence, while surface active humpback whales were likely to transition to traveling in the presence of vessels. These short-term changes in movement and behavior in response to whale-watching vessels could lead to cumulative, long-term consequences, negatively impacting the health and predictability of the resource on which the industry relies. Current formal vessel approach regulations and voluntary guidelines should be revisited to reduce vessel pressure and mitigate potential negative effects of this growing whale-watching industry.
Introduction
Whale watching has been the world’s fastest growing wildlife-based activity since its inception in the 1950’s (Hoyt and Parsons, 2014). Global whale-watching tourism opportunities have grown concomitantly with the recovery of many whale populations from historic whaling pressure (Best, 1993; O’Connor et al., 2009). More than 13 million people in 119 countries engaged in whale-watching activities in 2008, yielding a global annual economic value of $2.1 billion, including $1 billion in revenue in the United States alone (O’Connor et al., 2009). As a non-consumptive use of cetaceans, whale watching is often labeled as “eco-friendly,” “green” or “sustainable” tourism. However, as the industry grows globally and the number of human-whale interactions increases, the whale-watching industry could be exploiting the resource on which they rely (Parsons, 2012; Cressey, 2014).
There is mounting evidence that whale watching can lead to negative effects ranging from short-term behavioral changes that disrupt life functions (e.g., feeding) to long-term consequences such as declines in physical fitness and habitat alteration (Lusseau and Bejder, 2007). In the presence of whale-watching vessels, whales have exhibited short-term behavioral changes including alteration of swimming speed and direction (Scheidat et al., 2004), diving and foraging patterns (Stamation et al., 2010; Christiansen et al., 2013b), aerial behaviors (Stamation et al., 2010; Di Clemente et al., 2018), resting patterns (Lusseau, 2003; Lundquist et al., 2012; Steckenreuter et al., 2012), group size and cohesion (Steckenreuter et al., 2012), and acoustic communication (Sousa-Lima and Clark, 2008). If short-term avoidance behaviors by whales (e.g., traveling at higher speeds, frequent changes in direction, movement away from whale-watching vessels) negatively affects body maintenance behaviors such as foraging and resting, there could be long-term declines in vital rates (Lusseau and Bejder, 2007; Parsons, 2012). If whale-watching vessels are repeatedly perceived as a threat, hormonal responses leading to chronic stress can suppress growth and limit reproduction (Atkinson et al., 2015). For females, reduction in time spent foraging could compromise energetic budgets required to produce and raise a calf, resulting in population decline (Bejder et al., 2006a; Parsons, 2012; New et al., 2015). Over time, whales may also alter habitat use and distribution in response to whale-watching vessel presence (Bejder et al., 2006b; Cartwright et al., 2012).
To mitigate the potential negative consequences of disturbance from whale-watching vessels, guidelines and regulations have been established for commercial whale-watching operations in over 40 countries (Carlson, 2013). In the United States, all cetaceans are protected under the Marine Mammal Protection Act of 1972 (MMPA), and endangered cetaceans are further protected under the Endangered Species Act of 1973 (ESA). In accordance with the MMPA, the National Oceanic and Atmospheric Administration (NOAA) has established formal whale-watching regulations and voluntary guidelines aimed to protect marine mammals from injury and behavioral disturbance. Each NOAA Fisheries Regional Office has developed marine mammal viewing regulations and guidelines tailored to the specific needs of the region. In U.S. Atlantic and Alaska regions NOAA and the Whale and Dolphin Conservation sponsor Whale SENSE (NOAA Fisheries Whale and Dolphin Conservation, 2018), a voluntary conservation and stewardship program that recognizes participating commercial whale-watching operators. Participating tour companies complete training, undergo evaluation, and agree to follow regional whale-watching guidelines.
Humpback whales (Megaptera novaeangliae) exist in every major ocean basin (Muto et al., 2018) and are a popular focal point of many whale-watching tours (O’Connor et al., 2009). Southeast Alaska is a productive feeding ground for the Central North Pacific humpback whale stock, designated as “depleted” by the MMPA (Muto et al., 2018). The ESA further categorizes stocks into Distinct Population Segments (DPS) based on their breeding areas. In Southeast Alaska, 94% of humpback whales belong to the Hawaii DPS (Calambokidis et al., 2001; Baker et al., 2013; National Oceanic and Atmospheric Administration, 2016) and 6% belong to the Mexico DPS (Muto et al., 2017). In 2016, the Hawaii DPS was delisted and designated as “not at risk” under the ESA, while the Mexico DPS was listed as “threatened” (National Oceanic and Atmospheric Administration, 2016). Overall, the humpback whale population in the North Pacific has grown at approximately 7% per year (Muto et al., 2017) with an estimated 1,585 individuals in Southeast Alaska in 2008 (Hendrix et al., 2012). Humpback whales migrate to Southeast Alaska from low latitude breeding grounds and are reliably present from May to September (Lopez and Pearson, 2017). Acquiring nutrients and energy on the Alaskan feeding grounds is essential for body maintenance and preparation for migration to low-latitude breeding grounds where humpback whales fast due to limited prey availability (Irvine et al., 2017).
Whale watching has become a lucrative industry and an important source of income to many Southeast Alaska coastal communities, with an estimated annual value of $32 million in the city of Juneau alone (O’Connor et al., 2009). To meet the demand of the rapid growth of tourism in Juneau, the whale-watching industry has tripled since 2000. Currently, there are approximately 65 vessels that offer dedicated whale-watching tours, in addition to fishing charter vessels which view whales opportunistically (Di Clemente et al., 2018). Due to this surge in vessel presence, it is unclear if the current Alaska whale-watching regulations, established in 2001 (Table 1), are sufficient to mitigate potential disturbance to humpback whales.
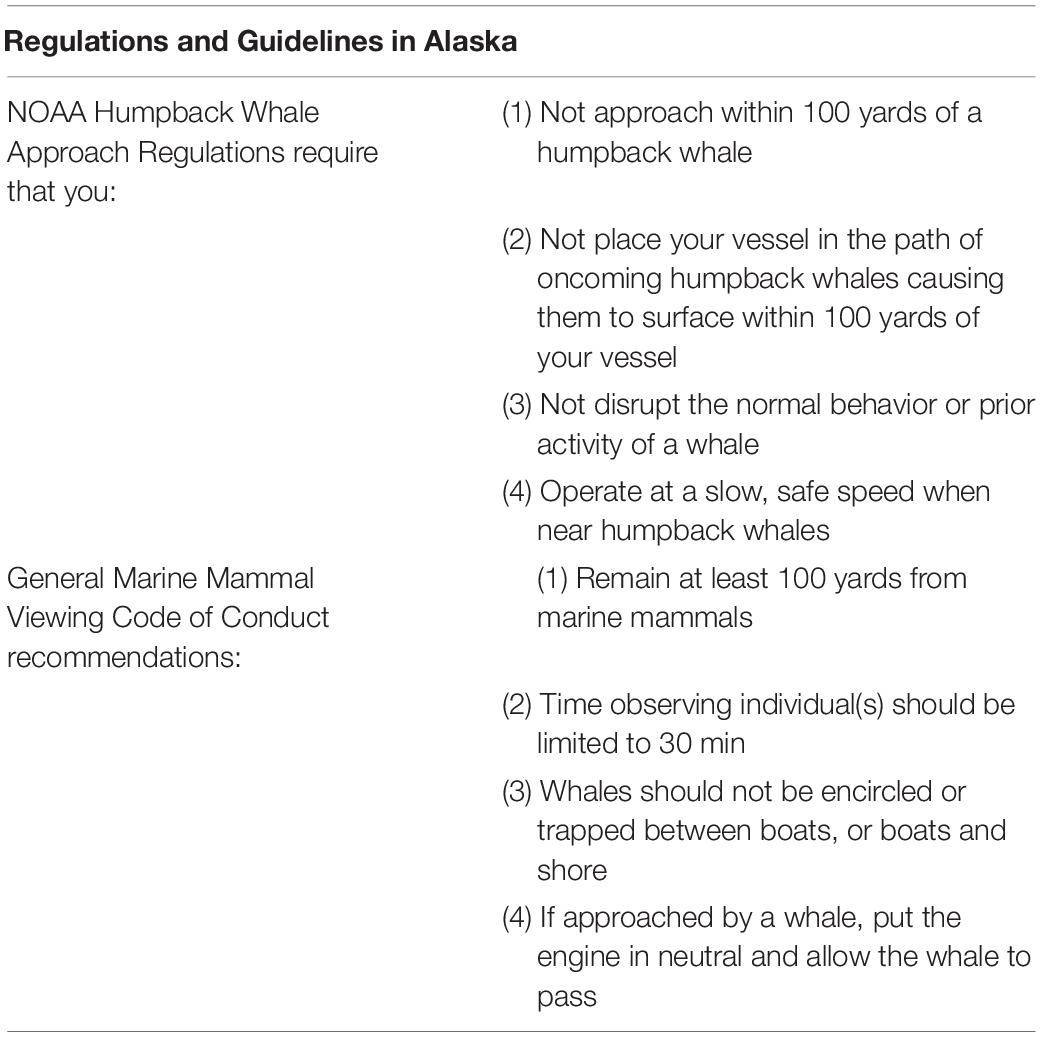
Table 1. Federal regulations and guidelines for viewing humpback whales and marine mammals in Alaska (National Oceanic and Atmospheric Administration, 2016).
Crowding of whales is of particular concern in the Juneau whale-watching tour area due to complex geography consisting of narrow channels and obstructed waterways (Weingartner et al., 2009). Compared to other whale-watching locations (e.g., Stellwagen Bank, Hawaiian Islands) characterized by open waters for viewing humpback whales, opportunities for vessel dispersion in Southeast Alaska are limited. All tours in Juneau depart out of a single port, Auke Bay, concentrating all whale-watching vessels in a tour area approximately 30 × 15 km in size (Teerlink et al., 2018). Throughout the summer, humpback whales vary in number and distribution throughout the region, which influences the number of vessels watching an individual or group at any given time. For example, bubble net feeding groups (i.e., highly conspicuous, and cooperative surface-feeding groups that release underwater bubble streams to corral schools of Pacific herring toward the surface, Sharpe, 2001), can attract as many as 30 vessels (Teerlink, 2017) at once. Furthermore, the long daylight hours during the Alaskan summer allows whale-watching vessels to operate for extended periods from 7:00 am to 9:00 pm. As a result, whales may be exposed to whale-watching vessels for the majority of the 24-h cycle. The sustainability of the Juneau whale-watching industry will likely have far-reaching and long-term ecological and economic effects throughout Southeast Alaska as some of the same humpback whales travel between Juneau and other popular tourist communities such as Ketchikan, Sitka, Hoonah, Skagway, and Glacier Bay (Hendrix et al., 2012).
In order to ensure that proper mitigation measures are in place to protect the resource upon which the industry relies, the goal of the present study was to assess the effects of whale-watching vessels on humpback whales in Juneau, Alaska. The objectives were to determine how humpback whale movement and behavioral patterns are affected by (1) vessel presence and the number of vessels present and (2) time spent in the presence of vessels, and to assess the effect of (3) vessel presence on humpback whale behavioral state transitions.
Materials and Methods
Data Collection
Land based observations occurred at Point Lena (58.39140 N, 134.77495 W) and Point Retreat (58.41308 N, 134.95644 W) (Figure 1). Data were collected using a Sokkia DT-5A theodolite connected to a laptop computer using the data acquisition software, Mysticetus1. Data collection required a team of at least 3 people, consisting of a theodolite operator, computer operator, and 1–2 spotters. Humpback whales were observed using a Konus 20 × 60 spotting scope and Leica 10 × 42 binoculars. Behavioral events and states were classified using an ethogram (Table 2). During each field day, environmental variables, including visibility, cloud cover, precipitation, and Beaufort sea state, were collected every hour or as conditions changed. Due to daily tidal fluctuations of up to 5 m, theodolite station height was measured approximately every 15 min using vertical markers on the shoreline. To ensure reliable data collection, observations were made only in Beaufort sea state of ≤3, little or no rain, and <15 kt wind.
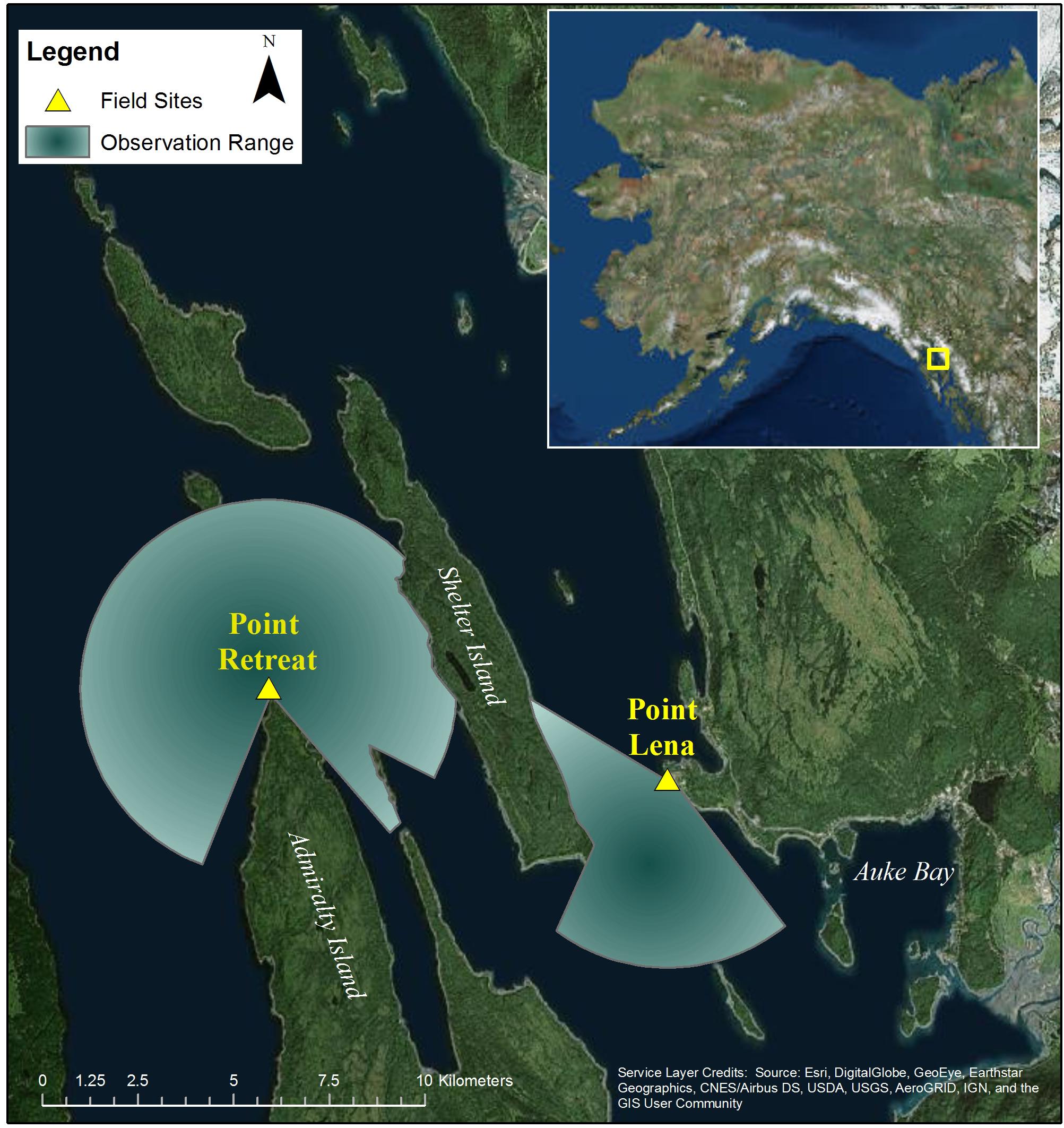
Figure 1. Map of Juneau whale watch tour area with field sites and corresponding observation ranges up to 5 km. Field sites were located at Point Lena (58.39140 N, 134.77495 W), at the NOAA Ted Steven’s Marine Research Institute, and Point Retreat (58.41308 N, 134.95644 W), at the lighthouse on the northern point of Admiralty Island. Most whale watching vessels depart from Auke Bay, with both sites located within the typical tour area. Each site was positioned at a high vantage point necessary for observing and tracking whales using a theodolite accurately up to 5 km (station height of 37.2 m, and 21.9 m, respectively).
Humpback whale movement and behavioral data with respect to whale-watching vessel presence were collected using theodolite tracking methods described in Würsig et al. (1991) and behavioral observation methods described in Di Clemente et al. (2018). A “sighting” (i.e., observation period of behaviors for a humpback whale) began when an individual was spotted < 5 km from the field site. Focal follow observations with all-occurrence sampling (Altmann, 1974) was used to record behavioral events (Table 2) and continuous sampling was used to record behavioral state, heading, and number of vessels present within a 500 m radius of the whale at the time of the behavioral event. A 500 m threshold was used previously by Di Clemente et al. (2018) as an intermediate distance between distance thresholds previously used in whale-watching studies on humpback whales [300 m (Corkeron, 1995; Morete et al., 2007) and 1000 m (Gulesserian et al., 2011; Schaffar et al., 2013)]. Vessels were considered present when within 500 m of the whale and observed approaching, departing, or tracking (i.e., following a whale in a straight line or in a parallel line according to the last heading observed of the whale). Simultaneously, the theodolite was used to obtain successive latitude/longitude coordinates of humpback whale positions, which were plotted as polyline tracks on Mysticetus. A “track” of the individual included all positions recorded along with time, distance from field site, and number of vessels within 500 m. Distinct physical markings (e.g., unique coloration, scarring, fluke notching, or dorsal fin shape) were recorded throughout the observation to aid in confirming the focal animal. If a second humpback whale was spotted < 5 km from the theodolite station, sighting and track information were collected on both individuals as long as accuracy was maintained. Groups > 1 whale (e.g., bubble net feeding groups and mother-calf pairs) were not included in the dataset because the focal animal could not be reliably determined throughout the follow. However, this excluded only a small portion of the dataset.
No limit was set for the duration of sightings or tracks. The mean dive time for humpback whales in Southeast Alaska is <10 min (Dolphin, 1987), therefore a sighting ceased once an individual went undetected for >20 min or had been absent from the study area >10 min. An individual was considered “absent” if it was out of range (i.e., >5 km from the theodolite, verified on Mysticetus) or out of sight due to trees or presence of islands. This distance has been recommended for stations between 20 and 45 m above mean low sea level to reduce errors in precision from targets at greater distances (Würsig et al., 1991; Piwetz et al., 2018). This method was similarly used by Di Clemente et al. (2018) to minimize the chance of sampling an individual that was different from the original focal animal.
In the summer of 2017, 20 companies advertised whale-watching tours or charters in Juneau, AK (Schuler, unpublished data). The industry included vessels ranging in size from 8 to 32 m and accommodating between 6 and 144 passengers. Each vessel departed from Auke Bay Harbor (Figure 1), conducting up to 3 tours per day that averaged 2.5–3 h each. In addition to these commercial whale-watching vessels, private vessels would also opportunistically track humpback whales.
Variables
Movement
Movement metrics
Successive positions of a humpback whale were used to obtain deviation, speed, and directionality and used to calculate changes in movement (Würsig et al., 1991). Deviation is the relative turning angle between three consecutive positions (Christiansen et al., 2013b). Deviation indicates path predictability for each position in the track by estimating the angle between the path taken and the straight-line path predicted (Williams et al., 2002; Christiansen et al., 2013b). Deviation ranges between 0° (linear movement) and 180° (erratic movement) (Christiansen et al., 2013b). Speed (kph) is the distance traveled between two coordinates divided by the time interval between those two coordinates (Lundquist et al., 2012). Directionality measured the linearity of movement of each surfacing in the track, calculated by dividing the distance between the two end-points of the specific section of the track by the actual distance of the track line of the section (Christiansen et al., 2013b). Directionality ranged between 0 (circular movement) and 1 (linear movement) (Christiansen et al., 2013b). Microsoft Excel (2016) was used to calculate deviation, speed, and directionality using data points recorded on Mysticetus.
Behavior
Behavioral metrics
Inter-breath interval (IBI) and respiration rate (RESP) were used to measure behavioral changes. Humpback whale IBIs were estimated as the time elapsed between two consecutive blows (Christiansen et al., 2013b). A fluke-up dive was typically associated with a longer IBI, therefore “Dive Type” for each interval was identified as “No fluke” or “Fluke-Up.” RESP (blows/min) was calculated by dividing the number of blows observed during a sighting by the total duration of the sighting.
Behavioral state transitions
Each behavioral event was categorized into one of four behavioral states (Table 2): feeding (FED), resting (RES), surface active behavior (SAB), and traveling (TRA). Over the course of a sighting, the behavioral states comprised a whale’s behavioral budgets [i.e., the proportion of time an animal spends in different behavioral states (Christiansen et al., 2013a)]. A change in behavioral state during a sighting was considered a behavioral transition. Each behavioral transition was categorized into ‘presence’ situations (i.e., observations in which ≥1 vessels were present <500 m of the focal whale) and ‘absence’ situations (i.e., observations in which no vessel was present <500 m radius of the focal whale).
Statistical Methods
Presence and Number of Whale-Watching Vessels (Obj. 1)
Regression analyses were performed using the open-source software R v.3.4.3 (R Core Team, 2018). To assess the significance of whale-watching vessels in explaining variations in deviation, speed, and IBI, linear mixed effects models (LMM) were fit by restricted maximum likelihood using the lme function in the R package nlme (Pinheiro et al., 2018). Covariates considered for the “base model” included behavioral state, location, time of day (indicated as a percentile of daylight hours, where sunrise = 0 and sunset = 1), Julian date, year, and environmental variables (i.e., visibility, Beaufort sea state) as fixed effects. Sighting distance associated with fixes (i.e., individual data records collected in Mysticetus) were included as an explanatory variable for deviation and speed analysis to account for potential vertical angle measurement errors associated with larger distances from shore. Time between fixes was included for deviation and dive type was included for IBI analysis to account for variability in direction changes and breathing intervals associated with longer dive times. Stepwise model selection based on the corrected Akaike Information Criterion (AICc) was used to select the combination of covariates that best explained the variations in each metric. As humpback whales may exhibit individually specific behaviors (Peterson, 2001), whale ID was included in each model as a random effect (intercept) to account for variability in mean deviation, speed, or IBI among individual whales. Two individuals (IDs 1538 and 1839 from the Alaska catalog) were seen frequently in the study area and could be readily identified based on their distinctive pigmentation on the underside of their flukes and dorsal fin shape. All observations of these identifiable individuals (i.e., 1538 and 1839) were included under the same unique identification numbers.
For each metric, a series of alternative models were used to test the possible effects of whale-watching vessels. The first model included vessel presence (vessels = 0 vs. vessels > 0) as a fixed effect to compare whale movement and behavior when vessels were present to those in which vessels were absent. The second model included the number of vessels as a linear fixed effect to understand how increasing numbers of vessels may influence movement and behavior. The third model included a categorical variable for the number of vessels to examine results for possible non-linearities and threshold effects. For deviation and speed, spatial autocorrelation was accounted for since measurements closer in space were likely to be more similar than those farther apart. For IBI, temporal autocorrelation was accounted for by including a first-order autoregressive term to model the possible dependence between consecutive residuals as εt = ϕ∗εt–1 + νt, where ϕ is the first-order auto-regressive coefficient and the errors (innovations νt) are assumed to follow a Gaussian distribution. Errors (νt) were examined for normality and homoscedasticity. The AICc was used to select the best-fit model while maintaining model parsimony.
Percent Time With Vessels (Obj. 2)
The percent time spent with vessels (PERC) was calculated by taking the sum of intervals spent in the presence of vessels and dividing it by the total length of the track or sighting. Linear regression was used to test for possible effects of PERC on directionality and RESP, while controlling for overall behavioral state (i.e., traveling, feeding, resting, surface active, and mixed), Julian date, year, location, and duration of track or sighting.
Behavioral State Transitions (Obj. 3)
To examine changes in whale activity budget with respect to whale-watching vessels, first-order Markov chain analyses were used to build transition matrices and run Monte Carlo simulations in situations in which whales were in the presence of vessels and in the absence of vessels (presence vs. absence). A first-order Markov chain was deemed appropriate for accounting for temporal autocorrelation while quantifying the dependence of a succeeding behavioral state (Lusseau, 2003). Based on the resulting contingency tables, presence and absence transition probability matrices were calculated (for details, see Di Clemente et al., 2018).
Monte Carlo simulations were also used to estimate the activity budgets of whales during vessel presence and absence situations (for details, see Christiansen et al., 2013a; Di Clemente et al., 2018). For presence and absence situations, simulations were repeated 1000 times, each representing a 7.5 h long time series either in the presence or the absence of whale-watching vessels. The resulting distribution was plotted using the relative proportion of each behavioral state in each situation. Initial model runs indicated that RES could not be included in Monte Carlo simulations due to its infrequent occurrence that resulted in a 100% transition probability. RES was therefore not included in the simulations.
Results
Data were collected on 147 days (111 days from Point Lena and 36 days from Point Retreat) from June through October 2016 and May through September 2017, totaling 843 h of effort (621 h from Point Lena, 223 h from Point Retreat) (Table 3). Only sightings consisting of ≥10 min of observed behaviors were included to ensure adequate IBI sample size. Tracks composed of ≥4 fixes were included to ensure at least two data points were included for deviation analysis. With these considerations, 201 humpback whale sightings and 178 whale tracks were included for analysis, totaling 162 h of behavioral observations (Table 3). The mean duration of a sighting was 49 ± 47.7 SD min (range = 12 – 447 min). The mean number of fixes in a track was 18 ± 24.3 (range = 4 – 213).
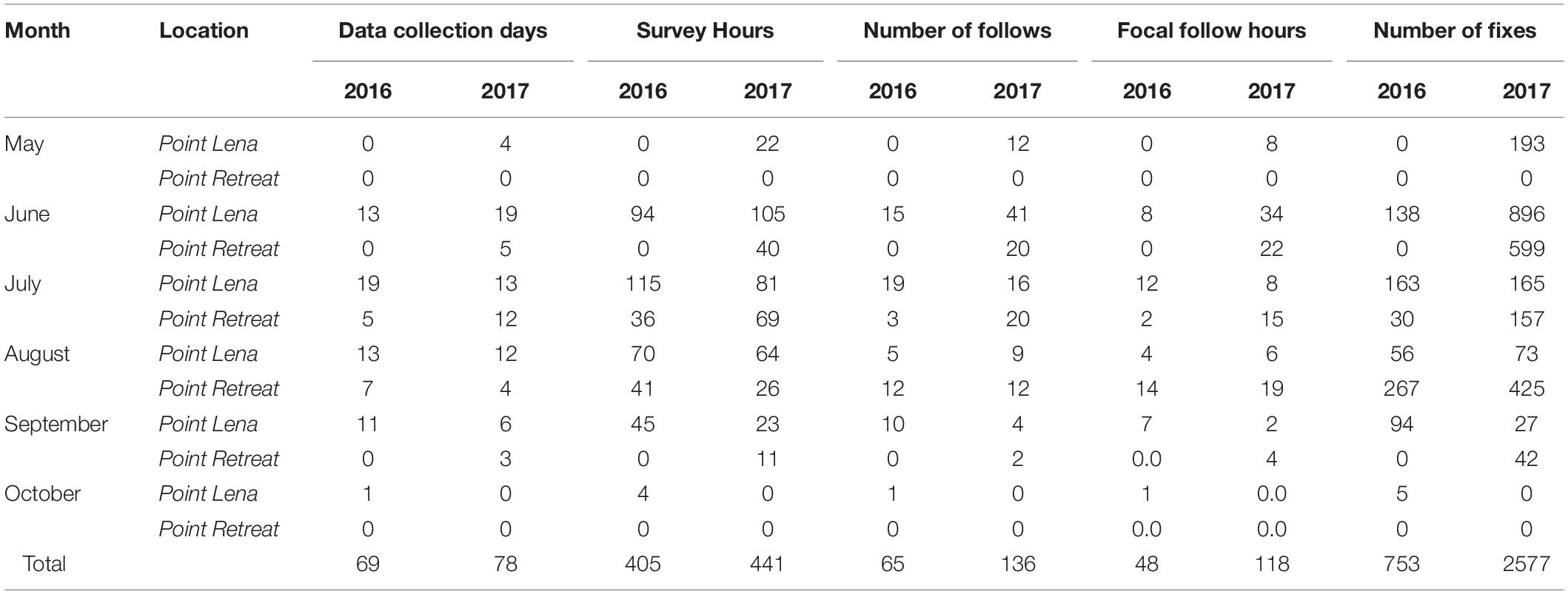
Table 3. Summary of research effort for single adult whales at Point Lena and Point Retreat for 2016 and 2017 field seasons.
Presence and Number of Vessels (Obj. 1)
Summary statistics for deviation, speed, and IBI were calculated in the presence and absence of vessels (Table 4). The mean number of vessels observed for each fix was 0.8 ± 1.41 (range = 0 – 8). In the presence of vessels, 59.9% of fixes included more than one vessel (n = 743). The estimated whale deviation from their straight-line course was 38.9% higher in the presence of vessels than in the absence of vessels based on the AICc-best model (p < 0.001, Table 5). Using the number of vessels instead of vessel presence in the same model resulted in an estimated increase in deviation by 6.2% per additional vessel (p = 0.022), but the model had less support (ΔAICc = 14.77). Whale swimming speed was 5.4% faster in the presence vs. absence of vessels (p = 0.047, Table 6). However, the number of vessels did not have a significant effect on speed. While IBI was 6.7% shorter in the presence vs. absence of vessels (p = 0.025), AICc model comparisons indicated that a linear effect of the number of vessels on IBI resulted in a better model (ΔAICc = 5.78), suggesting a 3.4% decrease in IBI for each additional vessel (p = 0.001, Table 7). The categorical model for number of vessels did not indicate an obvious threshold effect for deviation, speed or IBI.
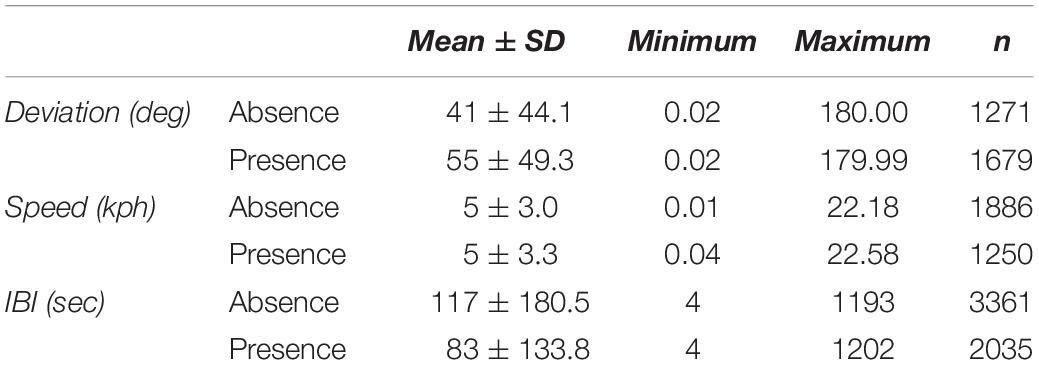
Table 4. Summary statistics for movement and behavioral metrics, deviation, speed, and inter-breath interval (IBI).
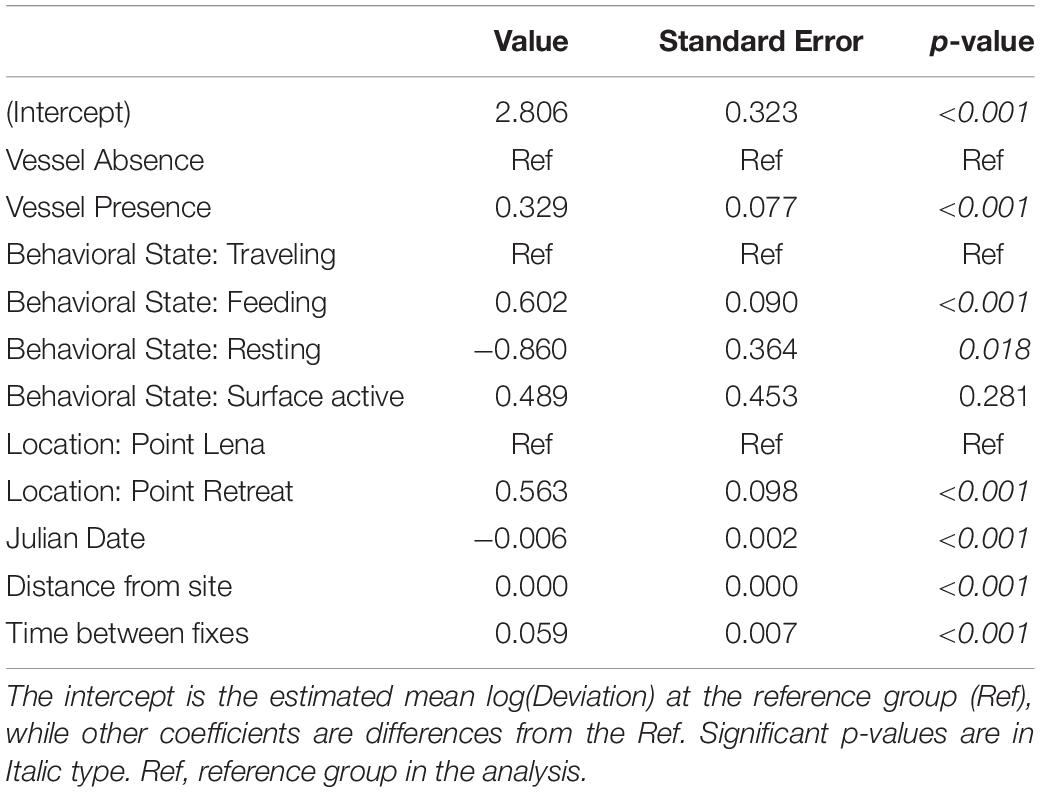
Table 5. Estimated coefficients and standard errors from the AICc-best linear mixed effects model of the effects of vessel presence on log(Deviation) (n = 2950 fixes) while controlling for behavioral state, location, Julian date, Beaufort sea state, distance from site, and time between fixes.
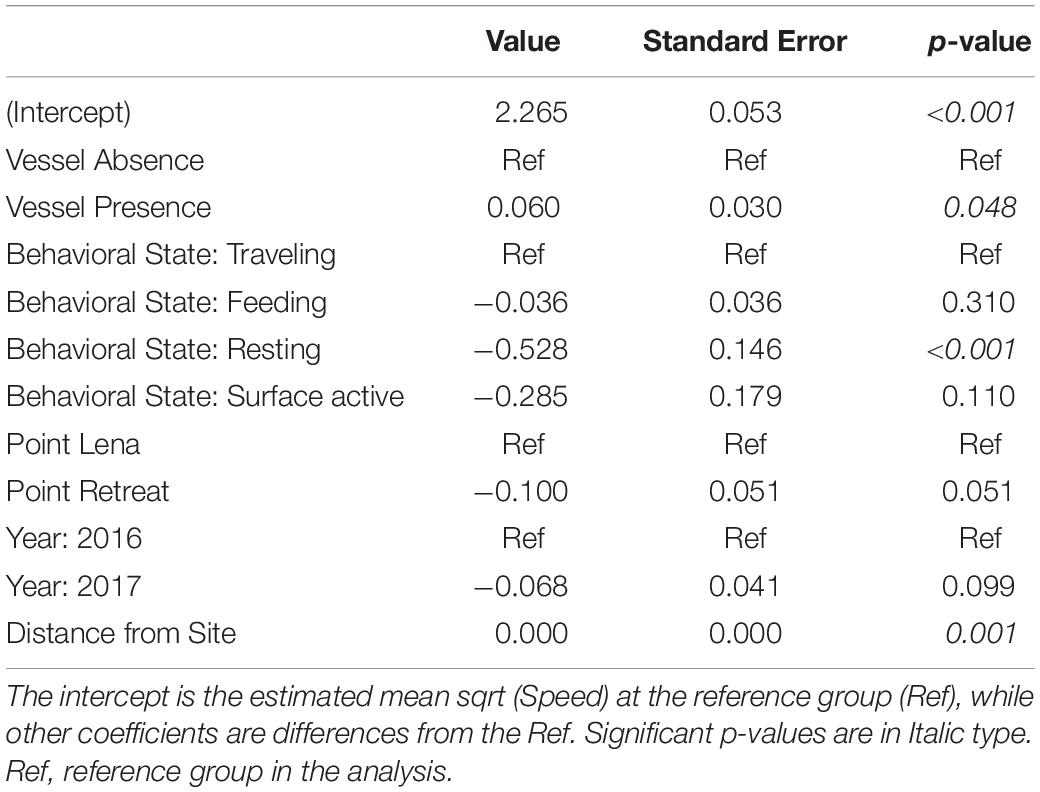
Table 6. Estimated coefficients and standard errors from the AICc-best linear mixed effects model of the effect of vessel presence on sqrt (Speed) (n = 3,136 fixes) while controlling for behavioral state, year, location, and distance from site.
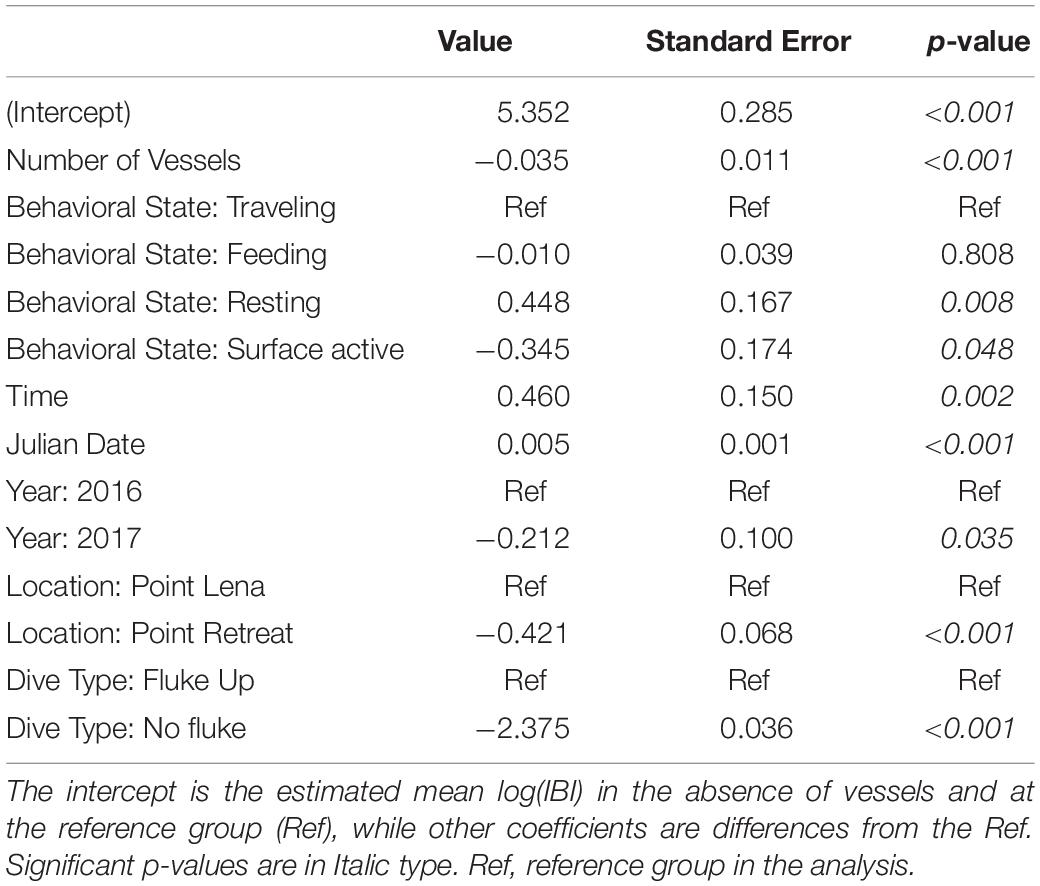
Table 7. Estimated coefficients and standard errors from the AICc-best linear mixed effects model of the simple linear effect of number of vessels on log(Inter-Breath Interval) (IBI) (n = 5,396 observations), while controlling for behavioral state, location, time, Julian date, year, and dive type.
Percent Time Spent With Vessels (Obj. 2)
The mean percent time humpback whales were observed in the presence of vessels was 33% ± 39.2% (range = 1–100%) during tracks and 29% ± 36.7% (range = 1–100%) during sightings. During 40% of tracks and 44% of sightings, no vessels were present. During 16% of tracks and 11% of sightings, vessels were present for the entire time. Humpback whale directionality averaged 0.72 ± 0.308 (range = 0.01–1.00, n = 177) and RESP averaged 0.55 ± 0.26) breaths/min (range = 0.16–1.52, n = 201). Percent time spent in the presence of vessels did not significantly affect directionality. However, the best fit model for RESP included percent time spent with boats, behavioral state, date, and location, and indicated that the more time a whale spent in the presence of vessels during a track, the higher the RESP (p = 0.011).
Behavioral State Transitions (Obj. 3)
A total of 5,489 behavioral events were recorded during 201 sightings (presence: 40.3%, n = 2213; absence: 59.7%, n = 3,276; Table 8). In the presence and absence of vessels, the predominant behavioral state observed was TRA (presence: 66.6%, absence: 76.1%), followed by FED (presence: 31.7%, absence: 21.5%), RES (presence: 1.4%, absence: 1.6%), and SAB (presence: 0.3%, absence: 0.8%). Markov chain analysis including TRA, FED, and SAB indicated that the transition probability between behavioral states differed by vessel presence (goodness-of-fit test, χ2 = 33.4; df = 4; p < 0.001). In the presence and absence of vessels, whales were likely to continue engaging in FED and TRA (FED → FED, presence = 96.7%, absence = 95.7%; TRA → TRA, presence = 98.2%, absence = 98.5%). In the presence of vessels, SAB more frequently transitioned to FED (SAB → FED, presence = 42.9%, absence = 3.7%) or TRA (SAB → TRA, presence = 14.3%, absence = 7.4%), while in the absence of vessels, whales were more likely to continue SAB (SAB → SAB, absence = 88.9%, presence = 42.9%).
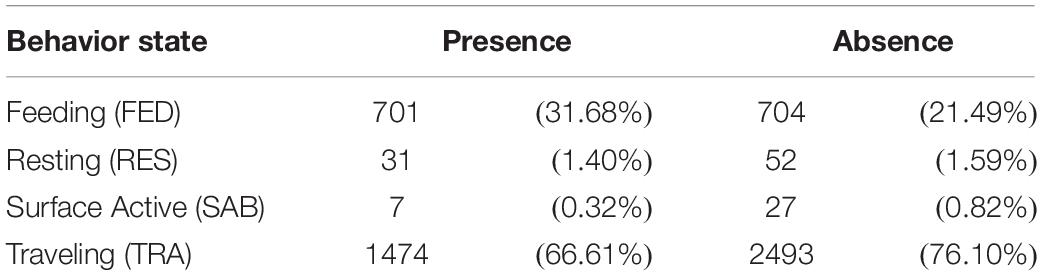
Table 8. Number of observations of whales in Juneau waters according to behavior states for presence (with whale watching vessels) and absence (without whale watching vessels) situations.
Monte Carlo simulations indicated that in the presence of vessels, the activity budgets of whales included less TRA and SAB (Figure 2). In the presence of whale-watching vessels, TRA composed 71.1% of the activity budget, compared to 80.7% in the absence of vessels, and SAB was 0.2% in the presence of vessels, compared to 0.9% in the absence of vessels. FED was also a large proportion of the activity budgets of whales, consisting of 28.6% in the presence of vessels and 18.4% in the absence (Table 9).
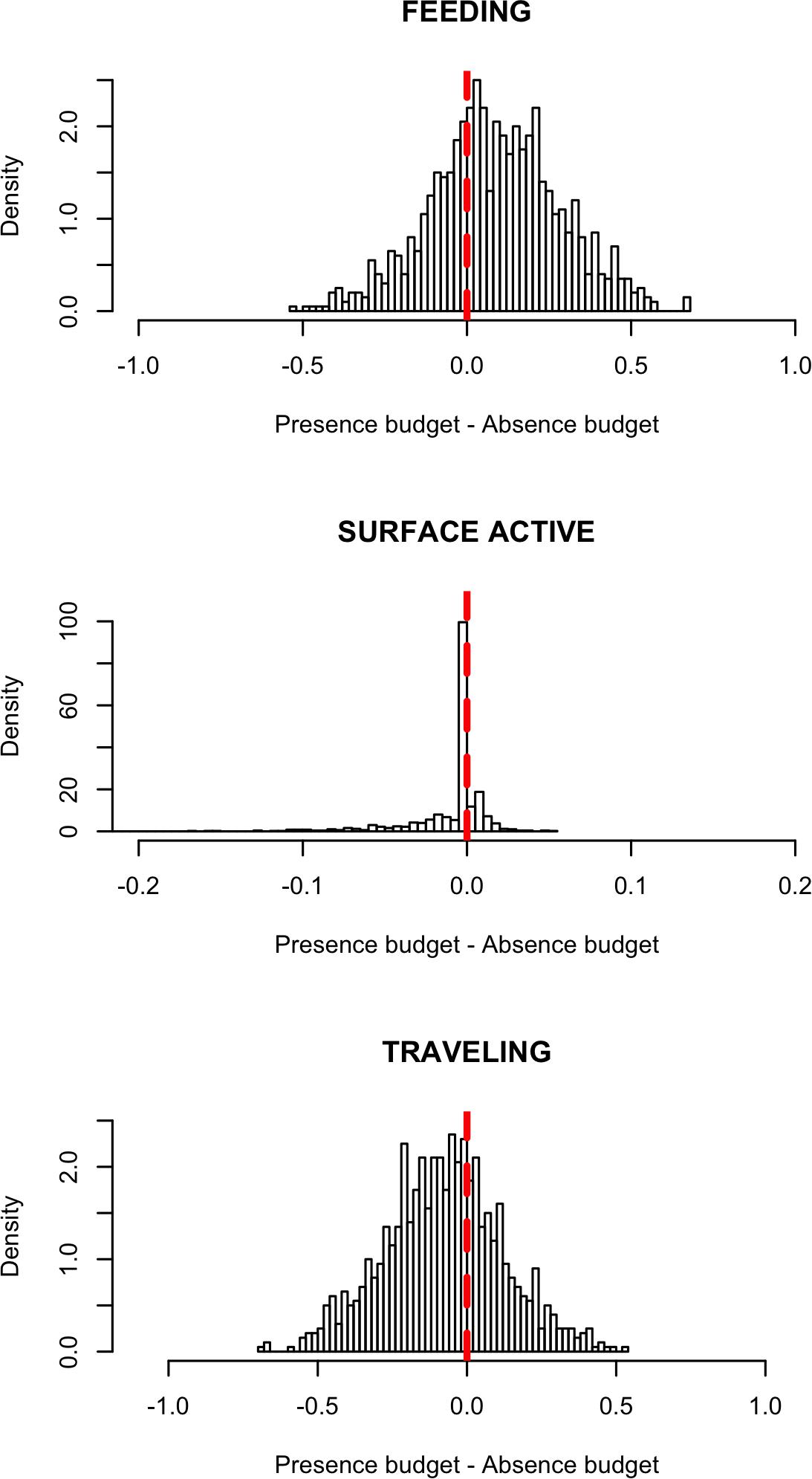
Figure 2. Difference in activity budgets (proportion of time spent in behavioral states) between vessel presence and vessel absence situations. The density distributions derive from 1000 Monte Carlo simulated sightings, each representing a 7.5-h long time series either in the presence or absence of whale-watching vessels. An increase in each state in the presence of vessels is indicated by a positive difference, whereas decrease in each state in the presence of vessels is shown as a negative difference. No difference is represented by red dashed lines.
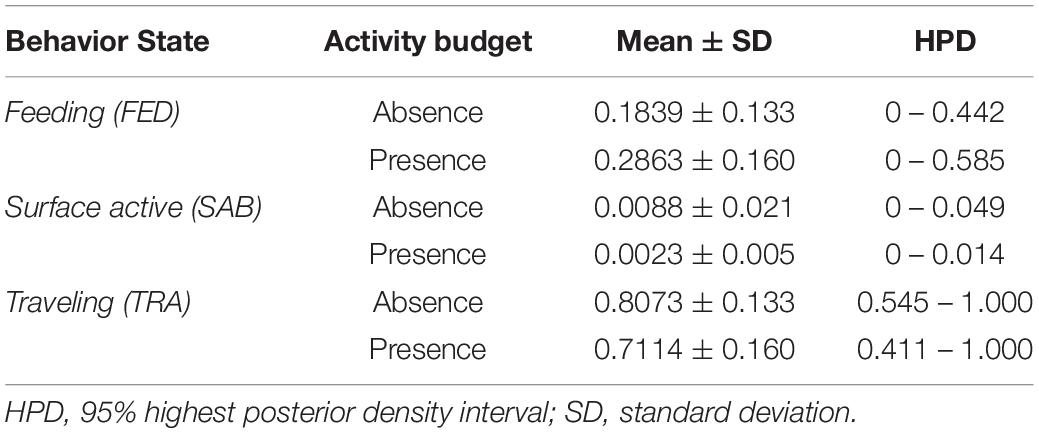
Table 9. Activity budgets of whales during vessel presence and vessel absence situations, estimated by Monte Carlo simulations.
Discussion
The results of this study reveal differences in humpback whale movement and behavior according to the presence of vessels, number of vessels, and time spent with vessels. Overall, in the presence of vessels, humpback whales demonstrated a larger deviation from their straight-line path, higher swimming speeds, and shorter IBIs. Higher deviation and shorter IBIs were also associated with the number of vessels, deviation significantly increasing and IBI significantly decreasing with each additional vessel present. A higher percentage of time spent with vessels was also correlated with higher RESP. Humpback whales that were feeding or traveling were likely to continue in those behavioral states whether in the presence or absence of vessels, but individuals were more likely to cease surface activity in the presence of vessels.
Whale-Watching Vessels (Obj. 1)
Horizontal movement metrics, such as changes in direction and increased swimming speed, are often used to identify vessel avoidance strategies by whales (Baker and Herman, 1989; Schaffar et al., 2013; Christiansen et al., 2014). The present study detected both a 38.5% higher deviation and 5% higher speed in the presence of vessels. Humpback whales have previously demonstrated increased path sinuosity (Schaffar et al., 2009; Stamation et al., 2010) and avoidance orientation in relation to vessels (Baker and Herman, 1989). Increased speeds in the presence of vessels has also been detected on humpback whale breeding grounds in the South Pacific (Schaffar et al., 2013) and South Atlantic (Morete et al., 2007). Direction changes and increased speeds are an energetically efficient strategy for humpback whales to avoid a perceived threat (Ford and Reeves, 2008; Senigaglia et al., 2016). While this ‘flight’ response may require less energy than a ‘fight’ response (i.e., surface-active behaviors) (Pitman et al., 2017), increased energetic expenditure spent avoiding vessels in highly trafficked areas, like Juneau, could be cumulative and decrease physical fitness over time.
Indicators of disturbance include changes in dive patterns and surfacing intervals (Bejder and Samuels, 2003). In the present study, shorter IBI in the presence of vessels indicates an increase in blow frequency. Furthermore, as the number of vessels increased, humpback whales exhibited significantly shorter IBI. These results support a meta-analysis by Senigaglia et al. (2016), which determined that mysticetes (i.e., baleen whales) tended to decrease IBI when whale-watching vessels were present. Previous studies in Juneau have also associated the presence of vessels with changes in breathing intervals (Baker et al., 1986) and increased variability in time spent at the surface (Peterson, 2001). Similarly, fin whales (Balaenoptera physalus) off the coast of Maine exhibited higher respiration rates and reduced dive durations, surface durations, and number of blows per surfacing sequence when whale-watching vessels were nearby (Stone et al., 1992). A decrease in IBI during interactions with whale-watching vessels can be of particular concern when it indicates a relative decrease in foraging efficiency, as seen in minke (B. acutorostrata) and fin whales (Christiansen et al., 2013b; Lesage et al., 2017). Furthermore, shorter IBI in the presence of vessels can reflect increased energetic expenditure and result in higher levels of oxygen consumption needed to recover (e.g., from increased speed to avoid vessels) (Christiansen et al., 2014).
Time Spent With Vessels (Obj. 2)
As the percent time spent with vessels increased, the respiration rate increased. Over the course of a track, whales may be disturbed by vessel noise of tracking whale-watching vessels (Richardson et al., 1995) due to the strong or rapid changes in engine and propeller speed (Watkins, 1986). Alterations in whale breathing patterns to persistent vessel exposure can indicate vessel avoidance of these sound levels. Multiple vessels simultaneously tracking a whale will accentuate this effect (Holt et al., 2009). Vessel characteristics (e.g., size and engine type) and vessel approach (e.g., angle and speed) are also likely to elicit different responses in whales. However, these factors could not be isolated due to the large proportion of humpback whale observations collected in the presence of >1 vessel. Furthermore, while the present study considered vessels present as those tracking whales within 500 m, it is likely that the vessel detection range for whales is >500 m (Baker and Herman, 1989; Richardson et al., 1995) as cetaceans respond to stimuli as far as tens of kilometers away (Baker and Herman, 1989; Richardson et al., 1995). Therefore, disturbance from vessel noise may be heightened in heavily trafficked areas, like Juneau. While not measured in the current study, information regarding detectable ranges of vessel engine sound in the tour area, and observations of whale movement and behavior with larger vessel distance thresholds, would help to validate this supposition.
Behavioral State Transitions (Obj. 3)
The present study reflects results from data collected in Juneau by Di Clemente et al. (2018), which demonstrated that humpback whale activity budgets in the presence of vessels consisted of less traveling and more feeding than in the absence of vessels. This could also reflect that whale-watching tours are more likely to select a feeding whale to watch over a traveling whale. However, humpback whales did not alter their feeding or traveling behaviors based on vessel presence. Previous research in Alaska has indicated that feeding humpback whales are less likely to alter their behavioral state in response to approaching vessels than non-feeding whales (Krieger and Wing, 1984, 1986; Peterson, 2001), suggesting that the need to build energy reserves while on the feeding grounds supersedes abandoning feeding even if disturbed. Responses may also occur beneath the surface, such as decreases in humpback whales feeding efficiency as seen with increased sound levels from vessels (Blair et al., 2016). By contrast, minke whales in Faxafloìi Bay, Iceland, decreased feeding behavior as a result of whale-watching vessel presence (Christiansen et al., 2013b). Differences in observed responses may vary by prey type, prey densities, and availability of food elsewhere (Krieger and Wing, 1986). Furthermore, the low number of recorded transitions between traveling and feeding could reflect the inability of shore-based observations to confirm humpback whales feeding at depth. Due to the importance of feeding in the area, it is possible that some straight, directional movement observed at the surface, could have also included feeding beneath the surface.
The only significant effect of vessel presence on humpback whale activity budgets was with respect to surface active behavior. Similar to Di Clemente et al. (2018), humpback whales in the absence of vessels were more than twice as likely to continue surface active behaviors, and whales in the presence of vessels were twice as likely to transition from surface active behavior to traveling. In other studies in Alaska, humpback whales increased surface active behaviors in the presence of vessels, potentially representing aggressive (e.g., tail breaching and lob tailing) and negative responses to vessels (Baker and Herman, 1989; Peterson, 2001). Transitions from vocal communication to non-vocal surface-generated communication (i.e., breaching, tail-slapping) by humpback whales have also been detected in noisy environments (i.e., increased background noise, high wind speeds) (Dunlop et al., 2010). However, surface active behavior has also been attributed to communication to distant groups (Kavanagh et al., 2017). This indicates that cessation of surface active behavior could result from acoustic masking due to vessel noise and subsequent inability to communicate (Richardson et al., 1995; Gabriele et al., 2018). However, due to the relatively small proportion of time whales spend resting and in surface active behaviors, in comparison to feeding and traveling, a larger sample size is needed to support the findings of the present study.
Potential Long-Term Effects
The present study did not directly address long-term consequences of vessel presence; however, it is possible that the measured short-term effects on whale movement and behavior could have cumulative effects on individuals and local populations in areas of high disturbance. Increased energetic expenditure and potential decreases in feeding efficiency, as evidenced by increased direction changes, higher traveling speeds, and changes in breathing and diving patterns, could have negative long-term effects. Prolonged energetic expenditure due to vessel avoidance behaviors may deplete energy reserves and disrupt homeostasis, ultimately causing physical fitness decline in whales (Beale, 2007). These physiological consequences, if extreme enough, could cause population decline, influencing reproductive success or decreasing chances of survival (Bejder and Samuels, 2003).
Humpback whales exhibit a high degree of feeding site fidelity (Calambokidis et al., 2001; Weinrich and Corbelli, 2009) to both general feeding areas (Baker et al., 1990) and to specific regions within the feeding grounds (Weinrich, 1998; Stevick et al., 2006). This may be an adaptive strategy to maximize prey intake by feeding on “known” prey resources that are predictable in time and space (Stevick et al., 2006). Humpback whales that remain in highly trafficked areas despite high exposure to vessel presence and associated stressors may do so because they rely on the resources from that area or they no longer perceive the presence of vessels as a threat.
Negative effects on whales may be minimized over time by habituation to vessel presence (Watkins, 1986). On the New England feeding grounds, Watkins (1986) determined that since whale watching began in 1976, humpback whales in the presence of vessels are more likely to continue their previous activity or respond by approaching vessels. Furthermore, despite high vessel pressure in the Juneau tour area, several individual humpback whales continue to return in subsequent years, often being observed multiple times a season (Teerlink, 2017). In a study by Teerlink et al. (2018), humpback whales in the Juneau area at the end of a whale-watching season (September) did not exhibit higher levels of the stress hormone, cortisol, compared to more remote regions. The lack of physiological stress responses detected in humpback whales in Juneau could indicate that repeated short-term disturbances may not be causing chronic stress. However, it is also possible that whales more sensitive to the presence of vessels had already left the tour area to avoid vessels, and the samples of whales in Juneau were biased toward whales more tolerant of vessels (Bejder et al., 2006a).
The expansion and persistence of vessel presence could result in alteration of humpback whale ranging patterns or habitat utilization. Changes in humpback whale distribution in an effort to avoid vessels could be detrimental not only to the whales, but to the profitability of the Juneau tour industry. In nearby Glacier Bay, habitat abandonment by humpback whales in response to vessel exposure has already been documented (Dean et al., 1985). Similarly, individual humpback whale singers in Brazil have responded to high vessel traffic by either moving out of recording range or discontinuing songs (Sousa-Lima and Clark, 2008). Bottlenose dolphins in Milford Sound, New Zealand have also adjusted their seasonal residency patterns in response to heavy vessel traffic (Lusseau, 2005).
Management Recommendations
Regulations were established in Alaska to prevent “take,” defined under the MMPA as an action to “harass, hunt, capture, or kill, or attempt to harass, hunt, capture, or kill any marine mammal.” The present study revealed that there are significant differences in short-term whale movement and behavioral patterns in the presence of vessels. This indicates that the current regulations, including to “not disrupt the normal behavior or prior activity of a whale” (Table 1), may not be adequate to reduce takes of humpback whales. Therefore, revisiting the humpback whale approach regulation and expanding the existing vessel approach limit to create a larger buffer around the whales should be considered by management to help reduce vessel impacts on humpback whales. The present study also determined that changes in deviation and IBI occurred in response to the number of vessels. Restrictions on the maximum number of vessels around whales or limits to the number of vessels in the industry does not currently exist. Managing the number of vessels watching whales would minimize vessel effects on whale movements and behavior.
The management approach in Alaska should continue to be supplemented with education programs encouraging operator participation in voluntary best practices. Whale SENSE was introduced in Alaska in 2015 (NOAA Fisheries Whale and Dolphin Conservation, 2018). In 2018, 44% of the companies that offered dedicated whale-watching tours in Juneau participated in the program, consisting of approximately 67% of vessels in the industry (Schuler, unpublished data). This program aims to minimize harassment of whales through the education of naturalists, captains, and passengers and promotion of responsible whale-watching practices. Programs such as Whale SENSE can help to manage tourist viewing expectations and encourage operator compliance (Filby et al., 2015). By combining evidenced-based regulations with effective educational programs, a sustainable whale-watching industry can be maintained.
Conclusion
The whale-watching industry in Juneau, Alaska, has grown alongside increasing humpback whale populations and rising tourist demand over the past 20 years. The present study revealed significant short-term differences in humpback whale movement and behavior in the presence of vessels that could negatively impact the health and predictability of the resource on which the industry relies. To determine if the short-term behavioral effects observed in this study manifest into long-term fitness consequences, the population should continue to be monitored. In the meantime, it is recommended that management bodies revisit the current regulations and guidelines and incorporate recent scientific research findings to ensure the sustainability of the industry. The precautionary principle, which should provide a buffer for scientific uncertainty (Reynolds et al., 2009), and proactive approaches should be implemented in order to ensure the viability of the whale-watching industry and the species upon which it depends.
Data Availability Statement
The datasets generated for this study are available on request to the corresponding author.
Author Contributions
AS collected and analyzed the data and wrote the manuscript. SP provided guidance on study design and data analysis, and wrote the manuscript. JD helped with study design, and data analysis, and wrote the manuscript. DS provided guidance on study design and data analysis, and reviewed the manuscript. FM helped with data analysis and wrote the manuscript. HP conceived the study, obtained funding, provided guidance on study design and data analysis, and wrote the manuscript.
Funding
This research was sponsored by Alaska Sea Grant, University of Alaska Fairbanks (project # R/112-05), with funds from the National Oceanic and Atmospheric Administration (Grant NA14OAR4170079), and from the University of Alaska with funds appropriated by the state of Alaska. Additional funds were provided by Alaska EPSCoR NSF (Grant Number OIA-1208927) and the NOAA Ernest F. Hollings Undergraduate Scholarship Program.
Conflict of Interest
DS was employed by company Mysticetus LLC.
The remaining authors declare that the research was conducted in the absence of any commercial or financial relationships that could be construed as a potential conflict of interest.
The reviewer BW declared a shared affiliation, with no collaboration, with several of the authors, AS, FM, and HP to the handling Editor at the time of review.
Acknowledgments
This project would not have been possible without data collection assistance by Chase Ammon, Madison Bargas, Taelor Brandon, Cody Crumpton, Dana Flerchinger, Holly Kelchner, Anneliese Moll, Breanna O’Farrell, Lindsey Overcast, Robert Schuler, and Margaret Sherman. Logistical support at the National Oceanic and Atmospheric Administration Ted Steven’s Marine Research Institute was provided by John Moran and Gordon Garcia, and access to the Point Retreat Lighthouse field site was generously provided by Dave Benton and Lori Tefler of the Alaska Lighthouse Association.
Footnotes
References
Atkinson, S., Crocker, D., Houser, D., and Mashburn, K. (2015). Stress physiology in marine mammals: how well do they fit the terrestrial model? J. Compar. Physiol. B 185, 463–486. doi: 10.1007/s00360-015-0901-0
Baker, C. S., and Herman, L. M. (1989). Behavioral Responses of Summering Humpback Whales to Vessel Traffic: Experimnetal and Opportunistic Observations. Anchorage, AK: U.S. Department of the Interior.
Baker, C. S., Herman, L. M., Perry, A., Lawton, W. S., Straley, J. M., Wolman, A. A., et al. (1986). Migratory movement and population structure of humpback whales (Megaptera novaeanglieae) in the central and eastern North Pacific. Mar. Ecol. Prog. Ser. 31, 105–119. doi: 10.3354/meps031105
Baker, C. S., Palumbi, S. R., Lambertsen, R. H., Weinrich, M. T., Calambokidis, J., and O’Bien, S. J. (1990). Influence of seasonal migration on geographic distribution of mitochondrial DNA haplotypes in humpback whales. Nature 344, 238–240. doi: 10.1038/344238a0
Baker, C. S., Steel, D., Calambokidis, J., Falcone, E. A., Gonzalez-Peral, U., Barlow, J., et al. (2013). Strong maternal fidelity and natal philopatry shape genetic structure in North Pacific humpback whales. Mar. Ecol. Prog. Ser. 494, 291–296.
Beale, C. M. (2007). The behavioral ecology of disturbance responses. Int. J. Compar. Psychol. 20, 111–120.
Bejder, L., and Samuels, A. (2003). “Evaluating the effects of nature-based tourism on cetaceans,” in Marine Mammals: Fisheries, Tourism and Management Issues eds. N. Gales, M. Hindell, and R. Kirkwood (Victoria: CSIRO Publishing), 229–256.
Bejder, L., Samuels, A., Whitehead, H., and Gales, N. (2006a). Interpreting short-term behavioural responses to disturbance within a longitudinal perspective. Ani. Behav. 72, 1149–1158. doi: 10.1016/j.anbehav.2006.04.003
Bejder, L., Samuels, A., Whitehead, H., Gales, N., Mann, J., Connor, R., et al. (2006b). Decline in relative abundance of bottlenose dolphins exposed to long-term disturbance. Conserv. Biol. 20, 1791–1798. doi: 10.1111/j.1523-1739.2006.00540.x
Best, P. B. (1993). Increase rates in severely depleted stocks of baleen whales. ICES J. Mar. Sci. 50, 169–186. doi: 10.1006/jmsc.1993.1018
Blair, H. B., Merchant, N. D., Friedlaender, A. S., Wiley, D. N., and Parks, S. E. (2016). Evidence for ship noise impacts on humpback whale foraging behaviour. Biol. Lett. 12:20160005. doi: 10.1098/rsbl.2016.0005
Calambokidis, J., Steiger, G., Straley, J., Herman, L., Cerchio, S., Salden, D., et al. (2001). Movements and population structure of humpback whales in the North Pacific. Mar. Mamm. Sci. 17, 769–794.
Carlson, C. (2013). A Review of Whale Watch Guidelines and Regulations Around the World Version 2012 [Online]. Report Submitted to the International Whaling Commission. Available at: https://iwc.int/index.php?cID=3107&cType=document (accessed December 2017).
Cartwright, R., Gillespie, B., Labonte, K., Mangold, T., Venema, A., Eden, K., et al. (2012). Between a rock and a hard place: habitat selection in female-calf humpback whale (Megaptera novaeangliae) pairs on the Hawaiian breeding grounds. PLoS One 7:e38004. doi: 10.1371/journal.pone.0038004
Christiansen, F., Rasmussen, M. H., and Lusseau, D. (2013a). Inferring activity budgets in wild animals to estimate the consequences of disturbances. Behav. Ecol. 24, 1415–1425. doi: 10.1093/beheco/art086
Christiansen, F., Rasmussen, M., and Lusseau, D. (2013b). Whale watching disrupts feeding activities of minke whales on a feeding ground. Mar. Ecol. Prog. Ser. 478, 239–251. doi: 10.3354/meps10163
Christiansen, F., Rasmussen, M. H., and Lusseau, D. (2014). Inferring energy expenditure from respiration rates in minke whales to measure the effects of whale watching boat interactions. J. Exp. Mar. Biol. Ecol. 459, 96–104. doi: 10.1016/j.jembe.2014.05.014
Corkeron, P. J. (1995). Humpback whales Megaptera novaeangliae in hervey bay, queensland: behaviour and responses to whale-watching vessels. Can. J. Zool. 73, 1290–1299. doi: 10.1139/z95-153
Dean, F. C., Jurasz, C. M., Palmer, V. P., Curby, C. H., and Thomas, D. L. (1985). Analysis of Humpback Whale (Megaptera Novaeangliae) Blow Interval Data/Glacier Bay Alaska, 1976-1979. Fairbanks, AK: US National Park Service.
Di Clemente, J., Christiansen, F., Pirotta, E., Steckler, D., Wahlberg, M., and Pearson, H. C. (2018). Effects of whale watching on the activity budgets of humpback whales, megaptera novaeangliae (borowski, 1781), on a feeding ground. Aquat. Conserv. 28, 1–11. doi: 10.1002/aqc.2909
Dolphin, W. F. (1987). Ventilation and dive patterns of humpback whales, megaptera novaeangliae, on their alaskan feeding grounds. Can. J. Zool. 65, 83–90. doi: 10.1242/jeb.023366
Dunlop, R. A., Cato, D. H., and Noad, M. J. (2010). Your attention please: increasing ambient noise levels elicits a change in communication behaviour in humpback whales (Megaptera novaeangliae). Proc. R. Soc. B 227, 2521–2529. doi: 10.1098/rspb.2009.2319
Filby, N. E., Stockin, K. A., and Scarpaci, C. (2015). Social science as a vehicle to improve dolphin-swim tour operation compliance? Mar. Policy 51, 40–47. doi: 10.1016/j.marpol.2014.07.010
Ford, J. K., and Reeves, R. R. (2008). Fight or flight: antipredator strategies of baleen whales. Mamm. Rev. 38, 50–86. doi: 10.1111/j.1365-2907.2008.00118.x
Gabriele, C. M., Ponirakis, D., Clark, C. W., Womble, J., and Vanselow, P. B. S. (2018). Underwater acoustic ecology metrics in an alaska marine protected area reveal marine mammal communication masking and management alternatives. Front. Mar. Sci. 5:17. doi: 10.3389/fmars.2018.00270
Gulesserian, M., Slip, D., Heller, G., and Harcourt, R. (2011). Modelling the behaviour state of humpback whales Megaptera novaeangliae in response to vessel presence off sydney Australia. Endanger. Species Res. 15, 255–264. doi: 10.3354/esr00380
Hendrix, A. N., Straley, J., Gabriele, C. M., Gende, S. M., and Chen, Y. (2012). Bayesian estimation of humpback whale (Megaptera novaeangliae) population abundance and movement patterns in southeastern Alaska. Can. J. Fish. Aquat. Sci. 69, 1783–1797. doi: 10.1139/f2012-101
Holt, M. M., Noren, D. P., Veirs, V., Emmons, C. K., and Viers, S. (2009). Speaking up: killer whales (Orcinus orca) increase their call amplitude in response to vessel noise. J. Acous. Soc. Am. 125, EL27–EL32. doi: 10.1121/1.3040028
Hoyt, E., and Parsons, E. C. M. (2014). “The whale-watching industry: historical development,” in Whale-Watching, Sustainable Tourism, and Ecological Management, eds J. Higham, L. Bejder, and R. Williams (New York, NY: Cambridge University Press), 57–70. doi: 10.1017/cbo9781139018166.006
Irvine, L. G., Thums, M., Hanson, C. E., McMahon, C. R., and Hindell, M. A. (2017). Quantifying the energy stores of capital breeding humpback whales and income breeding sperm whales using historical whaling records. R. Soc. Open Sci. 4:160290. doi: 10.1098/rsos.160290
Kavanagh, A. S., Owen, K., Williamson, M. J., Blomberg, S. P., Noad, M. J., Goldizen, A. W., et al. (2017). Evidence for the functions of surface-active behaviors in humpback whales (Megaptera novaeangliae). Mar. Mamm. Sci. 33, 313–334. doi: 10.1111/mms.12374
Krieger, K. J., and Wing, B. L. (1984). Hydroacoustic Surveys and Identification of humpback Whale Forage in Glacier Bay, Stephens Passage, and Frederick Sound, Southeastern Alaska. Summer 1983. Auke Bay, AK: National Marine Fisheries Service.
Krieger, K. J., and Wing, B. L. (1986). Hydroacoustic Monitoring of Prey to Determine Humpback Whale Movements NOAA Technical Memorandum NMFS F/NWC-98. Auke Bay, AK: National Marine Fisheries Service.
Lesage, V., Omrane, A., Doniol-Valcroze, T., and Mosnier, A. (2017). Increased proximity of vessels reduces feeding opportunities of blue whales in the st. lawrence Estuary, Canada. Endanger. Species Res. 32, 351–361. doi: 10.3354/esr00825
Lopez, G., and Pearson, H. C. (2017). Can whale watching be a conduit for spreading educational and conservation messages? A case study in Juneau, Alaska. Tourism Mar. Environ. 12, 95–104. doi: 10.3727/154427316X14779456049821
Lundquist, D., Gemmell, N. J., and Wursig, B. (2012). Behavioural responses of dusky dolphin groups (Lagenorhynchus obscurus) to tour vessels off kaikoura, New Zealand. PLoS One 7:e41969. doi: 10.1371/journal.pone.0041969
Lusseau, D. (2003). Effects of tour boats on the behavior of bottlenose dolphins: using markov chains to model anthropogenic impacts. Conserv. Biol. 17, 1765–1793.
Lusseau, D. (2005). Residency pattern of bottlenose dolphins tursiops spp. in milford sound, new zealand is related to boat traffic. Mar. Ecol. Prog. Ser. 295, 265–272. doi: 10.3354/meps295265
Lusseau, D., and Bejder, L. (2007). The long-term consequences of short-term responses to disturbance experiences from whalewatching impact assessment. Int. J. Comp. Psychol. 20, 228–236.
Morete, M. E., Bisi, T. L., and Rosso, S. (2007). Mother and calf humpback whale responses to vessels around the abrolhos archipelago, bahia, Brazil. Cetacean Resour. Manag. 9, 241–248.
Muto, M. M., Helker, V. T., Angliss, R. P., Allen, B. A., Boveng, P. L., Breiwick, J. M., et al. (2017). “Alaska marine mammal stock assessments, 2016,” in NOAA Technical Memorandum NMFS-AFSC-355, (Seattle, WA: U.S. Department of Commerce).
Muto, M. M., Helker, V. T., Angliss, R. P., Allen, B. A., Boveng, P. L., Breiwick, J. M., et al. (2018). “Alaska marine mammal stock assessments, 2017”, in NOAA Technical Memorandum NMFS-AFSC-378, (Seattle, WA: U.S. Department of Commerce).
National Oceanic and Atmospheric Administration, (2016). Endangered and Threatened Species; Identification of 14 Distinct Population Segments of the Humpback Whale (Megaptera novaeangliae) and Revision of Species-Wide Listing. Washington, D.C: U.S. Deartment of Commerce.
New, L. F., Hall, A. J., Harcourt, R., Kaufman, G., Parsons, E. C. M., Pearson, H. C., et al. (2015). The modelling and assessment of whale-watching impacts. Ocean Coast. Manag 115, 10–16. doi: 10.1016/j.ocecoaman.2015.04.006
NOAA Fisheries Whale and Dolphin Conservation, (2018). Whale SENSE: Promoting Responsible Whale Watching. Available at: www.whalesense.org (accessed November 1, 2018).
O’Connor, S., Campbell, R., Cortez, H., and Knowles, T. (2009). Whale Watching Worldwide: Tourism Numbers, Expenditures and Expanding Economic Benefits. Yarmouth MA: IFAW.
Parsons, E. C. M. (2012). The negative impacts of whale-watching. J. Mar. Biol. 2012:807294. doi: 10.1155/2012/807294
Peterson, H. A. (2001). Whale Behavioral Responses and Human Perceptions: An Assessment of Humpback Whales (Megaptera novaeanglie) and Vessel Activity Near Juneau, Alaska. Durham: Duke University.
Pinheiro, J., Bates, D., DebRoy, S., and Sarkar, D. R Core Team, (2018). nlme: Linear and Nonlinear Mixed Effects Models in: R Package Version 3.1-137.
Pitman, R. L., Deecke, V. B., Gabriele, C. M., Srinivasan, M., Black, N., Denkinger, J., et al. (2017). Humpback whales interfering when mammal-eating killer whales attack other species: mobbing behavior and interspecific altruism? Mar. Mamm. Sci. 33, 7–58. doi: 10.1111/mms.12343
Piwetz, S., Gailey, G., Munger, L., Lammers, M. O., Jefferson, T. A., and Würsig, B. (2018). Theodolite tracking in marine mammal reserach: from roger payne to the present. Aquat. Mamm. 44, 683–693. doi: 10.1578/AM.44.6.2018.683
R Core Team, (2018). R: A Language and Environment for Statistical Computing. Vienna: R Foundation for Statistical Computing.
Reynolds, J. E. III, Marsh, H., and Ragen, T. J. (2009). Marine mammal conservation. Endanger. Species Res. 7, 23–28. doi: 10.3354/esr00179
Richardson, J. W., Greene, C. Jr., Malme, C., and Thomson, D. (1995). Marine Mammals and Noise. San Diego: Academic Press.
Schaffar, A., Madon, B., Garrigue, C., and Constantine, R. (2009). “Avoidance of whale watching boats by humpback whales in their main breeding ground in New Caledonia,” in Report Presented to the Scientific Committee of the International Whaling Commission SC/61/WW6.
Schaffar, A., Madon, B., Garrigue, C., and Constantine, R. (2013). Behavioural effects of whale-watching activities on an endangered population of humpback whales wintering in New Caledonia. Endanger. Species Res. 19, 245–254. doi: 10.3354/esr00466
Scheidat, M., Castro, C., Gonzalez, J., and Williams, R. (2004). Behavioural responses of humpback whale (Megaptera novaeangliae) to whalewatching boats near Isla de la plata. machalilla National Park, ecuador. J. Cetacean Resour. Manag. 6, 63–68.
Senigaglia, V., Christiansen, F., Bejder, L., Gendron, D., Lundquist, D., Noren, D. P., et al. (2016). Meta-analyses of whale-watching impact studies: comparisons of cetacean responses to disturbance. Mar. Ecol. Prog. Ser. 542, 251–263. doi: 10.3354/meps11497
Sharpe, F. A. (2001). Social Foraging of the Southeast Alaskan Humpback Whale Megaptera novaeangliae. Doctor of Philosophy. Burnaby, BC: Simon Fraser University.
Sousa-Lima, R. S., and Clark, C. W. (2008). Modeling the effect of boat traffic on the fluctuation of humpback whale singing activity in the Abrolhos National Marine Park. Brazil Can. Acoust. 36, 174–181.
Stamation, K. A., Croft, D. B., Shaughnessy, P. D., Waples, K. A., and Briggs, S. V. (2010). Behavioral responses of humpback whales (Megaptera novaeangliae) to whale-watching vessels on the southeastern coast of Australia. Mar. Mamm. Sci. 26, 98–122. doi: 10.1111/j.1748-7692.2009.00320.x
Steckenreuter, A., Moller, L., and Harcourt, R. (2012). How does Australia’s largest dolphin-watching industry affect the behaviour of a small and resident population of Indo-Pacific bottlenose dolphins? J. Environ. Manag. 97, 14–21. doi: 10.1016/j.jenvman.2011.11.002
Stevick, P. T., Allen, J., Clapham, P. J., Katona, S. K., Larsen, F., Lien, J., et al. (2006). Population spatial structuring on the feeding grounds in North Atlantic humpback whales (Megaptera novaeangliae). J. Zool. 270, 244–255. doi: 10.1111/j.1469-7998.2006.00128.x
Stone, G. S., Katona, S. K., Mainwaring, A., Allen, J. M., and Corbett, H. D. (1992). Respiration and Surfacing Rates Of Fin Whales (Balaenoptera physalus) Observed From a Lighthouse Tower. Cambridge: International Whaling Commision.
Teerlink, S. (2017). Humpback Whales And Humans: a Multi-Disciplinary Approach to Exploring The Whale-Watching Industry in Juneau, Alaska. Fairbanks, AK: Unviersity of Alaska Fairbanks.
Teerlink, S., Horstmann, L., and Witteveen, B. (2018). Humpback whale (Megaptera novaeangliae) blubber steroid hormone concentration to evaluate chronic stress response from whale-watching vessels. Aquat. Mamm. 44, 411–425. doi: 10.1578/AM.44.4.2018.411
Watkins, W. A. (1986). Whale reactions to human activites in cape cod waters. Mar. Mamm. Sci. 2, 251–262. doi: 10.1111/j.1748-7692.1986.tb00134.x
Weingartner, T., Eisner, L., Eckert, G. L., Danielson, S., and Bellwood, D. (2009). Southeast alaska: oceanographic habitats and linkages. J. Biogeogr. 36, 387–400. doi: 10.1111/j.1365-2699.2008.01994.x
Weinrich, M. (1998). Early experience in habitat choice by humpback whales (Megaptera novaeangliae). J. Mammal. 79, 163–170. doi: 10.2307/1382851
Weinrich, M. T., and Corbelli, C. (2009). Does whale watching in Southern New england impact humpback whale (Megaptera novaeangliae) calf production or calf survival? Biol. Conserv. 142, 2931–2940. doi: 10.1016/j.biocon.2009.07.018
Whale and Dolphin Conservation, (2015). WDC Ethogram- Humpback Whale Behavior. Available at: https://whalesenseblog.files.wordpress.com/2015/04/ethogram_mn2.pdf (accessed Ocotober 18, 2018).
Williams, R., Trites, A. W., and Bain, D. E. (2002). Behavioural responses of killer whales (Orcinus orca) to whale-watching boats: opportunistic observations and experimental approaches. J. Zool. 256, 255–270. doi: 10.1017/s0952836902000298
Keywords: whale watching, tourism effects, disturbance, humpback whale, movement, Alaska, regulation, behavior
Citation: Schuler AR, Piwetz S, Di Clemente J, Steckler D, Mueter F and Pearson HC (2019) Humpback Whale Movements and Behavior in Response to Whale-Watching Vessels in Juneau, AK. Front. Mar. Sci. 6:710. doi: 10.3389/fmars.2019.00710
Received: 23 June 2019; Accepted: 05 November 2019;
Published: 20 November 2019.
Edited by:
Lyne Morissette, M – Expertise Marine, CanadaReviewed by:
Benjamin de Montgolfier, Independent Researcher, Sainte-Luce, MartiniqueBree Witteveen, University of Alaska Fairbanks, United States
Copyright © 2019 Schuler, Piwetz, Di Clemente, Steckler, Mueter and Pearson. This is an open-access article distributed under the terms of the Creative Commons Attribution License (CC BY). The use, distribution or reproduction in other forums is permitted, provided the original author(s) and the copyright owner(s) are credited and that the original publication in this journal is cited, in accordance with accepted academic practice. No use, distribution or reproduction is permitted which does not comply with these terms.
*Correspondence: Alicia R. Schuler, YXJzY2h1bGVyQGFsYXNrYS5lZHU=