- 1Red Sea Research Center, King Abdullah University of Science and Technology, Thuwal, Saudi Arabia
- 2The UWA Oceans Institute, The University of Western Australia, Crawley, WA, Australia
- 3Australian Institute of Marine Science, Townsville, WA, Australia
Concern over the deterioration of the oceans has generated a demand for a system able to assess ocean health globally, and approaches to assess ocean health globally and an observing system delivering the data to support this assessment are now being developed. In parallel with public health systems, a system to assess ocean health should evaluate the occurrence and severity of a set of syndromes of concern through a series of indicators composed from a parsimonious set of ecosystem essential ocean variables delivered by a coherent and robust observing system. The development of such approach for the global assessment of ocean health will help inform policies acting upon the drivers causing ocean syndromes and help ensure a healthy ocean for all.
Introduction
In a much-quoted US Supreme Court opinion on pornography in the film industry, Justice Potter Stewart stated “I shall not today attempt further to define the kinds of material I understand to be embraced within that shorthand description (referring to pornography); and perhaps I could never succeed in intelligibly doing so. But I know it when I see it.” Defining Ocean Health has proven just as challenging for scientists (Tett et al., 2013), and yet most scientists, managers and citizens would feel reasonably confident they can identify a healthy ocean ecosystem within the region they operate or live when they see it. Difficulties in the capacity to define ocean health in an operational way poses a major challenge to deliver on the assessments of the state of the ocean that society increasingly demands.
There are growing concerns that accumulated pressures on the global oceans, from syndromes such as overfishing, pollution, coastal development, climate change, and ocean acidification (Table 1) are causing serious deterioration of the health of ocean ecosystems (Jackson et al., 2001; Mooney et al., 2009; Duarte, 2014), and that left unabated there is risk of catastrophic loss of biodiversity, habitats and productivity. This widely shared belief is epitomized in the statement “Humans have put the oceans under risk of irreversible damage” accompanying the Oceans Compact declaration by UN Secretary General Ban Ki Moon (United Nations, 2012). In responding to this risk, the assessment of ocean health has reached the status of legal mandate in some nations, such as the EU through the Marine Strategy Framework Directive (MSFD) (European Union, 2008) and the US Executive Order 13547 on the Stewardship of the Ocean, Our Coasts, and the Great Lakes (US Government, 2010). The concept of ecosystem health has taken a pivotal role in framing the goals of environmental policy, as reflected in Principle Seven of the Río Declaration on Environment and Development, which reads “States shall cooperate in a spirit of global partnership to conserve, protect and restore the health and integrity of the Earth's ecosystems.”
The assessment of ocean health has recently been added, through the United Nations World Ocean Assessment (WOA) (Regular Process for Global Reporting of the State of the Marine Environment) to the regular global assessments the UN conducts on the state of the planet, including the assessments of the climate system conducted by the IPCC since 1990 and biodiversity to be conducted by the International science-policy Platform on Biodiversity and Ecosystem Services (IPBES). In its first report, the WOA adopted the DPSIR (Driver-Pressure-State-Impact-Response) approach to assess the status of the ocean, and in doing so listed ecosystem health as a state that changes in response to human pressures (Inniss et al., 2016). However, the assessment was challenged by the lack of an agreed framework to assess ocean health globally. In its first assessment, the WOA explored a number of approaches, such as the Ocean Health Index (OHI, Halpern et al., 2012), the ecological quality objectives (EcoQOs) for an ocean area (OSPAR, 2007), and the indicators adopted in 2017 under the EU MSFD (cf. https://eur-lex.europa.eu/legal-content/EN/TXT/?qid=1495097018132&uri=CELEX:32017D0848). Yet, the delivery of a global assessment based, for instance, on the OHI, was hampered by the paucity of the data required to apply these approaches at the global scale, and thus required a large degree of expert judgement to reach any conclusions (Inniss et al., 2016). Specifically, the WOA concludes “The OHI depends crucially on the availability of satisfactory data across many fields, and on the expert judgements made about the weighting to be given to the different fields covered. Much of the necessary data is not available, and estimates of various kinds have to be used instead. The scale of the expert judgements needed means that there is a substantial subjective component in any results” (Inniss et al., 2016). The outcome consisted, therefore, of DPSIR narratives at the habitat level, a tabulated list of pressures on the marine environment, along with the corresponding impacts on the marine environment and socio-economic aspects of the marine environment (Chapter 54 in Inniss et al., 2016). While some comparable assessments, such as the IPCC, also contain qualitative, narrative components (e.g., impacts sections), the WOA could not fully meet its mandate to review the state of the marine environment because a system delivering the required data was not in place (Inniss et al., 2016). Indeed, assessing and attributing impacts on climate change on ecosystems and biodiversity is challenging, and so is the assessment of ocean health, where multiple candidate variables, many of which are unavailable at the global scale, maybe required.
Efforts to progress toward a global observing system to assess ocean health should meet two major requirements: (1) identify the components and processes required to operationalize the assessment, and (2) articulate an effective observational basis to deliver the data underpinning a robust assessment, and make these data openly available. Our goal here is to examine the maintenance of ocean health as an aspirational goal, and to offer an overarching conceptual basis, based on a parallel with the evaluation of human health, to develop a robust global observation system to assess ocean health.
Implementation of the Assessment of Ocean Health
The concept of ecosystem health applies public understanding of the notion of human health to ecosystems, which is intuitively grasped by the general public and policy makers (Costanza, 1992). Statutory mandates to assess ocean health require a definition of ocean health but fail to recognize that it is in reality a metaphor (e.g., Fock and Kraus, 2016) referring to the perceived integrity of the structure and functioning of ocean ecosystems. As a metaphor it is not directly amenable to simple definition or quantification (Tett et al., 2013). Efforts to define ocean health typically yield subsidiary metaphors such as “the condition of a system that is self-maintaining, vigorous, resilient to externally imposed pressures, and able to sustain services to humans” (Tett et al., 2013). The broader concept of ecosystem health has similar problems, as a healthy ecosystem is defined as being “stable and sustainable”; “maintaining its organization and autonomy over time and its resilience to stress” (Costanza, 1992), each of those being qualities that are no closer to being operational than that of “healthy.” Some frameworks emphasize the examination of trajectories of ecosystems under pressure, which are complex and often non-linear, to diagnose their health, which is then embedded in the DPSIR paradigm (Tett et al., 2013).
Directives to assess ocean health against properties that are qualitative and non-operational risk being ineffective as the robustness of the outcomes cannot be audited. The EU Marine Strategy aims at maintaining “oceans and seas which are clean, healthy and productive” (European Union, 2008). While a definition of ocean health in is lacking in the EU MFSD, the assessments are guided by a definition of Good Environmental Status, and multiple metrics of status and pressures are aggregated into indices to provide diagnostics on environmental status (Borja et al., 2016; Heiskanen et al., 2016). However, this framework is resource-demanding and, therefore, very difficult to automatize or implement at the global scale. The OHI was proposed to provide a quantitative, reproducible metric of ocean health, where a healthy ocean was defined as “one that sustainably delivers a range of benefits to people now and in the future” (Halpern et al., 2012, 2015, 2017). The OHI provides “a framework to evaluate sustainable delivery of benefits people want from healthy oceans by measuring progress toward 10 widely-held societal goals” (Longo et al., 2017). The OHI scores ocean health on the basis of societal goals related to human use (e.g., food provision from fisheries, natural products extraction, and tourism and recreation) as well as conservation objectives (e.g., clean waters and biodiversity) of the marine ecosystems evaluated, and is argued to be particularly suited as a basis for ecosystem-based management of these ecosystems (Longo et al., 2017). As most of the components of the OHI relate to human use of marine ecosystems, this index quantifies benefits humans derived from a healthy ocean and is, therefore, best suited for ocean areas under intense human use. Indeed, although the OHI can be applied anywhere, application of the OHI focusses on economic exclusive zones (Halpern et al., 2012), which have been the focus of global OHI assessments (Halpern et al., 2012, 2015, 2017). Thus far international waters (i.e., International Waters and Areas Beyond National Jurisdiction), which comprise 64 percent of the ocean surface, have not yet been addressed in OHI assessments, although the OHI has been recently applied to areas with less intense human use, such as the Southern Ocean (Longo et al., 2017). The calculation of the OHI is also demanding on data, because as 10 traits are evaluated (Halpern et al., 2012), many, such as “biodiversity” and “clean water,” are aggregated traits that require multiple data streams, which can be an impediment for its broad application (Inniss et al., 2016). Yet, this is not exclusive of the OHI as any rigorous assessment of ocean health will likely be data demanding. The OHI has proven particularly useful as an assessment of human stressors and as a lagging indicator of ocean health. However, where health assessments point at departures from healthy states, remedial action will benefit from the support provided by an observation system reporting on the status or trends in key marine ecosystem traits on which management actions can be targeted and assessed. Ideally, the ocean observation system identifying departures from healthy state will also allow to objectively measure effectiveness of management actions. For instance, fisheries management provides an example where such objective measures of stock status allow to adaptively manage the human impact on stocks and associated ecosystems. Indeed, Samhouri et al. (2012) provide an example of how to set targets for sustainable fisheries under the framework of the application of the OHI. We need a similarly robust approach to measuring other actionable components of marine ecosystems.
The central role of ecosystems services in the assessment of the OHI, has thus far proven somewhat problematic, as it is unclear how the choice of ecosystem services included affect the assessment of ecosystem health (Jacobsen and Hanley, 2009; Fock and Kraus, 2016). In addition, assessments based on the OHI are sensitive to the weights given to these services, which affect how changes in specific ecosystem services, which may not result from human pressures alone but also from natural fluctuations, translate into changes in ecosystem health. Most importantly, prescriptive approaches based on expected outcomes for society are necessarily arbitrary and difficult to transpose across regions, cultures and societal values, and, therefore, cannot be shared universally, because expected outcomes and aspirations from ocean conservation measures depend, among many other factors, on wealth, culture, and background (Jacobsen and Hanley, 2009). Indeed, assessments based on indices driven by interpretations of the “ocean health” metaphor jeopardize scientific specificity and rigor, because these are not entirely based on data but values, which also render them vulnerable to political disagreements based on different interpretations (Fock and Kraus, 2016).
An effort to “ground” the metaphor of ecosystem health concluded that this concept is better operationalized and communicated when defined by the absence of disease and distress (Rapport et al., 1998; Fock and Kraus, 2016), as a healthy ecosystem is also defined as one free of signs of ecosystem distress (Costanza, 1992). Similarly, whereas World Health Organization defines human health as “a state of complete physical, mental and social well-being and not merely the absence of disease or infirmity” (www.who.int/about/mission/en/) it is the absence of disease or infirmity, rather than the many dimensions of well-behind, that are targeted in routine assessment systems. Hence, operationally, systematic assessments of individual health in public health systems focus on detecting the presence of syndromes conducive to unhealthy states. Likewise, whereas the concept of ocean health maybe more encompassing than just the absence of syndromes, observing systems to assess ocean health are more easily operationalized when guided to the detection of syndromes. Drawing a parallel with the assessment of human health in public health systems, the assessment of syndromes affecting ocean health must involve three key components (Table 1): (1) a limited set of syndromes, defined as a group of signs and symptoms that occur together and characterize a particular abnormality in ocean ecosystem state, or maladies of concern (e.g., increase in coronary diseases, morbid obesity, malnutrition, diarrheic diseases, or cancer, in public health), such as overfishing, ocean acidification, pollution or hypoxia; (2) the identification of a parsimonious set of robust indicators of the occurrence of such syndromes (e.g., cholesterol levels, body mass index, blood nutrient levels, in public health), such as habitat loss, increased harmful algal blooms, decline in fish stocks, and a series of variables required to calculate the indicators (weight and height, iodine, iron and cholesterol concentration in blood, in public health), such as fish biomass, habitat extent, nutrient concentrations, oxygen and chlorophyll a concentration; and (3) the identification of a set of drivers causing the syndromes (e.g., lack of physical exercise, inadequate water and food quantity and quality, in public health), such as pollutants (nutrients, persistent organic pollutants and heavy metals), increased atmospheric CO2, anthropogenic climate change, overfishing, and coastal development (Table 1). Acute disruptions of human health, where individuals suffer pain or are at risk, are typically managed reactively by the subjects visiting emergency rooms. Indeed, routine health assessments, health checks, are conducted precisely to diagnose the most common syndromes that may, if not addressed, lead to such acute problems. Likewise, critical ecological issues in ecosystems in risk of collapse are likely to be managed reactively through a series of urgent measures, whereas the goal of an assessment of ocean health is, indeed, to proactively detect syndromes that may, if not addressed, lead to risk of collapse. Providing a necessary, sufficient but parsimonious set of drivers, syndromes, indicators and variables to support the assessment of syndromes affecting ocean health, for which Table 1 provides just some examples to highlight the parallel with the assessment of human health, is a critical, but challenging task. It also requires the definition of the frequency, scale and standardized protocols for observation of the variables, that will require a broad community effort. Whereas many efforts are currently being allocated to the definition of essential variables (Constable et al., 2016), these are not aligned with the sole index proposed thus far, the OHI (Halpern et al., 2012), which, in turn is not specifically designed to identify specific syndromes. This highlights the need to complement assessments of ocean health based on the OHI with the development of an observational system informing on the presence of syndromes of interest. The risk of failing to do so is that the components of the observing system may not be consistent, therefore failing to derive useful, actionable diagnostics.
A sustained and robust observing system, measuring variables required to produce such indicators would allow the assessment of whether and where syndromes characteristic of poor ocean health occur or change in magnitude, with the corresponding trends providing a diagnostic on shifts in ocean health (Figure 1). This assessment then informs policy actions on the drivers believed to trigger the syndromes, with the observing system subsequently verifying whether the actions were indeed effective at halting or reducing the spread of syndromes (Figure 1), allowing the assessment of the benefits, in terms of enhanced ecosystem services, to society derived from the OHI. The feed-back between the assessment of ocean health, actions to remediate syndromes detected and the evaluation of the response (Figure 1) is a case for adaptive management (see below). It is also possible to integrate the indicators of marine ecosystem state into a meta-indicator that provides a metric of the risk of failure to remain healthy of a particular ecosystem. This is an approach developed in industrial failure analysis, where multiple sensors are integrated into a meta-sensor, defined as a virtual sensor that compresses the information from several sensors in an optimal manner (Butters et al., 2015). Integrated, meta-indicators have been developed in the context of marine biodiversity assessments in European seas (e.g., Andersen et al., 2014; Borja et al., 2016; Heiskanen et al., 2016; Uusitalo et al., 2016). Developing such meta-sensor of ocean health requires big-data approaches allowing machine learning algorithms, such as deep-learning, to feed on multiple cases of failure of marine ecosystems around the world including time series of data of the various candidate indicators to be integrated. Such big-data approach, including machine-learning diagnostics informed by mechanistic understanding of marine ecosystem responses to pressures, would eventually allow real-time diagnostics supporting management actions to address incipient challenges to ocean health.
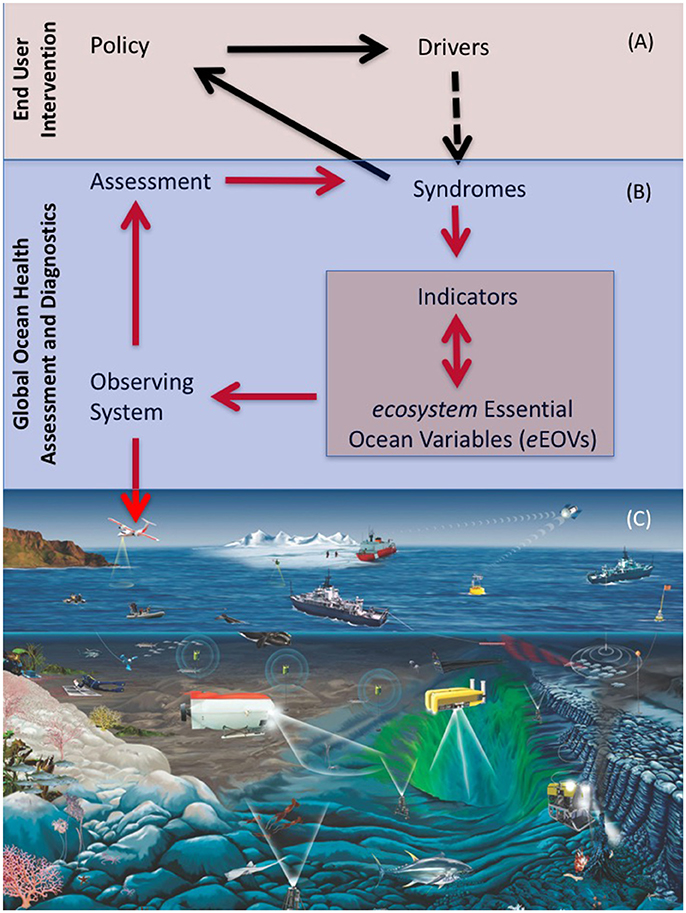
Figure 1. A framework for the global assessment of ocean health identifying the policy actors that take actions to improve ocean health (A) on the basis of diagnostics resulting from the assessment of ocean health (B), supported by the technology and observational platforms required to deliver the data on the ecosystem Essential Ocean Variables informing the process (C). Black arrows identify feedback between diagnostics and policy and managerial intervention, and red arrows identify inputs and interactions within the ocean health assessment process. Image (B) by E. Paul Oberlander, provided courtesy of the Census of Marine Life and reproduced with permission under the terms of the Creative Commons Attribution License.
The quest for such global system to assess ocean health can be inspired by existing frameworks to assess good environmental status of marine ecosystems. These include the assessments to be conducted under the EU Marine Strategy Framework Directive (MSFD; 2008/56/EC), the reference legal frameworks for the protection of marine biodiversity in European Seas. The MSFD evaluates the ecological status of the marine environment based on 11 descriptors, comprising biodiversity (biological diversity; food-webs; and seafloor integrity) and pressure (non-indigenous species; fisheries; eutrophication; hydrological conditions; contaminants in the environment and in seafood; litter; energy and noise) descriptors (Heiskanen et al., 2016). The EU Commission Decision 2010/477/EU provides 29 criteria and 56 associated “indicators” that should be monitored for the assessment of the environmental status (Heiskanen et al., 2016). The HELCOM holistic assessment of the state of the Baltic Sea, initiated in 2010, provides an earlier, comprehensive example of an operational assessment, at a regional scale, of ocean health. The HELCOM holistic assessment is a system of coordinated monitoring among 9 nations with a unified set of indicators and thresholds (http://www.helcom.fi/baltic-sea-trends and the latest assessment: http://stateofthebalticsea.helcom.fi/), supported by assessment tools for integrated assessments (http://www.helcom.fi/action-areas/monitoring-and-assessment/monitoring-manual/) and operational links to policy processes. Hence, the HELCOM holistic assessment of the state of the Baltic Sea complies with the steps in the framework we recommend (Figure 1).
Delivering an Effective Observational Basis to Assess Ocean Health: What is Required?
In 2009, more than 600 of the world's leading oceanographers identified the requirement for a framework to guide the design and coordination of a multidisciplinary sustained global ocean observing system that would deliver the data and information needed for monitoring status and trends of ocean physics, biogeochemistry and biology/ecosystem health (Fischer et al., 2010).
In response, a Framework for Ocean Observing (FOO; Lindstrom et al., 2012) was developed, adapting the model employed by the Intergovernmental Oceanographic Commission and its partners to build the global ocean observing system for climate. For over 20 years, this system has provided the basis for building a comprehensive set of remotely sensed (satellite) and in-situ observations to support climate science and to inform policy and actions under the UN Framework Convention on Climate Change.
The FOO takes an engineering approach to designing and building an integrated ocean observing system serving the needs of UNFCCC, as well as the myriad bodies interested in assessing and managing ocean health, and the emerging oceans services sector that supports end users spanning safety and industries involved in the “blue economy” (World Bank United Nations Department of Economic Social Affairs, 2017). It has recently been used as a foundation for discussions in the ocean biology, biodiversity and ecosystems (Constable et al., 2016) communities on what needs to be observed/measured and how these measurements will be used to inform policy and regulation. In doing so, the FOO reverts the process followed by many assessment processes, which identify first what data are available and then ask what relevant information on ocean health can be derived from existing data, to first ask what is it that need be assessed, and what data are required to inform the assessment with high confidence. Hence, these discussions focus on the development of an agreed set of ecosystem “essential ocean variables” (eEOVs, Constable et al., 2016) and “essential biodiversity variables” (eBOV's, Muller-Karger et al., 2018). In the absence of the equations of state that govern physical oceanography and the prioritization of observations of ocean physics, the challenge for the ocean biology and ecosystem communities is to determine how best to define, and agree on, what is “essential” for a sustained observation system (Constable et al., 2016). The heterogeneity and complexity of ecosystems and ecosystem responses to pressures, along with the diversity of perspectives among biologists, make this a difficult challenge.
The first, and fundamentally critical step in meeting this challenge, is to understand the data and information requirements of end users. In the case of ocean ecosystem health assessment, this requires scientists working with policy makers and regulators, at local, national or intergovernmental levels, to drill down from “metaphor level” objectives and indicators to those that can be objectively and robustly evaluated. Halpern et al. (2017) emphasize that currently “all indicators face the substantial challenge of informing policy for progress toward broad goals and objectives with insufficient monitoring and assessment data. If countries and the global community hope to achieve and maintain healthy oceans, we will need to dedicate significant resources to measuring what we are trying to manage”. However, resourcing assessments is not enough, and cooperation toward a global assessment requires agreeing on the variables to be observed, standardized methods to do so and how these are to be aggregated to deliver the assessment (Figure 1), all of which requires strong scientific cooperation and leadership. Examples, at regional levels, do exist, such as the HELCOM holistic assessment described above, but scaling from a few nations, like the 9 nations in that assessment, to hundreds of nations is challenging. Such standardized global assessment need also have the flexibility to recognize regional differences, and, thus, probably develop region-specific thresholds, which may refer to individual variables or aggregated multi-metric indicators used with appropriate assessment tools to issue diagnostics, as for instance used in the regionalized thresholds for assessments under the EU MSFD or the HELCOM holistic assessment described above. Different frameworks are available that may provide already a blueprint for this global-scale regionalization, such as the Large Marine Ecosystem (Sherman and Hempel, 2009) or the Longhurst Biogeochemical Provinces (Longhurst et al., 1995), or a hybrid of these.
A Conceptual Model Guiding the Selection of Essential Ocean Variables
Encouragingly, there are now many groups working to define a set of “fit-for-purpose” eEOV for ocean biology, biodiversity, habitats and ecosystems in the context of the Global Ocean Observing System Ocean [GOOS, see Constable et al., 2016, Table 3], as well as other assessment frameworks, such as the development of Essential Biodiversity Indicators developed under the Marine Biodiversity Observation Network (MBON) within the Biodiversity Observation Network (GEO BON, Muller-Karger et al., 2018), and the selection of marine biodiversity indicators under the EU MSFD (Heiskanen et al., 2016). There is a need for these efforts to converge and agree on essential variable indicators of ocean health, and steps have been taken by the GOOS and GEO BON programs to converge (Muller-Karger et al., 2018). While these programs share a broad objective of building data and indicator time series to inform end-users on the status and trends of ocean ecosystems, we argue there is a pressing need for these groups, and the scientific community in general, to be more closely aligned with those in the policy, industry and community sectors for whom the data is intended. Moreover, the essential variables to be monitored by observing systems supporting assessment of ocean health need be assembled into indicators useful to governments and end-users (Niemeijer, 2002). The European Union's COPERNICUS Program, in which remotely sensed and in situ observations of the ocean are driven by the services and applications of a broad suite of end users, is a useful example of how tight alignment of data provider and user communities is providing significant societal benefit. While governance models for ensuring alignment will likely differ across the globe, we argue that the establishment of formal partnerships between providers and users would be of huge benefit. These partnerships will likely be essential if the United Nations Decade of Ocean Science for Sustainable Development (2021–2030), recently proclaimed by the United Nations General Assembly (A/RES/72/73, December 2017) is to achieve its goals.
It would also be efficient if agreement was reached on a conceptual model of ocean health assessment including characterization of the syndromes to be detected and the indicators required to diagnose their occurrence and severity (Table 1). Such a model should identify the syndromes potentially compromising ocean health and their anthropogenic drivers, and allow the attribution of changes in the occurrence and severity of the syndromes to these drivers. Furthermore, it should guide the selection of the most suitable and operational indicators, as well as the eEOV's required to evaluate them, to diagnose the occurrence of the syndromes (Table 1). The selection of syndromes, indicators and eEOVs should be guided by a principle of parsimony to ensure efficiency.
Ambitious Technological Developments to Deliver the Needed Data
The global assessment of ocean health demands and offers opportunities for technological advances comparable to those developed to explore the most distant galaxies or explore the Martian environment. That similarly powerful integrated platforms to assess ocean health are yet to be developed should not be considered acceptable. A global system to observe ocean health should involve ambitious targets to guide the development of revolutionary technologies including observing eEOV's from space and autonomous marine vehicles such as sea gliders, ocean robots or sensors mounted on marine organisms (Figure 1) loaded with miniaturized technologies to assess marine life, such as those emerging from the use of environmental DNA (Bourlat et al., 2013), combined with forthcoming in situ sequencing technologies and molecular chips for high-throughput screening of microbial activities (Tu et al., 2014), as a remote sensing tool (Bohmann et al., 2014; Valentini et al., 2016). Yet, a system that measures everything will not be either feasible nor useful. Reaching agreement on the key useful and feasible eEOV's (Constable et al., 2016) will be an important milestone propelling the conceptualization and development of technology for the integrated assessment of ocean health.
The technological breakthrough allowing the systematic observation of EOV's required to assess global ocean health is an ambitious, but achievable goal. This is exemplified by the Global Climate Observing System/Global Ocean Observing System Ocean (GCOS /GOOS) program, which developed the ARGO buoys, free-drifting profiling floats that measure temperature and salinity of the upper 2,000 m of the ocean (Gould et al., 2004). The ARGO program maintains a network of about 3,800, globally distributed ARGO floats and makes all data publicly available within hours after collection to monitor and assess global ocean climate dynamics (cf. http://www.argo.ucsd.edu).
The challenges that global assessments of ocean health, such as the World Ocean Assessment, face derive partly from our collective failure to design and deploy a monitoring system delivering the necessary data, so that the indices and metrics adopted cannot be quantified due to insufficient data availability, eventually leading to expert judgment and narratives replacing the use of indices (e.g., Inniss et al., 2016). We submit that future attempts at assessing global ocean health will continue to fail to deliver robust assessments unless the observing platform delivering the necessary data is deployed. Funding will indeed be required, but need not be an impediment. Each ARGO float costs about US $ 50,000, with a nominal life span of 5 years, but typical operational life span of 3 years (i.e., an annual investment of about US $ 60 million to maintain the 3,800 ARGO float fleet). Assuming deployment, typically using ships of opportunity, and operation to require 30% of the infrastructure costs, the ARGO program would run with about US $ 80 million per year. These costs are distributed among participating nations, rendering this an achievable goal within the forthcoming decade of the oceans. The ARGO approach has been one of a bottom up mode, where researchers, having previously agreed on the EOV's and the technology to be developed, made a value proposition on the benefits of joining the ARGO program to the corresponding national funding agency. Note that the costs of the program are mostly borne by developed nations, but the data are openly available to all.
Adaptive Management
The model to assess ocean health must be adaptive, flexible, and responsive to user needs to cope with dynamic and complex events since the syndromes impairing ocean health are dynamic. For instance, realization, a decade ago, that ocean acidification is a major challenge affecting marine calcifiers (Orr et al., 2005) led to a major impetus to monitor relevant properties, such as pH and the saturation state for carbonate minerals. A constant feedback approach should be adopted to evaluate, improve and refine the EOVs, indicators and the relevant syndromes and the management actions resulting from the assessment of ocean health (Figure 1). The adaptive management approach proposed (Figure 1) is a well-proven approach to manage natural resources (Schreibner et al., 2004), such as fisheries, applied in this context to manage ocean health as an asset supporting the flow of ecosystems services to society. The desired outcomes are better processes and more informed decisions to help ensure the health of the ocean in the presence of shifting pressures. Yet, the time required to detect a syndrome may be significant. For instance, changes attributable to climate change require time series of the order of 20 years (Parmesan et al., 2013), and time lags between managerial intervention to address a syndrome and recovery of the ecosystem may be long and is affected by shifting boundary conditions (e.g., Duarte et al., 2009). Hence, the system to assess ocean health must remain consistent over decadal time scales, and both scientists and the public need have expectations of the outcomes to be expected from adaptive management that are consistent with the time-scales for detection of responses imposed by ecosystem time lags and shifting baselines.
Effective Communication of the Assessments
The assessment of ocean health is not a requirement to progress in our understanding of the ocean ecosystem, which is based on testing more rigorous hypothesis and theories than the “ecosystem health” metaphor. Indeed, ocean health assessments are demanded by policy makers (e.g., UN, EU), who are the end users of these assessments. Policy-makers, managers and the general public are all interested in the “health of the ocean” at multiple levels and should be able to access the assessments at various levels of complexity and detail. This requires both an open data policy as well as a system for the effective communication of the outcome of the assessments of ocean health, in a synthetic, rigorous and accessible manner. Summarizing the outcome of environmental assessments has proved challenging in existing processes (Sterman, 2011), both in terms of adequately reflecting the complexity, nuances and limitation of the indicators used and in avoiding jargon and statements so qualified as to be devoid of content. Anticipating that the assessment may provide cause for concern on ocean health, ocean scientists should develop links with ocean users to ensure an understanding of need and partnerships with psychologists, sociologists, and other social scientists, to communicate the outcome of the assessments in ways that foster hope and action rather than denial and despair, as recommended to climate scientists (Sterman, 2008). A recent analysis of the challenges to communicate ocean health issues to the public (Schuldt et al., 2016) recommended that ocean health matters be framed in terms of their consequences for public health to increase public support for pro-environmental policy.
A Resilient Ocean Health Assessment System
The Global Ocean Observing System [GOOS, UN Global Ocean Observing System (GOOS)., 2011], a program run by the Intergovernmental Oceanographic Commission (IOC) of UNESO, has served over the last two decades as a very effective coordinating mechanism to identify essential components/indicators of the global climate system and develop systems, including innovative technology (e.g., ARGO floats, Roemmich et al., 2009) and global partnerships to collect the data supporting climate assessments. The expansion of GOOS to develop a system to deliver the observations and data required for the global assessment of ocean health is, therefore, an obvious step. Unfortunately, the IOC itself and the coordination for the programs it runs have been at times impacted by withdrawal of funding by some of the states, but has managed to maintain most programs in place. Resilient governance and support systems, dependent on an extensive network of committed partners, will be required to avoid such unexpected events delivering fatal impacts to an emerging program assessing ocean health globally.
As demands for the systematic and comprehensive assessment of ocean health grow, so has the recognition that the observational base available for most components of global ocean ecosystems is inadequate. Implementing the approach proposed here to develop a robust system to assess ocean health globally is an imperative as concern about the health of the oceans rises in parallel to awareness of the major role a healthy ocean plays in ensuring a sustainable future for humanity. The forthcoming OceanObs19 conference (http://www.oceanobs19.net), whose goal is to further develop effective strategies for a sustained, multidisciplinary and integrated ocean observing system, and to better connect user communities and observers, will provide an opportunity to discuss and consolidate the basis for the future assessment of Ocean Health, an effort that could be informed by the suggestions provided here.
Author Contributions
All authors listed have made a substantial, direct and intellectual contribution to the work, and approved it for publication.
Conflict of Interest Statement
The authors declare that the research was conducted in the absence of any commercial or financial relationships that could be construed as a potential conflict of interest.
Acknowledgments
This research was supported by funding from the Australian Institute of Marine Science, the UWA Oceans Institute and King Abdullah University of Science and Technology (KAUST) through baseline funding to CD.
References
Andersen, J. H., Dahl, K., Göke, C., Hartvig, M., Murray, C., Rindorf, A., et al. (2014). Integrated assessment of marine biodiversity status using a prototype indicator-based assessment tool. Front. Mar. Sci. 1:55. doi: 10.3389/fmars.2014.00055
Bohmann, K., Evans, A., Gilbert, M. T. P., Carvalho, G. R., Creer, S., Knapp, M., et al. (2014). Environmental DNA for wildlife biology and biodiversity monitoring. Trends Ecol. Evol. 29, 358–367. doi: 10.1016/j.tree.2014.04.003
Borja, A., Elliott, M., Snelgrove, P. V. R., Austen, M. C., Berg, T., Cochrane, S., et al. (2016). Bridging the gap between policy and science in assessing the health status of marine ecosystems. Front. Mar. Sci. 3:175. doi: 10.3389/fmars.2016.00248
Bourlat, S. J., Borja, A., Gilbert, J., Taylor, M. I., Davies, N., Weisberg, S. B., et al. (2013). Genomics in marine monitoring: new opportunities for assessing marine health status. Mar. Pollut. Bull. 74, 19–31. doi: 10.1016/j.marpolbul.2013.05.042
Butters, T. D., Guttel, S., Shapiro, J. L., and Sharpe, T. J. (2015). “Automatic real-time fault detection for industrial assets using metasensors,” in IET Conference Proceedings. (London, UK).
Constable, A. J., Costa, D. P., Schofield, O., Newman, L., Urban, E. R. Jr., Fulton, E. A., et al. (2016). Developing priority variables (“ecosystem Essential Ocean Variables” - eEOVs) for observing dynamics and change in Southern Ocean ecosystems. J. Mar. Syst. 161, 26–41. doi: 10.1016/j.jmarsys.2016.05.003
Costanza, R. (1992). “Ecosystem health” in New Goals for Environmental Management, eds R. Costanza, B. G. Norton, and B. D. Haskell (New York, NY: Island Press). 239–256.
Duarte, C. M. (2014). Global change and the future ocean: a grand challenge for marine sciences. Front. Mar. Sci. 1:63. doi: 10.3389/fmars.2014.00063
Duarte, C. M., Conley, D. J., Carstensen, J., and Sánchez-Camacho, M. (2009). Return to neverland: shifting baselines affect eutrophication restoration targets. Estuaries Coasts 32, 29–36 doi: 10.1007/s12237-008-9111-2
European Union (2008). Directive 2008/56/EC of the European Parliament and of the Council of 17 June 2008 Establishing a Framework for Community Action in the Field of Marine Environmental Policy (Marine Strategy Framework Directive). Marine Strategy Framework Directive of the European Union. Available online at: http://eur-lex.europa.eu/legal-content/EN/TXT/?uri=CELEX:32008L0056
Fischer, A. S., Hall, J., Harrison, D. E., Stammer, D., and Benveniste, J. (2010). “Conference summary-ocean information for society: sustaining the benefits, realizing the potential,” in Proceedings of OceanObs'09: Sustained Ocean Observations and Information for Society, Vol. 1, eds J. Hall, D. E. Harrison, and D. Stammer (Venice: ESA Publication), 23.
Fock, H. O., and Kraus, G. (2016). From metaphors to formalism: a heuristic approach to holistic assessments of ecosystem health. PLoS ONE 11:e0159481. doi: 10.1371/journal.pone.0159481
Gould, J., Roemmich, D., Wijffels, S., Freeland, H., Ignaszewsky, M., Jianping, X., et al. (2004). Argo profiling floats bring new era of in situ ocean observations. Eos Trans. Am. Geophys. Union 85, 185–191. doi: 10.1029/2004EO190002
Halpern, B. S., Frazier, M., Afflerbach, J., O'Hara, C., Katona, S., Stewart Lowndes, J. S., et al. (2017). Drivers and implications of change in global ocean health over the past five years. PLoS ONE 12:e0178267. doi: 10.1371/journal.pone.0178267
Halpern, B. S., Longo, C., Hardy, D., McLeod, K. L., Samhouri, J. F., Katona, S. K., et al. (2012). An index to assess the health and benefits of the global ocean. Nature 488, 615–620. doi: 10.1038/nature11397
Halpern, B. S., Longo, C., Lowndes, J. S. S., Best, B. D., Frazier, M., Katona, S. K., et al. (2015). Patterns and emerging trends in global ocean health. PLoS ONE 10:e0117863. doi: 10.1371/journal.pone.0117863
Heiskanen, A.-S., Berg, T., Uusitalo, L., Teixeira, H., Bruhn, A., Krause-Jensen, D., et al. (2016). Biodiversity in marine ecosystems—european developments toward robust assessments. Front. Mar. Sci. 3:184. doi: 10.3389/fmars.2016.00184
Inniss, L., Simcock, A., Ajawin, A. Y., Alcala, A. C., Bernal, P., Calumpong, H. C., et al. (2016). The First Global Integrated Marine Assessment World Ocean Assessment I. United Nations. Available online at: www.un.org/Depts/los/woa
Jackson, J. B., Kirby, M. X., Berger, W. H., Bjorndal, K. A., Botsford, L. W., Bourque, B. J., et al. (2001). Historical overfishing and the recent collapse of coastal ecosystems. Science 293, 629–637. doi: 10.1126/science.1059199
Jacobsen, J. B., and Hanley, N. (2009). Are there income effects on global willingness to pay for biodiversity conservation? Environ. Resour. Econ. 43, 137–160. doi: 10.1007/s10640-008-9226-8
Lindstrom, E. J., Gunn, A., Fischer, A., and McCurdy, and, L. K. (2012). A Framework for Ocean Observing. By the Task Team for an Integrated Framework for Sustained Ocean Observing, UNESCO 2012, IOC/INF-1284 rev.
Longhurst, A., Sathyendranath, S., and Platt, T. Caverhill, C. (1995). An estimate of global primary production in the ocean from satellite radiometer data. J. Plankton Res. 17, 1245–1271.
Longo, C. S., Frazier, M., Doney, S. C., Rheuban, J. E., Humberstone, J. M., and Halpern, B. S. (2017). Using the ocean health index to identify opportunities and challenges to improving southern ocean ecosystem health. Front. Mar. Sci. 4:20. doi: 10.3389/fmars.2017.00020
Mooney, H., Larigauderie, A., Cesario, M., Elmquist, T., Hoegh-Guldberg, O., Lavorel, S., et al. (2009). Biodiversity, climate change, and ecosystem services. Curr. Opin. Environ. Sustain. 1, 46–54. doi: 10.1016/j.cosust.2009.07.006
Muller-Karger, F. E., Miloslavich, P., Bax, N. J., Simmons, S., Costello, M. J., Sousa Pinto, I., et al. (2018). Advancing marine biological observations and data requirements of the complementary essential ocean variables (EOVs) and essential biodiversity variables (EBVs) frameworks. Front. Mar. Sci. 5:211. doi: 10.3389/fmars.2018.00211
Niemeijer, D. (2002). Developing indicators for environmental policy: data-driven and theory-driven approaches examined by example. Environ. Sci. Policy 5, 91–103. doi: 10.1016/S1462-9011(02)00026-6
Orr, J. C., Fabry, V. J., Aumont, O., Bopp, L., Doney, S. C., Feely, R. A., et al. (2005). Anthropogenic ocean acidification over the twenty-first century and its impact on calcifying organisms. Nature 437, 681–686. doi: 10.1038/nature04095
OSPAR (2007). EcoQO Handbook-Handbook for the Application of Ecological Quality Objectives in the North Sea. OSPARBiodiversity Series 2007/307, 39.
Parmesan, C., Burrows, M., Duarte, C. M., Poloczanska, E., Richardson, A., Schoeman, D., et al. (2013). Beyond climate change attribution in conservation and ecological research. Ecol. Lett. 16, 58–71. doi: 10.1111/ele.12098.
Rapport, D. J., Costanza, R., and McMichael, A. J. (1998). Assessing ecosystem health. Trends Ecol Evol. 13, 397–402.
Roemmich, D., Johnson, G. C., Riser, S., Davis, R., Gilson, J., Owens, W. B., et al. (2009). The Argo program: observing the global ocean with profiling floats. Oceanography 22, 34–43. doi: 10.5670/oceanog.2009.36
Samhouri, J. F., Lester, S. E., Selig, E. R., Halpern, B. S., Fogarty, M. J., Longo, C., et al. (2012). Sea sick? Setting targets to assess ocean health and ecosystem services. Ecosphere 3, 1–18. doi: 10.1890/ES11-00366.1
Schreibner, E. S. G., Bearlin, A. R., Nicol, S. J., and Todd, C. R. (2004). Adaptive management: a synthesis of current understanding and effective application. Ecol. Manag. Rest. 5, 177–182. doi: 10.1111/j.1442-8903.2004.00206.x
Schuldt, J. P., McComas, K. A., and Byrne, S. E. (2016). Communicating about ocean health: theoretical and practical considerations. Phil. Trans. R. Soc. B 371:20150214. doi: 10.1098/rstb.2015.0214
Sherman, K., and Hempel, G. (2009). “The UNEP large marine ecosystem report: a perspective on changing conditions in lmes of the world's regional seas,” United Nations Environment Programme Regional Seas Report and Stud- Ies (Nairobi), 872.
Sterman, J. D. (2008). Risk communication on climate: mental models and mass balance. Science 322, 532–533. doi: 10.1126/science.1162574
Sterman, J. D. (2011). Communicating climate change risks in a skeptical world. Clim. Change 108:811. doi: 10.1007/s10584-011-0189-3
Tett, P., Gowen, R. J., Painting, S. J., Elliott, M., Forster, R., Mills, D. K., et al. (2013). Framework for understanding marine ecosystem health. Mar. Ecol. Prog. Ser. 494, 1–27. doi: 10.3354/meps10539
Tu, Q., Yu, H., He, Z., Deng, Y., Wu, L., Van Nostrand, J. D., et al. (2014). GeoChip 4: a functional gene-array-based high-throughput environmental technology for microbial community analysis. Mol. Ecol. Resour. 14, 914–928. doi: 10.1111/1755-0998.12239
United Nations (2012). UN Oceans Compact. Available online at: http://www.un.org/depts/los/ocean_compact/SGs%20OCEAN%20COMPACT%202012-EN-low%20res.pdf
UN Global Ocean Observing System (GOOS). (2011). Available online at: http://www.ioc-goos.org
US Government (2010). Executive Order 13547 on the Stewardship of the Ocean, Our Coasts, and the Great Lakes. Available online at: https://obamawhitehouse.archives.gov/the-press-office/executive-order-stewardship-ocean-our-coasts-and-great-lakes
Uusitalo, L., Blanchet, H., Andersen, J. H., Beauchard, O., Berg, T., Bianchelli, S., et al. (2016). Indicator-based assessment of marine biological diversity–lessons from 10 case studies across the european seas. Front. Mar. Sci. 3:159. doi: 10.3389/fmars.2016.00159
Valentini, A., Taberlet, P., Miaud, C., Civade, R., Herder, J., Thomsen, P. F., et al. (2016). Next-generation monitoring of aquatic biodiversity using environmental DNA metabarcoding. Mol. Ecol. 25, 929–942. doi: 10.1111/mec.13428
World Bank and United Nations Department of Economic and Social Affairs (2017). The Potential of the Blue Economy: Increasing Long-term Benefits of the Sustainable Use of Marine Resources for Small Island Developing States and Coastal Least Developed Countries. Washington, DC: World Bank. Available online at: https://sustainabledevelopment.un.org/content/documents/15434Blue_EconomyJun1.pdf
Keywords: ocean health, ecosystem function, global change, observing systems, climate change
Citation: Duarte CM, Poiner I and Gunn J (2018) Perspectives on a Global Observing System to Assess Ocean Health. Front. Mar. Sci. 5:265. doi: 10.3389/fmars.2018.00265
Received: 14 February 2018; Accepted: 16 July 2018;
Published: 03 August 2018.
Edited by:
Jesper H. Andersen, NIVA Denmark Water Research, DenmarkReviewed by:
Alistair James Hobday, Commonwealth Scientific and Industrial Research Organisation (CSIRO), AustraliaSamuli Korpinen, Finnish Environment Institute, Finland
Copyright © 2018 Duarte, Poiner and Gunn. This is an open-access article distributed under the terms of the Creative Commons Attribution License (CC BY). The use, distribution or reproduction in other forums is permitted, provided the original author(s) and the copyright owner(s) are credited and that the original publication in this journal is cited, in accordance with accepted academic practice. No use, distribution or reproduction is permitted which does not comply with these terms.
*Correspondence: Carlos M. Duarte, Y2FybG9zLmR1YXJ0ZUBrYXVzdC5lZHUuc2E=