- Department of Primary Industries and Regional Development, Perth, WA, Australia
Sillago schomburgkii inhabits coastal waters of south-western Australia, where it occurs over a wide latitudinal range (22–35°S). The species has occupied this range for at least the past century. It is a valuable fishery species, particularly in northern (warmer) areas where it has historically been most abundant. Fishery trends indicate a gradual poleward shift in the center of abundance since the 1950s, coinciding with gradual ocean warming over the same period. This shift dramatically accelerated after the 2010/2011 marine heatwave, when abundance abruptly declined at the northern edge of the range but increased in southern areas. The heatwave drew attention to the significant, but previously unrecognized, distributional shift of S. schomburgkii. It also helped to elucidate the recruitment-related mechanism by which the shift is occurring. There was evidence of exceptionally strong recruitment by the 2010/2011 year class in southern areas, but weaker recruitment at the northern edge. Spawning by S. schomburgkii is associated with ocean temperatures of approximately 20–26circC. Temperatures are in this range for most of the year in the north, but only briefly during the height of summer in the south. During the heatwave, coastal temperatures were up to 5 degrees above average. It is likely that the spawning period was reduced in the north but extended in the south, due to higher temperatures, which would explain the recruitment trends in each area. All evidence suggests the distributional shift is primarily due to altered spawning success by resident fish in each area (i.e., self-recruitment), rather than by the movement of adults, or larval dispersal. The accelerated shift during the heatwave was probably due to major changes in self-recruitment, but possibly supplemented by atypical southward larval dispersal. For commercial and recreational fisheries that capture this species, the ongoing poleward range shift could have significant negative, and positive impacts in the northern and southern areas, respectively. Fisheries in southern areas are expected to benefit from an increasing availability of this species, although a predicted climate-induced loss of critical habitats could negate any gains at the southern edge of the range.
Introduction
A global redistribution of marine species is predicted to occur in response to climate change (Pinsky et al., 2013; Poloczanska et al., 2016). Poleward range shifts have already been documented for many fish species in response to warming temperatures (Perry et al., 2005; Dulvy et al., 2008; Nye et al., 2009; Last et al., 2011; Cheung et al., 2013; Fossheim et al., 2015). These range shifts pose major challenges for fisheries, which will need to adapt to minimize the socio-economic costs of the changes, take advantage of emerging opportunities, and ensure appropriate regulations are in place to allow adaptation whilst preventing overexploitation of declining or new fishery resources (Rice and Garcia, 2011; Madin et al., 2012; Pinsky and Fogarty, 2012; Bell et al., 2016; Caputi et al., 2017).
As oceans warm, marine heatwaves (MHWs) are becoming more frequent (Lima and Wethey, 2012; Frölicher and Laufkötter, 2018; Oliver et al., 2018). These events can have damaging and long-term impacts on ecosystems, but can also provide a useful “preview” into future ecosystem changes, and the human responses to them, that will occur as temperatures continue to rise and become more variable (Mills et al., 2013). MHWs can therefore assist fisheries to prepare for production losses, or gains, associated with future climate change.
In the austral summer of 2010/2011, an unprecedented MHW affected about 2000 km of the western Australian coastline. During this La Niña-driven event, sea surface temperatures (SST) along the coast between 22 and 34°S exceeded the typical summer level by 3°C on average, including peaks of 5°C above average at numerous sites over this range (Feng et al., 2013; Pearce and Feng, 2013; Wernberg et al., 2013). The MHW was superimposed on a long term, increasing SST trend of about 0.1°C per decade off south-western Australia (Pearce and Feng, 2007; Pearce et al., 2016).
Dramatic effects of the 2010/2011 MHW included mortality of fish, invertebrates, seagrasses and habitat-forming algae, coral bleaching, and the sudden appearance of tropical species in temperate waters (Pearce et al., 2011; Moore et al., 2012; Wernberg et al., 2013, 2016; Arias-Ortiz et al., 2018). Less obvious effects, such as impacts to growth or recruitment of longer-lived species, have also become apparent in the years following the event (Foster et al., 2014; Cure et al., 2015, 2018; Lenanton et al., 2017).
Sillago schomburgkii (Peters, 1864) (Sillaginidae) is a temperate marine fish that is endemic to south-western Australia, where it is distributed over a wide latitudinal range (22–35°S) (Hutchins and Swainston, 1986). It occurs on sheltered, sand flats in shallow coastal waters and the saline parts of estuaries (Hyndes et al., 1996; Hyndes and Potter, 1997). The species, commonly known as “yellowfin whiting,” is a popular target for shore-based commercial and recreational fishers. In 2014, the commercial catch of S. schomburgkii unexpectedly rose to a record high level in the Peel-Harvey Estuary (Figure 1), generating concerns among fishery managers, and stakeholders about the sustainability of this fishery. It then became apparent that changes in S. schomburgkii catches were also occurring in other fisheries across the species range. This study was undertaken to (i) determine the full extent of spatio-temporal changes in abundance of S. schomburgkii and (ii) identify reasons for the observed changes.
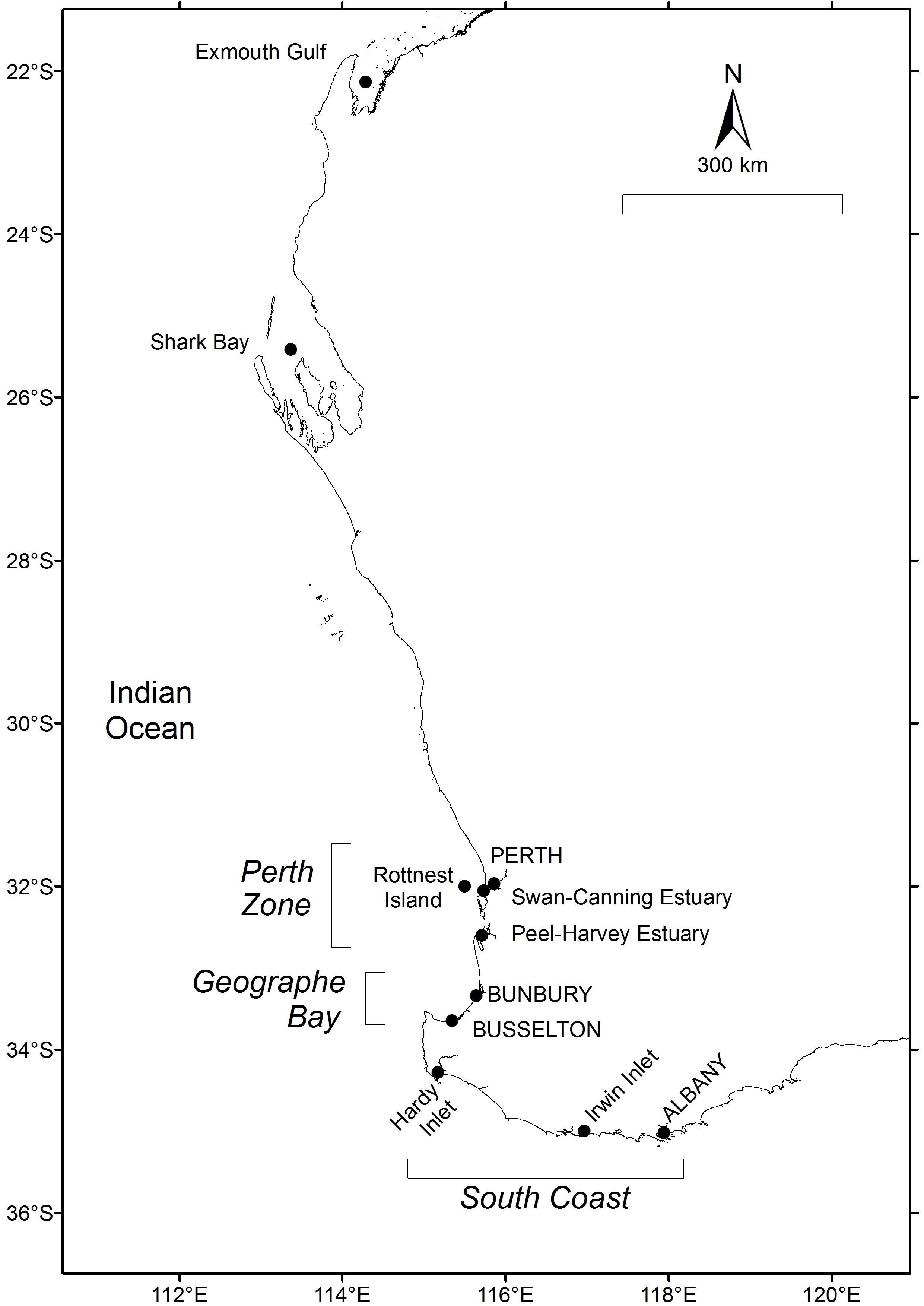
Figure 1. Location of fisheries and sampling locations for Sillago schomburgkii in Western Australia.
Materials and Methods
Biology of Sillago schomburgkii
In Western Australia (WA), S. schomburgkii occurs from Exmouth (22°S, 114.5°E) to Albany (35°S, 118°E) (Figure 1). A second, separate population of this species occurs in South Australia from Spencer Gulf to Fleurieu Peninsula (33–35°S, 136–138.4°E). The population structure in WA has not been directly investigated. However, there is evidence of low rates of alongshore movement by adults, and thus low connectivity of adults at different latitudes in WA (Smith, unpubl. data). The entire life cycle, including spawning, is completed in sheltered, shallow waters (mostly <2 m) (Hyndes and Potter, 1997; R. Lenanton pers. comm). Adults and juveniles inhabit estuaries and ocean waters, although spawning occurs in ocean waters only. Similar to other sillaginids, S. schomburgkii has a protracted spawning period in which multiple batches of pelagic eggs are released by each female (Lenanton, 1970; Hyndes and Potter, 1997; Ferguson, 2000; Coulson, 2003). Fecundity is regarded as indeterminate and thus total annual fecundity is likely to be a function of the duration of the spawning period. S. schomburgkii has a maximum recorded total length (TL) of 427 mm and age of 12 years (Hyndes and Potter, 1997; Brown et al., 2013). Both sexes attain maturity at approximately 200 mm TL and age 2 years (Hyndes and Potter, 1997; Coulson et al., 2005).
Ocean Temperature Data
Ocean temperatures from 1981 onward at Exmouth Gulf (22.375°S, 114.375°E), Shark Bay (25.625°S, 113.635°E), Perth Zone (near Rottnest Island, 32.125°S, 115.625°E), and South Coast (near Albany, 34.875°S, 118.625°E) (Figure 1) were obtained from satellite-derived continuous daily sea surface temperature (SST) data from the NOAA OIv2 dataset at 1/4 degree (∼28 km) resolution1.
In Geographe Bay mean daily ocean temperatures from February 2001 onward were obtained from the Busselton Jetty Underwater Observatory (33.630°S, 115.338°E). Water temperatures at the Observatory (1.7 km from the shore) are recorded hourly at about 4 m depth using automatic temperature loggers accurate to ∼±0.2°C2.
To examine temperature variations during the main S. schomburgkii spawning period (see section “Results”), the “spring” (October-December) and “summer” (January–March) temperature anomalies at each location were calculated against mean levels in pre-MHW years (i.e., 2001/2002–2009/2010 for Busselton; 1981/1982–2009/2010 for other locations). For a more detailed view of the progression of the MHW at each location, mean monthly ocean temperatures in 2010/2011, 2011/2012, and 2012/2013, which all had exceptionally warm summers, were plotted against mean (±SD) monthly temperatures in pre-MHW years.
Compared to satellite-derived coastal SST, shallow nearshore waters in WA typically experience slightly higher (0 to °C) summer maxima, and substantially lower (∼2°C) winter minima (e.g., Baldock et al., 2014; Pearce et al., 2016). Thus, SST is generally indicative of spring and summer temperatures experienced by S. schomburgkii in nearshore waters at the same latitude. SST may have underestimated the temperatures experienced by S. schomburgkii during the MHW. In late February/early March 2011, SST anomalies averaged +2.7°C along the west coast of WA but anomalies of +3 to +5°C were recorded at very shallow sites due to atypically high air-sea flux (Depczynski et al., 2013; Feng et al., 2013; Pearce and Feng, 2013).
Fishery Descriptions and Catch Rates
Sillago schomburgkii is taken commercially and recreationally throughout its range in WA. Commercial catches are taken by numerous small-scale net fisheries that are beach or estuary-based. Since 1976, it has been compulsory for commercial fishers in WA to provide monthly summaries of their catch (kilograms of each species, for each gear type) and effort (number of crew, total days fished by each gear type). Prior to 1976, more limited catch and effort data are available and only for certain fisheries. To assess trends in S. schomburgkii abundance, annual catches and catch rates were examined in a suite (n = 7) of commercial fisheries that collectively span the full latitudinal range of this species (Table 1). In these fisheries, S. schomburgkii is taken as part of a multi-species catch. Due to the monthly aggregation of reported data, effort spent directly targeting S. schomburgkii is not known for any fishery. Therefore, a mean annual “targeted catch rate” was developed by restricting the catch and effort data to the primary gear type, the primary fishing months, and the primary boats that captured S. schomburgkii in each fishery (Table 1). Targeted catch rates of S. schomburgkii for each commercial fishery were calculated for recent years, 1990–2017.
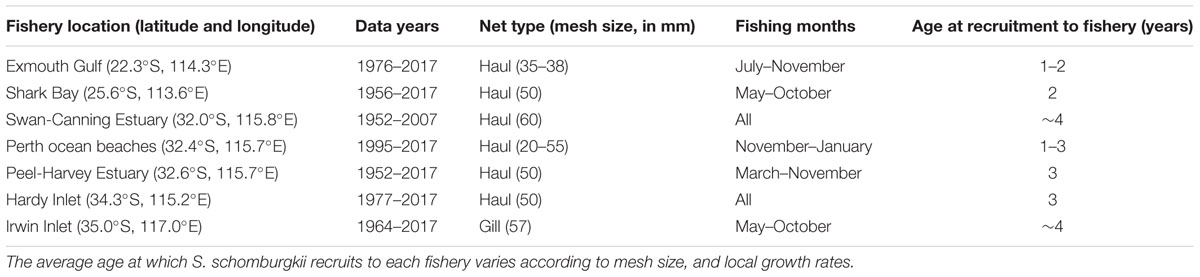
Table 1. Location of commercial fisheries for which catch rates were examined, years for which annual catch and effort data are available, and the primary fishing gear and primary fishing months for capturing S. schomburgkii.
The targeted catch rate was regarded as the most reliable index of abundance of S. schomburgkii for each fishery. In earlier years, where insufficient data were available to calculate targeted catch rates, nominal (raw) annual catch rates (kg/day or kg/boat), and total catches were plotted to provide longer term indicators of abundance. S. schomburgkii has always fetched relatively high wholesale prices (approximately twice the average price per kilogram for finfish captured in these fisheries). It is targeted when available and always retained. Hence the catch in these fisheries is a reasonable indicator of S. schomburgkii availability. The nominal annual catch rate for each fishery was calculated from the total annual catch of S. schomburgkii and total annual effort. The best available measure of long-term annual effort (sum of fishing days by all boats, or mean monthly number of active boats) was used for each fishery. The methods used by each fishery have not substantially changed over time and so fishing efficiency was assumed to be constant. Note: effort in these fisheries has declined substantially since 1970 as a result of a WA government policy to reduce the number of commercial fishers in nearshore and estuarine areas (Anonymous, 1999).
Limited data are available about recreational catches of S. schomburgkii in WA. The annual recreational catch level is not monitored. Most recreational catches of S. schomburgkii are taken in summer, and occur on ocean beaches with smaller amounts also taken in estuaries. To provide an indication of recreational catch rate trends in ocean waters, catch and effort data recorded by an avid recreational angler who fished regularly on ocean beaches in Geographe Bay (Figure 1), were examined. A mean annual catch rate of S. schomburgkii was calculated from the total catch (number of fish, kept, and released) and total effort (number of fishing days) recorded in a voluntary daily logbook by the angler from 2005/2006 to 2016/2017. All fishing was shore-based and restricted to the warmer months (November–February).
Sampling and Analysis of Fish
During 2014–2017, the age composition of S. schomburgkii was sampled from commercial catches at Shark Bay, Perth Zone (in Peel-Harvey Estuary), Geographe Bay (on ocean beaches near Busselton and Bunbury), and South Coast (in Hardy Inlet) (Figure 1). Recreational fishery catches were sampled from the Perth Zone (in Peel-Harvey Estuary and on ocean beaches), and from the South Coast (in Hardy Inlet).
For each fish, the TL (in mm), total weight (g), sex and gonad weight (g) was recorded, and sagittal otoliths were extracted. Fish were later aged by enumerating the number of annually deposited opaque zones in their sagittal otoliths (Hyndes and Potter, 1997; Coulson et al., 2005). Age in months was estimated, assuming a nominal birthday of 1 October at Shark Bay, and a nominal birthday of January 01 at other locations (Hyndes and Potter, 1997; Coulson et al., 2005). Otoliths were aged by two independent readers. Agreement between readers was achieved for all otoliths.
Fish were sampled from commercial nets with a mesh size of 50 mm, which retain fish of approximately 260 mm TL and greater (i.e., 50% selection at this length). This length corresponds to an age of 2 or 3 years depending on sex and region (Hyndes and Potter, 1997; Coulson et al., 2005; Smith, unpubl. data). The selectivity of the recreational fishery was unclear, but the age composition of the recreational catch was similar to that of the local commercial catch, suggesting similar ages at selection. In this study, fish were assumed to be fully selected by each fishing gear at >2 years.
At each site, age composition was similar for females and males, and also similar for commercial and recreational fisheries, so age data for all fish were pooled at each site. To assess recruitment variability, age frequencies of fully selected year classes were loge transformed, and then residuals were examined against a simple linear regression, i.e., a “regression catch curve” approach (Maceina, 1997). This method assumes all fully selected age classes are subject to a similar rate of total mortality (Z), represented by the regression slope, and positive (or negative) residuals indicate above (or below) average recruitment.
The timing of spawning was determined from monthly trends in gonad development at four latitudinal zones: 26°S (Shark Bay), 32°S (Perth Zone), 33.5°S (Geographe Bay), and 34.5°S (South Coast) (Figure 1). Samples were obtained in each month in each zone, except Shark Bay where only 6 months were represented. To provide adequate monthly sample sizes at each latitude, all available gonad data from that latitude were pooled, including additional fish from other sites not used in the analysis of age composition. The majority of South Coast fish were obtained from Hardy Inlet, with the remainder from various estuaries located between Hardy Inlet and Albany. There were no obvious differences in the timing of gonad development between estuaries and so all South Coast sites were pooled. A gonadosomatic index (GSI) for each fish was calculated as GSI = 100 × [W1/(W2-W1)], where W1 is weight of the gonad and W2 is total weight of the fish. Gonads from fish below the length at 50% maturity (L50) were excluded. L50 values previously estimated by Coulson et al. (2005) were assumed, i.e., 220/195 mm TL for females/males in Shark Bay, and 200/190 mm TL for females/males elsewhere. A monthly mean (+ SD) GSI for each sex was calculated at each location. Mean monthly GSI is a good indicator of relative spawning activity in S. schomburgkii (Hyndes and Potter, 1997; Coulson et al., 2005).
Results
Ocean Temperatures
Along the WA coast, mean monthly ocean temperatures generally follow a latitudinal gradient, with highest temperatures in northern areas, and lowest in southern areas (Figure 2). The exception in this study was Geographe Bay, where temperatures in autumn/winter are substantially lower than those of the Perth and South Coast Zones.
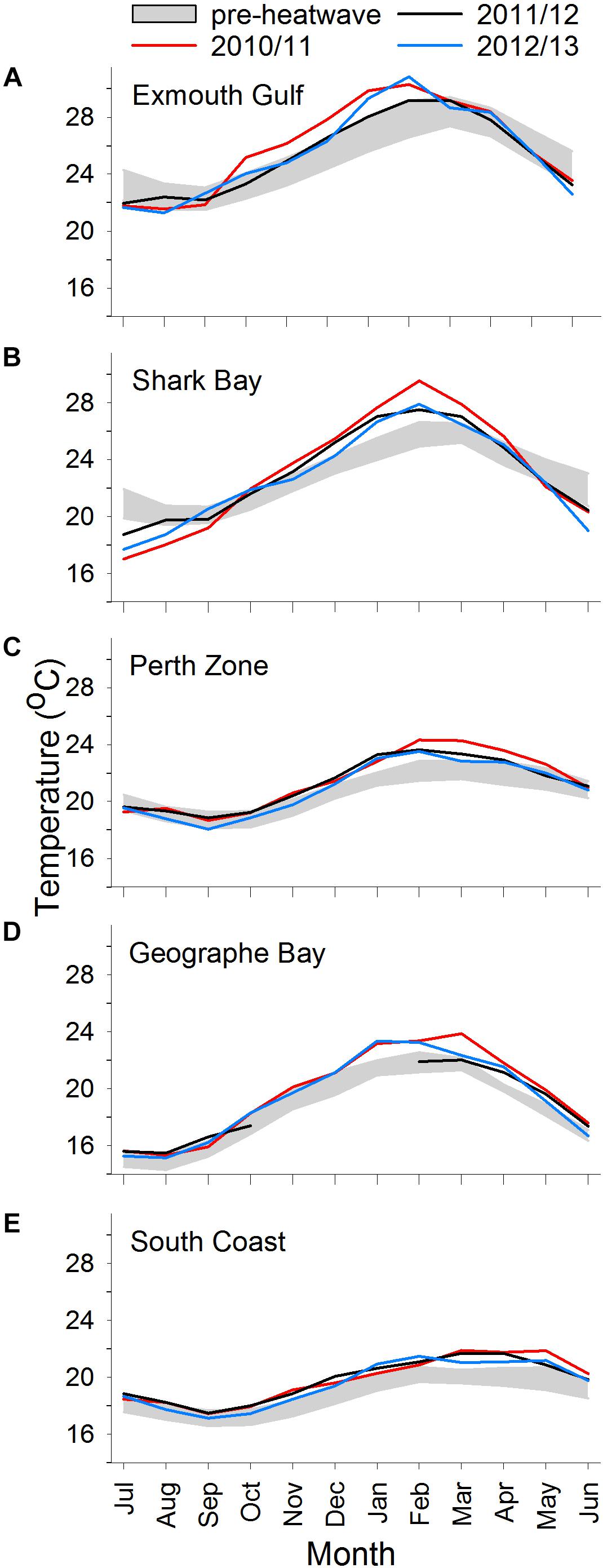
Figure 2. Mean monthly ocean temperatures in individual years 2010/2011, 2011/2012, and 2012/2013 vs. pre-heatwave years (years pooled; shaded area is mean ± SD) at (A) Exmouth, (B) Shark Bay, (C) Perth Zone, (D) Geographe Bay, and (E) South Coast. Pre-heatwave years are 1981/1982–2009/2010, except for Geographe Bay which are 2001/2002–2009/2010.
In pre-MHW years, the mean monthly SST ranged from 22°C (in August/September) to 28°C (March) at Exmouth, 20°C (August/September) to 26°C (February/March) at Shark Bay, 19°C (September/October) to 22°C (February-April) at Perth, and 17°C (September/October) to 20°C (February-April) on the South Coast (Figure 2). In Geographe Bay, mean monthly temperature ranged from 15°C (July/August) to 22°C (February/March) (Figure 2D).
During 2010/2011, summer temperatures were well above their pre-MHW mean levels at all sites. In 2010/2011, the summer temperature anomaly was ∼3°C at Shark Bay, ∼°C at Exmouth, Perth, and Geographe Bay, and ∼1°C on the South Coast (Figure 3). At Exmouth the spring temperature anomaly was also high (∼2°C) in 2010/2011. In 2011/2012 and 2012/2013, spring and summer temperatures were generally lower than in 2010/2011 but still relatively high compared to pre-MHW levels, with the exception of relatively cool summer temperatures at Geographe Bay in 2011/2012. Notably, on the South Coast the summer temperatures in 2011/2012 and 2012/2013 were both higher than in 2010/2011. In Geographe Bay, warm conditions continued in 2014/2015 and 2015/2016, when spring temperatures were higher than in 2010/2011.
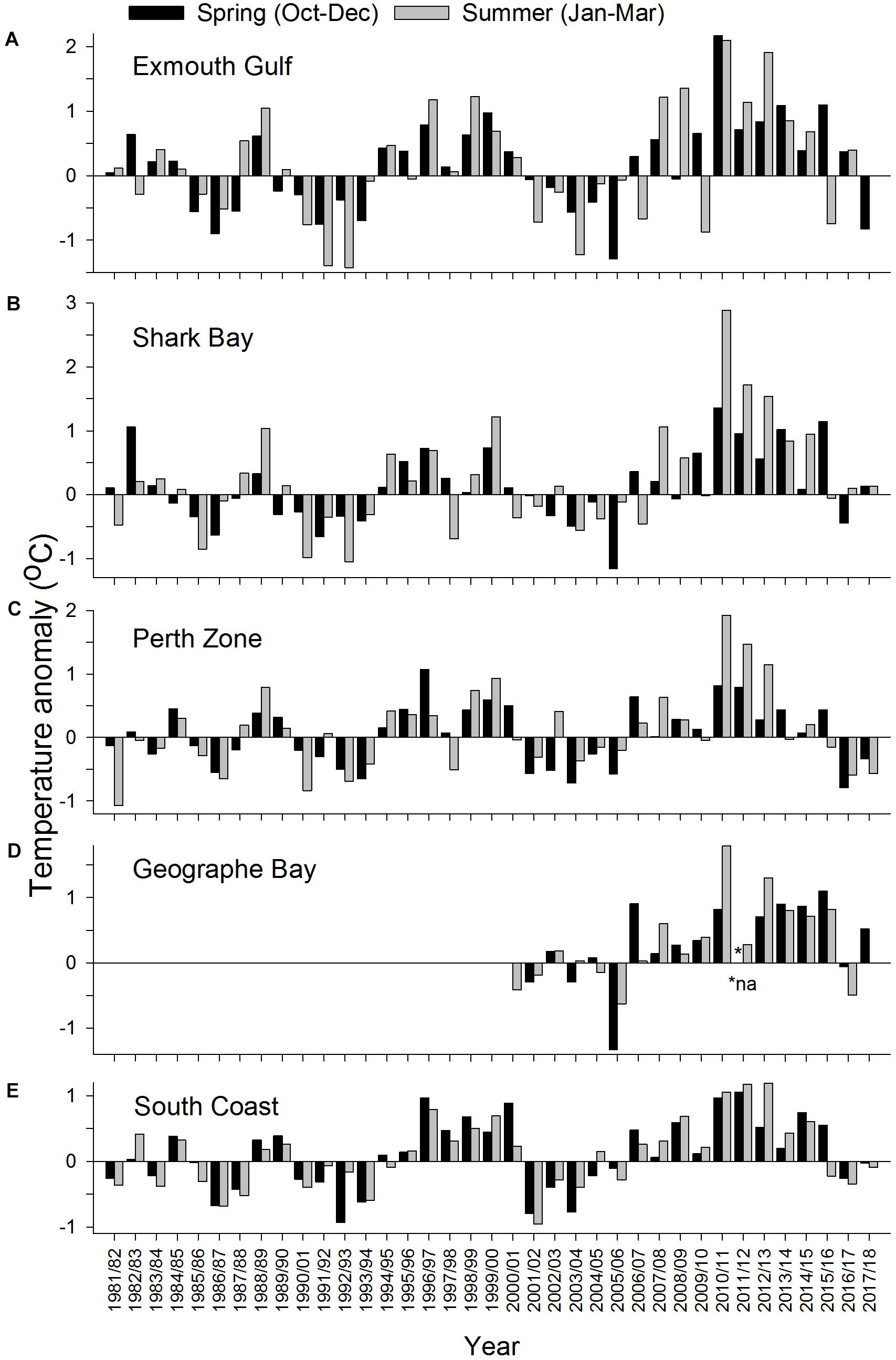
Figure 3. Anomalies in mean ocean temperature during spring (October–December) and summer (January–March) at (A) Exmouth, (B) Shark Bay, (C) Perth Zone, (D) Geographe Bay, and (E) South Coast.
Catch and Catch Rate Trends
In Exmouth Gulf, the nominal commercial catch rate of S. schomburgkii was variable but displayed no obvious directional trend between 1976 and 2017 (Figure 4A). There was a shift toward a higher number of crew per boat, which contributed to higher nominal catch rates in the mid-2000s. The targeted catch rate (which was standardized for crew number) followed a declining trend during 1990–2017 (Figure 5A). From a peak in 1994, it gradually declined to low levels during 2012–2014, then increased slowly until 2017. The targeted catch rate was also relatively low in 2001.
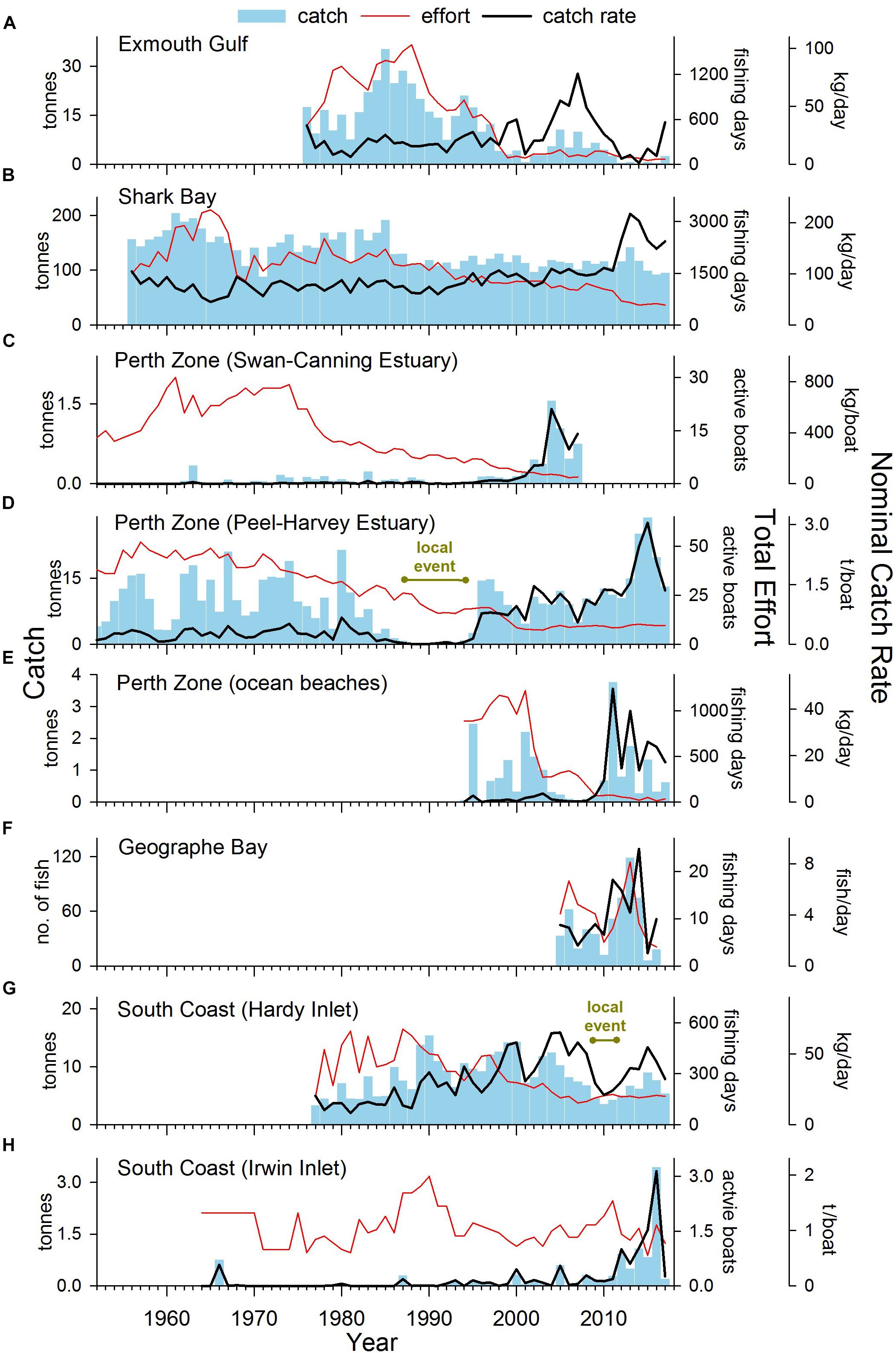
Figure 4. Total annual catch, effort, and nominal catch rates of S. schomburgkii for commercial fisheries in (A) Exmouth Gulf, (B) Shark Bay, (C) Perth Zone (Swan-Canning Estuary), (D) Perth Zone (Peel-Harvey Estuary), (E) Perth Zone (ocean beaches), (G) South Coast (Hardy Inlet) and (H) South Coast (Irwin Inlet), and (F) recorded by a recreational fisher on ocean beaches in Geographe Bay. “Year” refers to calendar years except for (E–G) which are fiscal years.
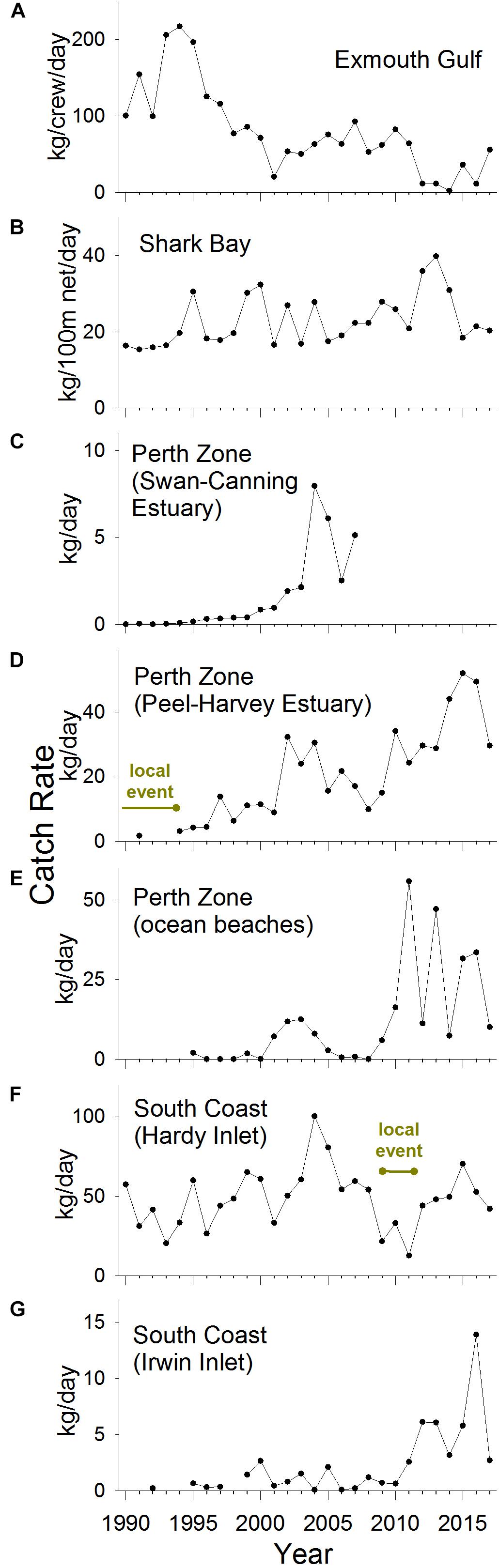
Figure 5. Targeted annual commercial catch rates of S. schomburgkii, 1990–2017, in (A) Exmouth Gulf, (B) Shark Bay, (C) Perth Zone (Swan-Canning Estuary), (D) Perth Zone (Peel-Harvey Estuary), (E) Perth Zone (ocean beaches), (F) South Coast (Hardy Inlet), and (G) South Coast (Irwin Inlet). “Year” refers to calendar years except for (E,F) which are fiscal years.
In Shark Bay, the nominal commercial catch rate was stable from the mid-1960s until 1991, then followed an increasing trend until 2017, including a pronounced peak in 2013 (Figure 4B). After 1991, there was a shift toward the use of larger nets, which contributed to the rise in nominal catch rates. During 1990–2017, the targeted catch rate (which was standardized for net length) varied, but displayed no clear directional trend. There was a relatively small peak in the targeted catch rate in 2013 (Figure 5B).
In the Perth Zone, the commercial catch of S. schomburgkii in the Swan-Canning Estuary was negligible prior to 2000. The nominal and targeted catch rates both abruptly increased after 2000 to peak in 2004 and remained relatively high until 2007 when this fishery ceased (Figures 4C, 5C). In the Peel-Harvey Estuary, the nominal commercial catch rate followed a gradual increasing trend from 1952 until 2013, except during 1987–1994 when it was almost zero (Figure 4D). The nominal catch rate peaked sharply during 2014–2016, then declined in 2017. The targeted catch rate followed an overall increasing trend during 1990–2017, including two localized peaks during 2002–2004, and 2015–2016 (Figure 5D). On ocean beaches in the Perth Zone, the nominal commercial catch rate was relatively low until 2009/10, then increased abruptly in 2011/2012 and remained relatively high until 2017/2018 (Figure 4E). The targeted catch rate was negligible until 2000/2001, increased to a small peak in 2003/2004 then returned to zero (Figure 5E). The targeted catch rate increased sharply to peak in 2011/2012, then remained relatively high until 2017/2018.
In Geographe Bay, the recreational logbook fisher reported his highest catch of S. schomburgkii in 2013/2014 (Figure 4F). His catch rate was relatively high during 2011/2012–2014/2015, compared to other years (Figure 4F).
On the South Coast, the nominal commercial catch rate in Hardy Inlet steadily increased during 1977/1978–2008/2009, then fell during 2009/2010–2012/2013, before returning to relatively high levels (Figure 4G). The targeted catch rate increased slightly during 1990/1991–2008/2009, fell during 2009/2010–2011/2012, then returned to pre-2009/2010 levels in subsequent years (Figure 5F). In Irwin Inlet, the commercial catch of S. schomburgkii was typically zero until 1986, then relatively low until 2011 (Figure 4H). The nominal and targeted catch rates in Irwin Inlet were both relatively low until 2011, then increased sharply to a peak in 2016, then fell in 2017 (Figures 4H, 5G).
Age Composition and Recruitment Variability
The age composition of the commercial catch of S. schomburgkii in Shark Bay was sampled in 2014. The ages of both females and males ranged from 1 to 9 years at this site. There was no evidence of recruitment variation, i.e., no obviously strong or weak year classes (Figure 6A).
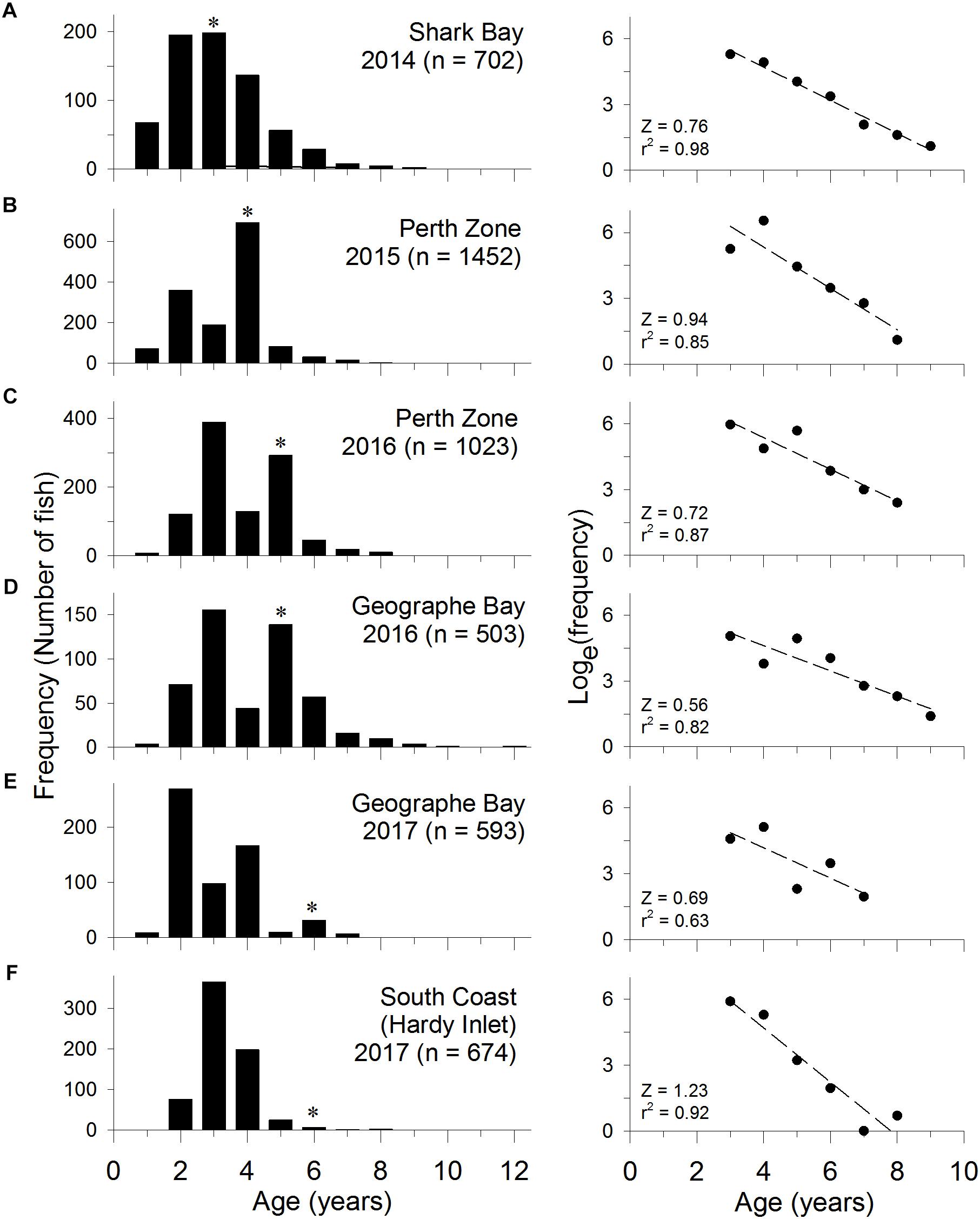
Figure 6. Age frequency distribution of S. schomburgkii in fishery catches sampled at (A) Shark Bay in 2014, (B) Perth Zone in 2015, (C) Perth Zone in 2016, (D) Geographe Bay in 2016, (E) Geographe Bay in 2017, and (F) South Coast (Hardy Inlet only) in 2017. (∗year class spawned during 2010/2011). Raw frequencies shown in left column and natural log transformed values in right column, with linear regression fitted to fully selected ages.
In the Perth Zone, the age composition of the commercial catch was sampled in the Peel-Harvey Estuary in 2015 and 2016. Recreational catches were sampled in 2016 in this estuary and in ocean waters. At this location the ages of females ranged from 1 to 9 years, while males ranged from 1 to 8 years. In all samples a strong year class, which was aged 4 years in 2015 and 5 years in 2016, was evident (Figures 6B,C). This age class was estimated to have been spawned during summer 2010/2011.
In Geographe Bay, the age composition of the commercial catch was sampled in 2016 and 2017. Ages of females ranged from 1 to 10 years, while males ranged from 2 to 12 years. The 2010/2011 year class was relatively strong in 2016 (then aged 5 years) and again in 2017 (aged 6 years) (Figures 6D,E). The 2011/2012 year class (aged 4 years in 2016, 5 years in 2017) was relatively weak. Despite not being fully selected by the fishing gear, the 2014/2015 year class (age 2 years) was the most abundant age group in 2017, which suggests that it was relatively strong (Figure 6E). Overall, there was evidence of more variability in annual recruitment strength at this location than Shark Bay or the Perth Zone.
On the South Coast, the age composition of commercial and recreational catches were sampled in Hardy Inlet in 2017. Females ranged from 1 to 6 years, and males from 2 to 8 years. Catches in Hardy Inlet were dominated by fish aged 4 years or less, which were spawned in 2012/2013 or later (Figure 6F). The proportion of older fish (>4 years) was lower than at other sites. Due to the truncated nature of the age composition, it was not possible to discern the relative strength of any age classes in Hardy Inlet.
Monthly Spawning Trends at Each Latitude
At latitude 26°S (Shark Bay), almost all gonads of S. schomburgkii were collected during May, June, August, or September. Only 9 fish (all male) were collected outside these months. Male and female mean GSI values at 26°S were relatively high in August-September and low in the other sampled months (Figure 7A). At latitude 32°S (Perth Zone) and 33.5°S (Geographe Bay), gonads were collected in every month. Mean GSI values were elevated from November to March at 32°S, and from November to February at 33.5°S (Figures 7B,C). At latitude 34.5°S (South Coast), gonads were collected in all months except April and May. GSI values at 34.5°S were relatively high for both sexes from November to January, with some relatively high values for males also observed in February and October (Figure 7D).
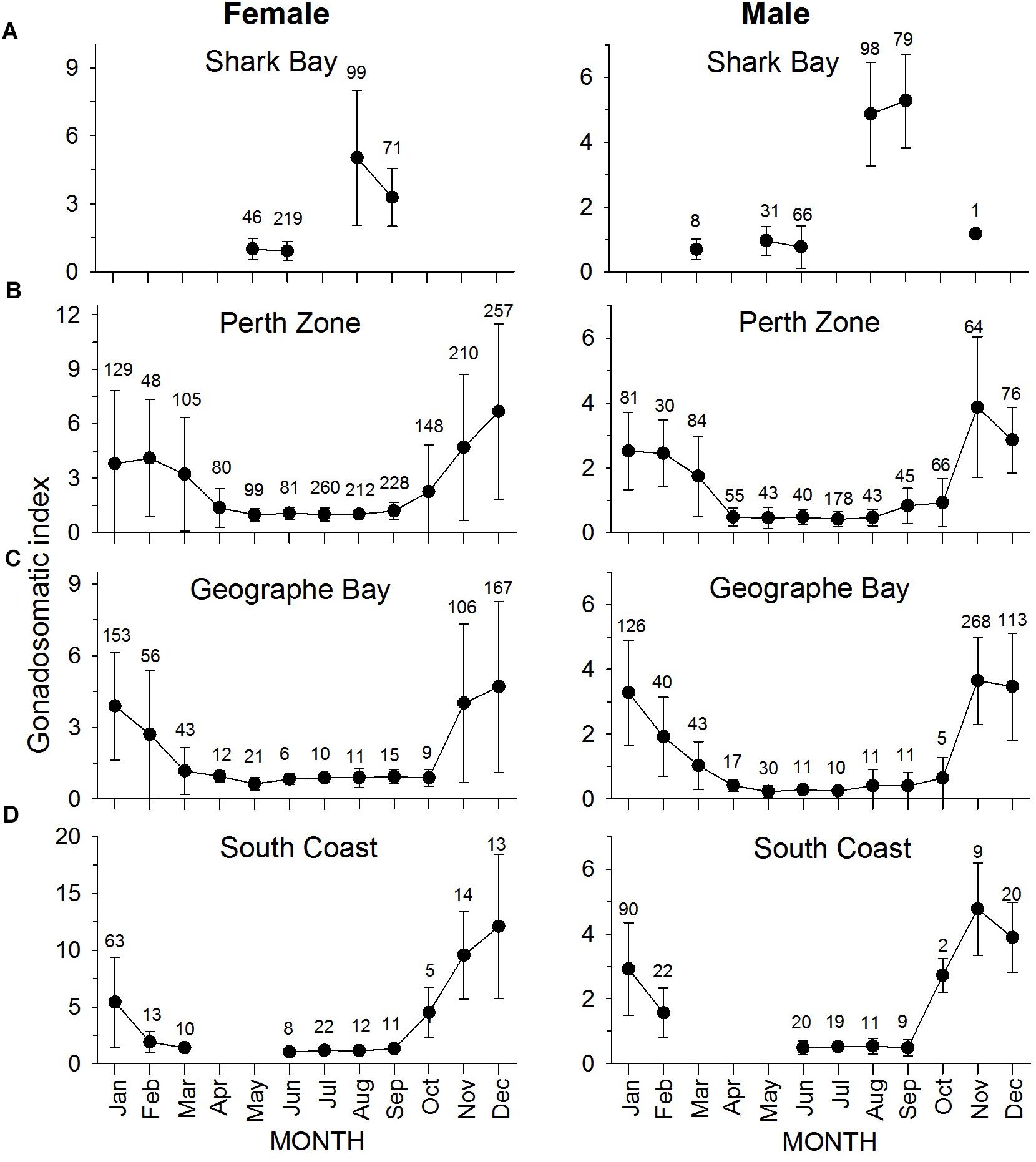
Figure 7. Monthly mean (±SD) gonadosomatic index for female (left column) and male (right column) S. schomburgkii in (A) Shark Bay, (B) Perth Zone, (C) Geographe Bay, and (D) South Coast. Each data point is labeled with number of fish sampled.
Discussion
Evidence for Poleward Shift by S. schomburgkii
There is evidence of a multi-decadal, poleward shift in the center of abundance of S. schomburgkii in WA, including a gradual increase in abundance in the southern part of its range since the 1950s, stable abundance in the center (also since the 1950s), and a more recent decline in abundance at the northern edge of its range since 1990.
In WA, intermittent commercial fishing records for S. schomburgkii commence around the start of the 20th century and, in combination with anecdotal reports, provide good information about the historical distribution of this species. Records of commercial “whiting” catches that are almost certainly S. schomburgkii are available from 1899 in the Peel-Harvey Estuary and from 1904 in estuaries around Albany (Lenanton, 1984). “Whiting” was reported to be relatively abundant in the 1920s in Shark Bay (Lenanton, 1970) and the 1950s in Geographe Bay (Gaynor et al., 2008). Specific catches of S. schomburgkii are recorded from south coast estuaries since the 1940s (Lenanton, 1984). Thus S. schomburgkii has occupied its current latitudinal range in WA (i.e., 22–35°S) since at least the 1940s and probably much earlier.
Of the sites examined in this study, Shark Bay is the closest to the center of the species range. Shark Bay hosts the largest commercial fishery for S. schomburgkii and has consistently yielded relatively large annual catches since it commenced in the 1940s (Lenanton, 1970). Apart from a period of overfishing in the early 1960s (Lenanton, 1970), there is no evidence of major changes in abundance of S. schomburgkii in Shark Bay since records commenced.
About 700 km to the south, fishery trends in the Peel-Harvey and Swan-Canning Estuaries provide long-term records of abundance in the Perth Zone. These data indicate a gradual rise in abundance of S. schomburgkii since the 1950s. In adjacent ocean waters, fishery records are limited (commencing in 1995) but these also follow an increasing trend.
At the southern edge of the species range, early recorded catches of S. schomburgkii in various estuaries on the South Coast of WA were typically small and infrequent, indicating relatively low historical abundance in this region (Lenanton, 1984). In Hardy Inlet, which currently yields the largest commercial and recreational fishery catches of S. schomburgkii on the south coast, this species was reported to be rare or absent in the 1940s and 1950s (“there were no yellowfin whiting to be caught” C. Price, retired commercial fisher, in Gaynor et al., 2008). By 1974/1975, when commercial catch records commence, S. schomburgkii had become relatively abundant in Hardy Inlet (Lenanton, 1977). The commercial catch rate indicates a steady increase in the abundance of S. schomburgkii in this estuary between the 1970s and the late 2000s. Limited observations of the recreational fishery in Hardy Inlet also suggest an increase. In 1974, S. schomburgkii was estimated to be the most common species retained by recreational fishers, comprising 42% of the total recreational catch in this estuary (Caputi, 1976). By 2005, the species was estimated to comprise 58% of the recreational catch in this estuary (Prior and Beckley, 2007), suggesting a further increase in abundance. In Irwin Inlet, located about 180 km southeast of Hardy Inlet, the earliest recorded catches of S. schomburgkii were in 1946, but the catch rate suggests abundance was very low until 1990, and then began to gradually rise.
The increase in abundance of S. schomburgkii at high latitudes is not restricted to WA. There is also evidence of increasing abundance in South Australian waters, where commercial catch rates have steadily increased since 1990, following a similar trend to WA catch rates at the same latitudes (33–35°S) (Steer et al., 2018).
After the 2010/2011 MHW, the southward shift of S. schomburgkii temporarily accelerated. At the northern edge of the range, abundance in Exmouth Gulf abruptly declined to a very low level as indicated by historically low catch rates during 2012–2014. In the southern half of the range, all sites examined in this study (except Hardy Inlet, but see section “Importance of Habitat” discussion below for reasons why) experienced a major spike in abundance of S. schomburgkii after the MHW. For example, catch rate trends implied an approximately twofold increase in abundance in the Peel-Harvey Estuary, and a 10-fold increase in Irwin Inlet. Toward the center of the range, in Shark Bay, S. schomburgkii abundance also briefly increased after the MHW, as suggested by a peak in the catch rate in 2013. However, the magnitude of the increase was less than at southern sites. After the MHW, fishery trends suggested that abundances at each site returned to levels consistent with the long-term trends in that area.
Reproductive Output Is Controlled by Ocean Temperature
The poleward distributional shift of S. schomburgkii (both long-term and during the MHW) can be largely explained in terms of changes in reproductive output at each latitude in response to rising ocean temperatures. Field observations in WA indicate that spawning by S. schomburgkii is associated with a temperature range of approximately 20–26°C (Hyndes and Potter, 1997; Coulson et al., 2005; this study). The same temperature range has also been indicated for spawning by S. ciliata and S. japonica, which are morphologically and ecologically similar (Hotta et al., 2001, 2003; Payne et al., 2016). Sillago species in temperate and subtropical regions predominantly spawn in spring and/or summer, suggesting that rising water temperature and/or photoperiod may be environmental cues for gonad maturation (Tongnunui et al., 2006; Kendall and Gray, 2009; Wang et al., 2010; Lowerre-Barbieri et al., 2011). The onset of spawning (i.e., actual release of gametes) in these species is likely to be primarily controlled by temperature (Lee, 1981; Lee and Hirano, 1985; Hotta et al., 2001; Tongnunui et al., 2006). Thus, we propose for S. schomburgkii that (i) rising temperature and/or photoperiod is required for gonad development and (ii) spawning is restricted to a temperature range of approximately 20–26°C.
The duration of the spawning period of S. schomburgkii declines from latitude 26°S to 35°S, consistent with the declining gradient in ocean temperatures over the same range. At 26°S (Shark Bay), spawning primarily occurs over a 5-month period from August to December, with small amounts of spawning in other months (Coulson et al., 2005; this study). At 32°S (Perth Zone), spawning also occurs over a 5-month period, from November to March, but with little evidence of spawning in other months (this study). Further south, the spawning period commences at approximately the same time but is progressively shorter with increasing latitude, i.e., November-February at 33.5°S (Geographe Bay) and November–January at 34–35°S (South Coast) (this study). Additionally, S. schomburgkii populations in South Australia (latitude 33–35°S) have a spring/summer spawning period of 3 or 4 months (Ferguson, 1999, 2000), similar to WA populations at the same latitudes.
In Shark Bay, SST typically remains within the preferred range (20–26°C) all year, potentially facilitating spawning throughout the year. In contrast, SST at the southern edge of the species range rarely exceeds 20°C, and at the northern edge is often above 26°C during spring/summer, limiting spawning potential in both cases. During the 2010/11 MHW, significantly higher spring/summer SST in each region could have dramatically increased spawning activity at the southern edge, and reduced it at the northern edge. Relatively warm summer SST in 2011/2012 and 2012/2013, particularly on the South Coast, could have altered spawning activity in these years too. The dramatic 10-fold increase in abundance in Irwin Inlet may be the product of three consecutive years of elevated summer temperatures and enhanced local spawning success.
It is noteworthy that the 5-month spawning period in the Perth Zone observed in our study is longer than the 3-month spawning period reported from the same area in the early 1990s (Hyndes and Potter, 1997). This difference is consistent with the substantially higher summer SST in this Zone during our study.
Localized Variations in Recruitment Linked to Local Ocean Temperatures
The evidence presented here indicates that the changes in S. schomburgkii abundance that occurred in each region after the 2010/2011 MHW were primarily due to variations in juvenile recruitment strength in each region and not due to movement between regions by older fish. In each fishery, a lag of one or more years between the MHW and the catch rate response indicates that older fish did not migrate in/out of the fishery area during, or immediately after, the MHW. In each fishery, the magnitude of the lag was consistent with the age at which S. schomburgkii becomes vulnerable to capture by their respective fishing gears. This suggests that the increase (or decrease) in each catch rate was due to strong (or weak) recruitment into each fishery by the year class that was spawned during 2010/2011. Thus, the catch rate trends imply that the MHW led to weaker recruitment at the northern edge of the range, but led to stronger recruitment in southern areas.
The age composition of S. schomburgkii sampled during 2015–2017 provides further evidence of stronger recruitment by the 2010/2011 year class in the southern part of the range. In the Perth Zone and Geographe Bay, fish spawned during the MHW were two or three times more abundant than expected, based on the relative strengths of each age class. The recruitment of the 2010/2011 year class into local fisheries would account for the abrupt increase in their catch rates 1–3 years later, and for the maintenance of elevated catch rates for several years while this year class survived in the population.
In Shark Bay, toward the center of the range, the age composition sampled in 2014 provided no indication of strong recruitment by the 2010/2011 year class and was similar to the age composition prior to the MHW [sampled in 2001–2003 (P. Coulson, unpubl. data)]. The age data are inconsistent with the catch rate trends at this site, which suggested an increase in abundance after the MHW. However, the abundance increase was relatively small compared to the increases at southern sites, so there may have been a minor change in recruitment that was not detected in the age composition at Shark Bay.
The unique temperature regime in Geographe Bay provides an explanation for the stronger variability in annual recruitment by S. schomburgkii observed here compared to other sites. Geographe Bay lies outside the influence of the warm leeuwin current (LC), which flows poleward along the west coast and extends eastward along the south coast (Feng et al., 2003). The LC has a distinct seasonal cycle, with southward transport reaching a maximum during autumn/winter (April–August), thus maintaining relatively warm ocean temperatures along the south coast in winter (Smith et al., 1991; Feng et al., 2003). The LC bypasses Geographe Bay and, as a consequence, the Bay typically experiences colder winter temperatures than the Perth Zone and South Coast (Fahrner and Pattiaratchi, 1995). Additionally, the cold capes current (CC) exerts a stronger influence in Geographe Bay, which can cause summer temperatures here to be significantly cooler than nearshore waters in the Perth Zone (Fahrner and Pattiaratchi, 1995; Pearce and Pattiaratchi, 1999). The CC originates near Cape Leeuwin (34°S) as cold, upwelled water, which flows into Geographe Bay and then northward against the coast in summer. Hence the fish populations in Geographe Bay and Perth are exposed to quite different temperature regimes, despite being separated by an alongshore distance of only ∼100 km, which is likely to result in differences in the duration of spawning and strength of local recruitment. Relatively weak recruitment in 2011/2012 in Geographe Bay compared to Perth was consistent with the summer temperature anomalies at each site, i.e., low in Geographe Bay and high in Perth.
Larval Dispersal or Self-Recruitment?
Broadly speaking, there are two mechanisms by which an increase in juvenile recruitment at higher latitudes could potentially occur: (i) an influx of remotely spawned recruits dispersed by ocean currents (“larval dispersal”), or (ii) an increase in the production of locally spawned recruits (“self-recruitment”). All lines of evidence suggest that enhanced self-recruitment by populations at higher latitudes, and not southward larval dispersal, is facilitating the gradual poleward shift by S. schomburgkii.
Overall, the location and the timing of spawning by S. schomburgkii greatly limits the potential for alongshore dispersal of their larvae. On the west coast of WA, alongshore flow in nearshore waters is not driven by the LC, but rather, is largely driven by the prevailing winds which result in primarily northward flows between September and February and no dominant direction in other months (Fahrner and Pattiaratchi, 1995; Woo et al., 2006; Zaker et al., 2007; Gallop et al., 2012; Ruiz-Montoya and Lowe, 2014). Thus, under typical oceanographic conditions, there is limited potential for the poleward dispersal of eggs or larvae of S. schomburgkii that are primarily spawned in spring/summer. At this time, larvae spawned in nearshore waters are likely to be retained within their natal region, with a low probability of being advected large (>200 km) distances alongshore, either northward or southward (assuming a larval duration of 3–4 weeks; Feng et al., 2010; Berry et al., 2012). The very shallow (mostly <2 m), sheltered habitats occupied by S. schomburgkii further reduces the likelihood of larval dispersal. Along the west coast, this habitat type is concentrated within marine embayments, such as Exmouth Gulf, Shark Bay and Geographe Bay, that are sheltered from the direct influence of alongshore currents, and where larval retention rates are high (Fahrner and Pattiaratchi, 1995; Nahas et al., 2003; Feng et al., 2010; Breheny et al., 2012).
The region from Cape Naturaliste to Cape Leeuwin is a prominent geographical feature that acts as a partial barrier to dispersal from the west coast to the south coast, particularly for summer-spawned larvae (Berry et al., 2012). The CC originates in this area during spring and flows into Geographe Bay, potentially capturing larvae that have been advected from northern areas (Pearce and Pattiaratchi, 1999). On the south coast, Hardy Inlet lies in the shadow of Cape Leeuwin, beyond the direct influence of the southward flowing LC. The probability of larvae from “upstream” spawning areas on the west coast being entrained in the LC and then recruiting into Hardy Inlet is particularly low (Feng et al., 2010; Berry et al., 2012). Despite this isolation, there has been a gradual increase in the abundance of S. schomburgkii in Hardy Inlet over many decades, which suggests that self-recruitment is maintaining this population.
The accelerated shift during the 2010/2011 MHW was probably due to greatly enhanced self-recruitment at higher latitudes, but may also have been supplemented by atypical larval dispersal. In 2010/2011, three unusual factors combined to greatly increase the likelihood of southward advection of S. schomburgkii larvae. First, the early onset of the LC provided atypical southward transport in spring and summer along the outer shelf (Feng et al., 2013; Pearce and Feng, 2013). Secondly, at higher latitudes, suppression of the CC resulted in unseasonal southward flows over the inner shelf in February/March (Pearce and Feng, 2013; Benthuysen et al., 2014). Thirdly, at higher latitudes, an extension of the spawning period due to warmer nearshore temperatures may have increased the production of larvae in late summer/early autumn when the LC velocity was peaking. Together, these conditions provided an unprecedented opportunity for southward advection of S. schomburgkii larvae along the west coast in 2010/2011. The larvae of numerous tropical species, including summer-spawning fish, were dispersed to higher latitudes in 2010/2011 (Pearce et al., 2011; Wernberg et al., 2013; Caputi et al., 2014; Cure et al., 2017; Lenanton et al., 2017), which suggests that the southward advection of S. schomburgkii at this time is possible.
The Importance of Habitat Availability
In WA, further increases in abundance of S. schomburgkii at higher latitudes may be limited by the availability of suitable habitats, particularly along the south coast. This coastline is subject to high wave energy and so the shallow, sheltered habitats favored by S. schomburgkii are largely restricted to estuaries. However, the quality and accessibility of estuarine habitats in south-western Australia is predicted to decline in future due to the impacts of climate change and catchment processes (Hallett et al., 2018). In particular, declining streamflow and ongoing eutrophication of estuaries are likely to strongly affect S. schomburgkii, as already demonstrated in several estuaries.
In the Peel-Harvey Estuary, commercial catches of S. schomburgkii almost ceased during 1986–1994, coinciding with a period of intense algal blooms in this estuary due to eutrophication (Brearley, 2005). The abrupt decline in catch was partly due to fishing nets becoming clogged with algae and difficult to deploy in many parts of the estuary (Lenanton et al., 1985). However, the abundance of S. schomburgkii probably also declined as benthic habitats within the estuary deteriorated. Firstly, there was reduced availability of bare sand habitat due to an increase in macro-algae. Secondly, hypoxia in bottom waters led to fish kills and presumably reduced the availability of benthic invertebrate prey (Potter et al., 1983). In 1994, the construction of an artificial entrance (the “Dawesville Channel”) led to increased tidal exchange, higher salinity, reduced nutrient loads, and a reduction in algal blooms in the estuary basin (Kelsey et al., 2011). By 1996, commercial catches of S. schomburgkii had resumed.
In Hardy Inlet, the commercial catch of S. schomburgkii started to decline in late 2009 and remained at a relatively low level until 2013. As a consequence of declining streamflow and eutrophication, nuisance blooms of macroalgae and cyanobacteria occurred in this estuary prior to and during 2010, which reduced the quantity and quality of bare sand habitat (White, 2012). Very few S. schomburgkii were observed in mid-2010, and their abundance in Hardy Inlet had reportedly been declining for several years prior to 2010 (T. Price, commercial fisher, pers. comm.). Additionally, the sand bar at the entrance (normally permanently open) temporarily closed in 2010 after low winter rainfall (White, 2012; Brearley, 2013), preventing juvenile recruitment into the estuary. Juvenile S. schomburgkii use this estuary, as well as adjacent coastal habitats, as a nursery area (Lenanton, 1977; Valesini et al., 1997). Hence, unfavorable conditions prior to and during 2010–2012 may have encouraged adult fish to emigrate from Hardy Inlet, and restricted recruitment into this estuary. The age composition sampled in 2017 had a low representation of fish spawned prior to 2012/13, which is consistent with poor recruitment during 2010–2012.
Conclusion
In the coastal waters of WA, S. schomburgkii occurs over a very wide latitudinal range (22 to 35°S) which it has occupied for at least the past century. Since the 1950s, there is evidence of a gradual, poleward shift in the center of S. schomburgkii abundance, coinciding with long-term ocean warming off South-Western Australia. The distributional shift briefly accelerated after the 2010/2011 MHW, further implicating temperature as the key driver.
Multiple lines of evidence suggest the range shift has primarily occurred via changes in spawning success by resident fish in each area (i.e., self-recruitment). There is no evidence of alongshore migration by adults of this species, including during the MHW, and the alongshore dispersal of larvae is probably very limited in most years. Unprecedented conditions during the MHW could have resulted in some atypical larval dispersal, and this may have also contributed to the accelerated range shift.
Sillago schomburgkii appears to spawn within a temperature range of approximately 20–26°C. Temperatures are typically in this range for several months in northern areas, but only briefly during the height of summer in southern areas. Being a multiple batch spawner, the length of the spawning period is a key factor determining the annual reproductive output of S. schomburgkii. During the 2010/2011 MHW, ocean temperatures were up to 5°C above average in some nearshore areas (Feng et al., 2013). As a consequence, it is likely that the spawning period was reduced in the north but extended in the south, which would explain the recruitment trends observed in each area. The age composition and fishery catch rates examined in this study provided evidence of exceptionally strong recruitment by the 2010/2011 year class in southern areas, but not in northern areas.
The dramatic response of S. schomburgkii to the 2010/2011 MHW focused attention on the significant, yet previously unrecognized, distributional shift by this species. It also helped to elucidate the mechanism by which this shift is occurring. The insights gained about the effects of ongoing ocean warming on S. schomburgkii have important implications for the commercial and recreational fisheries that harvest this valuable species. In future, fisheries at lower latitudes are expected to suffer a decline in availability of this species while those at higher latitudes (32–35°S) are expected to benefit from increasing availability, although gains on the south coast could be negated by the loss of critical estuarine habitats.
Data Availability
The datasets generated for this study are available on request to the corresponding author.
Author Contributions
KS conceived the study, prepared the manuscript, and analyzed the data and aged fish. CD and JB collected the fish and conducted the laboratory processing and ageing of fish.
Conflict of Interest Statement
The authors declare that the research was conducted in the absence of any commercial or financial relationships that could be construed as a potential conflict of interest.
Footnotes
- ^ https://www.esrl.noaa.gov/psd/data/gridded/data.noaa.oisst.v2.highres.html
- ^ https://www.busseltonjetty.com.au
References
Anonymous. (1999). Management Directions for Western Australia’s Estuarine and Marine Embayment Fisheries - A Strategic Approach to Management. Fisheries Management Paper No. 131. Perth, WA: Fisheries Department of Western Australia.
Arias-Ortiz, A., Serrano, O., Masqué, P., Lavery, P. S., Mueller, U., Kendrick, G. A., et al. (2018). A marine heatwave drives massive losses from the world’s largest seagrass carbon stocks. Nat. Clim. Change 8:338. doi: 10.1038/s41558-018-0096-y
Baldock, J., Bancroft, K. P., Williams, M., Shedrawi, G., and Field, S. (2014). Accurately estimating local water temperature from remotely sensed satellite sea surface temperature: a near real-time monitoring tool for marine protected areas. Ocean Coast. Manag. 96, 73–81. doi: 10.1016/j.ocecoaman.2014.05.007
Bell, J., Cheung, W., De Silva, S., Gasalla, M., Frusher, S., Hobday, A., et al. (2016). “Chapter 4.5: Impacts and effects of ocean warming on the contributions of fisheries and aquaculture to food security,” in Explaining Ocean Warming: Causes, Scale, Effects and Consequences, eds D. Laffoley and J. M. Baxter (Gland: International Union for Conservation of Nature and Natural Resources (IUCN)), 409–437.
Benthuysen, J., Feng, M., and Zhong, L. (2014). Spatial patterns of warming off Western Australia during the 2011 Ningaloo Niño: quantifying impacts of remote and local forcing. Cont. Shelf Res. 91, 232–246. doi: 10.1016/j.csr.2014.09.014
Berry, O., England, P., Fairclough, D., Jackson, G., and Greenwood, J. I. M. (2012). Microsatellite DNA analysis and hydrodynamic modelling reveal the extent of larval transport and gene flow between management zones in an exploited marine fish (Glaucosoma hebraicum). Fish. Oceanogr. 21, 243–254. doi: 10.1111/j.1365-2419.2012.00623.x
Brearley, A. (2005). Ernest Hodgkin’s Swanland. Estuaries and Coastal Lagoons of South-western Australia. Crawley, WA: University of Western Australia Press.
Brearley, A. (2013). Revisiting the Blackwood River and the Hardy Inlet, 40 Years of Change. An Environmental Review of the Blackwood River estuary Western Australia 1974–2010. Perth: Ernest Hodgkin Trust for Estuary Education and Research.
Breheny, N. B., Beckley, L. E., and Wakefield, C. B. (2012). Ichthyoplankton assemblages associated with pink snapper (Pagrus auratus) spawning aggregations in coastal embayments of southwestern Australia. J. R. Soc. West. Aust. 95, 103–114.
Brown, J., Dowling, C., Hesp, A., Smith, K., and Molony, B. (2013). Status of Nearshore finfish Stocks in Southwestern Western Australia. Part 3: Whiting (Sillaginidae). Fisheries Research Report No. 248. Perth, WA: Department of Fisheries.
Caputi, N. (1976). Creel census of amateur line fishermen in the Blackwood River Estuary, Western Australia, during 1974-75. Mar. Freshw. Res. 27, 583–593. doi: 10.1071/MF9760583
Caputi, N., de Lestang, S., Feng, M., and Jackson, G. (2017). Management adaptation to climate change effect on fisheries in Western Australia. Clim. Change Impacts Fish. Aquacult. 2, 603–638. doi: 10.1002/9781119154051.ch18
Caputi, N., Jackson, G., and Pearce, A. (2014). The Marine Heat Wave off Western Australia During the Summer of 2010/11 – 2 Years on. Fisheries Research Report No. 250. Perth, WA: Department of Fisheries.
Cheung, W. W. L., Watson, R., and Pauly, D. (2013). Signature of ocean warming in global fisheries catch. Nature 497, 365–368. doi: 10.1038/nature12156
Coulson, P. G. (2003). Comparisons Between the Biology of Two Species of Whiting (Sillaginidae) in Shark Bay, Western Australia. Honours thesis, Murdoch University, Perth.
Coulson, P. G., Hesp, S. A., Potter, I. C., and Hall, N. G. (2005). Comparisons between the biology of two co-occurring species of whiting (Sillaginidae) in a large marine embayment. Environ. Biol. Fishes 73, 125–139. doi: 10.1007/s10641-004-4568-8
Cure, K., Hobbs, J. P. A., and Harvey, E. S. (2015). High recruitment associated with increased sea temperatures towards the southern range edge of a Western Australian endemic reef fish Choerodon rubescens (family Labridae). Environ. Biol. Fishes 98, 1059–1067. doi: 10.1007/s10641-014-0339-3
Cure, K., Hobbs, J. P. A., Langlois, T. J., Abdo, D. A., Bennett, S., and Harvey, E. S. (2018). Distributional responses to marine heat waves: insights from length frequencies across the geographic range of the endemic reef fish Choerodon rubescens. Mar. Biol. 165:1. doi: 10.1007/s00227-017-3259-x
Cure, K., Thomas, L., Hobbs, J. P. A., Fairclough, D. V., and Kennington, W. J. (2017). Genomic signatures of local adaptation reveal source-sink dynamics in a high gene flow fish species. Sci. Rep. 7:8618. doi: 10.1038/s41598-017-09224-y
Depczynski, M., Gilmour, J. P., Ridgway, T., Barnes, H., Heyward, A. J., Holmes, T. H., et al. (2013). Bleaching, coral mortality and subsequent survivorship on a West Australian fringing reef. Coral Reefs 32, 233–238. doi: 10.1007/s00338-012-0974-0
Dulvy, N. K., Rogers, S. I., Jennings, S., Stelzenmüller, V., Dye, S. R., and Skjoldal, H. R. (2008). Climate change and deepening of the North Sea fish assemblage: a biotic indicator of warming seas. J. Appl. Ecol. 45, 1029–1039. doi: 10.1111/j.1365-2664.2008.01488.x
Fahrner, C. K., and Pattiaratchi, C. B. (1995). “The Physical Oceanography of Geographe Bay, Western Australia”. in Geographe Bay Summary Report, Wastewater 2040 Strategy for the South-West Region. Perth, WA: Water Authority of Western Australia, 3–12.
Feng, M., McPhaden, M. J., Xie, S. P., and Hafner, J. (2013). La Niña forces unprecedented Leeuwin Current warming in 2011. Nat. Sci. Rep. 3:1277. doi: 10.1038/srep01277
Feng, M., Meyers, G., Pearce, A., and Wijffels, S. (2003). Annual and interannual variations of the Leeuwin Current at 32 S. J. Geophys. Res. Oceans 108, 19–21. doi: 10.1029/2002JC001763
Feng, M., Slawinski, D., Beckley, L. E., and Keesing, J. K. (2010). Retention and dispersal of shelf waters influenced by interactions of ocean boundary current and coastal geography. Mar. Freshw. Res. 61, 1259–1267. doi: 10.1071/MF09275
Ferguson, G. (1999). Yellow fin whiting (Sillago schomburgkii). November 1999. South Australian Fisheries Assessment Series 99/10. Adelaide, SA: SARDI.
Ferguson, G. (2000). Yellow fin whiting (Sillago schomburgkii). Fishery Assessment Report to PIRSA for the Marine Scalefish Fishery Management Committee. South Australian Fisheries Assessment Series Report No. 00/10. Adelaide, SA: SARDI.
Fossheim, M., Primicerio, R., Johannesen, E., Ingvaldsen, R. B., Aschan, M. M., and Dolgov, A. V. (2015). Recent warming leads to a rapid borealization of fish communities in the Arctic. Nat. Clim. Change 5:673. doi: 10.1038/nclimate2647
Foster, T., Short, J. A., Falter, J. L., Ross, C., and McCulloch, M. T. (2014). Reduced calcification in Western Australian corals during anomalously high summer water temperatures. J. Exp. Mar. Biol. Ecol. 461, 133–143. doi: 10.1016/j.jembe.2014.07.014
Frölicher, T. L., and Laufkötter, C. (2018). Emerging risks from marine heat waves. Nat. Commun. 9:650. doi: 10.1038/s41467-018-03163-6
Gallop, S. L., Verspecht, F., and Pattiaratchi, C. B. (2012). Sea breezes drive currents on the inner continental shelf off southwest Western Australia. Ocean Dyn. 62, 569–583. doi: 10.1007/s10236-011-0515-3
Gaynor, A., Kendrick, A., and Westera, M. (2008). An Oral History of Fishing and Diving in the Capes Region of South-West Western Australia. Perth, WA: The University of Western Australia.
Hallett, C. S., Hobday, A. J., Tweedley, J. R., Thompson, P. A., McMahon, K., and Valesini, F. J. (2018). Observed and predicted impacts of climate change on the estuaries of south-western Australia, a Mediterranean climate region. Reg. Environ. Change 18, 1357–1373. doi: 10.1007/s10113-017-1264-8
Hotta, K., Tamura, M., Watanabe, T., Nakamura, Y., Adachi, S., and Yamauchi, K. (2001). Changes in spawning characteristics of Japanese whiting Sillago japonica under control of temperature. Fish. Sci. 67, 1111–1118. doi: 10.1046/j.1444-2906.2001.00368.x
Hotta, K., Watanabe, T., Kishida, C., Nakamura, Y., Ohkubo, N., Matsubara, T., et al. (2003). Seasonality of serum levels of vitellogenin in male Japanese whiting, Sillago japonica, reared under natural temperature and photoperiod. Fish. Sci. 69, 555–562. doi: 10.1046/j.1444-2906.2003.00656.x
Hutchins, B., and Swainston, R. (1986). Sea Fishes of Southern Australia: Complete Field Guide for Anglers and Divers. Perth, WA: Swainston Publishing.
Hyndes, G. A., and Potter, I. C. (1997). Age, growth and reproduction of Sillago schomburgkii in south-western Australian, nearshore waters and comparisons of life history styles of a suite of Sillago species. Environ. Biol. Fishes 49, 435–447. doi: 10.1023/A:1007357410143
Hyndes, G. A., Potter, I. C., and Lenanton, R. C. (1996). Habitat partitioning by whiting species (Sillaginidae) in coastal waters. Environ. Biol. Fishes 45, 21–40. doi: 10.1007/BF00000625
Kelsey, P., Hall, J., Kretschmer, P., Quinton, B., and Shakya, D. (2011). Hydrological and Nutrient Modelling of the Peel-Harvey catchment. Water Science Technical Series, Report no. 33. Perth, WA: Department of Water.
Kendall, B. W., and Gray, C. A. (2009). Reproduction, age and growth of Sillago maculata in south-eastern Australia. J. Appl. Ichthyol. 25, 529–536. doi: 10.1111/j.1439-0426.2009.01252.x
Last, P. R., White, W. T., Gledhill, D. C., Hobday, A. J., Brown, R., Edgar, G. J., et al. (2011). Long-term shifts in abundance and distribution of a temperate fish fauna: a response to climate change and fishing practices. Glob. Ecol. Biogeogr. 20, 58–72. doi: 10.1111/j.1466-8238.2010.00575.x
Lee, C. S. (1981). Factors affecting egg characteristics in the fish Sillago sihama. Mar. Ecol. Prog. Ser. 4, 361–363. doi: 10.3354/meps004361
Lee, C. S., and Hirano, R. (1985). Effects of water temperature and photoperiod on the spawning cycle of the sand borer, Sillago sihama. Prog. Fish Cult. 47, 225–230 doi: 10.1577/1548-8640(1985)47<225:EOWTAP>2.0.CO;2.
Lenanton, R. C. J. (1970). The Biology of the Commercially Fished Whiting (Sillago spp.) in Shark Bay, Western Australia. Perth, WA: University of Western Australia. M.Sc. Thesis.
Lenanton, R. C. J. (1977). Aspects of the Ecology of Fish and Commercial Crustaceans of the Blackwood River Estuary Western Australia. Fisheries Research Bulletin 19. Perth, WA: Western Australian Department of Fisheries and Fauna.
Lenanton, R. C. J. (1984). The Commercial Fisheries of Temperate Western Australian Estuaries : Early Settlement to 1975. Report No. 62. Perth, WA: Department of Fisheries and Wildlife.
Lenanton, R. C. J., Dowling, C. E., Smith, K. A., Fairclough, D. V., and Jackson, G. (2017). Potential influence of a marine heatwave on range extensions of tropical fishes in the eastern Indian Ocean - Invaluable contributions from amateur observers. Reg. Stud. Mar. Sci. 13, 19–31. doi: 10.1016/j.rsma.2017.03.005
Lenanton, R. C. J., Loneragan, N. R., and Potter, I. C. (1985). Blue-green algal blooms and the commercial fishery of a large Australian estuary. Mar. Pollut. Bull. 16, 477–482. doi: 10.1016/0025-326X(85)90380-7
Lima, F. P., and Wethey, D. S. (2012). Three decades of high-resolution coastal sea surface temperatures reveal more than warming. Nat. Commun. 3:704. doi: 10.1038/ncomms1713
Lowerre-Barbieri, S. K., Ganias, K., Saborido-Rey, F., Murua, H., and Hunter, J. R. (2011). Reproductive timing in marine fishes: variability, temporal scales, and methods. Mar. Coast. Fish. 3, 71–91. doi: 10.1080/19425120.2011.556932
Maceina, M. J. (1997). Simple application of using residuals from catch-curve regressions to assess year-class strength in fish. Fish. Res. 32, 115–121. doi: 10.1016/S0165-7836(97)00051-9
Madin, E. M., Ban, N. C., Doubleday, Z. A., Holmes, T. H., Pecl, G. T., and Smith, F. (2012). Socio-economic and management implications of range-shifting species in marine systems. Glob. Environ. Change 22, 137–146. doi: 10.1016/j.gloenvcha.2011.10.008
Mills, K. E., Pershing, A. J., Brown, C. J., Chen, Y., Chiang, F. S., Holland, D. S., et al. (2013). Fisheries management in a changing climate: lessons from the 2012 ocean heat wave in the Northwest Atlantic. Oceanography 26, 191–195.
Moore, J. A., Bellchambers, L. M., Depczynski, M. R., Evans, R. D., Evans, S. N., Field, S. N., et al. (2012). Unprecedented mass bleaching and loss of coral across 12 of latitude in Western Australia in 2010–11. PLoS One 7:e51807. doi: 10.1371/journal.pone.0051807
Nahas, E. L., Jackson, G., Pattiaratchi, C. B., and Ivey, G. N. (2003). Hydrodynamic modelling of snapper Pagrus auratus egg and larval dispersal in Shark Bay, Western Australia: reproductive isolation at a fine spatial scale. Mar. Ecol. Prog. Ser. 265, 213–226. doi: 10.3354/meps265213
Nye, J. A., Link, J. S., Hare, J. A., and Overholtz, W. J. (2009). Changing spatial distribution of fish stocks in relation to climate and population size on the Northeast United States continental shelf. Mar. Ecol. Prog. Ser. 393, 111–129. doi: 10.3354/meps08220
Oliver, E. C., Donat, M. G., Burrows, M. T., Moore, P. J., Smale, D. A., Alexander, L. V., et al. (2018). Longer and more frequent marine heatwaves over the past century. Nat. Commun. 9:1324. doi: 10.1038/s41467-018-03732-9
Payne, N. L., Smith, J. A., van der Meulen, D. E., Taylor, M. D., Watanabe, Y. Y., Takahashi, A., et al. (2016). Temperature dependence of fish performance in the wild: links with species biogeography and physiological thermal tolerance. Funct. Ecol. 30, 903–912. doi: 10.1111/1365-2435.12618
Pearce, A., and Feng, M. (2007). Observations of warming on the Western Australian continental shelf. Mar. Freshw. Res. 58, 914–920. doi: 10.1071/MF07082
Pearce, A., Hutchins, B., Hoschke, A., and Fearns, P. (2016). Record high damselfish recruitment at Rottnest Island, Western Australia, and the potential for climate-induced range extension. Reg. Stud. Mar. Sci. 8, 77–88. doi: 10.1016/j.rsma.2016.09.009
Pearce, A., Lenanton, R., Jackson, G., Moore, J., Feng, M., and Gaughan, D. (2011). The “Marine Heat Wave” off Western Australia During the Summer of 2010/11. Fisheries Research Report No. 222. Perth, WA: Department of Fisheries.
Pearce, A., and Pattiaratchi, C. (1999). The capes current: a summer countercurrent flowing past Cape Leeuwin and Cape Naturaliste, Western Australia. Cont. Shelf Res. 19, 401–420. doi: 10.1016/S0278-4343(98)00089-2
Pearce, A. F., and Feng, M. (2013). The rise and fall of the “marine heat wave” off Western Australia during the summer of 2010/2011. J. Mar. Syst. 111, 139–156. doi: 10.1016/j.jmarsys.2012.10.009
Perry, A. L., Low, P. J., Ellis, J. R., and Reynolds, J. D. (2005). Climate change and distribution shifts in marine fishes. Science 308, 912–915. doi: 10.1126/science.1111322
Peters, W. C. H. (1864). Über Einige Neue Säugethiere, Amphibien und Fische. Monatsberichte der Kniglichen Preussischen (Berlin: Akademie der Wissenschaften zu Berlin), 381–399.
Pinsky, M. L., and Fogarty, M. (2012). Lagged social-ecological responses to climate and range shifts in fisheries. Clim. Change 115, 883–891. doi: 10.1007/s10584-012-0599-x
Pinsky, M. L., Worm, B., Fogarty, M. J., Sarmiento, J. L., and Levin, S. A. (2013). Marine taxa track local climate velocities. Science 341, 1239–1242. doi: 10.1126/science.1239352
Poloczanska, E. S., Burrows, M. T., Brown, C. J., García Molinos, J., Halpern, B. S., Hoegh-Guldberg, O., et al. (2016). Responses of marine organisms to climate change across oceans. Front. Mar. Sci. 3:62. doi: 10.3389/fmars.2016.00062
Potter, I. C., Loneragan, N. R., Lenanton, R. C. J., and Chrystal, P. J. (1983). Blue-green algae and fish population changes in a eutrophic estuary. Mar. Pollut. Bull. 14, 228–233. doi: 10.1016/0025-326X(83)90257-6
Prior, S. P., and Beckley, L. E. (2007). Characteristics of recreational anglers in the Blackwood Estuary, a popular tourist destination in southwestern Australia. Tour. Mar. Environ. 4, 15–28. doi: 10.3727/154427307784835679
Rice, J. C., and Garcia, S. M. (2011). Fisheries, food security, climate change, and biodiversity: characteristics of the sector and perspectives on emerging issues. ICES J. Mar. Sci. 68, 1343–1353. doi: 10.1093/icesjms/fsr041
Ruiz-Montoya, L., and Lowe, R. J. (2014). Summer circulation dynamics within the Perth coastal waters of southwestern Australia. Cont. Shelf Res. 77, 81–95. doi: 10.1016/j.csr.2014.01.022
Smith, R. L., Huyer, A., Godfrey, J. S., and Church, J. A. (1991). The Leeuwin current off western Australia, 1986–1987. J. Phys. Oceanogr. 21, 323–345. doi: 10.1175/1520-0485(1991)021<0323:tlcowa>2.0.co;2
Steer, M. A., Fowler, A. J., McGarvey, R., Feenstra, J., Smart, J., Rogers, P. J., et al. (2018). Assessment of the South Australian Marine Scalefish Fishery in 2017. Report to PIRSA Fisheries and Aquaculture. SARDI Publication No. F2017/000427-2. SARDI Research Report Series No. 1002. Adelaide: South Australian Research and Development Institute (Aquatic Sciences).
Tongnunui, P., Sano, M., and Kurokura, H. (2006). Reproductive biology of two sillaginid fishes, Sillago sihama and S. aeolus, in tropical coastal waters of Thailand. La Mer. 44, 1–16.
Valesini, F. J., Potter, I. C., Platell, M. E., and Hyndes, G. A. (1997). Ichthyofaunas of a temperate estuary and adjacent marine embayment. Implications regarding choice of nursery area and influence of environmental changes. Mar. Biol. 128, 317–328. doi: 10.1007/s002270050097
Wang, N., Teletchea, F., Kestemont, P., Milla, S., and Fontaine, P. (2010). Photothermal control of the reproductive cycle in temperate fishes. Rev. Aquacult. 2, 209–222. doi: 10.1111/j.1753-5131.2010.01037.x
Wernberg, T., Bennett, S., Babcock, R. C., De Bettignies, T., Cure, K., Depczynski, M., et al. (2016). Climate-driven regime shift of a temperate marine ecosystem. Science 353, 169–172. doi: 10.1126/science.aad8745
Wernberg, T., Smale, D. A., Tuya, F., Thomsen, M. S., Langlois, T. J., De Bettignies, T., et al. (2013). An extreme climatic event alters marine ecosystem structure in a global biodiversity hotspot. Nat. Clim. Change 3:78. doi: 10.1038/nclimate1627
White, K. S. (2012). Hardy Inlet Water Quality Improvement Plan: Stage One - the Scott River Catchment. Available at: https://www.water.wa.gov.au/__data/assets/pdf_file/0012/3261/103163.pdf (accessed July 9, 2019).
Woo, M., Pattiaratchi, C., and Schroeder, W. (2006). Summer surface circulation along the Gascoyne continental shelf, Western Australia. Cont. Shelf Res. 26, 132–152. doi: 10.1016/j.csr.2005.07.007
Keywords: yellowfin whiting, ocean warming, fisheries impact, spawning duration, Western Australia, Peel-Harvey
Citation: Smith KA, Dowling CE and Brown J (2019) Simmered Then Boiled: Multi-Decadal Poleward Shift in Distribution by a Temperate Fish Accelerates During Marine Heatwave. Front. Mar. Sci. 6:407. doi: 10.3389/fmars.2019.00407
Received: 14 April 2019; Accepted: 02 July 2019;
Published: 17 July 2019.
Edited by:
Thomas Wernberg, The University of Western Australia, AustraliaReviewed by:
David John Booth, University of Technology Sydney, AustraliaKatherine Cure, Australian Institute of Marine Science (AIMS), Australia
Copyright © 2019 Smith, Dowling and Brown. This is an open-access article distributed under the terms of the Creative Commons Attribution License (CC BY). The use, distribution or reproduction in other forums is permitted, provided the original author(s) and the copyright owner(s) are credited and that the original publication in this journal is cited, in accordance with accepted academic practice. No use, distribution or reproduction is permitted which does not comply with these terms.
*Correspondence: Kimberley A. Smith, a2ltLnNtaXRoQGRwaXJkLndhLmdvdi5hdQ==