- 1Thünen Institute of Baltic Sea Fisheries, Rostock, Germany
- 2Institute for Marine Ecosystem and Fisheries Science, University of Hamburg, Hamburg, Germany
- 3Max Planck Institute for Chemistry, Department of Climate Geochemistry, Mainz, Germany
Despite centuries of human exploitation and research on Atlantic herring (Clupea harengus) in Europe, there is still much uncertainty on where major nursery areas are located. However, understanding the quantitative contribution of particular coastal systems to adult fish populations is of utmost importance to secure sustainable fish resources. Routinely, marker elements indicating certain hydrological conditions, which are incorporated into calcified structures, the so-called otoliths, are used to trace the origin of fish. However, as in the Baltic Sea, small and large scale salinity gradients potentially masking specific salinity signals. Based on the entire elemental inventory of the otolith core region, indicating the chemical signature of the spawning area, we developed a unique elemental fingerprinting index (EFI), allowing comparisons of multi-elemental chemical signatures from within and between herring juvenile areas. Our results show significantly distinct chemical “fingerprints” on the scale of particular bays and estuaries, which were not detectable with the usual marker elements. We further demonstrate that heavy metals levels drive the potential to distinguish natal origin of herring. These findings provide an essential baseline for further studies on the impact of small scale productivity for exploited fish resources and central components of marine food webs.
Introduction
Coastal ecosystems are highly productive, serving as crucial spawning and juvenile habitats for many fish species worldwide (Blaber and Blaber, 1980; Orth et al., 1984; Shulman, 1985). At the same time, coastal systems are under increasing anthropogenic pressure, resulting in global losses of species biodiversity and habitat degradation (Lotze et al., 2006; Worm et al., 2006; Waycott et al., 2009). However, management of natural fish resources is widely limited to fishery regulations for adult stocks. Regional coastal zone management rarely includes a certain focus on important fish nursery areas although impacts can have drastic consequences on resilience and recruitment strength of a fish population. The definition after Beck et al. (2001) emphasizes the concept of a nursery area, revealing the importance to identify the quantitative contribution of particular areas to the adult population of fish or invertebrates. Accordingly, a nursery area is not just one of multiple areas inhabited by juveniles of a population, but is defined as an area, which contributes a greater than average number of individuals to the adult population (Beck et al., 2001; Dahlgren et al., 2006). According to these quantitative aspects, research is nowadays increasingly focused on studies on juvenile-adult habitat connectivity and dependency of fish species on certain habitats (Thorrold et al., 2001; Forrester and Swearer, 2002; Gillanders, 2002a). This connectivity and dependency aspect is highly relevant to evaluate the relation between habitat availability and fish population dynamics and at the same time exposed a great lack of knowledge on general life history and migratory patterns of economic important fish species with a great uncertainty of obligate or facultative habitat use (Able, 2005).
Otoliths are the calcified ear stones in teleost fish and were formerly used for annual age and growth estimations (Beamish, 1979; Hoyer et al., 1985). During the last decades the scope of otolith research further expanded and resulted in new scientific applications, such as otolith microstructure analyses for age estimates of early life stages on a daily scale (Nishimura and Yamada, 1984; Campana and Moksness, 1991), otolith shape analyses for stock identification (Campana and Casselman, 1993; Tracey et al., 2006) and chemical analyses of the otolith core region to investigate elemental signals and spatial differences in juvenile fish otoliths (De Pontual et al., 1999; Gillanders and Kingsford, 2003; Hamer et al., 2003). This otolith chemistry approach takes advantage of specific fish otolith characteristics, being metabolically inert, growing continuously without resorption and incorporating elements into their growing surface. Hence, these calcified structures reflect the physical and chemical characteristics of the ambient water, where the fish is residing during the early ontogeny (Campana, 1999). With the implementation of otolith microchemistry, a new age of fishery research was established, resulting in studies concerning stock discrimination analyses (Geffen et al., 2011; Heidemann et al., 2012) and fish migration patterns (Limburg, 1995; Barnett-Johnson et al., 2008), mainly based on elements indicating hydrologic conditions like strontium and barium, which are known as markers for salinity gradients (Kennedy et al., 2002; Elsdon and Gillanders, 2005). Further approaches to investigate habitat connectivity were predominately based on a few selected elements in tropic and sub-tropic fishes (Milton et al., 1997; Thorrold et al., 2001). Studies concerning the elemental inventory of fish otoliths and habitat connectivity of economically important fish species in the North Atlantic region, such as flatfishes, cod and herring, are rather scarce (but see Brophy et al., 2003; Vasconcelos et al., 2008; Thorisson et al., 2011).
The Atlantic herring (Clupea harengus, L. 1958) is one of the most economically important marine fishery target species in the world (FAO, 2016). Moreover, this small pelagic fish is of high ecological relevance, linking different trophic levels in the marine food web (Casini et al., 2004; Möllmann et al., 2004). In the Baltic Sea, C. harengus is a highly migratory fish, accomplishing annual migrations between their feeding grounds in the Kattegat-Skagerrak and their coastal spawning grounds located in bays, estuaries and lagoons along the coastal Western Baltic Sea during spring, showing a distinct homing behavior (Wheeler and Winters, 1984; McQuinn, 1997). This herring population is of high economic value for the Western Baltic Sea region. Herring reproduction success is affected by multiple abiotic and biotic parameters (Holliday and Blaxter, 1960; Laine and Rajasilta, 1999; Peck et al., 2012; Kotterba et al., 2017), as well as by human-induced stressors, such as pollution (Dethlefsen et al., 1996; Incardona et al., 2012) or eutrophication (Aneer, 1987; Rahikainen et al., 2017; von Nordheim et al., 2018). Eutrophication processes in the past decades led to severe habitat degradation of important spawning areas in Baltic coastal waters (Kääriä et al., 1988; Kanstinger et al., 2016). Western Baltic herring recruitment decreased during the past two decades and remained on a low level ever since, reaching the lowest value of the time-series in 2017 (ICES, 2018). The reasons for this decline are still unclear, but evidence mounts for a synergy of eutrophication, climate change and resulting ecological cascades negatively affecting the survival of early life stages (Moll et al., 2018). However, quantitative studies on the contribution of single coastal juvenile habitats to the adult herring population are lacking, but are of vital importance to understand the impact of local hazards to fish population dynamics and to establish adequate coastal zone management strategies.
The objectives of this study are to identify differences in the elemental composition of age-0 herring otoliths from different inshore spawning areas along the Western Baltic coastline. Hypothesizing that herring otoliths from different juvenile areas differ in their elemental composition, we created an elemental fingerprinting index (EFI) based on a multi-element approach.
Materials and Methods
Age-0 Herring Sampling
All four inner coastal sampling sites are anthropogenic-influenced spawning areas for the Western Baltic spring spawning herring population, located along the German coastline of the Western Baltic Sea. In June 2016, sampling was conducted in the eutrophic Schlei Fjord (SF: ∼54.62°N, 09.09°E) and in Kiel Canal (KC; ∼54.33°N, 09.72°E), an artificial waterway with heavy ship traffic, connecting the Baltic Sea and the North Sea. Furthermore, young-of-the-year (YOY) herring was sampled in the Warnow Estuary (WE; ∼54.12°N, 12.10°E), with its dockyard and landing pier for cruise liners and ferries, and the eutrophic Greifswald Bay (GB; ∼54.25°N, 13.50°E) (see Figure 1). YOY herring was caught by beach seine (7 m opening, 5 mm mesh size, wing height 1.5 m) in the SF sampling area and by purse seine (40 m length, 4 mm mesh size, 3 m height) in KC, both nets were pulled by two people. Sampling areas WE and GB were sampled with a ring trawl of 1 m diameter (1550 μm mesh size) onboard a research vessel. Hydrological parameters [sea surface temperatures (SST) and salinity] were either recorded with a hand held field probe (YSI Professional Plus) or a remotely operated CTD. The characteristics of each sampling site, including abiotic parameters during sampling, mean total length (TL mm) of YOY herring, as well as numbers of individuals per sampling site are summarized in Table 1. Herring were frozen immediately after the catch and were transported to the laboratory for otolith dissection.
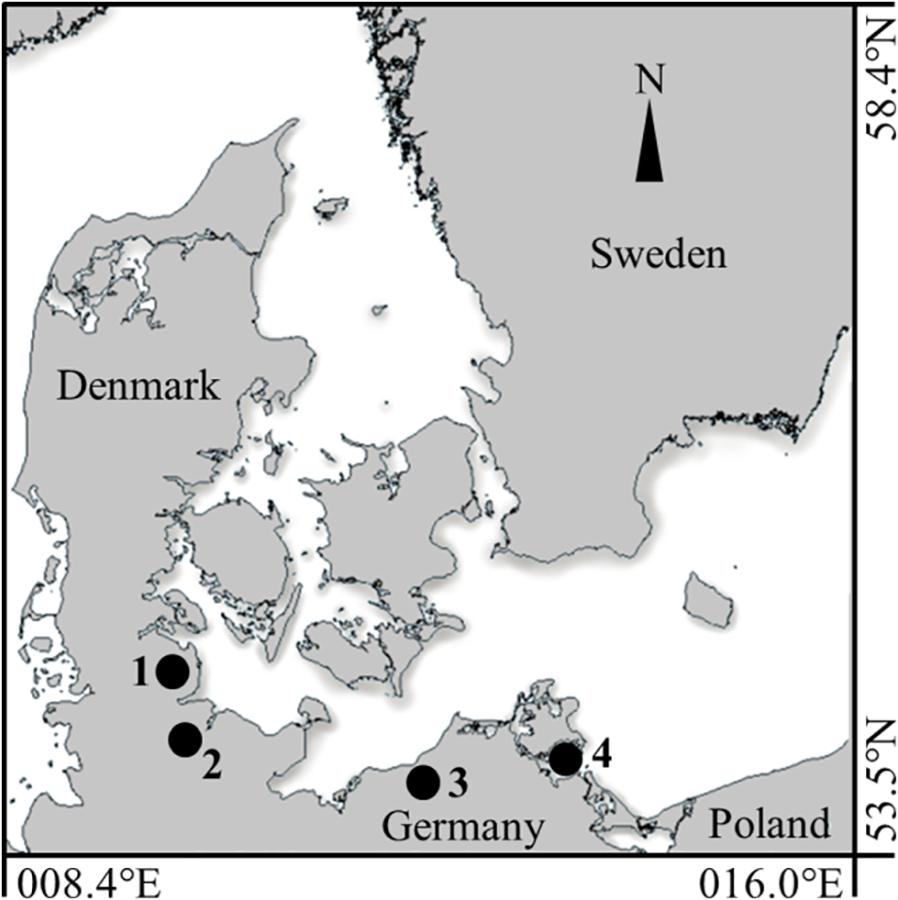
Figure 1. Inshore sampling locations along the German coastline of the Western Baltic Sea. The four inshore spawning sites, sampled in summer 2016, are indicated by black circles (1) Schlei Fjord (SF), (2) Kiel Canal (KC), (3) Warnow Estuary (WE) and (4) Greifswald Bay (GB).
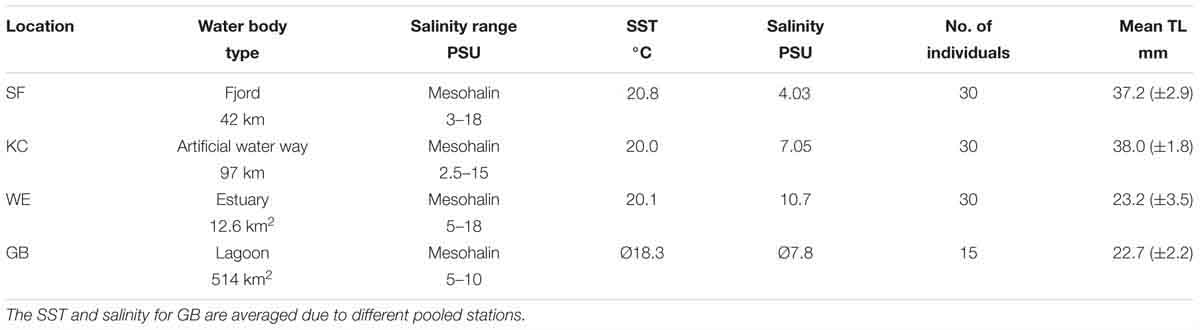
Table 1. Description of each inshore sampling area [Schlei Fjord (SF), Kiel Canal (KC), Warnow Estuary (WE), Greifswald Bay (GB)] with respective water body type, prevailing salinity ranges in PSU (Messner and von Oertzen, 1990; Feibicke, 1997; Kafemann et al., 2000), abiotic parameters at sampling location (SST and salinity), number of analyzed individuals and mean total length TL [mm] of caught age-0 herring with standard deviation.
Young-of-the-year herring sampling was conducted under current licenses for wild fish sampling according to Mecklenburg-Western Pomeranian (Germany) fishery law (§11 LFischG, Landesamt für Landwirtschaft, Lebensmittelsicherheit und Fischerei, Mecklenburg-Vorpommern).
Sample Processing
Prior to otolith dissection, microscope slides were washed in 2% nitric acid (Rotipuran Supra 69%, Carl Roth), were rinsed with ultrapure water and were dried under a flow cabin to keep them free from dust particles. Double-sided adhesive transparent pads, placed on the cleaned glass slides, were used as otolith fixing material (for further information, see Supplementary Figure 1). Herring otoliths were dissected under a microscope (Olympus SD 30) and sagittal otoliths were removed with non-metallic equipment to minimize potential contamination. After dissection, each otolith (either left or right sagittal otolith) was rinsed in ultrapure water several times to remove any adhesive tissue and 30 otoliths per sampling area were used for chemical analysis of habitat-specific signatures, except for GB (n = 15). The chosen sample size was above the commonly accepted standard for this kind of analysis (Gillanders, 2002a; Hamer et al., 2003) and was assumed to be adequate to test our hypotheses. Otoliths were transferred and placed on the fixing material. Microscope slides with attached otoliths were stored in a plastic vials for further processing in the laboratory.
Analytical Methods
Elemental composition of herring otolith core regions were analyzed with the laser ablation-inductively coupled plasma-mass spectrometry (LA-ICP-MS) technique, using a ThermoFinnigan ELEMENT2 ICP-MS with a Nd:YAG UP213 laser ablation system at the Max Planck Institute of Chemistry in Mainz, Germany. The certified reference material NIST612 (National Institute of Standards and Technology) and the micro analytical reference material MACS3 (United States Geological Survey) were used for calibration (Jochum et al., 2007; Jochum et al., 2011) and were analyzed prior to sample measurement and reference material measurements were constantly repeated to ensure quality. Otolith core regions were ablated with 80 μm laser spot size at 60% power with a pulse repetition rate of 10 Hz and energy densities of ∼10.2 J cm-2. Dwell durations of otoliths were operated manually, due to individual otolith size and thickness. Further technical information of the New Wave laser ablation system and the operating parameters for the ELEMENT2 ICP-MS (further information is given in Supplementary Table 1).
Data Analysis
Values near detection limits were excluded and only elements with no more than two missing values were considered for the analysis. In total, the following 17 elements were used: lithium (Li), boron (B), sodium (Na), magnesium (Mg), silicon (Si), manganese (Mn), iron (Fe), cobalt (Co), copper (Cu), zinc (Zn), rubidium (Rb), strontium (Sr), tin (Sn), barium (Ba), lead (Pb), thallium (Tl) and uranium (U). Potential chemical differences between different sampling locations were visualized by multidimensional scaling (MDS-plot), using the PRIMER Software (Version 6.1.13) and the dissimilarity matrix of calculating the Euclidean distances. Elemental concentrations were log(x+1)-transformed for standardization. Statistical tests were performed with the software SPSS, statistical significance (significance level of p < 0.05) was tested by using one-way analysis of variance (ANOVA). Data sets were tested for variance homoscedasticity by Levene’s test. Due to variance heteroscedasticity and unequal sample sizes, statistical significances were tested with ANOVA including Welch correction (Welch, 1938) which also compensates for non-normal distributions and post-hoc test for inhomogeneous variances and sample sizes (Games Howell test). EFI values were tested for significant differences within and between sampling areas using Welch Test and Games Howell post-hoc test to investigate significant differences between the four sampling sites.
Elemental Fingerprinting Index (EFI)
We visualized chemical otolith signatures for each spawning area by radar plots, based on the relative mean proportion of each element to the highest concentration found among the total of 105 individuals included in this study. We modified a well-established ecological overlap index (Schoener index, Schoener, 1974) to create the EFI, a novel measure of similarity between 2 fish individuals considering numerous chemical signatures in their otoliths. The EFIi,j describes the comparison of two fish individuals that includes 17 elements and was calculated according to the following equation:
Where conc_xi and conc_xj represent the concentrations of element x in fish i and fish j, respectively, while min (conc_xi; conc_xj) represents the lower value of these two concentrations (for detailed information, see Supplementary Figures 2, 3). The EFI ranges from 0 to 1, where a value of 0 indicates that two compared individuals are most different in otolith elemental composition, whereas a value of 1 indicating the highest similarity in elemental composition of two comparing individuals. We conducted elemental concentration comparisons for all individuals within one sampling area resulting in = 435 pairs for each sampling area (except for GB: = 105 pairs).
And comparisons of all individuals between the different sampling areas:
pairs for each comparison (GB:).
Subsequently, we tested whether the variance among the EFIs derived from comparisons within the areas differed significantly from the EFIs obtained from comparisons of individuals from differing areas.
Results
Chemical Signatures in Herring Otoliths
In total, 39 elements could be determined in young-of-the-year otolith samples from different inshore spawning areas. Altogether, 17 elements were used to distinguish the chemical otolith signatures from different sampling sites. The mean elemental concentrations are given in Supplementary Table 2. The element concentrations found in all 105 individuals were included in an MDS-plot for visualizing the differences in chemical signatures for each sampling area (Figure 2). Otolith signals from the western sampling areas Schlei Fjord (SF) and Kiel Canal (KC) are more similar in their elemental concentrations compared to the eastern sampling areas Warnow Estuary (WE) and Greifswald Bay (GB) with some intermingling signals from KC, GB and WE. Elemental otolith compositions from WE showed a higher variability in individual element concentration. However, a clear separation between the western and eastern spawning areas is discernible (Figure 2).
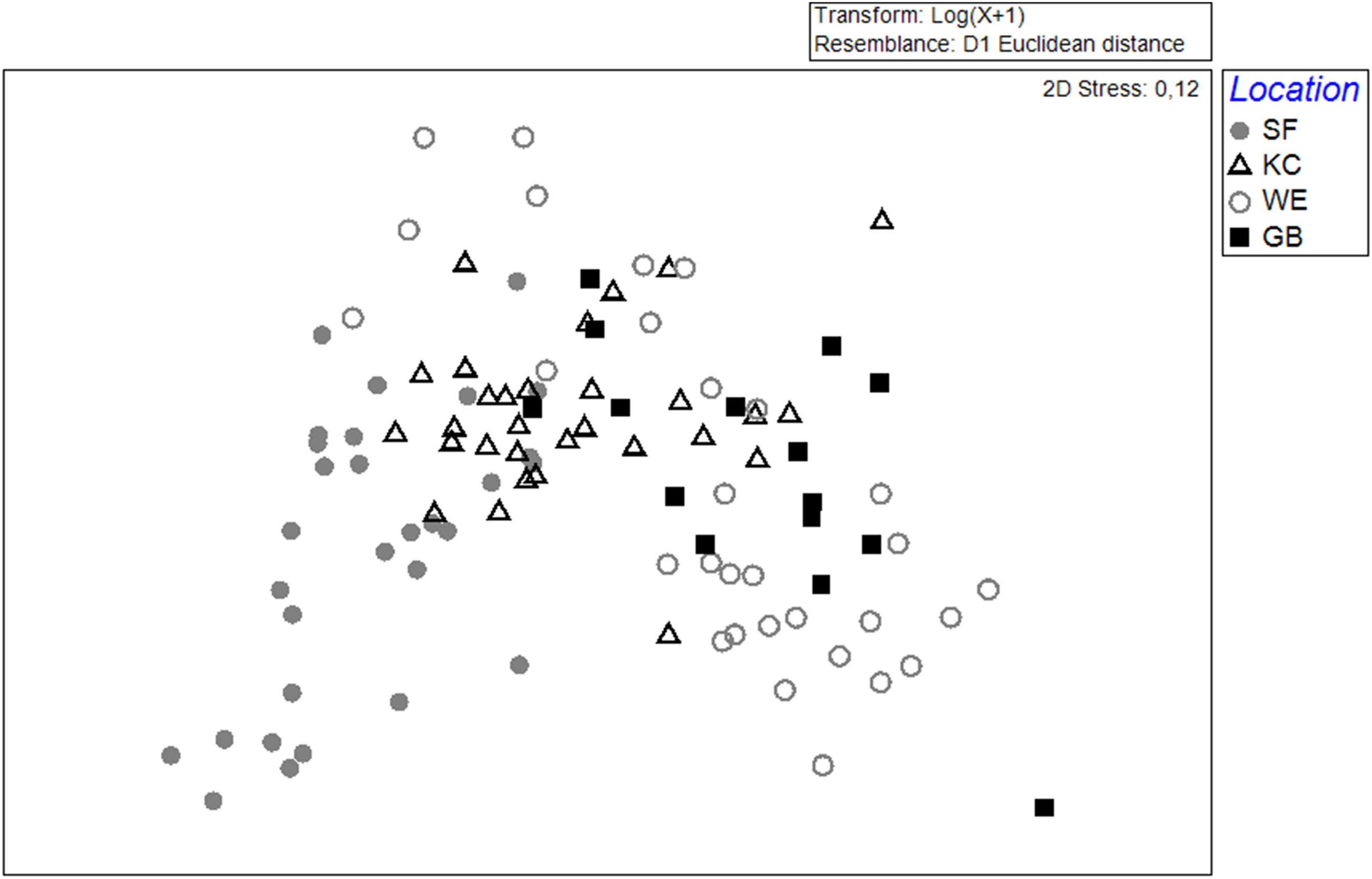
Figure 2. MDS-Plot, using the Euclidean distance to classify groups of age-0 herring otoliths (based on 17 elements). Data were log(x+1)-transformed for standardization. The Schlei Fjord (SF, gray circles) and Kiel Canal (KC, black open triangles) are located along the western German coastline. The Warnow Estuary (WE, gray open circles) and Greifswald Bay (GB, black squares) represented samples from the eastern spawning areas. Each data point represents a single otolith and individual fish (n = 105).
Significant Differences in Elemental Compositions
We found continuously high significant differences (p ≤ 0.001) in element concentrations between all four sampling areas and significant differences (p = 0.008) for tin (Sn) (Table 2). Based on these results we performed post-hoc tests for single elements to identify habitat-specific significant differences between single sampling areas. Detailed information on the results for each element is given in Table 3. We found 13 elements to be significantly different between SF-WE comparison. The comparisons SF-KC and SF-GB revealed significant differences of 11 elements with uranium (U), being significantly different between SF-GB. Ten elements were significantly different within the KC-WE comparison. Furthermore, 7 elements were significantly different between KC-GB. The comparison WE-GB revealed significant differences in 6 elements (Table 3).
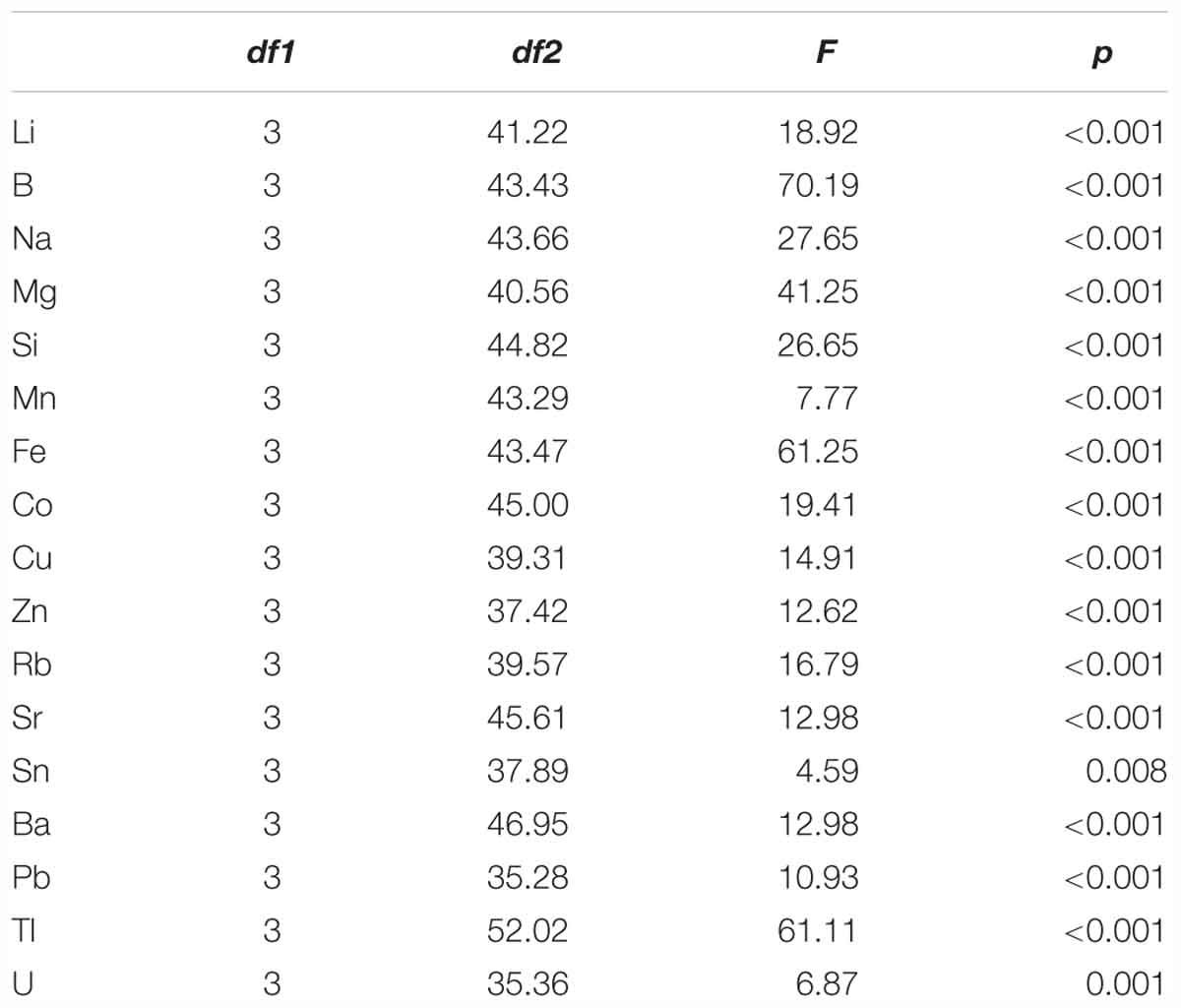
Table 2. Results of ANOVA with Welch correction to identify statistical differences in age-0 herring otolith element concentration between the four juvenile inshore areas [Schlei Fjord, Kiel Canal, Warnow Estuary (n = 30) and Greifswald Bay (n = 15)], with degrees of freedom (df1 and df2), F-value and significance level (p) for each single element.
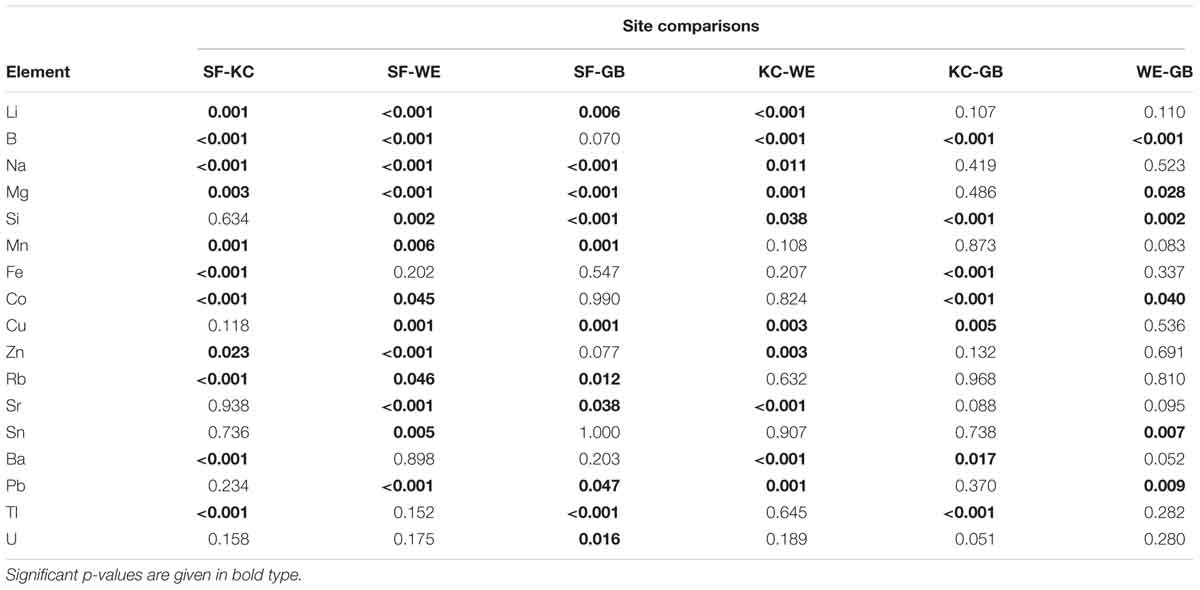
Table 3. Results of Games Howell post-hoc test to identify site-specific significant differences for each element with respective significant levels (p ≤ 0.05), including sample sizes of n = 30 for Schlei Fjord (SF), Kiel Canal (KC) and Warnow Estuary (WE) and n = 15 for Greifswald Bay (GB).
Elemental Fingerprinting Indices (EFI)
The entire chemical otolith inventory for each inshore coastal spawning area revealed 4 habitat-specific fingerprint plots with similarities in the generally dominance of single elements, but clear differences in their relative element ratio (Figure 3). We conducted multi-elemental concentration pairwise comparisons for individuals within one sampling site and pairwise comparisons of individuals between different sampling sites, using EFI to identify similarities in element concentrations within one group and differences between groups. Elemental concentrations of individuals within each of the SF, KC and GB sample groups were rather stable, whereas WE samples were slightly more variable in their multi-element composition (lower mean EFI). Comparisons of individual fingerprints between two sampling groups revealed clear differences between WE and SF, whereas comparisons between SF-KC and KC-GB were more similar in their element signatures (Table 4). EFI values for comparisons of SF-GB, WE-KC and WE-GB were smaller than EFI values of within-group comparisons, indicating that elemental concentrations between all four groups are more different than within groups (Table 4). Regarding statistical differences between two group comparisons, the EFI values showed highly significant differences for all comparisons (GB-KC: F(2,288.7) = 253.2, p < 0.001; GB-WE: F(2,345.1) = 144.8, p < 0.001; GB-SF: F(2,289.1) = 410.8, p < 0.001; WE-KC: F(2,1767) = 959.5, p < 0.001; WE-SF: F(2,1013.4) = 1413.6, p < 0.001; SF-KC: F(2,975.5) = 563.2, p < 0.001). Post-hoc test results are shown in Supplementary Table 3.
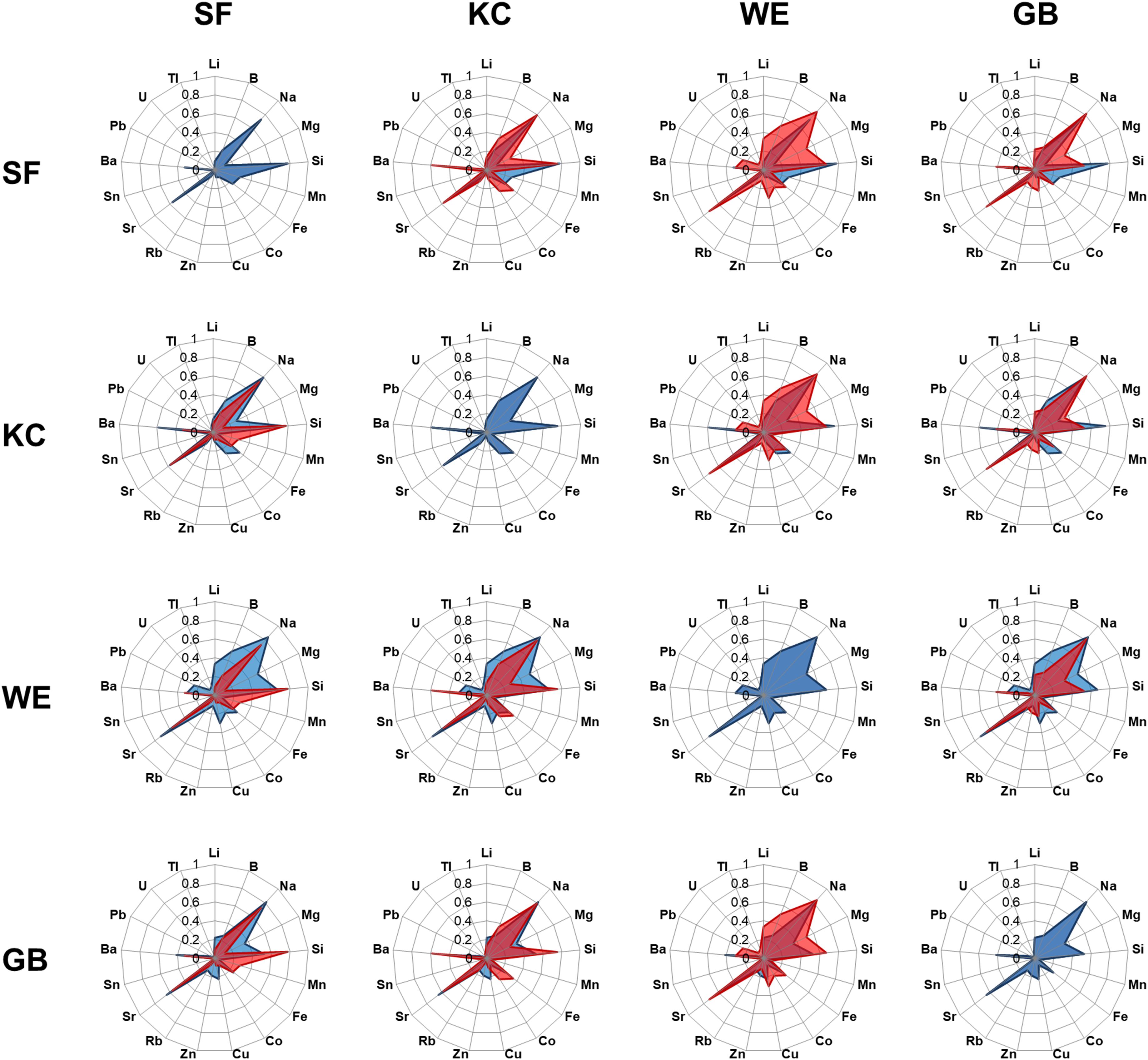
Figure 3. Mean standardized concentrations of each single element for each sampling area are shown in a defined order, revealing radar plots with site-specific elemental fingerprints (blue radar plots in horizontal rows). Data were standardized to deal with different concentration units. Each site-specific radar plot was compared with the respective other three site-specific radar plots (shown by column in red) to visualize similarity or differences in elemental concentrations of western locations [Schlei Fjord (SF), Kiel Canal (KC)] and eastern locations [Warnow Estuary (WE) and Greifswald Bay (GB)].
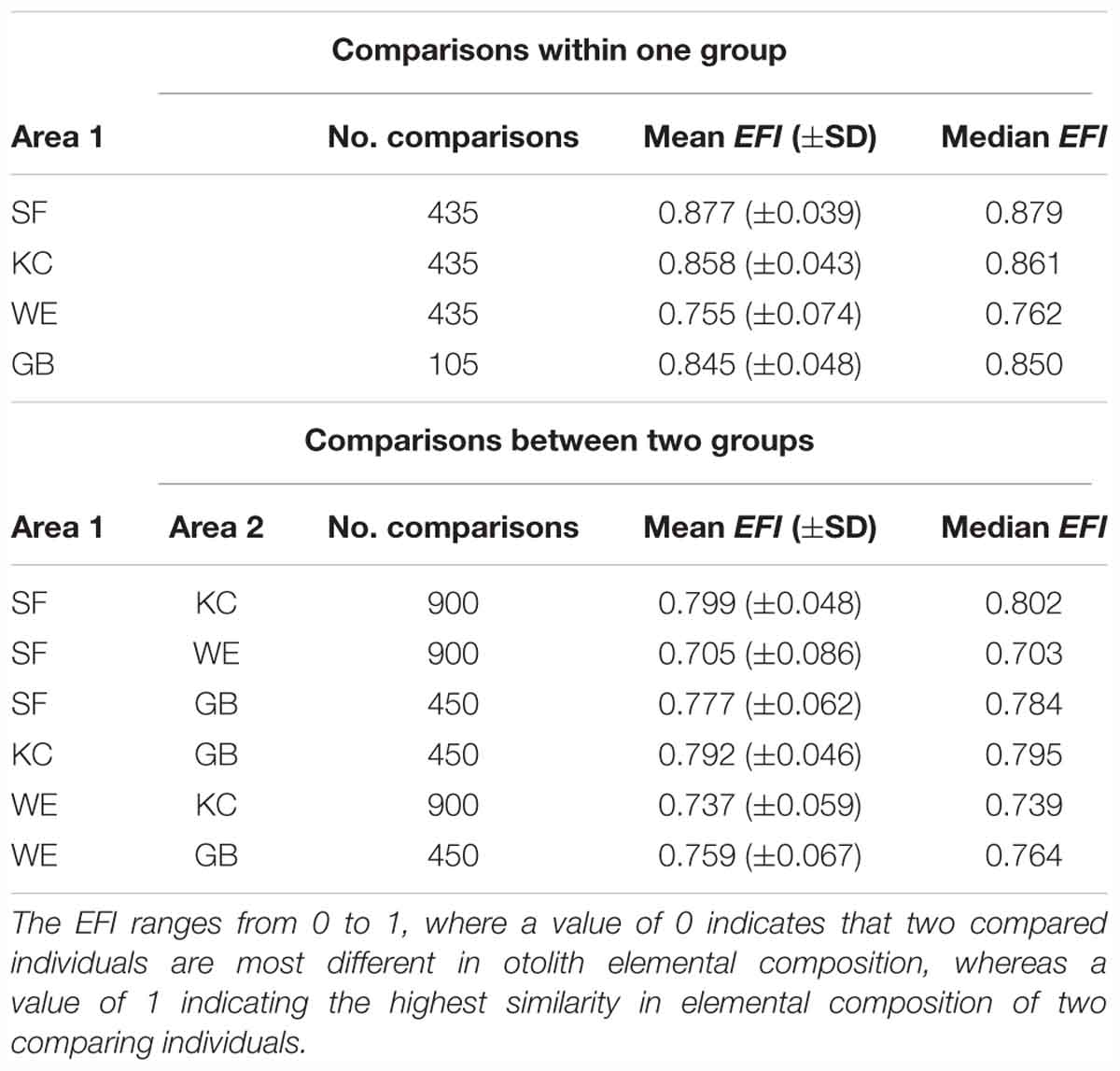
Table 4. Elemental fingerprinting indices {mean EFI [with standard deviation (±SD)] and median EFI} with number of comparisons for each sampling area (upper table) and results of comparisons between respective groups (lower table) with number of comparisons.
Discussion
The successful outcome of otolith microchemistry studies strongly depends on spatial variability of elemental concentrations between investigated habitats. There is abundant evidence that fish otoliths from different basins and even sites within basins show spatial variability in their elemental composition (De Pontual et al., 1999; Rooker et al., 2001; Yamane et al., 2010). Our approach included the entire inventory of otolith-core elements summarized by a unique EFI, based on 17 elements. To our best knowledge, this approach allows comparing multi-elemental chemical composition of otoliths within and between herring juvenile areas for the first time. Our results show significantly distinct chemical “fingerprints” on the scale of particular bays and estuaries providing a chemical “birth certificate” of their natal origin.
Environmental Factors
The incorporation of elements into the growing surface of fish otoliths is related to environmental variables, such as water temperatures, salinity and the availability of specific elements in the water (Tzeng et al., 1997; Elsdon and Gillanders, 2003). In addition, endogenous factors (such as physiology, genetics and growth effects) control incorporation processes (Fowler et al., 1995; Martin and Thorrold, 2005; Barnes and Gillanders, 2013). Elemental uptake by prey digestion might be marginal, at least for some species (Farrell and Campana, 1996; Milton and Chenery, 2001; Buckel et al., 2004). The impact of environmental variables on element incorporation is sufficiently studied, but results are rather contradictory (Kalish, 1989; Radtke et al., 1990; Townsend et al., 1992; Hoff and Fuiman, 1995), indicating that interactions between environmental and endogenous factors might be species-specific. However, it is stated that non-physiologically regulated elements (such as Sr, Ba, Mn) are usable for life history studies (Elsdon and Gillanders, 2003). Woodcock et al. (2012) indicated that Mg, an element often used in microchemistry studies, might also be physiologically regulated in fish otoliths. Hence, validation experiments for the species of interest are highly recommended to verify interpretations (Kalish, 1989).
Whereas studies on the elemental uptake are almost entirely focused on single elements (see review in Elsdon and Gillanders, 2003), multi-element investigations are rather scarce (Fowler et al., 1995; Elsdon and Gillanders, 2002). With our multi-element approach, including also heavy metals, we assume that observed chemical patterns are more related to habitat-specific differences than affected by endogenous factors. However, more research is required to understand the impact and interaction of different factors on elemental incorporation in herring otoliths.
Potential temporal variability of element concentrations is one important aspect to be considered in future connectivity studies (Milton et al., 1997; Hamer et al., 2003; Swearer et al., 2003; Arkhipkin et al., 2009). Although some studies showed high temporal variability in elemental concentrations, other studies revealed inter-annual consistency of elements (Patterson et al., 1999; Rooker et al., 2001), indicating that variability might be habitat-specific. Gillanders (2002b) stated to build up a library of elemental fingerprints over several years for each study area to increase the interpretation success of fingerprint patterns from juvenile fish, regarding contribution estimates of single reproduction areas to the overall fish population. A multi-year sampling should be considered in the future to investigate temporal consistency of the found habitat-specific signals.
Element Concentrations in Herring Otoliths
The concentrations levels found in YOY herring otoliths corresponded to levels for single elements (Mg, Zn, Sr, Ba, Pb) from a study by Brophy et al. (2003), except for the two elements Sr and Ba. Those in particular are marker elements for an origin relative to certain salinity conditions, with higher Sr concentrations in marine habitats and higher Ba concentrations in freshwater habitats (Tzeng and Tsai, 1994; Elsdon and Gillanders, 2005). The concentrations found in otolith samples from inshore juvenile areas were generally lower in Sr and higher in Ba, compared to herring otoliths from the North Sea (Brophy et al., 2003), reflecting the brackish Baltic Sea ecosystem, with high salinity gradients (from rather marine salinities of ∼30 PSU) in adult herring feeding areas up North in the Kattegat and Skagerrak to more brackish conditions within the Western Baltic Sea. In addition to the overall Baltic Sea salinity gradient from Southwest to Northeast, there are multiple local inshore-offshore salinity gradients composed by river estuaries or other transitional waters, making it difficult to interpret results solely based on these salinity signatures. Hence, we focused on the multiple-element approach to find differences in elemental composition of herring otolith from different coastal spawning grounds.
Sampling in four historically known juvenile areas was performed in summer 2016. Due to sampling site-specific time of spawning and larval growth, we were not able to sample YOY herring otoliths of the same size. However, high concentration variations between different otolith sizes could not be observed during our measurements. Using the LA-ICP-MS approach for unpolished herring otoliths within a specific size-range was applicable, at least for the Western Baltic Sea, since all herring samples were collected within their inshore coastal reproduction areas. Moreover, the approach of solution-based ICP-MS is an approved method, where whole otoliths are dissolved in nitric acid to analyze chemical signatures and habitat-specific patterns (Patterson et al., 1999; Rooker et al., 2003). However, the choice of sample processing and analytical methods is depending on single fish species and their particular habitat utilization.
Multi-Element Approach
Although we were able to detect concentration values of 39 elements in total with the LA-ICP-MS approach, we had to exclude all rare earth metals and some heavy metals (such as Cd and Hg), due to concentration levels below the instrument detection threshold. All together 17 elements could be used for data analysis, which is still an appreciable element number. In most studies, differences in elemental compositions were mainly based on a few established elements (e.g., Ba, Mg, Mn, Sr) (see Thorrold et al., 1998; Campana et al., 2000) with some exceptions, focusing also on multiple elements (Edmonds et al., 1991; Leakey et al., 2009). For our interdisciplinary multiple-element approach, we created a unique and innovative EFI to describe differences and similarities of the otolith chemical inventory. The EFIs were more similar within one group/sampling area than between different sampling areas, indicating that habitat-specific chemical signatures were more stable within a particular area and more variable between different sampling areas, except for the comparison GB-WE, due to higher variability in chemical composition of WE samples. Our results show a clear separation between the western and eastern spawning areas, based on site-specific water chemistry, including salinity and pollutant signals. We can only speculate, if these patterns reflect the historical pollutant signals of different water management strategies, caused by the boundary of the “German Wall,” since pollutants are known to accumulate into the sediment over decades (Deely and Fergusson, 1994; Ruiz, 2001; Buccolieri et al., 2006) or if different elemental signals are based on site-specific alterations (ship traffic, industrial and agricultural discharge).
The lack of knowledge on the quantitative contribution of single reproduction areas to the overall herring population is problematic, since herring show a distinct homing behavior to their traditional spawning areas. If important nursery areas are affected by human-induced habitat degradation and environmental conditions of important nurseries become unfavorable for successful herring reproduction, but herring continue their migrations to these specific anthropogenic-stressed habitats, this might have severe impacts for the population persistence. This unknown habitat dependency demonstrates the relevance of our results, which set the baseline for future estimates on habitat productivity, which could lead to a more directed coastal zone management of important spawning grounds and coastal nursery areas in the future.
Author Contributions
DM, PK, LvN, and PP conceptualized the study. DM wrote the manuscript, performed the sampling and was responsible for laboratory processing and sample preparation. KJ and DM performed the measurements with the LA-ICP-MS. DM and PK analyzed the data. PK contributed the EFI calculations and EFI statistical analyses. PK, LvN, PP, and KJ revised the manuscript.
Funding
The research leading to these results received funding from BONUS INSPIRE (DM) and BONUS BIO-C3 (PK), the joint Baltic Sea research and development program by BONUS (Art 185), funded jointly by the European Union and the Federal Ministry of Education and Research of Germany (BMBF 03F0681; 03F0682). LvN received funds from the German Federal Environmental Foundation (DBU). PP and DM were funded by the EU Data Collection Framework (DCF). Open Access publication fee was funded by the Thünen Institute of Baltic Sea Fisheries, Rostock, Germany.
Conflict of Interest Statement
The authors declare that the research was conducted in the absence of any commercial or financial relationships that could be construed as a potential conflict of interest.
Acknowledgments
We would like to thank all colleagues from the Thünen Institute of Baltic Sea Fisheries, who contributed to the study. We would like to thank the editor and the reviewers for their helpful comments. We would also like to thank Rüdiger Neukamm from the Landessportfischerverband Schleswig-Holstein e.V., Matthias Nanz and the crew of the research vessel FRV CLUPEA for their help during sampling. Thanks are extended to Brigitte Stoll and Ulrike Weis from the Max Planck Institute for Chemistry in Mainz, Germany, for their help during the measurements.
Supplementary Material
The Supplementary Material for this article can be found online at: https://www.frontiersin.org/articles/10.3389/fmars.2019.00191/full#supplementary-material
References
Able, K. W. (2005). A re-examination of fish estuarine dependence: evidence for connectivity between estuarine and ocean habitats. Estuar. Coast. Shelf Sci. 64, 5–17. doi: 10.1016/j.ecss.2005.02.002
Aneer, G. (1987). High natural mortality of Baltic herring (Clupea harengus) eggs caused by algal exudates? Mar. Biol. 94, 163–169. doi: 10.1007/BF00392928
Arkhipkin, A. I., Schuchert, P. C., and Danyushevsky, L. (2009). Otolith chemistry reveals fine population structure and close affinity to the Pacific and Atlantic oceanic spawning grounds in the migratory southern blue whiting (Micromesistius australis australis). Fish. Res. 96, 188–194. doi: 10.1016/j.fishres.2008.11.002
Barnes, T. C., and Gillanders, B. M. (2013). Combined effects of extrinsic and intrinsic factors on otolith chemistry: implications for environmental reconstructions. Can. J. Fish. Aquat. Sci. 70, 1159–1166. doi: 10.1139/cjfas-2012-0442
Barnett-Johnson, R., Pearson, T. E., Ramos, F. C., Grimes, C. B., Bruce, and MacFarlane, R. (2008). Tracking natal origins of salmon using isotopes, otoliths, and landscape geology. Limnol. Oceanogr. 53, 1633–1642. doi: 10.4319/lo.2008.53.4.1633
Beamish, R. J. (1979). Differences in the age of Pacific hake (Merluccius productus). J. Fish. Res. Board Can. 36, 141–151. doi: 10.1139/f79-023
Beck, M. W., Heck, K. L., Able, K. W., Childers, D. L., Eggleston, D. B., Gillanders, B. M., et al. (2001). The identification, conservation, and management of estuarine and marine nurseries for fish and invertebrates. BioScience 51, 633–641. doi: 10.1641/0006-35682001051
Blaber, S. J. M., and Blaber, T. G. (1980). Factors affecting the distribution of juvenile estuarine and inshore fish. J. Fish. Biol. 17, 143–162. doi: 10.1111/j.1095-8649.1980.tb02749.x
Brophy, D., Danilowicz, B. S., and Jeffries, T. E. (2003). The detection of elements in larval otoliths from Atlantic herring using laser ablation ICP-MS. J. Fish. Biol. 63, 990–1007. doi: 10.1046/j.1095-8649.2003.00223.x
Buccolieri, A., Buccolieri, G., Cardellicchio, N., Dell’Atti, A., Di Leo, A., and Maci, A. (2006). Heavy metals in marine sediments of Taranto Gulf (Ionian Sea, Southern Italy). Mar. Chem. 99, 227–235. doi: 10.1016/j.marchem.2005.09.009
Buckel, J. A., Sharack, B. L., and Zdanowicz, V. S. (2004). Effect of diet on otolith composition in Pomatomus saltatrix, an estuarine piscivore. J. Fish. Biol. 64, 1469–1484. doi: 10.1111/j.0022-1112.2004.00393.x
Campana, S. E. (1999). Chemistry and composition of fish otoliths: pathways, mechanisms and applications. Mar. Ecol. Prog. Ser. 188, 263–297. doi: 10.3354/meps188263
Campana, S. E., and Casselman, J. M. (1993). Stock discrimination using otolith shape analysis. Can. J. Fish. Aquat. Sci. 50, 1062–1083. doi: 10.1139/f93-123
Campana, S. E., Chouinard, G. A., Hanson, J. M., Fréchet, A., and Brattey, J. (2000). Otolith elemental fingerprints as biological tracers of fish stocks. Fish. Res. 46, 343–357. doi: 10.1016/S0165-7836(00)00158-2
Campana, S. E., and Moksness, E. (1991). Accuracy and precision of age and hatch date estimates from otolith microstructure examination. ICES J. Mar. Sci. 48, 303–316. doi: 10.1093/icesjms/48.3.303
Casini, M., Cardinale, M., and Arrhenius, F. (2004). Feeding preferences of herring (Clupea harengus) and sprat (Sprattus sprattus) in the southern Baltic Sea. ICES J. Mar. Sci. 61, 1267–1277. doi: 10.1016/j.icesjms.2003.12.011
Dahlgren, C. P., Kellison, G. T., Adams, A. J., Gillanders, B. M., Kendall, M. S., Layman, C. A., et al. (2006). Marine nurseries and effective juvenile habitats: concepts and applications. Mar. Ecol. Prog. Ser. 312, 291–295. doi: 10.3354/meps312291
De Pontual, H., Lagardère, F., Troadec, H., Batel, A., DéSaunay, Y., and Koutsikopoulos, C. (1999). Otoliths imprinting of sole (Solea solea) from the bay of biscay: a tool to discriminate individuals from nursery origins? Oceanol. Acta 23, 497–513. doi: 10.1016/S0399-1784(00)00140-7
Deely, J. M., and Fergusson, J. E. (1994). Heavy metal and organic matter concentrations and distributions in dated sediments of a small estuary adjacent to a small urban area. Sci. Total Environ. 153, 97–111. doi: 10.1016/0048-9697(94)90106-6
Dethlefsen, V., Von Westernhagen, H., and Cameron, P. (1996). Malformations in North Sea pelagic fish embryos during the period 1984–1995. ICES J. Mar. Sci. 53, 1024–1035. doi: 10.1006/jmsc.1996.0128
Edmonds, J., Caputi, N., and Morita, M. (1991). Stock discrimination by trace-element analysis of otoliths of orange roughy (Hoplostethus atlanticus), a deep-water marine teleost. Mar. Freshw. Res. 42, 383–389. doi: 10.1071/MF9910383
Elsdon, T. S., and Gillanders, B. M. (2002). Interactive effects of temperature and salinity on otolith chemistry: challenges for determining environmental histories of fish. Can. J. Fish. Aquat. Sci. 59, 1796–1808. doi: 10.1139/f02-154
Elsdon, T. S., and Gillanders, B. M. (2003). Reconstructing migratory patterns of fish based on environmental influences on otolith chemistry. Rev. Fish Biol. Fish. 13, 219–235. doi: 10.1023/B:RFBF.0000033071.73952.40
Elsdon, T. S., and Gillanders, B. M. (2005). Alternative life-history patterns of estuarine fish: barium in otoliths elucidates freshwater residency. Can. J. Fish. Aquat. Sci. 62, 1143–1152. doi: 10.1139/f05-029
FAO (2016). The State of World Fisheries and Aquaculture 2016: Contributing to Food Security and Nutrition for All. Rome: FAO.
Farrell, J., and Campana, S. E. (1996). Regulation of calcium and strontium deposition on the otoliths of juvenile tilapia, Oreochromis niloticus. Comp. Biochem. Physiol. A Physiol. 115A, 103–109. doi: 10.1016/0300-9629(96)00015-1
Feibicke, M. (1997). Impact of nitrate addition on phosphorus availability in sediment and water column and on plankton biomass —Experimental field study in the shallow brackish schlei Fjord (Western Baltic, Germany). Water Air Soil Pollut. 99:445. doi: 10.1007/BF02406884
Forrester, G. E., and Swearer, S. E. (2002). Trace elements in otoliths indicate the use of open-coast versus bay nursery habitats by juvenile California halibut. Mar. Ecol. Prog. Ser. 241, 201–213. doi: 10.3354/meps241201
Fowler, A. J., Campana, S. E., Thorrold, S. R., and Jones, C. M. (1995). Experimental assessment of the effect of temperature and salinity on elemental composition of otoliths using laser ablation ICPMS. Can. J. Fish. Aquat. Sci. 52, 1431–1441. doi: 10.1139/f95-138
Geffen, A. J., Nash, R. D. M., and Dickey-Collas, M. (2011). Characterization of herring populations west of the British Isles: an investigation of mixing based on otolith microchemistry. ICES J. Mar. Sci. 68, 1447–1458. doi: 10.1093/icesjms/fsr051
Gillanders, B. M. (2002a). Connectivity between juvenile and adult fish populations: do adults remain near their recruitment estuaries? Mar. Ecol. Prog. Ser. 240, 215–223. doi: 10.3354/meps240215
Gillanders, B. M. (2002b). Temporal and spatial variability in elemental composition of otoliths: implications for determining stock identity and connectivity of populations. Can. J. Fish. Aquat. Sci. 59, 669–679. doi: 10.1139/f02-040
Gillanders, B. M., and Kingsford, M. J. (2003). Spatial variation in elemental composition of otoliths of three species of fish (family Sparidae). Estuar. Coast. Shelf Sci. 57, 1049–1064. doi: 10.1016/S0272-7714(03)00009-X
Hamer, P. A., Jenkins, G. P., and Gillanders, B. M. (2003). Otolith chemistry of juvenile snapper Pagrus auratus in Victorian waters: natural chemical tags and their temporal variation. Mar. Ecol. Prog. Ser. 263, 261–273. doi: 10.3354/meps263261
Heidemann, F., Marohn, L., Hinrichsen, H. H., Huwer, B., Hüssy, K., Klügel, A., et al. (2012). Suitability of otolith microchemistry for stock separation of Baltic cod. Mar. Ecol. Prog. Ser. 465, 217–226. doi: 10.3354/meps09922
Hoff, G. R., and Fuiman, L. A. (1995). Environmentally induced variation in elemental composition of red drum (Sciaenops ocellatus) otoliths. Bull. Mar. Sci. 56, 578–591.
Holliday, F. G. T., and Blaxter, J. H. S. (1960). The effects of salinity on the developing eggs and larvae of the herring. J. Mar. Biol. Assoc. UK 39, 591–603. doi: 10.1017/S0025315400013564
Hoyer, M. V., Shireman, J. V., and Maceina, M. J. (1985). Use of otoliths to determine age and growth of largemouth bass in florida. Trans. Am. Fish. Soc. 114, 307–309. doi: 10.1577/1548-8659(1985)114<307:UOOTDA>2.0.CO;2
ICES (2018). Report of the Herring Assessment Working Group for the Area South of 62°N (HAWG). Copenhagen: ICES HQ, 960.
Incardona, J. P., Vines, C. A., Anulacion, B. F., Baldwin, D. H., Day, H. L., French, B. L., et al. (2012). Unexpectedly high mortality in Pacific herring embryos exposed to the 2007 Cosco Busan oil spill in San Francisco Bay. Proc. Natl. Acad. Sci. U.S.A. 109, E51–E58. doi: 10.1073/pnas.1108884109
Jochum, K. P., Stoll, B., Herwig, K., and Willbold, M. (2007). Validation of LA-ICP-MS trace element analysis of geological glasses using a new solid-state 193 nm Nd:YAG laser and matrix-matched calibration. J. Anal. At. Spectrom. 22, 112–121. doi: 10.1039/B609547J
Jochum, K. P., Weis, U., Stoll, B., Kuzmin, D., Yang, Q., Raczek, I., et al. (2011). Determination of reference values for NIST SRM 610–617 glasses following ISO guidelines. Geostand. Geoanal. Res. 35, 397–429. doi: 10.1111/j.1751-908X.2011.00120.x
Kääriä, J., Eklund, J., Hallikainen, S., Kääriä, R., and Rajasilta, M. (1988). Effects of coastal eutrophication on the spawning grounds of the Baltic herring in the SW Archipelago of Finland. Kieler Meeresforsch. 6, 348–356.
Kafemann, R., Adlerstein, S., and Neukamm, R. (2000). Variation in otolith strontium and calcium ratios as an indicator of life-history strategies of freshwater fish species within a brackish water system. Fish. Res. 46, 313–325. doi: 10.1016/S0165-7836(00)00156-9
Kalish, J. M. (1989). Otolith microchemistry: validation of the effects of physiology, age and environment on otolith composition. J. Exp. Mar. Biol. Ecol. 132, 151–178. doi: 10.1016/0022-0981(89)90126-3
Kanstinger, P., Beher, J., Grenzdörffer, G., Hammer, C., Huebert, K. B., Stepputtis, D., et al. (2016). What is left? Macrophyte meadows and Atlantic herring (Clupea harengus) spawning sites in the greifswalder bodden, Baltic Sea. Estuar. Coast. Shelf. Sci. 201, 72–81. doi: 10.1016/j.ecss.2016.03.004
Kennedy, B. P., Klaue, A., Blum, J. D., Folt, C. L., and Nislow, K. H. (2002). Reconstructing the lives of fish using Sr isotopes in otoliths. Can. J. Fish. Aquat. Sci. 59, 925–929. doi: 10.1139/f02-070
Kotterba, P., Moll, D., Hammer, C., Peck, M. A., Oesterwind, D., and Polte, P. (2017). Predation on Atlantic herring (Clupea harengus) eggs by the resident predator community in coastal transitional waters. Limnol. Oceanogr. 62, 2616–2628. doi: 10.1002/lno.10594
Laine, P., and Rajasilta, M. (1999). The hatching success of Baltic herring eggs and its relation to female condition. J. Exp. Mar. Biol. Ecol. 237, 61–73. doi: 10.1016/S0022-0981(98)00213-5
Leakey, C. D. B., Attrill, M. J., and Fitzsimons, M. F. (2009). Multi-element otolith chemistry of juvenile sole (Solea solea), whiting (Merlangius merlangus) and European seabass (Dicentrarchus labrax) in the Thames estuary and adjacent coastal regions. J. Sea Res. 61, 268–274. doi: 10.1016/j.seares.2008.12.002
Limburg, K. E. (1995). Otolith strontium traces environmental history of subyearling American shad Alosa sapidissima. Mar. Ecol. Prog. Ser. 119, 25–35. doi: 10.3354/meps119025
Lotze, H. K., Lenihan, H. S., Bourque, B. J., Bradbury, R. H., Cooke, R. G., Kay, M. C., et al. (2006). Depletion, degradation, and recovery potential of estuaries and coastal seas. Science 312:1806. doi: 10.1126/science.1128035
Martin, G. B., and Thorrold, S. R. (2005). Temperature and salinity effects on magnesium, manganese, and barium incorporation in otoliths of larval and early juvenile spot Leiostomus xanthurus. Mar. Ecol. Prog. Ser. 293, 223–232. doi: 10.3354/meps293223
McQuinn, I. H. (1997). Metapopulations and the Atlantic herring. Rev. Fish. Biol. Fish. 7, 297–329. doi: 10.1023/A:1018491828875
Messner, U., and von Oertzen, J.-A. (1990). Recent changes in the phytal zone of greifswald bay. Limnologica 20, 183–186.
Milton, D. A., and Chenery, S. R. (2001). Sources and uptake of trace metals in otoliths of juvenile barramundi (Lates calcarifer). J. Exp. Mar. Biol. Ecol. 264, 47–65. doi: 10.1016/S0022-0981(01)00301-X
Milton, D. A., Chenery, S. R., Farmer, M. J., and Blaber, S. J. M. (1997). Identifying the spawning estuaries of the tropical shad, terubok Tenualosa toli, using otolith microchemistry. Mar. Ecol. Prog. Ser. 153, 283–291. doi: 10.3354/meps153283
Moll, D., Kotterba, P., von Nordheim, L., and Polte, P. (2018). Storm-Induced Atlantic herring (Clupea harengus) egg mortality in baltic sea inshore spawning areas. Estuar. Coast. 41, 1–12. doi: 10.1007/s12237-017-0259-5
Möllmann, C., Kornilovs, G., Fetter, M., and Köster, F. W. (2004). Feeding ecology of central Baltic Sea herring and sprat. J. Fish. Biol. 65, 1563–1581. doi: 10.1111/j.0022-1112.2004.00566.x
Nishimura, A., and Yamada, J. (1984). Age and growth of larval and juvenile walleye pollock, Theragra chalcogramma (Pallas), as determined by otolith daily growth increments. J. Exp. Mar. Biol. Ecol. 82, 191–205. doi: 10.1016/0022-0981(84)90104-7
Orth, R. J., Heck, K. L., and van Montfrans, J. (1984). Faunal communities in seagrass beds: a review of the influence of plant structure and prey characteristics on predator-prey relationships. Estuaries 7, 339–350. doi: 10.2307/1351618
Patterson, H. M., Thorrold, S. R., and Shenker, J. M. (1999). Analysis of otolith chemistry in Nassau grouper (Epinephelus striatus) from the Bahamas and Belize using solution-based ICP-MS. Coral Reefs 18, 171–178. doi: 10.1007/s003380050176
Peck, M. A., Kanstinger, P., Holste, L., and Martin, M. (2012). Thermal windows supporting survival of the earliest life stages of Baltic herring (Clupea harengus). ICES J. Mar. Sci. 69, 529–536. doi: 10.1093/icesjms/fss038
Radtke, R. L., Townsend, D. W., Folsom, S. D., and Morrison, M. A. (1990). Strontium:calcium concentration ratios in otoliths of herring larvae as indicators of environmental histories. Environ. Biol. Fishes 27, 51–61. doi: 10.1007/bf00004904
Rahikainen, M., Hoviniemi, K.-M., Mäntyniemi, S., Vanhatalo, J., Helle, I., Lehtiniemi, M., et al. (2017). Impacts of eutrophication and oil spills on the Gulf of Finland herring stock. Can. J. Fish. Aquat. Sci. 74, 1218–1232. doi: 10.1139/cjfas-2016-0108
Rooker, J. R., Secor, D. H., Zdanowicz, V. S., De Metrio, G., and Relini, L. O. (2003). Identification of Atlantic bluefin tuna (Thunnus thynnus) stocks from putative nurseries using otolith chemistry. Fish. Oceanogr. 12, 75–84. doi: 10.1046/j.1365-2419.2003.00223.x
Rooker, J. R., Secor, D. H., Zdanowicz, V. S., and Itoh, T. (2001). Discrimination of northern bluefin tuna from nursery areas in the Pacific Ocean using otolith chemistry. Mar. Ecol. Prog. Ser. 218, 275–282. doi: 10.3354/meps218275
Ruiz, F. (2001). Trace metals in estuarine sediments from the Southwestern Spanish coast. Mar. Pollut. Bull. 42, 481–489. doi: 10.1016/S0025-326X(00)00192-2
Schoener, T. W. (1974). Resource partitioning in ecological communities. Science 185:27. doi: 10.1126/science.185.4145.27
Shulman, M. J. (1985). Recruitment of coral reef fishes: effects of distribution of predators and shelter. Ecology 66, 1056–1066. doi: 10.2307/1940565
Swearer, S. E., Forrester, G. E., Steele, M. A., Brooks, A. J., and Lea, D. W. (2003). Spatio-temporal and interspecific variation in otolith trace-elemental fingerprints in a temperate estuarine fish assemblage. Estuar. Coast. Shelf Sci. 56, 1111–1123. doi: 10.1016/S0272-7714(02)00317-7
Thorisson, K., Jónsdóttir, I. G., Marteinsdottir, G., and Campana, S. E. (2011). The use of otolith chemistry to determine the juvenile source of spawning cod in Icelandic waters. ICES J. Mar. Sci. 68, 98–106. doi: 10.1093/icesjms/fsq133
Thorrold, S. R., Jones, C. M., Swart, P. K., and Targett, T. E. (1998). Accurate classification of juvenile weakfish Cynoscion regalis to estuarine nursery areas based on chemical signatures in otoliths. Mar. Ecol. Prog. Ser. 173, 253–265. doi: 10.3354/meps173253
Thorrold, S. R., Latkoczy, C., Swart, P. K., and Jones, C. M. (2001). Natal homing in a marine fish metapopulation. Science 291, 297–299. doi: 10.1126/science.291.5502.297
Townsend, D. W., Radtke, R. L., Corwin, S., and Libby, D. A. (1992). Strontium:calcium ratios in juvenile Atlantic herring Clupea harengus L. otoliths as a function of water temperature. J. Exp. Mar. Biol. Ecol. 160, 131–140. doi: 10.1016/0022-0981(92)90115-Q
Tracey, S. R., Lyle, J. M., and Duhamel, G. (2006). Application of elliptical Fourier analysis of otolith form as a tool for stock identification. Fish. Res. 77, 138–147. doi: 10.1016/j.fishres.2005.10.013
Tzeng, W. N., Severin, K. P., and Wickström, H. (1997). Use of otolith microchemistry to investigate the environmental history of European eel Anguilla anguilla. Mar. Ecol. Prog. Ser. 149, 73–81. doi: 10.3354/meps149073
Tzeng, W. N., and Tsai, Y. C. (1994). Changes in otolith microchemistry of the Japanese eel, Anguilla japonica, during its migration from the ocean to the rivers of Taiwan. J. Fish. Biol. 45, 671–683. doi: 10.1111/j.1095-8649.1994.tb00933.x
Vasconcelos, R. P., Reis-Santos, P., Tanner, S., Maia, A., Latkoczy, C., Günther, D., et al. (2008). Evidence of estuarine nursery origin of five coastal fish species along the Portuguese coast through otolith elemental fingerprints. Estuar. Coast. Shelf Sci. 79, 317–327. doi: 10.1016/j.ecss.2008.04.006
von Nordheim, L., Kotterba, P., Moll, D., and Polte, P. (2018). Impact of spawning substrate complexity on egg survival of Atlantic herring (Clupea harengus, L.) in the Baltic sea. Estuar. Coast. 41, 549–559. doi: 10.1007/s12237-017-0283-5
Waycott, M., Duarte, C. M., Carruthers, T. J. B., Orth, R. J., Dennison, W. C., Olyarnik, S., et al. (2009). Accelerating loss of seagrasses across the globe threatens coastal ecosystems. Proc. Natl. Acad. Sci. U.S.A. 106:12377. doi: 10.1073/pnas.0905620106
Welch, B. L. (1938). The significance of the difference between two means when the population variances are unequal. Biometrika 29, 350–362. doi: 10.2307/2332010
Wheeler, J. P., and Winters, G. H. (1984). Homing of Atlantic herring (Clupea harengus harengus) in Newfoundland waters as indicated by tagging data. Can. J. Fish. Aquat. Sci. 41, 108–117. doi: 10.1139/f84-010
Woodcock, S. H., Munro, A. R., Crook, D. A., and Gillanders, B. M. (2012). Incorporation of magnesium into fish otoliths: determining contribution from water and diet. Geochim. Cosmochim. Acta 94, 12–21. doi: 10.1016/j.gca.2012.07.003
Worm, B., Barbier, E. B., Beaumont, N., Duffy, J. E., Folke, C., Halpern, B. S., et al. (2006). Impacts of biodiversity loss on ocean ecosystem services. Science 314, 787–790. doi: 10.1126/science.1132294
Keywords: Clupea harengus, Western Baltic Sea, inshore spawning areas, age-0 herring, otolith chemistry, elemental fingerprinting, LA-ICP-MS
Citation: Moll D, Kotterba P, Jochum KP, von Nordheim L and Polte P (2019) Elemental Inventory in Fish Otoliths Reflects Natal Origin of Atlantic Herring (Clupea harengus) From Baltic Sea Juvenile Areas. Front. Mar. Sci. 6:191. doi: 10.3389/fmars.2019.00191
Received: 21 November 2018; Accepted: 26 March 2019;
Published: 11 April 2019.
Edited by:
Mario Barletta, Universidade Federal de Pernambuco (UFPE), BrazilReviewed by:
Brian R. MacKenzie, Technical University of Denmark, DenmarkJosé Lino Vieira De Oliveira Costa, Universidade de Lisboa, Portugal
Copyright © 2019 Moll, Kotterba, Jochum, von Nordheim and Polte. This is an open-access article distributed under the terms of the Creative Commons Attribution License (CC BY). The use, distribution or reproduction in other forums is permitted, provided the original author(s) and the copyright owner(s) are credited and that the original publication in this journal is cited, in accordance with accepted academic practice. No use, distribution or reproduction is permitted which does not comply with these terms.
*Correspondence: Dorothee Moll, ZG9yb3RoZWUubW9sbEB0aHVlbmVuLmRl