- 1Changing Oceans Lab, Grant Institute, School of GeoSciences, University of Edinburgh, Edinburgh, United Kingdom
- 2Institute of Life Science, School of Energy, Geoscience, Infrastructure and Society, Heriot-Watt University, Edinburgh, United Kingdom
- 3Marine Scotland, Marine Laboratory, Aberdeen, United Kingdom
Discovery and understanding of fragile deep-sea habitats like sponge aggregations, are being outpaced by anthropogenic resource exploitation. Sustainable ocean development in the Faroe-Shetland Channel Nature Conservation Marine Protected Area (FSC NCMPA; northeast Atlantic), which harbors sponge aggregations, now requires adaptive management in the face of encroachment of multisectorial activities in this area (e.g., fishing, oil and gas, shipping) and climate change. We examined sponge morphotype composition, richness, diversity, density and body-size distribution inside and outside the FSC NCMPA, and the role of environmental variability and human impact in these sponge aggregations. Analyses were based on the examination of 465 high resolution images from 13 towed-camera transects. A catalog for regional sponge morphotypes was also developed and applied for these analyses. Analysis revealed that morphotype composition did not differ between inside and outside the FSC NCMPA but richness, diversity and densities of massive/spherical/papillate and flabellate/caliculate sponges were higher inside than outside the boundary. The sponge aggregations occurred within a narrow zone between 450 and 530 m depth, within relatively warm and saline water masses. Furthermore, multiple size cohorts of sponges were recorded inside the FSC NCMPA, in contrast to the single cohort outside. Distance-based linear modeling showed that demersal fisheries, substratum, salinity and temperature explained a statistically-significant amount of variation (48%, p < 0.001) of sponge density across the study area. Findings on density and size cohorts suggest that the FSC NCMPA boundary currently encloses the most vulnerable area, which also demonstrates normal ecosystem functions (e.g., recruitment). However, sponges were constrained to a narrow environmental niche shaped by fisheries and interactions of FSC NCMPA water masses with the slope that in turn likely determine, food supply to the sponge aggregations. Our study illustrated the vulnerability of the FSC NCMPA sponge aggregations to fisheries and changes to water mass properties over time. The morphotype catalog and suite of indicators (i.e., density and body-size distribution) allow for baseline and future assessments of anthropogenic and climate change impacts on sponge aggregations’ environmental status in the FSC NCMPA, thus guiding management as sectoral encroachment continues in this area.
Introduction
Sponges (Phylum Porifera) are widespread benthic organisms being found from the intertidal zone down to the abyss (Maldonado et al., 2017). Studies on shallow-water ecosystems have revealed the important role of sponges in habitat supply and nutrient cycling (e.g., Bell, 2008 and references therein). On the contrary, available information about sponges in the deep sea (i.e., generally areas below 200 m water depth), even for basic aspects like sponges’ biogeographic distribution, biology and ecological roles, is very limited (Klitgaard and Tendal, 2004; Cathalot et al., 2015; Kazanidis et al., 2016, 2018).
Technological developments like the use of towed cameras, remotely operated vehicles (ROVs) and autonomous underwater vehicles (AUVs) can help us map the distribution of deep-sea sponges and the ecosystems they form i.e., deep-sea sponge grounds (Hogg et al., 2010). However, in order to maximize, the impact of these video/image-based methods, there is an urgent need to develop and establish common protocols and procedures (Henry and Roberts, 2014a,b). Method standardization (e.g., units used in reporting faunal density) will enable the comparability of findings among studies and will enable the identification of gaps, challenges, strengths, and opportunities for the exploration of sponge grounds and deep-sea ecosystems, in overall. Another important step for advancing our understanding on deep-sea sponge grounds is to unravel the environmental parameters that shape their distribution, which in turn will facilitate the development of efficient conservation strategies (Howell et al., 2016; Johnson et al., 2018). This is of uttermost importance as deep-sea sponges are slow-growing organisms which makes them particularly vulnerable to human activities such as bottom trawling (Kenchington et al., 2014; Pusceddu et al., 2014; Victorero et al., 2018) and oil and gas activities (Vad et al., 2018). Indeed, deep-sea sponge grounds fulfill the Food and Agriculture Organization of the United Nations (FAO) definition of Vulnerable Marine Ecosystems (VMEs) and have also been included in the Oslo/Paris Convention for the Protection of the Marine Environment of the North-East Atlantic (OSPAR) List of threatened and/or declining species and habitats in the northeast Atlantic (OSPAR, 2008). Existing studies (both modeling and observations) about the role of water mass properties on the environmental parameters shaping the distribution of deep-sea sponge grounds suggest important roles of hydrography and temperature (Rice et al., 1990; Bett, 2001; Murillo et al., 2012), ocean chemistry (e.g., salinity and silicate) (Beazley et al., 2015; Howell et al., 2016), and biological parameters such as the concentration of particulate organic carbon (Barthel et al., 1996; Howell et al., 2016).
The Faroe-Shetland Chanel (FSC, hereafter) in the northeast Atlantic hosts complex hydrographic and sedimentary features (Bett, 2003). Multisectoral collaborations between industry, government and academia have allowed detailed investigations of how the ecology of deep-sea benthic communities is shaped by the hydrography and sedimentary regimes in FSC (Bett, 2001; Henry and Roberts, 2004; Jones et al., 2006; Narayanaswamy et al., 2010), including the discovery of sponge aggregations within a narrow bathymetric zone (Bett, 2001; Axelsson, 2003). The area hosting these deep-sea sponge aggregations was designated in 2014 as the “Faroe-Shetland Channel Nature Conservation Marine Protected Area” (FSC NCMPA hereafter)1. Mixed sponge assemblages are recorded there including boreal “ostur” communities (Klitgaard and Tendal, 2004) a mixture of geodiid sponges such as Geodia barretti, G. macandrewi, G. atlantica, G. phlegraei (reported as Isops phlegraei) and several other flabellate, lobose, stipitate and encrusting species (Bett, 2001; Howell et al., 2010; Henry and Roberts, 2014a2). Sponge density ranged from 0.001 to 0.818 sponges/m2 (Axelsson, 2003; Henry and Roberts, 2014a). More detailed information on density, bathymetric distribution and body size of different species is currently missing. Furthermore, how anthropogenic activities and environmental parameters shape the distribution of FSC NCMPA sponge aggregations is also unknown. This is an important knowledge gap because sponges in FSC NCMPA provide habitat for Munida crabs, the ophiuroid Ophiactis balli and sessile polychaetes (Howell et al., 2010) and also because of oil and gas, shipping and telecommunications3 encroachment in the region. Oil exploration and production activities take place in the FSC since early 1990s (Vad et al., 2018). Investigations on the physical disturbance caused from drilling on the megafaunal communities in FSC have shown smothering of the seafloor in a radius of 50–120 m around the drilling site (Jones et al., 2006). This disturbance caused significant reduction in abundance, diversity and species richness of megafauna (e.g., sponges, cnidarians, molluscs) (Jones et al., 2006, 2007, 2012). Furthermore, studies on FSC have shown that a part of the fishing activity (8% of total time) is carried out sub-zero temperatures; however, best catches of Greenland halibut (Reinhardtius hippoglossoides) and Roughhead grenadier (Macrourus berglax) and most of the fishing activity were recorded in boundary waters (0–4°C) (Bullough et al., 1998). In addition, surveys in the area have revealed disturbance in sediments and sponges, assumed to result from trawling (Bett, 2000). Currently, there are no FSC NCMPA specific management measures in place (e.g., for fisheries) and the statutory agency Marine Scotland is in the process of developing a joint recommendation to implement measures under the Common Fisheries Policy (Marine Scotland, 2017)4.
Hátún et al. (2017) have shown a decline over the last 25 years in silicate concentrations in North Atlantic including the FSC NCMPA. Changes in the temperature and salinity profiles of FSC NCMPA water masses over the last two decades, have also been shown (Turrell et al., 1999; Sherwin et al., 2006; Berx et al., 2013; Broadbridge and Toumi, 2015; McKenna et al., 2016). Reduction in concentration of silicate can have detrimental effects on deep-sea sponges as silicate concentrations shape primary production (and thus food-supply) and also silicate is an integral compound of sponges’ skeleton (Maldonado et al., 2011).
Deep-sea sponge aggregations of the FSC NCMPA are impacted by both human activities and climate change. Advancing, thus, our knowledge is essential for evaluating the existing FSC NCMPA boundary and for the development of conservation strategies for these VMEs (Johnson et al., 2018).
In the present study we have analyzed video/image material from inside and outside the FSC NCMPA to address three fundamental questions:
(1) Which sponge morphotypes are encountered inside and outside the NCMPA?
(2) How does sponge morphotype (a) richness and diversity, (b) density and (c) body-size distribution differ between inside and outside the FSC NCMPA?
(3) Which is the role of environmental variability and human activities in controlling the distribution and density of sponge morphotypes?
Materials and Methods
Faroe-Shetland Channel Hydrography and Habitat Features
The Faroe-Shetland Channel (FSC) is characterized by complex hydrography as five water masses flow through it: North Atlantic Water (NAW), Modified North Atlantic Water (MNAW), Modified East Icelandic Water (MEIW), Norwegian Sea Arctic Intermediate Water (NSAIW), and Norwegian Sea Deep Water (NSDW) (Hansen and Østerhus, 2000). The distribution of these five water masses in the FSC is indicated by their characteristic temperature and salinity values which are accompanied by a specific spectrum of chemical and biological characteristics (see Table 1 in McKenna et al., 2016 for details). In the FSC, water masses above 400 m water depth are composed from NAW (9.5–10.5°C; 35.35–35.45 psu) or MNAW (7–8.5°C; 35.10–35.30 psu). NAW flows along the Shetland Shelf Edge while MNAW enters the FSC from the north of the Faroe Islands and recirculates within it. At greater depths (∼400–800 m) water masses are composed from MEIW (2–4.5°C; 34.76–34.99 psu) and NSAIW (-0.5–0.5°C; 34.89–34.91 psu). Bottom water mass NSDW (<-0.5°C; 34.91–34.92 psu) which has the greatest contribution in the southwards overflow through the Faroe Bank Channel and across the Wyville Thomson Ridge (Berx, 2012; Broadbridge and Toumi, 2015; McKenna et al., 2016). The boundary area occurring between the warm and cold-waters (found between ∼350–650 m) is particularly interesting as within it (∼550 m) rapid changes in seawater temperature (decrease up to 7°C within 1 h) have been recorded (Bett, 2001). These changes in seawater temperature are thought to be created by internal waves created by water flow across the Wyville Thomson Ridge. These internal waves propagate in the FSC at the boundary between warm and cold-water masses (Sherwin, 1991). Apart from the unusual temperature profile, the FSC is also interesting in terms of sedimentary habitat types that include areas of iceberg ploughmarks and North Sea Fan among others (Masson, 2001 for details; see also Bett, 2003; Narayanaswamy et al., 2010).
Visual Survey of Sponge Aggregations
In September 2014, a visual survey was conducted by Marine Scotland on board the MRV Scotia (“MoreDeep” cruise) to investigate the deep-sea sponge aggregations inside and outside the FSC NCMPA. The visual survey was conducted using a Seatronics5 towed body hosting a Kongsberg Maritime OE14-366 PAL color zoom camera6. The system was also equipped with six high-intensity lights and sensors that measured at 1 s intervals, the altitude (the distance between the camera system and the seabed), depth (±0.1 m), and pitch and roll of the system (see McIntyre et al., 2013, 2016 for details). Seawater temperature was recorded (±0.01°C) through the sensor attached to the camera system. In total, 13 camera transects were conducted, which were grouped into one area inside (i.e., Area 2) and three areas (i.e., Area 1, Area 3, and Area 4) outside the FSC NCMPA (Figure 1). The average duration of each transect was ∼1 h 21 min at a speed of 1.5 m/sec. The altitude was 2–6 m. Because a camera positioning system was not available, positions recorded are those of the ship. Starting-ending points and depth range of the camera transects as well as values of temperature are given in Table 1. Sponge samples during the transects were not collected.
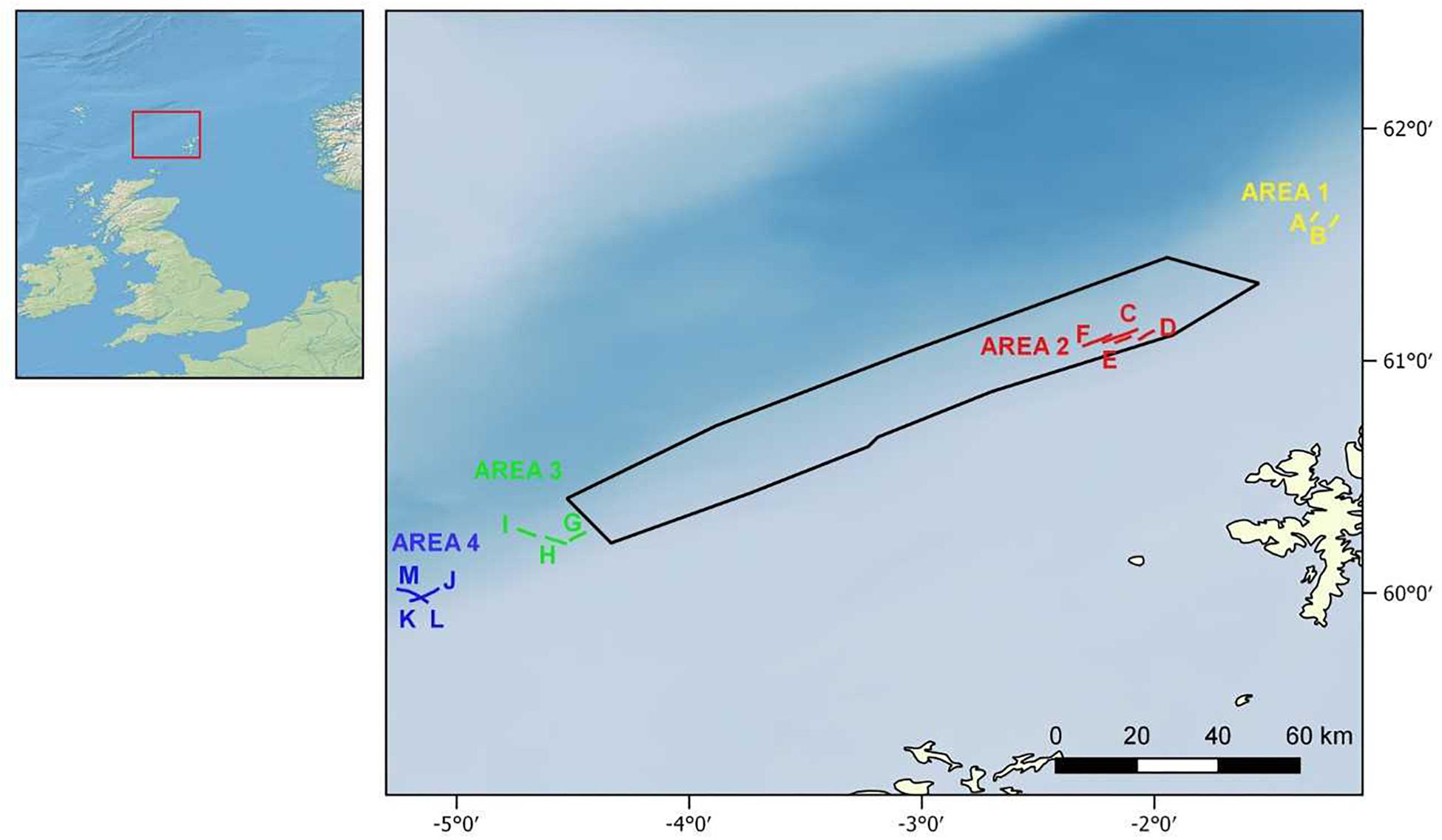
Figure 1. Wider study area (Left) and camera transects inside (Area 2: transects C–F) and outside the Faroe-Shetland Channel Nature Conservation Marine Protected Area (FSC NCMPA) (Area 1: transects A,B; Area 3: transects G–I; Area 4: transects J–M) (Right). See also Table 1.
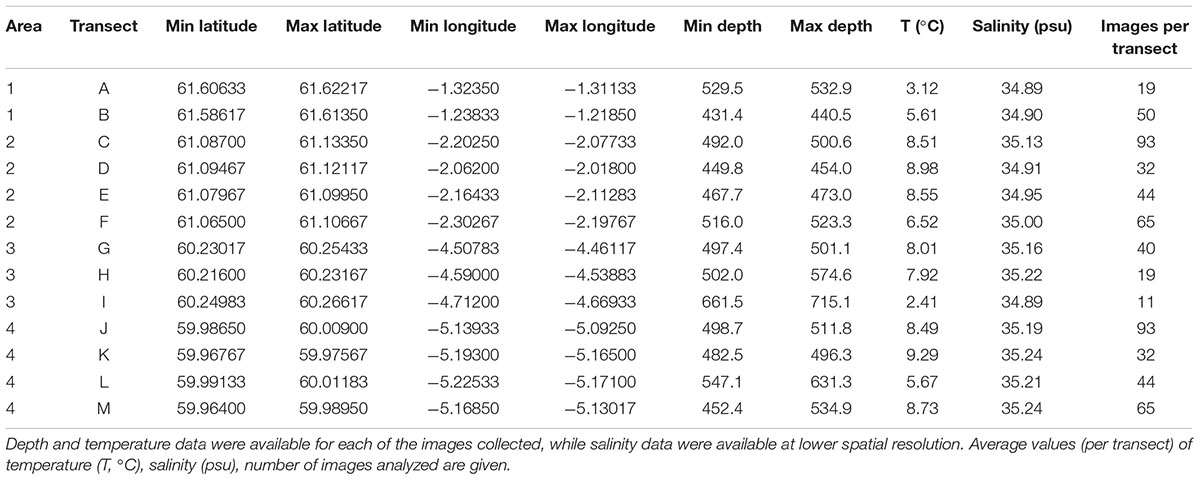
Table 1. Coordinates and depth values for each of the transects inside (Area 2) and outside (Area 1, Area 3, Area 4) the Faroe-Shetland Channel Nature Conservation Marine Protected Area (FSC NCMPA).
Image Analysis
The camera recorded continuous high definition (HD) video from which individual image frames were extracted every 20 s. Extraction was carried out with freely available software VLC. The frames were subjected to quality check with low-visibility frames been discarded. The resolution in images was 6544 × 3680 pixels in dimension with a 350 pixel/inch DPI. In total, 465 high quality image frames were subjected to further analysis (see below).
Sponge Morphotype Categories
Sponge morphotype categories were mainly based on the “Thesaurus of Sponge Morphology” (Boury-Esnault and Rützler, 1997) accompanied by studies on the distribution and structure of deep-sea sponge aggregations in the North Atlantic (Bett, 2001; Axelsson, 2003; Klitgaard and Tendal, 2004; Ackers et al., 2007; Cárdenas et al., 2013; Henry and Roberts, 2014a; McIntyre et al., 2016). The construction of the sponge morphotype catalog was also based on the examination of images collected by ROVs over several years in the wider FSC area. Five sponge morphotype categories were used (Table 2). Each sponge specimen recorded in the high-quality image frames of the present study was grouped in one of the five morphotype categories, and counted. Sponge morphotype categories were based on the “Thesaurus of Sponge Morphology” (Boury-Esnault and Rützler, 1997). Sponges were grouped in the five following categories: Category 1: encrusting (Figure 2A); Category 2: arborescent (“tree-like”) (Figure 2A); Category 3: massive, spherical and papillate (Figures 2B,C,G); Category 4: flabellate (“fan-shaped”) and caliculate (“cup-shaped”) (Figures 2F,G); Category 5: stipitate (“stalked”) and clavate (“club-shaped”) (Figures 2D,E).
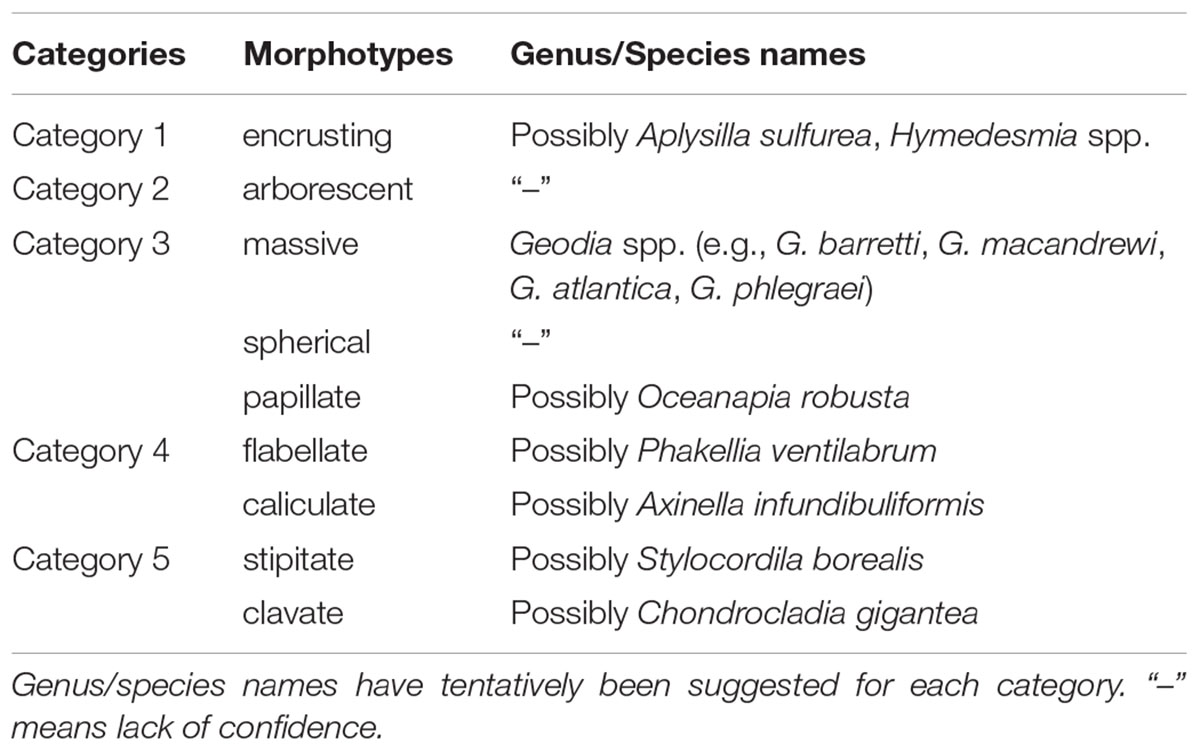
Table 2. Categories of sponge morphotypes in the Faroe Shetland Channel Nature Conservation Marine Protected Area (FSC NCMPA).
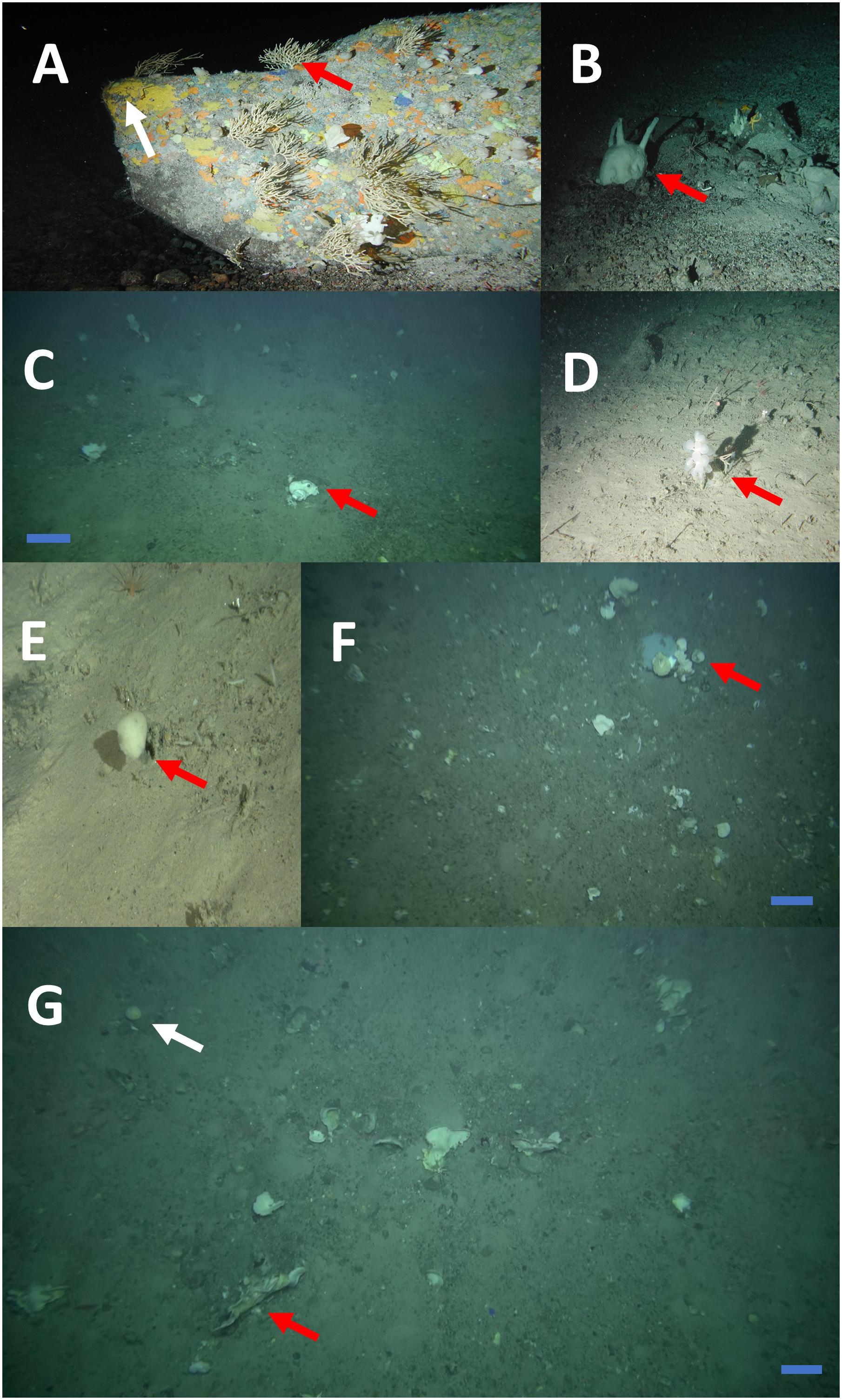
Figure 2. Categories of sponge morphotypes from the Faroe-Shetland Channel Nature Conservation Marine Protected Area (FSC NCMPA). Category 1: encrusting (A, white arrow), Category 2: arborescent (“tree-like”) (A, red arrow), Category 3: massive (C), spherical (G, white arrow) and papillate (B). Category 4: flabellate (“fan-shaped”) (G, red arrow) and caliculate (“cup-shaped”) (F). Category 5: stipitate (“stalked”) (E) and clavate (“club-shaped”) (D). All scale bars: 30 cm. See also Table 2.
Density and Body Size of Sponge Morphotypes
The surface of the seabed seen in each of the HD image frames (field of view) was quantified (in m2) by matching the time point that a frame was captured with the relevant data (altitude and pitch angle of the towed camera system) provided by the sensor attached to the system. A detailed description of the methodology followed for measuring the field of view is given in McIntyre et al. (2013). In brief, altitude and pitch of the towed body were used in the survey area calculations using trigonometric equations. To correct for the effect of the distance on the visibility of the seabed, image analysis techniques in MATLAB7 Image Processing Toolbox were used to construct a correction factor. The latter was a proportion of visible seabed in each image(see McIntyre et al., 2013 for details).
Using the number of sponge specimens from each morphotype Category recorded in each image and the surface of the seabed, values of sponge density were measured (sponge individuals per Category/m2). These measurements of sponge morphotype density were carried out for categories 2, 3, 4, and 5 while specimens from sponge morphotype Category 1 (“encrusting”) were logged in terms of presence/absence. Furthermore, using the camera’s laser points as a reference (7.5 cm), we measured the width of flabellate sponges and the average value between “length” and “width” for the massive/spherical/papillate and the caliculate sponges (Figure 3). Body size measurements were grouped into 5 cm size classes following Bo et al. (2012) Measurements on body size were mainly carried out for transects A, B, C, D, E, F; unfortunately the position of the camera did not allow appropriate measurements to be taken in transects G, H, I, J, K, L, and M (Figure 1).
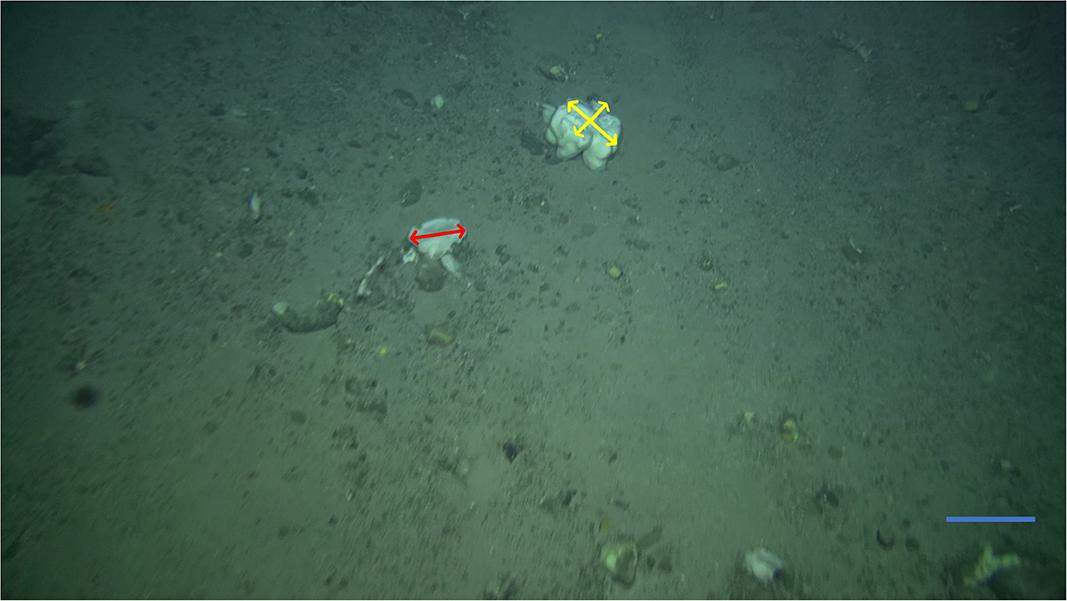
Figure 3. Measurements carried out on the width of a flabellate (“fan-shaped” sponges, pointed with the red arrows) and “length” and “width” in a massive sponge (pointed with the yellow arrows). Scale bar: 30 cm.
Fisheries and Environmental Data
The distribution of sponge aggregations inside and outside the FSC NCMPA was investigated in relation to water depth (m), type of substratum, temperature (°C), salinity (psu), water density (g/ml), oxygen saturation and demersal fisheries. Data on depth, type of substratum and temperature were available for each of the images collected during the visual survey of the present study. The type of the substratum in each of the 465 high quality image frames was grouped in one of the four categories (following Bett, 2003): (1) cobble (Figure 4A), (2) cobble with boulder (Figure 4B), (3) sand (Figure 4C), and (4) sand with boulder (Figure 4D). Data on salinity, water density and oxygen saturation (derived from equivalent temperature and salinity using a MATLAB routine8) from the FSC were supplied from Marine Scotland, at a coarser spatial scale. These data came from locations inside the transects of the visual survey (Table 1) at similar depths.
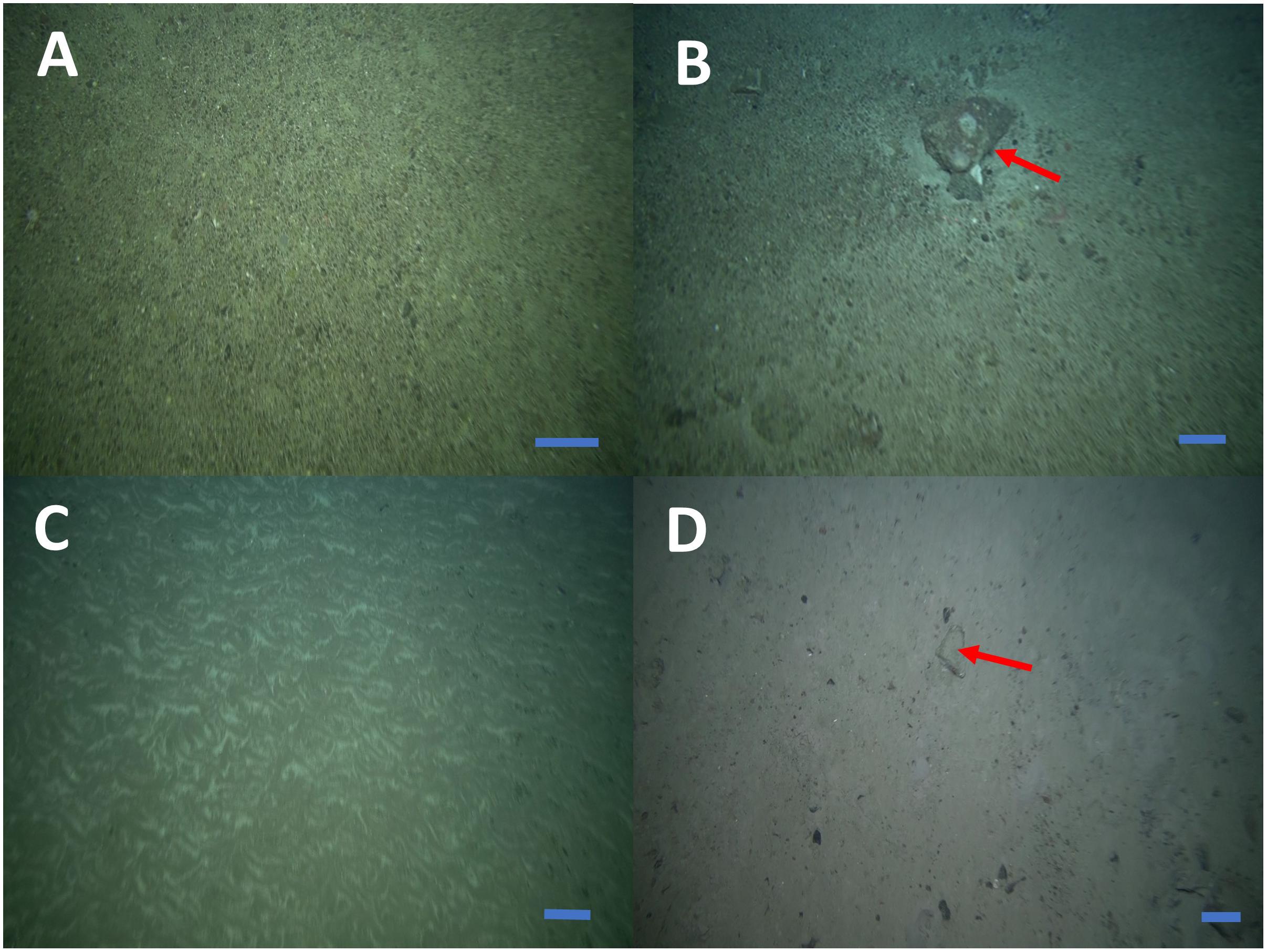
Figure 4. Categories of substrate types inside and outside the Faroe-Shetland Channel Nature Conservation Marine Protected Area FSC NCMPA. Cobble (A), cobble with boulder (B), sand (C), and sand with boulder (D). Scale bars: 30 cm. Red arrows show boulders.
Data on demersal fishing activity for Area 1, Area 2, Area 3, and Area 4, were extracted with qGIS9 from the Marine Scotland MAPS NMPI (National Marine Plan Interactive) website using the layer entitled “Fishing statistics – Demersal landings (tons) from Scottish waters [per International Council for the Exploration of the Sea (ICES) statistical rectangle]”10. Data for this layer were available only for 5 years (2012–2016) enabling thus an assessment of recent demersal fishing impact on the sponge communities. For each of the ICES rectangles taken into account (i.e., rectangle 52E8 for Area 1; 51E7 for Area 2; 49E5 for Area 3; 48E4 for Area 4) an average value was calculated over the time period 2012–2016. The average value for the annual demersal landings were: 113 tons in Area 1, 64 tons in Area 2, 2836 tons in Area 3, and 3011 tons in Area 4.
Statistical Analysis
The number of sponge morphotypes (S) and Shannon’s diversity index (H) were calculated using Primer-7 (Clarke et al., 2014) and these indices were compared between inside and outside the FSC NCMPA. Furthermore, the density of sponges was compared (1) inside versus outside the FSC NCMPA, (2) across the four areas (i.e., Area 1, Area 2, Area 3, and Area 4), and (3) across all transects. Sponge size was compared inside versus outside the FSC NCMPA. These comparisons were carried out in the software R (R Core Team, 2018). The Shapiro-Wilk test was applied to test for normality, followed by the Bartlett-test or the F-test to verify the equality of variances (for two or three groups, respectively). If the distributions were normal and the variances were equal, then the hypothesis that the groups have the same mean was tested using the two-sample t-test or the one-way ANOVA (for two or three groups, respectively). If distributions were normal but variances were not equal, the hypothesis that the means of the groups were the same was examined through the Welch’s two sample t-test or one-way analysis of means (not assuming equal variances) (for two groups or three groups, respectively). If distributions were not normal, the hypothesis that the groups have the same median was tested through a Wilcoxon rank sum test or a Kruskal-Wallis rank sum test (for two or three groups, respectively). All values in the text are presented as mean ± standard error (SE).
The size-frequency distributions were analyzed applying the Bhattacharya analysis using the FISAT software package (Gayanilo and Pauly, 199711). Size-frequency distributions were compared between inside and outside the FSC NCMPA; comparisons were carried out for morphotype Categories 3, 4 and for all morphotypes.
The grouping of the transects was analyzed in Primer-7. Specifically, non-transformed data on the density (individuals/m2) of each sponge morphotype were used in the calculation of Bray–Curtis similarities and similarity matrices. Based on these matrices, clustering analysis was carried out. Finally, the role of environmental parameters and demersal fisheries in shaping the density of sponge morphotypes was analyzed through distance-based linear modeling (“Dist-LM” routine) in Primer 7.
Results
Environmental Data
Preliminary investigation of environmental parameters (i.e., Draftsman Plots in Primer-7 software; see below for details) showed high correlations between salinity-density and between salinity-oxygen saturation and thus density and oxygen saturation data were not used further (see below for details).Average values of temperature ranged from 2.41°C (transect I) to 9.29°C (transect K) and average salinity ranged from 34.89 psu (A, I transects) to 35.24 psu (transects K, M) (Table 1). Hard substrata (i.e., cobble, cobble with boulder) dominated most transects while soft sediments were the major type of substrate only in J and K transects (Figure 5).
Biological Data
Sponge Morphotypes Encountered Inside and Outside the FSC NCMPA
In the present study we recorded only three categories (Category 1, Category 3, and Category 4 in Table 2) out five previously recorded in FSC; there were no records of Category 2 or Category 5 (Figures 2, 6 and Table 2). Category 1 sponges were recorded in all transects except from transect I. Sponge morphotypes from Categories 1, 3, and 4 were found both inside and outside the FSC NCMPA (Figure 6). In Category 3, >90% of sponge specimens recorded were massive; similarly, in Category 4, >90% of sponges were flabellate. The number of sponge morphotypes (S) was significantly higher inside than outside the FSC NCMPA. Specifically, the number of sponge morphotypes (S) inside the FSC NCMPA was 1.2 ± 0.0 sponge morphotypes vs. 0.1 ± 0.0 sponge morphotypes outside the FSC NCMPA (Wilcoxon rank sum test = 46716, p < 0.0001; n = 465). Similarly, (H) was significantly higher inside than outside the FSC NCMPA. (H) inside the FSC NCMPA was 0.22 ± 0.01 while (H) outside the FSC NCMPA was 0.01 ± 0.00 (Wilcoxon rank sum test = 36628, p < 0.0001, n = 465).
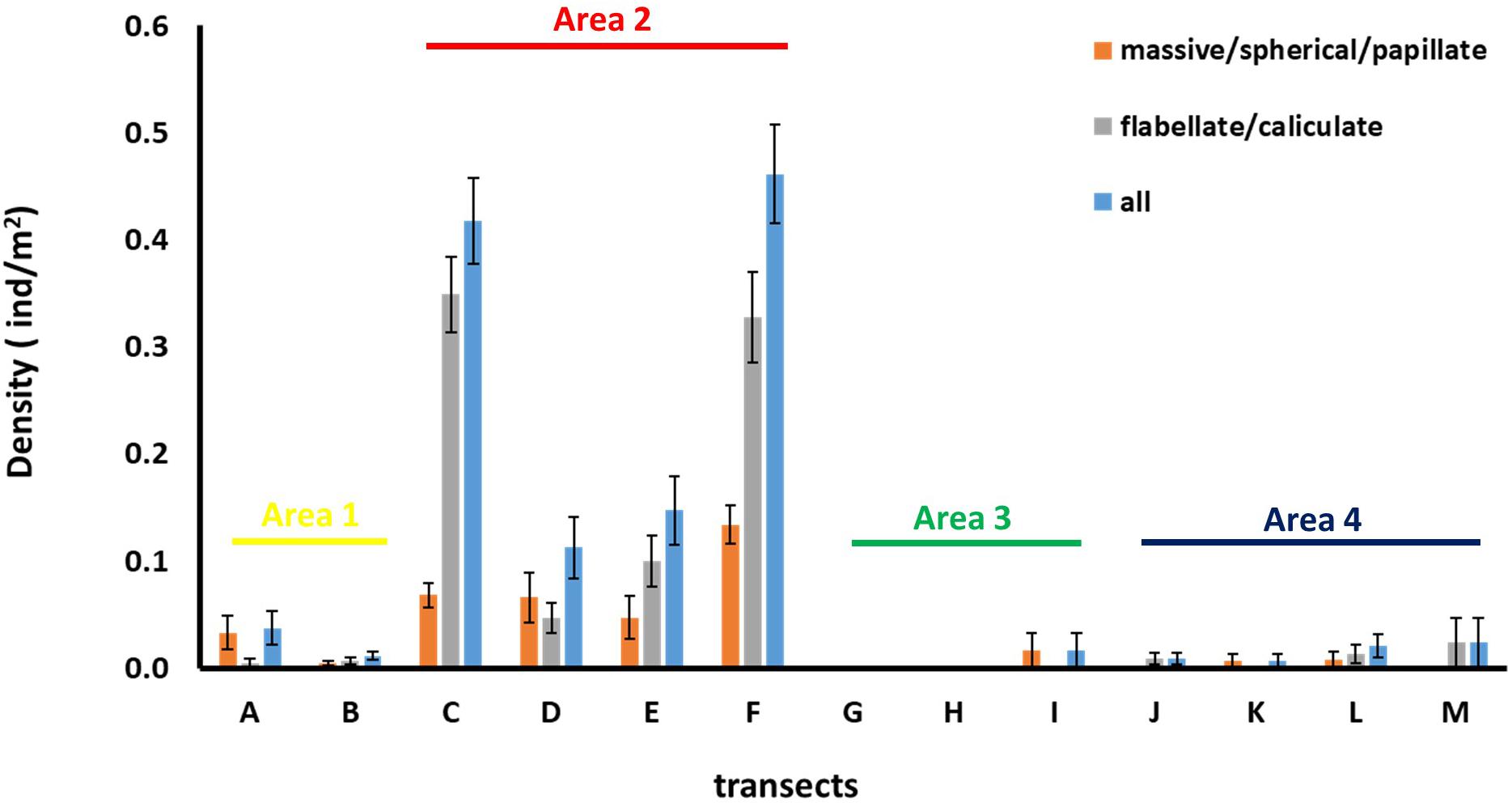
Figure 6. Average ± standard error values of sponge density for each of the sponge morphotype categories across the thirteen transects.
Density of Sponge Morphotypes Inside and Outside the FSC NCMPA
Density of all sponge morphotypes was significantly higher inside than outside the FSC NCMPA (Table 3). Significant differences were also detected across the four areas (i.e., Area 1, Area 2, Area 3, and Area 4); higher values were recorded in Area 2 (Table 3 and Figure 6). Clustering analysis using density values for each sponge morphotype revealed a higher level of similarity (∼50%) for transects in Area 2 (i.e., inside the FSC NCMPA) than transects in Area 1, Area 3, and Area 4 (i.e., outside the FSC NCMPA) (Figure 7). Finally, significant differences in density were recorded across all transects (Table 3 and Figure 6). The highest density for Category 3 was found in transect F, for Category 4 in transect C and for all sponge morphotypes in transect F (Table 3 and Figure 6).
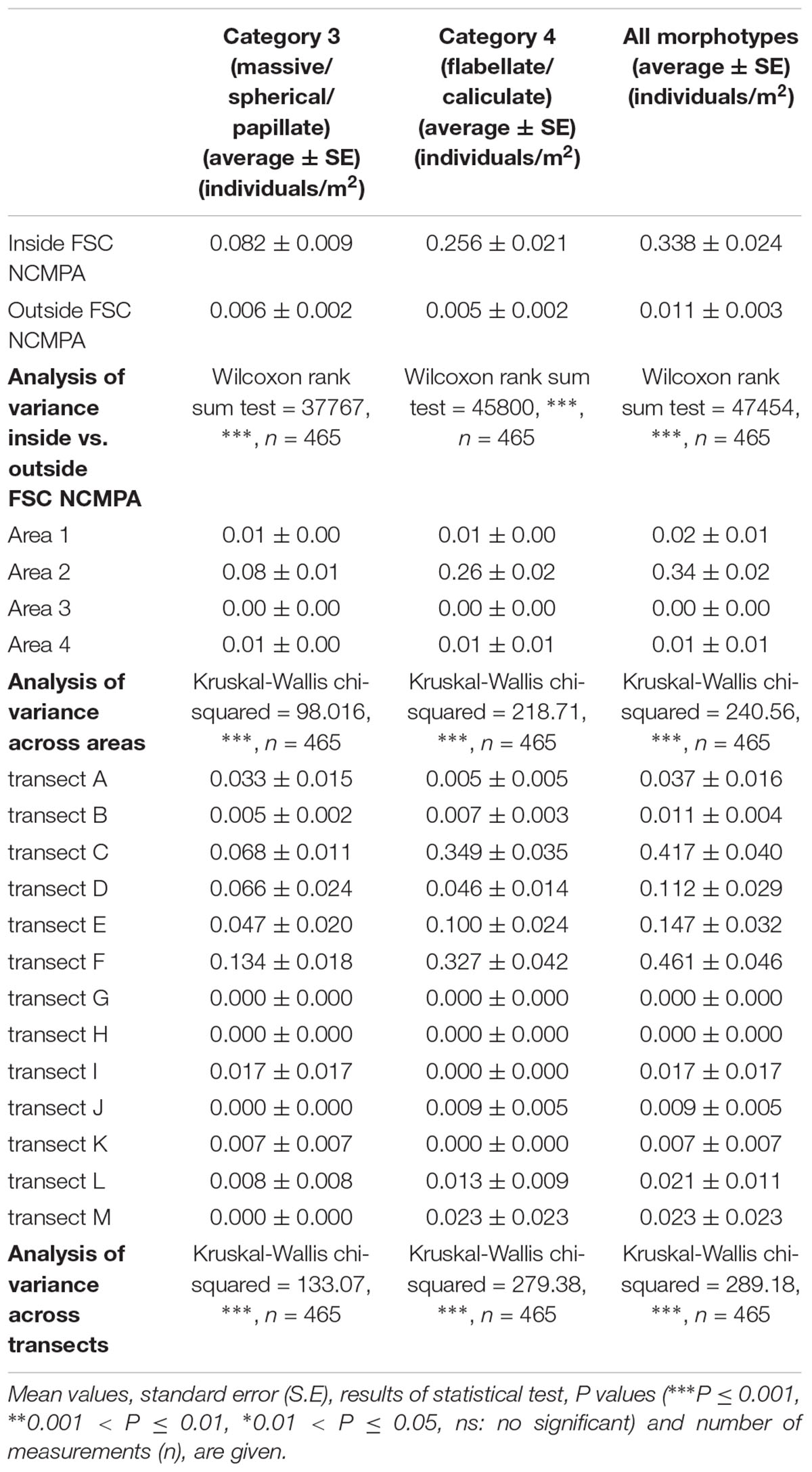
Table 3. Density of Category 3 (massive/spherical/papillate) and Category 4 (flabellate/caliculate) sponges inside and outside the Faroe-Shetland Channel Nature Conservation Marine Protected Area (FSC NCMPA), across areas (Area 1, Area 2, Area 3, and Area 4) and across transects.
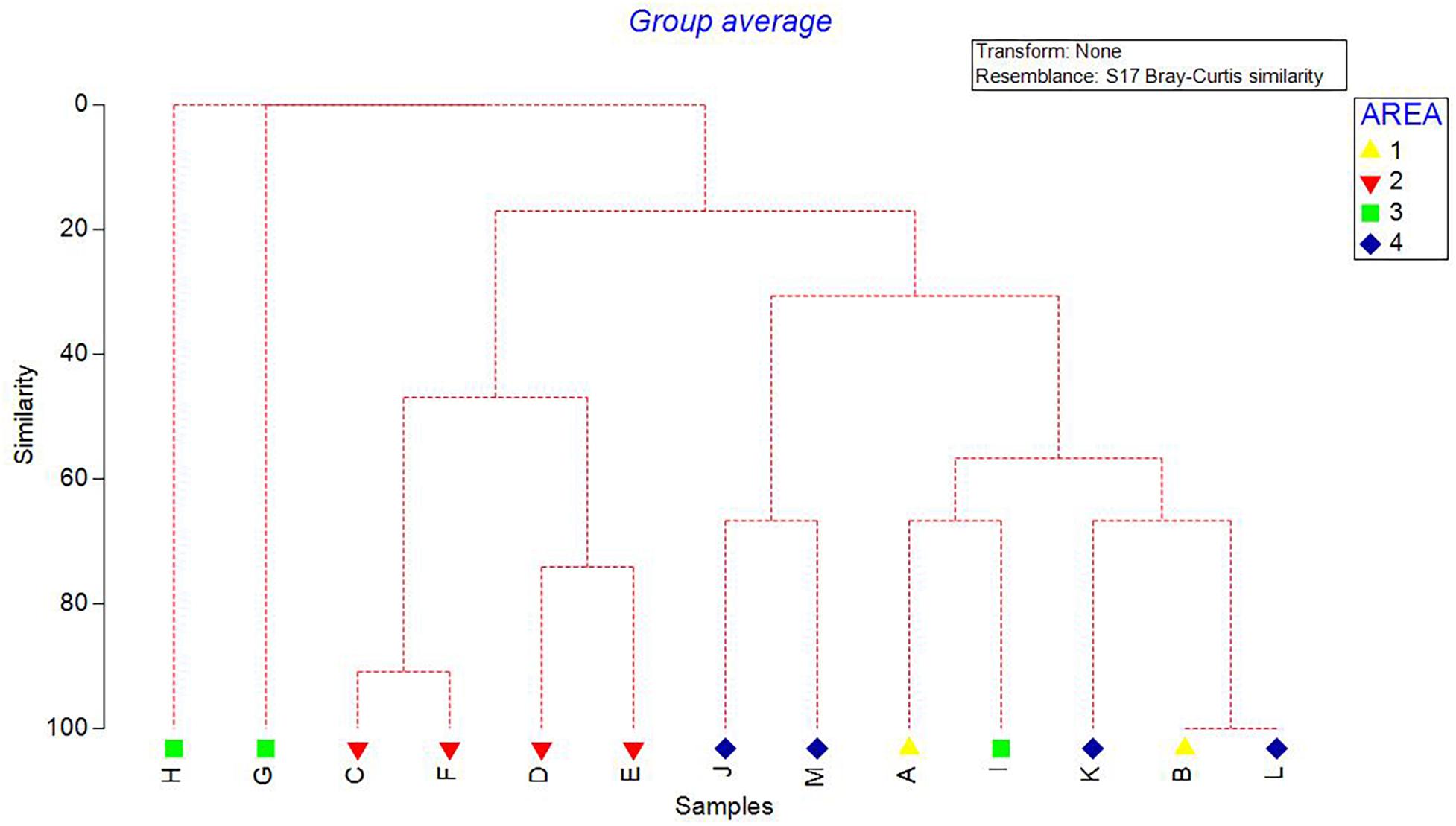
Figure 7. Dendrogram showing the clustering of transects/areas (Area 1, Area 2, Area 3, and Area 4). Analysis was based on the density values (individuals/m2) of sponge morphotype categories.
Density of Sponge Morphotypes Across Depth
Density values for both Categories 3 and 4 showed a peak within a narrow zone (450–530 m) (Figures 8A–C). This peak was recorded inside the FSC NCMPA. The maximum values of density recorded for Category 3 was 0.79 individuals/m2 (Figure 8A) and for Category 4 was 1.93 individuals/m2 (Figure 8B).
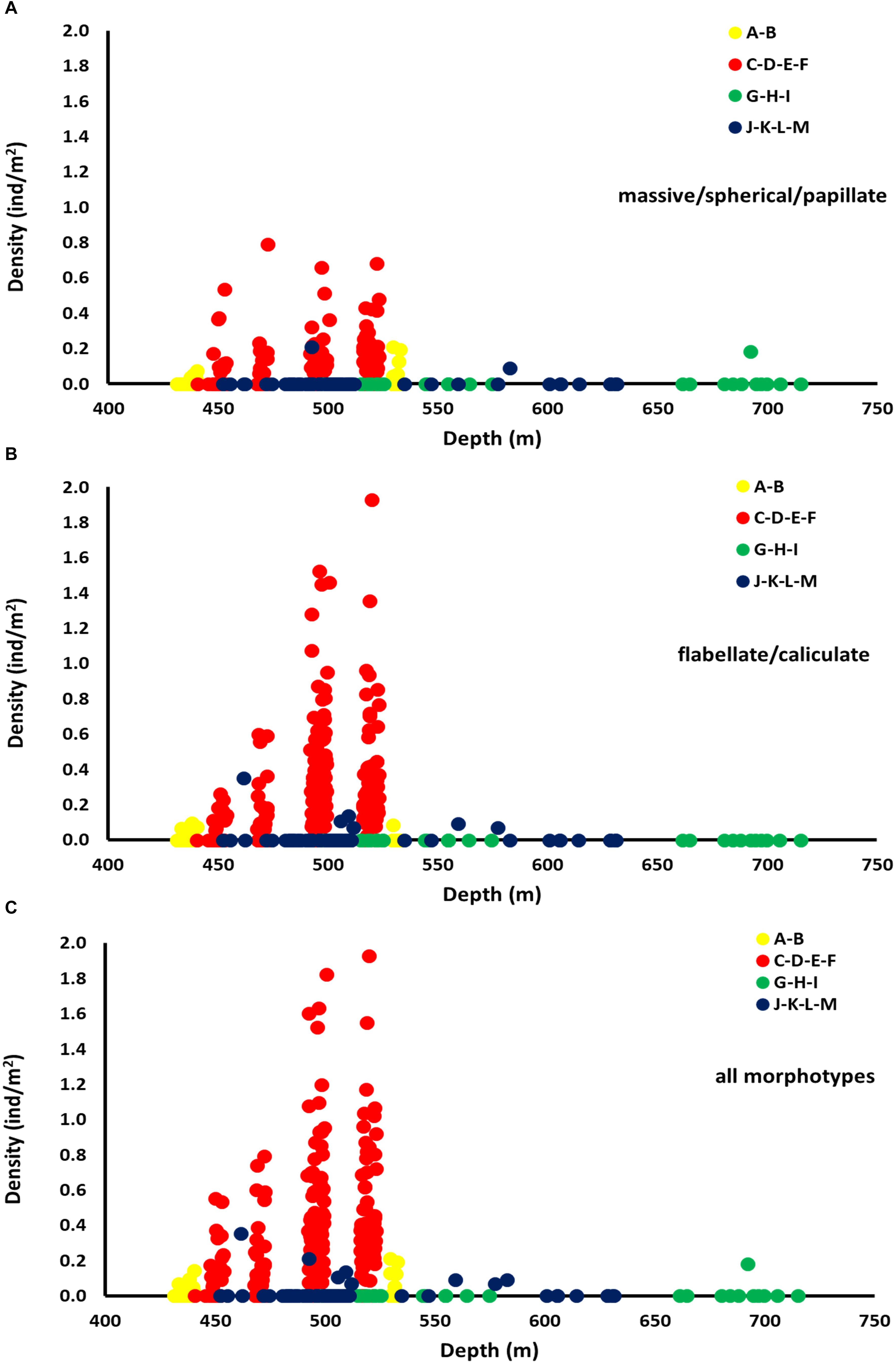
Figure 8. Density (individuals/m2) of sponge morphotypes across depth in each of the four areas: Area 1 (transects A-B), Area 2 (transects C-D-E-F), Area 3 (transects G-H-I), Area 4 (transects J-K-L-M). Data shown for Category 3 (massive/spherical/papillate) (A), Category 4 (flabellate/caliculate) (B), and for all sponge morphotypes (C).
Size of Sponge Morphotypes Inside and Outside the FSC NCMPA
The average size for Category 3 inside the FSC NCMPA was significantly higher than outside the FSC NCMPA i.e., 13.3 ± 0.8 vs. 9.2 ± 3.1 cm, respectively (Wilcoxon rank sum test = 944.5, p < 0.05, n = 145). In Category 4, there were no statistically-significant differences in the average size between transects inside and outside the FSC NCMPA i.e., 12.3 ± 0.4 vs. 11.7 ± 2.4 cm, respectively (Wilcoxon rank sum test = 2713.5, p > 0.05, n = 469). For all the sponge morphotypes, the average size inside the FSC NCMPA was not different from the average size outside the FSC NCMPA i.e., 12.6 ± 0.4 vs. 10.5 ± 1.9 cm, respectively (Wilcoxon rank sum test = 7621.5, p > 0.05, n = 614).
The analysis of the body-size distribution through the Bhattacharya method revealed clear differences between inside and outside the NCMPA. Specifically, in Category 3 there were two size cohorts in transects inside the FSC NCMPA and one size cohort outside the FSC NCMPA. In Category 4 there were three size cohorts inside the FSC NCMPA and one outside the NCMPA. The analysis of Categories 3 and 4 pooled together showed the existence of four size cohorts inside the FSC NCMPA and one size cohort outside the FSC NCMPA (Table 4 and Figure 9).
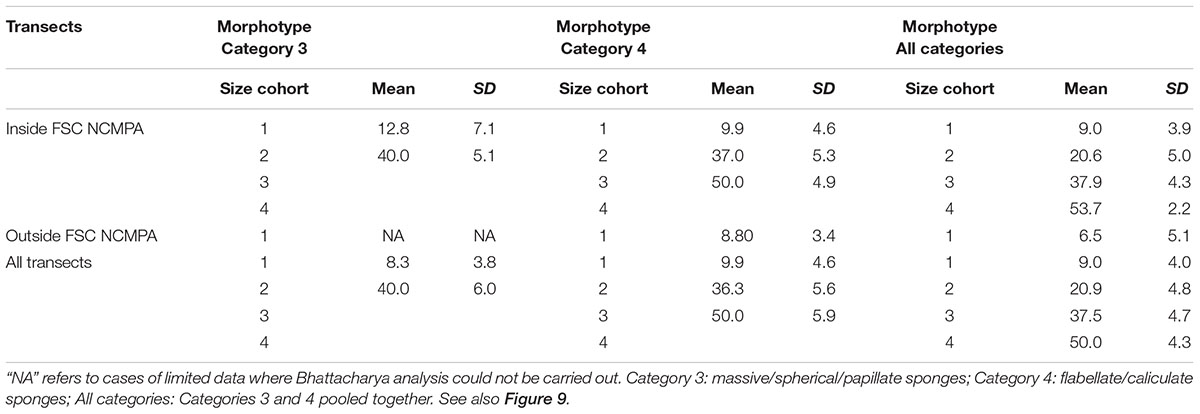
Table 4. Size cohorts (mean ± standard deviation for each cohort) in transects (a) inside the Faroe-Shetland Channel Nature Conservation Marine Protected Area (FSC NCMPA), (b) outside the FSC NCMPA, and (c) across all transects.
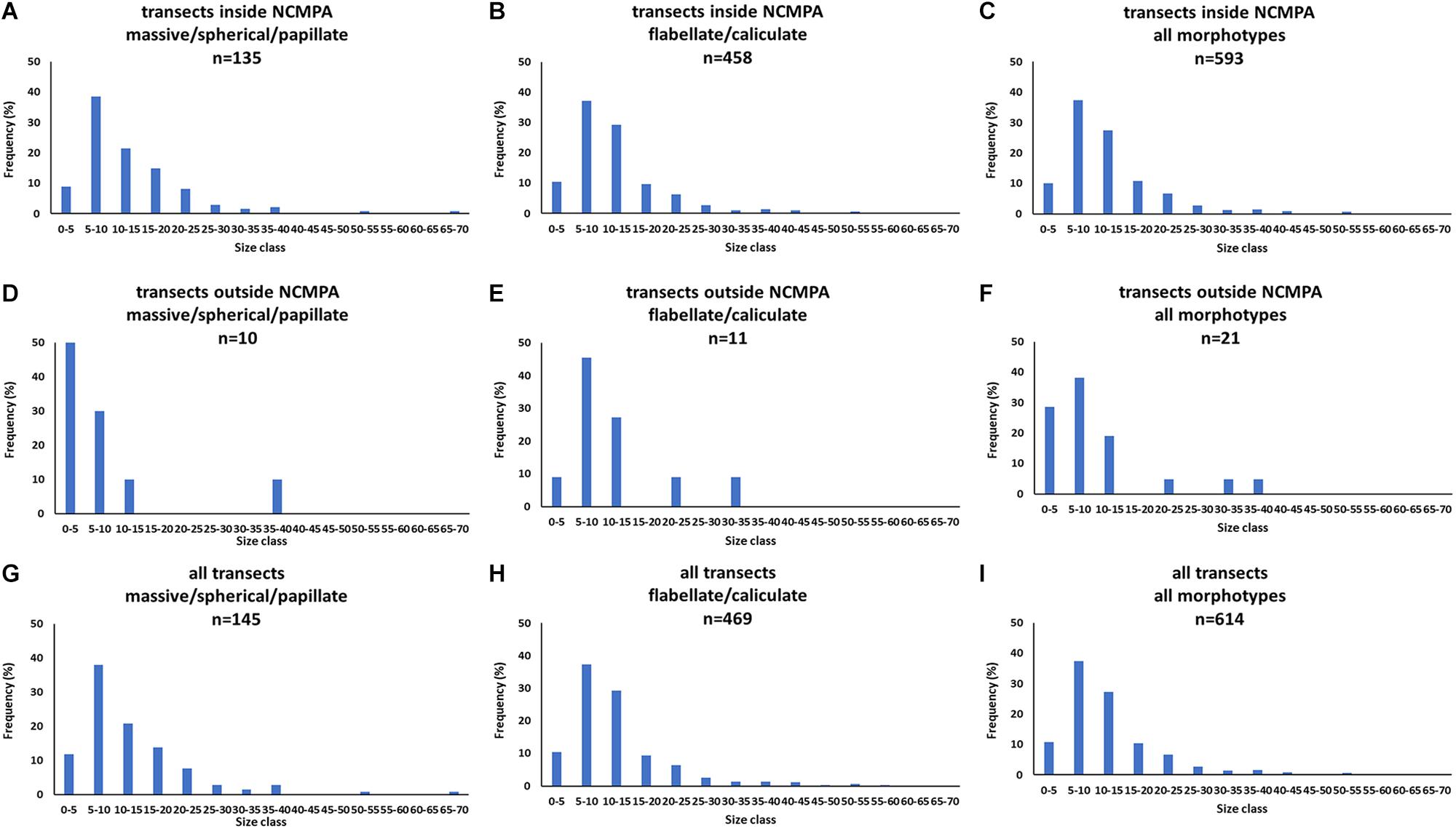
Figure 9. Size-frequency distributions of sponge size (cm) for transects (i) inside the Faroe-Shetland Channel Nature Conservation Marine Protected Area (FSC NCMPA) (A–C), (ii) outside the FSC NCMPA (D–F), (iii) all transects (G–I). Data are shown for Category 3 (massive/spherical/papillate), Category 4 (flabellate/caliculate), and for all sponge morphotypes.
Role of Fisheries and Environmental Parameters in Density of Sponge Morphotypes
Fisheries and environmental variability explained a total of 47.98% of the variation in the density of sponge morphotypes. Variables that explained significant amounts of variation included demersal fisheries pressure (29.75%), cobble with boulder (12.02%), salinity (4.89%) and temperature (1.07%) (Table 5). Inside the FSC NCMPA the density of Category 3 and Category 4 sponges was higher than outside the FSC NCMPA. Furthermore, the highest densities of Category 3 and Category 4 sponges were found in transects dominated by cobble with boulder substrata. The temperature and salinity values in transects with highest measured densities ranged from 6.52 to 8.98°C and 34.91 to 35.13 psu, respectively, indicating that the sponge aggregations were mainly sitting within the relatively warm and saline NAW, MNAW, and MEIW.
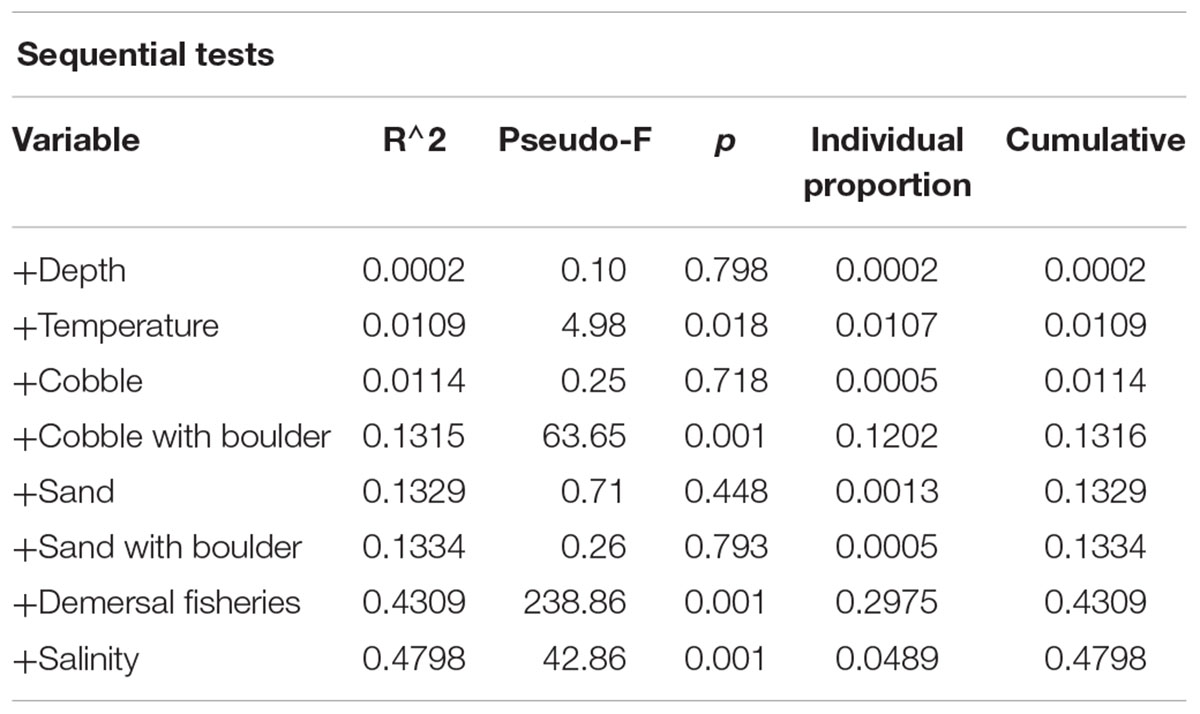
Table 5. Outcome of distance-based linear modeling on the role of environmental parameters in the variation of density of sponge morphotypes.
Discussion
The present study showed that sponge morphotype composition did not differ between inside and outside the FSC NCMPA boundary, but richness, diversity and densities of Category 3 and Category 4 sponges (Tables 2, 3 and Figures 2, 6) were higher inside than outside this boundary. Multiple size cohorts of sponges were recorded inside the FSC NCMPA, in contrast to the single cohort outside (Table 4 and Figure 9). Variation in density of sponges was mostly explained by fisheries pressure: higher densities of sponges were found in the area- with lowest values of demersal landings (i.e., Area 2) while densities were significantly lower in the areas with higher demersal landings (i.e., Area 1, Area 3, and Area 4). Type of substratum explained also a significant amount of variation in sponge density: highest densities were found in cobble with boulder. Finally, water temperature and salinity explained also a significant (but lower compared to fisheries and substratum) amount of variation in sponge density: highest values were found in Area 2 where temperature and salinity ranged from 6.52 to 8.98°C and 34.91 to 35.13 psu, respectively, indicating that the sponge aggregations were mainly sitting within the relatively warm and saline NAW, MNAW, and MEIW.
Richness/Diversity, Body-Size Distribution and Density of Sponge Morphotypes Inside and Outside the FSC NCMPA – The Role of Human Activities and Environmental Variability
Richness and Diversity of Sponge Morphotypes
Sponge aggregations inside and outside the FSC NCMPA were mainly composed of massive (most likely Geodia spp.) and flabellate (most likely Phakellia ventilabrum) sponges (Table 2). Unfortunately, biological samples were not collected during the transects and thus the taxonomic identification of specimens seen in images was not possible. Findings of the present study are in agreement with previous studies in the wider FSC that recorded Geodia and Phakellia in this area (Bett, 2001; Axelsson, 2003; Klitgaard and Tendal, 2004; Howell et al., 2010; Henry and Roberts, 2014a).
Although the same morphotypes were encountered inside and outside the FSC NCMPA, their richness and diversity were significantly higher inside than outside this boundary. Studies have shown that bottom fishing has an impact on the morphotypes of benthic organisms and the same may hold true for our findings on FSC NCMPA. Specifically, a photographic survey in the Georges Bank (northwest Atlantic Ocean) provided evidence that erect forms like hydrozoans, bushy bryozoans, sponges and sedentary polychaetes had higher cover in undisturbed sites while encrusting bryozoans were the only colonial group not affected by fishing (Asch and Collie, 2008). As regards the role of hydrography, Bell and Barnes (2000) have shown that massive and encrusting sponge morphotypes were more abundant at high-flow areas in the sublittoral zone, due to a high basal area to volume ratio which decreases removal from the substrates; on the contrary, pedunculate, papillate and arborescent morphotypes were more abundant at the low flow areas as these morphotypes may prevent sedimentation on sponges (see also Ginn et al., 2000; Bell et al., 2002; George et al., 2018). Bell and Barnes (2000) also showed that diversity of sponge morphotypes decreased with increasing flow due to the removal of delicate morphotypes. Studies on the hydrography of the FSC have shown the existence of active mesoscale variability (Sherwin et al., 2006). The role, however, of this hydrographic variability in shaping richness and diversity of FSC NCMPA sponge aggregations, is currently unknown.
Body-Size Distribution of Sponge Morphotypes
Our study showed that the number of body-size cohorts sponges was higher inside than outside the FSC NCMPA (Table 4 and Figure 9). Although the available time series on demersal fisheries inside and outside the FSC NCMPA are limited the findings indicate the impact that fisheries can have on the FSC NCMPA sponge aggregations. However, due to this limited data availability, the impact of fisheries should be treated with caution. Actually, we did not observe trawl marks in any of the 465 images analyzed (see Figure 4 in Roberts et al., 2000). Hydrography, however, in FSC NCMPA is dynamic (Sherwin et al., 2006) and trawl marks could have been erased. In addition, fishermen may intentionally now avoid the FSC NCMPA sponge aggregations in order to limit damage to fishing gear or due to relatively little fish biomass present in sponge aggregations (e.g., see Munoz et al., 2012; Kenchington et al., 2013; Kutti et al., 2014).
Present findings on sponge richness/diversity and body-size distribution are a strong indication about the presence inside the FSC NCMPA of more favorable conditions for reproduction, recruitment and growth of sponges. Data on these very important parameters, however, and their role in shaping deep-sea sponge aggregations are extremely limited (Witte, 1996; Fallon et al., 2010). Long-term studies in the abyssal northeast Pacific have shown a correlation between the increase in food supply and density of two glass sponges, suggesting that increased flux of particulate organic carbon may cause recruitment or regeneration in deep-sea sponges (Kahn et al., 2012). Furthermore, studies on reef-forming glass sponges in British Columbia showed that recruitment took place where hard substrate (sponge skeletons) was available (Kahn et al., 2016). Apart from the parameters shaping body-size distribution information on the number and structure of body-size cohorts themselves is also limited and when available, a detailed analysis like the one carried out here has not been completed. Available studies have shown unimodal distribution with long tails (e.g., in Pachastrella monilifera and Poecillastra compressa – Bo et al., 2012; various taxa – Rooper et al., 2016) and multimodal distributions (e.g., in Pheronema carpenteri – Rice et al., 1990; in Geodia mesotriaena – Klitgaard and Tendal, 2004).
Density
Density had significantly higher values inside than outside the FSC NCMPA (Table 3 and Figure 6). The average value when all sponge morphotypes within the FSC NCMPA were summed, was 0.28 individuals/m2 which is well within the range reported for deep-sea areas in the North Atlantic e.g., the Traena Coral Field (0.14 individuals/m2 for geodiids and unidentified sponges – Cathalot et al., 2015), the Rosemary Bank Seamount Nature Conservation Marine Protected Area (1.2 individuals/m2 for various demosponges – McIntyre et al., 2016), the Norwegian shelf (∼0.2 individuals/m2 for various demosponges – Buhl-Mortensen et al., 1995), and Norwegian cold-water coral reefs (from 0.007 to 2.254 individuals/m2 for Mycale lingua; from 0.011 to 0.139 individuals/m2 for Geodia barretti – Purser et al., 2013) (see also Rice et al., 1990; Barthel et al., 1996; Kutti et al., 2013).
Densities of sponges peaked within a highly constrained depth zone of about 80 m (Figure 8) with substratum having a statistically-significant contribution. Highest values were recorded where cobble/cobble with boulder were the dominant or even the only type of substrate recorded (Tables 2, 3 and Figures 5, 6). This finding is in agreement with available information about the presence of Geodia and Phakellia sponges on hard substrates (Hoffmann et al., 2003; Klitgaard and Tendal, 2004; Ackers et al., 2007; Gates and Jones, 2012; Tjensvoll et al., 2013).
Apart from fisheries pressure and substratum, a whole suite of other variables related to chemical oceanography exerted strong control over sponge aggregations in the FSC NCMPA in similar ways found in other North Atlantic regions. This suggests that these same factors shape distribution and structure of sponge grounds at the basin scale. These variables include water temperature, salinity and silicate concentrations (Murillo et al., 2012; Knudby et al., 2013; Beazley et al., 2015; Howell et al., 2016), topography, internal waves, current speeds, food supply (Rice et al., 1990; Klitgaard and Tendal, 2004; Knudby et al., 2013; Howell et al., 2016). Establishing a precise relationship between the contribution of each of the environmental parameters mentioned above and the distribution of deep-sea sponges/deep-sea sponge aggregations is still an open question given the limited availability of data on (a) species’ physiological tolerances, (b) geographical/bathymetric distribution of these organisms and (c) measurements of environmental data at appropriate spatial scales (Tjensvoll et al., 2013; Beazley et al., 2015; Strand et al., 2017). In the present study, the values of temperature per transect inside the FSC NCMPA ranged from 6.5°C (transect F) to ∼8.9°C (transect D) and salinity values ranged from 34.9 psu (transect D) to 35.1 psu (transect C; Table 1); these values fall within the temperature and salinity range reported up to now for Geodia sponges in the North East Atlantic (Klitgaard and Tendal, 2004; Bett, 2012). Despite this agreement, it is not clear to what extent the high density of sponge aggregations recorded inside the FSC NCMPA is due to the physical/chemical parameters of the water masses per se and/or due to the outcome of the interaction of these water masses (i.e., NAW, MNAW, MEIW, NSAIW, NSDW) with the slope creating internal waves supplying food particles to deep-sea benthos (Mienis et al., 2009; Mohn et al., 2014). Taking into account, however, that (a) rapid changes in temperature have been recorded at the Faroe-Shetland Channel (up to 7°C within 1 h) at a depth of 550 m (Bett, 2001) and that deep-sea sponges feed mainly on particulate organic matter (e.g., Kazanidis et al., 2018 and references therein), it is likely that the hydrographic conditions (i.e., internal waves) play a more important role than temperature and salinity themselves in promoting the proliferation of sponges within the FSC NCMPA. Previous works have also highlighted the role of enhanced kinetic energy increasing the supply of food particles to sponges (Rice et al., 1990; Klitgaard and Tendal, 2004; Beazley et al., 2015).
Suggestions made above about the role of water mass characteristics and hydrography in promoting the proliferation of sponge aggregations within the FSC NCMPA gain further interest considering long-term changes in major chemical features in North Atlantic including the FSC NCMPA (Hátún et al., 2017). On top of changes in silicate concentration, long-term measurements (1994–2011) in the FSC NCMPA have also shown that surface layers (NAW and MNAW) have become warmer and more saline over the past two decades: temperatures have been increasing by 0.5°C per decade and salinities by approximately 0.07 (Berx et al., 2013). These temperature- and salinity-related changes in FSC water masses may pose additional pressures on the vulnerable sponge aggregations through changes like a decrease in oxygen concentration (e.g., Sweetman et al., 2017).
Conclusion and Suggestions Facilitating Adaptive Management in the FSC NCMPA
The present study provided evidence that the FSC NCMPA boundary currently encloses the most vulnerable area encompassing sponge communities with high richness, diversity, density and several size classes. Sponge communities were constrained to a narrow environmental niche shaped by relatively low fishing impact and the interactions of the five FSC water masses with the slope, supplying in this way food to sponges. This suggests that the deep-sea sponge aggregations are vulnerable not only to demersal fishing but could also be susceptible to changes in oceanic conditions over time.
The findings of the present study can contribute to the establishment of monitoring programs and efficient conservation strategies in the FSC NCMPA. Our study presents simple indicators that can be monitored during annual demersal camera surveys of the FSC NCMPA, including sponge richness/diversity, density, and body-size distribution. The present study serves both baseline and future assessments of anthropogenic and climate change impacts on deep-sea sponge ecosystem environmental status in the FSC NCMPA guiding thus adaptive management in FSC NCMPA.
Author Contributions
FN designed and led the ship survey and collated the video footage. GK and JV processed the video/image material. GK, JV, and L-AH designed the statistical comparisons, while GK and JV analyzed the data with assistance from KG. BB provided oceanographic data from the FSC. All authors helped to write the manuscript.
Funding
JV acknowledges support from the Natural Environment Research Council Centre for Doctoral Training in Oil and Gas, received through Heriot-Watt University (James Watt Scholarship scheme) and the British Geological Survey (British University Funding Initiative scheme). This study received funding from the European Union’s Horizon 2020 research and innovation programme under grant agreement no. 678760 (ATLAS). This output reflects only the authors’ view and the European Union cannot be held responsible for any use that may be made of the information contained therein. The MRV Scotia survey was funded by the Scottish Government (MOREDEEP project).
Conflict of Interest Statement
The authors declare that the research was conducted in the absence of any commercial or financial relationships that could be construed as a potential conflict of interest.
Acknowledgments
We are grateful for camera technical support from Neil Collie and Mike Stewart (Marine Scotland).
Footnotes
- ^ http://jncc.defra.gov.uk/page-6483
- ^ http://jncc.defra.gov.uk/pdf/NEFSC_SACO_v1.0.pdf)
- ^ https://marinescotland.atkinsgeospatial.com/nmpi/
- ^ https://www.gov.scot/Topics/marine
- ^ http://www.seatronics-group.com
- ^ www.km.kongsberg.com
- ^ Release, 2017b; www.matlab.com
- ^ http://mooring.ucsd.edu/software/matlab/doc/ocean/oxsat.html
- ^ www.qgis.org/en/site/
- ^ https://marinescotland.atkinsgeospatial.com/nmpi/
- ^ http://www.fao.org/fishery/topic/16072/en
References
Ackers, R. G., Moss, D., Picton, B. E., Stone, S. M. K., and Morrow, C. C. (2007). Sponges of the British Isles (“Sponge V”). A Colour Guide and Working Document. London: Marine Conservation Society, 165.
Asch, R. G., and Collie, J. S. (2008). Changes in a benthic megafaunal community due to disturbance from bottom fishing and the establishment of a fishery closure. Fish. Bull. 106, 438–456.
Axelsson, M. B. (2003). The Deep-Seabed Environment of the UK Continental Margin – Integration and Interpretation of Geological and Biological Data. PhD thesis, University of Southampton, Southampton.
Barthel, D., Tendal, O. S., and Thiel, H. (1996). A wandering population of the hexactinelIid sponge Pheronema carpenteri on the continental slope off Morocco, Northwest Africa. Mar. Ecol. 17, 603–616. doi: 10.1111/j.1439-0485.1996.tb00420.x
Beazley, L., Kenchington, E., Yashayaev, I., and Murillo, F. J. (2015). Drivers of epibenthic megafaunal composition in the sponge grounds of the sackville spur, northwest Atlantic. Deep Sea Res. I 98, 102–114. doi: 10.1016/j.dsr.2014.11.016
Bell, J. J. (2008). The functional roles of marine sponges. Estuar. Coast. Shelf Sci. 79, 341–353. doi: 10.1016/j.ecss.2008.05.002
Bell, J. J., and Barnes, D. K. A. (2000). The influences of bathymetry and flow regime upon the morphology of sublittoral sponge communities. J. Mar. Biol. Assoc. 80, 707–718. doi: 10.1017/S0025315400002538
Bell, J. J., Barnes, D. K. A., and Shaw, C. (2002). Branching dynamics of two species of arborescent demosponge: the effect of flow regime and bathymetry. J. Mar. Biol. Assoc. 82, 279–294. doi: 10.1017/S0025315402005465
Berx, B. (2012). The hydrography and circulation of the faroe-shetland channel. Ocean Chall. 19, 15–19.
Berx, B., Hansen, B., Osterhus, S., Larsen, K. M., Sherwin, T., and Jochumsen, K. (2013). Combining in situ measurements and altimetry to estimate volume, heat and salt transport variability through the faroe–shetland channel. Ocean Sci. 9, 639–654. doi: 10.5194/os-9-639-2013
Bett, B. (2001). UK atlantic margin environmental survey: introduction and overview of bathyal benthic ecology. Cont. Shelf Res. 21, 917–956. doi: 10.1016/S0278-4343(00)00119-9
Bett, B. (2003). An Introduction to the Benthic Ecology of the Faroe-Shetland Channel (SEA4). London: Department of Trade and Industry, 84.
Bett, B. (2012). Seafloor Biotope Analysis of the Deep Waters of the SEA4 Region of Scotland’s Seas. JNCC Report No. 472. Peterborough: Joint Nature Conservation Committee, 104.
Bett, B. J. (2000). Signs and Symptoms of Deepwater Trawling on the Atlantic Margin. Man-Made Objects on the Seafloor. London: Society for Underwater Technology, 107–118.
Bo, M., Bertolino, M., Bavestrello, G., Canese, S., Giusti, M., Angiolillo, M., et al. (2012). Role of deep sponge grounds in the mediterranean sea: a case study in southern italy. Hydrobiologia 687, 163–177. doi: 10.1007/s10750-011-0964-1
Boury-Esnault, N., and Rützler, K. (1997). Thesaurus of sponge morphology. Smithson. Contrib. Zool. 596, 1–55. doi: 10.5479/si.00810282.596
Broadbridge, M. B., and Toumi, R. (2015). The deep circulation of the faroe-shetland channel: opposing flows and topographic eddies. J. Geophys. Res. Oceans 120, 5983–5996. doi: 10.1002/2015JC010833
Buhl-Mortensen, P., Hovland, M., Brattegard, T., and Farestveit, R. (1995). Deepwater bioherms of the scleractinian coral Lophelia pertusa (L.) at 64oN on the Norwegian shelf: structure and associated megafauna. Sarsia 80, 145–158. doi: 10.1080/00364827.1995.10413586
Bullough, L. W., Turrell, W. R., Buchan, P., and Priede, I. G. (1998). Commercial deep water trawling at sub-zero temperatures - observations from the faroe-shetland channel. Fish. Res. 39, 33–41. doi: 10.1016/S0165-7836(98)00170-2
Cárdenas, P., Rapp, H. T., Klitgaard, A. B., Best, M., Thollesson, M., and Tendal, O. S. (2013). Taxonomy, biogeography and DNA barcodes of Geodia species (Porifera, Demospongiae, Tetractinellida) in the Atlantic boreo-arctic region. Zool. J. Linn. Soc. 169, 251–311. doi: 10.1111/zoj.12056
Cathalot, C., van Oevelen, D., Cox, T. J. S., Kutti, T., Lavaleye, M., Duineveld, G., et al. (2015). Cold-water coral reefs and adjacent sponge grounds: hot spots of benthic respiration and organic carbon cycling in the deep sea. Front. Mar. Sci. 2:37. doi: 10.3389/fmars.2015.00037
Clarke, K. R., Gorley, R. N., Somerfield, P. J., and Warwick, R. M. (2014). Change in Marine Communities: an Approach to Statistical Analysis and Interpretation, 3nd Edn. Plymouth: PRIMER-E.
Core Team, R. (2018). R: A Language and Environment for Statistical Computing. Vienna: R Foundation for Statistical Computing.
Fallon, S. J., James, K., Norman, R., Kelly, M., and Ellwood, M. J. (2010). A simple radiocarbon dating method for determining the age and growth rate of deep-sea sponges. Nucl. Instrum. Methods Phys. Res. B 268, 1241–1243. doi: 10.1016/j.nimb.2009.10.143
Gates, A. R., and Jones, D. O. B. (2012). Recovery of benthic megafauna from anthropogenic disturbance at a hydrocarbon drilling well (380 m depth in the Norwegian Sea). PLoS One 7:e44114. doi: 10.1371/journal.pone.0044114
Gayanilo, F. C. J., and Pauly, D. (1997). The FAO–ICLARM Stock Assessment Tools (FiSAT) Reference Manual. Rome: FAO Computerized Information Series.
George, A. M., Brodie, J., Daniell, J., Capper, A., and Jonker, M. (2018). Can the sponge morphologies act as environmental proxies to biophysical factors in the great barrier reef. Australia? Ecol. Indic. 93, 1152–1162. doi: 10.1016/j.ecolind.2018.06.016
Ginn, B. K., Logan, A., and Thomas, M. L. H. (2000). Sponge ecology on sublittoral hard substrates in a high current velocity area. Est. Coast. Shelf Sci. 50, 403–414. doi: 10.1006/ecss.1999.0563
Hansen, B., and Østerhus, S. (2000). North atlantic–nordic seas exchanges. Prog. Oceanogr. 45, 109–208. doi: 10.1016/S0079-6611(99)00052-X
Hátún, H., Azetsu-Scott, K., Somavilla, R., Rey, F., Johnson, C., Mathis, M., et al. (2017). The subpolar gyre regulates silicate concentrations in the North Atlantic. Sci. Rep. 7:14576. doi: 10.1038/s41598-017-14837-4
Henry, L.-A., and Roberts, J. M. (2004). The Biodiversity, Characteristics and Distinguishing Features of Deep-Water Epifaunal Communities from the Wyville-Thomson Ridge, Darwin Mounds and Faeroe Plateau. AFEN: Report to the Atlantic Frontier Environment Network, 44.
Henry, L.-A., and Roberts, J. M. (2014a). Applying the OSPAR Habitat Definition of Deep-sea Sponge Aggregations to Verify Suspected Records of the Habitat in UK Waters. JNCC Report No 508. Peterborough: Joint Nature Conservation Committee.
Henry, L.-A., and Roberts, J. M. (2014b). Recommendations for best practice in deep-sea habitat classification: bullimore et al. as a case study. ICES J. Mar. Sci. 71, 895–898. doi: 10.1093/icesjms/fst175
Hoffmann, F., Rapp, H. T., Zöller, T., and Reitner, J. (2003). Growth and regeneration in cultivated fragments of the boreal deep water sponge Geodia barretti Bowerbank, 1858 (Geodiidae, Tetractinellida, Demospongiae). J. Biotechnol. 100, 109–118. doi: 10.1016/S0168-1656(02)00258-4
Hogg, M. M., Tendal, O. S., Conway, K. W., Pomponi, S. A., van Soest, R. W. M., Gutt, J., et al. (2010). Deep-Sea Sponge Grounds: Reservoirs of Biodiversity. UNEP-WCMC Biodiversity Series No. 32. Cambridge: UNEP-WCMC.
Howell, K., Davies, J. S., and Narayanaswamy, B. E. (2010). Identifying deep-sea megafaunal epibenthic assemblages for use in habitat mapping and marine protected area network design. J. Mar. Biol. Assoc. 90, 33–68. doi: 10.1017/S0025315409991299
Howell, K. L., Piechaud, N., Downie, A. L., and Kenny, A. (2016). The distribution of deep-sea sponge aggregations in the North Atlantic and implications for their effective spatial management. Deep Sea Res. I 115, 309–320. doi: 10.1016/j.dsr.2016.07.005
Johnson, D., Ferreira, M. F., and Kenchington, E. (2018). Climate change is likely to severely limit the effectiveness of deep-sea ABMTs in the north atlantic. Mar. Policy 87, 111–122. doi: 10.1016/j.marpol.2017.09.034
Jones, D. O. B., Gates, A. R., and Lausen, B. (2012). Recovery of deep-water megafaunal assemblages from hydrocarbon drilling disturbance in the faroe-shetland channel. Mar. Ecol. Prog. Ser. 461, 71–82. doi: 10.3354/meps09827
Jones, D. O. B., Hudson, I. A., and Bett, B. J. (2006). Effects of physical disturbance on the cold-water megafaunal communities of the faroe–shetland channel. Mar. Ecol. Prog. Ser. 319, 43–54. doi: 10.3354/meps319043
Jones, D. O. B., Wigham, B. D., Hudson, I. R., and Bett, B. J. (2007). Anthropogenic disturbance of deep-sea megabenthic assemblages: a study with remotely operated vehicles in the faroe-shetland channel. NE Atlantic. Mar. Biol. 151, 1731–1741. doi: 10.1007/s00227-007-0606-3
Kahn, A. S., Ruhl, H. A., and Smith, K. L. Jr. (2012). Temporal changes in deep-sea sponge populations are correlated to changes in surface climate and food supply. Deep Sea Res. Part I 70, 36–41. doi: 10.1016/j.dsr.2012.08.001
Kahn, A. S., Vehring, L. J., Brown, R. R., and Leys, S. P. (2016). Dynamic change, recruitment and resilience in reef-forming glass sponges. J. Mar. Biol. Assoc. U.K. 96, 429–436. doi: 10.1017/S0025315415000466
Kazanidis, G., Henry, L.-A., Roberts, J. M., and Witte, U. F. M. (2016). Biodiversity of Spongosorites coralliophaga (Stephens, 1915) on coral rubble at two contrasting cold-water coral reef settings. Coral Reefs 35, 193–208. doi: 10.1007/s00338-015-1355-2
Kazanidis, G., van oevelen, D., Veuger, B., and Witte, U. F. M. (2018). Unravelling the versatile feeding and metabolic strategies of the cold-water ecosystem engineer Spongosorites coralliophaga (Stephens, 1915). Deep Sea Res. Part I Oceanogr. Res. Papers 141, 71–82. doi: 10.1016/j.dsr.2018.07.009
Kenchington, E., Murillo, F. J., Lirette, C., Sacau, M., Koen-Alonso, M., Kenny, A., et al. (2014). Kernel density surface modelling as a means to identify significant concentrations of vulnerable marine ecosystem indicators. PLoS One 9:e109365. doi: 10.1371/journal.pone.0109365
Kenchington, E., Power, D., and Koen-Alonso, M. (2013). Associations of demersal fish with sponge grounds on the continental slopes of the northwest Atlantic. Mar. Ecol. Prog. Ser. 477, 217–230. doi: 10.3354/meps1012
Klitgaard, A. B., and Tendal, O. S. (2004). Distribution and species composition of mass occurrences of large-sized sponges in the northeast Atlantic. Prog. Oceanogr. 61, 57–98. doi: 10.1016/j.pocean.2004.06.002
Knudby, A., Kenchington, E., and Murillo, F. J. (2013). Modeling the distribution of Geodia sponges and sponge grounds in the Northwest Atlantic. PLoS One 8:e82306. doi: 10.1371/journal.pone.0082306
Kutti, T., Bannister, R. J., and Fossa, J. H. (2013). Community structure and ecological function of deep-water sponge grounds in the traenadypet MPA—Northern Norwegian continental shelf. Cont. Shelf Res. 69, 21–30. doi: 10.1016/j.csr.2013.09.011
Kutti, T., Bergstad, O. A., Fosså, J. H., and Helle, K. (2014). Cold-water coral mounds and sponge-beds as habitats for demersal fish on the Norwegian shelf. Deep Sea Res. II 99, 122–133. doi: 10.1016/j.dsr2.2013.07.021
Maldonado, M., Aguilar, R., Bannister, R. J., Bell, J. J., Conway, K. W., Dayton, P. K., et al. (2017). “Sponge grounds as key marine habitats: a synthetic review of types, structure, functional roles, and conservation concerns,” in Marine Animal Forests the Ecology of Benthic Biodiversity Hotspots, eds S. Rossi, L. Bramanti, A. Gori, and C. Orejas (Berlin: Springer), 145–183.
Maldonado, M., Navarro, L., Grasa, A., Gonzalez, A., and Vaquerizo, I. (2011). Silicon uptake by sponges: a twist to understanding nutrient cycling on continental margins. Sci. Rep. 1:30. doi: 10.1038/srep00030
Marine Scotland (2017). Northern North Sea Proposals. JNCC Feedback on Proposed Fisheries Management Measures. Available at: https://fiskeristyrelsen.dk/media/9068/northern-north-sea-proposal.pdf (accessed March 27, 2019).
Masson, D. G. (2001). Sedimentary processes shaping the eastern slope of the faeroe-shetland channel. Cont. Shelf Res. 21, 825–857. doi: 10.1016/S0278-4343(00)00115-1
McIntyre, F. D., Collie, N., Stewart, M., Scala, L., and Fernandes, P. G. (2013). A visual survey technique for deep-water fishes: estimating anglerfish Lophius spp. abundance in closed areas. J. Fish Biol. 83, 739–753. doi: 10.1111/jfb.12114
McIntyre, F. D., Drewery, J., Eerkes-Medrano, D., and Neat, F. C. (2016). Distribution and diversity of deep-sea sponge grounds on the rosemary bank seamount. NE Atlantic. Mar. Biol. 63, 142–152. doi: 10.1007/s00227-016-2913-z
McKenna, C., Berx, B., and Austin, W. E. N. (2016). The decomposition of the faroe-shetland channel water masses using parametric optimum multi-parameter analysis. Deep Sea Res. I 107, 9–21. doi: 10.1016/j.dsr.2015.10.013
Mienis, F., de Stigter, H. C., de Haas, H., and van Weering, T. C. E. (2009). Near-bed particle deposition and resuspension in a cold-water coral mound area at the southwest rockall trough margin, NE. Atlantic. Deep Sea Res. I 56, 1026–1038. doi: 10.1016/j.dsr.2009.01.006
Mohn, C., Rengstorf, A., White, M., Duineveld, G., Mienis, F., Soetaert, K., et al. (2014). Linking benthic hydrodynamics and cold-water coral occurrences: a high-resolution model study at three cold-water coral provinces in the NE Atlantic. Prog. Oceanogr. 122, 92–104. doi: 10.1016/j.pocean.2013.12.003
Munoz, P. D., Sayago-Gil, M., Patrocinio, T., González-Porto, M., Murillo, F. J., Sacau, M., et al. (2012). Distribution patterns of deep-sea fish and benthic invertebrates from trawlable grounds of the hatton bank, north-east Atlantic: effects of deep-sea bottom trawling. J. Mar. Biol. Assoc. 92, 1509–1524. doi: 10.1017/S002531541200015X
Murillo, F. J., Muñoz, P. D., Cristobo, J., Ríos, P., González, C., Kenchington, E., et al. (2012). Deep-sea sponge grounds of the flemish cap, flemish pass and the grand banks of newfoundland (Northwest Atlantic Ocean): distribution and species composition. Mar. Biol. Res. 8, 8842–8854. doi: 10.1080/17451000.2012.682583
Narayanaswamy, B. E., Bett, B. J., and Hughes, D. J. (2010). Deep-water macrofaunal diversity in the faroe-shetland region (NE Atlantic): a margin subject to an unusual thermal regime. Mar. Ecol. 31, 237–246. doi: 10.1111/j.1439-0485.2010.00360.x
OSPAR. (2008). OSPAR List of Threatened and/or Declining Species and Habitats. Reference Number: 2008–2006. Paris: OSPAR Commission.
Purser, A., Orejas, C., Gori, A., Tong, R., Unnithan, V., and Thomsen, L. (2013). Local variation in the distribution of benthic megafauna species associated with cold-water coral reefs on the Norwegian margin. Cont. Shelf Res. 54, 37–51. doi: 10.1016/j.csr.2012.12.013
Pusceddu, A., Bianchelli, S., Martín, J., Puig, P., Palanques, A., Masqué, P., et al. (2014). Chronic and intensive bottom trawling impairs deep-sea biodiversity and ecosystem functioning. Proc. Natl. Acad. Sci. 111, 8861–8866. doi: 10.1073/pnas.140545411
Rice, A. L., Thurston, M. H., and New, A. L. (1990). Dense aggregations of a hexactinellid sponge, Pheronema carpenteri, in the porcupine seabight (northeast Atlantic ocean), and possible causes. Prog. Oceanogr. 24, 179–196. doi: 10.1016/0079-6611(90)90029-2
Roberts, J. M., Harvey, S. M., Lamont, P. A., Gage, J. D., and Humphery, J. D. (2000). Seabed photography, environmental assessment and evidence for deep-water trawling on the continental margin west of the Hebrides. Hydrobiologia 441, 173–183. doi: 10.1023/A:1017550612340
Rooper, C. N., Sigler, M. F., Goddard, P., Malecha, P., Towler, R., Williams, K., et al. (2016). Validation and improvement of species distribution models for structure-forming invertebrates in the eastern bering sea with an independent survey. Mar. Ecol. Prog. Ser. 551, 117–130. doi: 10.3354/meps11703
Sherwin, T. J. (1991). “Evidence of a deep internal tide in the Faroe-Shetland Channel,” in Tidal Hydrodynamics, ed. B. B. Parker (New York, NY: John Wiley & Sons), 469–488.
Sherwin, T. J., Williams, M. O., Turrell, W. R., Hughes, S. L., and Miller, P. (2006). A description and analysis of mesoscale variability in the faroe-shetland channel. J. Geophys. Res. 111:C03003. doi: 10.1029/2005JC002867
Strand, R., Whalan, S., Webster, N. S., Kutti, T., Fang, J. K. H., Luter, H. M., et al. (2017). The response of a boreal deep-sea sponge holobiont to acute thermal stress. Sci. Rep. 7:1660. doi: 10.1038/s41598-017-01091-x
Sweetman, A. K., Thurber, A. R., Smith, C. R., Levin, L. A., Mora, C., Wei, C.-L., et al. (2017). Major impacts of climate change on deep-sea benthic ecosystems. Elem. Sci. Anthr. 5:4. doi: 10.1525/elementa.203
Tjensvoll, I., Kutti, T., Fosså, J. H., and Bannister, R. J. (2013). Rapid respiratory responses of the deep-water sponge Geodia barretti exposed to suspended sediments. Aquat. Biol. 19, 65–73. doi: 10.3354/ab00522
Turrell, W. R., Slesser, G., Adams, R. D., Payne, R., and Gillibrand, P. A. (1999). Decadal variability in the composition of faroe shetland channel bottom water. Deep Sea Res. Part I 46, 1–25. doi: 10.1016/S0967-0637(98)00067-3
Vad, J., Kazanidis, G., Henry, L.-A., Jones, D. O. B., Tendal, O. S., Christiansen, S., et al. (2018). Potential impacts of offshore oil and gas activities on deep-sea sponges and the habitats they form. Adv. Mar. Biol. 79, 33–60. doi: 10.1016/bs.amb.2018.01.001
Victorero, L., Watling, L., Deng Palomares, M. L., and Nouvian, C. (2018). Out of sight, but within reach: a global history of bottom-trawled deep-sea fisheries from 400 m depth. Front. Mar. Sci. 5:98. doi: 10.3389/fmars.2018.00098
Keywords: deep-sea sponges, marine protected area, fisheries, oil and gas, environmental health, climate change
Citation: Kazanidis G, Vad J, Henry L-A, Neat F, Berx B, Georgoulas K and Roberts JM (2019) Distribution of Deep-Sea Sponge Aggregations in an Area of Multisectoral Activities and Changing Oceanic Conditions. Front. Mar. Sci. 6:163. doi: 10.3389/fmars.2019.00163
Received: 16 November 2018; Accepted: 15 March 2019;
Published: 09 April 2019.
Edited by:
Cristina Gambi, Marche Polytechnic University, ItalyReviewed by:
Lorenzo Angeletti, Istituto di Scienze Marine (ISMAR), ItalyTina Molodtsova, P.P. Shirshov Institute of Oceanology (RAS), Russia
Copyright © 2019 Kazanidis, Vad, Henry, Neat, Berx, Georgoulas and Roberts. This is an open-access article distributed under the terms of the Creative Commons Attribution License (CC BY). The use, distribution or reproduction in other forums is permitted, provided the original author(s) and the copyright owner(s) are credited and that the original publication in this journal is cited, in accordance with accepted academic practice. No use, distribution or reproduction is permitted which does not comply with these terms.
*Correspondence: Georgios Kazanidis, Z2Vvcmdpb3Mua2F6YW5pZGlzQGVkLmFjLnVr J Murray Roberts, bXVycmF5LnJvYmVydHNAZWQuYWMudWs=