- 1Posgrado en Ciencias del Mar y Limnología, Universidad Nacional Autónoma de México, Ciudad de México, Mexico
- 2Instituto de Ciencias del Mar y Limnología, Unidad Académica de Sistemas Arrecifales, Universidad Nacional Autónoma de México, Cancún, Mexico
Partial mortality (PM) is increasingly common in the Elkhorn coral Acropora palmata and, depending on the causative agent, is potentially lethal. The effects of PM on growth, reproduction and total lipid content in A. palmata were studied by sampling apparently healthy (AH) colonies in comparison with colonies showing signs of PM. Branch growth rates and lesion regeneration rates were estimated using monthly photographs over a four-month period prior to the summer spawning season. No differences were found in the growth rates of colonies with PM compared to AH colonies. The areas affected by PM did not regenerate during the period of the study. Colonization of the lesions by competing species and sediment cover were documented and did not show major changes. During the spawning season, percent fertilization, egg volume and embryonic development were evaluated for comparison between AH colonies and those with PM. Total lipids were also quantified in tissues from three branches per colony. Percentage fertilization was similar in both AH colonies and those with PM. Embryonic development was normal, regardless of proximity to the lesion borders. However, egg volume was significantly lower in PM colonies than in AH colonies. Lower lipid concentrations were found at the edges of the lesions and similar to those found at the growing edges of the branches. The lack of regeneration may be explained by the low lipid concentration, because the polyps adjacent to the lesion do not have an adequate energy budget as a result of the damage. This would also affect their ability to compete against organisms that colonize the site of the lesion, a distinct situation to the rapid regeneration rates characteristic of lesions due to physical injury of the colony. Therefore, we conclude that partial mortality in A. palmata affects the colony, inducing energetic stress due to both competition and decreased egg quality.
Introduction
Coral reefs are complex formations created by the accumulation of calcium carbonate deposited by animals and algae. They are considered to be the marine ecosystems with the widest biological diversity on the planet (Bellwood and Hughes, 2001; Hughes et al., 2017). However, because they need strict conditions for their formation (Kleypas et al., 1999; Muir et al., 2015) they are restricted to less than 0.1% of the ocean’s surface (Spalding and Grenfell, 1997). Despite this, they provide important ecological and economic services (Bell et al., 2013; Cooper et al., 2014; Mitra and Zaman, 2015; Spalding et al., 2017). Hermatypic corals are primarily responsible for the formation of coral reefs (Cairns et al., 2009). For Caribbean reefs, Acropora spp., specifically Acropora palmata is of great importance due to their ability to withstand high energy conditions, high growth rates, ability to produce fragments and regenerate as well as their three-dimensional architecture, which provides habitat for many species (Lirman, 2002).
Acropora palmata, like most hermatypic corals, lives in a symbiotic relationship with a dinoflagellate alga called Symbiodinium that contributes to its host coral products that are derived from photosynthesis, in exchange for protection from external conditions and a constant supply of inorganic nutrients from the efficient recycling of the coral’s metabolic waste (Davy et al., 2012; Pernice et al., 2012). The contribution of energy by the algae is more than the coral’s daily requirement, so the surplus carbon, mainly glucose, is transformed into triglycerides and waxes to form large energy reserves (Oku et al., 2003). These energy reserves allow the coral to maintain its high productivity and sustain metabolic processes such as growth and sexual reproduction (Edmunds and Davies, 1986; Grottoli et al., 2004).
Lipids are key energetic biomolecules for hermatypic corals in particular, representing up to 40% of their dry weight (Harland et al., 1993; Oku et al., 2003; Imbs, 2013), although this percentage can vary in terms of environmental conditions such as light intensity and water temperature, as well as the coral’s nutritional and health status (Harland et al., 1992; Oku et al., 2002). Under normal circumstances there is a balance between the energy that is produced and that which is consumed (Grottoli et al., 2004), however, under stressful conditions this balance can be broken, because the energy reserves, in addition to supporting growth and reproduction, are also the source of energy to combat the consequences of the stress (Seemann et al., 2013). Lipids represent up to 40% of coral tissue dry weight and, as such, are the primary energy reserve, therefore decreases or increases in the concentrations of total lipids are useful indicators of the use or accumulation, respectively, of these reserves (Stimson, 1987; Ward, 1995; Oku et al., 2002, 2003; Grottoli et al., 2004; Imbs, 2013; Lin et al., 2013; Conlan et al., 2017, 2018). For this reason, lipid concentrations are considered to be a good indicator of coral stress.
Corals can suffer injuries or lesions that are caused by events such as non-lethal diseases, predation, high or prolonged sedimentation or as a result of physical damage caused by various types of disturbances such as hurricanes or tropical storms (Lewis, 1997; Meesters et al., 1997; Wesseling et al., 2001; Cantin and Lough, 2014). Coral bleaching, whereby the symbiotic algae leave or are expelled from the coral tissue, can also result in lesions on the colony, especially if the bleaching event is prolonged (Gleason, 1993; Jones, 2008). Corals have mechanisms that respond immediately to an injury, which initiate the regeneration of the skeleton and tissue in the damaged area. The regeneration process consumes large amounts of energy, involving all the adjacent polyps up to 15 cm around the lesion (Oren et al., 2001; Klingenberg, 2008, 2014; Cocito and Sgorbini, 2014). On occasion, the regeneration process cannot cover the entire lesion, probably due to various factors such as the origin, shape, and size of the lesion relative to colony size, environmental conditions, and the high energy cost involved (Bak and Steward-Van Es, 1980; Oren et al., 1997, 2001; Denis et al., 2013). Consequently, the area becomes covered by colonizing organisms or sediment and the lesions become permanent, becoming what is known as partial mortality.
Corals are modular organisms and can survive partial tissue loss (Oren et al., 2001). However, because recovery from partial mortality represents a major expenditure of energy for the coral, in an effort to maintain colony integrity, it may interrupt other vital processes that require energy reserves such as reproduction and growth (Oren et al., 1997, 2001). Many studies have determined the effects of partial mortality on coral energy reserves focusing on reproduction (Kojis and Quinn, 1985; Rinkevich and Loya, 1989; Van Veghel and Bak, 1994; Oren et al., 2001; Graham and van Woesik, 2013; Riegl and Purkis, 2015), and growth (Bak, 1983; Fang et al., 1989; Guzmán et al., 1994; Meesters et al., 1994; Lirman, 2000; Alvarado and Acosta, 2009) while regeneration is active. To our knowledge, no studies have documented the effects, if any, once regeneration has stopped and partial mortality is permanent. This is relevant to the coral’s energy budget if these lesions represent an area of high energy demand, i.e., the amount of lipids near the lesion is less than that in areas at a distance from the lesion. In this case, areas of partial mortality could continue to be a drain on the coral’s energy and negatively affect other processes, such as growth and reproduction. Therefore, the objective of this contribution is to evaluate if there is a prolonged effect of partial mortality on the energy budget and its possible implications on branch growth and egg size, as well as embryonic development in the coral A. palmata, by comparing the growing edge of apparently healthy colonies to the borders of the lesions of colonies with partial mortality.
Materials and Methods
Study Area
Coral tissue samples were collected from three sites, all located within the Puerto Morelos Reef National Park, Mexico (Figure 1). Growth measurements were also conducted in the same three sites whereas gamete bundles were collected only from Limones Reef. The northern site (20° 52′ 25.02″ N, 86° 51′ 4.67″ W) was located in Limones Reef, the central site (20° 55′ 4.01″ N, 86° 49′ 46.91″ W) was located in Cuevones Reef, and the southern site (20° 59′ 18.74″ N, 86° 47′ 26.66″ W) was located at “La Bocana” Reef. The sites were chosen based on the abundance of A. palmata and the presence of partial mortality (Banaszak and Álvarez-Filip, 2014).
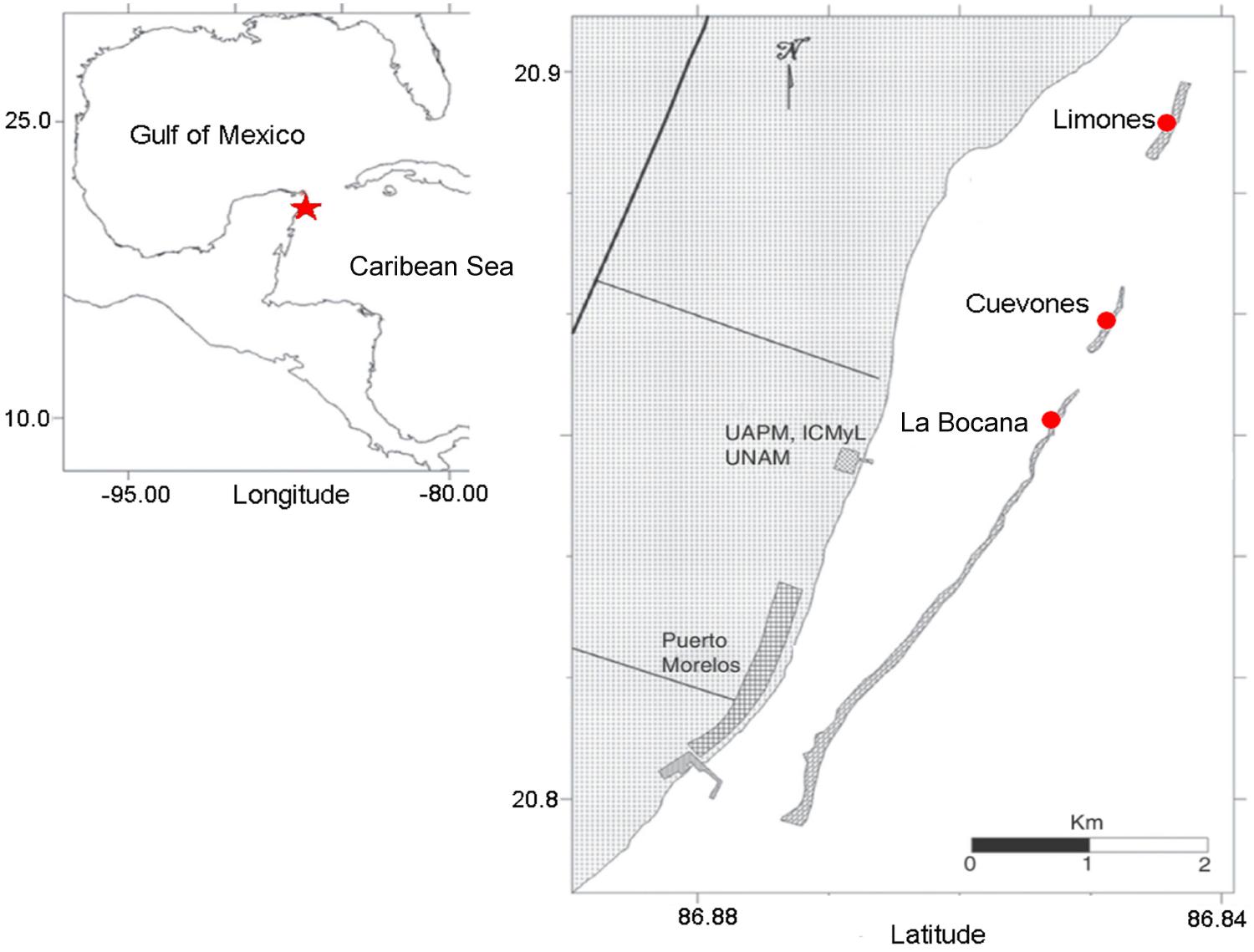
FIGURE 1. The study area located in the Western Atlantic at Puerto Morelos Reef National Park, Mexico. The star denotes the location of the National Park and the circles show the location of the three study sties.
The study was carried out prior to and during the A. palmata spawning season from March to August 2016 and again during the spawning season in 2017. A total of 16 colonies were used in the study: three colonies of apparently healthy A. palmata (AH) and three colonies with partial mortality (PM) were selected from the Cuevones and Limones sites and two of each category from the La Bocana site, due to the smaller number of healthy colonies at that site. All colonies were tagged and ranged between 80 and 100 cm maximum diameter to ensure that the colonies were of reproductive size (Bruckner and Hourigan, 2000).
Growth Measurements
Three branches from each of the 16 A. palmata colonies were selected for the measurement of surface growth rates (defined as changes in surface area). A 1 cm diameter PVC disk was glued onto each of the branches at 5 cm from the apical limit to serve as a reference point (Figure 2). To document changes in surface area, ten photographs of each branch were taken at monthly intervals for four consecutive months using a Nikon Coolpix AW130 digital camera attached to an aluminum support that fitted over the disk. These photographs were used to calculate changes in the surface areas, from the disk to the growth edge, in branches unaffected by partial mortality as well as branches affected by partial mortality. Benthic composition and cover by organisms colonizing the lesion space were also documented from the photographs. The latter was based on the Caribbean Coastal Marine Productivity method (CARICOMP, 2001).
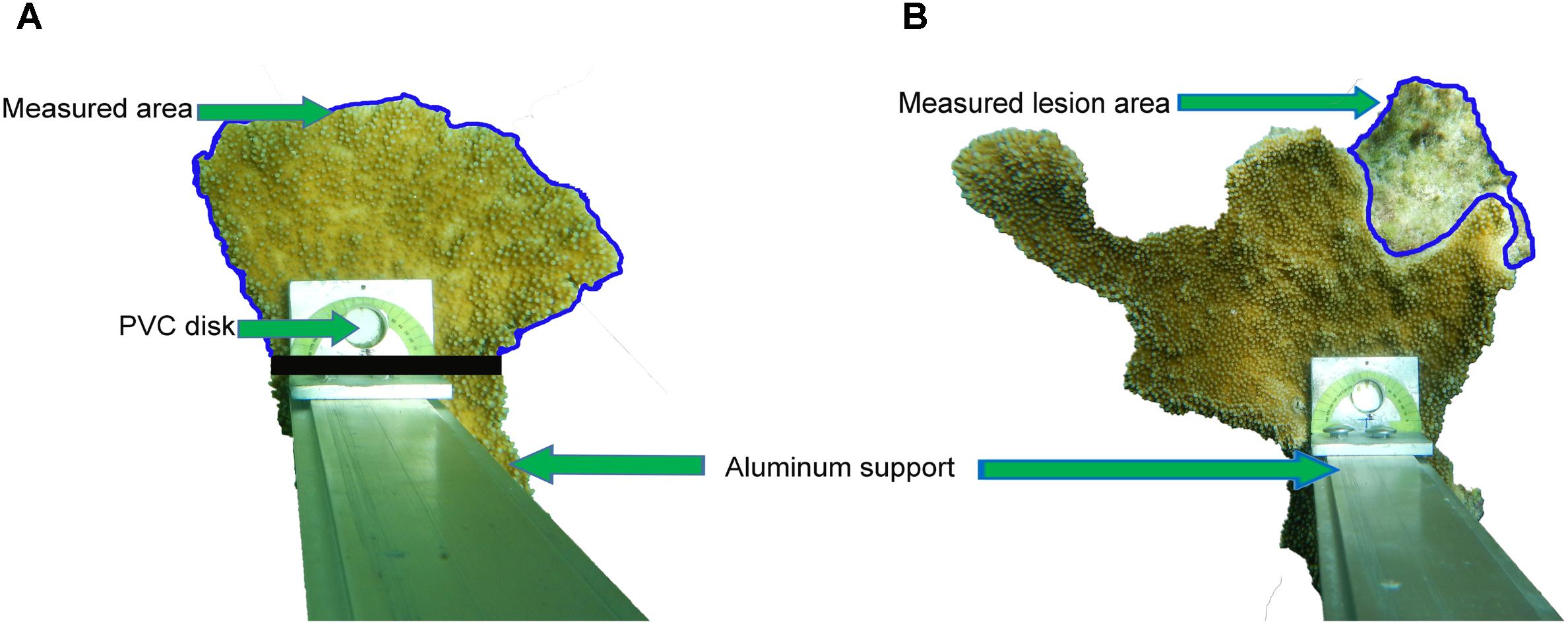
FIGURE 2. The technique for the measurement of surface growth rates of Acropora palmata branches is shown. A camera was attached to an aluminum support that was fixed to a PVC disk. The blue outline indicates the area measured in an apparently healthy colony (A) and in (B) the blue outline indicates the measured area of a lesion in a colony with old partial mortality. The measured area of healthy tissue in colonies with old partial mortality were measured as in (A).
Developmental Anomalies
Gamete samples were collected only at Limones Reef due to logistical reasons. Specially designed collecting nets, approximately 1.5 m high and 90 cm in maximum diameter, were used to obtain gamete bundles. The bundles were transported to the laboratory where cross-fertilizations were carried out with the first samples taken 1 h after the first division was observed to calculate fertilization rates. Egg size was determined using Adobe Photoshop CS5 Extended v12.0 on digital photographs taken using an optical microscope (Leica DM500) at 100 times magnification. Further samples were taken every hour during the 4 h following the first embryonic division and then every 12 h until the planula larva stage was reached. The samples were photographed under an optical microscope (Leica DM500) at 100 times magnification. The anomalies present during development were evaluated and cataloged as malformations or delays in embryo development (López et al., 2012) at the four-cell stage.
Total Lipid Extraction and Determination
To determine the concentration of lipids in coral tissue, three samples of approximately 2 cm2 each were taken. The first sample was taken from the branch edge and subsequent samples were taken at 1.5 cm intervals toward the branch base in AH individuals of A. palmata. For the colonies with PM, the first sample was taken at the edge of the lesion and at 1.5 cm intervals toward the edge of the branch. All samples were taken 11 days before the A. palmata spawning event. The samples were frozen immediately in liquid nitrogen and stored at -80°C for further analysis.
Total lipid extraction was carried out using the methods proposed by Harland et al. (1991) and modified by Harland et al. (1993) and Grottoli-Everett and Kuffner (1995) using the coral tissue samples stored at -80°C. The samples were measured, photographed, and weighed before starting the extraction protocol. All the glassware used was washed two times with tap water, then with Milli-Q water and finally with chloroform before each extraction. A blank reference was run with each extraction cycle.
Data Analysis
Changes in surface area of each branch as well as the evaluation of anomalies and malformations in embryos were estimated using Adobe Photoshop CS5 Extended v12.0. All of the data were processed in R version 3.3.3 and normality and homoscedasticity tests were carried out to determine if the distribution of the data was normal. To compare monthly growth rates between AH and PM colonies a repeated measures ANOVA with Welch’s correction was performed to compare the growth rates of both conditions (AH and PM) for each month using a repeated measures ANOVA test. This is a robust test for heteroscedastic data without the need to apply transformations (Derrick et al., 2016). We calculated growth rates of the individual branches rather than using an average value per colony to avoid over- or under-estimation (Weisburd and Britt, 2014) and thus obtain reliable growth values. Differences in the measured areas between the first (March–April) and the last month (June–July) of measurements, differences in the percentage of anomalies, changes in colonizing organism percent cover and branch growth, both together and separated by site, were analyzed using the nonparametric Wilcoxon test.
The percentage fertilization rates and comparison of egg volumes of eggs produced by AH and PM corals were analyzed using the Student’s t-test with Welch’s correction. A Wilcoxon test was performed to evaluate differences in the percentage of anomalies in developing embryos. Total lipid results for the three sites were grouped due to low sample numbers, to increase statistical power even though it was likely that variability would be higher due to differences between sites. The Student’s t-test was used to analyze for differences in lipid concentrations between AH and PM colonies at each distance sampled (0–1 cm, 2.5–3.5 cm, and 5–6 cm). An ANOVA test with Welch’s correction was used to compare lipid concentrations between distances for each condition. The data are expressed as median ± median absolute deviation (MAD) due to their wide variability. The data, including outliers, are shown in box and whisker plots where the horizontal lines within the boxes indicate the median, the boxes indicate the second and third quartiles, and the whiskers denote the first and fourth quartiles.
Results
Area Occupied by Partial Mortality
The analysis of the 24 lesions on A. palmata colonies showed that they were maintained over time, with no significant differences between the first (March–April) and last (June–July) month of sampling (p = 0.190, power test = 95%). The total area of partial mortality per colony branch was 49.2 cm2 ± 4.45 in March–April 2016, which reduced to 38.8 cm2 ± 12.6 by June–July of the same year, however, the difference was not statistically significant (p = 0.190). The variability in the areas occupied by lesions was greater in June than in March.
Colonization of Lesions
The composition of colonizing organisms that occupied the areas of partial mortality in the A. palmata branches changed (Figure 3), although there were no statistically significant differences in percent cover (p > 0.05, power test ≥ 75%) over the four-month sampling period. The percent cover occupied by the dominant algal group presented trends that were not statistically significant. Turf algae decreased from 50.5% ± 10.5 to 39.3% ± 5.25 with a concurrent increase in fleshy macroalgae from 10% ± 7.5 to 20.25% ± 18.7. Percent cover occupied by sand did not change over the four-month period.
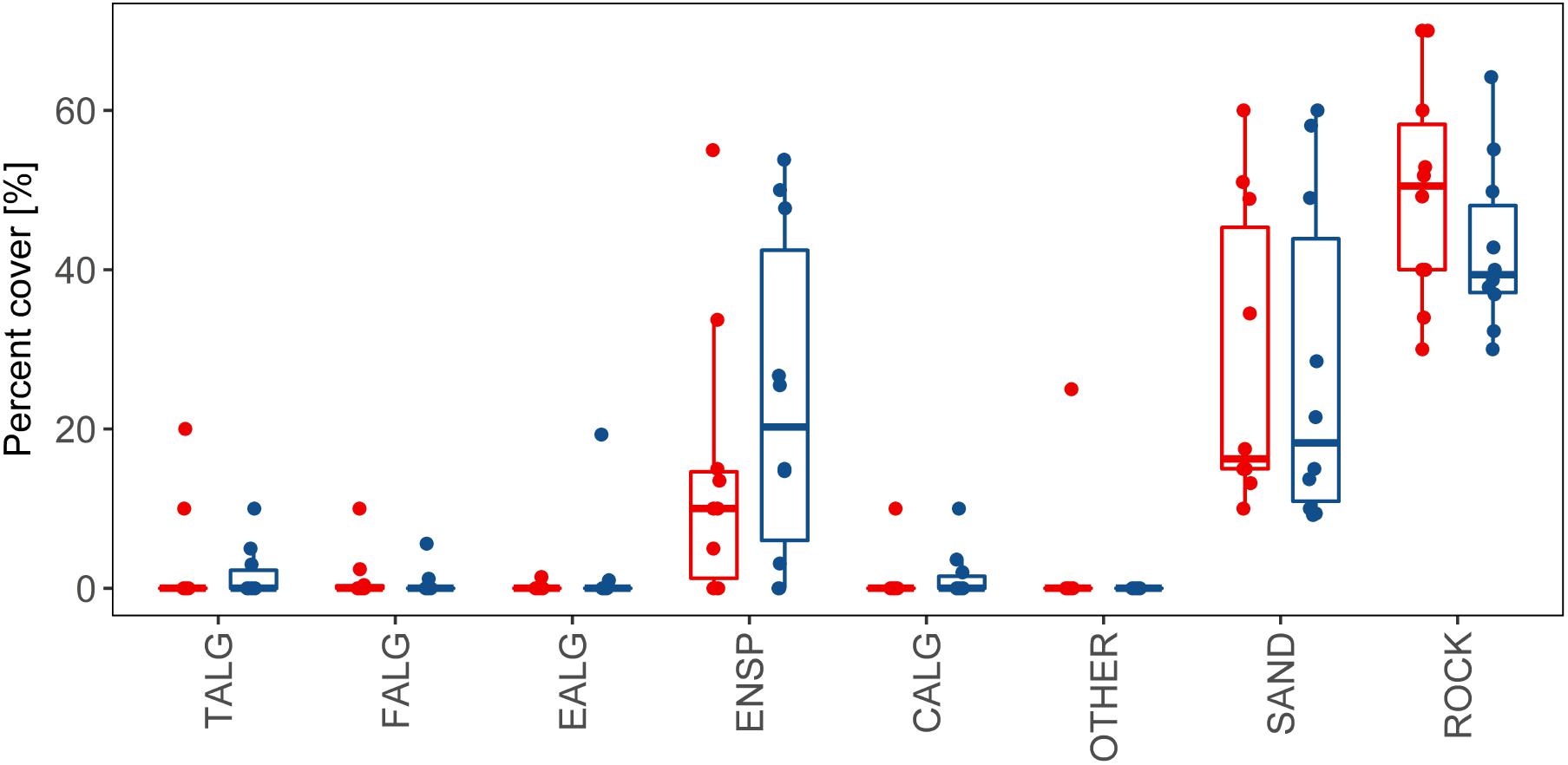
FIGURE 3. Percent cover of the categories of organisms that colonized or non-biological material that covered the areas of partial mortality in branches of the coral A. palmata during the months of March (red bars) and June (blue bars), 2016. Groupings: TALG, Turf algae; EALG, Encrusting algae; ENSP, Encrusting sponges; CALG, Calcareous algae; FALG, Fleshy macroalgae; SAND, Sand; Substrates: ROCK, Rock; OTHER, Other.
Branch Growth Rates
The variations in the surface growth rates of the branches were high at all sites (Figure 4). The lowest positive daily growth rate recorded during the four-month period was 0.01 cm2 day-1, registered in Limones Reef in May–June, whereas the highest was daily growth rate was seventy times higher at 0.7 cm2 day-1 in Limones Reef in April–May. Negative growth rates are due to the breakage of the branch during the previous month. Due to these large variations, we considered it more appropriate to analyse growth rates of the individual branches rather than using an average value per colony.
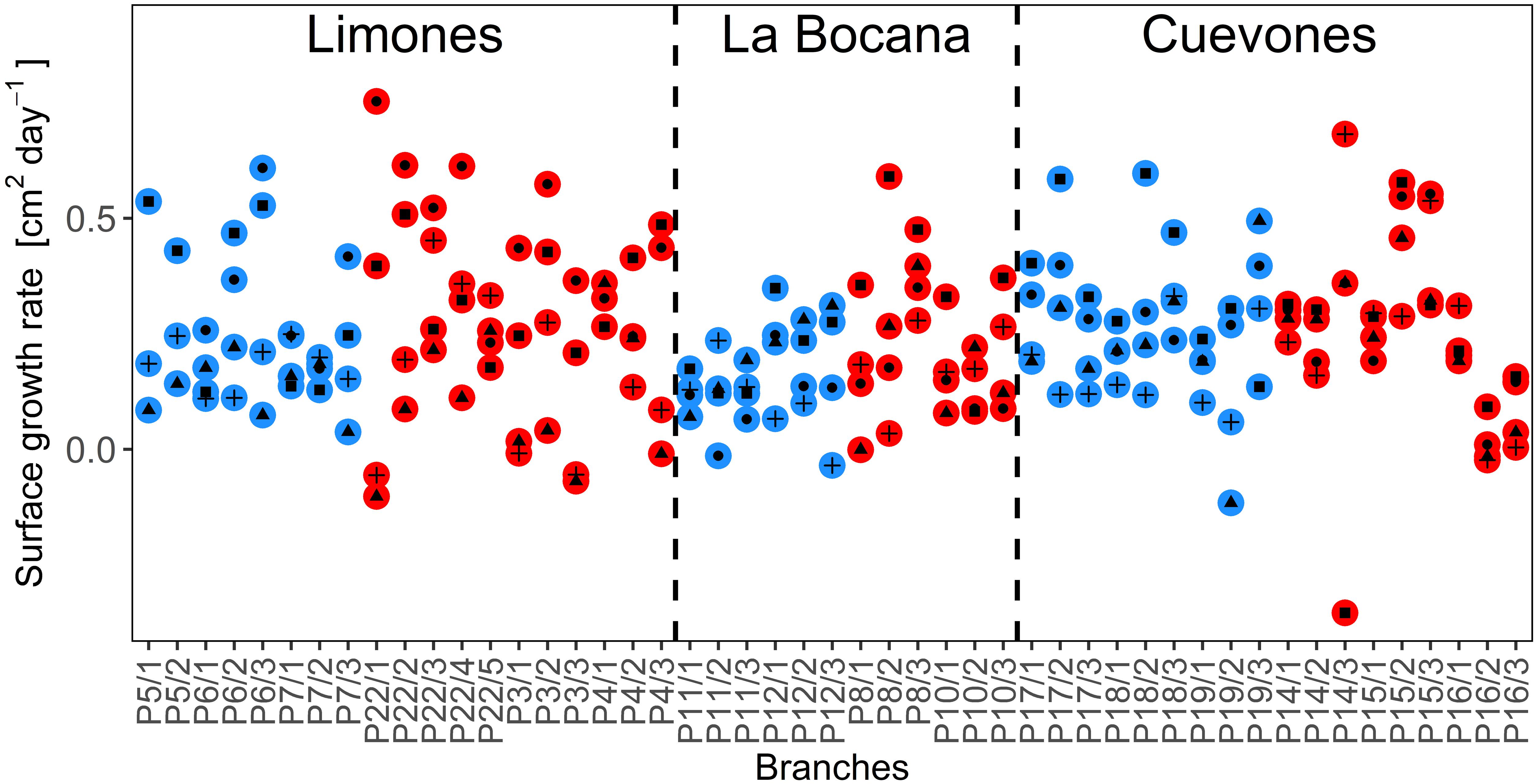
FIGURE 4. The surface growth rates (cm2 day-1) of each branch that was measured at the three sampling sites during the 4 months of the study, for apparently healthy (AH, blue circles) colonies and those with partial mortality (PM, red circles). Within the colored circles, black circles represent the rates for March, triangles for April, squares for May, and plus signs for June.
Analysis of the growth rates at each site (Figures 5A–C) indicates that there were no significant differences between the AH branches and the branches with PM, for the four-month period (p > 0.05) at any of the sites. However, significant monthly variation was found in the growth rates for both AH and PM colonies (p < 0.05, power test ≥ 95%) in Limones Reef between the months of April–May (0.14 cm2 day-1 ± 0.4) when compared with March–April (0.31 cm2 day-1 ± 0.8) for AH colonies, as well as for the months of March–April (0.43 cm2 day-1 ± 0.13) compared with June–July (0.16 cm2 day-1 ± 0.17) and April–May (0.08 cm2 day-1 ± 0.12). For colonies with PM, differences were found (P < 0.05) between May–June (0.32 cm2 day-1 ± 0.09) and April–May (0.08 cm2 day-1 ± 0.12). At Cuevones Reef, only the AH branches showed differences (p = 0.006) between the months of June–July (0.11 cm2 day-1 ± 0.02) when compared to May–June (0.32 cm2 day-1 ± 0.09). At La Bocana Reef, over the four-month period, there were no statistically significant changes detected. Finally, there were no significant differences in growth rates of AH branches relative to the branches with PM for any site. However, there is high variation in the growth rates of the branches over time.
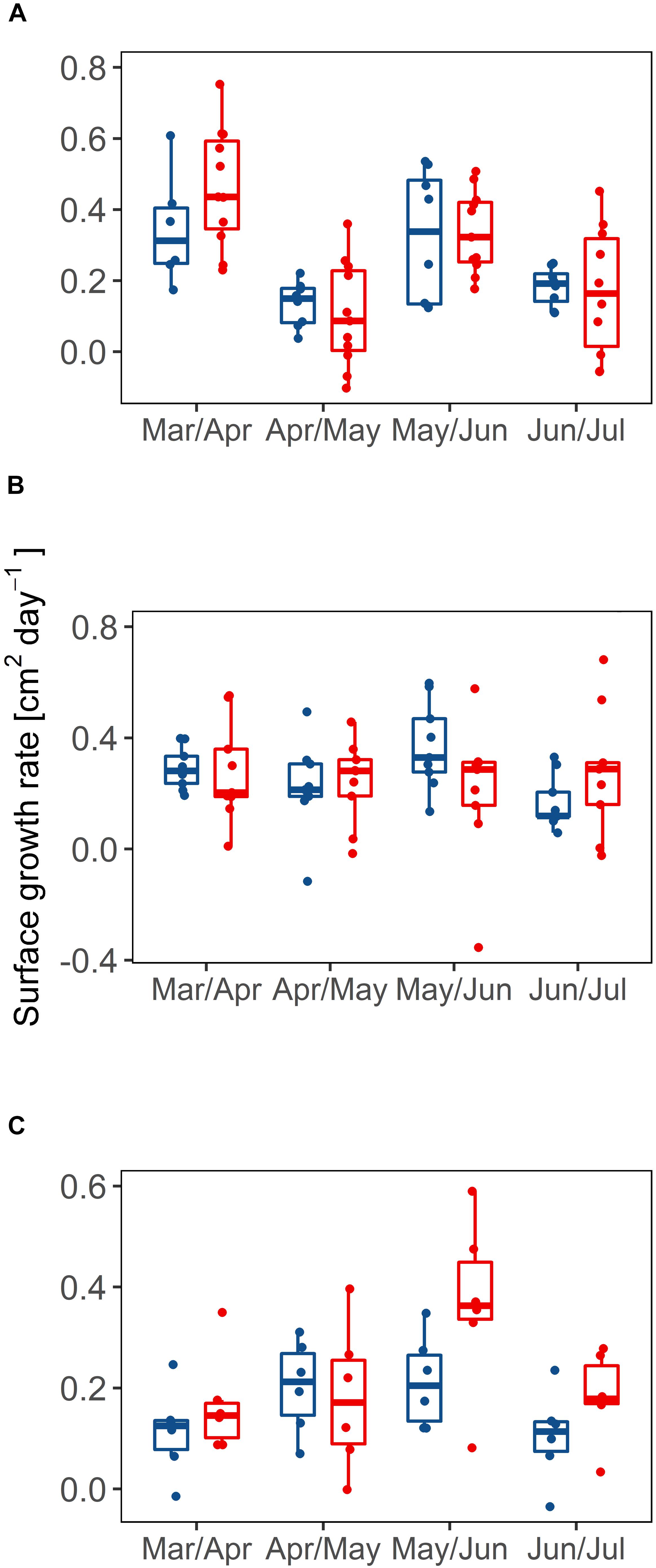
FIGURE 5. Surface growth rates (cm2 day-1) for branches measured in Limones Reef (A), Cuevones Reef (B) and La Bocana Reef (C). Shown are growth rates of branches with partial mortality (PM, red bars) vs. apparently healthy branches (AH, blue bars) throughout the months of March to June, 2016.
Gamete Quality
The first two-celled embryos were observed at 2.5 h after fertilization of the gametes collected from both AH colonies and colonies with PM. Fertilization rates were not significantly different (p > 0.05, power test = 89%) for samples collected from colonies with PM (median of 80.75% ± 0.54) when compared to samples from AH colonies (median of 71.45% ± 6.46). The fertilization percentage of AH corals shows a much wider variability in comparison to that observed in corals with PM (Figure 6A). The percentage of anomalies (Figure 6B), were variable and did not present significant differences (p > 0.05, power test = 35%) between the eggs of AH colonies (median of 14.98% ± 6.04) and those from colonies with PM (median of 15.55% ± 7.63). In terms of egg volume, the median volume of the eggs produced by AH colonies was 0.026 mm3 ± 0.005, while those produced by colonies with PM was 0.021 mm3 ± 0.005 (Figure 7). The eggs from AH colonies were significantly larger than those from colonies with PM (p < 0.05, power test = 99%).
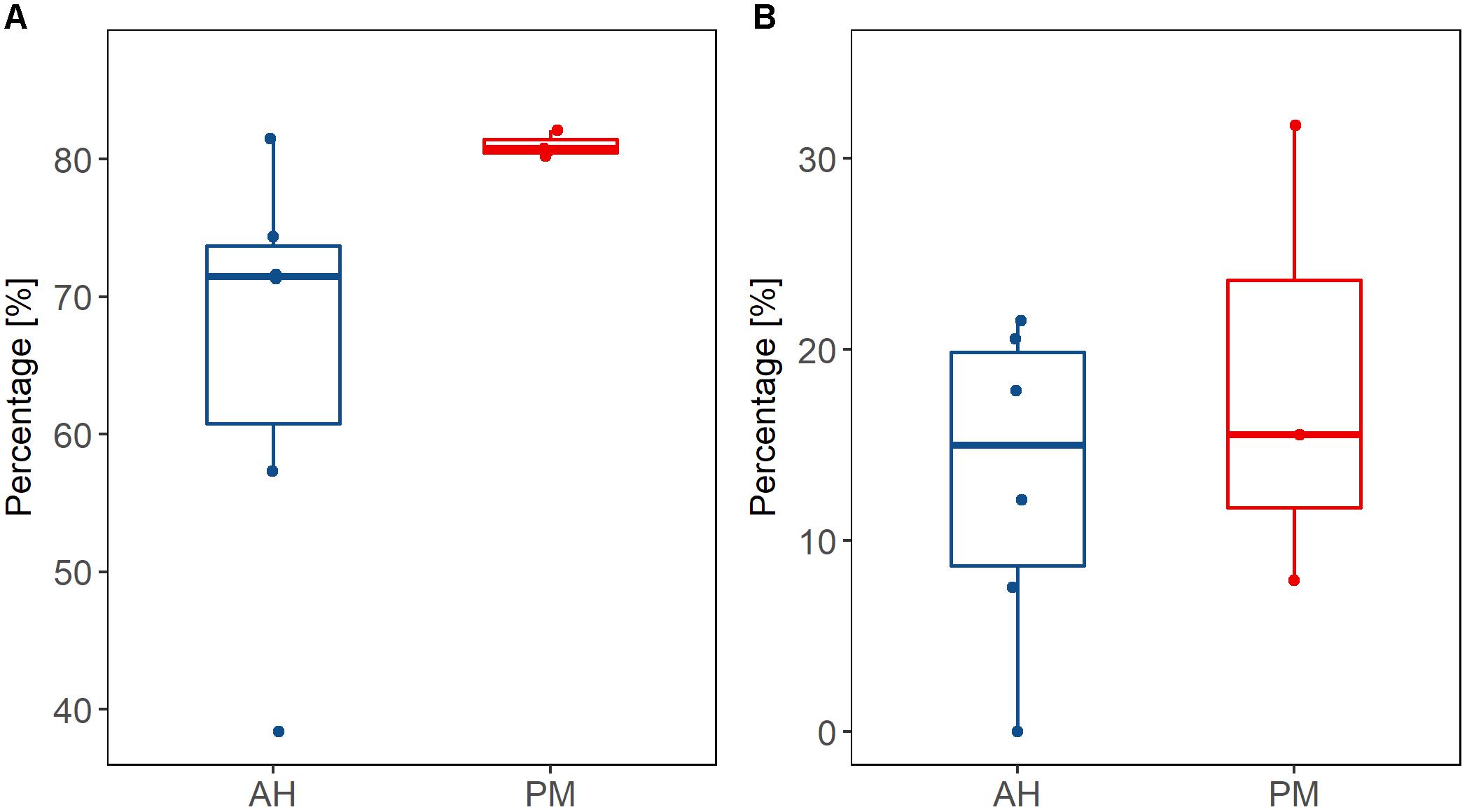
FIGURE 6. Percentage fertilization (A) and percentage of anomalies (B) of gametes collected from apparently healthy (blue bars) colonies vs. those obtained from colonies with partial mortality (red bars), collected from the Limones site.
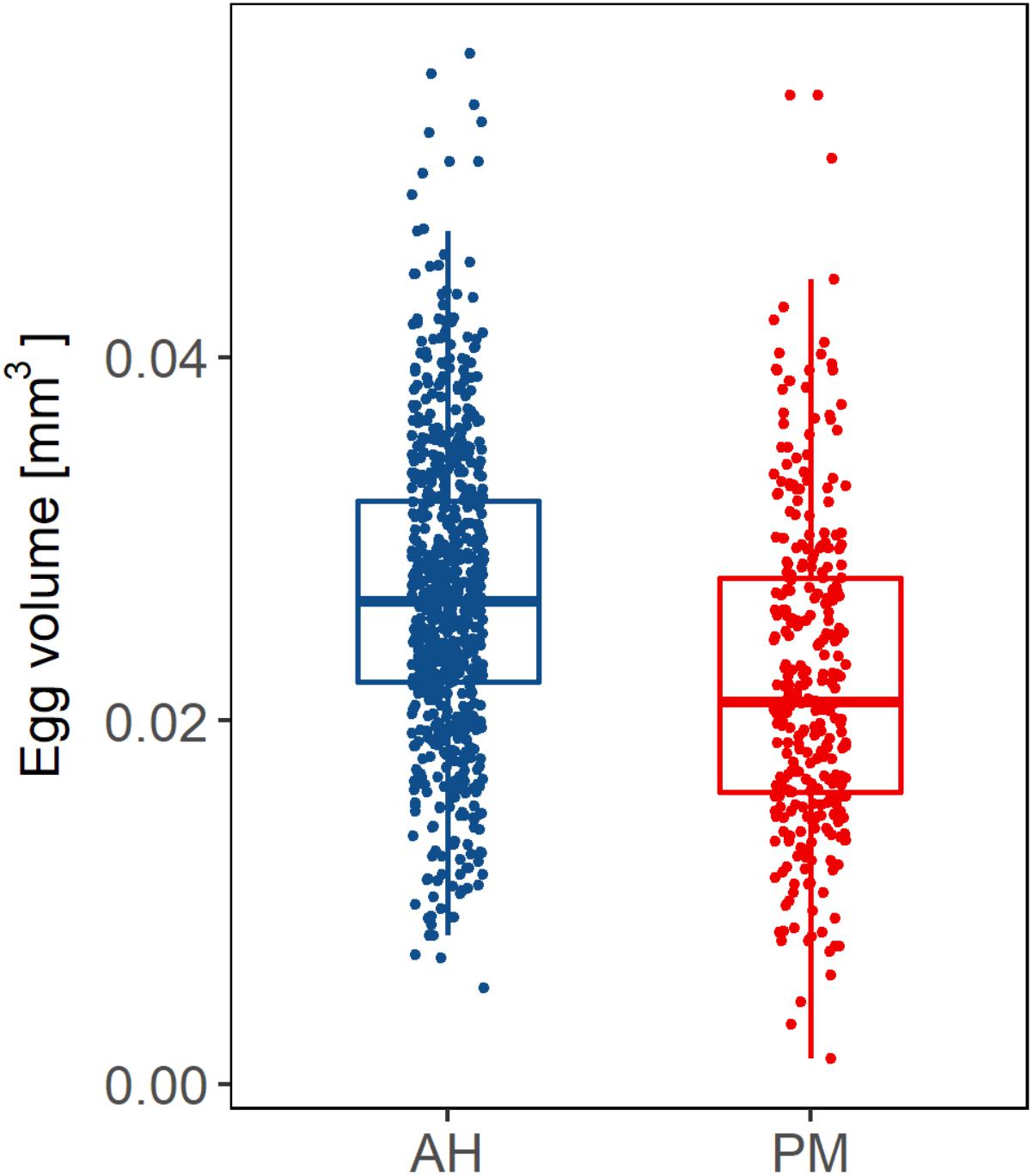
FIGURE 7. Volume of eggs produced by apparently healthy (blue bars) colonies vs. colonies with partial mortality (red bars) from the Limones site, collected during the spawning season in 2017.
Lipids in Tissues
The results of the total lipid analysis (Figure 8) showed that there were no significant differences in the amount of lipids between the branches with PM and the AH branches (p > 0.05, power test ≥ 30%). Of the colonies that were sampled, there are no significant differences in the amount of lipids at the lesion borders in colonies with PM when compared with the growth edges of AH colonies. This pattern holds for at least a 5 cm distance from the lesions in colonies with PM. On the other hand, the amount of lipids did vary significantly between sampling distances, both in the AH branches (p = 0.013, power test = 99%) and in the branches with PM (p = 0.01, power test = 99%). The post hoc multi-comparison test, after the ANOVA with Welch’s correction, showed that the coral tissue at distances of 2.5–3.5 cm and 5–6 cm from the edge did not contain significant differences (p > 0.05) in the amounts of total lipids. This held true for both conditions of colonies: AH and PM. However, at a distance of 0–1 cm from the growth edge in AH colonies and the lesion border for colonies with PM, there are significantly lower concentrations of lipids with respect to the other two distances (p < 0.05). The results show clear evidence of a low energy zone at the growth edge for AH colonies and at the lesion border for colonies with PM. From at least 2.5 to 3.5 cm distance from the growth edge of AH colonies and lesion border of colonies with PM, lipid concentrations increase.
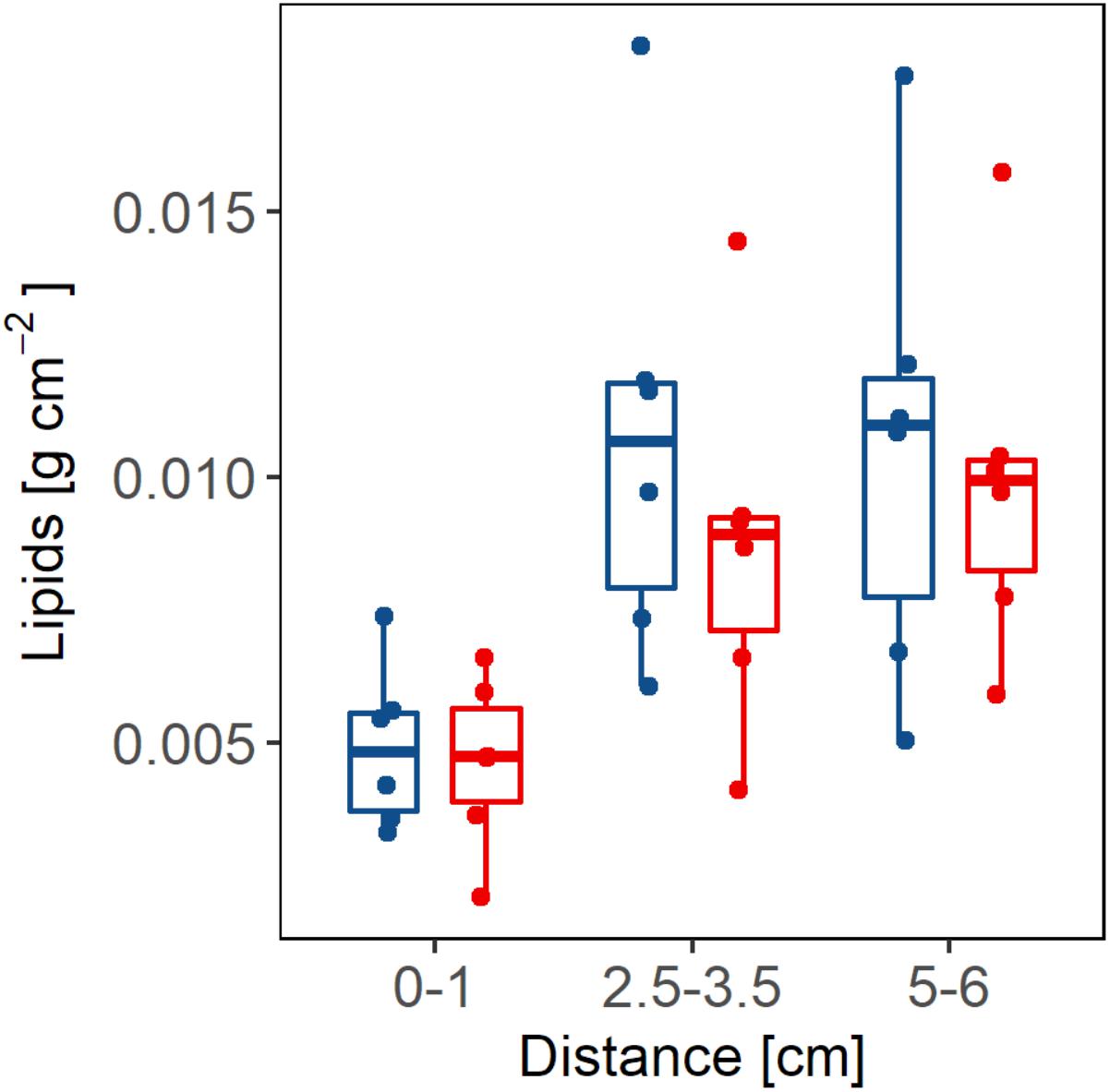
FIGURE 8. The concentration of lipids (g) per tissue area (cm2) is shown at different distances (cm) from the border of the lesion in branches with partial mortality (red bars) and growth edge in apparently healthy (blue bars) branches.
Discussion
Partial mortality in A. palmata colonies has a negative effect on their energy budget as shown by the results obtained in this study. The negative effects were detected in colonies with old partial mortality, where the lesions were completely colonized by other species or covered in sediment. Certain aspects of the reproduction biology of A. palmata were affected by the presence of old partial mortality, whereas colony branch growth appeared to be unaffected.
All studies to date, on the effects of partial mortality on reproduction and growth have only focused on the first stages of the injury or lesion when regeneration tends to be accelerated. Reported results differ widely ranging from a reduction in reproductive activity in Stylophora pistillata (Rinkevich and Loya, 1989) and massive Porites spp. (Welsh et al., 2015) and fecundity in Goniastrea favulus (Kojis and Quinn, 1985), to cases where the effects are localized and only affected up to a 15 cm radius around injured areas in Favia favus (Oren et al., 2001). Negative effects also vary depending on the morphotype of colonies of Montastrea annularis (Van Veghel and Bak, 1994). For A. palmata it has been reported that injured colonies completely stop gamete production (Lirman, 2000).
The area occupied by partial mortality in individuals of A. palmata did not change during the period of study, designed to coincide with the months leading up to the summer spawning season. No significant changes were found between the months of March–April and June–July (Figures 5A–C). This is likely explained by the fact that regeneration is a gradual process that decreases with time reaching the point where it stops, even when the injury has not completely regenerated. The most accepted explanation for this fact is that regeneration is stopped in order to not continue with the excessive energy expenditure caused by this process (Oren et al., 1997, 2001). However, the lipid results obtained in this study contradict this explanation because they show that even after the regeneration process was stopped, the lesions continue to represent areas of high energy demand, as reflected by the significantly lower lipid concentrations at the borders of the lesions (Figure 8).
Furthermore, our results show that the amount of lipids at the borders of the lesions does not differ from the lipid concentrations at the edges of maximum growth of the A. palmata branches. This would indicate that large amounts of energy are being consumed near the borders of the partial mortality, which is similar to the expense that supports the accelerated growth in the tips of the branches in A. palmata. Despite the possibility that there are variations in physico-chemical properties between the three sites, the edges of the zones with partial mortality consistently exhibited lower lipid concentrations (Figure 8) even when the data are separated by site.
Based on our results, we infer that the explanation for the arrest or reduction of regeneration and the low amount of lipids at the edges of the lesions (Figure 8) is produced by a possible competitive relationship induced by the organisms that colonize the injured areas, rather than a mechanism to stop energy expenditure. The space exposed by the lesions, as analyzed in this study, was dominated by filamentous algae (38%) and fleshy algae (20%), which are organisms that are characterized by engaging in competitive relationships with coral species by activating chemical mechanisms that allow them to maintain the space they have gained and, if possible, to overgrow the coral. Their very presence represents a physical obstacle that prevents the adequate regeneration of injured areas (Titlyanov et al., 2005, 2006) and promotes the accumulation of organic and inorganic sediment that in turn provides nutrients and support for algal development (Nugues and Roberts, 2003; Titlyanov and Titlyanova, 2008). This coincides with our results, because sediment, in addition to the algae, is the substrate that dominates the lesions with a median of around 20% (Figure 3). Therefore, the coral needs to activate defensive mechanisms, which require significant amounts of energy, and which would explain the results of the low amount of lipids at the borders of the lesions.
On the other hand, Oren et al. (1997, 2001) also mentions that excessive energy expenditure such as that which occurs when regeneration is active can affect other processes such as reproduction and growth since they depend on the same reserves. This energy expenditure has been estimated at about 33% of the total available energy in the coral Porites porites (Edmunds and Davies, 1986). Such a sizeable energy expenditure would affect processes such as growth and reproduction. Therefore, taking into account our results where it is shown that old lesions continue to be large energy sinks, one would expect that these negative effects would continue even after the regeneration stopped. However, our results showed that growth is not affected in corals with partial mortality in any of the three sites sampled (Figure 4), which is unusual and differs from previous studies. For example, Meesters et al. (1994) found that lesions in Montastrea annularis reduced the growth capacity of the coral even after regeneration was stopped. Bak (1983) found that branches of A. palmata that presented lesions that were within 25 cm of the growth edge of the branch had considerably lower linear extension rates. However, if the lesion was located at more than 25 cm from the growth edge, there was no negative effect on its growth (Bak, 1983). This is a plausible explanation for the results obtained in this work since the position of the lesions analyzed were greater than 25 cm from the growth edge.
As seen from the results presented here, the presence of old partial mortality did affect some aspects of the reproductive process in A. palmata colonies. These colonies were capable of producing and releasing gametes. However, the volume of the eggs released in gamete bundles by corals suffering from partial mortality was significantly lower than eggs released by corals that were apparently healthy (p = 8.52e-15). The eggs were almost 20% lower in terms of volume. This result could be due to the energy imbalance produced by partial mortality on the coral such that the eggs from colonies suffering from old injuries have lower lipid contents. These eggs with lower energy reserves would be at a disadvantage during embryonic development and long-distance dispersal in comparison to eggs produced by apparently healthy colonies with larger reserves. Decreased energy reserves have also been found in adult corals that have previously bleached (Jones and Berkelmans, 2011). Bleached colonies produce fewer eggs with lower lipid levels compared to healthy colonies (Ward et al., 2000; Sudek et al., 2012).
The difference in egg size did not affect the ability to be fertilized (Figure 6A). In fact, fertilization percentage was on average higher and much less variable in colonies with signs of partial mortality than the fertilization percentage from apparently healthy colonies. Another process that was not affected by the volume of the eggs was abnormalities in the developing embryos (Figure 6B). This would indicate then, that while smaller, the eggs produced by colonies suffering from partial mortality were of equivalent quality to the eggs produced by apparently healthy colonies. However, small egg size may have post-developmental repercussions such as reduced dispersal distances, ability to settle and colony growth.
With the results of this work we can conclude that partial mortality does affect the coral A. palmata and the negative effect is observed mainly as a constant energy expenditure, due to the process of competition against the colonizing organisms occupying the injured area, which in turn causes a decrease in the common energy reserves for other processes. Although the results show no negative effects on growth, we did detect a significant effect on the reproductive capacity of corals, specifically in the volume of the eggs, however, due to the low sample size used here and the large variability of this process more studies are needed to confirm these results. Finally, it is important to bear in mind that partial mortality is a condition that remains over time, consuming large amounts of energy and possibly weakening the coral, so that it is very likely that its resistance will decrease in the face of future stress events.
Author Contributions
VP-G and AB developed the concept for this study and wrote the manuscript. VP-G conducted the field work, collected the data, and undertook statistical analyses.
Funding
This study was funded by project number 608 from the Instituto de Ciencias del Mar y Limnología, Universidad National Autónoma de México as well as project numbers 153260 and 247765 from the Consejo Nacional de Ciencia y Tecnología (Mexico) to AB. VP-G was supported by a postgraduate fellowship from the Consejo Nacional de Ciencia y Tecnología (Mexico).
Conflict of Interest Statement
The authors declare that the research was conducted in the absence of any commercial or financial relationships that could be construed as a potential conflict of interest.
Acknowledgments
We thank Dr. Andrea Grottoli for providing helpful advice and protocols on lipid extractions.
References
Alvarado, E., and Acosta, A. (2009). Lesiones naturales y regeneración de tejido en ramets del coral Montastraea annularis (Scleractinia: Faviidae) en un arrecife degradado del Caribe Colombiano. Rev. Biol. Trop. 4, 939–954.
Bak, R. P. M. (1983). Neoplasia, regeneration and growth in coral reef-building Acropora palmata. Mar. Biol. 77, 221–227. doi: 10.1007/BF00395810
Bak, R. P. M., and Steward-Van Es, Y. (1980). Regeneration of superficial damage in the scleractinian corals Agaricia agaricites F. purpurea and Porites astreoides. Bull. Mar. Sci. 30, 883–887.
Banaszak, A. T., and Álvarez-Filip, L. (2014). Diagnóstico y estado de Conservación de las Poblaciones de Acropora palmata en el Parque Nacional Arrecife de Puerto Morelos. Final Report. Quintana Roo: PROCER/PNAPM02/01/2014 Concepto 9.2, 57.
Bell, J. D., Ganachaud, A., Gehrke, P. C., Griffiths, S. P., Hobday, A. J., Hoegh-Guldberg, O., et al. (2013). Mixed responses of tropical Pacific fisheries and aquaculture to climate change. Nat. Clim. Change 3, 591–599. doi: 10.1038/nclimate1838
Bellwood, D. R., and Hughes, T. P. (2001). Regional-scale assembly rules and biodiversity of coral reefs. Science 292, 1532–1534. doi: 10.1126/science.1058635
Bruckner, A. W., and Hourigan, T. F. (2000). “Proactive management for conservation of Acropora cervicornis and Acropora palmata: application of the U. S. Endangered Species Act,” in Proceedings 9th International Coral Reef Symposium, Bali, 23–27.
Cairns, S. D., Gershwin, L., Brook, F. J., Pugh, P., Dawson, E. W., Ocana, O., et al. (2009). “Phylum Cnidaria: corals, medusae, hydroids, myxozoans,” in New Zealand Inventory of Biodiversity, ed. D. P. Gordon (Christchurch: Canterbury University Press), 59–101.
Cantin, E. N., and Lough, J. M. (2014). Surviving coral bleaching events: Porites growth anomalies on the Great Barrier Reef. PLoS One 9:e88720. doi: 10.1371/journal.pone.0088720
CARICOMP (2001). Methods Manual Levels 1 and 2: Manual of Methods for Mapping and Monitoring of Physical and Biological Parameters in the Coastal Zone of the Caribbean. Kingston: CARICOMP Data Management Center University of the West Indies.
Cocito, S., and Sgorbini, S. (2014). Long-term trend in substratum occupation by a clonal, carbonate bryozoan in a temperate rocky reef in times of thermal anomalies. Mar. Biol. 161, 17–27. doi: 10.1007/s00227-013-2310-9
Conlan, J. A., Humphrey, C. A., Severati, A., and Francis, D. S. (2018). Intra-colonial diversity in the scleractinian coral, Acropora millepora: identifying the nutritional gradients underlying physiological integration and compartmentalised functioning. PeerJ 6:e4239. doi: 10.7717/peerj.4239
Conlan, J. A., Rocker, M. M., and Francis, D. S. (2017). A comparison of two common sample preparation techniques for lipid and fatty acid analysis in three different coral morphotypes reveals quantitative and qualitative differences. PeerJ 5:e3645. doi: 10.7717/peerj.3645
Cooper, E. L., Hirabayashi, K., Strychar, K. B., and Sammarco, P. W. (2014). Corals and their potential applications to integrative medicine. Evid. Based Complement. Alternat. Med. 2014:184959. doi: 10.1155/2014/184959
Davy, S. K., Allemand, D., and Weis, V. M. (2012). Cell biology cnidarian-dinoflagellate symbiosis. Microbiol. Mol. Biol. Rev. 76, 229–261. doi: 10.1128/MMBR.05014-11
Denis, V., Guillaume, M. M. M., Goutx, M., de Palmas, S., Debreuil, J., Andrew, C. B., et al. (2013). Fast growth may impair regeneration capacity in the branching coral Acropora muricata. PLoS One 8:e72618. doi: 10.1371/journal.pone.0072618
Derrick, B., Toher, D., and White, P. (2016). Why Welch’s test is type I error robust. Quant. Methods Psychol. 12, 30–38. doi: 10.20982/tqmp.12.1.p030
Edmunds, P. J., and Davies, P. S. (1986). An energy budget for Porites porites (Scleractinia). Mar. Biol. 92, 339–347.
Fang, L., Chen, Y., and Chen, C. (1989). Why does the white tip of stony coral grow so fast without zooxanthellae? Mar. Biol. 103, 359–363. doi: 10.1007/BF00397270
Gleason, M. G. (1993). Effects of disturbance on coral communities bleaching in Moorea, French Polynesia. Coral Reefs 12, 193–201. doi: 10.1007/BF00334479
Graham, J. E., and van Woesik, R. (2013). The effects of partial mortality on the fecundity of three common Caribbean corals. Mar. Biol. 160, 2561–2565. doi: 10.1007/s00227-013-2248-y
Grottoli, A. G., Rodrigues, L., and Juarez, C. (2004). Lipids and stable carbon isotopes in two species of Hawaiian corals, Porites compressa and Montipora verrucosa, following a bleaching event. Mar. Biol. 145, 621–631. doi: 10.1007/s00227-004-1337-3
Grottoli-Everett, A. G., and Kuffner, I. B. (1995). “Uneven bleaching within colonies of the Hawaiian coral Montipora verrucosa,” in Ultraviolet Radiation and Coral Reefs, eds D. Gulko and P. L. Jokiel (Kaneohe, HI: HIMB Technical Report), 115–120.
Guzmán, H. M., Burns, K. A., and Jackson, J. B. C. (1994). Injury, regeneration and growth of Caribbean reef corals after a major oil spill in Panama. Mar. Ecol. Prog. Ser. 105, 231–241. doi: 10.3354/meps105231
Harland, A. D., Davies, P. S., and Fixter, L. M. (1992). Lipid content of some Caribbean corals in relation to depth and light. Mar. Biol. 113, 357–361. doi: 10.1007/BF00349159
Harland, A. D., Fixter, L. M., Davies, P. S., and Anderson, R. A. (1991). Distribution of lipids between the zooxanthellae and animal compartment in the symbiotic sea anemone Anemonia viridis: wax esters, triglycerides and fatty acids. Mar. Biol. 110, 13–19. doi: 10.1007/BF01313087
Harland, A. D., Navarro, J. C., Davies, P. S., and Fixter, L. M. (1993). Lipids of some Caribbean and Red Sea corals: total lipid, wax esters, triglycerides and fatty acids. Mar. Biol. 117, 113–117. doi: 10.1007/BF00346432
Hughes, T. P., Barnes, M. L., Bellwood, D., Cinner, J. E., Cumming, G. S., Jackson, J. B. C., et al. (2017). Coral reefs in the Anthropocene. Nature 546, 82–90. doi: 10.1038/nature22901
Imbs, A. B. (2013). Fatty acids and other lipids of corals: composition, distribution, and biosynthesis. Russ. J. Mar. Biol. 39, 153–168. doi: 10.1134/S1063074013030061
Jones, A. M., and Berkelmans, R. (2011). Tradeoffs to thermal acclimation: energetics and reproduction of a coral reef with heat tolerant Symbiodinium Type-D. J. Mar. Biol. 2011:185890. doi: 10.1155/2011/185890
Jones, R. J. (2008). Coral bleaching, bleaching-induced mortality, and the adaptive significance of the bleaching response. Mar. Biol. 154, 65–80. doi: 10.1007/s00227-007-0900-0
Kleypas, J. A., McManus, J. W., and Menez, L. A. B. (1999). Environmental limits to coral reef development: Where do we draw the line? Am. Zool. 39, 146–159. doi: 10.1093/icb/39.1.146
Klingenberg, C. P. (2008). Morphological integration and developmental modularity. Annu. Rev. Ecol. Evol. Syst. 39, 115–132. doi: 10.1146/annurev.ecolsys.37.091305.110054
Klingenberg, C. P. (2014). Studying morphological integration and modularity at multiple levels: concepts and analysis. Philos. Trans. R. Soc. Lond. B Biol. Sci. 369:20130249. doi: 10.1098/rstb.2013.0249
Kojis, B. L., and Quinn, N. J. (1985). “Puberty in Goniastrea favulus age or size limited?,” in Proceedings of the Fifth International Coral Reef Congress, Tahiti, 289–293.
Lewis, J. B. (1997). Abundance, distribution and partial mortality of the massive coral Siderastrea siderea on degrading coral reefs at Barbados, West Indies. Mar. Pollut. Bull. 34, 622–627. doi: 10.1016/S0025-326X(96)00184-1
Lin, C., Wang, L., Meng, P., Chen, C., and Tsai, S. (2013). Lipid content and composition of oocytes from five coral species: potential implications for future cryopreservation efforts. PLoS One 8:e57823. doi: 10.1371/journal.pone.0057823
Lirman, D. (2000). Fragmentation in the coral branching Acropora palmata (Lamarck): growth, survivorship, and reproduction of colonies and fragments. J. Exp. Mar. Biol. Ecol. 251, 41–57. doi: 10.1016/S0022-0981(00)00205-7
Lirman, D. (2002). “Population dynamics and life-history traits of Acropora palmata: costs and benefits of fragmentation,” in Proceedings of the Caribbean Acropora workshop: Potential Application of the U.S. Endangered Species Act as a Conservation Strategy, ed. A. W. Bruckner (Miami, FL: Southeast Fishery Science Center), 18–27.
López, G. J., Felices, A. U., and Povedano, M. C. (2012). Manual de Laboratorio Para el Análisis del Semen. México: Omnia Publisher SL. doi: 10.3926/oss.5
Meesters, E. H., Noordeloos, M., and Bak, R. P. M. (1994). Damage and regeneration: links to growth in the coral reef-building Montastrea annularis. Mar. Ecol. Prog. Ser. 112, 119–128. doi: 10.3354/meps112119
Meesters, E. H., Wesseling, I., and Bak, R. P. M. (1997). Coral colony tissue damage in six species of reef-building corals: partial mortality in relation with depth and surface area. J. Sea Res. 37, 131–144. doi: 10.1016/S1385-1101(96)00004-4
Mitra, A., and Zaman, S. (2015). Blue Carbon Reservoir of the Blue Planet. Berlin: Springer. doi: 10.1007/978-81-322-2107-4
Muir, P. R., Wallace, C. C., Done, T., and Aguirre, J. D. (2015). Limited scope for latitudinal extension of reef corals. Science 348, 1135–1138. doi: 10.1126/science.1259911
Nugues, M. M., and Roberts, C. M. (2003). Coral mortality an interaction with algae in relation to sedimentation. Coral Reefs 22, 507–516. doi: 10.1007/s00338-003-0338-x
Oku, H., Yamashiro, H., Onaga, K., Iwasaki, H., and Takara, K. (2002). Lipid distribution in branching coral Montipora digitata. Fish. Sci. 68, 517–522. doi: 10.1046/j.1444-2906.2002.00456.x
Oku, H., Yamashiro, H., Onaga, K., Sakai, K., and Iwasaki, H. (2003). Seasonal changes in the content and composition of lipids in the coral Goniastrea aspera. Coral Reefs 22, 83–85.
Oren, U., Benayahu, Y., Lubinevsky, H., and Loya, Y. (2001). Colony integration during regeneration in the stony coral Favia favus. Ecology 82, 802–813. doi: 10.2307/2680199
Oren, U., Rinkevich, B., and Loya, Y. (1997). Oriented intra-colonial transport of 14C labeled materials during coral regeneration. Mar. Ecol. Prog. Ser. 161, 117–122. doi: 10.3354/meps161117
Pernice, M., Meibom, A., Van Den Heuvel, A., Kopp, C., Domart-Coulon, I., Hoegh-Guldberg, O., et al. (2012). A single-cell view of ammonium assimilation in coral-dinoflagellate symbiosis. ISME J. 6, 1314–1324. doi: 10.1038/ismej.2011.196
Riegl, B., and Purkis, S. (2015). Coral population dynamics across consecutive mass mortality events. Global Change Biol. 21, 3995–4005. doi: 10.1111/gcb.13014
Rinkevich, B., and Loya, Y. (1989). Reproduction in regenerating? colonies of the coral Stylophora pistillata. Environ. Qual. Ecosyst. 4, 257–265.
Seemann, J., Sawall, Y., Auel, H., and Richter, C. (2013). The use of lipids and fatty acids to measure the trophic plasticity of the coral Stylophora subseriata. Lipids 48, 275–286. doi: 10.1007/s11745-012-3747-1
Spalding, M., Burke, L., Wood, S. A., Ashpole, J., Hutchison, J., and zu Ermgassen, P. (2017). Mapping the global value and distribution of coral reef tourism. Mar. Policy 82, 104–113. doi: 10.1016/j.marpol.2017.05.014
Spalding, M. D., and Grenfell, A. M. (1997). New estimates of global and regional coral reef areas. Coral Reefs 16, 225–230. doi: 10.1007/s003380050078
Stimson, J. S. (1987). Location, quantity and rate of change in quantity of lipids in tissue of hawaiian hermatypic corals. Bull. Mar. Sci. 41, 889–904.
Sudek, M., Aeby, G. S., and Davy, S. K. (2012). Localized bleaching in Hawaii causes tissue loss and a reduction in the number of gametes in Porites compressa. Coral Reefs 31, 351–355. doi: 10.1007/s00338-011-0844-1
Titlyanov, E. A., and Titlyanova, T. V. (2008). Coral-algal competition on damaged reefs. Russ. J. Mar. Biol. 34, 199–219. doi: 10.1371/journal.pone.0114525
Titlyanov, E. A., Titlyanova, T. V., Yakovleva, I. M., and Sergeeva, O. S. (2006). Three stages of injuries regeneration on scleractinian corals. Galaxea 8, 39–50. doi: 10.3755/jcrs.8.39
Titlyanov, E. A., Tylyanovaa, T. V., Yakovlevaa, I. M., Nakanob, Y., and Bhagooli, R. (2005). Regeneration of artificial injuries on scleractinian corals and coral / algal competition for newly formed substrate. J. Exp. Mar. Biol. Ecol. 323, 27–42. doi: 10.1016/j.jembe.2005.02.015
Van Veghel, M. L. J., and Bak, R. P. M. (1994). Reproductive characteristics of the polymorphic Caribbean coral reef building Montastrea annularis. III. Reproduction in damaged and regenerating colonies. Mar. Ecol. Prog. Ser. 109, 229–233. doi: 10.3354/meps109229
Ward, S. (1995). The effect of damage on the growth, reproduction and storage of lipids in the scleractinian coral Pocillopora damicornis (Linnaeus). J. Exp. Mar. Biol. Ecol. 187, 193–206. doi: 10.1016/0022-0981(94)00180-L
Ward, S., Harrison, P., and Hoegh-Guldberg, O. (2000). “Coral bleaching reduces reproduction of scleractinian corals and increases susceptibility to future stress,” in Proceedings 9th International Coral Reef Symposium, Bali,23–27.
Weisburd, D., and Britt, C. (2014). Statistics in Criminal Justice. Boston: Springer. doi: 10.1007/978-1-4614-9170-5
Welsh, J. Q., Bonaldo, R. M., and Bellwood, D. R. (2015). Clustered parrotfish feeding scars trigger partial coral mortality of massive Porites colonies on the inshore Great Barrier Reef. Coral Reefs 34, 81–86. doi: 10.1007/s00338-014-1224-4
Keywords: Elkhorn coral, branch growth, developmental anomalies, lipid content, sexual reproduction, spawning
Citation: Piñón-González VM and Banaszak AT (2018) Effects of Partial Mortality on Growth, Reproduction and Total Lipid Content in the Elkhorn Coral Acropora palmata. Front. Mar. Sci. 5:396. doi: 10.3389/fmars.2018.00396
Received: 31 May 2018; Accepted: 09 October 2018;
Published: 05 November 2018.
Edited by:
Noga Stambler, Bar-Ilan University, IsraelReviewed by:
Douglas Fenner, Independent Researcher, United StatesCharles Birkeland, University of Hawaii, United States
Copyright © 2018 Piñón-González and Banaszak. This is an open-access article distributed under the terms of the Creative Commons Attribution License (CC BY). The use, distribution or reproduction in other forums is permitted, provided the original author(s) and the copyright owner(s) are credited and that the original publication in this journal is cited, in accordance with accepted academic practice. No use, distribution or reproduction is permitted which does not comply with these terms.
*Correspondence: Anastazia T. Banaszak, YmFuYXN6YWtAY21hcmwudW5hbS5teA==