- 1School of Science, University of Waikato, Hamilton, New Zealand
- 2National Institute of Water and Atmospheric Research, Hamilton, New Zealand
- 3Waikato Regional Council, Hamilton, New Zealand
The supply of ecosystem services (ES) that benefit humanity are derived from multiple, interacting ecological functions and processes. Focusing on the ecological mechanisms that underpin ES delivery allows bundles of services to be identified, bridging a critical gap with management. Work in marine systems has not yet progressed to the identification of ES bundles, as a result of data scarcity and complications arising from system complexity and connectivity, as opposed to terrestrial systems where ES bundles have been more widely applied based on spatial clustering. To demonstrate the approach, identification of ES bundles provided by shellfish is used as a case-study. Shellfish provide a number of known ES that need to be strategically managed to ensure sustainable use. As a result of global degradations in shellfish beds ES have been lost, and restoration efforts emphasize the importance in regaining these services. A literature review, including 146 papers aimed specifically at linking shellfish to either ecosystem functions or ES, was conducted to establish key linkages between processes, functions and services. Based on co-occurrence of services and shared linkages, four bundles of services are identified, including Marine resources, Coastal health and quality, Habitat modification, and Biological structuring. Our study emphasizes the underpinning ecological mechanisms and the importance of interactions between services, expressed in the formation of bundles by mutual drivers and processes, as well as between services in different bundles, as either synergies or trade-offs. The approach enables the translation of ecological knowledge and creates generality to inform policy making and management, thereby providing a format useful for ecologists, managers and other stakeholders.
Introduction
Ecosystem services (ES) are a means of linking the natural environment to various benefits that humans are able to extract, utilize or experience (Daily, 1997; Millennium Ecosystem Assessment [MEA], 2005; de Groot et al., 2010). This explicit recognition can facilitate improved environmental resource management. To implement this concept, it is important to understand how the structure, processes and functions of ecosystems relate to the generation of different services (Müller and Burkhard, 2007; de Groot et al., 2010; QUINTESSENCE Consortium, 2016; Culhane et al., 2018). These relationships are numerous and complex and do not necessarily show a one-to-one correspondence, as exemplified by Snelgrove et al. (2014) who showed the multiple, complex linkages between biodiversity, processes, functions and services for seafloor environments. On top of this complexity, processes span multiple spatial and temporal scales, which affect where, when and how services are delivered (Raffaelli and White, 2013). Bennett et al. (2009) provide a typology for ES relationships, including the impact of drivers on multiple ES as well as the level of interactions, thereby demonstrating the need to study multiple, rather than individual services. Hence, understanding the relationships between services (effectively their inter-dependence and collinearity) has been proven important (Bennett et al., 2009; Lester et al., 2013; Howe et al., 2014) and resulted in the development of the ES bundles concept (Raudsepp-Hearne et al., 2010). ES bundles, defined as “sets of associated services that appear together repeatedly across space and/or time” (Raudsepp-Hearne et al., 2010) allow the assessments of trade-offs and synergies among services in complex and changing environments.
Most work to date on ES bundles has focused on terrestrial environments, using cluster methods to identify spatial patterns in service delivery (Raudsepp-Hearne et al., 2010; Turner et al., 2014; Queiroz et al., 2015). However, marine, and especially estuarine and coastal environments, provide ES that need to be strategically managed to ensure sustainable use (Barbier et al., 2011). Studies in the marine environment are fewer than those of terrestrial systems as a result of data scarcity, and complications arising from system complexity and connectivity, spatial scales and context, and the 3-dimensional use of these environments (Guerry et al., 2012; Townsend et al., 2018). Even though the importance of understanding links between biodiversity, ecosystem function and service delivery is emphasized (Kremen and Ostfeld, 2005; Haines-Young and Potschin, 2010; Nagendra et al., 2013), few studies have been able to incorporate this in ES identification and quantification. In terrestrial systems, functional traits of plants have been used as a way of including ecological mechanisms in determining ecosystem service delivery (de Bello et al., 2010; Lavorel et al., 2011; Lavorel and Grigulis, 2012; Lamarque et al., 2014). While research in the marine environment has focused on the links between biodiversity and ecosystem functions (Solan et al., 2006; Stachowicz et al., 2007; Gamfeldt et al., 2015), links to ES and especially bundles of services in this environment remain unclear.
The idea of bundles of services, whether or not ecologically underpinned, is useful for resource managers, policy makers, communities and as an interdisciplinary tool helping stakeholders understand the value of multiple services. Work on individual and multiple ES in the marine environment can be used to inform ecosystem based management (EBM) and marine spatial planning (MSP) (McLeod and Leslie, 2009; Granek et al., 2010; Lester et al., 2013). Application of the ES bundle approach and understanding the underpinning ecology can facilitate sustainable management of resources, a key aspect of current and future marine management to ensure the continuation of the services they provide (Martínez-Harms and Balvanera, 2012). It will also contribute to conservation of biodiversity and ecosystem resilience to prevent tipping points in ES provision (Bennett et al., 2009; Maes et al., 2012b). Loss of ES has been a key motivation for ecological restoration of degraded habitats (Bullock et al., 2011). For example, shellfish beds and reefs have degraded globally (Beck et al., 2011), which has resulted in negative impacts on environmental health (Grabowski and Peterson, 2007) and recovering lost ES has motivated shellfish restoration efforts (Coen and Luckenbach, 2000; Cerco and Noel, 2007; Coen et al., 2007; Schulte et al., 2009; Beck et al., 2011). Shellfish provide a number of ES beyond the provision of food, including regulating services like water quality regulation, and sediment or shoreline stabilization, as well as a number of habitat and supporting services, such as habitat provision and increasing biodiversity (Figure 1; Grabowski et al., 2012; van der Schatte Olivier et al., 2018). Thousands of ecological studies world-wide provide insight in the structure and processes created and altered by shellfish. However, the multitude of services they provide have not been explicitly linked back to these linkages in underlying functions and processes, nor have interactions between services been studied.
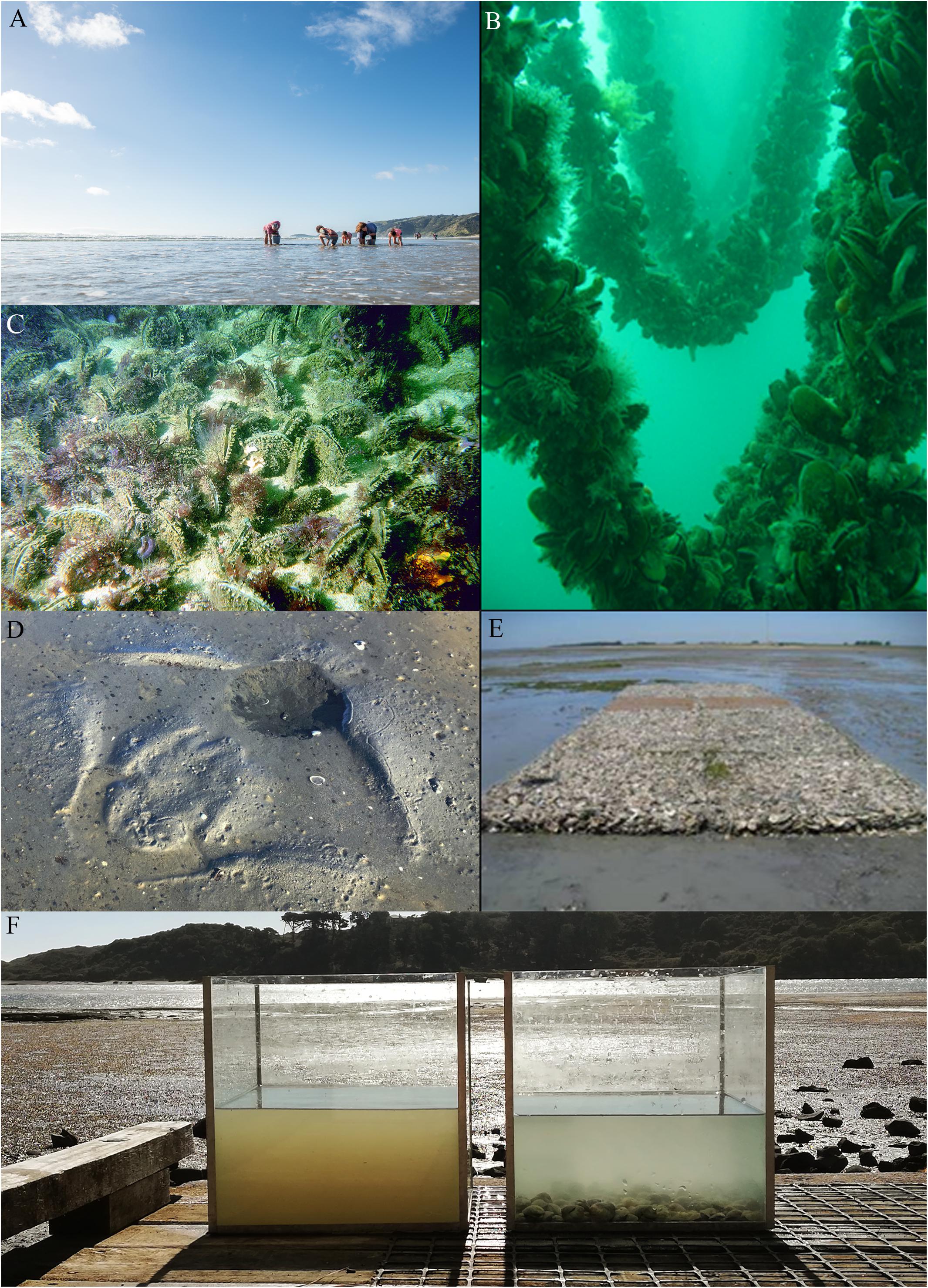
Figure 1. Examples of ecosystem services provided by shellfish. (A) Recreational gathering of shellfish for food in New Zealand (Chris Williams, NZ Story), (B) mussel (Perna canaliculas) aquaculture (Chris Woods), (C) habitat provision by horse mussel (Atrina zelandica) beds (Simon Thrush), (D) infaunal shellfish (Austrovenus stutchburyi, Macomona liliana) as a food source for stingrays on the intertidal sandflat (Helen Cadwallader), (E) sediment and shoreline stabilization by artificial oyster (Crassostrea gigas) reefs (NIOZ, EcoShape), and (F) water clarity improvements and contaminant removal through filter feeding, comparing aquaria with (right) and without (left) shellfish (A. stutchburyi) (Vera Rullens). Images reproduced with permission.
The aim of the current study was to review the ecological mechanisms that underpin service delivery to determine ES bundles, applied to shellfish dominated systems as a case study. Shellfish-associated processes and functions were then linked to ES to investigate the potential for complex interactions. The role of shellfish in estuarine and coastal environments, and how they affect ecosystem functions, has been studied extensively, although our understanding of how services are generated and what drivers or stressors might affect them remains unconsolidated. This work will enable investigations of the interactions between services, including the potential for tradeoffs and synergies within and between bundles. This provides an example of a different approach identifying ES bundles in data-sparse (marine) environments, and could be applied to other habitats or key species where sufficient ecological knowledge is available to elucidate these linkages and relationships. The approach is novel as it shifts toward a focus on ecological processes driving services supply, and provides a format useful for ecologists, managers and other stakeholders to translate and generalize ecological knowledge into the ES framework.
Materials and Methods
Scope of Review
To explore the complex linkages and interactions between processes, functions and services, a literature review was conducted to extract the current ecological knowledge on the mechanisms that underpin shellfish service delivery. The aim of this review was on the higher level ecological mechanisms, with a focus on well-understood, generalizable concepts, and on elucidating the key linkages in service generation. Although there are thousands of peer-reviewed ecological publications that focus on shellfish, we limited our review to studies specifically targeting ecosystem functions and services. In this study, shellfish habitats are defined as a location where shellfish dominate the benthic biomass, and function as ecosystem engineers (sensu Jones et al., 1994). Both epifaunal reefs and infaunal shellfish beds are therefore considered, as both can significantly create, modify and maintain habitats, thereby changing the physical state of the environment, controlling the availability of resources to other species, and affecting the ecosystem functioning of the system at a scale larger than the habitat itself (Jones et al., 1994; Gutiérrez et al., 2003; Bouma et al., 2009). In addition to natural habitats (reefs and beds), studies that focused on highly modified (aquaculture) settings were also included within this review.
Literature Review Details
This research was based on findings reported in 146 peer-reviewed papers. Papers were derived from a literature search in ISI Web of Science database (December 2017), using combinations of search terms applied to title, themes, and abstracts. The search terms used included combinations of either “Ecosystem service” or “Ecosystem function” with a search term for shellfish, i.e., “Shellfish,” “Bivalve,” “Clam,” etc. In total, 202 papers matched these search criteria, which were then screened for relevance based on title, abstract and/or paper content. Papers were excluded if they were not specific to marine shellfish, did not focus on the links between shellfish and processes that affect functions or service delivery, or were not focused on current studies in estuarine or coastal habitats (i.e., excluding for example paleo-ecological studies, or studies in deep-sea or polar environments).
The resulting 146 papers were reviewed to extract general information on study type (e.g., experimental, observational, review) and provide an overview of what was studied, where and when, the species and environment studied. Specific emphasis was placed on the species type, categorized for “epifaunal suspension feeders,” “infaunal suspension feeders,” “infaunal deposit feeders” and the more general “bivalves” if not further specified. Emphasis is placed on these distinct groups as they affect ecosystem functioning differently, driven by their feeding mechanisms (suspension vs. deposit) and position in or on the sediment (infaunal vs. epifaunal, respectively). The list of presented services was not specified prior to the review but was guided by the literature to ensure all those discussed were included. Shellfish not only provide “final” services that can be directly utilized (Fisher et al., 2009), but also a number of “intermediate” (or supporting) services that contribute to maintaining high-level functioning and resilience of coastal systems, which were included to maintain the nuances found in the reviewed literature. Services indirectly provided by other species or habitats that flow on from the supporting services provided by shellfish were beyond the scope of this paper. This resulted in the inclusion of two provisioning services, five regulating services, and five habitat and supporting services (Table 1). To allow comparison with more generalized frameworks, the TEEB classification categories (de Groot et al., 2010) are included in Table 1 for these services. Cultural services were excluded as they are more subjective and context specific, and are strongly underpinned by social variables, like identity, country of origin, ethnicity, religion, and income level (Stephenson, 2008; Dickinson and Hobbs, 2017), rather than the ecological processes and functions that this review targeted.
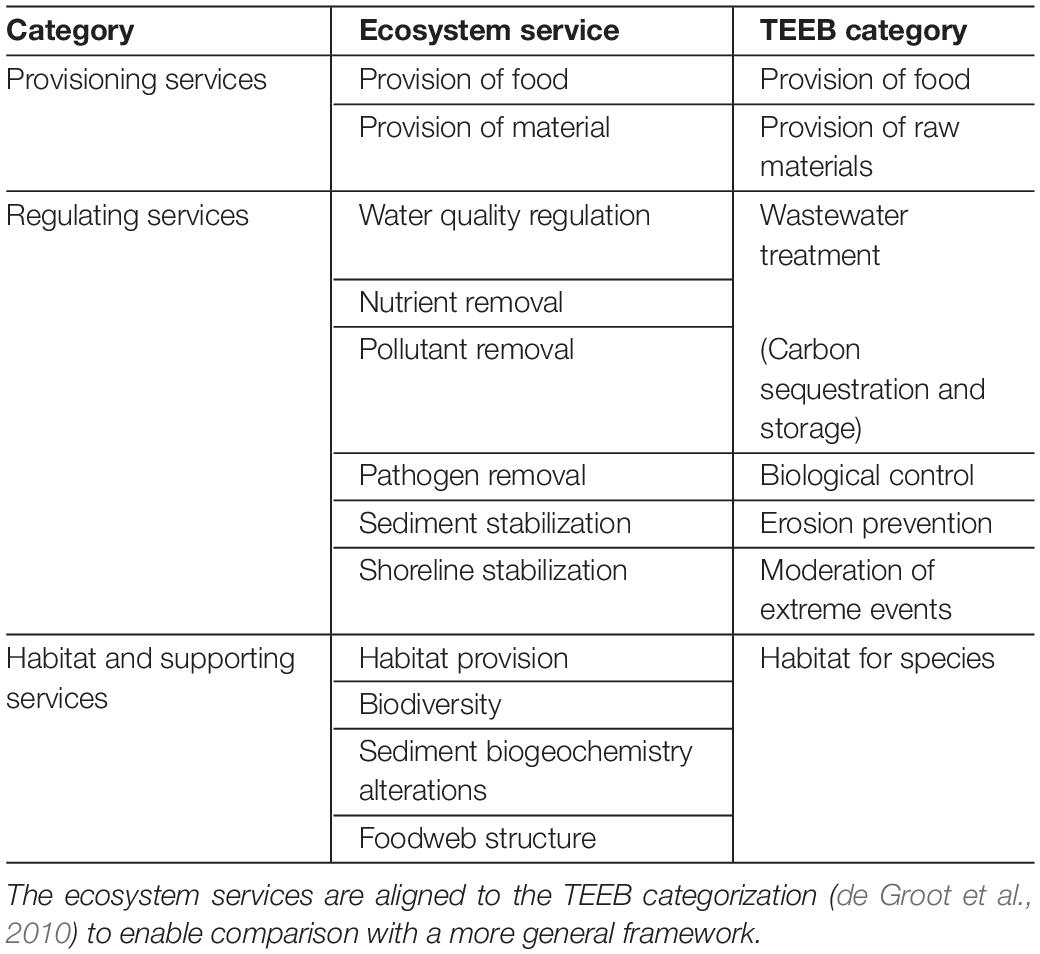
Table 1. Overview of the ecosystem services found in the reviewed literature as function of service category.
The main focus of the review was to establish the links between processes, functions and services, which were documented for each paper in the review. Most papers discussed only one ecosystem function or service, hence they were documented as one input that described the identified link between process-function-service. A number of studies discussed multiple services and links with processes and functions that needed to be summarized. When studies discussed multiple services resulting from the same underpinning processes and functions, their input was as one, while if they were underpinned by different processes, they were treated separately. For example, if a paper included information on how filter feeding affects both the services of water quality regulation, and nutrient cycling/removal, this was considered one input. However, if they studied two services that were underpinned by different processes, like growth underpinning food provision, and filter feeding underpinning water quality regulation, these were considered as two separate inputs from the same paper. Furthermore, for each paper, the main driver or stressor was noted as well as their impact on service delivery (positive, negative, or neutral) with further explanations.
Bundle Identification, Cascades, and Interactions
The links identified between processes, functions and services (see Appendix 2 in Supplementary Materials) formed the basis for bundle identification. Linkages were quantified per service in terms of the number of papers discussing a specific link (Appendix 1 in Supplementary Materials). Links were included if described in three or more papers and if they were well established and understood. If the same linkage was found for more than one service, these services were grouped together to form the initial bundles. Once grouped, all included linkages were examined for similarities in their effects on the services and the services within each group for their co-occurrence to form the four final bundles. Each service was only categorized for one bundle, but underpinning processes or functions could be used more than once, due to the ecological complexity of the system and key processes provided by shellfish. Overlapping processes and/or functions were therefore allowed, if their effects on service delivery differed, for example, biological vs. physical effects. Each of the identified bundles contained two to four services, and were underpinned by key processes and functions. To provide more detail about the ecological mechanisms behind each of the bundles, the underpinning processes and functions were mapped and simplified to represent the main links from the literature to the provisioning of the services in cascade diagrams. In the section on “ES bundles for shellfish” below, the four bundles are described, followed by further explanation and examples of the mechanisms generating them.
Even when services are bundled, they are not completely independent, as interactions i.e., synergies and tradeoffs, between bundles are still possible. Synergies are defined as “a situation where the use of one ES directly increases the benefits supplied by another service,” while tradeoffs are defined as “a situation where the use of one ES directly decreases the benefits supplied by another” (Turkelboom et al., 2015). A subset of the data was used to study these interactions, by including those that look at multiple services from different bundles, which could be underpinned by the same process and function, in which case they are considered as “interactions,” or those that were treated separately, as explained above. In some cases, a service was mentioned as a driver or stressor of another service, in which case they were also included in the subset looking at interactions. For example, aquaculture was considered as the main driver or stressor of the delivery of other services, whilst not discussing the provisioning benefits generated by aquaculture itself.
ES Bundles for Shellfish
Ecosystem Services Bundles
From the obtained linkages in the literature review, four bundles of services are identified for shellfish (Figure 2) based on 21 key linkages (Appendix 1 in Supplementary Materials). The first bundle, Marine resources, contains all services related to the provision of goods, including food and shell material. In total, information from 25 papers are included in this bundle, mainly with a focus on the provision of food (24 papers total) from either natural environments (11 papers) or artificially through aquaculture (13 papers). The second bundle, Coastal health and quality, includes the effects of shellfish on water quality regulation and the removal of contaminants from the water, including nutrients, pollutants and pathogens. Data from 51 papers supports the linkages for this bundle, with the majority of papers focusing on water quality regulation (27 papers) and/or nutrient cycling/removal (25 papers), while less emphasis is placed on removal of other pollutants (9 papers) and pathogens (4 papers). The Habitat modification bundle includes the physical effects shellfish have on the environment, through their role as ecosystem engineers, as discussed in 44 papers. This includes their effect on stabilizing shorelines (6 papers) and sediments (7 papers), and their effect on altering sediment biogeochemistry (25 papers). The final bundle Biological structuring, includes the services by which shellfish have biological effects on the environment by supporting other species and communities. This is based on data from 54 papers, that demonstrate shellfish providing habitat (22 papers), altering biodiversity (26 papers) and food web structure (19 papers), which results in and contributes to the intrinsic value and resilience of the system.
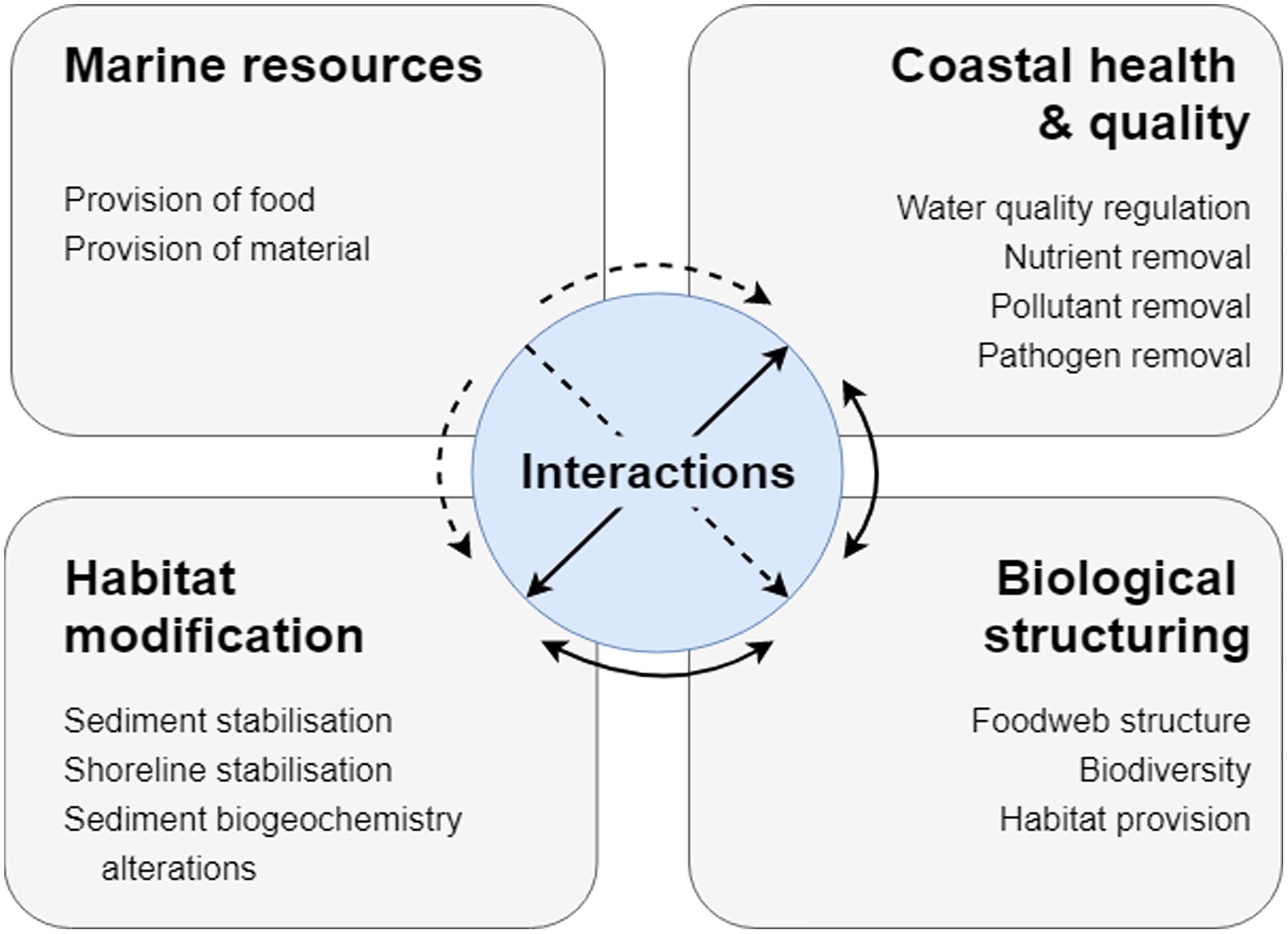
Figure 2. Bundles of ecosystem services provided by shellfish. Each box contains the services grouped for the respective bundles. Arrows between bundles in the center of the figure indicate the interactions between bundles either as synergies (black bi-directional arrows) or trade-offs (dashed one-directional arrows).
Linkages Cascades per Bundle
Marine Resources
The Marine resources bundle is underpinned by three main processes: shellfish survival, growth, and recruitment, which determine the amount of biomass generated in the system that can be harvested (Figure 3). In the review, 15 papers described the contribution of biomass production to the delivery of goods. Food provision (i.e., the production of edible shellfish biomass) is strongly dependent on the biomass produced in a system and the production yield in a region. Biomass can be harvested through commercial or recreational collection of natural populations, or from artificial (aquaculture) set-ups specifically aimed to grow shellfish as a food source. Aquaculture examples are discussed where shellfish are viewed as being increasingly important for the production of proteins and thereby as an alternative for exploiting natural resources (Kluger et al., 2017), resulting in benefits to local economies and employment (Ferreira and Bricker, 2016). In some cases, invasive species are used for aquaculture (Ruesink et al., 2006; Humphreys et al., 2015), where the annual yield generated by these species can exceed the historical landing of native species (Ruesink et al., 2006). Limited information was available in the review on the provision of material, except for some papers discussing shell formation. Shell formation and calcification were discussed in relation to the impact of ocean acidification and thermal stress, which have a negative impact on shell growth and thickness (Hiebenthal et al., 2013; Lacoue-Labarthe et al., 2016), that not only affects the provision of materials but could also impact bivalve fisheries revenues (Marshall et al., 2017).
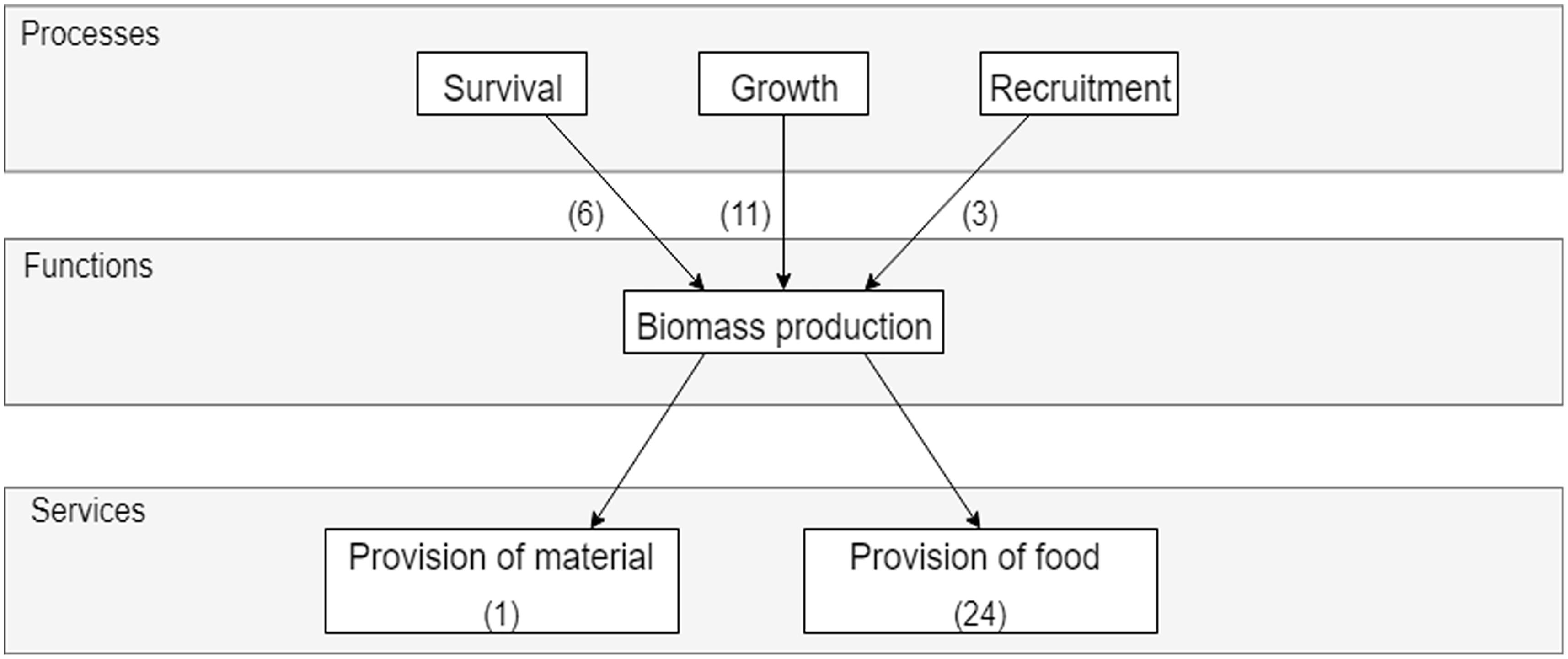
Figure 3. Simplified cascade showing the links between processes, functions, and services in the Marine resources bundle. The number of papers discussing a service is indicated in brackets within the service box, while the number of times links between processes and functions were discussed is indicated in brackets next to the arrows.
The effect of growth was discussed 11 times as the underpinning process for this bundle, where growth rates of shellfish determine how much biomass is generated over time, with higher growth rates resulting in higher service delivery. Growth rates are dependent on environmental variables and can vary temporally (Li et al., 2012) and spatially, as is shown for mussels along the Swedish coast (Bergstrom et al., 2015) where models and empirical studies were used to spatially determine growth rates over a 2 month timeframe. Survival (or mortality) of shellfish affects the amount of biomass available, as discussed in six reviewed papers, where high survival rates result in greater service delivery. Stressors generally have a negative impact on survival rates. For example, emergence of infaunal shellfish from the sediment when stressed by macroalgal blooms or hypoxia, and physical distress from high temperatures can alter mortality rates (Lewis and DeWitt, 2017). Disease outbreaks (Wilkie et al., 2013) can affect survival rates of farmed and wild shellfish populations, but also could make them unsafe for harvest and human consumption, thereby resulting in a loss of value. Finally, recruitment of juveniles affects biomass production, particularly in restoration areas (Marsden and Adkins, 2010). The methods used for harvesting can also affect recruitment, where Toupoint et al. (2016) show that hand raking, a method of recreational harvesting, inhibited primary recruitment, whereas aquaculture promoted primary recruitment intensity.
Coastal Health and Quality
The key process underpinning the Coastal health and quality bundle is filter feeding by shellfish, as discussed in 38 out of 51 papers for this bundle. Through filter feeding, shellfish act as biofilters, removing suspended particles from the water column, thereby affecting all services in this bundle (Figure 4). Water quality regulation is the service most frequently discussed in the scientific literature (27 times), with a strong focus on epifaunal-suspension feeders (e.g., oyster and mussel reefs). By removing phytoplankton and suspended sediments from the water column, filtration improves water clarity by reducing turbidity and increasing light penetration. This, together with the effect shellfish have on the exchange of biomass, energy, or nutrients between the sediment and water column, i.e., benthic-pelagic coupling, results in the service of water quality regulation. Filtration rates are size and density dependent, and depend on a number of variables including phytoplankton, organic matter or seston concentrations (MacDonald and Ward, 2009; Galimany et al., 2017b), temperature (Zu Ermgassen et al., 2013a), dissolved oxygen, and turbulence (Li et al., 2012). A number of studies have focused on the effect of oyster reef declines and restoration effort on filtration rates. For example, an 80% decline in filtering capacity was found when comparing past and present situations in Chesapeake bay (Zu Ermgassen et al., 2013b) and restoration of reefs can help regain lost services (Coen and Luckenbach, 2000; Grizzle et al., 2008; Baggett et al., 2015; Milbrandt et al., 2015). Filtration rates for invasive versus native species were compared, where invasive mussels were more efficient than native species that occupied similar niche space (Galimany et al., 2017a), thereby outcompeting them (Ruesink et al., 2006).
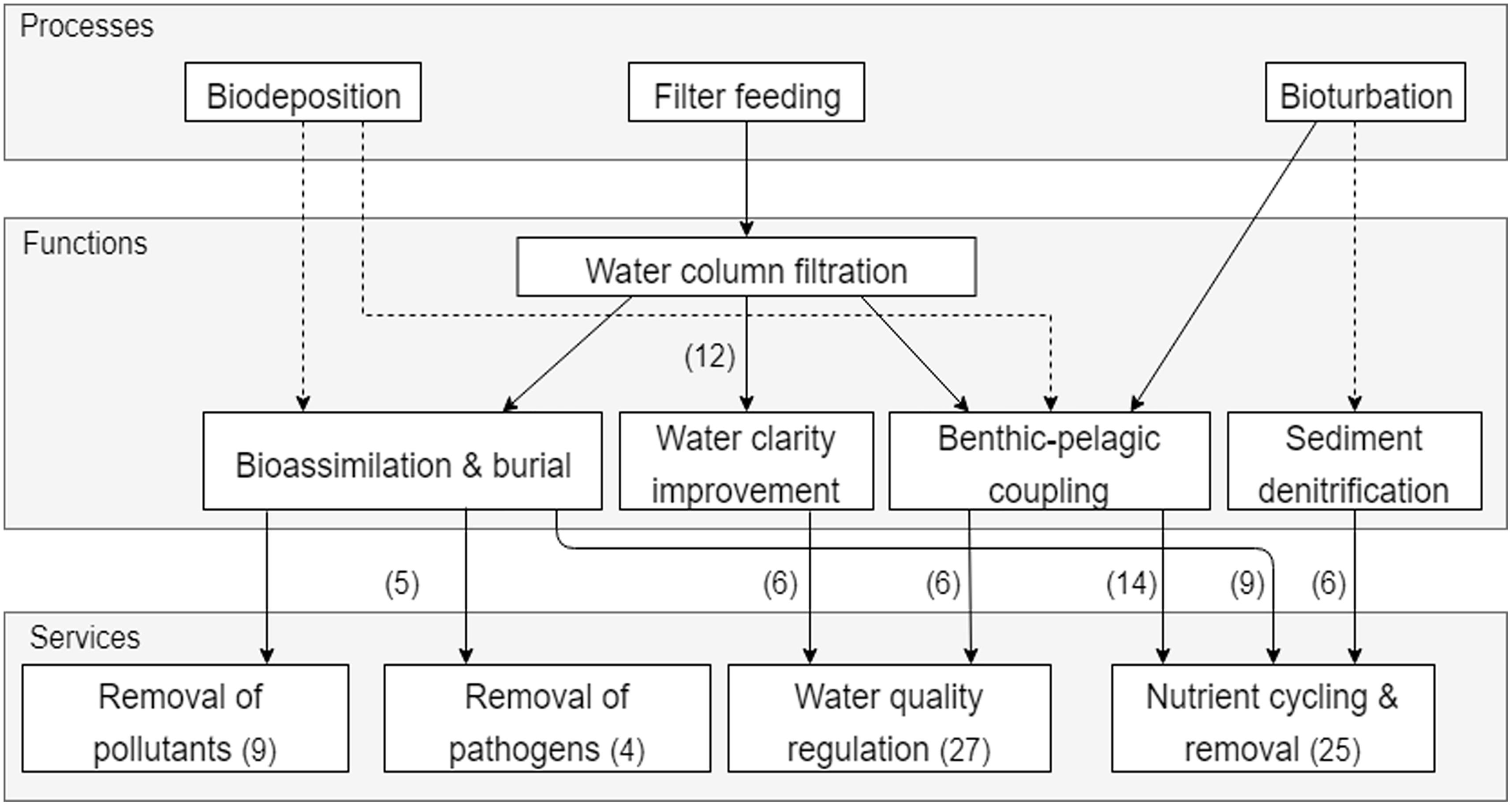
Figure 4. Simplified cascade showing the links between processes, functions, and services in the Coastal health and quality bundle. The number of papers discussing a service is indicated in brackets within the service box, while the number of times links between processes and functions were discussed is indicated in brackets next to the arrows. Dashed lines indicate links that are inferred from expert knowledge.
Benthic-pelagic coupling drives both water quality regulation, as well as nutrient cycling and removal. Shellfish contribute to benthic-pelagic coupling through filter feeding, by moving particles from the water column to the sediment as biodeposits (Kent et al., 2017a), and can alter pelagic community structure and trophic interactions in the system (Orlova et al., 2006; Sunda et al., 2006; Filgueira et al., 2016). Biodeposition by both suspension and deposit feeders also increases sedimentation rates and modifies the physical, chemical, and bacterial composition properties of settling particles (Karlson et al., 2010; Kanaya, 2014) altering rates of nutrient cycling (including denitrification) and burial (Cerco, 2015; Kent et al., 2017a). Modifications in the physico-chemical benthic environments, through for example bioturbation, can affect the cycling and removal of nutrients by affecting ammonia fluxes at the sediment water interface (Thrush et al., 2006; Jones et al., 2011; Filgueira et al., 2016; Lohrer et al., 2016) and denitrification in the sediment that removes bio-available nitrogen from the system to the atmosphere (Kellogg et al., 2013; Cerco, 2015; Welsh et al., 2015). Nutrient cycling and removal is also affected by bioassimilation of nutrients in tissue and burial of organic matter, as well as nutrient excretion by shellfish. A number of studies quantify nutrient removal from the system through assimilation in tissue or shell and often in relation to aquaculture settings (Sebastiano et al., 2015; Galimany et al., 2017c). Eutrophication can have direct negative impacts on estuarine ecosystems through phytoplankton blooms, as well as indirect effects on denitrification, some of which could be mitigated by bivalve filter feeding through phytoplankton biomass control and should be considered in management decisions (Ferreira and Bricker, 2016).
The removal of pollutants and pathogens from the system is also linked to bioassimilation and burial (Figure 4). Bivalves can act as a filter for bacteria and contaminants thereby removing them from the system (Volety et al., 2014; Broszeit et al., 2016). Once ingested, toxins or bacteria can either be assimilated in tissue or discarded in biodeposits that are buried in the sediment. Burge et al. (2016) reviewed the role of filter feeders on pathogen removal through augmentation and reduction, with emphasis on the role of bivalves. Their findings suggested that the effects of filter feeding on pathogen transmission and disease risk can be either positive or negative depending on the bivalve and pathogen specific selectivity or mechanisms. Pathogens can be removed from the system by degradation and released in biodeposits. If pathogens are able to resist degradation and are bioassimilated, this can pose a threat to humans and wildlife upon ingestion through biomagnification. Bivalves can remove a number of pollutants from the system by bioremediation of wastes (Broszeit et al., 2016) and biotransformation of contaminants that alter their bioavailability (Montes et al., 2012). As a result of the bioassimilation in tissue, shellfish are often considered as bioindicators of toxins in estuarine habitats (Chapman et al., 2013; Burge et al., 2016). Carbon sequestration is a form of pollution removal occurring on a much longer temporal scale, but is underpinned by the same processes and functions of bioassimilation and burial. A couple of examples were found in the literature showing how shellfish can contribute to carbon removal through burial (Cerco, 2015) or through carbon sequestration in shells (Talmage and Gobler, 2010; Volety et al., 2014).
Habitat Modification
Habitat modification is linked to the role of shellfish as ecosystem engineers, where they modify habitat by interacting with the physical environment around them (Figure 5). Infaunal shellfish interact with their environment mostly by bioturbation, thereby reworking the sediment (supported by 9 papers), whereas epifaunal shellfish do so by reef formation (supported by 6 papers). Sediment reworking by infaunal shellfish drives a number of ecosystem functions and the resulting services of sediment biogeochemistry alterations and sediment stabilization (as discussed in 24 and 7 papers, respectively). Shellfish alter sediment biogeochemistry through the burial of organic matter to depth (Maire et al., 2006; Sousa et al., 2009; Kanaya, 2014), cause changes in benthic metabolism and nutrient fluxes between the sediment-water interface in soft sediment-habitats (Rossi et al., 2008; Sandwell et al., 2009; Lohrer et al., 2010; Lohrer et al., 2012; Norkko et al., 2013; Premo and Tyler, 2013), as well as changes in the Redox Potential Discontinuity Layer depth (Clare et al., 2016). Sediment biogeochemistry alterations are affected by key species composition (Michaud et al., 2009) and density (Sandwell et al., 2009; Clare et al., 2016; Sospedra et al., 2017), predator presence affecting burrowing behavior (Maire et al., 2010), and feeding behavior or the availability of food (Maire et al., 2006; Karlson et al., 2010). This supporting service is important in soft sediment habitats as it affects productivity and overall condition of the system, but is susceptible to a number of physical stressors. Terrestrial sediment deposits of a couple of mm can have a significant effect on this service (Pratt et al., 2014), as not only will the deposition alter physical properties of the sediment, but has also been shown to cause subtle changes in behavior of adults and juvenile shellfish (Hohaia et al., 2014; McCartain et al., 2017). Similarly, smothering or hypoxia can greatly impair the benthic communities, and in particular shellfish, and their ability to contribute to sediment oxygen and nutrient fluxes (Rossi et al., 2008; Lohrer et al., 2010; Villnas et al., 2012).
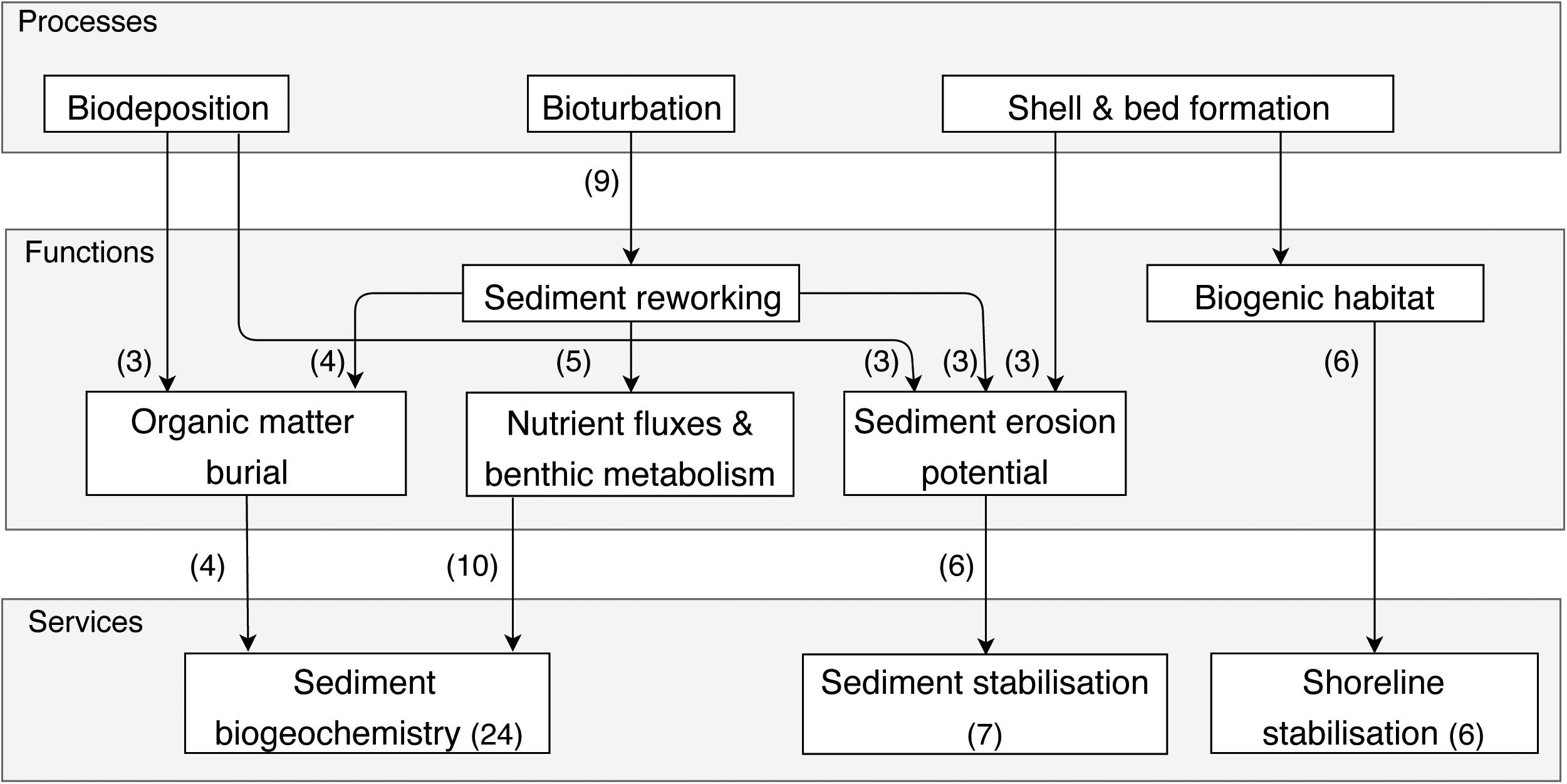
Figure 5. Simplified cascade showing the links between processes, functions, and services in the Habitat modification bundle. The number of papers discussing a service is indicated in brackets within the service box, while the number of times links between processes and functions were discussed is indicated in brackets next to the arrows.
Through sediment reworking, the sediment erosion potential is altered by changes in near-bed flow dynamics, sedimentary properties (e.g., grain size distribution, microbial activity) and bottom roughness (Sousa et al., 2009). The effect shellfish have can be either stabilizing or destabilizing, depending on a number of factors, including densities, size distribution, patch scale, etc. Eriksson et al. (2010) show that the effect on sediment stability can be species dependent, where they found a stabilizing effect at high densities of oysters, mussels and cockles in a pre-disturbed scenario, whereas deposit feeders (lugworms) resulted in sediment destabilization in a human disturbed scenario. Similarly, Harris et al. (2015), found differences between juvenile and adult Macomona liliana (deposit feeding shellfish), where adults had a stabilizing effect on the sediment, compared to juveniles, indicating a shift in species functioning. Through biodeposition and mucus production, sediment erosion potential can also be reduced (Donadi et al., 2013), as discussed in three papers. Six papers look into the effects epifaunal bivalves have on shoreline stabilization by reef formation, thereby creating biogenic habitat (Brumbaugh and Coen, 2009) and form natural breakwaters and reduce wave attenuation. Oysters can be used as living shorelines to improve shoreline protection by reef restoration efforts (Baggett et al., 2015) or through creating artificial breakwaters from oyster shell bags. These breakwaters have been shown to provide similar shoreline stabilizing services, but also create more habitat for other species, compared to other artificial breakwaters (Scyphers et al., 2015).
Biological Structuring
Shellfish provide a number of supporting services by biological structuring in estuarine and coastal habitats (Figure 6). By supporting and altering species and communities, these services contribute to the intrinsic value of the system and make them more resilient to change. Both infaunal and epifaunal shellfish contribute to habitat alterations and have been shown to be habitat providers, as discussed in 22 papers. Most of this service is attributed to epifaunal shellfish that alter and provide habitat through the formation of reefs (15 papers). Reefs can result in the creation of refuge, feeding, or nursing habitats (Guidetti and Boero, 2004; Coen et al., 2007; Volety et al., 2014). Dinesen and Morton (2014) describe the habitat provided by horse mussel reefs, that create three layers of habitat, with the first layer residing on the shell debris, the second layer for mobile megafauna and the third layer for mobile or sedentary macrofauna that live in the crevices. Reefs form refuge areas from predation for other bivalves (Glaspie and Seitz, 2017) or benthic macroinvertebrate species (Micheli and Peterson, 1999) and juvenile fish. An important indirect service resulting from nursery and feeding habitat provided by shellfish is increased secondary production of higher tropic levels, such as fishes and crabs, (Coen et al., 2007; Volety et al., 2014), that if harvested, indirectly result in increased food provision (Kent et al., 2017b). Infaunal shellfish also alter and provide habitat (as discussed in four papers) through creating colonizable substrate from shells (Sousa et al., 2009) and by sediment reworking. An example of the latter is the work by Queiros et al. (2011), showing that the invasive Manila clams can modify the functioning of the invaded system through their effect on sediment reworking by bioturbation. Thereby they can provide variability in habitat characteristics and community composition. These effects can be context dependent, as they can be mediated by structuring vegetation, or sediment granulometry and compaction (Queiros et al., 2011).
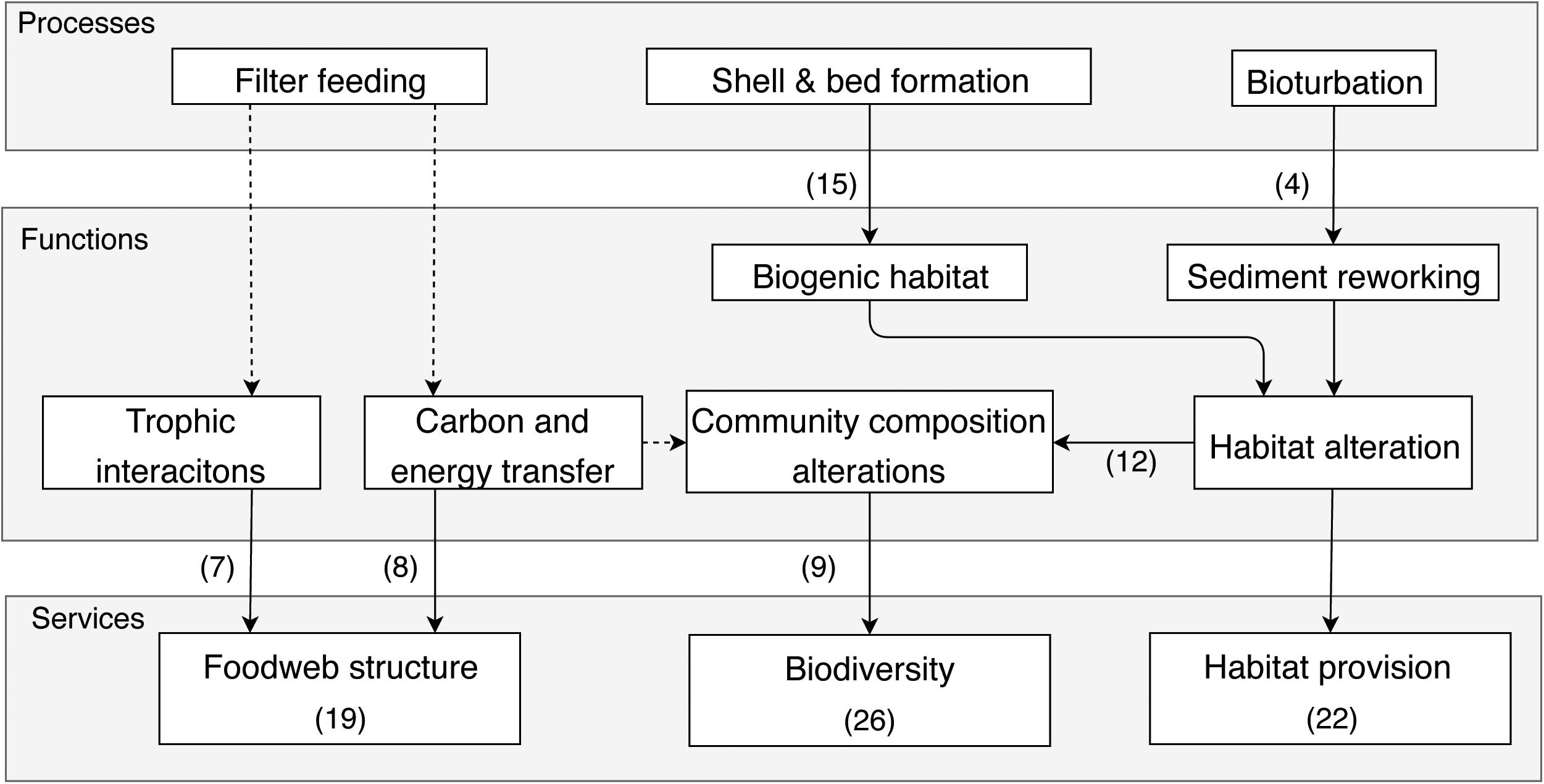
Figure 6. Simplified cascade showing the links between processes, functions, and services in the Biological structuring bundle. The number of papers discussing a service is indicated in brackets within the service box, while the number of times links between processes and functions were discussed is indicated in brackets next to the arrows. Dashed lines indicate links that are inferred from expert knowledge.
As discussed in 11 papers in the review, habitat alterations is crucial for increasing biodiversity (Coen and Luckenbach, 2000; Volety et al., 2014; Kasoar et al., 2015; Gittman et al., 2016; Kent et al., 2017b). Shellfish have been shown to have positive effects on biodiversity in the system, by altering both macrofauna and microbial communities. A number of papers (nine) have shown the link between shellfish and community composition alterations in general, showing changes in community assemblages (Kluger et al., 2016), species richness, abundance and biomass (Quan et al., 2012; van der Zee et al., 2015). The majority of the papers focused on the changes in macrofauna (invertebrate) or meiofauna communities or fish population dynamics (Boldina et al., 2014; Winberg and Davis, 2014; Van Colen et al., 2015). There are some examples of how shellfish affect microbial communities also, for example, Deng et al. (2015) show that the presence of ark shells resulted in higher archaea diversity in intertidal sediments, and Liu et al. (2009) show that clam culturing systems have an effect on both microbial and macrobenthos biomass and diversity. Stressors can have negative effects on community composition and biodiversity, as is shown for anoxia effects (Riedel et al., 2014) and extreme flooding in an eutrophication recovery site (Cardoso et al., 2008).
Foodweb structure is affected by trophic interactions in the system and the transfer of carbon and energy from primary producers to higher trophic levels. Bivalves are primary consumers and form an important link between primary producers (either phytoplankton or microphytobenthos) and higher trophic levels (Vinagre et al., 2015), as they are an important food source for shorebirds (Caldow et al., 2007), fish and rays, thereby transfer carbon and energy up the foodweb. There are two possible pathways, benthic or pelagic trophic interactions, which can be determined using stable isotope data. Christianen et al. (2017) found that microphytobenthos was the main food source for Limecola (Macoma) balthica (a deposit-feeder), while suspension-feeding cockles had a pelagic food source. Shellfish can have significant impacts on the phytoplankton dynamics through their feeding, which can be affected by hydrodynamics, immersion time, and shellfish density (Grangere et al., 2010). Important also is the transfer of energy to benthic communities by organic matter deposition that can fuel microbial communities (Franzo et al., 2016). Non-consumptive interactions can also cause changes in foodweb dynamics, like mortality events (Long et al., 2014; Jones et al., 2016) or predation (Barrios-O’Neill et al., 2017).
Interactions
Even though services are bundled, there are still likely interactions between services across bundles which must be considered in their utilization and management. In the review, 37 papers considered interactions and 17 papers discussed multiple services. Understanding the interactions between services is key, which can manifest as either trade-offs or synergies (Figure 2), and are highly relevant for coastal management.
Trade-Offs
Trade-offs are often found between provisioning and regulating/supporting or cultural services. In our study, this relates to the marine resources bundle, where nine papers looked at multiple services or interactions and an additional 12 papers included either aquaculture or fishing/harvesting as a driver or stressor of other services. The harvesting of biomass for either food or material will result in the loss of other services as shellfish are removed from the system. Furthermore, shellfish harvesting methods can have negative impacts on the delivery of other services. As Toupoint et al. (2016) demonstrated, recreational fishing can reduce primary recruitment intensity and fisheries can negatively affect biodiversity and foodweb structure through the effect on habitat provision. For example, a loss of nursery habitat on rocky reefs can affect fish population dynamics, through the dismantling of rocky substrate by date-mussel fisheries, thereby creating barrens (Guidetti and Boero, 2004), while clam digging can alter nematode and copepods aggregated in spatial structures (Boldina et al., 2014). We trade off the benefits generated for food and the economic value to the delivery of other services in natural systems, so we need to consider what is lost if shellfish disappear or become functionally extinct.
Aquaculture differs from the provision of food, as the active growing of shellfish for food will result in a different mixture blend and differing levels of service provision. Aquaculture is linked to services in the Coastal health and quality bundle (discussed in six papers), as their presence in the system will result in water filtration and removing particles, thereby positively contributing to the delivery of the services in this bundle (Grant et al., 2007). For example, Ruesink et al. (2006) discuss the use of invasive species for aquaculture, thereby generating food that exceeds the yield from historic landings and at the same time contributing to water quality regulation through filtration. Nutrient cycling and removal is affected by aquaculture through excretion (Filgueira et al., 2016) and the cycling and removal of nutrients from the system (Saurel et al., 2014). Shellfish from aquaculture can contribute to the mitigation of eutrophication symptoms by removing excess nitrogen (Sebastiano et al., 2015; Ferreira and Bricker, 2016). However, these services vary depending on the age and biomass structure of the farmed population, and are only provided when the shellfish are present, and disappear when harvested.
Aquaculture is discussed (seven times) in the context of impacts on habitat provision and biodiversity. By creating new habitat, aquaculture settings can have positive impacts on biodiversity, as compared to open mudflats, with more diverse epibenthic organisms (Ruesink et al., 2006), as well as microbial communities (Liu et al., 2009). However, other examples exist describing negative effect of aquaculture on biodiversity (Bendell, 2014), with a drop in primary producers and consumers in a 100 year scenario, resulting in a loss of resilience (Kluger et al., 2017). Another potential downside is that aquaculture may provide habitat or structure for undesirable invasive species or biofouling (Bendell, 2014). Additional trade-offs may arise from indirect services that flow on from supporting services. For example, food provision can be increased indirectly through secondary production of harvested fish and crab species (Kent et al., 2017b). This is often a primary driver for shellfish restoration projects, but design considerations can contribute to trade-offs with other services, particularly in the Habitat modification bundle and water quality outcomes. Habitat provision from complex 3D reefs favor juvenile fish and invertebrate species (Coen et al., 2007) compared to shellfish beds and aggregations that allow for high shellfish densities. However, the exact degree in which habitat provision contributes to secondary production and resulting trade-offs will be dependent on the species present and the shapes or topography of the 3D structure and can vary geographically (Dinesen and Morton, 2014).
Synergies
Synergies exist also, where the delivery of services enhances each other. For example, there is a strong link between sediment biogeochemistry alterations and nutrient cycling and removal (discussed in 17 papers). One of the key aspects related to sediment biogeochemistry is the exchange of nutrients across the sediment-water interface, as caused by sediment reworking. In soft sediment habitats, bioturbation by macrofauna enhances the release of ammonium from the sediment and oxygenates the sediment (Rossi et al., 2008; Lohrer et al., 2010; Wrede et al., 2017). A number of studies have looked at the effect of shellfish on nutrient exchange between the sediment and water column, and included the effect of bivalve densities (Sandwell et al., 2009; Lohrer et al., 2016; Sospedra et al., 2017), or the effect of large adults by removing them (Thrush et al., 2006; Norkko et al., 2013). Furthermore, sediment denitrification, the function of removing bio-available nitrogen, is often strongly coupled with the oxygenation of the sediment. Through sediment biogeochemistry alterations, including organic matter inputs and sediment oxygenation, shellfish can enhance denitrification (Welsh et al., 2015).
Other key interactions are between biogenic habitat generation and a range of services. Biogenic habitat drives services in the Habitat modification bundle, by stabilizing sediments and shorelines, which again result in improved water clarity (as less sediment and particles will be re-suspended) and hence water quality in the Coastal health and quality bundle. Through shoreline protection, shellfish habitats can also contribute to the creation of new habitats, like seagrass meadows. One of the key linkages is between biogenic habitat or habitat alterations and biodiversity, as discussed above. Not only is this link discussed in a number of papers (12 times), but also reef restoration is often discussed as one of the key drivers for service delivery (nine times in the interaction subset). Habitat provision and alterations also interact with food web structure, as microbenthic engineering has been shown to help sustain the smaller components of the food web (Braeckman et al., 2011). Foodweb structure and biodiversity are also linked to sediment biogeochemistry alterations (Michaud et al., 2009; Kanaya, 2014), nutrient cycling and removal by altering the structure of benthic and planktonic communities (Orlova et al., 2006; Compton et al., 2013), and water quality regulation by shellfish clearance rates (Jones et al., 2016).
Discussion
Our review provides a new approach in identifying bundles of ES, focused on the use of key linkages in elucidating the mechanisms underpinning these bundles. As opposed to the majority of work in the bundles literature (Spake et al., 2017; Saidi and Spray, 2018), our work does not identify bundles as a spatial representation of where services are being delivered. Instead, its strength lies in providing a generalization of well understood ecological mechanisms resulting in bundle identification, without requiring any spatial or proxy data that is often lacking in marine environments (Guerry et al., 2012; Townsend et al., 2018). We were able to identify associated services, each underpinned by one or two key processes or functions. This information on mutual drivers will result in a better understanding on how services are generated and provide insight in interdependencies between services (Bennett et al., 2009; Wu and Li, 2019).
However, as these bundles are never independent, and non-linear relationships are possible, interactions among services between bundles should also be considered (Barbier et al., 2008; Lester et al., 2013). As commonly found, provisioning services in the Marine resources bundle tend to generate trade-offs with other services, while synergies are mostly found between regulating and supporting/intermediate services in the other bundles (Lee and Lautenbach, 2016). For terrestrial systems, biomass production generates trade-offs with other ecosystem functions, as they are underpinned by different ecosystem attributes (Wu and Li, 2019). By including the effect of shellfish on biological structuring and habitat modification, the importance of intermediate services was captured for service supply. These services contribute to biodiversity effects and overall functioning and resilience of the system. Biodiversity has both intrinsic and utilitarian values contributing to human well-being (Haines-Young and Potschin, 2010), and overall findings indicate positive, but complex, linkages between biodiversity attributes and ES (Harrison et al., 2014). Biodiversity loss in ocean ecosystems is reducing the oceans capacity to provide ES, like food provision and water quality regulation, as well as reduced recovery from perturbations and a loss in resilience (Worm et al., 2006).
Issues often identified for bundle methodology are related to location or context specific findings using clustering methods and a lack of causal understanding (Spake et al., 2017). This complicates cross-study comparisons, as studies are often conducted at different scales, studying different services and using different proxies or indicators for quantification (Grêt-Regamey et al., 2014; Rodríguez-Loinaz et al., 2015). This scale and context specificity could cause mismatches with management, and hence should be taken into consideration when used in decision making processes, as recommendations may be non-transferable or could affect management outcomes (Raudsepp-Hearne and Peterson, 2016). Our approach provides an insight in the mechanism resulting in service provision and would therefore be more readily transferable. However, it does not quantify the amount of services generated, nor does it include where these services are being consumed or benefitted from. Flow of services from the place of supply to societal demand is important in marine ecosystems due to connectivity and complexity in structure and flow (through wind, currents, and tides) (Townsend et al., 2018).
Many ecological processes and functions are density and/or size dependent, often in a non-linear manner that have implications for service delivery. Shellfish densities can affect the provision of ES, where for example, a reduction in water column turbidity can be directly proportional to shellfish abundance (Newell, 2004) if there is a linear scaling of filtration capacity, whereas a density threshold is predicted for nitrogen removal, as this service is reliant on the exchange across the oxic-anoxic sediment interface. Burrowing behavior and spacing by benthic macrofauna alters the amount of oxygen in the sediment. When a critical organism density is reached, surface area of the oxic-anoxic boundary is reduced across which exchange processes occur that are necessary for coupled nitrification-denitrification in sediments. Therefore, denitrification potential is highest at moderate densities, with a collapse at extreme densities (Gilbert et al., 2003; Newell, 2004). Similarly, Lohrer et al. (2016) show the complex direct and indirect effects of shellfish density on primary productivity and nutrient cycling in seagrass beds, with highest effect of cockles on primary production at intermediate densities. Shellfish size can also affect service delivery, where for example, the removal of large adults has a negative impact on nutrient and oxygen fluxes and hence affect alterations in sediment biogeochemistry (Thrush et al., 2006; Norkko et al., 2013). Koch et al. (2009) show the effect of non-linearity in wave attenuation by benthic habitats on shoreline stabilization, and the resulting changes in valuation of this service and the impact on coastal management if non-linearity is taken into consideration. Unexpected non-linearity in service delivery as a result of density dependency and dynamic ecosystem processes or functions, can therefore have a significant impact on the assessment of service supply and resulting decision making.
Predicting the delivery of ES is a key challenge in resource management which may benefit more from a mechanistic understanding of the supply of services rather than a collection of differing, context specific, and non-transferable case studies (Spake et al., 2017). Understanding which processes drive the generation of these service bundles and how they link with environmental variables will help us better predict and quantify service delivery at different spatial and temporal scales. In many cases, models and maps are used as tools to predict ecosystem service delivery to inform management and decision making (Tallis and Polasky, 2009; Burkhard et al., 2012; Maes et al., 2012a; Burkhard and Maes, 2017), by providing useful insights in where services are likely delivered.
The next step in applying our framework is to move from identification to ecological quantification of linkages and bundles, including shellfish density and size effects, and to consider the implications of interactions for management. When managing for individual services in isolation, interactions can be overlooked and tend to down-weight human perceptions of the total benefits provided (Rodríguez et al., 2006). Ecosystem models are based on ecological principles and processes and could be configured to derive information on interactions between services that would represent a step forward in predicting multiple services delivery. For example environmental carrying-capacity models predict shellfish growth and clearance rates (e.g., Dame and Prins, 1997) that could be used to quantify services in the water quality bundle. In some instances coupled models may be required, for example carrying-capacity and morphodynamical models (e.g., van Maanen et al., 2015) could be used to predict how shellfish distribution affects sediment and shoreline stability. Such advances would lead to a better understanding of interactions between multiple services and support a more holistic, EBM approach to marine systems (McLeod and Leslie, 2009).
Conclusion
The ES and bundles concept have been proposed as a step forward in EBM. Ecological understanding on how bundles are generated will contribute to sustainable management, as it will create a better understanding of what drives the supply of services, how they interact with each other, what stressors they are prone to, and what might be lost if not managed properly. As a result of global shellfish bed degradation, recovery of lost ES through shellfish bed restoration effort and methods form a large part of the ES literature on shellfish. Our case study is an example of how we should manage for more than the tangible services that have direct economic gain, as this bundle will often form trade-offs with other services that are generated through different processes. Shellfish, like many other marine organisms and habitats, are prone to multiple and cumulative stressors, and global losses are linked to a loss in service value, reduced habitat quality and affects the functioning of the system. As estuarine and coastal systems are prone to change, maintaining resilience through healthy systems should be a main concern for management. A holistic approach to managing shellfish beds and estuaries in general is in line with EBM and will help maintain resilience and ensure future use of the services they generate. More generally, our approach provides a format to translate ecological knowledge to advice decision makers and spatial planners, without getting caught up in case or location specific details, and will help connect ecological knowledge with social science and decision making.
Data Availability
All datasets generated for this study are included in the manuscript and/or the Supplementary Files.
Author Contributions
VR, AL, MT, and CP conceived the ideas presented in the manuscript. VR conducted the literature review and wrote the manuscript with input from AL, MT, and CP.
Funding
This work was supported by the Ministry of Business, Innovation and Employment (MBIE; Contract No. C01X1515) as part of the Sustainable Seas National Science Challenge, Valuable Seas program, Project 2.1.3 “Measuring Ecosystem Services and Assessing Impacts” (SUSS16202).
Conflict of Interest Statement
The authors declare that the research was conducted in the absence of any commercial or financial relationships that could be construed as a potential conflict of interest.
Supplementary Material
The Supplementary Material for this article can be found online at: https://www.frontiersin.org/articles/10.3389/fmars.2019.00409/full#supplementary-material
References
Baggett, L. P., Powers, S. P., Brumbaugh, R. D., Coen, L. D., DeAngelis, B. M., Greene, J. K. B., et al. (2015). Guidelines for evaluating performance of oyster habitat restoration. Restor. Ecol. 23, 737–745. doi: 10.1111/rec.12262
Barbier, E. B., Hacker, S. D., Kennedy, C., Koch, E. W., Stier, A. C., Silliman, B. R., et al. (2011). The value of estuarine and coastal ecosystem services. Ecol. Monogr. 81, 169–193.
Barbier, E. B., Koch, E. W., Silliman, B. R., Hacker, S. D., Wolanski, E., Primavera, J., et al. (2008). Coastal ecosystem-based management with nonlinear ecological functions and values. Science 319, 321–323. doi: 10.1126/science.1150349
Barrios-O’Neill, D., Bertolini, C., and Collins, P. C. (2017). Trophic cascades and the transient keystone concept. Biol. Conserv. 212, 191–195. doi: 10.1016/j.biocon.2017.06.011
Beck, M. W., Brumbaugh, R. D., Airoldi, L., Carranza, A., Coen, L. D., Crawford, C., et al. (2011). Oyster reefs at risk and recommendations for conservation, restoration, and management. BioScience 61, 107–116. doi: 10.1525/bio.2011.61.2.5
Bendell, L. I. (2014). Community composition of the intertidal in relation to the shellfish aquaculture industry in coastal British Columbia. Canada. Aquaculture 433, 384–394. doi: 10.1016/j.aquaculture.2014.06.027
Bennett, E. M., Peterson, G. D., and Gordon, L. J. (2009). Understanding relationships among multiple ecosystem services. Ecol. Lett. 12, 1394–1404. doi: 10.1111/j.1461-0248.2009.01387.x
Bergstrom, P., Lindegarth, S., and Lindegarth, M. (2015). Modeling and predicting the growth of the mussel, Mytilus edulis: implications for planning of aquaculture and eutrophication mitigation. Ecol. Evol. 5, S920–S933. doi: 10.1002/ece3.1823
Boldina, I., Beninger, P. G., and Le Coz, M. (2014). Effect of long-term mechanical perturbation on intertidal soft-bottom meiofaunal community spatial structure. J. Sea Res. 85, 85–91. doi: 10.1016/j.seares.2013.10.006
Bouma, T. J., Olenin, S., Reise, K., and Ysebaert, T. (2009). Ecosystem engineering and biodiversity in coastal sediments: posing hypotheses. Helgol. Mar. Res. 63, 95–106. doi: 10.1007/s10152-009-0146-y
Braeckman, U., Van Colen, C., Soetaert, K., Vincx, M., and Vanaverbeke, J. (2011). Contrasting macrobenthic activities differentially affect nematode density and diversity in a shallow subtidal marine sediment. Mar. Ecol. Prog. Ser. 422, 179–191. doi: 10.3354/meps08910
Broszeit, S., Hattam, C., and Beaumont, N. (2016). Bioremediation of waste under ocean acidification: reviewing the role of Mytilus edulis. Mar. Pollut. Bull. 103, 5–14. doi: 10.1016/j.marpolbul.2015.12.040
Brumbaugh, R. D., and Coen, L. D. (2009). Contemporary approaches for small-scale oyster reef restoration to address substrate versus recruitment limitation: a review and comments relevant for the olympia oyster, ostrea lurida carpenter 1864. J. Shellfish Res. 28, 147–161. doi: 10.2983/035.028.0105
Bullock, J. M., Aronson, J., Newton, A. C., Pywell, R. F., and Rey-Benayas, J. M. (2011). Restoration of ecosystem services and biodiversity: conflicts and opportunities. Trends Ecol. Evol. 26, 541–549. doi: 10.1016/j.tree.2011.06.011
Burge, C. A., Closek, C. J., Friedman, C. S., Groner, M. L., Jenkins, C. M., Shore-Maggiok, A., et al. (2016). The use of filter-feeders to manage disease in a changing world. Integr. Comp. Biol. 56, 573–587. doi: 10.1093/icb/icw048
Burkhard, B., Kroll, F., Nedkov, S., and Müller, F. (2012). Mapping ecosystem service supply, demand and budgets. Ecol. Indic. 21, 17–29. doi: 10.1016/j.ecolind.2011.06.019
Caldow, R. W. G., Stillman, R. A., Durell, S., West, A. D., McGrorty, S., Goss-Custard, J. D., et al. (2007). Benefits to shorebirds from invasion of a non-native shellfish. Proc. R. Soc. BBiol. Sci. 274, 1449–1455. doi: 10.1098/rspb.2007.0072
Cardoso, P. G., Raffaelli, D., Lillebo, A. I., Verdelhos, T., and Pardal, M. A. (2008). The impact of extreme flooding events and anthropogenic stressors on the macrobenthic communities’ dynamics. Estuar. Coast. Shelf Sci. 76, 553–565. doi: 10.1016/j.ecss.2007.07.026
Cerco, C. F. (2015). A multi-module approach to calculation of oyster (Crassostrea virginica) environmental benefits. Environ. Manage. 56, 467–479. doi: 10.1007/s00267-015-0511-3
Cerco, C. F., and Noel, M. R. (2007). Can oyster restoration reverse cultural eutrophication in Chesapeake Bay? Estuar. Coasts 30, 331–343. doi: 10.1007/bf02700175
Chapman, P. M., Wang, F. Y., and Caeiro, S. S. (2013). Assessing and managing sediment contamination in transitional waters. Environ. Int. 55, 71–91. doi: 10.1016/j.envint.2013.02.009
Christianen, M. J. A., Middelburg, J. J., Holthuijsen, S. J., Jouta, J., Compton, T. J., van der Heide, T., et al. (2017). Benthic primary producers are key to sustain the Wadden Sea food web: stable carbon isotope analysis at landscape scale. Ecology 98, 1498–1512. doi: 10.1002/ecy.1837
Clare, D. S., Spencer, M., Robinson, L. A., and Frid, C. L. J. (2016). Species densities, biological interactions and benthic ecosystem functioning: an in situ experiment. Mar. Ecol. Prog. Ser. 547, 149–161. doi: 10.3354/meps11650
Coen, L. D., Brumbaugh, R. D., Bushek, D., Grizzle, R., Luckenbach, M. W., Posey, M. H., et al. (2007). Ecosystem services related to oyster restoration. Mar. Ecol. Prog. Ser. 341, 303–307. doi: 10.3354/meps341303
Coen, L. D., and Luckenbach, M. W. (2000). Developing success criteria and goals for evaluating oyster reef restoration: ecological function or resource exploitation? Ecol. Eng. 15, 323–343. doi: 10.1016/s0925-8574(00)00084-7
Compton, T. J., Holthuijsen, S., Koolhaas, A., Dekinga, A., ten Horn, J., Smith, J., et al. (2013). Distinctly variable mudscapes: distribution gradients of intertidal macrofauna across the Dutch Wadden Sea. J. Sea Res. 82, 103–116. doi: 10.1016/j.seares.2013.02.002
Culhane, F. E., Frid, C. L., Royo Gelabert, E., White, L., and Robinson, L. A. (2018). Linking marine ecosystems with the services they supply: what are the relevant service providing units? Ecol. Appl. 28, 1740–1751. doi: 10.1002/eap.1779
Daily, G. (1997). Nature’s Services: Societal Dependence on Natural Ecosystems. Washington, DC: Island Press.
Dame, R. F., and Prins, T. C. (1997). Bivalve carrying capacity in coastal ecosystems. Aquat. Ecol. 31, 409–421.
de Bello, F., Lavorel, S., Díaz, S., Harrington, R., Cornelissen, J. H., Bardgett, R. D., et al. (2010). Towards an assessment of multiple ecosystem processes and services via functional traits. Biodivers. Conserv. 19, 2873–2893.
de Groot, R., Fisher, B., Christie, M., Aronson, J., Braat, L., Haines-Young, R., et al. (2010). “Integrating the ecological and economic dimensions in biodiversity and ecosystem service valuation,” in The Economics of Ecosystems and Biodiversity (TEEB): Ecological and Economic Foundations, ed. P. Kumar (London: Routledge).
Deng, F., Ma, Y., Li, J., Wang, Y. Z., Yan, Q. P., Yan, X. Z., et al. (2015). Archaeal community structure and response to ark shell bioturbation in typical intertidal mudflats, Southeast coast of China. Cont. Shelf Res. 106, 97–106. doi: 10.1016/j.csr.2015.07.004
Dickinson, D. C., and Hobbs, R. J. (2017). Cultural ecosystem services: characteristics, challenges and lessons for urban green space research. Ecosyst. Serv. 25, 179–194. doi: 10.1016/j.ecoser.2017.04.014
Dinesen, G. E., and Morton, B. (2014). Review of the functional morphology, biology and perturbation impacts on the boreal, habitat-forming horse mussel Modiolus modiolus (Bivalvia: Mytilidae: Modiolinae). Mar. Biol. Res. 10, 845–870. doi: 10.1080/17451000.2013.866250
Donadi, S., Westra, J., Weerman, E. J., van der Heide, T., van der Zee, E. M., van de Koppel, J., et al. (2013). Non-trophic interactions control benthic producers on intertidal flats. Ecosystems 16, 1325–1335. doi: 10.1007/s10021-013-9686-8
Eriksson, B. K., van der Heide, T., van de Koppel, J., Piersma, T., van der Veer, H. W., and Olff, H. (2010). Major changes in the ecology of the Wadden Sea: human impacts, ecosystem engineering and sediment dynamics. Ecosystems 13, 752–764. doi: 10.1007/s10021-010-9352-3
Ferreira, J. G., and Bricker, S. B. (2016). Goods and services of extensive aquaculture: shellfish culture and nutrient trading. Aquacult. Int. 24, 803–825. doi: 10.1007/s10499-015-9949-9
Filgueira, R., Guyondet, T., Comeau, L. A., and Tremblay, R. (2016). Bivalve aquaculture-environment interactions in the context of climate change. Glob. Change Biol. 22, 3901–3913. doi: 10.1111/gcb.13346
Fisher, B., Turner, R. K., and Morling, P. (2009). Defining and classifying ecosystem services for decision making. Ecol. Econ. 68, 643–653. doi: 10.1016/j.ecolecon.2008.09.014
Franzo, A., Auriemma, R., Nasi, F., Vojvoda, J., Pallavicini, A., Cibic, T., et al. (2016). Benthic ecosystem functioning in the severely contaminated Mar Piccolo of Taranto (Ionian Sea, Italy): focus on heterotrophic pathways. Environ. Sci. Pollut. Res. 23, 12645–12661. doi: 10.1007/s11356-015-5339-0
Galimany, E., Freeman, C. J., Lunt, J., Domingos, A., Sacks, P., Walters, L., et al. (2017a). Feeding competition between the native oyster Crassostrea virginica and the invasive mussel Mytella charruana. Mar. Ecol. Prog. Ser. 564, 57–66. doi: 10.3354/meps11976
Galimany, E., Lunt, J., Freeman, C. J., Reed, S., Segura-Garcia, I., and Paul, V. J. (2017b). Feeding behavior of eastern oysters Crassostrea virginica and hard clams Mercenaria mercenaria in shallow estuaries. Mar. Ecol. Prog. Ser. 567, 125–137. doi: 10.3354/meps12050
Galimany, E., Wikfors, G. H., Dixon, M. S., Newell, C. R., Meseck, S. L., Henning, D., et al. (2017c). Cultivation of the Ribbed Mussel (Geukensia demissa) for nutrient bioextraction in an Urban Estuary. Environ. Sci. Technol. 51, 13311–13318. doi: 10.1021/acs.est.7b02838
Gamfeldt, L., Lefcheck, J. S., Byrnes, J. E., Cardinale, B. J., Duffy, J. E., and Griffin, J. N. J. O. (2015). Marine biodiversity and ecosystem functioning: what’s known and what’s next? Oikos 124, 252–265. doi: 10.1111/oik.01549
Gilbert, F., Aller, R. C., and Hulth, S. (2003). The influence of macrofaunal burrow spacing and diffusive scaling on sedimentary nitrification and denitrification: an experimental simulation and model approach. J. Mar. Res. 61, 101–125. doi: 10.1357/002224003321586426
Gittman, R. K., Peterson, C. H., Currin, C. A., Fodrie, F. J., Piehler, M. F., Bruno, J. F., et al. (2016). Living shorelines can enhance the nursery role of threatened estuarine habitats. Ecol. Appl. 26, 249–263. doi: 10.1890/14-0716
Glaspie, C. N., and Seitz, R. D. (2017). Role of habitat and predators in maintaining functional diversity of estuarine bivalves. Mar. Ecol. Prog. Ser. 570, 113–125. doi: 10.3354/meps12103
Grabowski, J. H., Brumbaugh, R. D., Conrad, R. F., Keeler, A. G., Opaluch, J. J., Peterson, C. H., et al. (2012). Economic valuation of ecosystem services provided by oyster reefs. BioScience 62, 900–909. doi: 10.1525/bio.2012.62.10.10
Grabowski, J. H., and Peterson, C. H. (2007). Restoring oyster reefs to recover ecosystem services. Theor. Ecol. Ser. 4, 281–298. doi: 10.1016/s1875-306x(07)80017-7
Granek, E. F., Polasky, S., Kappel, C. V., Reed, D. J., Stoms, D. M., Koch, E. W., et al. (2010). Ecosystem services as a common language for coastal ecosystem-based management. Conserv. Biol. 24, 207–216. doi: 10.1111/j.1523-1739.2009.01355.x
Grangere, K., Lefebvre, S., Bacher, C., Cugier, P., and Menesguen, A. (2010). Modelling the spatial heterogeneity of ecological processes in an intertidal estuarine bay: dynamic interactions between bivalves and phytoplankton. Mar. Ecol. Prog. Ser. 415, 141–158. doi: 10.3354/meps08659
Grant, J., Bugden, G., Horne, E., Archambault, M. C., and Carreau, M. (2007). Remote sensing of particle depletion by coastal suspension-feeders. Can. J. Fish. Aquat. Sci. 64, 387–390. doi: 10.1139/f07-021
Grêt-Regamey, A., Weibel, B., Bagstad, K. J., Ferrari, M., Geneletti, D., Klug, H., et al. (2014). On the effects of scale for ecosystem services mapping. PLoS One 9:e112601. doi: 10.1371/journal.pone.0112601
Grizzle, R. E., Greene, J. K., and Coen, L. D. (2008). Seston removal by natural and constructed Intertidal Eastern Oyster (Crassostrea virginica) reefs: a comparison with previous laboratory studies, and the value of in situ methods. Estuar. Coasts 31, 1208–1220. doi: 10.1007/s12237-008-9098-8
Guerry, A. D., Ruckelshaus, M. H., Arkema, K. K., Bernhardt, J. R., Guannel, G., Kim, C.-K., et al. (2012). Modeling benefits from nature: using ecosystem services to inform coastal and marine spatial planning. Int. J. Biodiver. Sci. Ecosyst. Serv. Manag. 8, 107–121.
Guidetti, P., and Boero, F. (2004). Desertification of Mediterranean rocky reefs caused by date-mussel, Lithophaga lithophaga (Mollusca : Bivalvia), fishery: effects on adult and juvenile abundance of a temperate fish. Mar. Pollut. Bull. 48, 978–982. doi: 10.1016/j.marpolbul.2003.12.006
Gutiérrez, J. L., Jones, C. G., Strayer, D. L., and Iribarne, O. O. (2003). Mollusks as ecosystem engineers: the role of shell production in aquatic habitats. Oikos 101, 79–90. doi: 10.1034/j.1600-0706.2003.12322.x
Haines-Young, R., and Potschin, M. (2010). “The links between biodiversity, ecosystem services and human well-being,” in Ecosystem Ecology: A New Synthesis, eds C. L. J. Raffaelli and D. G. Frid (Cambridge: Cambridge University Press), 110–139. doi: 10.1017/cbo9780511750458.007
Harris, R. J., Pilditch, C. A., Hewitt, J. E., Lohrer, A. M., Van Colen, C., Townsend, M., et al. (2015). Biotic interactions influence sediment erodibility on wave-exposed sandflats. Mar. Ecol. Prog. Ser. 523, 15–30. doi: 10.3354/meps11164
Harrison, P., Berry, P., Simpson, G., Haslett, J., Blicharska, M., Bucur, M., et al. (2014). Linkages between biodiversity attributes and ecosystem services: a systematic review. Ecosyst. Serv. 9, 191–203. doi: 10.1016/j.ecoser.2014.05.006
Hiebenthal, C., Philipp, E. E. R., Eisenhauer, A., and Wahl, M. (2013). Effects of seawater pCO(2) and temperature on shell growth, shell stability, condition and cellular stress of Western Baltic Sea Mytilus edulis (L.) and Arctica islandica (L.). Mar. Biol. 160, 2073–2087. doi: 10.1007/s00227-012-2080-9
Hohaia, A., Vopel, K., and Pilditch, C. A. (2014). Thin terrestrial sediment deposits on intertidal sandflats: effects on pore-water solutes and juvenile bivalve burial behaviour. Biogeosciences 11, 2225–2235. doi: 10.5194/bg-11-2225-2014
Howe, C., Suich, H., Vira, B., and Mace, G. M. (2014). Creating win-wins from trade-offs? Ecosystem services for human well-being: a meta-analysis of ecosystem service trade-offs and synergies in the real world. Glob. Environ. Change 28, 263–275. doi: 10.1016/j.gloenvcha.2014.07.005
Humphreys, J., Harris, M. R. C., Herbert, R. J. H., Farrell, P., Jensen, A., Cragg, S. M., et al. (2015). Introduction, dispersal and naturalization of the Manila clam Ruditapes philippinarum in British estuaries, 1980-2010. J. Mar. Biol. Assoc. U. K. 95, 1163–1172. doi: 10.1017/s0025315415000132
Jones, C. G., Lawton, J. H., and Shachak, M. (1994). “Organisms as ecosystem engineers,” in Ecosystem Management, eds F. B. Samson and F. L. Knopf (New York, NY: Springer), 130–147. doi: 10.1007/978-1-4612-4018-1_14
Jones, H. F., Pilditch, C. A., Bruesewitz, D. A., and Lohrer, A. M. (2011). Sedimentary environment influences the effect of an infaunal suspension feeding bivalve on estuarine ecosystem function. PLoS One 6:e27065. doi: 10.1371/journal.pone.0027065
Jones, H. F., Pilditch, C. A., Hamilton, D. P., and Bryan, K. R. (2016). Impacts of a bivalve mass mortality event on an estuarine food web and bivalve grazing pressure. N. Z. J. Mar. Freshw. Res. 51, 370–392. doi: 10.1080/00288330.2016.1245200
Kanaya, G. (2014). Effects of infaunal bivalves on associated macrozoobenthic communities in estuarine soft-bottom habitats: a bivalve addition experiment in a brackish lagoon. J. Exp. Mar. Biol. Ecol. 457, 180–189. doi: 10.1016/j.jembe.2014.03.022
Karlson, A. M. L., Nascimento, F. J. A., Naslund, J., and Elmgren, R. (2010). Higher diversity of deposit-feeding macrofauna enhances phytodetritus processing. Ecology 91, 1414–1423. doi: 10.1890/09-0660.1
Kasoar, T., Ermgassen, P., Carranza, A., Hancock, B., and Spalding, M. (2015). New opportunities for conservation of a threatened biogenic habitat: a worldwide assessment of knowledge on bivalve-reef representation in marine and coastal Ramsar Sites. Mar. Freshw. Res. 66, 981–988.
Kellogg, M. L., Cornwell, J. C., Owens, M. S., and Paynter, K. T. (2013). Denitrification and nutrient assimilation on a restored oyster reef. Mar. Ecol. Prog. Ser. 480, 1–19. doi: 10.1371/journal.pone.0209799
Kent, F. E. A., Last, K. S., Harries, D. B., and Sanderson, W. G. (2017a). In situ biodeposition measurements on a Modiolus modiolus (horse mussel) reef provide insights into ecosystem services. Estuar. Coast. Shelf Sci. 184, 151–157. doi: 10.1016/j.ecss.2016.11.014
Kent, F. E. A., Mair, J. M., Newton, J., Lindenbaum, C., Porter, J. S., Sanderson, W. G., et al. (2017b). Commercially important species associated with horse mussel (Modiolus modiolus) biogenic reefs: a priority habitat for nature conservation and fisheries benefits. Mar. Pollut. Bull. 118, 71–78. doi: 10.1016/j.marpolbul.2017.02.051
Kluger, L. C., Filgueira, R., and Wolff, M. (2017). Integrating the concept of resilience into an ecosystem approach to bivalve aquaculture management. Ecosystems 20, 1364–1382. doi: 10.1007/s10021-017-0118-z
Kluger, L. C., Taylor, M. H., Rivera, E. B., Silva, E. T., and Wolff, M. (2016). Assessing the ecosystem impact of scallop bottom culture through a community analysis and trophic modelling approach. Mar. Ecol. Prog. Ser. 547, 121–135. doi: 10.3354/meps11652
Koch, E. W., Barbier, E. B., Silliman, B. R., Reed, D. J., Perillo, G. M., Hacker, S. D., et al. (2009). Non-linearity in ecosystem services: temporal and spatial variability in coastal protection. Front. Ecol. Environ. 7:29–37. doi: 10.1890/080126
Kremen, C., and Ostfeld, R. S. (2005). A call to ecologists: measuring, analyzing, and managing ecosystem services. Front. Ecol. Environ. 3:540–548. doi: 10.2307/3868610
Lacoue-Labarthe, T., Nunes, P., Ziveri, P., Cinar, M., Gazeau, F., Hall-Spencer, J. M., et al. (2016). Impacts of ocean acidification in a warming Mediterranean Sea: an overview. Reg. Stud. Mar. Sci. 5, 1–11. doi: 10.1016/j.rsma.2015.12.005
Lamarque, P., Lavorel, S., Mouchet, M., and Quétier, F. (2014). Plant trait-based models identify direct and indirect effects of climate change on bundles of grassland ecosystem services. Proc. Natl. Acad. Sci. U.S.A. 111, 13751–13756. doi: 10.1073/pnas.1216051111
Lavorel, S., and Grigulis, K. (2012). How fundamental plant functional trait relationships scale-up to trade-offs and synergies in ecosystem services. J. Ecol. 100, 128–140. doi: 10.1111/j.1365-2745.2011.01914.x
Lavorel, S., Grigulis, K., Lamarque, P., Colace, M. P., Garden, D., Girel, J., et al. (2011). Using plant functional traits to understand the landscape distribution of multiple ecosystem services. J. Ecol. 99, 135–147. doi: 10.1111/j.1365-2745.2010.01753.x
Lee, H., and Lautenbach, S. (2016). A quantitative review of relationships between ecosystem services. Ecol. Indic. 66, 340–351. doi: 10.1016/j.ecolind.2016.02.004
Lester, S. E., Costello, C., Halpern, B. S., Gaines, S. D., White, C., Barth, J. A., et al. (2013). Evaluating tradeoffs among ecosystem services to inform marine spatial planning. Mar. Policy 38, 80–89. doi: 10.1016/j.marpol.2012.05.022
Lewis, N. S., and DeWitt, T. H. (2017). Effect of green macroalgal blooms on the behavior, growth, and survival of cockles Clinocardium nuttallii in Pacific NW estuaries. Mar. Ecol. Prog. Ser. 582, 105–120. doi: 10.3354/meps12328
Li, Y. Q., Meseck, S. L., Dixon, M. S., Rivara, K., and Wikfors, G. H. (2012). Temporal variability in phytoplankton removal by a commercial, suspended eastern oyster nursery and effects on local plankton dynamics. J. Shellfish Res. 31, 1077–1089. doi: 10.2983/035.031.0419
Liu, J., Zhou, H. P., Qin, P., Zhou, J., and Wang, G. X. (2009). Comparisons of ecosystem services among three conversion systems in Yancheng National Nature Reserve. Ecol. Eng. 35, 609–629. doi: 10.1016/j.ecoleng.2008.09.007
Lohrer, A. M., Halliday, N. J., Thrush, S. F., Hewitt, J. E., and Rodil, I. F. (2010). Ecosystem functioning in a disturbance-recovery context: contribution of macrofauna to primary production and nutrient release on intertidal sandflats. J. Exp. Mar. Biol. Ecol. 390, 6–13. doi: 10.1016/j.jembe.2010.04.035
Lohrer, A. M., Townsend, M., Hailes, S. F., Rodil, I. F., Cartner, K., Pratt, D. R., et al. (2016). Influence of New Zealand cockles (Austrovenus stutchburyi) on primary productivity in sandflat-seagrass (Zostera muelleri) ecotones. Estuar. Coast. Shelf Sci. 181, 238–248. doi: 10.1016/j.ecss.2016.08.045
Lohrer, A. M., Townsend, M., Rodil, I. F., Hewitt, J. E., and Thrush, S. F. (2012). Detecting shifts in ecosystem functioning: the decoupling of fundamental relationships with increased pollutant stress on sandflats. Mar. Pollut. Bull. 64, 2761–2769. doi: 10.1016/j.marpolbul.2012.09.012
Long, W. C., Seitz, R. D., Brylawski, B. J., and Lipcius, R. N. (2014). Individual, population, and ecosystem effects of hypoxia on a dominant benthic bivalve in Chesapeake Bay. Ecol. Monogr. 84, 303–327. doi: 10.1890/13-0440.1
MacDonald, B. A., and Ward, J. E. (2009). Feeding activity of scallops and mussels measured simultaneously in the field: repeated measures sampling and implications for modelling. J. Exp. Mar. Biol. Ecol. 371, 42–50. doi: 10.1016/j.jembe.2009.01.002
Maes, J., Egoh, B., Willemen, L., Liquete, C., Vihervaara, P., Schägner, J. P., et al. (2012a). Mapping ecosystem services for policy support and decision making in the European Union. J. Ecosyst. Serv. 1, 31–39. doi: 10.1016/j.ecoser.2012.06.004
Maes, J., Paracchini, M., Zulian, G., Dunbar, M., and Alkemade, R. (2012b). Synergies and trade-offs between ecosystem service supply, biodiversity, and habitat conservation status in Europe. Biol. Conserv. 155, 1–12. doi: 10.1016/j.biocon.2012.06.016
Maire, O., Duchene, J. C., Rosenberg, R., de Mendonca, J. B., and Gremare, A. (2006). Effects of food availability on sediment reworking in Abra ovata and A. nitida. Mar. Ecol. Prog. Ser. 319, 135–153. doi: 10.3354/meps319135
Maire, O., Merchant, J. N., Bulling, M., Teal, L. R., Grémare, A., Duchêne, J. C., et al. (2010). Indirect effects of non-lethal predation on bivalve activity and sediment reworking. J. Exp. Mar. Biol. Ecol. 395, 30–36. doi: 10.1016/j.jembe.2010.08.004
Marsden, I. D., and Adkins, S. C. (2010). Current status of cockle bed restoration in New Zealand. Aquacult. Int. 18, 83–97. doi: 10.1007/s10499-009-9270-6
Marshall, K. N., Kaplan, I. C., Hodgson, E. E., Hermann, A., Busch, D. S., McElhany, P., et al. (2017). Risks of ocean acidification in the California Current food web and fisheries: ecosystem model projections. Glob. Change Biol. 23, 1525–1539. doi: 10.1111/gcb.13594
Martínez-Harms, M. J., and Balvanera, P. (2012). Methods for mapping ecosystem service supply: a review. Int. J. Biodiver. Sci. Ecosyst. Serv. Manag. 8, 17–25. doi: 10.1073/pnas.0804960105
McCartain, L. D., Townsend, M., Thrush, S. F., Wethey, D. S., Woodin, S. A., Volkenborn, N., et al. (2017). The effects of thin mud deposits on the behaviour of a deposit-feeding tellinid bivalve: implications for ecosystem functioning. Mar. Freshw. Behav. Physiol. 50, 239–255. doi: 10.1080/10236244.2017.1364123
McLeod, K., and Leslie, H. (2009). Ecosystem-Based Management for the Oceans. Washington, DC: Island Press.
Michaud, E., Desrosiers, G., Aller, R. C., Mermillod-Blondin, F., Sundby, B., and Stora, G. (2009). Spatial interactions in the Macoma balthica community control biogeochemical fluxes at the sediment-water interface and microbial abundances. J. Mar. Res. 67, 43–70. doi: 10.1357/002224009788597926
Micheli, F., and Peterson, C. H. (1999). Estuarine vegetated habitats as corridors for predator movements. Conserv. Biol. 13, 869–881. doi: 10.1046/j.1523-1739.1999.98233.x
Milbrandt, E. C., Thompson, M., Coen, L. D., Grizzle, R. E., and Ward, K. (2015). A multiple habitat restoration strategy in a semi-enclosed Florida embayment, combining hydrologic restoration, mangrove propagule plantings and oyster substrate additions. Ecol. Eng. 83, 394–404. doi: 10.1016/j.ecoleng.2015.06.043
Millennium Ecosystem Assessment [MEA] (2005). Ecosystems and Human Well-Being: Synthesis. Washington, DC: Island Press.
Montes, M. O., Hanna, S. K., Lenihan, H. S., and Keller, A. A. (2012). Uptake, accumulation, and biotransformation of metal oxide nanoparticles by a marine suspension-feeder. J. Hazard. Mater. 225, 139–145. doi: 10.1016/j.jhazmat.2012.05.009
Müller, F., and Burkhard, B. (2007). “An ecosystem based framework to link landscape structures, functions and services,” in Multifunctional Land Use, eds Ü Mander, H. Wiggering, and K. Helming (Berlin: Springer), 37–63. doi: 10.1007/978-3-540-36763-5_3
Nagendra, H., Reyers, B., and Lavorel, S. (2013). Impacts of land change on biodiversity: making the link to ecosystem services. Curr. Opin. Environ. Sustain. 5, 503–508. doi: 10.1016/j.cosust.2013.05.010
Newell, R. I. (2004). Ecosystem influences of natural and cultivated populations of suspension-feeding bivalve molluscs: a review. J. Shellfish Res. 23, 51–62.
Norkko, A., Villnäs, A., Norkko, J., Valanko, S., and Pilditch, C. (2013). Size matters: implications of the loss of large individuals for ecosystem function. Sci. Rep. 3:2646. doi: 10.1038/srep02646
Orlova, M. I., Telesh, I. V., Berezina, N. A., Antsulevich, A. E., Maximov, A. A., Litvinchuk, L. F., et al. (2006). Effects of nonindigenous species on diversity and community functioning in the eastern Gulf of Finland (Baltic Sea). Helgol. Mar. Res. 60, 98–105. doi: 10.1007/s10152-006-0026-7
Pratt, D. R., Lohrer, A. M., Pilditch, C. A., and Thrush, S. F. (2014). Changes in ecosystem function across sedimentary gradients in estuaries. Ecosystems 17, 182–194. doi: 10.1007/s10021-013-9716-6
Premo, K. M., and Tyler, A. C. (2013). Threat of predation alters the ability of benthic invertebrates to modify sediment biogeochemistry and benthic microalgal abundance. Mar. Ecol. Prog. Ser. 494, 29–39. doi: 10.3354/meps10561
Quan, W. M., Humphries, A. T., Shen, X. Q., and Chen, Y. Q. (2012). Oyster and associated benthic macrofaunal development on a created intertidal oyster (crassostrea ariakensis) reef in the yangtze river estuary, China. J. Shellfish Res. 31, 599–610. doi: 10.2983/035.031.0302
Queiros, A. D., Hiddink, J. G., Johnson, G., Cabral, H. N., and Kaiser, M. J. (2011). Context dependence of marine ecosystem engineer invasion impacts on benthic ecosystem functioning. Biol. Invas. 13, 1059–1075. doi: 10.1007/s10530-011-9948-3
Queiroz, C., Meacham, M., Richter, K., Norström, A. V., Andersson, E., Norberg, J., et al. (2015). Mapping bundles of ecosystem services reveals distinct types of multifunctionality within a Swedish landscape. AMBIO 44, 89–101. doi: 10.1007/s13280-014-0601-0
QUINTESSENCE Consortium (2016). Networking our way to better ecosystem service provision. Trends Ecol. Evol. 31, 105–115. doi: 10.1016/j.tree.2015.12.003
Raffaelli, D., and White, P. C. (2013). Ecosystems and their services in a changing world: an ecological perspective. Adv. Ecol. Res. 48, 1–70. doi: 10.1016/b978-0-12-417199-2.00001-x
Raudsepp-Hearne, C., and Peterson, G. D. (2016). Scale and ecosystem services: how do observation, management, and analysis shift with scale—lessons from Québec. Ecol. Soc. 21:16.
Raudsepp-Hearne, C., Peterson, G. D., and Bennett, E. (2010). Ecosystem service bundles for analyzing tradeoffs in diverse landscapes. Proc. Natl. Acad. Sci. U.S.A. 107, 5242–5247. doi: 10.1073/pnas.0907284107
Riedel, B., Pados, T., Pretterebner, K., Schiemer, L., Steckbauer, A., Haselmair, A., et al. (2014). Effect of hypoxia and anoxia on invertebrate behaviour: ecological perspectives from species to community level. Biogeosciences 11, 1491–1518. doi: 10.5194/bg-11-1491-2014
Rodríguez, J., Beard, T. D. Jr., Bennett, E., Cumming, G., Cork, S., Agard, J., et al. (2006). Trade-offs across space, time, and ecosystem services. Ecol. Soc. 11:28.
Rodríguez-Loinaz, G., Alday, J. G., and Onaindia, M. (2015). Multiple ecosystem services landscape index: a tool for multifunctional landscapes conservation. J. Environ. Manage. 147, 152–163. doi: 10.1016/j.jenvman.2014.09.001
Rossi, F., Gribsholt, B., Middelburg, J. J., and Heip, C. (2008). Context-dependent effects of suspension feeding on intertidal ecosystem functioning. Mar. Ecol. Prog. Ser. 354, 47–57. doi: 10.3354/meps07213
Ruesink, J. L., Feist, B. E., Harvey, C. J., Hong, J. S., Trimble, A. C., Wisehart, L. M., et al. (2006). Changes in productivity associated with four introduced species: ecosystem transformation of a ’pristine’ estuary. Mar. Ecol. Prog. Ser. 311, 203–215. doi: 10.3354/meps311203
Saidi, N., and Spray, C. (2018). Ecosystem services bundles: challenges and opportunities for implementation and further research. Environ. Res. Lett. 13:113001. doi: 10.1088/1748-9326/aae5e0
Sandwell, D. R., Pilditch, C. A., and Lohrer, A. M. (2009). Density dependent effects of an infaunal suspension-feeding bivalve (Austrovenus stutchburyi) on sandflat nutrient fluxes and microphytobenthic productivity. J. Exp. Mar. Biol. Ecol. 373, 16–25. doi: 10.1016/j.jembe.2009.02.015
Saurel, C., Ferreira, J. G., Cheney, D., Suhrbier, A., Dewey, B., Davis, J., et al. (2014). Ecosystem goods and services from Manila clam culture in Puget Sound: a modelling analysis. Aquacult. Environ. Interact. 5, 255–270. doi: 10.3354/aei00109
Schulte, D. M., Burke, R. P., and Lipcius, R. N. (2009). Unprecedented restoration of a native oyster metapopulation. Science 325, 1124–1128. doi: 10.1126/science.1176516
Scyphers, S. B., Powers, S. P., and Heck, K. L. (2015). Ecological value of submerged breakwaters for habitat enhancement on a residential scale. Environ. Manag. 55, 383–391. doi: 10.1007/s00267-014-0394-8
Sebastiano, D., Levinton, J. S., Doall, M., and Kamath, S. (2015). Using a shellfish harvest strategy to extract high nitrogen inputs in urban and suburban coastal bays: practical and economic implications. J. Shellfish Res. 34, 573–583. doi: 10.2983/035.034.0242
Snelgrove, P. V., Thrush, S. F., Wall, D. H., and Norkko, A. (2014). Real world biodiversity–ecosystem functioning: a seafloor perspective. Trends Ecol. Evol. 29, 398–405. doi: 10.1016/j.tree.2014.05.002
Solan, M., Raffaelli, D., Paterson, D., White, P., and Pierce, G. (2006). Marine biodiversity and ecosystem function: empirical approaches and future research needs: introduction. Mar. Ecol. Prog. Ser. 311, 175–178.
Sospedra, J., Falco, S., Morata, T., and Rodilla, M. (2017). Influence of organic enrichment and Spisula subtruncata (da Costa, 1778) on oxygen and nutrient fluxes in fine sand sediments. Estuar. Coasts 40, 726–740. doi: 10.1007/s12237-016-0174-1
Sousa, R., Gutierrez, J. L., and Aldridge, D. C. (2009). Non-indigenous invasive bivalves as ecosystem engineers. Biol. Invas. 11, 2367–2385. doi: 10.1007/s10530-009-9422-7
Spake, R., Lasseur, R., Crouzat, E., Bullock, J. M., Lavorel, S., Parks, K. E., et al. (2017). Unpacking ecosystem service bundles: towards predictive mapping of synergies and trade-offs between ecosystem services. Glob. Environ. Change 47, 37–50. doi: 10.1016/j.gloenvcha.2017.08.004
Stachowicz, J. J., Bruno, J. F., and Duffy, J. E. (2007). Understanding the effects of marine biodiversity on communities and ecosystems. Annu. Rev. Ecol. Evol. Syst. 38, 739–766. doi: 10.1146/annurev.ecolsys.38.091206.095659
Stephenson, J. (2008). The cultural values model: an integrated approach to values in landscapes. Landsc. Urban Plann. 84, 127–139. doi: 10.1016/j.scitotenv.2017.11.196
Sunda, W. G., Graneli, E., and Gobler, C. J. (2006). Positive feedback and the development and persistence of ecosystem disruptive algal blooms. J. Phycol. 42, 963–974. doi: 10.1111/j.1529-8817.2006.00261.x
Tallis, H., and Polasky, S. (2009). Mapping and valuing ecosystem services as an approach for conservation and natural-resource management. Ann. N. Y. Acad. Sci. 1162, 265–283. doi: 10.1111/j.1749-6632.2009.04152.x
Talmage, S. C., and Gobler, C. J. (2010). Effects of past, present, and future ocean carbon dioxide concentrations on the growth and survival of larval shellfish. Proc. Natl. Acad. Sci. U.S.A. 107, 17246–17251. doi: 10.1073/pnas.0913804107
Thrush, S. F., Hewitt, J. E., Gibbs, M., Lundquist, C., and Norkko, A. (2006). Functional role of large organisms in intertidal communities: community effects and ecosystem function. Ecosystems 9, 1029–1040. doi: 10.1007/s10021-005-0068-8
Toupoint, N., Barbier, P., Tremblay, R., Archambault, P., McKindsey, C. W., Winkler, G., et al. (2016). Influence of intertidal recreational fisheries and ’bouchot’ mussel culture on bivalve recruitment. Mar. Environ. Res. 117, 1–12. doi: 10.1016/j.marenvres.2016.03.006
Townsend, M., Davies, K., Hanley, N., Hewitt, J. E., Lundquist, C. J., Lohrer, A. M., et al. (2018). The challenge of implementing the marine ecosystem service concept. Front. Mar. Sci. 5:359. doi: 10.3389/fmars.2018.00359
Turkelboom, F., Thoonen, M., Jacobs, S., and Berry, P. (2015). Ecosystem service trade-offs and synergies. Ecol. Soc. 21:43.
Turner, K. G., Odgaard, M. V., Bøcher, P. K., Dalgaard, T., and Svenning, C. J. (2014). Bundling ecosystem services in Denmark: trade-offs and synergies in a cultural landscape. Landsc. Urban Plann. 125, 89–104. doi: 10.1016/j.landurbplan.2014.02.007
Van Colen, C., Thrush, S. F., Parkes, S., Harris, R., Woodin, S. A., Wethey, D. S., et al. (2015). Bottom-up and top-down mechanisms indirectly mediate interactions between benthic biotic ecosystem components. J. Sea Res. 98, 42–48. doi: 10.1016/j.seares.2014.10.016
van der Schatte Olivier, A., Jones, L., Vay, L. L., Christie, M., Wilson, J., Malham, S. K., et al. (2018). A global review of the ecosystem services provided by bivalve aquaculture. Rev. Aquacult. doi: 10.1111/raq.12301 [Epub ahead of print].
van der Zee, E. M., Tielens, E., Holthuijsen, S., Donadi, S., Eriksson, B. K., van der Veer, H. W., et al. (2015). Habitat modification drives benthic trophic diversity in an intertidal soft-bottom ecosystem. J. Exp. Mar. Biol. Ecol. 465, 41–48. doi: 10.1016/j.jembe.2015.01.001
van Maanen, B., Coco, G., and Bryan, K. R. (2015). On the ecogeomorphological feedbacks that control tidal channel network evolution in a sandy mangrove setting. Proc. R. Soc. A Math. Phys. Eng. Sci. 471:20150115. doi: 10.1098/rspa.2015.0115
Villnas, A., Norkko, J., Lukkari, K., Hewitt, J., and Norkko, A. (2012). Consequences of increasing hypoxic disturbance on benthic communities and ecosystem functioning. PLoS One 7:12. doi: 10.1371/journal.pone.0044920
Vinagre, C., Mendonca, V., Narciso, L., and Madeira, C. (2015). Food web of the intertidal rocky shore of the west Portuguese coast - determined by stable isotope analysis. Mar. Environ. Res. 110, 53–60. doi: 10.1016/j.marenvres.2015.07.016
Volety, A. K., Haynes, L., Goodman, P., and Gorman, P. (2014). Ecological condition and value of oyster reefs of the Southwest Florida shelf ecosystem. Ecol. Indic. 44, 108–119. doi: 10.1016/j.ecolind.2014.03.012
Welsh, D. T., Nizzoli, D., Fano, E. A., and Viaroli, P. (2015). Direct contribution of clams (Ruditapes philippinarum) to benthic fluxes, nitrification, denitrification and nitrous oxide emission in a farmed sediment. Estuar. Coast. Shelf Sci. 154, 84–93. doi: 10.1016/j.ecss.2014.12.021
Wilkie, E. M., Bishop, M. J., O’Connor, W. A., and McPherson, R. G. (2013). Status of the Sydney rock oyster in a disease-afflicted estuary: persistence of wild populations despite severe impacts on cultured counterparts. Mar. Freshw. Res. 64, 267–276.
Winberg, P. C., and Davis, A. R. (2014). Ecological response to MPA zoning following cessation of bait harvesting in an estuarine tidal flat. Mar. Ecol. Prog. Ser. 517, 171–180. doi: 10.3354/meps11052
Worm, B., Barbier, E. B., Beaumont, N., Duffy, J. E., Folke, C., Halpern, B. S., et al. (2006). Impacts of biodiversity loss on ocean ecosystem services. Science 314, 787–790.
Wrede, A., Dannheim, J., Gutow, L., and Brey, T. (2017). Who really matters: influence of German Bight key bioturbators on biogeochemical cycling and sediment turnover. J. Exp. Mar. Biol. Ecol. 488, 92–101. doi: 10.1016/j.jembe.2017.01.001
Wu, S., and Li, S. (2019). Ecosystem service relationships: formation and recommended approaches from a systematic review. Ecol. Indic. 99, 1–11. doi: 10.1016/j.ecolind.2018.11.068
Zu Ermgassen, P. S., Gray, M. W., Langdon, C. J., Spalding, M. D., and Brumbaugh, R. D. (2013a). Quantifying the historic contribution of Olympia oysters to filtration in Pacific Coast (USA) estuaries and the implications for restoration objectives. Aquat. Ecol. 47, 149–161. doi: 10.1007/s10452-013-9431-6
Keywords: ES bundles, ecological mechanisms, shellfish, ecosystem services, ecosystem functions, interactions, EBM
Citation: Rullens V, Lohrer AM, Townsend M and Pilditch CA (2019) Ecological Mechanisms Underpinning Ecosystem Service Bundles in Marine Environments – A Case Study for Shellfish. Front. Mar. Sci. 6:409. doi: 10.3389/fmars.2019.00409
Received: 05 April 2019; Accepted: 03 July 2019;
Published: 02 August 2019.
Edited by:
Katherine Dafforn, Macquarie University, AustraliaReviewed by:
Ana B. Bugnot, The University of Sydney, AustraliaChris Gillies, The Nature Conservancy, Australia
Copyright © 2019 Rullens, Lohrer, Townsend and Pilditch. This is an open-access article distributed under the terms of the Creative Commons Attribution License (CC BY). The use, distribution or reproduction in other forums is permitted, provided the original author(s) and the copyright owner(s) are credited and that the original publication in this journal is cited, in accordance with accepted academic practice. No use, distribution or reproduction is permitted which does not comply with these terms.
*Correspondence: Vera Rullens, dmVyYXJ1bGxlbnNAZ21haWwuY29t