- 1Laboratorio de Ecología Experimental, Departamento de Estudios Ambientales, Universidad Simón Bolívar, Caracas, Venezuela
- 2Grupo de Investigación y Desarrollo en Mecatrónica, Universidad Simón Bolívar, Caracas, Venezuela
- 3EDGE of Existence Program, Conservation and Policy, Zoological Society of London, London, United Kingdom
Global and local stressors are causing the worldwide loss of coral cover and structural complexity at an unprecedented pace on reefs. In consequence, the habitat of coral reef fish has suffered a profound degradation affecting the abundance, biodiversity and species composition of this taxonomic group. Thus, understanding the link between coral reef fish assemblages and their habitats is paramount to predict their responses to increasing human threats. Herein, we implemented Structure from Motion (SfM) techniques and digital mosaics to characterize the habitat of reef fish in terms of structural complexity and cover of benthic organisms, and we examined the relationships between these metrics and the variation in fish assemblages among sites using a multivariate approach. We found that attribute of fish assemblage varied across reef sites in Los Roques, depending on the highly specific features of the benthic habitat. Results indicate that 69% of the variation in species-specific abundances of fish (i.e., reef fish assemblage structure) was explained by cover of massive coral and turf algae, the number and sizes of holes, and the site. Furthermore, when fish biomass per species was utilized as a response variable, 64% of the variation in assemblage structures was explained by a model that included: cover of crustose coralline algae (CCA), variation and the maximum height of reef structures along the transect, the number of holes and the site. All these variables together also explained >60% of variation of total abundance, biomass and species richness. When data were sorted by trophic groups, CCA cover explained 70% of the variation in forager biomass, whereas the number of holes explained up to 60% of variation in carnivore biomass. These results suggest that each trophic group relates differently to the benthic habitat. We conclude that variation in fish assemblages among sites can be explained by features of the benthic habitat, but more importantly the absence of specific attributes may impact fish trophic groups differently.
Introduction
The role of habitat complexity in shaping ecological communities has been a major topic of research for decades and continues to be prominent. It is hypothesized that structurally complex habitats may provide more niches and diverse ways of exploiting environmental resources thus increasing local species diversity (Huston and DeAngelis, 1994; Willis et al., 2005). However, whilst there is copious evidence supporting this hypothesis in both terrestrial and marine ecosystems (Tews et al., 2004), the relative importance of different attributes of complexity in determining species diversity and small-scale heterogeneity in biodiversity and species composition remains poorly understood (St. Pierre and Kovalenko, 2014).
In marine ecosystems, there are multiple examples illustrating the key role structural complexity plays in determining the structure of ecological communities (Beck, 2000; Angel and Ojeda, 2001; Laegdsgaard and Johnson, 2001). This relationship is particularly strong for coral reefs, where the habitat is provided by sessile organisms such as scleractinian corals, which have a variety of shapes that provide a highly complex physical structure (Laforsch et al., 2008). In fact, a high diversity of fishes has been associated with the highly heterogeneous nature of these habitats at multiple spatial scales (Sale, 1977; Connell, 1978; Sale and Douglas, 1984). Furthermore, literature provides plenty of examples showing positive relationships between live coral cover, structural complexity, and attributes of fish assemblages (Risk, 1972; Luckhurst and Luckhurst, 1978; Birkeland and Neudecker, 1981; Bell and Galzin, 1984; Alvarez-Filip et al., 2011; Coker et al., 2012). The study of these relationships seems to be more important currently because coral reefs are losing coral cover and structural complexity worldwide as a consequence of multiple human-made stressors (i.e., human induced climate change, overfishing, pollution), and this would probably have negative effects on reef fish assemblages (Hughes et al., 2003; Jones et al., 2004; Munday et al., 2008; Pratchett et al., 2008; Alvarez-Filip et al., 2009; Jackson et al., 2014).
Over the past 40 years, the relationship between reef fishes and coral substrate has been studied extensively (Risk, 1972; Luckhurst and Luckhurst, 1978; Bell and Galzin, 1984; Chong-Seng et al., 2012; Coker et al., 2012; Komyakova et al., 2013). Studies focusing on reef fish-substrate relationships have provided valuable information derived from “in situ” estimations, using quadrats or line transects to determine benthic cover and chain link or qualitative estimations to determine structural complexity (i.e., rugosity) (Risk, 1972; Luckhurst and Luckhurst, 1978). However, these methods require investing a considerable amount of time in the field and, most importantly, reduce the structural complexity to a single variable (i.e., rugosity), underestimating its many dimensions (Agudo-Adriani et al., 2016). In the last decade, the development of technological advances have brought more affordable underwater photography and video equipment, allowing the development of new techniques that complement data acquisition in the field with data that can be analyzed in the laboratory in a more detailed fashion (Lirman et al., 2007; Reid et al., 2010; Burns J. et al., 2015; Ferrari et al., 2016). For instance, photomosaics can be used to determine benthic community structure from digital images (Lirman et al., 2007), and more recently, it is possible to generate three-dimensional reconstructions of the substrate and its elements using “Structure from Motion (SfM)” (Westoby et al., 2012; Burns J. et al., 2015; Lavy et al., 2015; Ferrari et al., 2016).
While these methods have well-identified limitations (Ferrari et al., 2016), they could offer a better approximation of the structural complexity of coral reefs in comparison to traditional techniques (e.g., chain and link method). For example, laboratory analysis of three-dimensional models supports estimation of multiple proxies of structural complexity, such as total surface, height of different structures in the reef, or even counting and measuring holes and crevices. In this paper, we have combined photomosaics to measure benthic cover and SfM to capture the multivariate nature of habitat complexity among reefs to explain the differences in fish assemblages. We hypothesized that fish assemblage would differ depending on structural complexity, whereby more complex reefs would support more biomass, abundance and diversity of fish, and also a different composition of species. To test this prediction, first, we created a series of photomosaics and 3D reconstructions of the benthic habitat along 50 m transects. Afterward, we derived multiple features describing the habitat for use in distance-based linear models designed to explain variation in fish assemblages, as described by total and species-specific abundance (fish assemblage structure) species richness, total and species-specific biomass and abundance and biomass of different trophic groups.
Materials and Methods
Study Area
The study was conducted at Archipelago Los Roques National Park (ALRNP); a marine protected area (MPA) located 170 km north off the central coast of Venezuela. Fieldwork was conducted at three sites: Dos Mosquises Sur (DMS), Cayo de Agua (CYA) and El Faro (FAR). Sites DMS and CAY are located at the west of the southern reef barrier, whereas FAR is at the eastern end of this barrier. Coral reefs across these sites are quite different in terms of their geomorphology, benthic communities, and structural complexity. Dos Mosquises is a fringing reef with high topographic heterogeneity provided by massive corals such as Orbicella faveolata, O. franksi, and Colpophyllia natans (>2 m height and/or width, Bastidas et al., 2012). Cayo de Agua is a flattened patch reef with scattered coral colonies (e.g., Diploria labyrinthiformis, Pseudodiploria strigosa, and Colpophyllia natans) and abundant octocorals. The site at FAR is a barrier reef with extensive areas of dead Acropora palmata stands covered by milleporids, zoanthids, and crustose coralline algae (CCA) (Croquer et al., 2016).
Data Collection in the Field
At each site, four 50 m long transects were laid on the substrate parallel to the coast at depths ranging from 6 to 8 m to avoid confounding effects from depth. Transects were separated by 5 m. Along each transect, a first diver characterized fish assemblages, and then a second diver took a video of the benthos covering an area of 50 × 2 m (100 m2). Videos were acquired at 30 frames per second from a height of 1.5–2.0 m above the reef contour with a standard digital camera (1440 × 1080 pixels resolution).
Description of Fish Assemblages
Underwater visual censuses were conducted to characterize fish assemblages at each site over the same sampling units (50 m transects). For this, a single observer swam along each transect counting and identifying fish to species level, and assigning each one to one of six size categories based on total length: (1)1–5 cm, (2) 6–10 cm, (3) 11–20 cm, (4) 21–30 cm, (5) 31–40 cm, and (6) over 40 cm. Each species abundance was converted to biomass using the allometric relationships outlined in www.fishbase.org. We also grouped each fish into one of six trophic categories: planktivores, foragers, small herbivores, small carnivores, and piscivores, according to descriptions found in www.fishbase.org.
Construction of 2D Mosaics
The first step to produce digital mosaics was obtaining overlapping frames from every video recorded along each transect (Figure 1). For this, the videos were split into fragments at a rate of 1.0–1.5 frames per second using ffmpeg v2.7 software (Bellard and Niedermayer, 2012) to produce multiple JPEG-format images with the highest quality setting (q = 1.0). The next step entailed using Hugin 2016 (D’Angelo, 2010) to find 8–40 matching points in consecutive frames that overlapped at least 35%. The identification of these points is necessary so the software can match consecutives frames. The final step consisted of stitching and blending the frames prior to optimization of translational parameters to correct potential video distortions using the default settings of the software (Figure 1). For each mosaic, we used photometric corrections of the JPEG output file to retrieve more accurate images that allowed a more reliable identification of benthic organisms. Furthermore, all images were calibrated using the total length of each transect. Complete mosaics (50-m transects) required too much computational time and were difficult to analyze using Coral Point Count with Excel extensions (CPCe; Kohler and Gill, 2006). Thus, for the analysis we produced several smaller mosaics for each transect. As swimming speed was not constant during acquisition of the video, the number of smaller mosaics varied between six and ten.
Description of Benthic Communities
A total of 200 points per mosaic were surveyed to identify major taxa (i.e., corals, octocorals, milleporids, sponge, zoanthids turf algae, and macroalgae) and abiotic substrates (i.e., dead coral, rubble, sand, and pavement). We used 200 points after constructing species accumulation curves using different quantities of random points. For this, we used CPCe software (Kohler and Gill, 2006). Only scleractinians corals and octocorals were identified down to species and genus level, respectively.
Construction of 3D Models of the Benthic Habitat
To create 3D models, we complemented existing tools such as Agisoft Photoscan (Agisoft LLC., St. Petersburg, Russia) and MeshLab with newly developed algorithms. Briefly, the reconstruction of the reef surface began with the extraction of enough frames from the input video to build reliable 3D models that preserved real structural features. To avoid poor overlap of images, distortion and other problems that potentially degraded the quality of the 3D model, we developed a tool (open source and freely available at1) that optimizes the process of frame selection by automatically selecting images with the least blurring, distortion and that overlap at least 65%. These frames were then uploaded into Agisoft Photoscan to follow standard procedures for 3D surface reconstruction (Figure 1). Every 3D model was imported into MeshLab and scaled to S.I. units, by using the transect length as reference (Figure 1).
Description of Habitat Complexity
We determined two sets of proxies aiming to describe habitat complexity at each site: (1) number and size of holes; and (2) metrics of structural complexity (i.e., a structural complexity index, maximum height of reef structure, average height of reef structure and variation the height of reef structure along the 50-m transects). The former set was derived from 2D mosaics, whereas the second set of variables were estimated by the 3D models. Thus, the metrics of complexity included an index of structural complexity, as well as maximum, average, variance and the kurtosis of heights for each structural element found within each transect. The index of surface complexity was calculated as the ratio between the actual 3D surface and the theoretical 2D surface of each transect (i.e., 50 × 3 m = 150 m2) using MeshLab. Heights were calculated as the perpendicular distances to the average plane recorded from each transect. This new plane was estimated using the plane fitting feature of the Point Cloud Library2, with RANSAC so the software could reject outliers.
Statistical Analysis
Data were analyzed following four steps: (1) visualization of spatial patterns of fish assemblages and the benthic substrate across sites, as we assume that similarities between these suggested an ecologically significant relationship. Patterns were identified with non- metric multidimensional scaling based on the Bray–Curtis index for benthic cover and fish assemblage and on Euclidian distance for proxies of structural complexity. Before analysis, benthic cover and fish biomass were square root transformed and structural complexity proxies were normalized (Clarke and Gorley, 2006). (2) We formally tested the null hypothesis (Ho) of no differences among: (a) structural complexity, (b) benthic community, and (c) fish assemblage, across sites. For this, we conducted a one-way permutation analysis of variance (PERMANOVA; Anderson, 2005) based on Bray–Curtis similarity index for the biological matrixes and Euclidean distance for the structural complexity matrix using site as a fixed factor. (3) Then we determined which combination of environmental variables better explained the pattern of variability in fish assemblage implementing the BIOENV routine, which matched the habitat-descriptor rectangular matrix (N transects × P variables) with the triangular matrix of Bray–Curtis similarities of fish assemblage structure. For this, we used data on both benthic assemblage and habitat complexity to identify correlations with: (a) total biomass, (b) species richness, (c) species density, and (d) structure of fish assemblages characterized by biomass and density of each species across sites. (4) A determination of the amount of variation explained by the variables suggested by BIOENV (i.e., all biological and structural complexity variables measured from each transect; Table 1) was done by building sequential linear models based on distance (DistLM). Along with environmental variables, site was included in the model as a categorical explanatory variable. We considered the best models those that explained the largest amount of variation using fewer variables. Before constructing linear models, we removed highly correlated variables (ρ > 0.8) to avoid redundancy and model overfitting. Finally, we also constructed models that explained the biomass of the four trophic groups, planktivores, foragers, small carnivores, and piscivores. All analyses were performed with Primer + PERMANOVA v6 (Anderson, 2005).
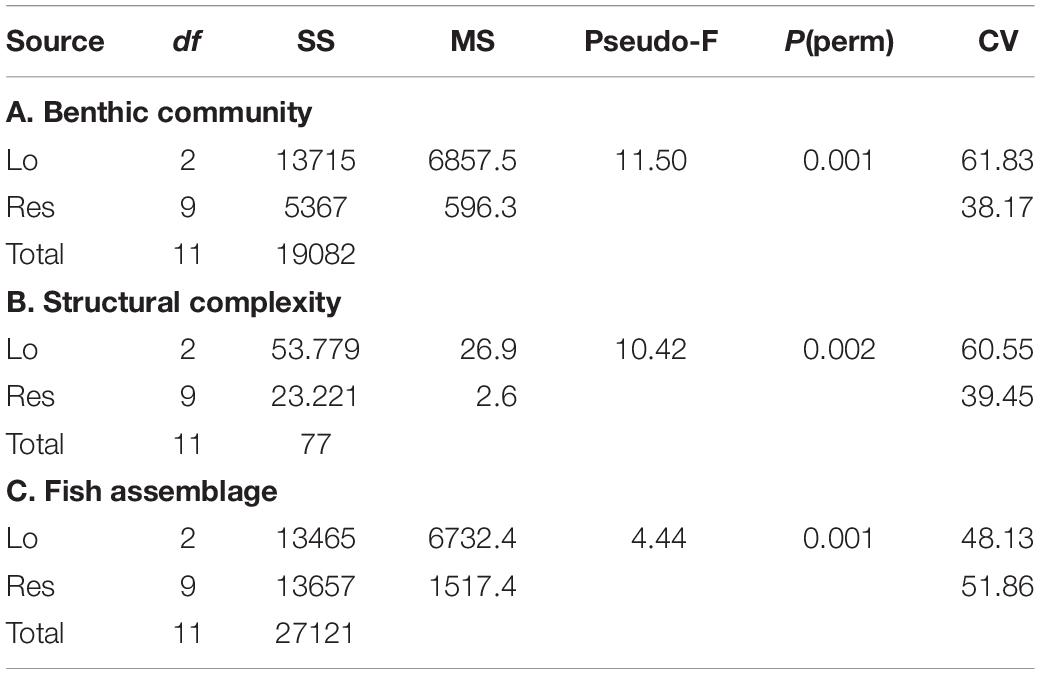
Table 1. Results of PERMANOVAs examining differences among benthic communities, proxies of structural complexity and fish assemblage.
Results
Benthic and Fish Assemblage Description and Patterns
Across sites, 1714 fishes belonging to 35 species and 10 families were observed. On average, the biomass of large parrotfishes such as Sparisoma viride and Scarus vetula was 1.5–3-fold higher at FAR compared to DMS and 3–10-fold when compared to CAY (Figure 2). These species along with Thallassoma bifasciatum, Chromis cyanea, Stegastes partitus, Clepticus parrae, Caranx ruber, Lutjanus cyanopterus, Kyphosus sectatrix, and Acanthurus coeruleus accounted for 75% of the abundance expressed as density (ind/100 m2) and biomass (g/100 m2) (Figures 2A,B).
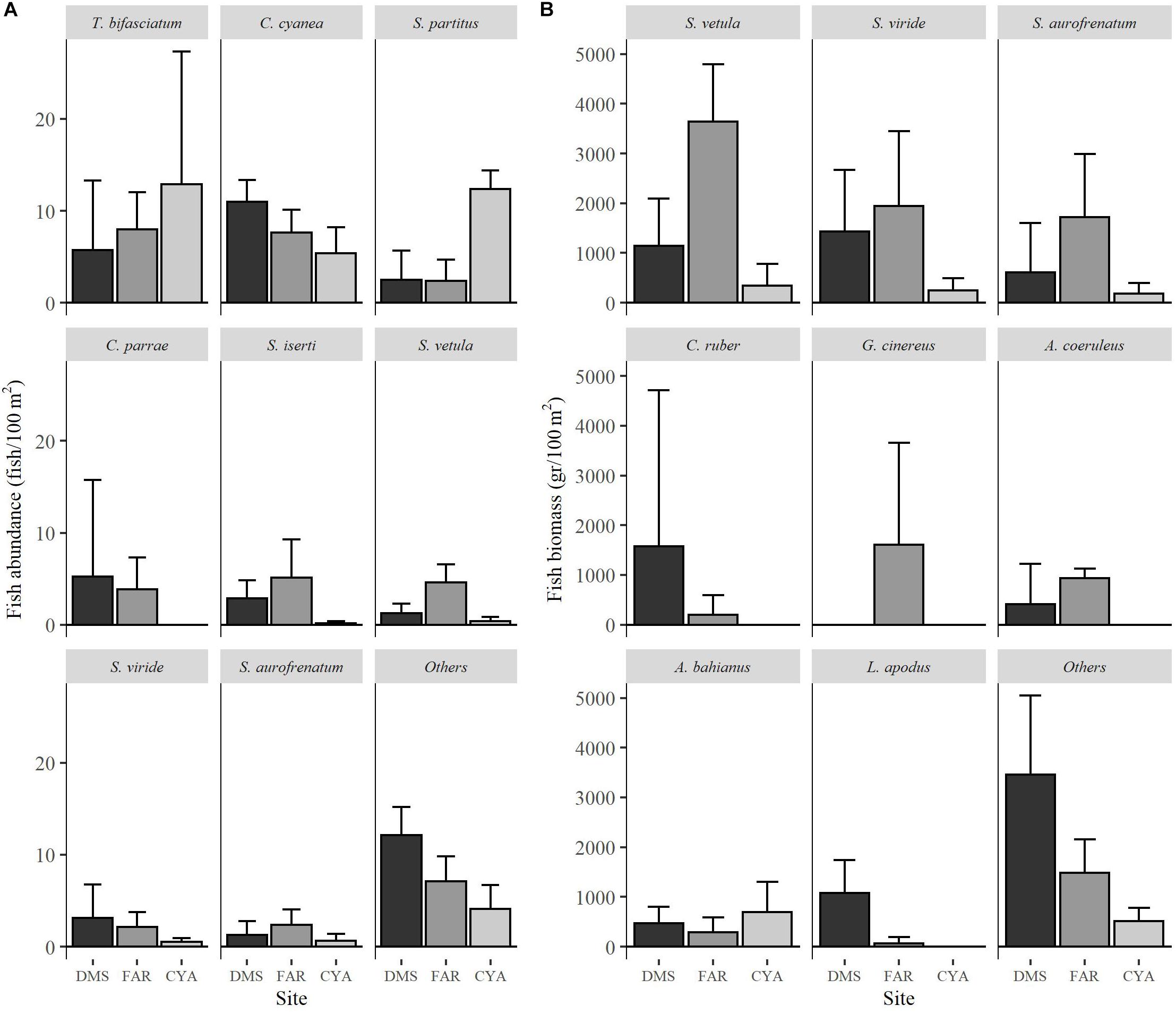
Figure 2. Abundance (number of individuals per 100 m2; A) and biomass (gr. per 100 m2; B) of the species composing 85% of the total abundances and biomass of fish. The planktonic species C. multilineata was not included for better visualization of the figure because of a single, large school (>600 individuals) was observed in Dos Mosquises. This species alone represented a 42% of total abundance of fish.
The reefs studied in Los Roques showed differences between them in both fish and benthic assemblages, as well as structural complexity. These patterns were visualized in the ordination plot and we tested if these differences were statistically significant. For instance, benthic assemblages differed significantly (F = 11.49 df = 2, p = 0.001) across reefs (Table 1A and Figures 3, 4). However, pair-wise comparisons showed no statistical differences in benthic communities (t = 1.8, df = 6, p = 0.155) between FAR and DMS (Figure 3); whereas statistical differences were found between CAY-DMS (t = 3.38, df = 6, p < 0.05) and CAY-FAR (t = 4.25, df = 6, p < 0.05). Likewise, attributes of habitat complexity varied significantly across sites (F = 10.22, df = 2, p = 0.01, Table 1B and Figure 3) similarly to benthic cover, pairwise comparisons indicated no statistical differences in structural complexity (t = 1.44, df = 6, p = 0.13) between FAR and DMS (Figure 2). We found similar patterns in two-dimensional ordination of data from benthic communities, habitat complexity and fish assemblages (Figure 4). Fish assemblage structure was statistically different across reefs (F = 4.16, df = 2, p = 0.001, Table 1C). In this case, pairwise comparisons showed statistically significant differences for every combination of sites (i.e., DMS-FAR, DMS-CAY, and CAY-FAR). Thus, our results suggest that benthic community structure, attributes of structural complexity and fish assemblages co-varied among sites.
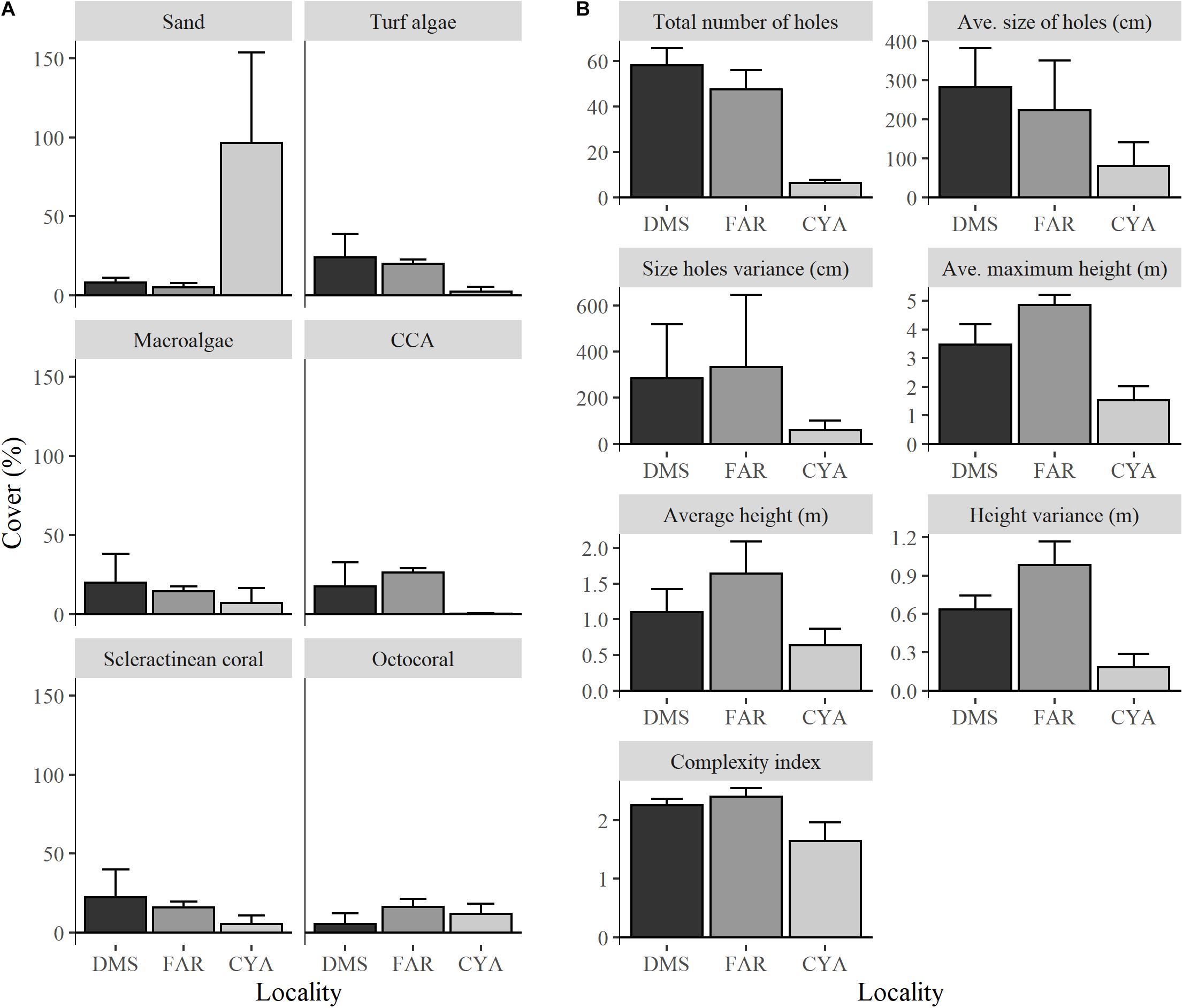
Figure 3. Average percent cover for benthic habitat (A) and proxies of structural complexity (B) at each site. Error bars represent standard deviations.
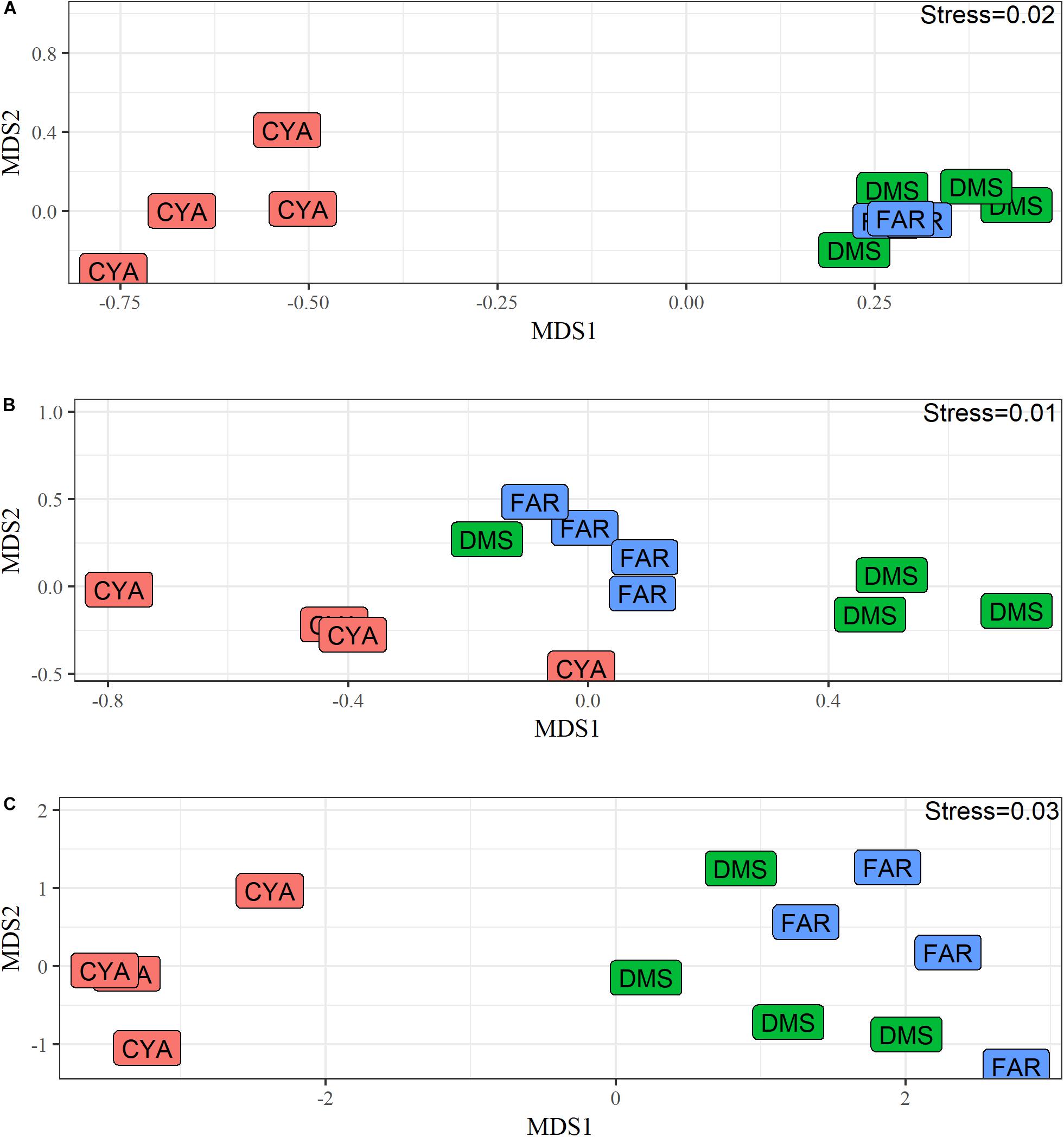
Figure 4. Non-metric Multidimensional Scaling (MDS) of benthic cover (A), proxies of structural complexity (B) and biomass of fish assemblages (C). Similarity matrix for benthic and fish assemblage were built using Bray–Curtis index, while Euclidean distances were used for complexity proxies. Note that benthic and fish data were square root transformed and complexity proxies were normalized.
Benthic Community and Structural Complexity as Predictors of Fish Assemblages
Our results from the BIOENV routine confirmed that attributes of fish assemblage, such as total fish biomass, abundance, and species richness were moderately correlated with coral cover and habitat complexity. Multivariate linear models based on distance (DistLM) explained 69%, 73% and 88% of the variation in total fish abundance, species richness, and biomass recorded across sites, respectively (Table 2). The results also showed that 69% (p-value = 0.04) of differences in species abundance (fish assemblage structure) among sites was explained by the percentage of cover of massive coral and turf algae, and the number and average size of holes. Furthermore, 64% (p-value = 0.04) of variability in terms of species-specific biomass was explained by CCA cover, variation in height of reef structure, site, the maximum height of reef structure and the number of holes (Table 2). On its own, the factor site alone only explained about 27–28% of the variance in species density and biomass and only added about 14% of explained variation to the model (Table 2).
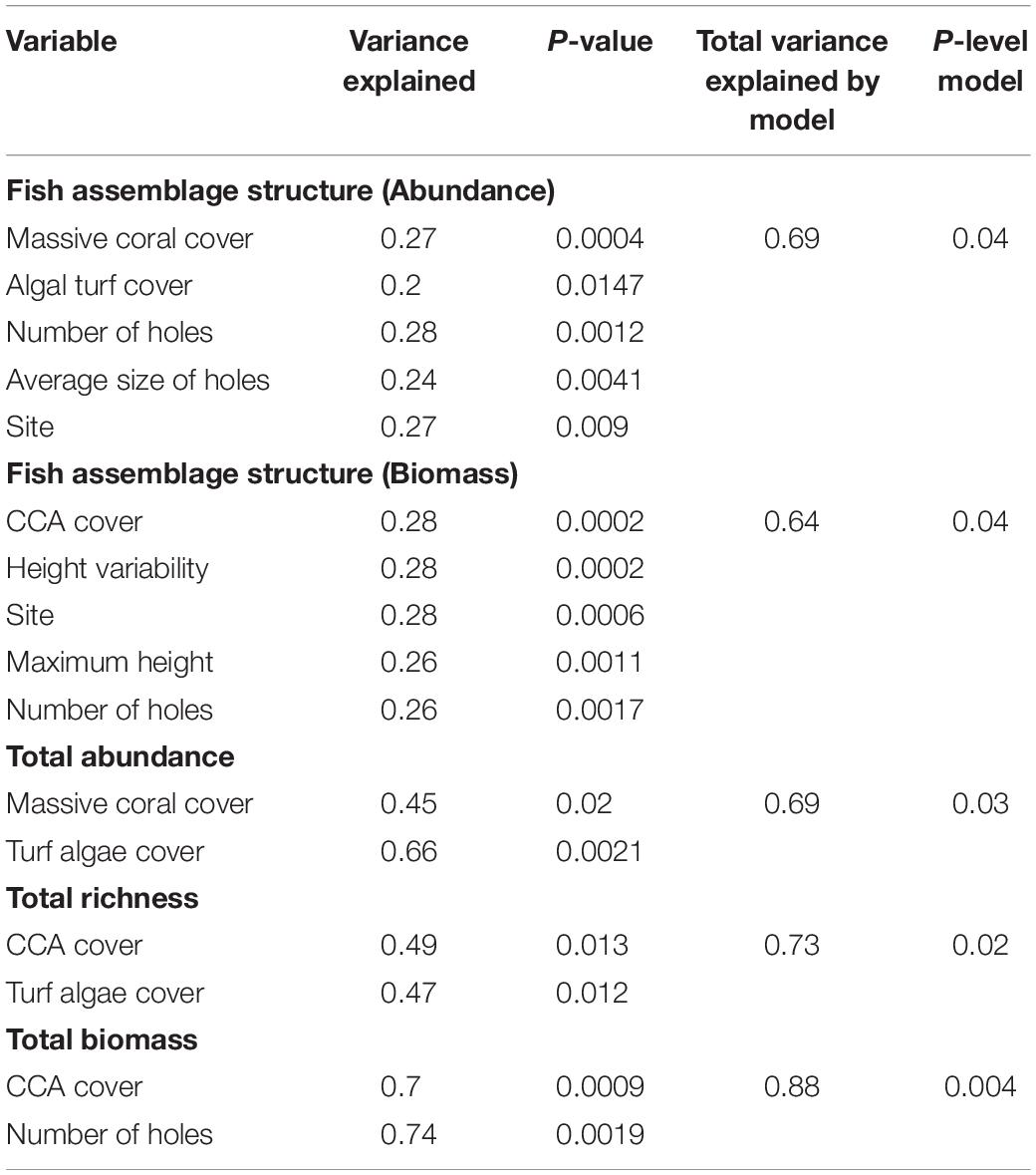
Table 2. Variables selected to construct linear models based on distances that best explained variation in abundances, biomass, richness and species-specific biomass of fish.
When looking at which variables were highly related to biomass of trophic groups, we observed that the variability in total biomass of forager fish (Families: Scaridae and Acanthuridae) was explained by only three variables: (1) cover of CCA, (2) the index of surface complexity, and (3) site, which together accounted for 74% of variation of the data (p-value = 0.01) (Table 3). In the case of small herbivores (Family Pomacentridae), 68% of the variation in biomass was explained (p-value = 0.53) by the number of holes and site, even though this correlation was not significant (Table 3). As for the density of planktivores, 70% (p-value = 0.03) was explained only by CCA cover and the number of holes. Furthermore, 78% (p-value = 0.014) of the variation in biomass of carnivores (Families: Serranidae, Lutjanidae, and Haemulidae) was explained by the average and the standard deviation in the size of holes. Lastly, 69% variability in biomass of piscivores (e.g., Sphyraena barracuda and some species of the family Carangidae) was explained by cover of turf algae (p-value = 0.62). However, this last model was not significant. In the case of small herbivores (Family Pomacentridae), 68% of the variation in biomass was explained (p-value = 0.0.0017) by the number of holes and site (Table 3).
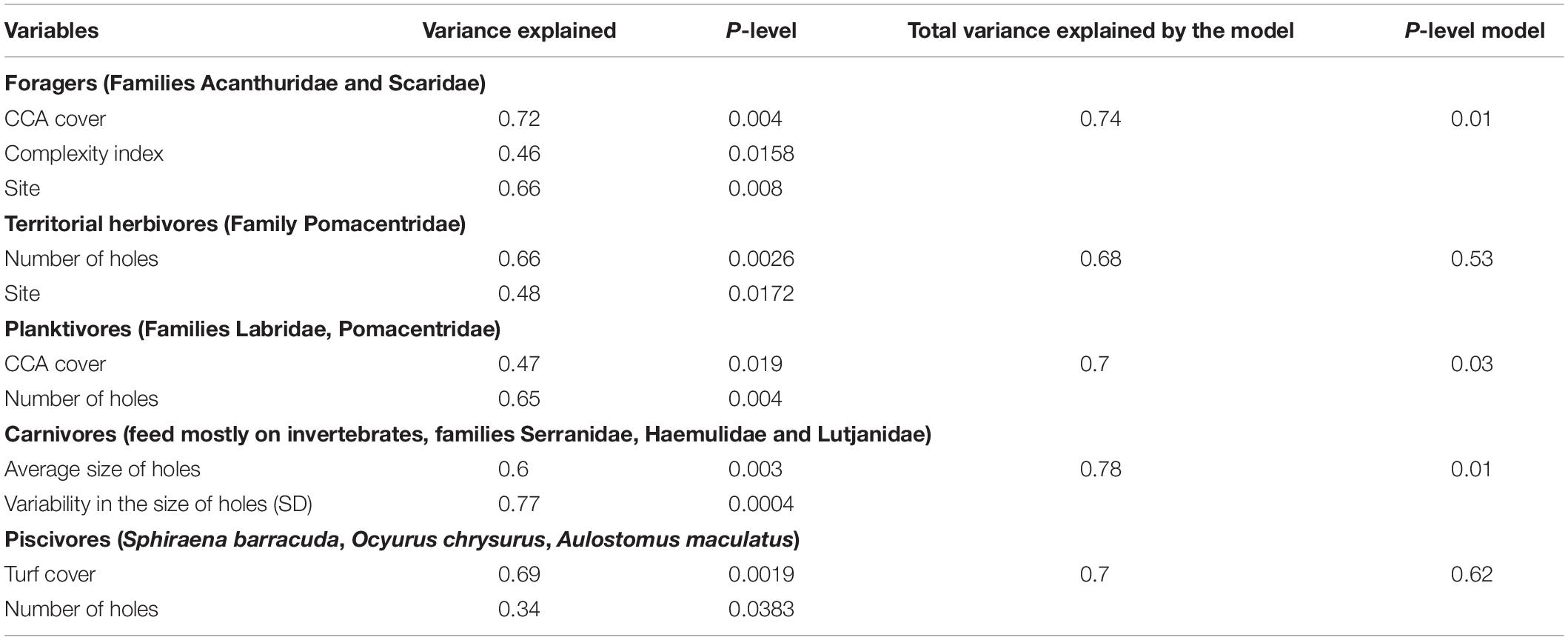
Table 3. Variables selected to construct linear models based on distances that best explained variation in biomass of fish with different trophic roles.
Discussion
We provided evidence that fish assemblages in Los Roques National Park vary significantly with specific features of the benthic habitat. We were able to explain more than 60% of the variability in total fish density, biomass, species richness and biomass of different species and trophic groups with only a few variables describing features of the benthic habitat. Benthic cover, live coral cover, turf algae, and CCA were good predictors of variation in fish assemblages. Additionally, several proxies of structural complexity (number and size of holes, the height of reef structures, and complexity index) also explained variation in fish assemblages between sites. Whilst live cover of massive scleractinian corals had an indisputable role in determining differences in benthic communities across sites and changes in fish assemblages in recent decades, this variable alone was not sufficient to explain the variability of fish assemblages at the scale of reefs as reported in other studies (Tolimieri, 1995; Sponaugle and Cowen, 1996; Friedlander et al., 2003; Wismer et al., 2009). Additionally, we found a high percentage of the differences in fish attributes were explained by the factor site. This result further suggests that factors not necessarily related to the attributes of the benthic habitat measured in this study (e.g., larvae supply, patterns of recruitment, wave exposure, and other oceanographic processes) also may drive differences in fish assemblage (Tolimieri, 1995; Sponaugle and Cowen, 1996; Friedlander et al., 2003; Wismer et al., 2009).
In most ecological assemblages, foundational species enhance ecological facilitation by providing habitat for other species and increasing niche availability, and in their absence, overall diversity often declines (Bruno and Bertness, 2000; Bruno et al., 2003; Bulleri et al., 2016). For example, effects of predation on diversity may be mitigated in more complex habitats since crevices provide refuge for smaller organisms that would otherwise be highly vulnerable to predation (Coull and Wells, 1983; Hereu et al., 2005; Komyakova et al., 2013). Likewise, the strength of competitive interactions leading to exclusion can be reduced in more complex habitats to allow species coexistence (Dayton, 1972; Stachowicz, 2001; Angelini et al., 2011). For coral reefs, multiple studies have affirmed that physical structure provided by scleractinian corals (Angelini et al., 2011; Burns J.H.R. et al., 2015) offers shelter and refuge from predators (Talbot et al., 1978; Roberts and Ormond, 1987; Caley and St. John, 1996; Friedlander and Parrish, 1998; Holbrook and Schmitt, 2004) as well as food resources for a myriad of fish species (Bouchon-Navaro and Bouchon, 1989; Stella et al., 2010, 2011; Graham and Nash, 2012). Many studies showed positive relationships between certain attributes of fish assemblages (i.e., abundances, richness or biomass) and live coral cover, structural complexity, or both (Risk, 1972; Luckhurst and Luckhurst, 1978; Birkeland and Neudecker, 1981; Bell and Galzin, 1984; Coker et al., 2012) while other studies showed a relationship between loss of coral cover and related structural complexity and fish abundances and diversity (Sano et al., 1984, 1987; Wilson et al., 2008). For example, structural complexity provided by massive and branching corals affected habitat selection by increasing the availability of suitable patches for territorial species of fish (Grol et al., 2011). Furthermore, holes and crevices may provide refuge for small fish and invertebrates that serve as food for piscivores and carnivores fish, respectively (Graham and Nash, 2012; Darling et al., 2017). Similarly, more complex habitat, as reflected by variables like total height, can provide vertical surfaces and large overhangs which could be used as refuge for larger foragers (Families Acanthuridae and Scaridae) too big to fit into small holes and crevices (Ménard et al., 2012).
The relationship observed between forager abundance and CCA can be a consequence of parrotfish and surgeonfish consuming turf algae and increasing space available for other benthic organisms like CCA (McManus et al., 2000; Ceccarelli et al., 2005; Hughes et al., 2007; Lokrantz et al., 2008; Mumby, 2009). Additionally, foragers were an important part of total fish biomass and are amongst the most biodiverse fish group on Caribbean reefs, which could explain the relationship between total fish biomass and species richness with CCA cover.
Our results indicate that fish biomass and species richness may increase with live coral cover and structural complexity. We found that sites such as DMS and FAR supported 4–6 times more fish biomass and 1.5-fold higher species richness compared to CAY, where the habitat was flatter and less structurally complex and the fish assemblage comprised mostly small demersal species like Stegastes partitus and Thallassoma bifasciatum. These species are considered omnivorous and generalist, often associated with degraded sites with low complexity and where competition with other species of damselfish is limited (Dominici-Arosemena and Wolff, 2006; Elise et al., 2017). Our results also indicated that only a few massive coral species (e.g., Orbicella faveolata, O. annularis, and Colpophyllia natans) contributed to live coral cover while increasing habitat structural complexity. Thus, our results support findings of recent studies showing that habitat complexity is not a simple function of live coral cover, but rather it varies at different scales depending on the species composition of the coral assemblage (Richardson et al., 2017b). However, the abundance of several small fish (e.g., territorial herbivores in the family Pomacentridae) seems to be related to live coral cover suggesting that coral cover could be playing an important role during recruitment and habitat selection in some species (Bell and Galzin, 1984; Gutiérrez, 1998).
The importance of habitat complexity in determining changes in biomass among sites, rather than a simple proportion of live-dead coral cover, was further illustrated by the features of the benthic coral habitat observed at FAR. At this site, we recorded the highest number of fish species (ca 12–15) and biomass (an average of 11.87 kg/m2), and the habitat consisted mainly of large, upright fragments of dead Acropora palmata, which added complexity to the substrate because these fragments mingled to form crevices, overhangs, and high topographic relief. In addition, the importance of variation of heights of structures on the reef in explaining the variation of fish assemblages suggested that the physical structure provided by dead but structurally complex corals still represents a suitable habitat for fish. Although there are other studies indicating that live coral cover is highly correlated to structural complexity (Darling et al., 2017), a dense cover of CCA might have maintained structural complexity by stabilizing coral skeletons and preventing bioerosion or flattening from physical abrasive forces (Sheppard et al., 2009). At FAR, A. palmata fragments were covered by CCA with no macroalgae and turf, which might have been due to a combination of herbivory from parrotfish (e.g., Scarus vetula, Sparisoma viride, and S. aurofrenatum), and long-spine urchins (Diadema antillarum), as well as low inputs of nutrients expected for oceanic coral reef systems like Los Roques (Birkeland, 2015; Bozec et al., 2015).
At DMS, live coral cover was reduced from 50 to 30% during a massive bleaching event that impacted Los Roques in the summer of 2010 (Bastidas et al., 2012). During this event, large Orbicella colonies suffered a massive die-off, leading to a rapid loss of coral tissues (Garzon-Ferreira et al., 2002; Villamizar et al., 2003; Cróquer et al., 2010; Bastidas et al., 2012). Nevertheless, 8 years later, the dead Orbicella colonies continue to contribute to a highly structurally complex habitat. Thus, our study suggested that loss of live coral cover might have different impacts on structural complexity depending on the identity of the coral species that are lost and if their physical structure remains intact after death (Sano et al., 1984, 1987; Richardson et al., 2017a). Striking changes in the fish community would be expected in cases of rapid decline of live coral cover leading to reef flattening (Sano et al., 1984; Alvarez-Filip et al., 2015; Newman et al., 2015), whereas minor impacts would be observed in cases where loss of live coral cover did not result in an immediate reduction of habitat complexity. It is also necessary to consider that the impacts of loss of live coral cover on fish biomass and richness are not linear and may be modulated by various processes like reduction in refuge availability. This may reduce recruitment of certain species, some of which might be highly specialist and dependent of certain coral species (Jones et al., 2004; Alvarez-Filip et al., 2015). These interactions have serious implications for selecting variables for inclusion in reef monitoring programs, for constructing models to predict fish abundances and species richness, as well as for management decisions regarding fisheries and fish populations that are based on these monitoring programs.
Recent studies have pinpointed Los Roques as a potential refuge for corals and fish because the MPA possesses higher coral cover than typical in the region and populations of important reef builders that provide highly heterogeneous reefs (Villamizar et al., 2003; Jackson et al., 2014; Elise et al., 2017). Severe bleaching events leading to rapid loss of coral cover have impacted several coral populations in Los Roques (Villamizar et al., 2008; Bastidas et al., 2012), and Los Roques also suffered the loss of most of the reef building Acropora palmata populations in past years (Croquer et al., 2016). Nevertheless, coral reefs in this MPA still support fish abundance that is above the Caribbean average (Elise et al., 2017). This finding highlights the ecological importance of this MPA at the regional scale for there is increasing evidence indicating that Caribbean reefs are losing most of their structural complexity (Alvarez-Filip et al., 2009) and concomitantly fish communities have changed rapidly (Alvarez-Filip et al., 2009, 2015).
In conclusion, this paper illustrates the complexity of fish-habitat interactions on coral reefs. Furthermore, it highlights the importance of preserving not only live coral cover as an essential variable (Miloslavich et al., 2018), but also the physical structure provided by corals to maintain ecosystem function.
Author Contributions
EA-A performed the experiments, analyzed the data, wrote the manuscript, prepared the figures and tables, and reviewed drafts of the manuscript. JC performed the experiments, contributed reagents, materials, and analysis tools, wrote the manuscript, and reviewed drafts of the manuscript. FC-B performed the experiments, wrote the manuscript, and reviewed drafts of the manuscript. AC performed the experiments, analyzed the data, wrote the manuscript, and reviewed drafts of the manuscript.
Funding
Field activities was provided through the EDGE of Existence Program Fellowships, Zoological Society of London.
Conflict of Interest
The authors declare that the research was conducted in the absence of any commercial or financial relationships that could be construed as a potential conflict of interest.
Acknowledgments
We acknowledge Territorio Insular Miranda and Instituto Nacional de Parques (INPARQUES) for providing the permits to conduct this study. We thank Andy Estep, Gina-Marie Maddix, Erica Johnson, and Monique Curtis for providing valuable comments for this manuscript. We also thank Mr. Jan Albarran for the logistic support provided on the field.
Field Study Permissions
This study was authorized by the Ministerio del Poder Popular de Ecosocialismo, Hábitat y Vivienda (Approval number: 0323) and Territorio Insular Miranda (Approval number: 006). No other permission was required from an ethics committee to conduct this study in compliance with local legislation.
Footnotes
References
Agudo-Adriani, E. A., Cappelletto, J., Cavada-Blanco, F., and Croquer, A. (2016). Colony geometry and structural complexity of the endangered species Acropora cervicornis partly explains the structure of their associated fish assemblage. PeerJ 4:e1861. doi: 10.7717/peerj.1861
Alvarez-Filip, L., Dulvy, N. K., Côteé, I. M., Watkinson, A. R., and Gill, J. A. (2011). Coral identity underpins architectural complexity on caribbean reefs. Ecol. Appl. 21, 2223–2231. doi: 10.1890/10-1563.1
Alvarez-Filip, L., Dulvy, N. K., Gill, J. A., Côté, I. M., and Watkinson, A. R. (2009). Flattening of caribbean coral reefs: region-wide declines in architectural complexity. Proc. R. Soc. Biol. Sci. U.S.A. 276, 3019–3025. doi: 10.1098/rspb.2009.0339
Alvarez-Filip, L., Paddack, M. J., Collen, B., Robertson, D. R., and Côté, I. M. (2015). Simplification of caribbean reef-fish assemblages over decades of coral reef degradation. PLoS One 10:e0126004. doi: 10.1371/journal.pone.0126004
Anderson, M. (2005). Permutational Multivariate Analysis of Variance. Auckland: The University of Auckland.
Angel, A., and Ojeda, F. P. (2001). Structure and trophic organization of subtidal fish assemblages on the northern Chilean coast: the effect of habitat complexity. Mar. Ecol. Prog. Ser. 217, 81–91. doi: 10.3354/meps217081
Angelini, C., Altieri, A. H., Silliman, B. R., and Bertness, M. D. (2011). Interactions among foundation species and their consequences for community organization, biodiversity, and conservation. Bioscience 61, 782–789. doi: 10.1525/bio.2011.61.10.8
Bastidas, C., Bone, D., Croquer, A., Debrot, D., Garcia, E., Humanes, A., et al. (2012). Massive hard coral loss after a severe bleaching event in 2010 at los roques Venezuela. Rev. Biol. Trop. 60, 29–37.
Beck, M. W. (2000). Separating the elements of habitat structure: independent effects of habitat complexity and structural components on rocky intertidal gastropods. J. Exp. Mar. Bio. Ecol. 249, 29–49. doi: 10.1016/S0022-0981(00)00171-174
Bell, J., and Galzin, R. (1984). Influence of live coral cover on coral-reef fish communities. Mar. Ecol. Prog. Ser. 15, 265–274. doi: 10.3354/meps015265
Bellard, F., and Niedermayer, M. (2012). FFmpeg. Available at: http//www.ffmpeg.org (accessed July, 2018).
Birkeland, C., and Neudecker, S. (1981). Foraging behavior of two Caribbean chaetodontids: Chaetodon capistratus and C. aculeatus. Copeia 1, 169–178.
Bouchon-Navaro, Y., and Bouchon, C. (1989). Correlations between chaetodontid fishes and coral communities of the Gulf of Aqaba (Red Sea). Environ. Biol. Fish. 25, 47–60. doi: 10.1007/978-94-009-2325-6_3
Bozec, Y. M., Alvarez-Filip, L., and Mumby, P. J. (2015). The dynamics of architectural complexity on coral reefs under climate change. Glob. Chang. Biol. 21, 223–235. doi: 10.1111/gcb.12698
Bruno, J. F., and Bertness, M. D. (2000). “Habitat modification and facilitation in benthic marine communities,” in Marine Community Ecology, eds M. D. Bertness, S. D. Gaines, and M. E. Hay, (Sunderland: Sinauer Associates), 201–218.
Bruno, J. F., Stachowicz, J. J., and Bertness, M. D. (2003). Inclusion of facilitation into ecological theory. Trends Ecol. Evol. 18, 119–125. doi: 10.1016/S0169-5347(02)00045-49
Bulleri, F., Bruno, J. F., Silliman, B. R., and Stachowicz, J. J. (2016). Facilitation and the niche: implications for coexistence, range shifts and ecosystem functioning. Funct. Ecol. 30, 70–78. doi: 10.1111/1365-2435.12528
Burns, J., Delparte, D., Gates, R., and Takabayashi, M. (2015). Integrating structure-from-motion photogrammetry with geospatial software as a novel technique for quantifying 3D ecological characteristics of coral reefs. PeerJ 3:e1077. doi: 10.7717/peerj.1077
Burns, J. H. R., Delparte, D., Gates, R. D., and Takabayashi, M. (2015). Utilizing underwater three-dimensional modeling to enhance ecological and biological studies of coral reefs. ISPRS 5, 61–66. doi: 10.5194/isprsarchives-XL-5-W5-61-2015
Caley, J., and St. John, J. (1996). Refuge availability structures assemblages of tropical reef fishes. J. Anim. Ecol. 65, 414–428.
Ceccarelli, D. M., Jones, G. P., and McCook, L. J. (2005). Foragers versus farmers: contrasting effects of two behavioural groups of herbivores on coral reefs. Oecologia 145, 445–453. doi: 10.1007/s00442-005-0144-y
Chong-Seng, K. M., Mannering, T. D., Pratchett, M. S., Bellwood, D. R., and Graham, N. (2012). The influence of coral reef benthic condition on associated fish assemblages. PLoS One 7:e42167. doi: 10.1371/journal.pone.0042167
Coker, D. J., Graham, N. A., and Pratchett, M. S. (2012). Interactive effects of live coral and structural complexity on the recruitment of reef fishes. Coral Reefs 31, 919–927. doi: 10.1007/s00338-012-0920-921
Connell, J. (1978). Diversity in tropical rain forests and coral reefs. Science 199, 1302–1310. doi: 10.1126/science.199.4335.1302
Coull, B. C., and Wells, J. B. J. (1983). Refuges from fish predation: experiements with phytal meiofauna from the New Zealand rocky intertidal. Ecology 64, 1599–1609. doi: 10.2307/1937513
Croquer, A., Cavada-Blanco, F., Zubillaga, A. L., Agudo-Adriani, E. A., and Sweet, M. (2016). Is Acropora palmata recovering? a case study in Los Roques national Park, venezuela. PeerJ 4:e1539. doi: 10.7717/peerj.1539
Cróquer, A., Debrot, D., Klein, E., Kurten, M., Rodríguez, S., and Bastidas, C. (2010). What can two years of monitoring tell us about venezuelan coral reefs? The Southern Tropical America node of the global coral reef monitoring network (STA-GCRMN). Rev. Biol. Trop. 58, 51–65.
D’Angelo, P. (2010). Hugin-Panorama Photo Stitcher. Available at: http//hugin.sourceforge.net (accessed July, 2018).
Darling, E. S., Graham, N. A. J., Januchowski-hartley, F. A., Nash, K. L., Pratchett, M. S., and Wilson, S. K. (2017). Relationships between structural complexity, coral traits, and reef fish assemblages. Coral Reefs 36, 561–575. doi: 10.1007/s00338-017-1539-z
Dayton, P. K. (1972). “Toward an understanding of community resilience and the potential effects of enrichments to the benthos at mcmurdo sound, Antartica,” in Proceedings of the Colloquium on Conservation Problems in Antarctica, Lawrence, KS.
Dominici-Arosemena, A., and Wolff, M. (2006). Reef fish community structure in the tropical eastern pacific (Panamá): living on a relatively stable rocky reef environment. Helgol. Mar. Res. 60, 287–305. doi: 10.1007/s10152-006-0045-44
Elise, S., Urbina, I., Hazael, B., Gil, B., Galindo, M., Ernesto, J., et al. (2017). Archipelago Los Roques: a potential baseline for reef fish assemblages in the southern caribbean. Aquat. Conserv. Mar. Freshw. Ecosyst. 27, 1–17. doi: 10.1002/aqc.2770
Ferrari, R., McKinnon, D., He, H., Smith, R., Corke, P., González-Rivero, M., et al. (2016). Quantifying multiscale habitat structural complexity: a cost-effective framework for underwater 3D modelling. Remote Sens. 8:113. doi: 10.3390/rs8020113
Friedlander, A., Brown, E. K., Jokiel, P. L., Smith, W. R., and Rodgers, K. S. (2003). Effects of habitat, wave exposure, and marine protected area status on coral reef fish assemblages in the hawaiian archipelago. Coral Reefs 22, 291–305. doi: 10.1007/s00338-003-0317-312
Friedlander, A. M., and Parrish, J. D. (1998). Habitat characteristics affecting fish assemblages on a Hawaiian coral reef. J. Exp. Mar. Bio. Ecol. 224, 1–30. doi: 10.1016/S0022-0981(97)00164-160
Garzon-Ferreira, J., Cortés, J., Corquer, A., Guzman, H., Leao, Z., and Rodríguez-Ramírez, N. (2002). “Status of coral reefs in southern tropical America in 2000–2002: Brazil, Colombia, Costa Rica, panama and Venezuela,” in Status of Coral Reefs of the World, ed. B. Wilkinson, (Townsville: Australian Institute of Marine Science), 343–360.
Graham, N. A. J., and Nash, K. L. (2012). The importance of structural complexity in coral reef ecosystems. Coral Reefs 32, 315–326. doi: 10.1007/s00338-012-0984-y
Grol, M., Nagelkerken, I., Bosch, N., and Meesters, E. (2011). Preference of early juveniles of a coral reef fish for distinct lagoonal microhabitats is not related to common measures of structural complexity. Mar. Ecol. Prog. Ser. 432, 221–233. doi: 10.3354/meps09175
Gutiérrez, L. (1998). Habitat selection by recruits establishes local patterns of adult distribution in two species of damselfishes: Stegastes dorsopunicans and S. planifrons. Oecologia 115, 268–277. doi: 10.1007/s004420050516
Hereu, B., Zabala, M., Linares, C., and Sala, E. (2005). The effects of predator abundance and habitat structural complexity on survival of juvenile sea urchins. Mar. Biol. 146, 293–299. doi: 10.1007/s00227-004-1439-y
Holbrook, S. J., and Schmitt, R. J. (2004). Population dynamics of a damselfish: effects of a competitor that also is an indirect mutualist. Ecology 85, 979–985. doi: 10.1890/03-0406
Hughes, T. P., Baird, A. H., Bellwood, D. R., Card, M., Connolly, S. R., Folke, C., et al. (2003). Climate change, human impacts, and the resilience of coral reefs. Science 3, 929–934. doi: 10.1126/science.1085046
Hughes, T. P., Rodrigues, M. J., Bellwood, D. R., Ceccarelli, D., Hoegh-Guldberg, O., McCook, L., et al. (2007). Phase shifts, herbivory, and the resilience of coral reefs to climate change. Curr. Biol. 17, 360–365. doi: 10.1016/j.cub.2006.12.049
Huston, M. A., and DeAngelis, D. L. (1994). Competition and coexistence: the effects of resource transport and supply rates. Am. Nat. 144, 954–977. doi: 10.1086/285720
Jackson, J. B. C., Donovan, M. K., Cramer, K. L., and Lam, W. (2014). Status and Trends of Caribbean Coral Reefs: 1970-2012. Gland: IUCN.
Jones, G. P., McCormick, M. I., Srinivasan, M., and Eagle, J. V. (2004). Coral decline threatens fish biodiversity in marine reserves. Proc. Natl. Acad. Sci. U.S.A. 101, 8251–8253. doi: 10.1073/pnas.0401277101
Kohler, K. E., and Gill, S. M. (2006). Coral point count with excel extensions (CPCe): a visual basic program for the determination of coral and substrate coverage using random point count methodology. Comput. Geosci. 32, 1259–1269. doi: 10.1016/j.cageo.2005.11.009
Komyakova, V., Munday, P. L., and Jones, G. P. (2013). Relative importance of coral cover, habitat complexity and diversity in determining the structure of reef fish communities. PLoS One 8:e83178. doi: 10.1371/journal.pone.0083178
Laegdsgaard, P., and Johnson, C. (2001). Why do juvenile fish utilise mangrove habitats? J. Exp. Mar. Bio. Ecol. 257, 229–253. doi: 10.1016/S0022-0981(00)00331-332
Laforsch, C., Christoph, E., Glaser, C., Naumann, M., Wild, C., and Niggl, W. (2008). A precise and non-destructive method to calculate the surface area in living scleractinian corals using X-ray computed tomography and 3D modeling. Coral Reefs 27, 811–820. doi: 10.1007/s00338-008-0405-404
Lavy, A., Eyal, G., Neal, B., Keren, R., Loya, Y., and Ilan, M. (2015). A quick, easy and non-intrusive method for underwater volume and surface area evaluation of benthic organisms by 3D computer modelling. Methods Ecol. Evol. 6, 521–531. doi: 10.1111/2041-210X.12331
Lirman, D., Gracias, N. R., Gintert, B. E., Gleason, A. C. R., Reid, R. P., Negahdaripour, S., et al. (2007). Development and application of a video-mosaic survey technology to document the status of coral reef communities. Environ. Monit. Assess. 125, 59–73. doi: 10.1007/s10661-006-9239-9230
Lokrantz, J., Nyström, M., Thyresson, M., and Johansson, C. (2008). The non-linear relationship between body size and function in parrotfishes. Coral Reefs 27, 967–974. doi: 10.1007/s00338-008-0394-393
Luckhurst, B. E., and Luckhurst, K. (1978). Analysis of the influence of substrate variables on coral reef fish communities. Mar. Biol. 49, 317–323. doi: 10.1007/bf00455026
McManus, J., Menez, L. A., Kesner-Reyes, K. N., Vergara, S. G., and Ablan, M. C. (2000). Coral reef fishing and coral-algal phase shifts: implications for global reef status. ICES J. Mar. Sci. 57, 572–578. doi: 10.1006/jmsc.2000.0720
Ménard, A., Turgeon, K., Roche, D. G., Binning, S. A., and Kramer, D. L. (2012). Shelters and their use by fishes on fringing coral reefs. PLoS One 7:e38450. doi: 10.1371/journal.pone.0038450
Miloslavich, P., Bax, N. J., Simmons, S. E., Klein, E., Appeltans, W., Aburto-Oropeza, O., et al. (2018). Essential ocean variables for global sustained observations of biodiversity and ecosystem changes. Glob. Chang. Biol. 24, 2416–2433. doi: 10.1111/gcb.14108
Mumby, P. J. (2009). Herbivory versus corallivory: are parrotfish good or bad for Caribbean coral reefs? Coral Reefs 28, 683–690. doi: 10.1007/s00338-009-0501-500
Munday, P. L., Jones, G. P., Pratchett, M. S., and Williams, A. J. (2008). Climate change and the future for coral reef fishes. Fish Fish. 9, 261–285. doi: 10.1111/j.1467-2979.2008.00281.x
Newman, S. P., Meesters, E. H., Dryden, C. S., Williams, S. M., Sanchez, C., Mumby, P. J., et al. (2015). Reef flattening effects on total richness and species responses in the Caribbean. J. Anim. Ecol. 84, 1678–1689. doi: 10.1111/1365-2656.12429
Pratchett, M. S., Munday, P. L., Wilson, S. K., Graham, N. A. J., Cinneri, J. E., Bellwood, D. R., et al. (2008). Effects of climate-induced coral bleaching on coral-reef fishes - ecological and economic consequences. Oceanogr. Mar. Biol. An Annu. Rev. 46, 251–296. doi: 10.1201/9781420065756.ch6
Reid, R. P., Lirman, D., Gracias, N., Negahdaripour, S., Gleason, A., and Gintert, B. (2010). Application of Landscape Mosaic Technology to Complement Coral Reef Resource Mapping and Monitoring. Florida: University of Miami.
Richardson, L. E., Graham, N. A. J., and Hoey, A. S. (2017a). Cross-scale habitat structure driven by coral species composition on tropical reefs. Sci. Rep. 7, 1–11. doi: 10.1038/s41598-017-08109-8104
Richardson, L. E., Graham, N. A. J., Pratchett, M. S., and Hoey, A. S. (2017b). Structural complexity mediates functional structure of reef fish assemblages among coral habitats. Environ. Biol. Fish. 100, 193–207. doi: 10.1007/s10641-016-0571-0
Risk, M. J. (1972). Fish diversity on a coral reef in the Virgin Islands. Atoll Res. Bull. 153, 1–6.
Roberts, C. M., and Ormond, R. (1987). Habitat complexity and coral reef fish diversity and abundance on Red Sea fringing reefs. Mar. Ecol. Prog. Ser. 41, 1–8. doi: 10.3354/meps041001
Sale, P. F. (1977). Maintenance of high diversity in coral reef fish communities. Am. Nat. 111, 337–359. doi: 10.1086/283164
Sale, P. F., and Douglas, W. A. (1984). Temporal variability in the community structure of fish on coral patch reefs and the relation of community structure to reef structure. Ecology 65, 409–422. doi: 10.2307/1941404
Sano, M., Shimizu, M., and Nose, Y. (1984). Changes in structure of coral reef fish communities by destruction of hermatypic corals: observational and experimental views. Pac. Sci. 38, 51–79.
Sano, M., Shimizu, M., and Nose, Y. (1987). Long-term effects of destruction of hermatypic corals by Acanthaster planci infestation on reef. Mar. Ecol. Prog. Ser. 37, 191–199. doi: 10.3354/meps037191
Sheppard, C. R., Davy, S. K., and Pilling, G. M. (2009). The Biology of Coral Reefs. Oxford: Oxford University Press.
Sponaugle, S., and Cowen, R. K. (1996). Larval supply and patterns of recruitment for two Caribbean reef fishes Stegastes partitus and Acanthurus bahianus. Mar. Freshw. Res. 47, 433–447. doi: 10.1071/MF9960433
St. Pierre, J. I., and Kovalenko, K. E. (2014). Effect of habitat complexity attributes on species richness. Ecosphere 5:art22. doi: 10.1890/ES13-00323.1
Stachowicz, J. J. (2001). Mutualism, facilitation, and the structure of ecological communities. Bioscience 51, 235–246. doi: 10.1126/science.aai8212
Stella, J. S., Jones, G. P., and Pratchett, M. S. (2010). Variation in the structure of epifaunal invertebrate assemblages among coral hosts. Coral Reefs 29, 957–973. doi: 10.1007/s00338-010-0648-648
Stella, J. S., Pratchett, M. S., Hutchings, P. A., and Jones, G. P. (2011). Coral-associated invertebrates. diversity, ecological importance and vulnerability to disturbance. Oceanogr. Mar. Biol. Annu. Rev. 49, 43–104.
Talbot, F., Russell, B., and Anderson, G. (1978). Coral reef fish communities: unstable, high-diversity systems? Ecol. Modell. 48, 425–440. doi: 10.2307/2937241
Tews, J., Brose, U., Grimm, V., Tielbörger, K., Wichmann, M. C., Schwager, M., et al. (2004). Animal species diversity driven by habitat heterogeneity/diversity: the importance of keystone structures. J. Biogeogr. 31, 79–92. doi: 10.1046/j.0305-0270.2003.00994.x
Tolimieri, N. (1995). Effects of microhabitat characteristics on the settlement and recruitment of a coral-reef fish at two spatial scales. Oecologia 102, 52–63. doi: 10.1007/s004420050096
Villamizar, E., Camisotti, H., Rodríguez, B., Pérez, J., and Romero, M. (2008). Impacts of the 2005 Caribbean bleaching event at archipiélago de Los Roques National park. Venezuela. Rev. Biol. Trop. 56, 255–270.
Villamizar, E., Posada, J., and Gomez, S. (2003). Rapid assesment of the coral reefs in the archipiélago de los roques National Park, Venezuela (part 1: stony corals and algae). Atoll Res. Bull. 496, 512–529. doi: 10.5479/si.00775630.496-28.512
Westoby, M. J., Brasington, J., Glasser, N. F., Hambrey, M. J., and Reynolds, J. M. (2012). “Structure-from-Motion” photogrammetry: a low-cost, effective tool for geoscience applications. Geomorphology 179, 300–314. doi: 10.1016/j.geomorph.2012.08.021
Willis, S. C., Winemiller, K. O., and Lopez-Fernandez, H. (2005). Habitat structural complexity and morphological diversity of fish assemblages in a Neotropical floodplain river. Oecologia 142, 284–295. doi: 10.1007/s00442-004-1723-z
Wilson, S. K., Fisher, R., Pratchett, M. S., Graham, N. A. J., Dulvy, N. K., Turner, R. A., et al. (2008). Exploitation and habitat degradation as agents of change within coral reef fish communities. Glob. Chang. Biol. 14, 2796–2809. doi: 10.1111/j.1365-2486.2008.01696.x
Keywords: digital mosaics, 3D models, Southern Caribbean, coral reef fishes, SfM
Citation: Agudo-Adriani EA, Cappelletto J, Cavada-Blanco F and Cróquer A (2019) Structural Complexity and Benthic Cover Explain Reef-Scale Variability of Fish Assemblages in Los Roques National Park, Venezuela. Front. Mar. Sci. 6:690. doi: 10.3389/fmars.2019.00690
Received: 29 October 2018; Accepted: 28 October 2019;
Published: 12 November 2019.
Edited by:
Thomas K. Frazer, University of Florida, United StatesReviewed by:
Douglas Fenner, Independent Researcher, Pago Pago, American SamoaCharles Alan Jacoby, St. Johns River Water Management District, United States
Copyright © 2019 Agudo-Adriani, Cappelletto, Cavada-Blanco and Cróquer. This is an open-access article distributed under the terms of the Creative Commons Attribution License (CC BY). The use, distribution or reproduction in other forums is permitted, provided the original author(s) and the copyright owner(s) are credited and that the original publication in this journal is cited, in accordance with accepted academic practice. No use, distribution or reproduction is permitted which does not comply with these terms.
*Correspondence: Esteban A. Agudo-Adriani, ZWFndWRvQGxpdmUudW5jLmVkdQ==; Aldo Cróquer, YWxkby5jcm9xdWVyQHRuYy5vcmc=; YWNyb3F1ZXJAdXNiLnZl