- Departament de Biologia Marina i Oceanografia, Institut de Ciències del Mar, CSIC, Barcelona, Spain
Human activities on land result in the high-level production of nutrients. When these nutrients reach coastal waters, they could drive the eutrophication process. Here we present the Land Uses Simplified Index (LUSI), an easy-to-use tool for assessing continental pressures on coastal waters. This assessment is done by indirectly estimating continental nutrient loads and concentrations, and their influence on coastal waters. LUSI is based on systematic information describing both the land uses that influence coastal waters by providing nutrient-rich freshwater inflows (urban, industrial, agricultural, and riverine) and the coastline morphology, which can modify this influence, as it determines the degree of coastal water confinement and therefore the likelihood that these inflows will be diluted. A low LUSI value indicates that coastal waters are not or only slightly influenced by continental pressures and/or that these pressures are diluted. On the contrary, a high LUSI value indicates that coastal waters are strongly influenced by continental pressures and/or that these pressures are not diluted. LUSI fulfills a methodological gap, as a simple method to assess coastal pressures when there is a lack of information. Furthermore, it fulfills the requirement of the Water Framework Directive for a true pressure assessment (i.e., not confounded with impact), which for coastal waters imply using pressure data from land. An additional and important feature of LUSI is that it allows the establishment of pressure-impact relationships with impact indicators, such as those related to the Biological Quality Elements of the above Directive. For example, a relationship based on LUSI, as a proxy of pressure, and on the chlorophyll-a concentration, as a proxy of phytoplanktonic biomass and, thus, of the eutrophication impact. By providing insights into the land uses that trigger eutrophication in coastal waters, LUSI aids in the design of measures aimed at remediating anthropogenic damage caused to the environment.
Introduction
In marine ecosystems, coastal areas are of major environmental, economic, and social importance. They are among the most diverse and biologically productive ecosystems on Earth, with the net primary production of phytoplankton estimated to be 50 Pg C y−1 (Malone et al., 2017). Coastal areas also provide other important ecological functions, including filtering terrestrial inflows, and offering food and shelter for a wide variety of organisms (Creel, 2003). For humans, they are “golden areas” of marine socioeconomic development (Zhang, 2012), sustaining major activities related to agriculture, fisheries, industry, urbanization, transport, and tourism (Springer et al., 1996; Cole and McGlade, 1998; San Vicente, 1999; Creel, 2003; Lopez y Royo et al., 2009). Coastal areas comprise 20% of the Earth's surface yet contain over 45% of the entire human population (Schäfer et al., 2010). The population in low-elevation coastal zones (below 10 m of elevation) in the year 2000 was 625.2 million people and is expected to increase to 938.9 million people by the year 2030 and 1,318.3 million people by the year 2060 (Neumann et al., 2015). Therefore, coastal areas are strongly affected by anthropogenic pressure, in the form of high population densities and intense human activities (Lopez y Royo et al., 2009). Coastal development has the potential to produce an excess of nutrients that are delivered to coastal waters via several routes, such as rivers, run-off, and submarine groundwater discharges. Moreover, human activities modify water flows, such as by the construction of dams, increased water extraction, and the deviation of rivers, resulting in profoundly altered nutrient loads, and concentrations (Scialabba, 1998) arriving at coastal waters. Consequently, the risk of eutrophication is higher in coastal waters than in other marine ecosystems.
Several policies have been enacted throughout the world with the aim of restoring and protecting coastal waters, including the Clean Water Act (United States Congress., 1972), The Water Act 2007 (Australia Office of Parliamentary Counsel., 2007), the Water Framework Directive (WFD) (European Commission, 2000), and the Marine Strategy Framework Directive (European Commission, 2008). For all of them, one of the main objectives is to combat eutrophication, which implies an ability to assess both its impact and the pressures that give rise to it, and therefore devise remediation measures. In the case of the WFD, it mandates assessments of true coastal pressures (i.e., not confounded with impact) and the establishment of pressure-impact relationships.
Pressure and impact related to coastal eutrophication have been broadly examined. Among the methods used to assess the degree of eutrophication of coastal waters, chlorophyll-a values are the most widely accepted impact indicator (Bricker et al., 2007; Ferreira et al., 2007; Nixon, 2009; Borja et al., 2012). Although coastal pressures are not easy to quantify and proof of their impact is hard to obtain, several assessment methods are available. Quantitative methods are typically based on readily measurable variables, such as wastewater discharges or human population size (Bonamano et al., 2016; Cai et al., 2016). Others evaluate land use from land cover maps (Comeleo et al., 1996; Jordan et al., 1997; Nedwell et al., 2002; Giupponi and Vladimirova, 2006; Rodriguez et al., 2007; Xian et al., 2007; Lopez y Royo et al., 2009, 2010; Tran et al., 2010) or satellite images (Barale and Folving, 1996; Stefanov et al., 2001; Lopez y Royo and Casazza, 2007; Xiao and Weng, 2007). Furthermore, Aighewi et al. (2013) proposed incorporating land use and land cover information into pressure models to obtain a more holistic assessment of coastal pressures. Semi-quantitative methods have also been proposed, including by Aubry and Elliott (2006); Lopez y Royo et al. (2009); European Commission (2011a); Neto and Juanes (2014), and Batista et al. (2014). These methods yield an approximation of a quantity, usually by a gradient of categories; for instances low, moderate or high population density. Other authors, including Andrulewicz and Witek (2002); Borja et al. (2011), and Weisberg et al. (2008), have advocated pressure assessments based on expert judgment. However, most of the various proposed methodologies to assess coastal pressures are complex or require large amounts of data.
Here we present the Land Uses Simplified Index (LUSI), an easy-to-use tool to assess continental pressures on coastal waters, and a case study to highlight its utility, from the Catalan coast (NW Mediterranean). The first aim of that study was to use LUSI to assess continental pressures on coastal waters and the second to establish a pressure-impact relationship based on LUSI and chlorophyll-a concentrations, as a proxy of phytoplanktonic biomass and, thus, of the eutrophication impact. Additional aims were to validate LUSI against dissolved inorganic nutrient concentrations measured in coastal waters and to determine whether land cover map selection influences the results of LUSI. Furthermore, LUSI utility to assess continental pressures and to establish pressure-impact relationships with the Biological Quality Elements of the WFD in other coastal areas were explored bibliographically. The results demonstrate the strengths of LUSI, its ability to improve scientific knowledge regarding coastal eutrophication, and its applications, especially in the development of remediation measures.
Land Uses Simplified Index (LUSI)
The main objective of LUSI is to assess coastal pressures related to eutrophication. LUSI serves as a proxy enabling the indirect assessment of continental nutrient loads and concentrations, and their dilution in coastal waters. Therefore, it estimates the eutrophication risk of coastal waters. It is based on systematic information describing both the anthropogenic land uses that influence coastal waters (urban, industrial, agricultural, and riverine) and coastline morphology. The latter determines the degree of coastal water confinement and therefore the likelihood that continental freshwater inflows and the nutrients they contain will be diluted. LUSI not only fulfills the methodological gap, by providing a simple method to assess coastal pressures when there is a lack of information, but also the requirements of the WFD, by yielding a true pressure assessment (i.e., not confounded with impact) and allowing the establishment of pressure-impact relationships with impact indicators, such as those related to the Biological Quality Elements of the WFD.
Rationale
Ketchum (1972) defined the coastal area as the band of dry land and adjacent ocean space (water and submerged land) in which terrestrial processes and land uses directly affect oceanic processes and uses, and vice versa. One of the most important coastal process boosted by continental pressures is eutrophication. Eutrophication is driven by nutrients and it has been greatly enhanced by human activities on land, which result in the high-level production of nutrients that reach coastal waters (Chislock et al., 2013). Accordingly, the rationale for LUSI is based on the following assumptions:
I Coastal land uses determine nutrient loads and concentrations of continental freshwater inflows reaching coastal waters. The nutrient richness of these inflows forms a gradient that ranges from freshwaters with minimum nutrient values, such as those within areas where anthropogenic activities are minimal, to the maximum values generated in areas where intense anthropogenic activities pose a high risk of inducing eutrophication (Table 1). Based on this gradient, continental freshwater inflows can be classified as: (i) natural and non-irrigated (rain-watered) agricultural sources, (ii) irrigated agricultural sources, (iii) industrial sources, and (iv) urban sources. The source indicates the pressure of a particular inflow on coastal waters. Coastal areas affected by riverine inflows are considered separately.
II Coastal waters with riverine inflows are influenced by the sum of watershed land uses. Therefore, in these cases the influence of the river should be added to the influence of the land uses performed in the neighboring continent (I). Riverine pressure is mainly a mixture of influences of natural and anthropic origin with different nutrient richness. However, for simplicity reasons LUSI does not include the discerning of the riverine pressure origin and assumes a standard nutrient concentration for riverine inflows. Accordingly, riverine pressure can be assessed using the mean coastal water salinity, as an inverse measure of freshwater content. A lower salinity implies the arrival of greater freshwater inflows from the continent and higher nutrient loads into coastal waters and, thus, a higher pressure. Maximum salinity occurs in the complete absence of freshwater inflows.
III Coastline morphology can modify the influence of continental pressures on coastal waters. It determines the degree of coastal water confinement and therefore the likelihood that continental freshwater inflows and the nutrients they contain will be diluted. In concave areas, such as bays, water is confined, residence times are long and water circulation is reduced. Consequently, continental freshwater inflows are diluted at a low rate, potentially leading to rising nutrient concentrations and an enhanced risk of eutrophication. By contrast, in convex areas, such as headlands, inflows are easily diluted, and the risk of eutrophication is attenuated. Straight coastlines do not modify the influence of continental pressures reaching coastal waters.
Requirements
LUSI requires information on the anthropogenic land uses that influence coastal waters and on coastline morphology. The pressures taken into account by LUSI include agricultural (irrigated land only), industrial, and urban land uses as well as riverine effects. Information on land uses and coastline morphology is available from different sources, including governmental sources, such as census data, satellite maps, such as those from Landsat or Google Earth, airplane, and drone survey images or combinations of them, as suggested by Lautenbach et al. (2011). However, land cover maps are the most useful for the calculation of LUSI, as they provide information on land use, the area occupied by the various types of land use, and the morphology of the coastline. There are several publicly available land cover maps with different degrees of coverage (continent, country, and region). Their appropriateness with respect to LUSI depends on the area of interest or whether distinct areas will be compared. For example, for Catalonia, in the NW Mediterranean, three maps are available: the Coordination of Information of the Environment land cover map [CORINE land cover map or CLCM; European Enviromental Agency (2012)], which covers Europe; the Sistema de Información sobre Ocupación del Suelo de España [SIOSE; Instituto Geográfico Nacional. (2011)], which covers Spain; and the Mapa de Cobertes del Sòl de Catalunya [MCSC; Centre de Recerca Ecològica i Aplicacions Forestals (2009)], which covers Catalonia. To determine riverine influences, the mean salinity value of the coastal water area of interest must be obtained. A truly representative value implies the need for a raw dataset acquired by a sampling frequency sufficient to capture the variability in the salinity. Such information is sometimes available from water management or environmental agencies. Ideally, for the calculation of LUSI, the land cover map and salinity dataset should cover the same time period.
Protocol
To calculate LUSI for a coastal water area, its quantitative information on pressures is classified into categories and assigned a score; then, all the scores are summed and multiplied by a correction factor related to coastline morphology. The protocol to calculate the LUSI is as follows:
I Pressure categories and their corresponding scores are assigned to describe urban, agricultural (irrigated land) and industrial pressures. The assignment depends on the percentage of land coverage accounted for by the respective activities in the continental area of study, which by definition extends from the coastline to 1.5 km inland. The percentage of land coverage is calculated using GIS software and a land cover map. Urban and agricultural pressures are divided into three categories and industrial pressure into two, and each category is associated with a score (Table 2). The three categories of urban and agricultural pressures were established considering low, moderate, and high percentage of land coverage and the two categories of industrial pressures considering low and high coverage, as a simple way to divide these gradients. Besides, the extension of the continental area of study was established as the land area that influences most directly coastal waters.
II A pressure category and its corresponding score are assigned to describe riverine pressure. In this case, the assignment depends on the mean salinity of the studied coastal water area. This gradient ranges from 0 to the maximum salinity occurring in the complete absence of freshwater inflows. For example, for Catalonia, the maximum salinity was established at 38.4. Riverine pressure is divided into three categories and each one is associated with a score (Table 2). These three categories were established following those of the Water Framework Directive Intercalibration Process for the Mediterranean Sea regarding the specific typology for Biological Quality Element Phytoplankton (Camp et al., 2016).
III The four pressure scores are summed. The resulting value summarizes the continental pressures reaching the studied coastal waters. Each score was established to have the same weight. However, similar categories of different pressures have different scores, following the gradient stated in the rationale regarding the nutrient richness of freshwater inflows affected by different land uses (I). For example, urban pressure was given higher scores than the other pressures.
IV The summed scores are multiplied by a coastline correction factor to obtain the LUSI value. This factor is used to consider the effect of coastline morphology on the influence of continental pressures on coastal waters and it is based on the shape of the coastline of the study area. For a concave coastline, where the confinement of coastal waters enhances the influence of continental pressures, the correction factor is 1.25; for a convex coastline, where the influence is diminished by the high dilution rates of freshwater inflows, the correction factor is 0.75; for a straight coastline, the influence is unchanged and the correction factor is 1.00 (Table 3). These values were chosen because they modify the influence of continental pressures on coastal waters but they cannot alter it in a considerable way. For example, coastal waters that receive large amounts of continental pressures will be highly influenced by them even if their dilution capacity is high. Thus, LUSI is calculated following Equation (1):
LUSI values provide a semi-quantitative assessment of continental pressures on the coastal waters of the studied area. They have no units and range from 0.75 to 8.75. A low LUSI value indicates that coastal waters are not or only slightly influenced by continental pressures and/or that these pressures are diluted. On the contrary, a high LUSI value indicates that coastal waters are strongly influenced by continental pressures and/or that these pressures are not diluted.
LUSI is to be applied where there is no previous information on continental pressures on coastal waters. However, if information from the area of study is available, such as punctual or diffuse continental nutrients loads reaching coastal waters or coastal waters residence times, the LUSI can be modified to include it. For example, for concave coastal areas with long residence time it can be assumed that the continental inflows would be diluted at a lower rate than in concave areas with short residence time. In the former case, the confinement correction factor for concave coastal morphology can be increased accordingly to the residence time to obtain a more accurate value of the influence of land pressures on the studied coastal area.
Case study: Catalan coast (NW Mediterranean)
Materials and Methods
Study Area
The Catalan coast (Figure 1) is located between 3° 19′ 59.94″ E and 42° 29′ 0.09″ N and between 0° 9′ 41.69″ E and 40° 31′ 27.56″ N. It occupies 7257.50 km2 (Agència Catalana de l'Aigua, 2005a) and delimits the Mediterranean Sea over 870.0 km (Institut d'Estadística de Catalunya., 2010). The geography, demographics, and socioeconomic development of the Catalan coast are representative of the NW Mediterranean coast (Flo et al., 2011b).
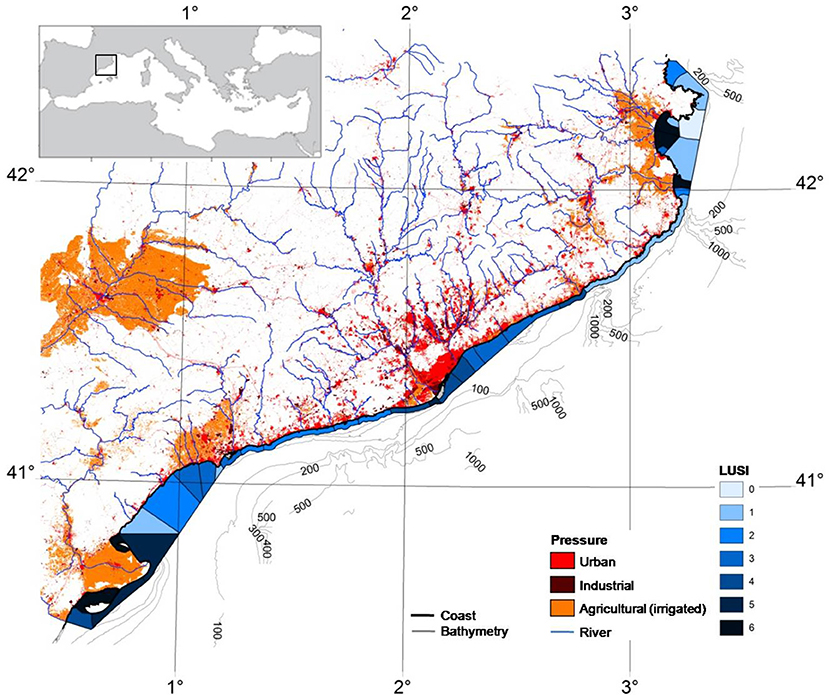
Figure 1. Map of the Catalan coast showing the continental pressures related to LUSI (Land Uses Simplified Index) and the LUSI values of the different coastal water bodies calculated using the 2009 Mapa de Cobertes del Sòl de Catalunya. Urban land use is shown in red, industrial land use in brown and agricultural (irrigated) land use in orange. Rivers are shown in blue and the coastline in black. Water bodies are also shown in blue, with the shade depending on the LUSI value (assigned based on direct integer values). Harbor water bodies were not assessed and are indicated in gray, as is the bathymetry.
The continental topography ranges from rocky and steep headlands to sandy and flat bays. There are also deltaic areas, the most important of which is the Ebre delta (Serra and Canals, 1992). This landscape encompasses several watersheds, which consist of ephemeral streams, nine medium to small rivers, and the Ebre River in the south, all of which open directly into the Mediterranean Sea. The Ebre River drains a watershed of 84,230 km2, with a mean water discharge at the river's mouth of 416 m3/s (Ludwig et al., 2009). Other major rivers in Catalonia drain an area of 13,400 km2 and have a mean water discharge of 0.3–16.3 m3/s (Liquete et al., 2009). Land use differs along the river basins, with agriculture accounting for 0.4–49.2%, forests for 17.2–77.2%, and urban areas for 5.5–81.7% (Institut d'Estadística de Catalunya, 2015). Agricultural land use is relatively important in southern river basins and urbanization in central river basins. In terms of surface area, 13.8% of the coastal zone is urbanized (Biblioteca del consorci el Far., 2010; Institut d'Estadística de Catalunya, 2015). The population in the coastal zone is 4,942,044 inhabitants, which represents 66% of the total population in Catalonia (Institut d'Estadística de Catalunya., 2016). However, the population density is highly variable along the coast, ranging from 33 inhabitants/km2 in the coastal area of the Ebre basin (Ferré, 2007) to 15,319.6 habitants/km2 in the Barcelona metropolitan area (Institut d'Estadística de Catalunya., 2016).
The underwater topography is complex: the continental shelf is usually narrow [average = 15–20 km; Maldonado (1995)] but wide along its border with the Ebre delta (maximum = 54.5 km), and almost non-existent (minimum = 1.6 km) in front of several canyons located along the coast (Platónov, 2002). The general surface circulation is due to the Liguro-Provençal current, which moves from the NE toward the SW; close to the shore, local superficial currents move toward the NE or also toward the SW (Agència Catalana de l'Aigua, 2005b). The tidal range is small and the sea weather, according to the Douglas scale (Harbord, 1897), is typically described as slight and occasionally as rough or very rough, especially during autumn (Agència Catalana de l'Aigua, 2005b).
Following the implementation of the WFD, the Catalan coast was divided into 36 water bodies (C01 to C35, from NE to SW), including the two bays situated in the north (T01) and south (T03) of the mouth of the Ebre River (Figure 1; Supplementary Table 4).
Data Collection
Pressure information to calculate LUSI was obtained from two sources. First, the land cover map of Catalonia, the MCSC, was used to determine the three land use pressures [urban, agricultural (irrigated), and industrial] and to establish the coastal morphology of each water body. The most recently available version of the map is from 2009. Second, the riverine pressure was determined by using the salinity data from the National Catalan Coastal Water Monitoring Program, conducted by the Catalan Water Agency (ACA) in collaboration with the Marine Science Institute (ICM-CSIC). As the implementation of the WFD follows 6-year cycles, the salinity data covered the period between 2007 and 2012 and matched the year represented by the land cover map.
Dissolved inorganic nutrient concentrations, which are used to validate LUSI, were also gathered for the same period and program.
To test whether the choice of the land cover map influences the results of LUSI, LUSI was also calculated using the land cover map of Europe, the CLCM, from 2006. The characteristics of the two maps are detailed in Table 4.
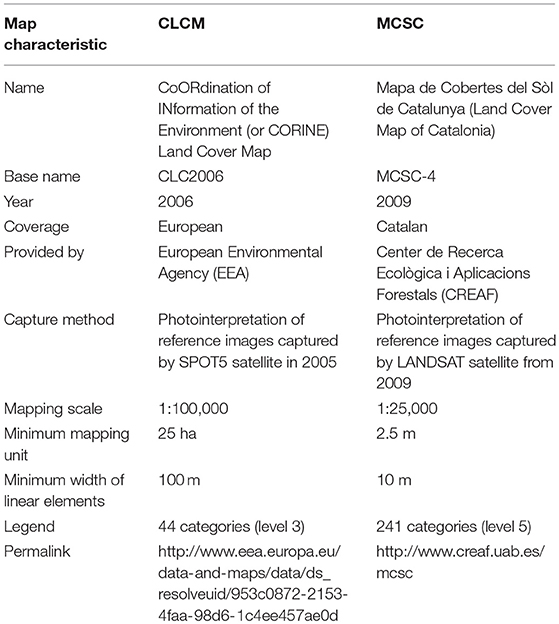
Table 4. Characteristics of the 2006 Coordination of Information of the Environment land cover map (CLCM) and the 2009 Mapa de Cobertes del Sòl de Catalunya (MCSC).
Chlorophyll-a concentrations to establish a pressure-impact relationship together with LUSI values were also obtained for the same period and Program.
Data on salinity, chlorophyll-a and dissolved inorganic nutrient concentrations were collected from 80 sampling stations in Catalan coastal inshore waters (Flo, 2014) (Supplementary Figure 1). These stations are located between 0 and 200 m from the shore, depending on the water depth (<2 m depth), and are distributed along all water bodies, with 1–7 stations per water body, depending on the coastal length. The surface water at the stations was sampled 4 or 12 times a year, depending on the variability of the measurements, previously established. Salinity was directly measured using a WTW probe (model 315). Total chlorophyll-a was quantified in 60-mL subsamples filtered through 25-mm Whatman GF/F glass-fiber filters that were stored frozen. The filters were subsequently extracted in 8 mL of 90% acetone for 48 h, and concentrations of chlorophyll-a (μg/L) were measured using a Turner Designs fluorometer, following the method of Yentsch and Menzel (1963). Dissolved inorganic nutrients were determined in 50-mL subsamples that had been frozen upon their arrival in the laboratory. Nitrate (NO3), nitrite (NO2), ammonium (NH4), phosphate (PO4), and silicate (SiO4) concentrations (μM) were measured using an autoanalyzer (Evolution II, from Alliance Instruments, and AA3 HR Bran+Luebbe, from Seal Analytical) and the colorimetric techniques of Grasshoff et al. (1983).
Data Processing and Statistics
Information on land use pressures was processed from CLCM and MCSC as follows. The 44 categories of the third-level legend of the CLCM and the 241 categories of the fifth-level legend of the MCSC were assigned either to the three land use pressure types considered within LUSI [urban, agricultural (irrigated), or industrial] or to the category “other” (Supplementary Tables 1, 2). The percentage of land coverage related to these pressures was established using Miramon software (Pons, 1994) for each map and water body, after which pressure scores were assigned accordingly. The low urban scores of two water bodies located in the Barcelona Metropolitan Area (C21-Llobregat and C22-El Prat de Llobregat-Castelldefels) were later changed by expert judgment to the maximum urban score. This modification was performed because the maps greatly underestimated the urban score in the area of land neighboring these water bodies. For example, several areas of land within the airport premises were classified as meadows and grasslands according to level 5 of the MCSC, but they should have been classified as airport. The same changes were made for the CLCM and MCSC.
Information on riverine pressure (salinity) and on dissolved inorganic nutrient and chlorophyll-a concentrations was processed as follows. During the study period, 3,562 samples were collected, with 23–300 samples per water body, depending on its size. The mean salinity of each water body was calculated and a riverine pressure score assigned accordingly. The 90th percentiles of the chlorophyll-a and dissolved inorganic nutrient concentrations were determined for each water body using six different methodologies [methodologies 4–9 from Hyndman and Fan (1996)]. This statistical parameter was chosen following the WFD intercalibration process agreements for chlorophyll-a. Since chlorophyll-a and dissolved inorganic nutrient concentrations are not normally distributed (as determined from histograms and Shapiro-Wilk tests), the obtained values were log-transformed following Equation (2):
as is commonly done for environmental data (Cassie, 1962; Legendre and Legendre, 1979; Heyman et al., 1984; Zar, 1984). The mean and standard deviation of the dissolved inorganic nutrient and chlorophyll-a concentrations were calculated for each water body with their six log-transformed 90th percentiles.
The continental pressures on coastal waters were then assessed by calculating LUSI for each water body based on the CLCM and MCSC maps, using the previously assigned pressure scores and taking into account the morphology of the coast.
To validate LUSI against dissolved inorganic nutrient concentrations and to establish a pressure-impact relationship based on LUSI and chlorophyll-a concentrations, first, the corresponding Spearman correlations (ρ) were determined together with the respective p-values. Linear models between LUSI values and dissolved inorganic nutrient concentrations and between LUSI values and chlorophyll-a concentrations were then adjusted, their p-values were calculated, the goodness of fit (R2) determined and the diagnostics checked. The means of the six transformed 90th percentiles of dissolved inorganic nutrient and chlorophyll-a concentrations were used to adjust the models; the standard deviations of the same variables were used to weight the models; and for each model the 90% confidence interval was calculated.
To test whether the choice of land cover map affects the results of LUSI, the LUSI values calculated with MCSC and CLCM were compared using the Wilcoxon matched-pairs signed-rank test. A regression line of the LUSI values calculated using MCSC and CLCM was then compared to an identity regression using the analyses of covariance (ANCOVAs). A first analysis included the interaction of the independent variables to determine whether the slopes were significantly different; in a second analysis, the interaction was omitted to determine whether the intercepts were significantly different.
The level of statistical significance for all performed tests was set at 0.01.
Statistical tests were performed using R (R Development Core Team, 2008) and STATISTICA software (StatSoft, 2003). Linear models were plotted using R software and the maps were drawn using Miramon software.
Results and Discussion
Assessment of Continental Pressures on Coastal Waters Using LUSI
Figure 1 (Supplementary Figure 2) shows that nearly the entire Catalan coast is influenced by continental pressures to some extent, with LUSI values ranging between 0.75 and 6.25. Lower LUSI values were obtained in areas where natural land use accounts for a high percentage of land coverage; as is the case for water body C05 (0.75), adjacent to the Cap de Creus marine and terrestrial natural park located in northeast Catalonia. Coastal areas that receive important fluvial inflows, such as water bodies located around the Ebre delta (C33 and C34; 5), the Ebre bays (T01 and T03; 6.25), or water bodies receiving the waters of the Muga (C07; 6.25), Fluvià (C08; 6.25), and Ter (C11; 6.25) rivers, located on the northeast Catalan coast, had the highest LUSI values. High LUSI values were also determined for coastal zones with the highest percentage of urban land coverage, primarily the Barcelona Metropolitan Area, in the center of the Catalan coast (C21; 5). However, the values in the metropolitan area were not as high as those of areas influenced by Catalonia's main rivers.
This assessment reveals the Catalan areas where continental pressures are able to fuel the eutrophication of coastal waters.
Validation of LUSI using dissolved inorganic nutrient concentrations
A validation implies a demonstration of a significant relationship between two independent datasets of indicators for the same parameter, comprising one of the datasets the results of the method to be tested. Accordingly, LUSI was validated by comparing its results with dissolved inorganic nutrient concentrations from coastal waters, given that land pressures, indirectly evaluated by LUSI, are mainly related to these concentrations.
The correlation and adjusted linear model based on the LUSI values and the dissolved inorganic nutrient concentrations were significant (Table 5; Supplementary Figure 3). The lowest correlation was 0.6 and the highest 0.87. All linear models showed positive relationships, with slope values ranging between 0.05 and 0.21.
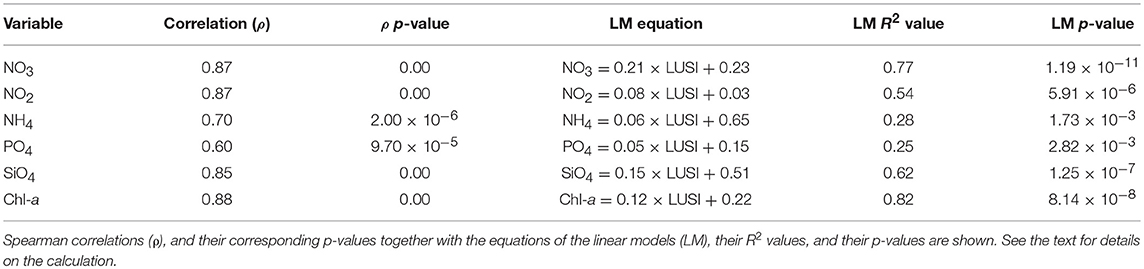
Table 5. Relationships between LUSI values calculated using the 2009 Mapa de Cobertes del Sòl de Catalunya and dissolved inorganic nutrient concentrations [log-transformed 90th percentiles of nitrate (NO3), nitrite (NO2), ammonium (NH4), phosphate (PO4), and silicate (SiO4), in μM] or chlorophyll-a (Chl-a; log-transformed 90th percentiles in μg/L), measured between 2007 and 2012 at water bodies along the Catalan coast.
These results validated LUSI as a proxy for indirectly assessing continental nutrient loads and concentrations and therefore continental pressures on coastal waters.
Comparison of LUSI calculated with different land cover maps
LUSI values calculated using two different land cover maps, with regional and European coverage, were compared to test whether the choice of the land cover map influences the results of LUSI.
European (CLCM) and regional (MCSC) land cover maps are based on different reference images, as CLCM uses images captured by SPOT5 and MSCS those from LANDSAT (Table 4). In addition, the images were captured during different times: the CLCM images in 2005 and those of MSCS in 2009. The two maps also differ in their legend levels, as level 3 of CLCM has 44 categories and level 5 of MSCS 241 categories. Nonetheless, 95% of the Catalan water bodies had similar LUSI values when calculated with MCSC and CLCM (1.25 was the maximum difference), and 67% of the water bodies had the same LUSI values. According to the Wilcoxon matched-pairs signed-rank test, there were no statistically significant differences between the two sets of LUSI values (p = 0.045). A comparison of a regression line of the LUSI values calculated using MCSC and CLCM and an identity regression also failed to reveal statistically significant differences between the slopes (p = 0.043) and the intercepts (p = 0.024) (Figure 2).
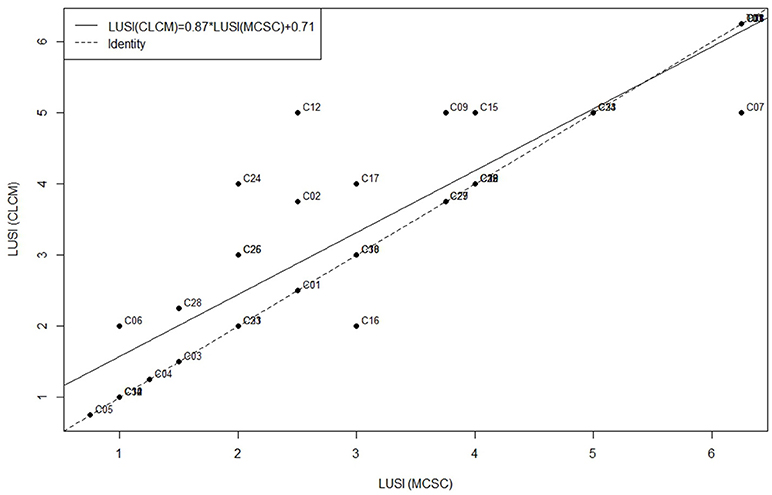
Figure 2. Regression line of the LUSI values calculated for the Catalan coast using the 2006 Coordination of Information of the Environment land cover map (CLCM) and the 2009 Mapa de Cobertes del Sòl de Catalunya (MCSC), drawn as a solid line. The dashed line corresponds to the identity regression. Codes of the water bodies are shown.
These results showed that the choice of land cover map does not significantly affect the calculation of LUSI, as similar continental pressure information is obtained.
Pressure-Impact Relationships
The WFD requires that pressure indicators are unambiguously linked with biological impact indicators, as this will allow an elucidation of the involved mechanisms and increase the probability that management actions will be effective. For coastal waters, these relationships should be established for the following Biological Quality Elements: phytoplankton, macrophytes, angiosperms, and macrofauna. Regarding coastal eutrophication, a pressure-impact relationship can be established between LUSI and chlorophyll-a, as a proxy of phytoplanktonic biomass and, thus, of the eutrophication impact. The mechanism underlying this relationship is related to nutrients: nutrient-rich freshwater and riverine inflows reach coastal waters, where nutrients boost phytoplanktonic growth, thus, enhancing eutrophication.
Establishment of a pressure-impact relationship based on LUSI and chlorophyll-a for the catalan coast
For the Catalan coast, the link between LUSI and chlorophyll-a is depicted in Figure 3: coastal waters adjacent to areas of land dominated by human activities or coastal waters receiving water from the Catalonia's main rivers had higher chlorophyll-a concentrations than areas with less human pressure or without rivers. Moreover, the effect of coastal morphology on the confinement of coastal waters could also be visualized: chlorophyll-a concentrations were higher in bays, where water is confined, than in the waters around headlands, where continental freshwater inflows become more diluted. The correlation and adjusted linear model (i.e., pressure-impact relationship) based on the LUSI values and chlorophyll-a concentrations were significant (Table 5; Figure 4; Supplementary Figure 4; Supplementary Table 3). The correlation (0.88) confirmed the relationship between LUSI and chlorophyll-a, while the positive slope coefficient (0.12) of the pressure-impact relationship implied that chlorophyll-a is positively related to LUSI. Therefore, water bodies receiving no or minimal pressure from the continent are either without signs of impact or the impact is minimal (low LUSI and chlorophyll-a values). Conversely, water bodies subject to continental pressures comprise more highly impacted waters (higher LUSI values are related to higher chlorophyll-a values). These results show that continental pressures are linked with eutrophication along the Catalan coast. However, it should be clarified that in the Mediterranean Sea high chlorophyll-a concentrations do not necessarily imply changes in the balance of organisms or in the development of hypoxia or anoxia, as is the case in other seas (Diaz and Rosenberg, 1995; Breitburg, 2002; Gray et al., 2002). Moreover, the obtained pressure-impact relationship between LUSI and chlorophyll-a fulfills the requirements of the WFD.
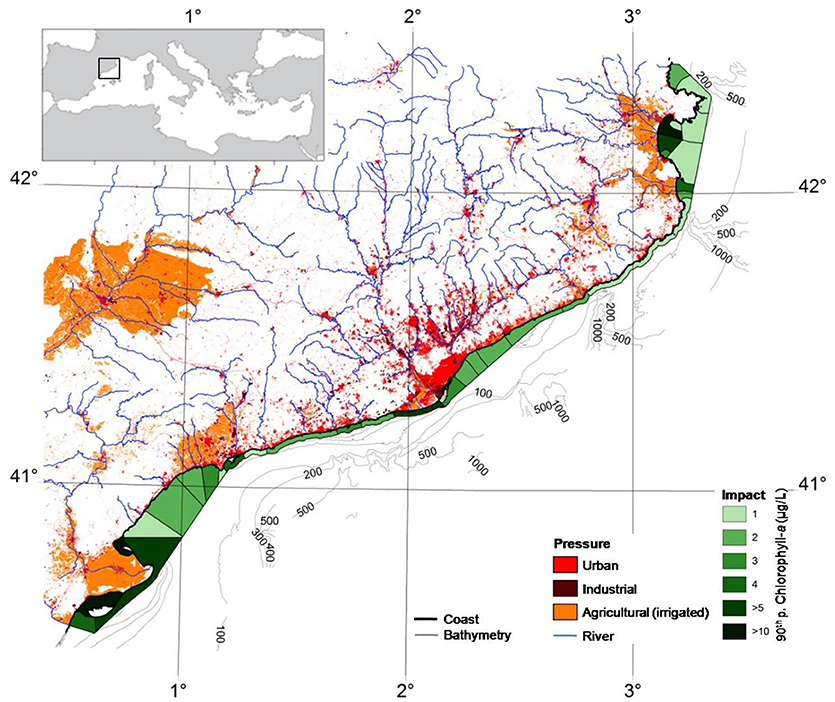
Figure 3. Map of the Catalan coast showing the continental pressures related to LUSI on land calculated using the 2009 Mapa de Cobertes del Sòl de Catalunya and the 90th percentile values of chlorophyll-a (μg/L) in coastal waters (shown as water bodies), as a measure of impact. Urban land use is shown in red, industrial land use in brown and agricultural (irrigated) land use in orange. Rivers are shown in blue and the coastline in black. Water bodies are shown in green, with the shade depending on the chlorophyll-a value (assigned based on direct integer values). There are no chlorophyll-a data for harbors, indicated, as for the bathymetry, in gray.
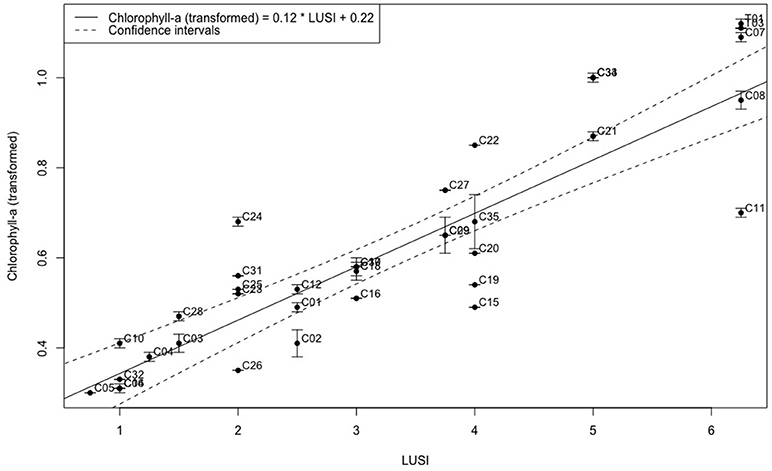
Figure 4. Pressure-impact relationship between LUSI values calculated using the 2009 Mapa de Cobertes del Sòl de Catalunya and chlorophyll-a concentrations (μg/L) for the water bodies of the Catalan coast (R2 value = 0.82 and p-value = 8.14 × 10−8). Chlorophyll-a was measured between 2007 and 2012; circles represent the means, and error bars the standard deviations of the log-transformed 90th percentiles. Chlorophyll-a confidence intervals of the relationship and the codes of the water bodies are shown. See the text for details on the calculation.
The theoretical background concentration of chlorophyll-a in the absence of continental pressures, calculated using the linear model, was compared with the natural background concentration of chlorophyll-a measured in coastal waters to validate the established pressure-impact relationship. The theoretical background concentration of chlorophyll-a was calculated using the minimum LUSI value of 0.75; the result was a minimum chlorophyll-a concentration of 1.04 μg/L. This value is similar to the 1.01 μg chlorophyll-a/L measured in the water body of Cap Norfeu (C05), located within the Cap de Creus marine and terrestrial natural park, in northeast Catalonia. Its LUSI value was 0.75, indicating that it is subject to minimum pressure from the continent. The similarity between the theoretical and measured minimum chlorophyll-a values supports the validity of the previously established pressure-impact relationship. Furthermore, 1.04 μg chlorophyll-a/L can be established as the reference condition for the Biological Quality Element Phytoplankton in the Catalan coast.
The above pressure-impact regression and the reference condition for the Biological Quality Element Phytoplankton are applicable for the entire Catalan coast, as all the water bodies of this area were considered in their establishment. However, this kind of exercise can be performed with subsets of data, considering the desired factor: land use pressure, riverine pressure, or coastline morphology. For example, to establish the reference conditions in the Catalan coast for the Biological Quality Element Phytoplankton within the Water Framework Directive Intercalibration Process for the Mediterranean Sea, three regressions were performed considering the three types of the specific typology for this element, which are based on salinity values and are the same of those categories established for riverine pressure; as a result, three different reference conditions were established (Camp et al., 2016).
Comparison of pressure-impact relationships based on LUSI and biological quality elements of the WFD from several coastal areas
Within the context of the WFD implementation, LUSI has been used together with several impact indicators related to all the Biological Quality Elements linked to coastal waters to establish pressure-impact relationships in the Mediterranean and Black Seas and in the Atlantic Ocean. The broad and successful use of LUSI was made possible by the open distribution of its rationale and its description within the Milestone 5 report regarding Intercalibration Phase 2 of the WFD (Flo et al., 2011a). An extended bibliographic exploration, including scientific articles but also oral presentations and technical reports, resulted in 48 relationships found, performed with independent datasets (Table 6). Most of the cases reinforced the well-performing of LUSI in assessing continental pressures and in establishing these relationships. Furthermore, they demonstrated the utility of LUSI beyond the Catalan coast, to other coastal areas of the Mediterranean Sea but also of the Black Sea and the Atlantic Ocean, and in relation to other Biological Quality Elements, in addition to phytoplankton.
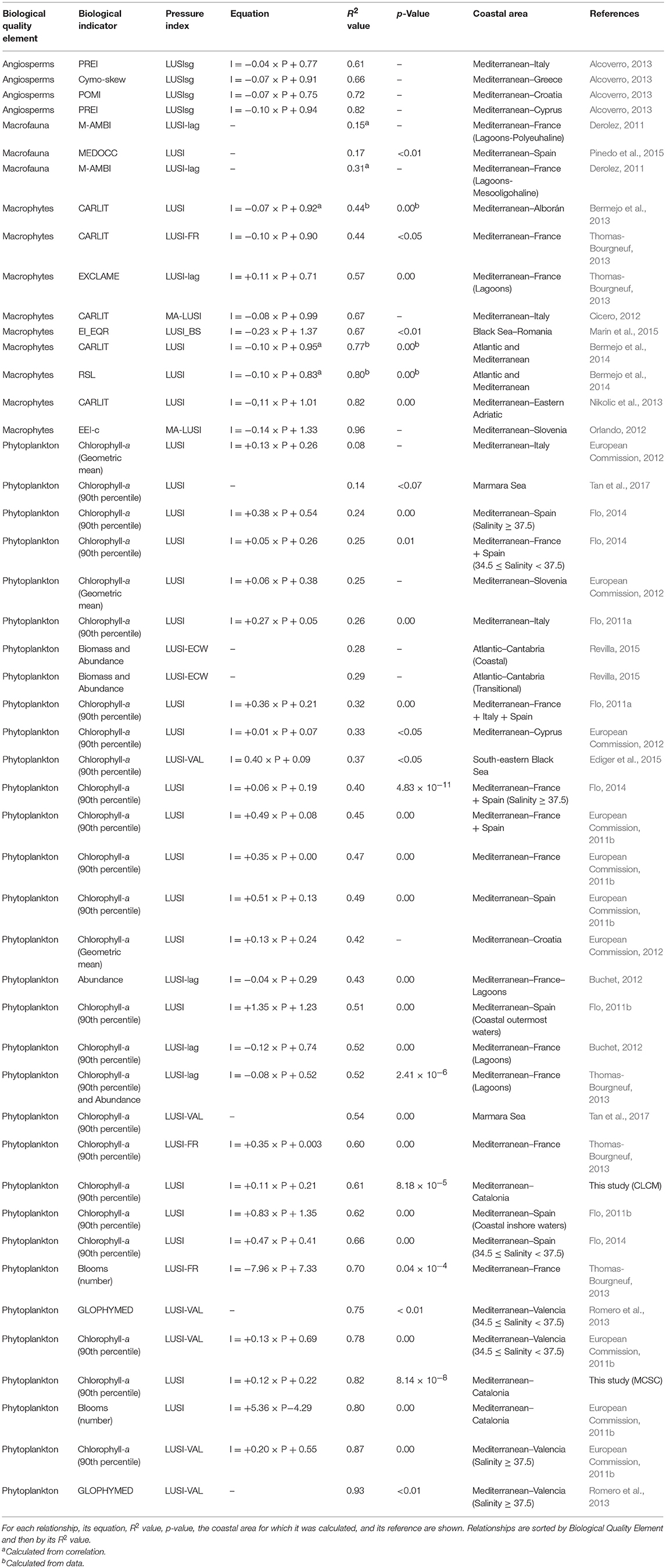
Table 6. Pressure-impact relationships between LUSI, as pressure (P), and biological indicators related to the Biological Quality Elements of the Water Framework Directive for coastal waters, as impact (I).
The comparison of the pressure-impact relationships based on LUSI values and biological impact indicators shows a very broad range of goodness of fit values, from 8 to 93% (Table 6). In some cases, well-defined, linear pressure-impact relationships could not be established, as indicated by the low goodness of fit values. In other cases, however, interactions between pressures and impacts were simpler, more readily identifiable and better known, resulting in liner relationships with a high goodness of fit. Relationships related to angiosperms showed acceptable goodness of fit (minimum value of 61%) while those related to macrofauna were generally poor fitted (maximum value of 31%). Macrophytes and phytoplankton relationships showed high and low goodness of fit. Nonetheless, in all cases the results should be carefully interpreted, as for example a high goodness of fit value does not always imply causality.
The disparity among goodness of fit values of the compared pressure-impact relationships exemplify the complexity of these relationships. For the marine environment and its functioning, the calculated pressure-impact relationships are a simplification. Generally, they are theoretical and linear, indicative of a unique and direct mechanism linking an identified pressure with a specific impact. These relationships, while a very useful tool in WFD implementation, especially for management purposes, are nonetheless rare in the environment, where multiple pressures, both natural and anthropogenic, interact in multiple ways to affect a wide range of organisms, resulting in numerous impacts throughout the ecosystem. In nature, interactions among pressure, and impact indicators create complex networks and involve physical, chemical, and biological mechanisms that coexist in time and space (Garcés and Camp, 2012). The influence of these factors on the area of study can modify the goodness of fit of the pressure-impact relationship.
An example of the influence of other factors in the fitting of a pressure-impact relationship is that of the distance to the coast of the sampling stations when establishing this relationship between LUSI and the Biological Quality Element phytoplankton. The relationship between continental nutrients and chlorophyll-a is more direct at the coastline than in outermost waters, where nutrient availability decreases and other complex oceanographic mechanisms that affect phytoplankton growth come into play (Basterretxea et al., 2018). For Mediterranean Spanish coastal waters, the goodness of fit of pressure-impact relationships between LUSI and chlorophyll-a concentration was higher when chlorophyll-a was sampled at the coastline (62%) than at coastal outermost waters (51%) (Table 6). Consequently, current sampling strategies for chlorophyll-a should be reconsidered: there should be greater focus on those sites where continental pressures are stronger and the risk of eutrophication is higher, thus where clearer pressure-impact relationships are more likely. These sites are near the coastline, not at the offshore coastal boundary, where the relationships are more complex and the risk of eutrophication is lower. This change will not only cut the expenses of sampling strategies, as those areas, at least in the Mediterranean Sea, are only reachable on food from the coastline and thus it is not necessary to use a boat, but also it will provide better information on the areas that are at higher risk of eutrophication (i.e., the coastal inshore waters).
Another example of factors that can influence the fitting of a pressure-impact relationship is LUSI modifications (Table 6). Several authors have adapted LUSI to a specific coastal area (Derolez, 2011; European Commission, 2011b; Cicero, 2012; Alcoverro, 2013; Thomas-Bourgneuf, 2013; Marin et al., 2015; Revilla, 2015) in order to include already know information of land pressures. These adaptations necessitated modifying some of LUSI's characteristics, such as adding more categories for a specific pressure, considering other kinds of pressures, changing the confinement correction factor, or taking into account a different continental area. Some modifications do not significantly affect the calculation of LUSI, such as take into account a different but similar continental area. However, the more closely adapted the modified index is to the specific characteristics of the coastal area of interest, the less useful it is for other coastal areas with different characteristics and, importantly, for subsequent comparisons between them. Accordingly, to compare pressures assessments of different coastal waters preformed with the original LUSI and with a modified LUSI an intercalibration exercise should be previously done. LUSI modifications could imply a loss of simplicity and a lack of comparability, however, they are a suitable option to obtain more accurate pressure assessments. In all cases when a modified LUSI is used, it is advisable to carefully interpret the results.
The comparison of several pressure-impact relationships (Table 6) has allowed to establish some evidences. First, the sea or ocean of study seems not to influence the performance of the relationships, as for example for the Mediterranean Sea there are well-defined relationships and others that are not acceptable. Second, for pressure-impact relationships based on LUSI and chlorophyll-a, the distance to the coast of the sampling stations is key, as LUSI has been developed to be confronted with impact data from the coastal inshore waters, where the pressures from land are more evident and pose a risk of eutrophication. Third, Biological Quality Elements phytoplankton, angiosperms, and macrophytes can provide well-defined relationships, while the examples found in the literature did not show any acceptable example for macrofauna. Fourth, LUSI modifications provide well-defined relationships when applied in the area for which it was developed. For example, LUSI-VAL applied in Valencia together with chlorophyll-a provided a goodness of fit of 87%. However, their performance it is not assured when applied to other areas. For example, LUSI-VAL together with chlorophyll-a provided an acceptable relationship in the Marmara Sea (54% of goodness of fit) but not in the South-eastern Black Sea (37% of goodness of fit). Even though these evidences, more information is needed to establish the differences and the commonalities regarding the factors that intervene in the calculation of LUSI and in the posterior establishment of pressure-impact relationships.
Guidance on Management Actions Provided by LUSI Regarding Eutrophication
The restoration and protection of coastal waters against eutrophication requires information on the pressures that strengthen its impact. With this knowledge, decision-makers can decide on where management actions are needed and the types of action that will be most effective. LUSI provides three kinds of information to guide this decision-making process.
First, the assessment of continental pressures using LUSI yields information on coastal areas vulnerable to eutrophication and thus at high risk (high LUSI values; Figure 1). These areas will require the implementation of a management plan if eutrophication is indeed detected. However, in the absence of evident eutrophication, the implementation of a monitoring plan as an early warning system will suffice.
Second, the pressure-impact relationship established between LUSI and chlorophyll-a concentrations identifies the coastal areas characterized by a mismatch between these values (Figure 4). For example, in coastal areas with a lower or higher than expected chlorophyll-a concentration, given their LUSI value, other mechanisms related to eutrophication are probably in play; for example, continental nutrients may be diluted at a different rate than expected or transport mechanisms are exporting or importing nutrients. In these cases, further studies aimed at a better understanding of the complexity of the area are warranted before the design of a management plan. Water body C19, located in front of Barcelona, is an example of a water body with a lower than expected chlorophyll-a concentration (2.43 vs. 4.01 μg/L) given its LUSI value (4). In this case, the city's wastewater systems, which include wastewater treatment plants, submarine outfalls, pumping stations, and upstream collectors (Agència Catalana de l'Aigua, 2011), are able to partially neutralize the continental pressures. This also demonstrates that disagreements between measured and expected chlorophyll-a values can be used to test the efficiency of wastewater treatment systems. Conversely, C22, located at the south of the Barcelona Metropolitan Area, is an example of a water body with a higher than expected chlorophyll-a concentration (6.06 vs. 4.01 μg/L) given its LUSI value (4). This water body receives nutrients that reached coastal waters in the northern adjacent water body C21. Continental nutrients from the Barcelona Metropolitan Area and from the Llobregat river reach water body C21 and then are transported by the general surface circulation dictated by the Liguro-Provençal current that moves from NE to SW to the water body C22. Thus, management of water body C22 should take into account the transfer of nutrients from adjacent areas, with subsequent actions directed at water body C21, where the nutrients reach coastal waters.
Third, the LUSI scores provides insights into the land uses that trigger the eutrophication of coastal waters and therefore guide the choice of management actions if an impact of eutrophication is detected. For the Catalan coast and the studied period, the assessment of the impact based on chlorophyll-a concentrations and on the methodology established by Camp et al. (2016) resulted in the identification of four water bodies impacted by eutrophication (Table 7; Supplementary Table 4). These water bodies require management plans aimed at remediating the negative effects of continental nutrient arrival. The LUSI scores of these coastal areas provides information on the human activities occurring on land that could be responsible for the eutrophication detected in coastal waters, and therefore on the type of management actions that should be included in these plans. In the case of the Catalan coast, the management plans for the four water bodies should include actions related to urban land uses, as all four were characterized by high scores for this source of pressure. Regarding other land-use pressures, the management actions for water bodies C07 and C22 should target agricultural land uses, and those for water body C27 industrial land uses. Only water body C07 show a high score related to salinity, and thus to riverine pressure, reflecting inflows of the Muga river. Therefore, the management plan for this coastal area should include actions related to land uses ongoing throughout the watershed. A study of land uses in the Muga watershed revealed agriculture and urban land uses as the main human activities. Accordingly, the management plan of C07 should include actions related to urban and agricultural land uses, with their implementation not only in the vicinity of the coastal waters of this water body but also across the whole watershed.
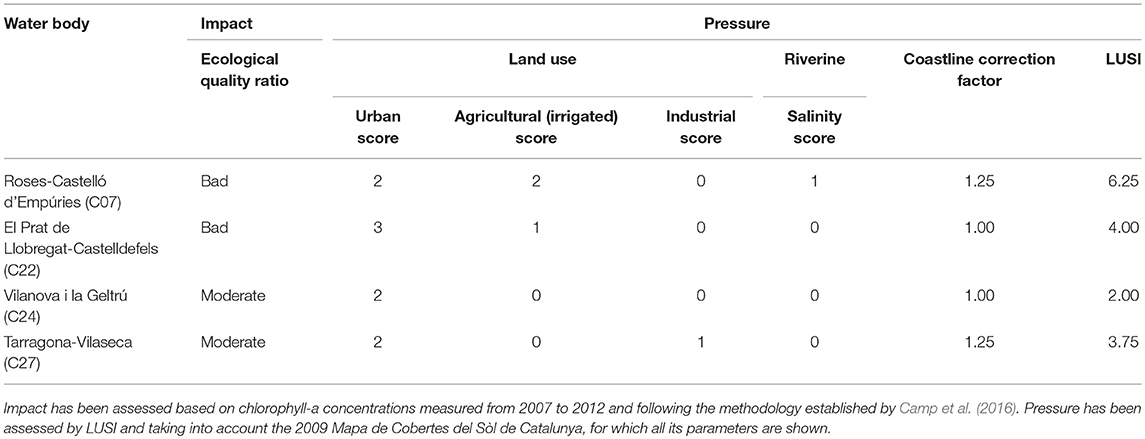
Table 7. Impact and pressure of the water bodies of the catalan coast where impact has been demonstrated.
General coastal management recommendations can be provided in addition and thus expand the scope of this study. First, actions should be implemented on land, where the nutrients that trigger coastal eutrophication are generated. Second, they should be implemented at a local scale, to respond to specific issues. Third, until integrated management plans are fully implemented, both precautions (Rice, 2003) and common sense are strongly recommended.
Further Considerations Regarding LUSI
LUSI is a method to assess continental pressures, manifested as the risk of eutrophication, on coastal waters and it fulfills most of the criteria proposed by Lopez y Royo et al. (2009) for this type of tool: simplicity, time and cost-effectiveness, repeatability, reliability and broad applicability. Other strength of LUSI is that it is based on publicly available and periodically updated data. LUSI provides a synthetic pressure assessment that integrates all potential sources of impact to yield a single indicator value that is comparable among coastal areas.
LUSI is neither quantitative nor accurate, but it is a simple and reliable proxy of continental pressures, as shown herein. However, according to Lopez y Royo et al. (2009), the use of simple pressure assessment methods implies an oversimplification of the information. The question then arises whether continental nutrient fluxes (load and concentration) must be evaluated quantitatively and precisely to obtain an accurate characterization of continental pressures, as recommended by Fedorko et al. (2005), Giupponi and Vladimirova (2006), and Rodriguez et al. (2007). The approaches of those authors have two main drawbacks. First, although information on continental pressures from an identified point source, such as river water and wastewaters, is available and updated, for continental diffuse pressures, such as those caused by runoff, data are scarce or still lacking. Second, an accurate characterization of continental pressures implies considerable investments of time, economic and human resources, and, if models are to be constructed, computational effort; thus, an accurate characterization is likely to be feasible only at local scales and nearly impossible at regional or larger scales. Despite these drawbacks, continental pressures assessed using more accurate approaches have been used to establish pressure-impact relationships. The choice between a simple vs. an accurate method to assess continental pressures may depend on the objectives of the study, specifically, on the spatial scale, and the required degree of detail. However, the goodness of fit values of pressure-impact relationships obtained with more accurate assessments of continental pressure (Böhmer et al., 2014; Gerakaris et al., 2017) were similar to those established by LUSI. Thus, in establishing pressure-impact relationships, simplicity is not necessarily at odds with accuracy, as our results showed that LUSI is both a reliable and an appropriate approach.
LUSI offers a broad range of applications in the fields of environmental science, environmental law and socio-economics. Direct applications include those related to environmental policies based on the drivers-pressures-state-impact-responses (DPSIR) framework (Organisation for Economic Co-operation Development, 1993), such as described in the WFD and presented herein. Moreover, LUSI has indirect applications related to the benefits of access to pressure information, especially regarding diffuse sources of nutrients. This information could lead to better protection of the environment and therefore human health and well-being. For example, in Europe, LUSI already provides useful information for the Nitrate Directive (European Communities Council, 1991b), the Habitats Directive (European Communities Council, 1992), the Bathing Water Quality Directive (European Communities Council, 1976), the Urban Wastewater Treatment (European Communities Council, 1991a), and the New Political Framework for Tourism (European Economic Social Committee, 2010), in addition to supporting Integrated Coastal Zone Management objectives.
Conclusion
LUSI is a method to assess the continental pressures on coastal waters. It serves as a proxy enabling the indirect assessment of continental nutrient loads and concentrations, and their influence on coastal waters. Therefore, it estimates the risk of eutrophication in coastal waters. It is based on systematic information on the land uses that influence coastal waters by providing nutrient-rich freshwater inflows (urban, industrial, agricultural, and riverine) and on coastline morphology, which can modify this influence, as it determines the degree of coastal water confinement and therefore the likelihood that these inflows will be diluted. LUSI was designed as a simple tool to assess coastal pressures when there is a lack of information, thus filling a methodological gap. Moreover, it also meets the requirements of the WFD regarding a true pressure assessment (i.e., not confounded with impact) and the establishment of pressure-impact relationships with impact indicators related to the Biological Quality Elements. The utility of LUSI was highlighted in a case study from the Catalan coast (NW Mediterranean), where continental pressures were assessed using LUSI, a pressure-impact relationship between LUSI and chlorophyll-a concentrations was established, and guidance on management actions regarding eutrophication provided. Furthermore, the well-performance of LUSI in assessing continental pressures and in establishing pressure-impact relationships was bibliographically demonstrated not only for the Biological Quality Element phytoplankton but also for angiosperms and macrophytes and its spatial scope broaden to the Black Sea and the Atlantic Ocean. Our results demonstrate the ability of LUSI to improve scientific knowledge regarding coastal eutrophication and to generate information that is useful to environmental managers in their efforts to restore and protect coastal waters against eutrophication and to achieve an integrated coastal management plan.
Author Contributions
All authors listed have made a substantial, direct and intellectual contribution to the work, and approved it for publication.
Funding
This study used data collected within the National Catalan Water Monitoring Program funded by contract CTN0802809 between the Catalan Water Agency (ACA) and the Marine Science Institute (ICM-CSIC). This research is a contribution to the GRADIENTS project (Estructura fina de los gradientes costeros a lo largo de la costa Mediterranea), funded by the Spanish National Program (CTM2012-39476-C02-02) and the DEVOTES project (Development of innovative tools for understanding marine biodiversity, and assessing good environmental status), funded by the European Union's Seventh Framework Programme for research, technological development, and demonstration under grant agreement number 308392.
Conflict of Interest Statement
The authors declare that the research was conducted in the absence of any commercial or financial relationships that could be construed as a potential conflict of interest.
Acknowledgments
R. Ventosa and M. Abad are acknowledged for dissolved inorganic nutrients analyses. J. Ballabrera for constructive discussions of the statistical approach and J. Jones, W. Ran, and two reviewers for helpful comments on earlier drafts of this work.
Supplementary Material
The Supplementary Material for this article can be found online at: https://www.frontiersin.org/articles/10.3389/fmars.2019.00018/full#supplementary-material
References
Agència Catalana de l'Aigua (2005a). Document IMPRESS: Caracterització de Masses D'aigua i Anàlisi del risc D'incompliment dels Objectius de la Directiva Marc de l'Aigua (2000/60/CE) a les Conques Internes de Catalunya. Barcelona: Generalitat de Catalunya.
Agència Catalana de l'Aigua (2005b). Document IMPRESS: Caracterització de Masses d'aigua i Anàlisi del Risc D'incompliment Dels Objectius de la Directiva Marc de l'Aigua (2000/60/CE) a les Conques Internes de Catalunya. Barcelona: Generalitat de Catalunya.
Agència Catalana de l'Aigua (2011). Estat de les masses d'aigua de Catalunya. Resultats del Programa de Seguiment i Control. Dades 2007–2010. Barcelona: Generalitat de Catalunya, 76.
Aighewi, I. T., Nosakhare, O. K., and Ishaque, A. B. (2013). Land use-land cover changes and sewage loading in the lower eastern shore watersheds and coastal bays of maryland: implications for surface water quality. J. Coastal Res. 29, 1073–1082. doi: 10.2112/JCOASTRES-D-11-00195.1
Alcoverro, T. (2013). Intercalibration of Biological Elements for Transitional and Coastal Water Bodies. Mediterranean geographical intercalibration group. Coastal Waters. Seagrasses. Barcelona: European Commission, 39.
Andrulewicz, E., and Witek, Z. (2002). Anthropogenic Pressure and Environmental Effects on the Gulf of Gdansk: Recent Management Efforts. Berlin: Springer-Verlag Berlin.
Aubry, A., and Elliott, M. (2006). The use of environmental integrative indicators to assess seabed disturbance in estuaries and coasts: application to the humber estuary, UK. Mar. Pollut. Bull. 53, 175–185. doi: 10.1016/j.marpolbul.2005.09.021
Australia Office of Parliamentary Counsel. (2007). An Act to Make Provision for the Management of the Water Resources of the Murray-Darling Basin, and to Make Provision for Other Matters of National Interest in Relation to Water and Water Information, and for Related Purposes (Water Act 2007).
Barale, V., and Folving, S. (1996). Remote sensing of coastal interactions in the Mediterranean region. Ocean Coast. Manag. 30, 217–233. doi: 10.1016/0964-5691(95)00059-3
Basterretxea, G., Torres-Serra, F. J., Alacid, E., Anglès, S., Camp, J., Ferrera, I., et al. (2018). Cross-shore environmental gradients in the western mediterranean coast and their influence on nearshore phytoplankton communities. Front. Mar. Sci. 5:78. doi: 10.3389/fmars.2018.00078
Batista, M. I., Henriques, S., Pais, M. P., and Cabral, H. N. (2014). Assessment of cumulative human pressures on a coastal area: integrating information for MPA planning and management. Ocean Coast. Manag. 102, 248–257. doi: 10.1016/j.ocecoaman.2014.09.020
Bermejo, R., de la Fuente, G., Vergara, J. J., and Hernandez, I. (2013). Application of the CARLIT index along a biogeographical gradient in the Alboran Sea (European Coast). Mar. Pollut. Bull. 72, 107–118. doi: 10.1016/j.marpolbul.2013.04.011
Bermejo, R., Mangialajo, L., Vergara, J. J., and Hernandez, I. (2014). Comparison of two indices based on macrophyte assemblages to assess the ecological status of coastal waters in the transition between the Atlantic and Mediterranean eco-regions. J. Appl. Phycol. 26, 1899–1909. doi: 10.1007/s10811-013-0226-x
Biblioteca del consorci el Far. (2010). Dades Bàsiques del Litoral Català. Població i Territori de les Comarques Litorals [Online]. Available online at: http://elfar.diba.es/cil/dadeslitoral/poblacio.htm (Accessed September 06, 2010).
Böhmer, J., Arbaciauskas, K., Benstead, R., Gabriels, W., Porst, G., Reeze, B., et al. (2014). Water Framework Directive Intercalibration Technical Report: Central Baltic Lake Benthic Invertebrate Ecological Assessment Methods (Luxembourg: Publications Office of the European Union).
Bonamano, S., Piermattei, V., Madonia, A., de Mendoza, F. P., Pierattini, A., Martellucci, R., et al. (2016). The civitavecchia coastal environment monitoring system (C-CEMS): a new tool to analyze the conflicts between coastal pressures and sensitivity areas. Ocean Sci. 12, 87–100. doi: 10.5194/os-12-87-2016
Borja, A., Barbone, E., Basset, A., Borgersen, G., Brkljacic, M., Elliott, M., et al. (2011). Response of single benthic metrics and multi-metric methods to anthropogenic pressure gradients, in five distinct European coastal and transitional ecosystems. Mar. Pollut. Bull. 62, 499–513. doi: 10.1016/j.marpolbul.2010.12.009
Borja, Á., Dauer, D. M., and Grémare, A. (2012). The importance of setting targets and reference conditions in assessing marine ecosystem quality. Ecol. Indic. 12, 1–7. doi: 10.1016/j.ecolind.2011.06.018
Breitburg, D. (2002). Effects of hypoxia, and the balance between hypoxia and enrichment, on coastal fishes and fisheries. Estuaries 25, 767–781. doi: 10.1007/BF02804904
Bricker, S., Longsta, B., Dennison, W., Jones, A., Boicourt, K., Wicks, C., et al. (2007). Efects of Nutrient Enrichment In the Nation's Estuaries: A Decade of Change. Silver Spring, MA: National Centers for Coastal Ocean Science.
Buchet, R. (2012). Assistance à la Coordination des Travaux Européens D'intercalibration des Indicateurs Biologiques de la Directive Cadre sur L'EAU (DCE). Rapport du contrat Ifremer/Hocer n°11/5210818, (Nantes: HOCER) p. 133+annexes.
Cai, M., Liu, Y., Chen, K., Huang, D., and Yang, S. (2016). Quantitative analysis of anthropogenic influences on coastal water – A new perspective. Ecol. Indic. 67, 673–683. doi: 10.1016/j.ecolind.2016.03.037
Camp, J., Flo, E., Vila, M., Arin, L., Re,ñé, A., Sampedro, N., et al. (2016). “Pros and Cons of biological quality element phytoplankton as a water-quality indicator in the NW Mediterranean Sea,” in Experiences from Ground, Coastal and Transitional Water Quality Monitoring: The EU Water Framework Directive Implementation in the Catalan River Basin District (Part II), eds A. Munné, A. Ginebreda, N. Prat (Cham: Springer International Publishing), 135–160.
Cassie, R. M. (1962). Frequency distribution models in the ecology of plankton and orher organisms. J. Anim. Ecol. 31, 65–91.
Centre de Recerca Ecològica i Aplicacions Forestals, C. (2009). Mapa de Cobertes del Sòl de Catalunya 2009 (MCSC). Available online at: http://www.creaf.uab.es/mcsc/descriptiu.htm
Chislock, M. F., Doster, E., Zitomer, R. A., and Wilson, A. E. (2013). Eutrophication: causes, consequences, and controls in aquatic ecosystems. Nat. Educ. Knowl. 4:10.
Cicero, A. M. (2012). “Ricerca e innovazione per la governance ambientale,” in Le Acque Marino-Costiere e di Transizione, eds ISPRA, I.S.p.l.P.e.l.R.A (Roma: Forum PA), 18.
Cole, J., and McGlade, J. (1998). Clupeoid population variability, the environment and satellite imagery in coastal upwelling systems. Rev. Fish Biol. Fish 8, 445–471. doi: 10.1023/A:1008861224731
Comeleo, R. L., Paul, J. F., August, P. V., Copeland, J., Baker, C., Hale, S. S., et al. (1996). Relationships between watershed stressors and sediment contamination in Chesapeake Bay estuaries. Landsc. Ecol. 11, 307–319. doi: 10.1007/BF02059858
Creel, L. (2003). Ripple Effects: Population and Coastal Regions in Measure Communication (Washington, DC: Population Reference Bureau).
Derolez, V. (2011). “La macrofaune benthique en lagunes,” in Séminaire Évaluation Dans le Contexte de la DCE IFREMER (Paris: IFREMER), 16.
Diaz, R. J., and Rosenberg, R. (1995). “Marine benthic hypoxia: A review of its ecological effects and the behavioural responses of benthic macrofauna,” in Oceanography and Marine Biology–an Annual Review Vol. 33, eds A. D. Ansell, R. N. Gibson, M. Barnes (London: U C L Press Ltd), 245–303.
Ediger, D., Beken, C. P., Feyzioglu, A. M., Sahin, F., and Tan, I. (2015). Establishing boundary classes for the quality classification of southeastern black sea using phytoplankton biomass. Turkish J. Fish. Aquat. Sci. 15, 723–731. doi: 10.4194/1303-2712-v15_3_16
European Commission (2000). Directive 2000/60/EC of the European Parliament and of the Council Establishing a Framework for the Community Action in the Field of Water Policy (Water Framework Directive).
European Commission (2008). Directive 2008/56/EC of the European Parliament and of the Council of 17 June 2008 Establishing a Framework for Community Action in the Field of Marine Environmental Policy (Marine Strategy Framework Directive).
European Commission (2011a). Water Framework Directive. Intercalibration Phase 2. Coastal Waters. North-East Atlantic Ocean Geographical Intercalibration Group. Macroalgae. Milestone 6 report. European Commission.
European Commission (2011b). Water Framework Directive. Intercalibration Phase 2. Milestone 4 report. Coastal Waters. Mediterranean Geographical Intercalibration Group. Biological Quality Element Phytoplankton. European Commission, 55.
European Commission (2012). Water Framework Directive. Intercalibration Phase 2. Technical Report. Coastal Waters. Mediterranean Geographical Intercalibration Group. Biological Quality Element Phytoplankton. European Commission, 24.
European Communities Council (1976). Council Directive 76/160/EEC of 8 December 1975 Concerning the Quality of Bathing Water.
European Communities Council (1991a). Council Directive 91/271/EEC of 21 May 1991 Concerning Urban Waste-Water Treatment.
European Communities Council (1991b). Council Directive 91/676/EEC of 12 December 1991 Concerning the Protection of Waters Against Pollution Caused by Nitrates From Agricultural Sources.
European Communities Council (1992). Council Directive 92/43/EEC of 21 May 1992 on the Conservation of Natural Habitats and of Wild fauna and Flora.
European Economic and Social Committee (2010). Opinion of the European Economic and Social Committee on the ‘Communication from the Commission to the European Parliament, the Council, the European Economic and Social Committee and the Committee of the Regions — Europe, the world's No 1 Tourist Destination — a New Political Framework for Tourism in Europe' COM(2010) 352 Final.
European Enviromental Agency (2012). Coordination of Information of the Environment Land Cover 2006 Seamless Vector Data (CORINE land cover map). Available online at: http://www.eea.europa.eu/data-and-maps/data/ds_resolveuid/953c0872-2153-4faa-98d6-1c4ee457ae0d
Fedorko, E. J., Pontius, R. G., Aldrich, S. P., Claessens, L., Hopkinson, C., and Wollheim, W. M. (2005). Spatial distribution of land type in regression models of pollutant loading. J. Spatial Hydrol. 5, 60–80. doi: 10.1086/BBLv207n2p173
Ferré, N. (2007). Nivells de Metalls Pesants a la Conca Catalana del riu EBRE. Avaluació del risc per a la població i l'ecosistema. Tarragona: Uiversitat Rovira i Virgili.
Ferreira, J. G., Vale, C., Soares, C. V., Salas, F., Stacey, P. E., Bricker, S. B., et al. (2007). Monitoring of coastal and transitional waters under the E.U. Water framework directive. Environ. Monitor. Assess. 135, 195–216. doi: 10.1007/s10661-007-9643-0
Flo, E. (2011a). “Water Framework directive. intercalibration phase 2. Mediterranean geographical intercalibration group. biological quality element phytoplankton. France, Spain and Italy intercalibration process in coastal and transitional waters,” in Validation workshop of the Water Framework Directive Intercalibration (Barza d'Ispra: European Commission), 55.
Flo, E. (2011b). Water Framework Directive. Intercalibration Phase 2. Technical Report. Coastal Waters. Spain. Biological Quality element Phytoplankton. European Commission, 8.
Flo, E. (2014). Water Framework Directive. Intercalibration Phase 2. Milestone 5. Coastal Waters. Mediterranean Geographical Intercalibration Group. Biological Quality Element Phytoplankton. France and Spain report. European Commission, 29.
Flo, E., Camp, J., and Garcés, E. (2011a). “Assessment of pressure methodology for biological quality element phytoplankton: land uses simplified index (LUSI),” in Mediterranean Geographical Intercalibration Group-Coastal waters-Biological quality element phytoplankton-France and Spain. Water Framework Directive Intercalibration Phase 2: Milestone 5 report. European Commission.
Flo, E., Garcés, E., Manzanera, M., and Camp, J. (2011b). Coastal inshore waters in the NW Mediterranean: physicochemical and biological characterization and management implications. Estuar. Coast. Shelf Sci. 93, 279–289. doi: 10.1016/j.ecss.2011.04.002
Garcés, E., and Camp, J. (2012). “Habitat changes in the Mediterranean Sea and the consequences for harmful algal blooms formation,” in Life in the Mediterranean Sea: a Look at Habitat Changes, ed N. Stambler (New York, NY: Nova Science), 519–541.
Gerakaris, V., Panayotidis, P., Vizzini, S., Nicolaidou, A., and Economou-Amilli, A. (2017). Effectiveness of Posidonia Oceanica Biotic Indices for Assessing the Ecological Status of Coastal Waters in Saronikos Gulf (Aegean Sea, Eastern Mediterranean).
Giupponi, C., and Vladimirova, I. (2006). Ag-PIE: a GIS-based screening model for assessing agricultural pressures and impacts on water quality on a European scale. Sci. Total Environ. 359, 57–75. doi: 10.1016/j.scitotenv.2005.07.013
Grasshoff, K., Ehrhardt, M., and Kremling, K. (1983). Methods of Seawater Analysis. Weinheim: Verlag Chemie.
Gray, J. S., Wu, R. S. S., and Or, Y. Y. (2002). Effects of hypoxia and organic enrichment on the coastal marine environment. Mar. Ecol. Prog. Ser. 238, 249–279. doi: 10.3354/meps238249
Harbord, J. B. (1897). Glossary of Navigation. A Vade Mecum for Practical Navigators. Glasgow: Griffin.
Heyman, U., Ryding, S. O., and Forsberg, C. (1984). Frequency distributions of water quality variables. Relationships between mean and maximum values. Water Res. 18, 787–794. doi: 10.1016/0043-1354(84)90262-8
Hyndman, R. J., and Fan, Y. N. (1996). Sample quantiles in statistical packages. Am. Statist. 50, 361–365.
Institut d'Estadística de Catalunya (2015). Usos del sòl 2015. Catalunya i Comarques. Available online at: http://www.idescat.es
Institut d'Estadística de Catalunya. (2010). Anuari Estadístic de Catalunya 2010 (Barcelona, Catalonia: Institut d'Estadística de Catalunya).
Institut d'Estadística de Catalunya. (2016). Densitat de Població 2016. Comarques i Aran, Àmbits i Províncies. Available online at: http://www.idescat.cat
Instituto Geográfico Nacional. (2011). Sistema de Información sobre Ocupación del Suelo de España (SIOSE). Available online at: http://www.siose.es/
Jordan, T. E., Correll, D. L., and Weller, D. E. (1997). Relating nutrient discharges from watersheds to land use and streamflow variability. Water Resour. Res. 33, 2579–2590. doi: 10.1029/97WR02005
Ketchum, B. H. (1972). “The water's edge: critical problems of the coastal zone,” in Coastal Zone Workshop (Woods Hole, MA: The MIT Press), 414. Available online at: http://hdl.handle.net/1721.1/1763
Lautenbach, S., Kugel, C., Lausch, A., and Seppelt, R. (2011). Analysis of historic changes in regional ecosystem service provisioning using land use data. Ecol. Indic. 11, 676–687. doi: 10.1016/j.ecolind.2010.09.007
Legendre, L., and Legendre, P. (1979). Ecologie Numérique. 1. Le Traitment des Donnes Ecologiques. Paris: Masson and Cie.
Liquete, C., Canals, M., Ludwig, W., and Arnau, P. (2009). Sediment discharge of the rivers of Catalonia, NE Spain, and the influence of human impacts. J. Hydrol. 366, 76–88. doi: 10.1016/j.jhydrol.2008.12.013
Lopez y Royo, C., and Casazza, G. (2007). Assessment of Pressures on the Coastal Zone by Using Satellite Images. Akyaka: Mediterranean Coastal Foundation-Medcoast Foundation.
Lopez y Royo, C., Casazza, G., Pergent-Martini, C., and Pergent, G. (2010). A biotic index using the seagrass Posidonia oceanica (BiPo), to evaluate ecological status of coastal waters. Ecol. Indic. 10, 380–389. doi: 10.1016/j.ecolind.2009.07.005
Lopez y Royo, C., Silvestri, C., Pergent, G., and Casazza, G. (2009). Assessing human-induced pressures on coastal areas with publicly available data. J. Environ. Manage. 90, 1494–1501. doi: 10.1016/j.jenvman.2008.10.007
Ludwig, W., Dumont, E., Meybeck, M., and Heussner, S. (2009). River discharges of water and nutrients to the Mediterranean and Black Sea: major drivers for ecosystem changes during past and future decades? Progress Oceanogr. 80, 199–217. doi: 10.1016/j.pocean.2009.02.001
Maldonado, A. (1995). La plataforma continental del litoral español. Ing. Agua 2, 31–54. doi: 10.4995/ia.1995.2664
Malone, T., Azzaro di Rosamarina, M., Bode, A., Brown, E., Duce, R., Kamykowski, D., et al. (2017). “Primary production, cycling of nutrients, surface layer and plankton,” in The First Global Integrated Marine Assessment: World Ocean Assessment I, ed Oceans and Law of the Sea, United Nations (Cambridge: Cambridge University Press), 119–148. doi: 10.1017/9781108186148.009
Marin, O., Berov, D., and Todorov, E. (2015). Macrophytobenthic communities from the romanian black sea coast–indicators of the ecological status of coastal water bodies. Cercet. Mar. 45, 195–205.
Nedwell, D. B., Dong, L. F., Sage, A., and Underwood, G. J. C. (2002). Variations of the nutrients loads to the mainland UK estuaries: correlation with catchment areas, urbanization and coastal Eutrophication. Estuarine Coastal Shelf Sci. 54, 951–970. doi: 10.1006/ecss.2001.0867
Neto, J. M., and Juanes, J. A. (2014). “Marine macroalgae and the assessment of ecological conditions,” in Marine Algae: Biodiversity, Taxonomy, Environmental Assessment, and Biotechnology, eds. L. Pereira and J.M. Neto (Coimbra, Portugal: CRC Press), 398. doi: 10.1201/b17540-4
Neumann, B., Vafeidis, A. T., Zimmermann, J., and Nicholls, R. J. (2015). Future coastal population growth and exposure to sea-level rise and coastal flooding - a global assessment. PLoS ONE 10:e0131375. doi: 10.1371/journal.pone.0118571
Nikolic, V., Zuljevic, A., Mangialajo, L., Antolic, B., Kuspilic, G., and Ballesteros, E. (2013). Cartography of littoral rocky-shore communities (CARLIT) as a tool for ecological quality assessment of coastal waters in the Eastern Adriatic Sea. Ecol. Indic. 34, 87–93. doi: 10.1016/j.ecolind.2013.04.021
Nixon, S. W. (2009). Eutrophication and the macroscope. Hydrobiologia 629, 5–19. doi: 10.1007/s10750-009-9759-z
Organisation for Economic Co-operation and Development (1993). “OECD core set of indicators for environmental performance reviews. A synthesis report by the Group on the State of the Environment,” in Environment Monographs (Paris, France).
Orlando, M. (2012). Metodologija vrednotenja ekološkega stanja obalnega morja z makroalgami v Sloveniji; EEI-c, Ekološko Stanje Voda V Sloveniji – Rezultati 2. Ljubljana: Faze Interkalibracije, 14.
Pinedo, S., Jordana, E., and Ballesteros, E. (2015). A critical analysis on the response of macroinvertebrate communities along disturbance gradients: description of MEDOCC (MEDiterranean OCCidental) index. Mar. Ecol. Evol. Persp. 36, 141–154. doi: 10.1111/maec.12126
Platónov, A. K. (2002). Aplicación de Imágenes de Satélite SAR, en los Estudios de Contaminación Marina y de Dinámica de Las Aguas en el Mediterráneo Noroccidental. Barcelona: Universitat Politècnica de Catalunya.
Pons, X. (1994). MiraMon. Sistema d'Informació Geogràfica i Software de Teledetecció. Centre de Recerca Ecològica i Aplicacions Forestals, C. R. E. A.
R Development Core Team (2008). R: A language and environment for statistical computing. (Vienna: R Foundation for Statistical Computing).
Revilla, M. (2015). “Eutrofización en el medio marino del País Vasco: influencia antrópica e impactos sobre el fitoplancton,” in Evaluación de la Presión de Eutrofización y Desarrollo de un Método de Evaluación del Fitoplancton Para Aguas de Transición, eds AZTI (Proyecto: IM14TROFI), 7.
Rice, J. (2003). Environmental health indicators. Ocean Coast. Manag. 46, 235–259. doi: 10.1016/S0964-5691(03)00006-1
Rodriguez, W., August, P. V., Wang, Y. Q., Paul, J. F., Gold, A., and Rubinstein, N. (2007). Empirical relationships between land use/cover and estuarine condition in the Northeastern United States. Landsc. Ecol. 22, 403–417. doi: 10.1007/s10980-006-9036-8
Romero, I., Paches, M., Martinez-Guijarro, R., and Ferrer, J. (2013). Glophymed: an index to establish the ecological status for the water framework directive based on phytoplankton in coastal waters. Mar. Pollut. Bull. 75, 218–223. doi: 10.1016/j.marpolbul.2013.07.028
Schäfer, K., Söding, E., and Zeller, M. (2010). World Ocean Review 1. Living With the Oceans. A Report on the State of the World's Oceans. Hamburg: maribus gGmbH.
Scialabba, N. (1998). Integrated Coastal Area Management and Agriculture, Forestry and Fisheries in: FAO Guidelines (Rome: FAO).
Serra, J., and Canals, M. (1992). “Morfologia submarina i litoral,” in Geologia II, Volum2, Història Natural dels Països Catalans, eds. J. Carreras, R. Folch, J. Gonsàlbez, X. Llimona and J. Terradas. (Barcelona: Enciclopedia Catalana), 390–438.
Springer, A. M., McRoy, C. P., and Flint, M. V. (1996). The Bering Sea Green Belt: shelf-edge processes and ecosystem production. Fish. Oceanogr. 5, 205–223. doi: 10.1111/j.1365-2419.1996.tb00118.x
Stefanov, W. L., Ramsey, M. S., and Christensen, P. R. (2001). Monitoring urban land cover change: an expert system approach to land cover classification of semiarid to arid urban centers. Remote Sens. Environ. 77, 173–185. doi: 10.1016/S0034-4257(01)00204-8
Tan, I., Beken, C. P., and Oncel, S. (2017). Pressure-impact analysis of the coastal waters of Marmara sea. Fresenius Environ. Bull. 26, 2688–2698.
Thomas-Bourgneuf, A. (2013). Guide Relatif aux Règles D'évaluation de L'état des Eaux littorales (Eaux Côtières et eaux de Transition) en vue de la Mise à jour de l'état des lieux 2013 (France: Ministère de l'Écologie, du Développement durable et de l'Énergie, MEDDE).
Tran, C. P., Bode, R. W., Smith, A. J., and Kleppel, G. S. (2010). Land-use proximity as a basis for assessing stream water quality in New York State (USA). Ecol. Indic. 10, 727–733. doi: 10.1016/j.ecolind.2009.12.002
United States Congress. (1972). Federal Water Pollution Control Act Amendments of 1972 (Clean Water Act).
Weisberg, S. B., Thompson, B., Ranasinghe, J. A., Montagne, D. E., Cadien, D. B., Dauer, D. M., et al. (2008). The level of agreement among experts applying best professional judgment to assess the condition of benthic infaunal communities. Ecol. Indic. 8, 389–394. doi: 10.1016/j.ecolind.2007.04.001
Xian, G., Crane, M., and Su, J. (2007). An analysis of urban development and its environmental impact on the Tampa Bay watershed. J. Environ. Manage. 85, 965–976. doi: 10.1016/j.jenvman.2006.11.012
Xiao, H. L., and Weng, Q. H. (2007). The impact of land use and land cover changes on land surface temperature in a karst area of China. J. Environ. Manage. 85, 245–257. doi: 10.1016/j.jenvman.2006.07.016
Yentsch, C. S., and Menzel, D. W. (1963). A method for the determination of phytoplankton chlorophyll and phaeophytin by fluorescence. Deep Sea Res. 10, 221–231. doi: 10.1016/0011-7471(63)90358-9
Keywords: land uses, pressure assessment, coastal waters, pressure-impact relationship, chlorophyll-a, dissolved inorganic nutrients, water framework directive
Citation: Flo E, Garcés E and Camp J (2019) Land Uses Simplified Index (LUSI): Determining Land Pressures and Their Link With Coastal Eutrophication. Front. Mar. Sci. 6:18. doi: 10.3389/fmars.2019.00018
Received: 16 August 2018; Accepted: 15 January 2019;
Published: 19 February 2019.
Edited by:
Jesper H. Andersen, NIVA Denmark Water Research, DenmarkReviewed by:
Joanne I. Ellis, King Abdullah University of Science and Technology, Saudi ArabiaAna C. Brito, Universidade de Lisboa, Portugal
Copyright © 2019 Flo, Garcés and Camp. This is an open-access article distributed under the terms of the Creative Commons Attribution License (CC BY). The use, distribution or reproduction in other forums is permitted, provided the original author(s) and the copyright owner(s) are credited and that the original publication in this journal is cited, in accordance with accepted academic practice. No use, distribution or reproduction is permitted which does not comply with these terms.
*Correspondence: Eva Flo, evaflo@icm.csic.es