- 1Leibniz Institute for Baltic Sea Research Warnemünde, Rostock, Germany
- 2Centre for Limnology, Estonian University of Life Sciences, Tartu, Estonia
Major Baltic inflow (MBI) events carry highly saline water from the North Sea to the central Baltic Sea and thereby affect both its environmental conditions and its biota. While bacterioplankton communities in the Baltic Sea are strongly structured by salinity, how MBIs impact the composition and distribution of bacteria is unknown. The exceptional MBI in 2014, which brought saline and oxygenated water into the basins of the central Baltic Sea, enabled the linkage of microbiological investigations to hydrographic and modeling studies of this MBI. Using sequence data of 16S ribosomal RNA (rRNA) and 16S rRNA genes (rDNA), we analyzed bacterioplankton community composition in the inflowing water and in the uplifted former bottom-water at stations reached by the MBI. Bacterial diversity data were compared with respective data obtained from previous, non-inflow conditions. Changes in bacterial community composition following the 2014 MBI were mainly apparent at the genus level. A number of specific taxa were enriched in the inflowing water, with large changes in the rRNA/rDNA ratios indicating the different activity levels between of the water masses. The relative similarity of the bacterial communities in the inflowing and uplifted waters as well as the results from an inflow-simulating numerical model showed that the inflowing water did not originate directly from the North Sea but mostly from adjacent areas in the Baltic Sea. This suggested that the inflow event led to a series of shifts in Baltic Sea water masses among the Baltic Sea basins and a gradual mixing of the water bodies. Dramatic changes in the bacterial community composition occurred when the bottom-water inflow reached the anoxic, sulfidic deep basins, resulting in an uplifting of the formerly anoxic bacterial community, dominated by Epsilonproteobacteria. Our study of the impact of MBIs on bacterioplankton communities therefore highlights two relevant underlying mechanisms that impact the distribution and possibly also the activities of planktonic bacteria in the Baltic Sea: (1) the successive dilution of inflowing North Sea water with ambient waters and (2) the uplifting of former bottom-water communities to higher water strata.
Introduction
In the land-locked Baltic Sea, water is exchanged only with the North Sea, via the straits of the Kattegat and Skagerrak. The low seawater inflow together with strong freshwater inputs from rivers, rain, and melt water accounts for the elongated, stable salinity gradient of the Baltic Sea, with high salinities in the Skagerrak (salinity > 25) and low salinities in the Gulf of Bothnia (salinity < 5) (Reissmann et al., 2009). Another result is a permanent halocline in the central Baltic Sea that prevents vertical mixing and leads to permanent stratification and subsequent oxygen deficiency in the deep basins (Reissmann et al., 2009).
Major Baltic inflow (MBI) events, forced by special atmospheric conditions, occur occasionally in the autumn and winter and carry a volume of saline water from the North Sea that is large enough to reach the central basins of the Baltic Sea (Matthäus and Franck, 1992). The inflowing water in winter typically has a higher salinity (17.5–19), a lower temperature (2–8°C), and higher oxygen content than the Baltic bottom-water (Matthäus and Franck, 1992; Omstedt et al., 2004). Several studies have shown that these inflowing, oxygenated waters can give rise to chemically and microbially mediated transformations in the deep, stratified basins, thereby affecting methane oxidation (Schmale et al., 2016), mercury species distribution (Kuss et al., 2017), manganese cycling (Dellwig et al., 2018), nutrient release from the sediments (Hall et al., 2017; Sommer et al., 2017), and macrozoobenthos communities (e.g., Laine et al., 1997), However, the mechanisms and magnitude by which MBIs change the composition, activity, and distribution of planktonic microbial communities in the Baltic Sea are currently not understood.
Nonetheless, that the Baltic Sea salinity gradient is a structuring force acting on bacterial community composition has been convincingly demonstrated in several studies (Riemann et al., 2008; Herlemann et al., 2011, 2016; Bergen et al., 2014), which also more generally confirmed that salinity is one of the most important determinants of the structure of microbial communities in aquatic systems (Lozupone and Knight, 2007; Nemergut et al., 2011). In estuaries, the dominant bacterial phyla/classes shift from a prevalence of Betaproteobacteria and Actinobacteria in oligohaline regions to one of Alpha- and Gamma-proteobacteria in higher salinity areas (e.g., Bouvier and Giorgio, 2002; Kirchman et al., 2005; Fortunato and Crump, 2011). This pattern also characterizes the Baltic Sea (Herlemann et al., 2011), where the salinity gradient is much more stable than in most estuaries. Additionally, an adapted bacterial community thrives in the brackish waters of the Baltic Sea and its composition differs significantly from that of its freshwater and marine counterparts. Because MBIs result in a major disturbance of the salinity gradient, particularly in deeper layers, and in the natural ventilation of the central basins (Matthäus and Franck, 1992), they can be expected to impact the structure of the Baltic’s bacterial communities.
In December 2014, an exceptionally strong MBI occurred after the long stagnation period that followed the last MBI, in 2003. The 2014 MBI ranked as the third strongest among the recorded MBIs (Mohrholz et al., 2015). Between December 13th and 25th, a total water volume of 358 km3 was pushed into the Baltic Sea by the continuous strong westerly winds. The inflowing water included a highly saline volume (salinity > 17) of 198 km3 and salt transport of 3.98 Gt. During the initial inflow phase, in December 2014, a mean salinity of 20.8, a mean temperature of 7.7°C, and a dissolved oxygen concentration of 6.5 mL/L were measured in the Arkona Basin (Naumann et al., unpublished data). As previously demonstrated for other MBIs (Umlauf et al., 2007) on its way to the Baltic Sea, the highly saline inflow water mixed with ambient waters and raised the former bottom-waters, such that new and distinct water masses were formed. The large volume of highly saline water propagated quickly through the western Baltic Sea and Bornholm Basin, passing the Slupsk Sill already in February 2015. This fast propagation was supported by a series of weaker inflows that had occurred earlier in 2014. The final arrival of the water mass and the oxygenation of the Gotland Deep at 240 m water depth occurred after this study, in mid April 2015 (Naumann et al., unpublished data).
In this study, we took advantage of this strong MBI to investigate the effect of inflowing saline water on the composition of bacterioplankton within the water column. Specifically, we asked whether characteristic marine bacterial assemblages are transported by the inflow to the central basins or does the inflowing marine bacterial community become diluted through successive mixing with adjacent water masses. To answer this question, we analyzed the bacterial community composition of the inflowing and uplifted water and compared the results with data collected in 2009 under non-inflow (stagnant) conditions (Herlemann et al., 2016). Differences in community composition were determined by next-generation sequencing (NGS) of partial 16S ribosomal RNA (rRNA) and 16S rRNA genes (rDNA), which also allowed the identification of potentially active taxa. Numerical hydrodynamic modeling was used to estimate the most likely origin of the sampled water masses (Gräwe et al., 2015), and thus to identify the initial location of the bacterial community in the inflowing water masses, despite the high turbulent mixing rates. The results provided the first insights into the changes in the bacterioplankton communities that occurred in response to the different water masses during the MBI that began in 2014. Thus, we were able to show that (1) during its passage from the North Sea to the interior of the Baltic, the inflowing water mixed with adjacent water masses such that the inflowing bacterial communities became increasingly similar to those already present, and (2) the displacement (uplifting) of whole water masses caused the transport of formerly anaerobic bacterial communities into water depths where they normally do not occur.
Materials and Methods
Sampling
Samples were taken in the Arkona Basin, Bornholm Basin, Slupsk Channel and the southwestern part of the Eastern Gotland Basin (Figure 1) during a cruise with the RV Elisabeth Mann Borgese in February 2015 (cruise EMB 95). Temperature, salinity, oxygen, and chlorophyll fluorescence were measured using a CTD SBE911+ combined with a bottle sampler rosette, which comprised 13 10-L free-flow bottles. Sampling depths were chosen at each station according to the characteristics of the physical water mass revealed in the CTD profiles. At each station, one sample was taken from the inflowing water body and one from the uplifted bottom-water body, distinguished by different salinities, temperatures, and oxygen concentrations (Figure 2).
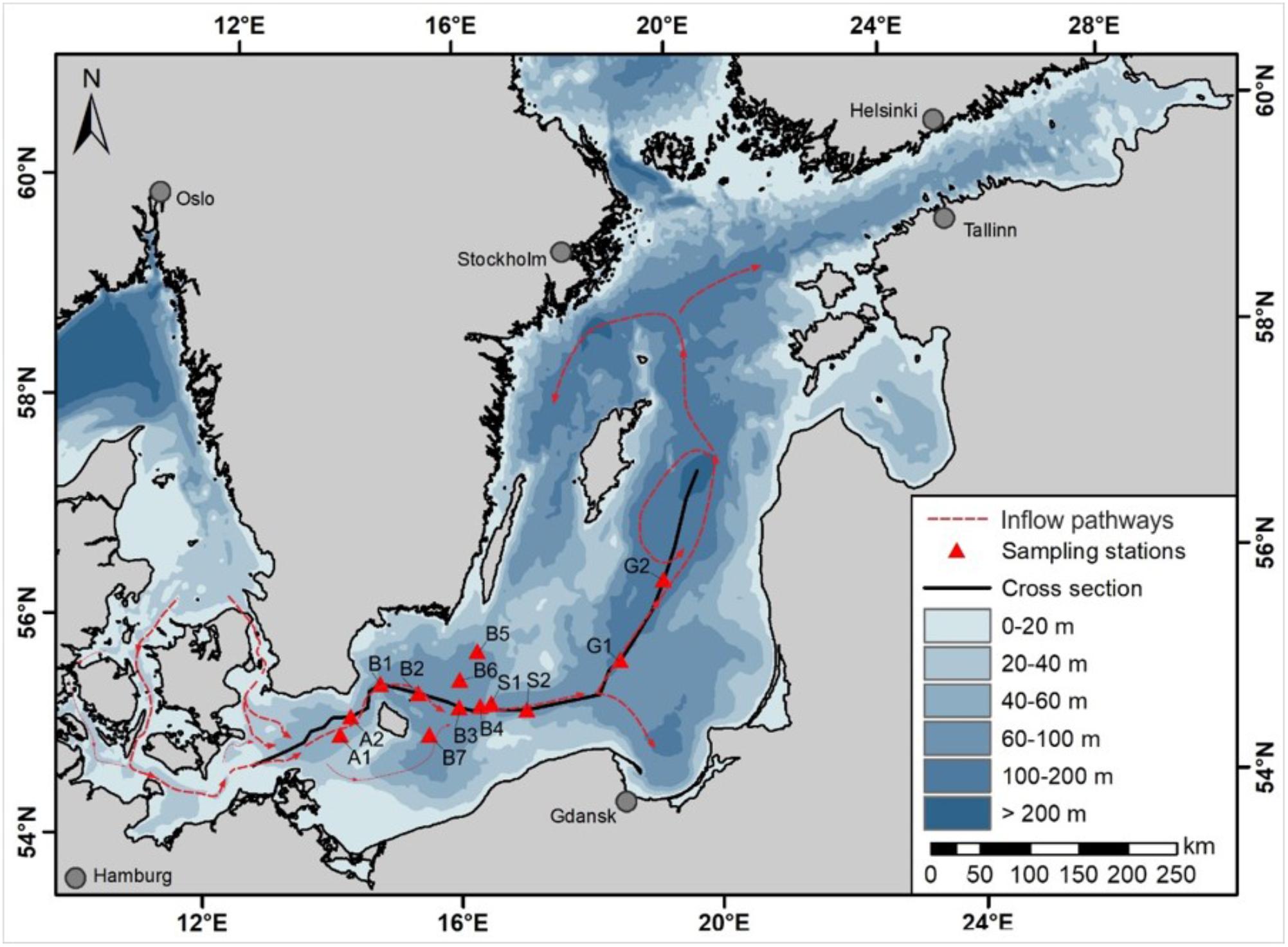
FIGURE 1. Map of the Baltic Sea, showing the sampling stations (dots) and estimated state of the 2014 Major Baltic inflow (MBI) during the time of sampling (red lines).
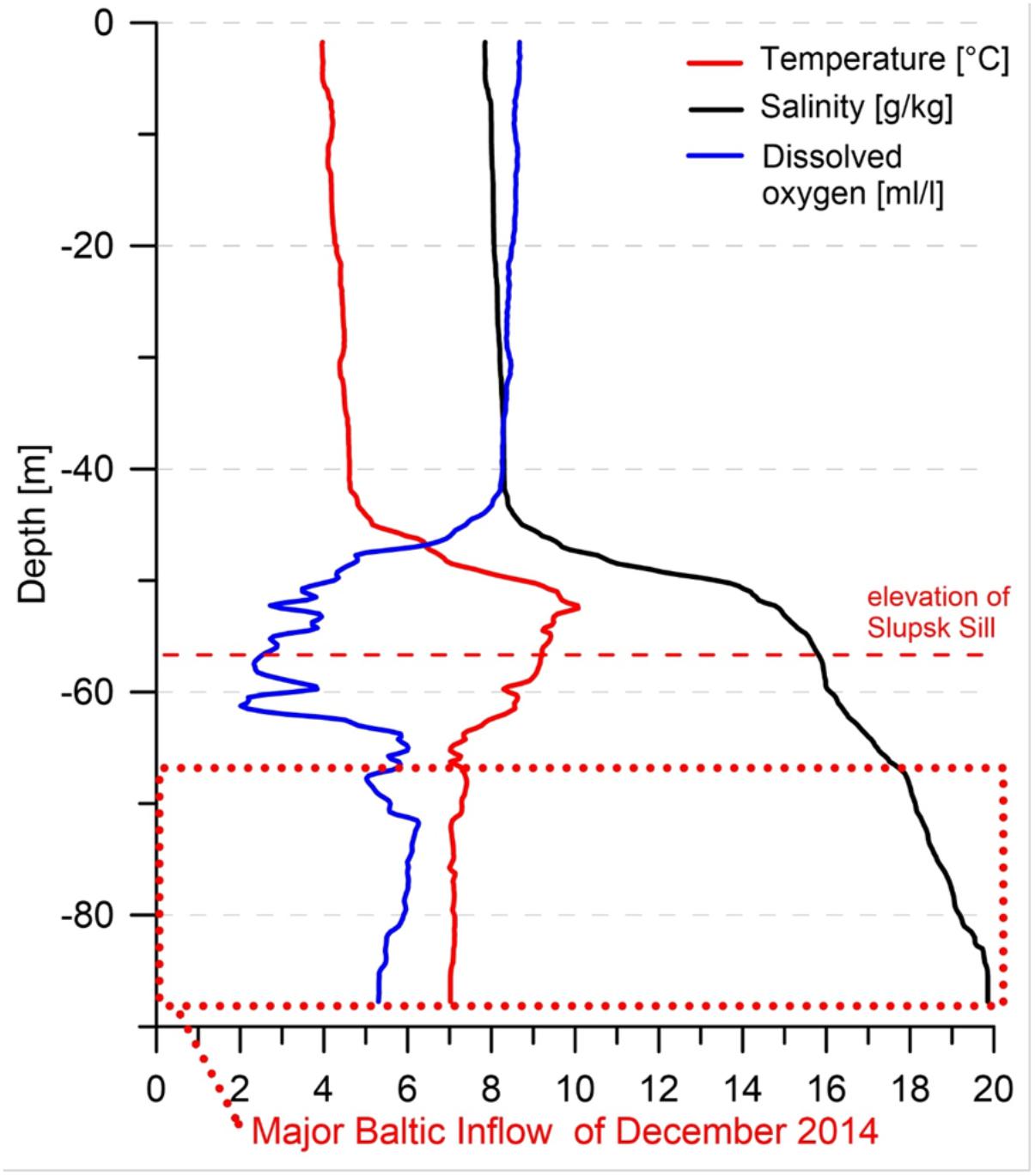
FIGURE 2. Example of a CTD profile (station B3, Bornholm basin), used to distinguish inflowing from uplifted bottom-water. The dotted line indicates the approximate depth layer of the inflowing water with a higher salinity and oxygen concentration and a lower temperature. Warmer, former bottom-water was uplifted and crossed the Slupsk Channel (dashed line) toward the eastern Gotland basin.
Water samples (1 L) used for DNA and RNA analysis were filtered onto 0.22-μm pore-size white polycarbonate filters. DNA and RNA were extracted according to Weinbauer et al. (2002). Concentrations of inorganic nutrients were analyzed as described by Grasshoff et al. (1983). For bacterial enumeration, subsamples (100 mL) were fixed for 1 h with 400 μl of 1% paraformaldehyde and 0.5% glutaraldehyde, shock-frozen in liquid nitrogen, and stored at -80°C until processed by flow cytometry. After staining with SYBR Green, the samples were analyzed on a FacsCalibur (Becton Dickinson) using a modification of the method of Gasol and del Giorgio (2000).
Amplicon Sequencing and Sequence Processing
For cDNA synthesis, DNA was removed from the RNA extracts by DNase I (Ambion/Applied Biosystems, Huntington, United Kingdom) digestion, following the manufacturer’s protocol. RNA samples were transcribed into cDNA by a reverse transcriptase step, using the iScript Select cDNA synthesis kit (Bio-Rad, Hercules, CA, United States) with the primer 1492r. The DNA extracts and the generated cDNA for bacterial diversity analysis were sent to LGC Genomics GmbH (Berlin, Germany) for further processing. There, the primers Bakt_341F (CCTACGGGNGGCWGCAG) and Bakt_805R (GACT ACHVGGGTATCTAATCC) (Herlemann et al., 2011) were used for PCR amplification and sequences were generated on the Illumina MiSeq V3 platform in a 2 bp × 300 bp paired-end run. Pairs of forward and reverse primer-trimmed sequences were combined using BBMerge 34.48 and processed using the SILVA NGS pipeline (Quast et al., 2013; Glöckner et al., 2017) based on SILVA release version 123 (Pruesse et al., 2007). SILVA NGS performs additional quality checks according to the SINA-based alignments (Pruesse et al., 2012) with a curated seed database in which PCR artifacts or non-SSU reads are excluded. The longest read serves as a reference for the taxonomic classification in a BLAST (version 2.2.28+) search against the SILVA SSURef dataset (Quast et al., 2013). The classification of the reference sequence of a cluster (98% sequence identity) is then mapped to all members of the respective cluster and to their replicates. Best BLAST hits were only accepted if they had a (sequence identity + alignment coverage)/2 ≥ 93 or otherwise defined as unclassified. The 16S rRNA and rDNA raw sequence data have been submitted to the European Nucleotide Archive (ENA) under the Accession No. PRJEB27018.
The bacterial community composition associated with the inflow event was compared with that of a typical situation, before the MBI, in approximately the same and adjacent areas of the Baltic Sea. For this purpose, we used sequence data from the western and central Baltic Sea obtained in February 2009 (for the positions of the 2009 stations see Supplementary Figure 1), as reported in Herlemann et al. (2016). DNA from the reference samples from 2009 was amplified using the same primers (Bakt_341F and Bakt_805R) as described in Herlemann et al. (2011). DNA from the 2009 samples was sequenced by Eurofins MWG GmbH on a 454 GS-FLX sequencer (Roche). For a detailed description of the amplification and 454 sequencing procedures, see Herlemann et al. (2011, 2016). To allow comparisons of the reference and inflow sequences, all sequences were processed together using the Silva NGS pipeline with standard settings.
Statistical Analyses
As the Gotland Basin samples derived from anoxic conditions and thus harbored very distinct bacterial communities, they were excluded from statistical analyses aimed at distinguishing the different bacterial communities in inflowing and uplifted waters. For all statistical tests, samples were grouped according to their origin. Explicet (Robertson et al., 2013) was used for providing bootstrapped-based subsampling for normalizing OTU counts in the bacterial alpha diversity. For this analysis all samples were reduced to 1000 reads. The difference between the bacterial communities were visualized by non-metric multidimensional scaling plots based on the Bray–Curtis dissimilarity matrix, using the software PAST (Hammer et al., 2001). For these analyses, the sum-normalized OTU abundances were combined with data from the investigation conducted during stagnant conditions in February/March 2009 (Herlemann et al., 2016). A linear discriminant analysis (LDA) effect size (LEfSe) (Segata et al., 2011)1 was used to test for discriminatory taxa in the different water masses, inflow and uplifted waters, based on relative rDNA and rRNA abundances. Based on a normalized relative abundance table, LEfSe applies the Kruskal–Wallis rank sum test to identify OTUs with significantly different abundances and performs an LDA to estimate the effect size of each feature. In this study, an alpha value of 0.05 and an effect size threshold of three were considered to indicate statistical significance.
Physical Numerical Model
To follow the inflowing water masses and quantify their transport pathways, a three-dimensional hydrodynamic model, the General Estuarine Transport Model (GETM), was used in a multi-nested downscaling framework extending from the North Atlantic (8-km resolution) to the Western Baltic Sea (600-m resolution). The numerical model provides hourly mean values of velocity, salinity, and temperature at every grid point and reproduced the 2014 MBI in detail (Gräwe et al., 2015; Mohrholz et al., 2015). Previous studies demonstrated the utility of the GETM in simulating inflow events and in allowing statistical analyses of inflows in the western Baltic Sea (Burchard et al., 2005; Gräwe et al., 2013). Our study used the same model setup as described in Gräwe et al. (2015). Based on the output of the hydrodynamic model, a particle-tracking tool was applied that enabled the water masses to be marked and followed, including tracking of the water masses back in time to estimate the potential sources and/or origins of distinct water parcels. Backtracking was started at the sampling stations in the Bornholm Gat (TF0145) and Slupsk Channel (TF0222) and was used to analyze the origin of the inflowing water masses in greater detail.
Results
Characterization of the Water Masses
The very strong inflow arising from the 2014/2015 MBI was clearly evident from the CTD profiles obtained from the Arkona Basin and Bornholm Basin; a representative example is shown in Figure 2 (Bornholm Deep). The highly saline bottom layer (salinity > 17) in the Bornholm Basin was ∼34 m thick, with a clearly higher oxygen concentration and lower temperature (Figure 2, dashed box) than the uplifted former bottom-water (at 50–60 m depth). This mixture of inflow water and uplifted former bottom-water (>8°C, salinity 12–17, 2–5 mL/L) passed the Slupsk Sill (58 m sill depth), which otherwise forms a natural barrier between the Bornholm and central Baltic basins, of which the Gotland Basin is the farthest to the northeast.
Figure 3 shows a hydrographic cross-section reaching from the Darss Sill to the Eastern Gotland Basin, including the sampling stations and sampling depths and the general situation along the “Baltic thalweg” (Burchard et al., 2005; Lilover et al., 2017) of water flowing into Baltic basins until the analyzed stage of the inflow event. During the sampling period, most of the MBI inflow water volume (about 90%) was estimated to be present in the Bornholm basin, below a water depth of 60 m (Naumann et al., unpublished data). The uplifted inflow water body, clearly apparent by its higher temperature (>7°C), lower salinity (12–15), and a lower dissolved oxygen concentration (2–4 mL/L), originated from a weak summer inflow in August of that year (Naumann et al., unpublished data). Anoxic/suboxic bottom-water in the Eastern Gotland Basin was clearly uplifted by this inflowing oxygenated, more saline water (station G1, Figure 3C), whereas deeper water (below 120 m depth) in the Gotland Basin remained anoxic and was not influenced at that stage of inflow propagation (station G2, Figure 3C).
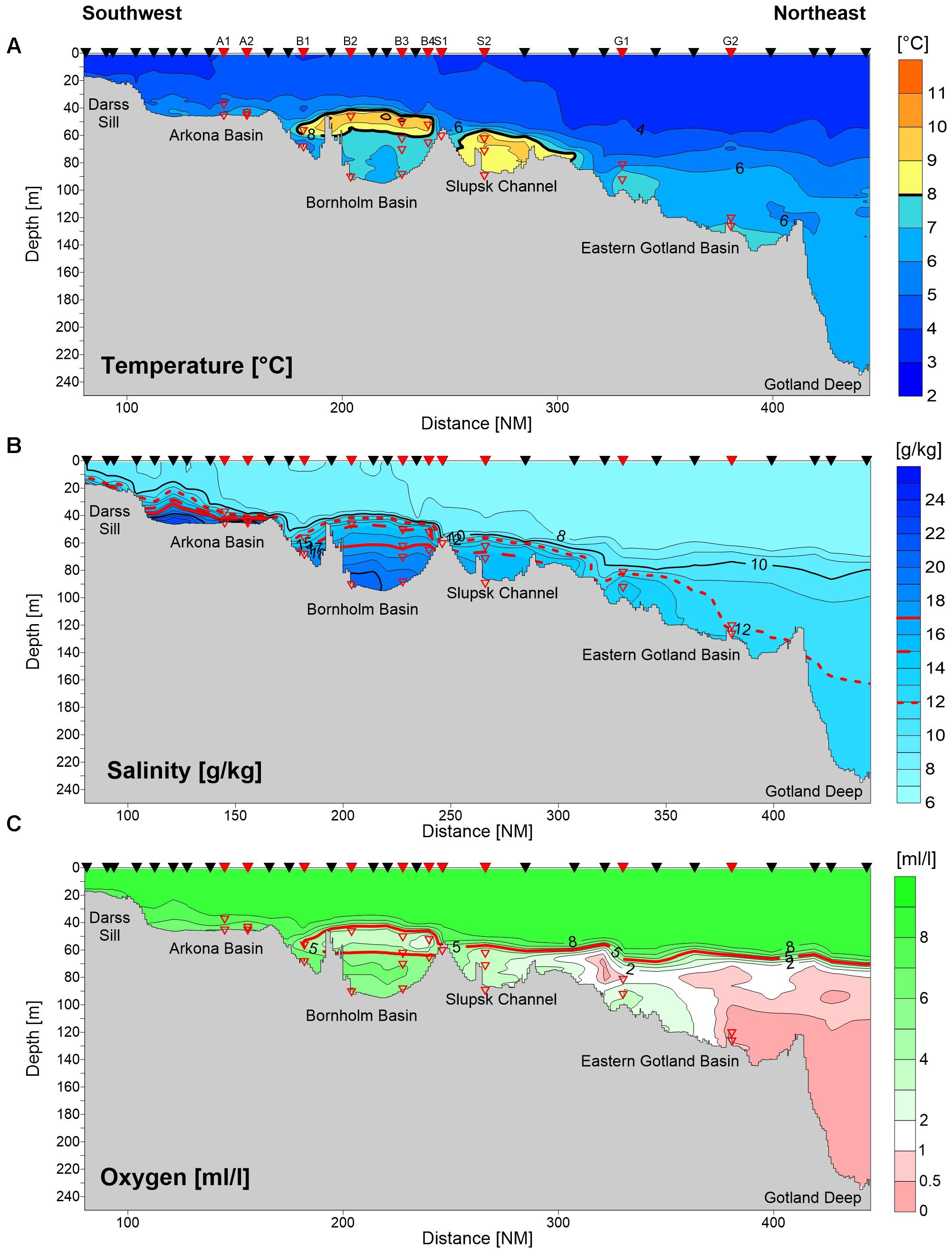
FIGURE 3. Bathymetric map of the southwestern Baltic Sea, showing the sampling locations and inflow pathways and a vertical cross-section, also showing the sampling locations (top of graphs; black triangles: only CTD measurements; red triangles: CTD and microbiological measurements) together with temperature (A), salinity (B), and oxygen concentrations (C).
An estimation of the flow pattern of the MBI along the stations sampled was obtained based on the features of the water masses determined from the CTD profiles and on information from previously published studies of this MBI (e.g., Gräwe et al., 2015; Mohrholz et al., 2015) (Figure 1). The stations first impacted by the MBI were those of the Arkoma Basin, A1 and A2, followed by stations B1 and B2 of the Bornholm Basin. Thereafter, the MBI spread in different directions, with the central and southern stations B3 and B7 probably reached more or less simultaneously, due to the deflection of gravity currents to the right by the Coriolis force at the northern hemisphere, followed shortly thereafter by station B4, then stations B6 and S1, stations B5 and S2, and finally Gotland Basin station G1. At the bottom-water stations B5 and B6, the water probably consisted of the less-mixed original inflow water of December 2014 (temperature < 7.4°C, salinity > 18, dissolved oxygen > 5.2 mL/L), whereas at the bottom of the sill stations S1 and S2 (temperature > 7.7°C, salinity 16.6, dissolved oxygen < 4.3 mL/L) the water was composed of new inflow water strongly mixed with former bottom-water from the Bornholm Basin and Slupsk Channel, as evidenced by the higher temperatures and lower oxygen concentrations. At the time of sample collection, the inflowing water had not yet reached Gotland Basin station G2, which still had anoxic bottom-water.
As expected, salinity and temperature were higher in the inflow water and the uplifted bottom-water than in the reference water from the stagnation period in winter 2009 (Table 1). The higher temperature of the sampled water could be attributed to the August 2014 and December 2014 inflows. During these events, the warmed summer and autumn surface water of the Mecklenburg Bight (overflow August 2014) and Kattegat (inflow December 2014) penetrated the deep water of the Baltic basins and was warmer than the bottom-water of the previous stagnation period. The salinity and temperature values as well as the phosphate, silicate, nitrite, and nitrate concentrations of the uplifted bottom-water, especially that of the Arkona Basin, were between those of the inflow and reference waters of 2009. A comparison of the concentrations of dissolved nitrogen compounds in the Arkona and Bornholm Basins, as determined in samples from this study, with those of the reference samples from winter 2009 revealed that, due to the inflow water, the ammonia concentration was significantly lower in both basins, the nitrite concentration was lower in the Arkona Basin, and the nitrate concentration was higher in both basins (Supplementary Figure 2). Total prokaryotic cell numbers were in the same range, 6.6–8.0 × 105 mL-1, with considerable variability between the stations but no apparent trend and with values roughly similar to those in samples from the stagnant period of 2009 (Table 1).
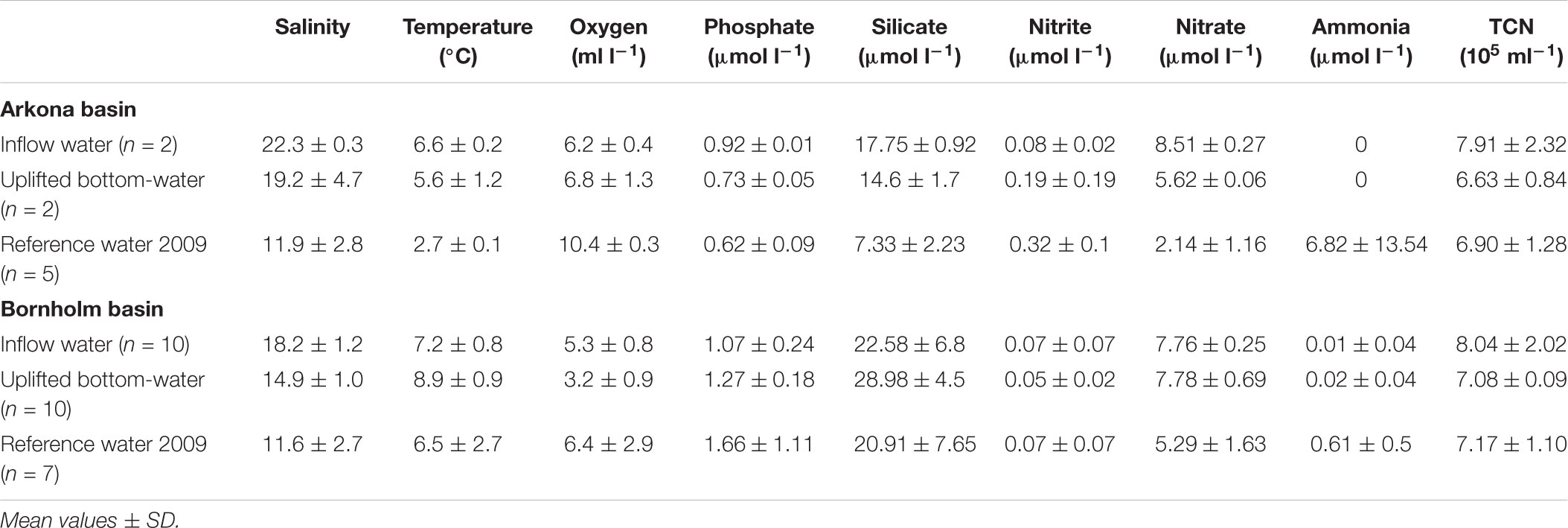
TABLE 1. Physicochemical parameters and total prokaryotic cell numbers (TCN) in the different water masses in the Arkona and Bornholm Basins.
Back-Trajectory of the Water Masses
The origin of the sampled inflow water, estimated by numerical modeling and particle tracking, is depicted in the probability maps of Figure 4. According to the maps, the sampled water mass likely originated from a specific region in the western Baltic Sea. For the two sampling stations, located in the Bornholm Gat (A2) and Slupsk Channel (S2, see Figure 1), the maps show similar results for the two time slices: a main inflow period during December 13–25, 2014 and a pre-inflow stage that occurred in mid-October 2014. During the main inflow period (upper panels in Figure 4), the possible origin of both sampled water masses may have been the Arkona Basin and the southern Danish Belt Sea. Thus, there was a high probability that both samples were in contact with the inflowing saline water. Due to the high degree of mixing (lateral but also vertical) during the inflow, the initial start position of the sampled water masses could not be further specified. The lower maps show the position of the sampled water in October, the pre-inflow stage, and indicate an area spanning from the Skagerrak to the Danish Belt Sea, which is a common finding for such events. During this pre-inflow stage, the saline water of the Danish Belt Sea was pushed farther north into the Kattegat, due to a lowering of the water level in the Baltic Sea and the resulting outflow. Furthermore, because of the Earth’s rotation, this outflowing water probably accumulated on the eastern side of the Kattegat. This specific pattern, illustrated in the lower panels of Figure 4, indicated that the water originated from the Danish Belt Sea and thus allowed an estimation of the origin of the sampled inflow water from this transition zone between the North Sea and the Baltic Sea.
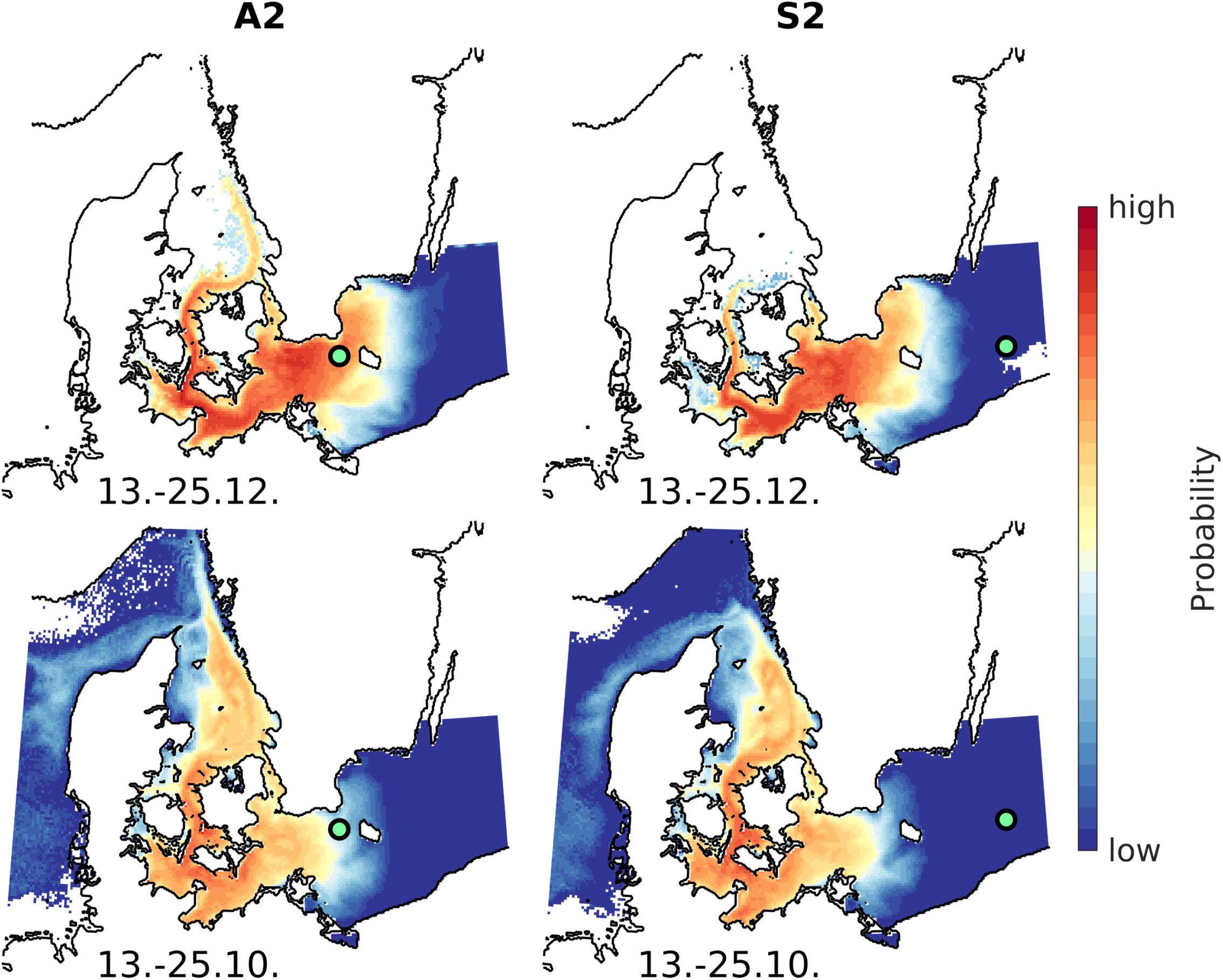
FIGURE 4. The possible origin of the sampled inflow water in the Bornholm Gat (TFO145, left panels) and in the Slupsk Channel (TFO222, right panels) for two different time slices (upper panels: during the inflow; lower panels: 2 months prior to the inflow). The maps show the most likely origin and were estimated based on numerical modeling.
Taxonomic Composition of the Microbial Communities in the Different Basins and in the Inflowing and Uplifted Water Bodies
We compared the bacterial community compositions of all the samples from this study (excluding the anoxic Gotland stations) with reference samples from the stagnant situation in winter 2009, which covered also those area of potential inflow water origin (Skagerrak, Belt Sea, Western Baltic) as well as the same locations as in this study (Arkona and Bornholm Basins) (Herlemann et al., 2016). The non-metric multidimensional scaling (NMDS) plot (Bray–Curtis dissimilarity) of the bacterioplankton community composition revealed a clear clustering of the respective bacterial communities according to their location (Figure 5A). The more saline stations from the Skagerrak, Kattegat, Belt Sea, and Western Baltic (reference 2009) were clearly separated from stations in the Arkona and Bornholm Basins. Samples from this study (Figure 5A, red symbols) clustered together for the Arkona and Bornholm Basins, slightly separated from samples from the reference study of 2009 (Figure 5A, blue symbols). The Bornholm samples from 2009 spread more widely whereas those from this study (including samples from the inflow and uplifted waters) were more tightly clustered (Figure 5A). A more detailed look at only the Bornholm Basin samples from this study showed the clear separation of the rRNA- and rDNA-based compositions on the first coordinate, with no overlap. Further, both the rRNA- and rDNA- based bacterial community compositions of the inflowing and uplifted water bodies were separated on the second coordinate (Figure 5B). To visualize the relationship between the variability in the community composition and the features of the water mass, the physicochemical parameters were plotted in the NMDS ordination as fitting environmental variables (only the Bornhom Basin, Supplementary Figure 3). The variation in community composition correlated with differences in salinity and oxygen (higher in the inflow water) and partly also with nutrient levels (higher in the uplifted water). Alpha diversity was significantly higher in the samples from the Arkona and Bornholm Basins obtained in this study than in all reference samples from 2009 (Supplementary Figure 4). A direct comparison of the inflowing and uplifted water masses revealed that for most stations diversity (OTU number and ShannonH) was slightly higher in the inflowing than in the uplifted water, both with the rDNA and the rRNA analyses (Supplementary Figure 5). Only the Gotland samples, containing anaerobic communities, deviated strongly from the other stations, showing a strongly reduced diversity (Supplementary Figure 5).
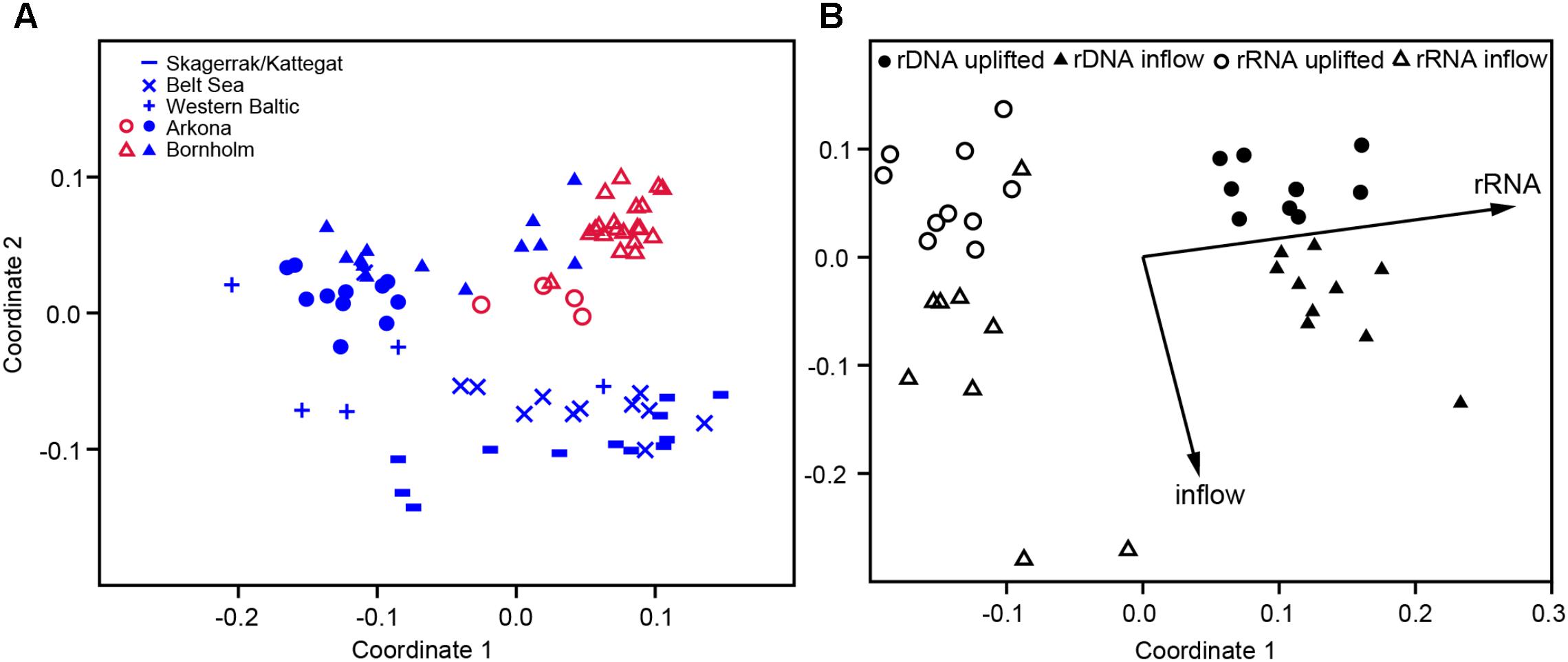
FIGURE 5. (A) Non-metric multidimensional scaling (NMDS) plots (Bray–Curtis dissimilarity, NMDS stress = 0.1133) of bacterioplankton community composition from different basins of the Baltic Sea, based on the rDNA analysis. The blue symbols represent samples from a reference study (Herlemann et al., 2016), which included the Skagerrak/Kattegat, Belt Sea, Western Baltic, Arkona and Bornholm Basins, and the red symbols samples from this study, including the Arkona and Bornholm Basins. (B) NMDS plot (Bray–Curtis dissimilarity, NMDS stress = 0.1046) of bacterioplankton community composition from the Bornholm Basin (this study only) based on rRNA and rDNA analyses. Full symbols indicate DNA-based and open symbols RNA-based bacterial communities. Dots represent the uplifted water masses and triangles the inflow water. The environmental variables inflow vs. uplifted water masses and rDNA vs. rRNA were added as post hoc vectors to the NMDS graph, representing the correlations between the environmental variables and NMDS scores.
To elucidate the broad taxonomic changes that occurred between the inflowing and uplifted water bodies in the different basins, both 16S rDNA and 16S rRNA sequence data were examined. According to the analysis at the phylum/class level, in which the data for the different basins were pooled, 87–90% of the sequences could be assigned to these major phyla/classes; only 10–13% were unclassified or belonged to rare phylogenetic groups (Figure 6). The bacterial composition at all stations until the inflow to station G1, including the Arkona Basin, Bornholm Basin, and Slupsk Channel, was comparable at this broad taxonomic resolution, and quite consistent between the rRNA- and rDNA-based analyses (Figure 6). Alpha- and Gammaproteobacteria were the most abundant groups in these samples, with an average relative abundance of 26 and 14%, respectively. Other important groups, with relative abundances of 5–10%, were Deltaproteobacteria, Bacteroidetes, Planctomycetes, Chloroflexi, and Actinobacteria. The rRNA- and rDNA-based compositions were only slightly different, with increased proportions of Firmicutes, Deltaproteobacteria and Chloroflexi, and decreased proportions of Bacteroidetes and Planctomycetes according to the rRNA data (Figure 6). The variations between the locations of the Arkona and Bornholm Basins and Slupsk Channel, and between the inflowing and uplifted waters, were rather small. However, in the Bornholm Basin, where the number of stations covered was sufficient to allow statistical tests, the inflowing and uplifted bacterial communities were significantly different at the phylum/class level (p < 0.01). A strikingly different community composition was apparent for the two Gotland basin stations G1 and G2, which were dominated by Epsilonproteobacteria. However, the water inflowing into station G1 (91 m depth), had a high overall similarity with the uplifted bottom-water from the Bornholm Basin, with a large proportion of Alphaproteobacteria, and only 6.8% Epsilonproteobacteria. By contrast, Epsilonproteobacteria constituted 59% of all sequences in the uplifted water at station G1 (82 m water depth). Station G2, which was not yet impacted by the MBI, was entirely dominated (85%) by Epsilonproteobacteria in the deeper waters (126 and 120 m) and contained only small proportions of the other phyla.
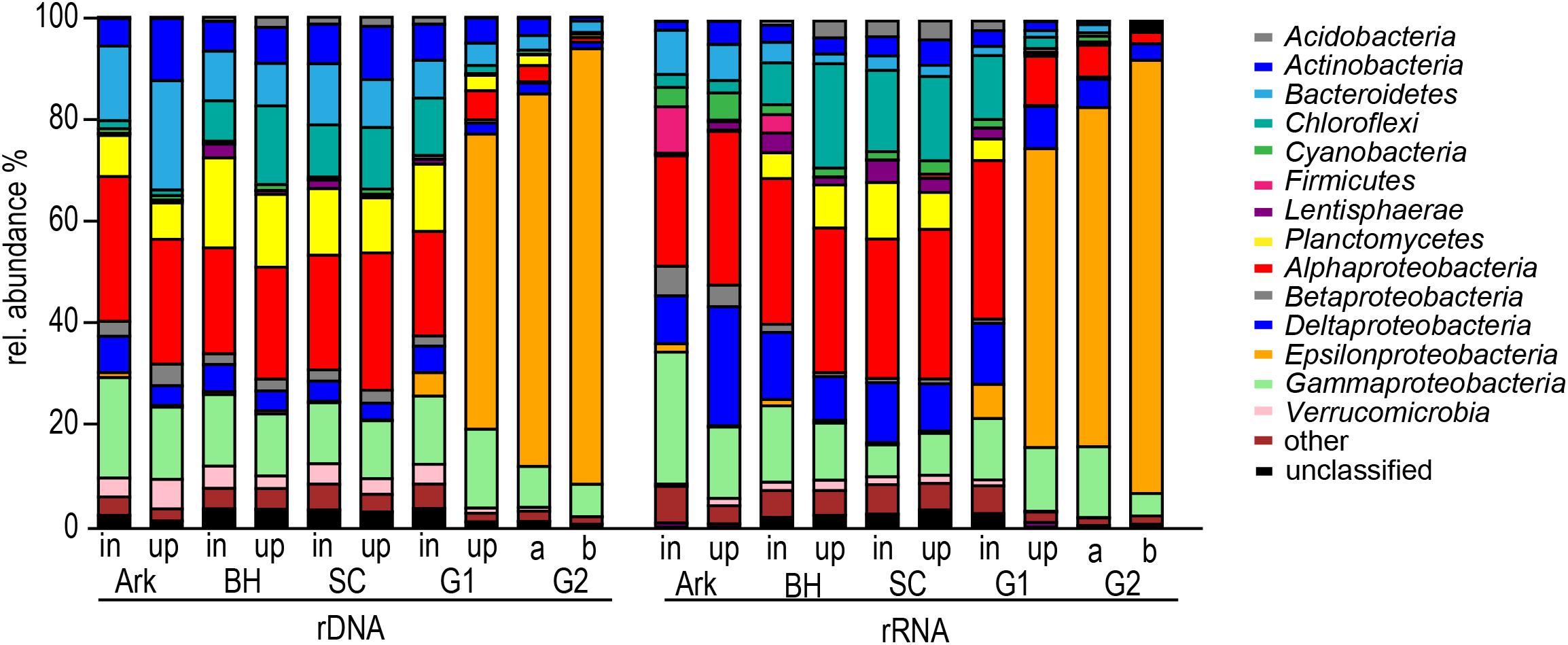
FIGURE 6. Bacterial community composition on the phylum/class level, mean values for the stations in the Arkona Basin (Ark, n = 2), Bornholm Basin (BH, n = 10), and Slupsk Channel (SC, n = 2), and single values for stations G1 and G2. As the MBI had not reached G2, data from two depths are shown for this station (a: 126 m, b: 120 m).
Distribution and rRNA/rDNA Ratios of Dominant OTUs
For the most abundant OTUs (>0.5% relative abundance across all stations), the relative proportions at the different stations were visualized in heatmaps. Similar trends were detected in the data from the two analyses, at the rDNA (Supplementary Figure 6) and rRNA level (Figure 7), but the latter showed a more pronounced pattern for some dominant OTUs and is therefore presented here. ANOSIM revealed significant differences at the rDNA OTU level (R = 0.721) between the inflow-water and uplifted bottom-water communities. Fifteen OTUs from various phyla were determined to be significantly enriched in the inflowing water bodies across the stations sampled (Supplementary Table 1). For some OTUs a characteristic alternating sequence between inflowing and uplifted waters became visible, as best seen for the OTU “unclassified SAR202 clade,” belonging to the Chloroflexi. In the inflowing water from the Arkona Basin this OTU was present in only modest abundance whereas it became the most abundant taxon in the uplifted water of the different stations in the Bornholm Basin, indicating that it dominated the bottom-waters of this basin, before being uplifted by the inflow. Alternating abundances in the inflowing and uplifted waters were also partly visible for other taxa such as the OTUs “unclassified Rhodospirillaceae” and “unclassified OM27.” There were also OTUs, such as the alphaproteobacterial OTU “unclassified T9d,” and the OTU “unclassified WCHB1,” that showed a reverse alternating pattern, with a higher abundance in the inflowing and a lower abundance in the uplifted water (Figure 7). A third pattern was exemplified by the OTU “unclassified Nitrospina,” a widespread marine nitrite oxidizer (Lücker et al., 2013). For this as well as a few other OTUs, a successive decline in relative abundance was determined, probably linked to the dilution of inflowing with existing water masses. The OTU “unclassified SAR86,” belonging to a widespread marine gammaproteobacterial group, was successively diluted and no longer detectable at stations toward the end of the Bornholm Basin (Figure 7). By contrast, the OTU Nitrospina declined with inflow propagation but was still detectable in considerable abundance in the inflowing water bodies. The epsilonproteobacterial OTU Sulfurimonas was present only in the Gotland Deep stations, but with a relative abundance > 55%, accompanied by only few other taxa.
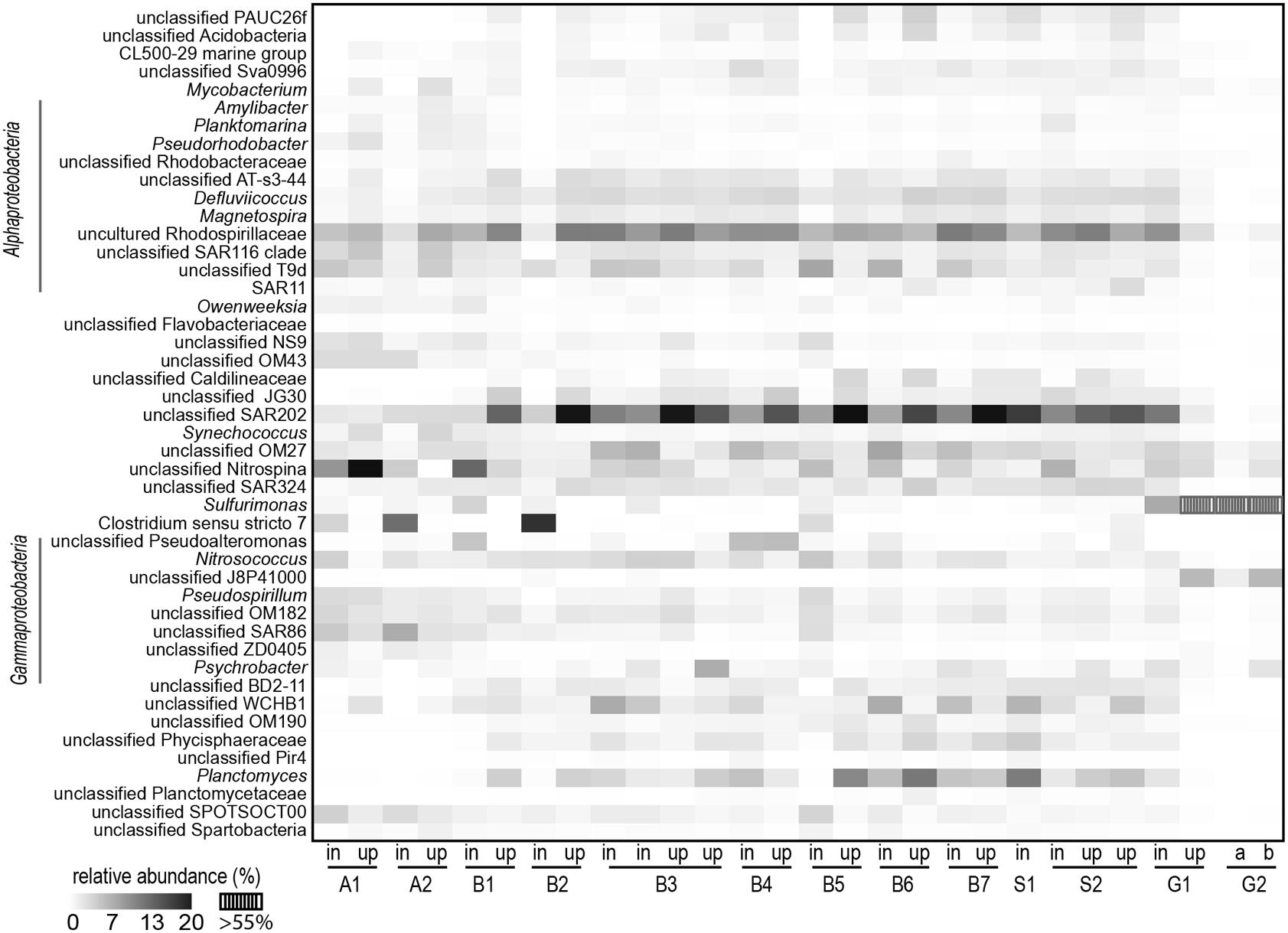
FIGURE 7. Heatmap of the abundant (total relative abundance > 0.5%) OTUs, based on the rRNA analysis, found in the inflow and uplifted water samples of all stations.
The rRNA/rDNA ratios for dominant bacterial OTUs in the different water masses and stations were determined, taking into account only those OTUs with a relative abundance of all sequence reads of >0.5% at both the rRNA and rDNA levels. The overall range in rRNA/rDNA ratios for the OTUs that met these criteria was quite large (Supplementary Table 2). Due to the variability between stations and because data were not available for all inflow/uplift pairs, the mean values for all inflow water bodies were not significantly different from those of the uplifting water bodies for an of the OTUs (Supplementary Table 2). However, several OTUs underwent strong changes between the inflow and uplifted water at some stations. For example, at the two Arkona basin stations (A1, A2), in the uplifted water there was a large increase in the ratio for the OTUs “unclassified Rhodospirillaceae,” “unclassified SAR116,” and Nitrospina (Supplementary Figure 7). For the latter, the ratio first increased by a factor of 5–7, but it then decreased in the Bornholm basin and leveled off to values mostly in the range of 2–4.
Discussion
Bacterial communities in estuaries and coastal margins differ spatially in their composition because of the prevalent gradients in salinity, nutrients, and the other components needed for bacterial growth. However, most estuaries are shaped by hydrodynamic forces, which are responsible for the mixing and characteristic short retention times of their waters. By contrast, in the Baltic Sea, the long water residence time (>5 years) (Reissmann et al., 2009) allows the adaptation of freshwater bacteria to brackish conditions (Riemann et al., 2008) and the coexistence of marine and freshwater lineages, together with presumed brackish water clades, in waters with a salinity of around 7 (Herlemann et al., 2011). Although several studies have addressed the distribution of freshwater and marine bacterial lineages in the Baltic Sea (Riemann et al., 2008; Herlemann et al., 2011, 2014; Piwosz et al., 2013; Bergen et al., 2014), the extent to which MBIs transport typical marine bacterial communities into the brackish parts of the Baltic Sea is unknown, as is the impact of inflowing water masses of relatively high salinity on the composition, distribution and activity of bacterioplankton during the transit of inflow-driven water bodies into the central Baltic Sea. This study is the first to address these questions, by taking advantage of the large MBI of 2014, investigated in detail with respect to its magnitude and temporal development, to link microbiological investigations with the hydrographic and modeling studies. Our data on the bacterioplankton communities, obtained using both rRNA- and rDNA-based community analyses, indicate a high degree of mixing of the inflowing water with the former bottom-waters, a conclusion consistent with the numerical modeling results. Moreover, we were able to show that, among the different water masses, specific bacterial taxa undergo changes in abundance and in their rRNA/rDNA ratios. We were also able to document an example of the displacement by the inflowing water of an entire bacterial assemblage into other water layers.
Characterization of the MBI Water Bodies
The development and dynamics of the 2014 MBI were investigated and followed in a series of cruises preceding our study. The data were complemented by CTD measurements of the permanent autonomous stations of the German Marine Monitoring network (MARNET), located at the Darss Sill and the Arkona Basin (Mohrholz et al., 2015), the autonomous Gotland Deep Environmental Sampling Station (GODESS) (Holtermann et al., 2017), and numerical modeling (Gräwe et al., 2015). The 2014 MBI is thus one of the best monitored MBIs in the Baltic Sea to date, with reliable estimates of inflow volume, as well as oxygen and salt transport. The data provided support for our proposed sequence of stations along which deep water transport and the uplifting of bottom-water presumably occurred (Figure 1).
An evaluation of the impact of the MBI on bacterioplankton communities required as a prerequisite a clear picture of the water mass transport through the western Baltic Sea and the deep basins. CTD measurements, in combination with an eddy-resolving hydrodynamic model, reproduced the timing and pattern of the inflowing saline water and revealed its successive mixing with the stagnant bottom-water on its way toward the interior of the Baltic Sea (Gräwe et al., 2015). The temporal evolution of salinity and temperature suggested that already one-third of the inflowing water had been mixed away by the time it reached the Bornholm Channel (Gräwe et al., 2015), the first sampling site of this study. The back-trajectory of the water masses sampled in the Arkona and Bornholm Basins suggested that the origin of the inflowing water was in the area between the Skagerrak and Danish Belt Sea. The widespread pattern of potential origins (Figure 4) indicated a high degree of mixing, both vertical and horizontal. However, given the extraordinary size of this inflow event, this strong mixing was not surprising.
When saline water enters the basins and uplifts existing bottom-water, some mixing of these water masses has to be assumed. The effects on bacterial assemblages are two-fold: first, different bacterial communities from the two water bodies are mixed; second, inputs of substrates and/or electron acceptors (oxygen, nitrate) may change the growth conditions of some taxa and modulate their activities. For the Bornholm Basin during the 2014 MBI, the situation was more complex because the uplifted bottom-water was not identical to the bottom-water present during the prolonged stagnation period in the Baltic Sea (2003–2014) that preceded the 2014 MBI. Rather, it already consisted of a mixture with the warmer water that derived from several smaller inflows into the Bornholm Basin earlier that same year (Naumann, 2015).
This study provides a snapshot of the MBI dynamics in February 2015, when the inflowing water had reached the southern edge of the Gotland Basin (station G1) but not yet the central basin. Later on, the MBI continued into the Gotland Basin and was followed by additional, smaller inflows, and two moderate MBIs in November 2015 and January–February 2016 (Naumann et al., unpublished data).
Impact of the MBI on the Composition and Distribution of the Baltic Sea Bacterial Communities on a Broad Phylogenetic Level
The relatively stable Baltic Sea salinity gradient, ranging from marine conditions in the Skagerrak to nearly limnic conditions in the Gulf of Bothnia, is an ideal natural model system to examine how salinity impacts the composition and functions of bacterioplankton communities. Studies within the last several years have revealed that Baltic Sea salinity determines the bacterial composition at broad phylogenetic levels, with Alpha- and Gamma-proteobacteria dominating at more marine, and Betaproteobacteria and Actinobacteria at more limnic conditions (Herlemann et al., 2011). Community shifts at the phylum level occur in the brackish area of the Baltic Sea within a critical salinity range of 5–8 (Herlemann et al. (2011). Although strong seasonal shifts in bacterial composition have been documented (Andersson et al., 2009), data collected across the whole Baltic Sea imply that salinity provides a stronger phylogenetic signal in bacterial community composition than seasonality (Herlemann et al., 2016). Moreover, metagenomic analyses indicate that salinity-related changes in bacterial community composition are associated with changes in metabolic functions (Dupont et al., 2014).
These insights into bacterial composition along the salinity gradient suggest that any inflow of saline waters into the Baltic Sea will also import different (i.e., mainly marine taxa) bacterial communities into the central Baltic Sea. However, the continuous entrainment of overlying water during the passage of new, deep water from the North Sea into the Baltic Sea’s basins also results in a successive dilution of the original communities. Therefore, the high similarity between the communities of the inflowing and former bottom-waters (uplifted) in the Arkona and Bornholm Basins, at least on a broad phylogenetic level (Figure 6), was not really surprising. This similarity was evident from not only the rDNA but also the rRNA analysis (Figure 6B); rRNA-based analyses have generally been used to assess the more “active” fraction of prokaryotes (e.g., Hunt et al., 2013). The successive dilution of inflowing water was also suggested by numerical modeling of the water transport (Figure 4). Other factors might also enhance the removal of imported marine taxa, such as inhibition due to more unfavorable conditions and losses due to viral lysis and grazing. Furthermore, the state of the deep water of the Bornholm Basin had probably already slightly changed compared to the previous stagnant conditions, due to smaller inflows of warmer water in 2014 that had mixed with old bottom-water. Despite these changes in the bottom-waters, the bacterial community compositions were rather similar to those of the reference data from 2009, as seen in the clustering of samples in the NMDS plots (Figure 5A). However, an inspection of only the Bornholm Basin stations, for which the most samples had been gathered, revealed obvious differences in the communities of the inflowing and uplifted water masses, both at the rDNA and the rRNA level (Figure 5B).
The most profound impact of the inflow water was visible in the Gotland Basin, which also represented the former stagnant water body and associated microbial communities because it had not been reached by the previous smaller inflows that occurred in 2004 (Naumann et al., unpublished data). In the Gotland Basin, a bacterial assemblage typical for the anoxic waters of the central Baltic Sea (e.g., Labrenz et al., 2007) was largely replaced by one that resembled the communities found in the adjacent oxygenated basins (Arkona, Bornholm) (Figure 6). Epsilonproteobacteria, which dominate near oxic-anoxic interfaces in the Baltic Sea and depend on the availability of sulfide and nitrate for chemoautotrophic denitrification (Grote et al., 2012), were a good indicator of the former anaerobic community. The inflowing saline water mass uplifted the former oxygen-deficient bottom-water, and thus also its anaerobic bacterial community. Interestingly, Epsilonproteobacteria were found, in a small proportion (5–7%), also in the inflowing water of the southern Gotland station (G1) (Figure 6). As this bacterial group was not detected at the (oxic) stations before G1, its proportion can be considered indicative of the degree of mixing that occurred when the inflowing water uplifted the existing bottom-water. This observation demonstrates that in addition to ventilating the Baltic Sea’s deep basins (Omstedt et al., 2004) MBIs can directly shift bacterial communities to water strata where they are normally not found.
Impact of the MBI on the Composition and Distribution of the Baltic Sea Bacterial Communities on a Fine Phylogenetic Level
The Epsilonproteobacteria in the oxygen-deficient basins of the Baltic Sea consist nearly entirely of the Sulfurimonas group (Grote et al., 2012). In the oxic water column, Sulfurimonas spp. may serve as a strong indicator of displaced anoxic bottom-water, as this taxon is able to survive in the presence of oxygen but only proliferates around the oxic-anoxic interface (Grote et al., 2012). The previous appearance of Sulfurimonas in Baltic Sea surface waters, together with other anaerobic taxa, has been interpreted as the result of an upwelling event, that transported chemocline water to the surface (Lindh et al., 2015).
At a finer phylogenetic resolution (OTUs), further differences between the studied water masses became apparent, including the enrichment of several taxa in the inflow water compared to the former bottom-water (Supplementary Table 1). These taxa mainly belonged to Bacteroidetes, Chloroflexi, Planctomycetes, and Alpha-, Delta-, and Gamma-proteobacteria (Supplementary Table 1), with some of them related to known marine taxa (e.g., Brown et al., 2009). The SAR202 clade within the Chloroflexi is widespread in the mesopelagic and deep ocean (Morris et al., 2004) but has also been detected in high abundance in Baltic Sea sediments (Klier et al., 2018). This suggests an input of Chloroflexi cells from sediments into the water column, driven by the turbulence generated by inflowing water. The resuspension of sediments due to inflowing water has been observed (Naumann et al., unpublished data). Planctomycetes diversity was high in the inflow water. Members of this group have been found in the Baltic Sea, including two potential anaerobic ammonium-oxidizing phylotypes from the suboxic zone of the central Baltic (Brettar et al., 2012) and several related OTUs in the sea’s brackish and marine areas (Herlemann et al., 2011). An analysis by Rieck et al. (2015) of the particle-associated bacterial communities in three salinity zones of the Baltic Sea showed a dominance of particle-associated Planctomycetes in the central Baltic. These Planctomycetes mainly consisted of the OTU CL500-3, which was also enriched in the inflow water of this study. The origin and reason for the enrichment of this group in the inflow water are unclear but a broader distribution than previously recognized seems probable. The Alphaproteobacteria enriched in the inflow water mainly comprised Rhodobacteraceae and Rhodospirillaceae. Enriched Gammaproteobacteria were exclusively represented by Oceanospirillales. These groups have also been detected in marine areas of the Baltic Sea (Herlemann et al., 2011) and their immigration with the inflow water was therefore likely.
At the OTU level, interesting patterns along the inflow stations became apparent (Figure 7). Whereas some OTUs were successively diluted with progression of the inflow, others showed alternating abundances between the inflow and uplifted water bodies, indicating that they had occurred in higher abundance in the bottom-water and became uplifted by the inflow. The higher overall OTU richness (alpha diversity) in the Bornholm and Arkona Basins during our study compared to the reference samples from 2009, based on the rDNA analysis, also indicated that partial mixing of different water bodies with different bacterial communities increased the overall bacterial diversity (Supplementary Figure 3).
rRNA/rDNA Ratios
The parallel analysis of rDNA- and rRNA-based community composition also allowed calculation of the rRNA/rDNA ratios of the dominant OTUs. This ratio has been used to distinguish the different growth strategies of marine bacteria (oligotrophs vs. copiotrophs) (Lankiewicz et al., 2015), but also to decipher seasonal changes in the activity status of specific taxa as well as differences between free-living and particle-associated OTUs (Denef et al., 2016). The dominant bacterial OTUs for which a reliable sequence threshold was reached for both rRNA and rDNA all showed a considerable variation in this ratio, but within the range determined in other studies (e.g., Denef et al., 2016). Although the average values of these ratios did not significantly differ between inflowing and uplifted water bodies (Supplementary Table 2), the pattern across the stations suggested strong changes in some of the taxa between these water bodies (Supplementary Figure 5). This, together with the large changes in relative abundance along the stations (Figure 7) probably indicates different growth conditions in the different water bodies.
A striking example of differing rRNA/rDNA ratios was Nitrospina, for which large increases in its ratios in the uplifted water of the Arkona Basin were determined (Supplementary Figure 5). The genus Nitrospina is a widespread marine nitrite oxidizer, mainly below the euphotic zone and in sediments (Ngugi et al., 2015; Daims et al., 2016). This OTU may have been transported with the inflow water, and its potential activity (e.g., nitrite oxidation) was enhanced by the newly encountered nutrient conditions. Indeed, the concentrations of inorganic nitrogen compounds in the inflow water differed from those usually found in the study area (Supplementary Figure 2). The low concentrations of ammonia and nitrite and the high concentration of nitrate might have resulted from enhanced nitrification in the inflow water. Ammonia-oxidizing bacteria were not detected in higher abundance in our samples, but it is known that ammonia oxidation in the deeper waters of the Baltic Sea is dominated by ammonia-oxidizing archaea (Berg et al., 2015), which were not covered by our primers.
Conclusion
This study is the first to describe the impact of an MBI on the composition and distribution of Baltic Sea bacterioplankton. Our results provide evidence of two major mechanisms that explain the observed impact on the bacterial communities of the central Baltic Sea basins: first, successive mixing of inflowing saline water along the transit through the Baltic Sea resulted in relatively similar bacterial assemblages between inflowing and former bottom-water communities on a broad phylogenetic level. Therefore, a typical marine bacterial assemblage did not arrive by the MBI in the central Baltic Sea. However, significant community changes were apparent at the OTU level, revealing the enrichment of certain taxa with the inflowing water, different patterns of changing abundance and varying rRNA/rDNA ratios in the different water bodies. Second, the uplifting of former bottom communities, with their key indicator taxa, resulted in their displacement into different water strata. This was best illustrated by the arrival of the 2014 MBI at a station in the Gotland Basin, where the former anoxic bottom community, dominated by Epsilonproteobacteria, was shifted to shallower water depths. The implications of these shifts in water masses and microbial communities for microbially mediated ecosystem functions are not yet fully understood. However, they can perhaps be determined in studies that examine the functional performance of key bacterial taxa and their physiological changes in response to mixing with inflowing water or to the uplifting to new water layers with different environmental conditions.
Ethics Statement
All authors declare that the research was conducted in the absence of any commercial or financial relationships that could be construed as a potential conflict of interest. This article does not contain any studies with human participants or animals performed by any of the authors.
Author Contributions
BB, MN, ML, and KJ planned, initiated, and conducted the study. MN gathered and provided hydrographic data. UG conducted the backtracking numerical model. DH helped with the analysis of bacterial diversity data. BB and KJ wrote the first draft of the manuscript. All authors discussed the results and edited the manuscript.
Funding
This work was funded by the Deutsche Forschungsgemeinschaft (DFG) (projects JU367/15-1, JU367/16-1 to KJ and LA1466/8-1 to ML). DH was supported by the European Regional Development Fund and the Estonian Research Council Mobilitas Plus Top Researcher grant “MOBTT24.” UG was supported by the BMBF project “Hydrodynamic observations and simulations of munition in the sea,” a subproject of the collaborative project “Environmental monitoring for the delaboration of munitions in the sea” (Grant No. #03F0747C).
Conflict of Interest Statement
The authors declare that the research was conducted in the absence of any commercial or financial relationships that could be construed as a potential conflict of interest.
Acknowledgments
We thank the captain and crew of the RV Elisabeth Mann Borgese for assistance in sampling during the EMB 95 cruise. The sampling campaign was done within the framework of the IOW’s long-term data program, which was quickly adapted to take advantage of the special inflow situation with additional “task force inflow” sampling. We also thank Stephanie Mothes for technical assistance at sea.
Supplementary Material
The Supplementary Material for this article can be found online at: https://www.frontiersin.org/articles/10.3389/fmars.2018.00383/full#supplementary-material
Footnotes
References
Andersson, A. F., Riemann, L., and Bertilsson, S. (2009). Pyrosequencing reveals contrasting seasonal dynamics of taxa within Baltic Sea bacterioplankton communities. ISME J. 4, 171–181. doi: 10.1038/ismej.2009.108
Berg, C., Vandieken, V., Thamdrup, B., and Jürgens, K. (2015). Significance of archaeal nitrification in hypoxic waters of the Baltic Sea. ISME J. 9, 1319–1332. doi: 10.1038/ismej.2014.218
Bergen, B., Herlemann, D. P. R., Labrenz, M., and Jürgens, K. (2014). Distribution of the verrucomicrobial clade S partobacteria along a salinity gradient in the Baltic Sea. Environ. Microbiol. Rep. 6, 625–630. doi: 10.1111/1758-2229.12178
Bouvier, T. C., and Giorgio, P. A. (2002). Compositional changes in free-living bacterial communities along a salinity gradient in two temperate estuaries. Limnol. Oceanogr. 47, 453–470. doi: 10.4319/lo.2002.47.2.0453
Brettar, I., Christen, R., and Höfle, M. G. (2012). Analysis of bacterial core communities in the central Baltic by comparative RNA–DNA-based fingerprinting provides links to structure–function relationships. ISME J. 6, 195–212. doi: 10.1038/ismej.2011.80
Brown, M. V., Philip, G. K., Bunge, J. A., Smith, M. C., Bissett, A., Lauro, F. M., et al. (2009). Microbial community structure in the North Pacific ocean. ISME J. 3, 1374–1386. doi: 10.1038/ismej.2009.86
Burchard, H., Lass, H. U., Mohrholz, V., Umlauf, L., Sellschopp, J., Fiekas, V., et al. (2005). Dynamics of medium-intensity dense water plumes in the Arkona Basin, Western Baltic Sea. Ocean Dyn. 55, 391–402. doi: 10.1007/s10236-005-0025-2
Daims, H., Lücker, S., and Wagner, M. (2016). A new perspective on microbes formerly known as nitrite-oxidizing bacteria. Trends Microbiol. 24, 699–712. doi: 10.1016/j.tim.2016.05.004
Dellwig, O., Schnetger, B., Meyer, D., Pollehne, F., Häusler, K., and Arz, H. W. (2018). Impact of the major baltic inflow in 2014 on manganese cycling in the Gotland Deep (Baltic Sea). Front. Mar. Sci. 5:248. doi: 10.3389/fmars.2018.00248
Denef, V. J., Fujimoto, M., Berry, M. A., and Schmidt, M. L. (2016). Seasonal succession leads to habitat-dependent differentiation in ribosomal RNA:DNA ratios among freshwater lake bacteria. Front. Microbiol. 7:606. doi: 10.3389/fmicb.2016.00606
Dupont, C. L., Larsson, J., Yooseph, S., Ininbergs, K., Goll, J., Asplund-Samuelsson, J., et al. (2014). Functional tradeoffs underpin salinity-driven divergence in microbial community composition. PLoS One 9:e89549. doi: 10.1371/journal.pone.0089549
Fortunato, C. S., and Crump, B. C. (2011). Bacterioplankton community variation across river to ocean environmental gradients. Microb. Ecol. 62, 374–382. doi: 10.1007/s00248-011-9805-z
Gasol, J. M., and del Giorgio, P. A. (2000). Using flow cytometry for counting natural planktonic bacteria and understanding the structure of planktonic bacterial communities. Sci. Mar. 64, 197–224. doi: 10.3989/scimar.2000.64n2197
Glöckner, F. O., Yilmaz, P., Quast, C., Gerken, J., Beccati, A., Ciuprina, A., et al. (2017). 25 years of serving the community with ribosomal RNA gene reference databases and tools. J. Biotechnol. 261, 169–176. doi: 10.1016/j.jbiotec.2017.06.1198
Grasshoff, K., Ehrhardt, M., and Kremling, K. (1983). Methods of Seawater Analysis, 2nd Edn. Weinheim: Verlag Chemie.
Gräwe, U., Friedland, R., and Burchard, H. (2013). The future of the western Baltic Sea: two possible scenarios. Ocean Dyn. 63, 901–921. doi: 10.1007/s10236-013-0634-0
Gräwe, U., Naumann, M., Mohrholz, V., and Burchard, H. (2015). Anatomizing one of the largest saltwater inflows into the Baltic Sea in December 2014. J. Geophys. Res. Ocean 120, 7676–7697. doi: 10.1002/2015JC011269
Grote, J., Schott, T., Bruckner, C. G., Glckner, F. O., Jost, G., Teeling, H., et al. (2012). Genome and physiology of a model Epsilonproteobacterium responsible for sulfide detoxification in marine oxygen depletion zones. Proc. Natl. Acad. Sci. U.S.A. 109, 506–510. doi: 10.1073/pnas.1111262109
Hall, P. O. J., Almroth Rosell, E., Bonaglia, S., Dale, A. W., Hylén, A., Kononets, M., et al. (2017). Influence of natural oxygenation of Baltic proper deep water on benthic recycling and removal of phosphorus, nitrogen, silicon and carbon. Front. Mar. Sci. 4:27. doi: 10.3389/fmars.2017.00027
Hammer,Ø, Harper, D. A. T., and Ryan, P. D. (2001). PAST: paleontological statistics software package for education and data analysis. Palaeontol. Electron. 4:9.
Herlemann, D. P. R., Labrenz, M., Jürgens, K., Bertilsson, S., Waniek, J. J., and Andersson, A. F. (2011). Transitions in bacterial communities along the 2000 km salinity gradient of the Baltic Sea. ISME J. 5, 1571–1579. doi: 10.1038/ismej.2011.41
Herlemann, D. P. R., Lundin, D., Andersson, A. F., Labrenz, M., and Jürgens, K. (2016). Phylogenetic signals of salinity and season in bacterial community composition across the salinity gradient of the Baltic Sea. Front. Microbiol. 7:1883. doi: 10.3389/fmicb.2016.01883
Herlemann, D. P. R., Woelk, J., Labrenz, M., and Jürgens, K. (2014). Diversity and abundance of “Pelagibacterales” (SAR11) in the Baltic Sea salinity gradient. Syst. Appl. Microbiol. 37, 601–604. doi: 10.1016/j.syapm.2014.09.002
Holtermann, P. L., Prien, R., Naumann, M., Mohrholz, V., and Umlauf, L. (2017). Deepwater dynamics and mixing processes during a major inflow event in the central Baltic Sea. J. Geophys. Res. 122, 6648–6667. doi: 10.1002/2017JC013050
Hunt, D. E., Lin, Y., Church, M. J., Karl, D. M., Tringe, S. G., Izzo, L. K., et al. (2013). Relationship between abundance and specific activity of bacterioplankton in open ocean surface waters. Appl. Environ. Microbiol. 79, 177–184. doi: 10.1128/AEM.02155-12
Kirchman, D. L., Dittel, A. I., Malmstrom, R. R., and Cottrell, M. T. (2005). Biogeography of major bacterial groups in the Delaware estuary. Limnol. Oceanogr. 50, 1697–1706. doi: 10.4319/lo.2005.50.5.1697
Klier, J., Dellwig, O., Leipe, T., Jürgens, K., and Herlemann, D. P. R. (2018). Benthic bacterial community composition in the oligohaline-marine transition of surface sediments in the Baltic Sea based on rRNA analysis. Front. Microbiol. 9:236. doi: 10.3389/fmicb.2018.00236
Kuss, J., Cordes, F., Mohrholz, V., Nausch, G., Naumann, M., Krüger, S., et al. (2017). The impact of the major Baltic inflow of December 2014 on the mercury species distribution in the Baltic Sea. Environ. Sci. Technol. 51, 11692–11700. doi: 10.1021/acs.est.7b03011
Labrenz, M., Jost, G., and Jürgens, K. (2007). Distribution of abundant prokaryotic organisms in the water column of the central Baltic Sea with an oxic-anoxic interface. Aquat. Microb. Ecol. 46, 177–190. doi: 10.3354/ame046177
Laine, A. O., Sandler, H., Andersin, A.-B., and Stigzelius, J. (1997). Long-term changes of macrozoobenthos in the Eastern Gotland Basin and the Gulf of Finland (Baltic Sea) in relation to the hydrographical regime. J. Sea Res. 38, 135–159. doi: 10.1016/S1385-1101(97)00034-8
Lankiewicz, T. S., Cottrell, M. T., and Kirchman, D. L. (2015). Growth rates and rRNA content of four marine bacteria in pure cultures and in the Delaware estuary. ISME J. 10, 823–832. doi: 10.1038/ismej.2015.156
Lilover, M.-J., Elken, J., Suhhova, I., and Liblik, T. (2017). Observed flow variability along the thalweg, and on the coastal slopes of the Gulf of Finland, Baltic Sea. Estuar. Coast. Shelf Sci. 195, 23–33. doi: 10.1016/j.ecss.2016.11.002
Lindh, M. V., Sjöstedt, J., Andersson, A. F., Baltar, F., Hugerth, L. W., Lundin, D., et al. (2015). Disentangling seasonal bacterioplankton population dynamics by high-frequency sampling. Environ. Microbiol. 17, 2459–2476. doi: 10.1111/1462-2920.12720
Lozupone, C. A., and Knight, R. (2007). Global patterns in bacterial diversity. Proc. Natl. Acad. Sci. U.S.A. 104, 11436–11440. doi: 10.1073/pnas.0611525104
Lücker, S., Nowka, B., Rattei, T., Spieck, E., and Daims, H. (2013). The genome of Nitrospina gracilis illuminates the metabolism and evolution of the major marine nitrite oxidizer. Front. Microbiol. 4:27. doi: 10.3389/fmicb.2013.00027
Matthäus, W., and Franck, H. (1992). Characteristics of major Baltic inflows - a statistical analysis. Cont. Shelf Res. 12, 1375–1400. doi: 10.1016/0278-4343(92)90060-W
Mohrholz, V., Naumann, M., Nausch, G., Krüger, S., and Gräwe, U. (2015). Fresh oxygen for the Baltic Sea—An exceptional saline inflow after a decade of stagnation. J. Mar. Syst. 148, 152–166. doi: 10.1016/j.jmarsys.2015.03.005
Morris, R. M., Rappé, M. S., Urbach, E., Connon, S. A., and Rappe, M. S. (2004). Prevalence of the Chloroflexi-related SAR202 bacterioplankton cluster throughout the mesopelagic zone and deep ocean. Appl. Environ. Microbiol. 70, 2836–2842. doi: 10.1128/AEM.70.5.2836
Naumann, M. (2015). Cruise Report r/v E. Mann-Borgese No. 100 – SECOS Project & MBI December 2014 Research Cruise, Leibniz-Institute for Baltic Sea Research. Available at: http://www.io-warnemuende.de/tl_files/forschung/pdf/cruise-reports/cremb100.pdf
Nemergut, D. R., Costello, E. K., Hamady, M., Lozupone, C., Jiang, L., Schmidt, S. K., et al. (2011). Global patterns in the biogeography of bacterial taxa. Environ. Microbiol. 13, 135–144. doi: 10.1111/j.1462-2920.2010.02315.x
Ngugi, D. K., Blom, J., Stepanauskas, R., and Stingl, U. (2015). Diversification and niche adaptations of Nitrospina-like bacteria in the polyextreme interfaces of Red Sea brines. ISME J. 10, 1383–1399. doi: 10.1038/ismej.2015.214
Omstedt, A., Pettersen, C., Rodhe, J., and Winsor, P. (2004). Baltic Sea climate: 200 yr of data on air temperature, sea level variation, ice cover, and atmospheric circulation. Clim. Res. 25, 205–216. doi: 10.3354/cr025205
Piwosz, K., Salcher, M. M., Zeder, M., Ameryk, A., and Pernthaler, J. (2013). Seasonal dynamics and activity of typical freshwater bacteria in brackish waters of the Gulf of Gdansk. Limnol. Oceanogr. 58, 817–826. doi: 10.4319/lo.2013.58.3.0817
Pruesse, E., Peplies, J., and Glöckner, F. O. (2012). SINA: accurate high-throughput multiple sequence alignment of ribosomal RNA genes. Bioinformatics 28, 1823–1829. doi: 10.1093/bioinformatics/bts252
Pruesse, E., Quast, C., Knittel, K., Fuchs, B. M., Ludwig, W., Peplies, J., et al. (2007). SILVA: a comprehensive online resource for quality checked and aligned ribosomal RNA sequence data compatible with ARB. Nucleic Acids Res. 35, 7188–7196. doi: 10.1093/nar/gkm864
Quast, C., Pruesse, E., Yilmaz, P., Gerken, J., Schweer, T., Yarza, P., et al. (2013). The SILVA ribosomal RNA gene database project: improved data processing and web-based tools. Nucleic Acids Res. 41, 590–596. doi: 10.1093/nar/gks1219
Reissmann, J. H., Burchard, H., Feistel, R., Hagen, E., Lass, H. U., Mohrholz, V., et al. (2009). Vertical mixing in the Baltic Sea and consequences for eutrophication - A review. Prog. Oceanogr. 82, 47–80. doi: 10.1016/j.pocean.2007.10.004
Rieck, A., Herlemann, D. P. R., Jürgens, K., and Grossart, H.-P. (2015). Particle-associated differ from free-living bacteria in surface waters of the Baltic Sea. Front. Microbiol. 6:1297. doi: 10.3389/fmicb.2015.01297
Riemann, L., Leitet, C., Pommier, T., Simu, K., Holmfeldt, K., Larsson, U., et al. (2008). The native bacterioplankton community in the central Baltic Sea is influenced by freshwater bacterial species. Appl. Environ. Microbiol. 74, 503–515. doi: 10.1128/AEM.01983-07
Robertson, C. E., Harris, J. K., Wagner, B. D., Granger, D., Browne, K., Tatem, B., et al. (2013). Explicet: graphical user interface software for metadata-driven management, analysis and visualization of microbiome data. Bioinformatics 29, 3100–3101. doi: 10.1093/bioinformatics/btt526
Schmale, O., Krause, S., Holtermann, P., Power Guerra, N. C., and Umlauf, L. (2016). Dense bottom gravity currents and their impact on pelagic methanotrophy at oxic/anoxic transition zones. Geophys. Res. Lett. 43, 5225–5232. doi: 10.1002/2016GL069032
Segata, N., Izard, J., Waldron, L., Gevers, D., Miropolsky, L., Garrett, W. S., et al. (2011). Metagenomic biomarker discovery and explanation. Genome Biol. 12:R60. doi: 10.1186/gb-2011-12-6-r60
Sommer, S., Clemens, D., Yücel, M., Pfannkuche, O., Hall, P. O. J., Almroth-Rosell, E., et al. (2017). Major bottom water ventilation events do not significantly reduce basin-wide benthic N and P release in the Eastern Gotland Basin (Baltic Sea). Front. Mar. Sci. 4:18. doi: 10.3389/fmars.2017.00018
Umlauf, L., Arneborg, L., Burchard, H., Fiekas, V., Lass, H. U., Mohrholz, V., et al. (2007). Transverse structure of turbulence in a rotating gravity current. Geophys. Res. Lett. 34:08601. doi: 10.1029/2007GL029521
Keywords: bacterioplankton, Baltic Sea, Major Baltic inflow, saltwater intrusions, RNA, DNA
Citation: Bergen B, Naumann M, Herlemann DPR, Gräwe U, Labrenz M and Jürgens K (2018) Impact of a Major Inflow Event on the Composition and Distribution of Bacterioplankton Communities in the Baltic Sea. Front. Mar. Sci. 5:383. doi: 10.3389/fmars.2018.00383
Received: 31 May 2018; Accepted: 28 September 2018;
Published: 26 October 2018.
Edited by:
Elinor Andrén, Södertörn University, SwedenReviewed by:
Pradeep Ram Angia Sriram, UMR 6023, Laboratoire Microorganismes Génome Et Environnement (LMGE), FranceAnyi Hu, Institute of Urban Environment (CAS), China
Copyright © 2018 Bergen, Naumann, Herlemann, Gräwe, Labrenz and Jürgens. This is an open-access article distributed under the terms of the Creative Commons Attribution License (CC BY). The use, distribution or reproduction in other forums is permitted, provided the original author(s) and the copyright owner(s) are credited and that the original publication in this journal is cited, in accordance with accepted academic practice. No use, distribution or reproduction is permitted which does not comply with these terms.
*Correspondence: Klaus Jürgens, a2xhdXMuanVlcmdlbnNAaW8td2FybmVtdWVuZGUuZGU=