- 1United States Environmental Protection Agency, National Exposure Research Laboratory, Research Triangle Park, NC, United States
- 2Maritime Studies Program, Williams College-Mystic Seaport, Mystic, CT, United States
- 3Department of Biology, Williams College, Williamstown, MA, United States
Recent years have witnessed growing appreciation for the ways in which human-mediated species introductions have reshaped marine biogeography. Despite this we have yet to grapple fully with the scale and impact of anthropogenic dispersal in both creating and determining contemporary distributions of marine taxa. In particular, the past several decades of research on marine biological invasions have revealed that broad geographic distributions of coastal marine organisms—historically referred to simply as “cosmopolitanism”—may belie complex interplay of both natural and anthropogenic processes. Here we describe a framework for understanding contemporary cosmopolitanism, informed by a synthesis of the marine bioinvasion literature. Our framework defines several novel categories in an attempt to provide a unified terminology for discussing cosmopolitan distributions in the world's oceans. We reserve the term eucosmopolitan to refer to those species for which data exist to support a true, natural, and prehistorically global (or extremely broad) distribution. While in the past this has been the default assumption for species observed to exhibit contemporary cosmopolitan distributions, we argue that given recent advances in marine invasion science this assignment should require positive evidence. In contrast, neocosmopolitan describes those species that have demonstrably achieved extensive geographic ranges only through historical anthropogenic dispersal, often facilitated over centuries of human maritime traffic. We discuss the history and human geography underpinning these neocosmopolitan distributions, and illustrate the extent to which these factors may have altered natural biogeographic patterns. We define the category pseudocosmopolitan to encompass taxa for which a broad distribution is determined (typically after molecular investigation) to reflect multiple, sometimes regionally endemic, lineages with uncertain taxonomic status; such species may remain cosmopolitan only so long as taxonomic uncertainty persists, after which they may splinter into multiple geographically restricted species. We discuss the methods employed to identify such species and to resolve both their taxonomic status and their biogeographic histories. We argue that recognizing these different types of cosmopolitanism, and the important role that invasion science has played in understanding them, is critically important for the future study of both historical and modern marine biogeography, ecology, and biodiversity.
“To trace the courses that Dennis, Barnstable, and Truro navigators pricked off between 1815 and 1850, would be to draw a network over all the oceans of the world.”
- Henry Kittredge, Cape Cod, Its People and Their History (1930, Houghton Mifflin)
The Need for a Typology of Cosmopolitanism
Students of marine biodiversity have long eyed the phenomenon of cosmopolitanism with some skepticism. Among the seeds of a fundamental shift in questioning the existence of cosmopolitan species were those planted by Grassle and Grassle (1976), who in a seminal paper announced that a classic example of a globally-distributed worm, Capitella capitata, in fact consisted of six sibling species in New England waters alone. Carlton (1975) commented that the “amazingly wide distributions of some marine organisms may reflect more the marvels of taxonomy than of nature.” And by the early 1980s polychaete systematist Mary Petersen was already referring to the “characteristic species disease” afflicting many polychaete taxa, a common ailment of what were misconstrued as single broadly distributed species, despite the fact that they often exhibited substantial variation throughout their ranges (Petersen, 1984). The paradigm of predominantly cosmopolitan distributions among marine annelids, in slow demise since the late 1970s, has now been partially supplanted by taxonomic descriptions of geographically restricted taxa (Hutchings and Kupriyanova, 2018), even as many polychaete species around the world still retain cosmopolitan nomenclature. Despite clear advances, the notion of cosmopolitanism among largely shallow water benthic marine species persists in many corners, in the face of growing apprehension that this may be a reflection less of biogeography than of taxonomic uncertainty. Observations ranging from “suspiciously cosmopolitan” algal species (Sherwood, 2007) to “implausible cosmopolitanism” of some bryozoan taxa (Harmelin et al., 2012) and “complexes of (pseudo-) cryptic species” among marine meiofauna (Van Steenkiste et al., 2018) all highlight the reluctance to shed a concept that has dominated thinking about marine biodiversity for nearly 200 years.
Mounting wariness of cosmopolitan distributions can be attributed to a number of factors, including increased and more internationally collaborative taxonomic efforts (Hutchings and Kupriyanova, 2018), recognition of overconservative systematics among many taxonomic groups (Klautau et al., 1999), and the advent and rapidly expanding accessibility of molecular genetic data (Brasier et al., 2016; Leray and Knowlton, 2016). But of critical general importance has also been the emergence and maturation of marine invasion biology as a field of scientific inquiry. The burgeoning literature describing anthropogenic introductions of marine taxa, similarly nascent in the late 1970s, has paralleled increasing circumspection directed at the paradigm of marine cosmopolitanism. This relationship can in part be traced to two related but separable aspects of marine invasion biology, each of which has influenced thinking about cosmopolitan species in different ways.
First, the past several decades of research have laid bare the extensive influence that humans have had on the global distribution of marine species. This realization is rooted not only in recognition of the importance of contemporary anthropogenic vectors of biotic homogenization and the ongoing role humans play in reshaping marine biogeography (Bax et al., 2003; Carlton and Ruiz, 2015), but also—as suggested by the above epigraph—in acknowledgement that humans have been playing that role for many centuries, if not millennia (Carlton, 2003; Gollasch et al., 2009; Rick et al., 2013). The extent of marine species introductions in the Anthropocene—that geological era dominated by the influence of our own species (Capinha et al., 2015)—may dwarf current estimates based solely on historical observations of novel incursions outside of known native ranges, as many human-mediated distributional shifts may have preceded by centuries any concerted attempts to catalog marine biodiversity (Carlton, 2009). Acceptance of this fact weighs heavily against the default assumption that observed contemporary cosmopolitanism must reflect solely the effects of natural processes driving community assembly over ecological and evolutionary time.
Second, greater attention leveled at the phenomenon of anthropogenic introductions of marine species—either for the sake of informing management of costly invasions (Darling, 2015; Darling et al., 2017) or as unique “experiments” offering insight into fundamental processes in evolution and ecology (Rius et al., 2015)—has led to considerable investment in attempts to reconstruct invasion histories. These attempts, powered often by application of increasingly sophisticated molecular genetic tools (Cristescu, 2015; Viard et al., 2016), have frequently unearthed unexpected diversity among widespread introduced marine species, leading naturally to questions about their taxonomic and biogeographic status (Geller et al., 2010). Such observations have become so commonplace that the literature is rich with papers hypothesizing cryptic species-level diversity among introduced marine taxa; one may be tempted to stop using the term “unexpected” to describe the diversity revealed by these investigations. Similar revelations have derived from general studies of marine biodiversity, with genetic evidence indicating that some groups harbor truly astonishing levels of cryptic diversity (Brasier et al., 2016). These discoveries, demonstrating the power of the molecular hammer to shatter species into multiple lineages of uncertain status, have only further eroded confidence in the phenomenon of marine cosmopolitanism.
We describe here a framework for understanding cosmopolitanism in the context of lessons learned over three decades of marine invasion biology. This effort is motivated by the conviction that we are in need of both a unified language to discuss marine cosmopolitanism and a common understanding of how to resolve the status of broadly distributed marine species. Our framework (Figure 1) thus comprises both a typology of cosmopolitanism and a structure for describing how changes in knowledge about marine species allow their categorization within this typology.
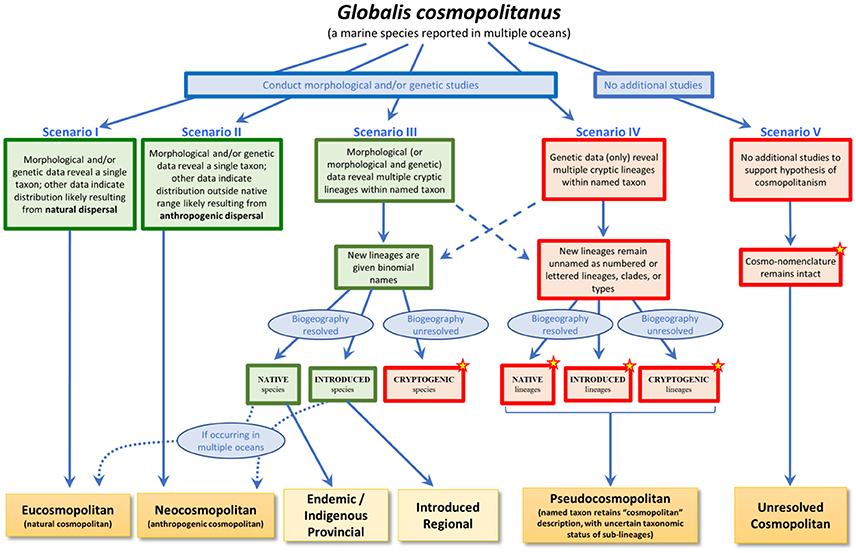
Figure 1. A framework for understanding the various types of cosmopolitanism. A species with an observed contemporary cosmopolitan distribution (the fictional Globalis cosmopolitanus, reported from multiple oceans) can fall into different categories depending on different trajectories of changing states of knowledge, represented by the five scenarios illustrated here. Red boxes indicate situations in which lineages are only identifiable by genetic means or otherwise remain unresolved either taxonomically or biogeographically; green boxes indicate situations in which lineages have binomial names and known biogeographic history. Stars identify those situations in which the number of introduced species is likely to be underestimated. Dashed arrows indicate the possibility that morphological and genetic data may not always result in new binomial names; similarly, genetic data alone may in rare cases be sufficient to erect new or resurrect old names. Curved dotted arrows reflect the possibility that newly identified species may still exhibit cosmopolitan distributions.
To accomplish our goals, we find it useful to re-introduce or propose several terms, which together constitute our typology (see Box 1 for a summary of definitions). We use the term eucosmopolitan to refer to a subset of those species previously referred to simply as “cosmopolitan,” but here restricted to species whose broad distribution in multiple oceans can clearly be attributed to natural processes acting over eco-evolutionary timescales. An important emphasis of our framework is thus that assignment of eucosmopolitan status requires positive evidence (below, and Scenario I in Figure 1), and should no longer be taken as the default assumption for broadly distributed species. This cautious approach signals a “once bitten, twice shy” attitude, an appreciation for the sheer repetitive force of innumerable studies in which presumed “natural” cosmopolitan distributions have been shown to reflect either recent anthropogenic spread or the global occurrence of multiple provincial species. In contrast, we define neocosmopolitan species as those that have demonstrably achieved cosmopolitan distributions through anthropogenic dispersal (Scenario II). We believe that this distinction is critical, as the two categories have markedly different implications for inferences about marine biogeographic theory and history and the ecological and evolutionary processes underlying community assembly, as we discuss further below.
Neocosmopolitan species are distinguished from eucosmopolitan species by a set of criteria that have been developed to differentiate non-native from native species (Carlton, 1979; Chapman and Carlton, 1994; Pociecha et al., 2016). Neocosmopolitan species are thus recognized in a given region by their historical absence (including, if applicable, absence from the archeological and paleontological record), association with a human-mediated vector, a known or suspected region of origin, and for many (but by no means all) species, presence in a disturbed, anthropogenic habitat (such as artificial substrates in ports and harbors). In contrast, eucosmopolitan species could have evidence of historical, archeological, or paleontological presence predating any possible human influence on their distribution, would be unlikely to be commonly associated with or transported by human-mediated vectors, would not be associated with any particular region of endemicity, and would not be frequently associated with anthropogenic habitats. In addition, eucosmopolitan species should possess dispersal capabilities (at some stage of their life cycle) that would suggest the capacity to sustain both transoceanic and interoceanic dispersal for months if not years on ocean currents (Hilario et al., 2015). In order to maintain a genetic structure suggestive of a single eucosmopolitan species, such dispersal would further have to be continuous in ecological time to prevent allopatric speciation in different ocean basins.
By definition, then, eucosmopolitan species would not include introduced species. This said, we recognize that under certain circumstances, such species could be engaged by a vector such as shipping (for example, a vessel ballasting in the open ocean taking in a pelagic species that would be released in another ocean). Such dispersal could in theory result in anomalous genetic exchange across unexpectedly large distances, or even in the appearance of the species beyond its known eucosmopolitan range.
Additional scenarios involve changes to the taxonomic status of broadly distributed marine species. Some of these changes may draw on novel morphological (or morphological and genetic) evidence, typically resulting in assignment of new (or resurrected) binomials for previously unresolved lineages (Scenario III). Cosmopolitan species may thus dissolve into complexes of endemic (or indigenous) or introduced regional species (Box 1); alternatively, one or more of the species in these complexes may still exhibit broad distributions across multiple oceans, requiring categorization as either eucosmopolitan or neocosmopolitan depending on biogeographic history (Figure 1, lower left dotted blue arrows). Species currently with invasions only within the same ocean where they are native (that is, introduced regional species), would become neocosmopolitan species if dispersed to another ocean.
Other changes in knowledge, most often provided by molecular genetic studies, frequently result in identification of evolutionary lineages sufficiently highly diverged to raise suspicions of the existence of multiple species (Scenario IV). In the absence of non-genetic corroboration of species-level distinctions, however, these lineages normally remain unnamed (Pante et al., 2015a), leaving us with a single accepted binomial describing a taxon with cosmopolitan distribution that we nevertheless suspect may represent a complex of multiple species—some or all of which may, in fact, be geographically restricted provincials. We define such taxa, now numerous in the literature, as pseudocosmopolitan to reflect the possibility that their current cosmopolitan status may be an illusory effect of overconservative taxonomy; that effect could be—and ultimately sometimes is—dispelled by future integrated taxonomic revision of the taxon.
Finally, we propose the term unresolved cosmopolitanism to describe what remains. We define this category as encompassing those species—perhaps the majority of species still described as “cosmopolitan”—which are said to exhibit a contemporary cosmopolitan distribution but have not yet been subjected to rigorous investigation into their taxonomic status and/or biogeographic history (Scenario V). In effect, taxa assigned unresolved cosmopolitan status are in a holding pattern, awaiting morphological, genetic, historical, and/or other analyses to resolve their status. We propose this category, then, as the new default. We feel such caution is warranted given what has been learned over the last several decades, and the suspicion with which marine biologists have grown to view the legacy of cosmopolitanism inherited largely from systematics of the 19th and 20th centuries.
These terms are not entirely new, but their usage in the past has been either obscure or inconsistent. Most notably, the two terms eucosmopolitan and neocosmopolitan were first proposed by Rapoport and Marino (1998) relative to terrestrial plant invasions. They used the terms much as we use them here, defining them simply as either “true or natural cosmopolitan species” or “human-transported cosmopolitans.” Gartner (1998), apparently independently, used eucosmopolitan to refer to what was thought to be a “circumglobal” mesopelagic (deep water) fish species; the term was repeated in the same sense by Rees et al. (2017). We have found no further use of the word neocosmopolitan prior to our current work, and the terms have clearly not attained broad usage in the literature. Pseudocosmopolitan was used by Fernald (1926) to refer to New Zealand flowering plants mis-identified as European species. Hoagstrom and Berry (2006) also used pseudocosmopolitan in a restricted sense to refer to less-than-widespread distributions of fish within Great Plains drainage basins. Closer to our usage is that of Nikulina et al. (2007) who defined pseudocosmopolitans as “complexes of regionally restricted cryptic species” (we include native, introduced, and cryptogenic species within pseudocosmopolitanism), and Kuklinksi and Taylor (2008) who referred to pseudocosmopolitans as species with “seemingly cosmopolitan distribution(s) (that are) probably spurious.” By placing these sporadically used terms into a comprehensive framework we hope to confer upon them sufficient weight that they might be adopted more broadly to ground future discussion and investigation.
In the remainder of this review we provide evidence from the literature supporting this framework, identify examples illustrative of each of the scenarios described briefly above, and describe how various lines of evidence are brought to bear to elucidate the appropriate status of particular species. We focus largely on neocosmopolitan and pseudocosmopolitan species, primarily because these categories align most closely with the two ways in which marine invasion biology has influenced thinking about cosmopolitanism over the past several decades. On the one hand, growing understanding of the pervasive influence of maritime traffic on species distributions has led to identification of a large and growing number of neocosmopolitan species; on the other, increasing application of molecular genetic and other tools to understand invasion histories has resulted in a similar proliferation of pseudocosmopolitan taxa.
These categories thus capture important lines of evidence linking invasion biology to a broader understanding of marine biogeography. We intentionally exclude from our study a substantial literature investigating cosmopolitan taxa that lack a known history of anthropogenic introductions, in order to focus specifically on the indispensable role of invasion biology in informing our framework. We hope through this exploration to illustrate the significant influence that invasion biology has had on marine biodiversity studies, and more broadly to provide a systematic approach for interpreting cosmopolitan distributions of marine species in the Anthropocene.
Neocosmopolitanism: Human-Mediated Spread and the Creation of Cosmopolitan Species
A vast literature accompanies the rapidly growing recognition since the 1980s of both the historic and modern-day scale of global dispersal of marine organisms by human activity. There are now many hundreds of papers that address the depth and breadth of the introduction of non-native marine and estuarine protists, invertebrates, fish, algae, seagrasses, and halophytes on most coastlines of the world. In contrast, Carlton (1979) was able to cite only one peer-reviewed paper (Lachner et al., 1970) that summarized selected examples of introduced marine species. This surge of modern literature reflects in part the vastly increased globalization of maritime trade since World War II that has led to a mushrooming of invasions, and in part to the realization that hundreds if not thousands of species invasions occurred prior to the 1900s that were simply never remarked on, mistaken for native species, or were members of poorly-studied taxa (Carlton, 2009).
The anthropogenic vectors that have historically transported, continue to move, or will persist in translocating marine species both within and between oceans have been reviewed in recent years (Carlton et al., 1995, 2017, 2018; Carlton, 2001; Minchin and Gollasch, 2002; Carlton and Ruiz, 2005; Minchin et al., 2005; Hewitt et al., 2007; Galil et al., 2015; Muirhead et al., 2015). These vectors have been or now include the extensive niche areas on or in ocean-going vessels that transport fouling, entangled, and ballasted organisms, sea level (such as the Suez) and lock (Panama) canals, mariculture (aquaculture), the aquarium and saltwater bait industries, and long-distance rafting on anthropogenic debris. Reviews of some marine bionvasions successfully introduced via these mechanisms or corridors are available, for example, for North America (Cohen and Carlton, 1995; Ruiz et al., 2000, 2011, 2015), Europe (Wolff, 2005; Gollasch, 2006; Galil et al., 2014), the Mediterranean (Galil, 2009; Rilov and Galil, 2009; Ulman et al., 2017), the Azores (Cardigos et al., 2006) and Madeira (Canning-Clode et al., 2013), South Africa (Mead et al., 2011; Alexander et al., 2016), Argentina (Orensanz et al., 2002), Brazil (Ferreira et al., 2009); the Pacific coast of Mexico (Low-Pfeng and Peters-Recagno, 2012), Chile (Villasenor-Parada et al., 2017), the Hawaiian Islands (Carlton and Eldredge, 2009, 2015), Japan (Otani, 2006), China (Xiong et al., 2017), Korea (Park et al., 2017), Australia (Wyatt et al., 2005, and references therein; Sliwa et al., 2009), and New Zealand (Hayden et al., 2009). The majority of these introduced species—whether phytoplankton, foraminifera, other protists, invertebrates, fish, or plants—are recognized as neocosmopolitan taxa based upon morphological and other criteria noted above.
We remark here briefly on the extent and antiquity of ship-mediated transport of species, and how this antiquity may have led to an under-assessment of the scale of neocosmopolitanism. When humans first sailed significant distances within, across or between oceans such that biogeographic barriers were bridged, is not known (Erlandson, 2017; Lawler, 2018). Whatever the antiquity and range of early wooden craft, there is no doubt that marine animals and plants became attached to, burrowed into, or were accidentally drawn inside these vessels (Carlton, 1999, 2011). Within the past 1,000–500 years these craft became sufficiently advanced to permit long-distance ocean voyages, such that northern European cultures (including the Norse) explored North America, Chinese empires reached East Africa (discussed further below), and Polynesians reached the Hawaiian Archipelago, leading to a global web of voyages that eventually connected virtually all shores of the world (Woods Hole Oceanographic Institution (WHOI), 1952; Natkiel and Preston, 1986; Casson, 1995; Woodman, 1997; McGrail, 2015). As vessel size and technology continued to evolve, expanding fleets of vessels sailing out of Europe commencing largely in the 1500s roamed the “Seven Seas,” which in modern times constitute the North and South Atlantic Ocean, the North and South Pacific, the Indian Ocean, and Arctic and Antarctic waters. Within the Atlantic Ocean alone, for example, between the 1,500s and 1,800s, a staggering 36,000 sailing voyages have been recorded that transported over 10 million Africans into slavery to North and South America (Eltis, 2007).
That ancient voyages may have long confounded our understanding of marine biogeography is perhaps no better illustrated than by the distributions of many hundreds–thousands?–of species that are believed to “naturally” occur over the vast distances from the Red Sea to the North Pacific Ocean (Carlton, 1987). A great many species of invertebrates and algae are described as ranging from the Red or Arabian Seas throughout the Indian Ocean, across Indonesia north to the China and Philippine Seas and southern Japan, and, often, continuing on through all of Oceania (Melanesia, Micronesia, Polynesia, and Australasia), if not to the Hawaiian Archipelago. To explain such distributions, taxonomists, biogeographers, and ecologists typically infer either “rafting” or larval dispersal over distances exceeding 20,000 km, implying that such natural dispersal mechanisms effectively insure a remarkable level of gene flow sufficient to prevent speciation.
In contrast, if we look through the lens of maritime history coupled with its imperceptible boundary with the history of marine biodiversity, ancient ports are positioned throughout this vast Indo-West Pacific theater. As an example, the island state of Singapore, at the tip of the Malay Peninsula in Southeast Asia, has been a strategic entrepot since the 1,300s. While not without interruption in terms of scale and intensity, the Port of Singapore presumably has been a hub for the dispersal of marine animals and plants across a vast sweep of the Indian and Pacific Oceans for at least 700 years (Wang, 1339; Turnbull, 2009). As Turnbull (2009) has remarked, “…since time immemorial the Straits of Singapore lay at the heart of a rich trading region in which great Malay empires had flourished.”
Over the centuries, a maritime Silk Road (commencing centuries B.C.E. and continuing into the 1,400s), also known as the Porcelain Road, settled into place, connecting European ports with those of the Indo-West Pacific and Asia (Figure 2A; Rapoport and Marino, 1998; Lawler, 2014; Church, 2018). The famous Chinese mariner, Admiral Zheng He, departed Suzhou in the East China Sea in July 1405 with no fewer than 317 vessels to sail to Southeast Asia, India, and Africa (Levathes, 1996; Dreyer, 2006; Liu et al., 2014), the first of seven naval expeditions that lasted until 1,433. It gives pause to begin to imagine the diversity of marine fouling and boring organisms carried by these homogenizing fleets alone for more than a quarter century.
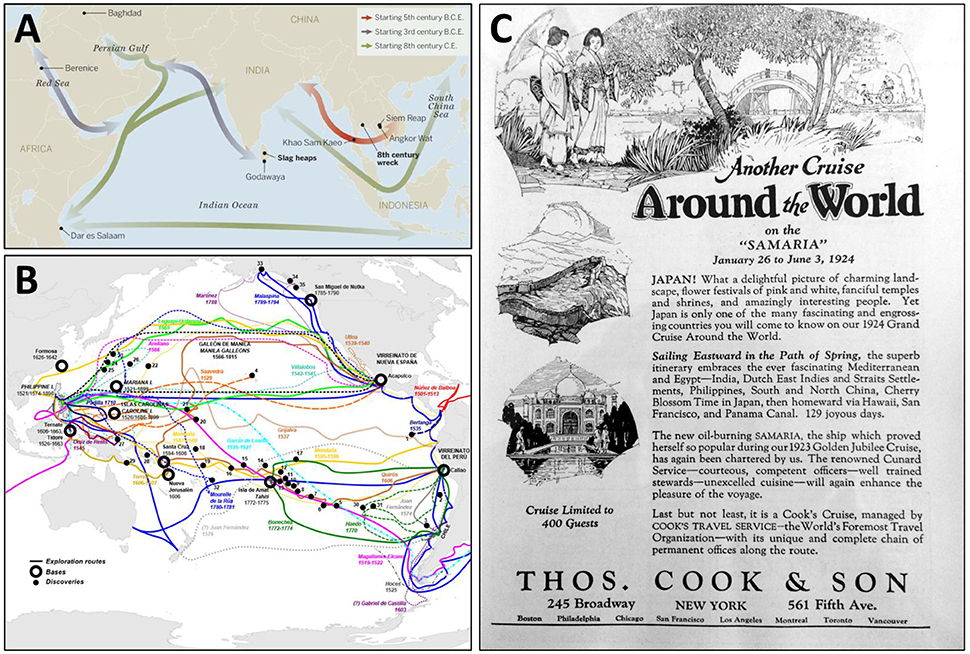
Figure 2. (A) Shipping lanes of the “Maritime Silk Road,” starting in the fifth century B.C.E., connected the Red Sea to the South China Sea and beyond. From Lawler (2014); reprinted with permission from AAAS. (B) Spanish expeditionary routes and bases of exploration of the North and South Pacific Oceans over 240 years, touching virtually the entire Pacific rim from 1535 (to the Galapagos Islands) to 1775 (to the Alexander Archipelago, in what is now southeast Alaska). From https://commons.wikimedia.org/wiki/File:SpanishPacific.svg, open use without restriction through Wikimedia Commons (https://creativecommons.org/licenses/by-sa/4.0). (C) An advertisement for “Another cruise around the world” aboard the Samaria from January 26 to June 3, 1924 in ”129 joyous days“: New York to the Mediterranean and Egypt through the Suez Canal to India, the Dutch East Indies and Straits Settlements (which included Singapore), Philippines, China, Japan, Hawaii, San Francisco, and then back to New York through the Panama Canal. From National Geographic Magazine 44(3), September 1923.
That vessels were plying many of these waters long before the 1,300s is without doubt. Since the 1,300s, hundreds of thousands of voyages crisscrossed the Indian and Western Pacific Oceans alone, marked by expeditions of exploration and colonization that were replaced by trade routes that endured for centuries (Figure 2B). Punctuating the development of commerce and supporting port systems were intense pulses of shipping related to perceived riches (such as the nineteenth century gold rushes of California and Australia) or to wars, all of which fundamentally both changed and expanded the hubs and spokes of the sea routes of empires. By the early Twentieth century, a new layer of global tourism on ocean-going cruise ships had developed as well (Figure 2C). Remarkable is how little reference to these centuries of global shipping is often made in the marine systematic, ecological, and biogeographic literature.
The continuous introductions and reintroductions of species along these routes may have created what today would be nearly undecipherable homogenized global gene pools, making the origin of many species difficult to determine. How many such species–ranging from foraminiferans, sponges, hydroids, flatworms, polychaetes, amphipods, and sea spiders to bryozoans and sea squirts–have become Flying Dutchmen, sailing the seas without a known home, we have as yet no idea. David et al. (2016) and David and Loveday (2017) refer to the widespread dispersal of individuals by anthropogenic vectors as “cryptic dispersal.” Observing that human-mediated movement may be an important contributor to gene flow, they note that such movements may conceal or erode signals of past phylogeographic structure. “If cryptic dispersal has been occurring across long timescales” (as we suggest here for Flying Dutchmen), “even genetic patterns inferred from mtDNA may be obscured via reshuffling of ancient haplotypes due to past translocation events” (David and Loveday, 2017).
While a reliable estimate of the total number of marine species invasions worldwide is not available (the summary statistics in Ahyong et al., 2018 omit many species and include non-established species), the number may be considerably more than 2,000 taxa. Even this estimate, however, overlooks what may be at least the same number of species that remain unrecognized as invasions, a significant fraction of which may be microscopic species [a reflection of what is known as the “smalls rule of invasion ecology,” defined as the inverse correlation of body size with the probability of being recognized as a non-native species (Carlton, 2009)]. For example, Carlton (2009) noted while at least 500 distinct phytoplankton taxa are recognized in San Francisco Bay, California, and despite the 100 or more years of vectors that have released non-native diatoms, dinoflagellates, or other phytoprotists into the Bay, no phytoplankton are recognized there as introduced. Doubtless, however, the largest fraction of unrecognized invasions are what are now either classified as native or cryptogenic species, the classical default in biogeography, evolutionary biology and ecology being to regard the latter as the former.
In Table 1 we present a few (33 taxa in 9 phyla) examples of neocosmopolitan species for which robust morphological, or morphological and genetic, data suggest that a single species is involved. It remains unclear how many of the hundreds of presumed neocosmopolitan species discussed above would withstand such inquiry to retain their current taxonomic assignments. We maintain that this stringent condition for formal categorization as neocosmopolitan—that the taxon be subjected to rigorous examination of its taxonomic status throughout its cosmopolitan range—is indispensable given rapidly mounting evidence for morphologically cryptic provincial species disguised as cosmopolitans (see below). Indeed, even given such exacting investigation it would not be surprising if cryptic species remain undetected amongst those taxa where only morphological evidence is available to support probable conspecificity. A number of species listed as pseudocosmopolitan in Table 2 have been subjected to morphological analysis by researchers forearmed with information about cryptic lineages disclosed by genetic data; even in such cases, distinctive morphological traits separating those lineages may remain elusive (Lessios et al., 2001; Xavier et al., 2010; Perez-Portela et al., 2013; Knapp et al., 2015). Low-hanging fruit for future such investigations include those morphospecies said to occur (and reproduce) from cold temperate (including freezing conditions) to tropical latitudes, such as the sea anemone Diadumene lineata and the barnacles Amphibalanus improvisus (whose tropical populations were not studied by Wrange et al., 2016) and Amphibalanus eburneus.
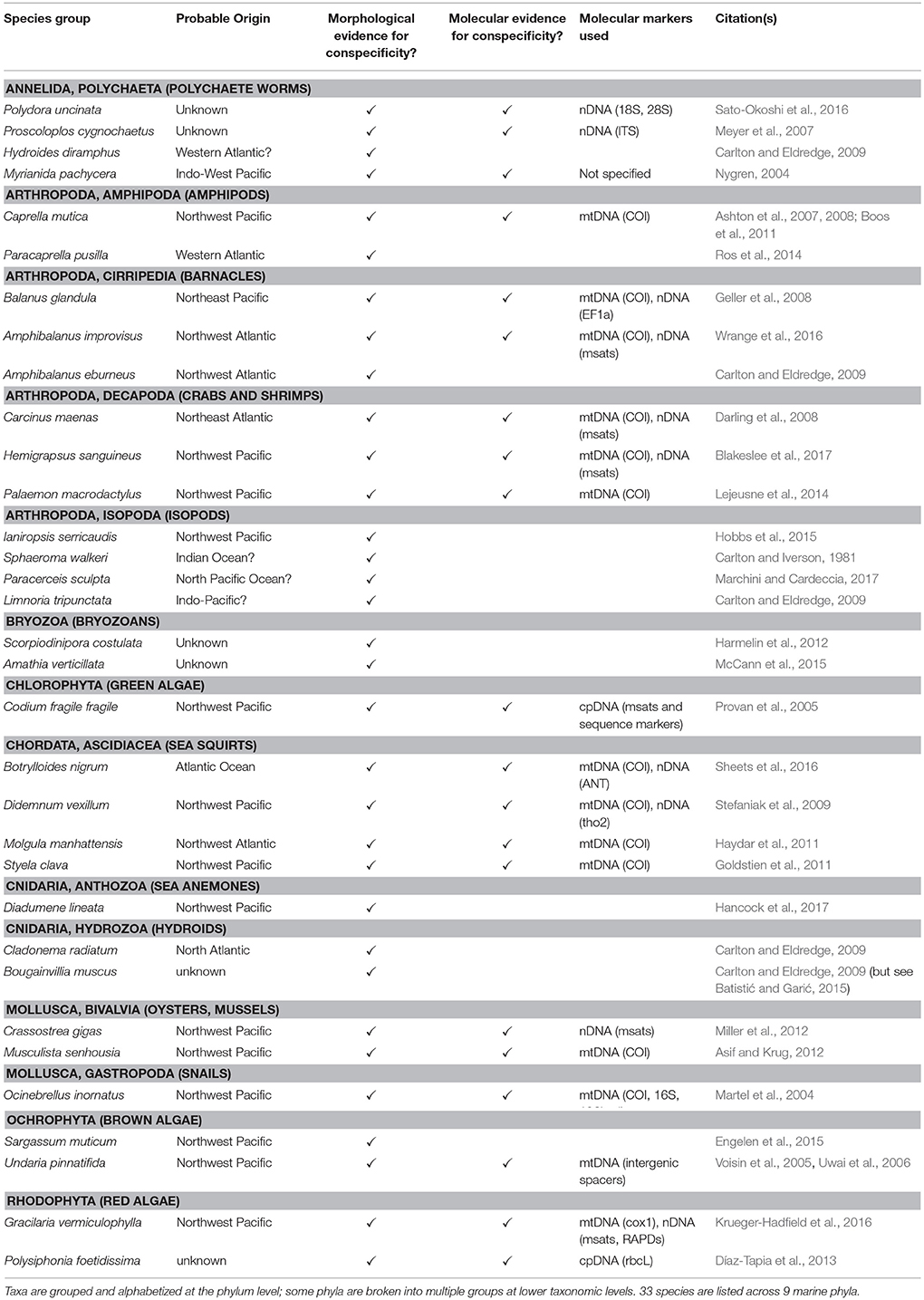
Table 1. Examples of neocosmopolitan species fitting Scenario II from Figure 1.
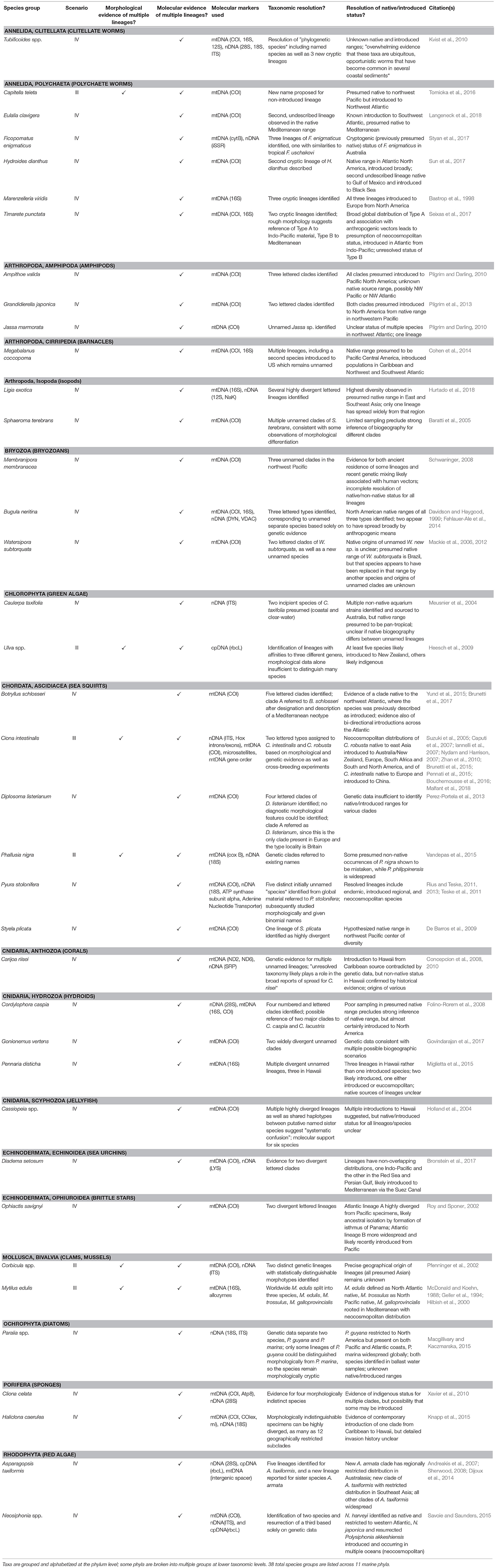
Table 2. Examples of provincial native and regional introduced (Scenario III) and pseudocosmopolitan (Scenario IV) species.
Neocosmopolitan and Eucosmopolitan: A Distinction With a Difference
We conclude these brief thoughts by underscoring that to fail to distinguish natural (eucosmopolitan) from anthropogenically-created (neocosmopolitan) distributions undermines many of the core assumptions of evolutionary community ecology and biogeography. Classical ecosystem and community marine studies–such as those of rocky intertidal shores, soft-bottom benthos, and salt marshes–assume that the species assemblages under investigation, and the processes regulating such communities, including competition, predation, and disturbance, are the result of long-term evolutionary interactions. Thus it is that, unbeknownst to, or overlooked by the researchers at the time of their work, a number of definitive studies on the ecological interactions of presumed native species were in fact based upon one or more members of the community that were introduced. Steneck and Carlton (2001) have already remarked that fundamental studies on the regulatory role of the periwinkle snail Littorina littorea on New England intertidal shores do not mention that it arrived in North America in the 1,800s; as a result, what would be today regarded as iconic studies on the impact of a marine invader often go without mention in reviews of the effects of non-indigenous species. Of note is that Steneck and Carlton's chapter on human alterations of marine communities is found in an end-of-book section on “Conservation Issues,” rather than under a leading section of “Processes influencing pattern in marine communities.”
Many other studies seeking to elucidate natural patterns were also founded inadvertently on non-native species. Sutherland's (1974) classic work on “multiple stable points in natural communities” was based in part on the seasonal phenology of the ascidian Styela plicata, now known to be native to the Western Pacific Ocean (De Barros et al., 2009; Pineda et al., 2011; Carlton, unpublished, relative to its Asian origin). Harger's (1972a,b) work on the ecological interactions of two intertidal native mussels, Mytilus edulis, and Mytilus californianus, in southern California, we now know to be studies on the introduced mussel Mytilus galloprovincialis and the native species M. californianus. Eyebrow-raising is the resolution that the amphipod Corophium volutator, a species long known to be central to trophic dynamics and benthic processes in the Bay of Fundy, is in fact native to Europe (Einfeldt and Addison, 2015), having been introduced by shipping in the nineteenth century. Hair-raising is the discovery that the saltmarsh cordgrass Spartina alterniflora, a dominant member of the coastal marine flora of the Atlantic coast of North America, is also a nineteenth century invasion, leading to the conclusion that what were bare mudflats are now endless expanses of rooted vegetation that have led to “vast unrecorded and thus overlooked shifts in bird, fish and invertebrate biodiversity, and immense shifts in algal vs. detritus production, with the concomitant trophic cascades that these changes imply” (Bortolus et al., 2015).
These are only a few such examples. To our knowledge, few of these realizations that presumptive indigenous species are not native (thus leading to significant re-interpretations of the work conducted on the communities in question) are reflected in most broader (let alone textbook) treatments on the structure and function of marine ecosystems. This illustrates the wider point, that failure to appropriately categorize taxa as either eu- or neocosmopolitan may guide researchers to inferences regarding community assembly, dispersal, and other critical ecological processes that rest on shifting sands. To continue to refer to all marine taxa with cosmopolitan distributions as merely “cosmopolitan” thus potentially obscures a conceptual distinction of critical importance.
Pseudocosmopolitanism: Genetic Evidence for Cryptic Lineages
The splintering of marine species into complexes of cryptic lineages is a common recent phenomenon, particularly among certain taxonomic groups (Nygren, 2014; Leray and Knowlton, 2016). Even when considering only the subset of species known to be anthropogenically introduced beyond their native ranges, examples abound of studies revealing previously unrecognized diversity. Table 2 provides a summary of over three dozen such studies; while not an exhaustive account, it represents a fair sampling of the breadth of evidence for cryptic diversity described in the invasion biology literature. Examples span 11 marine phyla, including both plants and animals, and are predictably dominated by groups well-known to harbor large numbers of introduced species.
Of the 38 species groups captured in Table 2, only 6 of them have been subjected to sufficiently rigorous integrated taxonomic analysis to allow resolution of cryptic status into multiple named species; these constitute examples of our Scenario III from Figure 1. The remainder, all investigated with molecular genetic approaches only (or including only superficial or limited non-genetic evidence), represent Scenario IV. As our collection has been neither exhaustive nor entirely systematic, it is difficult to say with confidence whether this ratio is representative of the state of the science or merely our own biased knowledge of the literature; however, we believe it likely that the literature is dominated largely by studies that introduce, rather than resolve, taxonomic uncertainty.
This pattern may be unsurprising, given the relative ease with which genetic data can be generated compared to the kind of information required to conduct integrated taxonomic revision of a species or species group. In the vast majority of cases, inference of multiple cryptic lineages from genetic data derives from one or very few standard genomic loci, most commonly those loci frequently adopted for DNA barcoding (Hajibabaei et al., 2007): mitochondrial loci such as cytochrome C oxidase subunit I (COI, 71% of cases in Table 2) and 16S (24%), the chloroplast marker RuBisCO (rbcL, 75% of plant taxa), or nuclear loci such as ribosomal 18S (13%), 28S (11%), or the internal transcribed spacer (ITS, 16%). Remarkably, over half of the taxa listed were investigated using only a single genetic marker, most often the “standard” animal barcode locus COI. This means that inference of evolutionary lineages perhaps deserving independent species status typically depends on one or very few lines of evidence, and almost universally on only two criteria: reciprocal monophyly (lineages form distinct branches with strong statistical support in phylogenetic trees) and average inter-lineage genetic distance greater than would be expected of intra-specific relationships.
These limitations may help explain the caution with which most molecular studies approach the task of declaring multiple cryptic species. Since the emergence of DNA barcoding as a powerful tool in molecular ecology and biodiversity studies, the connection between single-locus genetic divergence and species discovery has been fraught with controversy (Desalle et al., 2005). Most practitioners view such evidence as merely one small component of the much broader effort required to recognize novel species (Pante et al., 2015b). Nevertheless, information even from limited genetic surveys can sometimes be compelling. For instance, it is common practice to consider genetic divergence of approximately 3% at the COI locus to represent expected limits on intra-specific variation (Meyer and Paulay, 2005), despite some well-known exceptions (Huang et al., 2008). However, studies in Table 2 regularly report variation at the COI locus dramatically exceeding these limits: roughly 5% among specimens of the amphipod Ampithoe valida (Pilgrim and Darling, 2010), 8.4% in the sponge Cliona celata (Xavier et al., 2010), 12% for the hydroid Cordylophora caspia (Folino-Rorem et al., 2008), 18% in the ascidian Botryllus schlosseri (Yund et al., 2015), 20% in the ascidian Diplosoma listerianum (Perez-Portela et al., 2013), and 21% in the polychaete Timarete punctata (Seixas et al., 2017). For specimens ostensibly belonging to the same species these divergences range from the merely remarkable to the truly astounding. It is little wonder that they have been sufficient to raise the prospect of multiple species, notwithstanding evidence being limited in some cases to a single locus. Interestingly, in several of these cases additional, non-genetic lines of evidence have been shown to further support the hypothesis of multiple species. These cases inspire the suggestion that when multiple lineages are detected, reported lineage descriptions should include as many morphological, biological (reproductive, physiological, or other traits), or ecological characters as are known to the authors for each newly resolved lineage.
Still, in the absence of thorough taxonomic revision the preference has clearly been to maintain the observed cryptic entities as numbered or lettered lineages or clades, without attachment of binomial names. This is true even when data from multiple loci (derived, in many cases, from both mitochondrial and nuclear genomes) provide consistent evidence of evolutionarily divergent lineages.
What's in a Name? The Problem of Unnamed Lineages
This reluctance to attach names to cryptic lineages identified through molecular means alone is nearly universal. In some cases, practitioners of molecular genetic analysis—particularly those with appropriate systematic expertise within the groups being studied—may be bold enough to suggest outright the existence of unnamed new species (Mackie et al., 2006) or to refer genetic clades to previously existing species names (Teske et al., 2011). But in the vast majority of cases, molecular data result only in unresolved hypotheses of multiple species, particularly when associated morphological analysis was either not undertaken or failed to turn up any diagnostic features enabling more complete taxonomic revision. This creates a significant challenge for interpreting the cosmopolitan status of these species, now revealed to be potentially species complexes. The single species name persists, attached still to a distribution that is considered cosmopolitan. There is therefore no formal recognition of anything but a single cosmopolitan species, even though molecular data may suggest that the species actually represents a group of originally geographically restricted lineages (one or more of which may then have been transported by human activity to other locations). This ambiguity—the lingering question of whether future analysis might provide sufficient data, morphological or otherwise, to resolve a cosmopolitan species into multiple species—leads us to characterize such species as pseudocosmopolitan, a designation reflecting the likelihood that the cosmopolitan distributions of these species are, at least in part, an artifact of overconservative taxonomy.
A number of the species cataloged in Table 2 provide especially compelling examples of this phenomenon. For example, analyses conducted by Bronstein et al. (2017) confirmed strong evidence of two largely geographically non-overlapping lineages of the widely distributed sea urchin Diadema setosum, “clade a” in the Indo-West Pacific and “clade b” in the Arabian Peninsula (with the latter introduced to the Mediterranean). These two clades exhibit sequence dissimilarity at the COI locus of approximately 8%, suggesting an estimated time of divergence 3-5 million years ago despite the lack of any as yet detected diagnostic morphological features (Lessios et al., 2001). Nevertheless, the species formally retains a distribution encompassing this entire range across multiple oceans. Figure 3 presents evidence from a particularly illustrative study revealing at least four genetic lineages of the globally distributed ascidian Diplosoma listerianum (Perez-Portela et al., 2013). One of these lineages, designated Clade A, exhibits a global distribution with a historical center in the British Isles (Figure 3A), and may represent a neocosmopolitan species. The remaining clades, however, have considerably more limited geographic ranges: Clades B and D apparently remain restricted to their native ranges in east Asia and the western Atlantic, respectively, while Clade B exhibits a disjunct distribution in South Africa and Panama. The hypothesis that these clades represent multiple species is supported by extraordinary genetic divergence, comparable with inter-specific distances observed in other colonial ascidians. Similar patterns of cosmopolitan “species” comprising provincial genetic lineages have been observed in isopods (Hurtado et al., 2018), polychaetes (Sun et al., 2017), and multiple hydrozoans (Miglietta et al., 2015; Govindarajan et al., 2017). In the absence of formal taxonomic revision, all of these species appear based on genetic evidence to harbor multiple lineages—possibly themselves all distinct species—that are most decidedly not cosmopolitan.
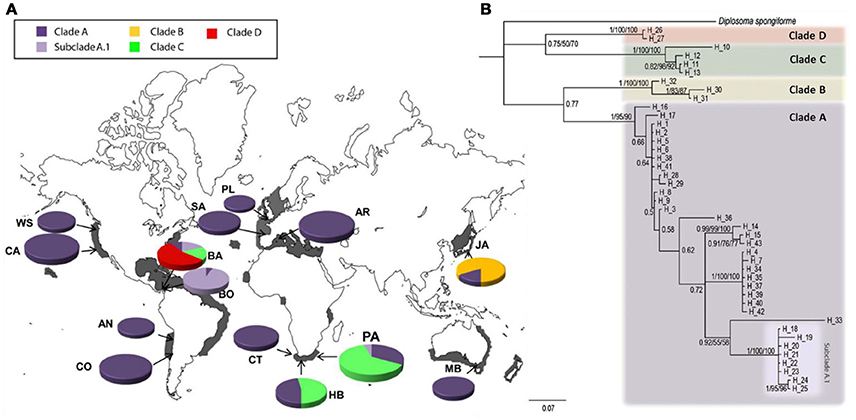
Figure 3. Geographic distributions of the multiple genetic lineages of the ascidian Diplosoma listerianum, from (Perez-Portela et al., 2013). (A) Global distributions of clades identified based on analysis of COI sequences. Clade A is widely dispersed, likely exhibiting a neocosmopolitan distribution, Clade B is cryptogenic, and Clades C and D display limited distributions consistent with provincial lineages. (B) Phylogenetic analysis revealing dramatic divergence between clades; interclade divergence was as great as 20% sequence dissimilarity at the COI locus, consistent with independent species status. Figures reprinted under the Creative Commons Attribution-Share Alike 4.0 International license.
These examples reveal the importance of genetic data not only for identifying cryptic diversity, but also for attempting to disentangle complex invasion histories that are often very poorly documented in the historical record. Figure 1 registers these twin challenges associated with cryptic diversity—not only resolution of taxonomic identity, but also resolution of biogeographic history. The latter task can be extremely difficult depending on sample availability, marker resolution, and evolutionary history (Geller et al., 2010; Cristescu, 2015), resulting in many cases where biogeographic history may remain unsettled. For instance, the amphi-Atlantic distribution of Diplosoma listerianum Clade B could not be resolved based on existing genetic data, leaving unclear whether that lineage may be native to Panama or South Africa, or to neither of these locations (Perez-Portela et al., 2013); we note that a fourth choice, that Clade B may be native to both locations, is highly improbable, thus rendering this clade cryptogenic in its known locations.
Indeed, a large number of lineages identified across various efforts cataloged in Table 2 remain cryptogenic despite attempts to discern biogeographic patterns. Nevertheless, many studies have successfully resolved invasion histories for cryptic lineages emerging from genetic analysis. Sometimes a newly discovered lineage exhibits only a limited geographic distribution, in which case it is typically assumed to be a regional endemic (e.g., D. listerianum Clades B and D in Figure 3). However, often one or more novel lineages retains a cosmopolitan distribution, and the question then turns to the nature of that distribution, and whether it has been influenced by anthropogenic dispersal. As we have already noted, D. listerianum Clade A appears to be a globally distributed independent evolutionary lineage; similarly, Clade A of the polychaete Hydroides dianthus has been found in the northwest and southwest Atlantic, Mediterranean, and western Pacific (Sun et al., 2017); and Clade D of the isopod Ligia exotica may be native to East Asia but has been identified from South Africa, India, the western Atlantic, and Hawaii (Hurtado et al., 2018). In all cases, molecular genetic as well as historical evidence support the hypothesis that these clades represent as-yet unnamed neocosmopolitan species.
This clarity is obviously useful, but without taxonomic resolution these taxa should continue to be described formally as pseudocosmopolitan, given that demonstrably provincial lineages remain subsumed under the single accepted binomial. This categorization of these taxa is important for two reasons. First, attaching this category promotes much-needed transparency and broader recognition of the uncertainty concerning taxonomic status that now burdens numerous marine species. Adoption of a unified terminology to describe this condition may help us to better understand the extent to which it poses a challenge for marine biodiversity studies. Second, the pseudocosmopolitan label can serve as acknowledgement that even uncertainty may represent an improvement in our comprehension of marine biogeographic history. The conversion of “unknown unknowns” to “known unknowns” is progress, although it sometimes may not appear that way. In an ideal world, pseudocosmopolitan species do not remain in that category indefinitely.
Successful Resolution of Taxonomic Uncertainty: A Success Story and a Cautionary Tale
The fact that the uncertainty associated with pseudocosmopolitanism can be ultimately resolved by integrated taxonomic assessment is revealed by the existence of multiple species groups populating Scenario III. Of the six examples of such integrated assessments listed in Table 2, clearly the most exhaustive involves resolution of the globally introduced ascidian Ciona intestinalis into two sibling species, C. intestinalis and C. robusta (Figure 4). In addition to being recognized as a widely distributed marine pest with an extensive record of historical introductions, C. intestinalis has also long been embraced as a model organism due to its global availability, amenability to experimental manipulation, chordate body plan, and substantial molecular genetic resources, including a whole genome sequence completed in 2002 (Caputi et al., 2007). Early genomic analysis revealed unexpected divergence between individuals from Northern European and Pacific sources, suggestive of cryptic species (Suzuki et al., 2005). Ensuing genetic investigations based on multiple nuclear and mitochondrial markers as well as analysis of mitochondrial gene order further supported recognition of two evolutionarily distinct lineages commonly labeled species A and species B (Caputi et al., 2007; Iannelli et al., 2007; Nydam and Harrison, 2007). Despite this wide-ranging and compelling molecular genetic evidence for multiple species, formal taxonomic revision of C. intestinalis failed to materialize until 2015. In that year, two publications thoroughly described morphological differences in both adult and larval specimens of sp. A and sp. B, and recognized diagnostic features previously associated with the Japanese species C. robusta, which had been synonymized with C. intestinalis (Brunetti et al., 2015; Pennati et al., 2015). The subsequent association of C. robusta with sp. A and C. intestinalis with sp. B completed the taxonomic resolution, and the acknowledged species-level distinction has since been further supported by experimental cross-breeding, although interspecific hybridization has been frequently observed (Malfant et al., 2018).
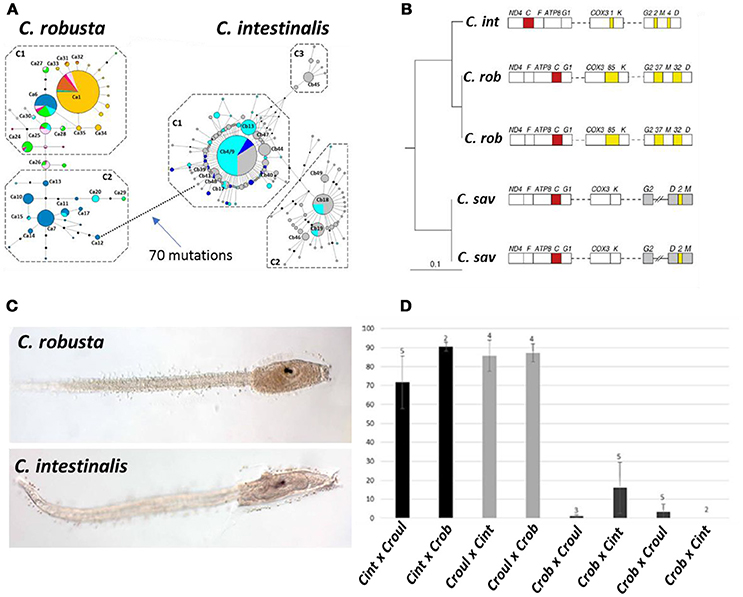
Figure 4. Genetic, morphological, and reciprocal crossing evidence for species-level divergence between two lineages of the ascidian Ciona intestinalis (Type A and Type B). Figures are relabeled with the new names for these lineages, Type A = C. robusta and Type B = C. intestinalis. (A) Median-joining haplotype network based on COX3-ND1 sequences, showing 70 mutational steps between the two lineages; colors indicate geographic affinities, which are not shown (Bouchemousse et al., 2016). (B) Differences in gene order on the mitochondrial genomes of C. intestinalis, C. robusta, and C. savigni (Iannelli et al., 2007). (C) Larval morphology of the two species; a discriminant function obtained from larval measurements correctly classified >93% of larvae (Pennati et al., 2015). (D) Fertilization rate of heterospecific crosses between C. intestinalis, C. roulei, and C. robusta; homospecific crosses resulted in >80% fertilization rates for all species, but heterospecific crosses with C. robusta as the maternal lineage (listed first) showed extremely low success rates (Malfant et al., 2018). (A,B,D) Reprinted under the Creative Commons license (Attribution 4.0 International (CC BY 4.0)); (C) reprinted with permission.
These extensive efforts undertaken to resolve taxonomic uncertainty have also been accompanied by comprehensive examination of introduction history for the two sibling species. Historical records suggest that C. robusta, the more broadly distributed species, has been introduced widely from its native range in east Asia to Australia and New Zealand, Europe (including the Mediterranean), South Africa, both coasts of South America, and western North America; C. intestinalis has been presumed native to the northeast Atlantic and either cryptogenic or introduced to the northwest Atlantic, and has also been introduced to China (Bouchemousse et al., 2016). The broad outlines of this historical picture have been roughly confirmed by genetic analysis. For C. robusta, observed patterns of genetic variation appear consistent with introductions to the northeast Pacific (including some evidence for multiple independent introductions), the southeast Pacific, and the northeast Atlantic (with genetic signatures of a more recent introduction to that region); populations from Australia and New Zealand were not examined in that study (Bouchemousse et al., 2016). The same study showed that for C. intestinalis, genetic evidence (though not unequivocal) was consistent with a natural amphi-Atlantic distribution of the species, with introduced populations in the Bohai and Yellow Seas. The current state of knowledge thus supports characterization of both C. robusta and C. intestinalis as neocosmopolitan species, i.e., taxa now occurring in multiple oceans. These results were consistent with an earlier 2010 study by Zhan et al. conducted prior to resurrection of C. robusta for sp. A. Interestingly, that earlier report suggested the existence of not only two, but rather four possible cryptic species, including a sp. D (apparent sister species to C. robusta and geographically restricted to the Black Sea) and sp. C (a rare and highly divergent lineage restricted to the Mediterranean). Thus, while the unambiguous association of sp. A with C. robusta and sp. B with C. intestinalis allows us to remove those species from the pseudocosmopolitan category, independent lineages C and D remain undescribed and attest to a measure of lingering uncertainty in this species group as a whole.
The case of Ciona is unusual in its comprehensiveness. But the considerable efforts devoted to disentangling the taxonomic and biogeographic mysteries of C. intestinalis offer a persuasive success story describing the resolution of pseudocosmopolitan status. They also sound an important cautionary note. It is significant that sp. A and sp. B did not receive proper names for a full decade after initial indications of likely cryptic species status. This was despite the fact that those 10 years witnessed proliferation of compelling genetic evidence for species-level divergence, driven in part by the availability of powerful molecular resources. This plainly highlights the centrality of morphological evidence for resolving taxonomic uncertainty. Indeed, identification of diagnostic morphological traits traditionally remains the one principle requirement for erecting new species names, often even in the face of overwhelming non-morphological evidence for the existence of multiple species. This fact is convincingly revealed by those multiple species for which morphological examination could not identify such diagnostic features even when investigators were aware of divergent genetic lineages and thus knew where to look (Lessios et al., 2001; Xavier et al., 2010; Perez-Portela et al., 2013; Knapp et al., 2015); in such cases, the original single binomial has been universally retained. Not all taxa listed in Table 2 adhere to this narrative, as initial questions about cryptic diversity are not always broached by genetic studies. In many examples of Scenario III, for instance, genetic data are brought to bear in attempts to clarify relationships between previously observed morphotypes (Pfenninger et al., 2002; Vandepas et al., 2015; Tomioka et al., 2016). Nevertheless, the vast majority of our examples of pseudocosmopolitanism represent cases where genetic inference of cryptic lineages precedes availability of any suggestive morphological data.
With the growing accessibility of genetic data for non-model organisms and increasingly sophisticated methods for the analysis of such data, it is reasonable to suppose that even more studies in the future will uncover persuasive multilocus evidence for species-level distinctions. Given that the accessibility of expert morphological taxonomy may be on the reverse trajectory (Pearson et al., 2011; Carlton and Fowler, 2018), we anticipate a widening gulf between those taxa for which genetic evidence urges revision and those for which sufficient morphological data can be obtained to effect it. Indeed, this gulf is often reflected amongst ecologists, field biologists, and naturalists who shy away from working with taxa which have been discovered to be species complexes but are bereft of scientific names representing the distinct clades. We thus believe it important to raise the possibility of future efforts relying solely on genetic data to describe and name new species. Although this has a ring of heresy to it, the International Commission on Zoological Nomenclature has made it clear that “new species can be described on the basis of DNA,” with the caveat that the type specimen from which DNA is extracted should be retained and appropriately vouchered (International Commission on Zoological Nomenclature ICOZ, 2018). Recent studies have established precedent for erecting new species names based largely or entirely on genetic data, not only for microbial taxa known to resist morphological characterization (John et al., 2014) but also for large metazoan taxa (Leaché and Fujita, 2010; Jörger and Schrödl, 2013). While integrated taxonomic revision—incorporating genetics, morphology, ecology, and other lines of evidence (Sheth and Thaker, 2017)— ultimately remains the ideal for resolving the status of cryptic lineages, we feel that the sheer breadth of the challenge faced and the practical limitations on available data and expertise recommend consideration of all available solutions.
Implications for Increased Resolution of Introduced and Cryptogenic Species
As indicated in Figure 1, our changing knowledge of the taxonomic and biogeographic status of cosmopolitan taxa also has important implications for our general understanding of introductions in marine systems. All possible outcomes of Scenario IV likely leave the number of known introduced species formally underestimated. Even when cryptic lineages can be convincingly identified as introduced in a region, the fact that they remain subsumed under a single original binomial masks the possibility that multiple introduced species exist where only one has been reported. This is true also of the case where taxonomy has been resolved, but biogeographic history—whether the species is native or introduced in a particular part of its range—remains uncertain. The abundance of such cryptogenic species has long been recognized as an indicator of our profound underestimation of human impacts on the composition of marine communities (Carlton, 1996), and more recent studies attest to the dramatic influence of cryptogenesis on our general understanding of coastal marine biogeography (Haydar, 2012).
The changing states of knowledge diagrammed in Figure 1 thus have implications not only for marine biodiversity studies, but also for management and policy related to marine bioinvasions. As biodiversity assessments including metrics reflecting the impacts of non-native species become more widely integrated into attempts to stay and reverse negative anthropogenic impacts on marine ecosystems (Darling et al., 2017), clear recognition of the uncertainties outlined in Figure 1—as well as approaches for their resolution—will no doubt become increasingly important.
Are There any Shallow-Water Benthic Eucosmopolitan Species?
Marine taxa in at least three habitats are still commonly alleged to often include eucosmopolitanism species. Many deep sea species are held to be identical cosmotaxa in multiple ocean basins (Costello et al., 2017), an evolutionary outcome presumably driven by gene flow via global deep sea currents that serves to inhibit allopatric speciation. Similarly, species occurring in the oceanic neustonic habitat, including planktonic foraminifers (Darling et al., 2000) and pelagic invertebrates such as the gooseneck barnacle Lepas anatifera, the nudibranch Fiona pinnata, and the amphipod Caprella andreae, have long been held to be naturally cosmopolitan species. This said, genetic studies are now revealing that these neustonic taxa are species complexes (Cabezas et al., 2013; Schiffer and Herbig, 2016; Trickey et al., 2016), although other species of Lepas, including L. anserifera and L. pectinata “might represent true global species” (Schiffer and Herbig, 2016). For the latter, global homogenization over many centuries via vessel hull fouling may have contributed to what may in fact be neocosmopolitanism in these oceanic taxa (Carlton et al., 1995; Carlton, 2002). Finally, many species of interstitial sandy beach meiofauna were long considered cosmopolitan (Todaro et al., 1996; Curini-Galletti et al., 2012). Schmidt and Westheide (2000) concluded that the “cosmopolitan nature” of the meiofaunal polychaete Hesionides arenaria “has been confirmed at the DNA level,” being a single clade, while demonstrating that another presumably cosmopolitan worm, Stygocapitella subterranea, was composed of three distinct clades. However, the conclusion that H. arenaria is “cosmopolitan” was based upon the study of European and Washington USA populations, reflecting a well-known pattern of the apparently largely natural circumboreal distributions of many marine species in the North Pacific and North Atlantic. Gerlach (1977) has noted that the long history of moving sand as ships' ballast around the world may have contributed to the perception of meiofauna cosmopolitanism; such taxa would now likely represent a broad mixture of introductions (neocosmopolitans) as well as pseudocosmopolitan and unresolved cosmopolitan species. These habitats aside, the question remains whether any shallow-water, benthic marine animal and plant, separated by continents and ocean basins, and in the absence of any data on their observed or potential for constant, consistent interoceanic rafting on natural materials, is eucosmopolitan. In this regard, for example, a very large number of what are said to be the same species of intertidal and shallow sublittoral algae (seaweeds) are reported from coastlines world-wide (http://www.algaebase.org/). None of these, to our knowledge, have been verified genetically as being eucosmopolitan.
This much, at least, is clear: it is far easier to find examples in the literature of neocosmopolitan and pseudocosmopolitan species than it is to find examples of presumed eucosmopolitan species that have withstood rigorous examination of their taxonomic and biogeographic status. There are two possible explanations for this observation. First, it may be that there is a bias among researchers (and possibly also editors) against publishing confirmation of eucosmopolitan status for species long assumed to be “natural” cosmopolitans. Under this hypothesis there may exist a large number of species for which considerable evidence exists supporting conspecificity across a cosmopolitan distribution, evidence that has not yet been made available in the peer-reviewed literature. If this is, indeed, the case, it is a problematic state of affairs in need of change. However, we find a second explanation much more likely: that most of the time, when workers take a closer look at a presumed eucosmopolitan species, it turns out either that it is a complex of evolutionary lineages masquerading as a single species, or that its broad distribution can be convincingly explained by anthropogenic dispersal.
We propose therefore, that there are likely no—or, at best, very few—eucosmopolitan neritic marine species. Rather, we predict that with morphological, genetic, historical, and related investigations (applying, in part, established criteria by which to distinguish native from non-native species as noted earlier) hundreds if not thousands of species of protists, invertebrates, and algae will prove to re-bin into neocosmopolitan, provincial, or pseudocosmopolitan categories. If the latter two, this is a further signal of the scale of underestimation of marine biodiversity. This hypothesis urges us, in the meantime, to describe what species remain as unresolved cosmopolitans. As noted previously, this category may encompass the vast majority of marine species to which are currently attributed cosmopolitan distributions.
The Way Forward
We offer here a framework that we believe accommodates most if not all circumstances that would explain why what are believed to be single species would occur in multiple oceans. Much work remains (see Box 2). Provincial (scenario III) species may yet be hidden within morphologically-determined neocosmopolitan (scenario II) taxa. Further resolution awaits a rapidly growing number of pseudocosmopolitan taxa; here, particularly pressing is that the proliferation of un-named lineages be steadily replaced with binomial nomenclature, with each named taxon delineated as clearly as possible by all available genetic, morphological, ecological, and distribution data. Thousands of unresolved cosmopolitan species also await resolution. As new records of widespread species–both known invasions and those ostensibly “native”–are detected, and as undescribed widespread species are found, we urge that consideration be given to where such taxa fit into this new global framework. Assigning as many species (both fauna and flora) as possible in regional biotas into our five categories may reveal lacunae in our model. More importantly, from such regional work may arise a global understanding of the relative diversity—the weight, in a sense—of each scenario, and thus lead to a more sophisticated understanding of the past, present, and future of marine biodiversity.
Box 2. Future research needs.
• Investment in integrated taxonomic expertise, with emphasis on skillsets bridging morphological and genetic data generation and analysis
• The development of novel criteria, and further elucidation of existing guidelines, for assignment of neocosmopolitan status based on history, distribution, association with anthropogenic vectors of introduction, etc.
• Rigorous investigation of presumed neocosmopolitan species with suspicious distributions bridging cold temperate and deep tropical latitudes
• Resolution of known pseudocosmopolitan taxa, replacing unnamed lineages with accepted binomials attached to diagnostic criteria accessible via morphological and/or genetic analysis
• Investigation of methods for assigning species names to lineages determined solely by multilocus genetic evidence coupled with development of formally accepted protocols for making such assignments (including development of standard protocols for incorporating genetic evidence for divergent evolutionary lineages into widely accessible taxonomic descriptions)
• Continued exploration of unresolved cosmopolitan species, with the aim of resolving taxonomic and biogeographic history sufficiently to assign provincial, neocosmopolitan, or eucosmopolitan status.
Author Contributions
JD and JC both developed the conceptual framework, assembled appropriate literature, and wrote the manuscript.
Conflict of Interest Statement
The authors declare that the research was conducted in the absence of any commercial or financial relationships that could be construed as a potential conflict of interest.
Acknowledgments
We are indebted to Agnese Marchini and Anna Occhipinti-Ambrogi for providing us this opportunity to synthesize and present our thoughts on cosmopolitan species in the ocean. We thank the American Association for the Advancement of Science for permission to reprint Figure 2A. We are grateful to three reviewers for comments that improved the manuscript. The United States Environmental Protection Agency, through its Office of Research and Development, supported the research described here. Though it has been subjected to Agency administrative review and approved for publication, its content does not necessarily reflect official Agency policy.
References
Ahyong, S., Costello, M. J., Galil, B. S., Gollasch, S., Hutchings, P., Katsanevakis, S., et al. (2018). World Register of Introduced Marine Species (WRIMS). Available online at: http://www.marinespecies.org/introduced (Accessed May, 2018)
Alexander, M. E., Simon, C., Griffiths, C. L., Sibanda, S., Miza, S., Groenewald, B., et al. (2016). Back to the future: reflections and directions of South African marine bioinvasion research. Afr. J. Mar. Sci. 38, 141–144. doi: 10.2989/1814232X.2016.1159984
Andreakis, N., Procaccini, G., Maggs, C., and Kooistra, W. H. (2007). Phylogeography of the invasive seaweed Asparagopsis (Bonnemaisoniales, Rhodophyta) reveals cryptic diversity. Mol. Ecol. 16, 2285–2299. doi: 10.1111/j.1365-294X.2007.03306.x
Ashton, G. V., Stevens, M. I., Hart, M. C., Green, D. H., Burrows, M. T., Cook, E. J., et al. (2008). Mitochondrial DNA reveals multiple Northern Hemisphere introductions of Caprella mutica (Crustacea, Amphipoda). Mol. Ecol. 17, 1293–1303. doi: 10.1111/j.1365-294X.2007.03668.x
Ashton, G. V., Willis, K. J., Cook, E. J., and Burrows, M. (2007). Distribution of the introduced amphipod, Caprella mutica Schurin, 1935 (Amphipoda: Caprellida: Caprellidae) on the west coast of Scotland and a review of its global distribution. Hydrobiologia 590, 31–41. doi: 10.1007/s10750-007-0754-y
Asif, J. H., and Krug, P. J. (2012). Lineage distribution and barriers to gene flow among populations of the globally invasive marine mussel Musculista senhousia. Biol. Invasions 14, 1431–1444. doi: 10.1007/s10530-011-0169-6
Baratti, M., Goti, E., and Messana, G. (2005). High level of genetic differentiation in the marine isopod Sphaeroma terebrans (Crustacea Isopoda Sphaeromatidae) as inferred by mitochondrial DNA analysis. J. Exp. Mar. Biol. Ecol. 315, 225–234. doi: 10.1016/j.jembe.2004.09.020
Bastrop, R., Jürss, K., and Sturmbauer, C. (1998). Cryptic species in a marine polychaete and their independent introduction from North America to Europe. Mol. Biol. Evol. 15, 97–103. doi: 10.1093/oxfordjournals.molbev.a025919
Batistić, M., and Garić, R. (2015). The case of Bougainvillia triestina Hartlaub 1911 (Hydrozoa, Cnidaria): a 100-year-long struggle for recognition. Mar. Ecol. 37, 145–154. doi: 10.1111/maec.12270
Bax, N., Williamson, A., Aguero, M., Gonzalez, E., and Geeves, W. (2003). Marine invasive alien species: a threat to global biodiversity. Mar. Policy 27, 313–323. doi: 10.1016/S0308-597X(03)00041-1
Blakeslee, A. M. H., Kamakura, Y., Onufrey, J., Makino, W., Urabe, J., Park, S., et al. (2017). Reconstructing the invasion history of the Asian shorecrab, Hemigrapsus sanguineus (De Haan 1835) in the Western Atlantic. Mar. Biol. 164:47. doi: 10.1007/s00227-017-3069-1.
Boos, K., Ashton, G. V., and Cook, E. J. (2011). “Invading Nature - Springer Series in Invasion Ecology, The Japanese skeleton shrimp Caprella mutica (Crustacea, Amphipoda): a global invader of coastal waters,” in In the Wrong Place - Alien Marine Crustaceans: Distribution, Biology and Impacts. Vol 6. eds B. Galil, P. Clark, and J. Carlton (Dordrecht: Springer), 129–156.
Bortolus, A., Carlton, J. T., and Schwindt, E. (2015). Reimagining South American coasts: unveiling the hidden invasion history of an iconic ecological engineer. Divers Distribut. 21, 1267–1283. doi: 10.1111/ddi.12377
Bouchemousse, S., Bishop, J. D., and Viard, F. (2016). Contrasting global genetic patterns in two biologically similar, widespread and invasive Ciona species (Tunicata, Ascidiacea). Sci. Rep. 6:24875. doi: 10.1038/srep24875
Brasier, M. J., Wiklund, H., Neal, L., Jeffreys, R., Linse, K., Ruhl, H., et al. (2016). DNA barcoding uncovers cryptic diversity in 50% of deep-sea Antarctic polychaetes. R. Soc. Open Sci. 3:160432. doi: 10.1098/rsos.160432
Bronstein, O., Georgopoulou, E., and Kroh, A. (2017). On the distribution of the invasive long-spined echinoid Diadema setosum and its expansion in the Mediterranean Sea. Mar. Ecol. Prog. Ser. 583, 163–178. doi: 10.3354/meps12348
Brunetti, R., Gissi, C., Pennati, R., Caicci, F., Gasparini, F., and Manni, L. (2015). Morphological evidence that the molecularly determined Ciona intestinalis type A and type B are different species: Ciona robusta and Ciona intestinalis. J. Zoolog. Syst. Evolut. Res. 53, 186–193. doi: 10.1111/jzs.12101
Brunetti, R., Manni, L., Mastrototaro, F., Gissi, C., and Gasparini, F. (2017). Fixation, description and DNA barcode of a neotype for Botryllus schlosseri (Pallas, 1766) (Tunicata, Ascidiacea). Zootaxa 4353, 29–50. doi: 10.11646/zootaxa.4353.1.2
Cabezas, M. P., Navarro-Barranco, C., Ros, M., and Guerra-Garcia, J. M. (2013). Long-distance dispersal, low connectivity and molecular evidence of a new cryptic species in the obligate rafter Caprella andreae Mayer, 1890 (Crustacea: Amphipoda: Caprellidae). Helgol. Mar. Res. 67, 483–497. doi: 10.1007/s10152-012-0337-9
Canning-Clode, J., Fofonoff, P., McCann, L., Carlton, J. T., and Ruiz, G. (2013). Marine invasions on a subtropical island: fouling studies and new records in a recent marina on Madeira Island (Eastern Atlantic Ocean). Aquat. Invas. 8, 261–270. doi: 10.3391/ai.2013.8.3.02
Capinha, C., Essl, F., Seebens, H., Moser, D., and Pereira, H. M. (2015). The dispersal of alien species redefines biogeography in the Anthropocene. Science 384, 1248–1251. doi: 10.1126/science.aaa8913
Caputi, L., Andreakis, N., Mastrototaro, F., Cirino, P., Vassillo, M., and Sordino, P. (2007). Cryptic speciation in a model invertebrate chordate. Proc. Natl. Acad. Sci. U.S.A. 104, 9364–9369. doi: 10.1073/pnas.0610158104
Cardigos, F., Tempera, F., Avila, S., Goncalves, J., Colaco, A., and Santos, R. S. (2006). Non-indigenous marine species of the Azores. Helgol. Mar. Res. 60, 160–169. doi: 10.1007/s10152-006-0034-7
Carlton, J. T. (1979). History, Biogeography, and Ecology of the Introduced Marine and Estuarine Invertebrates of the Pacific Coast of North America. Ph.D. dissertation, Davis: University of California.
Carlton, J. T. (1987). Patterns of transoceanic marine biological invasions in the Pacific Ocean. Bull. Mar. Sci. 41, 452–465.
Carlton, J. T. (1996). Biological invasions and cryptogenic species. Ecology 77, 1653–1655. doi: 10.2307/2265767
Carlton, J. T. (1999). “The scale and ecological consequences of biological invasions in the world's oceans,”in Invasive Species and Biodiversity Management, eds O. T. Sandlund, P. J. Schel, and Å. Viken (Dordrecht: Kluwer Academic Publishers), 195–212.
Carlton, J. T. (2001). Introduced Species in U.S. Coastal Waters: Environmental Impacts and Management Priorities. Arlington, TX: Pew Oceans Commission.
Carlton, J. T. (2002). “Bioinvasion ecology: assessing invasion impact and Scale,” in Invasive Aquatic Species of Europe. Distribution, Impacts, and Management, eds E. Leppäkoski, S. Gollasch, and S. Olenin (Dordrecht: Kluwer Academic Publishers), 7–19
Carlton, J. T. (2003). Community assembly and historical biogeography in the North Atlantic Ocean: the potential role of human-mediated dispersal vectors. Hydrobiologia 503, 1–8. doi: 10.1023/B:HYDR.0000008479.90581.e1
Carlton, J. T. (2009). “Deep invasion ecology and the assembly of communities in historical time,” in Biological Invasions in Marine Ecosystems: Ecological, Management, and Geographic Perspectives, eds. G. Rilov and J. A. Crooks (Berlin: Springer-Verlag), 13–56.
Carlton, J. T. (2011). “The global dispersal of marine and estuarine crustaceans,” in In the Wrong Place: Alien Marine Crustaceans – Distribution, Biology and Impacts, eds B. S. Galil, P. F. Clark, and J. T. Carlton (Dordrecht: Springer), 3–23.
Carlton, J. T., Chapman, J. W., Geller, J. B., Miller, J. A., Carlton, D. A., McCuller, M. I., et al. (2017). Tsunami-driven rafting: Transoceanic species dispersal and implications for marine biogeography. Science 357, 1402–1406. doi: 10.1126/science.aao1498
Carlton, J. T., Chapman, J. W., Geller, J. B., Miller, J. A., Ruiz, G. M., Carlton, D. A., et al. (2018). Ecological and biological studies of ocean rafting: Japanese tsunami debris in North America and the Hawaiian Islands. Aquat. Invas. 13, 1–19. doi: 10.3391/ai.2018.13.1.01
Carlton, J. T., and Eldredge, L. G. (2009). Marine Bioinvasions of Hawai'i. The Introduced and Cryptogenic Marine and Estuarine Animals and Plants of the Hawaiian Archipelago. Honolulu: Bishop Museum Bulletins in Cultural and Environmental Studies 4, Bishop Museum Press.
Carlton, J. T., and Eldredge, L. G. (2015). “Update and revision of the marine bioinvasions of Hawai'i: the introducedand cryptogenic marine and estuarine animals and plants of the hawaiian archipelago,” in Lucius, G. Eldredge III Memorial Volume: Tribute to a Polymath, eds N. L. Evenhuis and J. T. Carlton (Honolulu, HI: Bishop Museum Bulletin Zoology), 25–47.
Carlton, J. T., and Fowler, A. (2018). Ocean rafting and marine debris: A broader vector menu requires a greater appetite for invasion biology research support. Aquat Invas. 13, 11–15. doi: 10.3391/ai.2018.13.1.02
Carlton, J. T., and Iverson, E. W. (1981). Biogeography and natural history of Sphaeroma walkeri Stebbing (Crustacea: Isopoda) and its introduction to San Diego Bay, California. J. Nat. Hist. 15, 31–48. doi: 10.1080/00222938100770031
Carlton, J. T., Reid, D. M., and van Leeuwen, H. (1995). Shipping Study. The Role of Shipping in the Introduction of Non-indigenous Aquatic Organisms to the Coastal Waters of the United States (Other Than the Great Lakes) and an Analysis of Control Options. Report Number CG-D-11-95. Government Accession Number AD-A294809, The National Sea Grant College Program/Connecticut Sea Grant Project R/ES-6. Department of Transportation, United States Coast Guard, Washington, DC; Groton, CT, 213.
Carlton, J. T., and Ruiz, G. M. (2005). “The magnitude and consequences of bioinvasions in marine ecosystems: implications for conservation biology,” in Marine Conservation Biology: The Science of Maintaining the Sea's Biodiversity, E. A. Norse, and L. B. Crowder (Washington, DC: Island Press), 123–148
Carlton, J. T., and Ruiz, G. M. (2015). “Anthropogenic vectors of marine and estuarine invasions: an overview framework,” in Biological Invasions in Changing Ecosystems: Vectors, Ecological Impacts, Management and Predictions, ed J. Canning-Clode (Warsaw; Berlin: De Gruyter Open Ltd), 24–36.
Casson, L. (1995). Ships and Seamanship in the Ancient World. Baltimore, MD: Johns Hopkins University Press.
Chapman, J. W., and Carlton, J. T. (1994). Predicted discoveries of the introduced isopod Synidotea laevidorsalis (Miers, 1881). J. Crustacean Biol. 14, 700–714. doi: 10.2307/1548863
Church, S. K. (2018). The Eurasian silk road: its historical roots and the Chinese immigration. Cambridge J. Euras. Stud. 2:XW4ESF. doi: 10.22261/CJES.XW4ESF
Cohen, A. N., and Carlton, J. T. (1995). Biological Study. Nonindigenous Aquatic Species in a United States Estuary: A Case Study of the Biological Invasions of the San Francisco Bay and Delta. A Report for the United States Fish and Wildlife Service, Washington, D.C., and The National Sea Grant College Program, Connecticut Sea Grant, NTIS Report Number PB96-166525, 246.
Cohen, O. R., Walters, L. J., and Hoffman, E. A. (2014). Clash of the titans: a multi-species invasion with high gene flow in a globally invasive titan acorn barnacle. Biol. Invasions 16, 1743–1756. doi: 10.1007/s10530-013-0624-7
Concepcion, G. T., Crepeau, M. W., Wagner, D., Kahng, S. E., and Toonen, R. J. (2008). An alternative to ITS, a hypervariable, single-copy nuclear intron in corals, and its use in detecting cryptic species within the octocoral genus Carijoa. Coral Reefs 27, 323–336. doi: 10.1007/s00338-007-0323-x
Concepcion, G. T., Kahng, S. E., Crepeau, M. W., Franklin, E. C., Coles, S. L., and Toonen, R. J. (2010). Resolving natural ranges and marine invasions in a globally distributed octocoral (genus Carijoa). Mar. Ecol. Prog. Ser. 401, 113–127. doi: 10.3354/meps08364
Costello, M. J., Tsai, P., Wong, P. S., Cheung, A. K. L., Basher, Z., and Chaudhary, C. (2017). Marine biogeographic realms and species endemicity. Nat. Commun. 8:1057. doi: 10.1038/s41467-017-01121-2
Cristescu, M. E. (2015). Genetic reconstructions of invasion history. Mol. Ecol. 24, 2212–2225. doi: 10.1111/mec.13117
Curini-Galletti, M., Artois, T., Delogu, V., De Smet, W. H., Fontaneto, D., Jondelius, U., et al. (2012). Patterns of diversity in soft-bodied meiofauna: dispersal ability and body size matter. PLoS ONE 7:e33801. doi: 10.1371/journal.pone.0033801
Díaz-Tapia, P., Sook Kim, M., Secilla, A., Bárbara, I., and Cremades, J. (2013). Taxonomic reassessment of Polysiphonia foetidissima (Rhodomelaceae, Rhodophyta) and similar species, including P. schneideri, a newly introduced species in Europe. Eur. J. Phycol. 48, 345–362. doi: 10.1080/09670262.2013.842655
Darling, J. A. (2015). Genetic studies of aquatic biological invasions: closing the gap between research and management. Biol. Invasions 17, 951–971. doi: 10.1007/s10530-014-0726-x
Darling, J. A., Bagley, M. J., Roman, J., Tepolt, C. K., and Geller, J. B. (2008). Genetic patterns across multiple introductions of the globally invasive crab genus Carcinus. Mol. Ecol. 17, 4992–5007. doi: 10.1111/j.1365-294X.2008.03978.x
Darling, J. A., Galil, B., Carvalho, G. R., Rius, M., Viard, F., and Piraino, S. (2017). Recommendations for developing and applying genetic tools to assess and manage biological invasions in marine ecosystems. Mar. Policy 85, 54–64. doi: 10.1016/j.marpol.2017.08.014
Darling, K. F., Wade, C. M., Stewart, I. A., Kroon, D., Dingle, R., and Brown, A. J. L. (2000). Molecular evidence for genetic mixing of Arctic and Antarctic subpolar populations of planktonic foraminifers. Nature 405, 43–47. doi: 10.1038/35011002
David, A. A., and Loveday, B. R. (2017). The role of cryptic dispersal in shaping connectivity patterns of marine populations in a changing world. J. Mar. Biol. Assoc. U.K. 98, 647–655. doi: 10.1017/S0025315417000236
David, A. A., Matthee, C. A., Loveday, B. R., and Simon, C. A. (2016). Predicting the dispersal potential of an invasive polychaete pest along a complex coastal biome. Integr. Comp. Biol. 56, 600–610. doi: 10.1093/icb/icw011
Davidson, S. K., and Haygood, M. G. (1999). Identification of sibling species of the bryozoan Bugula neritina that produce different anticancer bryostatins and harbor distinct strains of the bacterial symbiont “Candidatus endobugula sertula”. Biol. Bull. 196, 273–280. doi: 10.2307/1542952
De Barros, R. C., Da Rocha, R. M., and Pie, M. R. (2009). Human-mediated global dispersion of Styela plicata (Tunicata, Ascidiacea). Aquat. Invas. 4, 45–57. doi: 10.3391/ai.2009.4.1.4
Desalle, R., Egan, M. G., and Siddall, M. (2005). The unholy trinity: taxonomy, species delimitation and DNA barcoding. Philos. Trans. R. Soc. B Biol. Sci. 360, 1905–1916. doi: 10.1098/rstb.2005.1722
Dijoux, L., Viard, F., and Payri, C. (2014). The more we search, the more we find: discovery of a new lineage and a new species complex in the genus Asparagopsis. PLoS ONE 9:e103826. doi: 10.1371/journal.pone.0103826
Dreyer, E. L. (2006). Zheng He. China and the Oceans in the Early Ming Dynasty: 1405–1433. London: Pearson.
Einfeldt, A. L., and Addison, J. A. (2015). Anthropocene invasion of an ecosystem engineer: resolving the history of Corophium volutator (Amphipoda: Corophiidae) in the North Atlantic. Biol. J. Linnean Soc. 115, 288–304 doi: 10.1111/bij.12507
Eltis, D. (2007). A brief overview of the trans-Atlantic slave trade. In, Voyages: The Trans-Atlantic Slave Trade Database. Available online at: http://www.slavevoyages.org/assessment/essays (Accessed July 2018).
Engelen, A., Serebryakova, A., Ang, P., Britton-Simmons, K., Mineur, F., Pedersen, M., et al. (2015). Circumglobal invasion by the brown seaweed Sargassum muticum. Oceanogr. Mar. Biol. 53, 81–126. doi: 10.1201/b18733-4
Erlandson, J. M. (2017). “Coastlines, marine ecology, and maritime dispersals in human history,” in Human Dispersal and Species Movement From Prehistory to the Present, eds N. Boivin, R. Crassard, and M. Petraglia (Cambridge: Cambridge University Press), 147–163.
Fehlauer-Ale, K. H., Mackie, J. A., Lim-Fong, G. E., Ale, E., Pie, M. R., and Waeschenbach, A. (2014). Cryptic species in the cosmopolitan Bugula neritina complex (Bryozoa, Cheilostomata). Zool. Scr. 43, 193–205. doi: 10.1111/zsc.12042
Fernald, M. L. (1926). The antiquity and dispersal of vascular plants. Quart. Rev. Biol. 1, 212–245. doi: 10.1086/394244
Ferreira, C. E. L., Junqueira, A., de, O. R., Villac, M. C., and Lopes, R. M. (2009). “Marine bioinvasions in the Brazilian coast: brief report on history of events, vectors, ecology, impacts, and management of non-indigenous species,” in Biological Invasions in Marine Ecosystems, eds G. Rilov and J. A. Crooks (Berlin: Springer-Verlag), 459–477.
Folino-Rorem, N. C., Darling, J. A., and D'ausilio, C. A. (2008). Genetic analysis reveals multiple cryptic invasive species of the hydrozoan genus Cordylophora. Biol. Invasions 11, 1869–1882. doi: 10.1007/s10530-008-9365-4
Galil, B. S. (2009). Taking stock: inventory of alien species in the Mediterranean sea. Biol. Invasions 11, 359–372. doi: 10.1007/s10530-008-9253-y
Galil, B. S., Boero, F., Campbell, M. L., Carlton, J. T., Cook, E., Fraschetti, W., et al. (2015). ‘Double trouble': the expansion of the Suez Canal and marine bioinvasions in the Mediterranean Sea. Biol. Invasions 17, 973–976. doi: 10.1007/s10530-014-0778-y
Galil, B. S., Marchini, A., Occhipinti-Ambrogi, A., Minchin, D., Narščius, A., Ojaveer, H., et al. (2014). International arrivals: widespread bioinvasions in European Seas. Ethol. Ecol. Evolut. 26, 152–171. doi: 10.1080/03949370.2014.897651
Gartner, J. V. Jr. (1998). “Are circumglobal lanternfish species (Pisces, Myctophidae) really circumglobal? A return to the question using Ceratoscopelus “warmingii” studies in the tropical-subtropical Atlantic Ocean and eastern Gulf of Mexico,” IOC Workshop Report No. 142, 114–119.
Geller, J., Sotka, E. E., Kado, R., Palumbi, S. R., and Schwindt, E. (2008). Sources of invasions of a northeastern Pacific acorn barnacle, Balanus glandula, in Japan and Argentina. Mar. Ecol. Prog. Ser. 358, 211–218. doi: 10.3354/meps07466
Geller, J. B., Carlton, J. T., and Powers, D. A. (1994). PCR-based detection of mtDNA haplotypes of native and invading mussels on the northeastern Pacific coast: latitudinal pattern of invasion. Mar. Biol. 119, 243–249. doi: 10.1007/BF00349563
Geller, J. B., Darling, J. A., and Carlton, J. T. (2010). Genetic perspectives on marine biological invasions. Ann. Rev. Mar. Sci. 2, 367–393. doi: 10.1146/annurev.marine.010908.163745
Goldstien, S. J., Dupont, L., Viard, F., Hallas, P. J., Nishikawa, T., Schiel, D. R., et al. (2011). Global phylogeography of the widely introduced North West Pacific ascidian Styela clava. PLoS ONE 6:e16755. doi: 10.1371/journal.pone.0016755
Gollasch, S. (2006). Overview on introduced aquatic species in European navigational and adjacent waters. Helgol. Mar. Res. 60, 84–89 doi: 10.1007/s10152-006-0022-y
Gollasch, S., Haydar, D., Minchin, D., Wolff, W. J., and Reise, K. (2009). “Introduced aquatic species of the North Sea coasts and adjacent brackish waters,” in Biological Invasions in Marine Ecosystems, eds G. Rilov and J. A. Crooks (Berlin Heidelberg: Springer-Verlag), 507–528.
Govindarajan, A. F., Carman, M. R., Khaidarov, M. R., Semenchenko, A., and Wares, J. P. (2017). Mitochondrial diversity in Gonionemus (Trachylina:Hydrozoa) and its implications for understanding the origins of clinging jellyfish in the Northwest Atlantic Ocean. PeerJ. 5:e3205. doi: 10.7717/peerj.3205
Grassle, J., and Grassle, J. P. (1976). Sibling species in the marine pollution indicator, Capitella (Polychaeta). Science 192, 567–569. doi: 10.1126/science.1257794
Hajibabaei, M., Singer, G. A., Hebert, P. D., and Hickey, D. A. (2007). DNA barcoding: how it complements taxonomy, molecular phylogenetics and population genetics. Trends Genet. 23, 167–172. doi: 10.1016/j.tig.2007.02.001
Hancock, Z. B., Goeke, J. A., and Wicksten, M. K. (2017). A sea anemone of many names: a review of the taxonomy and distribution of the invasive actiniarian Diadumene lineata (Diadumenidae), with records of its reappearance on the Texas coast. Zookeys 706, 1–15. doi: 10.3897/zookeys.706.19848
Harger, J. R. (1972a). Variation and relative “niche” size in the sea mussel Mytilus edulis in association with Mytilus californianus. Veliger 14, 275–283.
Harger, J. R. (1972b). Competitive co-existence: maintenance of interacting associations of the sea mussels Mytilus edulis and Mytilus californianus. Veliger 14, 387–410.
Harmelin, J.-G., Vieira, L. M., Ostrovsky, A. N., Cáceres-Chamizo, J. P., and Sanner, J. (2012). Scorpiodinipora costulata (Canu & Bassler, 1929) (Bryozoa, Cheilostomata), a taxonomic and biogeographic dilemma: complex of cryptic species or human-mediated cosmopolitan colonizer? Zoosystema 34, 123–138. doi: 10.5252/z2012n1a5
Haydar, D. (2012). What is natural? The scale of cryptogenesis in the North Atlantic Ocean. Divers. Distribut. 18, 101–110. doi: 10.1111/j.1472-4642.2011.00863.x
Haydar, D., Hoarau, G., Olsen, J. L., Stam, W. T., and Wolff, W. J. (2011). Introduced or glacial relict? Phylogeography of the cryptogenic tunicate Molgula manhattensis (Ascidiacea, Pleurogona). Divers. Distribut. 17, 68–80. doi: 10.1111/j.1472-4642.2010.00718.x
Hayden, B. J., Inglis, G. J., and Schiel, D. R. (2009). “Marine invasions in New Zealand: A history of complex supply-side dynamics,” in Biological Invasions in Marine Ecosystems, eds G. Rilov and J. A. Crooks (Berlin: Springer-Verlag), 409–423.
Heesch, S., Broom, J. E. S., Neill, K. F., Farr, T. J., Dalen, J. L., and Nelson, W. A. (2009). Ulva, Umbraulva and Gemina: genetic survey of New Zealand taxa reveals diversity and introduced species. Eur. J. Phycol. 44, 143–154. doi: 10.1080/09670260802422477
Hewitt, C. L., Campbell, M. L., and Schaffelke, B. (2007). Introductions of seaweeds: accidental transfer pathways and mechanism. Botanica Marina 50, 326–337. doi: 10.1515/BOT.2007.038
Hilario, A., Metaxas, A., Gaudron, S. M., Howell, K. L., Mercier, A., Mestre, N. L. C., et al. (2015). Estimating dispersal distance in the deep sea: challenges and applications to marine reserves. Front. Mar. Sci. 2:6. doi: 10.3389/fmars.2015.00006
Hilbish, T. J., Mullinax, A., Dolven, S. I., Meyer, A., Koehn, R. K., and Rawson, P. D. (2000). Origin of the antitropical distribution pattern in marine mussels (Mytilus spp.): routes and timing of transequatorial migration. Mar. Biol. 136, 69–77. doi: 10.1007/s002270050010
Hoagstrom, C. W., and Berry, C. R. Jr. (2006). Island biogeography of native fish faunas among Great Plains drainage basins: basin scale features influence composition. Am. Fish. Soc. Sympos. 48, 221–264.
Hobbs, N.-V., Lazo-Wasem, E., Faasse, M., Cordell, J., Chapman, J., Smith, C., et al. (2015). Going global: The introduction of the Asian isopod Ianiropsis serricaudis Gurjanova (Crustacea: Peracarida) to North America and Europe. Aquatic Invasions 10, 177–187. doi: 10.3391/ai.2015.10.2.06
Holland, B. S., Dawson, M. N., Crow, G. L., and Hofmann, D. K. (2004). Global phylogeography of Cassiopea (Scyphozoa: Rhizostomeae): molecular evidence for cryptic species and multiple invasions of the Hawaiian Islands. Mar. Biol. 145, 1119–1128. doi: 10.1007/s00227-004-1409-4
Huang, D., Meier, R., Todd, P. A., and Ming Chou, L. M. (2008). Slow mitochondrial COI sequence evolution at the base of the metazoan tree and its implications for DNA barcoding. J. Mol. Evol. 66, 167–174. doi: 10.1007/s00239-008-9069-5
Hurtado, L. A., Mateos, M., Wang, C., Santamaria, C. A., Jung, J., Khalaji-Pirbalouty, V., et al. (2018). Out of Asia: mitochondrial evolutionary history of the globally introduced supralittoral isopod Ligia exotica. Peer J. 6:e4337. doi: 10.7717/peerj.4337
Hutchings, P., and Kupriyanova, E. (2018). Cosmopolitan polychaetes—fact or fiction? Pers. Hist. Perspect. Inverteb. Syst. 32, 1–9. doi: 10.1071/IS17035
Iannelli, F., Pesole, G., and Gissi, C. (2007). Mitogenomics reveals two cryptic species in Ciona intestinalis. Trends Genet. 23, 419–422. doi: 10.1016/j.tig.2007.07.001
International Commission on Zoological Nomenclature ICOZ (2018). Can DNA Be a Type Specimen? [Online]. Available online at: http://iczn.org/content/can-dna-be-type-specimen [Accessed 4 May, 2018].
John, U., Litaker, R. W., Montresor, M., Murray, S., Brosnahan, M. L., and Anderson, D. M. (2014). Formal revision of the Alexandrium tamarense species complex (Dinophyceae) taxonomy: the introduction of five species with emphasis on molecular-based (rDNA) classification. Protist 165, 779–804. doi: 10.1016/j.protis.2014.10.001
Jörger, K. M., and Schrödl, M. (2013). How to describe a cryptic species? Practical challenges of molecular taxonomy. Front. Zool. 10:59. doi: 10.1186/1742-9994-10-59
Klautau, M., Russo, C. A. M., Lazoski, C., Boury-Esnault, N., Thorpe, J. P., and Sole-Cava, A.M. (1999). Does cosmopolitanism result from overconservative systematics? A cases study using the marine sponge Chondrilla nucula. Evolution 53, 1414–1422. doi: 10.1111/j.1558-5646.1999.tb05406.x
Knapp, I. S., Forsman, Z. H., Williams, G. J., Toonen, R. J., and Bell, J. J. (2015). Cryptic species obscure introduction pathway of the blue Caribbean sponge (Haliclona (Soestella) caerulea) (order: Haplosclerida) to Palmyra Atoll, Central Pacific. PeerJ 3:e1170. doi: 10.7717/peerj.1170
Krueger-Hadfield, S. A., Kollars, N., Strand, A., Byers, J., Shainker, S., Terada, R., et al. (2016). The identification of source and vector of a prolific marine invader. BioRxiv 083972. doi: 10.1101/083972
Kuklinksi, P., and Taylor, P. D. (2008). Arctic species of the cheilostome bryozoan Microporella, with a redescription of the type species. J. Nat. Hist. 42, 1893–1906. doi: 10.1080/00222930802126904
Kvist, S., Sarkar, I. N., and Erséus, C. (2010). Genetic variation and phylogeny of the cosmopolitan marine genus Tubificoides (Annelida: Clitellata: Naididae: Tubificinae). Mol. Phylogenet. Evol. 57, 687–702. doi: 10.1016/j.ympev.2010.08.018
Lachner, E. A., Robbins, C. R., and Courtenay, W. R. (1970). Exotic fishes and other aquatic organisms introduced into North America. Smiths. Contrib. Zool. 59:29. doi: 10.5479/si.00810282.59
Langeneck, J., Diez, M. E., Nygren, A., Salazar-Vallejo, S., Carrera-Parra, L. F., Vega Fernández, T., et al. (2018). Worming its way into patagonia: an integrative approach reveals the cryptic invasion by Eulalia clavigera (Annelida: Phyllodocidae). Mar. Biodiv. doi: 10.1007/s12526-018-0864-y
Lawler, A. (2018). Searching for a stone age odysseus. Science 360, 362–363. doi: 10.1126/science.360.6387.362
Leaché, A. D., and Fujita, M. K. (2010). Bayesian species delimitation in West African forest geckos (Hemidactylus fasciatus). Proc. R. Soc. B Biol. Sci. 277, 3071–3077. doi: 10.1098/rspb.2010.0662
Lejeusne, C., Saunier, A., Petit, N., Béguer, M., Otani, M., Carlton, J. T., et al. (2014). High genetic diversity and absence of founder effects in a worldwide aquatic invader. Sci. Rep. 4:5808. doi: 10.1038/srep05808
Leray, M., and Knowlton, N. (2016). Censusing marine eukaryotic diversity in the twenty-first century. Philos. Trans. R. Soc. Lond. B Biol. Sci. 371:20150331. doi: 10.1098/rstb.2015.0331
Lessios, H. A., Kessing, B. D., and Pearse, J. S. (2001). Population structure and speciation in tropical seas: global phylogeography of the sea urchin Diadema. Evolution 55, 955–975. doi: 10.1554/0014-3820(2001)055[0955:PSASIT]2.0.CO;2
Levathes, L. (1996). When China Ruled the Seas: The Treasure Fleet of the Dragon Throne 1405–1433. New York, NY: Oxford University Press, 256 pp.
Liu, Y., Chen, Z., and Blue, G. (2014). Zheng He's maritime voyages (1405-1433) and China's Relations with the Indian Ocean World. A Multilingual Bibliography. Leiden: Brill.
Low-Pfeng, A. M., and Peters-Recagno, E. M. (2012). Invertebrados marinos exóticos en el Pacífico mexicano. Geomare, AC: INE- SEMARNAT.
Macgillivary, M. L., and Kaczmarska, I. (2015). Paralia (Bacillariophyta) stowaways in ship ballast: implications for biogeography and diversity of the genus. J. Biol. Res. (Thessaloniki) 22:2. doi: 10.1186/s40709-015-0024-5
Mackie, J. A., Darling, J. A., and Geller, J. B. (2012). Ecology of cryptic invasions: latitudinal segregation among Watersipora (Bryozoa) species. Sci. Rep. 2:871. doi: 10.1038/srep00871
Mackie, J. A., Keough, M. J., and Christidis, L. (2006). Invasion patterns inferred from cytochrome oxidase I sequences in three bryozoans, Bugula neritina, Watersipora subtorquata, and Watersipora arcuata. Mar. Biol. 149, 285–295. doi: 10.1007/s00227-005-0196-x
Malfant, M., Darras, S., and Viard, F. (2018). Coupling molecular data and experimental crosses sheds light about species delineation: a case study with the genus Ciona. Sci. Rep. 8:1480. doi: 10.1038/s41598-018-19811-2
Marchini, A., and Cardeccia, A. (2017). Alien amphipods in a sea of troubles: cryptogenic species, unresolved taxonomy and overlooked introductions. Mar. Biol. 16:69. doi: 10.1007/s00227-017-3093-1
Martel, C., Viard, F., Bourguet, D., and Garcia-Meunier, P. (2004). Invasion by the marine gastropod Ocinebrellus inornatus in France, I. Scenario for the source of introduction. J. Exp. Mar. Biol. Ecol. 305, 155–170. doi: 10.1016/j.jembe.2003.11.011
McCann, L., Keith, I., Carlton, J., Ruiz, G., Dawson, T., and Collins, K. (2015). First record of the non-native bryozoan Amathia (= Zoobotryon) verticillata (delle Chiaje, 1822) (Ctenostomata) in the galápagos islands. Bioinvasions Rec. 4, 255–260. doi: 10.3391/bir.2015.4.4.04
McDonald, J. H., and Koehn, R. K. (1988). The mussels Mytilus galloprovincialis and Mytilus trossulus on the Pacific coast of North America. Mar. Biol. 99, 111–118. doi: 10.1007/BF00644984
McGrail, S. (2015). Early ships and seafaring. Water transport beyond Europe. Pen and Sword, 192 pp.
Mead, A., Carlton, J. T., Griffiths, C. L., and Rius, M. (2011). Introduced and cryptogenic marine and estuarine species of South Africa. J. Nat. Hist. 45, 2463–2524. doi: 10.1080/00222933.2011.595836
Meusnier, I., Valero, M., Olsen, J. L., and Stam, W. T. (2004). Analysis of rDNA ITS1 indels in Caulerpa taxifolia (Chlorophyta) supports a derived, incipient species status for the invasive strain. Eur. J. Phycol. 39, 83–92. doi: 10.1080/09670260310001646531
Meyer, A., Bleidorn, C., Rouse, G. W., and Hausen, H. (2007). Morphological and molecular data suggest a cosmopolitan distribution of the polychaete Proscoloplos cygnochaetus Day, 1954 (Annelida, Orbiniidae). Mar. Biol. 153, 879–889. doi: 10.1007/s00227-007-0860-4
Meyer, C. P., and Paulay, G. (2005). DNA barcoding: error rates based on comprehensive sampling. PLoS Biol. 3:e422. doi: 10.1371/journal.pbio.0030422
Miglietta, M. P., Odegard, D., Faure, B., and Faucci, A. (2015). 16:69. doi: 10.1007/s00227-017-3093-1
Miller, P. A., Elliott, N. G., Koutoulis, A., Kube, P. D., and Vaillancourt, R. E. (2012). Genetic diversity of cultured, naturalized, and native Pacific oysters, Crassostrea Gigas, determined from multiplexed microsatellite markers. J. Shellfish Res. 31, 611–617. doi: 10.2983/035.031.0303
Minchin, D., and Gollasch, S. (2002). “Vectors - How exotics get around,”in Invasive Aquatic Species of Europe: Distribution, Impacts and Management, eds E. Leppäkoski,S. Gollasch, and S. Olenin (Dordrecht: Kluwer Academic Publishers), 183–192.
Minchin, D., Gollasch, S., and Wallentinus, I. (2005). Vector Pathways and the Spread of Exotic Species in the Sea. ICES Cooperative Research Report 271. Copenhagen: ICES.
Muirhead, J. R., Minton, M. S., Miller, W. A., and Ruiz, G. M. (2015). Projected effects of the panama canal expansion on shipping traffic and biological invasions. Div. Distrib. 21, 75–87. doi: 10.1111/ddi.12260
Nikulina, E. A., Hanel, R., and Schäfer, P. (2007). Cryptic speciation and paraphyly in the cosmopolitan bryozoan Electra pilosa- Impact of the Tethys closing on species evolution. Mol. Phylogeneics Evol. 45, 765–776. doi: 10.1016/j.ympev.2007.07.016
Nydam, M. L., and Harrison, R. G. (2007). Genealogical relationships within and among shallow-water Ciona species (Ascidiacea). Mar. Biol. 151, 1839–1847. doi: 10.1007/s00227-007-0617-0
Nygren, A. (2004). Revision of Autolytinae (Syllidae: Polychaeta). Zootaxa 680, 1–314. doi: 10.5281/zenodo.157809
Nygren, A. (2014). Cryptic polychaete diversity: a review. Zool. Scr. 43, 172–183. doi: 10.1111/zsc.12044
Orensanz, J. M., Schwindt, E., Pastorino, G., Bortolus, A., Casas, G., Darrigran, G., et al. (2002). No longer the pristine confines of the world: a survey of exotic marine species in the southwestern Atlantic. Biol. Invasions 4, 115–143. doi: 10.1023/A:1020596916153
Otani, M. (2006). “Important vectors for marine organisms unintentionally introduced to Japanese waters,” in Assessment and Control of Biological Invasion Risks, eds F. Koike, M. N. Clout, and M. Kawamichi, De Poorter, M., and Iwatsuki, K (Gland: Shoukadoh Book Sellers, Kyoto, Japan and IUCN), 92–103
Pante, E., Puillandre, N., Viricel, A., Arnaud-Haond, S., Aurelle, D., Castelin, M., et al. (2015a). Species are hypotheses: avoid connectivity assessments based on pillars of sand. Mol. Ecol. 24, 525–544. doi: 10.1111/mec.13048
Pante, E., Schoelinck, C., and Puillandre, N. (2015b). From integrative taxonomy to species description: one step beyond. Syst. Biol. 64, 152–160. doi: 10.1093/sysbio/syu083
Pappalardo, P., Pringle, J. M., Wares, J. P., and Byers, J. E. (2015). The location, strength, and mechanisms behind marine biogeographic boundaries of the east coast of North America. Ecography 38, 722–731. doi: 10.1111/ecog.01135
Park, C., Tim, S.-T., Hong, J.-S., and Choi, K.-H. (2017). A rapid assessment survey of invasive species of macrobenthic invertebrates in Korean waters. Ocean Sci. J. 52, 387–395. doi: 10.1007/s12601-017-0024-5
Pearson, D. L., Hamilton, A. L., and Erwin, T. L. (2011). Recovery plan for the endangered taxonomy profession. Bioscience 61, 58–63. doi: 10.1525/bio.2011.61.1.11
Pennati, R., Ficetola, G. F., Brunetti, R., Caicci, F., Gasparini, F., Griggio, F., et al. (2015). Morphological differences between larvae of the Ciona intestinalis species complex: hints for a valid taxonomic definition of distinct species. PLoS ONE 10:e0122879. doi: 10.1371/journal.pone.0122879
Perez-Portela, R., Arranz, V., Rius, M., and Turon, X. (2013). Cryptic speciation or global spread? The case of a cosmopolitan marine invertebrate with limited dispersal capabilities. Sci. Rep. 3:3197. doi: 10.1038/srep03197
Petersen, M. E. (1984). Chaetopterus variopedatus (Annelida: Polychaeta): another victim of the “characteristic species” disease. Am. Zool. 23:62A.
Pfenninger, M., Reinhardt, F., and Streit, B. (2002). Evidence for cryptic hybridization between different evolutionary lineages of the invasive clam genus Corbicula (Veneroida, Bivalvia). J. Evol. Biol. 15, 818–829. doi: 10.1046/j.1420-9101.2002.00440.x
Pilgrim, E. M., Blum, M. J., Reusser, D. A., Lee, H., and Darling, J. A. (2013). Geographic range and structure of cryptic genetic diversity among Pacific North American populations of the non-native amphipod Grandidierella japonica. Biol. Invasions 15, 2415–2428. doi: 10.1007/s10530-013-0462-7
Pilgrim, E. M., and Darling, J. A. (2010). Genetic diversity in two introduced biofouling amphipods (Ampithoe valida & Jassa marmorata) along the Pacific North American coast: investigation into molecular identification and cryptic diversity. Divers. Distribut. 16, 827–839. doi: 10.1111/j.1472-4642.2010.00681.x
Pineda, M. C., López-Legentil, S., and Turon, X. (2011). The whereabouts of an ancient wanderer: global phylogeography of the solitary ascidian Styela plicata. PLoS ONE 6:e25495. doi: 10.1371/journal.pone.0025495
Pociecha, A., Solarz, W., Najberek, K., and Wilk-Wozniak, K. (2016). Native, alien, cosmopolitan, or cryptogenic? A framework for clarifying the origin status of rotifers. Aquat. Biol. 24, 141–149. doi: 10.3354/ab00644
Provan, J., Murphy, S., and Maggs, C. (2005). Tracking the invasive history of the green alga Codium fragile ssp tomentosoides. Mol. Ecol. 14, 189–194. doi: 10.1111/j.1365-294X.2004.02384.x
Rapoport, E. H., and Marino, C. R. (1998). “Patterns of commerce and the dispersal of weeds,” in Ecology Today: An Anthology of Contemporary Ecological Research, eds B. Gopal, P. S. Pathak, and K. G. Saxena (New Delhi: International Scientific Publications), 163–176.
Rees, D. J., Byrkjedal, I., and Sutton, T. T. (2017). Pruning the pearlsides: reconciling morphology and molecules in mesopelagic fishes (Maurolicus: Sternoptychidae). Deep Sea Res. II 137, 246–257. doi: 10.1016/j.dsr2.2016.04.024
Rick, T. C., Kirch, P. V., Erlandson, J. M., and Fitzpatrick, S. M. (2013). Archeology, deep history, and the human transformation of island ecosystems. Anthropocene 4, 33–45. doi: 10.1016/j.ancene.2013.08.002
Rilov, G., and Galil, B. (2009). Marine bioinvasions in the Mediterranean Sea—history, distribution, and ecology,” in eds G. Rilov and J. A. Crooks, Biological Invasions in Marine Ecosystems. (Berlin: Springer-Verlag), 549–575.
Rius, M., and Teske, P. R. (2011). A revision of the Pyura stolonifera species complex (Tunicata, Ascidiacea), with a description of a new species from Australia. Zootaxa 2754, 27–40.
Rius, M., and Teske, P. R. (2013). Cryptic diversity in coastal Australasia: a morphological and mitonuclear genetic analysis of habitat-forming sibling species. Zool. J. Linn. Soc. 168, 597–611. doi: 10.1111/zoj.12036
Rius, M., Turon, X., Bernardi, G., Volckaert, F., and Viard, F. (2015). Marine invasion genetics: from spatio-temporal patterns to evolutionary outcomes. Biol. Invasions 17, 869–885. doi: 10.1007/s10530-014-0792-0
Ros, M., Ashton, G. V., Lacerda, M. B., Carlton, J. T., Vázquez-Luis, M., Guerra-García, J. M., et al. (2014). The Panama Canal and the transoceanic dispersal of marine invertebrates: evaluation of the introduced amphipod Paracaprella pusilla Mayer, 1890 in the Pacific Ocean. Mar. Environ. Res. 99, 204–211. doi: 10.1016/j.marenvres.2014.07.001
Roy, M. S., and Sponer, R. (2002). Evidence of a human-mediated invasion of the tropical western Atlantic by the 'world's most common brittlestar'. Proc. R. Soc. Lond. Ser. B Biol Sci. 269, 1017–1023. doi: 10.1098/rspb.2002.1977
Ruiz, G. M., Fofonoff, P. W., Carlton, J. T., Wonham, M. J., and Hines, A. H. (2000). Invasion of coastal marine communities in North America: apparent patterns, processes, and biases. Annu. Rev. Ecol. Syst. 31, 481–531. doi: 10.1146/annurev.ecolsys.31.1.481
Ruiz, G. M., Fofonoff, P. W., Steves, B., Foss, S. F., and Shiba, S. N. (2011). Marine invasion history and vector analysis of California: a hotspot for Western North America. Divers. Distribut. 17, 362–373. doi: 10.1111/j.1472-4642.2011.00742.x
Ruiz, G. M., Fofonoff, P. W., Steves, B. P., and Carlton, J. T. (2015). Invasion history and vector dynamics in coastal marine ecosystems: a North American perspective. Aquat. Ecosyst. Health Manage. 18, 299–311. doi: 10.1080/14634988.2015.1027534
Sato-Okoshi, W., Abe, H., Nishitani, G., and Simon, C. A. (2016). And then there was one: Polydora uncinata and Polydora hoplura (Annelida: Spionidae), the problematic polydorid pest species represent a single species. J. Mar. Biol. Assoc. U. K. 97, 1675–1684. doi: 10.1017/S002531541600093X
Savoie, A. M., and Saunders, G. W. (2015). Evidence for the introduction of the Asian red alga Neosiphonia japonica and its introgression with Neosiphonia harveyi (Ceramiales, Rhodophyta) in the Northwest Atlantic. Mol. Ecol. 24, 5927–5937. doi: 10.1111/mec.13429
Schiffer, P. H., and Herbig, H.-G. (2016). Endorsing Darwin: Global biogeography of the epipelagic goose barnacles Lepas spp. (Cirripedia, Lepadomorpha) proves cryptic speciation. Zool. J. Linnean Soc. 177, 507–525. doi: 10.1111/zoj.12373
Schmidt, H., and Westheide, W. (2000). Are the meiofaunal polychaetes Hesionides arenaria and Stygocapitella subterranea true cosmopolitan species? Resuls of RAPD-PCR investigations. Zool. Scripta 29, 17–27. doi: 10.1046/j.1463-6409.2000.00026.x
Schwaninger, H. R. (2008). Global mitochondrial DNA phylogeography and biogeographic history of the antitropically and longitudinally disjunct marine bryozoan Membranipora membranacea L. (Cheilostomata): Another cryptic marine sibling species complex? Mol. Phylogenet. Evolut. 49, 893–908. doi: 10.1016/j.ympev.2008.08.016
Seixas, V. C., Zanol, J., Magalhães, W. F., and Paiva, P. C. (2017). Genetic diversity of Timarete punctata (Annelida: Cirratulidae): Detection of pseudo-cryptic species and a potential biological invader. Estuar. Coast. Shelf Sci. 197, 214–220. doi: 10.1016/j.ecss.2017.08.039
Sheets, E. A., Cohen, C. S., Ruiz, G. M., and Da Rocha, R. M. (2016). Investigating the widespread introduction of a tropical marine fouling species. Ecol. Evol. 6, 2453–2471. doi: 10.1002/ece3.2065
Sherwood, A. R. (2007). “Where are we now regarding Hawaiian stream algal systematics? (A suspiciously cosmopolitan flora),” in Biology of Hawaiian Streams and Estuaries eds N. L. Evenhuis and J. M. Fitzsimons (Honolulu, HI: Bishop Museum), 195–206.
Sherwood, A. R. (2008). Phylogeography of Asparagopsis taxiformis (Bonnemaisoniales, Rhodophyta) in the Hawaiian Islands: two mtdna markers support three separate introductions. Phycologia 47, 79–88. doi: 10.2216/07-39.1
Sheth, B. P., and Thaker, V. S. (2017). DNA barcoding and traditional taxonomy: An integrated approach for biodiversity conservation. Genome 60, 618–628. doi: 10.1139/gen-2015-0167
Sliwa, C., Migus, S., McEnnulty, F., and Hayes, K. R. (2009). “Marine bioinvasions in Australia,” in Biological Invasions in Marine Ecosystems, G. Rilov and J. A. Crooks (Berlin: Springer-Verlag), 425–437.
Spalding, M. D., Fox, H. E., Allen, G. R., Davidson, N., Ferdana, Z. A., Finlayson, M., et al. (2007). Marine ecoregions of the world: a bioregionalization of coastal and shelf areas. Bioscience 57, 573–583. doi: 10.1641/B570707
Stefaniak, L., Lambert, G., Gittenberger, A., Zhang, H., Lin, S., and Whitlatch, R. B. (2009). Genetic conspecificity of the worldwide populations of Didemnum vexillum Kott, 2002. Aquatic Invasions 4, 29–44. doi: 10.3391/ai.2009.4.1.3
Steneck, R., and Carlton, J. T. (2001). “Human alterations of marine communities: students beware!,” in Marine Community Ecology, eds M. D. Bertness, S. D. Gaines, and M. E. Hay (Sunderland: Sinauer Associates, Inc.), 445–468.
Styan, C., Mccluskey, C., Sun, Y., and Kupriyanova, E. (2017). Cryptic sympatric species across the Australian range of the global estuarine invader Ficopomatus enigmaticus (Fauvel, 1923) (Serpulidae, Annelida). Aquatic Invasions 12, 53–65. doi: 10.3391/ai.2017.12.1.06
Sun, Y., Wong, E., Keppel, E., Williamson, J. E., and Kupriyanova, E. K. (2017). A global invader or a complex of regionally distributed species? Clarifying the status of an invasive calcareous tubeworm Hydroides dianthus (Verrill, 1873) (Polychaeta: Serpulidae) using DNA barcoding. Mar. Biol. 164:28. doi: 10.1007/s00227-016-3058-9
Sutherland, J. P. (1974). Multiple stable points in natural communities. Am. Natur. 108, 859–873. doi: 10.1086/282961
Suzuki, M. M., Nishikawa, T., and Bird, A. (2005). Genomic approaches reveal unexpected genetic divergence within Ciona intestinalis. J. Mol. Evol. 61, 627–635. doi: 10.1007/s00239-005-0009-3
Teske, P. R., Rius, M., Mcquaid, C. D., Styan, C. A., Piggott, M. P., Benhissoune, S., et al. (2011). “Nested” cryptic diversity in a widespread marine ecosystem engineer: a challenge for detecting biological invasions. BMC Evol. Biol. 11, 176. doi: 10.1186/1471-2148-11-176
Todaro, M. A., Fleeger, J. W., Hu, Y. P., Hrincevich, A. W., and Foltz, D. W. (1996). Are meiofaunal species cosmopolitan? Morphological and molecular analysis of Xenotrichula intermedia (Gastrotricha: Chaetonotida). Mar. Biol. 125, 735–742. doi: 10.1007/BF00349256
Tomioka, S., Kondoh, T., Sato-Okoshi, W., Ito, K., Kakui, K., and Kajihara, H. (2016). Cosmopolitan or cryptic Species? A case study of Capitella teleta (Annelida: Capitellidae). Zool. Sci. 33, 545–554. doi: 10.2108/zs160059
Trickey, J. S., Thiel, M., and Waters, J. M. (2016). Transoceanic dispersal and cryptic diversity in a cosmopolitan rafting nudibranch. Invertebr. Syst. 30, 290–301. doi: 10.1071/IS15052
Turnbull, C. M. (2009). A History of Modern Singapore 1819-2005. Singapore: National University of Singapore Press.
Ulman, A., Ferrario, J., Occhpinti-Ambrogi, A., Arvanitidis, C., Bandi, A., Bertolino, M., Marchini, A., et al. (2017). A massive update of non-indigenos species records in Mediterranean marinas. PeerJ. 5:e3954. doi: 10.7717/peerj.3954
Uwai, S., Nelson, W., Neill, K., Wang, W. D., Aguilar-Rosas, L. E., Boo, S. M., et al. (2006). Genetic diversity in Undaria pinnatifida (Laminariales, Phaeophyceae) deduced from mitochondria genes – origins and succession of introduced populations. Phycologia 45, 687–695. doi: 10.2216/05-66.1
Van Steenkiste, N. W. L., Herbert, E. R., and Leander, B. S. (2018). Species diversity in the marine microturbellarian Astrotorhynchus bifidus sensu lato (Platyhelminthes: Rhabdocoela) from the Northeast Pacific Ocean. Mol. Phylogenet. Evol. 120, 259–273. doi: 10.1016/j.ympev.2017.12.012
Vandepas, L. E., Oliveira, L. M., Lee, S. S., Hirose, E., Rocha, R. M., and Swalla, B. J. (2015). Biogeography of Phallusia nigra: is it really black and white? Biol. Bull. 228, 52–64. doi: 10.1086/BBLv228n1p52
Viard, F., David, P., and Darling, J. A. (2016). Marine invasions enter the genomic era: three lessons from the past, and the way forward. Curr. Zool. 62, 629–642. doi: 10.1093/cz/zow053
Villasenor-Parada, C., Pauchard, A., and Macaya, E. C. (2017). Ecologia de invasiones marinas en Chile coninental: que sabemos y que nos falta por saber? Rev. Biol. Mar. Oceanogr. 52, 1–17. doi: 10.4067/S0718-19572017000100001
Voisin, M., Engel, C. R., and Viard, F. (2005). Differential shuffling of native genetic diversity across introduced regions in a brown alga: aquaculture vs. maritime traffic effects. Proc. Natl. Acad. Sci. 102, 5432–5437. doi: 10.1073/pnas.0501754102
Wang, D. (1339). Daoyi Zhilüe (岛夷志略; A brief account of island barbarians), Zhonghua Book Company. ISBN 7-101-02026-7 (in Chinese; not seen).
Wolff, W. J. (2005). Non-indigenous marine and estuarine species in the Netherlands. Zool. Mededelingen 79, 1–116.
Woodman, R. (1997). The History of the Ship: The Comprehensive Story of Seafaring From the Earliest Times to the Present Day. London: Conway Maritime Press.
Woods Hole Oceanographic Institution (WHOI) (1952). Marine Fouling and its Prevention. Annapolis: United States Naval Institute.
Wrange, A. L., Charrier, G., Thonig, A., Alm Rosenblad, M., Blomberg, A., Havenhand, J. N., et al. (2016). The story of a hitchhiker: population genetic patterns in the invasive barnacle Balanus (Amphibalanus) improvisus Darwin 1854. PLoS ONE 11:e0147082. doi: 10.1371/journal.pone.0147082
Wyatt, A. S. J., Hewitt, C. L., Walker, D. I., and Ward, T. J. (2005). marine introductions in the shark bay world heritage property, western australia: a preliminary assessment. Divers. Distribut. 11, 33–44. doi: 10.1111/j.1366-9516.2005.00109.x
Xavier, J. R., Rachello-Dolmen, P. G., Parra-Velandia, F., Schönberg, C. H., Breeuwer, J. A., and Van Soest, R. W. (2010). Molecular evidence of cryptic speciation in the “cosmopolitan” excavating sponge Cliona celata (Porifera, Clionaidae). Mol. Phylogenet. Evol. 56, 13–20. doi: 10.1016/j.ympev.2010.03.030
Xiong, W., Shen, C., Wu, Z., Lu, H., and Yan, Y. (2017). A brief overview of known introductions of non-native marine and coastal species into China. Aquat. Invas. 12, 109–115. doi: 10.3391/ai.2017.12.1.11
Yund, P. O., Collins, C., and Johnson, S. L. (2015). Evidence of a native northwest Altantic COI haplotype clade in the cryptogenic colonial ascidian Botryllus schlosseri. Biol. Bull. 228, 201–216. doi: 10.1086/BBLv228n3p201
Keywords: introduced species, marine biogeography, cosmopolitanism, eucosmopolitan, pseudocosmopolitan, neocosmopolitan, provincial, invasion biology
Citation: Darling JA and Carlton JT (2018) A Framework for Understanding Marine Cosmopolitanism in the Anthropocene. Front. Mar. Sci. 5:293. doi: 10.3389/fmars.2018.00293
Received: 23 May 2018; Accepted: 30 July 2018;
Published: 22 August 2018.
Edited by:
Stelios Katsanevakis, University of the Aegean, GreeceReviewed by:
Anja Schulze, Texas A&M University at Galveston, United StatesHanno Seebens, Senckenberg Biodiversität und Klima Forschungszentrum (SBiK-F), Germany
Rosana Moreira Rocha, Universidade Federal do Paraná, Brazil
Copyright © 2018 Darling and Carlton. This is an open-access article distributed under the terms of the Creative Commons Attribution License (CC BY). The use, distribution or reproduction in other forums is permitted, provided the original author(s) and the copyright owner(s) are credited and that the original publication in this journal is cited, in accordance with accepted academic practice. No use, distribution or reproduction is permitted which does not comply with these terms.
*Correspondence: John A. Darling, ZGFybGluZy5qb2huQGVwYS5nb3Y=