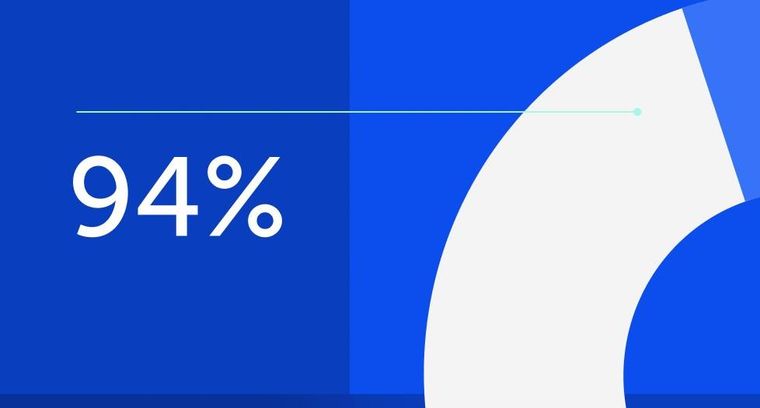
94% of researchers rate our articles as excellent or good
Learn more about the work of our research integrity team to safeguard the quality of each article we publish.
Find out more
ORIGINAL RESEARCH article
Front. Mar. Sci., 17 January 2019
Sec. Marine Conservation and Sustainability
Volume 5 - 2018 | https://doi.org/10.3389/fmars.2018.00513
This article is part of the Research TopicSeaFlower Biosphere Reserve: New Findings and Trends in the Largest Caribbean Marine Protected AreaView all 14 articles
The IUCN considers the stony coral Dendrogyra cylindrus as vulnerable. However, there is insufficient information on its population structure and dynamics, conservation status, or extinction risk and population decreases have been inferred from observations of habitat degradation. In 2002 and 2012, surveys using manta tows, circular plots and satellite images were performed in Old Providence and Santa Catalina Islands (Seaflower Biosphere Reserve) to determine changes in the condition and structure of a local population of D. cylindrus and its habitat. Size-frequency histograms were asymmetric and leptokurtic, showing positive distribution induced by colony fragmentation, which is indicative of reef degradation. Signs of degradation were more evident in 2012, when partial mortality of living tissue in the parent colony yielded 96.6% of the asexually produced fragments. Most of the fragments were from larger colonies (≥115 cm), which exhibited the highest partial and total mortality (>50%). Three of the four benthic habitats used by the species in 2002 were seen in 2012, but with reduced areas. The results suggest that the reduction of living tissue, the dominance of colonies produced asexually, and reduced size of fragments limit population growth and species viability in an unfavorable and changing habitat within this marine protected area (MPA) of the southwestern Caribbean. In the west of the reef complex of Old Providence, a synergy of multiple stressors could cause the habitat degradation and the fragmentation of colonies, limiting the potential recovery of the species and therefore the ability to create a healthy, genetically diverse and resilient population. Thus, these stressors must be minimized to prevent local extinction. Monitoring the population trends and recording sexual recruitment continues to be vital to understand the larvae’s habitat selection and determine whether these habitats are suitable for the survival of coral recruits. Other stressors to be monitored include anchor damage, diseases and bleaching. We recommend the MPA management program to include specific plans of conservation, recovery and restoration for coral reef builders species like D. cylindrus.
Dendrogyra cylindrus Ehrenberg, 1834 is a stony coral with a restricted distribution in the Caribbean. Despite its low abundance in its habitat, this species’ construction of vertical cylindrical columns makes it conspicuous in reefs (INVEMAR, 2010), covering significant horizontal and vertical extensions, and reaching heights of two to three meters (Almy and Carrion, 1963; Prahl and Erhardt, 1985). This species’ characteristic vertical growth increases the habitat’s three-dimensionality (Acosta and Acevedo, 2006), fostering local biodiversity and making it an excellent reef builder. D. cylindrus reproduces both asexually and sexually, releasing sperm and incubating fecundated eggs (Szmant, 1986; Richmond and Hunter, 1990; Marhaver et al., 2015). This coral is slow growing, 0.8–2 cm/year (Hughes, 1987; Hudson and Goodwin, 1997), long-lived, and can compete for space due to its asexual reproduction strategy (Hughes and Tanner, 2000; Darling et al., 2012). D. cylindrus is usually found at depths between 5 and 12 m, as part of fringing reefs along the sheltered shores of Caribbean islands, where the substrate is mainly sand and consolidated dead coral colonized by octocorals, mixed coral, and sponges (Prahl and Erhardt, 1985; Geister and Díaz, 1997; Díaz et al., 2000; Acosta and Acevedo, 2006). In Colombia, the habitat of D. cylindrus has been reported exclusively in the Seaflower Biosphere Reserve which is a marine protected area (MPA). Within this MPA, the reef complex of Old Providence Island hosts more than 90% of the total Colombian D. cylindrus population.
The Seaflower Biosphere Reserve was declared as an MPA in 2005 and covers all the Archipelago of San Andres and it includes the islands and cays of Providence, San Andrés, Bolivar, Alburquerque, Quitasueño, Serrana and Roncador. The reserve has created specific areas where particular activities are allowed or prohibited to safeguard the marine ecosystems. Although there are no coral management and conservation plans, there is a reef monitoring program, but it does not include a detailed monitoring of D. cylindrus population structure. In Old Providence Island, the only protected area is the McBean Lagoon National Natural Park in the northeast, where very few colonies of D. cylindrus remain.
The species’ limited sexual reproduction (Acosta and Acevedo, 2006; Brainard et al., 2011), asynchronous spawning (Marhaver et al., 2015) and propensity to fragmentation, bleaching and diseases contribute to its vulnerability. White plague is a highly virulent disease that affects this species and destroys tissue; type I destroys 3.1 mm day-1 and type II destroys 3.3 mm day-1 (Richardson et al., 1998; Weil, 2004). Its low reproductive output limits population connectivity and recovery within the metapopulation (Rockwood, 2006). These factors, combined with low juvenile survival rates, low observed recruitment, and, possibly, low frequency of reproductive colonies, have the potential to decrease population growth (Aronson et al., 2008).
According to Acosta and Acevedo (2006), the population could be maintained via asexual reproduction by fragmentation using two mechanisms. First, columns of the colony break off and fall and in some cases the ramet fixes to the substrate creating columns by positive phototropism, which allows it to grow vertically and horizontally. Alternatively, the living tissue on the columns dies by natural causes, leaving patches of live tissue on the skeleton, and creating physically isolated fragments (Meesters et al., 1997; Hughes and Tanner, 2000). Excess fragmentation, however, results in constant loss of parent colony live tissue (Lirman, 2000; Cooper et al., 2009), which is expensive to regenerate, high fragment mortality (Hughes and Jackson, 1980; Chadwick-Furman et al., 2000), reduction of average colony size (e.g., Orbicella annularis Pante et al., 2008; Alvarado-Chacón and Acosta, 2009b), and an imbalance in the relative proportion of size classes, resulting in lower population growth (Vandermeer and Goldberg, 2003).
The 2017 IUCN Red List of Threatened Species (Aronson et al., 2008) and NOAA (2014) list D. cylindrus as a vulnerable species because of its declining populations caused by the degradation and destruction of reef habitats (Wilkinson, 2008). The IUCN recommends research on population abundance and trends, ecology and habitat status, threats and resilience, restoration and management of new protected areas. Despite the IUCN’s suggestion, population structure analyses are still scarce for most stony corals, and D. cylindrus is no exception. Besides, it is unknown how disturbances in the last decade have altered the structure and colonization habitat of D. cylindrus on Caribbean coral reefs (Aronson et al., 2008).
Given that the changes in the abundance and distribution over time can be an indication of the health of populations of D. cylindrus, as well as their sustainability in the ecosystem, in this study we evaluate the changes in its population structure and habitat over a decade in the reefs of Old Providence and St. Catalina Islands, which are part of the Seaflower Biosphere Reserve MPA.
Surveys were conducted in the Old Providence and St. Catalina Islands reef complex in the Archipelago of San Andrés, Colombian Caribbean (Figure 1). This archipelago is part of the Seaflower MPA, which was recognized by UNESCO as a Biosphere Reserve. The islands have a shallow marine platform with a 285.2 km2 reef complex (Díaz et al., 2000). The study area covered the shallow reef complex (1–15 m depth) that surrounds the islands.
Figure 1. Study area in Old Providence and St. Catalina Islands. Black circles with stars: plots where colonies were found in 2002 and 2012. White circles with dots: plots where colonies were found in 2002, but not in 2012. Barrier reef: striped area east of the islands. Cambell 1, Cambell 2, and Tete’s Place are popular dive sites.
The data obtained by Acosta and Acevedo (2006) in 2002 was used as a baseline to evaluate the change in population structure for D. cylindrus in 2012. The manta tow technique, which consist of towing a diver with a small boat to produce a general description of large areas of the reef (Rogers et al., 1994), was used to locate and create a spatial distribution map, using zigzag band transects and covering 1.6 km2. In places where more than four colonies were observed, 60 m-diameter circular plots were set in situ to measure the size and condition of colonies and fragments (Figure 1). A total of 20 plots were assessed. The center point of each plot was georeferenced. The 2002 study determined that the habitat and areas with the highest population density were to the west of the islands. This information was used to plan the 2012 sampling. The same 20 plots surveyed in 2002 were resampled, all west of the islands where some of the best-known dive sites are located (Figure 1). No live colonies of D. cylindrus were found in ten of the 2012 plots; only skeletal remains existed, despite extending the diameter of the plots to 70 m to confirm the absence of live colonies (Figure 1).
The population structure for D. cylindrus was determined by measuring the size of the individuals (colonies and fragments) and constructing size-frequency histograms. The maximum height (cm) of each colony and the maximum length (cm) of each fragment were measured and then classified into size classes. The fragments could have two origins, as explained above: (a) physical detachment from the parent colony, where fragments were lying horizontally on the substrate around the colony, or (b) partial mortality of the parent colony, where remaining live tissue generated clones (fragments) on the standing colony. The colonies and fragments were grouped into ten categories according to their size following the Sturges Rule (Llinás and Rojas, 2006). Size-class distribution diagrams were created for the entire population, as well as for colonies and fragments separately to verify their respective contribution to the distribution based on the absolute frequency of individuals in each size class.
Population structure changes between 2002 and 2012 were assessed by comparing the size-class distribution diagrams, calculating their asymmetry (α3) and kurtosis (α4), which were used as base parameters to evaluate the bias of the two curves (skewness and peakedness relative to a normal distribution). We also compared the absolute frequencies of the colonies and fragments by size class, as well as the total average size of the colonies and fragments (height and length, respectively) by applying non-parametric Mann–Whitney U and Z tests and the Kolmogorov–Smirnov tests for two samples. Additionally, we calculated descriptive statistics [mode, geometric mean, and coefficient of variation (CV)] to analyze size variability, in this case, colony height and fragment length.
To determine the condition of the colonies and determine how the condition changed over time, we measured the partial mortality of each colony by identifying the lesions that physically separated part of the live tissue from the rest. We also measured the frequency of colonies with signs of white syndromes (i.e., bleaching and white diseases), as well as erosion at the base of the colony, manifested by a thinning of the colony base that can lead to a toppling. After using these three variables (i.e., average partial mortality by colony, absolute frequency of colonies with white syndromes, and absolute frequency of colonies with erosion at the base) to determine the condition of colonies, we compared the results between 2002 and 2012 using the Mann–Whitney U test. Non-parametric tests were employed because the Kolmogorov–Smirnov test with the Lilliefors correction and Levene test showed non-normality and non-homogeneity of variances of the five dependent variables both untransformed, and after being transformed by square root, log, natural log and Box-Cox.
We identified the habitats used by D. cylindrus in the shallow reef complex (<15 m depth) using the landscape units previously produced by Bernal-Sotelo (2015) for 2000 and 2012, as well as the georeferenced points of the circular plots. This identification was carried out by placing the georeferenced points on each of the maps to visualize the distribution areas of D. cylindrus. Using ArcGIS 10.0 and the shapefile maps of Bernal-Sotelo (2015), we assessed the relationship between the potential area of use of each habitat (i.e., the total area of each habitat where the species is present and which corresponds to the area that could potentially be colonized by D. cylindrus) and the active use observed in each habitat (i.e., the absolute frequency of colonies and fragments present in each plot and habitat). Similarly, we counted the number of times that D. cylindrus populations and their habitat changed within 10 years, and calculated the percentages of change in both cases. We then quantified the relationship between the rate of change of D. cylindrus populations and the rate of habitat change. Using the HaviStat 1.0 program (Montenegro and Acosta, 2008), we estimated Bailey’s confidence intervals for each year to evaluate whether the species used any of the habitats, and analyzed the changes in use by creating graphs of potential area and frequency of observed use.
In 2002, 283 individuals of D. cylindrus were quantified (20 plots, 0.06 km2), of which 213 were colonies and 70 were fragments (Acosta and Acevedo, 2006). In contrast, in 2012, 846 individuals were recorded, of which 261 were colonies and 585 were fragments (present in only ten plots, 0.03 km2). In 2012, the number of fragments increased by 515 in comparison to 2002 (an increase of 313%) which explains the relative increase in population after ten years. There were significant differences in the size of the total population (colonies and fragments) between 2002 and 2012 (U and ZK-S: p < 0.05, n = 1129). In both years, the size-frequency histograms were skewed to the right, showing a leptokurtic distribution (>3: 2002 = 3.7 and 2012 = 8.9), and had positive asymmetry (>0: 2002 = 1 and 2012 = 2.2). This trend was emphasized in 2012 (Figure 2). In 2002, ∼65% of the colonies and fragments were in the first four size classes (<15.1–75 cm), while in 2012, ∼60% of the total the population were in these classes (Figure 2). The average size of the colonies and fragments in 2012 was smaller than in 2002, but had a higher coefficient of variation compared to 2002 (Table 1).
Figure 2. Dendrogyra cylindrus population structure in terms of the frequency of colonies and/or fragments per size class. Total population (including colonies and fragments) in 2002 and 2012 (A,B). Colony contribution per size class in 2002 and 2012 (C,D). Relative contribution of fragments to the size classes in 2002 and 2012 (E,F). Fi: absolute frequency.
Table 1. Temporal comparison of the average size of colonies and fragments comprising the population of Dendrogyra cylindrus, measured as height of colonies and length of fragments in 2002 and 2012.
Colony frequency and height in all size classes were similar in 2002 and 2012 (U and ZK-S: p > 0.05, n = 474), showing a leptokurtic distribution (α4: 2002 = 3.5, 2012 = 4.3) and positive asymmetry (α3: 2002 = 0.9, 2012 = 1.3). In both years, most of the colonies (2002 = 67.6%, 2012 = 70.5%) were in the first five size classes (Figure 2). The colonies in 2012 were 4.7 cm higher (on average) than those in 2002 (Table 1). The frequency and length in all size classes of fragments changed significantly between 2002 and 2012 (U and ZK-S: p < 0.05, n = 655). The distribution of fragment sizes was also leptokurtic (>3) and had positive asymmetry (>0); this trend was heightened in 2012 (α4: 2002 = 4.6, 2012 = 6.0; α3: 2002 = 1.2, 2012 = 1.6). In 2002, ∼70% of the fragments were in the first four size classes, while in 2012, ∼78% were in the first two categories (Figure 2). The fragments in 2002 were, on average, larger than those in 2012 by 22.7 cm, a decrease of 47.3% (Table 1). This size reduction occurred because 96.6% of the fragments in 2012 were clones (living tissue surrounded by the skeleton), while in 2002 this type of fragmentation only represented 30% of the total. The fragments on the columns were smaller than the fragments lying on the substrate near the colony.
The number of colonies affected by some level of partial mortality in 2012 (61.3%) was significantly greater (U: p = 0.03, n = 474) than in 2002 (56.8%; Figure 3). In addition, partial colony mortality was higher in 2012 (25% ± 32.8%) than in 2002 (15.3% ± 21.9%). However, in both years, partial mortality had higher average values in the larger size categories (Figure 3). Partial mortality was significantly higher in 2012 than in 2002 in the largest size classes: 135.1–155 cm (U: p = 0.001, n = 23), 155.1–175 cm (U: p = 0.02, n = 21), and >175 cm (U: p = 0.02, n = 19). In 2012, the colonies in these three classes had an average of 50–80% dead tissue, while in 2002 the percent of dead tissue did not exceed 27% (Figure 3).
Figure 3. Mean proportion of live and dead tissue among colonies within each size class in 2002 (n = 213) and 2012 (n = 261).
The frequency of white syndromes in 2012 was lower compared to 2002, but the difference was not statistically significant (U: p = 0.1, n = 474). However, the number of colonies with erosion at the base was significantly different (U: p = 0, n = 474); more than 50% of colonies in 2002 were eroded, while in 2012, the percentage of colonies with eroded bases did not exceed 25% (Table 2). The most significant differences in frequency of erosion were found in three size classes: 75.1–95 cm (U: p = 0.01, n = 52), 115.1–135 cm (U: p = 0.03, n = 32), and 135.1–155 cm (U: p = 0.04, n = 23) (Table 2). The most notable change was observed in the 115.1–135 cm class, which went from 17 colonies with erosion in 2002 to only three colonies in 2012.
Table 2. Absolute frequency of colonies of D. cylindrus with white syndromes or erosion at the base in 2002 and 2012.
In 2002, D. cylindrus was present in four habitats of the reef complex, defined by the association of D. cylindrus with other dominant benthic species and substrate types (Figure 4, sensu Bernal-Sotelo, 2015). These habitats are represented in Figure 4 with the following numbers: 4 (IB: Acropora palmata-Pseudodiploria strigosa, Millepora spp.-dead coral, sand and octocorals-macroalgae), 10 (FR: Agaricia spp.-mixed corals, sponges-macroalgae and octocorals-antipatharia), 13 (FRp-Lp: Macroalgae, sand, octocorals and dead coral), and 26 (FR-BR-L: Sand and macroalgae). In 2002, 46.2% of sampling plots were in habitat 13, and 38.5%, were in habitat 10. In 2012, D. cylindrus was found in three habitats, two of them new (transformed from the original benthic composition): 11 (FR: Cyanobacteria, macroalgae-dead coral and mixed corals-octocorals-sand) and 12 (FRp: Dead coral, Orbicella spp.-mixed coral, macroalgae, sand and octocorals); and the species remained in habitat 13. Both habitat 11 and habitat 13 each had 42.9% of the sampling plots. In 2012, only dead colonies and eroded skeletons of D. cylindrus were found in habitats 4, 10, and 26 (Figure 4). The deterioration of habitat 4 was reflected in the loss of >70% of calcifying organisms (stony corals and hydrocorals), especially, A. palmata, as well as by the increase of more than 95% of dead coral coverage. Habitat 10 in 2002 was transformed into habitat 11 in 2012, because of the dominance of cyanobacteria, a new benthic component, which in habitat 11 covered 31.9%.
Figure 4. Habitats where D. cylindrus was present in the Old Providence reef complex and their changes over time: (A) 2002 and (B) 2012. Detailed changes in habitat 13 (C) and habitat 10 (D). Map legends are organized from habitats where D. cylindrus had the highest frequency (habitats 11 and 13) to habitats where D. cylindrus had the lowest frequency (habitats 12 and 26). In c and d, the 2000 and 2012 maps show changes in habitat distribution. Cumulative bar plots on the right show the changes in the total area and the area covered by the main components of each habitat. “∙” indicate habitat exclusive of 2012, whereas habitats without symbols were present in both years. The bars within dotted boxes represent the habitats corresponding to coral communities. The area (km2) of each habitat is presented on each bar. Other components: octocorals, rubble and sand. 4 = IB: A. palmata-P. strigosa, Millepora spp.-dead coral, sand and octocorals-macroalgae. 10 = FR: Agaricia spp.-mixed corals, sponges-macroalgae and octocorals-antipatharia. 11 = FR: Cyanobacteria, macroalgae-dead coral and mixed corals-octocorals-sand. 12 = FRp: Dead coral, Orbicella spp.-mixed corals, macroalgae, sand and octocorals. 13 = FRp-Lp: Macroalgae, sand, octocorals and dead coral. 22 = L: Sand, cyanobacteria and octocorals. 23 = FR-BR-L: Sand, macroalgae, rubble and octocorals, 24 FR-BR-L: Cyanobacteria and sand. 24 = FR-BR-L: Cyanobacteria and sand. 25 FR-BR-L: Sand and cyanobacteria. 26 = FR-BR-L: Sand and macroalgae. The first letters of each habitat refer to the geomorphological zone and the type of reef (when it is a coral formation). IB = Internal reef barrier. BR = Backreef terrace. FR = Forereef terrace. FRp = Patch reef on the forereef terrace. L = Lagoon. Lp = Reef in patch (or strip) of the lagoon. In grayscale are the areas where D. cylindrus was not observed. “+” indicate habiat exclusive of 2002.
According to Bailey’s confidence intervals, the population of D. cylindrus on the island primarily used habitat 13 because, in both years, it was the habitat with the highest potential area (2002 = 5.7 km2, 2012 = 4.4 km2) and the habitat with the highest observed use (2002 = 132 individuals, 2012 = 411 individuals), which means that the species used the most available habitat (Figure 5). Habitat 13, where most of the population of D. cylindrus was concentrated, was characterized by the dominance of components such as macroalgae, sand, octocorals, and dead coral, mainly on the leeward fore-reef terrace. Mixed sponges and octocorals (habitat 10) were also found to a lesser extent in 2002.
Figure 5. Habitat used by individual D. cylindrus (including colonies and fragments), in terms of absolute frequency (Fi, white) and area of the six potential habitats (black), in 2002 and 2012.
The unfavorable change in habitat was also reflected in the change of population’s size class structure (Table 3). Habitat 13 lost 23% of its area for D. cylindrus. This generated a population change 11 times greater than in 2002, largely because fragmentation followed partial mortality, whereas habitat 10 lost a relatively smaller area (8.4%) and had a relatively lower population growth (6.1 times). Habitats 4 and 26 were not analyzed because of the low number of colonies and fragments present in the two samples (n was not representative for a change analysis).
The right-skewed size distribution reported for most coral populations is usually considered an indicator of stable to moderately deteriorated environments (Bak and Meesters, 1998; Meesters et al., 2001; Smith et al., 2005), where the populations are recovering as a result of successful sexual recruitment (Bak and Meesters, 1999; Cooper et al., 2009; Rodríguez-Martínez et al., 2010). However, the increase in positive skew over time for D. cylindrus in Old Providence indicates the opposite; a response to a modified environment created an increase in the frequency of smaller sizes produced by fragmentation or fission, resulting from partial mortality with fragments remaining fixed to a parental colony that is slowly dying while standing (Figure 6).
Figure 6. Colonies of D. cylindrus in different condition including: (a) healthy, (b) healthy with associated fauna, (c) fragmented due to anchors breakage, (d) with tissue mortality at the base likely to lead to fragmentation, and (e) dead and colonized by macroalgae and sponges.
In 2012, 96.6% of the total fragments quantified were the result of partial colony mortality. This value surpasses reports of fragmentation for branching species like A. palmata that presented 40% of colonies fragmented after storms (Lirman, 2000) or for massive species such as O. annularis, which, in the Virgin Islands had 9% of colonies undergoing fission by partial mortality (Edmunds and Elahi, 2007). The increase in the average partial mortality per colony of D. cylindrus (2002 = 15.3% and 2012 = 25.0%) indicates that the Old Providence reefs are no longer in good condition; mortality even exceeds the expected values for highly degraded areas (Fong and Glynn, 2001; Bauman et al., 2013).
Larger colonies of D. cylindrus (>115.1 cm) were the most affected by partial and total mortality, contributing to the majority of fragments. The direct relationship between colony size and partial mortality has also been reported for other species from the Pacific and Caribbean (Babcock, 1991; Pante et al., 2008; Bauman et al., 2013). Partial mortality could be a strategy to maximize the area:volume ratio in a colony facing adverse environmental conditions, as less energy is required to sustain a smaller area of living tissue (Hughes and Tanner, 2000; Alvarado-Chacón and Acosta, 2009b). However, a smaller area of living tissue in D. cylindrus (–9.7% in 2012) may result in a reduction of sexual reproduction (van Woesik and Jordán-Garza, 2011). This situation is further exacerbated in the smaller fragments of D. cylindrus, which must invest the energy that was destined for reproduction to repair damaged tissue and compete against invaders.
The absence of sexual recruits could be explained by the lower coverage of D. cylindrus living tissue, which limits the reproductive output of the species (Marhaver et al., 2015). The absence of recruits affects the recovery by self-seeding, as observed by Hughes and Tanner (2000) and Alvarado-Chacón and Acosta (2009a) for other corals. Lack of recruitment could also be attributed to the reproductive biology of this species, which involves asynchronous spawning and rapid embryonic development (Marhaver et al., 2015), possibly preventing dispersal and crossbreeding between populations. Thus, the probability of recovery of this declining population in Old Providence by importing larvae from other populations is unlikely. Besides, the closest D. cylindrus population is 70 km away in Quitasueño Bank (Díaz et al., 2000). Therefore, the viability of the species on the island is dependent on the self-recruitment of large reproductive colonies, which are precisely the ones being lost.
Coral population skewness caused by asexual reproduction have been associated with factors such as temperature (Bauman et al., 2013), diseases (Edmunds and Elahi, 2007), overgrowth of macroalgae and cyanobacteria (Hughes and Tanner, 2000; Edmunds and Elahi, 2007), bioerosion (Fong and Glynn, 1998; McClanahan et al., 2008), eutrophication (Lewis, 1997), hurricanes (Bythell et al., 1993; Taylor et al., 2007), and sedimentation (Pante et al., 2008). All of these stressors have been observed in Old Providence in the last decades and affect the various size classes differently. The cumulative effect of these multiple stressors may explain the demise of D. cylindrus colonies present in the ten sampling plots with only skeletal remains of this species.
The prevalence and severity of coral diseases in the Caribbean have been intensified by factors such as eutrophication, sewage inputs and water temperature (Santavy et al., 2001; Weil, 2004; Cooper et al., 2009). This was not observed in the Old Providence reefs, where the incidence of white syndromes in D. cylindrus decreased from 9.4% in 2002 to 1.5% in 2012. Possible explanations for this result include a more intense impact of bleaching (in 2005 and 2010) and white diseases in the past. D. cylindrus is considered to have medium resistance to white plague and bleaching (Ward et al., 2006; Quinn and Kojis, 2008; Bruckner and Hill, 2009). For example, in Florida (Jaap, 1985), Honduras (Riegl et al., 2009), Jamaica (Quinn and Kojis, 2008) and Puerto Rico (Bruckner and Hill, 2009) from 1983 to 2008, 0–8% of D. cylindrus colonies experienced bleaching, whereas >40% of Acropora spp., Agaricia spp., Helioseris cucullata, O. annularis, Porites spp. and Siderastrea siderea colonies experienced bleaching.
The highest frequency of colonies with erosion at the base in 2002 (51.2% vs. 24.5% in 2012) may be explained by the presence of bioeroders such as Diadema antillarum (Acosta and Acevedo, 2006). In 2002, this urchin was found in the proximity of 13.8% of the colonies with signs of erosion, while in 2012, the urchins were only present in 3.8% of colonies. Also, in 2012, there was less associated fauna with the colonies, an indicator of deterioration of the system and reduced habitat quality. Echinoids of the genus Diadema and Echinometra can exert an adverse effect on Pocillopora spp., Porites spp. and also on massive corals through the mechanical abrasion caused by their spines and because they feed on living tissue and calcium carbonate, weakening the bases of the colonies (Glynn, 1988; Guzmán, 1988; Bak, 1994).
The cumulative effect of multiple stressors is the key factor affecting coral survival around the world (McClanahan et al., 2008). This was observed in this study at Old Providence where habitats 10 and 13 are located (habitats most used by D. cylindrus). Human population density is highest on the west coast of Old Providence (>1000 people/km2). This area contains 95% of dive sites, as well as high tourist use, and the Santa Isabel pier (Vides and Sierra-Correa, 2003; Vivas-Aguas et al., 2012). This implies a higher risk of contamination and physical damage (e.g., anchoring) to colonies. The west coast of Old Providence is also the area with the majority of permanent and occasional freshwater streams and where the sanitary landfill that discharges leachate to the sea is located. Furthermore, in Old Providence wastewater is discharged directly into the sea or disposed of in septic tanks that leak liquid waste into the sea (Vivas-Aguas et al., 2012). These stressors contribute to nutrient enrichment and decrease water quality. The average concentration of dissolved inorganic nitrogen and phosphorus (DIN and DIP) in Old Providence (Vivas-Aguas et al., 2012) indeed exceeded the corals’ limit of physiological stress (DIN = 14 μg l-1, DIP = 62 μg l-1; Fabricius, 2005).
The excess of nutrient loading has caused cyanobacteria and macroalgae to proliferate in Old Providence (Puyana et al., 2015). The habitat considered typical for D. cylindrus has changed because of the growth and dominance of cyanobacteria in habitat 10, which led to its transformation after ten years into habitat 11, where 31.9% of coverage was benthic cyanobacteria. This finding agrees with Ford et al. (2018) who found that mats of cyanobacteria have become prevalent on different reefs of the world, playing an important role in the ecosystem degradation. During blooms, planktonic cyanobacteria also affect the living tissue of D. cylindrus (Puyana et al., 2015). Benthic cyanobacteria colonize skeletal areas exposed between coral fragments, competing for space with living tissue (Ritson-Williams et al., 2005; Kuffner et al., 2006). Competition decreases the individual growth rate of remaining tissue and colonies by up to 30% in places with low water quality (Vermeij and Bak, 2002; Edmunds, 2007). D. cylindrus colonies at Old Providence were 4.7 cm higher in 2002 than in 2012, so colonies around the island may grow 0.47 mm per year (assuming a constant growth rate), which is lower than 0.8–2 cm/year (Hughes, 1987; Hudson and Goodwin, 1997).
Surviving colonies and fragments of D. cylindrus are enduring suboptimal conditions when we compare their current habitat quality with previous decades (Prahl and Erhardt, 1985; Geister and Díaz, 1997). Biogenetic simplification of the habitat or dominance of some unwanted components was the primary driver of the decrease in abundance and density of the associated fauna, as well as the loss of functional and taxonomic diversity (Bustamante et al., 2017). We argue this is the case in Old Providence, with a higher fragmentation of colonies in locations with a higher loss of usable habitat area used by D. cylindrus. For example, habitat 13 lost 23.4% of its suitable area in 10 years and had the number of colonies and fragments increase by 11 times in 2012 than in 2002.
Our results suggest that D. cylindrus populations in Colombia are under high extinction risk, and should be considered as extremely vulnerable. The fragmentation of colonies and habitat transformation is a consequence of the synergy of multiple stressors that can limit the potential recovery of the species in changing habitats. These stressors must be minimized to prevent the species’ local extinction, especially in the west of Old Providence. Therefore, to reduce the local stressors and help improve the resilience of local D. cylindrus populations to climate change, we suggest the following mediation efforts: (a) the improvement of water quality; (b) the evaluation of management strategies effectiveness of the Seaflower MPA and the regulation of land- and water-based activities; (c) the monitoring of population tendencies and recording of sexual recruitment to understand the larvae’s habitat selection and whether these habitats are suitable for recruit survival; (d) the monitoring of natural (e.g., hurricanes, temperature change) and anthropic stressors (e.g., anchors, diving, eutrophication), that can affect the species to collect evidence of the cause-effect relationships and for evidence-based decision making; and (e) the formulation of conservation, recovery and restoration plans that are specific for coral reef builders species like D. cylindrus and can be included in the MPA program. The Seaflower MPA currently has a no-take management zone (García et al., 2005), but diving and tourism activities have not been limited, which implies a risk to coral due to anchoring (Figure 6). The results of these mediation efforts should benefit not only D. cylindrus, but other species and habitats within the MPA, and will contribute to a greater understanding of larval habitat selection and recruitment habitat potential that will enhance the future D. cylindrus populations in Colombia.
KB-S, AA, and JC conceptualized the study and wrote the final manuscript. KB-S and AA did the field work. KB-S analyzed the data, performed the geographical component and structured the document with the supervision of AA and JC. AA made a complementary analysis of the species habitat use.
This work was supported by the University of Costa Rica (FR-082), Javeriana University (004318), and CONARE-CeNAT (Costa Rica) student grant.
The authors declare that the research was conducted in the absence of any commercial or financial relationships that could be construed as a potential conflict of interest.
The work was done with the permits of CORALINA (001-2012) and PNN McBean Lagoon (001-2013). We are grateful to CIMAR (Universidad de Costa Rica), INVEMAR, CORALINA, Catalina Benavides, Ana Fonseca, Trigal Velásquez, Julián González, members of PRIAS-CeNAT and Sirius dive shop. We thank to Lillian R. McCormick for the English review and to the reviewers who helped us to improve the quality of the manuscript.
Acosta, A., and Acevedo, A. (2006). “Population structure and colony condition of Dendrogyra cylindrus (Anthozoa: Scleractinia) in Providencia Island, Colombia Caribbean,” in Proceedings Of the 10th International Coral Reef Symposium, Okinawa, 1605–1610.
Almy, C., and Carrion, T. (1963). Shallow-water stony corals of Puerto Rico. Carib. J. Sci. 3, 133–162
Alvarado-Chacón, E. M., and Acosta, A. (2009a). Fertilidad y fecundidad de Montastraea annularis en un arrecife degradado. Bol. Invest. Mar. Cost. 38, 91–108.
Alvarado-Chacón, E. M., and Acosta, A. (2009b). Population size-structure of the reef-coral Montastraea annularis in two contrasting reefs of a marine protected area in the southern Caribbean Sea. Bull. Mar. Sci. 85, 61–76.
Aronson, R., Bruckner, A., Moore, J., Precht, B., and Weil, E. (2008). Acropora palmata, Dendrogyra cylindrus, Montastraea annularis, Montastraea faveolata, Montastraea franksi. The IUCN Red List of Threatened Species Version2014.3
Babcock, R. C. (1991). Comparative demography of three species of Scleractinian corals using age- and size-dependent classifications. Ecol. Monogr. 61, 225–244. doi: 10.2307/2937107
Bak, R. P. M. (1994). Sea urchin bioerosion on coral reefs: place in the carbonate budget and relevant variables. Coral Reefs 13, 99–103. doi: 10.1007/BF00300768
Bak, R. P. M., and Meesters, E. H. (1998). Coral population structure: the hidden information of colony size-frequency distributions. Mar. Ecol. Prog. Ser. 162, 301–306. doi: 10.3354/meps162301
Bak, R. P. M., and Meesters, E. H. (1999). Population structure as a response of coral communities to global change. Am. Zool. 39, 56–65. doi: 10.1093/icb/39.1.56
Bauman, A. G., Pratchett, M. S., Baird, A. H., Riegl, B., Heron, S. F., and Feary, D. A. (2013). Variation in the size structure of corals is related to environmental extremes in the Persian Gulf. Mar. Environ. Res. 84, 43–50. doi: 10.1016/j.marenvres.2012.11.007
Bernal-Sotelo, J. K. (2015). Cambio Espacio-Temporal (2000-2012) del Complejo Arrecifal de las Islas de Providencia y Santa Catalina, Caribe colombiano. M.Sc. thesis, Universidad de Costa Rica, San Pedro.
Brainard, R. E., Birkeland, C., Eakin, C. M., McElhany, P., Miller, M. W., Patterson, M., et al. (2011). Status Review Report of 82 Candidate Coral Species Petitioned Under the U.S. Endangered Species Act. Tech. Memo. NOAA-TM-NMFS-PIFSC-27. Honolulu, HI: NOAA.
Bruckner, A. W., and Hill, R. L. (2009). Ten years of change to coral communities off Mona and Desecheo Islands, Puerto Rico, from disease and bleaching. Dis. Aquat. Organ. 87, 19–31. doi: 10.3354/dao02120
Bustamante, M., Tajadura, J., Díez, I., and Saiz-Salinas, J. I. (2017). The potential role of habitat-forming seaweeds in modeling benthic ecosystem properties. J. Sea Res. 130, 123–133. doi: 10.1016/j.seares.2017.02.004
Bythell, J. C., Gladfelter, E. H., and Bythell, M. (1993). Chronic and catastrophic natural mortality of three common Caribbean reef corals. Coral Reefs 12, 143–152. doi: 10.1007/BF00334474
Chadwick-Furman, N. E., Goffredo, S., and Loya, Y. (2000). Growth and population dynamic model of the reef coral Fungia granulosa Klunzinger, 1879 at Eilat, northern Red Sea. J. Exp. Mar. Biol. Ecol. 249, 199–218. doi: 10.1016/S0022-0981(00)00204-5
Cooper, T. F., Gilmour, J. P., and Fabricius, K. E. (2009). Bioindicators of changes in water quality on coral reefs: review and recommendations for monitoring programs. Coral Reefs 28, 589–606. doi: 10.1007/s00338-009-0512-x
Darling, E. S., Alvarez-Filip, L., Oliver, T. A., McClanahan, T. R., and Côté, I. M. (2012). Evaluating life-history strategies of reef corals from species traits. Ecol. Lett. 15, 1378–1386. doi: 10.1111/j.1461-0248.2012.01861.x
Díaz, J. M., Barrios, L. M., Cendales, M. H., Garzón-Ferreira, J., Geister, J., López-Victoria, M., et al. (2000). Áreas Coralinas de Colombia. Santa Marta: INVEMAR.
Edmunds, P. J. (2007). Evidence for a decadal-scale decline in the growth rates of juvenile scleractinian corals. Mar. Ecol. Prog. Ser. 341, 1–13. doi: 10.3354/meps341001
Edmunds, P. J., and Elahi, R. (2007). The demographics of a 15-year decline in cover of the Caribbean reef coral Montastraea annularis. Ecol. Monogr. 77, 3–18. doi: 10.1890/05-1081
Ehrenberg, C. G. (1834). Beiträge zur physiologischen Kenntniss der Corallenthiere im Allgemeinen, und Besonders des Rothen Meeres, nebst einem Versuche zur Physiologischen Systematik Derselben, Vol. 1. Berlin: Abhandlungen der Königlichen Akademie der Wissenschaften, 225–380. Available at: http://bibliothek.bbaw.de/bibliothek-digital/digitalequellen/schriften/anzeige?band=07-abh/1832-1&seite:int=00000243
Fabricius, K. E. (2005). Effects of terrestrial runoff on the ecology of corals and coral reefs: review and synthesis. Mar. Pollut. Bull. 50, 125–146. doi: 10.1016/j.marpolbul.2004.11.028
Fong, P., and Glynn, P. W. (1998). A dynamic size-structured population model: does disturbance control size structure of a population of the massive coral Gardineroseris planulata in the Eastern Pacific? Mar. Biol. 130, 663–674. doi: 10.1007/s002270050288
Fong, P., and Glynn, P. W. (2001). Population abundance and size-structure of an Eastern Tropical Pacific reef coral after the 1997-98 ENSO: a simulation model predicts field measures. Bull. Mar. Sci. 69, 187–202.
Ford, A. K., Bejarano, S., Nugues, M. M., Visser, P. M., Albert, S., and Ferse, S. C. A. (2018). Reefs under siege-the rise, putative drivers, and consequences of benthic cyanobacterial mats. Front. Mar. Sci. 5, 1–15. doi: 10.3389/fmars.2018.00018
García, M. I., Howard, M., Charris, S., Hawkins, E., Taylor, M., Prada, M. C., et al. (2005). Plan de Manejo del ÁREA Marina Protegida Seaflower, Parte II. (Informe del proyecto Caribbean Archipelago - Biosphere Reserve: Regional Marine Protected Area System CO-GM-P066646). San Andrés: GEF-TOC-CORALINA.
Geister, J., and Díaz, J. M. (1997). “A field guide to oceanic barrier and atolls of the southwestern Caribbean (San Andrés y Providencia),” in Proceedings of the 8th International. Coral Reef Symposium, Panama.
Glynn, P. W. (1988). El Niño warming, coral mortality and reef framework destruction by echinoid bioerosion in the eastern Pacific. Galaxea 7,129–160.
Guzmán, H. M. (1988). Distribución y abundancia de organismos coralívoros en los arrecifes coralinos de la Isla del Caño, Costa Rica. Rev. Biol. Trop. 36, 191–207.
Hudson, J. H., and Goodwin, W. B. (1997). “Restoration and growth rate of hurricane damaged pillar coral (Dendrogyra cylindrus) in the Key Largo National Sanctuary, Florida,”. in Proceedings of the 8th International. Coral Reef Symposium, Smithsonian Tropical Research Institute, Panama, 567–570.
Hughes, T. P. (1987). Skeletal density and growth form of corals. Mar. Ecol. Prog. Ser. 35, 259–266. doi: 10.3354/meps035259
Hughes, T. P., and Jackson, J. B. C. (1980). Do corals lie about their age? some demographic consequences of partial mortality, fission and fusion. Science 209, 713–715. doi: 10.1126/science.209.4457.713
Hughes, T. P., and Tanner, J. E. (2000). Recruitment failure life histories, and long-term decline of Caribbean corals. Ecology 81, 2250–2263. doi: 10.1890/0012-9658(2000)081[2250:RFLHAL]2.0.CO;2
INVEMAR (eds) (2010). Corales Escleractíneos de Colombia. Santa Marta: Instituto de Investigaciones Marinas y Costeras José Benito Vives de Adréis.
Jaap, W. C. (1985). “An epidemic zooxanthellae expulsion during 1983 in the lower Florida Keys coral reefs: hyperthermic etiology,” in Proceedings of the. 5th International. Coral Reef Symposium, Tahiti, 143–148.
Kuffner, I., Walters, L., Becerro, M., Paul, V., Ritson-Williams, R., and Beach, K. (2006). Inhibition of coral recruitment by macroalgae and cyanobacteria. Mar. Ecol. Prog. Ser. 323, 107–117. doi: 10.3354/meps323107
Lewis, J. B. (1997). Abundance, distribution and partial mortality of the massive coral Siderastrea siderea on degrading coral reefs at Barbados, West Indies. Mar. Pollut. Bull. 34, 622–627. doi: 10.1016/S0025-326X(96)00184-1
Lirman, D. (2000). Fragmentation in the branching coral Acropora palmata (Lamarck): growth, survivorship, and reproduction of colonies and fragments. J. Exp. Mar. Biol. Ecol. 251, 41–57. doi: 10.1016/S0022-0981(00)00205-7
Llinás, H., and Rojas, C. (2006). Estadística Descriptiva y Distribuciones de Probabilidad. Barranquilla: Ediciones Uninorte.
Marhaver, K. L., Vermeij, M. J. A., and Medina, M. M. (2015). Reproductive natural history and successful juvenile propagation of the threatened Caribbean Pillar Coral Dendrogyra cylindrus. BMC Ecol. 15, 1–11. doi: 10.1186/s12898-015-0039-7
McClanahan, T. R., Ateweberhan, M., and Omukoto, J. (2008). Long-term changes in coral colony size distributions on Kenyan reefs under different management regimes and across the 1998 bleaching event. Mar. Biol. 153, 755–768. doi: 10.1007/s00227-007-0844-4
Meesters, E. H., Hilterman, M., Kardinaal, E., Keetman, M., de Vries, M., and Bak, R. P. M. (2001). Colony size-frequency distributions of scleractinian coral populations: spatial and interspecific variation. Mar. Ecol. Prog. Ser. 209, 43–54. doi: 10.3354/meps209043
Meesters, E. H., Wesseling, I., and Bak, R. P. M. (1997). Coral colony tissue damage in six species of reef-building corals: partial mortality in relation with depth and surface area. J. Sea. Res. 37, 131–144. doi: 10.1016/S1385-1101(96)00004-4
Montenegro, J. A., and Acosta, A. (2008). HaviStat v1.0: aplicación para evaluar uso y preferencia de hábitat. PANAMJAS 3, 2–4.
NOAA (2014). Endangered and Threatened Wildlife and Plants: Final Listing Determinations on Proposal to List 66 Reef-Building Coral Species and to Reclassify Elkhorn and Staghorn Corals. Final rule. Miami, FL: National Oceanic and Atmospheric Administration.
Pante, E., King, A., and Dustan, P. (2008). Short-term decline of a Bahamian patch reef coral community: rainbow gardens reef 1991-2004. Hydrobiologia 596, 121–132. doi: 10.1007/s10750-007-9062-9
Puyana, M., Acosta, A., Bernal-Sotelo, K., Velásquez-Rodríguez, T., and Ramos, F. (2015). Spatial scale of cyanobacterial blooms in old providence island, colombian caribbean. Univ. Sci. 20, 83–105. doi: 10.11144/Javeriana.SC20-1.sscb
Quinn, N., and Kojis, B. (2008). The recent collapse of a rapid phase-shift reversal on a Jamaican north coast coral reef after the 2005 bleaching event. Rev. Biol. Trop. 56 (Suppl. 1), 149–159.
Richardson, L. L., Goldberg, W. M., Carlton, R. G., and Halas, J. C. (1998). Coral disease outbreak in the Florida Keys: Plague type II. Rev. Biol. Trop. 46, 187–198.
Richmond, R. D., and Hunter, C. L. (1990). Reproduction and recruitment of corals: comparison among the Caribbean, the Tropical Pacific and the Red Sea. Mar. Ecol. Prog. Ser. 60, 185–203. doi: 10.3354/meps060185
Riegl, B., Purkis, S. J., Keck, J., and Rowlands, G. P. (2009). Monitored and modeled coral population dynamics and the refuge concept. Mar. Pollut. Bull. 58, 24–38. doi: 10.1016/j.marpolbul.2008.10.019
Ritson-Williams, R., Paul, V., and Bonito, V. (2005). Marine benthic cyanobacteria overgrow coral reef organisms. Coral Reefs 24:629. doi: 10.1007/s00338-005-0059-4
Rodríguez-Martínez, R. E., Ruíz-Renteria, F., van Tussenbroek, B., Barba-Santos, G., Escalante-Mancera, E., Jordán-Garza, G., et al. (2010). Environmental state and tendencies of the puerto morelos Caricomp site, Mexico. Rev. Biol. Trop. 58 (Suppl. 3), 23–43.
Rogers, C. S., Garrison, G., Grober, R., Hillis, Z. M., and Franke, M. A. (1994). Coral reef monitoring manual for the Caribbean and Western Atlantic. St. John: National Park Service.
Santavy, D. L., Mueller, E., Peters, E. C., MacLaughlin, L., Porter, J. W., Patterson, K. L., et al. (2001). Quantitative assessment of coral diseases in the Florida keys: strategy and methodology. Hydrobiologia 460, 39–52. doi: 10.1023/A:1013194422440
Smith, L. D., Devlin, M., Haynes, D., and Gilmour, J. P. (2005). A demographic approach to monitoring the health of coral reefs. Mar. Pollut. Bull. 51, 399–407. doi: 10.1016/j.marpolbul.2004.11.021
Szmant, A. M. (1986). Reproductive ecology of Caribbean reef corals. Coral Reefs 5, 43–54. doi: 10.1007/BF00302170
Taylor, E., Hernández, D., Howard, F., Peñaloza, G., Posada, S., Howard, N., et al. (2007). Impacto en los arrecifes de coral ocasionados por el huracán Beta en la plataforma insular de Old Providencie y Santa Catalina. Bol. Cient. CIOH 25, 71–77. doi: 10.26640/01200542.25.71_77
van Woesik, R., and Jordán-Garza, A. G. (2011). Coral populations in a rapidly changing environment. J. Exp. Mar. Biol. Ecol. 408, 11–20. doi: 10.1016/j.jembe.2011.07.022
Vandermeer, J. H., and Goldberg, D. E. (2003). Population Ecology First Principles. Princeton, NJ: Princeton University Press.
Vermeij, M. J. A., and Bak, R. P. M. (2002). “Inferring demographic processes from population size structure in corals,” in Proceedings of the 9th International Coral Reef Symposium, Bali, 589–593.
Vides, M., and Sierra-Correa, P. (2003). Atlas de Paisajes Costeros de Colombia. Santa Marta: INVEMAR.
Vivas-Aguas, L. J., Garay, J. A., Espinosa, L., Abdulazis, P., Bent, O., Osorio, L., et al. (2012). “Calidad ambiental en las islas de San Andrés, Providencia y Santa Catalina”, in Atlas de la Reserva de Biósfera Seaflower Archipiélago de San Andrés, Providencia y Santa Catalina, eds D. I. Gómez-López, C. Segura-Quintero, P. C. Sierra-Correa, and J. Garay-Tinoco (Santa Marta: INVEMAR), 60–85.
Ward, J., Rypien, K., Bruno, J., Harvell, C., Jordán-Dahlgren, E., Mullen, K., et al. (2006). Coral diversity and disease in Mexico. Dis. Aquat. Organ. 69, 23–31. doi: 10.3354/dao069023
Weil, E. (2004). “Coral reef diseases in the Wider Caribbean” in Coral Health and Disease, eds E. Rosenberg, and Y. Loya (New York, NY: Springer), 35–68. doi: 10.1007/978-3-662-06414-6_2
Keywords: Dendrogyra cylindrus, population size distribution, habitat, partial mortality, fragmentation
Citation: Bernal-Sotelo K, Acosta A and Cortés J (2019) Decadal Change in the Population of Dendrogyra cylindrus (Scleractinia: Meandrinidae) in Old Providence and St. Catalina Islands, Colombian Caribbean. Front. Mar. Sci. 5:513. doi: 10.3389/fmars.2018.00513
Received: 11 July 2018; Accepted: 21 December 2018;
Published: 17 January 2019.
Edited by:
Sonia Bejarano, Leibniz Centre for Tropical Marine Research (LG), GermanyReviewed by:
Jose M. Fariñas-Franco, National University of Ireland Galway, IrelandCopyright © 2019 Bernal-Sotelo, Acosta and Cortés. This is an open-access article distributed under the terms of the Creative Commons Attribution License (CC BY). The use, distribution or reproduction in other forums is permitted, provided the original author(s) and the copyright owner(s) are credited and that the original publication in this journal is cited, in accordance with accepted academic practice. No use, distribution or reproduction is permitted which does not comply with these terms.
*Correspondence: Katherine Bernal-Sotelo, amthdGhlcmluZWJzQGdtYWlsLmNvbQ==
Disclaimer: All claims expressed in this article are solely those of the authors and do not necessarily represent those of their affiliated organizations, or those of the publisher, the editors and the reviewers. Any product that may be evaluated in this article or claim that may be made by its manufacturer is not guaranteed or endorsed by the publisher.
Research integrity at Frontiers
Learn more about the work of our research integrity team to safeguard the quality of each article we publish.