- 1Department of Bioscience, Arctic Research Centre, Aarhus University, Aarhus, Denmark
- 2Department of Bioscience, Aarhus University, Silkeborg, Denmark
- 3Department of Bioscience, Aarhus University, Roskilde, Denmark
- 4King Abdullah University of Science and Technology, Red Sea Research Center, and Computational Bioscience Research Center, Thuwal, Saudi Arabia
- 5Tønder Kommune, Tønder, Denmark
Until recently, Arctic kelp forests were so understudied that they did not even appear in global kelp forest maps. An increasing focus on Arctic coastal ecosystems has documented extended kelp forests along Arctic coastlines but the distribution of the forests is still not well documented and opens up for surprises. Here, we report kelp depth limits deeper than 61 m (median: 38.4 m, 10–90% percentiles: 10.8–54.4 m) in the Disko Bay region, Greenland, at latitudes of 67–70°N. We compiled, for comparison, existing records of kelp depth limits in high latitude (50°N) regions (median: 17.7 m, 10–90% percentiles: 9.0–38.1 m), which underline that the Disko Bay kelps hold a depth-record for this region. The deepest kelps were located at offshore sites beyond the Disko Island and the main coast. The clear waters offshore with euphotic zones (1% of surface irradiance) extending to 67 m depth provide, along with deep rocky seafloors and low density of sea urchins, the basis for these deep kelps. The sites were ice-covered for 77–133 days year-1, which is beyond the length of the polar night (30–60 days year-1) in the region, suggesting a potential for further depth penetration of kelp forests in a future with longer open water periods and more light potentially reaching the seafloor.
Introduction
Kelp forests are widely distributed along coastlines where rocky substratum and cool, clear, and relatively nutrient-rich waters fulfill their growth requirements and where sea urchins do not overgraze them (e.g., Steneck et al., 2002). In spite of their large ecological relevance in terms of habitat provision, productivity and carbon cycling (e.g., Teagle et al., 2017; Wernberg et al., 2019), kelp distribution is still widely unexplored, particularly in Arctic regions, which are often ignored in global reviews and maps of kelp forest ecosystems (Steneck et al., 2002; Graham et al., 2007; Krumhansl et al., 2016). Research efforts on kelp forests have focused in mid-latitude regions (30–60°) on both hemispheres, with much fewer observations from higher latitudes where kelps until recently were considered to be unable to attain forest status (Steneck et al., 2002). Only very recently, Greenland kelp forests appear in maps of global kelp distribution (Filbee-Dexter et al., 2019; Wernberg et al., 2019), despite the huge suitable habitat that Greenland offers to support kelps, which may potentially grow along much of the 44,000 km, 12% of the global, shoreline (Krause-Jensen and Duarte, 2014). While kelps are generally confined to temperate and Arctic regions, additional extended kelp forest habitats exist at 30–200 m depth in tropical regions, where the shallow zones are too warm and nutrient-limited to support kelp growth but where the deeper zones fulfill the habitat requirements of kelps (Graham et al., 2007; Assis et al., 2018).
Temperate and Arctic kelp forests typically extend down to maximum water depths of 30–40 m (Wernberg et al., 2019) with lack of light preventing deeper forests, as relatively high nutrient levels in kelp forest regions fuel considerable phytoplankton productivity, leading to relatively high light extinction coefficients (Lüning, 1990; Steneck et al., 2002; Graham et al., 2007). In line with this, a recent compilation showed, e.g., Canadian, Alaskan, and Norwegian kelp forests down to around 20–30 m (Filbee-Dexter et al., 2019). Arctic kelp forests in Svalbard also grow down to 18–20 m depth (Bartsch et al., 2016; Hop et al., 2016), with occurrences deeper than 25 m in some fjords (Tatarek et al., 2012; Smoła et al., 2017), along relatively protected coastlines and fjords of Greenland kelp grow down to ca. 15–40 m depth (Borum et al., 2002; Krause-Jensen et al., 2012) with the deepest occurrences and densest forests in areas with long open-water periods (Krause-Jensen et al., 2012), and along Russian coasts there are reports of kelps down to 15 m depth (Shoshina et al., 2016) as well as several cases of depth limits shallower than 10 m (Myagkov, 1975; Plotkin et al., 2005; Malavenda and Malavenda, 2012). However, the vast majority of studies on Arctic kelps represents fjords and bays, which experience stronger light attenuation than offshore and open coastal stretches due to planktonic production in combination with turbidity caused by river and glacier runoff (Murray et al., 2015). Hence, there is a need to balance available estimates with those derived from offshore, open coastal Arctic habitats, where the underwater light environment and deep rocky seafloors are expected to allow deeper penetration of kelp forests.
Here, we report Greenland kelps extending to maximum depths deeper than 61 m along offshore and open coastlines of the Disko Bay region (W. Greenland) at latitudes of 67–70°N. We compare these observations with a compilation of existing records of kelp depth limits from the temperate/Arctic region at latitudes >50°N.
Materials and Methods
New Observations of Kelp Depth Limits in the Disko Bay Area, Greenland
The vertical extension of kelp forests was studied during a cruise from Sisimiut to Nuussuaq in the Disko Bay, Greenland, in August/September 2009 (Hansen et al., 2013). A total of 33 transect lines, representing 11 sites with 3 transects each, were surveyed by a underwater video systems equipped with a depth sensor (UV-video system JH-Teknik Aps, Denmark), transmitting camera depth and surface positions from the ships navigation system to each recorded video frame. Four of the sites (Hareøen, Kronprinsens Ejland, Hunde Ejland, and Rifkol) represent islands located offshore from the Disko Island and the main coast. The remaining seven sites represented relatively open coastlines including bays and fjords along the western and southern coastline of the Disko Island, and the mainland north and south of the Disko Island (Figure 1). At each site, the three transect lines were, where possible, placed to represent different relative levels of exposure to wind and waves (visually assessed as protected, medium, exposed relative to the conditions at the specific site), which could affect, e.g., substratum characteristics. Three of the sites had solely exposed transects whereas the remaining eight sites allowed for comparison between exposure levels to test whether such small-scale variation affected the depth penetration of the kelp.
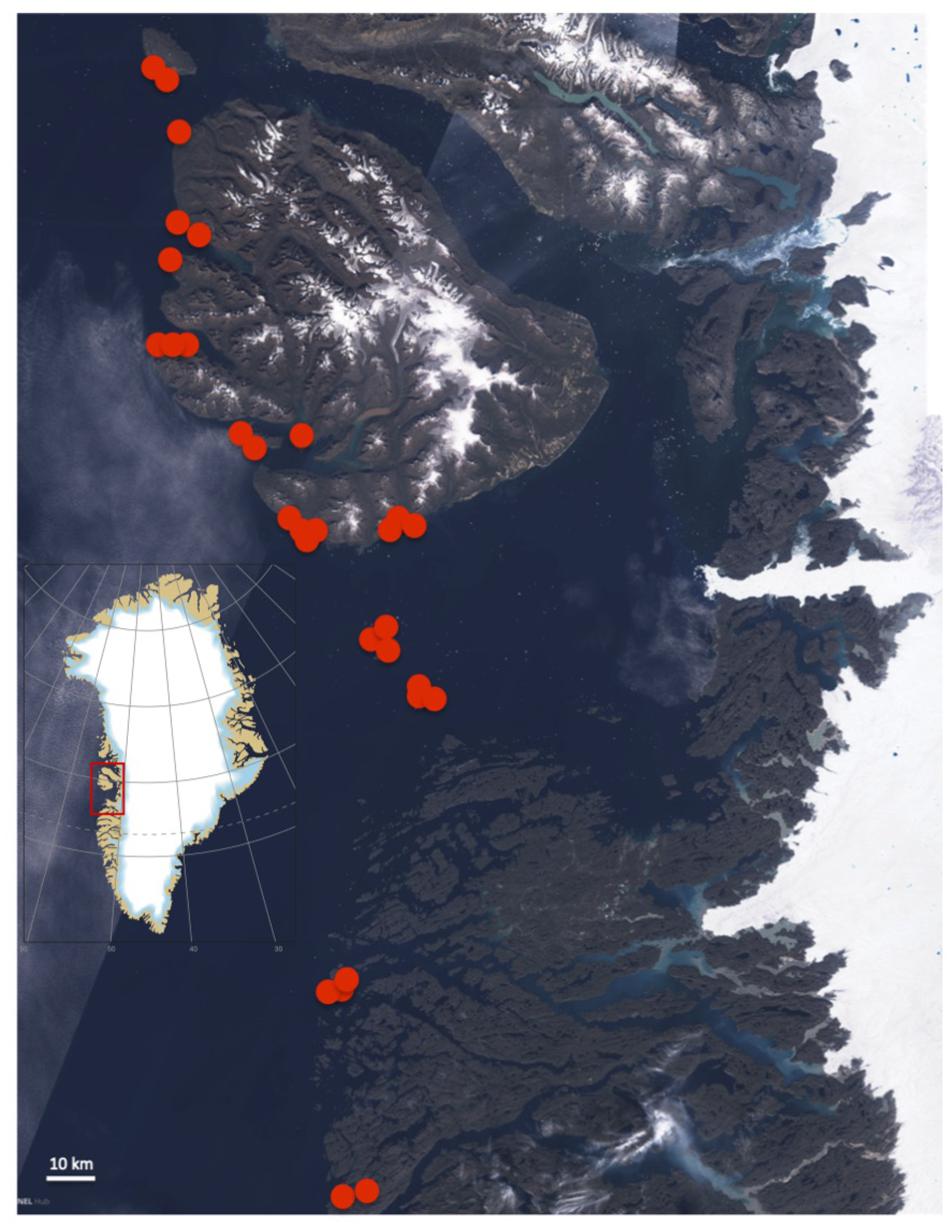
Figure 1. Map of stations representing this study’s observations of depth limits of Greenland kelp along the outer shores of the Disko Bay area, 67–70°N, on Greenland’s west coast. The satellite image shows plumes of turbid water in the inner parts of fjords and clear waters along the outer shores although some clouds cover part of the scene.
Each video recording encompassed the depth range from the shallow subtidal (typically 5–8 m depth) to the deepest occurrence of kelps, though in some cases kelps penetrated deeper than the deepest video images (61.4 m). The measured water depths were corrected for tidal level at the time of assessment based on tidal information from the nearest town1. The percentage kelp cover was estimated visually from the video frames within depth intervals of approximately 5 m. The kelp cover was estimated relative to the total area of the bottom irrespective of the presence of suitable substratum. The depth limit of kelps, here defined as the deepest occurrence of 1% cover, was identified, and we noted whether it was represented by Agarum clathratum Dumortier, for which the distinct holes in the blade made it easily distinguishable, or by either Saccharina latissima (Linnaeus) C. E. Lane, C. Mayes, Druehl and G. W. Saunders, S. longicruris (Bachelot de la Pylaie) Kuntze or Saccharina sp., for which a joint cover was estimated. The nature of the substratum (1 = rock/stones, 2 = mixed, 3 = sand) as well as the approximate density (# m-2) of sea urchins was equally assessed from the video frames along the depth gradients, and averaged for each transect. Data on sea ice cover representing each of the 11 sites was obtained from passive microwave imagery processed with the Enhanced NASA Team algorithm and archived and distributed by the National Snow and Ice Data Center; and the length of the ice-free period was calculated based on an algorithm (for details see Krause-Jensen et al., 2012).
Literature Compilation of Reported Depth Limits
We scanned the international literature for data on kelp depth limits in the high latitude (beyond 50°N) regions, extending the earlier compilations by Vadas and Steneck (1988), Gattuso et al. (2006), and Filbee-Dexter et al. (2019) for the Arctic. Among the additions are data from Greenland (Krause-Jensen et al., 2012), Iceland (Gunnarsson, 1991), and the Faroe Islands, located in the north Atlantic at about 62°N between Iceland and Norway (Bruntse et al., 1999).
Data Analysis
We compared the range and 10–90% percentiles of our observations of kelp depth limits from the Disko Bay area with our literature compilation and tested (one way ANOVA) for differences in mean depth limits among the data sets. For the Disko Bay data set, we further compared the range and 10–90% percentiles of observations of kelp depth limits from the offshore sites with those from the main land coast and the Disko Island coast and tested (one way ANOVA) for differences in mean depth limits among the data sets. For the eight sites where transect lines represented different exposure levels, we tested (paired t-test) for differences in depth limits between exposure levels (exposed vs. protected and medium-exposed). Average sea urchin cover and sediment composition at offshore vs. coastal sites were also tested for differences (one-way ANOVA, t-test). Analyses were performed using JMP version 13.0.
Results
The 33 observations of kelp depth limits from this study in the Disko Bay area represent a mean of 35.5 m (median 38.4 m) and 10–90% percentiles of 10.8–54.4 m (Figure 2). Three observations were deeper than 55 m with a maximum deeper than 61 m recorded at two sites, the small skerry islands “Kronprinsens Ejland” and “Hunde Ejland” off the coast of the Disko Island (Supplementary Table S1). The Disko Bay depth limits are considerably deeper than depth limits earlier reported for the high latitudes, which represented a mean level of only 18.4 m (median 17.7 m) and 10–90% percentiles of 9–31.8 m (Figure 2 and Supplementary Table S2). The difference in mean levels is significant (one way ANOVA, F-Ratio = 75.1; p < 0.0001). While export of kelps from shallow to deep waters do occur (e.g., Krause-Jensen and Duarte, 2016), we are confident that the depth limits reported here represent growing depths of the kelps. This is supported by the gradual decline in kelp cover recorded by underwater video along the depth along with a general decline in the size of the kelps near the depth limit.
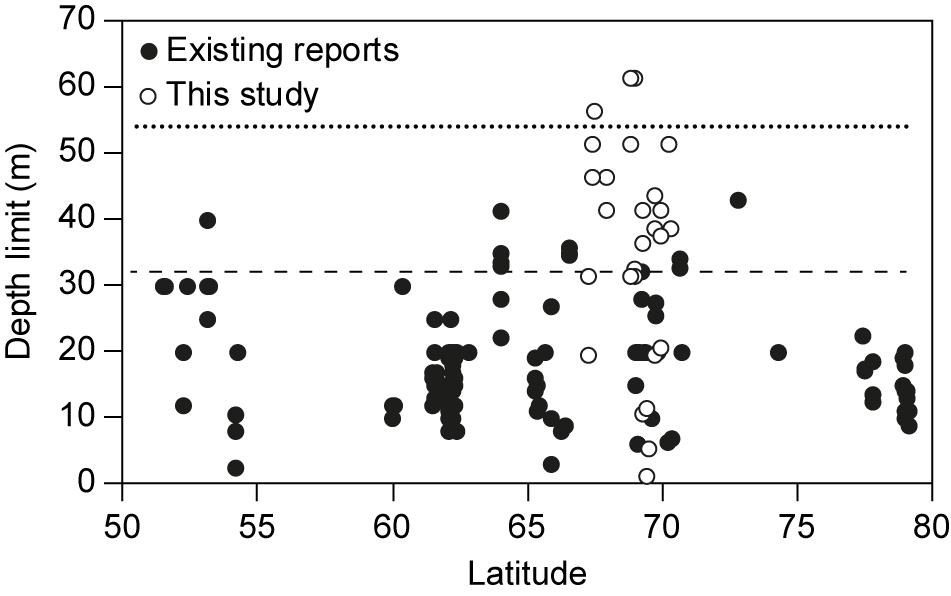
Figure 2. Reports of kelp depth limits from the Disko Bay region, Greenland at 67–70°N (this study, open circles) compared with a literature compilation of existing reports of depth limits from latitudes above 50°N (filled circles). The upper dotted line shows the 90% percentile (54.4 m) of the observations from the Disko Bay region while the lower dashed line shows the 90% percentile (31.8 m) of published reports.
A comparison among the 33 transects underlined that kelp forests grow markedly deeper at offshore sites (mean ± SE: 43.9 ± 3.1 and 10–90% percentiles of 31.5–60.4 m) than at sites located along the coast of the mainland and the Disko Island (mean ± SE: 30.7 ± 3.4 and 10–90% percentiles of 10.4–41.4 m, Figure 3). The difference is significant (one way ANOVA, F-Ratio = 6.75; p = 0.014) and is well in accord with the much clearer water offshore than along the coast as evidenced from satellite images of the region (Figure 1). The sites represented open water periods ranging from 232 to 288 days year-1. Across this narrow range, there was no relationship between the depth limit of kelps and the open water period (linear regression, p = 0.32). For sites where transects represented different exposure levels, depth limits did not depend on exposure as there was no indication that the linear relationship between depth limits at exposed vs. protected/medium exposed sites deviated from the 1:1 line (Supplementary Figure S1, paired t-test: t-ratio = -0.66719, DF = 7, prob < ltl = -0.664).
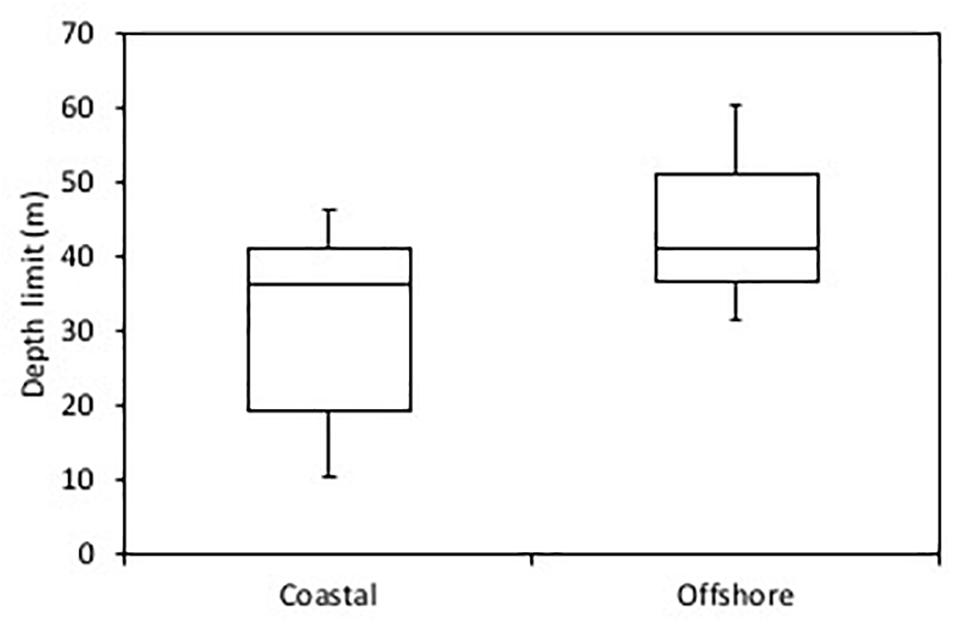
Figure 3. Depth limits of kelp forests from the Disko Bay region, Greenland at 67–70°N in offshore sites (right panel) as compared with coastal sites (left panel). The box represents 25–75% percentiles, the central line is the median and the whiskers represent 10–90% percentiles.
The three offshore sites with kelp depth limits deeper than 55 m all had no or low sea urchin density (Supplementary Figure S2). Conversely, the shallowest depth limits (1–11.4 m in the Disko Fjord) were associated with the highest occurrence of sea urchins (5–40 urchins m-2). However, low sea urchin density was no guarantee for deep penetration of kelps (Supplementary Figure S2). Still, sea urchin density was significantly lower in offshore sites (mean ± SE (min-max): 0.13 ± 0.1 (0–1) urchins m-2) as compared to coastal sites (mean ± SE (min-max): 5.5 ± 2.0 (0–40) urchins m-2) (t-test, t-ratio: -2.0; DF = 31, p(t < 1) = 0.027), which may contribute to the generally deeper depth limits offshore.
While suitable substrate at depth is also a requirement for deep depth limits, there was no significant relationship between depth limits and average substratum composition along the transect lines within the kelp distribution limits (Supplementary Figure S3, linear regression, R2 = 0.06, p = 0.17) or substratum type at the depth limit (one way ANOVA, F-ratio: 1.61, p = 0.22). Offshore sites were not characterized by significantly harder substratum than coastal sites within the depth ranges where kelps grew (One-way ANOVA, F-ratio: 2.18, p = 0.15). Still, e.g., at the coastal sites in the Disko Fjord and Laksebugten representing the shallowest depth limits, the substrate turned sandy already at shallow depths and at the offshore site Hunde Ejland, the two replicate transects supporting kelp depth limits down to 51 and 61 m had more rocky substrate than the third replicate transect where kelps penetrated solely to 31 m depth.
Along the vast majority of transects (30 out of 33 transects), A. clathratum was the deepest located kelp, sometimes in combination with other kelps (predominantly S. latissima and S. longicruris), while these other kelps alone only defined the depth limit in three cases.
Discussion
We documented that the deepest occurring kelps extend to record depths deeper than 61 m in the Disko Bay region at latitudes of 67–70°N. This is far deeper than previously reported depth limits for high latitude (beyond 50° N) regions (Figure 2). Earlier reports of kelp depth limits in Greenland were in the range of 12.6–43.6 m and predominantly represented relatively protected sites (Krause-Jensen et al., 2012), whereas the current dataset includes offshore sites and open coastal stretches. Indeed the data from the Disko Bay showed that the deepest kelp forests occurred at small islands isolated from the main coastlines, which are likely less influenced by high turbidity delivered by glacial run-off, resulting in clearer waters and less sedimentation of material on the rocky seafloors (Murray et al., 2015; Traiger and Konar, 2018). In addition to clearer waters, the reduced occurrence of sea urchins along with the presence of suitable substratum at depth likely support the deep penetration of kelps in the offshore regions, as also noted for, e.g., kelp forests in Iceland (Gunnarsson, 1991). In the Gulf of Maine, kelp forests offshore where also less affected by sea urchins, and recruitment limitation of sea urchins offshore was suggested as explanation (Witman and Lamb, 2018). A. clathratum shows marked resistance to sea urchin grazing (Gagnon et al., 2005) and is also a shade-tolerant species (Wernberg et al., 2019), which may explain why this species typically defined the depth limit.
Deeper kelp forests along open coasts relative to more inland locations have also been reported from the Faroe Islands with depth limits increasing from 12.3 ± 0.85 m (mean ± SE) in inner protected fjords, over 16.8 ± 1.2 m in medium exposed areas to 17.8 ± 0.9 m in the most exposed open settings (Bruntse et al., 1999). Lack of substrate often limits the depth distribution in the Faroe Islands where erosion coasts prevail. In Breidifjörður Bay, Iceland, depth limits increased from 11 to 15 m in inner, protected-medium exposed settings to 16–19 m in outer, exposed settings with clearer waters and with predominantly hard substratum and absence of sea urchins (Gunnarsson, 1991). Along 2,700 km of the littoral of Brittany, Derrien-Courtel et al. (2013) also reported depth limits varying from 1.6 to 32.2 m, increasing toward offshore, clearer sites. In Hornsund, Svalbard, kelps also grows deeper in the least turbid sites (Tatarek et al., 2012). In line with these observations, Traiger and Konar (2018) observed faster recruitment of kelps and more abundant kelps at oceanic sites than at glacial sites along a glacial gradient in Kachemak Bay, Alaska, and correlated this pattern with increased inorganic sedimentation rates at the glacial sites. Hence, there may be a general pattern of deeper depth limits toward more exposed, oceanic Arctic sites. The density and biomass of kelps and associated fish communities can also be considerably larger in offshore compared to coastal kelp forests (Witman and Lamb, 2018).
Kelp depth limits deeper than 61 m offshore in the Disko Bay area match well the extent of the euphotic zone [roughly defined as 1% of surface irradiance (SI)] reported to extend to a maximum of 67 m during summer in this region (Jensen et al., 1999). Depth limits extending to about 1% SI are in line with findings for kelps in general (Lüning and Dring, 1979; Lüning, 1990), with a range of 0.2–4.2% SI and absolute light levels of 10–559 mol photons year-1 at the depth limit (Gattuso et al., 2006, their Appendix D). Field-determined light requirements at the depth limit are available for, e.g., Greenland S. latissima (0.7% SI, 40 mol photons m-2 year-1, Borum et al., 2002), Icelandic kelps (0.6–1.9% SI, 34–108 mol photons m-2 year-1, Gunnarsson, 1991), Alaskan High Arctic Laminaria solidungula (down to 0.2% SI; 45 mol photons m-2 year-1, Dunton, 1990) and Laminaria hyperborea at Helgoland (0.7% SI, 70 mol photons m-2 year-1, Lüning and Dring, 1979). Low Arctic water temperature may be an advantage and a prerequisite for survival in the long dark period, as it keeps respiration costs low (Borum et al., 2002). The low Arctic temperatures could also be expected to facilitate deep penetration of kelps, but the presence of even deeper kelps in tropical regions (Graham et al., 2007; Assis et al., 2018) contradicts this explanation.
The Disko Bay is covered by sea ice in winter, which starts to break up in May, but as for most of the Arctic, spring sea ice coverage is decreasing (Sejr et al., 2007). On average, the bay is ice covered for 77–133 days year-1, which is beyond the length of the polar night (30–60 days year-1) in this region, suggesting a potential for more light penetrating to the seafloor on an annual basis as the open water period expands, given that water clarity remains high. Less sea-ice and resulting longer open water periods have been linked with deeper and more productive kelp forests along the Greenland coast on a spatial scale as well as over a decadal time series representing open water periods of varying length (Krause-Jensen et al., 2012). In Antarctica, similar patterns of colonization by kelp-like species (there are no Laminarian kelps in Antarctica) have followed the retraction of glaciers (Quartino et al., 2013; Deregibus et al., 2016). Depth limits of these Antarctic marine forests [compiled by Klöser et al. (1993)] with extremes of 90–100 m in Admiralty Bay (Zielinski, 1990) and even deeper in the Ross Sea (Zaneveld, 1966) confirm a large potential for depth colonization. The overall expectation is, therefore, that loss of sea ice at the poles will lead to both lateral and downward expansion and more productive kelp forests (Clark et al., 2013; Krause-Jensen and Duarte, 2014). Increased turbidity from melting glaciers may to some extent counteract the positive effect of melting sea ice on the light climate and lead to a shoreward shift of the zonation of algae with shallower depth limits (Bartsch et al., 2016; Hop et al., 2016). Hence, while kelp forests may overall expand poleward and toward deeper water in response to melting sea ice, large site-specific differences can be expected with shallower depth limits near melting glaciers.
The deep penetration of kelps reported here is probably not a unique phenomenon. Given the limited knowledge on Arctic seafloor habitats, further explorations may document similar hidden forests elsewhere in offshore clear waters where hard substratum is present and sea urchins do not prevail. Deep kelp penetration highlights the importance of benthic primary production for Arctic coastal ecosystems. With the extensive length of the Arctic coastline, exceeding the 407,680 km (34% of the world’s coastline) affected by the presence of permafrost (Lantuit et al., 2012), a deep penetration of kelps parallels a potentially huge area of kelp forest. Benthic primary producers (microphytobenthos and macroalgae combined) have a high capacity for low light acclimation, leading to examples of area-specific production rates of benthic producers exceeding pelagic production by a factor of two in coastal Greenland regions (Attard et al., 2016). For a specific fjord system (Young Sound, NE Greenland) benthic primary production contributed 34% of annual primary production (Glud and Rysgaard, 2007) and on a circum-Arctic scale, benthic primary production has been estimated to represent 26% of annual pelagic production (Attard et al., 2016). In addition to the 3D structure of kelp forests providing habitat and stimulating biodiversity, part of this primary production enters grazing and detrital food chains (Wernberg et al., 2019) and part of it is sequestered in oceanic carbon sinks (Krause-Jensen and Duarte, 2016). Offshore kelp forests, as reported here, may, due to the exposed settings, export a larger fraction of their biomass than protected kelp forests, subsidizing offshore benthic communities, and supporting carbon sequestration in offshore sedimentary basins.
Data Availability
All datasets generated for this study are included in the manuscript and/or the Supplementary Files.
Author Contributions
DK-J compiled the literature data, conducted the comparative analyses, and wrote the draft manuscript with support from MS and CD. AB, MR, and PC conducted the fieldwork with support from JH and SW in planning and reporting. GB contributed data from the Faroe Island. All authors contributed to improving the manuscript and approved its final version.
Funding
This study was a contribution to the Northeast Greenland Environmental Study Program, a collaboration between the Danish Centre for Environment and Energy (DCE, Aarhus University), the Greenland Institute of Natural Resources, and the Environmental Agency for Mineral Resource Activities of the Government of Greenland. Oil companies operating in Greenland are obliged to contribute to knowledge regarding environmental matters. The Strategic Environmental Impact Assessment and the background study program are funded under these commitments administered by the Mineral License and Safety Authority and the Environmental Agency for Mineral Resource Activities. The study was also a contribution to the Greenland Ecosystem Monitoring Program (http://g-e-m.dk/) and to the Biodiversa project MARFOR (FCT-BIODIVERSA/004/2015). DK-J received support from the Independent Research Fund Denmark (8021-00222 B, “CARMA”). MS received support from the EU Horizon2020 project INTAROS.
Conflict of Interest Statement
The authors declare that the research was conducted in the absence of any commercial or financial relationships that could be construed as a potential conflict of interest.
Acknowledgments
We are thankful to the late Poul Møller Pedersen who joined the data collection team in Greenland and contributed with his lifelong knowledge and expertise on Greenland macroalgae. We also thank Joao Rodrigues for computing the length of the ice-free period as reported in Krause-Jensen et al. (2012). Data from the Disko West area were collected as part of “Disko West strategic environmental impact assessment of hydrocarbon activities” supported by the Bureau of Minerals and Petroleum, Greenland Government.
Supplementary Material
The Supplementary Material for this article can be found online at: https://www.frontiersin.org/articles/10.3389/fmars.2019.00375/full#supplementary-material
Footnotes
References
Assis, J., Araújo, M. B., and Serrão, E. A. (2018). Projected climate changes threaten ancient refugia of kelp forests in the North Atlantic. Glob. Chang. Biol. 24, e55–e66. doi: 10.1111/gcb.13818
Attard, K. M., Hancke, K., Sejr, M. K., and Glud, R. N. (2016). Benthic primary production and mineralization in a High Arctic fjord: in situ assessments by aquatic eddy covariance. Mar. Ecol. Prog. Ser. 554, 35–50. doi: 10.3354/meps11780
Bartsch, I., Paar, M., Fredriksen, S., Schwanitz, M., Daniel, C., Hop, H., et al. (2016). Changes in kelp forest biomass and depth distribution in Kongsfjorden, Svalbard, between 1996–1998 and 2012–2014 reflect Arctic warming. Polar Biol. 39, 2021–2036. doi: 10.1007/s00300-015-1870-1
Borum, J., Pedersen, M., Krause-Jensen, D., Christensen, P., and Nielsen, K. (2002). Biomass, photosynthesis and growth of Laminaria saccharina in a high-arctic fjord. NE Greenland. Mar. Biol. 141, 11–19. doi: 10.1007/s00227-002-0806-9
Bruntse, G., Lein, T. E., and Nielsen, R. (1999). Marine Benthic Algae and Invertebrate Communities From the Shallow Waters of the Faroe Islands. A Baseline Study. Faroe Islands: Kaldbak Marine Biological Laboratory.
Clark, G. F., Stark, J. S., Johnston, E. L., Runcie, J. W., Goldsworthy, P. M., Raymond, B., et al. (2013). Light-driven tipping points in polar ecosystems. Glob. Chang. Biol. 19, 3749–3761. doi: 10.1111/gcb.12337
Deregibus, D., Quartino, M. L., Campana, G. L., Momo, F. R., Wiencke, C., and Zacher, K. (2016). Photosynthetic light requirements and vertical distribution of macroalgae in newly ice-free areas in Potter Cove, South Shetland Islands, Antarctica. Polar Biol. 39, 153–166. doi: 10.1007/s00300-015-1679-y
Derrien-Courtel, S., Le Gal, A., and Grall, J. (2013). Regional-scale analysis of subtidal rocky shore community. Helgol. Mar. Res. 67, 697–712. doi: 10.1007/s10152-013-0355-2
Dunton, K. H. (1990). Growth and production in Laminaria solidungula: relation to continuous underwater light levels in the Alaskan High Arctic. Mar. Biol. 106, 297–304. doi: 10.1007/bf01314813
Filbee-Dexter, K., Wernberg, T., Fredriksen, S., Norderhaug, K. M., and Pedersen, M. F. (2019). Arctic kelp forests: diversity, resilience and future. Glob. Planet Change 172, 1–14. doi: 10.1016/j.gloplacha.2018.09.005
Gagnon, P., Johnson, L. E., and Himmelman, J. H. (2005). Kelp patch dynamics in the face of intense herbivory: Stability of Agarum clathratum (Phaeophyta) stands and associated flora on urcin barrens. J. Phycol. 41, 498–505. doi: 10.1111/j.1529-8817.2005.00078.x
Gattuso, J. P., Gentili, B., Duarte, C. M., Kleypas, J. A., Middelburg, J. J., and Antoine, D. (2006). Light availability in the coastal ocean: impact on the distribution of benthic photosynthetic organisms and their contribution to primary production. Biogeoscience 3, 489–513. doi: 10.5194/bg-3-489-2006
Glud, R. N., and Rysgaard, S. (2007). Carbon cycling in Arctic marine ecosystems: case study young sound. Copenhagen: Danish Polar Center. Meddelelser om Grønland. Bioscience 58, 159–174.
Graham, M. H., Kinlan, B. P., Druehl, L. D., Garske, L. E., and Banks, S. (2007). Deep-water kelp refugia as potential hotspots of tropical marine diversity and productivity. Proc. Nat. Acad. Sci. U.S.A. 104, 16576–16580. doi: 10.1073/pnas.0704778104
Gunnarsson, K. (1991). Populations de Laminaria hyperborea et Laminaria digitata (Pheophycees) dans la baie de Breidifjordur, Island. J. Mar. Inst. Reykjavik 12:148.
Hansen, J. L. S., Hjorth, M., Rasmussen, M. B., Bruhn, A., Christensen, P. B., and Pedersen, P. M. (2013). “Mapping of macroalgae in the Disko West coastal zone,” in Disko West. A Strategic Environmental Impact Assessment of Hydrocarbon Activities, Vol. 71, eds D. Boertmann, A. Mosbech, D. Schiedek, and M. Dünweber (Aarhus: Aarhus University), 1–306.
Hop, H., Kovaltchouk, N. A., and Wiencke, C. (2016). Distribution of macroalgae in Kongsfjorden, Svalbard. Polar Biol. 39, 2037–2051. doi: 10.1007/s00300-016-2048-1
Jensen, H. M., Pedersen, L., Burmeister, A., and Winding Hansen, B. (1999). Pelagic primary production during summer along 65 to 72°N off West Greenland. Polar Biol. 21, 269–278. doi: 10.1007/s003000050362
Klöser, H., Ferreyra, G., Schloss, I., Mercuri, G., Laturnus, F., and Curtosi, A. (1993). Seasonal variation of algal growth conditions in sheltered Antarctic bays: the example of Potter Cove (King George Island, South Shetlands). J. Mar. Syst. 4, 289–301. doi: 10.1016/0924-7963(93)90025-H
Krause-Jensen, D., and Duarte, C. M. (2014). Expansion of vegetated coastal ecosystems in the future Arctic. Front. Mar. Sci. 1:77. doi: 10.3389/fmars.2014.00077
Krause-Jensen, D., and Duarte, C. M. (2016). Substantial role of macroalgae in marine carbon sequestration. Nat. Geosci. 9:737. doi: 10.1007/s13280-016-0849-7
Krause-Jensen, D., Marbà, N., Olesen, B., Sejr, M. K., Christensen, P. B., Rodrigues, J., et al. (2012). Seasonal sea ice cover as principal driver of spatial and temporal variation in depth extension and annual production of kelp in Greenland. Glob. Chang. Biol. 18, 2981–2994. doi: 10.1111/j.1365-2486.2012.02765.x
Krumhansl, K. A., Okamoto, D. K., Rassweiler, A., Novak, M., Bolton, J. J., Cavanaugh, K. C., et al. (2016). Global patterns of kelp forest change over the past half-century. Proc. Nat. Acad. Sci. U.S.A. 113, 13785–13790. doi: 10.1073/pnas.1606102113
Lantuit, H., Overduin, P. P., Couture, N., Wetterich, S., Aré, F., Atkinson, D., et al. (2012). The Arctic coastal dynamics database: a new classification scheme and statistics on Arctic permafrost coastlines. Est. Coasts. 35, 383–400. doi: 10.1007/s12237-010-9362-6
Lüning, K. (1990). Seaweeds. Their Environment, Biogeography, and Ecophysiology. New York, NY: Wiley.
Lüning, K., and Dring, M. J. (1979). Continuous underwater light measurement near Helgoland (North Sea) and its significance for characteristic light limits in the sublittoral region. Helgoländer wissenschaftliche Meeresuntersuchungen 32, 403–424. doi: 10.1007/bf02277985
Murray, C., Markager, S., Stedmon, C. A., Juul-Pedersen, T., Sejr, M. K., and Bruhn, A. (2015). The influence of glacial melt water on bio-optical properties in two contrasting Greenland fjords. Estuar. Coast. Shelf Sci. 163, 72–83. doi: 10.1016/j.ecss.2015.05.041
Myagkov, G. M. (1975). Composition, distribution and the seasonal dynamics of algal biomass in the Laminaria biocenosis of the bay of the White Sea USSR based on diving data. Bull. Leningr. State Univ. Ser. Biol. 3, 48–53.
Plotkin, A. S., Railkin, A. I., Gerasimova, E. I., Pimenov, A. Y., and Sipenkova, T. M. (2005). Subtidal underwater rock communities of the White Sea: Structure and interaction with bottom flow. Russ. J. Mar. Biol. 31, 335–343. doi: 10.1007/s11179-006-0001-9
Quartino, M. L., Deregibus, D., Campana, G. L., Latorre, G. E. J., and Momo, F. R. (2013). Evidence of macroalgal colonization on newly Ice-free areas following glacial retreat in Potter Cove (South Shetland Islands), Antarctica. PLoS One 8:e58223. doi: 10.1371/journal.pone.0058223
Sejr, M. K., Nielsen, T. G., Rysgaard, S., Risgaard-Petersen, N., Sturluson, M., and Blicher, M. E. (2007). Fate of pelagic organic carbon and importance of pelagic-benthic coupling in a shallow cove in Disko Bay, West Greenland. Mar. Ecol. Prog. Ser. 341, 75–88. doi: 10.3354/meps341075
Shoshina, E., Kapkov, V. I., and Belenikina, O. A. (2016). Ecological factors regulating growth of seaweeds in Arctic communities. Vestnik MSTU 19, 334–344. doi: 10.21443/1560-9278-2016-1/2-334-344
Smoła, Z. T., Tatarek, A., Wiktor, J. M., Wiktor, J. M., Kubiszyn, A., and Wêsławski, J. M. (2017). Primary producers and production in Hornsund and Kongsfjorden–comparison of two fjord systems. Pol. Polar Res. 38, 351–373. doi: 10.1515/popore-2017-0013
Steneck, R. S., Graham, M. H., Bourque, B. J., Corbett, D., Erlandson, J. M., Estes, J. A., et al. (2002). Kelp forest ecosystems: biodiversity, stability, resilience and future. Environ. Cons. 29, 436–459. doi: 10.1017/s0376892902000322
Tatarek, A., Wiktor, J., and Kendall, M. A. (2012). The sublittoral macroflora of Hornsund. Polar Res. 31:18900. doi: 10.3402/polar.v31i0.18900
Teagle, H., Hawkins, S. J., Moore, P. J., and Smale, D. A. (2017). The role of kelp species as biogenic habitat formers in coastal marine ecosystems. J. Exp. Mar. Biol. Ecol. 492, 81–98. doi: 10.1016/j.jembe.2017.01.017
Traiger, S. B., and Konar, B. (2018). Mature and developing kelp bed community composition in a glacial estuary. J. Exp. Mar. Biol. Ecol. 501, 26–35. doi: 10.1016/j.jembe.2017.12.016
Vadas, R. L., and Steneck, R. S. (1988). Zonation of deep water benthic algae in the Gulf of Maine. J. Phycol. 24, 338–346. doi: 10.1111/j.1529-8817.1988.tb04476.x
Wernberg, T., Krumhansl, K., Filbee-Dexter, K., and Pedersen, M. F. (2019). “Chapter 3 - Status and Trends for the World’s Kelp Forests,” in World Seas: an Environmental Evaluation, ed. C. Sheppard (Cambridge, MA: Academic Press), 57–78. doi: 10.1016/b978-0-12-805052-1.00003-6
Witman, J. D., and Lamb, R. W. (2018). Persistent differences between coastal and offshore kelp forest communities in a warming Gulf of Maine. PLoS One 13:e0189388. doi: 10.1371/journal.pone.0189388
Zaneveld, J. S. (1966). Vertical zonation of Antarctic and sub-Antarctic benthic marine algae. Antarct. J. U.S. 1, 211–213.
Keywords: macroalgae, Saccharina, Agarum, submerged vegetation, depth extension, Arctic
Citation: Krause-Jensen D, Sejr MK, Bruhn A, Rasmussen MB, Christensen PB, Hansen JLS, Duarte CM, Bruntse G and Wegeberg S (2019) Deep Penetration of Kelps Offshore Along the West Coast of Greenland. Front. Mar. Sci. 6:375. doi: 10.3389/fmars.2019.00375
Received: 23 March 2019; Accepted: 17 June 2019;
Published: 03 July 2019.
Edited by:
Michelle Jillian Devlin, Centre for Environment, Fisheries and Aquaculture Science (CEFAS), United KingdomReviewed by:
Dan Reed, University of California, Santa Barbara, United StatesJan Marcin Weslawski, Institute of Oceanology (PAN), Poland
Copyright © 2019 Krause-Jensen, Sejr, Bruhn, Rasmussen, Christensen, Hansen, Duarte, Bruntse and Wegeberg. This is an open-access article distributed under the terms of the Creative Commons Attribution License (CC BY). The use, distribution or reproduction in other forums is permitted, provided the original author(s) and the copyright owner(s) are credited and that the original publication in this journal is cited, in accordance with accepted academic practice. No use, distribution or reproduction is permitted which does not comply with these terms.
*Correspondence: Dorte Krause-Jensen, dkj@bios.au.dk