- 1Facultad de Ciencias del Mar y de Recursos Naturales, Universidad de Valparaíso, Viña del Mar, Chile
- 2Center for Climate and Resilience Research, University of Chile, Santiago, Chile
- 3Department of Oceanography, Faculty of Natural and Oceanographic Sciences, University of Concepción, Concepción, Chile
- 4Department of Geophysics, Faculty of Physics and Mathematics Sciences, University of Chile, Santiago, Chile
Using in situ hydro-chemical data and MODIS-SeaWiFS ocean color images as a proxy of river plumes and phytoplankton biomass from 2000 to 2014, this study documents the temporal co-variability of river discharge, plume area, nitrate and phosphate export and phytoplankton biomass in the coastal waters off Central Chile (33∘–37∘S). Five major rivers (Maipo, Mataquito, Maule, Itata, and Biobío) drain into this region with annual mean discharge ranging from 120 to 1000 m3 s−1. River discharge and coastal plume area present a marked seasonal cycle, reaching maximum values during the winter rainy season (June-September). Export of riverine nutrients also peaks in winter, leading to an increase in phytoplankton biomass within the plumes that can be twice larger than the background values in coastal areas away from the river mouths. Wintertime river discharge, plume area and nutrient export are also correlated at interannual time scales. During a recent extended dry period (2010–2014), river discharges, plume areas and nutrient export clearly decreased by about 50% compared to historical values, reducing significantly the size of the chlorophyll pool within plumes off Central Chile during winter. The potential impacts of droughts are discussed in terms of coastal ecology and primary production, a highly relevant issue considering the projections of a dry climate over Central Chile in the future. Systematic evidence of mega-drought effects upon coastal productivity still does not exist, but it remains a priority to further investigate and quantify these impacts.
Introduction
Phytoplankton biomass (PB) and primary production (PP) in coastal areas are highly variable over temporal and spatial scales, primarily regulated by nutrient supply, light availability and physical processes driving stratification/mixing and advection (e.g., Hickey and Banas, 2003). Organic matter, nutrients and particles from terrestrial sources are partially delivered into coastal areas through rivers, which can support both PB and PP, as well as regulate coastal biogeochemical cycles of Nitrate (NO3−), Phosphate (PO4−3), and Silicate [Si(OH)4] (Hopkinson and Vallino, 2005; Cotrim et al., 2007). Through their impact on precipitation, both natural variability (e.g., El Niño Southern Oscillation, ENSO) and anthropogenic climate change may alter river discharge into coastal areas over interannual and longer time scales, leading to considerable impacts on coastal PB and PP.
Several studies have reported a positive relationship between increased river discharge, nutrient input, and high PB in adjacent coastal areas to river mouths. For example, in coastal waters off Japan, chlorophyll (Chl-a) concentration from ocean color satellite estimates was two times higher during periods of high river discharge compared to periods of low discharge (Lihan et al., 2011). Similarly, high Chl-a levels were observed in winter and early spring, coinciding with an abrupt precipitation increase in the Apalachicola River in northwestern Florida (Morey et al., 2009). A similar trend of high Chl-a concentrations and productive plume waters, enriched by the Mississippi River nutrients, was observed across the shelf and along the coast of Louisiana (Wysocki et al., 2006). On the other hand, a reduction of freshwater input into coastal areas during droughts led to a marked decrease in Chl-a biomass over various shelf-coastal and estuarine environments, as observed in the North Atlantic and Pacific oceans (Cloern et al., 1983; Nichols, 1985; Wetz et al., 2011). Furthermore, ecological studies have indicated a deterioration of the planktonic food web during a period of drought in the coastal waters of the North Pacific, with negative consequences on fish populations due to cascading effects on higher trophic levels (Cloern et al., 1983; Nichols, 1985; Wetz et al., 2011).
Along eastern boundary systems, such as California, Humboldt and Benguela, the effects of the river discharge on coastal PB/PP are less apparent. This is due to the considerable influence of coastal upwelling, the process by which cold, nutrient-rich subsurface water ascends to the ocean’s surface, creating the most biologically productive areas of the global ocean (Pauly and Christensen, 1995). The importance of nutrient and particle supply from river discharge with respect to coastal upwelling is seasonally variable, and depends on physical processes (such as thermal and haline stratification, wind stress, and rainfall), as well as the geographical and topographical features of the coastal area. For example, the Columbia River has been characterized as a major nutrient source to the adjacent coastal area, promoting positive effects on PB and PP during extended periods of reduced coastal upwelling (Hickey et al., 2010). A similar situation occurred in the Canadian Beaufort shelf where the Mackenzie River re-injects nutrients into the surface with a high N:P or N:Si ratio, influencing the phytoplankton physiology (Tremblay et al., 2014).
Central Chile, along the subtropical west coast of South America (33°–38°S), is characterized by an archetypical Mediterranean climate with an annual mean precipitation ranging from 100 to 1000 mm, predominantly occurring during the austral winter. Because part of the winter precipitation is stored as snow in the Andes cordillera most of the rivers in this region exhibit a seasonal mixed pluvio-nival regime (e.g., Garreaud et al., 2017). On interannual scales, variations in river discharges are correlated with ENSO, since an increase in precipitation over Central Chile during El Niño years leads to high river flows (Aceituno and Vidal, 1990; Waylen et al., 1993; Pellicciotti et al., 2007). Conversely, La Niña is often associated with a decrease in precipitation and low rivers discharge (Aceituno and Vidal, 1990). The ENSO fluctuations, however, only account for about 50% of the total precipitation variation in Central Chile (Montecinos et al., 2011), and many dry years do not coincide with La Niña events. Of particular relevance, the recent “mega-drought” in Central Chile (Garreaud et al., 2017), an uninterrupted sequence of dry years beginning in 2010, coincides with mostly ENSO-neutral conditions. The mega-drought has produced rainfall deficits of about 30% across much of Central Chile, a relatively small decrease compared with other droughts in the past (e.g., >60% in 1924, 1968, and 1988) but its duration is unprecedented in the historical record and millennial reconstruction (Garreaud et al., 2017). Furthermore, approximately one third of the dry signal has been associated with climate change (Boisier et al., 2016).
In the coastal waters off Central Chile both river discharge and coastal upwelling occur, but their seasonal cycles are out of phase. Strong southerly upwelling favorable winds prevail during spring-summer (e.g., Rahn and Garreaud, 2014), leading to an intense increase in PB and PP (Montecino et al., 2006; Thiel et al., 2007). During fall and winter the southerly winds relax, whereas rainfall and river discharge simultaneously reaches a maximum. Thus, the seasonal signal of river plumes extension in the region detected using satellite ocean color images showed persistent and large river plumes during fall-winter period (Saldías et al., 2012). Indeed, major plume events are associated with the warm phases of ENSO and PDO when precipitation tends to be above normal (Saldías et al., 2016).
In this region, recent studies have shown evidences of the impact of the nutrient export in these river plumes on the carbon biogeochemistry and phytoplankton community of coastal waters off Central Chile in winter (Iriarte et al., 2012; Léniz et al., 2012; Pérez et al., 2015; Yevenes et al., 2016) and its possible contribution in maintaining high primary productivity rates in coastal waters (Testa et al., 2018). Nonetheless, there is a lack of a systematic analysis into the regional scale influence of the river discharges variability on nutrient loading and phytoplankton biomass in coastal waters. The present work investigates the impact of discharge of the five major rivers in Central Chile on river plumes, nutrient export, and phytoplankton biomass in coastal waters, based on datasets from 2000 to 2014, and discerns the effect of the extended mega-drought in theses coastal processes. We also discuss the representativeness of the satellite based PB estimates in coastal areas by contrasting those values against in situ data, the effects of the present mega-drought on the ecology and trophic dynamics of adjacent areas. A full assessment of the consequences of regional climate change on coastal hydro-biology is beyond the scope of this work, but this key issue is discussed on the basis of the observed impacts of the recent mega-drought that is relevant considering the prospect of a drier, warmer climate in central Chile (Fuenzalida et al., 2007; Bozkurt et al., 2017).
Materials and Methods
Hydrological River Survey
The study area spans from 33° to 37°S along the west coast of South America, including the outlets from the Maipo, Mataquito, Maule, Itata, and Biobío Rivers (Figure 1). The main features of these basins are presented in Table 1. Daily mean discharge in these rivers is recorded at fluviometric stations located about 20 km from the river mouth. Data from January 2000 to December 2014 is provided by the Department of Water at the Ministry of Public Works, Chile (Dirección General de Aguas, DGA1). Monthly mean discharge values are calculated for months when more than 70% of the daily values were available. The annual mean discharge varies from ∼120 m3 s−1 for the Maipo River to ∼1000 m3 s−1 for the Biobío River, reflecting the north-to-south precipitation increase as well as differences between catchment areas (Table 1).
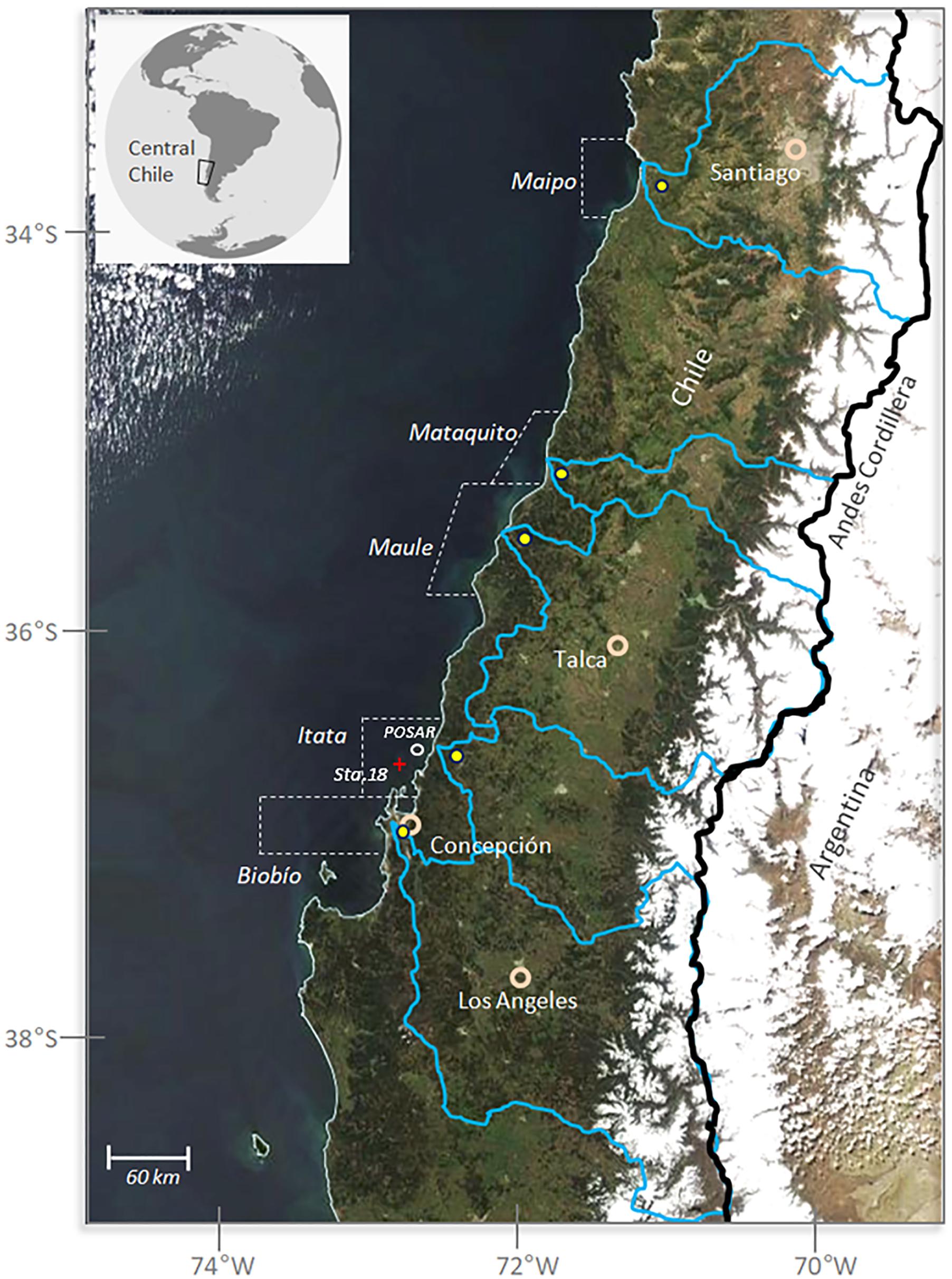
FIGURE 1. General map of the study area. The background picture is a TERRA-MODIS visible image (September 22, 2013) upon, which we have mapped the main river basin boundaries (blue lines) and the Chile-Argentina border (black line following the Andes crest level). Off Itata River we have mapped the position of the Oceanographic Station 18 and the coastal buoy POSAR. Also shown are coastal adjacent areas at the mouth of each river (dashed polygons) and major cities.
Nutrient (Nitrate-NO3− and Phosphate-PO4−3) concentrations in the rivers are measured on a quarterly basis at the same fluviometric stations. Thus, each year four simultaneous nutrient values are obtained for each river. Nutrient exportation (load) is estimated as a product of river discharge Q (m3 s−1) and nutrient concentration C (mg L−1). Estimates of the daily nutrient load (Q × C, kg day−1) are obtained using a load estimator (LOADEST, Runkel et al., 2004) as follow:
Where, Load is the pollutant burden, an∼1 are the regression coefficients and Q is the streamflow in a specific period. The regression coefficients are obtained using multiple predictors (flow, time, and other variables) and a maximum likelihood estimation method (Cohn, 2005). The daily loads are then aggregated to obtain the NO3− and PO4−3 river export over a monthly basis (expressed in Ton month−1).
Ocean Color Data: Plume Signal Detection and Phytoplankton Biomass
To study the seasonal and interannual variability of plumes extension and phytoplankton biomass in coastal waters we used ocean color (OC) datasets (4 km × 4 km pixels and 8-day composition) between January 2000 to December 2014, including chlorophyll and normalized water-leaving radiance at 555 nm (nLw555) from SeaWiFS (2000–2002) and MODIS-Aqua (Moderate Resolution Imaging Spectroradiometer, 2002–2014). Satellite images are obtained from the NASA Ocean Color web server2 and the GlobColour core dataset3. Ocean color, 8-day composition datasets (3 or 4 scenes per month) are used to reduce the loss of information due to cloud effects, with cloud-free data available for over 90% of the region (Saldías et al., 2012, 2016).
The phytoplankton biomass (PB) is derived from chlorophyll-a (Chl-a) based on standard NASA’s algorithms using OC datasets (O’Reilly et al., 2000; Hu et al., 2012), which are known to overestimate Chl-a concentrations in coastal waters (e.g., Lohrenz et al., 2008). Our study, however, focuses on the seasonal and interannual variability of PB rather than absolute values, for which the satellite timeseries estimates are a viable tool to represent the surface pigment dynamics in coastal waters as suggested before by other studies (Lohrenz et al., 2008; Hopkins et al., 2013). To further evaluate the satellite derived 8-day Chl-a concentrations we contrasted those valued against in situ surface measurements collected from 2006 to 2014 at Oceanographic Station 18 (77 match-up data points). Station 18 is under the influence of the Itata river plume and is located 18 nautical miles (30 km) from the coast (36°30′S–73°07′W; Figure 1). The correlation coefficient between both measurements on the logarithmic scale shows that both Chl-a series are well correlated (rs = 0.65, SD = 0.33, p < 0.001) supporting that satellite Chl-a retrieval can be useful to track and characterize the plume rivers.
The nLw555 band is analyzed to detect the river plume signal in the coastal zone. Previous studies have confirmed the use of this band to detect the river plume signal associated with discharge variability (Otero and Siegel, 2004; Nezlin et al., 2005; Thomas and Weatherbee, 2006). The river plume is identified as the area close to the river mouth where nLw555 values are higher than 1.50 mW cm−2 μm−1 sr−1, following criteria from Saldías et al. (2012).
Whenever the plume is present, area (expressed in km2) and mean Chl-a are calculated to produce monthly mean values. The plume areas ranged between 120 and 500 km2 (Supplementary Table 1) so that at least 10 pixels are used to define the previous statistics. Granted, higher spatial resolution will result in more accurate plume definitions but, once again, our focus is on the large month-to-month and year-to-year changes in plume’s features. In addition to the monthly values, river plume frequency is calculated on a yearly basis (number of 8-days scenes with plume signal by year). In order to provide context for the potential PB enhancement generated by the rivers, the mean Chl-a concentration is calculated over a larger coastal area adjacent to each river mouth. For each river, the adjacent area is outlined by the maximal plume extent on record (Figure 1) and remains fixed in time.
Results
Mean Seasonal Cycle
The coastal waters off Central Chile are characterized by marked seasonal differences in PB as shown in Figure 2. This figure shows satellite-derived maps of Chl-a during one week in winter (13–20 August 2008) and late spring (16–23 November 2008). In winter, Chl-a presents low values (≤2.50 mg m−3) along the coast with some patches of higher values surrounding the mouths of major rivers. The river plumes are clearly detected in coastal water by their large nLw555 values. In late spring, Chl-a values in excess of 5 mg m−3 are found along the coast, up to 100 km offshore. These high values in spring are due to coastal upwelling, whereas river plumes are undetected or considerably reduced compared to wintertime.
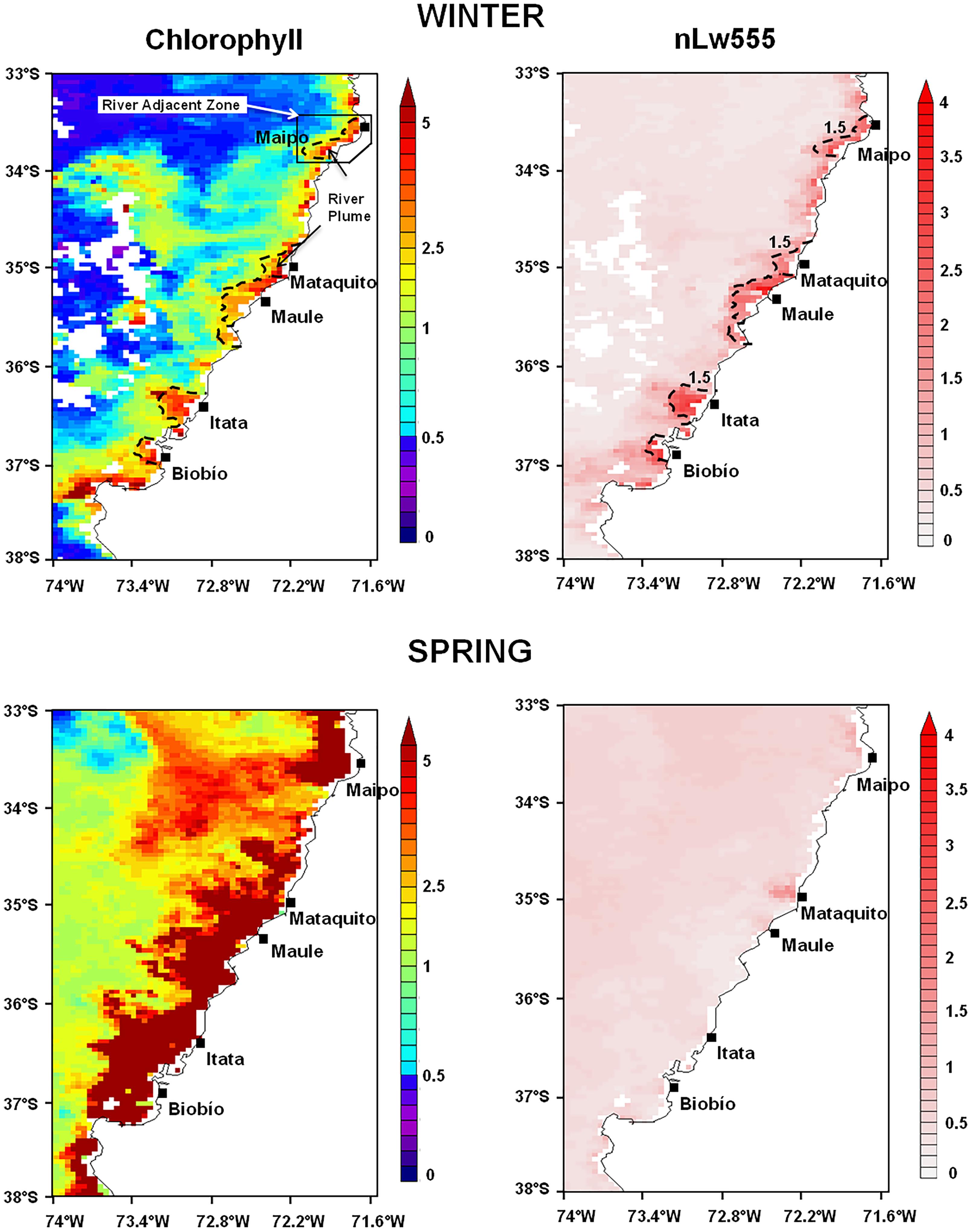
FIGURE 2. Satellite-derived Chlorophyll (mg m−3) and nLw555 (mW cm−2 μm−1 sr−1) conditions observed during winter (13–20 August 2008) and spring (16–23 November 2008) along the coastal area off Central Chile. Black dashed line indicates the winter river plume boundaries (nLw555 > 1.5 mW cm−2 μm−1 sr−1) detected in the adjacent coastal waters.
The seasonal cycles of river discharge and plume area are shown in Figure 3, using 15 years of hydrological records and satellite derived SeaWiFS-MODIS ocean color data. All rivers exhibit a clear seasonal cycle with higher discharge during winter (rainy season) compared to summer-fall (dry season). The areal extent of the river plumes follows a similar seasonal cycle, with a prominent maximum in all rivers during winter (Figure 3B). In general, mean wintertime plume areas are 3–12 times larger than in summer. During the fall-winter period (May-September) river plumes tend to be both larger and more frequent, detected during 85, 71, 99, 79, and 71% of the time in the Maipo, Mataquito, Maule, Itata, and Biobío Rivers, respectively.
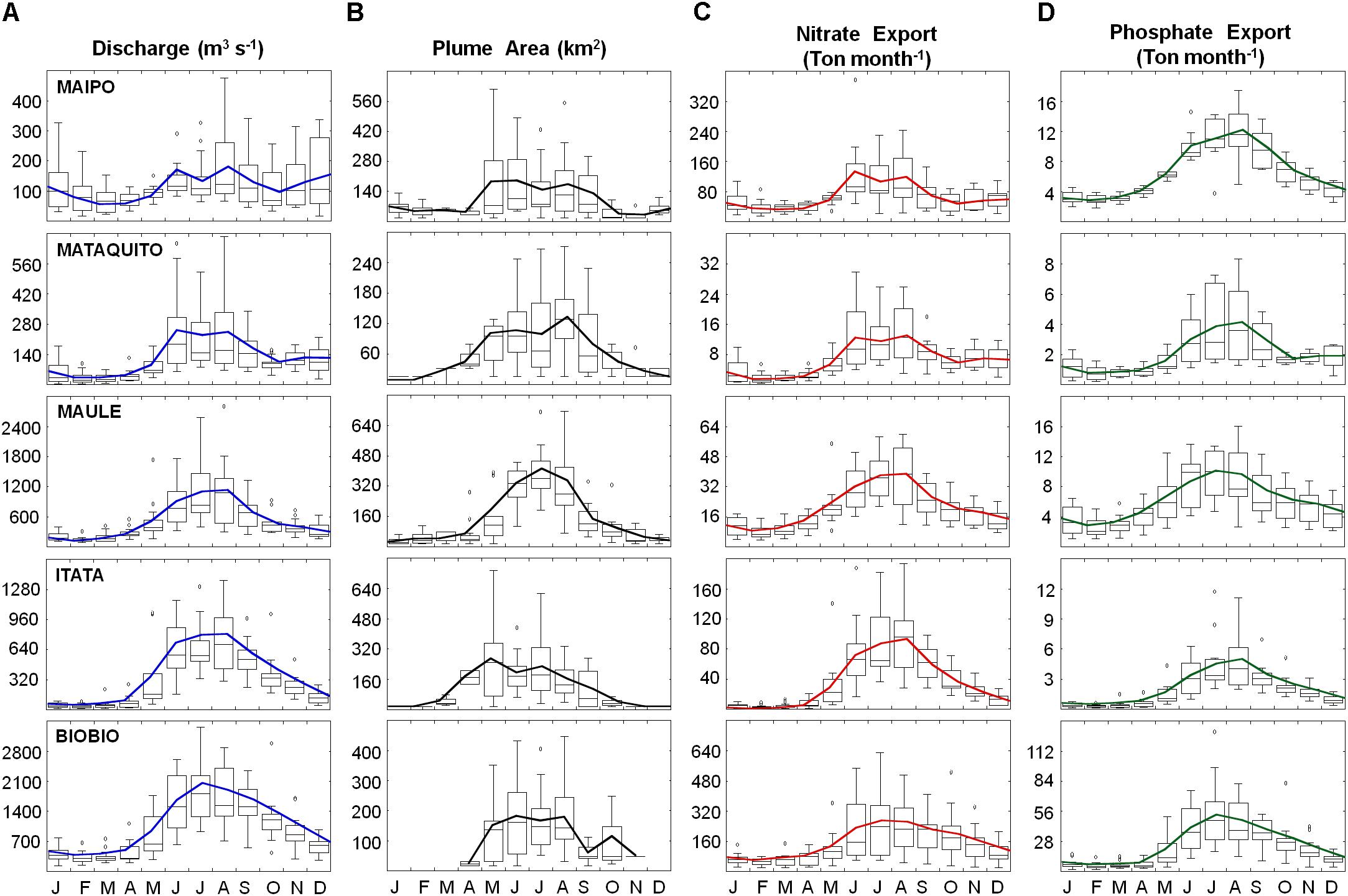
FIGURE 3. Seasonal cycles of (A) river discharge (m3 s−1), (B) satellite-derived river plume area (km2), (C) nitrate- NO3− and (D) phosphate-PO4−3 export (Ton month−1) for the Maipo, Mataquito, Maule, Itata, and Biobío rivers. Solid colored lines correspond to the monthly mean value.
The Maipo and Mataquito Rivers, located in the northern sector of the study area, exhibit water discharge with a secondary maximum in December due to snowmelt contribution. The secondary maxima do not cause a substantial increase in plume area, probably due to the plume signal being dissipated offshore through upwelling activity. Conversely, the Biobío River does not produce a detectable river plume from December to March, during minimum discharge (but still remains >500 m3 s−1). This is due to wind-driven dynamics that seasonally maintain the plume within a close reach of the river mouth, and therefore undetectable by the satellite (Saldías et al., 2012). It is also noted that in Central Chile the mean river plume area during winter is not proportional to the average discharge. For instance, both the Maipo and Biobío River plumes reach about 180 km2 in winter; however, the Biobío River flow is ∼10 times larger than the Maipo. Likewise, the largest wintertime plume is produced by the Maule River (>900 km2), that has an intermediate values of discharge. This inter-river variability in plume areas may reflect differences in lithology and land-use of the draining basins (and hence river loads). The offshore and alongshore extent of these plumes are also controlled by the interplay of different processes like coriolis effect and surface wind stress, as well as by the mixing of the plume waters with the coastal ocean waters. Here the satellite-derived plume areas provide a broad but reasonably characterization of the seasonal variability of the whole plume under these complex dynamic processes.
Nutrient concentrations measured near the mouth of each river are extremely variable and have a very weak seasonal cycle (Pizarro et al., 2010). NO3− concentrations range from 0.01 to 10.50 mg L−1, while mean PO4−3 concentration ranges from <0.005 to 0.98 mg L−1. In both cases, maximum NO3− and PO4−3 concentrations were found in the Maipo River. However, nutrient exportations into coastal areas show a seasonal cycle certainly controlled by river discharge, reaching a marked maximum during winter (Figures 3C,D). Maximum mean values of NO3− and PO4−3 export are observed in the Biobío River, with 245 and 45 Ton month−1 in winter, respectively, whereas minimum mean values are found in summertime for the Mataquito and Itata Rivers with 3.95 NO3− Ton month−1 and 0.56 PO4−3 Ton month−1 exported, respectively.
The phytoplankton biomass within river plumes (area mean Chl-a) remains fairly constant throughout the year, and similar between the rivers, with typical values about 4 mg m−3 (red lines in Figure 4). Thus, when the mean biomass is scaled by the plume area, a clear seasonal cycle of Chl-a pool size is observed, with higher values in winter (ca. June to August, 1.50 to 5.40 Chl-a Ton for the Mataquito and Maule Rivers, respectively). On the other hand, PB in coastal areas adjacent to the river mouth exhibit a marked seasonal cycle (displayed by the discontinuous lines in Figure 4), with summertime values being 2–4 times higher than in winter, following the wind-driven upwelling regime of the region. Notably, for all rivers in the study area, wintertime PB within the plume is 1.5–2 times larger than in the surrounding area (Figure 4). As discussed in section 4.2, locally increased PB near the river mouth, coupled with a large plume area and frequency, reinforces the crucial role of land-sourced nutrient inputs in winter when upwelling activity is at a minimum.
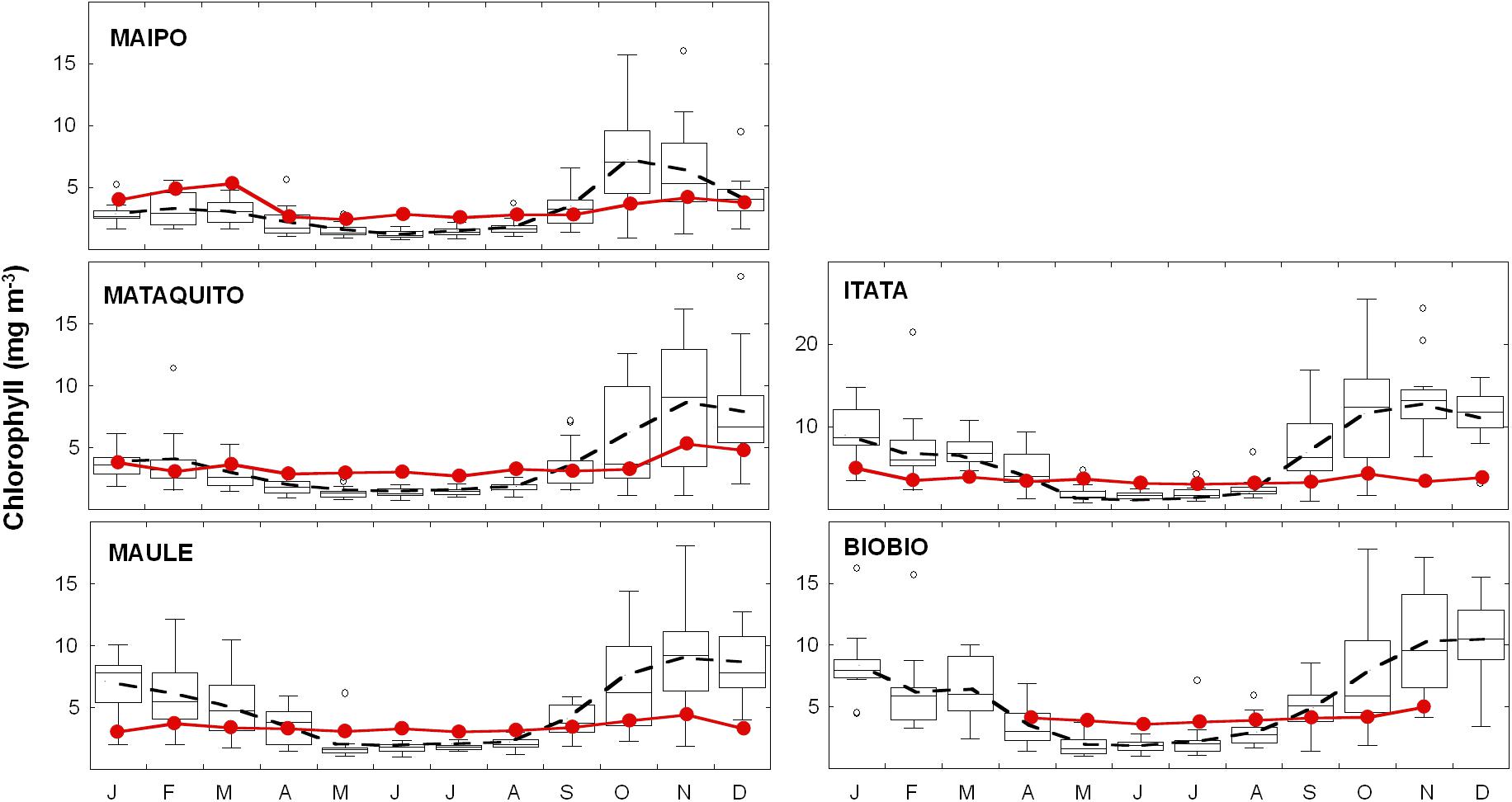
FIGURE 4. Seasonal cycle of phytoplankton biomass (Chlorophyll; mg m−3) in the river plume and coastal waters area adjacent to each river mouth. Solid red lines with circles and dashed black line indicate PB monthly mean for the river plume and coastal area adjacent to the river, respectively.
Inter-Annual Variability
Yearly variability of selected variables, averaged over fall-winter (May–September), is shown in Figure 5. Mean river discharge and plume area show a strong interannual variability, with a standard deviation of approximately half of the amplitude of the mean annual cycle. Not surprisingly, the year-to-year variability of river discharge is strongly correlated with the plume area for all rivers, except for the Biobío River (Spearman correlation coefficients larger than 0.50, Table 2).
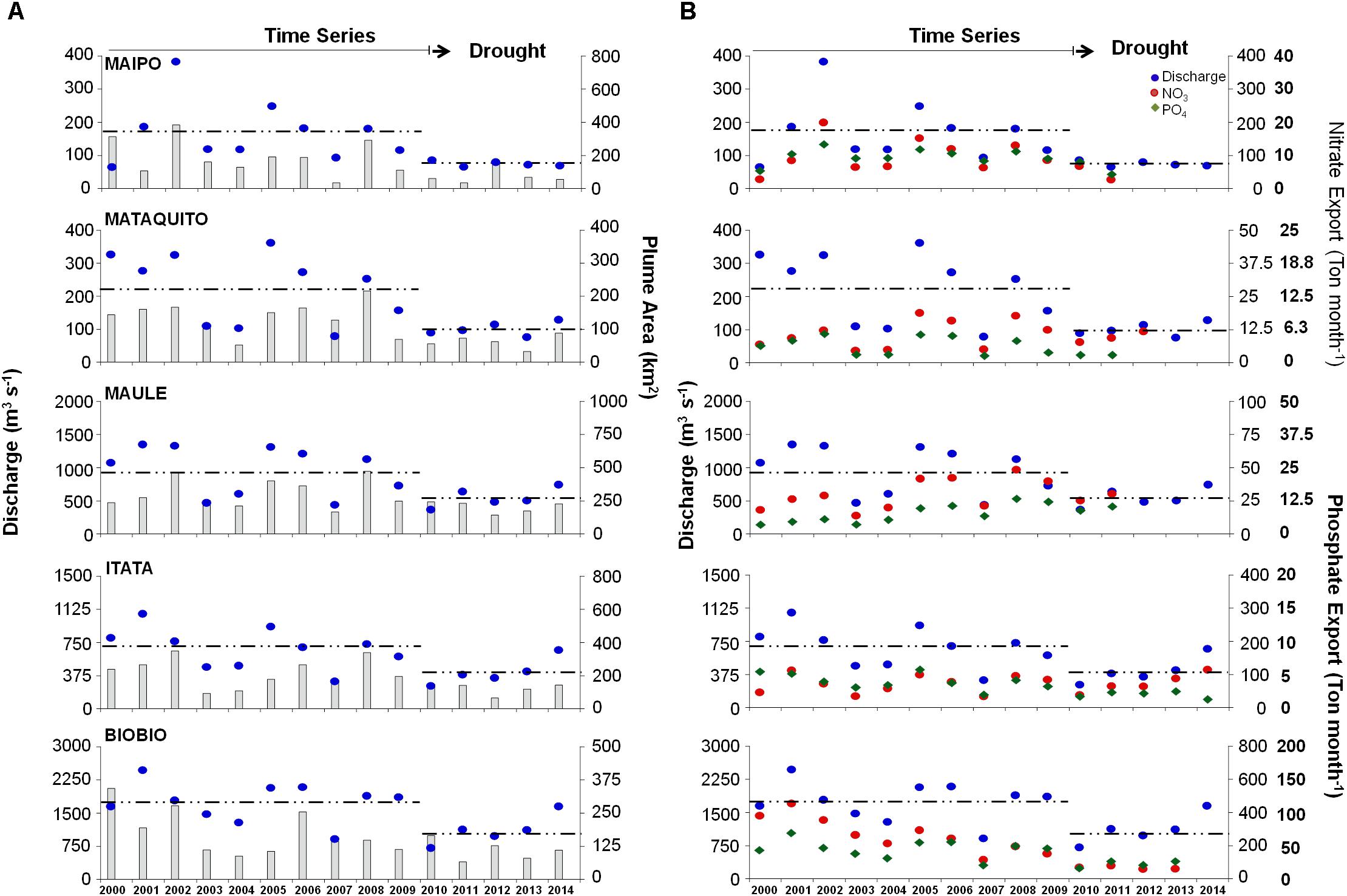
FIGURE 5. Inter-annual variability of (A) river discharge (m3 s−1; blue circles), river plume area (km2; gray bars) and (B) nitrate- NO3− (Ton month−1; red circle) and phosphate-PO4−3 export (Ton month−1; green diamond). Dashed lines indicate river discharge mean value during normal (2000–09) and drought (2010–14) periods, respectively.
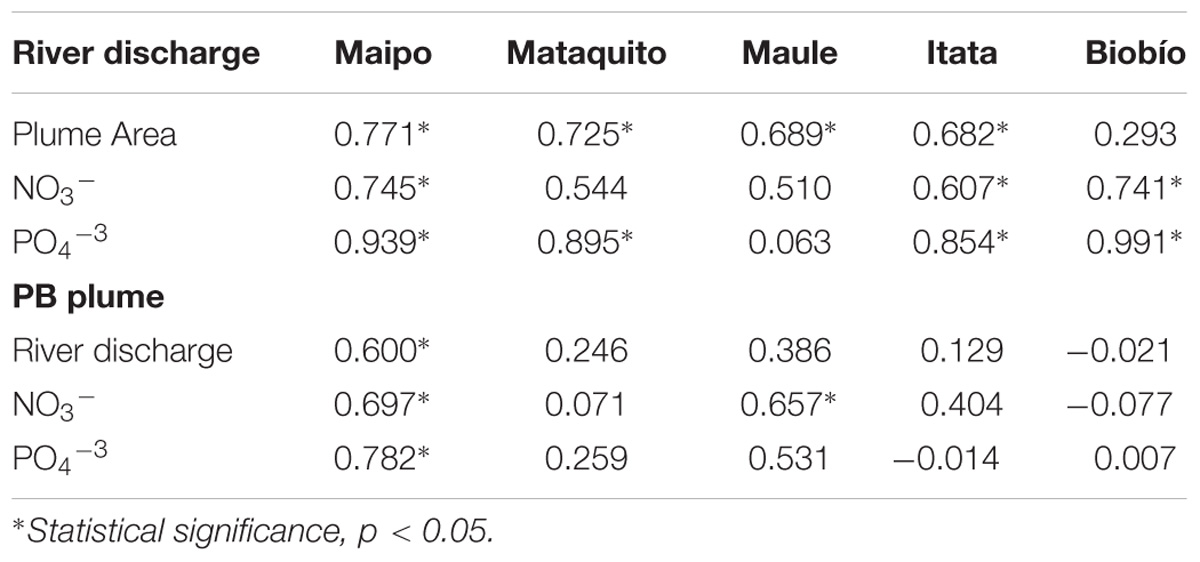
TABLE 2. Spearman correlation coefficients (rs) for the Maipo, Mataquito, Maule, Itata, and Biobío Rivers using 15 years of mean annual values of river discharge (m3 s−1), river plume area (km2), Nitrate- NO3−, and Phosphate-PO4−3 export (Ton month−1) and phytoplankton biomass plume (Chlorophyll; mg m−3).
Interannual variations in NO3− and PO4−3 concentrations is generally not correlated with discharge, whereas total nutrient export only shows a significant positive correlation with discharge in the Maipo, Itata, and Biobío Rivers (Figure 5 and Table 2). Likewise, year-to-year variability of PB is not significantly correlated with river discharge and nutrient exportation, except in the Maipo River (Table 2).
The Impact of the Mega-Drought
Two periods can be clearly distinguished in the timeseries of the discharge in all the rivers considered: a normal period between 2000 and 2009, and the mega-drought period between 2010 and 2014. The mean river discharge in winter during the mega-drought (2010–2014) is reduced to around half of the average of the previous decade (2000–2009) (Table 3 and Figure 6), as the drought propagates from rainfall to stream flow (Garreaud et al., 2017). Likewise, the mean plume area and nutrient loads during the mega-drought are only 40–60% of their corresponding “normal” values (2000–2009). On the contrary, the frequency of plume detection declines less markedly, and remains unchanged in the Maule River. However, the mega-drought period significantly affects the nutrients loads, its exportation and PB within the Maipo and Mataquito river plumes (Table 3). In all rivers, large plume events (area >2× annual mean for each river) are observed from May to September during the period between 2000 and 2009, but these events were reduced by nearly 80% during the mega-drought.
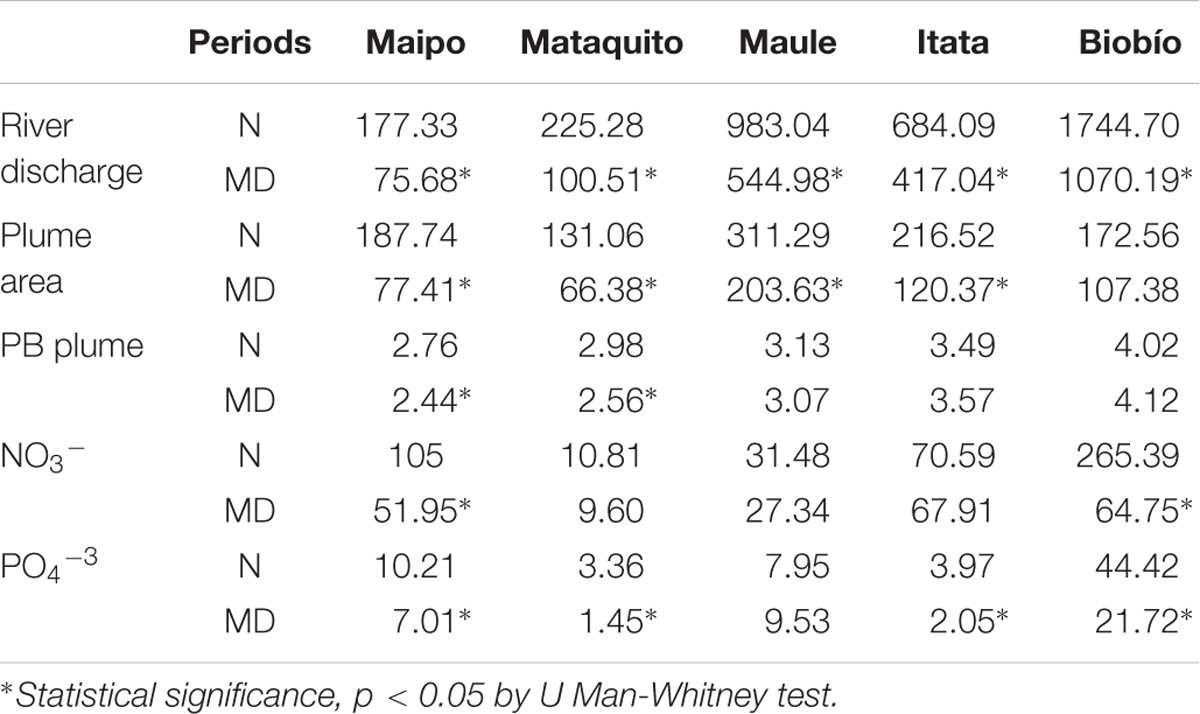
TABLE 3. Comparison between the “normal” 2000–2009 (N) and the “mega-drought” 2010–2014 (MD) periods using mean values of the river discharge (m3 s−1), river plume area (km2), Nitrate- NO3−, and Phosphate-PO4−3 export (Ton month−1) and phytoplankton biomass (Chlorophyll; mg m−3) in the river plume.
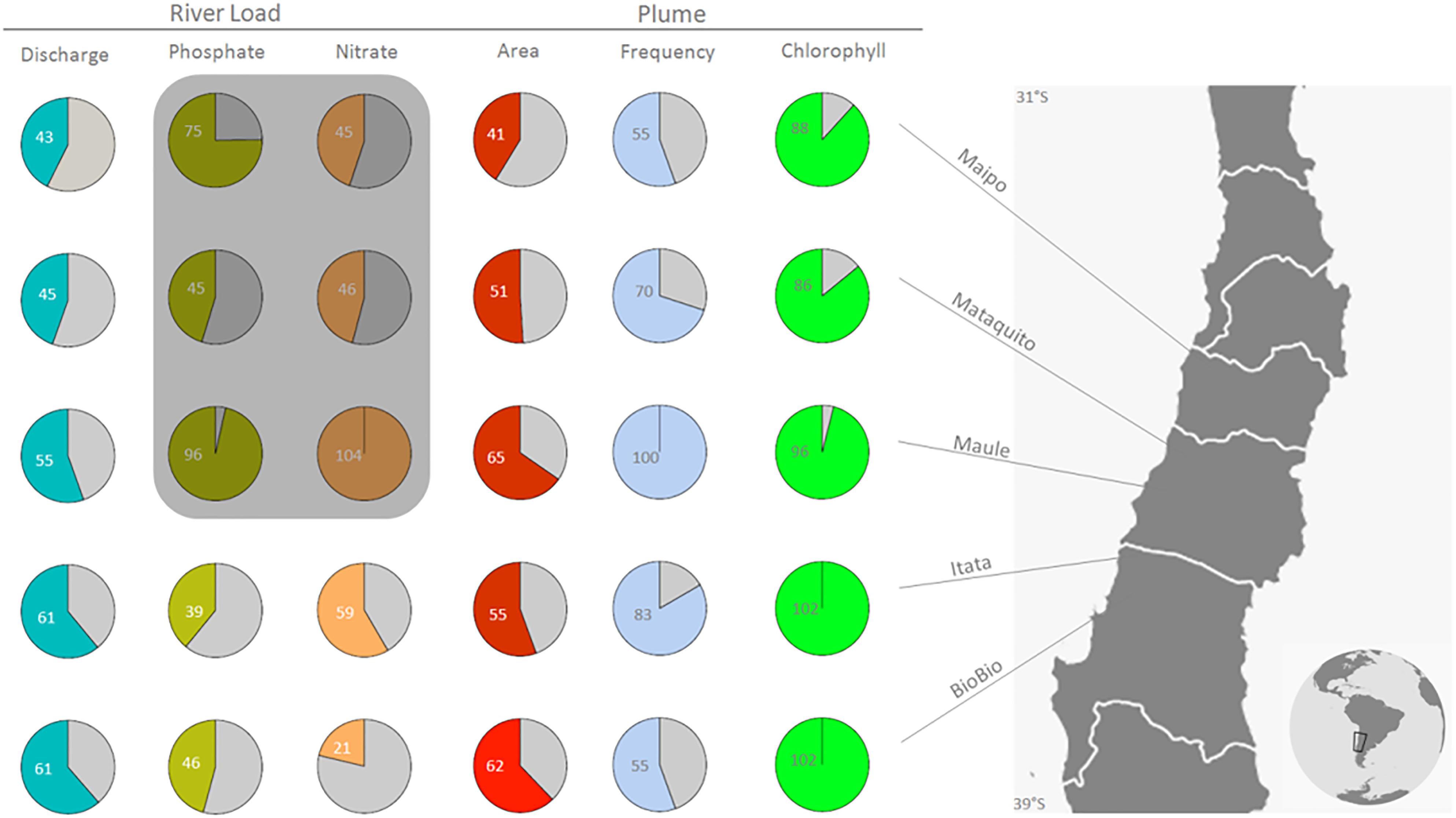
FIGURE 6. Impact of mega-drought in coastal areas off Central Chile in terms of river load (Discharge; m3 s−1, Phosphate-PO4−3 and Nitrate- NO3− export; Ton month−1), river plume area (km2), frequency, and phytoplankton biomass (Chlorophyll; mg m−3). Numbers and colored areas indicate the mega-drought (2010–2014), mean values as a percentage of the averages over the past decade (2000–2009) for five rivers in Central Chile. Shadow box indicates that Maipo, Mataquito, and Maule Rivers only have Phosphate-PO4−3 and Nitrate- NO3− export data until 2011.
Discussion
Rivers and Enhanced Phytoplankton Biomass
Off Central Chile, over seasonal and interannual time scales there is a strong correlation between river discharge and the size and frequency of river plumes (derived from ocean color satellite data) as well as nutrient export (estimated from in situ measurements). The high input of land-sourced nutrients (mainly NO3− and PO4−3) appears to enhance biological activity near river mouths in Central Chile, as the mean Chl-a concentrations within the river plumes are approximately twice the background PB values in coastal areas during winter, when discharge is at a maximum but upwelling is at a minimum. This result agrees with observations from the north Pacific and Atlantic oceans (Cloern et al., 1983; Mallin et al., 1993; Wysocki et al., 2006; Morey et al., 2009; Hickey et al., 2010).
In situ measurements of phytoplankton biomass verify the results obtained from satellite-based data. Previous studies in the Maipo River suggest that river discharge has a positive influence on phytoplankton biomass during upwelling-relaxed conditions (Wieters et al., 2003). Off the Itata River, evidence suggests that nutrient inputs have a positive influence on the pico-nanophytoplankton community (Iriarte et al., 2012). Furthermore, Itata River discharge during austral winter may partially support basal productivity around the river mouth, as a result of nutrient input and the influence of the fresh water plume on water column stratification (Testa et al., 2018). Recently, salinity (measured by a SBE 37 MicroCAT) and chlorophyll data (measured by a C3TM Submersible Fluorometer) was collected at approximately 1 m depth, taken from a coastal buoy located about 10 km off the mouth of the Itata River (Belmar et al., 2017). In late fall, when the river discharge was at a minimum and wind-driven upwelling has subsided, Chl-a was low (less than 200 RFU) and salinity was high (>33) (Figure 7). Winter storms caused an increase in river flow and episodes of low salinity in surface waters. Three weeks following the increase in base flow, the Chl-a exhibited a marked increase (>1000 RFU), indicating a delayed response of the phytoplankton population to nutrients availability. We also note that the maximum values of Chl-a during winter are of the same order of those observed in summer (Figure 7), when the region is exposed to strong upwelling, emphasizing the crucial role of the river in providing nutrients, thus increasing PB close to the river mouth.
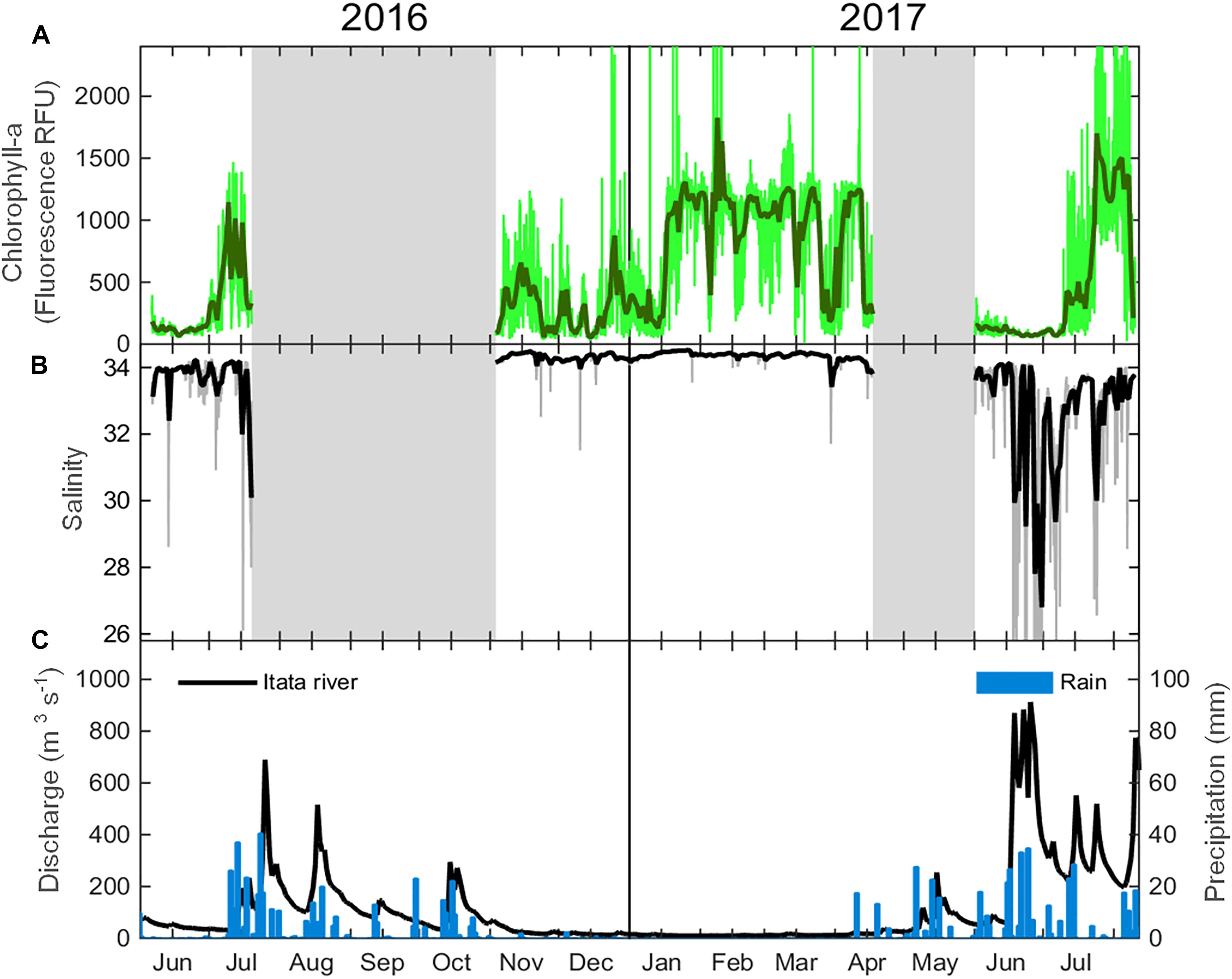
FIGURE 7. Daily mean (thick lines) and hourly (thin lines) variability of (A) phytoplankton biomass (as fluorescence of chlorophyll in RFU) and (B) salinity captured by POSAR, an automated monitoring buoy located at ∼10 km off the mouth of Itata River (see Figure 1), compared to (C) daily Itata river discharge (m3 s−1) and precipitation (mm) during 2016 to 2017 period (01 June 2016 to 10 August 2017).
Potential Impact of the River Plume on Coastal Ecological Processes
As showed by our results, these rivers can contribute to enhance biological activity in the coastal water off Central Chile. Although this contribution is small in comparison to that supplied by regional upwelling, they are probably sufficient to sustain the local ecosystem (e.g., larval survival and recruitment) during periods of minimum or delayed seasonal upwelling (winter and early spring). Significant levels of nutrient export from rivers over Central Chile is highly relevant to several larval fish taxa, as they present cross-shelf distribution, with an inner-shelf pattern concentrated mainly within >2 km offshore. For example, Helcogrammoides chilensis and Hypsoblennius sordidus larvae is observed in low abundances in slope waters (>10 km offshore) off the Chilean coast during winter and spring (Landaeta et al., 2008). Furthermore, river plumes have a direct influence on the development of rock and inter-tidal community (Vargas et al., 2006).
River influence is further appreciated when considering seasonal time scales, as the Chl-a pool (mean PB × plume area) in coastal areas near river mouths reach maximum levels during late winter and early spring (while wind-driven upwelling is low), coinciding with the larval recruitment of several fish (Castro et al., 2000; Landaeta et al., 2015). Previous studies in the Itata River have shown that high concentrations of Chl-a are accompanied by increased primary production in coastal waters (Salamanca and Pantoja, 2009). Moreover, it has been demonstrated that input from the Itata River, in particular during years of increased precipitation, can promote favorable environmental conditions for fish larvae and forage fish species (sardines and anchovies; Soto-Mendoza et al., 2010), as well as optimum conditions for growth and fish recruitment due to nutrient enriched waters (Grimes, 2001). Studies carried out in the Itata and Biobío Rivers suggest that river runoff and rainfall strongly influence local fish survival (e.g., Robalo, Eleginops maclovinus) during early life stages (Quiñones and Montes, 2001).
Future Projections
Model-based climate projections, under scenarios of high greenhouse gas emissions, consistently indicate a reduction in mean annual precipitation (up to 30% relative to current values) and an increase in surface air temperature (up to 2°C along the coast) over Central Chile for the 2070–2100 period (Fuenzalida et al., 2007; Bozkurt et al., 2017). It is expected that the rainfall decline will be most marked in winter due to the projected reduction in extratropical storms crossing this region. Given the small size of the Andean basins in central Chile and the previously found association between precipitation and river discharge (Vicuña et al., 2010; Bozkurt et al., 2017) and well correlated with nutrient exportation is probably expected to consistently decline over the 21st century, potentially causing detrimental impacts upon these river influenced coastal areas.
Studies elsewhere show that drought conditions are able to degrade coastal ecological processes. A decrease in diatoms and Chl-a biomass was associated with extremely low river flow during droughts in the Suisun Bay – San Francisco estuary and in the Neuse River estuary (Nichols, 1985; Lehman, 1996; Thompson et al., 2015). In the Neuse River estuary, droughts also caused significant shifts in the phytoplankton community, promoting cyanobacteria species (Paerl et al., 2010), as well as a decrease in phytoplankton productivity, directly associated with a decrease in nutrients (Wetz et al., 2011). Ecological studies in the San Francisco Bay demonstrate important changes in trophic webs under low river discharge, with the benthic community becoming more important with respect to the planktonic community, leading to negative consequences on fish populations in the estuary (Cloern et al., 1983; Nichols, 1985). The worst ever recorded red-tide event in the coastal waters of western Patagonia took place in summer/fall 2016, caused by an extensive bloom of Pseudochattonella sp. and Alexandrium catenella, was also connected with a very intense drought in southern Chile mediated by the marked reduction in fresh water input and subsequent alteration of the coastal hydrobiology (León-Muñoz et al., 2018).
This study shows that the recent mega-drought (2010–2014) has produced a consistent decrease in discharge (30–60% relative to historical values) and plume area (35 to 60%) for all the rivers considered within the study area (Figure 6). Furthermore, a reduction in phytoplankton biomass was observed in the coastal areas influenced by the Maipo and Mataquito rivers, situated in the northern (arid) sector of Central Chile (Figure 6). Given the intensity and duration of the mega-drought, it is considered to be an analog to future climate scenarios for this region (Fuenzalida et al., 2007; Bozkurt et al., 2017). Systematic evidence of mega-drought impacts upon coastal fish productivity still does not exist, however, it remains a priority to further investigate and quantify these impacts, considering the ongoing trend toward dry conditions (Boisier et al., 2016) and the persistence of this trend projected for the near future.
Author Contributions
IM, PA-R, LF, and RG designed the study. MY and LF carried out data analysis of in situ hydro-chemical data. IM, PA-R, and RG acquired and processed the remote-sensed MODIS-SeaWiFS ocean color images. LB contributed to data analysis of the coastal buoy POSAR. All authors provided contributions for writing and correcting the paper.
Funding
This research was funded by the Center of Excellence FONDAP-CONYCIT N° 15110009 and PA-R was supported by beca FIP UV/Doctorado. Coastal buoy POSAR was funded by FONDEQUIP-CONICYT project EQM140134.
Conflict of Interest Statement
The authors declare that the research was conducted in the absence of any commercial or financial relationships that could be construed as a potential conflict of interest.
Acknowledgments
PA-R acknowledges for support by the CID UV Centro de Observación Marino para Estudios de Riesgos del Ambiente Costero (COSTA-R). MY also acknowledges for support by the Fondecyt project 3150162. The authors thank NASA/GSFC Ocean Biology Processing Group and GlobColour – The European Service for Ocean Color for providing access to weekly L3 SeaWiFS and MODIS binned products. The authors also thank the Dirección General de Aguas (DGA) for nutrient and discharge data from fluviometric stations.
Supplementary Material
The Supplementary Material for this article can be found online at: https://www.frontiersin.org/articles/10.3389/fmars.2018.00423/full#supplementary-material
Footnotes
References
Aceituno, P., and Vidal, F. (1990). Variabilidad interanual en el caudal de ríos andinos en Chile central en relación con la temperatura de la superficie del mar en el Pacífico central. Revista de la Sociedad Chilena de Ingeniería Hidráulica 5, 7–19.
Belmar, L., Garreaud, R., and Farías, L. (2017). “Plataforma de Observación del Sistema Acoplado Océano Atmósfera (POSAR): un sistema de observación de alta frecuencia en la zona costera de Chile central,” in Proceedings of the XXXVII Congreso de Ciencias del Mar 2017, Valparaíso, CL.
Boisier, J. P., Rondanelli, R., Garreaud, R. D., and Muñoz, F. (2016). Anthropogenic and natural contributions to the Southeast Pacific precipitation decline and recent megadrought in central Chile. Geophys. Res. Lett. 43, 413–421. doi: 10.1002/2015GL067265
Bozkurt, D., Rojas, M., Boisier, J. P., and Valdivieso, J. (2017). Climate change impacts on hydroclimatic regimes and extremes over Andean basins in central Chile. Hydrol. Earth Syst. Sci. Discuss doi: 10.5194/hess-2016-690
Castro, L. R., Salinas, G. R., and Hernàndez, E. H. (2000). Environmental influences on winter spawning of the anchoveta Engraulis ringens off central Chile. Mar. Ecol. Prog. Ser. 197, 247–258. doi: 10.3354/meps197247
Cloern, J. E., Alpine, A. E., Cole, B. E., Wong, R. L. J., Arthur, J. F., and Ball, M. D. (1983). River discharge controls phytoplankton dynamics in northern San Francisco Bay estuary. Estuar. Coast. Shelf Sci. 16, 415–429. doi: 10.1016/0272-7714(83)90103-8
Cohn, T. A. (2005). Estimating contaminant loads in rivers: an application of adjusted maximum likelihood to type 1 censored data. Water Resour. Res. 41:W07003. doi: 10.1029/2004WR003833
Cotrim, da Cunha, L., Buitenhuis, E. T., Le Quéré, C., Giraud, X., and Ludwig, W. (2007). Potential impact of changes in river nutrient supply on global ocean biogeochemistry. Global Biogeochem. Cycles 21:GB4007. doi: 10.1029/2006GB002718
Fuenzalida, H., Aceituno, P., Falvey, M., Garreaud, R., Rojas, M., and Sánchez, R. (2007). “Study on Climate Variability for Chile during the 21st century,” in Proceedings of the Technical Report of the National Environmental Committee, Santiago, CL.
Garreaud, R., Alvarez-Garreton, C., Barichivich, J., Boisier, J. P., Christie, D., Galleguillos, M., et al. (2017). The 2010-2015 mega drought in Central Chile: Impacts on regional hydroclimate and vegetation. Hydrol. Earth Syst. Sci. 2017, 1–37. doi: 10.5194/hess-2017-191
Hickey, B. M., and Banas, N. S. (2003). Oceanography of the U.S. Pacific Northwest coastal ocean and estuaries with application to coastal ecology. Estuaries 26, 1010–1031. doi: 10.1007/BF02803360
Hickey, B. M., Kudela, R. M., Nash, J. D., Bruland, K. W., Peterson, W. T., MacCready, P., et al. (2010). River influences on shelf ecosystems: introduction and synthesis. J. Geophys. Res. 115:C00B17. doi: 10.1029/2009JC005452
Hopkins, J., Lucas, M., Dufau, C., Sutton, M., Stum, J., Lauret, O., et al. (2013). Detection and variability of the Congo River plume from satellite derived sea surface temperature, salinity, ocean colour and sea level. Remote Sens. Environ. 139, 365–385. doi: 10.1016/j.rse.2013.08.015
Hopkinson, C. S., and Vallino, J. J. (2005). Efficient export of carbon to the deep ocean through dissolved organic carbon. Nature 433, 142–145. doi: 10.1038/nature03191
Hu, C., Lee, Z., and Franz, B. (2012). Chlorophyll a algorithms for oligotrophic oceans: a novel approach based on three-band reflectance difference. J. Geophys. Res. 117, 1–25. doi: 10.1029/2011jc007395
Iriarte, J. L., Vargas, C. A., Tapia, F. J., Bermúdez, R., and Urrutia, R. E. (2012). Primary production and plankton carbon biomass in a river-influenced upwelling area off Concepción. Chile. Prog. Oceanogr. 92–95, 97–109. doi: 10.1016/j.pocean.2011.07.009
Landaeta, M. F., Contreras, J. E., Bustos, C. A., and Muñoz, G. (2015). Larval growth of two lanternfishes at nearshore waters from an upwelling zone based in the otolith microstructure analysis. J. Appl. Ichthyol. 31, 106–113. doi: 10.1111/jai.12639
Landaeta, M. F., Veas, R., Letelier, J., and Castro, L. R. (2008). Larval fish assemblages off central Chile upwelling ecosystem. Rev. Biol. Mar. Oceanogr. 43, 569–584. doi: 10.4067/S0718-19572008000300016
Lara, A., Solari, M. E., Prieto, M. R., and Peña, M. P. (2012). Reconstrucción de la cobertura de la vegetación y uso de suelo hacia 1550 y sus cambios a 2007 en la ecorregióın de los bosques valdivianos lluviosos de Chile (35° - 43° 30’ S). Bosque 33, 13–23. doi: 10.4067/S0717-92002012000100002
Lehman, P. W. (1996). “Changes in chlorophyll a concentration and phytoplankton community composition with water-year type in the upper San Francisco Bay estuary,” in San Francisco Bay: The Ecosystem. Pacific Division, American Association for the Advancement of Science, ed. J. T. Hollibaugh (San Francisco, CA: Bay), 351–374.
Léniz, B., Vargas, C. A., and Ahumada, R. (2012). Characterization and comparison of microphytoplankton biomass in the lower reaches of the Biobío River and the adjacent coastal area off Central Chile during autumn-winter conditions. Lat. Am. J. Aquat. Res. 40, 847–857. doi: 10.3856/vol40-issue4-fulltext-3
León-Muñoz, J., Urbina, M., Garreaud, R., and Iriarte, J. L. (2018). Hydroclimatic conditions trigger record harmful algal bloom in western Patagonia (summer 2016). Sci. Rep. 8:1330. doi: 10.1038/s41598-018-19461-4
Lihan, T., Mustapha, M. A., Rahim, S. A., Saitoh, S., and Iida, K. (2011). Influence of River plume on variability of chlorophyll a concentration using satellite images. J. Appl. Sci. 11, 484–493. doi: 10.3923/jas.2011.484.493
Lohrenz, S. E., Redalje, D. G., Cai, W.-J., Acker, J., and Dagg, M. (2008). A retrospective analysis of nutrients and phytoplankton productivity in the Mississippi River plume. Cont. Shelf Res. 28, 1466–1475. doi: 10.1016/j.csr.2007.06.019
Luzio, W., Casanova, M., and Seguel, O. (2010). Suelos de Chile. Santiago de Chile, CL: Universidad de Chile.
Mallin, M. A., Paerl, H. W., Rudek, J., and Bates, P. W. (1993). Regulation of estuarine primary production by watershed rainfall and river flow. Mar. Ecol. Prog. Ser. 93, 199–203. doi: 10.3354/meps093199
Montecino, V., Strub, P. T., Chavez, F., Thomas, A., Tarazona, J., Baumgartner, T., et al. (2006). “Chapter 10, Bio-physical interactions off western South America,” in The Coastal Ocean: Interdisciplinary Regional Studies and Synthesis, Vol. 14A, ed. A. R. Robinson (Cambridge: Harvard University Press), 329–390.
Montecinos, A., Kurgansky, M. V., Muñoz, C., and Takahashi, K. (2011). Non-ENSO interannual rainfall variability in central Chile during austral winter. Theor. Appl. Climatol. 106, 557–568. doi: 10.1007/s00704-011-0457-1
Morey, S. L., Dukhovskoy, D. S., and Bourassa, M. A. (2009). Connectivity of the Apalachicola River flow variability and the physical and bio-optical oceanic properties of the northern West Florida Shelf. Cont. Shelf Res. 29, 1264–1275. doi: 10.1016/j.csr.2009.02.003
Nezlin, N. P., DiGiacomo, P. M., Stein, E. D., and Ackerman, D. (2005). Stormwater runoff plumes observed by SeaWifs radiometer in the Southern California Bight. Remote Sens. Environ. 98, 494–510. doi: 10.1016/j.rse.2005.08.008
Nichols, F. H. (1985). Increased benthic grazing: an alternative explanation for low phytoplankton biomass in northern San Francisco Bay during the 1967-1977 drought. Estuar. Coast. Shelf Sci. 21, 379–388. doi: 10.1016/0272-7714(85)90018-6
O’Reilly, J. E., Maritorena, S., Siegel, D., O’Brien, M. C., Toole, D., Mitchell, B. G., et al. (2000). “SeaWiFS Postlaunch Calibration and Validation Analyses, Part 3,” in NASA Tech. Memo, TM-2000-206892, Vol. 11, eds S. B. Hooker and E. R. Firestone (Greenbelt, MD: NASA Goddard Space Flight Center), 49.
Otero, M. P., and Siegel, D. A. (2004). Spatial and temporal characteristics of sediment plumes and phytoplankton blooms in the Santa Barbara Channel. Deep Sea Res. II 51, 1129–1149. doi: 10.1016/j.dsr2.2004.04.004
Paerl, H. W., Rossignol, K. L., Hall, S. N., Peierls, B. L., and Wetz, M. S. (2010). Phytoplankton Community Indicators of Short- and Long-term Ecological Change in the Anthropogenically and Climatically Impacted Neuse River Estuary, North Caroline, USA. Estuaries Coast. 33, 485–497. doi: 10.1007/s12237-009-9137-0
Pauly, D., and Christensen, V. (1995). Primary production required to sustain global fisheries. Nature 374, 255–257. doi: 10.1038/374255a0
Pellicciotti, F., Burlando, P., and Van Vliet, K. (2007). “Recent trends in precipitation and streamflow in the Aconcagua River Basin, central Chile”, in Glacier Mass Balance Changes and Meltwater Discharge. Centre Ecol. Hydrol. 318, 17–38.
Pérez, C. A., DeGrandpre, M. D., Lagos, N. A., Saldías, G. S., Cascales, E.-K., and Vargas, C. A. (2015). Influence of climate and land use in carbon biogeochemistry in lower reaches of rivers in Central-Southern Chile: implications for the carbonate system in river-influenced rockyshore environments. J. Geophys. Res. Biogeosci. 120, 673–692. doi: 10.1002/2014JG002699
Pizarro, J., Vergara, P. M., Sanhueza, P. A., Rodríguez, J. A., and Castro, S. A. (2010). Nutrients dynamics in the main river basins of the centre-southern region of Chile. J. Hazard. Mater. 175, 608–613. doi: 10.1016/j.jhazmat.2009.10.048
Quiñones, R. A., and Montes, R. M. (2001). Relationship between freshwater input to the coastal zone and the historical landings of the benthic/demersal fish Eliginops maclovinus in central-south Chile. Fish. Oceanogr. 10, 311–328. doi: 10.1046/j.1365-2419.2001.00177.x
Rahn, D. A., and Garreaud, R. D. (2014). A synoptic climatology of the near-surface wind along the west coast of South America. Int. J. Climatol. 34, 780–792. doi: 10.1002/joc.3724
Runkel, R. L., Crawford, C. G., and Cohn, T. A. (2004). “Load estimator (LOADEST): a fortran program for estimating constituent loads in streams and rivers,” in Proceedings of the Techniques and Methods Book 4, Chapter A5, ed. U.S. Geological Survey (Reston, VA: Geological Survey), 75.
Salamanca, M. A., and Pantoja, S. (2009). ““Caracterización química en la zona marina adyacente a la desembocadura del río Itata”,” in La cuenca hidrográfica del río Itata. Aportes Científicos para su gestión sustentable, eds O. Parra, J. C. Castilla, H. Romero, R. Quiñones, and A. Camaño (Concepción, CL: Editorial Universidad de Concepción), 177–191.
Saldías, G. S., Largier, J. L., Mendes, R., Pérez-Santos, I., Vargas, C. A., and Sobarzo, M. (2016). Satellite-measured interannual variability of turbid river plumes off central- southern Chile: spatial patterns and the influence of climate variability. Prog. Oceanogr. 146, 212–222. doi: 10.1016/j.pocean.2016.07.007
Saldías, G. S., Sobarzo, M., Largier, J., Moffat, C., and Letelier, R. M. (2012). Seasonal variability of turbid river plumes off central Chile based on high-resolution MODIS imagery. Remote Sens. Environ. 123, 220–233. doi: 10.1016/j.rse.2012.03.010
Soto-Mendoza, S., Castro, L. R., and Llanos-Rivera, A. (2010). Variabilidad espacial y temporal de huevos y larvas de Strangomera bentincki y Engraulis ringens, asociados a la desembocadura del río Itata. Chile Rev. Biol. Mar. Oceanogr. 45, 471–487. doi: 10.4067/S0718-19572010000300012
Testa, G., Masotti, I., and Farias, L. (2018). Temporal variability in net primary production in an upwelling area off central Chile (36°S). Front. Mar. Sci. 5:179. doi: 10.3389/fmars.2018.00179
Thiel, M., Macaya, E. C., Acuña, E., Arntz, W. E., Bastias, H., Brokordt, K., et al. (2007). The humboldt current system of northern and central Chile. Oceanogr. Mar. Biol. Annu. Rev. 45, 195–344. doi: 10.1201/9781420050943.ch6
Thomas, A. C., and Weatherbee, R. A. (2006). Satellite-measured temporal variability of the Columbia River plume. Remote Sens. Environ. 100, 167–178. doi: 10.1016/j.rse.2005.10.018
Thompson, P. A., O’Brien, T. D., Paerl, H. W., Peierls, B. L., Harrison, P. J., and Robb, M. (2015). Precipitation as a driver of phytoplankton ecology in coastal waters: a climatic perspective. Estuar. Coast. Shelf Sci. 162, 119–129. doi: 10.1016/j.ecss.2015.04.004
Tremblay, J. -È, Raimbault, P., Garcia, N., Lansard, B., Babin, M., and Gagnon, J. (2014). Impact of river discharge, upwelling and vertical mixing on the nutrient loading and productivity of the Canadian Beaufort Shelf. Biogeosciences 11, 4853–4868. doi: 10.5194/bg-11-4853-2014
Vargas, C. A., Narváez, D. A., Piñones, A., Navarrete, S. A., and Lagos, N. A. (2006). River plume dynamics influences transport of barnacle larvae in the inner shelf off central Chile. J. Mar. Biol. Ass. 86, 1057–1065. doi: 10.1017/S0025315406014032
Vicuña, S., Garreaud, R., and McPhee, J. (2010). Climate change impacts on the hydrology of a snow-melt driven basin in semiarid Chile. Clim. Change 105, 3–4.
Waylen, P. R., Caviedes, C. N., and Juricic, C. (1993). El Niño-Southern Oscillation and the surface hydrology of Chile: a window on the future? Can. Water Resour. J. 18, 425–441. doi: 10.4296/cwrj1804425
Wetz, M. S., Hutchinson, E. A., Lunetta, R. S., Paerl, H. W., and Taylor, J. C. (2011). Severe droughts reduce estuarine primary productivity with cascading effects on higher trophic levels. Limnol. Oceanogr. 56, 627–638. doi: 10.4319/lo.2011.56.2.0627
Wieters, E. A., Kaplan, D. M., Navarrete, S. A., Sotomayor, A., Largier, J., Nielsen, K. J., et al. (2003). Alongshore and temporal variability in chlorophyll a concentration in Chilean nearshore waters. Mar. Ecol. Prog. Ser. 249, 93–105. doi: 10.3354/meps249093
Wysocki, L. A., Bianchi, T. S., Powell, R. T., and Reuss, N. (2006). Spatial variability in the coupling of organic carbon, nutrients, and phytoplankton pigments in surface waters and sediments of the Mississippi River plume. Estuar. Coast. Shelf Sci. 69, 47–63. doi: 10.1016/j.ecss.2006.03.022
Keywords: drought, river discharge, nutrient export, phytoplankton biomass, satellite remote sensing, central Chile
Citation: Masotti I, Aparicio-Rizzo P, Yevenes MA, Garreaud R, Belmar L and Farías L (2018) The Influence of River Discharge on Nutrient Export and Phytoplankton Biomass Off the Central Chile Coast (33°–37°S): Seasonal Cycle and Interannual Variability. Front. Mar. Sci. 5:423. doi: 10.3389/fmars.2018.00423
Received: 22 June 2018; Accepted: 23 October 2018;
Published: 20 November 2018.
Edited by:
Alejandro A. Murillo, EMBL Heidelberg, GermanyReviewed by:
José Pinho, University of Minho, PortugalRiccardo Briganti, University of Nottingham, United Kingdom
Copyright © 2018 Masotti, Aparicio-Rizzo, Yevenes, Garreaud, Belmar and Farías. This is an open-access article distributed under the terms of the Creative Commons Attribution License (CC BY). The use, distribution or reproduction in other forums is permitted, provided the original author(s) and the copyright owner(s) are credited and that the original publication in this journal is cited, in accordance with accepted academic practice. No use, distribution or reproduction is permitted which does not comply with these terms.
*Correspondence: Italo Masotti, aXRhbG8ubWFzb3R0aUB1di5jbA==