- 1Centre d’Etudes Biologiques de Chizé, UMR7372, Villiers-en-Bois, France
- 2Conservation Department, Phillip Island Nature Parks, Cowes, VIC, Australia
- 3H.T. Harvey & Associates Ecological Consultants, Los Gatos, CA, United States
- 4Departamento de Ecologia Evolutiva, Museo Nacional de Ciencias Naturales, CSIC, Madrid, Spain
- 5Global Penguin Society and Center for Ecosystem Sentinels, Department of Biology, University of Washington, Seattle, WA, United States
- 6Department of Zoology, Weber State University, Ogden, UT, United States
- 7School of Health and Life Sciences, Federation University Australia, Berwick, VIC, Australia
- 8Global Penguin Society and Department of Ecology, Environment and Evolution, La Trobe University, Melbourne, VIC, Australia
- 9Global Penguin Society and Centro Nacional Patagónico (CONICET), Puerto Madryn, Argentina
- 10Australian Antarctic Division, Department of Environment and Energy, Australian Government, Kingston, TAS, Australia
- 11Department of Zoology, University of Otago, Dunedin, New Zealand
- 12Woods Hole Oceanographic Institution, Woods Hole, MA, United States
- 13Centre for Environmental Sustainability and Remediation, School of Science, RMIT University, Melbourne, VIC, Australia
- 14Departament de Biologia Evolutiva, Ecologia i Ciències Ambientals, Universitat de Barcelona, Barcelona, Spain
- 15Aiuká, Praia Grande, Brazil
- 16International Fund for Animal Welfare (IFAW), Yarmouth Port, MA, United States
- 17FitzPatrick Institute of African Ornithology, DST-NRF Centre of Excellence, University of Cape Town, Rondebosch, South Africa
- 18Environment and Sustainability Institute, University of Exeter, Penryn, United Kingdom
- 19Bristol Zoological Society, Bristol, United Kingdom
- 20Institute of Research and Rehabilitation of Marine Animals, Cariacica, Brazil
- 21University of California, Davis, Davis, CA, United States
- 22Department of Biodiversity and Conservation Biology, University of the Western Cape, Belville, South Africa
- 23The Southern African Foundation for the Conservation of Coastal Birds, Cape Town, South Africa
- 24Marine and Antarctic Futures Centre, Institute for Marine and Antarctic Studies, University of Tasmania, Hobart, TAS, Australia
- 25BirdLife Tasmania, Hobart, TAS, Australia
- 26British Antarctic Survey, Natural Environment Research Council, Cambridge, United Kingdom
Penguins face a wide range of threats. Most observed population changes have been negative and have happened over the last 60 years. Today, populations of 11 penguin species are decreasing. Here we present a review that synthesizes details of threats faced by the world’s 18 species of penguins. We discuss alterations to their environment at both breeding sites on land and at sea where they forage. The major drivers of change appear to be climate, and food web alterations by marine fisheries. In addition, we also consider other critical and/or emerging threats, namely human disturbance near nesting sites, pollution due to oil, plastics and chemicals such as mercury and persistent organic compounds. Finally, we assess the importance of emerging pathogens and diseases on the health of penguins. We suggest that in the context of climate change, habitat degradation, introduced exotic species and resource competition with fisheries, successful conservation outcomes will require new and unprecedented levels of science and advocacy. Successful conservation stories of penguin species across their geographical range have occurred where there has been concerted effort across local, national and international boundaries to implement effective conservation planning.
Introduction
Penguins are one of the most iconic taxonomic groups of wild animals, with similar appeal to polar bears, humpback whales, orangutans, and giant pandas. As a seabird, they forage at sea but must return to land to breed. Flightless and slow out of water, they are gracious and agile in the ocean. Penguins have charismatic appeal, and have inspired award-winning documentaries and popular cartoon movies, as well as numerous books for children and coffee-table books for adults. Penguins attract millions of people every year to zoos and to natural places in the temperate or high latitudes of the Southern Hemisphere. Penguins are definitely high on the list in popular culture.
Ironically, despite their public appeal, penguin populations face a wide range of threats. Penguins evolved over 60 million years ago, and yet perhaps the biggest challenge will be for some of them to survive the next 50 years. Most observed population changes have been negative and have happened over the last 60 years. Today, populations of 11 of the 18 penguin species are decreasing (BirdLife International, 2018), especially those with restricted ranges living in temperate areas close to humans. The species located around the Antarctic continent where human contact is minimal are of lower concern; in general, these also have some of the largest populations and most extensive geographic ranges.
The layperson may plausibly ask: Why should we care about penguins? Three immediate answers would be: (i) penguins can tell us about the status of the oceans as they integrate changes occurring at lower levels in the trophic network; (ii) they act as flagship species, i.e., by protecting penguins, we can protect their ecosystems; and (iii) as a charismatic species that people identify with, they play a vital role in education to help explain environmental issues to the public. Yet, besides these utilitarian answers, should not the prime reason for caring about penguins simply be a moral duty toward living things? Humans have a natural tendency to be attracted by life in the biophilic perspective (Simaika and Samways, 2010). However, one can wonder if this perspective is still actual in a world where children grow increasingly detached from Nature. Loveable creatures like penguins are thus particularly important in reinforcing the bond between humans and the environment (Simaika and Samways, 2010).
A major challenge for the conservation of penguins is to quantify how multiple stressors interact at the community or ecosystem level. Therefore, to achieve meaningful conservation goals, it will be important to develop a detailed understanding of how each ecosystem supports a penguin species and to identify priority areas for research in order to inform conservation. Over the past five decades, there has been a growing level of information on penguin physiology, ecology, and population trends owing to the emergence of cutting-edge technologies, such as biotelemetry and bio-logging (e.g., Ropert-Coudert and Wilson, 2005), satellite facilitated remote sensing and unmanned aerial vehicles (e.g., Fretwell and Trathan, 2009; Borowicz et al., 2018), weighbridges (e.g., Chiaradia and Kerry, 1999; Green et al., 2006), stable isotope and fatty acid analyses (e.g., Cherel and Hobson, 2007; Connan et al., 2016), DNA analyses (e.g., Banks et al., 2006; Deagle et al., 2007), amongst others.
Ensuring policy makers and ecosystem managers have the most up-to-date scientific basis for making conservation decisions is vital. To this end, Trathan et al. (2015) identified pollution, habitat loss, introduction of alien species into their habitats, fishing, and climate change as critical threats to the conservation of penguin populations worldwide. Yet the rate of ecosystem change is so rapid that a constant re-appraisal is needed to keep conservation priorities up-to-date. Potential competition for resources between fisheries and natural predators, such as penguins, is becoming an increasing concern and estimated capture rates by fisheries may be underestimated (Pauly and Zeller, 2016), as is bycatch (Crawford et al., 2017). The demand for marine protein is almost certainly going to increase as the world human population moves toward the projection of ∼9.7 billion by 2050 (United Nations Department of Economic and Social Affairs [UN-DESA], 2015). Consequently, developing new management approaches is vital if human populations and natural systems are to survive in the future. Similarly, climate change is an ever-increasing threat, mitigation and adaptation to which is becoming more difficult than previously thought (Steffen et al., 2018) and requires urgent decision now (Rintoul et al., 2018). Taken together, major changes to marine ecosystems will likely lead to major losses within natural systems (Kolbert, 2014). In this paper, we compile new information and new perspectives elaborating upon the review of Trathan et al. (2015). We also identify those threats that are specific, as well as common to all species, and propose mitigation measures that should help protect these species.
For the purpose of this review we consider the following 18 species: emperor (Aptenodytes forsteri), king (Aptenodytes patagonicus), macaroni (Eudyptes chrysolophus), southern rockhopper (Eudyptes chrysocome), northern rockhopper (Eudyptes moseleyi), royal (Eudyptes schlegeli), snares (Eudyptes robustus), fiordland (Eudyptes pachyrhynchus), erect-crested (Eudyptes sclateri), little penguin (Eudyptula minor), yellow-eyed (Megadyptes antipodes), Adélie (Pygoscelis adeliae), chinstrap (Pygoscelis antarctica), gentoo (Pygoscelis papua), Humboldt (Spheniscus humboldti), African (Spheniscus demersus), Magellanic (Spheniscus magellanicus), and Galápagos (Spheniscus mendiculus). We have chosen not to include subdivisions such as eastern rockhopper penguin (Eudyptes chrysocome filholi) or Australian little penguin (Eudyptula minor novaehollandiae) that are not currently officially recognized by IUCN as separate species.
Influence of Climate Change on Penguins
The warming of the oceans has a profound impact on the structure and function of both physical and biological systems (Hoegh-Guldberg and Bruno, 2010). Oceanic temperature changes across the globe are uneven with some of the areas most impacted by global warming coinciding with marine regions that are used by seabirds, including penguins (Ramírez et al., 2017). Like many other species, penguin life cycles are affected by climate change directly through El Nino, increased frequency of heat waves and storms leading to egg and chick loss (Boersma, 1978; Boersma and Rebstock, 2014), or indirectly through changes in sea-ice dynamics (Barbraud and Weimerskirch, 2001; Jenouvrier et al., 2006), increased frequency of bushfires (Chambers et al., 2010), and climate-driven changes in prey abundance and distribution (Trathan et al., 2006; Vargas et al., 2006; Grémillet and Boulinier, 2009; Barbraud et al., 2012; Jenouvrier, 2013; Cristofari et al., 2018).
Indirect Effects of Climate Change
Changes in prey abundance and distribution are often the main mechanisms through which seabirds are affected by climatic conditions. Warming ocean waters can change the distribution of water currents and lead to changes in prey recruitment and growth, making finding food more difficult for many of the world’s penguin species (Cristofari et al., 2018; Morgenthaler et al., 2018). Among climate variables, many studies have focused on sea surface temperature (SST) because of the influence of SST on primary and secondary marine production (Behrenfeld et al., 2006). Changing winds also have a strong impact on productivity through effects on frontal zones (Bakun et al., 2015). For instance, intensifying winds in the Southern Ocean, owing to a positive Southern Annular Mode, are altering the depth of the mixed layer, which can affect the distribution of prey, and, by cascading effect, the foraging behavior, fitness components, and ultimately population size of penguins (e.g., Ropert-Coudert et al., 2009; Saraux et al., 2016), or by directly altering habitat (e.g., Fretwell and Trathan, 2018). Furthermore, prolonged drought periods in El Niño years resulted in reduced foraging range, low prey abundance and dietary diversity; leading to lower breeding success in little penguins (Preston et al., 2010; Kowalczyk et al., 2015).
Evidence of a shifting marine environment affecting the foraging and migration of penguins has become apparent in southern Africa in recent years. Crawford et al. (2015) found that African penguins have fared poorly due to reductions in prey caused by competing fisheries in conjunction with marine climate-induced spatial shifts in forage fish distribution. Similar patterns are being exhibited by penguins in the other heavily fished, wind driven boundary current systems along the south American west coast, i.e., Humboldt and Galápagos penguins (Boersma et al., 2013, 2015). Along the New Zealand coast, yellow-eyed adult and fledgling penguins exhibit sudden reductions in survival in response to difficulty in finding food during intermittent warm oceanic conditions (Mattern et al., 2017). In the Southern Ocean, climate variability has occasionally shifted the position of the Antarctic Polar Front, forcing king penguins to extend their foraging trips and as a result experience decreased breeding success (Bost et al., 2015) and even abandon some colonies altogether (Cristofari et al., 2018). Strong El Niño events, which are expected to increase in frequency as a result of climate change (Yeh et al., 2009), can also cause abrupt shifts in prey distribution with potentially dramatic consequences for seabirds, as demonstrated by the population crashes experienced by Humboldt and Galápagos penguins in 1982–1983 and 1997–1998 (Hays, 1986; Paredes and Zavalaga, 1998; Vargas et al., 2006). Likewise, regional instances of episodic warming of the marine environment have also been shown to cause mass starvation of penguins in Argentina and Australia (Cannell et al., 2012; Morgenthaler et al., 2018).
Direct Effect of Climate Change
Fewer studies have documented direct effects of climate change on penguins. Storms can have substantial effects on penguin colonies, leading to the flooding or collapse of nests (de Villiers, 2002; Demongin et al., 2010; Boersma and Rebstock, 2014). During unusually hot summer days, heat stress can lead penguins to desert their nests in large numbers (Kemper et al., 2007; Chapman et al., 2011; Boersma and Rebstock, 2014; Traisnel and Pichegru, 2018). The aforementioned severe drought periods can also increase the frequency of bushfires that destroy nesting habitat (Chambers et al., 2010). For high latitude Antarctic penguins, vagaries of sea ice, either too much or too little, can have important effects on breeding performance (Ainley et al., 2010). For example, early break-out of fast-ice may cause massive breeding failure and even adult mortality in emperor penguins (Barbraud and Weimerskirch, 2001; Jenouvrier et al., 2009; Kooyman and Ponganis, 2017). In contrast, for Adélie penguins, a late break-out of fast-ice can result in reproductive failure (Emmerson and Southwell, 2008), sometimes with dramatic proportions (Ropert-Coudert et al., 2018). Furthermore, sea level rise threatens to inundate important coastal penguin nesting sites, especially in places where natural barriers such as cliffs or human barriers, such as roads and developments, prevent penguins from moving inland. Landslides resulting from climate variability may also destroy penguin’s breeding sites (e.g., chinstrap and macaroni penguins at Nyrøysa; Niemandt et al., 2016). However, the negative aspect of such an extreme event should be measured on a longer time scale, as sites affected by the Nyrøysa landslide became available for penguins to breed following an earlier landslide. In this context, the geologic temporal scale response of penguins to sea level rise (e.g., Emslie and Patterson, 2007) indicates the potential for important changes in the future.
Variable Response of Penguin Populations to Climate
Across their latitudinal range, very few penguin populations are increasing in response to changing environmental conditions, and for those that are, increases in populations in one area are largely counter-balanced by decreases elsewhere. Despite prolonged periods of warm sea temperatures leading to abrupt population declines, Galápagos penguin populations are currently increasing (Boersma et al., 2013) and their recent population increases are expected to continue, with a trend of favorable cooler sea-surface temperatures expected in their foraging range (Karnauskas et al., 2015). Populations are increasing for gentoo penguins breeding at the South Orkney Islands and south of the South Shetland Islands where sea ice has decreased (Hinke et al., 2007; Carlini et al., 2009; Dunn et al., 2016). This is in stark contrast to declines reported for the more northern and larger Kerguelen gentoo population (Lescroël et al., 2009). Similarly, Adélie penguin populations at the South Orkney Islands and on the northern Western Antarctic Peninsula have declined (e.g., Dunn et al., 2016; Hinke et al., 2017), whereas Adélie penguins in east Antarctica have increased steadily over the last six decades with only a few populations plateauing or declining in recent years (Southwell et al., 2015; Che-Castaldo et al., 2017).
Future Penguin Responses to Climate Change
As environmental conditions change, predicting population responses is an increasingly important task for ecologists, if they are to guide the development of management and conservation strategies (Jenouvrier, 2013; Hinke et al., 2017). A number of studies have projected future penguin population, or distribution change, according to climate forecasts developed through the Intergovernmental Panel on Climate Change (IPCC) assessment reports (e.g., Iles and Jenouvrier, 2019). To our knowledge, the few that have done so focused on Antarctic1 penguin species: In Antarctica, climate warming, loss of sea ice, and more frequent anomalous events suggest that further reductions in ice-dependent penguin populations are likely (Ainley et al., 2010; Jenouvrier et al., 2012, 2014, 2017; Ballerini et al., 2015; Hinke et al., 2017). Broad-scale modeling projections suggest that both of the true Antarctic penguin populations (Adélie and emperor penguins) are expected to decline in an increasingly warm environment (Ainley et al., 2010; Cimino et al., 2016; Hinke et al., 2017; Jenouvrier et al., 2017).
Modeling the impacts from climate change will be most successful when it includes the complexity of habitats and movements between colonies, as dispersion is a key process for persistence in a changing environment (Crawford et al., 2015; Jenouvrier et al., 2017). For penguin species to persist, any shifts in their breeding habitat in response to climate change must also relate to the availability of suitable foraging habitat.
Penguin Adaptation to Climate Warming
Critically important is the notion that species responses to climate change are contingent upon their intrinsic sensitivity and plasticity and hence their capacity to buffer against poor conditions or to adapt their behavior to cope with change. Range shifts are primarily a short-term species response to rapid climate change, but are often hampered by natural or anthropogenic habitat fragmentation (Crawford et al., 2015). Furthermore, the ability of penguin species to disperse to new habitat remains an open question (Jenouvrier et al., 2017).
Changes in foraging behavior in terms of either horizontal or vertical distribution, or of prey species, could be one means of potential buffering. Southern rockhopper penguins are one of the few species that have displayed substantial male behavioral change in response to environmental change with the potential to increase reproductive outcomes (Pütz et al., 2018). Similarly, snares and fiordland penguins may be less susceptible to warming as they are more flexible in their foraging behavior when feeding at either the sub-Antarctic and/or sub-Tropical Fronts (Mattern et al., 2009). However, juvenile African penguins have been unable to adjust their foraging behavior and have found themselves caught in what has been termed an “ecological trap” (Sherley et al., 2017) that results in them mistakenly selecting habitats that do not favor population persistence.
Penguins Interacting With Fisheries
Most penguins are fish eaters, foraging on schooling pelagic fish that are also targeted by commercial fisheries. Thus, within all of the ocean basins, penguins experience a high degree of interaction with fisheries, the majority of which are coastal (Crawford et al., 2017; Gianuca et al., 2017). These interactions include both direct effects such as incidental bycatch in fishing gear (Crawford et al., 2017), as well as indirect effects such as competition for prey resources (Sherley et al., 2018), habitat modification by fishing gear (e.g., Mattern et al., 2013) or interaction with fisheries discards (Grémillet et al., 2008).
Incidental Bycatch in Fisheries
Bycatch, the unintentional capture of non-target animals in fisheries, has been a documented issue for seabirds since the 1970s (Tull et al., 1972), and by now has been widely studied and identified as a substantial threat to many species (Croxall et al., 2012). The bycatch of penguins in fishing gear, however, has been the subject of far fewer directed studies. Moreover, quantifying overall penguin bycatch levels and their relative importance compared to other threats is a challenge. That said, a recent review has indicated that 14 of the world’s 18 penguin species have been recorded as bycatch in fishing gear (Crawford et al., 2017).
Although evidence for bycatch is mostly anecdotal, substantive evidence of fisheries bycatch exists for Humboldt, Magellanic and yellow-eyed penguins (Crawford et al., 2017). Penguins, as pursuit divers, are most likely to interact with gillnets (Žydelis et al., 2013) and birds appear to be caught when they are actively foraging (Simeone et al., 1999; Pütz et al., 2011), transiting, and resting on the surface (Majluf et al., 2002). Penguins are also caught on occasion in trawl fisheries, as well as longline fisheries in low numbers (e.g., Nel et al., 2002; González-Zevallos and Yorio, 2006). For example, Magellanic penguins are captured by trawlers during the haul as the penguins attempt to feed on small fish that drop from the net (González-Zevallos and Yorio, 2006).
Population trends of long-lived birds are generally most sensitive to adult mortality (Sæther and Bakke, 2000; Stahl and Oli, 2006); bycatch is therefore currently of greatest conservation concern for the endangered yellow-eyed penguin in New Zealand (Crawford et al., 2017; Gianuca et al., 2017). Humboldt and Magellanic penguins are also at a great risk due to the intense gillnet fishing along the Humboldt current, with tens to thousands of penguins dying per year (Simeone et al., 1999; Schlatter et al., 2009). Further studies would be required to quantify the true extent of bycatch, particularly for those species deemed most susceptible.
Use of Discards
Fisheries produce huge amounts of waste – of approximately 120 million tons (Mt) of annual global landings, around 10% are dumped back into the sea (Zeller et al., 2018). Discards tend to occur in relatively predictable, highly concentrated patches and, thus for seabirds, require less energy to exploit than diving to catch prey naturally. It is perhaps unsurprising that this subsidy attracts large numbers of scavengers including more than half of all seabird species (Oro et al., 2013). However, seabirds have a relatively high metabolic rate, so discards may constitute an “attractive nuisance” if they are low in energy content relative to natural prey sources (Grémillet et al., 2008). While penguins appear less inclined to scavenge on discards than many other seabird species (Oro et al., 2013), the fact that some penguins associating with fishing vessels are taken as bycatch (see above), indicates that they may utilize fish discharged overboard (Gandini et al., 1999; González-Zevallos and Yorio, 2006; Roux et al., 2012). Incidence at boats does appear to be low, but penguins may be more difficult for on-board observers to spot than flying seabirds, possibly resulting in under-reporting. A directed effort is needed to research the issue.
Competition for Prey Resources
Penguins are important consumers; for instance, macaroni penguins alone may eat ∼9 Mt of food annually, or ∼7% of global fisheries catches in 2010 (Brooke, 2004; Zeller et al., 2018). Penguins consume the forage species that are central to marine food webs, i.e., squid, krill, and small pelagic schooling fish (Garcia-Borboroglu and Boersma, 2013). These prey are also targeted by commercial fisheries, which catch ∼20 Mt annually, or ∼30% of total global landings (Alder et al., 2008; Nicol et al., 2012). Such fisheries generally overlap with penguins in space, time, and size-classes of prey taken (e.g., Pichegru et al., 2009; Trathan et al., 2018; Warwick-Evans et al., 2018). Fisheries also have the potential to reduce prey abundance below levels that seabirds need to forage efficiently. To maintain successful reproduction and survival, penguins must contend with either localized prey depletion (Sherley et al., 2018) or exist within broader-scale ecosystem change (Sherley et al., 2017). However, while many of these fisheries are assumed to compete with penguins for food, in many instances, direct evidence is sparse (Trathan et al., 2014; Ratcliffe et al., 2015). Fisheries also deplete the adults of benthic fish, whose pelagic juveniles are important in the diet of species such as gentoo and macaroni penguin; population reduction has coincided with depletion of the adult stages of these fish (Ainley and Blight, 2009). Finally, the removal of larger-sized fish species that compete with penguins for food may lead to alteration of the food web and penguin population change (Ainley et al., 2017).
Arguably the best evidence for competition between commercial fisheries and penguins comes from southern Africa, where the local purse-seine fisheries compete with African penguins for their two main prey, anchovy Engraulis encrasicolus and sardine Sardinops sagax (e.g., Pichegru et al., 2009). In Namibia, overfishing in the 1950s and 1960s, and subsequent environmental change, precipitated a regime-shift that led sardine populations to collapse (Cury and Shannon, 2004). Low energy bearded goby Sufflogobius bibarbatus replaced sardines as the main penguin prey (Ludynia et al., 2010), but juvenile penguins from South Africa continue to disperse into this degraded habitat and suffer high mortality as a result (Sherley et al., 2017).
In South Africa, a decline in the relative abundance of adult anchovy and sardine after 2000 (e.g., Coetzee et al., 2008; Mhlongo et al., 2015) led penguin populations to plummet (Crawford et al., 2014). Adult survival (Sherley et al., 2014; Robinson et al., 2015), juvenile survival (Sherley et al., 2017), and chick growth rates (Sherley et al., 2013) decreased concurrently. Similarly, in south America, the huge take of anchoveta Engraulis ringens, in perhaps the largest fishery on the planet, has likely had similar negative effects on the population viability of Humboldt penguins and other seabirds feeding in the Humboldt Current (Duffy et al., 1984), even if other factors have been implicated (e.g., guano mining destroying nesting habitat).
In the Southern Ocean, chinstrap and Adélie penguins probably increased as a consequence of the commercial depletion of baleen whales (Ballance et al., 2006; Ainley et al., 2007), illustrating the importance of krill, Euphausia spp., and its availability, to penguins. In the Ross Sea, Adélie penguin populations have increased over recent decades owing initially to increasing persistence of coastal polynyas (Ainley et al., 2010) and more recently possibly due to increased prey availability as fisheries remove a direct competitor, the Antarctic toothfish Dissostichus mawsoni (Ainley et al., 2017). Likewise, the nascent recovery of whale populations, and that of krill-eating fur seals Arctocephalus spp., is leading to recent decreases of chinstrap, macaroni and other penguins, further illustrating the importance of krill (Trivelpiece et al., 2011; Lynch et al., 2012; Trathan et al., 2012). No recent estimates of prey removals by whales along the Antarctic Peninsula and Scotia Sea are available, making robust interpretation problematic. This is in part because whale numbers have been dramatically increasing in the past 50 years (e.g., Branch, 2011). Currently, the krill fisheries take about 300,000 t from the Scotia Sea/northern Antarctic Peninsula region annually, but this is only about half of the amount harvested in the 1980s (Brooks, 2013). Such a level of take, and fears that whale recovery would be compromised, led in 1982 to the creation of the Convention for the Conservation of Antarctic Marine Living Resources (CCAMLR), which calls for protecting the needs of krill-dependent predators in its management (Hofman, 2017). Moreover, while a proportion of current krill fishing grounds overlap with the foraging areas of penguin colonies (Trathan et al., 2018; Warwick-Evans et al., 2018), evidence that the fishery is becoming increasingly concentrated and that such concentration of effort could impact some colonies more than others is growing. In the future, the pressure for increased krill fishing will likely develop as demand has grown with the need to supply aquaculture and nutraceutical uses. Any relaxation of CCAMLR’s primary objective of conservation, as more fishing countries accede to the Convention, would not bode well for the future of “krill-dependent species” foraging either in the krill fishing grounds, or waters downstream of where fisheries operate, which may then become depleted in krill (Brooks et al., 2016; Jacquet et al., 2016; Hofman, 2017; Trathan et al., 2018).
Disturbed Ecosystems
Disturbance to marine ecosystems by humans can have profound impacts which may have long term consequences and lead to altered trophic relationships, especially following commercial removal of important trophic components. For example, the commercial removal of top predators in many systems has allowed other taxa to increase in abundance (e.g., Ballance et al., 2006; Ainley et al., 2007, Ainley and Blight, 2009; Trivelpiece et al., 2011; Trathan et al., 2012). For penguins, such altered trophic relationships can be significant, especially if certain relationships become a dominant link. Under natural conditions, interactions between native species should not be a conservation issue. However, under altered conditions, natural relationships can become a dominant factor for some species. For example, seals predating penguins has become an emerging concern for several penguin species. Fur seals and sea lions are normally fish and cephalopod specialists but Cape fur seals Arctocephalus pusillus pusillus predate or displace African penguins from breeding sites and are now considered a major driver of decreasing penguin population trends at some colonies (Crawford et al., 2001; Weller et al., 2016). Until recently, seal predation of penguins was considered to be a natural trophic interaction, but now in South Africa, it is managed by culling individuals that threaten penguin colonies.
Pollution and Penguins
In this section, we have focused on four main areas of environmental pollution documented in penguin habitats: plastic, mercury, Persistent Organic Pollutants (POPs) and oil pollution. We do not consider light pollution, which has received considerably less attention, though it has recently been shown that light pollution might actually be beneficial for penguins in some contexts (Rodríguez et al., 2018). Acoustic pollution also represents an issue that has not received adequate attention for species other than cetaceans, but there is preliminary evidence that penguins avoid areas of seismic surveys (Pichegru et al., 2017).
Plastic Pollution
There is increasing concern about the impacts of plastic pollution on marine organisms with two major impacts specific to birds: entanglement in plastics and other synthetic debris, and plastic ingestion (Kühn et al., 2015). Penguins are fortunate at this stage in not being severely impacted by either of these threats, in part because they mostly take live prey and pay little attention to floating objects, either a fish carcass or plastic objects, quite unlike petrels and albatross many of which are scavengers. However, recent reports that penguins are also feeding on gelatinous organisms may make penguins susceptible to ingestion of fragments of plastic bags (Thiebot et al., 2017). In addition, they are at risk of indirect contamination via microplastic transfer from their prey (Nelms et al., 2018).
Entanglement has been reported for 7 of the 18 penguin species, with most records from two temperate species, African and little penguins (Ryan, 2018). There are no published records from south American Spheniscus penguins, but this is plausibly due to the failure to report incidents, rather than an absence of entanglements. For example, Boersma removed plastic bags around feet and necks of two living Magellanic penguins. A high proportion of African penguins washed up on the South African south coast in the 1980s were entangled (28%; Ryan, 1990). This is clearly not a random sample of birds, as entanglement doubtless contributed to their stranding, but this is among the highest entanglement rates recorded for any seabird (e.g., Camphuysen, 2008). There have been very few records of entangled African penguins over the last decade, despite regular surveys for stranded birds. The reason for the decrease is not clear but may reflect a decrease in the abundance of entangling debris in the region, as well as a reduction in the African penguin population (which has more than halved over the same period; Crawford et al., 2011, 2015). Fishing gear is responsible for most entanglement incidents (net fragments, fishing line), but penguins also have been found entangled by six-pack rings, packing straps, lid rings and rubber bands (Ryan, 2018).
Plastic ingestion has seldom been recorded in most penguin species (Ryan, 2016). The comprehensive review by Kühn et al. (2015) reported ingestion of meso- and macro-plastic (>1 mm) by five species, but the record they list for southern rockhopper penguin is in error for northern rockhopper (the record was from Gough Island in 1984, prior to the species being split; Ryan, 1987). In four of the five species, ≤2% of individuals had ingested plastic (Kühn et al., 2015). In stark contrast, 22–35% of juvenile Magellanic penguins stranding in southern Brazil have plastic items in their stomach (Pinto et al., 2007; Tourinho et al., 2010; Brandão et al., 2011). This atypically high proportion probably reflects an exceptional dynamic where starving juveniles attempt to ingest any items (including plastic, but also plant fragments and other unusual items) in a desperate attempt to stave off hunger (Brandão et al., 2011). Birds that became stranded might also have ingested plastic because they are in poor health (cf. Ryan, 1987).
Most penguins almost certainly regularly consume microplastics contained within their prey, particularly fibers, which occur in a high proportion of small pelagic fish (Barrows et al., 2018). However, it is unclear whether these small fibers have any impact on the birds; they probably are excreted shortly after being ingested but could be vectors for transferring other pollutants (Browne et al., 2011).
Mercury Pollution
As meso-predators in marine ecosystems, penguins are at an elevated risk of bioaccumulation and biomagnification of mercury (Hg). In seabirds, exposure to Hg has been linked to increased oxidative stress, altered gene expression, and decreases in reproductive fitness, immunocompetence, and neurotransmitter functioning (reviewed in Ackerman et al., 2016). To date, feather Hg concentrations have been reported in the literature for adults of 12 species of penguins from at least 40 unique populations across seven ecosystems, with the most extensive data sets coming from populations on the Antarctic Peninsula, South Georgia, Crozet Islands, and Kerguelen Islands (Table 1). Adult feather total Hg concentrations range from 0.09 to 5.90 ppm (fw) with 37.5% of these populations having feather Hg concentrations <1.00 ppm. Only four populations had feather Hg concentrations over 5 ppm, the current lowest level above which observable adverse effects are known for seabirds (Wolfe et al., 1998; Evers et al., 2008): southern rockhopper penguins on Staten Island, Patagonian Shelf marine ecosystem (5.10 ± 1.46 ppm; Brasso et al., 2015), gentoo penguins in the Estacade population on the Kerguelen Islands (5.85 ± 3.00 ppm; Carravieri et al., 2013), gentoo penguins on the Crozet Islands (5.90 ± 1.91 ppm; Carravieri et al., 2016), and little penguins in Australia (5.01 ± 1.78 ppm; Brasso et al., 2015; Finger et al., 2015). Antarctic species generally have lower Hg concentrations than sub-Antarctic and subtropical species; however, there are no clear latitudinal gradients in Hg exposure across the Southern Hemisphere (Brasso et al., 2015; Becker et al., 2016; Carravieri et al., 2016).
Concurrent stable isotope analysis has been a valuable tool allowing the resolution of differences in Hg exposure among populations (Cherel et al., 2007; Carravieri et al., 2013, 2016; Brasso and Polito, 2013; Brasso et al., 2015; Polito et al., 2016; Renedo et al., 2018). Species that feed heavily on fish, and in particular myctophid fish, such as emperor and king penguins, tend to accumulate higher concentrations of Hg relative to their sympatric congeners (Carravieri et al., 2013, 2016; Renedo et al., 2018). Among sympatrically breeding, Pygoscelis penguins in the Antarctica Peninsula, Polito et al. (2016) found differences in Hg to be the result of a difference in dietary preference whereby chinstrap penguins consume a larger proportion of myctophids compared to Adélie and gentoo penguins (Polito et al., 2016). Gentoo penguins, in particular, show a high degree of inter-individual and population-level variation in foraging habits (dietary composition and foraging habitat) which seems to be a significant driver in differences in Hg exposure in this species (Carravieri et al., 2013, 2016; Brasso et al., 2015). While diet is a major driver of exposure risk to Hg, these studies have also identified potential “hot-spots” of Hg availability that result from local environmental conditions that enhance the production of methylmercury. These areas include the Crozet and Kerguelen Islands shelves (Carravieri et al., 2016; Renedo et al., 2018), southern Patagonian Shelf marine ecosystem and Port Phillip Bay (Brasso et al., 2015). Though Hg concentrations in most penguin populations fall well below current adverse effects levels, no study has directly tested for effects of Hg on penguins. Further research is warranted in populations with known elevated tissue concentrations as Hg may alter their ability to respond to more severe challenges posed by changes in prey availability and climate.
Persistent Organic Pollution
Persistent organic pollutants are synthetic, ubiquitous, hydrophobic chemicals that pose a significant risk to environmental and human health according to the United Nations Convention on POPs (Kelly et al., 2007; UNEP, 2009). They are globally distributed through long-range atmospheric transport, even to remote polar regions far from emission sources (Wania and Mackay, 1993; Beyer et al., 2000). POPs lipophilic properties allow them to accumulate in organisms and biomagnify through food chains, with top-order marine predators accumulating high levels (Jepson et al., 2016). In seabirds, higher level POPs exposure is broadly associated with wing asymmetry and reduced fecundity (e.g., Jara-Carrasco et al., 2015). Many POPs are known neurotoxins with negative reproductive effects, neurobehavioural development and immunosuppression (Jara-Carrasco et al., 2017). The level of contaminant exposure can affect populations differently; endocrine disruption and impaired immune function of individuals may result in reduced reproductive success and survival which can lead to population declines (Ellis et al., 2018).
Penguins have a high lipid content and slow metabolism, therefore most species have a very slow process of pollutant detoxification (Jara-Carrasco et al., 2017). Despite small fractions eliminated by guano (Falkowska and Reindl, 2015), POPs can persist and accumulate in penguins, with enhanced circulation of pollutants when animals are fasting and mobilize fat reserves (e.g., Dehnhard et al., 2017).
The earliest report of POPs in a penguin species (Adélie penguin) was in the early 1970s (Conroy and French, 1974). Since then, the bulk of studies have investigated contaminant loads found in Antarctic penguins (Pygoscelis spp., emperor penguins and Eudyptes spp.), reporting on concentrations found in eggs or blood (Ellis et al., 2018). The temporal trends of POPs within these Antarctic species appears to reflect global patterns of contamination and POPs contamination within the Southern Hemisphere, where penguins live, is rising (Goerke et al., 2004; Ellis et al., 2018). The full extent of POPs probable impact on penguin ecology and metabolic abilities remains an open question. Given the many other threats penguins are facing, attribution of cause for demographic changes due to POPs contamination will be challenging and their effect is likely to remain difficult to quantify at the population level.
Oil Pollution
Penguins are particularly vulnerable to oil and petroleum pollution and are often the most numerous group of birds affected by oil spills within their distributions (Gandini et al., 1994; Goldsworthy et al., 2000; García-Borboroglu et al., 2006; Wolfaardt et al., 2009; Ruoppolo et al., 2013). Oiling impacts penguins causing direct mortality of adults, juveniles and chicks and long-term physiological damage. Oiled feathers lose their waterproofing and insulating properties, causing the birds to lose heat rapidly (Erasmus et al., 1981; Leighton, 1993; Stephenson and Andrews, 1997). Oiled penguins apparently experience great discomfort, increase the time spent preening and, due to heat loss, decrease the time spent foraging, which leads them to a negative energetic balance and dehydration, with a rapid decrease in body mass (Morant et al., 1981; Erasmus and Wessels, 1985). If not rescued, they may drown or leave the water and eventually starve (Stephenson and Andrews, 1997; Crawford et al., 2013). Oil may cause chemical burns to the skin and eyes, and when ingested (e.g., during preening) it can cause gastrointestinal ulcers and bleeding (Gandini et al., 1994; Crawford et al., 2000). Avian embryos exposed to oil (e.g., due to incubation of the eggs by their oiled parents) may have increased mortality, delayed growth and skeletal deformities (Aibers, 1978).
Oiling events involving wildlife have increased globally during the second half of the 20th century, reflecting the increase in oil production and international maritime transport (Clark, 1986; García-Borboroglu et al., 2006, 2008). Penguins experience oil spills from vessels and pipelines, by chronic oil pollution from ship discharges, leaking sunken containers at sea, at terminals and from contaminated ballast water (Gandini et al., 1994; Parsons and Underhill, 2005).
They can be substantially impacted especially if pollution is chronic (Adams, 1994; Shannon and Crawford, 1999). In the late 1980s and early 1990s, it was estimated that over 40,000 Magellanic penguins died annually due to chronic oil pollution along the coast of Chubut Province, Argentina (Gandini et al., 1994), a situation that only improved when – among other measures – the provincial government moved tanker lanes 40 km farther offshore in 1997 (Boersma, 2008). Chronic oil pollution is still a significant ongoing threat to African, Magellanic and little penguins (Parsons and Underhill, 2005; Ruoppolo et al., 2014).
Attributing seabird population changes from oiling can be difficult without pre-spill baseline data, needed to separate estimates of mortality due to oiling from mortality due to environmental fluctuations, other sources of mortality, emigration and recruitment (e.g., Dunnet, 1982; Crawford et al., 2000). The numbers of penguins oiled in some of the largest historical oil spills, however, provide evidence that these events can have significant population level impacts (Table 2). Chronic oiling can also be a key driver of population decreases at some colonies (e.g., Weller et al., 2016). The disturbance associated with rescuing oiled seabirds, the oil spill response and clean-up operations can lead to further loss of eggs and chicks (Shannon and Crawford, 1999), and even successfully cleaned penguins may subsequently never breed as well as unaffected conspecifics (Wolfaardt et al., 2008, 2009). Oil spills can also have indirect population effects by disrupting molt cycles (Underhill et al., 1999) and pair bonds (Butler et al., 1988), with carry-over effects on the breeding success in subsequent years (Crawford et al., 2000). To the best of our knowledge, no study has investigated the indirect ecological impacts of oil spills on penguin populations, such as changes in prey availability.
Pathogens, Diseases and Penguins
Pathogens, including microbes and parasites, are active players in the regulation of host populations (Grenfell and Dobson, 1995). Mass mortality events caused by pathogens can have significant and immediate effects on host populations over a short period of time. Furthermore, pathogens can influence host populations in the long term by reducing individual life-history traits such as reproduction phenology, clutch or brood size (see revision in Moller, 1997). Pathogens can also reduce an individual’s ability to respond to other stressor like pollutants (Carrasco et al., 2001) or extreme environmental changes. Individuals fight against pathogens through their immune system which has coevolved with the well-established pathogens over time. However, when this balance is interrupted, a host’s immune system can fail to prevent and destroy a pathogen leading to infectious disease. The appearance of new pathogens, to which the immune system could be naïve is of potential conservation concern as this could cause of a catastrophic mass mortality event (Jarvi et al., 2001) or lead to a slow population decline due to reduced host fitness and breeding success.
Prevalence of Disease Outbreaks in Penguins
Penguins are affected by a wide number of parasites, pathogens and diseases (Clarke and Kerry, 2000; Barbosa and Palacios, 2009; Barbosa et al., 2014; Grimaldi et al., 2015a; Diaz et al., 2017) including emergent diseases, such as feather loss disorder that likely spread from African Penguins to Magellanic penguins (Kane et al., 2010; Barbosa et al., 2015; Grimaldi et al., 2015b). Information about the health status of penguin species and their populations is very scarce and generally has been focused on reporting the presence/absence of pathogens, parasites or diseases with little information of epidemiological variables such as prevalence or intensity of infestation. Mass mortality events caused by disease is often very difficult to assess (see Gartrell et al., 2017), but there have been some reports of penguin mass mortality due to outbreaks in yellow-eyed penguin (Alley et al., 2017), little penguin (Mykytowycz and Hesterman, 1957), southern rockhopper penguin (de Lisle et al., 1990; Keymer et al., 2001), macaroni penguin (Cooper et al., 2009), gentoo penguin (MacDonald and Conroy, 1971; Munro, 2007), chinstrap penguin [Government of South Georgia and South Sandwich Islands (GSGSSI), 2005] and Adélie penguin (Kerry et al., 1996; Leotta et al., 2006) due to infectious diseases. Recurring infections of avian malaria (Plasmodium spp.), (Grilo et al., 2016) are common causes of death in penguin species especially African, yellow-eyed and Magellanic penguins, whilst recurrent infections of diphtheric stomatis (ætiology unclear) regularly affect yellow-eyed penguin chicks (Alley et al., 2017). Subtle effects of diseases include decreased chick body mass (Palacios et al., 2012), which could compromise survival (Moreno et al., 1999), reduce breeding success (Mangin et al., 2003) and increase the transmission of vector-borne pathogens (Olsen et al., 1995; Schramm et al., 2014; Montero et al., 2016). In other cases, Antarctic wildlife have been showing signs of disease of unknown pathology including feather loss disease in Adélie and emperor penguins (Barbosa et al., 2014; Varsani et al., 2015).
Prevalence of Pathogens That Have Not Yet Been Demonstrated to Cause Problems
In a number of recent studies the prevalence of known pathogenic organisms have been isolated from penguins not presenting signs of disease. Organisms such as Mycoplasma, Campylobacter, Helicobacter, Neisseria (Dewar et al., 2013), Avian avulavirus, Infectious bursal disease Virus (Smeele et al., 2017; Grimaldi et al., 2018) and members of the viral genera Avulavirus, Treisepsilonpapillomavirus, Gammapolyomavirus, and Siadenovirus (Smeele et al., 2017). Little is known about whether or not these organisms cause disease or negatively influence life histories of penguins. In addition, new developments in genomic sequencing has identified novel viruses including papillomaviruses, polyomavirus, avian influenza virus, among others (Varsani et al., 2014, 2015; Hurt et al., 2016).
Factors Facilitating Pathogen Susceptibility
Not all penguin species are under the same risk and it highly depends on the regions they inhabit, the level of human activity in the region, and the presence of disease transmitting vectors. In areas where penguin populations have been in close contact with humans over the last two centuries, their immune systems could be better adapted to the higher prevalence and diversity of pathogens. The higher cellular immunity of Magellanic penguins compared to Antarctic species like the chinstrap or Adélie penguins would suggest so, but levels were similar in gentoo penguins (D’Amico et al., 2014). As Magellanic and gentoo penguins have a far more diverse and fish-based diet than the other two species, diet and gastrointestinal parasite diversity (and prevalence) are too strong confusing variables when comparing the immune response among these species to ascertain this. Nonetheless, human activity is increasing in the polar regions. Penguin colonies are one of the main tourist attractions in the Southern Hemisphere, particularly in Antarctica where the number of tourists increases each year, reaching to over 40,000 people2. As such, penguins living in these environments could be more vulnerable to disease due to the potential exposure to human-borne pathogens or to the human-mediated spread of pathogens among colonies (Grimaldi et al., 2011). For example, an outbreak of avian cholera that killed 2,500 to 3,000 chinstrap penguins at Cooper Bay, South Georgia, in 2004 was attributed to human visitation (Vanstreels, subm.).
There are several factors that interact with diseases increasing their potential effects. A number of penguin species live in dense aggregations that could increase the probability of disease transmissions. Reduced genetic diversity in species with small populations, such as the Galápagos (Bollmer et al., 2007; Nims et al., 2008), yellow-eyed (Boessenkool et al., 2010), and African penguins (Dalton et al., 2016) could put them at higher risk to an outbreak. Invasive species can also increase the risk of outbreaks due to the introduction of new parasites (i.e., ticks or fleas) and pathogens transported by these species (van Riper et al., 2002). In addition, invasive species could act as reservoirs of pathogens previously present in the habitat (Tompkins and Poulin, 2006). Thus, the introduction of pathogenic microorganisms that could give rise to emergent infectious disease is a serious concern for penguin conservation (Barbosa et al., 2013). Climate change can affect disease directly, as rising temperatures will probably increase the geographic range, and abundance at lower latitudes, of ectoparasites, as has been the case for ticks feeding on Adélie penguins during high temperature events in Antarctica (Benoit et al., 2009). These increases are linked with infections by vector borne diseases. Furthermore, when penguins shift their distribution in response to climate change this could also lead them to colonize areas where they are exposed to novel pathogens (Vanstreels et al., 2017). Shifts in prey distribution due to climate change can also have an indirect effect on susceptibility to disease and can increase a host’s exposure to new parasites (Xavier et al., 2013). Effect of disease on the prey can also affect penguin indirectly as is the case in the little penguin, when massive die-off of prey resulted in high penguin mortality and the lowest breeding success in 50-year record (Chiaradia et al., 2010). Factors affecting immunocompetence, such as pollutants (Jara-Carrasco et al., 2015) but also starvation, human disturbance, etc., can also be an additive factor reducing disease resistance.
Human Disturbance Impact on Penguins
Disturbance From Visitors
Many tourists anticipate close-up, personal encounters when visiting penguin breeding sites or landing beaches. Recent technological developments encourage people to share their experiences immediately via public media, often using tablets and smartphones, but these have not been developed with sensitive wildlife in mind. Unaware of wildlife sensitivities, many tourists behave in an irresponsible manner for example considering penguins as an entertainment option, disregarding reserve restrictions and regulations, and chase after fleeing birds for penguin photo opportunities (Weeden, 2013). For example, due to an increasing number of such incidents, a popular penguin-viewing hide in New Zealand has recently had to be dismantled to discourage visitor presence and thus to better protect a breeding area of the endangered yellow-eyed penguin.
In addition, habitat can degrade due to human presence (tourists but also researchers in some instances). For example, in the Puñihuil Islands in southern Chile, unregulated tourism activities led to an increased incidence of nesting burrow collapse and a decline in numbers of both Humboldt and magellanic penguins (Simeone and Schlatter, 1998). More difficult to manage are natural predators that can take advantage of the distraction caused by human presence at penguin breeding areas to snatch eggs and chicks (Giese, 1996; Descamps et al., 2005). At Cape Crozier, Antarctica, Adélie penguin breeding groups that were reduced in size through human disturbance were unable to resist skua Stercorarius spp. attacks (Oelke, 1978). Note human presence does not always bring negative outcome to penguins. In recent decades, African penguins have established new colonies near urban settlements which provide some level of protection against the pressure exerted by terrestrial predators, such as wild felids (Whittington et al., 1996).
More impactful, yet often overlooked, are the subtle and cumulative effects of frequent, low-level human disturbance on behavior and physiology of affected birds, which can ultimately affect breeding populations (Ellenberg, 2017; Dunn et al., 2018).
A solitary visitor quietly standing 20 m from an established Adélie penguin pathway resulted in a 70 m penguin detour that was maintained for several hours after the person had left. This caused the 12,000 birds using the track during a 10-h period to travel an extra estimated 840 km (Culik and Wilson, 1995). Yellow-eyed penguins will not even come ashore if people are visible on landing beaches. At frequently disturbed sites, this leads to reduced fledging weights, and ultimately lower first year survival and recruitment (Wright, 1998; McClung et al., 2004; Ellenberg et al., 2007).
Visitor guidelines are often based on overt behavioral responses. However, human disturbance can also disrupt vital behaviors and induce immobilization. Even without any behavioral reaction, human presence can increase energy demands and compromise the immune system through physiological stress responses (Regel and Pütz, 1997; Ellenberg et al., 2013). Effective mitigation of the potentially adverse effects of human visitation can arise only from detailed site- and species-specific research (Ellenberg and Seddon, 2009; Ellenberg, 2017). Using heart-rate recording dummy eggs to minimize observer effects, researchers found that heart rates of penguins can double or even triple during human approach (Nimon et al., 1995; Ecks, 1996; Ellenberg et al., 2006, 2009, 2013; Viblanc et al., 2012, 2015). Without any changes in behavior, the heart rate increase in response to the careful approach of a single human was greater than that during direct overflight of a predatory skua (Holmes et al., 2005). Once the human had retreated out of sight, Humboldt penguins needed up to 30 min to recover with little evidence of habituation to even minor human disturbance (Ellenberg et al., 2006). Because penguin heart rate is linearly correlated to metabolic rate (Green et al., 2005), human disturbance will increase energy consumption and the costs of repeated disturbance events can accumulate, which can be particularly challenging during times of limited energy reserves such as breeding or molting (McClung et al., 2004; Ellenberg et al., 2007, 2013).
Elevated heart rate is part of the vertebrate stress response, and is mediated by a rapid release of adrenaline. Short-term stress responses are often beneficial enabling individuals to escape from, or cope with, challenging situations. However, repeated stressful events can lead to the long-term elevation of corticosterone, which in turn can result in higher susceptibility to disease, lower fertility and reduced life expectancy (Walker et al., 2005a; Ellenberg et al., 2007).
Individual stress-coping styles differ markedly even within the same species depending upon a range of factors that we are just beginning to appreciate (Ellenberg, 2017). Behavioral responses may also differ depending on the stage of the breeding season (Villanueva et al., 2014) and on the past experiences of human interaction both at the individual and population levels (Villanueva et al., 2012; Pichegru et al., 2016; Cockrem et al., 2017). While the long-term exposure to tourist visitation leads to behavioral and physiological habituation, possibly with a decrease in adrenocortical function (Walker et al., 2005b, 2006; Villanueva et al., 2012, 2014), repeated instances of acute stress (e.g., capture and handling for research) in otherwise undisturbed areas can cause heightened behavioral and physiological responses rather than habituation (Carroll et al., 2016; Pichegru et al., 2016). Even closely related species may respond differently to human disturbance (Ellenberg et al., 2006). For example, breeding success of Magellanic penguins was not affected in Punta Tombo, Argentina, where visitors can walk freely among nests and approach penguins to within a few meters of nest sites (Yorio and Boersma, 1992); whereas a Humboldt penguin colony exposed to visitors at close range had virtually no reproductive output (Ellenberg et al., 2006) and has since ceased to exist.
A single disturbance event is generally not much of a problem; it is the accumulating impact of repeated, undermanaged human visitation that can adversely affect breeding populations (Ellenberg, 2017). For example, human passage through low-density breeding areas of African penguins caused not only egg loss and the exodus of birds, but prevented nest-site prospecting (Hockey and Hallinan, 1981). Similarly, human visits have adversely affected the recruitment of pre-breeding birds to Adélie penguin colonies (Woehler et al., 1994). Adélie penguin colonies exposed to recreational visits hatched only half the number of chicks compared to neighboring undisturbed areas, and chick survival was reduced by up to 80% at tourist sites due to ineffective brooding leading to retarded development of the embryos or hypothermia of chicks (Giese, 1996).
Disturbance From Aerial and Ground Transport
The effects of ground transport and/or traffic resulting from tourist visits, such as road kill associated with higher traffic to and from penguin viewing areas, are obvious and can severely impact populations (Heber et al., 2008). Proactive ecotourism managers have adopted measures seen to be very successful with other wildlife including wildlife traffic signs, closing access roads after dark, and/or installing penguin tunnels and fences to successfully mitigate traffic interaction (Clevenger et al., 2001; Little et al., 2002).
Aerial traffic can have devastating consequences. For example, approximately 7,000 king penguins died by asphyxiation when a single overflight by a Hercules aircraft caused a panic stampede on Macquarie Island (Rounsevell and Binns, 1991; Cooper et al., 1994). While an extreme example, this event does illustrate that even without bad intentions human disturbance can result in significant penguin mortality. In 2004, regulations were put in place to restrict aircraft flights near seabird colonies, at least in Antarctica and the sub-Antarctic Islands (Harris, 2005). These guidelines recommend no aircrafts fly over bird colonies below 610 m, and avoid landings within 0.5 nm (ca. 930 m) “if possible.” Most penguins do respond at considerably greater distances indicating current guidelines are inadequate (Ellenberg, 2017). The advent of Remote Control Vehicles, especially recreational drones, is providing new operational and conservation management challenges (see Rümmler et al., 2018; Weimerskirch et al., 2018).
Similarly, increasing human usage of coastal waters means that penguins, whether deep- or shallow-diving ones, are at risk of disturbance and injury from watercraft.
Mitigation
Focusing on the Major Threats
While the last IUCN Red List penguin assessment reported more than half of the world’s penguin species as threatened with extinction there was some good news for three species of penguins, Adélie, gentoo and little penguins, as these are faring better at the global species level than in the previous assessment. Nevertheless, it is evident that all penguin species are affected by each of the threats considered here, and the others considered by Trathan et al. (2015), but each to varying degrees (Figure 1). Occurrence (presence/absence) data for penguin species from BirdLife International3 were summed to identify the number of penguin species at any particular location. We then assessed the spatial congruence (overlap) between the global coastal distribution of penguins and the main threats in order to identify the species most highly impacted.
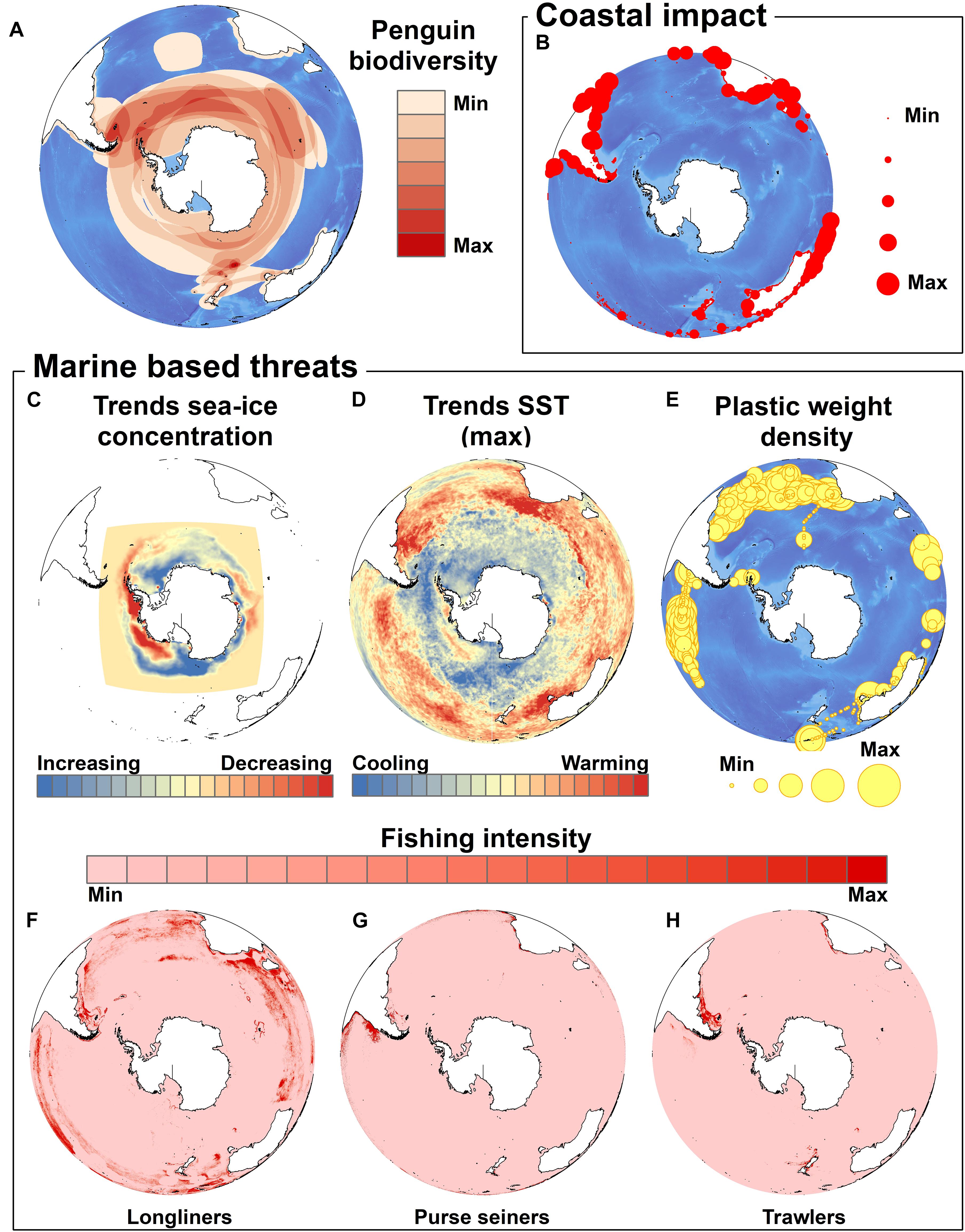
Figure 1. Major coastal and marine-based threats for penguins. The global occurrence of penguins is depicted as the number of penguin species at any particular location (data from Birdlife International) (A). The sum of coastal human populations within a 10 km, moving window around any coastal cell (B) was used as a proxy for the intensity of coastal impact (see Halpern et al., 2015). The main marine threats we considered are (C) sea ice trends over 38 years (1979–2017) using averaged monthly data for each year (Fetterer et al., 2017), (D) the slopes of least-square linear regressions of maximum annual SST for a given cell represented here as a proxy for the magnitude of SST change over the 1982–2016 time period (“optimum interpolation SST” sourced at http://www.esrl.noaa.gov/psd/), (E) plastic density (both count and weight density) for 1571 locations around the world (from Eriksen et al., 2014), and the spatial distribution of daily fishing effort (the period that a vessel spends at-sea fishing actively) for (F) longliners, (G) purse seiners and (H) trawlers for the 2012–2017 period (Global Fishing Watch, http://globalfishingwatch.org/; see also Kroodsma et al., 2018).
The ubiquitous nature of certain threats is best illustrated by the physical changes affecting the environment. The effects of climate change differ in intensity at regional scale. As such, some species are more at risk than others and are likely to fare more badly from alteration of their habitats. For example, in the Antarctic, the penguin species that are most at risk are probably those that depend upon sea ice – a rapidly changing habitat. Changes in the oceans in more temperate areas are also impacting other species such as African penguins. In this case, intensive exposure to resource competition from fisheries overlaying the consequences of climate change complicates the situation. The impact of climate change is expected to intensify, potentially making populations less resilient to non-climate related impacts (Crawford et al., 2017). Thus, how penguin populations cope and respond to further climate change will depend to some extent upon how other current terrestrial and marine threats are addressed (Trathan et al., 2015). While there is a sense of inevitability of climate change impacts on penguin populations (Mattern et al., 2017), efforts to improve the resilience of these animals to climate change through management of the marine and terrestrial environments are still possible and should be considered a matter of urgency (Boersma et al., 2015; Crawford et al., 2015, 2017; Trathan et al., 2015, 2018; Mattern et al., 2017).
Penguin biogeographic range distributions follow the major cold oceanographic currents; either boundary currents of intensive upwelling such as the Humboldt Current and Falkland Current in South America, the Benguela Current in southern Africa, and the West Australian Current, or more complex currents like the Antarctic Circumpolar Current whose northern boundary corresponds to a downwelling front. As these currents and SST change, so will penguin foraging habitat, with potential consequences on survival and productivity. There are evident regional differences in both sea ice change and SST change, with important consequences for future species persistence (e.g., Jenouvrier et al., 2014, 2017) (Figure 1). Greatest SST increases are likely to occur in the sub-Antarctic and temperate latitudes, with other major increasing trends evident in the waters off Tasmania and southeast Australia (little), Argentina and Uruguay (Magellanic), southern South Africa (African), and to a lesser degree southern mainland New Zealand (yellow-eyed and fiordland), southern Chile (Humboldt), and the Antarctic Peninsula (gentoo, chinstrap, Adélie).
Species breeding in regions with permanent human settlements in more temperate environments are more strongly impacted by the presence of human activity close to the breeding sites than those living in more remote areas. The index of human disturbance highlights a number of regions experiencing moderate to severe coastal impacts. The regions of highest coastal impacts include Tasmania and southeast Australia (little), the New Zealand mainland (yellow-eyed, fiordland, little), Argentina4 (Magellanic), Chile (Humboldt, Magellanic), Peru (Humboldt), and South Africa and Namibia (African), but not Antarctica, nor oceanic islands in the Pacific, Atlantic or Indian Oceans. Penguins breeding in areas with severe coastal impacts from human activities will be at risk from increased human disturbance, loss of nesting habitat to infrastructure development, increased pressures from companion animals such as cats and dogs, and from urban-adaptor invasive predators. Coastal foraging areas will be subject to increased vessel traffic and will be affected by the changes brought about by land-based activities that can modify or destroy natural habitats, cause runoff of sediments, nutrients, and pollutants, and even alter the flow of currents and tides (Trathan et al., 2015). Coastal marine pollution also originates from sources other than those described above. For example, coastal and inshore mining operation along Namibia’s southern coast threaten foraging habitats of African penguins through the large-scale release of sediment into coastal waters. Water turbidity can reduce prey availability and is likely to affect foraging behavior. Sediment movement also contributes to the formation of temporary land bridges to some islands, which allows access by land predators (Kemper, 2006).
Fishing, especially in the vicinity of penguin colonies has major potential consequences for penguins that rely upon the same species targeted commercially (e.g., Skewgar et al., 2007; Sherley et al., 2018; Trathan et al., 2018; Warwick-Evans et al., 2018). Data quantifying the intensity of fishing effort by trawlers and long-liners offshore exist from many areas, including from Namibia and South Africa (African penguins), Argentina and Uruguay (Magellanic penguins), southern Chile (Humboldt penguins), and New Zealand (yellow-eyed penguins), indicating hotspots of both trawler and long-liner activity around the Falklands Islands (Islas Malvinas) (Magellanic and southern rockhopper penguins), South Georgia, Crozet archipelago, and Heard Island (king, southern rockhopper, and macaroni penguins). High intensity purse-seine fisheries’ activity off the coasts of Chile, Argentina, and southwest South Africa are known to, or have the potential to, compete for the main prey species of Humboldt, Magellanic, and African penguins (e.g., Bertrand et al., 2012; Sherley et al., 2018). Currently, fine-scale data to document inshore gillnetting do not exist, but it is known that high intensity gillnet fishing does impact penguins (Crawford et al., 2017).
Proactive Management
Implementing mitigation measures to address threats to penguins is challenging. For example, climate change is a global issue, not influenced by political boundaries, that requires intergovernmental effort at a global scale, whilst limiting coastal development and regulating marine-capture fisheries continues to be a growing challenge as 250 additional humans are born every minute (The World Factbook, 2016/2017). With such pressures, one of the critical challenges facing ecologists is the attribution of cause through analyzing long-term data that describe changes in functional traits (e.g., body condition, foraging activity), vital rates (e.g., survival, fecundity) and population size (Jenouvrier et al., 2018; Otley et al., 2018). Few studies have accounted for the effects of stressors, such as climate change, on the complete life cycle of species, thereby accounting for multiple seasonal and carry-over effects of the stressor (Jenouvrier et al., 2012; Iles and Jenouvrier, 2019). Elucidating such whole life cycle impacts is challenging as the potential drivers may be numerous and diverse; moreover, many species are difficult to observe over large periods of their life history. Furthermore, some drivers have indirect effects in that they can alter the structure and functioning of marine systems as a whole (e.g., Croxall et al., 2012). For instance, penguin prey could benefit from increased nutrient availability, but then may also face increased competition, reducing overall prey abundance for penguins (Bulman et al., 2008; Jørgensen, 2009; Thompson et al., 2011). Although this may not always be sufficient, enhancing species’ resilience to environmental change could rely on addressing local-scale impacts (Mattern et al., 2017). For example, penguins at sea face many other (non-climate related) threats, which, if addressed locally, could enhance their resilience to threats from climate change (Crawford et al., 2017). The development of marine spatial planning leading to marine protected areas that include core foraging areas and traveling routes to and from foraging grounds (García Borboroglu et al., 2008; Trathan et al., 2014; Boersma et al., 2015), the establishment of new penguin colonies nearer the location of food (Crawford et al., 2015), and the identification and protection of refugia which have supported species through periods of intense past environmental change (Levy et al., 2016; Younger et al., 2016), could all help protect penguins. Where management resources are limited, efforts to protect the residual populations of penguins that have survived changes to date may prove the best management strategy (Crawford et al., 2015). However, none of the aforementioned mitigation measures are worth if, in parallel, mankind does not reduce its emissions for a sustainable long-term future.
Many of the successful conservation stories originate from locations that have some form of conservation plan in action. The mega colony of little penguins at Phillip Island, Australia illustrates how strong conservation actions can improve resilience to overcome severe threats. Little penguins suffered from a series of major threats in the 1990s: highland-introduced predation, rapid habitat destruction, a couple of strong El Niño years and a massive mortality of their major prey, sardines S. sagax (Chiaradia, 2013) – the largest single fish species mortality ever recorded in marine system (Jones et al., 1997). Remarkably, these pressures were counterbalanced by a wide range of conservation efforts. Introduced predators like red foxes Vulpes vulpes were systematically eradicated and an entire housing estate has been demolished and returned to penguin habitat by the State Government. Restoring natural conditions on land reversed the bleak prediction that this penguin colony was facing. The case of African penguins triggered a large-scale experiment which started in 2008; it sought to determine whether the local fisheries for sardine and anchovy contributed to observed penguin population declines. Temporally alternating closures based on the penguins’ core foraging area around two penguin breeding colonies were instigated to assess impacts on penguin foraging behavior and population dynamics. The closures increased prey and decreased foraging effort by breeding adults (Pichegru et al., 2012), also increasing chick survival and body condition (Sherley et al., 2015, 2018). Modeling suggests that the apparent benefits should have meaningful effects on long-term population growth, but are probably insufficient to reverse the observed decline in penguin numbers without additional, broad-scale fisheries management interventions (Sherley et al., 2017, 2018). As a direct result of these trial closures and many other research initiatives highlighting the importance of fisheries management and marine protection in general, the South African Department of Environmental Affairs created 20 new marine protected areas after nearly 5 years of extensive consultation and negotiation between all stakeholders. The designation and subsequent management of these protected areas begins in 2019 and will increase the size of protected South African marine territory from 0.4 to 5%. Of these 20 new MPAs, two (the expansion of the Addo Elephant National Park and the creation of Robben Island) include the protection of foraging areas, for species such as the African penguin, as reasons for/benefits of their designation. This includes the trial closure areas around the two African penguin breeding colonies and a large area surrounding another large, island breeding colony5.
Penguin conservation and management requires both national and international actions. Though animals from an individual colony may breed and forage within national waters, they may mix beyond the political jurisdiction of one state during the post breeding period. For instance, effects of disease outbreaks brought about by ever-increasing proximity to humans should be considered as a major threat for penguin species. However, relevant information on disease remains scarce, despite the high risk of catastrophic penguin die-offs. Similarly, there is still much to learn about what factors drive habituation or sensitization to human presence in wild populations (Ellenberg, 2017). Tourism managers, conservation authorities, and researchers need to work together to develop anticipatory management guidelines that benefit both the penguins and the tourists that come to see them (e.g., Dunn et al., 2018). The long-term sustainability of penguin tourism ventures relies on well-informed adaptive management strategies that effectively minimize any associated negative human impacts. Guidelines based on conservative approach distances for one species may trigger significant physiological responses with associated fitness consequences in another species. Some experimental approaches to understand tourist impacts on penguins have been ongoing for a number of years and conclusions, even after a decade, remained tentative (Trathan et al., 2008). With a longer time series results from the same study became clearer, but it is still difficult to be completely certain about the impacts (Dunn et al., 2018).
The capture and rehabilitation of those oiled penguins that reach shore (sometimes < 50% of the individuals concerned, e.g., García Borboroglu et al., 2008) is often a feasible approach for the mitigation of impacts of oil spills on these species. Some oiled penguins rescued and rehabilitated may, however, have lower breeding success than those that were never oiled, and some never breed again (Giese et al., 2000; Wolfaardt et al., 2008). Experiences from sub-Antarctic and Antarctic oil spills has shown that logistics place critical constraints on successful rehabilitation operations at remote locations (Ruoppolo et al., 2013; Guggenheim and Glass, 2014), suggesting that penguins at remote islands might be particularly vulnerable to oil spills. With continued tanker transport of petroleum, together with the increase in gas exploration and ship to ship bunkering, oil spills are likely to remain significant threats for penguins worldwide in the foreseeable future (García Borboroglu et al., 2008; Garcia-Borboroglu and Boersma, 2013; Woehler et al., 2014). Introductions of bans on Heavy Fuel Oil in the Antarctic are particularly welcome, and should be enacted elsewhere.
The major threats described above should not be the only ones receiving scientific attention. Emerging threats should be monitored and proactive actions should be applied. The increase in plastic particles in the environment of penguins is a good example. Our review highlighted the limited impact that plastics have caused so far to penguins but maps of plastics occurrence from at-sea surveys, despite being heavily influenced by the sampling effort (Figure 1, from Eriksen et al., 2014), suggest that the amount of plastic noted south of 50°S is more likely the result of a reduced monitoring effort in these regions. Dedicated surveys ought to be conducted on a regular basis to evaluate the impact that plastics really have on penguin species.
Conclusion
It is clear that there is a considerable amount of scientific information about the threats affecting penguin species. For some species, there is enough evidence to inform decision-making bodies, and there are evidently a few beacons of hope where there is a clearly link between science and improved conservation. However, a lack of political will, or a focus on other priorities, in a range of countries is potentially one of the single-most important problems contributing to penguin species declines. Indeed, to ensure the long term survival of penguins in the wild, available science must be translated into conservation and wildlife management actions. Only policy makers can bring about the changes needed. In the Antarctic, this is particularly topical; the anticipated ecosystem collapse under current CO2 emission scenarios will probably be accompanied by a socio-political collapse (Rintoul et al., 2018). Successful conservation of penguin species across their geographical range requires work across national and international boundaries (Boersma et al., 2015) for conservation planning and implementation to halt and reverse any negative population trends. In this context, all scientists have an important role to play in disseminating scientific advice, informing policy and engaging people in penguin conservation actions. They should also be active in facilitating development of species action plans, raising awareness, and coordinating and harnessing global efforts to protect penguins. However, it is also up to all of humanity to make the world of penguins a better place; there is still time for this to happen.
Author Contributions
YR-C, AC, and PT conceived the project. All authors wrote the review.
Funding
This work was supported by the WWF-UK and PEW Foundation. SJ is supported by NSF OPP PICA #1643901.
Conflict of Interest Statement
The authors declare that the research was conducted in the absence of any commercial or financial relationships that could be construed as a potential conflict of interest.
Acknowledgments
We thank our numerous scientific colleagues and collaborators for sharing ideas and information, WWF-UK, the PEW foundation, the IUCN Penguin Specialist Group, and all other institutions and people who promote or conduct penguin conservation efforts. We also thank T. Mattern, M. Young, Y. van Heezik, J. Fyfe, and L. Ropert-Kato for critical inputs. Polytrans provided useful technical help during the redaction.
Footnotes
- ^A similar approach – but not using the IPCC scenarios – has been conducted on little penguins (Chambers et al., 2011).
- ^https://iaato.org/home
- ^www.birdlife.org
- ^If considering wintering grounds one must also consider northern Argentina, Uruguay, and Brazil as regions with high coastal impacts on Magellanic penguins.
- ^https://www.environment.gov.za/mediarelease/cabinetapproves_representativenetworkofMPAs
References
Ackerman, J. T., Eagles-Smith, C. A., Herzog, M. P., Hartman, C. A., Peterson, S. H., Evers, D. C., et al. (2016). Avian mercury exposure and toxicological risk across western North America: a synthesis. Sci. Total Environ. 568, 749–769. doi: 10.1016/j.scitotenv.2016.03.071
Adams, N. J. (1994). Patterns and impacts of oiling of African penguins Spheniscus demersus: 1981–1991. Biol. Conserv. 68, 35–41.
Aibers, P. H. (1978). The effects of petroleum of different stages of incubation in bird eggs. Bull. Environ. Contam. Toxicol. 19, 624–630.
Ainley, D., Ballard, G., Ackley, S., Blight, L. K., Eastman, J. T., Emslie, S. D., et al. (2007). Paradigm lost, or is top-down forcing no longer significant in the Antarctic marine ecosystem? Antarct. Sci. 19, 283–290.
Ainley, D., Russell, J., Jenouvrier, S., Woehler, E., Lyver, P. O. B., Fraser, W. R., et al. (2010). Antarctic penguin response to habitat change as Earth’s troposphere reaches 2 C above preindustrial levels. Ecol. Monogr. 80, 49–66.
Ainley, D. G., and Blight, L. K. (2009). Ecological repercussions of historical fish extraction from the Southern Ocean. Fish Fish. 10, 13–38.
Ainley, D. G., Crockett, E. L., Eastman, J. T., Fraser, W. R., Nur, N., O’Brien, K., et al. (2017). How overfishing a large piscine mesopredator explains growth in Ross Sea penguin populations: a framework to better understand impacts of a controversial fishery. Ecol. Modell. 349, 69–75.
Alder, J., Campbell, B., Karpouzi, V., Kaschner, K., and Pauly, D. (2008). Forage fish: from ecosystems to markets. Annu. Rev. Environ. Resour. 33, 153–166. doi: 10.1146/annurev.environ.33.020807.143204
Alley, M. R., Suepaul, R. B., McKinlay, B., Young, M. J., Wang, J., Morgan, K. J., et al. (2017). Diphtheritic stomatitis in Yellow-eyed penguins (Megadyptes antipodes) in New Zealand. J. Wildl. Dis. 53, 102–110. doi: 10.7589/2015-07-195
Álvarez-Varas, R., Morales-Moraga, D., González-Acuña, D., Klarian, S. A., and Vianna, J. A. (2018). Mercury exposure in humboldt (Spheniscus humboldti) and chinstrap (Pygoscelis antarcticus) penguins throughout the Chilean coast and Antarctica. Arch. Environ. Contam. Toxicol. 75, 75–86. doi: 10.1007/s00244-018-0529-7
Bakun, A., Black, B. A., Bograd, S. J., Garcia-Reyes, M., Miller, A. J., Rykaczewski, R. R., et al. (2015). Anticipated effects of climate change on coastal upwelling ecosystems. Curr. Clim. Change Rep. 1, 85–93.
Ballance, L., Pitman, R. L., Hewitt, R. P., Siniff, D. B., Trivelpiece, W. Z., Clapham, P. J., et al. (2006). “The removal of large whales from the Southern Ocean: evidence for long-term ecosystem effects,” in Whales, Whaling and Ocean Ecosystems, eds J. A. Estes, D. P. DeMaster, D. F. Doak, T. M. Williams, and R. L. Brownell Jr. (Berkeley, CA: University of California Press),215–230.
Ballerini, T., Tavecchia, G., Pezzo, F., Jenouvrier, S., and Olmastroni, S. (2015). Predicting responses of the Adélie penguin population of Edmonson Point to future sea ice changes in the Ross Sea. Front. Ecol. Evol. 3:8. doi: 10.3389/fevo.2015.00008
Banks, J., Van Buren, A., Cherel, Y., and Whitfield, J. B. (2006). Genetic evidence for three species of rockhopper penguins, Eudyptes chrysocome. Polar Biol. 30, 61–67.
Barbosa, A., Colominas-Ciuró, R., Coria, N., Centurión, M., Sandler, R., Negri, A., et al. (2015). First record of feather-loss disorder in Antarctic penguins. Antarct. Sci. 27, 69–70.
Barbosa, A., Costa, E. S., Dewar, M., González-Acuña, D., Gray, R., Power, M., et al. (2014). Antarctic Wildlife Diseases. Antarctic Environments Portal.
Barbosa, A., De Mas, E., Benzal, J., Diaz, J. I., Motas, M., Jerez, S., et al. (2013). Pollution and physiological variability in gentoo penguins at two rookeries with different levels of human visitation. Antarct. Sci. 25, 329–338.
Barbosa, A., and Palacios, M. J. (2009). Health of Antarctic birds: a review of their parasites, pathogens and diseases. Polar Biol. 32, 1095–1115.
Barbraud, C., Rolland, V., Jenouvrier, S., Nevoux, M., Delord, K., and Weimerskirch, H. (2012). Effects of climate change and fisheries bycatch on Southern Ocean seabirds: a review. Mar. Ecol. Prog. Ser. 454, 285–307.
Barbraud, C., and Weimerskirch, H. (2001). Emperor penguins and climate change. Nature 411, 183–186.
Bargagli, R., Monaci, F., Sanchez-Hernandez, J. C., and Cateni, D. (1998). Biomagnification of mercury in an Antarctic marine coastal food web. Mar. Ecol. Prog. Ser. 169, 65–76.
Barrows, A. P. W., Cathey, S. E., and Petersen, C. W. (2018). Marine environment microfiber contamination: global patterns and the diversity of microparticle origins. Environ. Pollut. 237, 275–284. doi: 10.1016/j.envpol.2018.02.062
Becker, P. H., González-Solís, J., Behrends, B., and Croxall, J. (2002). Feather mercury levels in seabirds at South Georgia: influence of trophic position, sex and age. Mar. Ecol. Prog. Ser. 243, 261–269.
Becker, P. H., Goutner, V., Ryan, P. G., and González-Solís, J. (2016). Feather mercury concentrations in Southern Ocean seabirds: variation by species, site and time. Environ. Pollut. 216, 253–263. doi: 10.1016/j.envpol.2016.05.061
Behrenfeld, M. J., O’Malley, R. T., Siegel, D. A., McClain, C. R., Sarmiento, J. L., Feldman, G. C., et al. (2006). Climate-driven trends in contemporary ocean productivity. Nature 444, 752–755.
Benoit, J. B., Lopez-Martinez, G., Elnitsky, M. A., Lee, R. E., and Denlinger, D. L. (2009). Short Note: increase in feeding by the tick, Ixodes uriae, on Adelie penguins during a prolonged summer. Antarct. Sci. 21, 151–152.
Bertrand, S., Joo, R., Smet, C. A., Tremblay, Y., Barbraud, C., and Weimerskirch, H. (2012). Local depletion by a fishery can affect seabird foraging. J. Appl. Ecol. 49, 1168–1177. doi: 10.1111/j.1365-2664.2012.02190.x
Beyer, A., Mackay, D., Matthies, M., Wania, F., and Webster, E. (2000). Assessing long-range transport potential of persistent organic pollutants. Environ. Sci. Technol. 34, 699–703.
BirdLife International (2018). State of the World’s Birds: Taking the Pulse of the Planet. Cambridge: BirdLife International.
Boersma, P. D. (1978). Breeding patterns of Galapagos penguins as an indicator of oceanographic conditions. Science 200, 1481–1483.
Boersma, P. D., and Rebstock, G. A. (2014). Climate change increases reproductive failure in Magellanic penguins. PLoS One 9:e85602. doi: 10.1371/journal.pone.0085602
Boersma, P. D., Rebstock, G. A., and García-Borboroglu, P. (2015). Marine protection is needed for Magellanic penguins in Argentina based on long-term data. Biol. Conserv. 182, 197–204.
Boersma, P. D., Steinfurth, A., Merlen, G., Jiménez-Uzcátegui, G., Vargas, F., and Parker, P. G. (2013). “Galápagos penguin (Spheniscus mendiculus),” in Penguins Natural History and Conservation, eds P. G. Borboroglu and P. D. Boersma (Seattle, WA: University of Washington Press), 360.
Boessenkool, S., Star, B., Seddon, P. J., and Waters, J. M. (2010). Temporal genetic samples indicate small effective population size of the endangered yellow-eyed penguin. Conserv. Genet. 11, 539–546.
Bollmer, J. L., Vargas, F. H., and Parker, P. G. (2007). Low MHC variation in the endangered Galapagos penguin (Spheniscus mendiculus). Immunogenetics 59, 593–602.
Borowicz, A., McDowall, P., Youngflesh, C., Sayre-McCord, T., Clucas, G., Herman, R., et al. (2018). Multi-modal survey of Adélie penguin mega-colonies reveals the Danger Islands as a seabird hotspot. Sci. Rep. 8:3926. doi: 10.1038/s41598-018-22313-w
Bost, C. A., Cotté, C., Terray, P., Barbraud, C., Bon, C., Delord, K., et al. (2015). Large-scale climatic anomalies affect marine predator foraging behaviour and demography. Nat. Commun. 6:8220. doi: 10.1038/ncomms9220
Branch, T. A. (2011). Humpback whale abundance south of 60°S from three complete circumpolar sets of surveys. J. Cetacean Res. Manag. 9, 253–262.
Brandão, M. L., Braga, K. M., and Luque, J. L. (2011). Marine debris ingestion by Magellanic penguins, Spheniscus magellanicus (Aves: Sphenisciformes), from the Brazilian coastal zone. Mar. Pollut. Bull. 62, 2246–2249. doi: 10.1016/j.marpolbul.2011.07.016
Brasso, R. L., Chiaradia, A., Polito, M. J., Rey, A. R., and Emslie, S. D. (2015). A comprehensive assessment of mercury exposure in penguin populations throughout the Southern Hemisphere: using trophic calculations to identify sources of population-level variation. Mar. Pollut. Bull. 97, 408–418. doi: 10.1016/j.marpolbul.2015.05.059
Brasso, R. L., and Polito, M. J. (2013). Trophic calculations reveal the mechanism of population-level variation in mercury concentrations between marine ecosystems: case studies of two polar seabirds. Mar. Pollut. Bull. 75, 244–249. doi: 10.1016/j.marpolbul.2013.08.003
Brooke, M. D. L. (2004). The food consumption of the world’s seabirds. Proc. R. Soc. Lond. B Biol. Sci. 271(Suppl. 4), S246–S248. doi: 10.1098/rsbl.2003.0153
Brooks, C. M. (2013). Competing values on the Antarctic high seas: CCAMLR and the challenge of marine-protected areas. Polar J. 3, 277–300.
Brooks, C. M., Crowder, L. B., Curran, L. M., Dunbar, R. B., Ainley, D. G., Dodds, K. J., et al. (2016). Science-based management in decline in the Southern Ocean. Science 354, 185–187.
Browne, M. A., Crump, P., Niven, S. J., Teuten, E., Tonkin, A., Galloway, T., et al. (2011). Accumulation of microplastic on shorelines worldwide: sources and sinks. Environ. Sci. Technol. 45, 9175–9179. doi: 10.1021/es201811s
Bulman, C. M., Condie, S. A., Neira, F. J., Goldsworthy, S. G., and Fulton, E. A. (2008). The trophodynamics of small pelagic fishes in the southern Australian ecosystem and the implications for ecosystem modelling of southern temperate fisheries. FRDC Final Rep. 28:132.
Butler, R. G., Harfenist, A., Leighton, F. A., and Peakall, D. B. (1988). Impact of sublethal oil and emulsion exposure on the reproductive success of Leach’s storm-petrels: short and long-term effects. J. Appl. Ecol. 25, 125–143.
Calle, P., Alvarado, O., Monserrate, L., Cevallos, J. M., Calle, N., and Alava, J. J. (2015). Mercury accumulation in sediments and seabird feathers from the Antarctic Peninsula. Mar. Pollut. Bull. 91, 410–417. doi: 10.1016/j.marpolbul.2014.10.009
Camphuysen, C. J. (2008). Verstrikkingen van zeevogels in zwerfvuil en vistuig, 1970–2007. Sula 21, 88–92.
Cannell, B. L., Chambers, L. E., Wooller, R. D., and Bradley, J. S. (2012). Poorer breeding by little penguins near Perth, Western Australia is correlated with above average sea surface temperatures and a stronger Leeuwin Current. Mar. Freshw. Res. 63, 914–925.
Carlini, A. R., Coria, N. R., Santos, M. M., Negrete, J., Juares, M. A., and Daneri, G. A. (2009). Responses of Pygoscelis adeliae and P. papua populations to environmental changes at Isla 25 de Mayo (King George Island). Polar Biol. 32, 1427–1433.
Carrasco, L., Lima, J. S. Jr., Halfen, D. C., Salguero, F. J., Sánchez-Cordón, P., and Becker, G. (2001). Systemic aspergillosis in an oiled magellanic penguin (Spheniscus magellanicus). J. Vet. Med. Ser. B 48, 551–554.
Carravieri, A., Bustamante, P., Churlaud, C., and Cherel, Y. (2013). Penguins as bioindicators of mercury contamination in the Southern Ocean: birds from the Kerguelen Islands as a case study. Sci. Total Environ. 454, 141–148. doi: 10.1016/j.scitotenv.2013.02.060
Carravieri, A., Cherel, Y., Jaeger, A., Churlaud, C., and Bustamante, P. (2016). Penguins as bioindicators of mercury contamination in the southern Indian Ocean: geographical and temporal trends. Environ. Pollut. 213, 195–205. doi: 10.1016/j.envpol.2016.02.010
Carroll, G., Turner, E., Dann, P., and Harcourt, R. (2016). Prior exposure to capture heightens the corticosterone and behavioural responses of little penguins (Eudyptula minor) to acute stress. Conserv. Physiol. 4:cov061. doi: 10.1093/conphys/cov061
Chambers, L. E., Devney, C. A., Congdon, B. C., Dunlop, N., Woehler, E. J., and Dann, P. (2011). Observed and predicted effects of climate on Australian seabirds. Emu 111, 235–251.
Chambers, L. E., Renwick, L., and Dann, P. D. (2010). “Climate, fire and the little penguin,” in Australia’s Biodiversity and Climate Change: a Strategic Assessment of the Vulnerability of Australia’s Biodiversity to Climate Change. A Report to the Natural Resource Management Ministerial Council Commissioned by the Commonwealth Department of Climate Change, eds W. Steffen, A. A. Burbidge, L. Hughes, R. Kitching, D. Lindenmayer, W. Musgrave, et al. (Clayton, MO: CSIRO Publishing).
Chapman, E. W., Hofmann, E. E., Patterson, D. L., Ribic, C. A., and Fraser, W. R. (2011). Marine and terrestrial factors affecting Adélie penguin Pygoscelis adeliae chick growth and recruitment off the western Antarctic Peninsula. Mar. Ecol. Prog. Ser. 436, 273–289.
Che-Castaldo, C., Jenouvrier, S., Youngflesh, C., Shoemaker, K. T., Humphries, G., McDowall, P., et al. (2017). Pan-Antarctic analysis aggregating spatial estimates of Adélie penguin abundance reveals robust dynamics despite stochastic noise. Nat. Commun. 8:832.
Cherel, Y., and Hobson, K. A. (2007). Geographical variation in carbon stable isotope signatures of marine predators: a tool to investigate their foraging areas in the Southern Ocean. Mar. Ecol. Prog. Ser. 329, 281–287.
Cherel, Y., Hobson, K. A., Guinet, C., and Vanpe, C. (2007). Stable isotopes document seasonal changes in trophic niches and winter foraging individual specialization in diving predators from the Southern Ocean. J. Anim. Ecol. 76, 826–836.
Chiaradia, A. (2013). “The smallest penguin faces big challenges,” in Penguins: Their World, their Ways, ed. T. Roy (Clayton, MO: CSIRO Publishing).
Chiaradia, A., Forero, M. G., Hobson, K. A., and Cullen, J. M. (2010). Changes in diet and trophic position of a top predator ten years after a mass mortality of a key prey. ICES J. Mar. Sci. 67, 1710–1720.
Chiaradia, A. F., and Kerry, K. R. (1999). Daily nest attendance and breeding performance in the little penguin Eudyptula minor at Phillip Island, Australia. Mar. Ornithol. 27, 13–20.
Cimino, M. A., Lynch, H. J., Saba, V. S., and Oliver, M. J. (2016). Projected asymmetric response of Adélie penguins to Antarctic climate change. Sci. Rep. 6:28785.
Clevenger, A. P., Chruszcz, B., and Gunson, K. E. (2001). Highway mitigation fencing reduces wildlife-vehicle collisions. Wildl. Soc. Bull. 29, 646–653.
Cockrem, J. F., Candy, E. J., Barrett, D. P., Agnew, P., and Potter, M. A. (2017). Individual variation and repeatability of corticosterone responses of little penguins (Eudyptula minor) sampled in two successive years at Oamaru, New Zealand. Gen. Comp. Endocrinol. 244, 86–92. doi: 10.1016/j.ygcen.2016.01.010
Coetzee, J. C., Van der Lingen, C. D., Hutchings, L., and Fairweather, T. P. (2008). Has the fishery contributed to a major shift in the distribution of South African sardine? ICES J. Mar. Sci. 65, 1676–1688.
Connan, M., Hofmeyr, G. G., and Pistorius, P. A. (2016). Reappraisal of the trophic ecology of one of the world’s most threatened spheniscids, the African penguin. PLoS One 11:e0159402. doi: 10.1371/journal.pone.0159402
Conroy, J. W. H., and French, M. C. (1974). Organochlorine levels in two species of Antarctic birds. Br. Antarct. Surv. Bull. 38, 43–47.
Cooper, J., Avenant, N. L., and Lafite, P. W. (1994). Airdrops and king penguins: a potential conservation problem at sub-Antarctic Marion Island. Polar Rec. 30, 277–282.
Cooper, J. O. H. N., Crawford, R. J., De Villiers, M. S., Dyer, B. M., Hofmeyr, G. G., and Jonker, A. (2009). Disease outbreaks among penguins at sub-Antarctic Marion Island: a conservation concern. Mar. Ornithol. 37, 193–196.
Crawford, R., Ellenberg, U., Frere, E., Hagen, C., Baird, K., Brewin, P., et al. (2017). Tangled and drowned: a global review of penguin bycatch in fisheries. Endanger. Spec. Res. 34, 373–396.
Crawford, R. J., Makhado, A. B., Waller, L. J., and Whittington, P. A. (2014). Winners and losers–responses to recent environmental change by South African seabirds that compete with purse-seine fisheries for food. Ostrich 85, 111–117.
Crawford, R. J., Makhado, A. B., Whittington, P. A., Randall, R. M., Oosthuizen, W. H., and Waller, L. J. (2015). A changing distribution of seabirds in South Africa—the possible impact of climate and its consequences. Front. Ecol. Evol. 3:10. doi: 10.3389/fevo.2015.00010
Crawford, R. J. M., Altwegg, R., Barham, B. J., Barham, P. J., Durant, J. M., Dyer, B. M., et al. (2011). Collapse of South Africa’s penguins in the early 21st century. Afr. J. Mar. Sci. 33, 139–156.
Crawford, R. J. M., David, J. H. M., Shannon, L. J., Kemper, J., Klages, N. T. W., Roux, J. P., et al. (2001). African penguins as predators and prey—coping (or not) with change. Afr. J. Mar. Sci. 23, 435–447.
Crawford, R. J. M., Davis, S. A., Harding, R. T., Jackson, L. F., Leshoro, T. M., Me yer, M. A., et al. (2000). Initial impact of the Treasure oil spill on seabirds off western South Africa. Afr. J. Mar. Sci. 22, 157–176. doi: 10.2989/025776100784125645
Crawford, R. J. M., Kemper, J., and Underhill, L. G. (2013). “African penguin (Spheniscus demersus),” in Penguins Natural History and Conservation, eds P. Garcia-Borboroglu and P. D. Boersma (Seattle, WA: University of Washington Press), 211–231.
Cristofari, R., Liu, X., Bonadonna, F., Cherel, Y., Pistorius, P., Le Maho, Y., et al. (2018). Climate-driven range shifts of the king penguin in a fragmented ecosystem. Nat. Clim. Change 8, 245–251. doi: 10.1038/s41558-018-0084-2
Croxall, J. P., Butchart, S. H., Lascelles, B. E. N., Stattersfield, A. J., Sullivan, B., Symes, A., et al. (2012). Seabird conservation status, threats and priority actions: a global assessment. Bird Conserv. Inter. 22, 1–34. doi: 10.1017/s0959270912000020
Cury, P., and Shannon, L. (2004). Regime shifts in upwelling ecosystems: observed changes and possible mechanisms in the northern and southern Benguela. Prog. Oceanogr. 60, 223–243.
Dalton, D. L., Vermaak, E., Roelofse, M., and Kotze, A. (2016). Diversity in the toll-like receptor genes of the African penguin (Spheniscus demersus). PLoS One 11:e0163331. doi: 10.1371/journal.pone.0163331
D’Amico, V. L., Bertellotti, M., Díaz, J. I., Coria, N., Vidal, V., and Barbosa, A. (2014). Leucocyte levels in some Antarctic and non-Antarctic penguins. Ardeola 61, 145–152.
de Lisle, G. W., Stanislawek, W. L., and Moors, P. J. (1990). Pasteurella multocida infections in rockhopper penguins (Eudyptes chrysocome) from Campbell Island, New Zealand. J. Wildl. Dis. 26, 283–285.
de Villiers, M. (2002). Effect of a storm on breeding African penguins Spheniscus demersus at foxy beach, boulders penguin colony, Simon’s town. Bird Numbers 11, 7–9.
Deagle, B. E., Gales, N. J., Evans, K., Jarman, S. N., Robinson, S., Trebilco, R., et al. (2007). Studying seabird diet through genetic analysis of faeces: a case study on macaroni penguins (Eudyptes chrysolophus). PLoS One 2:e831. doi: 10.1371/journal.pone.0000831
Dehnhard, N., Jaspers, V. L., Demongin, L., Van den Steen, E., Covaci, A., Pinxten, R., et al. (2017). Organohalogenated contaminants in plasma and eggs of rockhopper penguins: does vitellogenin affect maternal transfer? Environ. Pollut. 226, 277–287. doi: 10.1016/j.envpol.2017.03.071
Demongin, L., Poisbleau, M., Strange, I. J., and Quillfeldt, P. (2010). Effects of severe rains on the mortality of southern rockhopper penguin (Eudyptes chrysocome) chicks and its impact on breeding success. Ornithol. Neotrop. 21, 439–443.
Descamps, S., Gauthier-Clerc, M., Le Bohec, C., Gendner, J. P., and Le Maho, Y. (2005). Impact of predation on king penguin Aptenodytes patagonicus in Crozet Archipelago. Polar Biol. 28, 303–310.
Dewar, M. L., Arnould, J. P., Dann, P., Trathan, P., Groscolas, R., and Smith, S. (2013). Interspecific variations in the gastrointestinal microbiota in penguins. Microbiologyopen 2, 195–204. doi: 10.1002/mbo3.66
Diaz, J. I., Fusaro, B., Vidal, V., González-Acuña, D., Costa, E. S., Dewar, M., et al. (2017). “Macroparasites in Antarctic penguins,” in Biodiversity and Evolution of Parasitic Life in the Southern Ocean, eds S. Klimpel, T. Kuhn, and H. Mehlhorn (Cham: Springer), 183–204.
dos Santos, I. R., Silva-Filho, E. V., Schaefer, C., Sella, S. M., Silva, C. A., Gomes, V., et al. (2006). Baseline mercury and zinc concentrations in terrestrial and coastal organisms of Admiralty Bay, Antarctica. Environ. Pollut. 140, 304–311.
Duffy, D. C., Hays, C., and Plenge, M. (1984). “The conservation status of Peruvian seabirds,” in Status and Conservation of the World’s Seabirds (No. 2), eds J. P. Croxall, P. G. Evans, and R. W. Schreiber (Princeton, NJ: Princeton University Press), 45–260.
Dunn, M. J., Forcada, J., Jackson, J. A., Waluda, C. M., Nichol, C., and Trathan, P. N. (2018). A long-term study of gentoo penguin (Pygoscelis papua) population trends at a major Antarctic tourist site, Goudier Island, Port Lockroy. Biodivers. Conserv. 28, 37–53.
Dunn, M. J., Jackson, J. A., Adlard, S., Lynnes, A. S., Briggs, D. R., Fox, D., et al. (2016). Population size and decadal trends of three penguin species nesting at Signy Island, South Orkney Islands. PLoS One 11:e0164025. doi: 10.1371/journal.pone.0164025
Dunnet, G. M. (1982). Oil pollution and seabird populations. Philos. Trans. R. Soc. Lond. B Biol. Sci. 297, 413–427.
Ecks, M. (1996). Einfluß von Störungen auf die Herzschlagrate Brütender Magellanpinguine Spheniscus Magellanicus. Diplomarbeit. Bonn: Friedrich-Wilhelms-Universität.
Ellenberg, U. (2017). “Penguin tourism,” in Ecotourism’s Promise and Peril – a Biological Perspective, ed. D. T. Blumstein (New York, NY: Springer International).
Ellenberg, U., Mattern, T., and Seddon, P. J. (2009). Habituation potential of yellow-eyed penguins depends on sex, character and previous experience with humans. Anim. Behav. 77, 289–296.
Ellenberg, U., Mattern, T., and Seddon, P. J. (2013). Heart rate responses provide an objective evaluation of human disturbance stimuli in breeding birds. Conserv. Physiol. 1:cot013. doi: 10.1093/conphys/cot013
Ellenberg, U., Mattern, T., Seddon, P. J., and Jorquera, G. L. (2006). Physiological and reproductive consequences of human disturbance in Humboldt penguins: the need for species-specific visitor management. Biol. Conserv. 133, 95–106.
Ellenberg, U., and Seddon, P. J. (2009). “Effective management decision-making depends on rigorous research,” in Ecotourism: Management, Development and Impact, eds A. Krause and E. Weir (Hauppauge, NY: Nova Science Publishers), 257–260.
Ellenberg, U., Setiawan, A. N., Cree, A., Houston, D. M., and Seddon, P. J. (2007). Elevated hormonal stress response and reduced reproductive output in Yellow-eyed penguins exposed to unregulated tourism. Gen. Comp. Endocrinol. 152, 54–63.
Ellis, D. S., Cipro, C. V., Ogletree, C. A., Smith, K. E., and Aronson, R. B. (2018). A 50-year retrospective of persistent organic pollutants in the fat and eggs of penguins of the Southern Ocean. Environ. Pollut. 241, 155–163. doi: 10.1016/j.envpol.2018.05.003
Emmerson, L., and Southwell, C. (2008). Sea ice cover and its influence on Adélie penguin reproductive performance. Ecology 89, 2096–2102.
Emslie, S. D., and Patterson, W. P. (2007). Abrupt recent shift in δ13C and δ15N values in Adélie penguin eggshell in Antarctica. Proc. Natl. Acad. Sci. U.S.A. 104, 11666–11669.
Erasmus, T., Randall, R. M., and Randall, B. M. (1981). Oil pollution, insulation and body temperatures in the jackass penguin Spheniscus demersus. Comp. Biochem. Physiol. A 69, 169–171.
Erasmus, T., and Wessels, E. D. (1985). Heat production studies on normal and oil-covered jackass penguins (Spheniscus demersus) in air and water. Afr. Zool. 20, 209–212.
Erasmus, Z. (1995). “A brief overview of the Apollo Sea incident,” in Proceedings of the Coastal Oil Spills: Effect on Penguin Communities and Rehabilitation Procedures, eds J. Barrett, Z. Erasmus, and A. J. Williams (Cape Town: Cape Nature Conservation), 5–7.
Eriksen, M., Lebreton, L. C., Carson, H. S., Thiel, M., Moore, C. J., Borerro, J. C., et al. (2014). Plastic pollution in the world’s oceans: more than 5 trillion plastic pieces weighing over 250,000 tons afloat at sea. PLoS One 9:e111913. doi: 10.1371/Journal.pone.0111913
Evers, D. C., Savoy, L. J., DeSorbo, C. R., Yates, D. E., Hanson, W., Taylor, K. M., et al. (2008). Adverse effects from environmental mercury loads on breeding common loons. Ecotoxicology 17, 69–81.
Falkowska, L., and Reindl, A. R. (2015). Dietary exposure to, and internal organ transfer of, selected halogenated organic compounds in birds eating fish from the Southern Baltic. J. Environ. Sci. Health A 50, 1029–1039. doi: 10.1080/10934529.2015.1038171
Fetterer, F., Knowles, K., Meier, W., Savoie, M., et al. (2017). Updated Daily. Sea Ice Index, Version 3. [Antarctic]. Boulder, CO: NSIDC.
Finger, A., Lavers, J. L., Dann, P., Nugegoda, D., Orbell, J. D., Robertson, B., et al. (2015). The little penguin (Eudyptula minor) as an indicator of coastal trace metal pollution. Environ. Pollut. 205, 365–377. doi: 10.1016/j.envpol.2015.06.022
Fretwell, P. T., and Trathan, P. N. (2009). Penguins from space: faecal stains reveal the location of emperor penguin colonies. Glob. Ecol. Biogeogr. 18, 543–552.
Fretwell, P. T., and Trathan, P. N. (2018). Emperor penguins at Halley Bay: wind, an environmental driver of colony location. Antarct. Sci. 25, 1–6.
Frias, J. E., Gil, M. N., Esteves, J. L., Borboroglu, P. G., Kane, O. J., Smith, J. R., et al. (2012). Mercury levels in feathers of Magellanic penguins. Mar. Pollut. Bull. 64, 1265–1269. doi: 10.1016/j.marpolbul.2012.02.024
Gandini, P., Boersma, P. D., Frere, E., Gandini, M., Holik, T., and Lichtschein, V. (1994). Magellanic penguins (Spheniscus magellanicus) affected by chronic petroleum pollution along coast of Chubut, Argentina. Auk 111, 20–27.
Gandini, P., Frere, E., and Boersma, P. D. (1996). Status and conservation of Magellanic penguins Spheniscus magellanicus in Patagonia, Argentina. Bird Conserv. Inter. 6, 307–316.
Gandini, P. A., Frere, E., Pettovello, A. D., and Cedrola, P. V. (1999). Interaction between Magellanic penguins and shrimp fisheries in Patagonia, Argentina. Condor 101, 783–789. doi: 10.2307/1370065
García Borboroglu, P., Boersma, P. D., Reyes, L. M., and Ruoppolo, V. (2008). Contaminación por Hidrocarburos y su Efecto Sobre el Pingüino de Magallanes. Puerto Madryn: Estado de Conservación del Mar Patagónico.
Garcia-Borboroglu, P., and Boersma, P. D. (eds). (2013). Penguins: Natural History and Conservation. Seattle, WA: University of Washington Press.
García-Borboroglu, P., Boersma, P. D., Reyes, L., and Skewgar, E. (2008). “Petroleum pollution and penguins: marine conservation tools to reduce the problem,” in Marine Pollution: New Research, ed. T. N. Hofer (New York: Nova Science Publishers Inc), 339–356.
García-Borboroglu, P., Boersma, P. D., Ruoppolo, V., Reyes, L., Rebstock, G. A., Griot, K., et al. (2006). Chronic oil pollution harms Magellanic penguins in the Southwest Atlantic. Mar. Pollut. Bull. 52, 193–198.
Gartrell, B., Agnew, D., Alley, M., Carpenter, T., Ha, H. J., Howe, L., et al. (2017). Investigation of a mortality cluster in wild adult yellow-eyed penguins (Megadyptes antipodes) at Otago Peninsula, New Zealand. Avian Pathol. 46, 278–288. doi: 10.1080/03079457.2016.1264568
Gianuca, D., Phillips, R. A., Townley, S., and Votier, S. C. (2017). Global patterns of sex-and age-specific variation in seabird bycatch. Biol. Conserv. 205, 60–76. doi: 10.1016/j.biocon.2016.11.028
Giese, M. (1996). Effects of human activity on Adelie penguin Pygoscelis adeliae breeding success. Biol. Conserv. 75, 157–164.
Giese, M., Goldsworthy, S. D., Gales, R., Brothers, N., and Hamill, J. (2000). Effects of the Iron Baron oil spill on little penguins (Eudyptula minor). III. Breeding success of rehabilitated oiled birds. Wildl. Res. 27, 583–591.
Goerke, H., Weber, K., Bornemann, H., Ramdohr, S., and Plötz, J. (2004). Increasing levels and biomagnification of persistent organic pollutants (POPs) in Antarctic biota. Mar. Pollut. Bull. 48, 295–302.
Goldsworthy, S. D., Gales, R. P., Giese, M., and Brothers, N. (2000). Effects of the Iron Baron oil spill on little penguins (Eudyptula minor). I. Estimates of mortality. Wildl. Res. 27, 559–571.
González-Zevallos, D., and Yorio, P. (2006). Seabird use of discards and incidental captures at the Argentine hake trawl fishery in the Golfo San Jorge, Argentina. Mar. Ecol. Prog. Ser. 316, 175–183.
Government of South Georgia and South Sandwich Islands (GSGSSI) (2005). “The GSGSSI address to the international association of Antarctic tour operators (IAATO),” in Proceedings of the 16th Annual Meeting. Government of South Georgia and South Sandwich Islands, Hamburg. Available at: http://www.sgisland.gs/index.php/%28g%29reports?useskin=gov (accessed July 13, 2016).
Green, C. J., Trathan, P. N., and Preston, M. (2006). A new automated logging gateway to study the demographics of macaroni penguins (Eudyptes chrysolophus) at Bird Island, South Georgia: testing the reliability of the system using radio telemetry. Polar Biol. 29, 1003–1010.
Green, J. A., White, C. R., and Butler, P. J. (2005). Allometric estimation of metabolic rate from heart rate in penguins. Comp. Biochem. Physiol. A Mol. Integr. Physiol. 142, 478–484.
Grémillet, D., and Boulinier, T. (2009). Spatial ecology and conservation of seabirds facing global climate change: a review. Mar. Ecol. Prog. Ser. 391, 121–137.
Grémillet, D., Pichegru, L., Kuntz, G., Woakes, A. G., Wilkinson, S., Crawford, R. J., et al. (2008). A junk-food hypothesis for gannets feeding on fishery waste. Proc. R. Soc. Lond. B Biol. Sci. 275, 1149–1156. doi: 10.1098/rspb.2007.1763
Grenfell, B. T., and Dobson, A. P. (eds). (1995). Ecology of Infectious Diseases in Natural Populations, Vol. 7. Cambridge: Cambridge University Press.
Grilo, M. L., Vanstreels, R. E. T., Wallace, R., García-Párraga, D., Braga,É. M., Chitty, J., et al. (2016). Malaria in penguins–current perceptions. Avian Pathol. 45, 393–407. doi: 10.1080/03079457.2016.1149145
Grimaldi, W., Ainley, D. G., and Massaro, M. (2018). Multi-year serological evaluation of three viral agents in the Adélie Penguin (Pygoscelis adeliae) on Ross Island, Antarctica. Polar Biol. 41, 2023–2031.
Grimaldi, W., Jabour, J., and Woehler, E. J. (2011). Considerations for minimising the spread of infectious disease in Antarctic seabirds and seals. Polar Rec. 47, 56–66.
Grimaldi, W. W., Hall, R. J., White, D. D., Wang, J., Massaro, M., and Tompkins, D. M. (2015a). First report of a feather loss condition in Adelie penguins (Pygoscelis adeliae) on Ross Island, Antarctica, and a preliminary investigation of its cause. Emu 115, 185–189.
Grimaldi, W. W., Seddon, P. J., Lyver, P. O. B., Nakagawa, S., and Tompkins, D. M. (2015b). Infectious diseases of Antarctic penguins: current status and future threats. Polar Biol. 38, 591–606.
Guggenheim, D. E., and Glass, T. (2014). “Disaster at Nightingale–the wreck of the MS Oliva at the world’s remotest island: lessons learned for resource managers in remote areas,” in Proceedings of the International Oil Spill Conference, Vol. 2014, (Washington, DC: American Petroleum Institute), 711–720.
Halpern, B. S., Frazier, M., Potapenko, J., Casey, K. S., Koenig, K., Longo, C., et al. (2015). Spatial and temporal changes in cumulative human impacts on the world’s ocean. Nat. Commun. 6:7615. doi: 10.1038/ncomms8615
Harris, C. M. (2005). Aircraft operations near concentrations of birds in Antarctica: the development of practical guidelines. Biol. Conserv. 125, 309–322.
Hays, C. (1986). Effects of the 1982–1983 El Nino on Humboldt penguin colonies in Peru. Biol. Conserv. 36, 169–180.
Heber, S., Wilson, K. J., and Molles, L. (2008). Breeding biology and breeding success of the blue penguin (Eudyptula minor) on the West Coast of New Zealand’s South Island. N. Z. J. Zool. 35, 63–71.
Hinke, J. T., Salwicka, K., Trivelpiece, S. G., Watters, G. M., and Trivelpiece, W. Z. (2007). Divergent responses of Pygoscelis penguins reveal a common environmental driver. Oecologia 153, 845–855.
Hinke, J. T., Trivelpiece, S. G., and Trivelpiece, W. Z. (2017). Variable vital rates and the risk of population declines in Adélie penguins from the Antarctic Peninsula region. Ecosphere 8:e01666. doi: 10.1002/ecs2.1666
Hockey, P. A. R., and Hallinan, J. (1981). Effect of human disturbance on the breeding behaviour of jackass penguins Spheniscus demersus. S. Afr. J. Wildl. Res. 11, 59–62.
Hoegh-Guldberg, O., and Bruno, J. F. (2010). The impact of climate change on the world’s marine ecosystems. Science 328, 1523–1528.
Hofman, R. J. (2017). Sealing, whaling and krill fishing in the Southern Ocean: past and possible future effects on catch regulations. Polar Rec. 53, 88–99. doi: 10.1017/S0032247416000644
Holmes, N., Giese, M., and Kriwoken, L. K. (2005). Testing the minimum approach distance guidelines for incubating Royal penguins Eudyptes schlegeli. Biol. Conserv. 126, 339–350.
Honda, K., Yamamoto, Y., Hidaka, H., and Tatsukawa, R. (1986). Heavy metal accumulations in Adélie penguin, Pygoscelis adeliae, and their variations with the reproductive processes. Mem. Natl. Inst. Polar Res. Spec. Issue 40, 443–453.
Hurt, A. C., Su, Y. C. F., Aban, M., Peck, H., Lau, H., Baas, C., et al. (2016). Evidence for the introduction, reassortment, and persistence of diverse influenza A viruses in Antarctica. J. Virol. 90, 9674–9682. doi: 10.1128/JVI.01404-16
Iles, D., and Jenouvrier, S. (2019). “Projected population consequences of climate change,” in Book Chapter for “Effects of Climate Change on Birds, eds A. Møller and P. O. Dunn (Oxford: Oxford University Press).
Jacquet, J., Blood-Patterson, E., Brooks, C., and Ainley, D. (2016). ‘Rational use in Antarctic waters. Mar. Policy 63, 28–34. doi: 10.1016/j.bbrep.2016.04.010
Jara-Carrasco, S., Barra, R., Espejo, W., Celis, J. E., González-Acuña, D., Chiang, G., et al. (2017). Persistent organic pollutants and porphyrin levels in excreta of penguin colonies from the Antarctic Peninsula area. Polar Rec. 53, 79–87.
Jara-Carrasco, S., González, M., González-Acuña, D., Chiang, G., Celis, J., Espejo, W., et al. (2015). Potential immunohaematological effects of persistent organic pollutants on chinstrap penguin. Antarct. Sci. 27, 373–381.
Jarvi, S. I., Atkinson, C. T., and Fleischer, R. C. (2001). Immunogenetics and resistance to avian malaria in Hawaiian honeycreepers (Drepanidinae). Stud. Avian Biol. 22, 254–263.
Jenouvrier, S. (2013). Impacts of climate change on avian populations. Glob. Change Biol. 19, 2036–2057. doi: 10.1111/gcb.12195
Jenouvrier, S., Barbraud, C., and Weimerskirch, H. (2006). Sea ice affects the population dynamics of Adélie penguins in Terre Adélie. Polar Biol. 29, 413–423.
Jenouvrier, S., Barbraud, C., Weimerskirch, H., and Caswell, H. (2009). Limitation of population recovery: a stochastic approach to the case of the emperor penguin. Oikos 118, 1292–1298.
Jenouvrier, S., Desprez, M., Fay, R., Barbraud, C., Weimerskirch, H., Delord, K., et al. (2018). Climate change and functional traits affect population dynamics of a long-lived seabird. J. Anim. Ecol. 87, 906–920. doi: 10.1111/1365-2656.12827
Jenouvrier, S., Garnier, J., Patout, F., and Desvillettes, L. (2017). Influence of dispersal processes on the global dynamics of Emperor penguin, a species threatened by climate change. Biol. Conserv. 212, 63–73.
Jenouvrier, S., Holland, M., Stroeve, J., Barbraud, C., Weimerskirch, H., Serreze, M., et al. (2012). Effects of climate change on an emperor penguin population: analysis of coupled demographic and climate models. Glob. Change Biol. 18, 2756–2770. doi: 10.1111/j.1365-2486.2012.02744.x
Jenouvrier, S., Holland, M., Stroeve, J., Serreze, M., Barbraud, C., Weimerskirch, H., et al. (2014). Projected continent-wide declines of the emperor penguin under climate change. Nat. Clim. Change 4, 715–718.
Jepson, P. D., Deaville, R., Barber, J. L., Aguilar,À., Borrell, A., Murphy, S., et al. (2016). PCB pollution continues to impact populations of orcas and other dolphins in European waters. Sci. Rep. 6:18573. doi: 10.1038/srep18573
Jones, J. B., Hyatt, A. D., Hine, P. M., Whittington, R. J., Griffin, D. A., and Bax, N. J. (1997). Australasian pilchard mortalities. World J. Microbiol. Biotechnol. 13, 383–392.
Kane, O. J., Smith, J. R., Boersma, P. D., Parsons, N. J., Strauss, V., Garcia-Borboroglu, P., et al. (2010). Feather-loss disorder in African and Magellanic penguins. Waterbirds 33, 415–422.
Karnauskas, K. B., Jenouvrier, S., Brown, C. W., and Murtugudde, R. (2015). Strong sea surface cooling in the eastern equatorial Pacific and implications for Galápagos Penguin conservation. Geophys. Res. Lett. 42, 6432–6437. doi: 10.1002/2015GL064456
Kelly, B. C., Ikonomou, M. G., Blair, J. D., Morin, A. E., and Gobas, F. A. (2007). Food web–specific biomagnification of persistent organic pollutants. Science 317, 236–239.
Kemper, J. (2006). Heading Towards Extinction?: Demography of the African Penguin in Namibia. Doctoral dissertation, University of Cape Town, Rondebosch.
Kemper, J., Underhill, L. G., and Roux, J. P. (2007). “Artificial burrows for African Penguins on Halifax Island, Namibia: do they improve breeding success,” in Final Report of the BCLME (Benguela Current Large Marine Ecosystem) Project on Top Predators as Biological Indicators of Ecosystem Change in the BCLME, ed. S. P. Kirkman (Cape Town: Avian Demography Unit), 101–106.
Kerry, K., Gardner, H., and Clarke, J. R. (1996). Penguin deaths: diet or disease. Microbiol. Aust. 17:16.
Keymer, I. F., Malcolm, H. M., Hunt, A., and Horsley, D. T. (2001). Health evaluation of penguins (Sphenisciformes) following mortality in the Falklands (South Atlantic). Dis. Aquat. Organ. 45, 159–169.
Kooyman, G. L., and Ponganis, P. J. (2017). Rise and fall of Ross Sea emperor penguin colony populations: 2000 to 2012. Antarct. Sci. 29, 201–208.
Kowalczyk, N. D., Reina, R. D., Preston, T. J., and Chiaradia, A. (2015). Environmental variability drives shifts in the foraging behaviour and reproductive success of an inshore seabird. Oecologia 178, 967–979. doi: 10.1007/s00442-015-3294-6
Kroodsma, D. A., Mayorga, J., Hochberg, T., Miller, N. A., Boerder, K., Ferretti, F., et al. (2018). Tracking the global footprint of fisheries. Science 359, 904–908. doi: 10.1126/science.aao5646
Kühn, S., Rebolledo, E. L. B., and van Franeker, J. A. (2015). “Deleterious effects of litter on marine life,” in Marine Anthropogenic Litter, eds M. Bergmann, L. Gutow, and M. Klages (Cham: Springer Open), 75–116.
Leighton, F. A., (1993). The toxicity of petroleum oils to birds. Environ. Rev. 1, 92–103. doi: 10.1139/a93-008
Leotta, G. A., Chinen, I., Vigo, G. B., Pecoraro, M., and Rivas, M. (2006). Outbreaks of avian cholera in Hope Bay, Antarctica. J. Wildl. Dis. 42, 259–270.
Lescroël, A., Bajzak, C., and Bost, C. A. (2009). Breeding ecology of the gentoo penguin Pygoscelis papua at Kerguelen Archipelago. Polar Biol. 32, 1495–1505.
Levy, H., Clucas, G. V., Rogers, A. D., Leaché, A. D., Ciborowski, K. L., Polito, M. J., et al. (2016). Population structure and phylogeography of the gentoo penguin (Pygoscelis papua) across the Scotia Arc. Ecol. Evol. 6, 1834–1853. doi: 10.1002/ece3.1929
Little, S. J., Harcourt, R. G., and Clevenger, A. P. (2002). Do wildlife passages act as prey-traps? Biol. Conserv. 107, 135–145.
Ludynia, K., Roux, J. P., Jones, R., Kemper, J., and Underhill, L. G. (2010). Surviving off junk: low-energy prey dominates the diet of African penguins Spheniscus demersus at Mercury Island, Namibia, between 1996 and 2009. Afr. J. Mar. Sci. 32, 563–572.
Lynch, H. J., Naveen, R., Trathan, P. N., and Fagan, W. F. (2012). Spatially integrated assessment reveals widespread changes in penguin populations on the Antarctic Peninsula. Ecology 93, 1367–1377.
MacDonald, J. W., and Conroy, J. W. H. (1971). Virus disease resembling puffinosis in the gentoo penguin (Pygoscelis papua) on Signy Island, South Orkney Islands. Br. Antarct. Surv. B 26, 80–82.
Majluf, P., Babcock, E. A., Riveros, J. C., Schreiber, M. A., and Alderete, W. (2002). Catch and bycatch of sea birds and marine mammals in the small-scale fishery of Punta San Juan, Peru. Conserv. Biol. 16, 1333–1343.
Mangin, S., Gauthier-Clerc, M., Frenot, Y., Gendner, J. P., and Le Maho, Y. (2003). Ticks Ixodes uriae and the breeding performance of a colonial seabird, king penguin Aptenodytes patagonicus. J. Avian Biol. 34, 30–34.
Mattern, T., Ellenberg, U., Houston, D. M., Lamare, M., Davis, L. S., van Heezik, Y., et al. (2013). Straight line foraging in yellow-eyed penguins: new insights into cascading fisheries effects and orientation capabilities of marine predators. PLoS One 8:e84381. doi: 10.1371/journal.pone.0084381
Mattern, T., Houston, D. M., Lalas, C., Setiawan, A. N., and Davis, L. S. (2009). Diet composition, continuity in prey availability and marine habitat—keystones to population stability in the snares penguin (Eudyptes robustus). Emu 109, 204–213.
Mattern, T., Meyer, S., Ellenberg, U., Houston, D. M., Darby, J. T., Young, M., et al. (2017). Quantifying climate change impacts emphasises the importance of managing regional threats in the endangered Yellow-eyed penguin. PeerJ 5:e3272. doi: 10.7717/peerj.3272
McClung, M. R., Seddon, P. J., Massaro, M., and Setiawan, A. N. (2004). Nature-based tourism impacts on yellow-eyed penguins Megadyptes antipodes: does unregulated visitor access affect fledging weight and juvenile survival? Biol. Conserv. 119, 279–285.
Mhlongo, N., Yemane, D., Hendricks, M., and van der Lingen, C. D. (2015). Have the spawning habitat preferences of anchovy (Engraulis encrasicolus) and sardine (Sardinops sagax) in the southern Benguela changed in recent years? Fish. Oceanogr. 24, 1–14. doi: 10.1111/fog.12061
Moldan, A., and Westphal, A. (1994). SANCCOB: the South African national foundation for the conservation of coastal birds. Penguin Conserv. 7, 13–16.
Moller, A. P. (1997). “Parasitism and the evolution of host life history,” in Host-Parasite Evolution. General Principles and Avian Models, eds D. H. Clayton and J. Moore (Oxford: Oxford University Press), 105–127.
Montero, E., González, L. M., Chaparro, A., Benzal, J., Bertellotti, M., Masero, J. A., et al. (2016). First record of Babesia sp. in Antarctic penguins. Ticks Tick Borne Dis. 7, 498–501. doi: 10.1016/j.ttbdis.2016.02.006
Morant, P. D., Cooper, J., and Randall, R. M. (1981). “The rehabilitation of oiled jackass penguins Spheniscus demersus, 1970–1980,” in Proceedings of the Symposium on Birds of the Sea and Shore’, ed. J. Cooper (Cape Town: African Seabird Group), 267–301.
Moreno, J., Barbosa, A., De Leon, A., and Fargallo, J. A. (1999). Phenotypic selection on fledging body size in the Chinstrap penguin Pygoscelis antarctica. J. Evol. Biol. 12, 507–513. doi: 10.1046/j.1420-9101.1999.00032.x
Morgenthaler, A., Frere, E., Rey, A. R., Torlaschi, C., Cedrola, P., Tiberi, E., et al. (2018). Unusual number of Southern rockhopper penguins, Eudyptes chrysocome, molting and dying along the Southern Patagonian coast of Argentina: pre-molting dispersion event related to adverse oceanographic conditions? Polar Biol. 41, 1041–1047. doi: 10.1371/journal.pone.0174850
Munro, G. (2007). Outbreak of Avian Pox Virus in Gentoo Penguins in the Falklands, February 2006. Stanley: Falklands Conservation.
Mykytowycz, R., and Hesterman, E. R. (1957). A note on tick infestation of the fairy penguin, Eudyptia minor, Forster. CSIRO Wildl. Res. 2, 165–166.
Nel, D. C., Ryan, P. G., and Watkins, B. P. (2002). Seabird mortality in the Patagonian toothfish longline fishery around the Prince Edward Islands, 1996–2000. Antarct. Sci. 14, 151–161.
Nelms, S. E., Galloway, T. S., Godley, B. J., Jarvis, D. S., and Lindeque, P. K. (2018). Investigating microplastic trophic transfer in marine top predators. Environ. Pollut. 238, 999–1007. doi: 10.1016/j.envpol.2018.02.016
Nicol, S., Foster, J., and Kawaguchi, S. (2012). The fishery for Antarctic krill–recent developments. Fish Fish. 13, 30–40. doi: 10.1111/j.1467-2979.2011.00406.x
Niemandt, C., Kovacs, K. M., Lydersen, C., Dyer, B. M., Isaksen, K., Hofmeyr, G. G., et al. (2016). Chinstrap and macaroni penguin diet and demography at Nyrøysa, Bouvetøya. Antarct. Sci. 28, 91–100.
Nimon, A. J., Schroter, R. C., and Stonehouse, B. (1995). Heart rate of disturbed penguins. Nature 374:415.
Nims, B. D., Vargas, F. H., Merkel, J., and Parker, P. G. (2008). Low genetic diversity and lack of population structure in the endangered Galápagos penguin (Spheniscus mendiculus). Conserv. Genet. 9, 1413–1420.
Oelke, H. (1978). Natürliche oder anthropogene populationsveränderungen von adéliepinguinen (Pygoscelis adeliae) im ross-meer-sektor der Antarktis. J. Ornithol. 119, 1–13.
Olsen, B., Duffy, D. C., Jaenson, T. G., Gylfe, A., Bonnedahl, J., and Bergström, S. (1995). Transhemispheric exchange of Lyme disease spirochetes by seabirds. J. Clin. Microbiol. 33, 3270–3274.
Oro, D., Genovart, M., Tavecchia, G., Fowler, M. S., and Martínez-Abraín, A. (2013). Ecological and evolutionary implications of food subsidies from humans. Ecol. Lett. 16, 1501–1514. doi: 10.1111/ele.12187
Otley, H., Edmonds, H., Hiscock, J., Newton, G., Tansell, J., Klink, P. V., et al. (2018). Assessing the population trend and threats to New Zealand’s Fiordland crested penguin using counting and demographic modelling approaches. N. Z. J. Ecol. 42, 125–136.
Palacios, M. J., Valera, F., and Barbosa, A. (2012). Experimental assessment of the effects of gastrointestinal parasites on offspring quality in chinstrap penguins (Pygoscelis antarctica). Parasitology 139, 819–824. doi: 10.1017/S0031182011002381
Paredes, R., and Zavalaga, C. B. (1998). Overview of the effects of El Niño 1997-98 on Humboldt penguins and other seabirds at Punta San Juan, Peru. Penguin Conserv. 11, 5–7.
Parsons, N. J., and Underhill, L. G. (2005). Oiled and injured African penguins Spheniscus demersus and other seabirds admitted for rehabilitation in the Western Cape, South Africa, 2001 and 2002. Afr. J. Mar. Sci. 27, 289–296.
Pauly, D., and Zeller, D. (2016). Catch reconstructions reveal that global marine fisheries catches are higher than reported and declining. Nat. Commun. 7: 10244. doi: 10.1038/ncomms10244
Pedro, S., Xavier, J. C., Tavares, S., Trathan, P. N., Ratcliffe, N., Paiva, V. H., et al. (2015). Mercury accumulation in gentoo penguins Pygoscelis papua: spatial, temporal and sexual intraspecific variations. Polar Biol. 38, 1335–1343.
Pichegru, L., Edwards, T. B., Dilley, B. J., Flower, T. P., and Ryan, P. G. (2016). African Penguin tolerance to humans depends on historical exposure at colony level. Bird Conserv. Int. 26, 307–322.
Pichegru, L., Nyengera, R., McInnes, A. M., and Pistorius, P. (2017). Avoidance of seismic survey activities by penguins. Sci. Rep. 7:16305. doi: 10.1038/s41598-017-16569-x
Pichegru, L., Ryan, P. G., Le Bohec, C., Van der Lingen, C. D., Navarro, R., Petersen, S., et al. (2009). Overlap between vulnerable top predators and fisheries in the Benguela upwelling system: implications for marine protected areas. Mar. Ecol. Prog. Ser. 391, 199–208.
Pichegru, L., Ryan, P. G., Van Eeden, R., Reid, T., Grémillet, D., and Wanless, R. (2012). Industrial fishing, no-take zones and endangered penguins. Biol. Conserv. 156, 117–125.
Pinto, M. B., Siciliano, S., and Di Beneditto, A. P. M. (2007). Stomach contents of the Magellanic penguin Spheniscus magellanicus from the northern distribution limit on the Atlantic coast of Brazil. Mar. Ornithol. 35, 77–78.
Polito, M. J., Brasso, R. L., Trivelpiece, W. Z., Karnovsky, N., Patterson, W. P., and Emslie, S. D. (2016). Differing foraging strategies influence mercury (Hg) exposure in an Antarctic penguin community. Environ. Pollut. 218, 196–206. doi: 10.1016/j.envpol.2016.04.097
Preston, T. J., Chiaradia, A., Caarels, S. A., and Reina, R. D. (2010). Fine scale biologging of an inshore marine animal. J. Exp. Mar. Biol. Ecol. 390, 196–202.
Pütz, K., Harris, S., Ratcliffe, N., Rey, A. R., Poncet, S., and Lüthi, B. (2018). Plasticity in the foraging behavior of male Southern Rockhopper Penguins (Eudyptes chrysocome) during incubation in the Falkland/Malvinas Islands. Polar Biol. 41, 1801–1814. doi: 10.1007/s00300-018-2320-7
Pütz, K., Hiriart-Bertrand, L., Simeone, A., Riquelme, V., Reyes-Arriagada, R., and Lüthi, B. (2011). Entanglement and drowning of a Magellanic Penguin (Spheniscus magellanicus) in a gill net recorded by a time-depth recorder in south-central Chile. Waterbirds 34, 121–125.
Ramírez, F., Afán, I., Davis, L. S., and Chiaradia, A. (2017). Climate impacts on global hot spots of marine biodiversity. Sci. Adv. 3:e1601198. doi: 10.1126/sciadv.1601198
Rand, R. W. (1969). Some hazards to seabirds. Ostrich 40, 515–520. doi: 10.1080/00306525.1969.9639146
Ratcliffe, N., Hill, S. L., Staniland, I. J., Brown, R., Adlard, S., Horswill, C., et al. (2015). Do krill fisheries compete with macaroni penguins? Spatial overlap in prey consumption and catches during winter. Divers. Distrib. 21, 1339–1348. doi: 10.1111/ddi.12366
Regel, J., and Pütz, K. (1997). Effect of human disturbance on body temperature and energy expenditure in penguins. Polar Biol. 18, 246–253.
Renedo, M., Amouroux, D., Pedrero, Z., Bustamante, P., and Cherel, Y. (2018). Identification of sources and bioaccumulation pathways of MeHg in subantarctic penguins: a stable isotopic investigation. Sci. Rep. 8:8865. doi: 10.1038/s41598-018-27079-9
Rintoul, S. R., Chown, S. L., DeConto, R. M., England, M. H., Fricker, H. A., Masson-Delmotte, V., et al. (2018). Choosing the future of Antarctica. Nature 558, 233–241. doi: 10.1038/s41586-018-0173-4
Robinson, W. M., Butterworth, D. S., and Plagányi, É. E. (2015). Quantifying the projected impact of the South African sardine fishery on the Robben Island penguin colony. ICES J. Mar. Sci. 72, 1822–1833.
Rodríguez, A., Holmberg, R., Dann, P., and Chiaradia, A. (2018). Penguin colony attendance under artificial lights for ecotourism. J. Exp. Zool. Part A Ecol. Integr. Physiol. 329, 457–464. doi: 10.1002/jez.2155
Ropert-Coudert, Y., Kato, A., and Chiaradia, A. (2009). Impact of small-scale environmental perturbations on local marine food resources: a case study of a predator, the little penguin. Proc. R. Soc. Lond. B Biol. Sci. 276, 4105–4109. doi: 10.1098/rspb.2009.1399
Ropert-Coudert, Y., Kato, A., Shiomi, K., Barbraud, C., Angelier, F., Delord, K., et al. (2018). Two recent massive breeding failures in an Adélie penguin colony call for the creation of a marine protected area in D’Urville Sea/Mertz. Front. Mar. Sci. 5:264. doi: 10.3389/fmars.2018.00264
Ropert-Coudert, Y., and Wilson, R. P. (2005). Trends and perspectives in animal-attached remote sensing. Front. Ecol. Environ. 3, 437–444.
Rounsevell, D., and Binns, D. (1991). Mass deaths of king penguins (Aptenodytes patagonica) at Lusitania Bay, Macquarie Island. Aurora 10, 8–10.
Roux, M. J., Brewin, P., Jurgens, E., and Winter, A. (2012). Scientific Report, Fisheries Cruise ZDLT1-08- 2012. Fisheries Department, Directorate of Natural Resources. Stanley: Falkland Islands Government.
Rümmler, M. C., Mustafa, O., Maercker, J., Peter, H. U., and Esefeld, J. (2018). Sensitivity of Adélie and Gentoo penguins to various flight activities of a micro UAV. Polar Biol. 41, 2481–2493.
Ruoppolo, V., Vanstreels, R. E. T. V., Woehler, E. J., Strauss, V., Parsons, N., Dann, P., et al. (2014). “Penguins clearly benefit from rehabilitation following exposure to oil,” in Proceedings of the Pacific Seabird Group Annual Meeting, Juneau, AK, 55.
Ruoppolo, V., Woehler, E. J., Morgan, K., and Clumpner, C. J. (2013). Wildlife and oil in the Antarctic: a recipe for cold disaster. Polar Rec. 49, 97–109. doi: 10.1017/S0032247411000763
Ryan, P. G. (1987). The incidence and characteristics of plastic particles ingested by seabirds. Mar. Environ. Res. 23, 175–206.
Ryan, P. G. (1990). “The marine plastic debris problem off Southern Africa: types of debris, their environmental effects, and control measures,” in Proceedings of the Second International Conference on Marine Debris, (Washington, DC: NOAA), 85–102.
Ryan, P. G. (2016). “Ingestion of plastics by marine organisms,” in Hazardous Chemicals Associated with Plastics in the Marine Environment. The Handbook of Environmental Chemistry, Vol. 78, eds H. Takada and H. Karapanagioti (Cham: Springer). doi: 10.1007/698_2016_21
Ryan, P. G. (2018). Entanglement of birds in plastics and other synthetic materials. Mar. Pollut. Bull. 135, 159–164. doi: 10.1016/j.marpolbul.2018.06.057
Saraux, C., Chiaradia, A., Salton, M., Dann, P., and Viblanc, V. A. (2016). Negative effects of wind speed on individual foraging performance and breeding success in little penguins. Ecol. Monogr. 86, 61–77.
Scheifler, R., Gauthier-Clerc, M., Bohec, C. L., Crini, N., Cśurdassier, M., Badot, P. M., et al. (2005). Mercury concentrations in king penguin (Aptenodytes patagonicus) feathers at Crozet Islands (sub-Antarctic): temporal trend between 1966–1974 and 2000–2001. Environ. Toxicol. Chem. 24, 125–128.
Schlatter, R. P., Paredes, E., Ulloa, J., Harris, J., Romero, A., Vásquez, J., et al. (2009). Mortandad de pingüino de Magallanes (Spheniscus magellanicus) en Queule, región de la Araucanía, Chile. Bol. Chil. Ornitol. 15, 78–86.
Schramm, F., Gauthier-Clerc, M., Fournier, J. C., McCoy, K. D., Barthel, C., Postic, D., et al. (2014). First detection of Borrelia burgdorferi sensu lato DNA in king penguins (Aptenodytes patagonicus halli). Ticks Tick Borne Dis. 5, 939–942. doi: 10.1016/j.ttbdis.2014.07.013
Shannon, L. J., and Crawford, R. J. M. (1999). Management of the African Penguin Spheniscus demersus- insights from modelling. Mar. Ornithol. 27, 119–128.
Sherley, R. B., Abadi, F., Ludynia, K., Barham, B. J., Clark, A. E., and Altwegg, R. (2014). Age-specific survival and movement among major African Penguin Spheniscus demersus colonies. Ibis 156, 716–728.
Sherley, R. B., Barham, B. J., Barham, P. J., Campbell, K. J., Crawford, R. J., Grigg, J., et al. (2018). Bayesian inference reveals positive but subtle effects of experimental fishery closures on marine predator demographics. Proc. R. Soc. B 285:20172443. doi: 10.1098/rspb.2017.2443
Sherley, R. B., Ludynia, K., Dyer, B. M., Lamont, T., Makhado, A. B., Roux, J. P., et al. (2017). Metapopulation tracking juvenile penguins reveals an ecosystem-wide ecological trap. Curr. Biol. 27, 563–568. doi: 10.1016/j.cub.2016.12.054
Sherley, R. B., Underhill, L. G., Barham, B. J., Barham, P. J., Coetzee, J. C., Crawford, R. J., et al. (2013). Influence of local and regional prey availability on breeding performance of African penguins Spheniscus demersus. Mar. Ecol. Prog. Ser. 473, 291–301.
Sherley, R. B., Winker, H., Altwegg, R., van der Lingen, C. D., Votier, S. C., and Crawford, R. J. (2015). Bottom-up effects of a no-take zone on endangered penguin demographics. Biol. Lett. 11:20150237. doi: 10.1098/rsbl.2015.0237
Simaika, J. P., and Samways, M. J. (2010). Biophilia as a universal ethic for conserving biodiversity. Conserv. Biol. 24, 903–906. doi: 10.1111/j.1523-1739.2010.01485.x
Simeone, A., Bernal, M., and Meza, J. (1999). Incidental mortality of Humboldt penguins Spheniscus humboldti in gill nets, central Chile. Mar. Ornithol. 27, 157–161.
Simeone, A., and Schlatter, R. P. (1998). Threats to a mixed-species colony of Spheniscus penguins in southern Chile. Colon. Waterbirds 21, 418–421.
Skewgar, E., Boersma, P. D., Harris, G., and Caille, G. (2007). Anchovy fishery threat to Patagonian ecosystem. Science 315:45.
Smeele, Z. E., Ainley, D. G., and Varsani, A. (2017). Viruses associated with Antarctic wildlife: from serology based detection to identification of genomes using high throughput sequencing. Virus Res. 243, 91–105. doi: 10.1016/j.virusres.2017.10.017
Southwell, C., Emmerson, L., McKinlay, J., Newbery, K., Takahashi, A., Kato, A., et al. (2015). Spatially extensive standardized surveys reveal widespread, multi-decadal increase in East Antarctic Adélie penguin populations. PLoS One 10:e0139877. doi: 10.1371/journal.pone.0139877
Stahl, J. T., and Oli, M. K. (2006). Relative importance of avian life-history variables to population growth rate. Ecol. Modell. 198, 23–39.
Steffen, W., Rockström, J., Richardson, K., Lenton, T. M., Folke, C., Liverman, D., et al. (2018). Trajectories of the earth system in the Anthropocene. Proc. Natl. Acad. Sci. U.S.A. 115, 8252–8259. doi: 10.1073/pnas.1810141115
Stephenson, R., and Andrews, C. A. (1997). The effect of water surface tension on feather wettability in aquatic birds. Can. J. Zool. 75, 288–294.
Sæther, B.-E., and Bakke, Ø. (2000). Avian life history variation and contribution of demographic traits to the population growth rate. Ecology 81, 642–653.
Thiebot, J. B., Arnould, J. P., Gómez-Laich, A., Ito, K., Kato, A., Mattern, T., et al. (2017). Jellyfish and other gelata as food for four penguin species–insights from predator-borne videos. Front. Ecol. Environ. 15, 437–441.
Thompson, P. A., Bonham, P., Waite, A. M., Clementson, L. A., Cherukuru, N., Hassler, C., et al. (2011). Contrasting oceanographic conditions and phytoplankton communities on the east and west coasts of Australia. Deep Sea Res. Part II Top. Stud. Oceanogr. 58, 645–663.
Tompkins, D. M., and Poulin, R. (2006). “Parasites and biological invasions,” in Biological Invasions in New Zealand, eds R. B. Allen and W. G. Lee (Berlin: Springer), 67–84.
Tourinho, P. S., do Sul, J. A. I., and Fillmann, G. (2010). Is marine debris ingestion still a problem for the coastal marine biota of southern Brazil? Mar. Pollut. Bull. 60, 396–401. doi: 10.1016/j.marpolbul.2009.10.013
Traisnel, G., and Pichegru, L. (2018). Possible drivers of nest usurpation in African Penguins Spheniscus demersus. Mar. Ornithol. 46, 85–88.
Trathan, P. N., Collins, M. A., Grant, S. M., Belchier, M., Barnes, D. K., Brown, J., et al. (2014). The South Georgia and the South Sandwich Islands MPA: protecting a biodiverse oceanic island chain situated in the flow of the Antarctic Circumpolar Current. Adv. Mar. Biol. 69, 15–78.
Trathan, P. N., Forcada, J., Atkinson, R., Downie, R. H., and Shears, J. R. (2008). Population assessments of gentoo penguins (Pygoscelis papua) breeding at an important Antarctic tourist site, Goudier Island, Port Lockroy, Palmer Archipelago, Antarctica. Biol. Conserv. 141, 3019–3028. doi: 10.1016/j.biocon.2008.09.006
Trathan, P. N., García-Borboroglu, P., Boersma, D., Bost, C. A., Crawford, R. J., Crossin, G. T., et al. (2015). Pollution, habitat loss, fishing, and climate change as critical threats to penguins. Conserv. Biol. 29, 31–41. doi: 10.1111/cobi.12349
Trathan, P. N., Murphy, E. J., Forcada, J., Croxall, J. P., Reid, K., and Thorpe, S. E. (2006). “Physical forcing in the southwest Atlantic: ecosystem control,” in Top Predators in Marine Ecosystems, eds I. L. Boyd, S. Wanless, and C. J. Camphuysen (Cambridge: Cambridge University Press).
Trathan, P. N., Ratcliffe, N., and Masden, E. A. (2012). Ecological drivers of change at South Georgia: the krill surplus, or climate variability. Ecography 35, 983–993. doi: 10.1111/j.1600-0587.2012.07330.x
Trathan, P. N., Warwick-Evans, V., Hinke, J. T., Young, E. F., Murphy, E. J., Carneiro, A. P. B., et al. (2018). Managing fishery development in sensitive ecosystems: identifying penguin habitat use to direct management in Antarctica. Ecosphere 9:e02392.
Trivelpiece, W. Z., Hinke, J. T., Miller, A. K., Reiss, C. S., Trivelpiece, S. G., and Watters, G. M. (2011). Variability in krill biomass links harvesting and climate warming to penguin population changes in Antarctica. Proc. Natl. Acad. Sci. U.S.A. 108, 7625–7628. doi: 10.1073/pnas.1016560108
Tull, C. E., Germain, P., and May, A. W. (1972). Mortality of Thick-billed Murres in the West Greenland salmon fishery. Nature 237, 42–44.
Underhill, L. G., Bartlett, P. A., Baumann, L., Crawford, R. J., Dyer, B. M., Gildenhuys, A., et al. (1999). Mortality and survival of African Penguins Spheniscus demersus involved in the Apollo Sea oil spill: an evaluation of rehabilitation efforts. Ibis 141, 29–37.
UNEP (2009). Stockholm Convention on Persistent Organic Pollutants, 22 May 2001 Stockholm Adoption of Amendments to Annexes A, B and C. Reference: C.N.524.2009.TREATIES-4 (Depository Notification). Geneva: United Nations Environment Programme.
United Nations Department of Economic and Social Affairs [UN-DESA] (2015). Worldcpssnm United Nations Department of Economic and Social Affairs (UN-DESA)cpesnm Population Projected to Reach 9.7 Billion by 2050. Available at: www.un.org/en/development/desa/news/population/2015-report.html (accessed September 14, 2018.)
van Riper, C., van Riper, S. G., and Hansen, W. R. (2002). Epizootiology and effect of avian pox on Hawaiian forest birds. Auk 119, 929–942.
Vanstreels, R. E. T., Uhart, M., Rago, V., Hurtado, R., Epiphanio, S., and Catao-Dias, J. L. (2017). Do blood parasites infect Magellanic penguins (Spheniscus magellanicus) in the wild? Prospective investigation and climatogeographic considerations. Parasitology 144, 698–705. doi: 10.1017/S0031182016002407
Vargas, F. H., Harrison, S., Rea, S., and Macdonald, D. W. (2006). Biological effects of El Niño on the Galápagos penguin. Biol. Conserv. 127, 107–114.
Varsani, A., Kraberger, S., Jennings, S., Porzig, E. L., Julian, L., Massaro, M., et al. (2014). A novel papillomavirus in Adelie penguin (Pygoscelis adeliae) faeces sampled at the Cape Crozier colony, Antarctica. J. Gen. Virol. 95, 1352–1365. doi: 10.1099/vir.0.064436-0
Varsani, A., Porzig, E. L., Jennings, S., Kraberger, S., Farkas, K., Julian, L., et al. (2015). Identification of an avian polyomavirus associated with Adelie penguins (Pygoscelis adeliae). J. Gen. Virol. 96, 851–857. doi: 10.1099/vir.0.000038
Viblanc, V. A., Smith, A. D., Gineste, B., Kauffmann, M., and Groscolas, R. (2015). Modulation of heart rate response to acute stressors throughout the breeding season in the king penguin Aptenodytes patagonicus. J. Exp. Biol. 218, 1686–1692. doi: 10.1242/jeb.112003
Viblanc, V. A., Valette, V., Kauffmann, M., Malosse, N., and Groscolas, R. (2012). Coping with social stress: heart rate responses to agonistic interactions in king penguins. Behav. Ecol. 23, 1178–1185.
Villanueva, C., Walker, B. G., and Bertellotti, M. (2012). A matter of history: effects of tourism on physiology, behaviour and breeding parameters in Magellanic Penguins (Spheniscus magellanicus) at two colonies in Argentina. J. Ornithol. 153, 219–228.
Villanueva, C., Walker, B. G., and Bertellotti, M. (2014). Seasonal variation in the physiological and behavioral responses to tourist visitation in Magellanic penguins. J. Wildl. Manage. 78, 1466–1476.
Walker, B. G., Boersma, P. D., and Wingfield, J. C. (2005a). Field endocrinology and conservation biology. Integr. Comp. Biol. 45, 12–18. doi: 10.1093/icb/45.1.12
Walker, B. G., Boersma, P. D., and Wingfield, J. C. (2005b). Physiological and behavioral differences in Magellanic Penguin chicks in undisturbed and tourist-visited locations of a colony. Conserv. Biol. 19, 1571–1577.
Walker, B. G., Dee Boersma, P., and Wingfield, J. C. (2006). Habituation of adult Magellanic penguins to human visitation as expressed through behavior and corticosterone secretion. Conserv. Biol. 20, 146–154.
Wania, F., and Mackay, D. (1993). Global fractionation and cold condensation of low volatility organochlorine compounds in polar regions. Ambio 22, 10–18.
Warwick-Evans, V., Ratcliffe, N., Lowther, A. D., Manco, F., Ireland, L., Clewlow, H. L., et al. (2018). Using habitat models for chinstrap penguins Pygoscelis antarctica to advise krill fisheries management during the penguin breeding season. Divers. Distrib. 24, 1756–1771.
Weimerskirch, H., Prudor, A., and Schull, Q. (2018). Flights of drones over sub-Antarctic seabirds show species-and status-specific behavioural and physiological responses. Polar Biol. 41, 259–266.
Weller, F., Sherley, R. B., Waller, L. J., Ludynia, K., Geldenhuys, D., Shannon, L. J., et al. (2016). System dynamics modelling of the Endangered African penguin populations on Dyer and Robben islands, South Africa. Fish Fish. 327, 44–56.
Whittington, P. (2002). Survival and Movements of African Penguins, Especially After Oiling. Doctoral dissertation, University of Cape Town, Cape Town.
Whittington, P. A., Hofmeyr, J. H., and Cooper, J. (1996). Establishment, growth and conservation of a mainland colony of Jackass Penguins Spheniscus demersus at Stony Point, Betty’s Bay, South Africa. Ostrich 67, 144–150.
Woehler, E. J., Ainley, D., and Jabour, J. (2014). “Human impacts to Antarctic wildlife: predictions and speculations for 2060,” in Antarctic Futures, eds T. Tin, P. T. Maher, D. Liggett, and M. Lamers (Dordrecht: Springer), 27–60.
Woehler, E. J., Penney, R. L., Creet, S. M., and Burton, H. R. (1994). Impacts of human visitors on breeding success and long-term population trends in Adélie penguins at Casey, Antarctica. Polar Biol. 14, 269–274.
Wolfaardt, A. C., Underhill, L. G., Nel, D. C., Williams, A. J., and Visagie, J. (2008). Breeding success of African penguins Spheniscus demersus at Dassen Island, especially after oiling following the Apollo Sea spill. Afr. J. Mar. Sci. 30, 565–580.
Wolfaardt, A. C., Williams, A. J., Underhill, L. G., Crawford, R. J. M., and Whittington, P. A. (2009). Review of the rescue, rehabilitation and restoration of oiled seabirds in South Africa, especially African penguins Spheniscus demersus and Cape gannets Morus capensis, 1983–2005. Afr. J. Mar. Sci. 31, 31–54.
Wolfe, M. F., Schwarzbach, S., and Sulaiman, R. A. (1998). Effects of mercury on wildlife: a comprehensive review. Environ. Toxicol. Chem. 17, 146–160. doi: 10.1002/etc.5620170203
Wright, M. (1998). Ecotourism on Otago Peninsula: preliminary studies of hoihos (Megadyptes antipodes) and Hookers sea lion (Phocarctos hookeri). Sci. Conserv. 68, 1173–2946.
Xavier, J. C., Barbosa, A., Agustí, S., Alonso-Sáez, L., Alvito, P., Ameneiro, J., et al. (2013). Polar marine biology science in Portugal and Spain: recent advances and future perspectives. J. Sea Res. 83, 9–29. doi: 10.1016/j.seares.2013.05.013
Yamamoto, Y., Kanesaki, S., Kuramochi, T., Miyazaki, N., Watanuki, Y., and Naito, Y. (1996). Comparison of trace element concentrations in tissues of chick and adult Adélie penguins. Proc. Natl. Inst. Polar Biol. 9, 253–262.
Yeh, S. W., Kug, J. S., Dewitte, B., Kwon, M. H., Kirtman, B. P., and Jin, F. F. (2009). El Niño in a changing climate. Nature 461, 511–514.
Yorio, P., and Boersma, P. D. (1992). The effects of human disturbance on Magellanic penguin Spheniscus magellanicus behaviour and breeding success. Bird Conserv. Inter. 2, 161–173. doi: 10.1017/S0959270900002410
Younger, J. L., Emmerson, L. M., and Miller, K. J. (2016). The influence of historical climate changes on Southern Ocean marine predator populations: a comparative analysis. Glob. Change Biol. 22, 474–493. doi: 10.1111/gcb.13104
Zeller, D., Cashion, T., Palomares, M., and Pauly, D. (2018). Global marine fisheries discards: a synthesis of reconstructed data. Fish Fish. 19, 30–39. doi: 10.1111/faf.12233
Keywords: Spheniscidae, threats, mitigation, pollution, climate change, fisheries
Citation: Ropert-Coudert Y, Chiaradia A, Ainley D, Barbosa A, Boersma PD, Brasso R, Dewar M, Ellenberg U, García-Borboroglu P, Emmerson L, Hickcox R, Jenouvrier S, Kato A, McIntosh RR, Lewis P, Ramírez F, Ruoppolo V, Ryan PG, Seddon PJ, Sherley RB, Vanstreels RET, Waller LJ, Woehler EJ and Trathan PN (2019) Happy Feet in a Hostile World? The Future of Penguins Depends on Proactive Management of Current and Expected Threats. Front. Mar. Sci. 6:248. doi: 10.3389/fmars.2019.00248
Received: 13 December 2018; Accepted: 24 April 2019;
Published: 28 May 2019.
Edited by:
Rob Harcourt, Macquarie University, AustraliaReviewed by:
Melinda Grace Conners, Old Dominion University, United StatesGail Schofield, Queen Mary University of London, United Kingdom
Copyright © 2019 Ropert-Coudert, Chiaradia, Ainley, Barbosa, Boersma, Brasso, Dewar, Ellenberg, García-Borboroglu, Emmerson, Hickcox, Jenouvrier, Kato, McIntosh, Lewis, Ramírez, Ruoppolo, Ryan, Seddon, Sherley, Vanstreels, Waller, Woehler and Trathan. This is an open-access article distributed under the terms of the Creative Commons Attribution License (CC BY). The use, distribution or reproduction in other forums is permitted, provided the original author(s) and the copyright owner(s) are credited and that the original publication in this journal is cited, in accordance with accepted academic practice. No use, distribution or reproduction is permitted which does not comply with these terms.
*Correspondence: Yan Ropert-Coudert, yan.ropert-coudert@cebc.cnrs.fr
†These authors have contributed equally to this work