- 1The University of Western Australia Oceans Institute, School of Biological Sciences, Indian Ocean Marine Research Centre, The University of Western Australia, Perth, WA, Australia
- 2School of Life and Environmental Sciences, Deakin University, Geelong, VIC, Australia
- 3Marine Biological Association of the United Kingdom, Plymouth, United Kingdom
- 4Ocean and Earth Science, National Oceanography Centre Southampton, University of Southampton, Southampton, United Kingdom
- 5Centre for Biological Sciences, University of Southampton, Southampton, United Kingdom
- 6Instituto de Física Interdisciplinar y Sistemas Complejos IFISC (CSIC-UIB), Palma, Spain
- 7ISI Foundation, Turin, Italy
- 8Australian Institute of Marine Science, Townsville, QLD, Australia
- 9Department of Biological Sciences, Macquarie University, Sydney, NSW, Australia
- 10The University of Western Australia Oceans Institute, Oceans Graduate School, Indian Ocean Marine Research Centre, The University of Western Australia, Perth, WA, Australia
- 11CIBIO/InBIO, Centro de Investigação em Biodiversidade e Recursos Genéticos, Universidade do Porto, Vairão, Portugal
- 12Department of Ecology and Evolutionary Biology, University of California, Santa Cruz, Santa Cruz, CA, United States
- 13Australian Institute of Marine Science, Indian Ocean Marine Research Centre (M096), The University of Western Australia, Perth, WA, Australia
- 14South Australian Research and Development Institute, Henley Beach, SA, Australia
- 15Ecology and Biodiversity Centre, Institute for Marine and Antarctic Studies, University of Tasmania, Hobart, TAS, Australia
- 16Alaska Ecosystems Program, National Marine Mammal Laboratory, National Oceanic and Atmospheric Administration Fisheries, Alaska Fisheries Science Center, Seattle, WA, United States
- 17U.S. Geological Survey, Alaska Science Center, Anchorage, AK, United States
- 18Department of Biological Sciences, San Jose State University, San Jose, CA, United States
- 19Minderoo Foundation, Dalkeith, WA, Australia
- 20Office of Naval Research, Arlington, VA, United States
- 21Red Sea Research Center and Computational Bioscience Research Center, King Abdullah University of Science and Technology, Thuwal, Saudi Arabia
Tracking data have led to evidence-based conservation of marine megafauna, but a disconnect remains between the many 1000s of individual animals that have been tracked and the use of these data in conservation and management actions. Furthermore, the focus of most conservation efforts is within Exclusive Economic Zones despite the ability of these species to move 1000s of kilometers across multiple national jurisdictions. To assist the goal of the United Nations General Assembly’s recent effort to negotiate a global treaty to conserve biodiversity on the high seas, we propose the development of a new frontier in dynamic marine spatial management. We argue that a global approach combining tracked movements of marine megafauna and human activities at-sea, and using existing and emerging technologies (e.g., through new tracking devices and big data approaches) can be applied to deliver near real-time diagnostics on existing risks and threats to mitigate global risks for marine megafauna. With technology developments over the next decade expected to catalyze the potential to survey marine animals and human activities in ever more detail and at global scales, the development of dynamic predictive tools based on near real-time tracking and environmental data will become crucial to address increasing risks. Such global tools for dynamic spatial and temporal management will, however, require extensive synoptic data updates and will be dependent on a shift to a culture of data sharing and open access. We propose a global mechanism to store and make such data available in near real-time, enabling a holistic view of space use by marine megafauna and humans that would significantly accelerate efforts to mitigate impacts and improve conservation and management of marine megafauna.
Introduction
The 35-year old Convention on the Conservation of Migratory Species of Wild Animals (CMS) aims to conserve terrestrial, aquatic, and avian migratory species throughout their range. The October 2017 CMS meeting in Manila, Philippines, highlighted current conservation concerns for migratory marine species by concluding that “these species face multiple anthropogenic threats over vast areas, including bycatch, over-harvesting, pollution, habitat degradation, marine noise impacts, and climate change” (CMS, 2017). The recent meeting of the Convention on Biological Diversity1 (Egypt, November 2018), an international treaty dedicated to promote sustainable development while maintaining the rich diversity of life on Earth, went further, calling for information sharing of scientific data on ecologically significant marine areas that are key for guiding area-based management across international organizations, including fishery agencies.
The approach of area-based management in the context of marine megafauna (e.g., mammals, seabirds, teleost fishes, sharks, reptiles) recognizes that these animals can move great distances and face a plethora of threats overlapping ecologically important areas. Such threats are already affecting populations across multiple space and time scales (McCauley et al., 2015). Therefore, identifying areas important to marine megafauna, for example in feeding or reproduction, presents major challenges for conservation because these seascapes are often located over large areas remote from observation or potential enforcement (Queiroz et al., 2016, 2019). To date, much highly relevant information about their movement, distribution and ecology in relation to anthropogenic threats has been collected using telemetry and bio-logging devices and holds tremendous potential for aiding conservation (Hays et al., 2019). However, most of these data are dispersed globally among researchers and other stakeholders, or are generally not available at the spatial scales relevant to populations. This lack of organization is likely to create problems in the future by slowing the accessibility and use of large quantities of near real-time data that could be used scientifically to underpin a step change in how marine megafauna are managed on the high seas in Areas Beyond National Jurisdiction (ABNJ).
To partly address such concerns, the United Nations General Assembly has launched negotiations to develop a treaty elaborating on the obligations described under the United Nations Convention on the Law of the Sea (UNCLOS articles 116-119 and 192). These negotiations, which began in September 2018 and are intended to close in mid-20202, aim to tackle the increasing number of threats in marine areas and to develop a mechanism for the creation of area-based management tools for the high seas in ABNJ. Other elements of the treaty will include provisions for environmental impact assessments, as well as, more focused mechanisms for capacity building and development and transfer of marine technology. Its main objective is to devise an international, legally binding instrument for the conservation and sustainable use of marine biodiversity, particularly in the high seas (for more details refer to Wright et al., 2018), referred to as Biodiversity Beyond National Jurisdictions (BBNJ).
Animal tracking data have been used to inform evidence-based conservation at local and regional scales in marine ecosystems (Hays et al., 2019). Examples include tracking data of Australian sea lions (Neophoca cinerea), which were used to quantify overlap with fisheries and bycatch impacts leading to targeted fisheries closures off South Australia (Goldsworthy et al., 2010), satellite data for leatherback (Dermochelys coriacea) and loggerhead turtles (Caretta caretta), which provided a framework for mitigating fisheries interactions off Hawaii (Howell et al., 2015), and the tracked movements of southern bluefin tuna (Thunnus maccoyii) in eastern Australia, which informed management to limit unwanted tuna captures in the longline fishery (Hobday et al., 2010) (Figure 1). Nevertheless, a disconnect remains between the large volume of extant tracking data and its use in conservation and management (Hays et al., 2016, 2019), and efforts thus far, focus mostly on conservation within exclusive economic zones (EEZs) largely ignoring the high seas (Harrison et al., 2018).
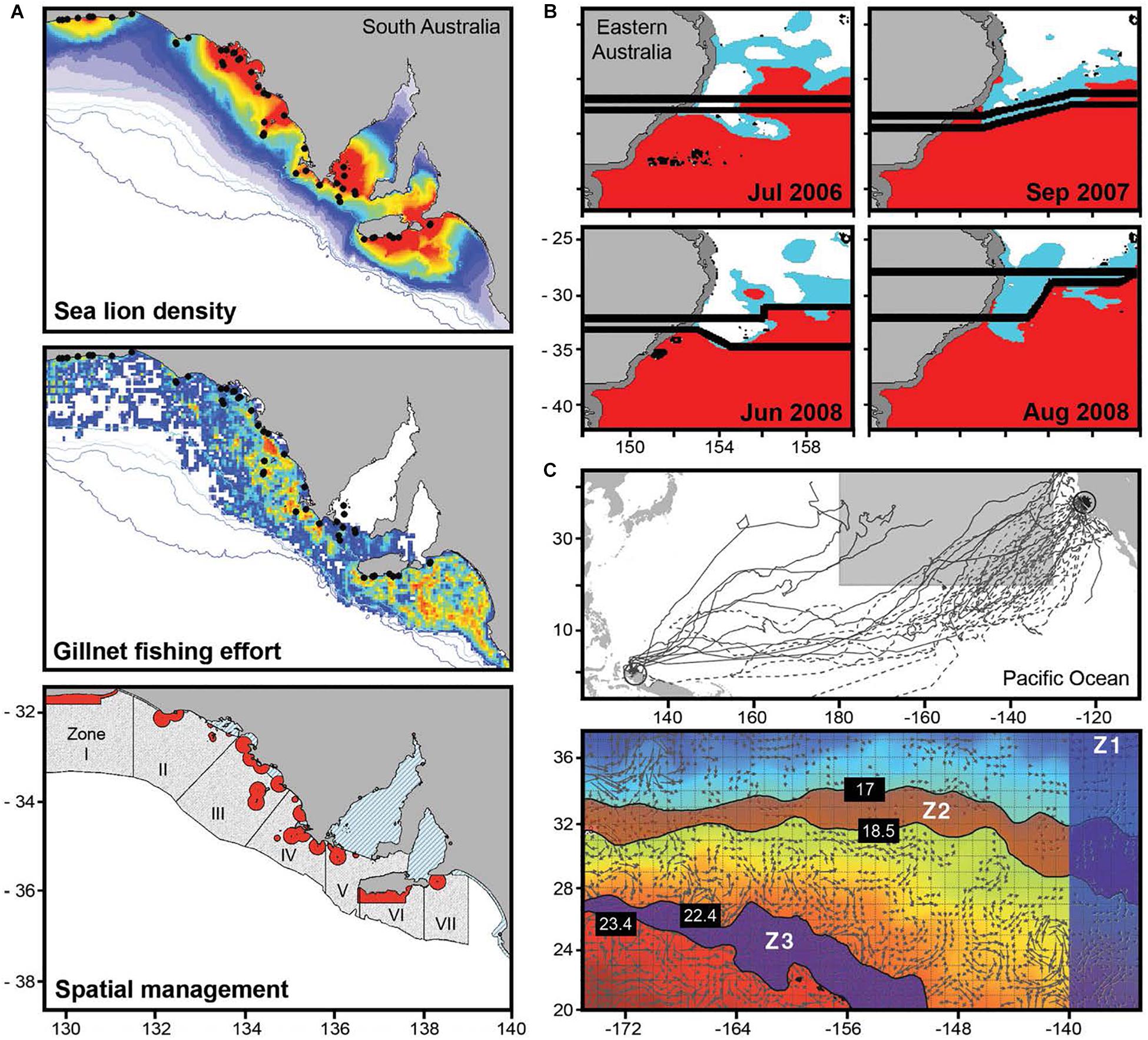
Figure 1. Impact of tracking data in the management of demersal gillnet fisheries in south Australia (A), eastern Australia longline fisheries (B), and pelagic longline fisheries in Hawaii (C). (A) Shows the detailed modeled at-sea density of Australian sea lions based on the combination of tracked movements from over 200 individuals from 48 breeding sites (black circles) with population models (top), which was integrated into a bycatch estimation model with the spatial distribution of demersal gillnet fishing effort (center) to estimate bycatch and develop spatial closures around all breeding colonies (in red, bottom) and bycatch trigger limits that enact temporal closures across seven zones (light gray, bottom) (adapted from Goldsworthy et al., 2010 and http://afma.gov.au). (B) Illustrates how management lines (black) that separate habitat types for southern bluefin tuna have been shifted according to habitat suitability predictions derived from tracking data. The figure shows habitat classed as ‘core’ where most tuna are predicted (80% suitability in red), ‘buffer’ representing a habitat transition zone (15% suitability in blue), and ‘OK’ where fewer tunas are expected (5% suitability in white) (adapted from Hobday et al., 2010). (C) Tracks of leatherback turtles (upper panel; n = 37, black solid and dashed lines) in relation to the region where > 95% of the Hawaii-based pelagic longline fishery operates (shaded box), and an example figure from TurtleWatch (lower panel) combining daily sea surface temperature (SST) and ocean current data with predicted locations of turtles. Zones Z1–Z3 are regions to avoid so as to reduce interactions with turtles (adapted from Howell et al., 2015).
Although at-sea tracking data exist for animal movements at regional and near global scales (Block et al., 2011; Hindell et al., 2018; Sequeira et al., 2018; Queiroz et al., 2019), efforts to identify dynamic shifts in the location of Areas of Ecological Significance over weeks to seasons and between years, and across ocean-basin scales, remains a most important challenge (Scales et al., 2014). Meeting this challenge with innovative approaches, technology and data will provide policy makers and marine managers with a new view of how marine megafauna respond to environmental changes, and will reveal spatio-temporal overlaps with anthropogenic threats.
Here, we argue that a global approach utilizing existing and emerging technologies (Thums et al., 2018), including artificial intelligence for data mining and pattern analyses, and big data approaches for synthesizing global datasets (e.g., Rodríguez et al., 2017), can be applied to deliver near real-time diagnostics on risks and threats at a global scale based on tracking movements of both marine megafauna (e.g., through animal-attached electronic tags) and human activities at sea (e.g., through automatic identification systems). Together with increasing our understanding of how megafauna movements vary with environmental changes, the global approach we propose would provide a new frontier in dynamic marine spatial management to help reduce potential impacts on migratory marine megafauna and allow great improvements to the conservation and management of these species. Such data could inform the designation and administration of dynamic area-based management tools, including marine protected areas (MPAs), and could also inform the development of long-term environmental impact assessments and larger scale strategic and regional environmental assessments, directly addressing key goals of the proposed UN treaty.
Potential of New Technology and Tracking Data
Marine vertebrates move over a range of spatial scales, often traveling many 1000s of kilometers through multiple national jurisdictions across EEZs and ABNJ, using areas well beyond the extent of even the world’s largest MPAs (Harrison et al., 2018). Tracking data enable researchers to quantify these scales of movement with the potential to follow individual animals for many months, or even years (Block et al., 2011; Lea et al., 2015; Abrahms et al., 2018).
Near Real-Time Tracking Data
Although large amounts of tracking data are currently available, these data and resulting analyses are typically unavailable to managers in near real-time or at the same temporal and spatial scales as changing threats, mostly because we lack a mechanism to integrate and manage data in real-time. Some types of tracking data need considerable post-processing, but the increasing use of GPS, especially to track vessels, can be of direct use with little processing. Such data can therefore, usefully highlight co-occurrence of threats across distributional ranges of megafauna, which in turn can be defined based on reliable dynamic habitat models that statistically relate the movements and distributions of tracked animals to remotely sensed oceanographic drivers in near real-time (Hazen et al., 2013, 2018; Hobday et al., 2014; Maxwell et al., 2015). Using such environmental data will facilitate defining and mapping dynamic areas with a high probability of species being present and can be complemented with probabilistic kernel methods to predict their occurrence at a given location at a specific time in the future (sensu Do et al., 2015). Hence, near real-time synoptic maps of modeled remotely sensed oceanographic variables that drive space use patterns of particular species can provide managers with information on where the greatest threats are likely co-occurring with megafauna.
Near real-time access to available spatial data on animal movement would no doubt lead to better informed management initiatives to reduce risks to marine megafauna. For example, knowing precisely where and when threatened marine megafauna migrate into areas with higher risk of capture, such as in longline fishing hotspots, could provide managers with data to inform target or bycatch reduction measures, including mobile protection zones or time-area closures to fisheries. Recently, spatial overlap between hotspots of shark space use and fishing effort was mapped showing overlaps as high as 76% for some species and highlighting limited refugia free from fishing are available to sharks in the open ocean (Queiroz et al., 2019). However, these estimates of overlap were available only several years after they occurred, reducing the likely success of time-dependent management interventions. Such interventions may become increasingly necessary in the future as marine megafauna movements and distributions shift in response to climate-driven environmental changes. At the same time, it is important that real-time tracking data are released in a tractable way, so it is not used to increase harvesting, e.g., by allowing fishermen to target their operations in high-use areas where they know there are tracked animals.
Dynamic Management Tools
The development of dynamic predictive products based on near real-time tracking and environmental data (e.g., using remotely sensed oceanography or outputs of forecasting circulation models) are likely to become increasingly important management tools. These will be crucial in the near future to tackle and mitigate risks for marine vertebrates (Zydelis et al., 2011; Howell et al., 2015; Hazen et al., 2017), particularly on the high seas. Such dynamic management tools offer the opportunity to develop adaptive management strategies that change in space and time to follow animals as they move through the ocean, and to maximize efficacy (i.e., as opposed to area-based closures for species conservation purposes). For example, recent studies showed that dynamic ocean management has the potential to reduce negative costs associated with static management, being significantly more efficient with fewer impacts on fishermen (Dunn et al., 2016) and therefore more useful for the management of highly migratory species (Lewison et al., 2015; Maxwell et al., 2015). Examples of existing dynamic tools include TurtleWatch3 (Howell et al., 2015), which allows the dynamic management of bycatch of leatherback and loggerhead turtles in the North Pacific Ocean, and WhaleWatch4 (Hazen et al., 2017), which provides a predictive tool for blue whale (Balaenoptera musculus) density in the California Current. The development of similar tools encompassing multiple species of threatened or endangered marine megafauna, as done recently for the California Current (Hazen et al., 2018), is now needed at a global scale for many more species, and will be crucial to manage fishing and shipping impacts, as well as other threats to megafauna in the high seas (Figure 1).
Global tools for dynamic management will however require extensive synoptic data of diverse potential anthropogenic threats that are updated at appropriately frequent intervals. For instance, to assess potential impacts of fisheries, data on 1000s of shipping vessels tracked with AIS (Automatic Identification System) and VMS (Vessel Monitoring System) (McCauley et al., 2016) should be made available quickly and, ideally, free of charge. Currently, for-profit companies maintain the hardware and structures needed to run these tools, suggesting that a shift in the culture may be needed to recognize these datasets as essential for improving wildlife conservation management and to find alternative ways to maintain the services that provide them. Because ocean resources are common property it will be essential that national governments or international assemblies routinely acquire such tools for the future management of threatened species. This could potentially be achieved through governmental sponsorship, by finding for-profit replacements based on products from those datasets, or at least, by specifying free access specifically for research (e.g., based on institutional login) or for other not-for-profit activities. Alternative for-profit replacements have been very successful in changing the culture of other highly profitable industries, including for example shark fisheries that have been successfully replaced with ecotourism in a number of locations (e.g., in Palau; Vianna et al., 2012). A stream of AIS and VMS data will also need to include a broader range of vessel sizes. AIS now covers two thirds of the large vessels traveling in the ocean but does not include smaller vessels and artisanal boats that are responsible for much of the fisheries bycatch (Robards et al., 2016). Tracking those vessels will be essential to have a global representation of the extent of impacts from fishing and bycatch.
New Technologies and Innovations
With recent developments in remote sensing and satellite technology, active monitoring of fishing and other vessels, and even large marine animals, at global scale is now possible. For example, smaller fishing vessels have been monitored using on-board GPS devices (Watson et al., 2018), and the WorldView satellites have enabled the detection and enumeration of whales from space (Fretwell et al., 2014; Cubaynes et al., 2019). Rapid innovations in drone technology can also provide means for further extending the surveillance of individual vessels or animals near shore (Kelaher et al., 2019) or around structures at sea, such as oil rigs or large vessels, which can also be mapped from space. Recently developed animal-borne tags can also identify the radar emissions from ships so as to detect the presence of ships in no-fishing zones and, therefore, enable targeted policing of vessels fishing illegally (Weimerskirch et al., 2018). This is an important example of how real-time tracking data can be of great use to fishery managers even before the post-processing commonly used to increase the accuracy and reliability of location estimates and obtain representative tracks. Such an increase in surveillance of at-sea activities will also require more sophisticated approaches to classify the information obtained.
The potential to increase surveillance of animal movements at-sea will also be catalyzed over the next decade by an increase in the number of smaller and inexpensive tracking devices and the advances in satellite receiver technologies enabling greater coverage and more frequent position estimates. An important recent advance has been the development of the ICARUS5 (International Cooperation for Animal Research Using Space) receiver and transmitter. The ICARUS receiver was installed on the International Space Station (ISS) in 2018, and its lower Earth orbit means lower energy is required by tags to communicate with it allowing for reductions in the size of tags as batteries for powering transmissions can be smaller. Alongside this, the very small ICARUS transmitter (weighing only 5 g), which is also solar-powered, will allow greater numbers of animals, including many smaller species about which little is known, to be tracked globally. Developments like ICARUS, and new sensor technologies, will make species location more affordable and accessible.
There are other technologies in advanced development that also hold particular promise for revolutionizing the global tracking of marine vertebrates. The Argos system6 has been central to key discoveries about marine megafauna ecology over the last few decades (for review see Hussey et al., 2015; Thums et al., 2018). New Argos receivers deployed on satellites starting in 2019 will allow tracking of very low power transmitters, thus reducing tag size for work on smaller species, or prolonging operational track times by maintaining existing tag package size but with provision of a greater battery load. Development is also underway for a new constellation of 20 dedicated nanosatellites (e.g., kineis.com) that will enable global localization and connectivity of several million small, mobile devices with low-power-consumption. Moreover, Argos coverage and throughput of data from satellite tags can be increased by using Motes (Jeanniard-du-Dot et al., 2017). Motes are stationary listening stations based on the ground (rather than on a satellite) that can log telemetry data captured within the range of the Mote (∼55 km). Collectively, such innovations present the possibility for longer track durations (years) and greater track resolutions (more locations per day) for global monitoring marine megafauna in near real-time and for better understanding the global threats faced by these species.
Threats to Marine Megafauna at Global Scale
Increasing fishing intensity, shipping density, plastic pollution, and warming represent four major threats to many marine species, including seabirds (e.g., Dias et al., 2019), sharks (Queiroz et al., 2019), and cetaceans (Harcourt et al., 2019b), that are now occurring at the full extent of the globe (Figures 2A–D). These threats, which can influence each other (e.g., Arctic ice melting due to climate change is leading to new shipping routes), are highly dynamic in space and time (e.g., Molinos et al., 2016), and occur simultaneously in most of the global ocean. Therefore, understanding levels of risk for marine megafauna will require dynamic approaches that also address these threats cumulatively (e.g., Maxwell et al., 2013).
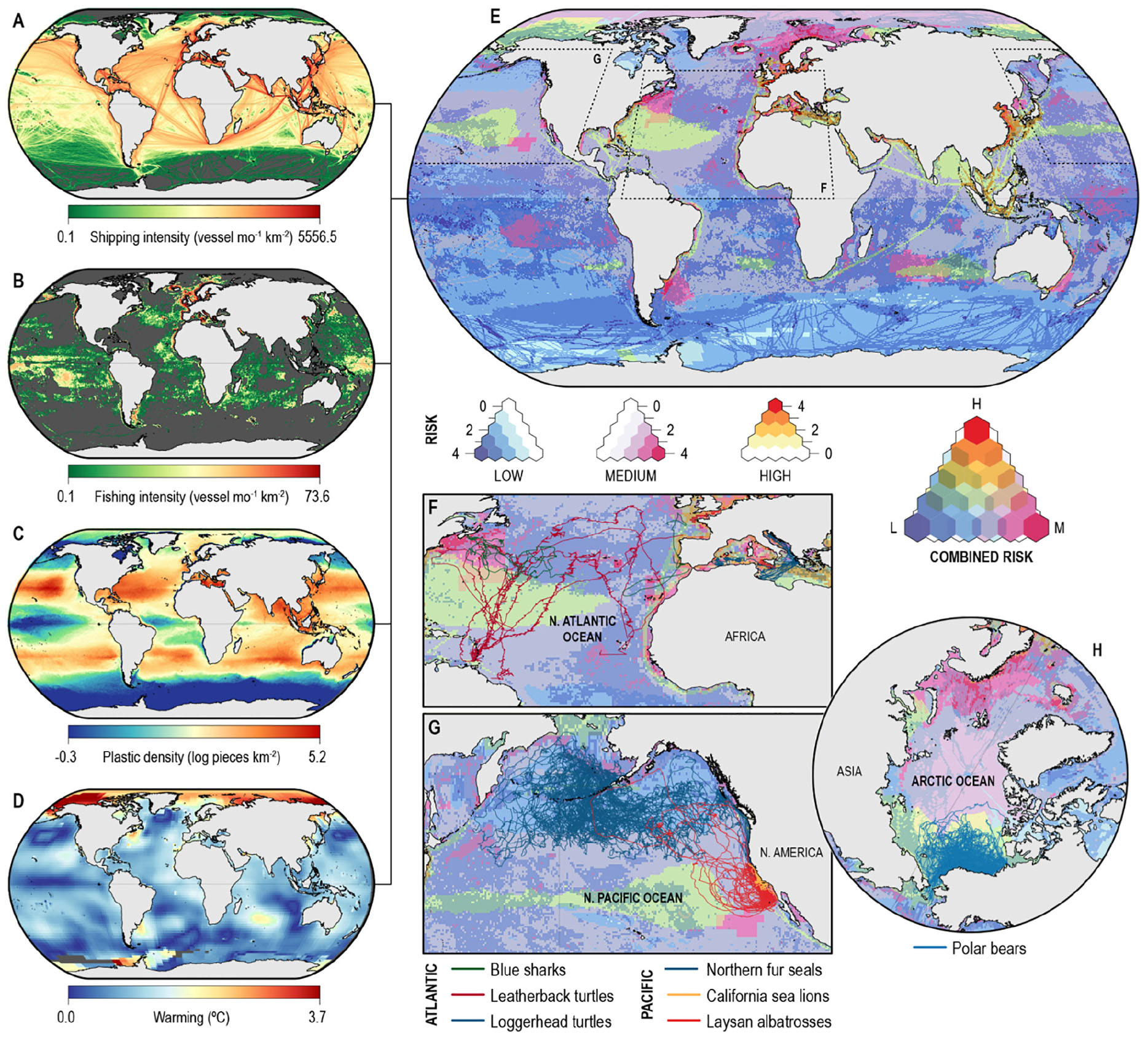
Figure 2. Global threats and potential for overlap with animal trajectories in the ocean (A–D) show the global distribution of shipping density (exactearth.com), fishing intensity (marinetraffic.com), plastic density (Eriksen et al., 2014), and warming (http://data.giss.nasa.gov/gistemp) (Hansen et al., 2010) in the world’s oceans, respectively. (E) Shows the overlap of threats presented in (A–D) with the prevalent color reflecting categories of risk as low (blue), medium (magenta), and red (high) and color intensity revealing the number of threats (1–4) in each category with 0 represented in white. (F–H) Show examples of overlaying marine megafauna tracks (lines) on the overlapped threats, showing a combination of all categories in the northern Atlantic (F) where blue sharks (Prionace glauca) and leatherback turtles (Dermochelys coriacea) migrate. (G) Highlights overlap of threats with different risk levels, including medium (magenta), relatively low (blue), and a patch where at least one of the risks is high (green) in the northern Pacific Ocean where California sea lions (Zalophus californianus), northern fur seals (Callorhinus ursinus), and Laysan albatrosses (Phoebastria immutabilis) travel, and (H) emphasizes the combination of a range of medium to high level of risks across the Chukchi Sea, Arctic Ocean, where Alaskan populations of polar bears (Ursus maritimus) move. These examples suggest that knowledge of where and when megafauna trajectories intersect locations prone to impacts from such threats can inform management actions to assist mitigating risks.
The development of a tiered system to classify global threats could greatly assist interpretation and analysis of the potential spatial extent of impacts. In Figure 2, we illustrate a simple approach to represent the cumulative risk from the different threats in different parts of the globe, specifically focusing on relevant threats to marine megafauna species. This figure highlights the different levels of threats and potential risk faced by marine megafauna while moving through the open ocean, after categorizing each threat as low, medium, and high risk. We used a 0.5° grid, and for any given grid cell we compared the difference between the value for each threat in that grid cell and the minimum value observed for that threat, with the difference between the minimum and the maximum observed values. This results in a ratio for the risk (R) expected in each grid cell (i) from each threat (j):
where A represents, for shipping density, fishing intensity, or plastic density, the logarithm of the non-null values for the threat being considered, and for warming, the non-null values for the expected temperature difference. We then calculated the probability density function for each risk and bounded the resulting function into three equal sections covering 1/3 of the values for each threat (refer to Supplementary Figure S1). We estimated the risk as ‘No risk’ if the observed value for the threat was null, ‘Low’ if R < 1/3, ‘Medium’ if 1/3 ≤ R < 2/3, and ‘High’ if R ≥ 2/3, with 1/3 and 2/3 referring to the split between each three equal sections containing the threat values (Supplementary Figure S1). We used these categories to represent the risk in each grid-cell. After classifying each threat into one of these four categories, we represented the combined risk by combining the number of threats in each category. We then simply mapped examples of existing tracks on the map with the cumulative risks to highlight that individuals travel through areas with different threats and where multiple threats occur synchronously. Areas with medium to high risk for all threats considered include the Mediterranean Sea, South East Asian, and Eastern United States waters (Figure 2E). Examples of animals that cross areas with different levels of risk are shown in Figures 2F–H, and include blue sharks, polar bears, and leatherback and loggerhead turtles.
Because different threats present different risk levels to different species, the application of a tiered system detailed above and in Figure 2, should be developed to classify threats specifically for each species, or at least, each taxa group. For instance, different types of vessels (e.g., fishing or seismic survey vessels) can be identified based on their pattern of tracked movements (Queiroz et al., 2016; Kroodsma et al., 2018), and could be sorted into categories of threats for particular marine megafauna. Such classification would be useful to identify movement associated for example with deployment of longlines, which would receive the highest-level threat category for sharks and some seabirds, or movement associated with large vessel transiting (e.g., tankers), which should receive a high-level threat category for whales and many other air-breathing species due to collision potential. These categories of fishing threats could be mapped automatically using detection algorithms (see Queiroz et al., 2016), particularly if the precise location of deployed fishing gear is available. The same type of threat-tiered system could also be applied to all other threats encountered by marine megafauna.
Addressing Issues of Global Governance
The technical advances described above will enable the tracking of a greater range of marine vertebrate species (e.g., extending to smaller taxa than now), and the increase in tracked individuals will also make studies at the level of whole-populations over multiple years possible for the first time. It is now timely to define recommendations for using the data and information that will derive from new technologies and include specific requirements within the international treaty being negotiated by the United Nations General Assembly. This treaty has the capacity to address issues of governance in the high seas, and to enhance implementation of informed and coordinated decisions on the sustainable use of marine resources (i.e., Marine Spatial Planning), including the necessary tools (and data) for dynamic management. For example, such developments in satellite tracking and resulting data could enable comprehensive long-term assessments of the efficacy of the 13,000 MPAs established worldwide (as of 2018) (O’Leary et al., 2018) in protecting migratory species. This will be relevant even in cases where the MPAs were not specifically focused on conserving highly migratory species, as it will assist in the identification of complementary measures needed for species conservation which will add value to the MPAs’ existence. Given that designated large-scale marine protected areas (LSMPAs) (sensu Spalding et al., 2007, i.e., large marine ecosystems ≥ 200,000 km2 used as units for practical application of management and with distinct characteristics) make up about 70% of the areas of ocean and EEZs that are included within current MPAs, a step change in satellite tracking capability combined with open access and near real-time accessibility will assist the protection of highly migratory marine vertebrates. This will directly address a major goal of the United Nations to develop an internationally legally binding instrument to conserve and sustain marine biodiversity (from ecosystems to genes) beyond national jurisdictions (BBNJ), and to clarify the level of protection afforded by area-based management tools from scales of days to years and from local to global. In so doing, this will allow extension of the concept of marine spatial planning (Douvere, 2008), thus far applied only locally and regionally, to be truly at the global ocean scale and to enable the incorporation of dynamic spatial management at those scales. Moreover, dynamic corridor protective measures to address highly migratory species’ connectivity between major areas for their occurrence could complement LSMPAs.
Global Data Gaps
A global mechanism to make available near real-time tracking data from animals and human activities that will be realized from the technological developments already mentioned, would significantly accelerate efforts to mitigate impacts and improve conservation and management of marine megafauna. It also would allow identification of spatial and taxonomic gaps in movement data to inform targeted data collection in the future and to identify countries that need assistance to develop their animal tracking programs and to contribute to the global dataset. For example, this will be particularly relevant to highlight coastal environments with high population density and high level of industrial activities at sea, but where tracking data may be sparse or non-accessible, and to identify and assign shared responsibilities for protection of species that spend time in multiple jurisdictions (Harrison et al., 2018). Furthermore, it could highlight the lack of data around developing nations that have limited means to monitor their natural resources and any shifts in distributions or declines in abundance of megafauna (Queiroz et al., 2019). The creation of affordable devices highlighted previously, will also allow researchers in developing nations to gain the ability to track megafauna occurring in their waters, and couple it with freely available near real-time vessel data to improve fisheries surveillance, for example. Additionally long-term trends in movement patterns, such as those associated with climate change, will be more easily assessed (Laidre et al., 2015; Hauser et al., 2017).
Biogeographic shifts in species occurrence are expected to occur with climate change, meaning that species might change their movement patterns, thereby gaining or losing habitat across different EEZs and high sea areas (Hazen et al., 2013). Changes in distribution are also expected to result from the predicted decline in ocean productivity as the oceans continue to warm (Kwiatkowski et al., 2017). Although these changes will have an impact on marine megafauna, many species can also be used as ‘observers’ of the environment and even act as sentinels for environmental change (Piersma and Lindstrom, 2004). Such dynamic changes, which are already taking place in the marine environment, further highlight the major role that dynamic management tools could play in informing climate related shifts in both fishing activities and patterns of habitat use by migratory marine megafauna (Hazen et al., 2013, 2018).
Other effects of climate change that could be detected include long-term modifications in the seascape that can lead to further changes in marine megafauna pathways. For example, the use of the Arctic Sea by shipping has already begun and the route has the potential to become a new shipping highway (Eguiluz et al., 2016; Hauser et al., 2018). Such changes in human activities can pose greater threats to animals using the Arctic environments (e.g., polar bears) (Pagano et al., 2018) beyond those already expected directly through climate change itself (e.g., melting of sea ice). Similar concerns apply in other ecologically important locations for marine megafauna impacted by heatwaves, and where human activities are increasing (e.g., Great Barrier Reef) (Hughes et al., 2018; Smale et al., 2019). The global mechanism and dynamic spatial management we propose would also accelerate our understanding of the full spatial extent of impacts from anthropogenic activities on marine megafauna. Because of the large geographic ranges of many of these species, there is still considerable uncertainty on the cause-effect relationships and short or long term impacts of threats associated with global change.
Need for Improved Data Sharing and Open Access
With global change happening at an increasing rate (Molinos et al., 2016; Poloczanska et al., 2016), sharing of existing data is becoming vital to tackle the growing challenges facing the management and conservation of marine megafauna (Thums et al., 2018; Harcourt et al., 2019a). Although data sharing has been recognized as imperative (Nguyen et al., 2017) to assist this process of integration, it is only slowly being implemented with a number of national efforts [e.g., Animal Telemetry Network (ATN), in the United States; the Australian Integrated Marine Observing System (IMOS); the European Ocean Observing System (EOOS)] and many global initiatives emerging to compile movement data (refer to Harcourt et al., 2019a for a list of these initiatives). The latter comprise initiatives with very different foci, for example, Movebank7 includes mostly data from terrestrial species, the BirdLife International Seabird Tracking Database8 focuses on seabirds, seaturtle.org contains data for turtles, Zoatrack contains mostly data from the Australian region (Dwyer et al., 2015) and SCAR-RAATD9 (Scientific Committee for Antarctic Research – Retrospective Analysis of Antarctic Tracking Data) is concerned with megafauna in the Antarctic region. Other existing initiatives with broader focus encompassing multiple taxa at the global scale, such as OBIS-SEAMAP10 (Ocean Biogeographic Information System – Spatial Ecological Analysis of Megavertebrate Populations), and the recent MiCO11 (Migratory Connectivity), have been successful to some extent, but have not alleviated issues of governance and of data ownership that can limit participation in such databases. To specifically address this, consortia such as MMMAP12 (Marine Megafauna Movement Analytical Program) and the Global Shark Movement Project13, are now directly engaging data providers as co-authors in the outputs derived from their datasets as an incentive to promote data sharing among vast, distributed networks of researchers in 100s of institutes across continents (Sequeira et al., 2018; Queiroz et al., 2019). This approach highlights that any potential mechanism to promote data sharing will need to ensure that data providers retain ownership of their data and have a say in the outputs resulting from their data. Nevertheless, current impediments to advance these efforts to enhance animal tracking data sharing include the lack of universal data standards to facilitate consolidation of datasets across technologies and methods, concerns for misuse or misinterpretation of the data if accessed without knowledge of critical context or accuracy, cross-jurisdictional standardization, sustained funding, and sovereignty over valuable resources (including knowledge). Most importantly, researchers in this field are increasingly realizing the value of data-sharing as a requirement to move analyses into the realm of big data (Thums et al., 2018). This is vital as the volume and types of data that can be obtained through tags increases. Given the ecological importance of marine megafauna and the fact that many species are threatened and highly migratory, the inability to use tracking data to their full potential is a wasted opportunity.
Recent research projects synthesizing large existing tracking datasets (Sequeira et al., 2018; Queiroz et al., 2019), are highlighting the power of bringing such datasets together, and revolutionizing the way tracking data analyses can be approached. There is, in theory, an abundance of movement data available to inform conservation and management, with the likelihood of even greater volumes of data being collected in the next ‘bio-logging decade’ (Lowerre-Barbieri et al., 2019). Currently, tracking data are generated by individual research groups with a growing tendency to manage the data through multiple online repositories (Campbell et al., 2016). However, these data are often not openly available to researchers (not even in metadata form), are incomplete (e.g., location data only), and there is no single common resource or data standard for archiving to facilitate the process of finding existing data. This hinders locating and accessing data, leading to tracking data remaining scattered and underutilized for the support of policy and management actions (Hays et al., 2019). A coordinated, collaborative, and strategic approach to the uptake and analysis of tracking data, including delivery of integrated products (e.g., megafauna space use and fishing effort overlap) to users in near real-time, will initiate an effective link between research, conservation, and management actions. Emerging projects initiatives are now trying to address the gap between data availability and potential use by policy makers, with strong focus on migratory pathways and species connectivity (e.g., mico.eco and gobi.org). However, to achieve a coherent evidence-based conservation strategy, we suggest that existing and future tracking datasets be made available within a global repository, including open-access data or metadata, so that existing data can be discovered, accessed, and used to support management decisions (e.g., Important Marine Mammal Areas, and Important Bird Areas), as outlined at the CMS COP12 (CMS, 2017).
A starting point for such a global repository could be based only on metadata using peer-to-peer technologies such as blockchain (e.g., FOAM14) where the risks of data management being held centrally are removed. Blockchain technologies store information across a network of computers and data is transferred directly from peer-to-peer, eliminating the need for a centralized data management company and making data access faster and cheaper. The decentralized structure of the network requires verification and approval from multiple computers prior to adding or editing data (Christidis and Devetsikiotis, 2016) making these peer-to-peer databases safe and secure. The use of technologies such as blockchain would allow easy discovery of datasets within a single location, and could act as a gateway to freely available data, but protocols for data standardization would still be required. ISO (International Organisation for Standardisation) standards (19110/19115) for biological data, as well as existing conventions for data discovery (e.g., http://wiki.esipfed.org/index.php/Attribute_Convention_for_Data_Discovery_1-3), whilst not comprehensive, can provide a starting point that can be further refined and tailored to tracking data (e.g., as used by ATN15). Ideally, data should be made open access through a single mechanism pointing to existing databases or a single repository akin to GenBank16. Additionally, improved fisheries and shipping data sharing would provide an increased opportunity to enhance sustainable management and to encourage cooperation and collaboration across sectors to better inform policy makers.
Actionable Recommendations
Tracks of marine megafauna, human activities at sea, and of changes to their dynamic environment, need to be combined to allow near real-time assessments of potential impacts to enable time-relevant management decisions. Such potential impacts can result from overlap between high fishing effort and megafauna hotspots, changes due to marine heatwaves associated with climate change, or industrial developments through creation of structures at-sea that might interfere with migratory paths of marine megafauna. Here, we suggest that a roadmap to meet the conservation concerns for marine migratory species highlighted by the Convention on Migratory Species in 2017, the need for the sharing of scientific data raised by the Convention on Biological Diversity in 2018, and to advance the objectives of the United Nations treaty now under negotiation, should include: (i) research development of dynamic assessment tools to address potential near real-time overlap between human activities at sea and areas of ecological importance for marine megafauna; (ii) investment in more, smaller, cheaper tags, and satellite observations to create a seascape of marine megafauna movements that can highlight potential changes already associated with climate change; (iii) international cooperation to fund near real-time monitoring in regions with severe data gaps and to sustain and expand current efforts; and (iv) global data sharing via a central mechanism (akin to GenBank, or specifically to allow networking of existing or developing databases), which can provide data in near real-time. We view the recent collaborative projects (e.g., Sequeira et al., 2018; Queiroz et al., 2019) as an important step on the path to making sure that all the available data are preserved, and as preceding the era where tracking datasets are made available through a global repository that will ensure the value of tracking data is maximized in the future for supporting management decisions.
We also advocate for stewardship of such a global resource to store and share tracking data, with clearly outlined governance, appropriate resourcing, and defined pathways for implementation, including: (i) adoption of agreed standards for reporting and documenting of data (including standardization of metadata); (ii) clear requirements for access to data; (iii) guarantee of uniqueness of datasets (e.g., through a unique digital object identifier as already used in some repositories (e.g., Movebank or ATN) to avoid data replication, and (iv) networking of existing or developing databases in support of a global open-access initiative. Overall, such a resource would also lead to tracking data being made available in a format that can be used effectively to inform policy makers. It would also help to ensure that tracking datasets are not lost when researchers retire or move to other fields, while allowing for inclusion of the many datasets that are never formally published beyond ‘gray literature.’
Conclusion
A global resource for storing and sharing tracking data at a global scale has the potential to provide a holistic view of space-use by marine megafauna and human-activities, and thus to identify threats across multiple taxa allowing key zones of multi-species space use (‘hotspots’) to be identified objectively in relation to stressor gradients, and facilitate timely interventions (e.g., against illegal fishing). Such a repository can provide individual researchers or groups a way to store and manage their datasets while keeping engaged with potential outputs resulting from their data contributions and allow engagement between multiple groups worldwide to address questions of global relevance, which are impossible to address when datasets remain scattered. Moreover, bringing all existing datasets together in one global repository would allow a clear assessment of how much more tracking data are needed and for which species. This will enhance efforts of collating existing data, help optimize the number of animals tracked, and minimize duplication of effort, to the benefit of our ethical responsibilities that arise from tagging and tracking wild animals (e.g., marinemammalscience.org/about-us/ethics), where a balance between the risks, consequences and benefits needs to be carefully considered (Hays et al., 2016).
Many nations have now committed through conventions (e.g., CMS) to the conservation of large migratory marine animals. Despite its growing volume, the inaccessibility of global tracking data (of animals and other ocean users) hinders developments that would make the provisions in these conventions more effective. A key point is that important questions about how much information is available or lacking are not yet answered, however, we now have a good idea of how much data are needed to address specific questions (Sequeira et al., 2019). Many questions will, however, require 1000s of tracks, particularly those associated with broad scale biodiversity assessments. A shift in the culture of agencies that fund animal tracking research, the journals that publish the research findings, and the scientists that undertake the work, to fully embrace the concept of open access data is imperative for achieving the conservation of these iconic ocean-roaming species that by definition are a globally-shared responsibility.
Author Contributions
AS obtained funding and organized the workshop with help from CD. AS, GH, VE, MRH, RH, HC, NQ, DC, JF-G, LF, SG, MM, JR, MT, and CD attended the workshop and contributed to discussions when preparing the first draft of the manuscript. AP, SS, MW, M-AL, DS, NQ, DC, and GH provided tracking data used in Figure 2. VE, JPR, JF-G, JR, and AS collated datasets on global threats. NQ, JPR, VE, JF-G, HC, and AS produced figures. AS led the writing with important additions from CD, GH, and DS, and with contributions from all co-authors. All commented on the final draft of the manuscript.
Funding
Workshop funding and support granted by ARC Grant No. DE170100841, AIMS, KAUST, and UWA Oceans Institute through a Research Impact Grant. AS was supported by an ARC Grant No. DE170100841 and AIMS. DS was supported by the UK Natural Environment Research Council (NE/R00997X/1) and the Save Our Seas Foundation. NQ was supported by FCT (Portugal). HC was supported by an Australian Government RTP scholarship at UWA.
Conflict of Interest
The authors declare that the research was conducted in the absence of any commercial or financial relationships that could be construed as a potential conflict of interest.
Acknowledgments
This manuscript was conceived at the fourth workshop of the Marine Megafauna Movement Analytical Program (MMMAP) in 2018. We thank S. Pei for attending the workshop. Any use of trade, firm, or product names is for descriptive purposes only and does not reflect endorsement by the U.S. Government.
Supplementary Material
The Supplementary Material for this article can be found online at: https://www.frontiersin.org/articles/10.3389/fmars.2019.00639/full#supplementary-material
Footnotes
- ^ cbd.int/cop
- ^ bit.ly/2MKrivz
- ^ oceanwatch.pifsc.noaa.gov/turtlewatch.html
- ^ https://www.fisheries.noaa.gov/west-coast/marine-mammal-protection/whalewatch
- ^ icarus.mpg.de/en
- ^ argos-system.org
- ^ movebank.org
- ^ seabirdtracking.org
- ^ bit.ly/2XVqMNl
- ^ seamap.env.duke.edu
- ^ mico.eco
- ^ mmmap.wordpress.com
- ^ globalsharkmovement.org
- ^ foam.space
- ^ catalog.data.gov/dataset/global-tagging-of-pelagic-predators-gtopp-animal-tracking-data#sec-dates
- ^ ncbi.nlm.nih.gov/genbank
References
Abrahms, B., Hazen, E. L., Bograd, S. J., Brashares, J. S., Robinson, P. W., Scales, K. L., et al. (2018). Climate mediates the success of migration strategies in a marine predator. Ecol. Lett. 21, 63–71. doi: 10.1111/ele.12871
Block, B. A., Jonsen, D., Jorgensen, S. J., Winship, A. J., Shaffer, S. A., Bograd, S. J., et al. (2011). Tracking apex marine predator movements in a dynamic ocean. Nature 475, 86–90. doi: 10.1038/nature10082
Campbell, H. A., Urbano, F., Davidson, S., Dettki, H., and Cagnacci, F. (2016). A plea for standards in reporting data collected by animal-borne electronic devices. Anim. Biotelem. 4:1. doi: 10.1186/s40317-015-0096-x
Christidis, K., and Devetsikiotis, M. (2016). Blockchains and smart contracts for the internet of things. IEEE Access 4, 2292–2303. doi: 10.1109/ACCESS.2016.2566339
CMS, (2017). The Conference of the Parties to the Convention on the Conservation of Migratory Species of Wild Animals - Adopted by the Conference of the Parties at its 12th Meeting. Manila: UNEP/CMS.
Cubaynes, H. C., Fretwell, P. T., Bamford, C., Gerrish, L., and Jackson, J. A. (2019). Whales from space: four mysticete species described using new VHR satellite imagery. Mar. Mamm. Sci. 35, 466–491. doi: 10.1111/mms.12544
Dias, M. P., Martin, R., Pearmain, E. J., Burfield, I. J., Small, C., Phillips, R. A., et al. (2019). Threats to seabirds: a global assessment. Biol. Conserv. 237, 525–537. doi: 10.1016/j.biocon.2019.06.033
Do, T. M. T., Dousse, O., Miettinen, M., and Gatica-Perez, D. (2015). A probabilistic kernel method for human mobility prediction with smartphones. Pervasive Mob. Comput. 20, 13–28. doi: 10.1016/j.pmcj.2014.09.001
Douvere, F. (2008). The importance of marine spatial planning in advancing ecosystem-based sea use management. Mar. Policy 32, 762–771. doi: 10.1016/j.marpol.2008.03.021
Dunn, D. C., Maxwell, S. M., Boustany, A. M., and Halpin, P. N. (2016). Dynamic ocean management increases the efficiency and efficacy of fisheries management. Proc. Natl. Acad. Sci. U.S.A. 113, 668–673. doi: 10.1073/pnas.1513626113
Dwyer, R. G., Brooking, C., Brimblecombe, W., Campbell, H. A., Hunter, J., Watts, M., et al. (2015). An open web-based system for the analysis and sharing and analysis of animal tracking data. Anim. Biotelem. 3, 1–11. doi: 10.1186/s40317-014-0021-8
Eguiluz, V. M., Fernandez-Gracia, J., Irigoien, X., and Duarte, C. M. (2016). A quantitative assessment of Arctic shipping in 2010-2014. Sci. Rep. 6:30682. doi: 10.1038/srep30682
Eriksen, M., Lebreton, L. C. M., Carson, H. S., Thiel, M., Moore, C. J., Borerro, J. C., et al. (2014). Plastic pollution in the world’s oceans: more than 5 trillion plastic pieces weighing over 250,000 tons afloat at sea. PLoS One 9:e111913. doi: 10.1371/journal.pone.0111913
Fretwell, P. T., Staniland, I. J., and Forcada, J. (2014). Whales from space: counting southern right whales by satellite. PLoS One 9:e88655. doi: 10.1371/journal.pone.0088655
Goldsworthy, S. D., Page, B., Shaughnessy, P. D., and Linnane, A. (2010). Mitigating Seal Interactions in the SRLF and the Gillnet Sector SESSF in South Australia. Final Report to the Fisheries Research and Development Corporation. Adelaide: South Australian Research and Development Institute (Aquatic Sciences).
Hansen, J., Ruedy, R., Sato, M., and Lo, K. (2010). Global surface temperature change. Rev. Geophys. 48:RG4004.
Harcourt, R., Sequeira, A. M. M., Zhang, X. L., Roquet, F., Komatsu, K., Heupel, M., et al. (2019a). Animal-borne telemetry: an integral component of the ocean observing toolkit. Front. Mar. Sci. 6:326. doi: 10.3389/fmars.2019.00326
Harcourt, R., van der Hoop, J., Kraus, S., and Carroll, E. L. (2019b). Future directions in eubalaena spp.: comparative research to inform conservation. Front. Mar. Sci. 5:530. doi: 10.3389/fmars.2018.00530
Harrison, A. L., Costa, D. P., Winship, A. J., Benson, S. R., Bograd, S. J., Antolos, M., et al. (2018). The political biogeography of migratory marine predators. Nat. Ecol. Evol. 2, 1571–1578. doi: 10.1038/s41559-018-0646-8
Hauser, D. D. W., Laidre, K. L., Stafford, K. M., Stern, H. L., Suydam, R. S., and Richard, P. R. (2017). Decadal shifts in autumn migration timing by Pacific Arctic beluga whales are related to delayed annual sea ice formation. Glob. Change Biol. 23, 2206–2217. doi: 10.1111/gcb.13564
Hauser, D. D. W., Laidrea, K. L., and Sterna, H. L. (2018). Vulnerability of Arctic marine mammals to vessel traffic in the increasingly ice-free northwest passage and northern sea route. Proc. Natl. Acad. Sci. U.S.A. 115, 7617–7622. doi: 10.1073/pnas.1803543115
Hays, G., Bailey, H., Bograd, S. J., Bowe, W. D., Campagna, C., Carmichael, R. H., et al. (2019). Translating marine animal tracking data into conservation policy and management. Trends Ecol. Evol. 34, 459–473. doi: 10.1016/j.tree.2019.01.009
Hays, G. C., Ferreira, L. C., Sequeira, A. M. M., Meekan, M. G., Duarte, C. M., Bailey, H., et al. (2016). Key questions in marine megafauna movement ecology. Trends Ecol. Evol. 31, 463–475. doi: 10.1016/j.tree.2016.02.015
Hazen, E. L., Jorgensen, S., Rykaczewski, R. R., Bograd, S. J., Foley, D. G., Jonsen, I. D., et al. (2013). Predicted habitat shifts of Pacific top predators in a changing climate. Nat. Clim. Change 3, 234–238. doi: 10.1038/nclimate1686
Hazen, E. L., Palacios, D. M., Forney, K. A., Howell, E. A., Becker, E., Hoover, A. L., et al. (2017). WhaleWatch: a dynamic management tool for predicting blue whale density in the California current. J. Appl. Ecol. 54, 1415–1428. doi: 10.1111/1365-2664.12820
Hazen, E. L., Scales, K. L., Maxwell, S. M., Briscoe, D. K., Welch, H., Bograd, S. J., et al. (2018). A dynamic ocean management tool to reduce bycatch and support sustainable fisheries. Sci. Adv. 4:eaar3001. doi: 10.1126/sciadv.aar3001
Hindell, M. A., Ropert-Coudert, Y., Jonson, I., Raymond, B., Reisinger, R. R., Bornemann, H., et al. (2018). “The SCAR retrospective analysis of antarctic tracking data,” in: XXXV SCAR Biennial Meetings, Arctic Science Summit Week. (Switzwerland: IASC Business Meetings and SCAR/IASC Open Science Conference). Available at: https://epic.awi.de/id/eprint/45900/
Hobday, A. J., Hartog, J. R., Timmiss, T., and Fielding, J. (2010). Dynamic spatial zoning to manage southern bluefin tuna (Thunnus maccoyii) capture in a multi-species longline fishery. Fish. Oceanogr. 19, 243–253. doi: 10.1111/j.1365-2419.2010.00540.x
Hobday, A. J., Maxwell, S. M., Forgie, J., McDonald, J., Darby, M., Seto, K., et al. (2014). Dynamic ocean management: integrating scientific and technological capacity with law, policy, and management. Stanf. Environ. Law J. 33, 125–165.
Howell, E. A., Hoover, A., Benson, S. R., Bailey, H., Polovina, J. J., Seminoff, J. A., et al. (2015). Enhancing the TurtleWatch product for leatherback sea turtles, a dynamic habitat model for ecosystem-based management. Fish. Oceanogr. 24, 57–68. doi: 10.1111/fog.12092
Hughes, T. P., Kerry, J. T., Baird, A. H., Connolly, S. R., Dietzel, A., Eakin, C. M., et al. (2018). Global warming transforms coral reef assemblages. Nature 556, 492–496. doi: 10.1038/s41586-018-0041-2
Hussey, N. E., Kessel, S. T., Aarestrup, K., Cooke, S. J., Cowley, P. D., Fisk, A. T., et al. (2015). Aquatic animal telemetry: a panoramic window into the underwater world. Science 348, 1221–1231. doi: 10.1126/science.1255642
Jeanniard-du-Dot, T., Holland, K., Schorr, G. S., and Vo, D. (2017). Motes enhance data recovery from satellite-relayed biologgers and can facilitate collaborative research into marine habitat utilization. Anim. Biotelem. 5, 17. doi: 10.1186/s40317-017-0132-0
Kelaher, B. P., Colefax, A. P., Tagliafico, A., Bishop, M. J., Giles, A., and Butcher, P. A. (2019). Assessing variation in assemblages of large marine fauna off ocean beaches using drones. Mar. Freshw. Res. doi: 10.1071/MF18375
Kroodsma, D. A., Mayorga, J., Hochberg, T., Miller, N. A., Boerder, K., Ferretti, F., et al. (2018). Tracking the global footprint of fisheries. Science 359, 904–908. doi: 10.1126/science.aao5646
Kwiatkowski, L., Bopp, L., Aumont, O., Ciais, P., Cox, P. M., Laufkotter, C., et al. (2017). Emergent constraints on projections of declining primary production in the tropical oceans. Nat. Clim. Change 7, 355–358. doi: 10.1038/nclimate3265
Laidre, K. L., Born, E. W., Heagerty, P., Wiig, O., Stern, H., Dietz, R., et al. (2015). Shifts in female polar bear (Ursus maritimus) habitat use in East Greenland. Polar Biol. 38, 879–893. doi: 10.1007/s00300-015-1648-5
Lea, J. S. E., Wetherbee, B. M., Queiroz, N., Burnie, N., Aming, C., Sousa, L. L., et al. (2015). Repeated, long-distance migrations by a philopatric predator targeting highly contrasting ecosystems. Sci. Rep. 5:11202. doi: 10.1038/srep11202
Lewison, R., Hobday, A. J., MAxwell, S., Elliott, H., Harthog, J. R., SDunn, D. C., et al. (2015). Dynamic ocean management: identifying the critical ingredients of dynamic approaches to ocean resource management. Bioscience 65, 486–498. doi: 10.1093/biosci/biv018
Lowerre-Barbieri, S. K., Kays, R., Thorson, J. T., and Wikelski, M. (2019). The ocean’s movescape: fisheries management in the bio-logging decade (2018–2028). ICES J. Mar. Sci. 76, 477–488. doi: 10.1093/icesjms/fsy211
Maxwell, S. M., Hazen, E. L., Bograd, S. J., Halpern, B. S., Breed, G. A., Nickel, B., et al. (2013). Cumulative human impacts on marine predators. Nat. Commun. 4:2688. doi: 10.1038/ncomms3688
Maxwell, S. M., Hazen, E. L., Lewison, R. L., Dunn, D. C., Bailey, H., Bograd, S. J., et al. (2015). Dynamic ocean management: defining and conceptualizing real-time management of the ocean. Mar. Policy 58, 42–50. doi: 10.1016/j.marpol.2015.03.014
McCauley, D. J., Pinsky, M. L., Palumbi, S. R., Estes, J. A., Joyce, F. H., and Warner, R. R. (2015). Marine defaunation: animal loss in the global ocean. Science 347:1255641. doi: 10.1126/science.1255641
McCauley, D. J., Woods, P., Sullivan, B., Bergman, B., Jablonicky, C., Roan, A., et al. (2016). Marine governance: ending hide and seek at sea. Science 351, 1148–1150. doi: 10.1126/science.aad5686
Molinos, J. G., Halpern, B. S., Schoeman, D. S., Brown, C. J., Kiessling, W., Moore, P. J., et al. (2016). Climate velocity and the future global redistribution of marine biodiversity. Nat. Clim. Change 6, 83–88. doi: 10.1038/nclimate2769
Nguyen, V., Brooks, J. L., Young, N., Lennox, R. J., Haddaway, N., Whoriskey, F., et al. (2017). To share or not to share in the emerging era of big data: perspectives from fish telemetry researchers on data sharing. Can. J. Fish. Aquat. Sci. 74, 1260–1274. doi: 10.1139/cjfas-2016-0261
O’Leary, B. C., Ban, N. C., Fernandez, M., Friedlander, A. M., Garcia-Borboroglu, P., Golbuu, Y., et al. (2018). Addressing criticisms of large-scale marine protected areas. Bioscience 68, 359–370. doi: 10.1093/biosci/biy021
Pagano, A. M., Durner, G. M., Rode, K. D., Atwood, T. C., Atkinson, S. N., Peacock, E., et al. (2018). High-energy, high-fat lifestyle challenges an Arctic apex predator, the polar bear. Science 359, 568–572. doi: 10.1126/science.aan8677
Piersma, T., and Lindstrom, A. (2004). Migrating shorebirds as integrative sentinels of global environmental change. IBIS 146, 61–69. doi: 10.1111/j.1474-919X.2004.00329.x
Poloczanska, E. S., Burrows, M. T., Brown, C. J., Molinos, J. G., Halpern, B. S., Hoegh-Guldberg, O., et al. (2016). Responses of marine organisms to climate change across oceans. Front. Mar. Sci. 3:62. doi: 10.3389/fmars.2016.00062
Queiroz, N., Humphries, N. E., Couto, A., Vedor, M., da Costa, I., Sequeira, A. M. M., et al. (2019). Global spatial risk assessment of sharks under the footprint of fisheries. Nature 572, 461–466. doi: 10.1038/s41586-019-1444-4
Queiroz, N., Humphries, N. E., Mucientes, G., Hammerschlag, N., Lima, F. P., Scales, K. L., et al. (2016). Ocean-wide tracking of pelagic sharks reveals extent of overlap with longline fishing hotspots. Proc. Natl. Acad. Sci. U.S.A. 113, 1582–1587. doi: 10.1073/pnas.1510090113
Robards, M., Silber, G., Adams, J., Arroyo, J., Lorenzini, D., Schwehr, K., et al. (2016). Conservation science and policy applications of the marine vessel automatic identification system (AIS) - a review. Bull. Mar. Sci. 92, 75–103. doi: 10.5343/bms.2015.1034
Rodríguez, J. P., Fernández-Gracia, J., Thums, M., Hindell, M. A., Sequeira, A. M. M., Meekan, M. G., et al. (2017). Big data analyses reveal patterns and drivers of the movements of southern elephant seals. Sci. Rep. 7, 1–10. doi: 10.1038/s41598-017-00165-0
Scales, K. L., Miller, P. I., Hawkes, L. A., Ingram, S. N., Sims, D. W., and Votier, S. C. (2014). On the front line: frontal zones as priority at-sea conservation areas for mobile marine vertebrates. J. Appl. Ecol. 51, 1575–1583. doi: 10.1111/1365-2664.12330
Sequeira, A., Rodríguez, J., Eguíluz, V., Harcourt, R., Hindell, M., Sims, D., et al. (2018). Convergence of marine megafauna movement patterns in coastal and open oceans. Proc. Natl. Acad. Sci. U.S.A. 115, 3072–3077. doi: 10.1073/pnas.1716137115
Sequeira, A. M. M., Heupel, M. R., Lea, M. A., Eguiluz, V. M., Duarte, C. M., Meekan, M. G., et al. (2019). The importance of sample size in marine megafauna tagging studies. Ecol. Appl. 29:e01947. doi: 10.1002/eap.1947
Smale, D. A., Wernberg, T., Oliver, E. C. J., Thomsen, M., Harvey, B. P., Straub, S. C., et al. (2019). Marine heatwaves threaten global biodiversity and the provision of ecosystem services. Nat. Ecol. Evol. 9, 306–331. doi: 10.1038/s41558-019-0412-1
Spalding, M. D., Fox, H. E., Halpern, B. S., McManus, M. A., Molnar, J., Allen, G. R., et al. (2007). Marine ecoregions of the world: a bioregionalization of coastal and shelf areas. Bioscience 57, 573–583. doi: 10.1641/B570707
Thums, M., Fernandez-Gracia, J., Sequeira, A. M. M., Eguiluz, V. M., Duarte, C. M., and Meekan, M. G. (2018). How big data fast tracked human mobility research and the lessons for animal movement ecology. Front. Mar. Sci. 5:21. doi: 10.3389/fmars.2018.00021
Vianna, G. M. S., Meekan, M. G., Pannell, D. J., Marsh, S. P., and Meeuwig, J. J. (2012). Socio-economic value and community benefits from shark-diving tourism in Palau: a sustainable use of reef shark populations. Biol. Conserv. 145, 267–277. doi: 10.1016/j.biocon.2011.11.022
Watson, J. T., Haynie, A. C., Sullivan, P. J., Perruso, L., O’Farrell, S., Sanchirico, J. N., et al. (2018). Vessel monitoring systems (VMS) reveal an increase in fishing efficiency following regulatory changes in a demersal longline fishery. Fish. Res. 207, 85–94. doi: 10.1016/j.fishres.2018.06.006
Weimerskirch, H., Filippi, D. P., Collet, J., Waugh, S. M., and Patrick, S. C. (2018). Use of radar detectors to track attendance of albatrosses at fishing vessels. Conserv. Biol. 32, 240–245. doi: 10.1016/j.fishres.2018.06.006
Wright, G., Rochette, J., Gjerde, K., and Seeger, I. (2018). The long and Winding Road: Negotiating a Treaty for the Conservation and Sustainable Use of Marine Biodiversity in Areas Beyond National Jurisdiction. Paris: IDDRI.
Keywords: global ocean conservation and policy, real-time management, marine megafauna tracking data, Areas Beyond National Jurisdiction, global repository, improved data sharing, Global Ocean Observing System
Citation: Sequeira AMM, Hays GC, Sims DW, Eguíluz VM, Rodríguez JP, Heupel MR, Harcourt R, Calich H, Queiroz N, Costa DP, Fernández-Gracia J, Ferreira LC, Goldsworthy SD, Hindell MA, Lea M-A, Meekan MG, Pagano AM, Shaffer SA, Reisser J, Thums M, Weise M and Duarte CM (2019) Overhauling Ocean Spatial Planning to Improve Marine Megafauna Conservation. Front. Mar. Sci. 6:639. doi: 10.3389/fmars.2019.00639
Received: 26 June 2019; Accepted: 30 September 2019;
Published: 01 November 2019.
Edited by:
Jeremy Kiszka, Florida International University, United StatesReviewed by:
Antonios D. Mazaris, Aristotle University of Thessaloniki, GreeceDaniel M. Palacios, Oregon State University, United States
Copyright © 2019 Sequeira, Hays, Sims, Eguíluz, Rodríguez, Heupel, Harcourt, Calich, Queiroz, Costa, Fernández-Gracia, Ferreira, Goldsworthy, Hindell, Lea, Meekan, Pagano, Shaffer, Reisser, Thums, Weise and Duarte. This is an open-access article distributed under the terms of the Creative Commons Attribution License (CC BY). The use, distribution or reproduction in other forums is permitted, provided the original author(s) and the copyright owner(s) are credited and that the original publication in this journal is cited, in accordance with accepted academic practice. No use, distribution or reproduction is permitted which does not comply with these terms.
*Correspondence: Ana Micaela Martins Sequeira, YW5hLnNlcXVlaXJhQHV3YS5lZHUuYXU=