- 1Ecosystem Sciences Division, Pacific Islands Fisheries Science Center, National Oceanic and Atmospheric Administration, Honolulu, HI, United States
- 2Joint Institute for Marine and Atmospheric Research, University of Hawai‘i at Mānoa, Honolulu, HI, United States
- 3Hawaii Division of Aquatic Resources, Honolulu, HI, United States
- 4The Nature Conservancy, Honolulu, HI, United States
Due to climate change, coral reefs have experienced mass bleaching, and mortality events in recent years. Although coral reefs are unlikely to persist in their current form unless climate change can be addressed, local management can have a role to play by extending the time frame over which there are functional reef systems capable of recovery. Here we consider the potential application of one form of local management – management of herbivorous fishes. The premise behind this approach is that increased herbivory could shift reef algal assemblages to states that are benign or beneficial for corals, thereby increasing corals’ ability to recover from destructive events such as bleaching and to thrive in periods between events. With a focus on Indo-Pacific coral reefs, we review what is known about the underlying processes of herbivory and coral-algal competition that ultimately affect the ability of corals to grow, persist, and replenish themselves. We then critically assess evidence of effectiveness or otherwise of herbivore management within marine protected areas (MPAs) to better understand why many MPAs have not improved outcomes for corals, and more importantly to identify the circumstances in which that form of management would be most likely to be effective. Herbivore management is not a panacea, but has the potential to enhance coral reef persistence in the right circumstances. Those include that: (i) absent management, there is an “algal problem” – i.e., insufficient herbivory to maintain algae in states that are benign or beneficial for corals; and (ii) management actions are able to increase net herbivory. As increased corallivory is a potentially widespread negative consequence of management, we consider some of the circumstances in which that is most likely to be a problem as well as potential solutions. Because the negative effects of certain algae are greatest for coral settlement and early survivorship, it may be that maintaining sufficient herbivory is particularly important in promoting recovery from destructive events such as mass bleaching. Thus, herbivore management can have a role to play as part of a wider strategy to manage and reduce the threats that currently imperil coral reefs.
Introduction
Coral reefs are important ecosystems because of the services they provide, including food-security, culture, tourism, recreation, and coastal protection (Woodhead et al., 2019). However, the corals that build reefs are sensitive to even relatively small increases in maximum temperature, and as a result, climate change has led to extensive and widespread coral bleaching and mortality in recent decades (Hoegh-Guldberg, 1999; Griffin et al., 2017; Hughes et al., 2017; Lough et al., 2018; Skirving et al., 2019; Sully et al., 2019). The scale of coral mortality associated with those events, along with an expectation of increasing frequency and severity of future events (van Hooidonk et al., 2016; Hughes et al., 2018), highlights an urgent need for strengthened informed and proactive management of these threatened ecosystems (Anthony et al., 2015; Norström et al., 2016; van Oppen et al., 2017; Hoegh-Guldberg et al., 2018; Mcleod et al., 2019).
Any solution for coral reefs must involve addressing global climate change, but local management could still have a role to play in improving corals’ ability to cope with destructive events such as mass bleaching – either by increasing scope for recovery from events, or by reducing other stressors so that corals are better able to thrive in periods between events. Although the potential for management to be effective in this regard is often framed in terms of resilience, which implies a return to pre-disturbance states, a more realistic management target might be “coral reef persistence,” i.e., extending the timeframe over which there are reefs capable of providing ecosystem services, and which are sufficiently intact to recover. Essentially, increased persistence would buy time for the larger problems to be dealt with, for coral acclimation or adaption to occur (Couch et al., 2017; Coles et al., 2018; DeCarlo et al., 2019), or for other solutions to be realized (Kennedy et al., 2013; Anthony et al., 2017).
While there are many management interventions that might promote coral reef persistence (Ladd et al., 2018; National Academies of Sciences, 2018; Mcleod et al., 2019), here we focus on one of those: management of herbivorous fishes. Although it has been widely believed that herbivore management is one of the keys to coral reef resilience (McClanahan et al., 2012; Chung et al., 2019), there has been recent push back against an overly simplistic expectation that protecting herbivorous fishes within marine protected areas (MPAs) will always or even generally benefit corals (Russ et al., 2015; Bruno et al., 2019). While it is certainly true that many coral reef MPAs have not improved outcomes for corals, some have been highly effective in this regard (Rasher et al., 2013; Bonito et al., 2014; Bonaldo et al., 2017). There is, therefore, a pressing need to better understand the circumstances in which MPAs and other forms of herbivore management are most likely to benefit corals, and equally when this approach is likely to be ineffective.
Given fundamental differences between Indo-Pacific and Caribbean reefs, especially in relation to the impacts of herbivory on algae and of algae on corals (Roff and Mumby, 2012; Doropoulos et al., 2016b; Mumby et al., 2016; Pawlik et al., 2016), and because there has been a recent review of herbivory and resilience on Caribbean reefs (Adam et al., 2015), we focus here on Indo-Pacific coral reefs. Our goals are to: (1) summarize what is known about the scope for herbivory to enhance coral vitality; (2) critically assess the evidence on the impacts of herbivore management on coral reef persistence; (3) consider which factors have contributed to the apparent failure of many MPAs to benefit corals; (4) highlight the main areas of uncertainty; and (5) provide guidance on the conditions in which herbivore management is most likely to be effective at increasing coral reef persistence.
How Might Herbivore Management Increase Coral Reef Persistence?
For herbivore management to increase coral reef persistence, a series of linked steps must occur: (1) management actions lead to increased grazing pressure, presumably by increasing the number, size or diversity of herbivores; (2) increased grazing pressure alters benthic algal assemblages, and corals are better able to thrive when benthic algae are in “cropped” states; and (3) the benefits to corals, such as increased growth or replenishment, or reduced mortality, outweigh any associated negative impacts, such as from increased corallivory (Bonaldo and Rotjan, 2018).
Regarding the first of those conditions, there is ample evidence that management can enhance coral reef herbivorous fish populations (Friedlander et al., 2007; Stockwell et al., 2009; Williams et al., 2009, 2016; Mumby et al., 2013a; Parravicini et al., 2014; Nash et al., 2016a; Bonaldo et al., 2017). Clearly, there are also circumstances in which that will not occur. For example, protection will not enhance herbivore populations much or at all where herbivores are lightly fished or unfished or where compliance is insufficient. Thus, an obvious prerequisite for implementing herbivore management at any particular location is that there can be a reasonable expectation that recovery of herbivore populations is feasible. We believe that local management should generally be able to identify the circumstances in which that is the case, and we do not consider that further here. Instead, we focus on the potential for herbivore management to enhance coral reef persistence, given an assumption that the first condition can be met.
We first consider what is known about the impacts of different types of benthic algae on corals at different life-stages, and the scope for higher grazing pressure to shift algal assemblages toward states that are more benign for corals. This part of our review therefore focuses on colony-scale processes such as coral growth, mortality, and reproduction and how those may be affected by herbivory. As we are ultimately concerned with coral reef persistence, we then shift focus to studies that have assessed reef-scale outcomes of herbivore management, all of which were conducted in MPAs and comparison sites.
Effects of Herbivory on Algal Assemblages
A large body of experimental and observational evidence indicates that herbivory has large and relatively predictable effects on coral reef algal assemblages (Hixon, 2015). Some of that comes from caging experiments which have consistently shown that, at locations where herbivorous fish populations are relatively intact, severely restricting grazing normally leads to substantial increases in algal biomass and canopy height, generally accompanied by shifts in algal communities toward domination by fleshy macroalgae, and dense turfs. Whereas, in control plots, where grazing is not restricted, algal assemblages are more generally dominated by crustose corralling algae (CCA), sparse turfs, and “bare” substrate (Hughes et al., 2007; Smith et al., 2010; Adam et al., 2011; Rasher et al., 2012; Humphries et al., 2014; Doropoulos et al., 2016b; Holbrook et al., 2016; Mumby et al., 2016).
One limitation of caging experiments is that they typically reduce grazing pressure by much more than would normally occur on even heavily fished reefs, e.g., by a factor of 10 or more (Hughes et al., 2007; Holbrook et al., 2016). However, multiple survey-based studies have shown broadly similar patterns across natural gradients of herbivory – i.e., negative associations between herbivore biomass and macroalgal cover on reefs in Australia, Hawaii, the Philippines, and American Samoa (Friedlander et al., 2007; Stockwell et al., 2009; Wismer et al., 2009; De’ath and Fabricius, 2010; Heenan and Williams, 2013). Similar reef-scale relationships between algal assemblages and intensity of herbivory were also evident in a study of herbivore management areas in Maui, Hawaii. Over the first 6 years of closure, during which time herbivore biomass increased, and parrotfish biomass more than doubled, CCA cover increased from ∼2 to ∼15%, macroalgal cover declined to near zero, and algal turfs shifted toward more heavily cropped states – i.e., sparse turfs and bare substrate. No similar changes occurred at eight comparison sites around Maui (Williams et al., 2016).
Of course, algal communities are shaped by many drivers other than herbivory, including sedimentation, turbidity, ocean chemistry, wave energy, nutrient levels, depth, temperature, substrate orientation, and preemption of space by other benthic organisms (Wismer et al., 2009; De’ath and Fabricius, 2010; Mumby et al., 2013a; Williams et al., 2015; Duran et al., 2018; Robinson et al., 2018). Reefs also vary in their capacity to support abundant herbivorous fishes (Heenan et al., 2016; Helyer and Samhouri, 2017), and there are also large differences among reefs in the extent to which herbivores have been depleted by fishing (Edwards et al., 2013). In addition, rates of herbivory and of algal growth and senescence vary seasonally (Done et al., 2007; Lefèvre and Bellwood, 2010; Brown et al., 2018). Consequently, the amount of grazing necessary to maintain algae in cropped states is likely to vary considerably among locations and time periods, as will the scope for management to raise herbivory sufficiently to cause meaningful shifts in benthic algal assemblages. Nevertheless, collectively there is strong evidence, that, where fleshy macroalgae and dense turf are abundant, increased grazing pressure could reasonably be expected to shift algae toward “cropped” states, i.e., CCA and sparse turfs.
Accepting that algal communities are affected in somewhat predictable ways by herbivory, it is less clear how much, and what type of herbivory is necessary for benthic algae to be maintained in desirable states. Certainly, parrotfishes are not the only important grazers, and instead it seems that maintaining herbivore functional and size diversity are important (Burkepile and Hay, 2008; Nash et al., 2016b). Additionally, feeding selectivity and limited diversity within some feeding groups can mean that a small number of species are particularly important at any particular location (Humphries et al., 2015; Plass-Johnson et al., 2015; Puk et al., 2016). For example, a study in Fiji found that 97% of macroalgal consumption was by four species, with little overlap among those in the algal taxa they consumed (Rasher et al., 2013).
Effects of Benthic Algae on Corals
Effects of Algae on Coral Condition, Growth, and Survival
In situ visual assessments of coral-algal interactions around coral colony boundaries generally show neutral or negative outcomes for coral tissue adjacent to macroalgae and turfs, i.e., tissue loss, damage, bleaching or overgrowth, but neutral or positive outcomes for tissue adjacent to CCA (Table 1). Interactions identified as corals “losing” likely have some cost to affected colonies, as they are associated with detrimental shifts in microbial communities and coral tissue hypoxia at the coral-algal interface (Barott et al., 2009). Corroboration that interactions with thick turfs and some macroalgae can translate into negative impacts on coral vitality comes from studies demonstrating reduced coral growth rate or lesion recovery, or higher tissue mortality (Table 1). In contrast, interactions between corals and either CCA or sparse or inconspicuous turfs tend to result in positive coral tissue growth at the interaction site (Haas et al., 2010; Jorissen et al., 2016).
The impacts of competition with turf and macroalgae vary considerably depending on the coral and algal species involved, as well as coral colony size and growth form (Barott et al., 2012; Swierts and Vermeij, 2016). Importantly, not all macroalgae have negative effects on corals; for example, the calcareous upright macroalgae Halimeda spp. has been shown to be a largely benign or neutral competitor (Barott et al., 2009; Brown et al., 2017). Allelopathy, i.e., chemical inhibition, appears to be a critically important mechanism. For example, transplantation of allelopathic algae (Chlorodesmis fastigiata and Galaxaura filamentosa) against coral colony edges led to tissue damage (bleaching, mortality, or overgrowth) for the majority of coral species tested, whereas transplanted non-allelopathic macroalgae (Sargassum polycystum and Turbinaria conoides) did not damage corals (Bonaldo and Hay, 2014). Close proximity with allelopathic macroalgae has also been associated with reduced rates of lesion repair for some but not all coral species (Bender et al., 2012).
Sub-lethal effects of excessive algal growth on corals potentially also include diminished reproductive capacity. For example, in long-term caging studies on the Great Barrier Reef, reproductive output was significantly reduced in coral fragments within macroalgal dominated cages (Hughes et al., 2007). Similarly, Isopora palifera colonies in plots dominated by macroalgae released around half as many larvae as colonies in plots where macroalgae was maintained at low levels over a 2 year period (Tanner, 1995).
Some studies have noted that small coral colonies (<5 cm diameter) are more likely to “win” interactions (judged by condition of coral tissue at the interaction site), perhaps because they devote a greater share of their resources to colony defense than larger colonies (Barott et al., 2012; Brown et al., 2017). However, probably more important to long-term outcomes of coral-algal interactions are that large colonies tend to: (1) have greater capacity to escape by height, i.e., portions of the colony grow up and away from the benthos and so a smaller portion of the colony perimeter directly interacts with algae (Barott et al., 2012; Bonaldo and Hay, 2014); and (2) have higher surface-area to perimeter ratios (George et al., 2018), and thus greater ability to absorb the energetic costs of competition around their boundaries. This may explain why growth rates of large colonies tend to be much less affected by competition with macroalgae than are small colonies (Ferrari et al., 2012), and why it is possible for reefs to have large and well-established corals alongside high macroalgae cover.
Effects of Algae on Coral Settlement and Early Survivorship
The clearest impacts of algae on corals are on corals’ early life stages. With few exceptions, experimental studies have shown that macroalgae generally inhibits coral settlement or reduces early survivorship (Table 2). Underlying mechanisms include physical overgrowth and abrasion, but there is also considerable evidence of the importance of allelopathic effects on corals in proximity to certain macroalgae (Vermeij et al., 2009; Dixson et al., 2014; Evensen et al., 2019). In some circumstances this can lead to reef-scale coral recruitment failure at locations where macroalgae have become very abundant (Doropoulos et al., 2014). Conversely, corals preferentially settle on some types of CCA, as shown by in situ surveys (O’Leary et al., 2012) and by experimental work, including that attachment of CCA chips to tile substrate increased coral settlement eightfold (Doropoulos et al., 2016a). Impacts of turf are more complex, but low turfs, i.e., with canopy height <∼ 3 mm, do not appear to negatively impact coral settlement or early survivorship, except when in combination with sediment (Birrell et al., 2005; Diaz-Pulido et al., 2010; Leong et al., 2018).
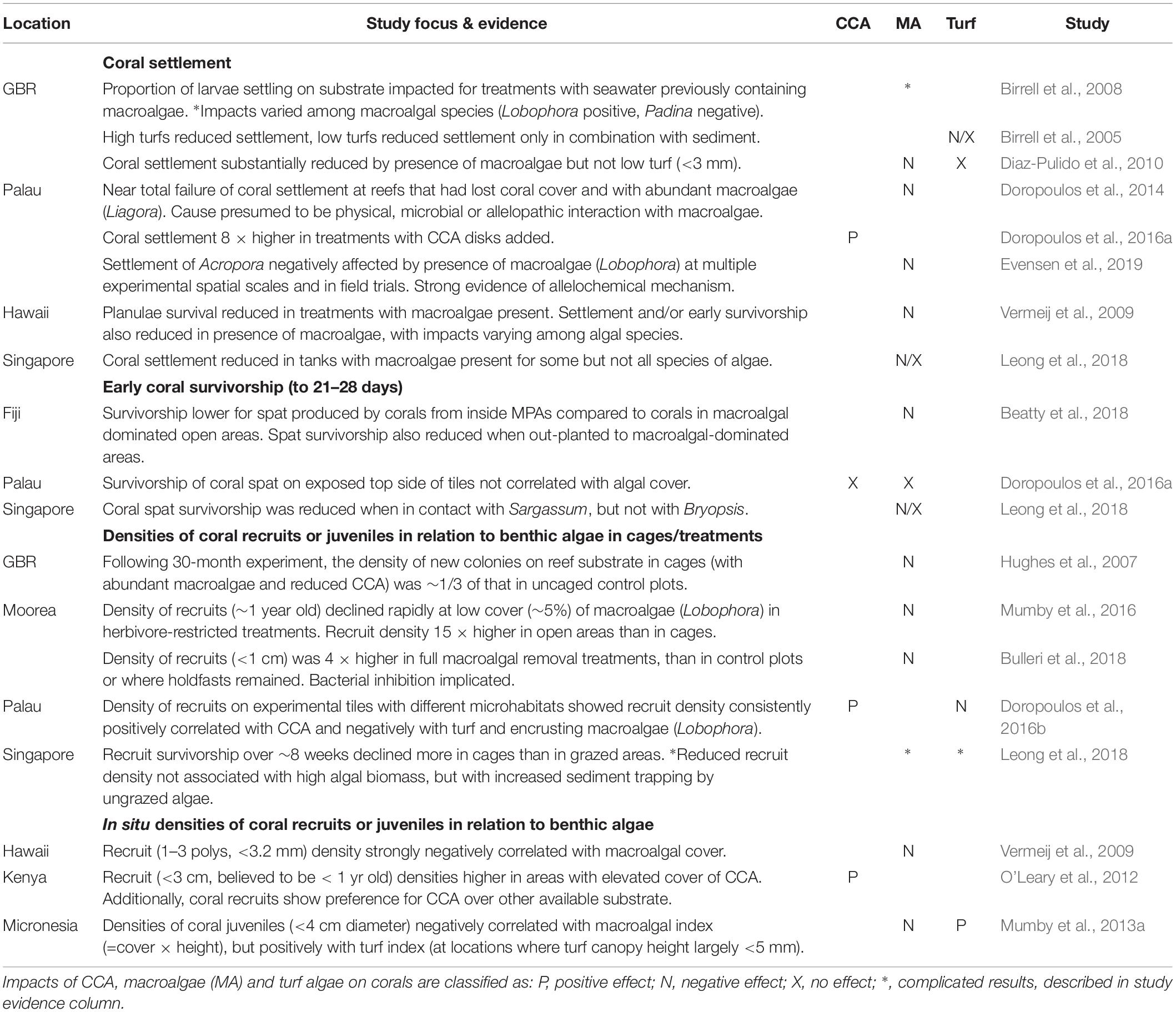
Table 2. Effects of benthic algae on coral replenishment – i.e., settlement, survivorship or density of early life stage corals.
Early stage corals have extremely high mortality rates, e.g., ∼1% of settling corals survive to ∼ 1 cm diameter (Doropoulos et al., 2015). At that highly vulnerable life stage, there are complex trade-offs for coral recruits between the negative effects of competition with uncropped algae in the form of shading, overgrowth, and smothering, vs. the risk of deliberate or incidental predation. Typically, corals preferentially settle into cryptic or sheltered microhabitats such as undersides of ledges and crevices, thereby avoiding the exposed upper surfaces where they would be more vulnerable to predation (Doropoulos et al., 2015, 2016a). These protected microhabitats can still be accessible to small herbivores that may partially control algal growth, but which are less likely to consume corals (Brandl et al., 2014).
Regardless of the relative importance of mechanisms that underlie survival of early-stage corals (Doropoulos et al., 2016b), experimental studies have consistently shown higher survivorship of early-stage corals in treatments that are accessible to herbivores and other fishes, in spite of higher predation rates (Hughes et al., 2007; Doropoulos et al., 2016a; Mumby et al., 2016). Corroboration of those experimental results in nature comes from a study of marine reserves in Fiji, where density of juvenile corals (<5 mm diameter) was around three times higher in two of three reserves (and non-significantly higher in the other), with those differences ascribed to lower macroalgal cover inside reserves (Bonaldo et al., 2017).
Although several studies have shown strong positive impacts of CCA on coral settlement and early survivorship (Table 1), there are important differences among CCA species, including that corals strongly avoid settling on some CCA species, and that spat survivorship tends to be low on non-preferred species, due to overgrowth or sloughing by CCA (Harrington et al., 2004; Price, 2010; O’Leary et al., 2012). However, there is some evidence that CCA species most favorable for coral settlement and survival tend also to be more abundant in the cryptic microhabitats favored by coral settlers (Steneck, 1986). Thus, although excessive algal growth can negatively impact corals in all life stages, perhaps what is most important for corals’ early life stages is that there is sufficient cover of benign CCAs and other preferred substrates in settlement microhabitats, and that broader reef algal communities are not dominated by allelopathic algae which can inhibit settlement or early survivorship at a distance (Evensen et al., 2019), potentially leading to reef-scale recruitment failure (Doropoulos et al., 2014).
Outcomes of Herbivore Management for Corals
Given the evidence above that, without sufficient herbivory reef benthic algae tend to shift toward states that are less desirable for coral wellbeing, one might expect there would be many cases in which boosting herbivory had led to increased coral cover or improved capacity of corals to recover from storms and other destructive events. In fact, evidence for the generality of those kinds of outcomes is weak (Bruno et al., 2019). Therefore, it is important to critically assess the relevant evidence to better understand why management has been ineffective in many cases, as well as to draw conclusions about where herbivore management has the greatest potential to improve outcomes for corals.
The only evidence we are aware of involving reef-scale outcomes of management comes from studies of MPAs. For example, several previous studies have synthesized evidence of MPA effects on coral cover and recovery, either as meta-analyses or in large-scale studies utilizing data from multiple MPAs and comparison areas at widely spread locations (Table 3). Those studies provide no indication that coral mortality from warming events was lower within MPAs (Ateweberhan et al., 2011; Selig et al., 2012). Also, although one study suggested that the rate of coral cover recovery following the 1998 Indian Ocean bleaching event was higher within protected areas than fished areas (Ateweberhan et al., 2011), other studies assessing recovery from major disturbances (storms, bleaching, and crown-of-thorns outbreaks) did not find evidence that coral recovery was enhanced within MPAs (Graham et al., 2011; Carassou et al., 2013).
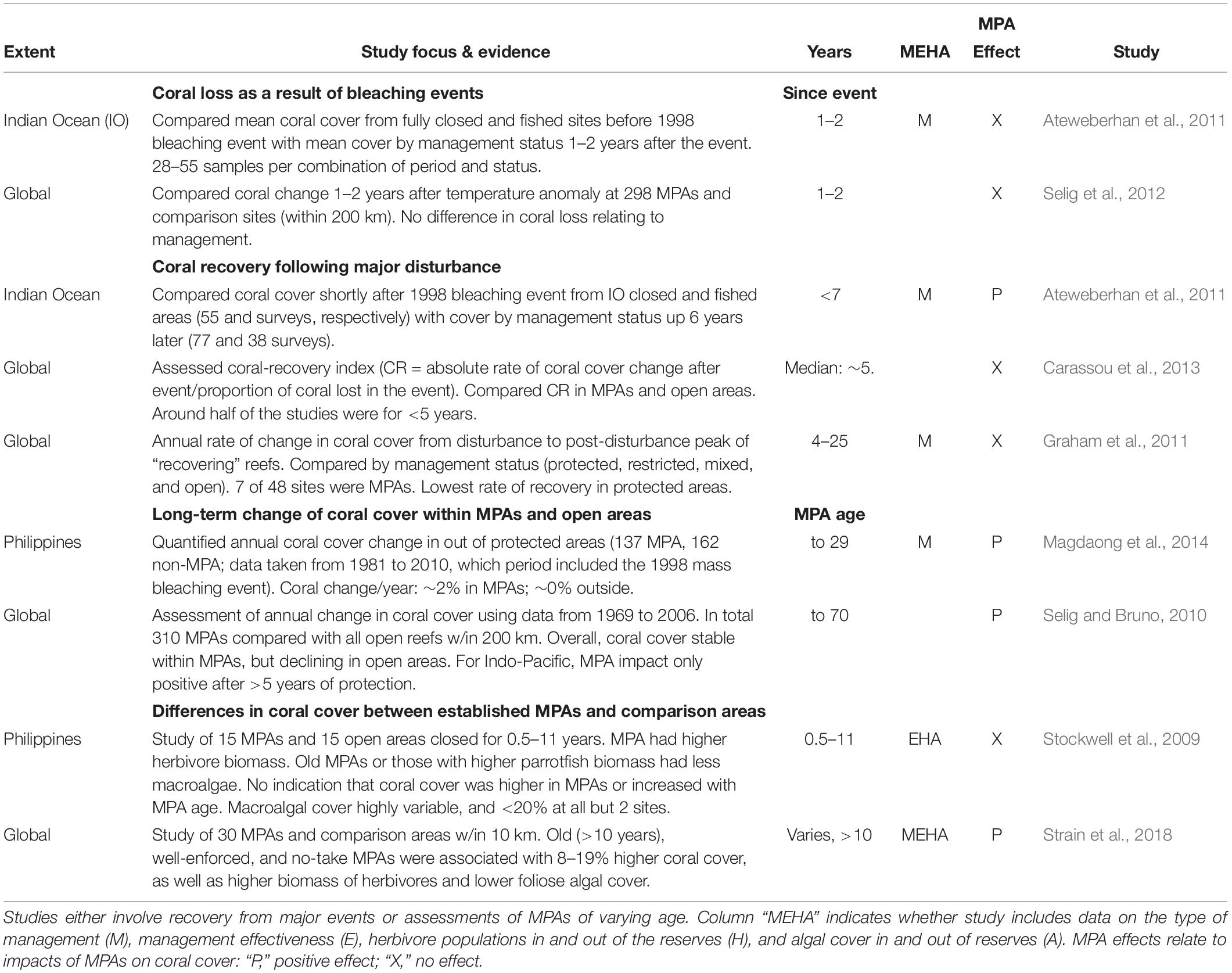
Table 3. Studies synthesizing MPA effects on coral cover or recovery (meta-analyses and large-scale data syntheses).
However, it is not always straightforward to draw conclusions on herbivore management from MPA studies. First, MPAs may have other protections that would benefit corals, such as no-anchoring rules; alternatively, they might be impacted by heavier recreational or tourist use. Also, MPAs are often established in atypical locations, undermining simple comparisons with unprotected reefs in their locality. Additionally, by no means do all coral reef MPAs enhance herbivore stocks. MPAs are likely to have only small effects in locations where herbivores are lightly fished or if compliance is weak, as was the case in some MPA studies that have addressed this topic (Carassou et al., 2013; Halpern et al., 2013). More importantly, the great majority of coral reef MPAs were not established to manage herbivores with a goal of sustaining or recovering corals, and in many cases it is unlikely they could ever be effective in that regard. For example, herbivorous fishes are not generally targeted by coral reef fisheries in either Florida or on the Great Barrier Reef in Australia (Kramer and Heck, 2007; Cheal et al., 2010), and thus there is no reason to expect that MPAs in those regions would boost herbivory. Notably, the majority of locations considered in the global studies showing no MPA effect on coral recovery were in the Caribbean or Australia – 14 of 17, and 5 of 7 MPAs, respectively (Graham et al., 2011; Carassou et al., 2013).
Other syntheses have shown modest positive benefits on the rate of change of coral cover from being within established (>∼5 years old) Indo-Pacific MPAs (Selig and Bruno, 2010; Magdaong et al., 2014). However, it is unclear how important herbivore management may have been in those patterns, as those studies do not contain information on relative abundance of herbivores or on other aspects of management effectiveness (Table 3). We found only two syntheses which included assessments of the impacts of protection on herbivorous fishes, as well as on algal and coral cover (Table 3). One of those compared 15 MPAs in the Philippines that had been closed for between 0.5 and 11 years with 15 comparison fished areas, and found no effect of protection on coral cover, in spite of higher herbivorous fish biomass and lower macroalgal cover in long-standing MPAs (Stockwell et al., 2009). The scope to draw broad conclusions on management from that study is undermined by high variability among these reefs – e.g., coral cover varied between 5.7 and 67.3% among sites – and because of the brief duration of protection –5 years or less at half the MPAs. The other synthesis, a global study, found evidence of a positive impact of protection on coral cover in some MPAs (Strain et al., 2018). Specifically, coral cover was 8–19% higher inside well-enforced no-take MPAs that were >10 years old than in comparison open areas. That difference was due in part to the higher biomass of herbivores and lower cover of foliose algae in those protected areas (Strain et al., 2018).
Although these results provide some indications that MPAs can benefit corals in at least some circumstances, our goal is not to determine whether MPAs are a universally effective tool, but rather to draw conclusions about the potential for herbivore management of Indo-Pacific reefs, assuming that management is able to meet the most basic standard for effectiveness that we highlight above – i.e., enhance stocks of herbivorous fishes. To that end we now give detailed consideration to studies of MPAs or groups of MPAs at Indo-Pacific locations that have been comprehensively studied over a number of years and which meet that standard (Table 4).
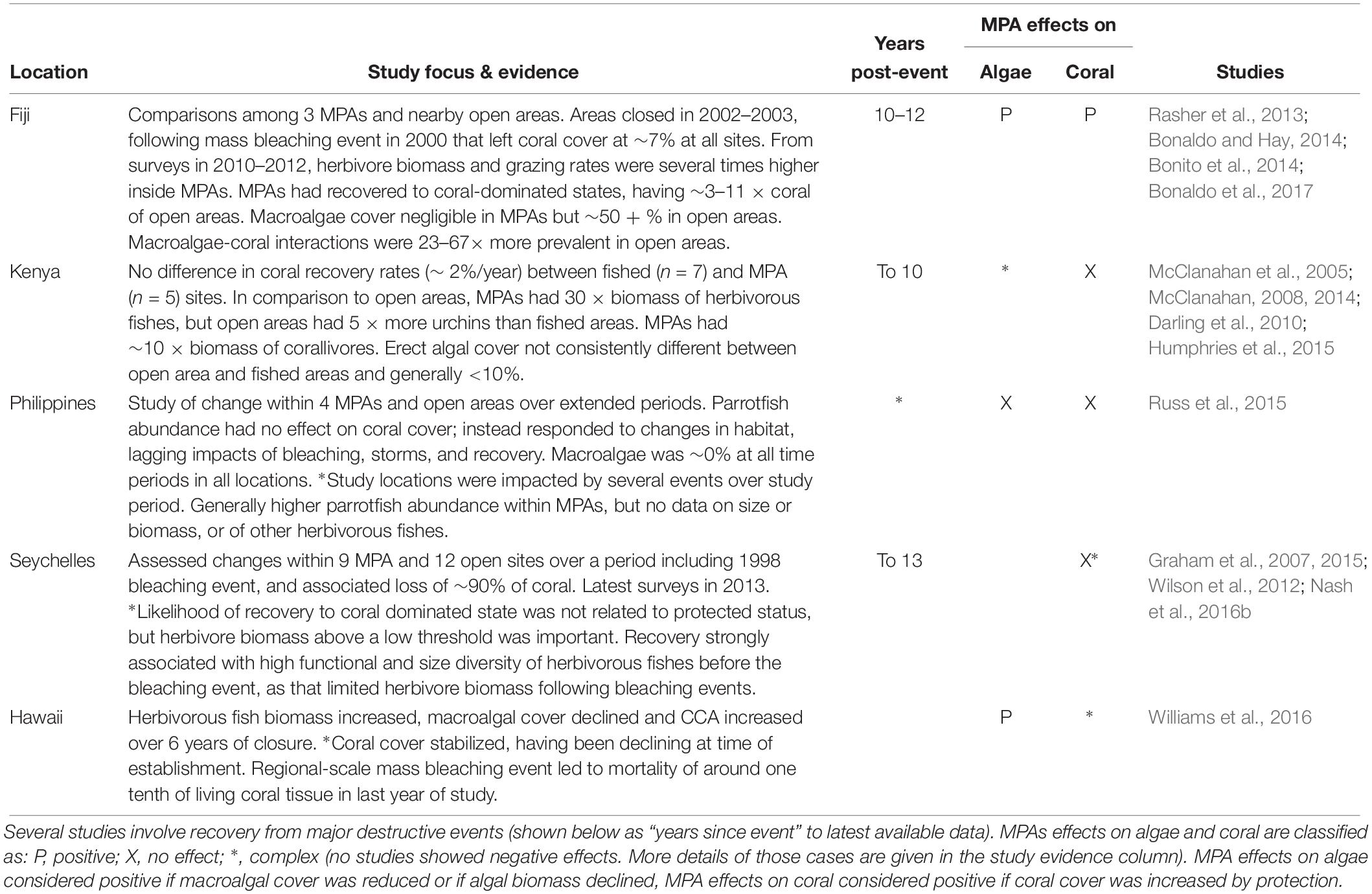
Table 4. Focal MPA studies, including only MPAs where there is evidence that herbivorous fishes were enhanced by management.
Conclusions From Focal MPA Studies
The studies summarized in Table 4 are from five locations in the Indo-Pacific. In all cases, there is comprehensive information on MPA effects on coral, herbivores and benthic algae, including data prior to the establishment of MPAs and for several years after. In four of these locations, reefs had experienced major bleaching or other destructive events immediately prior to or during the study (Table 4). These focused studies therefore allow us to draw a number of broad conclusions about the potential for herbivore management to enhance Indo-Pacific coral reef persistence including recovery from disturbance.
Herbivore Management Can Improve Outcomes for Corals in Some Circumstances
Clear evidence that marine reserves can be highly effective at promoting coral recovery comes from MPAs on the south coast of Viti Levu, Fiji. There, three small MPAs were established in 2002–2003, ∼ 2 years after a mass bleaching event that had left reefs in a degraded state, with coral cover of around 7% and macroalgal cover around 35–45% in both protected and comparison fished areas (Bonito et al., 2014). By 2010–2012, coral cover within MPAs was variously estimated at 16–23, 38–56, or 36–75% depending on the location of sampling, but had barely recovered – to around 10% – in fished areas (Rasher et al., 2013; Bonito et al., 2014; Bonaldo et al., 2017). Macroalgae continued to dominate on fished reefs but had become scarce within MPAs (Bonaldo et al., 2017). Multiple lines of evidence indicate that the reduction of the direct and indirect impacts of macroalgae was key to coral recovery within the MPAs, including demonstrated negative effects of macroalgae on coral recruitment, growth, and mortality (Bonaldo and Hay, 2014; Clements and Hay, 2015; Beatty et al., 2018; Clements et al., 2018). The obvious driver of differences in algal assemblages between these MPAs and the nearby fished areas was that herbivorous fish biomass and grazing rates had become 3–6 times higher, and herbivore diversity 2–3 times higher inside the MPAs than in fished area (Rasher et al., 2013; Bonito et al., 2014; Bonaldo et al., 2017). Thus, within those Fijian MPAs there was a clear link between protection of herbivores and the rapid and substantial recovery of coral assemblages.
A more equivocal case is that of the Kahekili herbivore fisheries management area (KHFMA) in Maui, Hawaii. The KHFMA was established in 2009 in response to concerns about the rapid decline of coral cover in that vicinity, during a period in which there had been several episodic but large-scale macroalgal blooms (Smith et al., 2005; Williams et al., 2016). By 2015, parrotfish biomass had more than doubled and algal assemblages had also changed, with cover of CCA and sparse turfs greatly increased, and macroalgae reduced to negligible levels. Coral cover, which had been declining at the time of KHFMA establishment, stabilized around 3 years after protection began. Unfortunately, any incipient coral recovery was arrested by a region-wide coral bleaching event in 2015, which caused the loss of around a tenth of the coral cover within the KHFMA (Williams et al., 2016). Thus, results from the KHFMA are not inconsistent with an expectation that herbivore management can benefit coral persistence, but definitive evidence for or against that will only become apparent over coming years as the reefs there and elsewhere in Hawaii recover from the 2015 event.
Herbivore Management Will Not Enhance Coral Reef Persistence Where There Is Already Sufficient Herbivory
In stark contrast to outcomes in Fiji and Hawaii, protection from fishing had no positive effects on coral reef recovery in Kenya from a major mortality event in 1998 (McClanahan, 2008; Darling et al., 2010). There, effective enforcement within reserves combined with high fishing pressure outside has led to dramatic differences in fish populations between fished and closed reefs, with closed reefs having around 30 times the biomass of herbivorous fishes (McClanahan, 2008). However, fishes that prey on sea urchins have also been heavily depleted in fished areas, with the consequence that superabundant sea urchins dominate herbivory on fished reefs (McClanahan et al., 2005; McClanahan, 2014). Overall, although CCA cover was reported to be higher inside closed areas, cover of fleshy algae was similar, and relatively low (<10%) in fished and closed areas (McClanahan, 2008, 2014; O’Leary et al., 2012). Thus, protection of fishes changed the nature, but not clearly the overall intensity, of herbivory inside these Kenyan closed areas.
Similarly, at four extremely well-studied MPAs and comparison areas in the Philippines, there were no positive impacts of protection on coral cover, and no evidence of improved recovery within reserves following a number of disturbances during up to 30 years of monitoring (Russ et al., 2015). Conversely, there was clear evidence of “bottom-up” effects, namely that parrotfish abundance increased as availability of preferred feeding substrata increased. However, at all studied areas, macroalgal cover remained at 0% at all times — i.e., in both fished and closed areas, grazing pressure was sufficient to maintain algae in cropped states throughout. Thus, in this case, there was no deficit of herbivory for management to address. While this highlights that simply establishing MPAs does not by itself improve outcomes for corals, it does not undermine the potential utility of herbivore management in locations where there is an “algal problem” to address.
Increased Corallivory Can Be a Detrimental Consequence of Herbivore Management
As well as differences in sea urchin densities, another key difference between Kenyan fished and closed areas was that corals in closed areas experienced much higher levels of tissue loss from coral predators, including the large parrotfishes and triggerfishes that had become abundant within MPAs (McClanahan et al., 2005). As increased number and size of parrotfishes is not only a likely outcome, but generally an explicit goal of herbivore management, there is clearly potential for increased corallivory to be a common undesirable consequence of protection. On Kenyan reefs, the primary corallivorous parrotfish was the steephead parrotfish, Chlorurus strongycephalus (McClanahan et al., 2005), a species that is known to consume large amounts of coral (Lokrantz et al., 2008). However, parrotfishes were by no means the only corallivorous fishes on those reefs; in fact, the main predator of branching corals within Kenyan MPAs was the red-lined triggerfish Balistapus undulatus (McClanahan et al., 2005). As B. undulatus is also the primary predator of sea urchins, more restricted management such as protecting only herbivores, might have led to better outcomes for corals in Kenyan protected areas. More generally, limited protection is likely also to be more palatable to local stakeholders (Cinner et al., 2009).
Inherent Complexity Can Overwhelm or Obscure Management Effects
A more complex case is that of coral reefs in the Seychelles that lost >90% of coral cover during the 1998 warming event (Graham et al., 2015). By 2011, 12 of 21 reefs studied had recovered to something approaching pre-1998 coral-dominated states, whereas coral cover remained low and reefs had become dominated by fleshy macroalgae in the other nine locations. For these Seychelles reefs, being located within an MPA did not increase the likelihood of recovery to coral-dominated states. However, compliance appears to have been weak in the Seychelles MPAs (Daw et al., 2011), which may also explain why herbivore biomass and MPAs effects varied so considerably among individual reserves, habitat types, and time periods (Graham et al., 2007). In addition, even if there were not clear protection effects on coral recovery, the state of herbivorous fish population was important, as maintaining herbivore biomass above a fairly low threshold was a predictor of coral recovery at Seychelles reefs (Graham et al., 2015). More recent studies of those reefs have shown that high functional and size diversity of herbivorous fish communities prior to the disturbance was actually key to the recovery to coral-dominated states, as that diversity enabled herbivorous fish populations to respond to the change in reef states as coral substrate was replaced by algae following the disturbance (Nash et al., 2016b). Therefore, while the Seychelles MPA studies are not supportive of a simple management effect, they do suggest that maintaining sufficient amount and types of herbivory will increase the scope for reef recovery following disturbance.
Summary
Coral vitality is negatively affected by interactions with certain types of algae, including some types of macroalgae as well as thick turf mats; whereas corals are generally better able to thrive when algal assemblages comprise sparse turfs, bare substrate, and CCA. Importantly, “problem algae” are not simply macroalgae, as: (i) there is considerable variability in the impacts of different species and growth forms of macroalgae; and (ii) there can be strong negative impacts of interactions with some types of CCA and turf. In particular, thick turfs, especially where those trap sediment, can have as negative impacts on corals as “problem” macroalgae.
The impacts of benthic algae on corals vary greatly depending on coral size, growth-form, and life stage. The clearest negative effects of some macroalgae and thick turfs are on corals’ ability to settle and survive into adult life stages. Although large corals can be vulnerable to extreme algal overgrowth and partial mortality, growth and total-mortality rates of large adult colonies are generally less impacted by algal interactions for two main reasons: (i) they can have greater capacity to escape by height, resulting in less of the colony being in close proximity to benthic algae; and (ii) their relatively high surface-area-to-perimeter ratios means that the costs of interactions at their boundaries are relatively smaller for the colony as a whole.
With considerable natural variability and several confounding factors, higher levels of herbivory will tend to shift algal communities toward states that are more benign for corals.
Increased corallivory, especially by large parrotfishes, has the potential to undermine benefits of herbivore management, but the importance of that factor will vary considerably depending on the species and size ranges present, as well as the particular form of management employed. More generally, herbivore management should not be focused only on restoring parrotfishes. Instead, maintaining diversity is probably key due to both feeding selectivity and complementarity. A high degree of herbivore functional and size diversity appears also to be a key factor facilitating herbivory community responses to major events such as mass coral mortality.
Herbivore management is not a panacea, but has the potential to enhance coral reef persistence in the right circumstances; essentially when there is a deficit of herbivory that management can solve. Specifically, that means: (i) that there is an “algal problem,” i.e., abundant macroalgae or dense turfs, or reason to believe that one might develop – perhaps because that had occurred on reefs elsewhere in the locality under broadly similar circumstances, or based on experimental evidence, such as manipulation of grazing pressure to determine whether local reefs are close to thresholds that could make them vulnerable to persisting phase shifts (Schmitt et al., 2019); and (ii) that herbivore stocks or functional diversity are depleted and management actions can reasonably be expected to increase those; which implies, among other things, that there can be sufficient compliance. Those condition have not been met at many existing MPAs, which should not be surprising as most MPAs were not established to enhance herbivory or to increase coral resilience. It is also important that any benefits to corals from increased herbivory are not overwhelmed by other factors, such as heavy sedimentation, that make areas poorly suited to coral growth (Rodgers et al., 2009; Stender et al., 2014). Understanding when those conditions apply requires local knowledge and good judgment. With the aim of grounding our conclusions, we consider application of those to the potential for applying herbivore management on reefs in Hawaii (Supplementary Appendix A).
Gaps and Research Directions
There is a pressing need to improve our understanding of the effects of parrotfish corallivory. There appears to be considerable variability among parrotfish species, size classes and regions (Bonaldo and Rotjan, 2018), as well as in the degree to which different coral species are able to sustain that form of predation. Ultimately, whether the effects of increased grazing along with increased corallivory is a net benefit or hindrance to corals is likely to vary greatly among locations, depending in part on the mix of parrotfish species and size classes present. Highly corallivorous species – those taking 20% or more of their bites on coral tissue – tend to be large-bodied excavators such as Bolbometopon muricatum, Cetoscarus bicolor, Chlorurus gibbus and C. strongycephalus (Bonaldo and Rotjan, 2018), but those are not abundant in all locations. The relative importance of corallivory is also likely to vary among reefs. For example, although “natural” reefs can have both high coral cover and abundant corallivorous parrotfishes, e.g., Wake Atoll (Muñoz et al., 2012), it may still be the case that certain parrotfish species or size-classes tend to have net negative impacts on corals, and perhaps especially so on degraded reefs where coral cover is lower, and thus corallivory is more concentrated (Rice et al., 2019). Intriguingly, the Fiji MPAs, where herbivore management was highly effective, had few large-bodied parrotfishes, likely in part due to the small size of those protected areas (Bonito et al., 2014; Bonaldo et al., 2017). It is therefore conceivable that management should not aim to recover or sustain the larger size classes of some species of parrotfishes. Clearly any such policy would have to balance any potential benefits against the important and distinct role of large parrotfishes as excavators and herbivores (Bellwood and Choat, 1990; Lokrantz et al., 2008; Ong and Holland, 2010).
While the impacts of management on herbivorous fishes have been relatively well studied, with a few exceptions, there has been much less focus on the direct and indirect effects of management on other coral reef herbivores, such as sea-urchins. This is in spite of the fact that sea-urchins can become the dominant herbivores on some reefs (O’Leary and McClanahan, 2010; Humphries et al., 2014), and are such effective grazers they have even been used as macroalgal biocontrol agents (Neilson et al., 2018). As we describe above, release from predation pressure and competition with other herbivores led to the explosion of sea-urchin abundance on fished reefs in Kenya (McClanahan, 2000). However, there appears to be considerable variability among locations in the importance of the various factors including predator density that drive sea-urchin abundance on coral reefs (Tebbett and Bellwood, 2018). Thus, for most reefs it remains difficult to predict the extent to which grazing by sea-urchins and other non-fish herbivores (Goatley et al., 2012; Altman-Kurosaki et al., 2018) may be able to compensate for loss of fish herbivory should herbivorous fishes become depleted, as well as how best and in what circumstances management might promote such herbivory.
Cleary there is complexity in the relative importance of factors that affect coral vitality, including not only interactions with algae, but also other factors, such as sedimentation, temperature, light intensity, wave exposure, biogeography, and nutrient levels (Williams et al., 2011, 2018; Gove et al., 2015; Goatley et al., 2016; Fox et al., 2018; Jouffray et al., 2019). It is also likely that grazing behavior and outcomes vary considerably depending on local conditions (Goatley et al., 2016) and also that critical ecological functions – in terms of herbivory – will change as reefs themselves change (Bellwood et al., 2018). Understanding the relative importance of the multiple factors affecting coral condition and recovery would greatly benefit from focused longitudinal studies of coral vital rates (e.g., reproduction, growth, and mortality) across a range of environments. The rapid development of computer-intensive technologies, such as high resolution three dimensional photo-mosaics (Burns et al., 2016; Edwards et al., 2017), has transformed researchers’ ability to precisely track outcomes at colony level, and to relate those to potential drivers. A focus on vital rates, allied with the improving capacity to generate large amounts of data, will help to clarify the amount and type of herbivory that is necessary to enhance coral persistence, and how that varies depending on a range of other environmental and habitat factors. That in turn would help to establish locally relevant management targets.
A fundamental question for managers considering herbivore management for coral reef persistence is at what stage that should be implemented. Self-evidently, there will be some lag before herbivore stocks recover sufficiently to begin to improve conditions for corals. The timespan involved will vary among species and depending on the degree of depletion but is likely to be at least several years, and could be much longer, given that some larger-bodied and longer-lived taxa can take a decade or more to approach new equilibria following protection (McClanahan et al., 2007; McClanahan, 2014). There is also growing evidence that undesirable feedbacks can mean that once reef benthic communities have shifted to alternate states, they have a tendency to remain there (Mumby et al., 2013b; van de Leemput et al., 2016). For example, once a reef algal community becomes dominated by large macroalgal plants, it can require greater amounts of herbivory to recover to a turf-dominated state than, absent the disruption, would have been necessary to maintain it in its former state (Schmitt et al., 2019). The relative unpalatability of some macroalgae can also mean that phase-shifted reefs have greater dependence on a relatively small number of herbivores, those that can consume those plants (Rasher et al., 2013; Puk et al., 2016). Collectively, therefore, it seems that proactive management of herbivores, i.e., in advance of anticipated coral loss, may be a more generally appropriate strategy than introducing herbivore management following destructive events. However, the scope for management to make wise judgments on where and when to implement herbivore management would be greatly improved by focused collaboration between managers and researchers, utilizing a mix of experimental, observational, and modeling approaches (Mumby et al., 2016; van de Leemput et al., 2016; Schmitt et al., 2019).
Finally, one reason it can be difficult to draw conclusions from previous studies is that widely used algal functional classifications such as “macroalgae” and “turf” are not only inherently broad categories, but also that those terms have not been consistently applied. “Turf” is perhaps an even broader and less clearly defined term than “macroalgae,” potentially encompassing such diverse assemblages as sparse mixed-species turfs; apparently bare substrate; clumps of filamentous algae; as well as dense turf mats comprising multiple algal species potentially including diminutive forms of macroalgal species (Connell et al., 2014). Thus, although these terms are useful, much complexity and nuance is lost when results are presented only in those terms, and it would be extremely valuable if more detail were provide, including for example canopy height, morphology, taxonomic composition.
Conclusion
We stress again that while herbivore management has the potential to benefit some coral reefs, herbivore management is at best buying time. Global warming is manifestly the foremost current threat to coral reefs, and must be addressed by the global community if reefs as we know them will have any chance to persist. However, the seriousness of the threat to coral reefs seems to necessitate action on multiple fronts. Herbivore management has a role as part of a larger strategy to build networks of resilient reefs that would provide the greatest time for the larger problems to be dealt with, and which would increase scope for eventual recovery (Beyer et al., 2018).
Author Contributions
IW, TK, and CC developed the initial outline. IW wrote the first draft with considerable help from all other authors. All authors made substantial contributions to the final version.
Funding
This work was supported by the NOAA’s Coral Reef Conservation Program (http://coralreef.noaa.gov) for project #31258, and by core funds of the NOAA Pacific Islands Fisheries Science Center.
Conflict of Interest Statement
The authors declare that the research was conducted in the absence of any commercial or financial relationships that could be construed as a potential conflict of interest.
Acknowledgments
We thank the staff of NOAA Pacific Island Fisheries Science Center in Honolulu, who assisted with internal review and editing of the manuscript prior to submission. We also thank Mariska Weijerman and Rusty Brainard for extremely useful comments and discussion on earlier versions of the manuscript.
Supplementary Material
The Supplementary Material for this article can be found online at: https://www.frontiersin.org/articles/10.3389/fmars.2019.00557/full#supplementary-material
References
Adam, T., Burkepile, D., Ruttenberg, B., and Paddack, M. (2015). Herbivory and the resilience of Caribbean coral reefs: knowledge gaps and implications for management. Mar. Ecol. Prog. Ser. 520, 1–20. doi: 10.3354/meps11170
Adam, T. C., Schmitt, R. J., Holbrook, S. J., Brooks, A. J., Edmunds, P. J., Carpenter, R. C., et al. (2011). Herbivory, connectivity, and ecosystem resilience: response of a coral reef to a large-scale perturbation. PLoS One 6:e23717. doi: 10.1371/journal.pone.0023717
Altman-Kurosaki, N. T., Priest, M. A., Golbuu, Y., Mumby, P. J., and Marshell, A. (2018). Microherbivores are significant grazers on Palau’s forereefs. Mar. Biol. 165:74. doi: 10.1007/s00227-018-3327-x
Anthony, K., Bay, L. K., Costanza, R., Firn, J., Gunn, J., Harrison, P., et al. (2017). New interventions are needed to save coral reefs. Nat. Ecol. Evol. 1, 1420–1422. doi: 10.1038/s41559-017-0313-5
Anthony, K. R. N., Marshall, P. A., Abdulla, A., Beeden, R., Bergh, C., Black, R., et al. (2015). Operationalizing resilience for adaptive coral reef management under global environmental change. Glob. Chang. Biol. 21, 48–61. doi: 10.1111/gcb.12700
Ateweberhan, M., McClanahan, T. R., Graham, N. A. J., and Sheppard, C. R. C. (2011). Episodic heterogeneous decline and recovery of coral cover in the Indian Ocean. Coral Reefs 30, 739–752. doi: 10.1007/s00338-011-0775-x
Barott, K., Smith, J., Dinsdale, E., Hatay, M., Sandin, S., and Rohwer, F. (2009). Hyperspectral and physiological analyses of coral-algal interactions. PLoS One 4:e8043. doi: 10.1371/journal.pone.0008043
Barott, K., Williams, G., Vermeij, M., Harris, J., Smith, J., Rohwer, F., et al. (2012). Natural history of coral-algae competition across a gradient of human activity in the Line Islands. Mar. Ecol. Prog. Ser. 460, 1–12. doi: 10.3354/meps09874
Beatty, D., Clements, C., Stewart, F., and Hay, M. (2018). Intergenerational effects of macroalgae on a reef coral: major declines in larval survival but subtle changes in microbiomes. Mar. Ecol. Prog. Ser. 589, 97–114. doi: 10.3354/meps12465
Bellwood, D. R., and Choat, J. H. (1990). A functional analysis of grazing in parrotfishes (family Scaridae): the ecological implications. Environ. Biol. Fishes 28, 189–214. doi: 10.1007/BF00751035
Bellwood, D. R., Streit, R. P., Brandl, S. J., and Tebbett, S. B. (2018). The meaning of the term ‘function’ in ecology: a coral reef perspective. Funct. Ecol. 33, 948–961. doi: 10.1111/1365-2435.13265
Bender, D., Diaz-Pulido, G., and Dove, S. (2012). Effects of macroalgae on corals recovering from disturbance. J. Exp. Mar. Biol. Ecol. 429, 15–19. doi: 10.1016/j.jembe.2012.06.014
Beyer, H. L., Kennedy, E. V., Beger, M., Chen, C. A., Cinner, J. E., Darling, E. S., et al. (2018). Risk-sensitive planning for conserving coral reefs under rapid climate change. Conserv. Lett. 11:e12587. doi: 10.1111/conl.12587
Birrell, C., McCook, L., Willis, B., and Harrington, L. (2008). Chemical effects of macroalgae on larval settlement of the broadcast spawning coral Acropora millepora. Mar. Ecol. Prog. Ser. 362, 129–137. doi: 10.3354/meps07524
Birrell, C. L., McCook, L. J., and Willis, B. L. (2005). Effects of algal turfs and sediment on coral settlement. Mar. Pollut. Bull. 51, 408–414. doi: 10.1016/j.marpolbul.2004.10.022
Bonaldo, R., and Rotjan, R. (2018). “The good, the bad, and the ugly: parrotfishes as coral predators,” in The Biology and Ecology of Parrotfishes, eds A. Hoey, and R. Bonaldo, (Didcot: Taylor & Francis), 197–2015.
Bonaldo, R. M., and Hay, M. E. (2014). Seaweed-coral interactions: variance in seaweed allelopathy, coral susceptibility, and potential effects on coral resilience. PLoS One 9:e85786. doi: 10.1371/journal.pone.0085786
Bonaldo, R. M., Pires, M. M., Guimarães, P. R., Hoey, A. S., and Hay, M. E. (2017). Small marine protected areas in fiji provide refuge for reef fish assemblages, feeding groups, and corals. PLoS One 12:e0170638. doi: 10.1371/journal.pone.0170638
Bonito, V., Simpson, R., and Waqairagata, F. (2014). Evaluating the Performance of LMMAs in the Districts of Korolevu-i-wai, Dawasamu, and Nakorotubu. NOAA Technical Report NA09NOS4630017. Available at: https://data.nodc.noaa.gov/coris/library/NOAA/CRCP/other/grants/International_FY09_Products/Final_Report_NA09NOS4630017.pdf (accessed May 7, 2015).
Brandl, S. J., Hoey, A. S., and Bellwood, D. R. (2014). Micro-topography mediates interactions between corals, algae, and herbivorous fishes on coral reefs. Coral Reefs 33, 421–430. doi: 10.1007/s00338-013-1110-5
Brown, K. T., Bender-Champ, D., Bryant, D. E. P., Dove, S., and Hoegh-Guldberg, O. (2017). Human activities influence benthic community structure and the composition of the coral-algal interactions in the central Maldives. J. Exp. Mar. Biol. Ecol. 497, 33–40. doi: 10.1016/j.jembe.2017.09.006
Brown, K. T., Bender-Champ, D., Kubicek, A., van der Zande, R., Achlatis, M., Hoegh-Guldberg, O., et al. (2018). The dynamics of coral-algal interactions in space and time on the Southern Great Barrier Reef. Front. Mar. Sci. 5:181. doi: 10.3389/fmars.2018.00181
Bruno, J. F., Côté, I. M., and Toth, L. T. (2019). Climate change, coral loss, and the curious case of the parrotfish paradigm: why don’t marine protected areas improve reef resilience? Ann. Rev. Mar. Sci. 11, 307–334. doi: 10.1146/annurev-marine-010318-095300
Bulleri, F., Thiault, L., Mills, S., Nugues, M., Eckert, E., Corno, G., et al. (2018). Erect macroalgae influence epilithic bacterial assemblages and reduce coral recruitment. Mar. Ecol. Prog. Ser. 597, 65–77. doi: 10.3354/meps12583
Burkepile, D. E., and Hay, M. E. (2008). Herbivore species richness and feeding complementarity affect community structure and function on a coral reef. Proc. Natl. Acad. Sci. U.S.A. 105, 16201–16206. doi: 10.1073/pnas.0801946105
Burns, J. H. R., Delparte, D., Kapono, L., Belt, M., Gates, R. D., and Takabayashi, M. (2016). Assessing the impact of acute disturbances on the structure and composition of a coral community using innovative 3D reconstruction techniques. Methods Oceanogr. 15–16, 49–59. doi: 10.1016/j.mio.2016.04.001
Carassou, L., Léopold, M., Guillemot, N., Wantiez, L., and Kulbicki, M. (2013). Does herbivorous fish protection really improve coral reef resilience? A case study from New Caledonia (South Pacific). PLoS One 8:e60564. doi: 10.1371/journal.pone.0060564
Cheal, A. J., MacNeil, M. A., Cripps, E., Emslie, M. J., Jonker, M., Schaffelke, B., et al. (2010). Coral–macroalgal phase shifts or reef resilience: links with diversity and functional roles of herbivorous fishes on the Great Barrier Reef. Coral Reefs 29, 1005–1015. doi: 10.1007/s00338-010-0661-y
Chung, A., Oliver, T., Gove, J., Gorospe, K., White, D., Davidson, K., et al. (2019). Translating resilience-based management theory to practice for coral bleaching recovery in Hawai‘i. Mar. Policy 99, 58–68. doi: 10.1016/J.MARPOL.2018.10.013
Cinner, J. E., McClanahan, T. R., Graham, N. A. J., Pratchett, M. S., Wilson, S. K., and Raina, J. B. (2009). Gear-based fisheries management as a potential adaptive response to climate change and coral mortality. J. Appl. Ecol. 46, 724–732. doi: 10.1111/j.1365-2664.2009.01648.x
Clements, C., Rasher, D., Hoey, A., Bonito, V., and Hay, M. (2018). Spatial and temporal limits of coral-macroalgal competition: the negative impacts of macroalgal density, proximity, and history of contact. Mar. Ecol. Prog. Ser. 586, 11–20. doi: 10.3354/meps12410
Clements, C. S., and Hay, M. E. (2015). Competitors as accomplices: seaweed competitors hide corals from predatory sea stars. Proc. R. Soc. B Biol. Sci. 282:20150714. doi: 10.1098/rspb.2015.0714
Coles, S. L., Bahr, K. D., Rodgers, K. S., May, S. L., McGowan, A. E., Tsang, A., et al. (2018). Evidence of acclimatization or adaptation in Hawaiian corals to higher ocean temperatures. PeerJ 6:e5347. doi: 10.7717/peerj.5347
Connell, S., Foster, M., and Airoldi, L. (2014). What are algal turfs? Towards a better description of turfs. Mar. Ecol. Prog. Ser. 495, 299–307. doi: 10.3354/meps10513
Couch, C. S., Burns, J. H. R. R., Liu, G., Steward, K., Gutlay, T. N., Kenyon, J., et al. (2017). Mass coral bleaching due to unprecedented marine heatwave in Papahānaumokuākea Marine National Monument (Northwestern Hawaiian Islands). PLoS One 12:e0185121. doi: 10.1371/journal.pone.0185121
Darling, E. S., McClanahan, T. R., and Côté, I. M. (2010). Combined effects of two stressors on Kenyan coral reefs are additive or antagonistic, not synergistic. Conserv. Lett. 3, 122–130. doi: 10.1111/j.1755-263X.2009.00089.x
Daw, T. M., Cinner, J. E., McClanahan, T. R., Graham, N. A. J., and Wilson, S. K. (2011). Design factors and socioeconomic variables associated with ecological responses to fishery closures in the Western Indian Ocean. Coast. Manag. 39, 412–424. doi: 10.1080/08920753.2011.589224
De’ath, G., and Fabricius, K. (2010). Water quality as a regional driver of coral biodiversity and macroalgae on the Great Barrier Reef. Ecol. Appl. 20, 840–850. doi: 10.1890/08-2023.1
DeCarlo, T. M., Harrison, H. B., Gajdzik, L., Alaguarda, D., Rodolfo-Metalpa, R., D’Olivo, J., et al. (2019). Acclimatization of massive reef-building corals to consecutive heatwaves. Proc. R. Soc. B Biol. Sci. 286:20190235. doi: 10.1098/rspb.2019.0235
Diaz-Pulido, G., Harii, S., McCook, L. J., and Hoegh-Guldberg, O. (2010). The impact of benthic algae on the settlement of a reef-building coral. Coral Reefs 29, 203–208. doi: 10.1007/s00338-009-0573-x
Dixson, D. L., Abrego, D., and Hay, M. E. (2014). Chemically mediated behavior of recruiting corals and fishes: a tipping point that may limit reef recovery. Science 345, 892–897. doi: 10.1126/science.1255057
Done, T., Turak, E., Wakeford, M., DeVantier, L., McDonald, A., and Fisk, D. (2007). Decadal changes in turbid-water coral communities at Pandora Reef: loss of resilience or too soon to tell? Coral Reefs 26, 789–805. doi: 10.1007/s00338-007-0265-3
Doropoulos, C., Roff, G., Bozec, Y.-M., Zupan, M., Werminghausen, J., and Mumby, P. J. (2016a). Characterising the ecological trade-offs throughout the early ontogeny of coral recruitment. Ecol. Monogr. 86, 20–44. doi: 10.1890/15-0668.1
Doropoulos, C., Roff, G., Visser, M.-S., and Mumby, P. J. (2016b). Sensitivity of coral recruitment to subtle shifts in early community succession. Ecology 98, 304–314. doi: 10.1002/ecy.1663
Doropoulos, C., Roff, G., Zupan, M., Nestor, V., Isechal, A. L., and Mumby, P. J. (2014). Reef-scale failure of coral settlement following typhoon disturbance and macroalgal bloom in Palau, Western Pacific. Coral Reefs 33, 613–623. doi: 10.1007/s00338-014-1149-y
Doropoulos, C., Ward, S., Roff, G., González-Rivero, M., and Mumby, P. J. (2015). Linking demographic processes of juvenile corals to benthic recovery trajectories in two common reef habitats. PLoS One 10:e0128535. doi: 10.1371/journal.pone.0128535
Duran, A., Collado-Vides, L., Palma, L., and Burkepile, D. E. (2018). Interactive effects of herbivory and substrate orientation on algal community dynamics on a coral reef. Mar. Biol. 165:156. doi: 10.1007/s00227-018-3411-2
Edwards, C. B., Eynaud, Y., Williams, G. J., Pedersen, N. E., Zgliczynski, B. J., Gleason, A. C. R., et al. (2017). Large-area imaging reveals biologically driven non-random spatial patterns of corals at a remote reef. Coral Reefs 36, 1291–1305. doi: 10.1007/s00338-017-1624-3
Edwards, C. B., Friedlander, A. M., Green, A. G., Hardt, M. J., Sala, E., Sweatman, H. P., et al. (2013). Global assessment of the status of coral reef herbivorous fishes: evidence for fishing effects. Proc. R. Soc. B Biol. Sci. 281:20131835. doi: 10.1098/rspb.2013.1835
Evensen, N., Doropoulos, C., Morrow, K., Motti, C., and Mumby, P. (2019). Inhibition of coral settlement at multiple spatial scales by a pervasive algal competitor. Mar. Ecol. Prog. Ser. 612, 29–42. doi: 10.3354/meps12879
Ferrari, R., Gonzalez-Rivero, M., and Mumby, P. (2012). Size matters in competition between corals and macroalgae. Mar. Ecol. Prog. Ser. 467, 77–88. doi: 10.3354/meps09953
Fox, M. D., Williams, G. J., Johnson, M. D., Radice, V. Z., Zgliczynski, B. J., Kelly, E. L. A., et al. (2018). Gradients in primary production predict trophic strategies of mixotrophic corals across spatial scales. Curr. Biol. 28, 3355.e4–3363.e4. doi: 10.1016/j.cub.2018.08.057
Friedlander, A. M., Brown, E., and Monaco, M. E. (2007). Defining reef fish habitat utilization patterns in Hawaii: comparisons between marine protected areas and areas open to fishing. Mar. Ecol. Prog. Ser. 351, 221–233. doi: 10.3354/meps07112
George, E. E., Mullinix, J., Meng, F., Bailey, B., Edwards, C., Felts, B., et al. (2018). Relevance of coral geometry in the outcomes of the coral-algal benthic war. bioRxiv 327031. doi: 10.1101/327031
Goatley, C. H. R., Bonaldo, R. M., Fox, R. J., and Bellwood, D. R. (2016). Sediments and herbivory as sensitive indicators of coral reef degradation. Ecol. Soc. 21:art29. doi: 10.5751/ES-08334-210129
Goatley, C. H. R., Hoey, A. S., and Bellwood, D. R. (2012). The role of turtles as coral reef Macroherbivores. PLoS One 7:e39979. doi: 10.1371/journal.pone.0039979
Gove, J., Williams, G., McManus, M., Clark, S., Ehses, J., and Wedding, L. (2015). Coral reef benthic regimes exhibit non-linear threshold responses to natural physical drivers. Mar. Ecol. Prog. Ser. 522, 33–48. doi: 10.3354/meps11118
Graham, N. A. J., Jennings, S., MacNeil, M. A., Mouillot, D., and Wilson, S. K. (2015). Predicting climate-driven regime shifts versus rebound potential in coral reefs. Nature 518, 94–97. doi: 10.1038/nature14140
Graham, N. A. J., Nash, K. L., and Kool, J. T. (2011). Coral reef recovery dynamics in a changing world. Coral Reefs 30, 283–294. doi: 10.1007/s00338-010-0717-z
Graham, N. A. J., Wilson, S. K., Jennings, S., Polunin, N. V. C., Robinson, J., Bijoux, J. P., et al. (2007). Lag effects in the impacts of mass coral bleaching on coral reef fish, fisheries, and ecosystems. Conserv. Biol. 21, 1291–1300. doi: 10.1111/j.1523-1739.2007.00754.x
Griffin, K. J., Hedge, L. H., González-Rivero, M., Hoegh-Guldberg, O. I., and Johnston, E. L. (2017). An evaluation of semi-automated methods for collecting ecosystem-level data in temperate marine systems. Ecol. Evol. 7, 4640–4650. doi: 10.1002/ece3.3041
Haas, A., El-Zibdah, M., and Wild, C. (2010). Seasonal monitoring of coral–algae interactions in fringing reefs of the Gulf of Aqaba, Northern Red Sea. Coral Reefs 29, 93–103. doi: 10.1007/s00338-009-0556-y
Halpern, B. S., Selkoe, K. A., White, C., Albert, S., Aswani, S., and Lauer, M. (2013). Marine protected areas and resilience to sedimentation in the Solomon Islands. Coral Reefs 32, 61–69. doi: 10.1007/s00338-012-0981-1
Harrington, L., Fabricius, K., De’ath, G., and Negri, A. (2004). Recognition and selection of settlement substrata determine post-settlement survival in corals. Ecology 85, 3428–3437. doi: 10.1890/04-0298
Heenan, A., Hoey, A. S., Williams, G. J., and Williams, I. D. (2016). Natural bounds on herbivorous coral reef fishes. Proc. R. Soc. B Biol. Sci. 283:20161716. doi: 10.1098/rspb.2016.1716
Heenan, A., and Williams, I. D. (2013). Monitoring Herbivorous fishes as indicators of coral reef resilience in American Samoa. PLoS One 8:e79604. doi: 10.1371/journal.pone.0079604
Helyer, J., and Samhouri, J. F. (2017). Fishing and environmental influences on estimates of unfished herbivorous fish biomass across the Hawaiian Archipelago. Mar. Ecol. Prog. Ser. 575, 1–15. doi: 10.3354/meps12235
Hixon, M. A. (2015). “Reef fishes, seaweeds, and corals,” in Coral Reefs in the Anthropocene, ed. C. Birkeland (Dordrecht: Springer), 195–215. doi: 10.1007/978-94-017-7249-5_10
Hoegh-Guldberg, O. (1999). Climate change, coral bleaching and the future of the world’s coral reefs. Mar. Freshw. Res. 50, 839–866. doi: 10.1071/MF99078
Hoegh-Guldberg, O., Kennedy, E. V., Beyer, H. L., McClennen, C., and Possingham, H. P. (2018). Securing a long-term future for coral reefs. Trends Ecol. Evol. 33, 936–944. doi: 10.1016/j.tree.2018.09.006
Holbrook, S. J., Schmitt, R. J., Adam, T. C., and Brooks, A. J. (2016). Coral reef resilience, tipping points and the strength of herbivory. Sci. Rep. 6:35817. doi: 10.1038/srep35817
Hughes, T. P., Anderson, K. D., Connolly, S. R., Heron, S. F., Kerry, J. T., Lough, J. M., et al. (2018). Spatial and temporal patterns of mass bleaching of corals in the Anthropocene. Science 359, 80–83. doi: 10.1126/science.aan8048
Hughes, T. P., Kerry, J. T., Álvarez-Noriega, M., Álvarez-Romero, J. G., Anderson, K. D., Baird, A. H., et al. (2017). Global warming and recurrent mass bleaching of corals. Nature 543, 373–377. doi: 10.1038/nature21707
Hughes, T. P., Rodrigues, M. J., Bellwood, D. R., Ceccarelli, D., Hoegh-Guldberg, O., McCook, L., et al. (2007). Phase shifts, herbivory, and the resilience of coral reefs to climate change. Curr. Biol. 17, 360–365. doi: 10.1016/j.cub.2006.12.049
Humphries, A., McClanahan, T., and McQuaid, C. (2014). Differential impacts of coral reef herbivores on algal succession in Kenya. Mar. Ecol. Prog. Ser. 504, 119–132. doi: 10.3354/meps10744
Humphries, A. T., McQuaid, C. D., and McClanahan, T. R. (2015). Context-dependent diversity-effects of seaweed consumption on coral reefs in Kenya. PLoS One 10:e0144204. doi: 10.1371/journal.pone.0144204
Jompa, J., and McCook, L. (2003a). Contrasting effects of turf algae on corals: massive Porites spp. are unaffected by mixed-species turfs, but killed by the red alga Anotrichium tenue. Mar. Ecol. Prog. Ser. 258, 79–86. doi: 10.3354/meps258079
Jompa, J., and McCook, L. (2003b). Coral-algal competition: macroalgae with different properties have different effects on corals. Mar. Ecol. Prog. Ser. 258, 87–95. doi: 10.3354/meps258087
Jompa, J., and McCook, L. J. (2002). Effects of competition and herbivory on interactions between a hard coral and a brown alga. J. Exp. Mar. Biol. Ecol. 271, 25–39. doi: 10.1016/S0022-0981(02)00040-0
Jorissen, H., Skinner, C., Osinga, R., de Beer, D., and Nugues, M. M. (2016). Evidence for water-mediated mechanisms in coral–algal interactions. Proc. R. Soc. B Biol. Sci. 283:20161137. doi: 10.1098/rspb.2016.1137
Jouffray, J., Wedding, L. M., Norström, A. V., Donovan, M. K., Williams, G. J., Crowder, L. B., et al. (2019). Parsing human and biophysical drivers of coral reef regimes. Proc. R. Soc. B Biol. Sci. 286:20182544. doi: 10.1098/rspb.2018.2544
Kennedy, E. V., Perry, C. T., Halloran, P. R., Iglesias-Prieto, R., Schönberg, C. H. L., Wisshak, M., et al. (2013). Avoiding coral reef functional collapse requires local and global action. Curr. Biol. 23, 912–918. doi: 10.1016/j.cub.2013.04.020
Kramer, K. L., and Heck, K. L. (2007). Top-down trophic shifts in Florida Keys patch reef marine protected areas. Mar. Ecol. Prog. Ser. 349, 111–123. doi: 10.3354/meps07083
Ladd, M. C., Miller, M. W., Hunt, J. H., Sharp, W. C., and Burkepile, D. E. (2018). Harnessing ecological processes to facilitate coral restoration. Front. Ecol. Environ. 16:239–247. doi: 10.1002/fee.1792
Lefèvre, C. D., and Bellwood, D. R. (2010). Seasonality and dynamics in coral reef macroalgae: variation in condition and susceptibility to herbivory. Mar. Biol. 157, 955–965. doi: 10.1007/s00227-009-1376-x
Leong, R. C., Marzinelli, E. M., Low, J., Bauman, A. G., Lim, E. W. X., Lim, C. Y., et al. (2018). Effect of coral-algal interactions on early life history processes in Pocillopora acuta in a highly disturbed coral reef system. Front. Mar. Sci. 5:385. doi: 10.3389/fmars.2018.00385
Lokrantz, J., Nyström, M., Thyresson, M., Johansson, C., Nystrom, M., Thyresson, M., et al. (2008). The non-linear relationship between body size and function in parrotfishes. Coral Reefs 27, 967–974. doi: 10.1007/s00338-008-0394-3
Lough, J. M., Anderson, K. D., and Hughes, T. P. (2018). Increasing thermal stress for tropical coral reefs: 1871-2017. Sci. Rep. 8, 1–8. doi: 10.1038/s41598-018-24530-9
Magdaong, E. T., Fujii, M., Yamano, H., Licuanan, W. Y., Maypa, A., Campos, W. L., et al. (2014). Long-term change in coral cover and the effectiveness of marine protected areas in the Philippines: a meta-analysis. Hydrobiologia 733, 5–17. doi: 10.1007/s10750-013-1720-5
McClanahan, T. R. (2000). Recovery of a coral reef keystone predator, Balistapus undulatus, in East African marine parks. Biol. Conserv. 94, 191–198. doi: 10.1016/s0006-3207(99)00176-7
McClanahan, T. R. (2008). Response of the coral reef benthos and herbivory to fishery closure management and the 1998 ENSO disturbance. Oecologia 155, 169–177. doi: 10.1007/s00442-007-0890-0
McClanahan, T. R. (2014). Recovery of functional groups and trophic relationships in tropical fisheries closures. Mar. Ecol. Prog. Ser. 497, 13–23. doi: 10.3354/meps10605
McClanahan, T. R., Donner, S. D., Maynard, J. A., MacNeil, M. A., Graham, N. A. J., Maina, J., et al. (2012). Prioritizing key resilience indicators to support coral reef management in a changing climate. PLoS One 7:e42884. doi: 10.1371/journal.pone.0042884
McClanahan, T. R., Graham, N. A. J., Calnan, J. M., and MacNeil, M. A. (2007). Toward pristine biomass: reef fish recovery in coral reef marine protected areas in Kenya. Ecol. Appl. 17, 1055–1067. doi: 10.1890/06-1450
McClanahan, T. R., Maina, J., Starger, C. J., Herron-Perez, P., and Dusek, E. (2005). Detriments to post-bleaching recovery of corals. Coral Reefs 24, 230–246. doi: 10.1007/s00338-004-0471-1
McCook, L. J. (2001). Competition between corals and algal turfs along a gradient of terrestrial influence in the nearshore central Great Barrier Reef. Coral Reefs 19, 419–425. doi: 10.1007/s003380000119
Mcleod, E., Anthony, K. R. N., Mumby, P. J., Maynard, J., Beeden, R., Graham, N. A. J., et al. (2019). The future of resilience-based management in coral reef ecosystems. J. Environ. Manage. 233, 291–301. doi: 10.1016/j.jenvman.2018.11.034
Mumby, P. J., Bejarano, S., Golbuu, Y., Steneck, R. S., Arnold, S. N., van Woesik, R., et al. (2013a). Empirical relationships among resilience indicators on Micronesian reefs. Coral Reefs 32, 213–226. doi: 10.1007/s00338-012-0966-0
Mumby, P. J., Steneck, R. S., and Hastings, A. (2013b). Evidence for and against the existence of alternate attractors on coral reefs. Oikos 122, 481–491. doi: 10.1111/j.1600-0706.2012.00262.x
Mumby, P. J., Steneck, R. S., Adjeroud, M., and Arnold, S. N. (2016). High resilience masks underlying sensitivity to algal phase shifts of Pacific coral reefs. Oikos 125, 644–655. doi: 10.1111/oik.02673
Muñoz, R. C., Zgliczynski, B. J., Laughlin, J. L., and Teer, B. Z. (2012). Extraordinary aggressive behavior from the giant coral reef fish, Bolbometopon muricatum, in a remote marine reserve. PLoS One 7:e38120. doi: 10.1371/journal.pone.0038120
Nash, K. L., Abesamis, R., Graham, N., McClure, E., and Moland, E. (2016a). Drivers of herbivory on coral reefs: species, habitat and management effects. Mar. Ecol. Prog. Ser. 554, 129–140. doi: 10.3354/meps11795
Nash, K. L., Graham, N. A. J., Jennings, S., Wilson, S. K., and Bellwood, D. R. (2016b). Herbivore cross-scale redundancy supports response diversity and promotes coral reef resilience. J. Appl. Ecol. 53, 646–655. doi: 10.1111/1365-2664.12430
National Academies of Sciences, (2018). A Research Review of Interventions to Increase the Persistence and Resilience of Coral Reefs. Washington, DC: National Academies Press.
Neilson, B. J., Wall, C. B., Mancini, F. T., and Gewecke, C. A. (2018). Herbivore biocontrol and manual removal successfully reduce invasive macroalgae on coral reefs. PeerJ 6:e5332. doi: 10.7717/peerj.5332
Norström, A. V., Nyström, M., Jouffray, J.-B. B., Folke, C., Graham, N. A. J. A., Moberg, F., et al. (2016). Guiding coral reef futures in the Anthropocene. Front. Ecol. Environ. 14:490–498. doi: 10.1002/fee.1427
O’Leary, J. K., and McClanahan, T. R. (2010). Trophic cascades result in large-scale coralline algae loss through differential grazer effects. Ecology 91, 3584–3597. doi: 10.1890/09-2059.1
O’Leary, J. K., Potts, D. C., Braga, J. C., and McClanahan, T. R. (2012). Indirect consequences of fishing: reduction of coralline algae suppresses juvenile coral abundance. Coral Reefs 31, 547–559. doi: 10.1007/s00338-012-0872-5
Ong, L., and Holland, K. N. (2010). Bioerosion of coral reefs by two Hawaiian parrotfishes: species, size differences and fishery implications. Mar. Biol. 157, 1313–1323. doi: 10.1007/s00227-010-1411-y
Parravicini, V., Villeger, S., McClanahan, T. R., Arias-Gonzalez, J. E., Bellwood, D. R., Belmaker, J., et al. (2014). Global mismatch between species richness and vulnerability of reef fish assemblages. Ecol. Lett. 17, 1101–1110. doi: 10.1111/ele.12316
Pawlik, J. R., Burkepile, D. E., and Thurber, R. V. (2016). A vicious circle? Altered carbon and nutrient cycling may explain the low resilience of Caribbean coral reefs. Bioscience 66, 470–476. doi: 10.1093/biosci/biw047
Plass-Johnson, J. G., Ferse, S. C. A., Jompa, J., Wild, C., and Teichberg, M. (2015). Fish herbivory as key ecological function in a heavily degraded coral reef system. Limnol. Oceanogr. 60, 1382–1391. doi: 10.1002/lno.10105
Price, N. (2010). Habitat selection, facilitation, and biotic settlement cues affect distribution and performance of coral recruits in French Polynesia. Oecologia 163, 747–758. doi: 10.1007/s00442-010-1578-4
Puk, L. D., Ferse, S. C. A., and Wild, C. (2016). Patterns and trends in coral reef macroalgae browsing: a review of browsing herbivorous fishes of the Indo-Pacific. Rev. Fish Biol. Fish. 26, 53–70. doi: 10.1007/s11160-015-9412-z
Rasher, D. B., Engel, S., Bonito, V., Fraser, G. J., Montoya, J. P., and Hay, M. E. (2012). Effects of herbivory, nutrients, and reef protection on algal proliferation and coral growth on a tropical reef. Oecologia 169, 187–198. doi: 10.1007/s00442-011-2174-y
Rasher, D. B., and Hay, M. E. (2010). Seaweed allelopathy degrades the resilience and function of coral reefs. Commun. Integr. Biol. 3, 564–566. doi: 10.4161/cib.3.6.12978
Rasher, D. B., Hoey, A. S., and Hay, M. E. (2013). Consumer diversity interacts with prey defenses to drive ecosystem function. Ecology 94, 1347–1358. doi: 10.1890/12-0389.1
Rice, M. M., Ezzat, L., and Burkepile, D. E. (2019). Corallivory in the Anthropocene: interactive effects of anthropogenic stressors and corallivory on coral reefs. Front. Mar. Sci. 5:525. doi: 10.3389/fmars.2018.00525
Robinson, J. P. W., Williams, I. D., Yeager, L. A., McPherson, J. M., Clark, J., Oliver, T. A., et al. (2018). Environmental conditions and herbivore biomass determine coral reef benthic community composition: implications for quantitative baselines. Coral Reefs 37, 1157–1168. doi: 10.1007/s00338-018-01737-w
Rodgers, K. S., Jokiel, P. L., Bird, C. E., and Brown, E. K. (2009). Quantifying the condition of Hawaiian coral reefs. Aquat. Conserv. Mar. Freshw. Ecosyst. 20, 93–105. doi: 10.1002/aqc.1048
Roff, G., and Mumby, P. J. (2012). Global disparity in the resilience of coral reefs. Trends Ecol. Evol. 27, 404–413. doi: 10.1016/j.tree.2012.04.007
Russ, G. R., Questel, S.-L. A., Rizzari, J. R., and Alcala, A. C. (2015). The parrotfish–coral relationship: refuting the ubiquity of a prevailing paradigm. Mar. Biol. 162, 2029–2045. doi: 10.1007/s00227-015-2728-3
Schmitt, R. J., Holbrook, S. J., Davis, S. L., Brooks, A. J., and Adam, T. C. (2019). Experimental support for alternative attractors on coral reefs. Proc. Natl. Acad. Sci. U.S.A. 116, 4372–4381. doi: 10.1073/pnas.1812412116
Selig, E. R., and Bruno, J. F. (2010). A global analysis of the effectiveness of marine protected areas in preventing coral loss. PLoS One 5:e9278. doi: 10.1371/journal.pone.0009278
Selig, E. R., Casey, K. S., and Bruno, J. F. (2012). Temperature-driven coral decline: the role of marine protected areas. Glob. Chang. Biol. 18, 1561–1570. doi: 10.1111/j.1365-2486.2012.02658.x
Skirving, W. J., Heron, S. F., Marsh, B. L., Liu, G., De La Cour, J. L., Geiger, E. F., et al. (2019). The relentless march of mass coral bleaching: a global perspective of changing heat stress. Coral Reefs 38, 547–557. doi: 10.1007/s00338-019-01799-4
Smith, J. E., Hunter, C. L., and Smith, C. M. (2010). The effects of top–down versus bottom–up control on benthic coral reef community structure. Oecologia 163, 497–507. doi: 10.1007/s00442-009-1546-z
Smith, J. E., Runcie, J. W., and Smith, C. M. (2005). Characterization of a large-scale ephemeral bloom of the green alga Cladophora sericea on the coral reefs of West Maui, Hawai’i. Mar. Ecol. Prog. Ser. 302, 77–91. doi: 10.3354/meps302077
Stender, Y., Jokiel, P. L., and Rodgers, K. S. (2014). Thirty years of coral reef change in relation to coastal construction and increased sedimentation at Pelekane Bay, Hawai‘i. PeerJ 2:e300. doi: 10.7717/peerj.300
Steneck, R. S. (1986). The ecology of coralline algal crusts: convergent patterns and adaptative strategies. Annu. Rev. Ecol. Syst. 17, 273–303. doi: 10.1146/annurev.ecolsys.17.1.273
Stockwell, B., Jadloc, C., Abesamis, R., Alcala, A., and Russ, G. (2009). Trophic and benthic responses to no-take marine reserve protection in the Philippines. Mar. Ecol. Prog. Ser. 389, 1–15. doi: 10.3354/meps08150
Strain, E. M. A., Edgar, G. J., Ceccarelli, D., Stuart-Smith, R. D., Hosack, G. R., and Thomson, R. J. (2018). A global assessment of the direct and indirect benefits of marine protected areas for coral reef conservation. Divers. Distrib. 25, 1–12. doi: 10.1111/ddi.12838
Sully, S., Burkepile, D. E., Donovan, M. K., Hodgson, G., and van Woesik, R. (2019). A global analysis of coral bleaching over the past two decades. Nat. Commun. 10:1264. doi: 10.1038/s41467-019-09238-2
Swierts, T., and Vermeij, M. J. (2016). Competitive interactions between corals and turf algae depend on coral colony form. PeerJ 4:e1984. doi: 10.7717/peerj.1984
Tanner, J. E. (1995). Competition between scleractinian corals and macroalgae - an experimental investigation of coral growth, survival and reproduction. J. Exp. Mar. Bio. Ecol. 190, 151–168. doi: 10.1016/0022-0981(95)00027-o
Tebbett, S. B., and Bellwood, D. R. (2018). Functional links on coral reefs: Urchins and triggerfishes, a cautionary tale. Mar. Environ. Res. 141, 255–263. doi: 10.1016/j.marenvres.2018.09.011
van de Leemput, I. A., Hughes, T. P., van Nes, E. H., and Scheffer, M. (2016). Multiple feedbacks and the prevalence of alternate stable states on coral reefs. Coral Reefs 35, 857–865. doi: 10.1007/s00338-016-1439-7
van Hooidonk, R., Maynard, J., Tamelander, J., Gove, J., Ahmadia, G., Raymundo, L., et al. (2016). Local-scale projections of coral reef futures and implications of the Paris agreement. Sci. Rep. 6:39666. doi: 10.1038/srep39666
van Oppen, M. J. H., Gates, R. D., Blackall, L. L., Cantin, N., Chakravarti, L. J., Chan, W. Y., et al. (2017). Shifting paradigms in restoration of the world’s coral reefs. Glob. Chang. Biol. 23, 3437–3448. doi: 10.1111/gcb.13647
Vermeij, M. J. A., Smith, J. E., Smith, C. M., Vega Thurber, R., and Sandin, S. A. (2009). Survival and settlement success of coral planulae: independent and synergistic effects of macroalgae and microbes. Oecologia 159, 325–336. doi: 10.1007/s00442-008-1223-7
Williams, G. J., Gove, J. M., Eynaud, Y., Zgliczynski, B. J., and Sandin, S. A. (2015). Local human impacts decouple natural biophysical relationships on Pacific coral reefs. Ecography 38, 751–761. doi: 10.1111/ecog.01353
Williams, G. J., Knapp, I. S., Maragos, J. E., and Davy, S. K. (2011). Proximate environmental drivers of coral communities at Palmyra Atoll: establishing baselines prior to removing a WWII military causeway. Mar. Pollut. Bull. 62, 1842–1851. doi: 10.1016/j.marpolbul.2011.05.002
Williams, G. J., Sandin, S. A., Zgliczynski, B. J., Fox, M. D., Gove, J. M., Rogers, J. S., et al. (2018). Biophysical drivers of coral trophic depth zonation. Mar. Biol. 165:60. doi: 10.1007/s00227-018-3314-2
Williams, I. D., Walsh, W. J., Claisse, J. T., Tissot, B. N., and Stamoulis, K. A. (2009). Impacts of a Hawaiian marine protected area network on the abundance and fishery sustainability of the yellow tang, Zebrasoma flavescens. Biol. Conserv. 142, 1066–1073. doi: 10.1016/j.biocon.2008.12.029
Williams, I. D., White, D. J., Sparks, R. T., Lino, K. C., Zamzow, J. P., Kelly, E. L. A., et al. (2016). Responses of Herbivorous fishes and benthos to 6 years of protection at the Kahekili Herbivore fisheries management area, Maui. PLoS One 11:e0159100. doi: 10.1371/journal.pone.0159100
Wilson, S. K., Graham, N. A. J., Fisher, R., Robinson, J., Nash, K., Chong-Seng, K., et al. (2012). Effect of macroalgal expansion and marine protected areas on coral recovery following a climatic disturbance. Conserv. Biol. 26, 995–1004. doi: 10.1111/j.1523-1739.2012.01926.x
Wismer, S., Hoey, A., and Bellwood, D. (2009). Cross-shelf benthic community structure on the Great Barrier Reef: relationships between macroalgal cover and herbivore biomass. Mar. Ecol. Prog. Ser. 376, 45–54. doi: 10.3354/meps07790
Keywords: coral resilience, climate change, herbivory, parrotfish, marine protected areas, coral reef, algae, Indo-Pacific
Citation: Williams ID, Kindinger TL, Couch CS, Walsh WJ, Minton D and Oliver TA (2019) Can Herbivore Management Increase the Persistence of Indo-Pacific Coral Reefs? Front. Mar. Sci. 6:557. doi: 10.3389/fmars.2019.00557
Received: 17 May 2019; Accepted: 23 August 2019;
Published: 10 September 2019.
Edited by:
Thomas K. Frazer, University of Florida, United StatesReviewed by:
Thomas C. Adam, University of California, Santa Barbara, United StatesLyndon Mark DeVantier, Coral Reef Research, Australia
Copyright © 2019 Williams, Kindinger, Couch, Walsh, Minton and Oliver. This is an open-access article distributed under the terms of the Creative Commons Attribution License (CC BY). The use, distribution or reproduction in other forums is permitted, provided the original author(s) and the copyright owner(s) are credited and that the original publication in this journal is cited, in accordance with accepted academic practice. No use, distribution or reproduction is permitted which does not comply with these terms.
*Correspondence: Ivor D. Williams, aXZvci53aWxsaWFtc0Bub2FhLmdvdg==