- 1Department of Evolutionary Ecology, Estación Biológica de Doñana, CSIC, Seville, Spain
- 2Marine Programme, SEO/BirdLife, Barcelona, Spain
- 3Centre d’Etudes Biologiques de Chizé, CNRS and Université de La Rochelle, Chizé, France
- 4BirdLife International, Cambridge, United Kingdom
- 5Marine and Environmental Sciences Centre (MARE), ISPA – Instituto Universitário, Lisbon, Portugal
- 6Island Conservation, Santa Cruz, CA, United States
- 7AZTI, Pasaia, Spain
- 8Department of Biology, Acadia University, Wolfville, NS, Canada
- 9Kaua’i Endangered Seabird Recovery Project, Hanapepe, HI, United States
- 10Departament de Biologia Evolutiva, Ecologia i Ciències Ambientals, Facultat de Biologia, Universitat de Barcelona, Barcelona, Spain
- 11Canary Islands’ Ornithology and Natural History Group (GOHNIC), Buenavista del Norte, Spain
- 12Canadian Wildlife Service, Environment and Climate Change Canada, Dartmouth, NS, Canada
- 13Biology Department, Queen’s University, Kingston, ON, Canada
- 14Biology Department, Trent University, Peterborough, ON, Canada
- 15Ecologie, Systématique et Evolution, Université Paris-Sud, CNRS, AgroParisTech, Université Paris-Saclay, Orsay, France
- 16David H. Smith Conservation Research Program, Society for Conservation Biology, Washington, DC, United States
- 17Norwegian Polar Institute, Fram Centre, Tromsø, Norway
- 18Theoretical and Computational Ecology Laboratory, Centre d’Estudis Avançats de Blanes, CSIC, Blanes, Spain
- 19Wildlife Research Division, Environment and Climate Change Canada, Mount Pearl, NL, Canada
- 20Oikonos Ecosystem Knowledge, Tacoma, WA, United States
- 21Black Bawks Data Science, Fort Augustus, United Kingdom
- 22UMR ENTROPIE, Université de la Réunion, Saint-Denis, France
- 23UMR Processus Infectieux en Milieu Insulaire Tropical, Université de la Réunion, Saint-Denis, France
- 24Washington Sea Grant, University of Washington, Seattle, WA, United States
- 25Psychology Department, Memorial University of Newfoundland, St. John’s, NL, Canada
- 26Parc National de la Réunion, LIFE+ Pétrels, Saint-Denis, France
- 27School of Biological Sciences, The University of Auckland, Auckland, New Zealand
- 28FitzPatrick Institute of African Ornithology, University of Cape Town, Cape Town, South Africa
- 29Animal Ecology and Demography Group, Instituto Mediterráneo de Estudios Avanzados, UIB-CSIC, Esporles, Spain
- 30Environment and Sustainability Institute, University of Exeter, Cornwall, United Kingdom
- 31Seabird Conservation Programme, BirdLife South Africa, Cape Town, South Africa
- 32Institute for Marine and Antarctic Studies, University of Tasmania, Hobart, TAS, Australia
- 33Conservation Department, Phillip Island Nature Parks, Cowes, VIC, Australia
- 34School of Biological Sciences, Monash University, Clayton, VIC, Australia
Shearwaters and petrels (hereafter petrels) are highly adapted seabirds that occur across all the world’s oceans. Petrels are a threatened seabird group comprising 124 species. They have bet-hedging life histories typified by extended chick rearing periods, low fecundity, high adult survival, strong philopatry, monogamy and long-term mate fidelity and are thus vulnerable to change. Anthropogenic alterations on land and at sea have led to a poor conservation status of many petrels with 52 (42%) threatened species based on IUCN criteria and 65 (52%) suffering population declines. Some species are well-studied, even being used as bioindicators of ocean health, yet for others there are major knowledge gaps regarding their breeding grounds, migratory areas or other key aspects of their biology and ecology. We assembled 38 petrel conservation researchers to summarize information regarding the most important threats according to the IUCN Red List of threatened species to identify knowledge gaps that must be filled to improve conservation and management of petrels. We highlight research advances on the main threats for petrels (invasive species at breeding grounds, bycatch, overfishing, light pollution, climate change, and pollution). We propose an ambitious goal to reverse at least some of these six main threats, through active efforts such as restoring island habitats (e.g., invasive species removal, control and prevention), improving policies and regulations at global and regional levels, and engaging local communities in conservation efforts.
Introduction
Humans have transformed ecosystems on an unprecedented global scale, driving a growing number of species to decline and extinction (Jenkins, 2003). The increasing human population living along coasts is putting a severe burden on marine and coastal environments through urban development, infrastructure for energy production and transport, fisheries, eutrophication, ocean acidification and invasion by alien species (Barnosky et al., 2016). Thus, it is not surprising that seabird populations have declined faster than other bird taxa during last decades (Croxall et al., 2012; Paleczny et al., 2015).
Seabirds are top predators and a significant component of marine ecosystems, making them key indicators of marine ecosystem functioning (including climate change). Changes and fluctuations in seabird population sizes, ranges, foraging ecology and breeding success have been used to detect environmental changes, document direct threats (e.g., poaching) and monitor success or failure of conservation management policies in protected areas and beyond (Lescroël et al., 2016; Dunlop, 2017).
The Order Procellariiformes (Class Aves) is one of the most endangered avian groups (Croxall et al., 2012) and the percentage of threatened species is higher than Aves overall (Figure 1). They are one of the most adapted groups of seabirds to the marine environment, traveling long distances and spending most of their lives over vast open oceans. In this review, we focus on the 124 species of three out of four families from the Order Procellariiformes: Procellaridae (petrels and shearwaters), Oceanitidae (southern storm-petrels), and Hydrobatidae (northern storm-petrels) (BirdLife International, 2018a), hereafter collectively referred to as “petrels.”
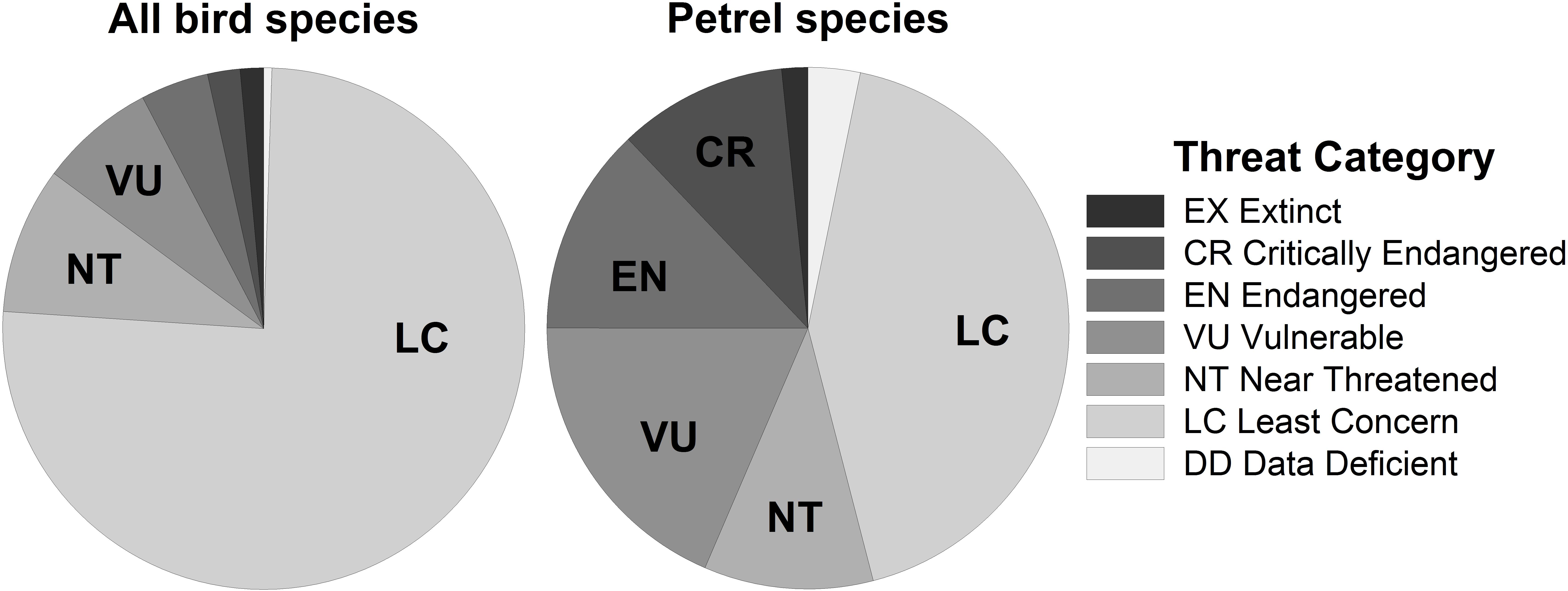
Figure 1. The percentage of species categorized by IUCN threat category for birds (left; n = 11,126 species) and for petrels (right; Procellariidae, Oceanitidae, and Hydrobatidae families; n = 124 species). Data from the IUCN Red List (BirdLife International, 2018a).
Petrels are colonial, nesting in cavities, crevices and burrows predominantly on isolated and inaccessible islands; most visit their colonies at night (Brooke, 2004a). These habits are thought to be mainly an attempt to avoid predation and piracy (Martin, 2017). These cryptic behaviors combined with their relative small size and high mobility at sea make petrels one of the most poorly known seabird groups, although some shearwater species are well studied. Petrels are perfect examples of slow species (Sæther and Bakke, 2000), exhibiting extended chick rearing periods, low fecundity (single egg clutch per breeding attempt), delayed maturity, long life spans, high adult survival, strong philopatry, monogamy, and long-term mate fidelity (Brooke, 2004a). They are highly adapted to exploit the marine environment and to cope with stable biological communities on breeding habitats (Brooke, 2004a). However, the exponential increase of the human population has resulted in stress and habitat transformation throughout natural petrel ecosystems. The poor conservation status of many petrel species (BirdLife International, 2018a), the importance of some as biodindicators (van Franeker and Law, 2015) and their regular role as keystone species in ecosystems (Brooke, 2004b) have resulted in many becoming flagships for research and conservation by both professional and citizen scientists.
Here, we take advantage of the experiences of seabird scientists working on petrels all over the world to review and synthesize threats that need to be addressed in future research, identify information gaps, and propose the most critical research needs to improve the conservation and management of petrels (including shearwaters, diving petrels, and storm-petrels).
Materials and Methods
We follow the taxonomy of the International Union for Conservation of Nature (IUCN) Red List of threatened species (BirdLife International, 2018a). We based this review on the 124 petrel species from three families of Order Procellariiformes: Procellariidae (including diving petrels Pelecanoides), Oceanitidae, and Hydrobatidae (BirdLife International, 2018a) and on the expertise of 38 petrel researchers from 34 institutions from 10 countries. We also used data from the BirdLife International Data Zone1 compiled and regularly updated by BirdLife Partners, scientists, ornithologists, conservationists and birdwatchers. Species are rated into the seven IUCN threat categories: Data Deficient (DD), Least Concern (LC), Near Threatened (NT), Vulnerable (VU), Endangered (EN), Critically Endangered (CR), and Extinct (EX). VU, EN, and CR species are referred to as threatened.
The assessment of threats followed the threat classification scheme of the IUCN (2012). This scheme defines threats as “the proximate human activities or processes that have impacted, are impacting, or may impact the status of the taxon being assessed. Direct threats are synonymous with sources of stress and proximate pressures” (IUCN, 2012). Each of the known threats were broken down into sections and written by groups of two to seven experts, showcasing impacts and potential solutions to the problems facing petrels worldwide. We subdivided the threat category “Biological resource use” into “bycatch” (stressor was “direct mortality by fisheries”), “overfishing” (stressor was “indirect ecosystem effects” of biological resource use, e.g., competition with fisheries or food depletion, and availability of fishery discards), and “human exploitation” (stressors were hunting, trapping, or harvesting). The category “Invasive and other problematic species, genes, and diseases” was partitioned into “invasive species,” “problematic native species,” and “diseases”; and “light pollution” was split from other forms of “pollution.” This gave a list of 12 threats: (1) invasive species, (2) light pollution, (3) bycatch, (4) human exploitation, (5) problematic native species, (6) climate change and severe weather, (7) residential and commercial development, (8) pollution, (9) disturbance, (10) energy production and mining, (11) overfishing, and (12) diseases. These threats were ordered in the text by the number of species affected according to the BirdLife International database (Figure 2). Some sections may overlap slightly given the multiple impacts of some activities. BirdLife International staff (led by MPD and RM) assessed (1) the timing of each threat (i.e., ongoing; past, likely to return; past, unlikely to return; future); (2) threat extent or scope (i.e., the proportion of the total population affected: minority; majority; whole); and (3) threat severity (i.e., the rate of population decline caused by the threat within its scope: Very rapid declines; Rapid declines; Slow, significant declines; Negligible declines; Causing/Could cause fluctuations) (IUCN, 2012). Finally, we classified for each species the impact of each ongoing threat in four levels based on scope and severity, from “very high” to “low” (Table 1; Garnett et al., 2018). In addition, we comment on two aspects we consider crucial for petrel conservation: improved understanding of petrel biology and ecology, and the role of an accurate taxonomy to develop taxon lists for conservation.
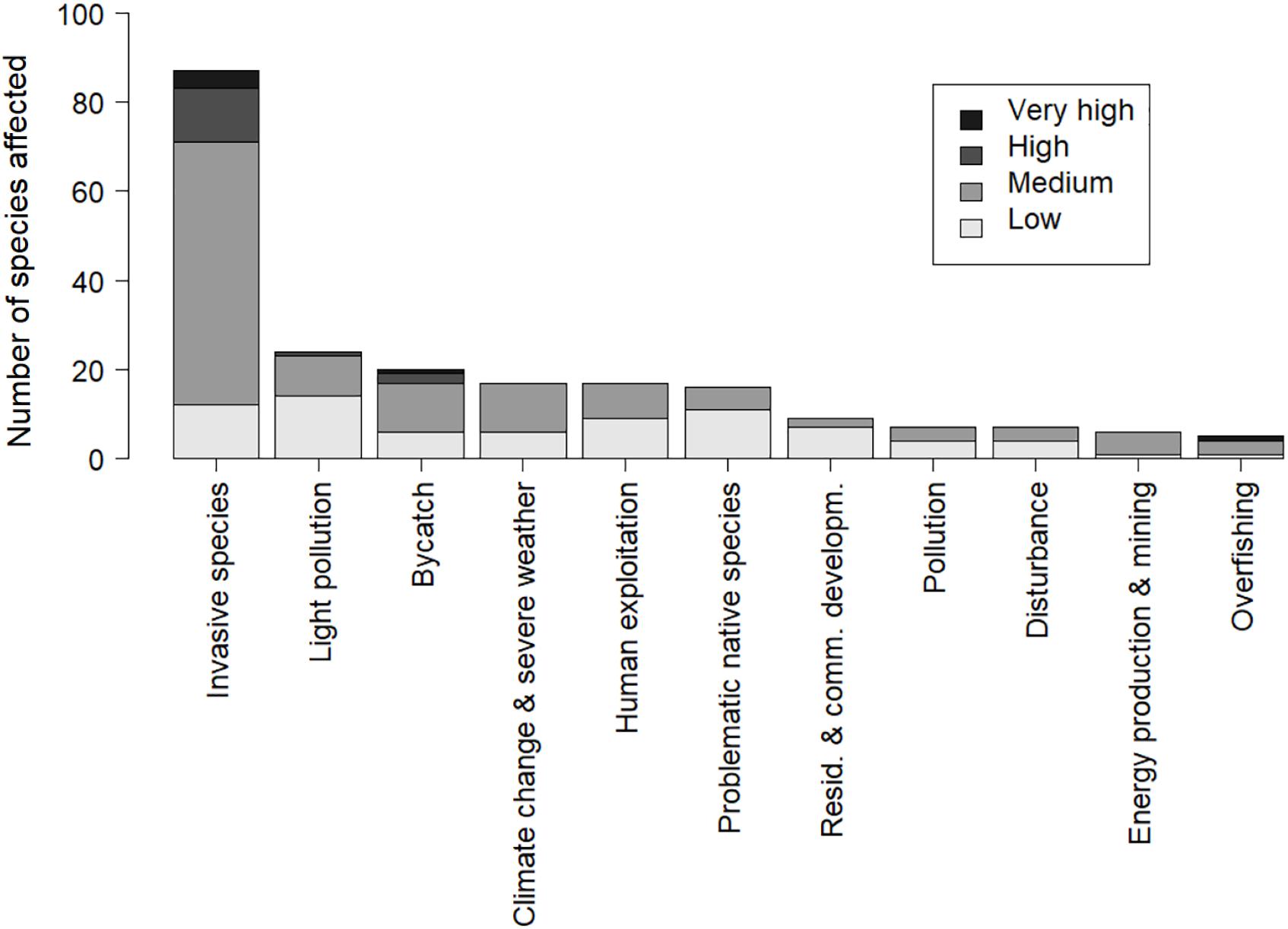
Figure 2. Threats to petrels sorted by the number of species affected according to BirdLife International (2018a).
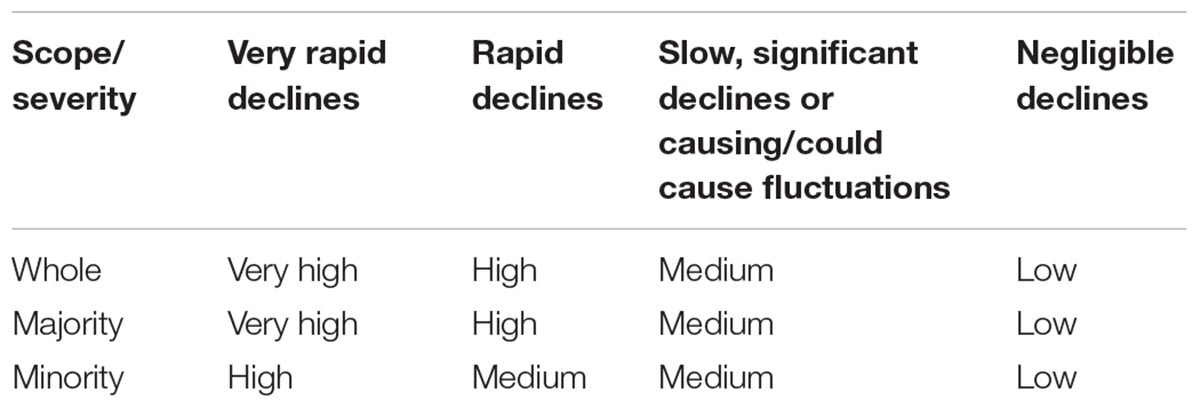
Table 1. Impact scoring system of each threat for species following the methodology of Garnett et al. (2018).
Invasive Species
Invasive species are non-native organisms whose introduction causes significant environmental harm. Invasive mammals are the most harmful of all threats to petrels (Figure 2). For some species, this threat is ongoing, high in scope and severity, and causing very rapid population declines, affecting several species across their entire range. Invasive mammals impact at least 78 petrel species, a critical contributing factor in all four species classified as extinct or possibly extinct since 1500 (Large St Helena Petrel Pterodroma rupinarum, Small St Helena Petrel Bulweria bifax, Jamaican Petrel P. caribbaea, and Guadalupe Storm-Petrel Hydrobates macrodactylus) (BirdLife International, 2018a). Invasive mammals are present on 171 (55%) of the 313 breeding islands of the 42 species classified as threatened on the IUCN Red List and 22 of those species occur with invasive mammals across their entire breeding range (Spatz et al., 2014).
Predation by invasive mammals – including by mice, rats, cats, pigs, and dogs – is a crucial threat, mainly where adult mortality occurs, driving colony extirpations, population declines and ultimately a higher risk of extinction. Rats (Rattus norvegicus, R. rattus, and R. exulans) are the most widespread invasive species affecting petrels (Figure 3), estimated to occur on 80% of the world’s island groups (Atkinson, 1985). Rats can prey on eggs, chicks, and adults, the relative severity depending on the size classes of the petrel. Smaller burrow-nesting species (<300 g) are most vulnerable (Jones et al., 2008), putting them at high risk of extinction. House mice Mus musculus have only recently been recognized as significant seabird predators – mainly of chicks and seldom adults – typically on islands where they are the only invasive mammal (Wanless et al., 2007; Caravaggi et al., 2018). Mice currently threaten at least six endangered or critically endangered petrel species. Cats Felis catus, both feral and free-ranging, or domestic and subsidized by humans, can also be significant predators of adult seabirds and chicks, including multiple threatened species (Bonnaud et al., 2011; Figure 3).
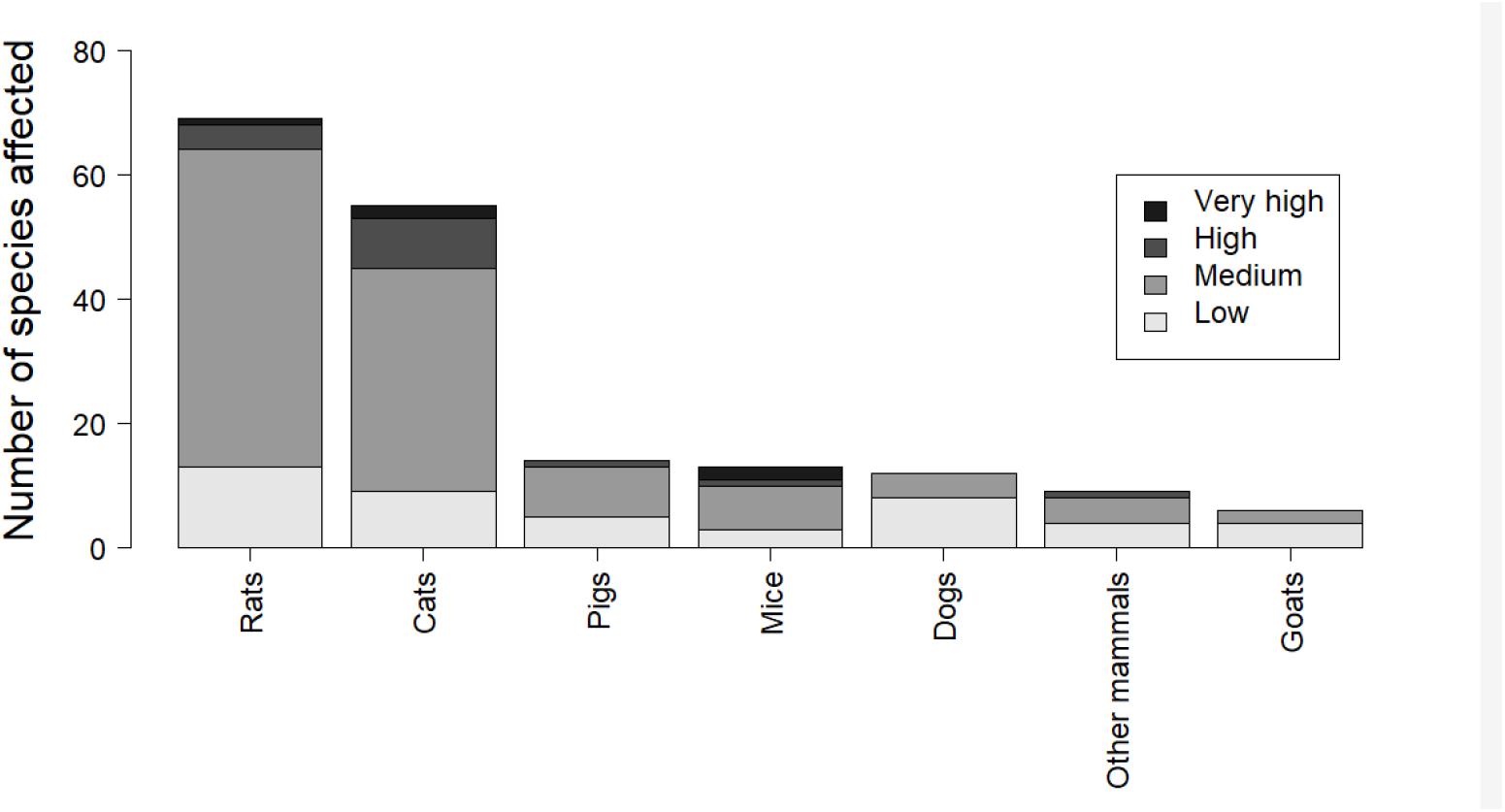
Figure 3. Number of petrel species (Procellariidae, Oceanitidae, and Hydrobatidae families) affected by introduced mammalian species according to BirdLife International database.
Introduced herbivores, including lagomorphs and ungulates, represent a threat primarily through destruction of breeding habitat, including alteration or trampling of burrows, compaction of soil, loss of vegetation leading to substrate instability and erosion (both of which can cause mortality for birds in burrows), or competition with petrels for burrows (Brodier et al., 2011; Shaw et al., 2011). Ungulates and pigs can also depredate petrels (Furness, 1988; Madeiros et al., 2012).
Invasive invertebrates, plants, and birds can also present threats to petrel populations. Invasive tramp ants can be particularly damaging, e.g., yellow crazy ants Anoplolepis gracilipes on islets off Oahu leading to Wedge-tailed Shearwater Ardenna pacifica nest abandonment, increased risk of chick mortality and ultimately colony decline. Invasive plants can threaten breeding habitat by changing vegetation structure, limiting access to burrows, or entangling individuals leading to injury or death, e.g., strawberry guavas Psidium cattleyanum impacting Hawaiian Petrels P. sandwichensis (VanZandt et al., 2014). Raptors introduced to islands have contributed to non-native predation and mortality, such as Masked Owl Tyto novaehollandiae predation on Little Shearwaters P. assimilis and Black-winged Petrels Pterodroma nigripennis on Lord Howe Island (Milledge, 2010), and Barn Owls Tyto furcata on multiple petrel species in Hawaii (Raine et al., 2017).
Invasive species can also induce indirect threats on petrels by affecting island ecosystems, including changes in community composition or trophic interactions among introduced and native species (Russell, 2011). An example is the case of invasive mice, overwintering Burrowing Owls Athene cunicularia and threatened Ashy Storm-Petrels Hydrobates homochroa on the Farallon Islands.
One of the most effective conservation actions has been the eradication of invasive species from islands. Worldwide, eradications have been attempted on more than 1200 islands with a success rate of 85%, thereby eliminating critical threats provided the islands remain free of threatening invasives (Jones et al., 2016). A review of 151 populations of 69 seabird species, including 28 petrel species, found a positive annual population growth rate (λ) of 1.119 (1.161 for petrels) after successful eradication (Brooke et al., 2018). The growth rate for many petrel populations is faster than the biological limit, indicating that recruitment of new breeders to these islands may play a key role in the re-establishment of some populations after an eradication (Harper, 1983; Smith et al., 2006; Bourgeois et al., 2013). Some important petrel breeding islands are not considered technically or socio-politically feasible to implement eradications due to their size or human population. Alternative conservation strategies to mitigate invasive mammals include sub-island actions such as predator-proof fencing to create predator-free environments, and localized control to reduce but not eliminate threats (Spatz et al., 2017).
The most critical future action remains to tackle the threat of invasive mammals, coupled with improved biosecurity for pest-free islands (Spatz et al., 2014). In many jurisdictions this requires strengthening of legislation and adequate resource allocation for monitoring and enforcement. Eradicating invasive mammals wherever technically feasible is key. Many suitable islands occur in countries and territories with limited precedent (e.g., Peru), requiring partnerships with land management agencies to undertake trial projects, and establish appropriate strategies (Russell et al., 2017a).
Eradicating and controlling invasive mammals from human-settled islands is a critical new conservation frontier for protecting several globally threatened species (e.g., Isla Floreana to protect Galapagos Petrel Pterodroma phaeopygia). This requires consideration of new technical challenges, such as commensal food waste, ensuring safe water supply, etc. As a result, strong local partnerships are required to understand social acceptability and alignment with community goals (Glen et al., 2013; Russell et al., 2018). Continued research and application of other restoration tools, including predator-proof fencing and reintroductions, invasive plant management, habitat restoration, and artificial nest construction, are required to protect remnant colonies on islands, and to achieve gains in efficacy and efficiency of pest control (Kappes and Jones, 2014). Improved reporting of conservation outcomes and knowledge transfer among seabird practitioners remains a key recommendation, including workshops, exchange programs, online databases and reporting in open access media. Investment in transformative innovations are also required (Campbell et al., 2015), that may allow unparalleled conservation goals such as Predator Free New Zealand (Russell et al., 2015).
Light Pollution
The use of artificial light at night, and the consequent increasing light pollution, is a major threat to biodiversity worldwide (Kyba et al., 2017). Light attraction and disorientation is a very well-documented behavior of petrels across the world, including coastal and insular communities (Reed et al., 1985; Troy et al., 2013; Rodríguez et al., 2015b), ships at sea (Glass and Ryan, 2013), and oil platforms (Montevecchi, 2006; Ronconi et al., 2015), with fog and rainy conditions exacerbating impacts. The life history of most petrel species includes underground nesting (95% of species), nocturnal flight around the colony, and underwater diving, with physiological and behavioral adaptation to low-light conditions. Artificial lights can confuse them, resulting in injury or mortality via collision with structures or the ground, or becoming “grounded.” Grounded birds are unlikely to be able to regain flight, and unless rescued, typically die from dehydration or starvation, or are killed by cats and dogs or run over by cars (Podolskyi et al., 1998; Rodríguez et al., 2012b, 2017c; Deppe et al., 2017). Impacts are particularly evident on islands with human communities, with fledglings affected during maiden flights to sea, especially during darker moon phases (Telfer et al., 1987; Le Corre et al., 2002; Rodríguez and Rodríguez, 2009; Fontaine et al., 2011; Rodríguez et al., 2014, 2015a).
Petrels are among the most at-risk seabirds from light pollution; at least 56 petrel species are affected, including 24 globally threatened species (Rodríguez et al., 2017b). In many cases, the light attraction is implicated in the long-term decline of petrel populations and can result in the grounding of more than thousands of individuals annually (Rodríguez et al., 2012c; Gineste et al., 2016; Raine et al., 2017). The increase in the number of grounded birds is often linked to an increase in light pollution levels of coastal areas (Rodrigues et al., 2012; Troy et al., 2013). Artificial light can not only affect birds breeding nearby but also birds flying over lighted areas to colonies many kilometers away, and even birds that have successfully fledged can be drawn back in from the sea (Montevecchi, 2006; Rodríguez et al., 2014, 2015b; Syposz et al., 2018).
Conservation actions include avoidance (turn off lights, part night lighting) and minimization (limit number of lights, shield lights, and prevent skyward light spill) during fledging periods in high-risk areas (Reed et al., 1985). Rescue campaigns recover a proportion of affected fledglings each year, though there is little data on post-release survivorship. Priority actions for future research include (1) testing avoidance and minimization measures at affected sites via education, light ordinance and enforcement, (2) investigating light characteristics (e.g., spectra and intensity) to reduce threat (Reed, 1986; Rodríguez et al., 2017a; Longcore et al., 2018), and (3) documenting rescued bird fate to assess the merit and effectiveness of rescue programs.
Bycatch
Fisheries bycatch (incidental mortality of non-target organisms in fishing gear) causes more than 500,000 seabird deaths annually, although this is expected to be a gross underestimate due to poor reporting rates from many fisheries (Žydelis et al., 2013). Procellariiformes are among the most caught groups, particularly albatrosses, but medium-size and large petrels also are affected, including several threatened species (Anderson et al., 2011; Žydelis et al., 2013). Diving abilities, foraging and social behavior, species size, and prey preferences are among the main factors influencing bycatch risk. Medium to large species foraging gregariously appear to be the most widely affected. The type of gear and the particularities of each fishery are also critical (Lewison et al., 2014). Demersal longline fisheries, which use relatively small hooks and bait, may be particularly dangerous for medium-sized petrels (Laneri et al., 2010; Cortés et al., 2017), while pelagic longline fisheries also cause extensive bycatch, mainly affecting medium-large-sized petrels (e.g., Macronectes and Procellaria petrels) that swallow the larger hooks and bait (Anderson et al., 2011; Yeh et al., 2013). Birds tend to get hooked or entangled during line setting, when the line is sinking, with the risk of bycatch increasing for deep diving species, as they can reach the bait at greater depths farther from the vessel. Diving species can facilitate access to non-diving seabirds, including petrels, by retrieving baited hooks to the surface from depth (Jiménez et al., 2012; Melvin et al., 2013, 2014). Gillnets can also cause entanglements and mortality by drowning. This gear type mainly affects proficient diving seabirds such as seaducks, cormorants and auks, but some diving or scavenging petrels are also caught incidentally (Žydelis et al., 2013). In trawl fisheries, petrels mostly collide with cables attached to the trawl net, while proficient divers (e.g., Puffinus and Ardenna shearwaters) may also be caught in the net during setting or hauling (González-Zevallos and Yorio, 2006; Sullivan et al., 2006, 2018; Maree et al., 2014). However, mortalities from cable collisions are difficult to quantify, leading to underestimates. Finally, there is increasing evidence of shearwater bycatch by purse-seine fisheries, particularly those targeting small pelagic fish. When birds are attracted to the shoaling fish, they can be injured or drowned during the closing and hauling of the net. Purse-seine bycatch has been reported with proficient divers such as the Balearic Shearwater Puffinus mauretanicus in Portugal, the Pink-footed Shearwater Ardenna creatopus in Chile, and the Flesh-flooted Shearwater Ardenna carneipes in Australia (Oliveira et al., 2015; Baker and Hamilton, 2016; Suazo et al., 2017).
Estimates of bycatch scale up to the thousands for some petrels, but population level effects are not as well-known as for albatrosses. Because petrels are long-lived species and their population dynamics are sensitive to changes in adult survival, petrel bycatch must be regarded as a severe threat (as for albatrosses). Recent evidence from the Mediterranean indicates that bycatch is the main factor driving the decline of the critically endangered Balearic Shearwater (45% of adult mortality) (Genovart et al., 2016). Bycatch also has a strong negative impact on Calonectris shearwaters demography, while adult survival is negatively related to exposure to bycatch risk (Ramos et al., 2012; Genovart et al., 2017, 2018). On the other hand, bycatch may differentially affect individuals within populations. Some studies show biases between sexes or age classes (Gianuca et al., 2017; Cortés et al., 2018), and behavioral traits of individual birds might also influence bycatch risk (Patrick and Weimerskirch, 2014; Tuck et al., 2015).
Seabird bycatch can be significantly reduced by applying operational and/or technical mitigation measures, some of which can be applied to multiple gear types (ACAP, 2014). A widely used operational measure is avoiding offal discharge or any discards during setting and hauling operations to avoid attracting scavenging seabirds. Night setting could reduce the bycatch of species that mainly forage by day (Barry Baker and Wise, 2005; Cortés and González-Solís, 2018), although many petrels present varying degrees of nocturnal foraging (Dias et al., 2012). Technical measures such as bird scaring lines (tori or streamer lines) and fast-sinking longlines also reduce bycatch significantly (ACAP, 2017). Bird-scaring lines, widely used in longline and trawl fisheries, exclude birds from the area astern of the vessel where bycatch risk is greatest. Fast sinking longlines (with attached or integrated weights) limit the time and distance astern that birds can access baited hooks. An extreme case is that of Chile, where demersal longlines were changed to a heavily weighted vertical longline configuration, which reduced bycatch to practically zero (Moreno et al., 2008). For pelagic longlines, hook-shielding devices have proved effective (Sullivan et al., 2018). Gillnet bycatch poses the greatest challenge at present, as few proven technical or operational measures have been identified. So far acoustic deterrents (pingers), visual panels and LEDs have been tested with varying results (Martin and Crawford, 2015; Mangel et al., 2018).
A collaborative approach involving fishermen is essential to mitigate bycatch. Their experience is highly valuable to develop practical and effective mitigation measures, and their acceptance is crucial for successful implementation (Wanless and Maree, 2014). Best practice mitigation measures should be safe, simple, easy to implement, technically feasible, cost-effective, and where possible should not reduce fishing profits (ACAP, 2014, 2017; Rouxel and Montevecchi, 2018). Moreover, such measures should not increase the bycatch of other marine biota. Experiences such as those of the Commission for the Conservation of Antarctic Marine Living Resources (CCAMLR) show how the problem may be tackled effectively with minimal effects in the fishing activity. Implementation of mandatory technical mitigation measures and seasonal closures over the past two decades reduced seabird bycatch from several thousands to tens of seabirds annually in most CCAMLR longline fisheries (Waugh et al., 2008).
Despite the attention that seabird bycatch has received in recent decades, there are still several knowledge gaps to address (Kirby and Ward, 2014). First, information on the extent of bycatch remains patchy due to the absence of onboard observers or data collection protocols in most fisheries. This gap is greater in artisanal fisheries, where a large number of vessels and the diversity of practices make systematic data gathering extremely difficult (Lewison et al., 2014). Improved observer program coverage and protocols, combined with self-reporting protocols and electronic monitoring (e.g., video monitoring) are critical to solving bycatch. Second, few studies are underway to explore the link between bycatch and petrel demography, which is a necessary step to understand the problem and to take the most appropriate conservation actions. Monitoring programs on the breeding performance of affected species should be promoted, as should monitoring initiatives to collect information on dead birds to assess bycatch mortality relative to other causes of mortality, as well as to understand how different components of the population are affected. Third, the adoption of mitigation measures requires raising awareness and building trust among fishermen to develop the most appropriate solution on a case-by-case basis. Developing toolkits of proven measures, from which fishermen choose the most appropriate combination for them, could be the most direct path to reduction of petrel mortality in world fisheries (Melvin et al., 2019).
Human Exploitation
Humans have been hunting petrels for food for centuries (Anderson, 1996), with devastating consequences for some species (e.g., Rando and Alcover, 2008). Breeding petrels are particularly vulnerable to harvesting as they typically breed synchronously and in large colonies, where adults, eggs, and chicks can easily be accessed (Hunter et al., 2000). Many petrels were also deliberately caught at sea for human consumption, and this practice continues in some areas. Although most hunting practices have ended, either due to regulation or the extinction of the species, some species may remain at risk.
Species such as the Short-tailed Ardenna tenuirostris and Sooty Shearwaters A. grisea are nowadays harvested. Approximately 360,000 ± 40,000 Sooty Shearwater chicks are estimated to be taken annually around Stewart Island/Rakiura, New Zealand, for commercial or personal use (Newman et al., 2009). Harvesting of Short-tailed Shearwater is managed for ‘recreational’ (private) and ‘commercial’ (indigenous) exploitation in Tasmania, with 52,000 ± 13,400 chicks taken annually for recreational use in the 2009–2017 period (DPIPWE, 2014, 2018). There are no public data on indigenous harvest, and the extent of chick poaching is unknown (Skira et al., 1996).
In the Atlantic Ocean, harvesting of petrels has occurred for generations at the Azores, Canary Islands and Selvagem Grande (Granadeiro et al., 2006; Lopez-Darias et al., 2011) and at the Tristan islands (Richardson, 1984), although currently only Great Shearwaters Ardenna gravis can be legally exploited. In the northeast Atlantic, the hunt was historically focused on the most abundant species, Cory’s Shearwater Calonectris borealis, and direct persecution may have been the major cause of a 90% reduction in the population size until the 1970s, when hunting was banned (Granadeiro et al., 2006; Lopez-Darias et al., 2011). Illegal hunting of this species still occurs on the Canary Islands to an unknown extent (Lopez-Darias et al., 2011). Unquantified numbers of Great and Sooty Shearwaters along the Atlantic coast of Canada and of Scopoli’s and Yelkouan Shearwaters Puffinus yelkouan in Malta are also illegally shot from boats (Merkel and Barry, 2008; BirdLife International, 2018a).
Regulations on quotas, as those from New Zealand, Australia, or the Arctic, have probably helped to reduce extinction risk. Future research must prioritize quantifying the impact of poaching relative to other threats, particularly on islands with small populations.
Problematic Native Species
Under natural conditions, interactions between native species and petrels should not be a conservation challenge. However, anthropogenic impacts can alter native species populations leading to effects of conservation concern. The best documented interactions types are predation on, competition with, or modification of the nesting habitat of petrels. Problematic native species have been mentioned as potential or real threats for about 20 petrel species (16 out of them threatened; BirdLife International, 2018a).
Like invasive species, native species can prey on petrels. Populations of several gull species (Larus spp.) are increasing due to anthropogenic factors (e.g., food supplementation), leading to higher predation pressure on petrels and their eggs and chicks (Vidal et al., 1998). For endangered Ashy Storm-Petrels, the Western Gull Larus occidentalis seems to be at least partly responsible for keeping numbers low at South Farallon, Santa Barbara, and Anacapa Islands (Ainley, 1995; Chandler et al., 2016). Heavy predation pressure by large Larus gulls is thought to be contributing to declines of some Leach’s Storm-Petrel populations in Atlantic Canada (Robertson et al., 2006). Paradoxically, however, regional populations of large gulls have also declined over the last several decades coincident with fishery closures and consequent removal of discards (Regular et al., 2013). In the Azores, some adults of the vulnerable Monteiro’s Storm-Petrel Hydrobates monteiroi are killed by resident Yellow-legged Gulls Larus michahellis (Oliveira, 2016). This gull species also preys on vulnerable Deserta Petrels Pterodroma deserta (IUCN, 2018a). Some individual gulls specialize in feeding on petrels. On Selvagem Islands, where its declaration as a reserve has led to increases in petrel numbers (see Human exploitation), a small gull colony (<20 pairs) consumes up to five petrel species (60% of the consumed biomass; Matias and Catry, 2010). Breeding habitat transformations can also lead to changes in interspecific interactions including predation. In the Mediterranean, the Yellow-legged Gull preys on European Storm-Petrels Hydrobates pelagicus at higher rates at sites illuminated by nearby coastal cities than sites facing the sea, i.e., unaffected by coastal light pollution (Oro et al., 2005). In this instance, after the selective culling of 16 specialized gull individuals, storm-petrel survival and breeding success increased by 16 and 23%, respectively (Sanz-Aguilar et al., 2009). Another case of predation by native species on petrels regulated by anthropogenic factors is from Farallon Islands, where the presence of invasive mice provides a reliable food source for migrating Burrowing Owls during autumn, which may encourage more owls to overwinter. When mouse populations crash in winter, Burrowing Owls switch prey to globally threatened Ashy Storm-Petrels (Chandler et al., 2016).
The predation conducted by native or vagrant owls is concerning for some fragile small populations of the smallest petrel species. For example, resident Long-eared Owls Asio otus kill up to 40 adult Monteiro’s Storm-petrels per year, which has a population of 295–999 mature individuals (Bolton et al., 2008; BirdLife International, 2018a). Extreme cases are associated with vagrant individuals such as a single Snowy Owl Bubo scandiacus which consumed 5% of endangered Bermuda Petrels Pterodroma cahow before it was eradicated (Madeiros et al., 2012; BirdLife International, 2018a), a Short-Eared Owl Asio flammeus which fed almost exclusively on Monteiro’s Storm-Petrels killing 1–2 individuals per night during 2 weeks on Praia islet, Azores (Bried, 2003) or vagrant falcons Falco spp. which could extinguish the threatened population of Macgillivray’s Prions Pachyptila macgillivrayi at Saint Paul Island (Jiguet et al., 2007).
Competition among seabirds for nesting habitat is another threat. Although the particular nesting habitat preferences of each petrel species helps to avoid burrow competition (Bourgeois and Vidal, 2007; Troy et al., 2016), some species share nesting habitat and fiercely compete for nesting sites. In such cases, larger species tend to monopolize available burrows (Ramos et al., 1997; Sullivan et al., 2000). Several storm-petrel species have reduced breeding success because of the presence of larger petrels (McClelland et al., 2008; Sato et al., 2010), and Tahiti Pseudobulweria rostrata and Providence Petrel Pterodroma solandri chicks are killed or ejected from burrows by Wedge-tailed Shearwaters A. pacifica (Villard et al., 2006; Priddel et al., 2010). The recovery of the Bermuda Petrel was initially hampered by competition from White-tailed Tropicbirds Phaethon lepturus for nest-sites until baffles were built in front of burrows (Madeiros et al., 2012; BirdLife International, 2018a) and Little Penguins occasionally kill Gray-faced Petrels (Friesen et al., 2016).
Native non-seabird species can modify the nesting habitat of petrels such as soil erosion by expanding populations of Antarctic fur seal Arctocephalus gazella in the breeding habitat of the White-chinned Petrels Procellaria aequinoctialis at South Georgia (Berrow et al., 2000).
Much of the available information on direct predation or competition for burrows by native species is anecdotal, with scant information for most species. Current evidence suggests that native species have little effect on breeding success, except for some highly threatened species (Gummer et al., 2015) or in some exceptional circumstances under unnatural conditions (Pierce, 1998). Identifying problematic species and assessing their demographic impacts on petrels is a priority. Until such information is obtained and following a precautionary principle, some measures with low impact to other species could be taken. For example, burrow competition and predation could be reduced for small petrels by installing artificial nests that exclude larger species (Libois et al., 2012; Gummer et al., 2015). This action has increased productivity of Monteiro’s and European Storm-Petrels (Bolton et al., 2004; Libois et al., 2012). For species returning later than competitors to breeding grounds, blocking burrows early in the season could ensure availability of burrows to threatened species that return later to shared colonies (Gardner and Wilson, 1999).
Climate Change and Severe Weather
The effects of climate change on petrels involve multiple pathways and can be direct, through increases in thermoregulation costs or loss of habitat, or indirect, through changes in the structure and functioning of marine (and terrestrial) ecosystems ultimately affecting food availability (Ramírez et al., 2016) and predation (McClelland et al., 2018).
Increasing sea or air temperatures have generally detrimental consequences for petrels (Figure 4). Warm temperatures are often associated with poorer body condition (Peck et al., 2004; Connan et al., 2008; Riou et al., 2011) and lower breeding success (Inchausti et al., 2003; Nevoux and Barbraud, 2006). Negative effects on survival and population size have also been reported (Veit et al., 1997; Barbraud and Weimerskirch, 2003; Jenouvrier et al., 2003). However, some studies have reported positive relationships between increasing temperatures and petrel vital rates or abundance (Thompson and Ollason, 2001; Jenouvrier et al., 2005; Slater and Byrd, 2009; Soldatini et al., 2014). The effects of warming temperature are often assessed using large-scale climate indices (Supplementary Material). In such cases, the relationships between changes in these large-scale indices and local temperatures are not always straightforward (Descamps et al., 2016), making their interpretation difficult (Oro, 2014). Sea-ice loss in polar environments is a direct consequence of climate warming that has important consequences for polar ecosystems (Post et al., 2013; Descamps et al., 2017; Ramírez et al., 2017b). The effects of sea-ice decline on petrels appear quite different than those of increasing temperatures (Supplementary Material). Overall, the consequences of climate warming on petrels are complex and can vary among species and even populations within species. They may be a function of, for example, the life-history trait (Barbraud and Weimerskirch, 2001), the period of the breeding cycle (Jenouvrier et al., 2005; Olivier et al., 2005; Waugh et al., 2015) or the rate of warming (Mauck et al., 2018).
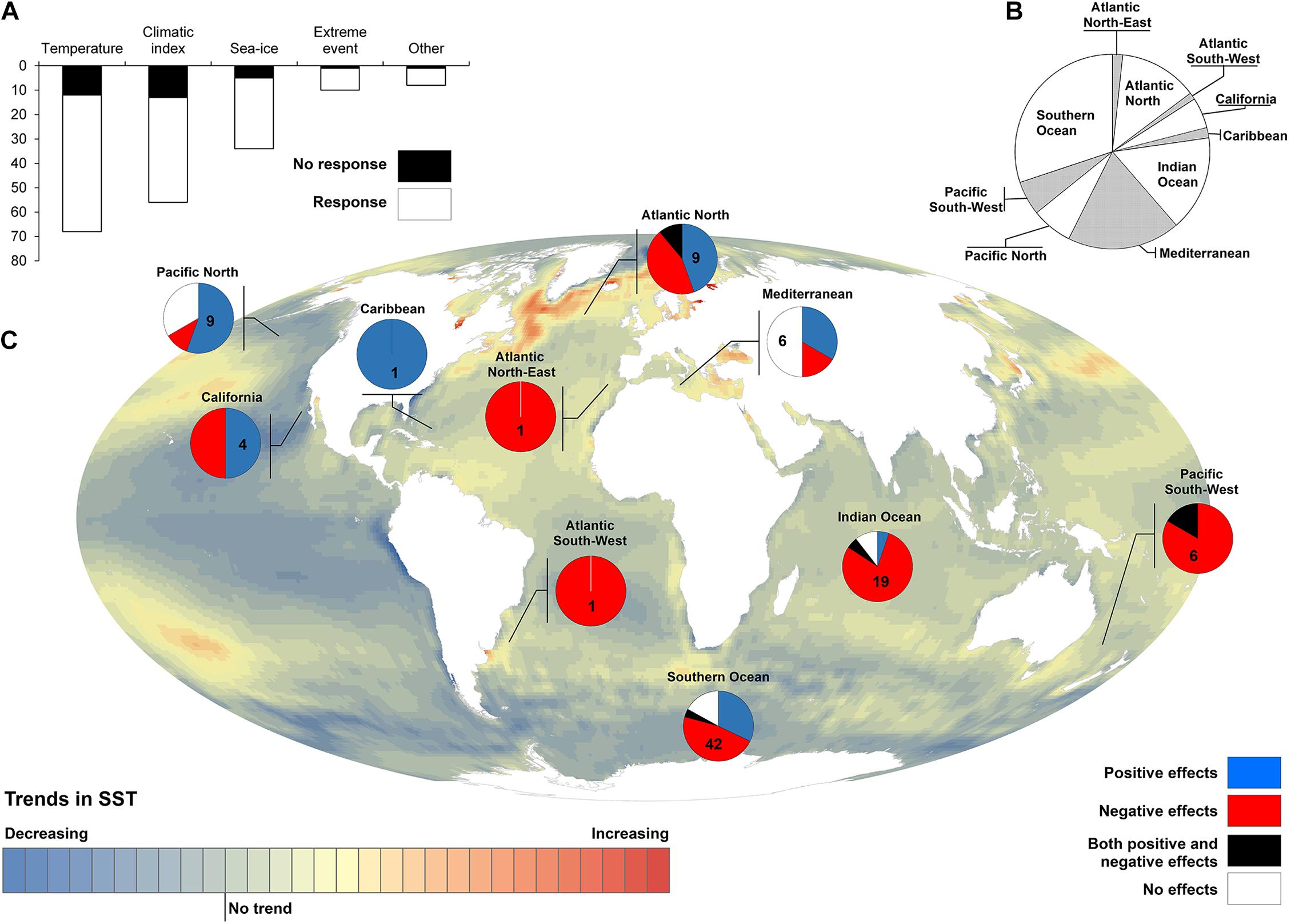
Figure 4. Reported seabird responses to climate driven environmental changes. We compiled 64 studies (Supplementary Material) reporting up to 176 cases in which petrel and shearwater species responded (any kind of response, 144) or not (32) to environmental features such as air or sea temperatures, large-scale climatic indexes, sea-level or extreme weather events (A). These responses were grouped geographically to elucidate the spatial heterogeneity in the distribution of reported responses (B). Responses that could be directly interpreted as a benefit or a threat for seabirds (e.g., changes in breeding performance or survival rates; but not in breeding phenology or distribution) when facing climate driven environmental changes (e.g., increasing temperatures and extreme weather events or decreasing extents of sea-ice; but not large-scale climatic indices) were selected and grouped geographically to show the spatially explicit effects of climate change on petrel and shearwater populations; colored background represents the pixel-basis long-term (1983–2014) trend in sea surface temperature (SST) (Ramírez et al., 2017a) (C).
Increasing extreme events represent one of the most imposing aspects of climate change that can affect petrels (Jentsch et al., 2007). Few studies have quantified their impacts on seabird individuals and populations but it seems clear that extreme events like snow storms or hurricanes strongly affect the survival, reproduction and movements of petrels (Franeker et al., 2001; Quillfeldt, 2001; Descamps et al., 2015). Some of these impacts may be exacerbated by ongoing sea-level rise for petrels that nest in low lying coastal zones. Rising sea level will reduce the availability of suitable breeding sites (Reynolds et al., 2015) that could also be susceptible to flooding associated with storms and hurricanes (Cadiou et al., 2010; Madeiros et al., 2012). In fact, 17 threatened petrel species are at high or medium risk of inundation due to sea level rise at their breeding sites (Spatz et al., 2017).
Ocean warming and acidification can affect trophic pathways from primary producers to upper-trophic levels through simplification of marine communities or changes in the abundance of prey (Burrows et al., 2011; García Molinos et al., 2015). Such climate-driven changes in seabirds’ diets, and their ultimate consequences on population parameters (e.g., breeding performance) are very often assumed but rarely confirmed (Connan et al., 2008).
Studies of the impact of climate change on seabirds are geographically biased toward higher latitudes (Poloczanska et al., 2016; Keogan et al., 2018; see also Figure 4 and Supplementary Material) and little is known about tropical species. Even in polar regions, there is a clear bias toward specific areas (e.g., Adélie Land in Antarctica) that are not necessarily the ones where climate change has been the most pronounced. Further studies should also address the role of intrinsic sources of variation in petrel responses to climate change such as age, sex, breeding state, or physical condition (Oro, 2014). Together with an understanding of the at-sea ecology and spatial distribution of seabirds (particularly for the smaller or rarer species), there is an urgent need to unravel the mechanisms linking climate and vital rates to identify the right spatial and temporal scale of climate effects and their link with changes in food availability. Acknowledging the non-linearity in climate change effects, their potential long-lasting consequences (i.e., carry-over effects) and their interactions with additional stressors (Darling and Côté, 2008; Montoya et al., 2017) is also necessary. Maintaining and promoting long-term studies is key to fill most of these knowledge gaps.
Climate change seems unlikely that it will stop or slow down in the coming decades. However, even if there is no real conservation measure to dampen the effects of climate change at the scale of a species or population, the reduction of human stressors operating locally (e.g., bycatch, invasive species or human-induced loss of breeding habitats) has been proposed as a more achievable way of retaining ecosystems within a “safe operating space” (Sensu; Rockström et al., 2009), where they remain resilient to ongoing climate change (Roberts et al., 2017; Ramírez et al., 2018).
Residential and Commercial Development
As human population continues to increase, land conversion can seriously impact numerous petrel species. The most obvious threat is the direct destruction of breeding habitat, particularly in coastal areas. Coastal habitats are desirable locations for residential communities, are more densely populated, and are developed at a faster rate than hinterland areas (Small and Nicholls, 2003; Neumann et al., 2015). These coastal sites also provide critical nesting habitat for many petrels. Urbanization can completely destroy colonies – either directly killing nesting birds in the process or significantly reducing breeding ranges. Breeding habitat also can be degraded or disturbed simply by being in close proximity to housing and commercial units and their associated artificial lighting, resulting in reduced reproductive success and lower survival rates.
Development also requires significant infrastructure; road systems, power plants, powerline networks, wind farms, oil platforms, communication towers, which leads to increased light pollution and noise pollution. Powerline networks and other tall structures increase collision risk – particularly if these span flyways from the sea to breeding colonies. Nocturnal seabirds are particularly at risk, as powerlines are difficult to detect at night. This is a poorly studied problem, but the impacts of powerline collisions on seabirds can be high enough to impact at a population-level (Raine et al., 2017). Often this is a hidden impact, as dead birds can be very hard to find, particularly as the terrain under lines is often not realistically searchable, may be scavenged and may not die immediately under the lines but could die of injuries kilometers away (Costantini et al., 2017). Quantitative approaches are needed to better estimate total mortality associated with powerlines (Costantini et al., 2017), but mitigative measures, such as buried lines, lowering and modifying existing lines, shielding by trees, rescue campaigns, and reduction of light attraction should be implemented to reduce risks (Rodríguez et al., 2017b).
Philopatric petrels could become victims of other threats, such as light pollution or introduced predators (see previous sections), once their natal or breeding grounds have been converted. Noise pollution is another consequence of urbanization and development. Its effects on petrels have not been assessed, though it has been suggested that can attract some individuals to dangerous areas (Miles et al., 2010).
Lastly, residential development will also result in a concomitant increase in the presence of introduced predators. These can be free-ranging house pets, unwanted feral animals, feral colonies fed by humans, or animals raised as livestock. The proximity of residential or commercial developments to petrel colonies can therefore also have a knock-on impact by introducing large numbers of these predators into the environment.
Pollution
Petrels are at risk to many types of marine pollution. Here we consider plastic, contaminant, oil, and radio-active sources of pollution.
Plastic Pollution – Ingestion and Entanglement
A candlestick in the gut of a Wilson’s Storm-Petrel Oceanites oceanicus was the first report of a seabird ingesting a man-made item (Couch, 1838). Since then hundreds of seabird species have been reported to ingest marine litter (Kühn et al., 2015). While petrels are vulnerable to plastic entanglement in ghost nets such as fishing gear or other large debris items (Ryan, 2018); they are particularly vulnerable to ingesting plastics because they feed at the surface where plastics float, and they retain ingested plastics for long periods due to the constricted structure of their pyloric valve (Ryan, 2015). Indeed the only official seabird monitoring program for marine plastics focuses on Northern Fulmars Fulmarus glacialis as a biological indicator in the North Sea (van Franeker et al., 2011; Provencher et al., 2017), although many other long-term studies collect data on the issue. Intergenerational transfer of plastics among seabirds have been demonstrated in petrels, illustrating how seabirds may be affected by marine plastics (Ryan, 1988; Carey, 2011a; Rodríguez et al., 2012a). Plastic ingestion could damage or block the digestive tract, reduce stomach volume and transfer chemical compounds both endogenous or absorbed while plastic items drifts at sea (Tanaka et al., 2015; Provencher et al., 2018). Petrels are particularly at risk from plastic-related toxins because they retain plastics in their stomach for protracted periods, giving the chemicals sufficient time to transfer into the birds (Tanaka et al., 2015). However, population level impacts from plastics have yet to be shown. This may be a function of the lack of long-term coordinated study efforts to date (Rochman et al., 2016; Avery-Gomm et al., 2018), but impact probably is only significant for individuals with large plastic loads, which comprise only a small proportion of birds even among species where virtually all individuals contain some ingested plastic (Ryan, 2019).
While the removal of marine debris could reduce entanglement mortality, plastic ingestion is a greater conservation concern for petrels. Given that plastic items larger than 5 mm account for more than 75% of plastic mass at sea (Lebreton et al., 2018), removing them from the environment now will have a major beneficial impact in future by preventing them from degrading into microplastics. Unfortunately, no coordinated international agreement on plastics exists to reduce the release of plastics into the environment (Borrelle et al., 2017). As clean-up efforts continue and plastic reduction policies come into effect, assessing if and how these measures reduce the impacts of plastics on vulnerable species such as petrels is critical to evaluating these approaches.
Contaminants
Anthropogenic environmental contaminants, including heavy metals and persistent organic pollutants (hereafter, POPs), can be taken up by and cause negative impacts to marine wildlife. Given that such contaminants have global distributions and some contaminants, such as mercury and POPs, tend to bio-magnify up food chains, petrels may be globally vulnerable to accumulating high levels of contaminants (Mallory and Braune, 2012). Negative effects vary by contaminant, species, and concentration but can include behavioral changes, physical deformities, mortality, and reduced reproductive success.
Contaminant accumulation (e.g., mercury, POPs, organochlorine pesticides, PCBs, and perfluorinated compounds) has been documented in several species (Braune et al., 2010; Carravieri et al., 2018; Escoruela et al., 2018). Blévin et al. (2013) reported mercury contamination in all 21 species of sympatric breeding seabird species at Kerguelen, nine of which are procellarids with documented bio-magnification according to trophic position. Petrels can also be exposed to heavy metals and contaminants via plastic ingestion (Tanaka et al., 2015; Lavers and Bond, 2016; Provencher et al., 2018). Future research priorities include evaluating: (1) the use of petrels as bio-monitors of contaminant levels in marine systems and the role of regulations on contaminant loads; (2) the implications of variability in contaminant levels in tissues throughout their annual cycle; and (3) the impact of contaminants, including those leached from ingested plastic debris, on vital rates.
Oil Pollution
The episodic nature of oil spills, blowouts and discharges are both chronic and acute in nature. Magnitude of oil impacts are determined by spill locations, duration, and more importantly, the spatial-temporal overlap with seasonal bird distributions (Burger, 1993). Quantification of these impacts is difficult, especially for pelagic birds where carcass detection and collection can be challenging or impossible. Chronic oil spills kill tens of thousands of seabirds each year in eastern Canada (Wiese and Robertson, 2004), although petrels accounted for less than 0.4% of the carcasses collected during the Deep Water Horizon blowout in the Gulf of Mexico (Haney et al., 2014b). Petrels are largely unreported in other major oil spills worldwide (ICES, 2005; Munilla et al., 2011) although possibly owing to spill location and timing but potentially due to undetected mortality.
Birds that survive initial oiling are vulnerable to hypothermia because of a decline in the waterproof properties of feathers when oil clumps and sticks to feathers. A reduction in body condition and reduced foraging opportunities can result in dehydration and mobilization of energy stores leading to starvation (Crawford et al., 2000). Birds that survive may also attempt to preen oil off, resulting in significant ingestion leading to a series of oil-induced diseases, such as aspergillosis, cachexia, haemolytic anemia, ulceration of the stomach, and immuno-suppressant effects (Crawford et al., 2000). In addition, indirect effects on habitat and prey could be severe (Zabala et al., 2011).
Assessment of oil spill impacts will require better estimates of at-sea distribution and abundance of petrel species which can be used in exposure probability models (Wilhelm et al., 2007; Haney et al., 2014a,b); damage assessment models may be informed further by telemetry data now being collected for some of the smallest petrel species (Pollet et al., 2014) which are difficult to detect and identify during surveys, especially during spills. Future work on oil spills in the vicinity of colonies should consider short- and medium-term management solutions and ecosystem restoration to mitigate the impacts of oil spills on petrels. For example, ship rats were eradicated from the Rakiura Titi Islands in New Zealand as compensation for the Command Oil Spill off California (McClelland et al., 2011).
Radioactivity
During the Fukushima nuclear disaster in 2011, reactor cooling waters were diverted into the Pacific Ocean (Reardon, 2011; Buesseler, 2014). Trans-equatorial migrants, such as Flesh-footed Shearwaters foraging within the contaminated marine zone (Reid et al., 2013) were likely exposed to radioactive isotopes, including caesium-137, which has a 30 year half-life, may bio-accumulate in seabird prey, and can be incorporated into animals’ bodies via the dermis or ingestion (Buesseler, 2014). The consequences of radioactive isotopes incorporated into the food chain could include reproductive failure, mutations, and stunted growth in seabirds (Buesseler, 2014). However, no formal research has been specifically conducted in petrels. Long-term monitoring is needed to determine the extent of these contaminant burden and if there are individual, sub-population or population-level impacts for exposed birds.
Disturbance
Human disturbance can have detrimental effects on wildlife including petrels (Carney and Sydeman, 1999; Carey, 2009). Given that petrels are highly pelagic seabirds, disturbance by humans is more frequent on land at breeding areas. Thus, all evidence of human disturbance on petrels comes from breeding colonies, mainly related with research or recreational activities.
Handling of eggs, chicks, and adults during research or burrow access hatches can lead to negative effects on breeding rates (Blackmer et al., 2004; Carey, 2009), although other studies did not find such effects (Vertigan et al., 2012). For example, manipulating the smaller species at egg stage could result in clutch abandon. Even the presence of observers during the hatching period can produce lower hatching and breeding success, as observed in Northern Fulmars (Ollason and Dunnet, 1980). Physiological effects have also been reported. Nest manipulations and human approaches by a single person on foot caused considerable increases in heart rates and modified energy expenditure during incubation of surface-nesting Northern Giant Petrels Macronectes halli (de Villiers et al., 2006).
In the Mediterranean, European Storm-Petrels were not chronically stressed (measured by adrenocorticotropic hormone levels) by the presence of tourist boats inside a tourist-exposed breeding cave, but birds breeding in undisturbed caves could be more susceptible to novel stressors (Soldatini et al., 2015). Nestling mortality was higher in areas exposed to high visitor pressure than remote areas at the Shetland Islands (Watson et al., 2014). Noise pollution may have a short-term negative effect on parental care and chick provisioning behavior of Scopoli’s Shearwaters (Cianchetti-Benedetti et al., 2018a).
In comparison with other seabirds like penguins and albatrosses, there are fewer field studies describing effects of human disturbance on breeding biology and physiology of petrels. Underground nesting behavior of petrels, which might make them less susceptible to human disturbance owing to the absence of direct visual contact, could explain the few studies (Watson et al., 2014). Long-term studies on anthropogenic stress will contribute to understanding the extent of consequences of human activities on animal populations, especially those of rare or endangered species (Carney and Sydeman, 1999; Carey, 2009).
Energy Production and Mining
Energy production and mining are highly lucrative industries with potential to impact pelagic seabirds offshore and at colonies. Direct impacts kill individuals, while indirect influences can modify movement behavior and remove or alter foraging and nesting habitats as detailed in previous sections.
Mining, Quarrying, and Other Terrestrial Infrastructure
Loss or degradation of breeding habitat through mining and quarrying is a threat for at least six IUCN Red-listed petrel species; for three overall impact is assessed as medium. For the endangered Peruvian Diving Petrel Pelecanoides garnotii, which burrows in thick guano, guano extraction is thought to have precipitated the massive historical declines along the Chilean and Peruvian coasts, and the extraction continues today, albeit at lower intensity (BirdLife International, 2018a). Nickel mining occurs within current and former breeding locations of the Tahiti Petrel, where feasibility of chick translocation as a means of mitigating the impact of mining is being investigated (BirdLife International, 2018a). For the critically endangered Beck’s Petrel Pseudobulweria becki, locating nesting colonies within the Bismarck Archipelago and Solomon Islands is paramount, as mining, logging and agriculture are rapidly removing forest nesting habitat (Bird et al., 2014). The impact and numbers of affected species worldwide by other mining operations is unknown. Permanent habitat loss likely precludes mitigation strategies, so conservation efforts should identify vulnerable colonies and limit development near these sites.
Offshore Oil and Gas
Impacts of offshore hydrocarbon development on seabirds have been poorly studied and specific information related to effects on petrels is extremely sparse, often anecdotal (Ronconi et al., 2015). Impacts include mortality associated with attraction to and collisions with platforms, lights and flares (Wiese et al., 2001; Montevecchi, 2006), increased exposure to oil (Fraser et al., 2006; Wilhelm et al., 2007), and potential changes to at-sea distribution of birds using habitats around platforms and drilling rigs (Baird, 1990; Burke et al., 2012).
Attraction to artificial night-lighting associated with offshore hydrocarbon platforms and ships is a major risk for petrels (see Light pollution section). In the northwest Atlantic, Leach’s Storm-Petrels Hydrobates leucorhous collide with and strand on offshore platforms (Ellis et al., 2013), and mortality assessments associated with attraction to both structure and flares is urgently needed (Hedd et al., 2018). Light attraction on migration and wintering grounds also requires study, e.g., in the oil and gas exploration and production fields off North-West and West Africa which provides important non-breeding habitat for several species (Pollet et al., 2014; Grecian et al., 2016). In the Falkland Islands, where there is a developing offshore hydrocarbon industry and regionally significant populations of petrels, data gaps around the distribution and movement of small petrels limit risk assessment for this vulnerable group (Blockley and Tierney, 2017; Augé et al., 2018). Best-practice recommendations include filling seasonal data gaps for distribution and abundance of vulnerable species, establishing monitoring and management plans at the outset of industrial development, and deploying independent observers on offshore platforms to quantify the occurrences and mortality of seabirds (Burke et al., 2012; Ronconi et al., 2015; Blockley and Tierney, 2017).
Oil and gas platforms and drill rigs also produce discharged water containing hydrocarbons and greasy drilling fluids that compromise seabird feather structure (O’Hara and Morandin, 2010), possibly contributing to cumulative mortality (Fraser et al., 2006; Ellis et al., 2013). Mitigation of this mortality is likely unfeasible for most platform operations, but cumulative impacts should be considered with environmental impact assessments and monitoring.
Renewable Energy
Marine and coastal renewable energy developments may also represent threats to petrels. Overall, the potential impacts of renewable energy installations on small petrels, whether positive or negative, are poorly understood.
Offshore wind farms may affect petrels directly via collision and displacement (Garthe and Hüppop, 2004; Cook et al., 2018). However, formal assessments conclude that impact risks are low for petrels (Furness et al., 2013; Bradbury et al., 2014), partly because of their low flight costs and flight altitudes below the arc of most turbines. Such studies are restricted to a small number of (mostly North-European) sites, therefore further work is needed to quantify the potential and realized risk on shearwaters and petrels over a much broader range of locations and species.
Land-based wind farms may also impact some species, particularly those breeding at high altitudes or inland, as they commute from terrestrial colonies to marine foraging areas. Land-based wind turbines are among potential threats for Newell’s Shearwater Puffinus newelli (BirdLife International, 2018a). For all species, the impact of collisions is either unknown or assessed as negligible-low.
Wave-powered energy installations have extremely low collision and displacement potential for flying birds, but are a direct collision risk to diving species (Grecian et al., 2010). Currently, most wave-powered devices are located in shallow coastal waters, whereas petrels frequently forage in pelagic waters, meaning minimal risks of impact. Research could, however, be targeted on understanding the diving behavior of some coastal small tubenoses in areas where wave-powered devices may be constructed.
Offshore wind farms and wave-powered energy installations may also indirectly impact petrels via changes in ocean habitat and foraging conditions. These processes are not well understood, but available research suggests that such indirect effects are beneficial (Inger et al., 2009). For instance, wind farms might act as de facto Marine Protected Areas (Campbell et al., 2014) and petrels could benefit from such effects, but formal research is lacking.
At-sea distribution during the breeding season, and particularly the non-breeding season, is poorly known for many species, and consequently so is our ability to assess spatial risk from the traditional (oil and gas) and renewable (wind, tidal) energy sectors. Current technology allows both fine-scale and year-round tracking of even the smallest species. Filling the research gaps about petrel seasonal marine distribution will improve our ability to assess potential impacts and should be a focus moving forward.
Overfishing
Food Depletion
Food depletion herein is considered to be the adverse consequence of human extractive activities (fisheries) influencing prey availability by direct exploitation with an implicit competition between fisheries and seabirds for forage fish (pelagic fish, crustaceans, and cephalopods) (Furness, 2006; Cury et al., 2011; Grémillet et al., 2018).
Among threatened species, two endangered (Peruvian diving-petrel and Hutton’s Shearwater Puffinus huttoni) and one critically endangered species (Balearic Shearwater) have been identified to be affected following IUCN assessments. There is evidence of the relationship between forage fish availability and breeding success in some species (Louzao et al., 2006). Prey reduction could adversely affect breeding success (Bourgeois and Vidal, 2008; Sommer et al., 2009) and species’ survival through increasing mortality from incidental bycatch. Low food availability may increase attraction to vessels, and thus, increase bycatch (Laneri et al., 2010; Soriano-Redondo et al., 2016).
Fishing activities can also affect petrel populations by reducing pelagic marine predator populations (e.g., Thunnus spp.) and therefore reducing their feeding opportunities, as tuna drive pelagic fishes to the ocean surface making them available to foraging seabirds. Only one endangered species (Newell’s Shearwater) has been shown to be affected by the removal of subsurface predators, through an increase of foraging effort at sea (Mitchell et al., 2005), although few studies have properly addressed this issue.
For most petrels, there is no evidence of the (in)direct effects of food depletion. Understanding the processes by which competition with fisheries may affect seabird foraging ecology and life-history traits are essential to quantify the interactions and impacts (Bertrand et al., 2012).
Proposed conservation actions could be directed to promote sustainable fishery management by studying both the forage fish and tuna populations to assess the degree of (over-)exploitation, and thus potentially limiting fish catches to secure prey availability and feeding opportunities. Fisheries could be also limited within specific seabird foraging grounds to secure prey availability in these localized areas through adaptive marine protected areas (Bertrand et al., 2012; Sherley et al., 2018). Other research actions should be directed to assess the impact of food depletion on the foraging ecology, breeding performance and survival by implementing long-term population monitoring (Arcos, 2011; Karris et al., 2018).
Discards
Discards provide important food for petrels, with the potential to support high numbers of scavengers, influencing movement and demography. Global fisheries produce ∼10 million tons of discards each year (Zeller et al., 2018), providing an important energetic subsidy for some species (Bicknell et al., 2013; Oro et al., 2013). Discards in the North Sea potentially supported ∼3.2 million Northern Fulmars annually during the 1990s (Garthe et al., 1996). Even Critically Endangered species such as the Balearic Shearwater may make extensive use of this resource, amounting up to 40% of their energy requirements (Arcos and Oro, 2002), which is likely reinforced by the overexploitation of their natural prey. Discard volumes are decreasing globally (Zeller et al., 2018), with the potential to impact upon currently dependent species, at least in the short-term (Genovart et al., 2018).
Discard availability can shape shearwater movement ecology when trawling activity provides a predictable foraging resource every weekday (Bartumeus et al., 2010). Northern Fulmars alter their at-sea movements even when they are as far as 35 km from a fishing boat (Pirotta et al., 2018). Furthermore, discard availability can alter seabird life-history traits (Bicknell et al., 2013), such as inter-annual variation in breeding performance (Louzao et al., 2006) or survival due to seabird interactions with multiple fishing gears. In the absence of discarding, Scopoli’s Shearwaters might switch from trawlers that generate considerable discards (with minimal bycatch) to long-liners which have a higher bycatch risk (Laneri et al., 2010; Soriano-Redondo et al., 2016; Cianchetti-Benedetti et al., 2018b). Such broad-scale impacts on seabird movement and bycatch risk are important in the context of understanding individuals’ behaviors, and in terms of marine spatial planning and conservation.
While most work on discard use by petrels is focussed on breeding birds, fisheries waste may also be important during non-breeding periods (Meier et al., 2017). Understanding fisheries interactions during the non-breeding period is important as this period typically represents >60% of the annual cycle. Further research is required to determine the extent to which movement of other species throughout the annual cycle is influenced and in what manner by fisheries. Only a limited understanding of the extent to which scavenging varies among and within species is currently available. A review of species-specific and regional differences in discard use is required to better understand the incidence and implications of discard use.
Diseases
Although disease emergence is recognized as a major threat for conservation, current knowledge on the ecology, epidemiology, and evolution, of infectious diseases in petrels, remains very limited. Transmission (both direct and vector-borne) of bacteria and viruses has been documented in other species of Procellariiformes, with sometimes devastating effects on the reproductive success of endangered species, e.g., Pasteurella multocida – the avian cholera causative agent – in Yellow-nosed albatrosses Thalassarche carteri (Jaeger et al., 2018). In petrels, Puffinosis has been recorded in Manx Shearwater Puffinus puffinus more than 30 years ago (Brooke, 2013), but the drivers of pathogen transmission are yet to be clearly determined. Negative effects associated with petrel infestation by ticks and other blood-parasites would also require further investigation (Dietrich et al., 2011). Studies on the mechanisms involved in transmission dynamics (e.g., population structure, environmental factors), but also on host-pathogen and pathogen-pathogen interactions (e.g., host susceptibility, co-infections with synergistic or antagonistic interactions) are required to fully assess the extent of pathogens and parasite transmission in petrels, and their consequences on conservation.
General Biology Knowledge
Effective conservation actions and assessments require well-documented knowledge on breeding biology, habitat use, as well as on population trends of the species that we aim to conserve or use as environmental indicators of the marine ecosystem. These specific fields of research are not often addressed for many petrel species, including both knowledge acquired on land in colonies and knowledge acquired at-sea during foraging trips, migration, and pelagic distribution. Indeed, despite a few extensive monographs on the petrels’ breeding biology (Warham, 1990, 1996; Brooke, 2004a), there are still major gaps in the aforementioned areas of research for many species. For example, only a few petrels are currently considered as well-known species: Cory’s Shearwater being the most studied species among the petrels, and Northern Fulmar (Fisher, 1952), Manx Shearwater (Brooke, 2013), and European Storm-Petrel (Lockley, 1983) providing the few specific monographs currently available. Therefore, we review here the current knowledge gaps in the breeding biology, habitat requirements, population size, and trends that could help in understanding the current conservation status of petrels and shearwaters.
Difficulties in Investigating Petrels on Land
The biology and ecology of petrels and shearwaters, especially the smaller species, can be challenging to study due to their specific behavior and nesting habits. First, some of these species are very sensitive to handling, such as most storm-petrels and small shearwaters, for which handling adults at specific time periods (e.g., incubation) can result in nest abandonment (Carey, 2011b). Second, most species usually visit their colonies exclusively at night, with only about 15% of petrel species attending their colony during daylight. Third, they often nest underground, in deep and inaccessible burrows, some of them excavated in soft soils, including sand. Fourth, they breed in remote locations, such as offshore islands, island summits or inaccessible cliffs. Thus, reaching burrows or colonies is sometimes difficult, e.g., tropical cliff-nesting petrels such as the Black-capped Petrel (Jodice et al., 2016). Indeed, colonies of, at least, eight species of petrels are only partly known: Elliot’s (or White-vented) Storm-Petrel Oceanites gracilis, Pincoya Storm-Petrel O. pincoyae, New Zealand Storm-Petrel Fregetta maoriana, Hornby’s Storm-Petrel Hydrobates hornbyi, Heinroth’s Shearwater Puffinus heinrothi, Fiji Petrel Pseudobulweria macgillivrayi, Beck’s Petrel Pseudobulweria beckii, and Jouanin’s petrel Bulweria fallax. Until very recently, some species were even considered extinct (Shirihai, 2008). Rediscovery of lost species could still be possible, e.g., the Jamaican Petrel Pterodroma caribbaea and the Guadalupe Storm-Petrel H. macrodactylus. Thus, the secretive breeding habits of petrels, not only hinder accurate our understanding of their breeding biology and their population estimates (see below), but also the identification of nesting areas and potential threats affecting these pelagic species on land.
Challenges to Understanding Ecology at Sea
Recent advances in miniaturization of tracking devices (e.g., in light-level geolocators, accelerometers, GPSs, and PTT-Argos devices) have provided detailed knowledge on the at-sea distribution and the foraging ecology of many petrels (Ramos et al., 2017; Hedd et al., 2018) as well as our understanding of their behavioral flexibility to deal with a changing environment (Dias et al., 2011). The number of datasets collected for petrels increased exponentially between 2005 and 2015 (BirdLife International, 2018b; Figure 5). In the same period, we saw an increase of the number of tracked species (Figure 5). Currently, the Seabird Tracking Database managed by BirdLife International holds data for 46 species. However, caveats remain for the spatial ecology of some of the rarest species as well as the smallest ones, i.e., most storm-petrels, diving petrels and some prions. From almost 500 datasets collected in the Seabird Tracking Database for petrels and shearwaters, only eight are for storm-petrels (Leach’s and Band-rumped Storm-Petrels). A particular challenge is lack of information on juvenile survival and biology at sea (Afán et al., 2019; Weimerskirch et al., 2019). As tracking technology use is growing in small petrel species and immatures, we foresee this data gap will be addressed, at least at some extent, over the next 5 years.
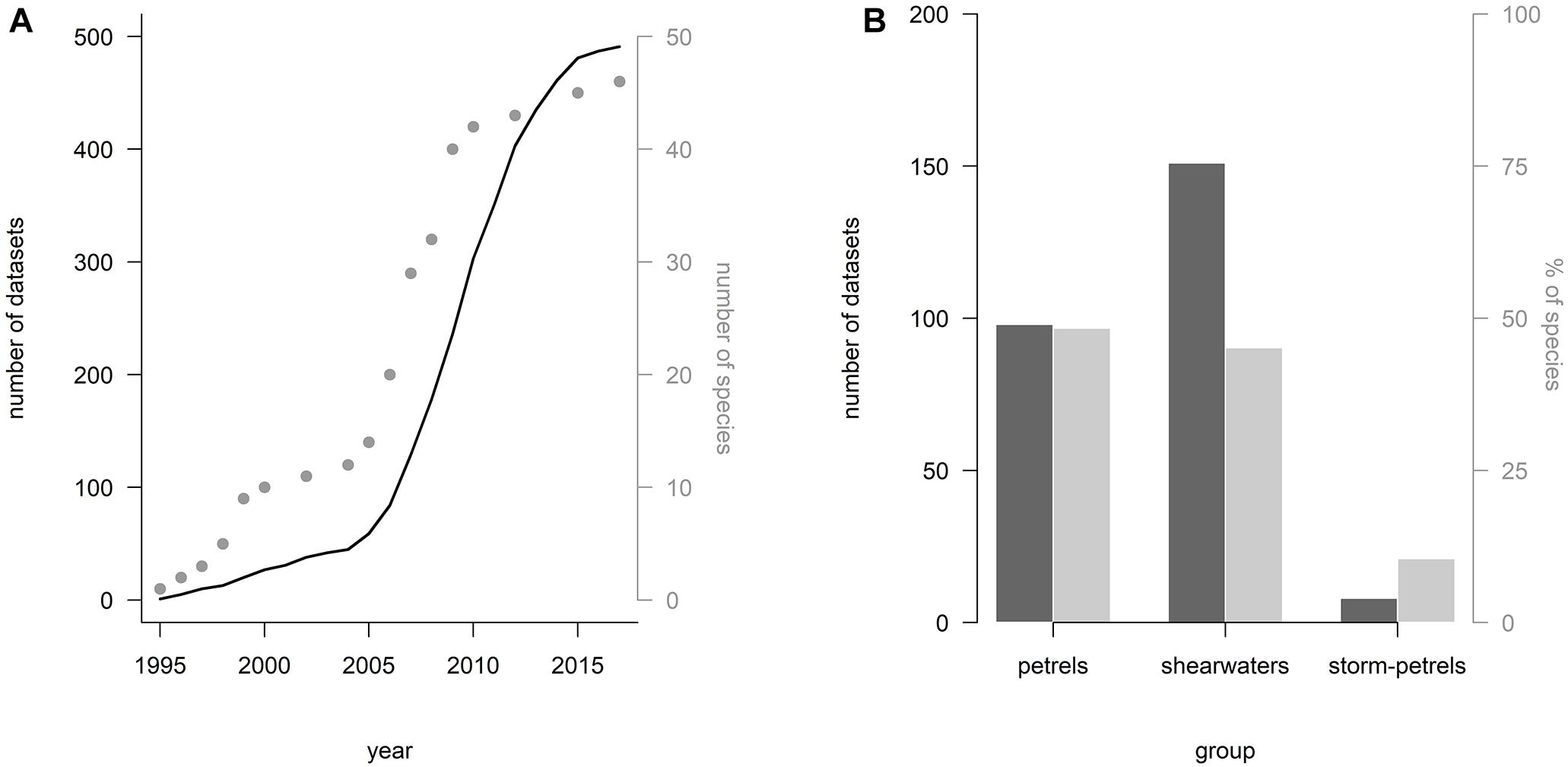
Figure 5. (A) Cumulative number of datasets (i.e., data collected for a single species in a given colony and with a specific type of device – GPS, PTT-Argos or GLS) collected between 1995 and 2018 (line), and cumulative number of species tracked during the same period (points). (B) Number of datasets per petrel groups (black bars) and respective percentages of species (gray bars). Data held (April 2018) in the Seabird Tracking Database (BirdLife International, 2018b).
Species distribution models (SDMs) can delineate habitat preferences of a given population based on spatial, count and tracking data (Guisan et al., 2013). SDMs enable researchers to draw species suitability maps based on the habitat preferences of certain populations. In this regard, important foraging grounds and habitat use for a given species may not be identified adequately by tracking a single (or a few) population(s), as dispersed populations across international boundaries will likely provide a more comprehensive picture of space/habitat use of the species (Ramos et al., 2013). This information is certainly needed to properly delineate key conservation areas and to inform conservation planning in the vast marine ecosystem. Finally, the use of intrinsic markers on the smallest species, such as stable isotope analysis (SIA), can ascertain specific features of their feeding and foraging ecology (Ramos and González-Solís, 2012). For instance, SIA of carbon (δ13C) and nitrogen (δ15N) on specific feathers have unraveled the year-round feeding ecology of the smallest seabirds breeding in the Atlantic Ocean (Quillfeldt et al., 2005; Hedd and Montevecchi, 2006; Paiva et al., 2018).
Challenges to Count and Estimate Population Size and Trends
Poor estimates limit our ability to accurately measure and report on status and trends, though these parameters are essential to develop conservation actions. Producing accurate estimates of population sizes or trends has proven extremely difficult, especially for nocturnal nest-burrowing seabirds (Sutherland and Dann, 2012). This is mainly because most of these species (a) breed in inaccessible colonies (e.g., cliffs) on remote islands, (b) nest in deep burrows, cavities or screes, (c) have nocturnal habits at breeding colonies, (d) share same breeding places with other species (more than 12 species may breed on a single island), and (e) have clumped colonial distributions so hard to extrapolate. Therefore, ensuring, assessing and counting their presence becomes difficult if not impossible (Pearson et al., 2013). Census techniques rarely account for inaccurate detection and habitat selection for nesting (Rayner et al., 2007; Whitehead et al., 2014). Detectability of nocturnal burrow-nesting seabirds can benefit from using both visual and acoustic detection (Barbraud and Delord, 2006). Records of the vocal activity of seabirds obtained from autonomous acoustic devices may be promising tools to detect occurrences or estimate population sizes of secretive species on remote islands, although such information requires validation to determine its value as a measure of abundance (Borker et al., 2014). Radar could be useful for estimating distribution and population trends, particularly for species breeding inland (Raine et al., 2017). Complementarily, SDMs can allow estimating suitable habitat for nesting, and, therefore, predicting nest density in a given area. Finally, integrating and combining these techniques (i.e., correction for detectability and SDMs) appears to be the best solution for providing reliable estimates of distribution, abundance and trends for these species (Oppel et al., 2014; Russell et al., 2017b).
Next Priorities
The key priorities to fill the gap in our knowledge of petrel biology include: (a) Identifying breeding localities of rare and threatened species. This does not mean necessarily finding the breeding colonies but at least the general breeding locations (e.g., island, mountain), to evaluate the extent of the colony, the approximate size and numbers of colonies, and their current threats. Several species are currently data deficient (all are storm-petrels). (b) Collecting data on at-sea distribution for less studied taxa or populations. Though improving, our current knowledge of the foraging ranges and distribution at sea is far from complete, especially for the south Pacific taxa, and for the juveniles of most species. (c) Standardized surveys to assess population trends. Censuses could be based on visual and/or acoustic detections, but they should be repeatable. (d) Searching for possibly extinct petrels. Unsuccessful searches for Guadalupe Storm-Petrel as well as Jamaican Petrel have already been conducted, but there is still hope for finding them. For instance, evidence of Pterodroma hasitata breeding on Jamaica has been found, so the survival of P. caribbaea is possible (Shirihai et al., 2010).
Finally, it should be stressed that knowledge on the breeding biology, especially for the rarest species, should not be targeted at any cost and unnecessary disturbances should be avoided. A sound evaluation of the costs and benefits of visiting breeding colonies, excavating burrows, or fitting tracking devices should be conducted for any threatened species before engaging in such operations.
Taxonomy
Taxonomy is tightly linked with conservation (Mace, 2004). Species concepts are extensively debated (De Queiroz, 2007), and so defining which populations should be recognized as distinct taxa, and therefore added to and assessed on species lists, such as the IUCN Red List and the Convention on International Trade in Endangered Species (CITES), can be difficult. However, this endeavor is necessary because ‘species’ are critical units for wider conservation planning and legislation (Mace, 2004; Ely et al., 2017).
The taxonomy of many petrels is still unresolved. The reasons for obscure and confusing taxonomy result from systematic decisions being based almost exclusively on morphological characters (biometrics and coloration), and many petrel groups are morphologically cryptic, i.e., similar in their external appearance (Brooke, 2004a). Two major factors can limit morphological change even between genetically divergent populations: use of non-visual reproductive signals, and constraints on morphology due to adaptation within a niche (Bickford et al., 2007). Behavioral cues used by petrels for reproductive isolation are mainly vocal, given that most species are nocturnal on their breeding grounds, and so differences in their calls are likely a better indicator of reproductive isolation than differences in morphology (Bretagnolle, 1989, 1995; Curé et al., 2010, 2016).
Procellariiformes have a well-developed sense of smell which facilitates finding their way to specific colonies (Brooke, 2004a; Gagliardo et al., 2013), and could therefore play a role in philopatry. Strong philopatry reduces genetic mixing between colonies and could also generate genetic divergence without morphological change. Their keen olfaction also appears to be used for individual recognition (Bonadonna, 2009; Bonadonna and Sanz-Aguilar, 2012). So we could hypothesize a role for scent in reproductive isolation, again without morphological change. This idea is untested and would be difficult to apply in experiments to determine levels of reproductive isolation between colonies (Zidat et al., 2017).
Many seabird populations diverge genetically without the presence of physical barriers to gene flow (Friesen, 2015). Divergence is often driven by adaptation to foraging in different ocean regimes, breeding phenology, and philopatry (Friesen et al., 2007; Gómez-Díaz et al., 2009; Friesen, 2015; Taylor et al., 2018). The lack of recognition that divergence can occur without physical barriers to gene flow until relatively recently, coupled with low morphological divergence, may have hindered recognition of seabird species. Indeed, recent recognition of cryptic species in petrels has flourished: for example C. borealis (Zidat et al., 2017), H. monteiroi (Bolton et al., 2008), Pelecanoides whenuahouensis (Fischer et al., 2018), and P. bannermani (Kawakami et al., 2018).
Testing for direct indicators of reproductive isolation between colonies of petrels, e.g., using playback experiments, is a good way of investigating species boundaries under the biological species concept (Bretagnolle, 1989; Bretagnolle and Lequette, 1990). However, these methods can be time consuming and logistically difficult (Bolton, 2006). Such experiments can and should continue to be carried out when possible. However, we encourage both academic researchers and applied conservation biologists to collect DNA samples of hard to sample and little known petrels to collaborate with conservation geneticists, as molecular investigation will be valuable for systematic and conservation biology. The shrinking costs of high-throughput DNA sequencing will continue to resolve the taxonomy of cryptic petrel lineages, such as for example Monteiro’s Storm-Petrel (Bolton et al., 2008), Bryan’s Shearwater Puffinus bryani (Pyle et al., 2011), and the Gray-faced Petrel Pterodroma gouldi (Wood et al., 2016).
A recent and ensuing debate about approaches to global taxonomy, especially regarding conservation practices, is bringing this issue to the forefront of the conservation priorities (Garnett and Christidis, 2017; Hollingsworth, 2017; Lambertz, 2017; Thomson et al., 2018). With many articles calling for more recognition, funding and training for taxonomic research (Mace, 2004; Ely et al., 2017), we hope to see a renewed vigor in the field of systematics given its relevance for applied conservation.
Synthesis
Effective conservation requires well documented knowledge (Simberloff, 1998). However, the cryptic behavior of petrels at breeding grounds (i.e., nocturnal colony visits, underground nesting, remote, and inaccessible reproduction areas), their small size, and their high mobility at sea hinder and, in some cases, prevent their study, management and conservation (Brooke, 2018). The petrel use of vast areas of the ocean does not help either, as it puts them under different regulations of many national and international jurisdictions, and boundaries (Lascelles et al., 2014; Harrison et al., 2018). Thus, there are major knowledge gaps in many species’ basic biology and our research is highly segregated by country and species, usually biased to the richest countries and to the most common, large body-sized species. A total of 16 out of 124 petrel species have unknown population status, and six out them are threatened (BirdLife International, 2018a). Under this scenario, assessing the importance of threats is subjective and probably biased by our knowledge on species or threats. Despite all these difficulties, ranking or prioritization lists have important implications for conservation and management.
In typical long-lived species such as petrels, the most pernicious threats must be those causing direct adult mortality. Given the extreme vulnerability of petrels on land due to their limited terrestrial locomotion, the introduction of invasive mammalian predators seems to be their most severe threat, occurring on over 50% of petrel breeding islands, impacting at least 78 petrel species and being a key contributing factor in the extinction of some petrel species (BirdLife International, 2018a). Other invasive species, such as invertebrates, plants, birds, and non-predatory mammals, threaten petrel populations by changing community composition, trophic interactions or habitat (Russell et al., 2017a).
As human demands on seafood increases, fisheries-related threats are expected to increase, even more if no correctional actions are taken (Table 2). Bycatch mortality seems to be already critical for some threatened medium-large species, although more information is urgently required on smaller petrel species. However, proper management could buffer the impact of the increase of seafood demand (Moreno et al., 2008; Waugh et al., 2008) and actions can be already put in practice to minimize it (Table 2). In addition, food depletion by overfishing could have also important consequences by reducing prey populations or mutualist predators during fishing such as tunas or cetaceans. Therefore, a better management of fisheries, with an ecosystem-based approach, is essential for the sustainability of this activity and the well-being of the marine ecosystem in the long term.
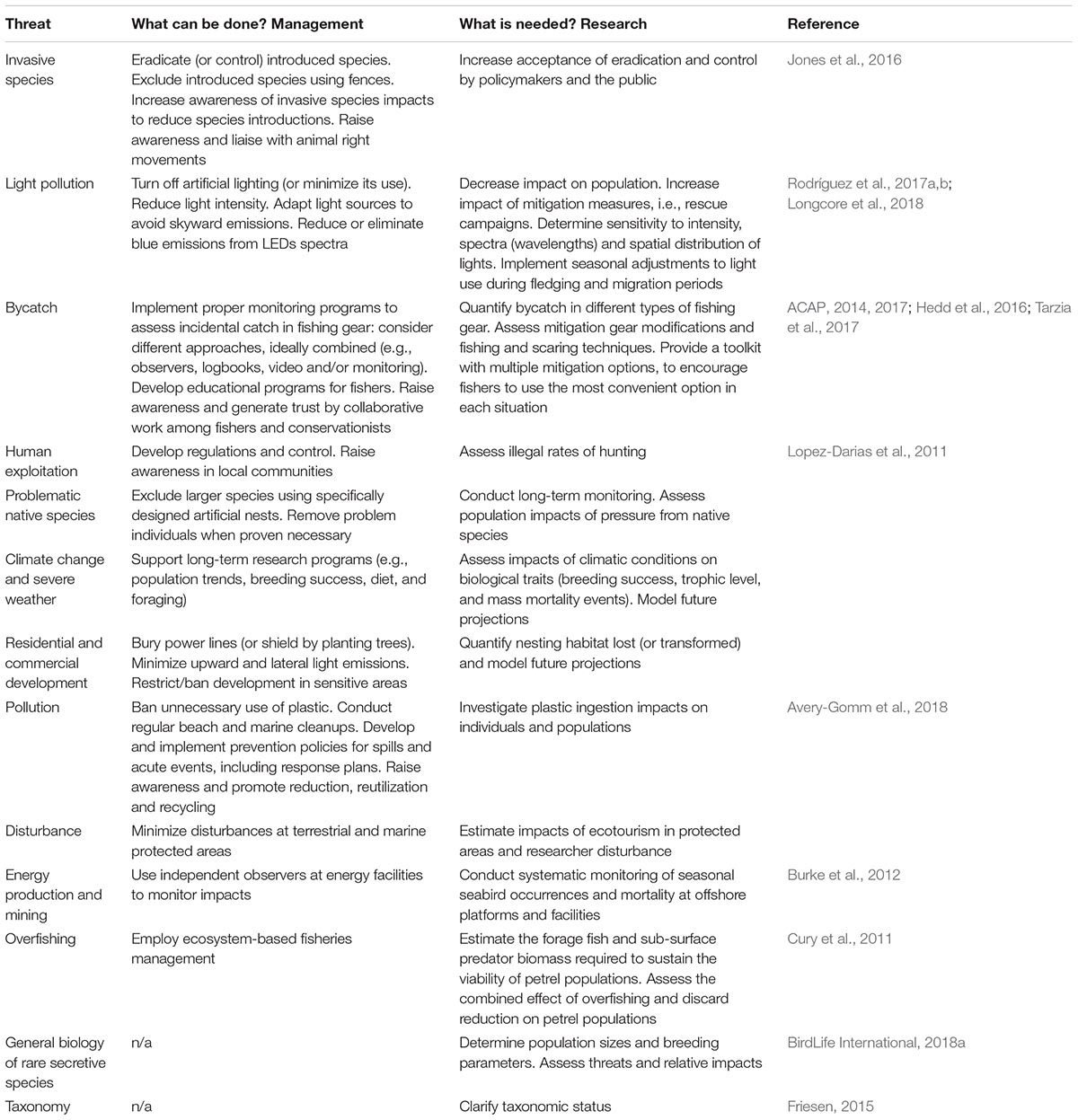
Table 2. Measures and future research needs to reduce the severity of threats to petrels around the world.
With human settlement on islands and the increase in density and development, loss and alteration of breeding habitat is expected to increase. Permanent habitat loss likely precludes mitigation strategies, so conservation efforts should identify vulnerable colonies and limit development near these sites. One of the main alterations of petrel breeding habitat is light pollution, which affects at least 56 petrel species (Rodríguez et al., 2017b), although impact is low for many of them (Figure 2). However, for some species with restricted breeding grounds on small islands densely inhabited by humans, this threat must be offset to reduce impacts on population dynamics by the high light-induced mortality of fledglings (Fontaine et al., 2011; Griesemer and Holmes, 2011; Gineste et al., 2016).
Global threats such as climate change or plastic pollution are impossible to address meaningfully without collaboration and commitment at a global scale to achieve stabilization of greenhouse gas concentrations in the atmosphere and reduction in the amount of plastic produced and released in the environment. Although our understanding is generally limited with regards to their impact on petrel populations, at least in the case of microplastics (Avery-Gomm et al., 2018), it is likely that there will be population-level consequences for many petrel species. Evidence indicates that climate change may affect petrel survival or reproduction through changes in the food chain (and thus prey availability) and an increase in extreme weather events, while plastics can cause mortality by ingestion and entanglement. Local or national agencies could also enhance ecosystem resilience to these global stressors by managing interacting stressors that operate at local or medium-scales, e.g., fisheries or sources of pollution (Ramírez et al., 2018). There is still a considerable lack of knowledge regarding thresholds of petrel stressors, and the manner in which these thresholds are lowered by additional stressors. In this regard, long-term studies, monitoring programs, and conservation plans will certainly improve our understanding of the thresholds of interacting stressors and the patterns and mechanisms by which these stressors are impacting petrels.
An ambitious goal to reverse the population declines is to address at least some of these six main threats (invasive species, bycatch, overfishing, light pollution, climate change, and plastic pollution). Tackling these main threats through active efforts such as restoring island habitats (e.g., invasive species removal, control and prevention), improving policies and regulations at global and regional levels, and engaging local communities in conservation efforts, will maximize opportunities to reverse the population declines of most petrel species. Raising awareness among general public will be crucial to drive changes in political will. Until such global decisions are collaboratively engaged by the international community, and scientists find responses to critical research questions, many actions can be already taken with the current knowledge (Table 2). Meanwhile, the improvement of communication and information exchanges between scientists and stakeholders are essential to help develop effective conservation strategies and activities to prevent petrel population declines and species extinctions (Croxall et al., 2012). This effort has already been enhanced by important knowledge sharing tools such as the Seabird Tracking Database, the Threatened Island Biodiversity Database, and the various IUCN databases (BirdLife International, 2018a,b; IUCN, 2018b). In addition, the bio-logging revolution, i.e., the miniaturization of tracking or logging devices and the development of new ones, which has enabled seabird researchers to answer questions that 20 years ago would have been unanswerable (Brooke, 2018), will continue to reveal the secret life of the most enigmatic, small-sized, shy petrels in the near future. The clear message that emerges from this review is the continued need for research and monitoring to inform and motivate effective conservation at the global level.
Data Availability
The datasets analyzed for this study can be found at BirdLife Data Zone (www.datazone.birdlife.org) and IUCN Red List of Threatened Species (www.iucnredlist.org).
Author Contributions
AR and AC conceived and designed the review. AR, MPD, and FR analyzed the data. MPD and RM contributed to data collection. JMA, VB, MPD, NDH, ML, JP, AFR, FR, BR, RAR, and RST led the topic research sections. All authors contributed to writing, and accepted a compiled version produced by AR and AC.
Funding
AR and FR were supported by Juan de la Cierva programme, Spanish Ministry of Economy, Industry and Competitiveness (IJCI-2015-23913 and IJCI-2015-24531). ML was funded by the Ramón y Cajal programme (RYC-2012-09897), Spanish Ministry of Economy, Industry and Competitiveness. WM was supported by an NSERC Discovery grant. MG was partially supported by the European Union (MINOUW Project, H2020-634495). JMA and VC were supported by ZEPAMED Project, Pleamar programme, Fundación Biodiversidad, Spanish Ministry of Ecological Transition. We are grateful for the logistic support and funding from the Phillip Island Nature Parks and the CSIC Open Access Publication Support Initiative through its Unit of Information Resources for Research (URICI).
Conflict of Interest Statement
The authors declare that the research was conducted in the absence of any commercial or financial relationships that could be construed as a potential conflict of interest.
Acknowledgments
BirdLife International support was crucial in providing information on the Red List. We thank the editor for inviting us to write this review and two reviewers for providing very useful criticism which contributed to improve earlier versions.
Supplementary Material
The Supplementary Material for this article can be found online at: https://www.frontiersin.org/articles/10.3389/fmars.2019.00094/full#supplementary-material
TABLE S1 | Reported effects of climatic environmental features on petrels and shearwaters.
Footnotes
References
ACAP (2014). Best Practice Seabird Bycatch Mitigation Criteria And Definition. Available at: https://www.bmis-bycatch.org/references/s62anjgw [accessed October 5, 2018].
ACAP (2017). ACAP Review and Best Practice Advice for Reducing the Impact of Pelagic Longline Fisheries on Seabirds. Available at: www.acap.aq/en/bycatch-mitigation/mitigation-advice [accessed October 5, 2018].
Afán, I., Navarro, J., Grémillet, D., Coll, M., and Forero, M. G. (2019). Maiden voyage into death: are fisheries affecting seabird juvenile survival during the first days at sea? R. Soc. Open Sci. 6:181151. doi: 10.1098/rsos.181151
Ainley, D. G. (1995). “Ashy storm-petrel Oceanodroma homochroma,” in The Birds of North America, No. 185, eds A. Poole and F. Gill (Philadelphia, PA: The Academy of Natural Sciences), 1–12.
Anderson, A. (1996). Origins of Procellariidae hunting in the Southwest Pacific. Int. J. Osteoarchaeol. 6, 403–410. doi: 10.1002/(SICI)1099-1212(199609)6:4<403::AID-OA296>3.0.CO;2-0
Anderson, O. R. J., Small, C. J., Croxall, J. P., Dunn, E. K., Sullivan, B. J., Yates, O., et al. (2011). Global seabird bycatch in longline fisheries. Endanger. Species Res. 14, 91–106. doi: 10.3354/esr00347
Arcos, J., and Oro, D. (2002). Significance of fisheries discards for a threatened Mediterranean seabird, the Balearic shearwater Puffinus mauretanicus. Mar. Ecol. Prog. Ser. 239, 209–220. doi: 10.3354/meps239209
Arcos, J. M. (2011). International Species Action Plan for the Balearic Shearwater, Puffinus mauretanicus. Cambridge: BirdLife & BirdLife International.
Atkinson, I. (1985). “The spread of commensal species of Rattus to oceanic islands and their effects on island avifaunas,” in Conservation of Island Birds, ed. P. Moors (Cambridge: International Council for Bird Preservation), 35–81.
Augé, A. A., Dias, M. P., Lascelles, B., Baylis, A. M. M., Black, A., Boersma, P. D., et al. (2018). Framework for mapping key areas for marine megafauna to inform marine spatial planning: the Falkland Islands case study. Mar. Policy 92, 61–72. doi: 10.1016/J.MARPOL.2018.02.017
Avery-Gomm, S., Borrelle, S. B., and Provencher, J. F. (2018). Linking plastic ingestion research with marine wildlife conservation. Sci. Total Environ. 63, 1492–1495. doi: 10.1016/j.scitotenv.2018.04.409
Baird, P. H. (1990). Concentrations of seabirds at oil-drilling rigs. Condor 92, 768–771. doi: 10.2307/1368697
Baker, B., and Hamilton, S. (2016). Impacts of Purse-Seine Fishing on Seabirds and Approaches to Mitigate Bycatch. Available at: https://www.bmis-bycatch.org/references/vmta6ld5 [accessed October 5, 2018].
Barbraud, C., and Delord, K. (2006). Population census of blue petrels Halobaena caerulea at Mayes Island, Iles Kerguelen. Antarct. Sci. 18, 199–204. doi: 10.1017/S095410200600023X
Barbraud, C., and Weimerskirch, H. (2001). Contrasting effects of the extent of sea-ice on the breeding performance of an Antarctic top predator, the Snow Petrel Pagodroma nivea. J. Avian Biol. 32, 297–302. doi: 10.1111/j.0908-8857.2001.320402.x
Barbraud, C., and Weimerskirch, H. (2003). Climate and density shape population dynamics of a marine top predator. Proc. R. Soc. B Biol. Sci. 270, 2111–2116. doi: 10.1098/rspb.2003.2488
Barnosky, A. D., Ehrlich, P. R., and Hadly, E. A. (2016). Avoiding collapse: grand challenges for science and society to solve by 2050. Elem. Sci. Anthr. 4:000094. doi: 10.12952/journal.elementa.000094
Barry Baker, G., and Wise, B. S. (2005). The impact of pelagic longline fishing on the flesh-footed shearwater Puffinus carneipes in Eastern Australia. Biol. Conserv. 126, 306–316. doi: 10.1016/J.BIOCON.2005.06.001
Bartumeus, F., Giuggioli, L., Louzao, M., Bretagnolle, V., Oro, D., and Levin, S. A. (2010). Fishery discards impact on seabird movement patterns at regional scales. Curr. Biol. 20, 215–222. doi: 10.1016/j.cub.2009.11.073
Berrow, S. D., Croxall, J. P., and Grant, S. D. (2000). Status of white-chinned petrels Procellaria aequinoctialis linnaeus 1758, at bird Island. South Georgia. Antarct. Sci. 12, 399–405. doi: 10.1017/S0954102000000468
Bertrand, S., Joo, R., Arbulu Smet, C., Tremblay, Y., Barbraud, C., and Weimerskirch, H. (2012). Local depletion by a fishery can affect seabird foraging. J. Appl. Ecol. 49, 1168–1177. doi: 10.1111/j.1365-2664.2012.02190.x
Bickford, D., Lohman, D. J., Sodhi, N. S., Ng, P. K. L., Meier, R., Winker, K., et al. (2007). Cryptic species as a window on diversity and conservation. Trends Ecol. Evol. 22, 148–155. doi: 10.1016/J.TREE.2006.11.004
Bicknell, A. W. J., Oro, D., Camphuysen, K. C. J., and Votier, S. C. (2013). Potential consequences of discard reform for seabird communities. J. Appl. Ecol. 50, 649–658. doi: 10.1111/1365-2664.12072
Bird, J. P., Carlile, N., and Miller, M. G. R. (2014). A review of records and research actions for the critically endangered Beck’s petrel Pseudobulweria becki. Bird Conserv. Int. 24, 287–298. doi: 10.1017/S0959270913000385
BirdLife International (2018a). IUCN Red List for Birds. Species Factsheets. Available at: www.birdlife.org [accessed June 1, 2018].
BirdLife International (2018b). Seabird Tracking Database, Tracking Ocean Wanderers. Cambridge: BirdLife International.
Blackmer, A. L., Ackerman, J. T., and Nevitt, G. A. (2004). Effects of investigator disturbance on hatching success and nest-site fidelity in a long-lived seabird, Leach’s storm-petrel. Biol. Conserv. 116, 141–148. doi: 10.1016/S0006-3207(03)00185-X
Blévin, P., Carravieri, A., Jaeger, A., Chastel, O., Bustamante, P., and Cherel, Y. (2013). Wide range of mercury contamination in chicks of Southern Ocean seabirds. PLoS One 8:e54508. doi: 10.1371/journal.pone.0054508
Blockley, D., and Tierney, M. (2017). “Addressing priority gaps in understanding ecosystem functioning for the developing Falkland Islands offshore hydrocarbon industry – the ‘Gap Project’. Phase I Final Report, September 2016,” in Report Prepared for the Falkland Islands Offshore Hydrocarbons Environment, (Stanley: South Atlantic Environmental Research Institute).
Bolton, M. (2006). Playback experiments indicate absence of vocal recognition among temporally and geographically separated populations of Madeiran storm-petrels Oceanodroma castro. IBIS 149, 255–263. doi: 10.1111/j.1474-919X.2006.00624.x
Bolton, M., Medeiros, R., Hothersall, B., and Campos, A. (2004). The use of artificial breeding chambers as a conservation measure for cavity-nesting procellariiform seabirds: a case study of the Madeiran storm petrel (Oceanodroma castro). Biol. Conserv. 116, 73–80. doi: 10.1016/S0006-3207(03)00178-2
Bolton, M., Smith, A. L., Gómez-Díaz, E., Friesen, V. L., Medeiros, R., Bried, J., et al. (2008). Monteiro’s storm-petrel Oceanodroma monteiroi: a new species from the Azores. IBIS 150, 717–727. doi: 10.1111/j.1474-919X.2008.00854.x
Bonadonna, F. (2009). Olfaction in petrels. Ann. N. Y. Acad. Sci. 1170, 428–433. doi: 10.1111/j.1749-6632.2009.03890.x
Bonadonna, F., and Sanz-Aguilar, A. (2012). Kin recognition and inbreeding avoidance in wild birds: the first evidence for individual kin-related odour recognition. Anim. Behav. 84, 509–513. doi: 10.1016/J.ANBEHAV.2012.06.014
Bonnaud, E., Medina, F. M., Vidal, E., Nogales, M., Tershy, B., Zavaleta, E., et al. (2011). The diet of feral cats on islands: a review and a call for more studies. Biol. Invasions 13, 581–603. doi: 10.1007/s10530-010-9851-3
Borker, A. L., Mckown, M. W., Ackerman, J. T., Eagles-Smith, C. A., Tershy, B. R., and Croll, D. A. (2014). Vocal activity as a low cost and scalable index of seabird colony size. Conserv. Biol. 28, 1100–1108. doi: 10.1111/cobi.12264
Borrelle, S. B., Rochman, C. M., Liboiron, M., Bond, A. L., Lusher, A., Bradshaw, H., et al. (2017). Opinion: why we need an international agreement on marine plastic pollution. Proc. Natl. Acad. Sci. 114, 9994–9997. doi: 10.1073/pnas.1714450114
Bourgeois, K., Ouni, R., Pascal, M., Dromzée, S., Fourcy, D., and Abiadh, A. (2013). Dramatic increase in the Zembretta Yelkouan shearwater breeding population following ship rat eradication spurs interest in managing a 1500-year old invasion. Biol. Invasions 15, 475–482. doi: 10.1007/s10530-013-0419-x
Bourgeois, K., and Vidal, E. (2008). The endemic Mediterranean yelkouan shearwater Puffinus yelkouan: distribution, threats and a plea for more data. Oryx 42, 187–194. doi: 10.1017/S0030605308006467
Bourgeois, K., and Vidal, É (2007). Yelkouan shearwater nest-cavity selection and breeding success. C. R. Biol. 330, 205–214. doi: 10.1016/j.crvi.2006.12.007
Bradbury, G., Trinder, M., Furness, B., Banks, A. N., Caldow, R. W. G., and Hume, D. (2014). Mapping seabird sensitivity to offshore wind farms. PLoS One 9:e106366. doi: 10.1371/journal.pone.0106366
Braune, B. M., Mallory, M. L., Butt, C. M., Mabury, S. A., and Muir, D. C. G. (2010). Persistent halogenated organic contaminants and mercury in northern fulmars (Fulmarus glacialis) from the Canadian Arctic. Environ. Pollut. 158, 3513–3519. doi: 10.1016/j.envpol.2010.08.023
Bretagnolle, V. (1989). Calls of Wilson’s storm petrel: functions, individual and sexual recognitions, and geographic variation. Behaviour 111, 98–112. doi: 10.1163/156853989X00600
Bretagnolle, V. (1995). Systematics of the Soft-plumaged petrel Pterodroma mollis (Procellariidae): new insight from the study of vocalizations. IBIS 137, 207–218. doi: 10.1111/j.1474-919X.1995.tb03241.x
Bretagnolle, V., and Lequette, B. (1990). Structural variation in the call of the Cory’s shearwater (Calonectris diomedea, Aves, Procellariidae). Ethology 85, 313–323. doi: 10.1111/j.1439-0310.1990.tb00410.x
Bried, J. (2003). Impact of vagrant predators on the native fauna: a short-eared owl (Asio flammeus) preying on Madeiran storm petrels (Oceanodroma castro) in the Azores. Arquipelago. Life Mar. Sci. 20, 57–60.
Brodier, S., Pisanu, B., Villers, A., Pettex, E., Lioret, M., Chapuis, J.-L., et al. (2011). Responses of seabirds to the rabbit eradication on Ile Verte, sub-Antarctic Kerguelen Archipelago. Anim. Conserv. 14, 459–465. doi: 10.1111/j.1469-1795.2011.00455.x
Brooke, M. (2018). Far From Land: The Mysterious Lives of Seabirds. Princenton, NJ: Princeton University Press.
Brooke, M. D. L. (2004b). The food consumption of the world’s seabirds. Proc. R. Soc. B Biol. Sci. 271, S246–S248. doi: 10.1098/rsbl.2003.0153
Brooke, M. D. L., Bonnaud, E., Dilley, B. J., Flint, E. N., Holmes, N. D., Jones, H. P., et al. (2018). Seabird population changes following mammal eradications on islands. Anim. Conserv. 21, 3–12. doi: 10.1111/acv.12344
Buesseler, K. (2014). Fukushima and ocean radioactivity. Oceanography 27, 92–105. doi: 10.5670/oceanog.2014.02
Burger, A. E. (1993). Estimating the mortality of seabirds following oil spills: effects of spill volume. Mar. Pollut. Bull. 26, 140–143. doi: 10.1016/0025-326X(93)90123-2
Burke, C. M., Montevecchi, W. A., and Wiese, F. K. (2012). Inadequate environmental monitoring around offshore oil and gas platforms on the grand bank of Eastern Canada: are risks to marine birds known? J. Environ. Manage. 104, 121–126. doi: 10.1016/j.jenvman.2012.02.012
Burrows, M. T., Schoeman, D. S., Buckley, L. B., Moore, P., Poloczanska, E. S., Brander, K. M., et al. (2011). The pace of shifting climate in marine and terrestrial ecosystems. Science 334, 652–655. doi: 10.1126/science.1210288
Cadiou, B., Bioret, F., and Chenesseau, D. (2010). Response of breeding European storm petrels Hydrobates pelagicus to habitat change. J. Ornithol. 151, 317–327. doi: 10.1007/s10336-009-0458-3
Campbell, K. J., Beek, J., Eason, C. T., Glen, A. S., Godwin, J., Gould, F., et al. (2015). The next generation of rodent eradications: innovative technologies and tools to improve species specificity and increase their feasibility on islands. Biol. Conserv. 185, 47–58. doi: 10.1016/J.BIOCON.2014.10.016
Campbell, M. S., Stehfest, K. M., Votier, S. C., and Hall-Spencer, J. M. (2014). Mapping fisheries for marine spatial planning: gear-specific vessel monitoring system (VMS), marine conservation and offshore renewable energy. Mar. Policy 45, 293–300. doi: 10.1016/j.marpol.2013.09.015
Caravaggi, A., Cuthbert, R. J., Ryan, P. G., Cooper, J., and Bond, A. L. (2018). The impacts of introduced House Mice on the breeding success of nesting seabirds on Gough Island. IBIS. doi: 10.1111/ibi.12664
Carey, M. J. (2009). The effects of investigator disturbance on procellariiform seabirds: a review. New Zeal. J. Zool. 36, 367–377. doi: 10.1080/03014220909510161
Carey, M. J. (2011a). Intergenerational transfer of plastic debris by Short-tailed Shearwaters (Ardenna tenuirostris). Emu 111, 229–234. doi: 10.1071/MU10085
Carey, M. J. (2011b). Investigator disturbance reduces reproductive success in Short-tailed Shearwaters Puffinus tenuirostris. IBIS 153, 363–372. doi: 10.1111/j.1474-919X.2011.01109.x
Carney, K. M., and Sydeman, W. J. (1999). A review of human disturbance effects on nesting colonial waterbirds. Waterbirds 22, 68–79. doi: 10.2307/1521995
Carravieri, A., Fort, J., Tarroux, A., Cherel, Y., Love, O. P., Prieur, S., et al. (2018). Mercury exposure and short-term consequences on physiology and reproduction in Antarctic petrels. Environ. Pollut. 237, 824–831. doi: 10.1016/J.ENVPOL.2017.11.004
Chandler, S. L., Tietz, J. R., Bradley, R. W., and Trulio, L. (2016). Burrowing owl diet at a migratory stopover site and wintering ground on Southeast Farallon Island, California. J. Raptor. Res. 50, 391–403. doi: 10.3356/JRR-16-00006.1
Cianchetti-Benedetti, M., Becciu, P., Massa, B., and Dell’Omo, G. (2018a). Conflicts between touristic recreational activities and breeding shearwaters: short-term effect of artificial light and sound on chick weight. Eur. J. Wildl. Res. 64:19. doi: 10.1007/s10344-018-1178-x
Cianchetti-Benedetti, M., Dell’Omo, G., Russo, T., Catoni, C., and Quillfeldt, P. (2018b). Interactions between commercial fishing vessels and a pelagic seabird in the southern Mediterranean Sea. BMC Ecol. 18:54. doi: 10.1186/s12898-018-0212-x
Connan, M., Mayzaud, P., Trouvé, C., Barbraud, C., and Cherel, Y. (2008). Interannual dietary changes and demographic consequences in breeding blue petrels from Kerguelen Islands. Mar. Ecol. Prog. Ser. 373, 123–135. doi: 10.3354/meps07723
Cook, A. S. C. P., Humphreys, E. M., Bennet, F., Masden, E. A., and Burton, N. H. K. (2018). Quantifying avian avoidance of offshore wind turbines: current evidence and key knowledge gaps. Mar. Environ. Res. 140, 278–288. doi: 10.1016/j.marenvres.2018.06.017
Cortés, V., Arcos, J., and González-Solís, J. (2017). Seabirds and demersal longliners in the northwestern Mediterranean: factors driving their interactions and bycatch rates. Mar. Ecol. Prog. Ser. 565, 1–16. doi: 10.3354/meps12026
Cortés, V., García-Barcelona, S., and González-Solís, J. (2018). Sex- and age-biased mortality of three shearwater species in longline fisheries of the Mediterranean. Mar. Ecol. Prog. Ser. 588, 229–241. doi: 10.3354/meps12427
Cortés, V., and González-Solís, J. (2018). Seabird bycatch mitigation trials in artisanal demersal longliners of the Western Mediterranean. PLoS One 13:e0196731. doi: 10.1371/journal.pone.0196731
Costantini, D., Gustin, M., Ferrarini, A., and Dell’Omo, G. (2017). Estimates of avian collision with power lines and carcass disappearance across differing environments. Anim. Conserv. 20, 173–181. doi: 10.1111/acv.12303
Couch, J. (1838). A letter on the occurrence of Wilson’s petrel (Procellaria wilsonii) on the British coast. Proc. Linn. Soc. London 1, 2–3.
Crawford, R., Davis, S., Harding, R., Jackson, L., Leshoro, T., Meyer, M., et al. (2000). Initial impact of the treasure oil spill on seabirds off western South Africa. South African J. Mar. Sci. 22, 157–176. doi: 10.2989/025776100784125645
Croxall, J. P., Butchart, S. H. M., Lascelles, B., Stattersfield, A. J., Sullivan, B., Symes, A., et al. (2012). Seabird conservation status, threats and priority actions: a global assessment. Bird Conserv. Int. 22, 1–34. doi: 10.1017/S0959270912000020
Curé, C., Aubin, T., and Mathevon, N. (2010). Intra-sex vocal interactions in two hybridizing seabird species (Puffinus sp.). Behav. Ecol. Sociobiol. 64, 1823–1837. doi: 10.1007/s00265-010-0994-0
Curé, C., Mathevon, N., and Aubin, T. (2016). Mate vocal recognition in the Scopoli’s shearwater Calonectris diomedea: do females and males share the same acoustic code? Behav. Process. 128, 96–102. doi: 10.1016/J.BEPROC.2016.04.013
Cury, P. M., Boyd, I. L., Bonhommeau, S., Anker-Nilssen, T., Crawford, R. J. M., Furness, R. W., et al. (2011). Global seabird response to forage fish depletion–one-third for the birds. Science 334, 1703–1706. doi: 10.1126/science.1212928
Darling, E. S., and Côté, I. M. (2008). Quantifying the evidence for ecological synergies. Ecol. Lett. 11, 1278–1286. doi: 10.1111/j.1461-0248.2008.01243.x
De Queiroz, K. (2007). Species concepts and species delimitation. Syst. Biol. 56, 879–886. doi: 10.1080/10635150701701083
de Villiers, M., Bause, M., Giese, M., and Fourie, A. (2006). Hardly hard-hearted: heart rate responses of incubating Northern Giant Petrels (Macronectes halli) to human disturbance on sub-Antarctic Marion Island. Polar Biol. 29, 717–720. doi: 10.1007/s00300-006-0137-2
Deppe, L., Rowley, O., Rowe, L. K., Shi, N., Gooday, O., and Goldstien, S. J. (2017). Investigation of fallout events in Hutton’s shearwaters (Puffinus huttoni) associated with artificial lighting. Notornis 64, 181–191.
Descamps, S., Aars, J., Fuglei, E., Kovacs, K. M., Lydersen, C., Pavlova, O., et al. (2017). Climate change impacts on wildlife in a High Arctic archipelago – Svalbard, Norway. Glob. Chang. Biol. 23, 490–502. doi: 10.1111/gcb.13381
Descamps, S., Tarroux, A., Lorentsen, S.-H., Love, O. P., Varpe,Ø, and Yoccoz, N. G. (2016). Large-scale oceanographic fluctuations drive Antarctic petrel survival and reproduction. Ecography 39, 496–505. doi: 10.1111/ecog.01659
Descamps, S., Tarroux, A., Varpe,Ø, Yoccoz, N. G., Tveraa, T., and Lorentsen, S.-H. (2015). Demographic effects of extreme weather events: snow storms, breeding success, and population growth rate in a long-lived Antarctic seabird. Ecol. Evol. 5, 314–325. doi: 10.1002/ece3.1357
Dias, M. P., Granadeiro, J. P., and Catry, P. (2012). Working the day or the night shift? Foraging schedules of Cory’s shearwaters vary according to marine habitat. Mar. Ecol. Prog. Ser. 467, 245–252. doi: 10.3354/meps09966
Dias, M. P., Granadeiro, J. P., Phillips, R. A., Alonso, H., and Catry, P. (2011). Breaking the routine: individual Cory’s shearwaters shift winter destinations between hemispheres and across ocean basins. Proc. R. Soc. B 278, 1786–1793. doi: 10.1098/rspb.2010.2114
Dietrich, M., Gómez-Díaz, E., and McCoy, K. D. (2011). Worldwide distribution and diversity of seabird ticks: implications for the ecology and epidemiology of tick-borne pathogens. Vector Borne Zoonotic. Dis. 11, 453–470. doi: 10.1089/vbz.2010.0009
DPIPWE (2014). Muttonbird. Game Tracks, Department Primary Industrial Park Water Environment. Available at: www.dpipwe.tas.gov.au/wmb [accessed October 10, 2018].
DPIPWE (2018). Short-Tailed Shearwater (Muttonbird). Hobart TAS: Department of Primary Industries, Parks, Water and Environment. Government of Tasmania.
Dunlop, J. (2017). Sentinel Seabirds: A Guide to Using Marine Birds to Monitor Marine Ecosystems in Western Australia. Geraldton Available at: https://www.nacc.com.au/wp-content/uploads/2017/11/1715-06C-01-Sentinel-Seabird.pdf [accessed October 11, 2018].
Ellis, J. I., Wilhelm, S. I., Hedd, A., Fraser, G. S., Robertson, G. J., Rail, J.-F., et al. (2013). Mortality of migratory birds from marine commercial fisheries and offshore oil and gas production in Canada. Avian Conserv. Ecol. 8:4. doi: 10.5751/ACE-00589-080204
Ely, C. V., Bordignon, S. A. D. L., Trevisan, R., and Boldrini, I. I. (2017). Implications of poor taxonomy in conservation. J. Nat. Conserv. 36, 10–13. doi: 10.1016/j.jnc.2017.01.003
Escoruela, J., Garreta, E., Ramos, R., González-Solís, J., and Lacorte, S. (2018). Occurrence of Per- and Polyfluoroalkyl substances in Calonectris shearwaters breeding along the Mediterranean and Atlantic colonies. Mar. Pollut. Bull. 131, 335–340. doi: 10.1016/J.MARPOLBUL.2018.04.032
Fischer, J. H., Debski, I., Miskelly, C. M., Bost, C. A., Fromant, A., Tennyson, A. J. D., et al. (2018). Analyses of phenotypic differentiations among South Georgian Diving Petrel (Pelecanoides georgicus) populations reveal an undescribed and highly endangered species from New Zealand. PLoS One 13:e0197766. doi: 10.1371/journal.pone.0197766
Fontaine, R., Gimenez, O., and Bried, J. (2011). The impact of introduced predators, light-induced mortality of fledglings and poaching on the dynamics of the Cory’s shearwater (Calonectris diomedea) population from the Azores, northeastern subtropical Atlantic. Biol. Conserv. 144, 1998–2011. doi: 10.1016/j.biocon.2011.04.022
Franeker, J. A., Van Creuwels, J. C. S., Veer, W., Van Der Cleland, S., et al. (2001). Unexpected effects of climate change on the predation of Antarctic petrels. Antarct. Sci. 13, 430–439. doi: 10.1017/S0954102001000591
Fraser, G. S., Russell, J., and von Zharen, W. M. (2006). Produced water from offshore oil and gas installations on the Grand Banks, Newfoundland and Labrador: are the potential effects to seabirds sufficiently known? Mar. Ornithol. 34, 147–156.
Friesen, M. R., Ross, J. R., Maitland, M., and Gaskin, C. P. (2016). First record of a petrel species killed by penguins: outcome of competition for artificial nesting boxes. Notornis 63, 112–115.
Friesen, V. L. (2015). Speciation in seabirds: why are there so many species…and why aren’t there more? J. Ornithol. 156, 27–39. doi: 10.1007/s10336-015-1235-0
Friesen, V. L., Smith, A. L., Gómez-Díaz, E., Bolton, M., Furness, R. W., González-Solís, J., et al. (2007). Sympatric speciation by allochrony in a seabird. Proc. Natl. Acad. Sci. U.S.A. 104, 18589–18594. doi: 10.1073/pnas.0700446104
Furness, R. W. (1988). Predation on ground-nesting seabirds by island populations of red deer Cervus elaphus and sheep Ovis. J. Zool. 216, 565–573. doi: 10.1111/j.1469-7998.1988.tb02451.x
Furness, R. W. (2006). “How many fish should we leave in the sea for seabirds and marine mammals?,” in Conservation Biology Series-Cambridge, (Cambridge: Press Syndicate of the University of Cambridge), 211. doi: 10.1017/CBO9780511541964.015
Furness, R. W., Wade, H. M., and Masden, E. A. (2013). Assessing vulnerability of marine bird populations to offshore wind farms. J. Environ. Manage. 119, 56–66. doi: 10.1016/j.jenvman.2013.01.025
Gagliardo, A., Bried, J., Lambardi, P., Luschi, P., Wikelski, M., and Bonadonna, F. (2013). Oceanic navigation in Cory’s shearwaters: evidence for a crucial role of olfactory cues for homing after displacement. J. Exp. Biol. 216, 2798–2805. doi: 10.1242/jeb.085738
García Molinos, J., Halpern, B. S., Schoeman, D. S., Brown, C. J., Kiessling, W., Moore, P. J., et al. (2015). Climate velocity and the future global redistribution of marine biodiversity. Nat. Clim. Chang. 6, 83–88. doi: 10.1038/nclimate2769
Gardner, P., and Wilson, K.-J. (1999). Burrow competition between Chatham petrels and broadbilled prions: the effectiveness of burrow blockading as a management strategy. Sci. Conserv. 131B, 23–37.
Garnett, S. T., Butchart, S. H. M., Baker, G. B., Bayraktarov, E., Buchanan, K. L., Burbidge, A. A., et al. (2018). Metrics of progress in the understanding and management of threats to Australian birds. Conserv. Biol. doi: 10.1111/cobi.13220
Garnett, S. T., and Christidis, L. (2017). Taxonomy anarchy hampers conservation. Nature 546, 25–27. doi: 10.1038/546025a
Garthe, S., Camphuysen, K. C., and Furness, R. W. (1996). Amounts of discards by commercial fisheries and their significance as food for seabirds in the North Sea. Mar. Ecol. Prog. Ser. 136, 1–11. doi: 10.3354/meps136001
Garthe, S., and Hüppop, O. (2004). Scaling possible adverse effects of marine wind farms on seabirds: developing and applying a vulnerability index. J. Appl. Ecol. 41, 724–734. doi: 10.1111/j.0021-8901.2004.00918.x
Genovart, M., Arcos, J. M., Álvarez, D., McMinn, M., Meier, R., Wynn, R. B., et al. (2016). Demography of the critically endangered Balearic shearwater: the impact of fisheries and time to extinction. J. Appl. Ecol. 53, 1158–1168. doi: 10.1111/1365-2664.12622
Genovart, M., Bécares, J., Igual, J.-M., Martínez-Abraín, A., Escandell, R., Sánchez, A., et al. (2018). Differential adult survival at close seabird colonies: the importance of spatial foraging segregation and bycatch risk during the breeding season. Glob. Chang. Biol. 24, 1279–1290. doi: 10.1111/gcb.13997
Genovart, M., Doak, D. F., Igual, J.-M., Sponza, S., Kralj, J., and Oro, D. (2017). Varying demographic impacts of different fisheries on three Mediterranean seabird species. Glob. Chang. Biol. 23, 3012–3029. doi: 10.1111/gcb.13670
Gianuca, D., Phillips, R. A., Townley, S., and Votier, S. C. (2017). Global patterns of sex- and age-specific variation in seabird bycatch. Biol. Conserv. 205, 60–76. doi: 10.1016/J.BIOCON.2016.11.028
Gineste, B., Souquet, M., Couzi, F.-X., Giloux, Y., Philippe, J.-S., Hoarau, C., et al. (2016). Tropical Shearwater population stability at Reunion Island, despite light pollution. J. Ornithol. 158, 385–394. doi: 10.1007/s10336-016-1396-5
Glass, J., and Ryan, P. (2013). Reduced seabird night strikes and mortality in the Tristan rock lobster fishery. African J. Mar. Sci. 35, 589–592. doi: 10.2989/1814232X.2013.860049
Glen, A. S., Atkinson, R., Campbell, K. J., Hagen, E., Holmes, N. D., Keitt, B. S., et al. (2013). Eradicating multiple invasive species on inhabited islands: the next big step in island restoration? Biol. Invasions 15, 2589–2603. doi: 10.1007/s10530-013-0495-y
Gómez-Díaz, E., González-Solís, J., and Peinado, M. (2009). Population structure in a highly pelagic seabird, the Cory’s shearwater Calonectris diomedea: an examination of genetics, morphology and ecology. Mar. Ecol. Prog. Ser. 382, 197–209. doi: 10.3354/meps07974
González-Zevallos, D., and Yorio, P. (2006). Seabird use of discards and incidental captures at the Argentine hake trawl fishery in the Golfo San Jorge, Argentina. Mar. Ecol. Prog. Ser. 316, 175–183. doi: 10.3354/meps316175
Granadeiro, J. P., Dias, M. P., Rebelo, R., Santos, C. D., and Catry, P. (2006). Numbers and population trends of Cory’s shearwater Calonectris diomedea at Selvagem Grande, Northeast Atlantic. Waterbirds 29, 56–60. doi: 10.2307/4132605
Grecian, W. J., Inger, R., Attrill, M. J., Bearhop, S., Godley, B. J., Witt, M. J., et al. (2010). Potential impacts of wave-powered marine renewable energy installations on marine birds. IBIS 152, 683–697. doi: 10.1111/j.1474-919X.2010.01048.x
Grecian, W. J., Witt, M. J., Attrill, M. J., Bearhop, S., Becker, P. H., Egevang, C., et al. (2016). Seabird diversity hotspot linked to ocean productivity in the canary current large marine ecosystem. Biol. Lett. 12:20160024. doi: 10.1098/rsbl.2016.0024
Grémillet, D., Ponchon, A., Paleczny, M., Palomares, M.-L. D., Karpouzi, V., and Pauly, D. (2018). Persisting worldwide seabird-fishery competition despite seabird community decline. Curr. Biol. 28, 4009–4013.e2. doi: 10.1016/j.cub.2018.10.051
Griesemer, A. M., and Holmes, N. D. (2011). Newell’s Shearwater Population Modeling for Habitat Conservation Plan and Recovery Planning. Honolulu, HI: University of Hawaii at Manoa.
Guisan, A., Tingley, R., Baumgartner, J. B., Naujokaitis-Lewis, I., Sutcliffe, P. R., Tulloch, A. I. T., et al. (2013). Predicting species distributions for conservation decisions. Ecol. Lett. 16, 1424–1435. doi: 10.1111/ele.12189
Gummer, H., Taylor, G., Wilson, K. J., and Rayner, M. J. (2015). Recovery of the endangered Chatham petrel (Pterodroma axillaris): a review of conservation management techniques from 1990 to 2010. Glob. Ecol. Conserv. 3, 310–323. doi: 10.1016/j.gecco.2014.12.006
Haney, J. C., Geiger, H. J., and Short, J. W. (2014a). Bird mortality from the Deepwater Horizon oil spill. I. Exposure probability in the offshore Gulf of Mexico. Mar. Ecol. Prog. Ser. 513, 225–237. doi: 10.3354/meps10991
Haney, J., Geiger, H., and Short, J. (2014b). Bird mortality from the Deepwater Horizon oil spill. II. Carcass sampling and exposure probability in the coastal Gulf of Mexico. Mar. Ecol. Prog. Ser. 513, 239–252. doi: 10.3354/meps10839
Harper, P. (1983). Biology of the Buller’s shearwater (Puffinus bulleri) at the Poor Knights Islands, New Zealand. Notornis 30, 299–318.
Harrison, A.-L., Costa, D. P., Winship, A. J., Benson, S. R., Bograd, S. J., Antolos, M., et al. (2018). The political biogeography of migratory marine predators. Nat. Ecol. Evol. 2, 1571–1578. doi: 10.1038/s41559-018-0646-8
Hedd, A., and Montevecchi, W. A. (2006). Diet and trophic position of Leach’s storm-petrel Oceanodroma leucorhoa during breeding and moult, inferred from stable isotope analysis of feathers. Mar. Ecol. Prog. Ser. 322, 291–301. doi: 10.3354/meps322291
Hedd, A., Pollet, I. L., Mauck, R. A., Burke, C. M., Mallory, M. L., McFarlane Tranquilla, L. A., et al. (2018). Foraging areas, offshore habitat use, and colony overlap by incubating Leach’s storm-petrels Oceanodroma leucorhoa in the Northwest Atlantic. PLoS One 13:e0194389. doi: 10.1371/journal.pone.0194389
Hedd, A., Regular, P. M., Wilhelm, S. I., Rail, J.-F., Drolet, B., Fowler, M., et al. (2016). Characterization of seabird bycatch in eastern Canadian waters, 1998-2011, assessed from onboard fisheries observer data. Aquat. Conserv. Mar. Freshw. Ecosyst. 26, 530–548. doi: 10.1002/aqc.2551
Hollingsworth, P. M. (2017). Taxonomy: avoid extra bureaucracy. Nature 546, 600–600. doi: 10.1038/546600a
Hunter, C. M., Moller, H., and Kitson, J. (2000). Muttonbirder selectivity of sooty shearwater (titi) chicks harvested in New Zealand. New Zeal. J. Zool. 27, 395–414. doi: 10.1080/03014223.2000.9518249
Inchausti, P., Guinet, C., Koudil, M., Durbec, J.-P., Barbraud, C., Weimerskirch, H., et al. (2003). Inter-annual variability in the breeding performance of seabirds in relation to oceanographic anomalies that affect the Crozet and the Kerguelen sectors of the Southern Ocean. J. Avian Biol. 34, 170–176. doi: 10.1034/j.1600-048X.2003.03031.x
Inger, R., Attrill, M. J., Bearhop, S., Broderick, A. C., Grecian, W. J., Hodgson, D. J., et al. (2009). Marine renewable energy: potential benefits to biodiversity? An urgent call for research. J. Appl. Ecol. 46, 1145–1153. doi: 10.1111/j.1365-2664.2009.01697.x
IUCN (2012). Threats Classification Scheme (Version 3.2). IUCN Red List Threat. Species. Gland: IUCN.
IUCN (2018a). The IUCN Red List of Threatened Species. Version 2017–2013. Available at: www.iucnredlist.org
IUCN (2018b). World Database on Protected Areas. Available at: https://www.iucn.org/theme/protected-areas/our-work/world-database-protected-areas [accessed October 18, 2018].
Jaeger, A., Lebarbenchon, C., Bourret, V., Bastien, M., Lagadec, E., Thiebot, J.-B., et al. (2018). Avian cholera outbreaks threaten seabird species on Amsterdam Island. PLoS One 13:e0197291. doi: 10.1371/journal.pone.0197291
Jenkins, M. (2003). Prospects for biodiversity. Science 302, 1175–1177. doi: 10.1126/science.1088666
Jenouvrier, S., Barbraud, C., and Weimerskirch, H. (2003). Effects of climate variability on the temporal population dynamics of southern fulmars. J. Anim. Ecol. 72, 576–587. doi: 10.1046/j.1365-2656.2003.00727.x
Jenouvrier, S., Barbraud, C., and Weimerskirch, H. (2005). Long-term contrasted responses to climate of two Antarctic seabird species. Ecology 86, 2889–2903. doi: 10.1890/05-0514
Jentsch, A., Kreyling, J., and Beierkuhnlein, C. (2007). A new generation of climate-change experiments: events, not trends. Front. Ecol. Environ. 5:365–374. doi: 10.1890/1540-9295 (2007)5[365:ANGOCE]2.0.CO;2
Jiguet, F., Robert, A., Micol, T., and Barbraud, C. (2007). Quantifying stochastic and deterministic threats to island seabirds: last endemic prions face extinction from falcon peregrinations. Anim. Conserv. 10, 245–253. doi: 10.1111/j.1469-1795.2007.00100.x
Jiménez, S., Domingo, A., Abreu, M., and Brazeiro, A. (2012). Bycatch susceptibility in pelagic longline fisheries: are albatrosses affected by the diving behaviour of medium-sized petrels? Aquat. Conserv. Mar. Freshw. Ecosyst. 22, 436–445. doi: 10.1002/aqc.2242
Jodice, P. G. R., Ronconi, R. A., Rupp, E., Wallace, G. E., and Satgé, Y. (2016). First satellite tracks of the endangered black-capped petrel. Endanger. Species Res. 29, 23–33. doi: 10.3354/esr00697
Jones, H. P., Holmes, N. D., Butchart, S. H. M., Tershy, B. R., Kappes, P. J., Corkery, I., et al. (2016). Invasive mammal eradication on islands results in substantial conservation gains. Proc. Natl. Acad. Sci. U.S.A. 113, 4033–4038. doi: 10.1073/pnas.1521179113
Jones, H. P., Tershy, B. R., Zavaleta, E. S., Croll, D. A., Keitt, B. S., Finkelstein, M. E., et al. (2008). Severity of the effects of invasive rats on seabirds: a global review. Conserv. Biol. 22, 16–26. doi: 10.1111/j.1523-1739.2007.00859.x
Kappes, P. J., and Jones, H. P. (2014). Integrating seabird restoration and mammal eradication programs on islands to maximize conservation gains. Biodivers. Conserv. 23, 503–509. doi: 10.1007/s10531-013-0608-z
Karris, G., Ketsilis-Rinis, V., Kalogeropoulou, A., Xirouchakis, S., Machias, A., Maina, I., et al. (2018). The use of demersal trawling discards as a food source for two scavenging seabird species: a case study of an eastern Mediterranean oligotrophic marine ecosystem. Avian Res. 9:26. doi: 10.1186/s40657-018-0118-5
Kawakami, K., Eda, M., Izumi, H., Horikoshi, K., and Suzuki, H. (2018). Phylogenetic position of endangered Puffinus lherminieri bannermani. Ornithol. Sci. 17, 11–18. doi: 10.2326/osj.17.11
Keogan, K., Daunt, F., Wanless, S., Phillips, R. A., Walling, C. A., Agnew, P., et al. (2018). Global phenological insensitivity to shifting ocean temperatures among seabirds. Nat. Clim. Chang. 8, 313–318. doi: 10.1038/s41558-018-0115-z
Kirby, D. S., and Ward, P. (2014). Standards for the effective management of fisheries bycatch. Mar. Policy 44, 419–426. doi: 10.1016/J.MARPOL.2013.10.008
Kühn, S., Bravo Rebolledo, E. L., and van Franeker, J. A. (2015). “Deleterious effects of litter on marine life,” in Marine Anthropogenic Litter, eds M. Bergmann, L. Gutow, and M. Klages (Cham: Springer International Publishing), 75–116. doi: 10.1007/978-3-319-16510-3_4
Kyba, C. C. M., Kuester, T., Sánchez, de Miguel, A., Baugh, K., Jechow, A., et al. (2017). Artificially lit surface of Earth at night increasing in radiance and extent. Sci. Adv. 3:e1701528. doi: 10.1126/sciadv.1701528
Lambertz, M. (2017). Taxonomy: retain scientific autonomy. Nature 546, 600–600. doi: 10.1038/546600b
Laneri, K., Louzao, M., Martínez-Abraín, A., Arcos, J., Belda, E., Guallart, J., et al. (2010). Trawling regime influences longline seabird bycatch in the Mediterranean: new insights from a small-scale fishery. Mar. Ecol. Prog. Ser. 420, 241–252. doi: 10.3354/meps08847
Lascelles, B., Notarbartolo, Di Sciara, G., Agardy, T., Cuttelod, A., Eckert, S., et al. (2014). Migratory marine species: their status, threats and conservation management needs. Aquat. Conserv. Mar. Freshw. Ecosyst. 24, 111–127. doi: 10.1002/aqc.2512
Lavers, J. L., and Bond, A. L. (2016). Ingested plastic as a route for trace metals in Laysan Albatross (Phoebastria immutabilis) and Bonin Petrel (Pterodroma hypoleuca) from Midway Atoll. Mar. Pollut. Bull. 110, 493–500. doi: 10.1016/j.marpolbul.2016.06.001
Le Corre, M., Ollivier, A., Ribes, S., and Jouventin, P. (2002). Light-induced mortality of petrels: a 4-year study from Réunion Island (Indian Ocean). Biol. Conserv. 105, 93–102. doi: 10.1016/S0006-3207(01)00207-5
Lebreton, L., Slat, B., Ferrari, F., Sainte-Rose, B., Aitken, J., Marthouse, R., et al. (2018). Evidence that the Great Pacific Garbage Patch is rapidly accumulating plastic. Sci. Rep. 8:4666. doi: 10.1038/s41598-018-22939-w
Lescroël, A., Mathevet, R., Péron, C., Authier, M., Provost, P., Takahashi, A., et al. (2016). Seeing the ocean through the eyes of seabirds: a new path for marine conservation? Mar. Policy 68, 212–220. doi: 10.1016/J.MARPOL.2016.02.015
Lewison, R. L., Crowder, L. B., Wallace, B. P., Moore, J. E., Cox, T., Zydelis, R., et al. (2014). Global patterns of marine mammal, seabird, and sea turtle bycatch reveal taxa-specific and cumulative megafauna hotspots. Proc. Natl. Acad. Sci. U.S.A. 111, 5271–5276. doi: 10.1073/pnas.1318960111
Libois, E., Gimenez, O., Oro, D., Mínguez, E., Pradel, R., and Sanz-Aguilar, A. (2012). Nest boxes: a successful management tool for the conservation of an endangered seabird. Biol. Conserv. 155, 39–43. doi: 10.1016/j.biocon.2012.05.020
Longcore, T., Rodríguez, A., Witherington, B., Penniman, J. F., Herf, L., and Herf, M. (2018). Rapid assessment of lamp spectrum to quantify ecological effects of light at night. J. Exp. Zool. Part A Ecol. Integr. Physiol. 329, 511–521. doi: 10.1002/jez.2184
Lopez-Darias, M., Luzardo, J., Martínez, R., González, D., García, E. A., and Cabrera, J. (2011). Poaching vs. patrolling: effects on conservation of Cory’s shearwater Calonectris diomedea borealis colonies. Bird Conserv. Int. 21, 342–352. doi: 10.1017/S0959270910000559
Louzao, M., Igual, J. M., McMinn, M., Aguilar, J. S., Triay, R., and Oro, D. (2006). Small pelagic fish, trawling discards and breeding performance of the critically endangered Balearic shearwater: improving conservation diagnosis. Mar. Ecol. Prog. Ser. 318, 247–254. doi: 10.3354/meps318247
Mace, G. M. (2004). The role of taxonomy in species conservation. Philos. Trans. R. Soc. Lond. B Biol. Sci. 359, 711–719. doi: 10.1098/rstb.2003.1454
Madeiros, J., Carlile, N., and Priddel, D. (2012). Breeding biology and population increase of the endangered Bermuda Petrel Pterodroma cahow. Bird Conserv. Int. 22, 35–45. doi: 10.1017/S0959270911000396
Mallory, M. L., and Braune, B. M. (2012). Tracking contaminants in seabirds of Arctic Canada: temporal and spatial insights. Mar. Pollut. Bull. 64, 1475–1484. doi: 10.1016/j.marpolbul.2012.05.012
Mangel, J. C., Wang, J., Alfaro-Shigueto, J., Pingo, S., Jimenez, A., Carvalho, F., et al. (2018). Illuminating gillnets to save seabirds and the potential for multi-taxa bycatch mitigation. R. Soc. Open Sci. 5:180254. doi: 10.1098/rsos.180254
Maree, B. A., Wanless, R. M., Fairweather, T. P., Sullivan, B. J., and Yates, O. (2014). Significant reductions in mortality of threatened seabirds in a South African trawl fishery. Anim. Conserv. 17, 520–529. doi: 10.1111/acv.12126
Martin, G. R. (2017). The Sensory Ecology of Birds. New York, NY: Oxford University Press. doi: 10.1093/oso/9780199694532.001.0001
Martin, G. R., and Crawford, R. (2015). Reducing bycatch in gillnets: a sensory ecology perspective. Glob. Ecol. Conserv. 3, 28–50. doi: 10.1016/J.GECCO.2014.11.004
Matias, R., and Catry, P. (2010). The diet of Atlantic yellow-legged gulls (Larus michahellis atlantis) at an oceanic seabird colony: estimating predatory impact upon breeding petrels. Eur. J. Wildl. Res. 56, 861–869. doi: 10.1007/s10344-010-0384-y
Mauck, R. A., Dearborn, D. C., and Huntington, C. E. (2018). Annual global mean temperature explains reproductive success in a marine vertebrate from 1955 to 2010. Glob. Chang. Biol. 24, 1599–1613. doi: 10.1111/gcb.13982
McClelland, G. T. W., Altwegg, R., van Aarde, R. J., Ferreira, S., Burger, A. E., and Chown, S. L. (2018). Climate change leads to increasing population density and impacts of a key island invader. Ecol. Appl. 28, 212–224. doi: 10.1002/eap.1642
McClelland, G. T. W., Jones, I. L., Lavers, J. L., and Sato, F. (2008). Breeding biology of Tristram’s storm-petrel Oceanodroma tristrami at French Frigate Shoals and Laysan Island, Northwest Hawaiian Islands. Mar. Ornitol. 36, 175–181.
McClelland, P. J., Coote, R., Trow, M., Hutchins, P., Nevins, H. R. M., Adams, J., et al. (2011). “The Rakiura Titi islands restoration project: community action to eradicate Rattus rattus and Rattus exulans for ecological restoration and cultural wellbeing,” in Island Invasives: Eradication and Management: Proceedings of the International Conference on Island Invasives, eds C. R. Veitch, M. N. Clout, and D. R. Towns (New Zealand: IUCN, Gland and the Centre for Biodiversity and Biosecurity (CBB)), 451–454.
Meier, R. E., Votier, S. C., Wynn, R. B., Guilford, T., McMinn Grivé, M., Rodríguez, A., et al. (2017). Tracking, feather moult and stable isotopes reveal foraging behaviour of a critically endangered seabird during the non-breeding season. Divers. Distrib. 23, 130–145. doi: 10.1111/ddi.12509
Melvin, E. F., Dietrich, K. S., Suryan, R. M., and Fitzgerald, S. M. (2019). Lessons from seabird conservation in Alaskan longline fisheries. Conserv. Biol. doi: 10.1111/cobi.13288 [Epub ahead of print].
Melvin, E. F., Guy, T. J., and Read, L. B. (2013). Reducing seabird bycatch in the South African joint venture tuna fishery using bird-scaring lines, branch line weighting and nighttime setting of hooks. Fish. Res. 147, 72–82. doi: 10.1016/J.FISHRES.2013.04.015
Melvin, E. F., Guy, T. J., and Read, L. B. (2014). Best practice seabird bycatch mitigation for pelagic longline fisheries targeting tuna and related species. Fish. Res. 149, 5–18. doi: 10.1016/J.FISHRES.2013.07.012
Merkel, F., and Barry, T. (2008). Seabird Harvest in the Arctic. CAFF International Secretariat. Available at: http://library.arcticportal.org/468/1/CAFF_Technical_Report_No.16_---_Seabird_Harvest_in_the_Arctic.pdf [accessed June 5, 2018].
Miles, W., Money, S., Luxmoore, R., and Furness, R. W. (2010). Effects of artificial lights and moonlight on petrels at St Kilda. Bird Study 57, 244–251. doi: 10.1080/00063651003605064
Milledge, D. (2010). Research to Inform the Eradication of the Introduced Masked Owl Population on Lord Howe Island. Suffolk Park, NSW: Landmark Ecological Services.
Mitchell, C., Ogura, C., Meadows, D. W., Kane, A., Strommer, L., Fretz, S., et al. (2005). Hawaii’s Comprehensive Wildlife Conservation Strategy. Honolulu, HI: Department of Land and Natural Resources Honolulu.
Montevecchi, W. (2006). “Influences of artificial light on marine birds,” in Ecological Consequences of Artificial Night Lighting, eds C. Rich and T. Longcore (Washington, D.C: Island Press), 94–113.
Montoya, J. M., Donohue, I., and Pimm, S. L. (2017). Planetary boundaries for biodiversity: implausible science, pernicious policies. Trends Ecol. Evol. 33, 71–73. doi: 10.1016/j.tree.2017.10.004
Moreno, C. A., Castro, R., Mújica, L. J., and Reyes, P. (2008). Significant conservation benefits obtained from the use of a new fishing gear in the Chilean Patagonian toothfish fishery. CCAMLR Sci. 15, 79–91.
Munilla, I., Arcos, J. M., Oro, D., Álvarez, D., Leyenda, P. M., and Velando, A. (2011). Mass mortality of seabirds in the aftermath of the Prestige oil spill. Ecosphere 2:art83. doi: 10.1890/ES11-00020.1
Neumann, B., Vafeidis, A. T., Zimmermann, J., and Nicholls, R. J. (2015). Future coastal population growth and exposure to sea-level rise and coastal flooding–a global assessment. PLoS One 10:e0118571. doi: 10.1371/journal.pone.0118571
Nevoux, M., and Barbraud, C. (2006). Relationships between sea ice concentration, sea surface temperature and demographic traits of thin-billed prions. Polar Biol. 29, 445–453. doi: 10.1007/s00300-005-0075-4
Newman, J., Scott, D., Bragg, C., McKechnie, S., Moller, H., and Fletcher, D. (2009). Estimating regional population size and annual harvest intensity of the sooty shearwater in New Zealand. New Zeal. J. Zool. 36, 307–323. doi: 10.1080/03014220909510157
O’Hara, P. D., and Morandin, L. A. (2010). Effects of sheens associated with offshore oil and gas development on the feather microstructure of pelagic seabirds. Mar. Pollut. Bull. 60, 672–678. doi: 10.1016/j.marpolbul.2009.12.008
Oliveira, N. (2016). Status Report for Monteiro’s Storm-petrel Hydrobates monteiroi. Lisboa: Sociedade Portuguesa para o Estudo das Aves.
Oliveira, N., Henriques, A., Miodonski, J., Pereira, J., Marujo, D., Almeida, A., et al. (2015). Seabird bycatch in Portuguese mainland coastal fisheries: an assessment through on-board observations and fishermen interviews. Glob. Ecol. Conserv. 3, 51–61. doi: 10.1016/j.gecco.2014.11.006
Olivier, F., Franeker, J. A., van, Creuwels, J. C. S., and Woehler, E. J. (2005). Variations of snow petrel breeding success in relation to sea-ice extent: detecting local response to large-scale processes? Polar Biol. 28, 687–699. doi: 10.1007/s00300-005-0734-5
Ollason, J. C., and Dunnet, G. M. (1980). Nest failures in the fulmar: the effect of observers. J. F. Ornithol. 51, 39–54.
Oppel, S., Hervias, S., Oliveira, N., Pipa, T., Silva, C., Geraldes, P., et al. (2014). Estimating population size of a nocturnal burrow-nesting seabird using acoustic monitoring and habitat mapping. Nat. Conserv. 7, 1–13. doi: 10.3897/natureconservation.7.6890
Oro, D. (2014). Seabirds and climate: knowledge, pitfalls, and opportunities. Front. Ecol. Evol. 2:79. doi: 10.3389/fevo.2014.00079
Oro, D., de León, A., Minguez, E., and Furness, R. W. (2005). Estimating predation on breeding European storm-petrels (Hydrobates pelagicus) by yellow-legged gulls (Larus michahellis). J. Zool. 265, 421–429. doi: 10.1017/S0952836905006515
Oro, D., Genovart, M., Tavecchia, G., Fowler, M. S., and Martínez-Abraín, A. (2013). Ecological and evolutionary implications of food subsidies from humans. Ecol. Lett. 16, 1501–1514. doi: 10.1111/ele.12187
Paiva, V. H., Ramos, J. A., Nava, C., Neves, V., Bried, J., and Magalhães, M. C. (2018). Inter-sexual habitat and isotopic niche segregation of the endangered Monteiro’s storm-petrel during breeding. Zoology 126, 29–35. doi: 10.1016/j.zool.2017.12.006
Paleczny, M., Hammill, E., Karpouzi, V., and Pauly, D. (2015). Population trend of the world’s monitored seabirds, 1950-2010. PLoS One 10:1–11. doi: 10.1371/journal.pone.0129342
Patrick, S. C., and Weimerskirch, H. (2014). Personality, foraging and fitness consequences in a long lived seabird. PLoS One 9:e87269. doi: 10.1371/journal.pone.0087269
Pearson, S. F., Hodum, P. J., Good, T. P., Schrimpf, M., and Knapp, S. M. (2013). A model approach for estimating colony size, trends, and habitat associations of burrow-nesting seabirds. Condor 115, 356–365. doi: 10.1525/cond.2013.110207
Peck, D. R., Smithers, B. V., Krockenberger, A. K., and Congdon, B. C. (2004). Sea surface temperature constrains wedge-tailed shearwater foraging success within breeding seasons. Mar. Ecol. Prog. Ser. 281, 259–266. doi: 10.3354/meps281259
Pierce, R. J. (1998). The impact of kiore Rattus exulans on two small seabird islands. Ostrich 69, 446.
Pirotta, E., Edwards, E. W. J., New, L., and Thompson, P. M. (2018). Central place foragers and moving stimuli: a hidden-state model to discriminate the processes affecting movement. J. Anim. Ecol. 87, 1116–1125. doi: 10.1111/1365-2656.12830
Podolskyi, R., Ainley, D. G., Spencer, G., Leah, D., and Nur, N. (1998). Mortality of Newell’s shearwaters caused by collisions with urban structures on Kauai. Colon. Waterbirds 21, 20–34. doi: 10.2307/1521727
Pollet, I. L., Hedd, A., Taylor, P. D., Montevecchi, W. A., and Shutler, D. (2014). Migratory movements and wintering areas of Leach’s Storm-Petrels tracked using geolocators. J. F. Ornithol. 85, 321–328. doi: 10.1111/jofo.12071
Poloczanska, E. S., Burrows, M. T., Brown, C. J., García Molinos, J., Halpern, B. S., Hoegh-Guldberg, O., et al. (2016). Responses of marine organisms to climate change across oceans. Front. Mar. Sci. 14, 217–224. doi: 10.3389/fmars.2016.00062
Post, E., Bhatt, U. S., Bitz, C. M., Brodie, J. F., Fulton, T. L., Hebblewhite, M., et al. (2013). Ecological consequences of sea-ice decline. Science 341, 519–524. doi: 10.1126/science.1235225
Priddel, D., Carlile, N., Evans, O., Evans, B., and McCoy, H. (2010). A review of the seabirds of Phillip Island in the Norfolk Island Group. Notornis 57, 113–127.
Provencher, J. F., Avery-Gomm, S., Liboiron, M., Braune, B. M., Macaulay, J. B., Mallory, M. L., et al. (2018). Are ingested plastics a vector of PCB contamination in northern fulmars from coastal Newfoundland and Labrador? Environ. Res. 167, 184–190. doi: 10.1016/J.ENVRES.2018.07.025
Provencher, J. F., Bond, A. L., Avery-Gomm, S., Borrelle, S. B., Bravo Rebolledo, E. L., Hammer, S., et al. (2017). Quantifying ingested debris in marine megafauna: a review and recommendations for standardization. Anal. Methods 9, 1454–1469. doi: 10.1039/C6AY02419J
Pyle, P., Welch, A. J., and Fleischer, R. C. (2011). A new species of shearwater (Puffinus) recorded from Midway Atoll, Northwestern Hawaiian Islands. Condor 113, 518–527. doi: 10.1525/cond.2011.100117
Quillfeldt, P. (2001). Variation in breeding success in Wilson’s storm petrels: influence of environmental factors. Antarct. Sci. 13, 400–409. doi: 10.1017/S0954102001000566
Quillfeldt, P., McGill, R. A. R., and Furness, R. W. (2005). Diet and foraging areas of Southern Ocean seabirds and their prey inferred from stable isotopes: review and case study of Wilson’s storm-petrel. Mar. Ecol. Prog. Ser. 295, 295–304. doi: 10.3354/meps295295
Raine, A. F., Holmes, N. D., Travers, M., Cooper, B. A., and Day, R. H. (2017). Declining population trends of Hawaiian Petrel and Newell’s Shearwater on the island of Kaua’i, Hawaii, USA. Condor 119, 405–415. doi: 10.1650/CONDOR-16-223.1
Ramírez, F., Afán, I., Davis, L. S., and Chiaradia, A. (2017a). Climate impacts on global hot spots of marine biodiversity. Sci. Adv. 3:e1601198. doi: 10.1126/sciadv.1601198
Ramírez, F., Tarroux, A., Hovinen, J., Navarro, J., Afán, I., Forero, M. G., et al. (2017b). Sea ice phenology and primary productivity pulses shape breeding success in Arctic seabirds. Sci. Rep. 7:4500. doi: 10.1038/s41598-017-04775-6
Ramírez, F., Afán, I., Tavecchia, G., Catalán, I. A., Oro, D., and Sanz-Aguilar, A. (2016). Oceanographic drivers and mistiming processes shape breeding success in a seabird. Proc. R. Soc. B 283:20152287. doi: 10.1098/rspb.2015.2287
Ramírez, F., Coll, M., Navarro, J., Bustamante, J., and Green, A. J. (2018). Spatial congruence between multiple stressors in the Mediterranean Sea may reduce its resilience to climate impacts. Sci. Rep. 8:14871. doi: 10.1038/s41598-018-33237-w
Ramos, J. A., Monteiro, L., Sola, E., Moniz, Z., and Encarnacion, S. (1997). Characteristics and competition for nest cavities in burrowing Procellariiformes. Condor 99, 634–641. doi: 10.2307/1370475
Ramos, R., Carlile, N., Madeiros, J., Ramírez, I., Paiva, V. H., Dinis, H. A., et al. (2017). It is the time for oceanic seabirds: tracking year-round distribution of gadfly petrels across the Atlantic Ocean. Divers. Distrib. 23, 794–805. doi: 10.1111/ddi.12569
Ramos, R., and González-Solís, J. (2012). Trace me if you can: the use of intrinsic biogeochemical markers in marine top predators. Front. Ecol. Environ. 10:258–266. doi: 10.1890/110140
Ramos, R., Granadeiro, J. P., Nevoux, M., Mougin, J.-L., Dias, M. P., and Catry, P. (2012). Combined spatio-temporal impacts of climate and longline fisheries on the survival of a trans-equatorial marine migrant. PLoS One 7:e40822. doi: 10.1371/journal.pone.0040822
Ramos, R., Granadeiro, J. P., Rodríguez, B., Navarro, J., Paiva, V. H., Bécares, J., et al. (2013). Meta-population feeding grounds of Cory’s shearwater in the subtropical Atlantic Ocean: implications for the definition of marine protected areas based on tracking studies. Divers. Distrib. 19, 1284–1298. doi: 10.1111/ddi.12088
Rando, J. C., and Alcover, J. A. (2008). Evidence for a second western Palearctic seabird extinction during the last Millenium: the Lava Shearwater Puffinus olsoni. IBIS 150, 188–192. doi: 10.1111/j.1474-919X.2007.00741.x
Rayner, M. J., Clout, M. N., Stamp, R. K., Imber, M. J., Brunton, D. H., and Hauber, M. E. (2007). Predictive habitat modelling for the population census of a burrowing seabird: a study of the endangered Cook’s petrel. Biol. Conserv. 138, 235–247. doi: 10.1016/j.biocon.2007.04.021
Reardon, S. (2011). Fukushima radiation creates unique test of marine life’s hardiness. Science 332:292. doi: 10.1126/science.332.6027.292
Reed, J. R. (1986). Seabird Vision: Spectral Sensitivity and Light-Attraction Behavior. Madison, WI: University of Wisconsin–Madison.
Reed, J. R., Sincock, J. L., and Hailman, J. P. (1985). Light attraction in endangered Procellariiform birds: reduction by shielding upward radiation. Auk 102, 377–383. doi: 10.2307/4086782
Regular, P., Montevecchi, W., Hedd, A., Robertson, G., and Wilhelm, S. (2013). Canadian fishery closures provide a large-scale test of the impact of gillnet bycatch on seabird populations. Biol. Lett. 9:20130088. doi: 10.1098/rsbl.2013.0088
Reid, T. A., Tuck, G. N., Hindell, M. A., Thalmann, S., Phillips, R. A., and Wilcox, C. (2013). Nonbreeding distribution of flesh-footed shearwaters and the potential for overlap with north Pacific fisheries. Biol. Conserv. 166, 3–10. doi: 10.1016/j.biocon.2013.06.006
Reynolds, M. H., Courtot, K. N., Berkowitz, P., Storlazzi, C. D., Moore, J., and Flint, E. (2015). Will the effects of sea-level rise create ecological traps for Pacific island seabirds? PLoS One 10:e0136773. doi: 10.1371/journal.pone.0136773
Richardson, M. E. (1984). Aspects of the ornithology of the Tristan da Cunha Group and Gough Island, 1972–1974. Cormorant 12, 122–201.
Riou, S., Gray, C. M., Brooke, M. D. L., Quillfeldt, P., Masello, J. F., Perrins, C., et al. (2011). Recent impacts of anthropogenic climate change on a higher marine predator in western Britain. Mar. Ecol. Prog. Ser. 422, 105–112. doi: 10.3354/meps08968
Roberts, C. M., O’Leary, B. C., McCauley, D. J., Cury, P. M., Duarte, C. M., Lubchenco, J., et al. (2017). Marine reserves can mitigate and promote adaptation to climate change. Proc. Natl. Acad. Sci. 114, 6167–6175. doi: 10.1073/pnas.1701262114
Robertson, G. J., Russell, J., Bryant, R., Fifield, D. A., and Stenhouse, I. J. (2006). Size and trends of Leach’s storm-petrel Oceanodroma leucorhoa breeding populations in Newfoundland. Atl. Seabirds 8, 41–50.
Rochman, C. M., Browne, M. A., Underwood, A. J., van Franeker, J. A., Thompson, R. C., and Amaral-Zettler, L. A. (2016). The ecological impacts of marine debris: unraveling the demonstrated evidence from what is perceived. Ecology 97, 302–312. doi: 10.1890/14-2070.1
Rockström, J., Steffen, W., Noone, K., Persson,Å, Chapin, F. S., Lambin, E. F., et al. (2009). A safe operating space for humanity. Nature 461, 472–475. doi: 10.1038/461472a
Rodrigues, P., Aubrecht, C., Gil, A., Longcore, T., and Elvidge, C. (2012). Remote sensing to map influence of light pollution on Cory’s shearwater in São Miguel Island, Azores Archipelago. Eur. J. Wildl. Res. 58, 147–155. doi: 10.1007/s10344-011-0555-5
Rodríguez, A., Burgan, G., Dann, P., Jessop, R., Negro, J. J., and Chiaradia, A. (2014). Fatal attraction of short-tailed shearwaters to artificial lights. PLoS One 9:e110114. doi: 10.1371/journal.pone.0110114
Rodríguez, A., Dann, P., and Chiaradia, A. (2017a). Reducing light-induced mortality of seabirds: high pressure sodium lights decrease the fatal attraction of shearwaters. J. Nat. Conserv. 39, 68–72. doi: 10.1016/j.jnc.2017.07.001
Rodríguez, A., Holmes, N. D., Ryan, P. G., Wilson, K.-J., Faulquier, L., Murillo, Y., et al. (2017b). Seabird mortality induced by land-based artificial lights. Conserv. Biol. 31, 986–1001. doi: 10.1111/cobi.12900
Rodríguez, A., Moffett, J., Revoltós, A., Wasiak, P., McIntosh, R. R., Sutherland, D. R., et al. (2017c). Light pollution and seabird fledglings: targeting efforts in rescue programs. J. Wildl. Manage. 81, 734–741. doi: 10.1002/jwmg.21237
Rodríguez, A., García, D., Rodríguez, B., Cardona, E., Parpal, L., and Pons, P. (2015a). Artificial lights and seabirds: is light pollution a threat for the threatened Balearic petrels? J. Ornithol. 156, 893–902. doi: 10.1007/s10336-015-1232-3
Rodríguez, A., Rodríguez, B., and Negro, J. J. (2015b). GPS tracking for mapping seabird mortality induced by light pollution. Sci. Rep. 5:10670. doi: 10.1038/srep10670
Rodríguez, A., and Rodríguez, B. (2009). Attraction of petrels to artificial lights in the Canary Islands: effects of the moon phase and age class. IBIS 151, 299–310. doi: 10.1111/j.1474-919X.2009.00925.x
Rodríguez, A., Rodríguez, B., and Carrasco, M. N. (2012a). High prevalence of parental delivery of plastic debris in Cory’s shearwaters (Calonectris diomedea). Mar. Pollut. Bull. 64, 2219–2223. doi: 10.1016/j.marpolbul.2012.06.011
Rodríguez, A., Rodríguez, B., Curbelo,ÁJ., Pérez, A., Marrero, S., and Negro, J. J. (2012b). Factors affecting mortality of shearwaters stranded by light pollution. Anim. Conserv. 15, 519–526. doi: 10.1111/j.1469-1795.2012.00544.x
Rodríguez, A., Rodríguez, B., and Lucas, M. P. (2012c). Trends in numbers of petrels attracted to artificial lights suggest population declines in Tenerife, Canary Islands. IBIS 154, 167–172. doi: 10.1111/j.1474-919X.2011.01175.x
Ronconi, R. A., Allard, K. A., and Taylor, P. D. (2015). Bird interactions with offshore oil and gas platforms: review of impacts and monitoring techniques. J. Environ. Manage. 147, 34–45. doi: 10.1016/j.jenvman.2014.07.031
Rouxel, Y., and Montevecchi, W. (2018). Gear sustainability assessment of the Newfoundland inshore northern cod fishery. Ocean Coast. Manag. 163, 285–295. doi: 10.1016/J.OCECOAMAN.2018.05.018
Russell, J. C. (2011). “Indirect effects of introduced predators on seabird islands,” in Seabird Islands: Ecology, Invasion and Restoration, eds C. P. H. Mulder, W. B. Anderson, D. R. Towns, and P. J. Bellingham (Oxford: Oxford University Press), 261–280. doi: 10.1093/acprof:osobl/9780199735693.003.0009
Russell, J. C., Innes, J. G., Brown, P. H., and Byrom, A. E. (2015). Predator-free New Zealand: conservation country. Bioscience 65, 520–525. doi: 10.1093/biosci/biv012
Russell, J. C., Meyer, J.-Y., Holmes, N. D., and Pagad, S. (2017a). Invasive alien species on islands: impacts, distribution, interactions and management. Environ. Conserv. 44, 359–370. doi: 10.1017/S0376892917000297
Russell, J. C., Welch, J. R., Dromzée, S., Bourgeois, K., Thoresen, J., Earl, R., et al. (2017b). Developing a National Framework for Monitoring the Grey-Faced Petrel (Pterodroma gouldi) as an Indicator Species. DOC Research and Development Series 350. Wellington: Department of Conservation, 19.
Russell, J. C., Taylor, C. N., and Aley, J. P. (2018). Social assessment of inhabited islands for wildlife management and eradication. Austr. J. Environ. Manag. 25, 24–42. doi: 10.1080/14486563.2017.1401964
Ryan, P. G. (1988). Effects of ingested plastic on seabird feeding: evidence from chickens. Mar. Pollut. Bull. 19, 125–128. doi: 10.1016/0025-326X(88)90708-4
Ryan, P. G. (2015). How quickly do albatrosses and petrels digest plastic particles? Environ. Pollut. 207, 438–440. doi: 10.1016/j.envpol.2015.08.005
Ryan, P. G. (2018). Entanglement of birds in plastics and other synthetic materials. Mar. Pollut. Bull. 135, 159–164. doi: 10.1016/j.marpolbul.2018.06.057
Ryan, P. G. (2019). “Ingestion of plastics by marine organisms,” in Hazardous Chemicals Associated with Plastics in the Environment The Handbook of Environmental Chemistry, eds H. Takada and H. K. Karapanagioti (Cham: Springer International Publishing), 235–266.
Sanz-Aguilar, A., Martínez-Abraín, A., Tavecchia, G., Mínguez, E., and Oro, D. (2009). Evidence-based culling of a facultative predator: efficacy and efficiency components. Biol. Conserv. 142, 424–431. doi: 10.1016/J.BIOCON.2008.11.004
Sato, F., Karino, K., Oshiro, A., Sugawa, H., and Hirai, M. (2010). Breeding of Swinhoe’s storm-petrel Oceanodroma monorhis in the Kutsujima Islands, Kyoto, Japan. Mar. Ornithol. 38, 133–136.
Shaw, J., Terauds, A., and Bergstrom, D. (2011). Rapid commencement of ecosystem recovery following aerial baiting on sub-Antarctic Macquarie Island. Ecol. Manag. Restor. 12, 241–244. doi: 10.1111/j.1442-8903.2011.00611.x
Sherley, R. B., Barham, B. J., Barham, P. J., Campbell, K. J., Crawford, R. J. M., Grigg, J., et al. (2018). Bayesian inference reveals positive but subtle effects of experimental fishery closures on marine predator demographics. Proc. R. Soc. B 285:20172443. doi: 10.1098/rspb.2017.2443
Shirihai, H. (2008). Rediscovery of Beck’s petrel Pseudobulweria becki, and other observations of tubenoses from the Bismarck archipelago, Papua New Guinea. Bull. Br. Ornithol. Club 128, 3–16.
Shirihai, H., Bretagnolle, V., and Wege, D. (2010). Petrels of the Caribbean: the Jamaica Petrel Pelagic Expedition. A Pelagic Expedition Off Jamaica, and Off the Islands of Guadeloupe and Dominica. Available at: https://www.birdscaribbean.org/wp-content/uploads/2015/BCPEWG/Shirihai_Jamaica_AtSea_Nov09.pdf [accessed November 9, 2018].
Simberloff, D. (1998). Flagships, umbrellas, and keystones: is single-species management passe in the landscape era? Biol. Conserv. 83, 247–257. doi: 10.1016/S0006-3207(97)00081-5
Skira, I., Brothers, N., and Pemberton, D. (1996). Distribution, abundance and conservation status of short-tailed shearwaters Puffinus tenuirostris in Tasmania, Australia. Mar. Ornithol. 24, 1–14.
Slater, L., and Byrd, G. V. (2009). Status, trends, and patterns of covariation of breeding seabirds at St Lazaria Island, Southeast Alaska, 1994–2006. J. Biogeogr. 36, 465–475. doi: 10.1111/j.1365-2699.2008.02050.x
Small, C., and Nicholls, R. J. (2003). A global analysis of human settlement in coastal zones. J. Coast. Res. 19, 584–599. doi: 10.2307/4299200
Smith, D. G., Shiinoki, E. K., and VanderWerf, E. A. (2006). Recovery of native species following rat eradication on Mokoli’i Island, O’ahu, Hawai’i. Pacific Sci. 60, 299–303. doi: 10.1353/psc.2006.0012
Soldatini, C., Albores-Barajas, Y. V., Massa, B., and Gimenez, O. (2014). Climate driven life histories: the case of the Mediterranean storm petrel. PLoS One 9:e94526. doi: 10.1371/journal.pone.0094526
Soldatini, C., Albores-Barajas, Y. V., Tagliavia, M., Massa, B., Fusani, L., and Canoine, V. (2015). Effects of human disturbance on cave-nesting seabirds: the case of the storm petrel. Conserv. Physiol. 3, 1–10. doi: 10.1093/conphys/cov041
Sommer, E., Bradfield, P., Dunlop, K., Harrow, G., Morrisey, M., Walford, D., et al. (2009). Population trends, breeding success and predation rates of Hutton’s shearwater (Puffinus huttoni): a 20 year assessment. Notornis 56, 144–153.
Soriano-Redondo, A., Cortés, V., Reyes-González, J. M., Guallar, S., Bécares, J., Rodríguez, B., et al. (2016). Relative abundance and distribution of fisheries influence risk of seabird bycatch. Sci. Rep. 6:37373. doi: 10.1038/srep37373
Spatz, D. R., Holmes, N. D., Reguero, B. G., Butchart, S. H. M., Tershy, B. R., and Croll, D. A. (2017). Managing invasive mammals to conserve globally threatened seabirds in a changing climate. Conserv. Lett. 10, 736–747. doi: 10.1111/conl.12373
Spatz, D. R., Newton, K. M., Heinz, R., Tershy, B., Holmes, N. D., Butchart, S. H. M., et al. (2014). The biogeography of globally threatened seabirds and island conservation opportunities. Conserv. Biol. 28, 1282–1290. doi: 10.1111/cobi.12279
Suazo, C. G., Oliveira, N., Debski, I., Mangel, J. C., Alfaro-Shigueto, J., Azocar, J., et al. (2017). Seabird Bycatch in Purse Seine Fisheries: Status of Knowledge and Mitigation Measures | Bycatch Management Information System (BMIS). Available at: https://www.bmis-bycatch.org/references/pbqazjhs [accessed October 5, 2018].
Sullivan, B. J., Kibel, B., Kibel, P., Yates, O., Potts, J. M., Ingham, B., et al. (2018). At-sea trialling of the Hookpod: a ‘one-stop’ mitigation solution for seabird bycatch in pelagic longline fisheries. Anim. Conserv. 21, 159–167. doi: 10.1111/acv.12388
Sullivan, B. J., Reid, T. A., and Bugoni, L. (2006). Seabird mortality on factory trawlers in the Falkland Islands and beyond. Biol. Conserv. 131, 495–504. doi: 10.1016/J.BIOCON.2006.02.007
Sullivan, W. J., Wilson, K.-J., and Paterson, A. (2000). Influence of artificial burrows and microhabitat on burrow competition between Chatham petrels and broad-billed prions. Emu 100, 329–333. doi: 10.1071/MU00058
Sutherland, D. R., and Dann, P. (2012). Improving the accuracy of population size estimates for burrow-nesting seabirds. IBIS 154, 488–498. doi: 10.1111/j.1474-919X.2012.01234.x
Syposz, M., Gonçalves, F., Carty, M., Hoppitt, W., and Manco, F. (2018). Factors influencing Manx shearwater grounding on the west coast of Scotland. IBIS 160, 846–854. doi: 10.1111/ibi.12594
Sæther, B.-E., and Bakke, Ø (2000). Avian life history variation and contribution of demographic traits to the population growth rate. Ecology 81, 642–653. doi: 10.2307/177366
Tanaka, K., Takada, H., Yamashita, R., Mizukawa, K., Fukuwaka, M., and Watanuki, Y. (2015). Facilitated leaching of additive-derived PBDEs from plastic by seabirds’ stomach oil and accumulation in tissues. Environ. Sci. Technol. 49, 11799–11807. doi: 10.1021/acs.est.5b01376
Tarzia, M., Mulligan, B., Campos, B., and Small, C. (2017). Seabird Bycatch Mitigation in the Mediterranean. Available at: http://www.unepmap.org/index.php?module=content2&catid=001001001 [accessed October 15, 2018].
Taylor, R. S., Bailie, A., Gulavita, P., Birt, T., Aarvak, T., Anker-Nilssen, T., et al. (2018). Sympatric population divergence within a highly pelagic seabird species complex (Hydrobates spp.). J. Avian Biol. 49, 1. doi: 10.1111/jav.01515
Telfer, T. C., Sincock, J. L., Byrd, G. V., and Reed, J. R. (1987). Attraction of hawaiian seabirds to lights: conservation efforts and effects of moon phase. Wildl. Soc. Bull. 15, 406–413.
Thompson, P. M., and Ollason, J. C. (2001). Lagged effects of ocean climate change on fulmar population dynamics. Nature 413, 417–420. doi: 10.1038/35096558
Thomson, S. A., Pyle, R. L., Ahyong, S. T., Alonso-Zarazaga, M., Ammirati, J., Araya, J. F., et al. (2018). Taxonomy based on science is necessary for global conservation. PLoS Biol. 16:e2005075. doi: 10.1371/journal.pbio.2005075
Troy, J., Holmes, N., Veech, J., and Green, M. (2013). Using observed seabird fallout records to infer patterns of attraction to artificial light. Endanger. Species Res. 22, 225–234. doi: 10.3354/esr00547
Troy, J. R., Holmes, N. D., Joyce, T., Behnke, J. H., and Green, M. C. (2016). Characteristics associated with Newell’s Shearwater (Puffinus newelli) and Hawaiian Petrel (Pterodroma sandwichensis) burrows on Kauai, Hawaii, USA. Waterbirds 39, 199–204. doi: 10.1675/063.039.0211
Tuck, G. N., Thomson, R. B., Barbraud, C., Delord, K., Louzao, M., Herrera, M., et al. (2015). An integrated assessment model of seabird population dynamics: can individual heterogeneity in susceptibility to fishing explain abundance trends in Crozet wandering albatross? J. Appl. Ecol. 52, 950–959. doi: 10.1111/1365-2664.12462
van Franeker, J. A., Blaize, C., Danielsen, J., Fairclough, K., Gollan, J., Guse, N., et al. (2011). Monitoring plastic ingestion by the northern fulmar Fulmarus glacialis in the North Sea. Environ. Pollut. 159, 2609–2615. doi: 10.1016/j.envpol.2011.06.008
van Franeker, J. A., and Law, K. L. (2015). Seabirds, gyres and global trends in plastic pollution. Environ. Pollut. 203, 89–96. doi: 10.1016/j.envpol.2015.02.034
VanZandt, M., Delparte, D., Hart, P., Duvall, F., and Penniman, J. (2014). Nesting characteristics and habitat use of the endangered Hawaiian petrel (Pterodroma sandwichensis) on the island of Lâna’i. Waterbirds 37, 43–51. doi: 10.1675/063.037.0107
Veit, R., McGowan, J., Ainley, D., Wahl, T., and Pyle, P. (1997). Apex marine predator declines ninety percent in association with changing oceanic climate. Glob. Chang. Biol. 3, 23–28. doi: 10.1046/j.1365-2486.1997.d01-130.x
Vertigan, C., McMahon, C. R., Andrews-Goff, V., and Hindell, M. A. (2012). The effect of investigator disturbance on egg laying, chick survival and fledging mass of short-tailed shearwaters (Puffinus tenuirostris) and little penguins (Eudyptula minor). Anim. Welf. 21, 101–111. doi: 10.7120/096272812799129493
Vidal, E., Medail, F., and Tatoni, T. (1998). Is the yellow-legged gull a superabundantbird species in the Mediterranean? Impacton fauna and flora, conservation measuresand research priorities. Biodivers. Conserv. 7, 1013–1026. doi: 10.1023/A:1008805030578
Villard, P., Dano, S., and Bretagnolle, V. (2006). Morphometrics and the breeding biology of the Tahiti Petrel Pseudobulweria rostrata. IBIS 148, 285–291. doi: 10.1111/j.1474-919X.2006.00528.x
Wanless, R. M., Angel, A., Cuthbert, R. J., Hilton, G. M., and Ryan, P. G. (2007). Can predation by invasive mice drive seabird extinctions? Biol. Lett. 3, 241–244. doi: 10.1098/rsbl.2007.0120
Wanless, R. M., and Maree, B. A. (2014). Problems and solutions for seabird bycatch in trawl fisheries. Anim. Conserv. 17:534. doi: 10.1111/acv.12183
Warham, J. (1996). The Behaviour, Population Biology and Physiology of the Petrels. San Diego, CA: Academic Press.
Watson, H., Bolton, M., and Monaghan, P. (2014). Out of sight but not out of harm’s way: human disturbance reduces reproductive success of a cavity-nesting seabird. Biol. Conserv. 174, 127–133. doi: 10.1016/j.biocon.2014.03.020
Waugh, S. M., Baker, G. B., Gales, R., and Croxall, J. P. (2008). CCAMLR process of risk assessment to minimise the effects of longline fishing mortality on seabirds. Mar. Policy 32, 442–454. doi: 10.1016/J.MARPOL.2007.08.011
Waugh, S. M., Barbraud, C., Adams, L., Freeman, A. N. D., Wilson, K.-J., Wood, G., et al. (2015). Modeling the demography and population dynamics of a subtropical seabird, and the influence of environmental factors. Condor 117, 147–164. doi: 10.1650/CONDOR-14-141.1
Weimerskirch, H., Pinet, P., Dubos, J., Andres, S., Tourmetz, J., Caumes, C., et al. (2019). Wettability of juvenile plumage as a major cause of mortality threatens endangered Barau’s petrel. J. Avian Biol. 50. doi: 10.1111/jav.02016
Whitehead, A. L., Lyver, P. O. B., Jones, C. J., Bellingham, P. J., MacLeod, C. J., Coleman, M., et al. (2014). Establishing accurate baseline estimates of breeding populations of a burrowing seabird, the grey-faced petrel (Pterodroma macroptera gouldi) in New Zealand. Biol. Conserv. 169, 109–116. doi: 10.1016/j.biocon.2013.11.002
Wiese, F. K., Montevecchià, W. A., Davorenà, G. K., Huettmann, F., and Diamond, A. W. (2001). Seabirds at risk around oil shore platforms in the North-west Atlantic. Mar. Pollut. Bull. 42, 1285–1290. doi: 10.1016/S0025-326X(01)00096-0
Wiese, F. K. F. K., and Robertson, G. J. G. J. (2004). Assessing seabird mortality from chronic oil discharges at sea. J. Wildl. Manage. 68, 627–638. doi: 10.2193/0022-541X (2004)068[0627:ASMFCO]2.0.CO;2
Wilhelm, S. I., Robertson, G. J., Ryan, P. C., and Schneider, D. C. (2007). Comparing an estimate of seabirds at risk to a mortality estimate from the November 2004 Terra Nova FPSO oil spill. Mar. Pollut. Bull. 54, 537–544. doi: 10.1016/j.marpolbul.2006.12.019
Wood, J. R., Lawrence, H. A., Scofield, R. P., Taylor, G. A., Lyver, P. O., and Gleeson, D. M. (2016). Morphological, behavioural, and genetic evidence supports reinstatement of full species status for the grey-faced petrel, Pterodroma macroptera gouldi (Procellariiformes: Procellariidae). Zool. J. Linn. Soc. 179, 201–216. doi: 10.1111/zoj.12432
Yeh, Y.-M., Huang, H.-W., Dietrich, K. S., and Melvin, E. (2013). Estimates of seabird incidental catch by pelagic longline fisheries in the South Atlantic Ocean. Anim. Conserv. 16, 141–152. doi: 10.1111/j.1469-1795.2012.00588.x
Zabala, J., Zuberogoitia, I., Martínez-Climent, J. A., and Etxezarreta, J. (2011). Do long lived seabirds reduce the negative effects of acute pollution on adult survival by skipping breeding? A study with European storm petrels (Hydrobates pelagicus) during the “Prestige” oil-spill. Mar. Pollut. Bull. 62, 109–115. doi: 10.1016/j.marpolbul.2010.09.004
Zeller, D., Cashion, T., Palomares, M., and Pauly, D. (2018). Global marine fisheries discards: a synthesis of reconstructed data. Fish Fish. 19, 30–39. doi: 10.1111/faf.12233
Zidat, T., Dell’Ariccia, G., Gabirot, M., Sourrouille, P., Buatois, B., Celerier, A., et al. (2017). Reproductive isolation maintains distinct genotypes, phenotypes and chemical signatures in mixed colonies of the two European Calonectris shearwaters (Procellariiformes: Procellariidae). Zool. J. Linn. Soc. 181, 711–726. doi: 10.1093/zoolinnean/zlx002
Keywords: management, marine environment, marine predator, population dynamics, Procellariiformes, research priorities, seabird conservation, threats
Citation: Rodríguez A, Arcos JM, Bretagnolle V, Dias MP, Holmes ND, Louzao M, Provencher J, Raine AF, Ramírez F, Rodríguez B, Ronconi RA, Taylor RS, Bonnaud E, Borrelle SB, Cortés V, Descamps S, Friesen VL, Genovart M, Hedd A, Hodum P, Humphries GRW, Le Corre M, Lebarbenchon C, Martin R, Melvin EF, Montevecchi WA, Pinet P, Pollet IL, Ramos R, Russell JC, Ryan PG, Sanz-Aguilar A, Spatz DR, Travers M, Votier SC, Wanless RM, Woehler E and Chiaradia A (2019) Future Directions in Conservation Research on Petrels and Shearwaters. Front. Mar. Sci. 6:94. doi: 10.3389/fmars.2019.00094
Received: 20 November 2018; Accepted: 18 February 2019;
Published: 18 March 2019.
Edited by:
Mark Meekan, Australian Institute of Marine Science (AIMS), AustraliaReviewed by:
Luke Einoder, Northern Territory Government, AustraliaScott A. Shaffer, San Jose State University, United States
Copyright © 2019 Rodríguez, Arcos, Bretagnolle, Dias, Holmes, Louzao, Provencher, Raine, Ramírez, Rodríguez, Ronconi, Taylor, Bonnaud, Borrelle, Cortés, Descamps, Friesen, Genovart, Hedd, Hodum, Humphries, Le Corre, Lebarbenchon, Martin, Melvin, Montevecchi, Pinet, Pollet, Ramos, Russell, Ryan, Sanz-Aguilar, Spatz, Travers, Votier, Wanless, Woehler and Chiaradia. This is an open-access article distributed under the terms of the Creative Commons Attribution License (CC BY). The use, distribution or reproduction in other forums is permitted, provided the original author(s) and the copyright owner(s) are credited and that the original publication in this journal is cited, in accordance with accepted academic practice. No use, distribution or reproduction is permitted which does not comply with these terms.
*Correspondence: Airam Rodríguez, YWlyYW1yZ3VlekBlYmQuY3NpYy5lcw== André Chiaradia, YWNoaWFyYWRpYUBwZW5ndWlucy5vcmcuYXU=