- 1Integrative Oceanography Division and Center for Marine Biodiversity and Conservation, Scripps Institution of Oceanography, La Jolla, CA, United States
- 2Florida State University Coastal and Marine Laboratory, St. Teresa, FL, United States
Ocean deoxygenation has become a topic of increasing concern because of its potential impacts on marine ecosystems, including oxygen minimum zone (OMZ) expansion and subsequent benthic effects. We investigated the influence of oxygen concentration and organic matter (OM) availability on metazoan meiofauna within and below an OMZ in bathyal sediments off Costa Rica, testing the hypothesis that oxygen and OM levels are reflected in meiofaunal community structures and distribution. Mean total densities in our sampling cores (400–1800 m water depth) were highest with 3688 ind. 10 cm−2 at the OMZ core at 400 m water depth, decreasing rapidly downslope. Nematodes were overall dominant, with a maximum of 99.9% in the OMZ core, followed by copepods (13%), nauplii (4.8%), and polychaetes (3%). Relative copepod and nauplii abundance increased consistently with depth and increasing bottom-water O2. Meiofaunal composition was significantly different among sites, with lower taxonomic diversity at OMZ sites relative to deeper, oxygenated sites. Vertical distribution patterns within sediments showed that in strongly oxygen-depleted sites less meiofauna was concentrated in the surface sediment than at deeper slope sites. Highest meiofaunal abundance and lowest diversity occurred under lowest oxygen and highest pigment levels, whereas highest diversity occurred under highest oxygen-concentrations and low pigments, as well as high quality of sedimentary pigment (chl a/phaeo) and organic carbon (C/N). The lower meiofaunal diversity, and lower structural and trophic complexity, at oxygen-depleted sites raises concerns about changes in the structure and function of benthic marine ecosystems in the face of OMZ expansions.
Introduction
Large areas of the bathyal eastern Pacific continental margin harbor significant fisheries resources associated with upwelling, but are lined by oxygen-depleted waters (O2 < 0.5 ml L−1 or <22 μmol kg−1) known as oxygen minimum zones (OMZs) usually found between 100 and 1200 m water depth (Levin, 2003). OMZs are most prevalent in the eastern Pacific Ocean, the Arabian Sea, and off west Africa (Karstensen et al., 2008; Paulmier and Ruiz-Pino, 2009). The world’s largest OMZ occurs along the eastern Pacific covering over 360,000 km2 of seabed, and varying in intensity and distribution with climate variability (Helly and Levin, 2004). Global warming resulting from climate change has exacerbated ocean deoxygenation in coastal and oceanic regions (Keeling et al., 2010; Schmidtko et al., 2017; Breitburg et al., 2018; Levin, 2018), leading to expansion of tropical OMZs in recent decades (Stramma et al., 2008; IPCC, 2014; Gobler and Baumann, 2016). Ocean deoxygenation has become a topic of increasing concern for scientists because of the damage that climate-driven oxygen loss will cause to ocean ecosystems (Stramma et al., 2010; Levin and Breitburg, 2015; Levin, 2018).
Oxygen minimum zones result from oxygen depletion and respiration often combined with sluggish circulation and oxygen-poor source waters. Where OMZs intercept the continental margins, strong oxygen and organic-matter gradients, often highly heterogeneous, are formed (Levin, 2003). These gradients influence the biogeochemical properties of sediments (Cowie and Levin, 2009), with consequences for the benthic ecosystem (Gooday et al., 2010; Stramma et al., 2010). Dissolved oxygen in the ocean is a key variable structuring communities because it modifies the energy flow in ecosystems (Wright et al., 2012), which can cause shifts in community structure and trophic transfer, as well as changes in productivity and biogeochemical cycling (Arntz et al., 2006). At OMZs, severe reductions of macro- and megafauna abundance and diversity occur (Levin, 2003; Gooday et al., 2009). Extended periods of hypoxia may negatively affect meiofaunal communities, reducing total densities and the most sensitive taxa and life stages (Murrell and Fleeger, 1989; Sergeeva and Zaika, 2013). However, there is evidence that metazoan meiofauna are much less negatively affected by low oxygen than the macrofauna (Cook et al., 2000; Neira et al., 2001b,c; Sellanes and Neira, 2006; Zeppilli et al., 2015).
Meiofauna are the most abundant metazoans in deep-sea sediments and play a critical role in trophic ecology and ecosystem functioning (Zeppilli et al., 2015; Schratzberger and Ingels, 2017). They have a short life cycle and respond quickly to disturbance and pollution, making them ideal subjects to study ecological change (Mahmoudi et al., 2005; Danovaro et al., 2008; Van Oevelen et al., 2011; Schratzberger and Ingels, 2017). There is consensus that metazoan meiofauna cope better with hypoxia than macrofauna and megafauna (Neira et al., 2001b). Thus, under oxygen decline (Schmidtko et al., 2017) and OMZ expansion (Stramma et al., 2008), an infaunal functional shift toward meiofauna may occur (Neira et al., 2013), with likely consequences for essential ecosystem functions. Although meiofauna represent the major metazoan component within the eastern Pacific OMZ, they remain poorly studied (Neira et al., 2001c,a, 2005, 2013; Neira and Decraemer, 2009; Veit-Köhler et al., 2009) and their community responses to oxygen depletion have not often been investigated.
In spite of considerable knowledge gained in recent decades on deep-sea meiobenthos in extreme marine ecosystems (Zeppilli et al., 2015, 2018), very little is known about them in OMZs. To our knowledge, there are no quantitative meiofauna studies in deep-sea areas associated with the OMZ in the geographic region off Costa Rica. The Pacific coast of Costa Rica, 1164 km in length, has a large diversity of coastal habitats such as sandy, muddy and rocky beaches, mangroves, estuaries, islands, bays, and gulfs (Cortés and Jiménez, 2003; Quesada-Alpízar and Cortés, 2006), and a tropical fjord (Golfo Dulce), an anoxic basin that intermittently receives oxygen-enriched water (Nichols-Driscoll, 1976; León-Morales and Vargas, 1998).
Previous studies for Costa Rica where meiofauna have been considered are very scarce and are restricted to the Gulf of Nicoya, and focused on meiofauna community structure variations in soft bottom beaches (e.g., De la Cruz and Vargas, 1986, 1987; Vargas, 1988) and coral reefs around Isla del Caño (Guzmán et al., 1987). The OMZ depicts substantial habitat heterogeneity, e.g., step gradients in oxygen, variation in the OM quantity and quality, redox potential, pH, multiple water masses with different physical–chemical characteristics (Gooday et al., 2010; Levin et al., 2010), providing a natural scenario to address whether these changes are reflected in differing meiofauna assemblages and spatial distributions along the Costa Rica slope. The aim of the present work was to investigate the effects of the OMZ on meiofaunal communities in terms of abundance, composition, taxon diversity and spatial distribution in the sediment column, and identify environmental factors that most likely structure communities along a bathymetric transect (400–1800 m depth) on the Costa Rica margin. The overarching hypothesis guiding this study is that spatial habitat heterogeneity driven by bottom-water oxygen and OM availability within the OMZ selects for distinct meiofaunal community structure and spatial distribution relative to deeper, more oxygenated slope sites.
Materials and Methods
Study Area and Sample Processing
Between February 21 and March 6, 2009 slope sediment samples were collected during a cruise on board of R/V Atlantis (Leg AT 15-44) off Costa Rica’s Pacific margin. This was complementary to a larger study focused on macrofauna inhabiting methane seep carbonates. The area is characterized by an intense, well-developed, mid-water OMZ which impinges on the margin at depths between 300 and 700 m (Levin et al., 2015). Along the Costa Rica margin there is a high degree of local and regional heterogeneity caused by carbonates, submarine fluid venting sites, methane seeps, hydrothermal seeps, mud mounds, seamount subduction scars, and slope failures as well-strong vertical hydrographic gradients (Han et al., 2004; Mau et al., 2006; Sahling et al., 2008; Füri et al., 2010; Levin et al., 2012, 2015). Surface net primary production (NPP) is relatively high, as a result of macronutrient supply associated with thermocline shoaling, mixing and wind-driven upwelling, and exhibits intra-annual variability with highest primary production in September–October and lowest in January–February (Nielsen-Muñoz and Quesada-Alpízar, 2006; Pennington et al., 2006). Thus, the OMZ along with both topography and hydrography result in strong gradients in bottom-water oxygen concentration, temperature, and pH over a relatively short depth range (Levin et al., 2015).
Sediment samples were collected with a multicorer (9.6 cm core liners i.d.) at six open slope sites (400, 620, 800, 1000, 1200, and 1800 m water depth; Figure 1). At each depth, three independent multicorer deployments were conducted (except the 1200 m site n = 2). Only undisturbed cores with clear overlying water were used. Subsamples were taken simultaneously from the same corer, for meiofauna and sediment properties, using Perspex tubes (3.6 cm inner diameter, 10 cm−2). All sediment cores were sectioned vertically on board ship at 0–1, 1–2, 2–3, 3–5, and 5–10 cm. Sections were preserved in 4% buffered formalin (Pfannkuche and Thiel, 1988) for meiofauna analysis, while fractions for sediment properties were frozen until analysis. Water content (WC) was determined by weight loss after freeze-drying sediment aliquots. Sediment total organic carbon (TOC) and total nitrogen (TN) were determined from freeze-dried, homogenized sediment using a Costech CHN analyzer (Valencia, CA, United States) after removal of inorganic carbonates. Chl a and phaeopigments were determined spectrophotometrically from freeze-dried sediment (Hagerthey et al., 2006) after extraction with 90% acetone (Danovaro, 2010). Chloroplastic pigment equivalent (CPE), i.e., the sum of chl a and phaeopigments was used as a measure of OM of phytodetrital origin. The ratio chl a to phaeopigments was used as a proxy of ‘freshness’ of the OM derived from primary production (García and Thomsen, 2008). The chl a to TOC ratio was used to indicate bioavailability of the bulk organic matter (OM; i.e., reflecting contribution of organic carbon of phytoplanktonic origin, Ramalho et al., 2014). Net primary production values for study sites were provided by M. Kahru (SIO) and were estimated from satellite data of chl-a concentration, photosynthetically active radiation and sea-surface temperature using an empirical algorithm (Kahru et al., 2012). Hydrographic data including temperature, salinity, dissolved oxygen, and pH were obtained from CTD casts.
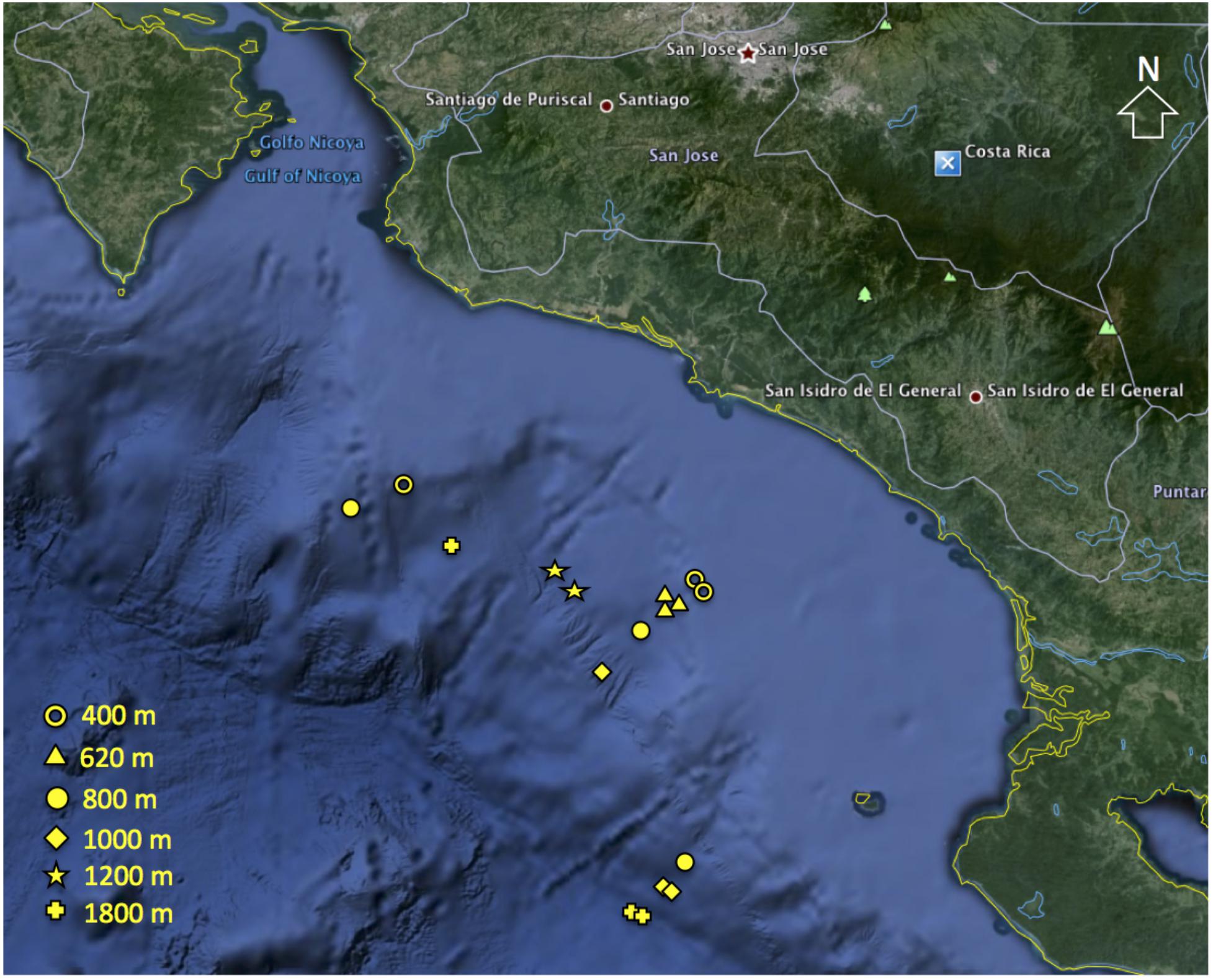
FIGURE 1. Location of meiofaunal sampling sites along the Costa Rica margin (Map data: 2009 Google Earth).
Metazoan meiofauna were extracted from sediment by suspension-decantation (Pfannkuche and Thiel, 1988) using a 40 μm-sieve as the lower limit. The procedure was repeated at least five times for the 1-cm sections, 8–10 times for the larger fractions. Residual sediment was examined for extraction efficiency (>98%, Neira et al., 2013). All meiofaunal organisms were counted and classified to higher taxon level under a stereoscopic microscope after staining with Rose Bengal (0.5 g L−1) (Pfannkuche and Thiel, 1988; Giere, 2009). Nauplii were counted separately given their ecological differences in size and diet compared to adults and copepodites (Decho and Fleeger, 1988).
Data Analysis
Meiofaunal density, taxon richness (number of higher taxa) and diversity indices [Pielou’s evenness (J′), Shannon–Wiener (H′ log10) and Rank 1 dominance (R1D, the proportion of the most abundant taxon, which is an inverse indicator of assemblage evenness)] were used to describe meiofauna assemblage structure. Taxon richness was also examined through rarefaction curves (number of taxa per number of individuals sampled – Hurlbert, 1971).
Meiofauna community composition (multivariate, square root transformed, Bray–Curtis Similarity) and total meiofauna abundance (univariate, square root transformed, Euclidean Distance) were analyzed with PERMANOVA tests (three-factor design: water depth, sediment depth, core). The random factor ‘core ‘was inserted as nested in water depth to account for the dependency of the sediment layers within each core; this approach has been used in several studies as a good solution to the sediment layer dependence issue (e.g., Ingels and Vanreusel, 2013; Rosli et al., 2016). This design allows us to investigate differences between water depths and sediment layers simultaneously. Additional PERMANOVA tests were performed on the meiofauna community matrix and the individual diversity indices (based on pooled 0–10 cm sediment data). Pairwise tests were performed for main factors and interactions when significant results were obtained. PERMDISPs were performed when PERMANOVA returned significant results, to investigate heterogeneity in dispersions. Monte Carlo tests were applied when the number of available permutations was <100. Non-parametric multidimensional scaling ordination (nMDS, square root transformation, Bray–Curtis Similarity) was used to visualize the dissimilarity in meiofaunal community structure among sites. Similarity percentage (SIMPER) analysis was used to determine which taxa were responsible for observed differences (Clarke, 1993).
Environmental data were subjected to univariate PERMANOVA analysis, testing differences between water depths for each variable separately (no transformation, Euclidean distance). Spearman-rank correlations were performed to examine collinearity between environmental variables, and possible relationships between environmental variables and meiofaunal taxa abundance. Redundancy analysis (RDA) was used to examine correlations between meiofaunal community composition and environmental variables (Ter Braak and Šmilauer, 2012) in order to assess the extent of the contribution of each variable to variance in community composition. Prior to RDA, a forward stepwise selection procedure was performed to minimize redundancy of variables (between-variable correlations) and select a set of explanatory environmental variables that account for the composition variation (Lepš and Šmilauer, 2003). This was followed by a Monte Carlo permutation test to determine significant relationships between the taxa and environment.
All above analyses were performed with PRIMER v6 (Clarke and Gorley, 2006) and the PERMANOVA+ add-on (Anderson et al., 2008), except for the Spearman Rank correlations (JMP Pro 12.0.1., SAS Institute, Inc.) and the RDA ordination and gradient analysis [CANOCO for Windows 5 (Ter Braak and Šmilauer, 2012)].
Results
Environmental Properties
Environmental and sediment properties are summarized in Table 1. Bottom-water temperature decreased progressively with depth along the entire transect, while bottom-water dissolved oxygen (DO) values progressively increased with depth, with a minimum of 0.05 ml L−1 at the 400 m site, and maximum of 1.61 ml L−1 at the 1800 m site. Bottom-water pH fluctuated between 7.68 and 7.77 along the transect. The OMZ 400 (core) and 620 m sites were enriched in TOC (∼2.5%DW) and CPE (147 μg g−1) compared to the deepest oxygenated site (∼1.1%DW, 8.2 μg g−1, respectively). The chl a value at the OMZ core site (400 m) was very high, as was the phaeopigment concentration, leading to a lower ‘freshness’ (chl a/phaeo) value than was observed at 800 m and deeper.
Quality of available OM was least refractory at the deepest site (1800 m), with lowest C/N (7.7) and highest chl a/phaeo (1.54). The vertical profile of chl a/phaeo values along the transect is shown in Figure 2. Sediment environmental variables were significantly different between study sites (PERMANOVA; Supplementary Table S1). Surface water chl a has been considered an indicator of the flux of phytodetrital OM to the seabed and thereby food availability to benthic animals (Rex and Etter, 2010). The relatively high and homogeneous NPP in surface waters for the study area (271–332 mg C m−2 d−1) calculated for the sampling period (Table 1) was reflected in the partially degraded phytopigments found in sediments. Indeed, sediment C/N ratio compared with δ13C signatures indicate that deposited sedimentary OM is predominantly of marine origin (Figure 3).
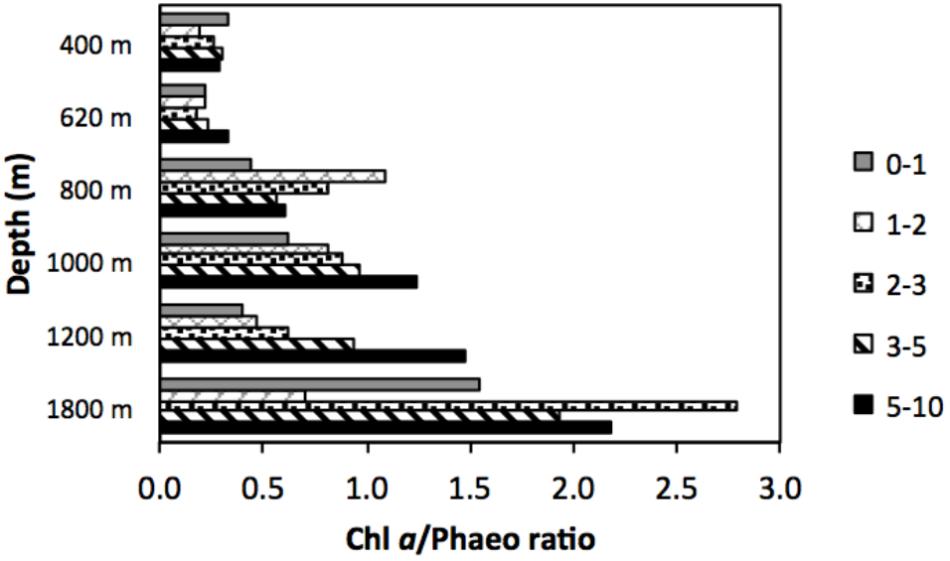
FIGURE 2. Vertical profile of the ratios of chlorophyll a to phaeopigments (chl a/phaeo) in slope sediments off Costa Rica.
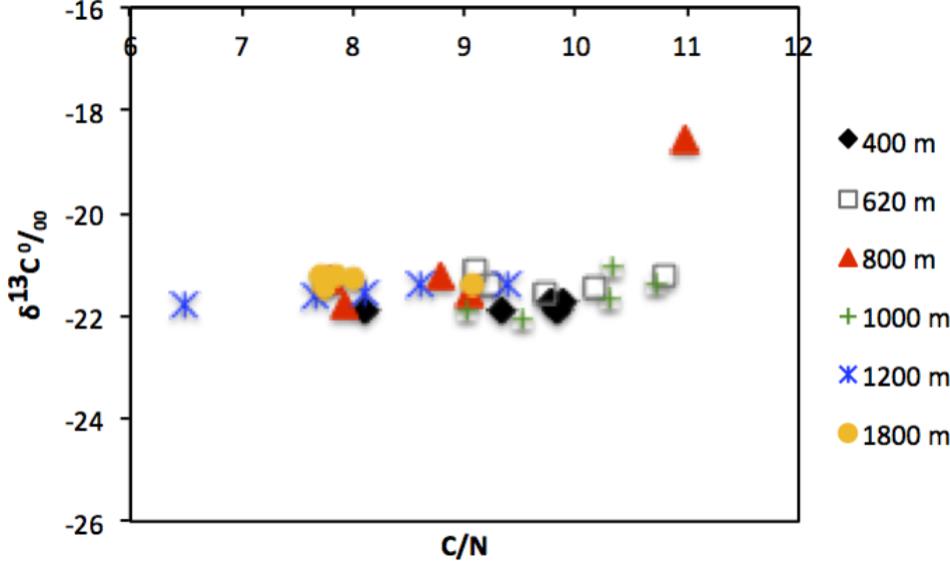
FIGURE 3. Scatter plot showing relationship between C/N ratio and δ13Corg measured in surface sediments (0–10 cm) of the study sites off Costa Rica.
Several correlations between environmental variables were significant (Supplementary Table S2), but most prominent were the significant relations between DO, bottom-water temperature, and chl a. Bottom temperature was inversely related to DO (r = −1.000), and positively to chl a (r = 1.000). In general, variables related to food quantity, freshness, and availability correlated strongly with each other. As a result, DO correlated significantly, negatively with TOC, TN, TOC/TN, Phaeo, CPE, chl a/TOC and positively with chl a/Phaeo.
Meiofaunal Density, Composition, and Diversity
Density
Mean total meiofaunal densities (0–10 cm depth) were highest (3685 ± 1 SE 995 ind. 10 cm−2) at the OMZ core site (400 m), decreased dramatically toward the 620 m site and the lower OMZ boundary site (800 m) (250 ± 1 SE 100 ind. 10 cm−2), and increased slightly at the deeper, more oxygenated sites (but still < 500 ind. 10 cm−2; Figure 4). Nematodes were dominant at all sites with an overall mean relative abundance of 79.7%; with a maximum at the OMZ core (99.9%), followed by copepods (13.0%), nauplii (4.8%), and polychaetes (3.0%) (Table 2).
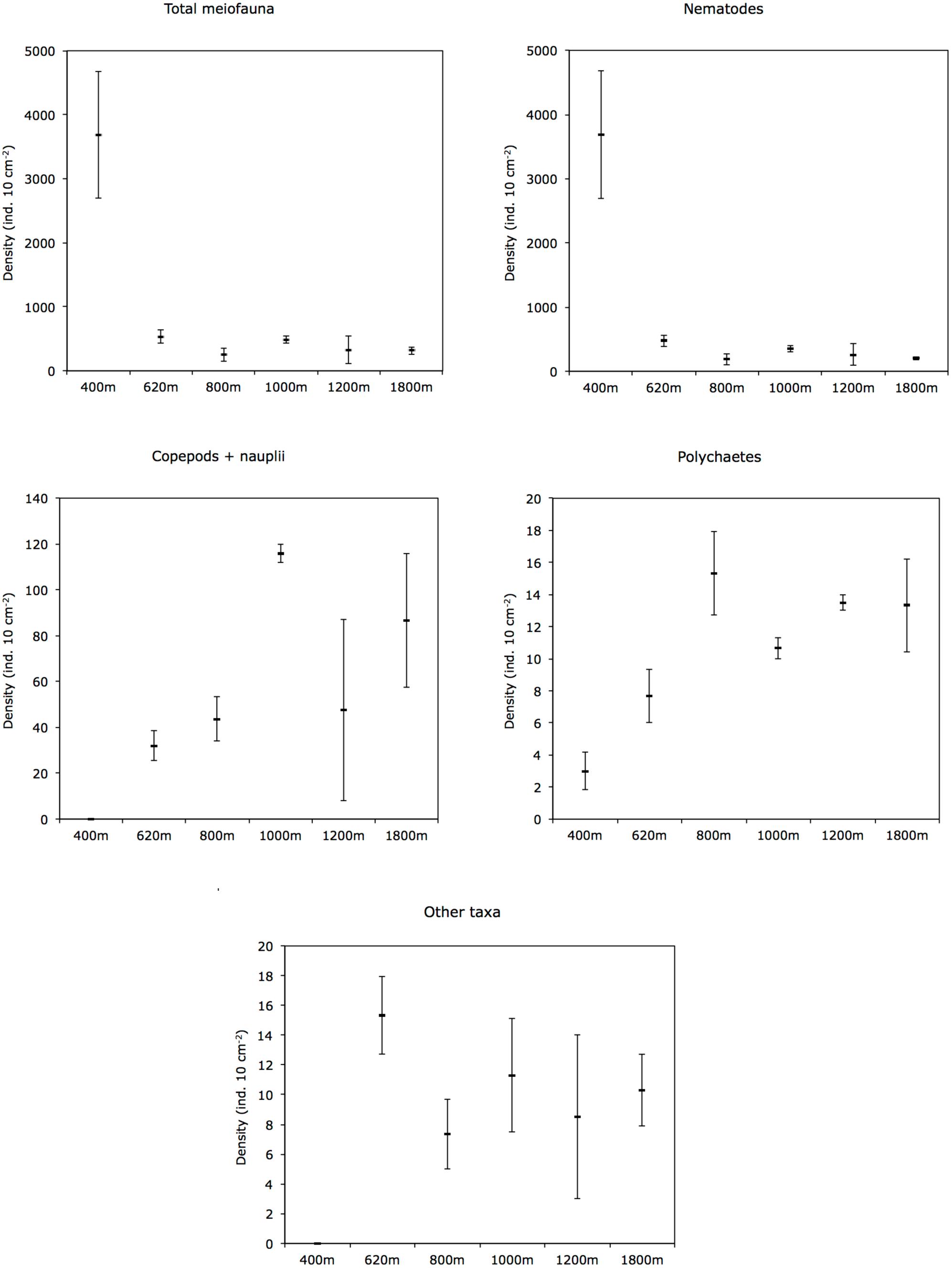
FIGURE 4. Cross-margin distribution of total meiofauna, nematodes, copepods + nauplii, polychaetes, and other taxa abundances (mean ± 1 SE) in slope sediments (0–10 cm) off Costa Rica. Other taxa include kinorhynchs, ostracods, turbellarians, bivalves, gastrotrichs, oligochaetes, isopods, tanaids, priapulids, aplacophorans, amphipods, cnidarians, gastropods, and cumaceans.
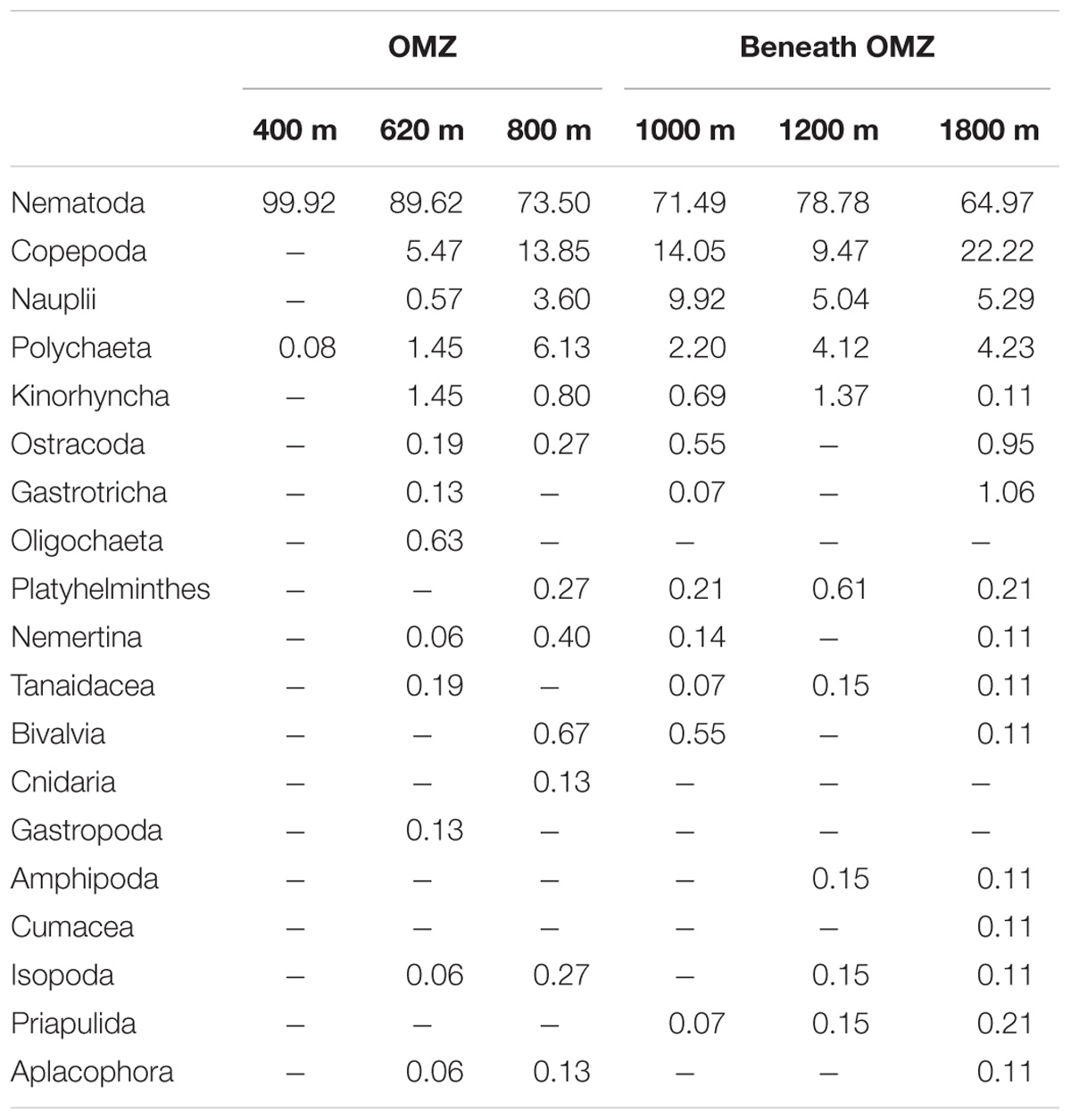
TABLE 2. Relative abundance (%) of meiofaunal taxa at six sites sampled along the slope off Costa Rica.
Other taxa present included kinorhynchs, ostracods, turbellarians, bivalves, gastrotrichs, nemerteans, oligochaetes, isopods, tanaids, priapulids, aplacophorans, amphipods, cnidarians, gastropods, and cumaceans (listed in decreasing order of relative abundance) and together represented ∼2.3% of total abundance (Figure 5 and Table 2). The PERMANOVA test on meiofauna density showed significant effects on the level of water depth (p = 0.002) and sediment layers (p = 0.001), with significant interaction effects (Supplementary Table S3). Pairwise comparisons showed that water depth differences were mainly caused by the contrasting density differences between 400 m and the other stations, and this mainly for the top sediment layers (0–1, 1–2, 2–3 cm). Sediment depth layer differences were significant in all pairwise comparisons, but when separated per station, it became clear that differences between the surface 0–1 cm layer and the deeper layers were more prominent outside the OMZ influence (Supplementary Table S3). PERMDISP results indicated that the 400 m station exhibited significant dispersion heterogeneity compared to the other stations, which is not surprising given the large density differences and the fact that density is a univariate measure.
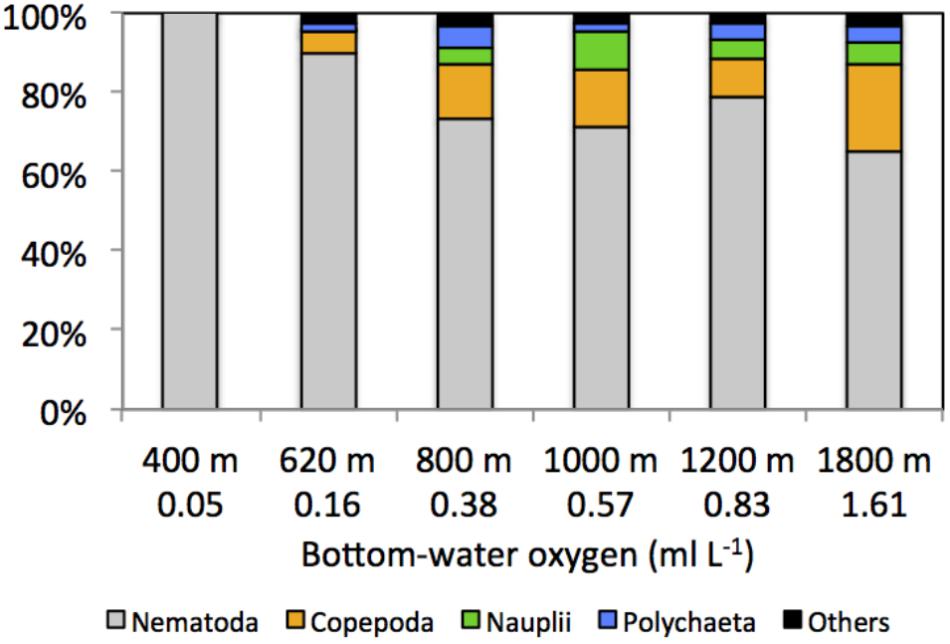
FIGURE 5. Composition (%) of main meiofaunal taxa along the Costa Rica margin as a function of depth gradient and bottom-water oxygen concentration. Others include kinorhynchs, ostracods, turbellarians, bivalves, gastrotrichs, nemerteans, oligochaetes, isopods, tanaids, priapulids, aplacophorans, amphipods, cnidarians, gastropods, and cumaceans.
Composition
The PERMANOVA result (not considering sediment layers separately; i.e., based on 0–10 cm) indicated significant differences between mainly the 400 and 620 m OMZ stations, and the deeper sites (Table 3). These water depth distinctions are clearly visible on the accompanying nMDS (Figure 6). Based on integrated 0–10 cm community data, similarity (SIMPER) within sites ranged from 53% at the 1200-m site to 87% in the OMZ core. Assemblage dissimilarities were highest between the OMZ-core site and all other sites (53.5–68.8%, SIMPER) (Table 3), driven by the high abundance of nematodes at the OMZ core. The lowest dissimilarity occurred between the 1000 and 1800-m sites (23.1%, SIMPER) and the 800 and 1800-m sites (26.7%) (Table 3), driven by an increased relative abundance contribution of copepods, nauplii, and other taxa such as gastrotrichs, kinorhynchs, and ostracods. The PERMANOVA test on community data is remarkably similar to that performed on total density, with significant differences between water depths (p = 0.003), sediment layers (p = 0.001), and their interaction (p = 0.001) (Supplementary Table S4). None of the PERMDISP analyses came out significant. Pairwise comparisons indicated that water depth differences were caused by contrasting meiofauna composition between the OMZ core 400 m station and the other sites and mainly for the top 3 cm of the sediment. Sediment layer differences occurred between all layers, but these differences changed depending on the water depth considered, with for instance significant composition differences between 0–1 and 1–2 cm at 1800 m, but not at 400 m (Supplementary Table S4).
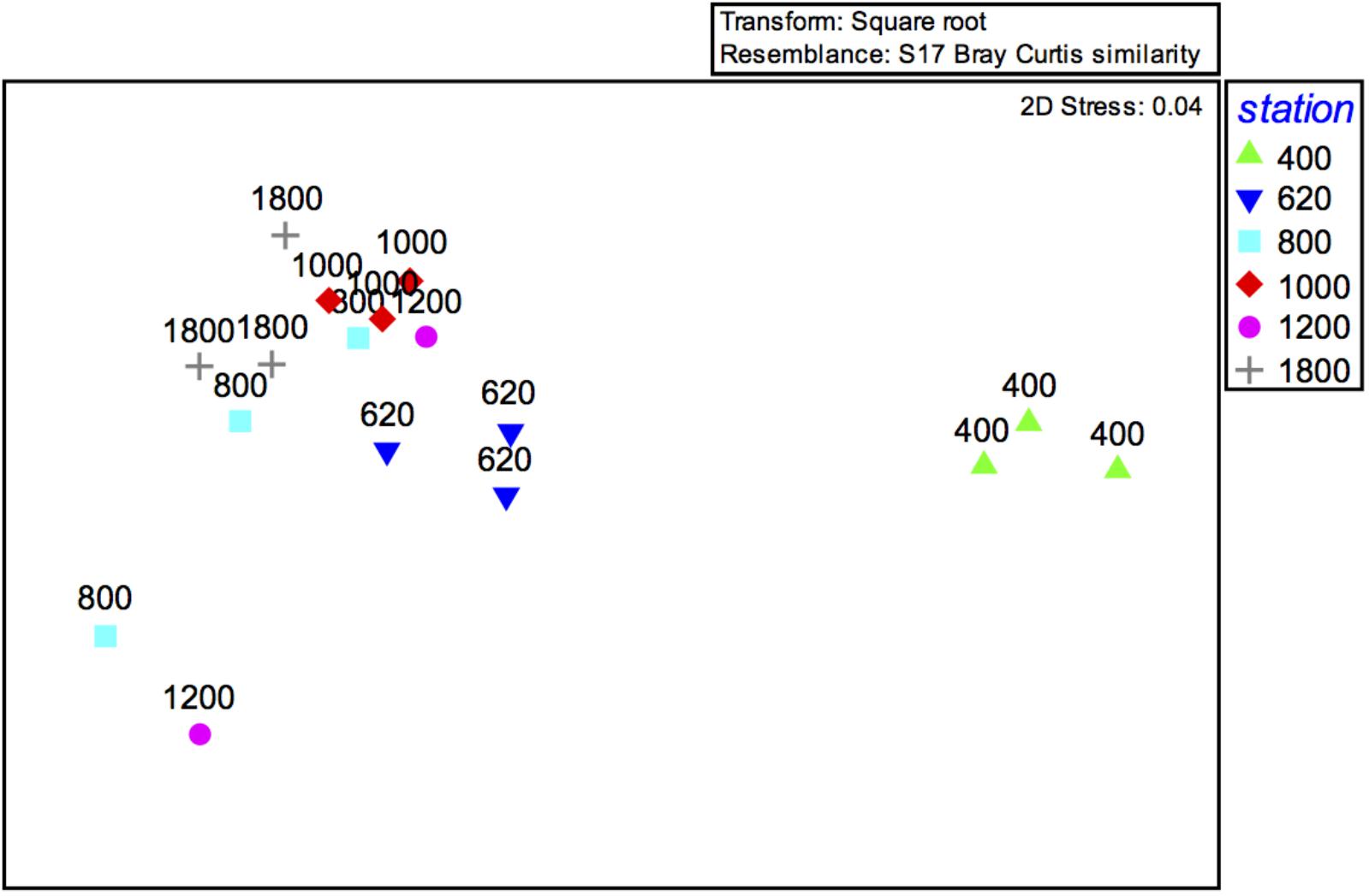
FIGURE 6. MDS ordination plot of meiofaunal community structure (0–10 cm) along the depth transect off Costa Rica.
Diversity
Taxon richness, H′ diversity, and J′ evenness were highest and rank 1 dominance (an inverse indicator of assemblage evenness) was lowest at the deepest site (1800 m), while the opposite was observed at the OMZ core site (400 m) (Table 4). In addition, comparative rarefaction curves of OMZ sites pooled together (i.e., 400, 620, and 800 m) vs. non-OMZ sites (i.e., 1000, 1200, and 1800 m), clearly reflecting the higher community diversity at the oxygenated sites (Figure 7 and Table 4). All diversity indices differed significantly between stations, with pairwise comparisons showing that the 400- and 620-m stations were the contrasting sites, setting them apart from the other stations (Supplementary Table S5).
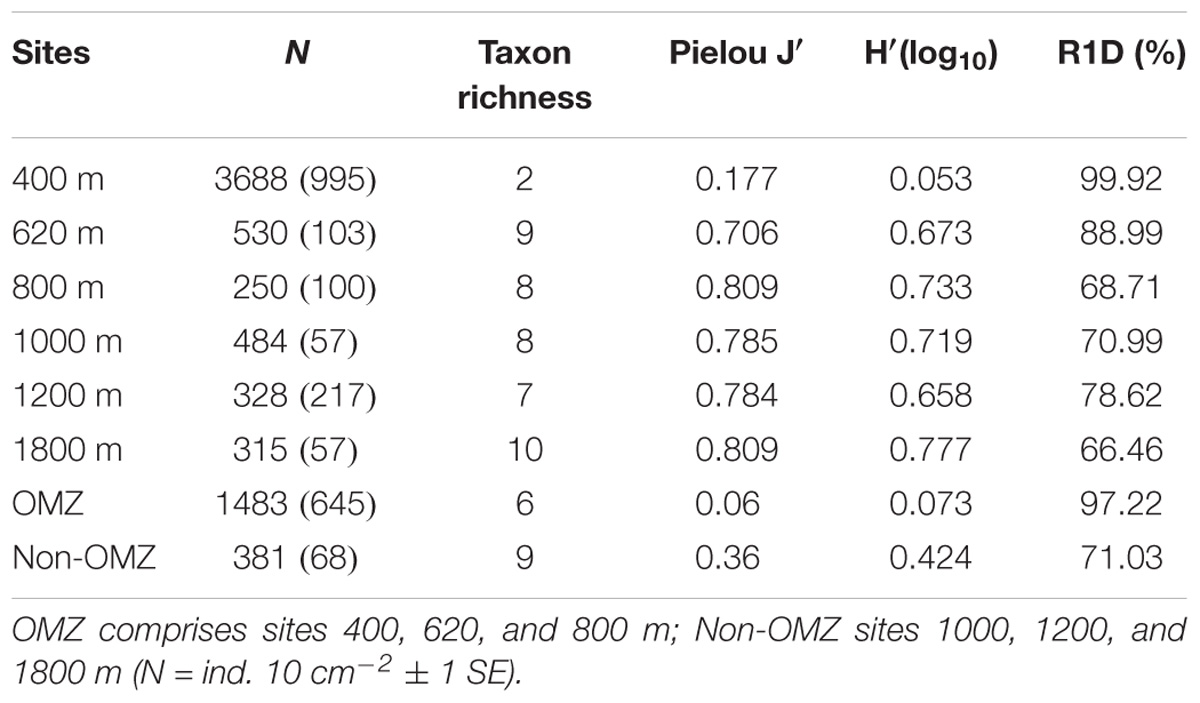
TABLE 4. Mean density, taxon richness, Pielou’s evenness (J′), Shannon–Wiener (H′) and rank 1 dominance (R1D) for meiofauna (0–10 cm).
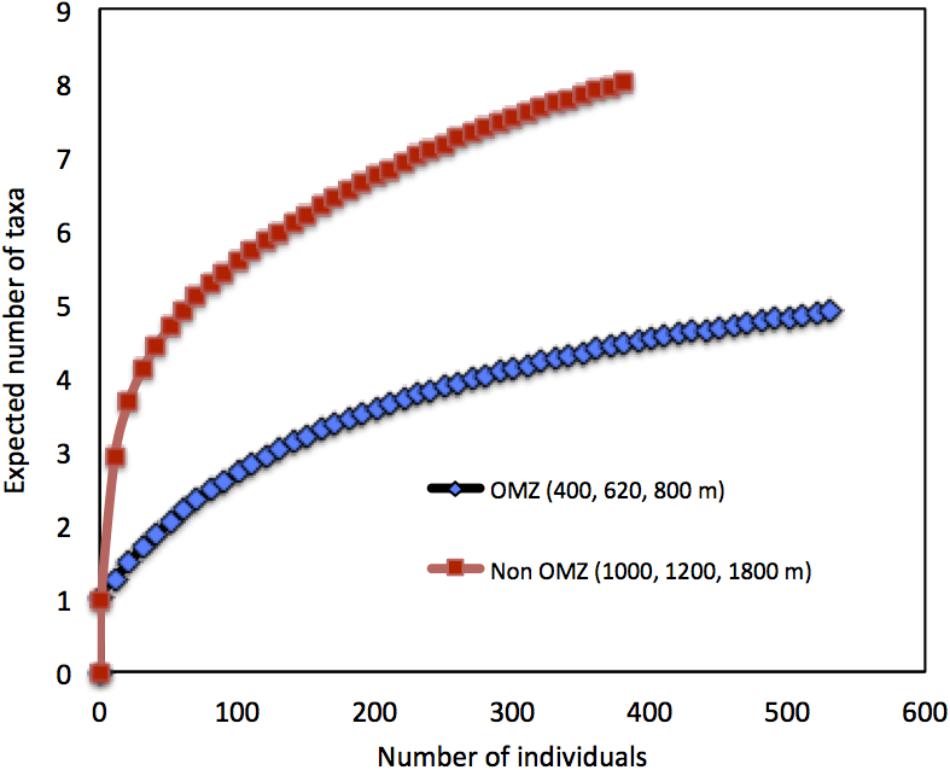
FIGURE 7. Rarefaction curves depicting diversity of meiofaunal assemblages at sites within the OMZ (<0.5 ml L−1; 400, 620, and 800 m) vs. sites beneath the OMZ (>0.5 ml L−1, 1000, 1200, and 1800 m) off Costa Rica.
Vertical Distribution of Meiofauna
In the OMZ core (400 m), most meiofauna was concentrated in the upper 2 cm, almost divided equally between the 0–1 and 1–2-cm horizons, while densities at the adjacent OMZ site (620 m) peaked in the 1–2 cm layer, but with relatively high numbers at the surface (0–1 cm) (Figure 8). At the deeper, more oxygenated sites, however, meiofauna are mostly concentrated in the top 1 cm, declining consistently with depth in the sediment (Figure 8). In strongly oxygen-depleted sites (∼0.05–0.16 ml L−1) about 20–40% of meiofauna abundance is concentrated in the uppermost 1 cm, while at deeper slope sites (>800–1800 m water depth), 55–70% of meiofauna were found in the top 1 cm sediment layer (Figure 9A). The relative importance of copepods and nauplii increased (and nematodes decreased) toward the deeper (620 to 1800 m), more oxygenated sites (Figures 4, 9B). The PERMANOVA results on density and community composition reported above support these observations statistically, with sediment layer differences being more evident at the OMZ core and adjacent sites (620 and 800 m) where abundances do not significantly change in the upper fraction (∼0–3 cm). In contrast, the more oxygenated sites (1000 and 1800 m) show significant differences between the 0–1 cm fraction and deeper layers, reflecting the higher abundances concentrated at the sediment surface (Supplementary Tables S3, S4).
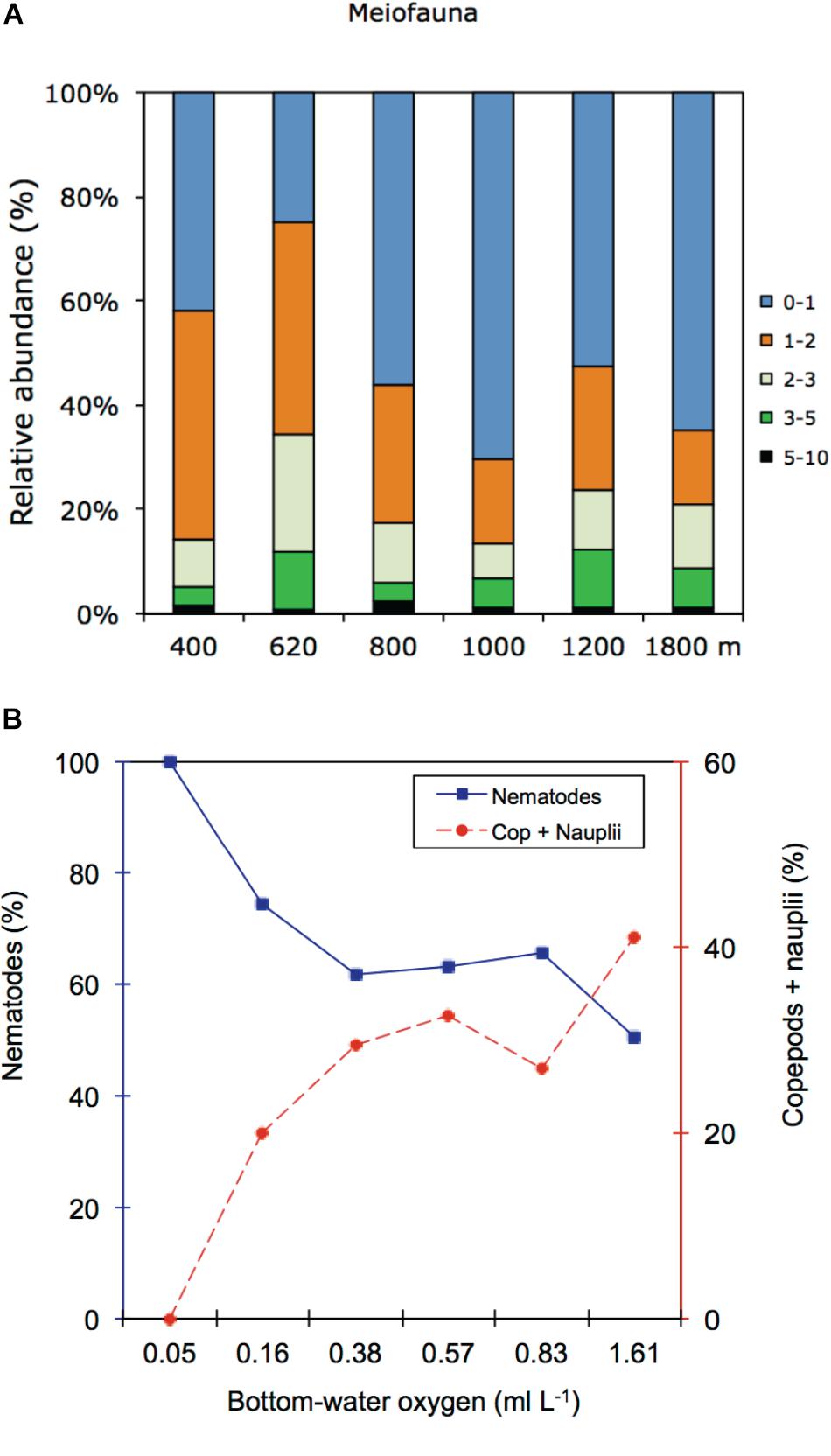
FIGURE 9. (A) Relative abundance (%) of meiofauna per sediment layer at each study site. (B) Relative abundances (%) distribution of nematode and copepods + nauplii for the upper 1 cm sediment along the bottom-water oxygen gradient.
Meiofauna in Relation to the Environment
Spearman’s correlation coefficients are shown in Supplementary Table S6. Copepod and nauplii densities were positively correlated with DO (p < 0.05) and freshness of the OM (chl a/phaeo) (p < 0.05) (Figure 9B). At the same time, their densities were negatively correlated with chl a and phaeo (p < 0.05) and bioavailability of the OM (chl a/TOC) (p < 0.001). Polychaetes and other taxa showed no significant relationships with the measured environmental variables. Nematodes on the other hand correlated significantly and positively with sediment TOC (p < 0.05).
The forward stepwise selection procedure identified a suite of environmental variables that in combination offer the best explanatory value for meiofauna community structure (cf. RDA ordination model visualized for meiofauna community in Figure 10). Those environmental variables that were selected are shown in Supplementary Table S7 and include chl a/TOC, phaeopigments, chl a, water depth, and DO. The RDA analysis yielded four axes that explained 98.02% of the variance between meiofaunal community structure and environmental variables, which were significant (p = 0.002) for all canonical axes. The direction of vectors indicates that OM related descriptors (chl a, phaeo, chl a/TOC) increased along the first axis, and are associated with presence of nematodes, which are markedly dominant at the OMZ core site. Copepods, nauplii as well as other crustaceans such as amphipods, ostracods and cumaceans, mostly occur in the uppermost sediment layer, where they are likely associated with increased DO availability. Other taxa such as kinorhynchs, tanaids, and oligochaetes do not appear to be associated with these environmental variables over the range tested (Figure 10).
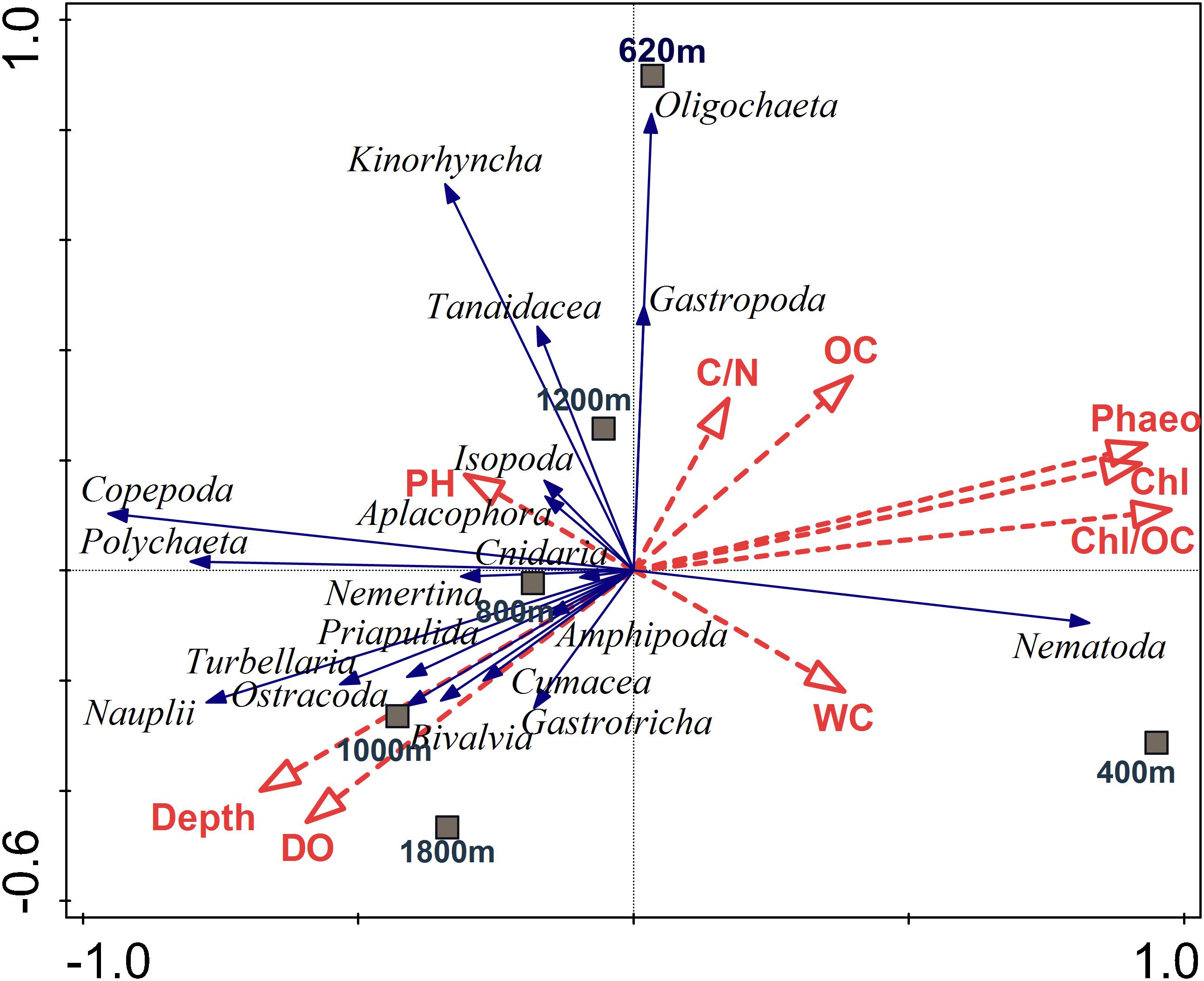
FIGURE 10. Redundancy analysis (RDA) depicting the meiofauna community on the Costa Rica margin in relation to environmental variables and depth. Dashed red arrows represent the environmental explanatory variables (vectors): OC (total organic carbon), C/N (total organic carbon/total nitrogen ratio), Chl (chlorophyll a), Phaeo (phaeopigments), Chl/OC (chlorophyll a/total organic carbon ratio), WC (water content), BO (bottom water oxygen), PH (pH bottom-water pH). Solid blue arrows are the meiofaunal taxa (responses). Arrows pointing in the same direction are correlated, and longer arrows indicate increasing values. The first two axes explained 84.8% of the total variance of taxa-environment variance. The relationships were highly significant (p = 0.002; 999 Monte Carlo permutations).
Discussion
Environmental Properties
Sediment TOC levels were not significantly different within the different OMZ sites (400-m core, 620-m adjacent site, and 800-m lower boundary site) but they were higher in the OMZ compared to those at the deeper, more oxygenated sites (Supplementary Table S1). The strong inverse correlation between TOC (and other food-related variables) and DO follows a similar trend to those observed previously in other OMZs, such as off Peru (Neira et al., 2001b), off central Chile both shelf (Sellanes and Neira, 2006) and slope (Veit-Köhler et al., 2009; Neira et al., 2013), and in the Arabian Sea (Gooday et al., 2000; Levin et al., 2000). This strong correlation suggests that DO may play a primary role in controlling TOC distribution on the Costa Rica margin, potentially through regulation of biological activity resulting in variable consumption and degradation of TOC. Also, very high levels of sinking OM may lead to rapid consumption and aerobic degradation (Stukel et al., 2016), quickly consuming the available oxygen in low-ventilation waters and leaving relatively large amounts of OM in the sediment under hypoxic conditions where anaerobic degradation by microbial organisms takes over (McKew et al., 2013). In such a scenario other factors such as bioturbation, burial, sediment sorption, as well as sediment transport, sedimentation rates and local topography may additionally influence margin TOC distributions (Cowie and Levin, 2009; Cowie et al., 2009).
The TOC levels (∼2–2.5%DW) of the OMZ sediments were comparable to those reported for the Golfo Dulce (Costa Rica), a nearshore oxygen-depleted basin (∼100–200 m depth) located southeast of the present study region (Nichols-Driscoll, 1976; Thamdrup et al., 1996) and Panama Basin (3900 m) (Aller et al., 1998), but lower than in other eastern Pacific OMZs such as off Chile (∼122–309 m) (Neira et al., 2001c, 2013; Veit-Köhler et al., 2009). However, at the Costa Rica margin, C/N (molar) ratios were much lower (7.7–9.7; Table 1) and similar to more oceanic waters with OM of phytoplanktonic origin, compared to the nearshore Golfo Dulce (6.1–12.9), where terrestrial OM input with enhanced content of N-poor detritus occurs (Thamdrup et al., 1996). Higher C/N ratios can be indicative of preferential use of nitrogen during the remineralization of OM of phytoplanktonic origin (Sánchez and Carriquiry, 2007). The relatively high chl a/phaeo values at deeper sites suggest that material deposited is more fresh relative to that of OMZ sites (400 and 620 m). Furthermore, C/N ratios and carbon isotopic composition shows little spatial variability between sites (Figure 3). This suggests that little degradation of OM or at least only partial degradation occurs in the overlying water column (Cowie et al., 2009), and that the deposited OM is of marine origin, with δ13C signatures typically between −18 and −22o/oo and C/N ratios of 6–11. Spatial variability of C/N ratios among sites reflects the diagenetic state, reactivity and the quality of the particulate OM as well as bioturbation and bio-irrigation by fauna (Cowie, 2005; Cowie et al., 2009; Lessin et al., 2018).
Meiofaunal Density and Diversity
Metazoan densities along the outer shelf-slope margin generally decrease with water depth and surface primary productivity (Rex et al., 2006). Table 5 summarizes information on mean total meiofauna abundances, number of taxa, and relative abundance of nematodes for the Costa Rica margin and compares it with studies conducted in other regions along the eastern Pacific. The mean densities registered at the OMZ core site (400 m) off Costa Rica (∼3688 ind. 10 cm−2) are one the highest compared to other OMZ core sites (independent of water depth) along the eastern Pacific, such as off Concepción (< 2000 ind. 10 cm−2) (Neira et al., 2001c, 2013; Sellanes et al., 2003; Sellanes and Neira, 2006), off Callao, Peru (∼1500 ind. 10 cm−2), and almost double that reported for an OMZ off Antofagasta (Veit-Köhler et al., 2009). The drastic decline in total abundance of meiofauna from the OMZ core toward the adjacent site (620 m) with increasing oxygen, but still within the OMZ, was a pattern similar to that observed at the Peru margin (Neira et al., 2001b).
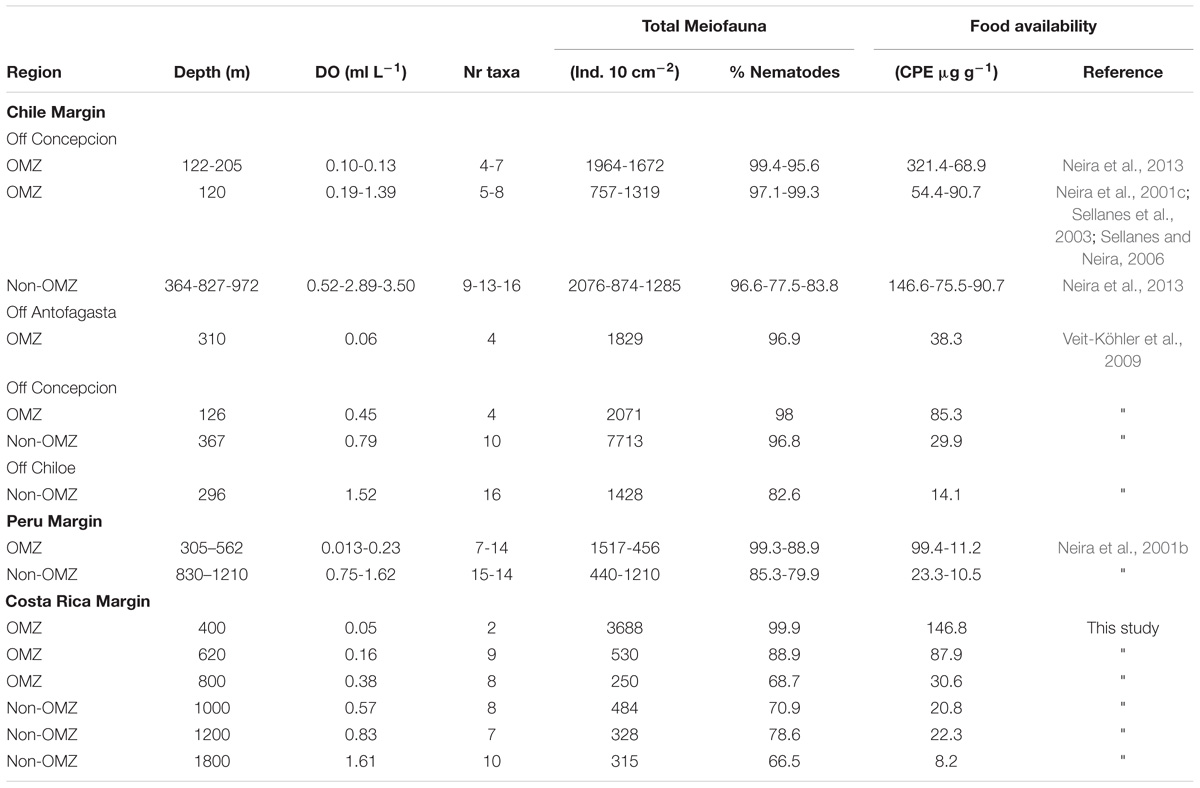
TABLE 5. Comparison of meiofaunal densities, number of meiofaunal taxa present, relative abundance of nematodes in and outside the OMZ along the eastern Pacific margin (range depths: 120–1800 m) in relation to bottom-water DO and CPE as food availability.
Marine benthic diversity usually increases with increasing water depth along the upper continental slope (Menot et al., 2010; Rex and Etter, 2010). In this study, a total of 19 meiofaunal taxa were found, but at the OMZ core site, only two taxa were collected (Nematoda and Polychaeta), with nematodes completely dominating meiofaunal composition (99.9% at the 400 m site, Table 2). This low number was even lower than reported for other Pacific OMZ sites such as off Antofagasta (4 taxa, Veit-Köhler et al., 2009), central Chile (4–8 taxa, Neira et al., 2001c; Sellanes and Neira, 2006) and, off Peru (7 taxa, Neira et al., 2001b) (Table 5). Differing diversity between OMZ vs. non-OMZ sites was also clearly reflected in diversity indices (Table 4 and Supplementary Table S5). Studies off Peru suggest that beyond the OMZ habitat, sediment heterogeneity has more relevance in shaping metazoan meiofaunal diversity compared to within the OMZ core, where oxygen is the main limiting factor (Neira et al., 2001b; Gooday et al., 2010; Sellanes et al., 2010). Oxygen availability in sediments plays a key role in controlling meiofaunal diversity (Gooday et al., 2010; Zeppilli et al., 2015). Reduced conditions may negatively impact meiofaunal diversity (e.g., Neira and Rackemann, 1996), and nematode community structure and trophic diversity (Ingels et al., 2011; Neira et al., 2013). Interestingly, this is illustrated by the changing diversity of Foraminifera from a site off Pakistan, showing consistently higher diversity during the inter-monsoon season (when the study site was outside the OMZ) compared to during the monsoon season (when the site was within the OMZ) (Larkin and Gooday, 2009; Gooday et al., 2010). Similarly, off Pakistan macrofaunal (>300 μm) and megafaunal diversity was strongly reduced where DO was lowest, but diversity recovered rapidly at slightly higher oxygen levels (Gooday et al., 2009; Levin et al., 2009). These observations are likely attributable to an ‘edge effect’ where a release from oxygen stress combined with an abundant food supply occurs (Murty et al., 2009; Gooday et al., 2010). Veit-Köhler et al. (2009) reported 10 taxa at an OMZ site off Chile that had slightly elevated oxygen levels (0.79 ml L−1) compared to 4 taxa from the lower-oxygen sites, suggesting comparable processes may be taking place in that area. For the Costa Rica margin, this is reflected by the increase in the number of meiofauna taxa from 2 (400 m) to 9 (620 m) within a gradient of DO from 0.05 to 0.16 ml L−1 (Table 5), and likely related to the relatively low C/N values and higher food quality (resulting from fresher OM, i.e., higher chl a/phaeo) toward the more oxygenated sites (Figure 2 and Table 1).
Factors Influencing Meiofauna Community Structure
Meiofaunal community distribution is influenced by numerous factors such as physical sediment properties (Giere, 2009), interactions with macrofauna (Pfannkuche and Thiel, 1987; Lambshead et al., 1994; Van Colen et al., 2009) or microbiota (Danovaro et al., 1995, 2000), habitat heterogeneity (Van Gaever et al., 2009), and oxygen availability (Levin et al., 2002; Sellanes and Neira, 2006). The potential role of oxygen is reflected in the RDA plot (Figure 10) where oxygen is positively correlated with a number of taxa. In fact, there are distinctly divergent meiofaunal responses across OMZ-influenced settings compared to deeper, more oxygenated ones (Figures 6, 7). The most oxygen-depleted sites were characterized by lower meiofaunal taxon richness, lower diversity (H′) and lower taxonomic evenness (J′), but higher rank 1 dominance (R1D) (Table 4). The more similar evenness (J′) and diversity (H′) exhibited at deeper, better-oxygenated sites suggests a more equitable distribution of meiofaunal assemblages relative to the OMZ core site (400 m) (Figure 6 and Table 4). Nematode densities at our Costa Rica sites were enhanced at low-oxygen sites, consistent with other OMZs studies (e.g., Levin et al., 1991; Cook et al., 2000; Neira et al., 2001b,c, 2013; Sellanes and Neira, 2006). The very high densities and total numerical dominance of nematodes at the site with lowest oxygen concentration, and the proportional decline of nematode abundance with increasing oxygen (Figure 9B) supports previous observations of a potential positive effect of low-oxygen levels on nematode abundance (e.g., Neira et al., 2001b), including the indirect effect of decreasing hypoxia-intolerant predators and competitors (Neira et al., 2001b; Levin et al., 2002; Levin, 2003). Time series data at a shelf site (98 m) off Callao, Peru has shown fluctuations in nematode density and a community 100% dominated by nematodes. Such extreme dominance, termed a “nematode state” was suggested to take place during extreme La Niña conditions, when bottom-water oxygen concentrations are at their lowest and macrofaunal densities are strongly reduced (Gutiérrez et al., 2008). This is likely reflected by the consistent decline in abundance of meiofaunal-size (and larger) polychaetes toward the most oxygen-depleted sites (Figure 4). Also crustaceans, such as copepods and nauplii, isopods, amphipods and ostracods are sensitive to low-oxygen conditions (Josefson and Widbom, 1988; Wetzel et al., 2001; De Troch et al., 2013) and compete with nematodes for space and resources, so their absolute and relative decline with decreasing oxygen levels likely allows nematodes to dominate the sediment system. Copepods are active swimmers and their metabolism requires more oxygen than other meiofaunal groups (Moodley et al., 1997; Modig and Olafsson, 1998; Giere, 2009). Summer hypoxic conditions (1985/86) on the Louisiana shelf resulted in the loss of copepods for a month, with moderate loss of nematode and kinorhynch densities. After the habitat returned to normal oxygen conditions, the copepods recovered slowly (Murrell and Fleeger, 1989). Oxygen depletion may lead to a feeding stop and unsuccessful reproduction in adult copepods (Veit-Köhler et al., 2009, 2018; De Troch et al., 2013) and hence absence of nauplii and adult copepods at the OMZ core. Recovery, speed and extent of colonization of meiofauna after hypoxic events are variable. For instance, the high mobility of copepods and ostracods promotes a rapid recolonization of azoic sediments, compared to nematodes which require more time to cover similar distances (Chandler and Fleeger, 1983). Experiments in the Wadden Sea reduced sediments (“black spots”) showed that meiofauna recolonized sediments only after redevelopment of an oxidized sediment layer (Neira and Rackemann, 1996). Van Colen et al. (2009) found strong changes in nematode community composition after exposing them to experimentally-induced hypoxia, but this did not result in complete nematode mortality. For instance, under El Niño conditions, which cause enhanced bottom-water oxygen concentrations, there was an increased representation of copepods and nauplii by one order of magnitude at sites influenced by the OMZ (Neira et al., 2001c). We suspect that the most important driver of meiofaunal communities is bottom-water oxygen, but that the co-occurrence of low oxygen and chl a/phaeo, and high chl a, phaeo, and chl a/TOC (and hence food quality and availability) lie behind some of the causality of the correlations.
The quality of food resources, in addition to oxygen, can play an important role in structuring faunal communities. For meiofauna, the role of food availability has been highlighted in many studies as one of the most important factors structuring communities and spatial biodiversity patterns (Danovaro et al., 1995; Neira et al., 2001b; Giere, 2009; Pusceddu et al., 2009). The importance of food quantity and availability on nematode abundance and diversity in OMZs has also been suggested by Cook et al. (2000) for the Arabian Sea. Food availability is a regulating factor along the vertical sediment profile (Ingels et al., 2009, 2011; Ingels and Vanreusel, 2013). At the Costa Rica margin, meiofauna densities declined with increasing depth in the sediment, a recurrent gradient observed in marine sediments (Levin et al., 2002; Soltwedel et al., 2005). However, at the lowest oxygen sites of Costa Rica (400 and 620 m), meiofauna, dominated by nematodes, were concentrated in subsurface sediments (∼41–45% in the 1–2 cm layer); whereas toward deeper sites, the surface 1-cm layer harbors most of the meiobenthos (Figure 9A). The vertical distribution of meiofauna was similar to that observed at the OMZ off Callao (transect 305–1210 m, Neira et al., 2001b), where densities declined consistently with depth in the sediment only at the oxygenated sites (Figure 8). The high meiofaunal densities as well as the high dominance of nematodes at the OMZ sites, and their high subsurface densities, are most likely attributable to the extensive availability of food, and relatively fresh phytodetritus (Figure 2) even in the deeper sediment layers (e.g., CPE ranging from 147 μg g−1 at 0–1 cm to 88 μg g−1 at 5–10 cm depth at site 400 m), as well as the relative tolerance of nematodes to low-oxygen levels (Neira et al., 2001a, 2005; Neira and Decraemer, 2009; Sajan et al., 2010), and reduced competition for food by exclusion of the more oxygen-sensitive macro- and meiofauna (Neira et al., 2001b; Levin et al., 2002; Sajan et al., 2010; Jeffreys et al., 2012). Macrofaunal activity may also influence vertical meiofauna patterns in the sediment through bioturbation. For instance, tuna crab (Pleuroncodes sp.), common on the Central American margin (Pineda et al., 2016), can sift out the food material present in the substrate and extract small animals such as nematodes, and other invertebrates (Boyd, 1962, 1967; Lovrich and Thiel, 2011). During Alvin dives in 2017, large masses of galatheid crabs were observed apparently feeding on the sediment bottom of Quepos slide, an active seep area (bacterial mats) influenced by the OMZ and nearby our 400 m site (L. A. Levin personal observation). Galatheid crabs such as Pleuroncodes spp. have often been seen associated with oxygen-depleted waters (Sellanes et al., 2007; Lovrich and Thiel, 2011; Gallardo et al., 2017) and can form dense aggregations swarming the seafloor (Thiel and Lovrich, 2011; Pineda et al., 2016). Bioturbating macrofauna, mainly annelids tolerant to hypoxic conditions found at OMZs, including at very low oxygen sites (e.g., Levin et al., 2000, 2002; Smith et al., 2000; Sellanes et al., 2007), may induce mixing downward, advecting “fresh” and nutritional material deep into the sediment (Levin et al., 1997; Gutiérrez et al., 2000), which, in turn, may support higher nematode survival rates in subsurface and deeper sediments (Braeckman et al., 2010).
Conclusion
To our knowledge, this is the first quantitative study of OMZ influence on meiofauna conducted along the Costa Rica margin. Results indicate that lowest oxygen levels along with highest CPE yielded the highest meiofaunal abundances and lowest diversity, whereas the inverse occurred under highest oxygen and lowest CPE and TOC conditions (even though food quality was higher as deduced from lower C/N and higher chl a/phaeo ratios).
High abundance of nematodes in bathyal sediments is enhanced in low oxygen settings where food is plentiful, although not necessarily fresh. In addition, low-oxygen conditions may have an additional indirect positive effect on nematode abundances by reducing potential predators and competitors. Low-oxygen conditions at the OMZ core site seem unfavorable for copepods, nauplii, and other meiobenthos. Increasing meiofaunal diversity and presence of animals such as copepods and nauplii downslope – mostly concentrated in the surface sediment – are most likely promoted by enhanced bottom-water oxygen and freshness of available food outside the OMZ. The abrupt transition in meiofaunal abundance and diversity from the OMZ core (400 m) to the adjacent within OMZ site (620 m) and deeper sites suggests a strong response to oxygen thresholds (Levin et al., 2009) interacting with OM availability and quality. The overall presence and dominance of nematodes across the vertical profile of the sediment in association with gradients in food quantity, quality, and oxygen, even in the deeper sediment layers, reflect the well-developed nutritional selectivity, wide range of diets, and different oxygen and food requirements attributed to meiofauna (Giere, 2009; Neira and Decraemer, 2009; Ingels et al., 2011; Neira et al., 2013; Schratzberger and Ingels, 2017).
More local and regional information on meiofaunal community structure and biodiversity, as well as understanding their responses to current environmental conditions will contribute to elucidating the role of food sources and oxygen and hypoxia thresholds (Vaquer-Sunyer and Duarte, 2008, 2011; Levin et al., 2009) in structuring meiobenthic fauna and will help to make predictions about future responses to ocean deoxygenation and OMZ expansion.
Author Contributions
LL designed and funded hydrographic measurements and field sampling. The original manuscript was written by CN. GM, CN, and JI conducted statistical analyses, and prepared the figures and tables. All authors contributed equally to the reworking and editing of the original manuscript toward the submitted version.
Funding
The research was supported by the National Science Foundation (NSF; Grant No. OCE 0826254).
Conflict of Interest Statement
The authors declare that the research was conducted in the absence of any commercial or financial relationships that could be construed as a potential conflict of interest.
Acknowledgments
We are grateful to captain, crew and science participants of R/V Atlantis Leg 15-44. We are indebt to P. López, H. Carson, and T. Rathburn, for multicoring and processing samples at sea. We are grateful to C. Smith, C. Puritty, J. Gonzalez, and M. Corrales for assistance in the laboratory and with sorting of meiofauna. We thank R. Lee for conducting elemental and stable isotope analyses. We also thank H. Molina, R. Vargas, J. Vargas, and A. Morales (CIMAR) for facilitating operations at sea and on the ground.
Supplementary Material
The Supplementary Material for this article can be found online at: https://www.frontiersin.org/articles/10.3389/fmars.2018.00448/full#supplementary-material
References
Aller, R. C., Hall, P. O. J., Rude, P. D., and Aller, J. Y. (1998). Biogeochemical heterogeneity and suboxic diagenesis in hemipelagic sediments of the Panama Basin. Deep-Sea Res. I 45, 133–165. doi: 10.1016/S0967-0637(97)00049-6
Anderson, M. J., Gorley, R. N., and Clarke, K. R. (2008). PERMANOVA + for PRIMER: Guide to Software and Statistical Methods. Plymouth: PRIMER-E.
Arntz, W. E., Gallardo,V. A., Gutiérrez, D., Isla, E., Levin, L. A., Mendo, J., et al. (2006). El Niño and similar perturbation effects on the benthos of the Humboldt, California, and benguela current upwelling ecosystems. Adv. Geosci. 6, 243–265. doi: 10.5194/adgeo-6-243-2006
Boyd, C. M. Jr. (1962). The Biology of a Marine Decapod Crustacean, Pleuroncodes Planipes Stimpson, 1860. Ph.D. thesis, University of California, San Diego, San Diego, CA.
Boyd, C. M. (1967). The benthic and pelagic habitats of the red crab, Pleuroncodes planipes. Pacific Sci. 21, 394–403.
Braeckman, U., Van Colen, C., Soetaert, K., Vincx, M., and Vanaverbeke, J. (2010). Contrasting macrofaunal activities differentially affect nematode density and diversity in a shallow subtidal marine sediment. Mar. Ecol. Prog. Ser. 422, 179–191. doi: 10.3354/meps08910
Breitburg, D., Levin, L. A., Oshlies, A., Grégoire, M., Chavez, F. P., Conley, D. J., et al. (2018). Declining oxygen in the global ocean and coastal waters. Science 359:eaam7240. doi: 10.1126/science.aam7240
Chandler, G. T., and Fleeger, J. W. (1983). Meiofaunal colonization of azoic estuarine sediment in Louisiana: mechanisms of dispersal. J. Exp. Mar. Biol. Ecol. 69, 175–188. doi: 10.1016/0022-0981(83)90066-7
Clarke, K. R. (1993). Non-parametric multivariate analyses of changes in community structure. Austr. J. Ecol. 18, 117–143. doi: 10.1111/j.1442-9993.1993.tb00438.x
Cook, A. A., Lambshead, P. J., Hawkins, L. E., Mitchell, N., and Levin, L. A. (2000). Nematode abundance at the oxygen minimum zone in the Arabian Sea. Deep-Sea Res. II 47, 75–85. doi: 10.1016/S0967-0645(99)00097-1
Cortés, J., and Jiménez, C. E. (2003). “Corals and coral reefs of the Pacific of Costa Rica: history, research and status,” in Latin American Coral Reefs, ed. J. Cortes (Amsterdam: Elsevier Science), 361–385. doi: 10.1016/B978-044451388-5/50017-5
Cowie, G. (2005). The biogeochemistry of Arabian Sea surficial sediments: a review of recent studies. Prog. Oceanogr. 65, 260–289. doi: 10.1016/j.pocean.2005.03.003
Cowie, G. L., and Levin, L. A. (2009). Benthic biological and biogeochemical patterns and processes across an oxygen minimum zone (Pakistan margin, NW Arabian Sea). Deep-Sea Res. II 56, 261–270. doi: 10.1016/j.dsr2.2008.10.001
Cowie, G. L., Mowbray, S., Lewis, M., Matheson, H., and McKenzie, R. (2009). Carbon and nitrogen elemental and stable isotopic compositions of surficial sediments from the Pakistan margin of the Arabian Sea. Deep-Sea Res. II 56, 271–282. doi: 10.1016/j.dsr2.2008.05.031
Danovaro, R. (2010). Methods for the Study of Deep-Sea Sediments, their Functioning and Biodiversity. Boca Ration, FL: CRC Press, Taylor & Francis Group, 428.
Danovaro, R., Della Croce, N., Eleftheriou, A., Papadopoulou, N., Smith, C., and Tselepides, A. (1995). Meiofauna of the deep Eastern Mediterranean Sea: distribution and abundance in relation to bacterial biomass, organic matter composition and other environmental factors. Prog. Oceanogr. 36, 329–341. doi: 10.1016/0079-6611(96)00002-X
Danovaro, R., Gambi, C., Dell’Anno, A., Corinaldesi, C., Fraschetti, S., Vanreusel, A., et al. (2008). Exponential decline of deep-sea ecosystem functioning linked to benthic biodiversity loss. Curr. Biol. 18, 1–8. doi: 10.1016/j.cub.2007.11.056
Danovaro, R., Tselepides, A., Otegui, A., and Della Croce, N. (2000). Dynamics of meiofaunal assemblages on the continental shelf and deep-sea sediments of the Cretan Sea (NE Mediterranean): relationships with seasonal changes in food supply. Prog. Oceanogr. 46, 367–400. doi: 10.1016/S0079-6611(00)00026-4
De la Cruz, E., and Vargas, J. A. (1986). Estudio preliminar de la meiofauna de la playa fangosa de Punta Morales, Golfo de Nicoya, Costa Rica. Brenesia 25/26, 89–97.
De la Cruz, E., and Vargas, J. A. (1987). Abundancia y distribucion vertical de la meiofauna en la playa fangosa de Punta Morales, Golfo de Nicoya, Costa Rica. Rev. Biol. Trop. 35, 363–367.
De Troch, M., Roelofs, M., Riedel, B., and Grego, M. (2013). Structural and functional responses of harpacticoid copepods to anoxia in the Northern Adriatic: an experimental approach. Biogeoscience 10, 4259–4272. doi: 10.5194/bg-10-4259-2013
Decho, A. W., and Fleeger, J. W. (1988). Ontogenetic feeding shifts in the meiobenthic harpacticoid copepod Nitocra lacustris. Mar. Biol. 97, 191–197. doi: 10.1007/BF00391302
Füri, E., Hilton, D. R., Tryon, M. D., Brown, K. M., McMurtry, G. M., Brückmann, W., et al. (2010). Carbon release from submarine seeps at the Costa Rica fore arc: implications for the volatile cycle at the Central America convergent margin. Geochem. Geophys. Geosys. 11:Q04S21. doi: 10.1029/2009GC002810
Gallardo, M. A., González López, A. E., Ramos, M., Mujica, A., Muñoz, P., Sellanes, J., et al. (2017). Reproductive patterns in demersal crustaceans from the upper boundary of the OMZ off north-central Chile. Cont. Shelf Res. 141, 26–37. doi: 10.1016/j.csr.2017.04.011
García, R., and Thomsen, L. (2008). Bioavailable organic matter in surface sediments of the Nazaré canyon and adjacent slope (Western Iberian Margin). J. Mar. Syst. 74, 44–59. doi: 10.1016/j.jmarsys.2007.11.004
Giere, O. (2009). Meiobenthology. The Microscopic Motile Fauna of Aquatic Sediments, 2nd Edn. Berlin: Springer.
Gobler, C. J., and Baumann, H. (2016). Hypoxia and acidification in ocean ecosystems: coupled dynamics and effects on marine life. Biol. Lett. 12:201500976. doi: 10.1098/rsbl.2015.0976
Gooday, A. J., Bernhard, J. M., Levin, L. A., and Suhr, S. B. (2000). Foraminifera in the Arabian Sea oxygen minimum zone and other oxygen-deficient settings: taxonomic composition, diversity, and relation to metazoan faunas. Deep-Sea Res. II 47, 25–54. doi: 10.1016/S0967-0645(99)00099-5
Gooday, A. J., Bett, B. J., Escobar, E., Ingole, B., Levin, L. A., Neira, C., et al. (2010). Habitat heterogeneity and its influence on benthic biodiversity in oxygen minimum zones. Mar. Ecol. 31, 125–147. doi: 10.1111/j.1439-0485.2009.00348.x
Gooday, A. J., Levin, L. A., Aranda, da Silva, A., Bett, B. J., Cowi, G. L., et al. (2009). Faunal responses to oxygen gradients on the Pakistan margin: a comparison of foraminiferans, macrofauna and megafauna. Deep-Sea Res. II 56, 488–502. doi: 10.1016/j.dsr2.2008.10.003
Gutiérrez, D., Enríquez, E., Purca, S., Quipúzcoa, L., Marquina, R., Flores, G., et al. (2008). Oxygenation episodes on the continental shelf of central Peru: remote forcing and benthic ecosystem response. Pro. Oceanogr. 79, 177–189. doi: 10.1016/j.pocean.2008.10.025
Gutiérrez, D., Gallardo, V. A., Mayor, S., Neira, C., Vásquez, C., Sellanes, J., et al. (2000). Effects of dissolved oxygen and fresh organic matter on the bioturbation potential of macrofauna in sublittoral bottoms off centra Chile, during the 1997-1998 El Niño. Mar. Ecol. Prog. Ser. 201, 81–99. doi: 10.3354/meps202081
Guzmán, H. H., Obando, V. L., and Cortés, J. (1987). Meiofauna associated with a Pacific coral reef in Costa Rica. Coral Reefs 6, 107–112. doi: 10.1007/BF00301379
Hagerthey, S. E., Louda, J. W., and Mongkronsri, P. (2006). Evaluation of pigment extraction methods and recommended protocol for periphyton chlorophyll a determination and chemotaxonomic assessment. J. Phycol. 42, 1125–1136. doi: 10.1111/j.1529-8817.2006.00257.x
Han, X., Suess, E., Sahling, H., and Wallmann, K. (2004). Fluid venting activity on the Costa Rica margin: new results from authigenic carbonates. Int. J. Earth Sci. 93, 596–611. doi: 10.1007/s00531-004-0402-y
Helly, J. J., and Levin, L. A. (2004). Global distribution of naturally occuring marine hypoxia on continental margins. Deep-Sea Res. I 51, 1159–1168. doi: 10.1016/j.dsr.2004.03.009
Hurlbert, S. H. (1971). The nonconcept of species diversity: a critique and alternative parameters. Ecology 52, 577–586. doi: 10.2307/1934145
Ingels, J., Billett, D. S. M., Kiriakoulakis, K., Wolff, G. A., and Vanreusel, A. (2011). Structural and functional diversity of Nematoda in relation with environmental variables in the Setúbal and Cascais canyons. Deep-Sea Res. II 58, 2354–2368. doi: 10.1016/j.dsr2.2011.04.002
Ingels, J., Kiriakoulakis, K., Wolff, G. A., and Vanreusel, A. (2009). Nematode diversity and its relation to quantity and quality of sedimentary organic matter in the Nazaré Canyon, Western Iberian margin. Deep-Sea Res. I 56, 1521–1539. doi: 10.1016/j.dsr.2009.04.010
Ingels, J., and Vanreusel, A. (2013). The importance of different spatial scales in determining structural and functional characteristics of deep-sea infauna communities. Biogeosciences 10, 4547–4563.
IPCC (2014). Climate Change 2014: Synthesis Report. Contribution of Working Groups I, II and III to the Fifth Assessment report of the Intergovernmental Panel on Climate Change, Core Writing Team, R. K. Pachauri, and L. A. Meyer. Geneva: IPCC, 151.
Jeffreys, R. M., Levin, L. A., Lamont, P. A., Woulds, C., Whitcraft, C. R., Mendoza, G. F., et al. (2012). Living on the edge: single-species dominance at the Pakistan oxygen minimum zone boundary. Mar. Ecol. Prog. Ser. 470, 79–99. doi: 10.4319/lo.2000.45.7.1576
Josefson, A. B., and Widbom, B. (1988). Differential response of benthic macrofauna and meiofauna to hypoxia in the Gullmar Fjord basin. Mar. Biol. 100, 31–40. doi: 10.1007/BF00392952
Kahru, M., Kudela, R. M., Manzano-Sarabia, M., and Mitchell, B. G. (2012). Trends in the surface chlorophyll of the California current: merging data from multiple ocean color satellites. Deep-Sea Res. II 7, 89–98. doi: 10.1016/j.dsr2.2012.04.007
Karstensen, J., Stramma, L., and Visbeck, M. (2008). Oxygen minimum zones in the eastern tropical Atlantic and Pacific oceans. Prog. Oceanogr. 77, 331–350. doi: 10.1016/j.pocean.2007.05.009
Keeling, R. F., Kortzinger,A., and Gruber, N. (2010). Ocean deoxygenation in a warming world. Ann. Rev. Mar. Sci 2, 199–229. doi: 10.1146/annurev.marine.010908
Lambshead, P. J. D., Elce, B. J., Thistle, D., Eckman, J. E., and Barnett, P. R. O. (1994). A comparison of the biodiversity of deep-sea marine nematodes from three stations in the Rockall Trough, Northeast Atlantic, and one station in the San Diego Trough, Northeast Pacific. Biodivers. Lett. 2, 21–33. doi: 10.2307/2999713
Larkin, K. E., and Gooday, A. J. (2009). Foraminiferal faunal responses to monsoon-driven changes in organic matter and oxygen availability at 140 m and 300 m water depth in the NE Arabian Sea. Deep-Sea Res. II 56, 403–421. doi: 10.10.1016/j.dsr2.2008.05.037
León-Morales, R., and Vargas, J. A. (1998). Macrofauna of a tropical fjord-like embayment: golfo dulce, Costa Rica. Rev. Biol. Trop. 46, 81–90.
Lepš, J., and Šmilauer, T. (2003). Multivariate Analysis of Ecological Data Using CANOCO. Cambridge: Cambridge University Press.
Lessin, G., Artioli, Y., Almroth-Rosell, E., Blackford, J. C., Dale, A. W., Glud, R. N., et al. (2018). Modelling marine sediment biogeochemistry: current knowledge gaps, challenges, and some methodological advice for advancement. Front. Mar. Sci. 5:19. doi: 10.3389/fmars.2018.00019
Levin, L., Blair, N., DeMaster, D., Plaia, G., Fornes, W., Martin, C., et al. (1997). Rapid subduction of organic matter by maldanid polychaetes on the North carolina slope. J. Mar. Res. 55, 595–611. doi: 10.1357/0022240973224337
Levin, L., Gutiérrez, D., Rathburn, A., Neira, C., Sellanes, J., Muñoz, P., et al. (2002). Benthic processes on the Peru margin: a transect across the oxygen minimum zone during the 1997-98 El Niño. Prog. Oceanogr. 53, 1–27. doi: 10.1016/S0079-6611(02)00022-8
Levin, L. A. (2003). Oxygen minimum zone benthos: adaptation and community response to hypoxia. Oceanogr. Mar. Biol. Ann. Rev. 41, 1–45.
Levin, L. A. (2018). Manifestation, drivers, and emergence of open ocean deoxygenation. Ann. Rev. Mar. Sci. 10, 229–260. doi: 10.1146/annurev-marine-121916-063359
Levin, L. A., and Breitburg, D. L. (2015). Linking coasts and seas to address ocean deoxygenation. Nat. Clim. Change 5, 401–403. doi: 10.1038/nclimate2595
Levin, L. A., Gage, J. D., Martin, C., and Lamont, P. A. (2000). Macrobenthic community structure within and beneath the oxygen mínimum zone, NW Arabian Sea. Deep-Sea Res. II 47, 189–226. doi: 10.1016/S0967-0645(99)00103-4
Levin, L. A., Huggett, C. L., and Wishner, K. F. (1991). Control of deep-sea benthic community structure by oxygen and organic-matter gradients in the eastern Pacific Ocean. J. Mar. Res. 49, 763–800. doi: 10.1357/002224091784995756
Levin, L. A., Mendoza, G. F., Grupe, B. M., Gonzalez, J. P., Jellison, B., Rouse, G., et al. (2015). Biodiversity on the rocks: macrofauna inhabiting authigenic carbonate at Costa Rica methane seeps. PLoS One 10:e0131080. doi: 10.1371/journal.pone.0131080
Levin, L. A., Orphan, V. J., Rouse, G. W., Rathburn, A. E., Ussler, W. III, Cook, G. S., et al. (2012). A hydrothermal seep on the Costa Rica margin: middle ground in a continuum of reducing ecosystems. Proc. R. Soc. B-Biol. Sci. 279, 2580–2588. doi: 10.1098/rspb.2012.0205
Levin, L. A., Sibuet, M., Gooday, A. J., Smith, C. R., and Vanreusel, A. (2010). The roles of habitat heterogeneity in generating and maintaining biodiversity on continental margins: an introduction. Mar. Ecol. 31, 1–5. doi: 10.1111/j.1439-0485.2009.00358.x
Levin, L. A., Whitcraft, C. R., Mendoza, G. F., Gonzalez, J. P., and Cowie, G. (2009). Oxygen and organic matter thresholds for benthic faunal activity on the Pakistan margin oxygen minimum zone (700-1100 m). Deep-Sea Res. II 56, 449–471. doi: 10.1016/j.dsr2.2008.05.032
Lovrich, G. A., and Thiel, M. (2011). “Ecology, physiology, feeding and trophic role of squat lobsters,” in The Biology of Squat Lobsters, eds G. C. B. Poore, S. T. Ahyong, and J. Taylor (Boca Raton, FL: CRC Press), 183–222.
Mahmoudi, E., Essid, N., Beyrem, H., Hedfi, A., Boufahja, F., Vitiello, P., et al. (2005). Effects of hydrocarbon contamination on a free living community: results from microcosm experiments. Mar. Pollut. Bull. 50, 1197–1204. doi: 10.1016/j.marpolbul.2005.04.018
Mau, S., Sahling, H., Rehder, G., Suess, E., Linke, P., and Soeding, E. (2006). Estimates of methane output from mud extrusions at the erosive convergent margin off Costa Rica. Mar. Geol. 225, 129–144. doi: 10.1016/j.margeo.2005.09.007
McKew, B. A., Dumbrell, A. J., Taylor, J. D., McGenity, T. J., and Underwood, G. J. C. (2013). Differences between aerobic and anaerobic degradation of microphytobenthic biofilm-derived organic matter within intertidal sediments. FEMS Microbiol. Ecol. 84, 495–509. doi: 10.1111/1574/6941.12077
Menot, L., Sibuet, M., Carney, R.S., Levin, L.A., Rowe, G.T., Billet, D.S.M. et al. (2010). “New preceptions of continental margin biodiversity,” in Life in the World’s Oceans: Diversity, Distribution, and Abundance, ed. A. D. McIntyre (Cambridge, MA: Harvard University Press), 79–101.
Modig, H., and Olafsson, E. (1998). Responses of baltic benthic invertebrates to hypoxic events. J. Exp. Mar. Biol. Ecol. 229, 133–148. doi: 10.1016/S0022-0981(98)00043-4
Moodley, L., Schaub, B. E. M., van der Zwaan, G. G. J., and Herman, P. M. L. (1997). Tolerance of benthic foraminifera (Protista: Sarcodina). Mar. Ecol. Prog. Ser. 158, 151–163. doi: 10.3354/Meps169077
Murrell, M., and Fleeger, J. W. (1989). Meiofauna abundance on the Gulf of Mexico continental shelf affected by hypoxia. Cont. Shelf Res. 9, 1049–1062. doi: 10.1016/0278-4343(89)90057-5
Murty, S. J., Bett, B. J., and Gooday, A. J. (2009). Megafaunal responses to strong oxygen gradients on the Pakistan Margin of the Arabian Sea. Deep-Sea Res. II 56, 472–487. doi: 10.1016/j.dsr2.2008.05.029
Neira, C., and Decraemer, W. (2009). Desmotersia levinae, a new genus and new species of free-living nematode from bathyal oxygen minimum zone sediments off Callao, Peru, with discussion on the classification of the genus Richtersia (Chromadorida: Selachinematidae). Org. Divers. Evol. 9, 1–15. doi: 10.1016/j.ode.2008.09.004
Neira, C., Decraemer, W., and Backeljau, T. (2005). A new species of glochinema (Epsilonematidae: Nematoda) from the oxygen minimum zone off Baja California, NE Pacific and phylogenetic relationships at species level within the family. Cah. Biol. Mar. 46, 105–126.
Neira, C., King, I., Mendoza, G., Sellanes, J., De Ley, P., and Levin, L. A. (2013). Nematode community structure along a central Chile margin transect influenced by the oxygen minimum zone. Deep-Sea Res. I 78, 1–15. doi: 10.1016/j.dsr.2013.04.002
Neira, C., and Rackemann, M. (1996). Black spots produced by buried macroalgae in intertidal sandy sediments of the Wadden Sea: effects on the meiobenthos. J. Sea Res. 36, 153–170.
Neira, C., Gad, G., Arroyo, N. L., and Decraemer, W. (2001a). Glochinema bathyperuvensis sp. n. (Nematoda, Epsilonematidae): a new species from Peruvian bathyal sediments, SE Pacific Ocean. Contrib. Zool. 70,147–159.
Neira, C., Sellanes, J., Levin, L. A., and Arntz, W. E. (2001b). Meiofaunal distributions on the Peru margin: relationship to oxygen and organic matter availability. Deep-Sea Res. I 48, 2453–2472. doi: 10.1016/S0967-0637(01)00018-8
Neira, C., Sellanes, J., Soto, A., Gutiérrez, D., and Gallardo, V. A. (2001c). Meiofauna and sedimentary organic matter off central Chile: response to changes caused by the 1997–1998 El Niño. Oceanol. Acta 24, 313–328. doi: 10.1016/S0399-1784(01)01149-5
Nichols-Driscoll, J. (1976). Benthic invertebrate communities in Golfo Dulce, Costa Rica, an anoxic basin. Rev. Biol. Trop. 24, 281–297. doi: 10.15517/rbt.v24i2.25889
Nielsen-Muñoz, V., and Quesada-Alpízar, M. A. (eds.). (2006). Ambientes Marinos Costeros de Costa Rica. Informe Tecnico, Comisión Interdiciplinaria Marino Costera de la Zona Económica Exclusiva de Costa Rica. The Nature Conservancy (TNC), 219. Available at: http://www.ucipfg.com/Repositorio/BAAP/BAAP06/Publicaciones/Infome_ambientes_marino_costeros.pdf
Paulmier, A., and Ruiz-Pino, D. (2009). Oxygen minimum zones (OMZs) in the modern ocean. Prog. Oceanogr. 80, 113–128. doi: 10.1016/j.pocean.2008.08.001
Pennington, J. T., Mahoney, K. L., Kuwahara, V. S., Kolber, D. D., Calienes, R., and Chavez, F. P. (2006). Primary production in the eastern tropical Pacific: a review. Prog. Oceanogr. 69, 285–317. doi: 10.1016/j.pocean.2006.03.012
Pfannkuche, O., and Thiel, H. (1987). Meiobenthic stocks and benthic activity on the NE-Svalbard Shelf and in the Nansen Basin. Polar Biol. 7, 253–266. doi: 10.1007/BF00443943
Pfannkuche, O., and Thiel, H. (1988). “Sample processing,” in Introduction to the Study of Meiofauna, eds R. P. Higgins and H. Thiel (Washington, DC: Smithsonian Institution Press), 134–145.
Pineda, J., Cho, W., Starczak, V., Govindarajan, A. F., Guzman, H. M., Girdhar, Y., et al. (2016). A crab swarm at an ecological hotspot: patchiness and population density from AUV observations at a coastal, tropical seamount. PeerJ 4:e1770. doi: 10.7717/peerj.1770
Pusceddu, A., Gambi, C., Zepelli, D., Bianchelli, S., and Danovaro, R. (2009). Organic matter composition, metazoan meiofauna,, and nematode biodiversity in sediments of the deep Mediterranean Sea. Deep-Sea Res. II 56, 755–762. doi: 10.1016/j.dsr2.2008.10.012
Quesada-Alpízar, M. A., and Cortés, J. (2006). Los ecosistemas marinos del Pacifico sur de Costa Rica: estado del conocimiento y perspectivas de manejo. Rev. Biol. Trop. 54, 101–145.
Ramalho, S. P., Adão, H., Kiriakoulakis, K., Wolff, G. A., Vanreusel, A., and Ingels, G. (2014). Temporal and spatial variation in the Nazare canyon (Western Iberian margin): inter-annual and canyon heterogeneity effects on meiofauna biomass and diversity. Deep-Sea Res. I 83, 102–114. doi: 10.1016/j.dsr.2013.09.010
Rex, M. A., and Etter, R. J. (2010). Deep-sea Biodiversity: Pattern and Scale. Cambridge: Harvard University Press, 354.
Rex, M. A., Etter, R. J., Morris, J. S., Crouse, J., McClain, C. R., Johnson, N. A., et al. (2006). Global bathymetric patterns of standing stock and body size in the deep-sea benthos. Mar. Ecol. Prog. Ser. 317, 1–8. doi: 10.3354/meps317001
Rosli, N., Leduc, D., Rowden, A. A., Clark, M. R., Probert, P. K., Berkenbusch, K., and Neira, C. (2016). Differences in meiofauna communities with sediment depth are greater than habitat effects on the New Zealand continental margin: implications for vulnerability to anthropogenic disturbance. PeerJ 4:e2154.
Sahling, H., Masson, D. G., Ranero, C. R., Huhnerbach, V., Weinrebe, W., et al. (2008). Fluid seepage at the continental margin offshore Costa Rica and southern Nicaragua. Geochem. Geophys. Geosys. 9:Q05S05. doi: 10.1029/2008GC001978
Sajan, S., Joidas, T. V., and Damodaran, R. (2010). Meiofauna of the western continental shelf of India, Arabian Sea. Estuar. Coast. Shelf Sci. 86, 665–674. doi: 10.1016/j.ecss.2009.11.034
Sánchez, A., and Carriquiry, J. (2007). Accumulation of corg, norg, Porg and BSi off the magdalena margin, BCS (Mexico), during the past 26 kyr. Cienc. Mar. 33, 23–35.
Schmidtko, S., Stramma, L., and Visbeck, M. (2017). Decline in global oceanic oxygen content during the past five decades. Nature 542, 335–339. doi: 10.1038/nature21399
Schratzberger, M., and Ingels, J. (2017). Meiofauna matters: the roles of meiofauna in benthic ecosystems. J. Exp. Mar. Biol. Ecol. 502, 12–25. doi: 10.1016/j.jembe.2017.01.007
Sellanes, J., and Neira, C. (2006). ENSO as a natural experiment to study meiofaunal communities. Mar. Ecol. 27, 31–43. doi: 10.1111/j.1439-0485.2005.00069.x
Sellanes, J., Neira, C., and Quiroga, E. (2003). Composition, structure and energy flux of the meiobenthos off central Chile. Rev. Chil. Hist. Nat. 76, 401–415.
Sellanes, J., Neira, C., Quiroga, E., and Teixido, N. (2010). Diversity patterns along and across Chilean margin: a continental slope encompassing oxygen gradients and methane seep benthic habitats. Mar. Ecol. 31, 111–124. doi: 10.1111/j.1439-0485.2009.00332.x
Sellanes, J., Quiroga, E., Neira, C., and Gutiérrez, D. (2007). Changes of macrobenthos composition under different ENSO cycle conditions on the continental shelf off central Chile. Cont. Shelf Res. 27, 1002–1016. doi: 10.1016/j.csr.2007.01.001
Sergeeva, N. G., and Zaika, V. (2013). The black sea meiobenthos in permanently hypoxic habitat. Acta Zool. Bulg. 65, 139–150.
Smith, C. R., Levin, L. A., Hoover, D. J., McMurtry, G., and Gage, J. D. (2000). Variations in bioturbation across the oxygen minimum zone in the northwest Arabian Sea. Deep-Sea Res. II 47, 227–257. doi: 10.1016/S0967-0645(99)00108-3
Soltwedel, T., Hasemann, C., Queric, N. V., and von Juterzenka, K. (2005). Gradients in activity and biomass of the small benthic biota along a channel system in the deep Western Greenland Sea. Deep-Sea Res. I 52, 815–835. doi: 10.1016/j.dsr.2004.11.011
Stramma, L., Johnson, G. C., Sprintall, J., and Mohrholz, V. (2008). Expanding oxygen minimum zones in the tropical oceans. Science 320, 655–658. doi: 10.1126/science.1153847
Stramma, L., Schmidtko, S., Levin, L. A., and Johnson, G. C. (2010). Ocean minima expansions and their biological impacts. Deep-Sea Res. I 57, 1–9. doi: 10.1016/j.dsr.2010.01.005
Stukel, M. R., Benitez-Nelson, C. R., Décima, M., Taylor, A. G., Buchwald, C., and Landry, M. R. (2016). The biological pump in the Costa Rica Dome: an open-ocean upwelling system with high new production and low export. J. Phytopl. Res. 38, 348–365. doi: 10.1093/plankt/fbv097
Ter Braak, C. J. F., and Šmilauer, P. (2012). Canoco Reference Manual and User’s Guide: Software for Ordination (Version 5.0). Ithaca, NY: Microcomputer Power, 496.
Thamdrup, B., Canfield, D. E., Ferdelman, T. G., Glud, R. N., and Gundersen, J. K. (1996). A biogeochemical survey of the anoxic basin Golfo Dulce, Costa Rica. Rev. Biol. Trop. 44, 19–33. doi: 10.15517/rbt.v44i3.29402
Thiel, M., and Lovrich, G. A. (2011). “Agonistic behavior and reproductive biology of squat lobsters,” in The Biology of Squat Lobsters, eds G. Poore, S. T. Ahyong, and J. Taylor (Boca Raton, FL: CRC Press), 223–247.
Van Colen, C., Monserrat, F., Verbist, K., Vincx, M., Steyaert, M., Vanaverbeke, J., et al. (2009). Tidal flat nematode responses to hypoxia and subsequent macrofauna-mediated alterations of sediment properties. Mar. Ecol. Prog. Ser. 381, 189–197. doi: 10.3354/meps07914
Van Gaever, D., Galéron, J., Sibuet, M., and Vanreusel, A. (2009). Deep-sea habitat heterogeneity influence on meiofaunal communities in the Gulf of Guinea. Deep-Sea Res. II 56, 2259–2269. doi: 10.1016/j.dsr2.2009.04.008
Van Oevelen, D., Soetaert, K., Garcia, R., de Stigter, H. C., Cunha, M. R., Pusceddu, A., et al. (2011). Canyon conditions impact carbon flows in food webs of three sections of the Nazaré canyon. Deep-Sea Res. II 58, 2461–2476. doi: 10.1016/j.dsr2.2011.04.009
Vaquer-Sunyer, R., and Duarte, C. M. (2008). Thresholds of hypoxia for marine biodiversity. Proc. Natl. Acad. Sci. U.S.A. 105, 15452–15457. doi: 10.1073/pnas.0803833105
Vaquer-Sunyer, R., and Duarte, C. M. (2011). Temperature effects on oxygen thresholds for hypoxia in marine benthic organisms. Glob. Change Biol. 17, 1788–1797. doi: 10.1111/j.1365-2486.2010.02343.x
Vargas, J. A. (1988). A survey of the meiofauna of an eastern tropical Pacific intertidal mud flat. Rev. Biol. Trop. 36, 541–544.
Veit-Köhler, G., Durst, S., Schuckenbrock, J., Hauquier, F., Durán Suja, L., Dorschel, B., et al. (2018). Oceanographic and topographic conditions structure benthic meiofauna communities in the Waddell Sea, Bransfield Strait and Drake Passage. Prog. Oceanogr. 162, 240–256. doi: 10.1594/PANGAEA/882582
Veit-Köhler, G., Gerdes, D., Quiroga, E., Hebbeln, D., and Sellanes, J. (2009). Metazoan meiofauna within the oxygen minimum zone off Chile: results of the 2001-Puck expedition. Deep-Sea Res. II 56, 1105–1111. doi: 10.1016/j.dsr2.2008.09.013
Wetzel, M. A., Fleeger, J. W., and Powers, S. P. (2001). “Effects of hypoxia and anoxia on meiofauna: a review with new data from the Gulf of Mexico,” in Coastal Hypoxia: Consequences for Living Resources and Ecosystems, eds N. N. Rabalais and R. E. Turner (Washington, DC: AGU), 165.
Wright, J. J., Konwar, K. M., and Hallam, S. J. (2012). Microbial ecology of expanding oxygen minimum zones. Nat. Rev. Microbiol. 10, 381–394. doi: 10.1038/nrmicro2778
Zeppilli, D., Leduc, D., Fontanier, C., Fontaneto, D., Fuchs, S., Gooday, A. J., et al. (2018). Characteristics of meiofauna in extreme marine ecosystems: a review. Mar. Biodiv. 48, 35–71. doi: 10.1007/s12526-017-0815-z
Keywords: meiofauna, oxygen minimum zone, eastern Pacific, bathyal, community structure, Costa Rica margin
Citation: Neira C, Ingels J, Mendoza G, Hernandez-Lopez E and Levin LA (2018) Distribution of Meiofauna in Bathyal Sediments Influenced by the Oxygen Minimum Zone Off Costa Rica. Front. Mar. Sci. 5:448. doi: 10.3389/fmars.2018.00448
Received: 29 August 2018; Accepted: 08 November 2018;
Published: 04 December 2018.
Edited by:
Cinzia Corinaldesi, Università Politecnica delle Marche, ItalyReviewed by:
Daniela Zeppilli, Institut Français de Recherche pour l’Exploitation de la Mer (IFREMER), FranceFederica Semprucci, Università degli Studi di Urbino Carlo Bo, Italy
Copyright © 2018 Neira, Ingels, Mendoza, Hernandez-Lopez and Levin. This is an open-access article distributed under the terms of the Creative Commons Attribution License (CC BY). The use, distribution or reproduction in other forums is permitted, provided the original author(s) and the copyright owner(s) are credited and that the original publication in this journal is cited, in accordance with accepted academic practice. No use, distribution or reproduction is permitted which does not comply with these terms.
*Correspondence: Carlos Neira, cneira@ucsd.edu Jeroen Ingels, jingels@fsu.edu