- Biosciences, Swansea University, Swansea, United Kingdom
Enhancing habitat complexity and thereby biodiversity is a main motivation for the creation of artificial reefs in the marine and coastal environment. Uncertainty remains, however, regarding which types of reef best deliver this aim, and how material properties impact faunal communities. The objective of this study was to assess the macrobenthic infauna in standardized reef-units made from different types of shell and rock and to quantify factors explaining community properties. 70 × 75 × 25 cm reef-units were made from cockle, mussel and oyster shells and rocks. Replicate units were placed on an intertidal sand flat of Swansea Bay (Wales, UK). After 5 months the benthic fauna was washed out of the reef-units and identified to species level. The volume of reef material, interstitial space and trapped sediment in each unit was quantified. A total of 45 invertebrate species were recorded in artificial reef-units compared with 12 species in the reef-free surrounding sands; 37 species were exclusively found in reefs. There was no significant difference between the infauna communities in different reef types in terms of univariate or multivariate diversity descriptors, but multivariate dispersion was lower among rock than shell-reef replicates. Distance-based linear models (DistLM) showed that the volume of interstitial space per reef-unit was the factor best explaining community structure, followed by properties of the trapped sediment. Species richness was significantly correlated with the volume of interstitial space and trapped sediment. Species seemed to use the reef-units fleetingly as shelter during low water, more permanently for protection, or as hunting ground for prey. The study demonstrated that artificial reef-units made of loose shell material and rocks can significantly enhance infauna diversity in sandy coastal environments. The identity of the material seems less relevant as long as it maximizes interstitial space and allows trapping of sediment. This provides practitioners with a degree of creative freedom when designing artificial reefs with the aim to enhance infauna diversity.
Introduction
Artificial reefs are man-made discrete areas of firm material arising from the surrounding seafloor. OSPAR defines them as “a submerged structure deliberately placed on the seafloor to mimic some functions of a natural reef such as protection, regeneration, concentration and/or enhancing population of living marine resources” (OSPAR CCOMMISSION., 1999). The motivation for designing and constructing reefs include fisheries protection and production, habitat protection and restoration, research and recreation (OSPAR COMMISSION, 2009). In Europe over 200 artificial reefs have been deployed over the past 40 years (Fabi et al., 2011). Projects range from small nature conservation initiatives to major programs developing regional fisheries or protecting large areas from trawling (Jensen, 2002).
Artificial reefs are either designed to enhance habitat complexity and provide space for a generally more diverse epifauna, or they are built to attract specific species (Baine, 2001). They may protect or enhance fish stocks, restore marine habitats or create sites for recreational diving and fishing (Jensen, 2002). Most artificial reefs are inadvertent reefs; they are coastal and marine infrastructure such as coastal defense measures, moorings, breakwaters or increasingly foundations of off-shore wind turbines (Langhamer, 2012; Lawless and Seitz, 2014). Off-shore wind turbines and other marine renewable energy devices are generally constructed on soft bottom substratum, and the submerged parts of the infrastructure, turbine foundation as well as scour protection can potentially turn exposed, biodiversity-poor areas into species rich ecosystems (Inger et al., 2009; Broadhurst and Orme, 2014).
One function of artificial reefs is to provide attachment surfaces for sessile species, and they may offer shelter from wave and current exposure, or from predators. The magnitude and nature of the impact of artificial reefs is influenced by their location, how well they are connected with other reefs, their height, size and complexity and the surrounding habitat (Lenihan, 1999; Grabowski, 2004; Grabowski et al., 2008). Structural complexity of a reef, besides other factors such as reef diversity, is linked with its impact on the abundance of fish and invertebrates as well as species diversity (Hunter and Sayer, 2009). Further, the reef community is determined by the presence of fouling species, in particular the amount of meroplankton in surrounding waters and the bioengineering potential of the fouling species (Ambrose and Swarbrick, 1989; Moffitt et al., 1989; Einbeinder et al., 2006). They can increase or decrease benthic invertebrates or have little effect (Langois et al., 2006). An assessment of European reefs indicated that only 50% of case studies met their objectives while the remainders had little or no effect (Baine, 2001).
Artificial reefs can be created from a plethora of different materials, such as concrete modules, natural rocks and boulders, tires, pulverized fuel ash (PFA), PVC, wood, trees, rope, netting or stabilized quarry dust slurry (Baine, 2001; Jensen, 2002; Fabi et al., 2011; La Peyre et al., 2014). Gravel and boulders may be used to protect offshore energy infrastructure from scour (Langhamer, 2012). OSPAR guidelines advocate the use of inert materials (non-polluting through leaching, physical or chemical weathering and/or biological activity), and they should not be made of wastes (Baine, 2001).
In shallow coastal areas bivalve shells from mussels or oysters are increasingly used as reef material (Fabi et al., 2011). Bivalve reefs such as mussel or oyster beds diversify the habitat, particularly in sedimentary sub- and intertidal areas, and they thereby engineer the resident benthic communities (Jones et al., 1994). Such features are recognized globally as providing ecosystem services disproportionate to their size (Lundquist et al., 2017). Artificial shell reefs mimic the structural element of natural biogenic reefs; they lack functional services such as water filtration. Oyster shells are ubiquitously used to enhance natural oyster populations for harvesting, the stabilization of intertidal sandflats or wave attenuation and coastal defense (Levine et.al., 2017). Bivalve reefs are part of an entire portfolio of coastal eco-engineering concepts for flood defenses (van Loon-Steensma et al., 2014). These are particularly applied at locations that have sufficient space between urbanized areas and the coastline to accommodate the creation of ecosystems, such as shellfish reefs, tidal marshes or mangroves, which have the natural capacity to reduce storm waves and storm surges and can keep up with sea-level rise by natural accretion of mineral and biogenic sediments (Temmerman et al., 2013). Like other bio-engineering species, bivalve reefs form complex structures that provide spaces for feeding and nesting, and they are a refuge from predation and environmental exposure (Bartol et al., 1999; Bartholomew et al., 2000; Gutiérrez et al., 2003; Coen et al., 2007).
Studies on the impact of artificial reefs focus on epifauna and fish communities and infauna is often ignored (Fabi et al., 2011). Many designed reef structures as well as moorings or breakwaters may indeed not provide habitat suitable for small invertebrates which require narrow internal spaces. However, it is possible to consider artificial reef designs that offer an open structural matrix with interstitial space to be exploited by invertebrates. The impact of different reef material on infauna can be difficult to compare due to the variable structures of the reefs they create and challenges for standardized sampling (Baine, 2001).
In this study a field experiment was designed with standardized reef-units made from oyster, mussel and cockle shells and from limestone rocks. The basic design was an adaptation of a commercial ecological engineering product called rock-roll, which is primarily used for river bank stabilization (SALIX, 2018). Artificial shell-reefs resemble natural biogenic reefs such as mussel and oyster beds, and they have potentially similar functions for associated fauna (Largaespada et al., 2012). It was hypothesized that bivalve shells may have a greater benefit for biodiversity than aggregations of limestone rocks. Further, using bivalve shells for artificial reef structures would provide shellfish processors and coastal managers with an opportunity to return shell by-products from the fishing industry to their natural environment.
The objectives of this study were to:
1) Determine the difference between artificial reef-units and sandy intertidal surroundings in terms of macrobenthic species richness and abundance.
2) Compare diversity properties and community structure of the associated benthic fauna among oyster, cockle and mussel shells and limestone rocks.
3) Quantify the degree to which habitat properties of reef materials (trapped sediment, interstitial space) explain variation in the associated benthic community.
Materials and Methods
Effects of shell and stone-filled artificial reef-units on benthic fauna were tested in intertidal sandflat areas of Swansea Bay, Wales, UK. Swansea Bay is a shallow embayment on the northern coastline of the Bristol Channel exposed to severe hydrodynamic forces due to strong winds and tides (Callaway, 2016). Reef-units were constructed from polypropylene mesh-tubes with a mesh size of 2.5 × 2.5 cm; the material is generally used in landscape engineering. Mesh-bags were filled with four types of material: Shells of the bivalves Cerastoderma edule (cockles), Mytilus edulis (mussels), Ostrea edulis (oysters) and limestone rock of about 4 cm diameter (Figure 1). Cockle and mussel shells were supplied by a local shellfish processor who stores the shells as a waste product, and the oyster shells were collected from Swansea Bay. All shells were dried for 2 months on land to sterilize them from attached fauna, and they were then filled into the prepared mesh bags to form reef-units. Individual reef-units were 70 cm long and had a diameter of 25 cm, therefore covering an area of 1,750 cm2. Rock-filled units were created and supplied by the landscape engineering company SALIX (UK).
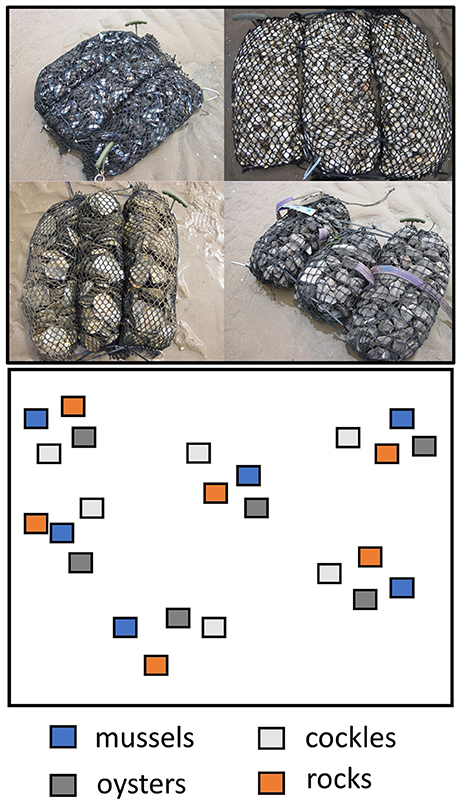
Figure 1. Schematic design of the field experiment with different types of reef-units arranged in blocks, and images of the experimental reefs made of oyster, cockle and mussel shells and limestone rocks.
Field Experiment
In June 2014 the reef-units were placed on an intertidal sandflat in Swansea Bay. In the field three units filled with the same material were lined up side by side and stitched together to cover an area of about 75 × 70cm2. Such a triple-unit would constitute one reef. The reefs were arranged in blocks of four: one of each reef type (oyster, cockle and mussel shells and stones) was placed at a distance of about 20 m to each other so that there was no interaction between them. Altogether six replicate block sets, each composed of the four reef types, were established (Figure 1). Sets were located about 50 m apart along the lower intertidal area of the sandflat. No other natural or artificial reef was in close proximity to avoid interaction with the experimental units. Reef-units were fixed in the sand with specialist pegs to avoid their movement or dislocation. However, in the proceeding months two replicates with cockle shells and one unit filled with stones were lost, most likely washed away by currents.
The experimental reefs were left in the field for 5 months. In November 2014 the reef units were collected for laboratory processing. Additionally, sediment core samples were taken from the intertidal sandflat as reference samples. Altogether six core samples were taken in the vicinity of each of the six replicate sets of reef-units; samples covered 300 cm2, 10 cm deep.
Laboratory Processing
One unit of each reef replicate covering a 25 × 70 cm surface area (1,750 cm2) was processed for benthos and sediments analysis; of the three units stitched together the sampled unit was always from the outside and never the middle unit. In the laboratory, each mesh-bag was submerged in a seawater filled container and rinsed thoroughly to wash out all sediment and benthic invertebrates. The sediment was then left to settle in the container for approximately 10 min, and subsequently the sample was treated similarly to benthos grab samples. The water was decanted carefully to avoid re-suspension of sediment and to lose as little very fine sediment as possible. It was poured through a 1 mm sieve to retain any suspended benthic fauna. The volume of the sediment was then determined, and a sediment sample (200 g) was taken for grain size analysis. A sample for grain size analysis was also removed from the reference sediment core samples. The remaining sediment of each sample was washed through a 1 mm sieve to retain macroinfauna; in November few juveniles were present in the benthos and most macrofauna had reached a size >1 mm. The sieve residue was fixed in 4% buffered formalin and subsequently preserved in 70% ethanol for benthic infauna analysis. Each rinsed, sediment-free artificial reef-unit mesh bag was then opened and emptied into a container and the volume of the shells or stones was determined. In order to quantify the volume of interstitial space (the space between the reef-unit material), the container was then filled with water until it just covered the shells or stones. The water was drained into a separate container and the total volume of the water was measured, representing the volume of interstitial space.
Shells and stones were checked for attached epibenthos. The infauna was separated from the preserved sieve residue and identified to species level. Sediment samples were air dried and passed through a series of sieves from 2 mm to 63 μm according to the Wentworth-Udden classification scale to determine particle-size.
Data Analysis
Differences between abiotic properties of reef units and reference sediment core samples as well as differences between reef materials were tested with ANOVA, followed by Tukey's pairwise comparison (volume of material per reef unit, trapped sediment, interstitial space). Differences in the structure of the benthic community in the different reef-units and the reference samples were explored by multi-dimensional-scaling (MDS) (PRIMER v6 software) based on a resemblance matrix of Bray-Curtis similarities. To down-weight abundant species and increase the importance of less-abundant ones the data was square-root transformed. Statistical differences in community structure between the different reef types and between blocks (Figure 1) were tested with PERMANOVA. In order to test for variation within and between reef types, homogeneity of multivariate dispersion was tested with PERMDISP (PRIMER v6 software). The degree to which different environmental factors explained the variation in the benthic community of reef-units was explored by constructing Distance-based linear models (DistLM in PERMANOVA). These were based on Bray-Curtis similarities of square-root transformed abundance data. R2 was calculated for each explanatory factor, and the best overall model was selected according to the Akaike Information Criterion (AIC). Results of the DistLM were visualized by distance-based redundancy analysis (dbRDA). A vector overlay was added to the ordination diagram of the dbRDA, with one vector for each predictor variable. Univariate diversity indices were calculated for each sample (species richness S, abundance N, evenness J, Shannon diversity H'), and their link with environmental factors was also tested by DistLM. Differences between diversity indices were tested with t-test (reef units vs reference core samples) and ANOVA (differences between reef materials), except for abundances where data were not normally distributed. Differences between abundances were therefore tested with the equivalent non-parametric tests (Mann-Whitney U test and Kruskal-Wallis). The sediment parameters mean grain size (x), sorting (σ), skewness (Sk), and kurtosis (K) were calculated with GRADISTAT (Blott and Pye, 2001).
Results
Differences Between Artificial Reef-Units and Reference Sediment Core Samples
Reef units and reference sediment core samples contained similar amounts of sediment; reef units trapped 3.6 ± 1.9 l of sediment (n = 21) and core samples contained 3.5 ± 0.5 l of sediment (n = 6) (ANOVA between all reef materials and sediment core samples p = 0.62).
A total of 45 invertebrate species were recorded in artificial reef-units compared with 12 species in the sediment core samples (Figure 2, Supplementary Table 1); 37 species were exclusively found in reefs, 4 exclusively in reference sediment cores. Of the 45 species in reef units, 32 were mobile, 5 sessile depending on hard substratum and 8 sessile depending on sediment. In sediment core samples 9 of the 12 species were mobile and 3 sessile. Most of the invertebrates were polychaetes (20 in reefs, 6 in sediment cores), followed by crustaceans (13 in reefs, 3 in sediment cores) and bivalves (8 in reefs, 2 in sediment cores).
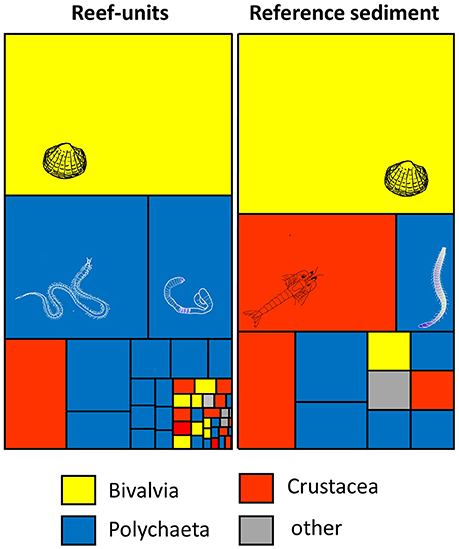
Figure 2. Tree-map illustrating the number of invertebrate species in reef-units and reef-free sediment core samples. The size of tiles reflects the relative abundance of individual species (mean m−2), the colour the taxonomic group; full species list in S1.
Average numbers of species were significantly higher in reef-units (8.1 ± 3.2, mean ± sd, n = 21) compared with sediment cores (4.3 ± 1.5, mean ± sd, n = 6) (t-test, p < 0.01). However, this comparison ought to be viewed with caution: despite the similarity in the amount of sediment in reef-units and reference sediment core samples it needs to be kept in mind that the area covered by reef-units was larger (1,750 cm2) than that of the reference samples (300 cm2). Numbers of individuals per unit area were not statistically significantly different: 317 ± 253 m−2 in reef-units (n = 21) compared with 294 ± 159 m−2 (n = 6) in sediment core samples (Mann-Whitney test p = 0.88).
The most common species in sediment core samples as well as reef units were juvenile < 5 mm Cerastoderma edule (sediment cores 128 ± 85 m2, reefs 123 ± 133 m2). In the experimental reef units, the scavenging polychaete Phyllodoce maculata was the second most common species (67 ± 77 m2), but it was absent from sediment cores. The amphipods Melita palmata, Echinogammarus stoerensis, and Gammarus zaddachi were exclusively recorded in reef units, while the mysid Praunus inermis and the sediment dwelling amphipod Urothoe brevicornis were predominantly found in the reef-free sediment cores. Nephtys spp. were common in reference and reef samples (sediment cores 50 ± 51 m2, reefs 13 ± 11 m2; ns difference Mann Whitney test p = 0.21).
Differences Among Reef Materials
Due to the manufacturing process rock filled reef-units contained significantly more material than the shell reefs (ANOVA p < 0.001, Figure 3); there was no significant difference between the volume of material in shell filled reef units (Tukey's multiple comparison test). Despite almost twice as much material in rock-units than in shell-reefs there was no significant difference between the amount of sediment trapped by the four different types of reefs (ANOVA, p = 0.56).
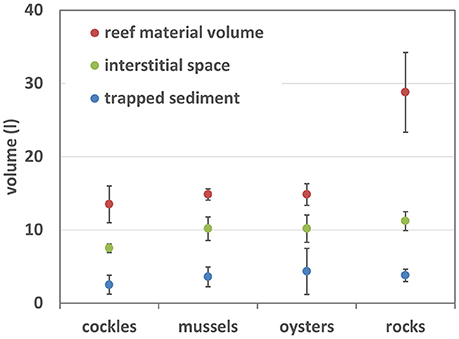
Figure 3. Abiotic properties of experimental reef-units: volume of shell and rock material within each sample unit, interstitial space within the units and amount of trapped sediment.
The amount of interstitial space was significantly larger in rock-filled units than in cockle shell reefs (ANOVA, p = 0.013), but there was no difference between other materials (Figure 3). However, the ratio “interstitial space/volume of material” was significantly smaller for rocks than for shell-reefs (rocks 0.40 ± 0.06, cockles 0.57 ± 0.09, mussels 0.68 ± 0.08, oysters 0.68 ± 0.11), meaning that bivalve shells provided significantly more interstitial space per unit volume than rocks (ANOVA p < 0.0001; Tukey's test < 0.05 between rocks and all shell types).
Species richness ranged from 6.5 ± 3.0 in cockle reefs to 10.2 ± 2.0 in rock reef units and was not statistically significantly different (ANOVA p = 0.35). Similarly, abundances per reef unit were not significantly different between the four materials (Kruskal-Wallis p = 0.09).
The infauna community composition between the four different reef materials was not significantly different (PERMANOVA between benthic communities in oyster, cockle and mussel shells and rock reef, Bray-Curtis similarities, √-transformation of abundances, p = 0.22); there was also no significant block effect (p = 0.1). The MDS ordination (Figure 4) visualizes that the communities in the different reef materials did not form discrete clusters, and the same species colonized all reef materials (Figure 5). However, PERMDISP analysis showed significant differences in homogeneity of multivariate dispersion among the four reef types, which are visualized in the MDS ordination (F 23.91, p = 0.0004). Dispersion was lowest among rock-reef replicates and largest among mussel and cockle shell-reefs (average deviation from centroid, mussels 49.3, cockles 46.7, oysters 35.0, rocks 21.9). Pairwise comparisons indicated significant differences (p < 0.05) between rock-reefs and the three types of shell-reef.
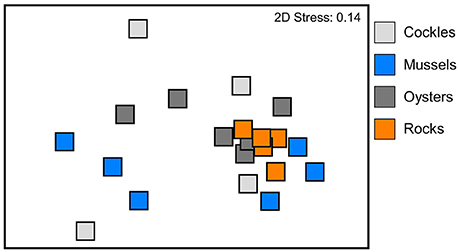
Figure 4. Non-metric multi-dimensional scaling (MDS) of benthic communities within experimental reef units consisting of cockle, mussel and oyster shells as well as rocks. Ordination based on Bray Curtis similarities of square-root transformed abundances of macrobenthic species per reef unit.
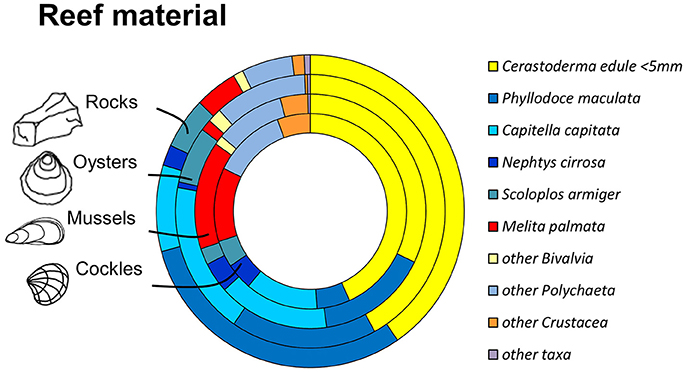
Figure 5. Most abundant species and taxonomic groups in experimental reef-units. From outside to inside: rock-reefs (n = 5), oysters (n = 6), mussels (n = 6), cockles (n = 4). Abundances for materials based on mean densities per reef unit.
Abiotic Factors Driving Artificial Reef Communities
Distance-based linear models (DistLM) were constructed to quantify the degree to which different abiotic factors explained the variation in the benthic communities of reef-units. The explanatory factors entered into the model were (1) volume of interstitial space, (2) volume of reef material (shells or rocks), (3) volume of trapped sediment, (4) mean grain size of sediment, (5) sorting of sediment, (6) sorting skewness of sediment, (7) sorting kurtosis.
The volume of interstitial space provided by a reef unit explained more of the variation in the benthic community than the other factors (Table 1). The two factors “interstitial space” and “skewness of sediment sorting” had the lowest AIC, therefore providing the overall best model solution (Figure 6). Since C. edule happened to be the most common species in the reef units but is generally an unlikely reef dweller, DistLMs were additionally constructed which excluded cockles. The purpose was to establish if the presence of C. edule had a profound impact on the model. Again, interstitial space explained more of the variation in the benthic community (cockles excluded) than other factors; interstitial space was the only factor individually explaining significant amounts of variation in the data (R2 = 0.10, p = 0.041). The lowest AIC was provided by interstitial space and sediment sorting. All factors combined explained 47% of the variation.
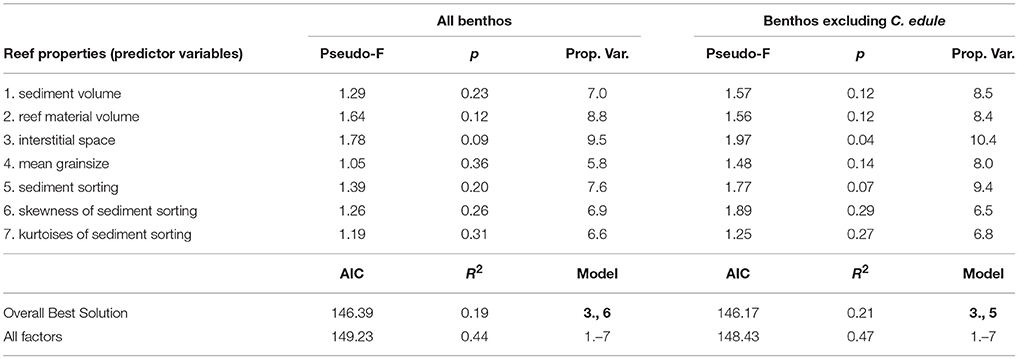
Table 1. Distance based linear model (DistLM) outputs for relationship between reef properties and benthic infauna in experimental reef units.
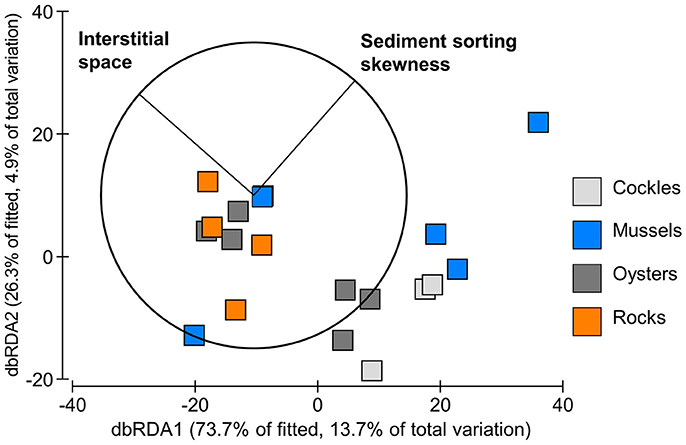
Figure 6. dbRDA ordination of the fitted DistLM model for benthic fauna in experimental reef units filled with shell and rock material (based on Bray Curtis similarities after square-root transformed abundances). Reef characteristics best explaining the variation in the community data are superimposed.
Species richness was significantly correlated with the volume of interstitial space as well as the volume of sediment trapped in the reef units (Figure 7). Shannon diversity H' was also significantly correlated with volume of trapped sediment (r = 0.54, R2 = 0.29, p = 0.015, n = 20), but there were no other significant relationships between univariate diversity indices and abiotic factors.
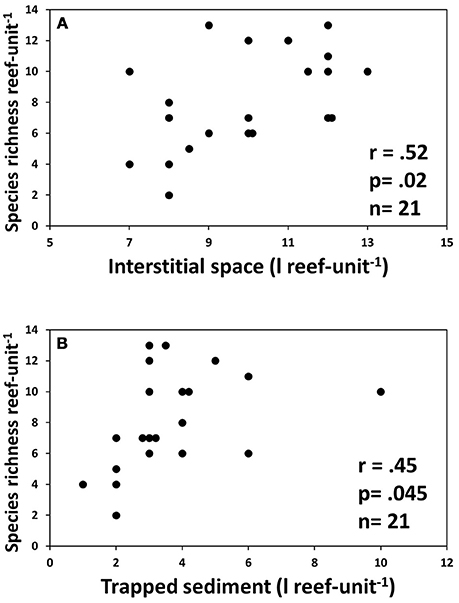
Figure 7. Correlation between abiotic reef properties and species richness. (A) Volume of interstitial space within each reef unit; (B) Amount of sediment trapped by reef units.
Discussion
This field experiment demonstrated that artificial reefs made of loose shell material and rocks had a significant impact on the diversity of benthic infauna species in a sandy intertidal environment: the reefs promoted species richness. Generally, complex habitats created by bivalve reefs have the potential to support a suite of species not found in nearby habitats (Coen and Luckenbach, 2000; Tolley and Volety, 2005; Scyphers et al., 2011; Brown et al., 2014). Particularly mobile invertebrates appear to benefit from small-scale habitat complexity of artificial material while sessile species seem to prefer smooth surfaces (Lavender et al., 2017). Whether or not shells or other reef material impact fauna appears to depend on the local assemblage and species-specific responses to the hard substratum (Langhamer, 2012); artificial oyster shell reefs for restoration projects, for example, had different impacts on faunal communities, which appears to be location specific (Schulte et al., 2009; La Peyre et al., 2014; George et al., 2015). Shells do not necessarily enrich the benthic community: in a Brazilian estuary the presence or absence of shells in sediments had no measurable effect on the community structure (Sandrini-Neto and da Cunha Lana, 2014). A review of European artificial reefs indicated a strong interaction between local environmental conditions, the local ecology and the specific reef designs, creating unique systems that may not be reproducible elsewhere (Baine, 2001). Therefore, caution needs to be applied when extrapolating results of this Swansea Bay (Wales, UK) experiment to other locations. Given that the infauna species were typical for intertidal sandflats in North-Western Europe (Hayward and Ryland, 2017), it seems plausible to presume that results would be similar for intertidal sandflats of this geographical region, and broader principles such as the importance of interstitial space may have wider relevance.
Interstitial Space
In this study biodiversity increased with the amount of interstitial space, independent from the reef material, indicating that the space created drove colonization. Differences in the amount of interstitial space were also found for fresh and dredged oyster shells, where material providing more interstitial space was preferentially chosen by crustaceans in mesocosm experiments (Levine et al., 2017). Due to the different ecologies of the infauna species in this field experiment they will have used the space in species-specific ways. Highly mobile crustaceans such as Nototropis swammerdamei or Idotea pelagica are likely to have used the space as a temporary shelter before migrating back into the water column at high tide (Callaway, 2006). The majority of species were mobile polychaetes that may have used the reefs as refuge from predators like larger crabs, or for protection from challenging environmental conditions. Juvenile cockles C. edule were probably passively transported into the reef-units together with mobile sands. Some may have been trapped in the interstitial space, although cockles, particularly juvenile ones, are capable of active movement and migration (Armonies, 1992). It is possible that some pockets of the reef-units offered suitable conditions for the juvenile cockles to survive, meaning enough water circulation to provide oxygen and food.
Some macrobenthic predators may have been attracted to the reefs as hunting ground. The predatory, scavenging Phyllodoce maculata was found in significantly larger number in the reef units compared with the surrounding sandflat. The polychaete is highly mobile and likely to have actively explored the interstitial space of the reef-units for meiofauna, juvenile macrofauna or dead organisms. The species appears to be attracted to biogenic reefs and has also been found in significantly higher abundances among Lanice conchilega aggregations (Callaway, 2006).
It ought to be kept in mind that the positive correlation between interstitial space and species richness cannot simply be extrapolated; if space between material becomes too large it may no longer fit the needs of the invertebrates. Further research into the interaction of benthic invertebrates with gaps between reef material and how the space is utilized could clarify the positive relationship between interstitial space and biodiversity. It was suggested that traits such as interstitial space of habitats created from various materials should be added to the list of issues considered when natural communities are to be restored in oyster reefs and other environments (Levine et al., 2017).
Reef Material
The study showed that different materials can have a similar positive impact on benthic infauna. This was also found in studies of “accidental reefs”: waste material such as wood, metal and tires, which was dumped in an estuary, was colonized at similar rates compared with sandstone reefs (Chapman and Clynick, 2006).
The three bivalve shell types resembled each other in terms of the interstitial space they provided, although oyster shells are considerable bigger than cockle and mussel shells. The surface texture of the shells differs in that oysters are roughly layered, mussels are relatively smooth with only fine groves, and C. edule is strongly ribbed; due to the absorbent properties of cockle shells they are used for removing pollutants from wastewater (Kazemi et al., 2016). Still, the differences in texture and size of shells had no significant effect on the infauna they hosted.
Bivalve shell-reefs showed no greater benefit for biodiversity than limestone rock aggregations. There were, however, significant differences in the multivariate dispersion among the reef types. Rock-reefs showed the lowest dispersion, i.e., the benthic communities in rock-reef replicates were more similar than those of shell-reefs, with the communities in mussel shells-reefs replicates being most different from each other. Homogeneity of multivariate dispersion is an indicator of beta diversity (Anderson et al., 2006) and the results suggest that shell reefs potentially create higher beta diversity. High dispersion levels are, however, also interpreted to reflect stress levels in marine communities (Warwick and Clarke, 1993), and the result may suggest that the shell reefs suffered greater disturbance while rock-reefs offered more constancy. Generally, this result must be viewed with caution since within-group sample size was less than n = 10, which limits the power of the multivariate test.
Further, it needs to be considered that the rock-reefs contained significantly more material than the shell-reefs and had a smaller ratio “interstitial space/volume of material,” or in other words, due to the larger size of rocks compared with bivalve shells, rock-reefs created less interstitial space per unit volume than shells. In efforts to create habitat for benthic macroinvertebrates, rock-reefs would need to be about double the size of bivalve reefs to produce similar volumes of interstitial space.
Impact of Artificial Shell Reefs Compared With Live Biogenic Reefs
Reefs of living bivalves have different properties than clusters of dead shells. Bivalves filter quantities of water and thereby move particles, excrete feces and pseudofeces, which agglomerate sediments, enriching the organic content and affect nutrient flux (Largaespada et al., 2012). Predators of the bivalves such as whelks or starfish would unlikely be attracted to empty shell reefs (Gosnell et al., 2012). Their live bodies have a different shape and volume compared with empty shells, and therefore the space they create is different. However, while both physical and biological properties of mussels mediate the positive effect of mussels on their recruits (positive intraspecific feedback), physical properties alone can explain the interspecific positive engineering effect of mussels on diversity of associated species (Largaespada et al., 2012).
The results of this study therefore also allow, to a limited degree, a comparison between mussel and oyster banks in terms of their respective impact on biodiversity. Comparisons of different natural bivalve reefs and their associated fauna can be challenging as it may not be possible to standardize sampling methods for different types of reef, or because mussels and oysters occur interlaced (Drent and Dekker, 2013). However, evidence from the Dutch Wadden Sea suggests that oyster and mussel reefs provide habitat for similar macrobenthic communities, except for the barnacle Elminius modestus, which was found predominantly with oysters (Drent and Dekker, 2013). This field experiment would support the result from the Wadden Sea: barnacles were found on some of the rocks and oyster shells, and only few individuals on mussel shells.
Epibenthic Species and Birds
The barnacle Elminius modestus was the only recorded epibenthic species and therefore epibenthic colonization played a small role in this study. Generally, epibenthic species use bivalve reefs as attachment surface and may also indirectly benefit from shell-reefs. For example, the sea cucumber Apostichopus japonicus was found to grow faster on artificial oyster shell-reefs because of increased food supply by diatoms colonizing the shells (Zhang et al., 2014). Whelks were shown to be positively affected by the presence of dense mussel beds that affected their feeding, growth and interaction with other species (Gosnell et al., 2012). Biogenic shell banks provide feeding habitat for coastal birds, but mussel and oyster beds support different species; reefs of the Pacific oyster Crassostrea gigas are used by curlews and oyster catchers, while herring gulls prefer mussel beds (Markert et al., 2013); copious footprints of gulls around the reef-units in this experiment indicated that birds were attracted to the features, but no direct observations of the use were made.
Production vs. Aggregation
There is an ongoing discussion if artificial reefs produce new biomass, or if they act as a focal point for existing organisms which aggregate on or in the newly formed reef (Cresson et al., 2014). This is considered to depend on the species present and their limitation by food, refuge, territory, and/or behavioral requirements. Colonisation of new artificial reefs generally follows two main processes: migration of post-larval individuals and settling of larvae. The recruitment success depends on the presence of larvae and mobile invertebrates in the water column, and the nature of the infauna communities near the reef.
The fauna in reef-units of this experiment comprised of species also found in surrounding sand flats, but an additional 37 species were exclusively found in the reefs (of a total of 45 species). Given the relatively short duration of the experiment (5 months), it is likely that they migrated into the reef units as opposed to being new biomass, and therefore the reefs would have re-distributed existing biomass. It seems, however, plausible that over time the reefs could be attractive nurseries for benthic fauna and enhance biomass production; there is evidence that mussel and oyster banks are attractive habitats for juvenile fish and crustaceans (Kochmann et al., 2008; Seitz et al., 2014). The crab Hemigrapsus sanguineus, for example, uses mussel beds only during early life stages to be protected from intra and interspecific predation (Pezy and Dauvin, 2014). Artificial reef structures could have a similar nursery function.
Conclusion
Material accretions with an open matrix that provide interstitial space and trap sediment have the potential to enhance benthic biodiversity. Like other coastal reefs the main function seems to be protection from severe environmental conditions and predation. However, the field experiment suggested that artificial reefs may also be attractive hunting grounds for polychaete predators, and it would be useful to better understand this function of reefs. Given the likely short-term use of reef structures by some species, it is possible that they interact with surface layers more extensively than with deeper reef areas. Further studies need to clarify this and could have implications for the surface design of coastal infrastructure.
This study indicated that it is possible to create man-made reef units which provide biodiversity enhancing services. Different types of material seem to be suitable for loose-material reefs. Bivalve shells provided suitable structures, but just piles of small rocks were equally effective. It is therefore possible that other materials could achieve a similar effect, such as gastropod shells, different types of natural stone or slate, or recycled material such crushed concrete. Considerations such as the suitability of these materials in a specific coastal environment need to be evaluated, but in terms of infauna-enhancing measure there seems to be creative flexibility.
Author Contributions
RC is responsible for the creation of the project, the experimental design, conducting the experiment, data processing and analysis and the interpretation of the results. She is the sole writer of the paper.
Conflict of Interest Statement
The author declares that the research was conducted in the absence of any commercial or financial relationships that could be construed as a potential conflict of interest.
Acknowledgments
This study was supported by the SEACAMS project, which is part-funded by the EU European Regional Development Fund (ERDF) through the Welsh Government. Many thanks to David Holland from SALIX for providing us with reef-netting and experimental rock-rolls. Technical support was provided by Chiara Bertelli (constructing of reefs, field work, species identification); Anouska Mendzil processed the sediment samples. Ian Dodkins, Christine Grey and Jake Scolding assisted with field work. Particular thanks to the skipper Keith Naylor for his creative use of the RV Noctiluca to deploy and retrieve the reef units. Drs Lise Chapman and John Griffin made constructive suggestions on an earlier draft of the manuscript.
Supplementary Material
The Supplementary Material for this article can be found online at: https://www.frontiersin.org/articles/10.3389/fmars.2018.00288/full#supplementary-material
References
Ambrose, R. F., and Swarbrick, S. L. (1989). Comparison of fish assemblages on artificial reefs off the coast of Southern California. Bull. Mar. Sci. 44, 718–733.
Anderson, M. J., Ellingsen, K. E., and McArdle, B. H. (2006). Multivariate dispersion as a measure of beta diversity. Ecol. Lett. 9, 683–693. doi: 10.1111/j.1461-0248.2006.00926.x
Armonies, W. (1992). Migratory rhythms of drifting juvenile molluscs in tidal waters of the Wadden Sea. Mar. Ecol. Prog. Ser. 83, 197–206.
Baine, M. (2001). Artificial reefs: a review of their design, application, management and performance. Ocean Coast. Manag. 44, 241–259. doi: 10.1016/S0964-5691(01)00048-5
Bartholomew, A., Diaz, R., and Cicchetti, G. (2000). New dimensionless indices of structural habitat complexity: predicted and actual effects on a predator's foraging success. Mar. Ecol. Prog. Ser. 206, 45–58. doi: 10.3354/meps206045
Bartol, I. K., Mann, R., and Luckenbach, M. W. (1999). Growth and mortality of oysters (Crassostrea virginica) on constructed intertidal reefs: effects of tidal height and substrate level. J. Exp. Mar. Biol. Ecol. 237, 157–184.
Blott, S. J., and Pye, K. (2001). GRADISTAT: a grain size distribution and statistics package for the analysis of unconsolidated sediments. Earth Surface Process. Landforms 26, 1237–1248. doi: 10.1002/esp.261
Broadhurst, M., and Orme, C. D. (2014). Spatial and temporal benthic species assemblage responses with a deployed marine tidal energy device: a small scaled study. Mar. Environ. Res. 99, 76–84. doi: 10.1016/j.marenvres.2014.03.012
Brown, L. A., Furlong, J. N., Brown, K. M., and La Peyre, M. K. (2014). Oyster reef restoration in the northern Gulf of Mexico: effect of artificial substrate and age on nekton and benthic macroinvertebrate assemblage use. Restor. Ecol. 22, 214–222. doi: 10.1111/rec.12071
Callaway, R. (2006). Tube worms promote community change. Mar. Ecol. Prog. Ser. 308, 49–60. doi: 10.3354/meps308049
Callaway, R. (2016). Historical data reveal 30-year persistence of benthic fauna associations in heavily modified waterbody. Front. Mar. Sci. 3:141. doi: 10.3389/fmars.2016.00141
Chapman, M. G., and Clynick, B. G. (2006). Experiments testing the use of waste material in estuaries as habitat for subtidal organisms. J. Exp. Mar. Biol. Ecol. 338, 164–178. doi: 10.1016/j.jembe.2006.06.018
Coen, L. D., Brumbaugh, R. D., Bushek, D., Grizzle, R., Luckenbach, M. W., Posey, M. H., et al. (2007). Ecosystem services related to oyster restoration. Mar. Ecol. Prog. Ser. 341, 303–307. doi: 10.3354/meps341303
Coen, L. D., and Luckenbach, M. W. (2000). Developing success criteria and goals for evaluating oyster reef restoration: ecological function or resource exploitation?. Ecol. Eng. 15, 323–343. doi: 10.1016/S0925-8574(00)00084-7
Cresson, P., Ruitton, S., and Harmelin-Vivien, M. (2014). Artificial reefs do increase secondary biomass production: mechanisms evidenced by stable isotopes. Mar. Ecol. Prog. Ser. 509, 15–26. doi: 10.3354/meps10866
Drent, J., and Dekker, R. (2013). Macrofauna Associated With Mussels, Mytilus edulis L., in the Subtidal of the Western Dutch Wadden Sea. NIOZ-Report, 2013-7, hdl:10670/1.bj5lbd
Einbeinder, S., Perelberg, A., Ben-Shaprut, O., Foucart, M. H., and Shashar, N. (2006). Effects of artificial reefs on fish grazing in their vicinity: evidence from algae presentation experiments. Mar. Environ. Res. 61, 110–119. doi: 10.1016/j.marenvres.2005.07.001
Fabi, G., Spagnolo, A., Bellan-Santini, D., Charbonnel, E., Çiçek, B. A., Goutayer, J. J., et al. (2011). Overview on artificial reefs in Europe. Br. J. Oceanogr. 59, 155–166. doi: 10.1590/S1679-87592011000500017
George, L. M., De Santiago, K., Palmer, T. A., and Beseres Pollack, J. (2015). Oyster reef restoration: effect of alternative substrates on oyster recruitment and nekton habitat use. J. Coast. Conserv. 19, 13–22. doi: 10.1007/s11852-014-0351-y
Gosnell, J. S., DiPrima, J. B., and Gaines, S. D. (2012). Habitat complexity impacts persistence and species interactions in an intertidal whelk. Mar. Biol. 159, 2867–2874. doi: 10.1007/s00227-012-2047-x
Grabowski, J. H. (2004). Habitat complexity disrupts predator–prey interactions but not the trophic cascade on oyster reefs. Ecology 85, 995–1004. doi: 10.1890/03-0067
Grabowski, J. H., Hughes, A. R., and Kimbro, D. L. (2008). Habitat complexity influences cascading effects of multiple predators. Ecology 89, 3413–3422. doi: 10.1890/07-1057.1
Gutiérrez, J. L., Jones, C. G., Strayer, D. L., and Iribarne, O. O. (2003). Mollusks as ecosystem engineers: the role of shell production in aquatic habitats. Oikos 101, 79–90. doi: 10.1034/j.1600-0706.2003.12322.x
Hayward, P. J., and Ryland, J. S. (2017). Handbook of the Marine Fauna of North-West Europe. Oxford: Oxford University Press. 880.
Hunter, W. R., and Sayer, M. D. J. (2009). The comparative effects of habitat complexity on faunal assemblages of northern temperate artificial and natural reefs. ICES J. Mar. Sci. 66, 691–698. doi: 10.1093/icesjms/fsp058
Inger, R., Attrill, M. J., Bearhop, S., Broderick, A. C., Grecian, W. J., Hodgson, D. J., Godley, B. J., et al. (2009). Marine renewable energy: potential benefits to biodiversity? An urgent call for research. J. Appl. Ecol. 46, 1145–1153. doi: 10.1111/j.1365-2664.2009.01697.x
Jensen, A. (2002). Artificial reefs of Europe: perspective and future. ICES J. Mar. Sci. 59, S3–S13. doi: 10.1006/jmsc.2002.1298
Jones, C. G., Lawton, J. H., and Shachak, M. (1994). Organisms as ecosystem engineers. Oikos 69, 373–386.
Kazemi, S. Y., Biparva, P., and Ashtiani, E. (2016). Cerastoderma lamarcki shell as a natural, low cost and new adsorbent to removal of dye pollutant from aqueous solutions: equilibrium and kinetic studies. Ecol. Eng. 88, 82–89. doi: 10.1016/j.ecoleng.2015.12.020
Kochmann, J., Buschbaum, C., Volkenborn, N., and Reise, K. (2008). Shift from native mussel to alien oysters: diffential effects of ecosystem engineers. J. Exp. Mar. Biol. Ecol. 364, 1–10. doi: 10.1016/j.jembe.2008.05.015
La Peyre, M., Furlong, J., Brown, L. A., Piazza, B. P., and Brown, K. (2014). Oyster reef restoration in the northern Gulf of Mexico: extent, methods and outcomes. Ocean Coast. Manag. 89, 20–28. doi: 10.1016/j.ocecoaman.2013.12.002
Langhamer, O. (2012). Artificial reef effect in relation to offshore renewable energy conversion: state of the art. Sci. World J. 2012:386713. doi: 10.1100/2012/386713
Langois, T. J., Anderson, M. J., and Babcock, R. C. (2006). Inconsistent effects of reefs on different size classes of macrofauna in adjacent sand habitats. J. Exp. Mar. Biol. Ecol. 334, 269–282. doi: 10.1016/j.jembe.2006.02.001
Largaespada, C., Guichard, F., and Archambault, P. (2012). Meta-ecosystem engineering: nutrient fluxes reveal intraspecific and interspecific feedbacks in fragmented mussel beds. Ecology 88, 82–89. doi: 10.1890/10-2359.1
Lavender, J. T., Dafforn, K. A., Bishop, M. B., and Johnston, E. L. (2017). Small-scale habitat complexity of artificial turf influences the development of associated invertebrate assemblages. J. Exp. Mar. Biol. Ecol. 492, 105–112. doi: 10.1016/j.jembe.2017.01.025
Lawless, A. S., and Seitz, R. D. (2014). Effects of shoreline stabilization and environmental variables on benthic infaunal communities in the Lynnhaven River system of Chesapeake Bay. J. Exp. Mar. Biol. Ecol. 457, 41–50. doi: 10.1016/j.jembe.2014.03.010
Lenihan, H. S. (1999). Physical–biological coupling on oyster reefs: how habitat structure influences individual performance. Ecol. Monogr. 69, 251–275.
Levine, E. A., Gosnell, J. S., Goetz, E. M., and Malinowski, C. R. (2017). Natural cultch type influences habitat preference and predation, but not survival, in reef-associated species. Restor. Ecol. 25, 101–111. doi: 10.1111/rec.12385
Lundquist, C. J., Bulmer, R. H., Clark, M. R., Hillman, J. R., Nelson, W. A., Norrie, C. R., et al. (2017). Challenges for the conservation of marine small natural features. Biol. Conserv. 211, 69–79. doi: 10.1016/j.biocon.2016.12.027
Markert, A., Esser, W., Frank, D., Wehrmann, A., and Exo, K. M. (2013). Habitat change by the formation of alien Crassostrea-reefs in the Wadden sea and its role as feeding sites for waterbirds. Estuarine Coast. Shelf Sci. 131, 41–51. doi: 10.1016/j.ecss.2013.08.003
Moffitt, R. B., Parrish, F. A., and Polovina, J. J. (1989). Community structure, biomass and productivity of deepwater artificial reefs in Hawaii. Bull. Mar. Sci. 44, 616–630.
OSPAR CCOMMISSION. (1999). OSPAR Guidelines on Artificial Reefs in Relation to Living Marine Resources. London: OSPAR 99/15/1-E, Annex 6.
OSPAR COMMISSION (2009). Assessment of Construction or Placement of Artificial Reefs. London: Biodiversity Series, publication No. 438/2009. 27.
Pezy, J. P., and Dauvin, J. C. (2014). Are mussel beds a favourable habitat for settlement of Hemigrapsus sanguineus (De Haan, 1835)? Aqua. Invasions 10, 51–56. doi: 10.3391/ai.2015.10.1.05
SALIX (2018). Available online at: https://www.salixrw.com/product/rock-rolls/ (Accessed April 24 2018)
Sandrini-Neto, L., and da Cunha Lana, P. (2014). Does mollusc shell debris determine patterns of macrofaunal recolonisation on a tidal flat? Experimental evidence from reciprocal transplantations. J. Exp. Mar. Biol. Ecol. 452, 9–21. doi: 10.1016/j.jembe.2013.11.012
Schulte, D. M., Burke, R. P., and Lipcius, R. N. (2009). Unprecedented restoration of a native oyster metapopulation. Science 325, 1124–1128. doi: 10.1126/science.1176516
Scyphers, S. B., Powers, S. P., Heck, K. L., and Byron, D. (2011). Oyster reefs as natural breakwaters mitigate shoreline loss and facilitate fisheries. PLoS ONE 6:e22396. doi: 10.1371/journal.pone.0022396
Seitz, R. D., Wennhage, H., Bergström, U., Lipcius, R. N., and Ysebaert, T. (2014). Ecological value of coastal habitats for commercially and ecologically important species. ICES J. Mar. Sci. 71, 648–665. doi: 10.1093/icesjms/fst152
Temmerman, S., Meire, P., Bouma, T. J., Herman, P. M., Ysebaert, T., and De Vriend, H. J. (2013). Ecosystem-based coastal defence in the face of global change. Nature 504, 79–83. doi: 10.1038/nature12859
Tolley, S. G., and Volety, A. K. (2005). The role of oysters in habitat use of oyster reefs by resident fishes and decapod crustaceans. J. Shellfish Res. 24, 1007–1012. doi: 10.2983/0730-8000(2005)24[1007:TROOIH]2.0.CO;2
van Loon-Steensma, J. M., Schelfhout, H. A., and Vellinga, P. (2014). Green adaptation by innovative dike concepts along the Dutch Wadden Sea coast. Environ. Sci. Pol. 44, 108–125. doi: 10.1016/j.envsci.2014.06.009.
Warwick, R. M., and Clarke, K. R. (1993). Increased variability as a symptom of stress in marine communities. J. Exp. Mar. Biol. Ecol. 172, 215–226.
Keywords: benthos, biodiversity, biogenic reef, cockle, infauna, mussel, oyster
Citation: Callaway R (2018) Interstitial Space and Trapped Sediment Drive Benthic Communities in Artificial Shell and Rock Reefs. Front. Mar. Sci. 5:288. doi: 10.3389/fmars.2018.00288
Received: 26 April 2018; Accepted: 27 July 2018;
Published: 17 August 2018.
Edited by:
Andrew James Guerin, Newcastle University, United KingdomReviewed by:
Valeriya Komyakova, Norwegian Institute of Marine Research (IMR), NorwayJuan Moreira Da Rocha, Universidad Autonoma de Madrid, Spain
Copyright © 2018 Callaway. This is an open-access article distributed under the terms of the Creative Commons Attribution License (CC BY). The use, distribution or reproduction in other forums is permitted, provided the original author(s) and the copyright owner(s) are credited and that the original publication in this journal is cited, in accordance with accepted academic practice. No use, distribution or reproduction is permitted which does not comply with these terms.
*Correspondence: Ruth Callaway, ci5tLmNhbGxhd2F5QHN3YW5zZWEuYWMudWs=