- 1Department of Earth, Ocean, and Atmospheric Science, Florida State University, Tallahassee, FL, United States
- 2Florida Fish and Wildlife Research Institute, St. Petersburg, FL, United States
- 3Department of Biology, University of Central Florida, Orlando, FL, United States
The environment and climate in which sea turtle eggs incubate affects how successful and viable hatchlings are. Therefore, it is crucial to understand how local climate impacts sea turtle hatchling production as well as how potential changes in climate may impact future hatchling production. In this study, we investigated the effects of five climate variables at different temporal scales on loggerhead sea turtle (Caretta caretta) hatchling production from North Florida, USA. Humidity, air temperature, and accumulated precipitation were the main climatic drivers of hatchling production, while sea surface temperature and wind speed did not demonstrate to have strong effects. Climate projections show air temperatures increasing at the nesting beaches throughout the 21st century, while precipitation and humidity projections vary between sites and projection scenarios. Due to the temperate nature of these nesting beaches, increases in hatching success for nests that incubate undisturbed (not affected by depredation and storm-related impacts) are projected for this region by 2100. This study demonstrates how different climate variables and their interactions can have a determining effect on an important marine species.
Introduction
Climate change is a major threat to biodiversity; changes in climate are already impacting on species distributions, physiology, phenology, behavior, and reproduction, with predictions for further impacts as climate change progresses (Walther et al., 2002; Root et al., 2003; Bellard et al., 2012). Sea turtles are thought to be particularly vulnerable to climate change (Hawkes et al., 2009; Hamann et al., 2013) since their reproductive success is highly dependent on the nest environment (Miller, 1985; Howard et al., 2014), with extreme temperatures and dry/saturated substrates being unfavorable for egg development (Foley et al., 2006; Best, 2017). Successful incubation of sea turtle eggs occurs within a narrow thermal range of 25–35°C (Miller, 1985; Ackerman, 1997), with different species and populations having different thermal tolerances (Howard et al., 2015). Despite this physiological plasticity, high temperatures have shown to be deleterious to the embryonic development and hatching success and emergence (Matsuzawa et al., 2002; Mickelson and Downie, 2010; Laloë et al., 2017). Likewise, humidity and precipitation can also influence egg survival and hatchling physiology so that hatchling production is reduced when the incubating environment is too moist or too dry (Ackerman, 1997; Katselidis et al., 2012; Santidrián Tomillo et al., 2012; Wyneken and Lolavar, 2015).
The effects of warming temperatures have already been observed through malformations in hatchlings, higher proportions of females being produced, and lower hatching success (Pike et al., 2006; Booth, 2017). As a result, there is concern over the potential impacts of projected increases in temperature on hatchling production and consequently population growth and stability (Fuentes et al., 2011; Santidrián Tomillo et al., 2012; Laloë et al., 2014). Most of the studies exploring the impacts of climate change on sea turtles have focused on the potential impacts on the primary sex ratio being produced (Houghton et al., 2007; Fuentes et al., 2009; Hays et al., 2017; Jensen et al., 2018). However, impacts of climate change on hatchling production (hatching success and emergence), and thus population stability, may be of greater concern (Santidrián Tomillo et al., 2015; Laloë and Hays, 2017). Studies investigating how hatchling production is influenced by climatic variables have often focused on the influences of air temperature (Santidrián Tomillo et al., 2012; Gasparrini et al., 2017). However, air temperature is one of several interacting climate variables, such as sea surface temperature, precipitation, and humidity, which influence the incubating environment (Fuentes et al., 2010b; Katselidis et al., 2012; Girondot and Kaska, 2015; Sifuentes-Romero et al., 2017) and consequently hatchling production (Lamont and Fujisaki, 2014; Lolavar and Wyneken, 2015; Santidrián Tomillo et al., 2015).
Considering the potential consequences of climate change on hatchling production, it is crucial to understand how embryonic development is influenced by the local climate and predict potential impacts as the climate continues to change. To expand from previous studies, here we explore the influences of five climatic variables (air and sea surface temperature, precipitation, humidity, and wind speed) on loggerhead sea turtle, Caretta caretta, hatchling production on temperate nesting grounds used by the Northern Gulf of Mexico (NGM) Recovery Unit that nest along the Florida Panhandle, USA. This allowed us to identify which variable(s) and combination of variables best predict hatchling production in North Florida. Additionally, we predicted potential impacts on future hatching success using extreme and conservative climate change scenarios throughout the 21st century.
Materials and Methods
Study Species and Location
Different sea turtle species are organized into units of protection known as Regional Management Units (Wallace et al., 2010). The focal species of this study was the loggerhead sea turtle of the NGM loggerhead Recovery Unit, one of the smallest Recovery Units in the United States (Shamblin et al., 2012), which is part of the Northwest Atlantic Regional Management Unit. Field work was conducted at T.H. Stone Memorial St. Joseph Peninsula (SJP) State Park and St. George Island (SGI) State Park, Florida, two of the most important nesting grounds in Florida for the NGM loggerhead Recovery Unit (Figure 1)1, with an average of 143 and 82 loggerhead nests a season, respectively. These sites were selected because they have relatively high nesting densities for loggerhead sea turtles in the NGM Recovery Unit, are relatively pristine being State Parks, and therefore add no confounding influence (e.g., human disturbance, sand compaction) on hatchling production. Both SJP and SGI are 23 km long, relatively flat, and open without vegetation.
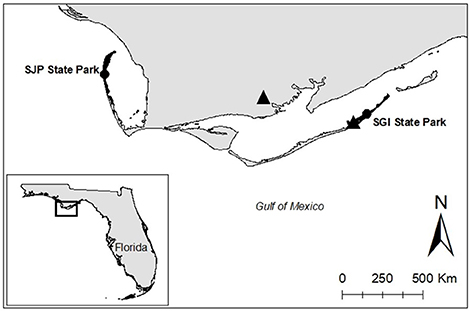
Figure 1. Study sites (west to east): T.H. Stone Memorial St. Joseph Peninsula (SJP) State Park and St. George Island (SGI) State Park are shaded black and are indicated with a black circle. Triangles represent weather stations used for this study.
Nest Data
Nest data (location, day laid, first date of emergence, inventory day) and hatchling production information (emergence rate = number of hatchlings that emerged from the nest in relation to the total hatched eggs, and hatching success = number of hatched eggs within a clutch) were obtained from the Florida Fish and Wildlife Research Institute, which obtains data from sea turtle patrol teams who collect information throughout Florida nesting beaches between March–October each year. Patrol teams collected loggerhead nest data during morning surveys conducted daily from 2013 to 2016. Variability in the data exists due to temporal distributions of nesting, depredation, and storm-related impacts (Table 1). All nests were left in situ. For analyses, we only considered nests that were excavated after hatching, had data on hatching success and emergence rate, and had no recorded disturbance (e.g., depredation and storm-related impacts).
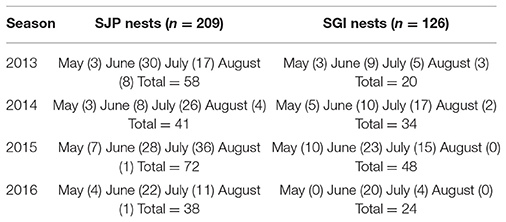
Table 1. Loggerhead sea turtle nest data from SJP and SGI considered in this study (n = 335) from 2013 to 2016, the number of nests considered is indicated in parenthesis.
Climate Data: Current and Projected
Local climate data for SGI from 2013 to 2016, were obtained from a weather station within the State Park, which collects daily averages for air temperature in degree Fahrenheit (°F), precipitation (in), and wind speed (mph). These values were converted to degrees Celsius (°C), millimeters (mm), and meters per second (m/s), respectively. Local climate data for SJP between 2013 and 2016 were obtained from the Apalachicola Municipal Airport, which is located 38.9 km from SJP. The Apalachicola Municipal Airport weather station records daily averages for air temperature (°F), humidity (%), precipitation (in), and wind speed (mph), which were converted to SI units. Humidity values from Apalachicola Municipal Airport were also used for SGI climate. This airport is located 27.8 km from SGI and 38.9 km from SJP. Skin temperature for an adjacent grid cell to the GPS coordinates for both locations were collected to eliminate influences from land surface measurements. Skin temperature refers to the temperature of the surface of the earth, which can be obtained via satellite (Gelaro et al., 2017). These values were obtained from the Modern-Era Retrospective analysis for Research and Applications, Version 2 (MERRA-2). MERRA-2 is a long-term meteorological reanalysis of space-based observations (Gelaro et al., 2017). Open water skin temperatures and sea surface temperatures differ by 0.2 K or less (Donlon et al., 2002); however open water skin temperature values used here will be considered sea surface temperatures (SST). Daily average values were computed from hourly averaged values. Open water skin temperature is skin temperature without terrestrial grid cells.
To explore how projected changes in climate may impact future hatchling production, we used projections for air temperature (°C), precipitation (mm), and humidity (%) from KNMI Climate Explorer (https://climexp.knmi.nl/start.cgi) centered on each State Park. We used multi-model means for all climate models under Coupled Model Intercomparison Project Phase 5 (CMIP5) and Representative Concentration Pathways (RCP) 8.5 and 4.5. The most extreme scenario, RCP8.5, predicts an extreme increase in global temperatures by the year 2100 due to continued increases in atmospheric concentrations of greenhouse gases (Meinshausen et al., 2011). RCP4.5 reflects a more conservative scenario where greenhouse gas concentrations stabilize, thus predicting a smaller increase in global air temperatures (Meinshausen et al., 2011).
Analysis
Hatching success and emergence rate proportions were transformed to arcsin values. A one-way ANOVA was used to compare the normalized hatching success and emergence rate between the State Parks and across seasons. Levene tests were used to test the homogeneity of variances for both hatching success and emergence rate.
To explore how local climate influences hatchling production, we used Generalized Linear Mixed—Effects Models, with the binomial family specified, in the lme4 package (Bates et al., 2018). To better fit these models, hatching success and emergence rate data were presented in successes and failures. In other words, hatching success consisted of number of eggs that hatched vs. those that did not hatch, while emergence rate consisted of the number of hatchlings that emerged vs. the number of hatchlings that did not from hatched eggs. The predictor variables used were: air temperature (temp), open water skin temperature (SST), accumulated rainfall (acc_rain), humidity (humid), and wind speed (wind). These predictors were explored at various temporal scales: the month nests were laid (0_climate variable), the month nests were laid and 1-month prior (0_1_climate variable), the month nests were laid and 2 months prior (0_2_climate variable), 2 months prior to nesting (2_climate variable), and during the incubation period (inc_climate variable). Previous research indicates that air temperature and precipitation have significant influences on hatchling production due to the variability that may exist in precipitation throughout the nesting season (i.e., dry and wet seasons) (Santidrián Tomillo et al., 2015); therefore, we chose to combine different precipitation predictors with average air temperature during incubation to explore their combined effects on hatchling production. There was a correlation between humidity and precipitation; therefore, we did not analyze the effects of air temperature in combination with humidity.
Corrected Akaike Information Criterion (AICc) was used to identify the best model at each State Park. The random effect used was year to allow for analysis and predictions to occur across and within years. We did not project emergence rate due to how impacted it can be by external factors that were not measured here (e.g., sand compaction, substrate type, etc.) (Mota, 2009). R version 3.4.2 was used for all analyses.
Results
Hatching Production
At SJP, 209 nests were considered for this study (36.5% of total loggerhead nests laid in 2013–2016), while at SGI 126 nests were considered (38.7% of total loggerhead nests laid in 2013–2016). Hatching success of undisturbed nests (not affected by predation and storm-related impacts) varied between both State Parks and across years, with a significant difference in hatching success between the State Parks [one-way ANOVA, F(23.7) = 6.95, DF = 1, p = 0.009] (Table 2, Figure 2A). SJP had a higher mean hatching success (87.3% ± 17.8 SD) than SGI (80.8% ± 26.4 SD; Table 2, Figure 2A). Emergence rate varied between sites and across years, with no significant difference in emergence rate between the sites [one-way ANOVA, F(21.7) = 0.009, DF = 1, p = 0.93) (Table 2, Figure 2B). SJP had a higher emergence rate as well, 84.9% ± 20.4 SD, while the mean emergence rate at SGI was 76.6% ± 28.5 SD (Table 2, Figure 2B).

Table 2. Average hatching success and emergence rate for undisturbed nests (not affected by predation and storm-related impacts), with their respective standard deviations and number of nests in parenthesis, at SJP and SGI throughout the study period.
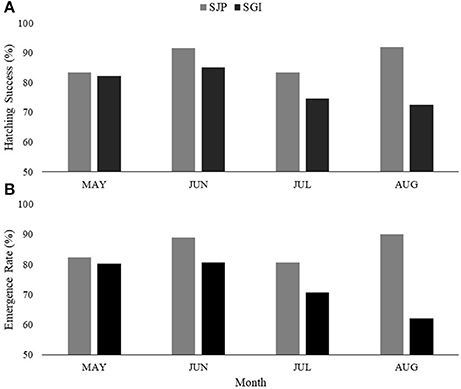
Figure 2. Average (A) hatching success and (B) emergence rate at T.H. Stone Memorial St. Joseph Peninsula (SJP) (gray) and St. George Island (SGI) (black) for each month (May–August) throughout the years (2013–2016) considered in this study.
Local Climate
The loggerhead nesting and hatching season in Florida occurs during the warmer and wetter months of the year (May–October). At both SGI and SJP, the hottest month is July (SGI = 29.03°C ± 1.5, SJP = 28.6°C ± 1.6), which is when the highest proportion of nests are laid at SJP (Figure 3A). SST is highest in August (SGI = 29.81°C ± 0.5, SJP = 29.84°C ± 0.5) when females have ended their nesting and hatchlings are still incubating and emerging (Figure 3C), The most humid month is September (79.9% ± 6.3) when there is no nesting, but hatchlings are still incubating and emerging (Figure 3D). The windiest month during the nesting season is May for SJP (3.3 m/s ± 0.9), which is the beginning of the nesting season (Figure 3E). Whereas, October is when wind speeds are highest in SGI (4.05 m/s ± 1.3) when there is no nesting and hatchlings are finishing their incubation (Figure 3E). SGI is the warmer and wetter of the two sites (Figure 3). Accumulated rainfall is at its highest in June at SGI (634.5 mm ± 9.1) and in July at SJP (633.5 mm ± 12.8; Figure 3B). At SGI, the highest proportion of nests occurs in June when accumulated precipitation is highest (Figure 3B).
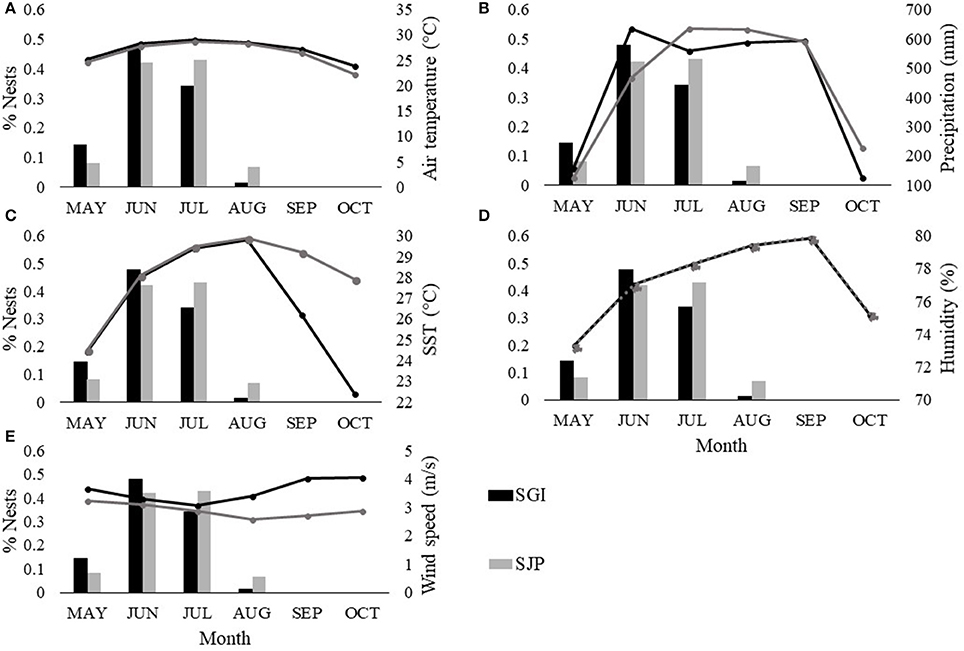
Figure 3. Monthly averaged climatic conditions (lines) at T.H. Stone Memorial St. Joseph Peninsula (SJP) (gray) and St. George Island (SGI) (black) and monthly proportions of nests laid (bars) between 2013 and 2016 at SJP (gray) and SGI (black). Climate variables include: (A) air temperature (°C), (B) accumulated rainfall (mm), (C) sea surface temperature (°C), (D) humidity (%), and (E) wind speed (m/s). Dotted line in (D) represents overlapping values. Between 2013 and 2016 at SJP, a total of 17 loggerhead nests were laid and left undisturbed in May, 90 in June, 90 in July, and 14 in August. At SGI, a total of 18 loggerhead nests were laid and left undisturbed in May, 44 in June, 41 in July, and 5 in August.
Climate Effects on Hatchling Production
At SJP, the model with the lowest AICc and high significance for hatching success was air temperatures during incubation (p < 0.001) in combination with accumulated precipitation during the month nests were laid (p < 0.001) (Figure 4A, Table S1 in Supplementary material). This model indicated that higher air temperatures and higher precipitation slightly increases hatching success at SJP throughout the study period (Figure 4A). The model with the lowest AICc and high significance for emergence rate at SJP was the average humidity during incubation (Figure 4C, Table S1 in Supplementary material). There was a strong negative and significant effect indicating high humidity values decreased emergence rate throughout the study period at SJP (p < 0.001; Figure 4C).
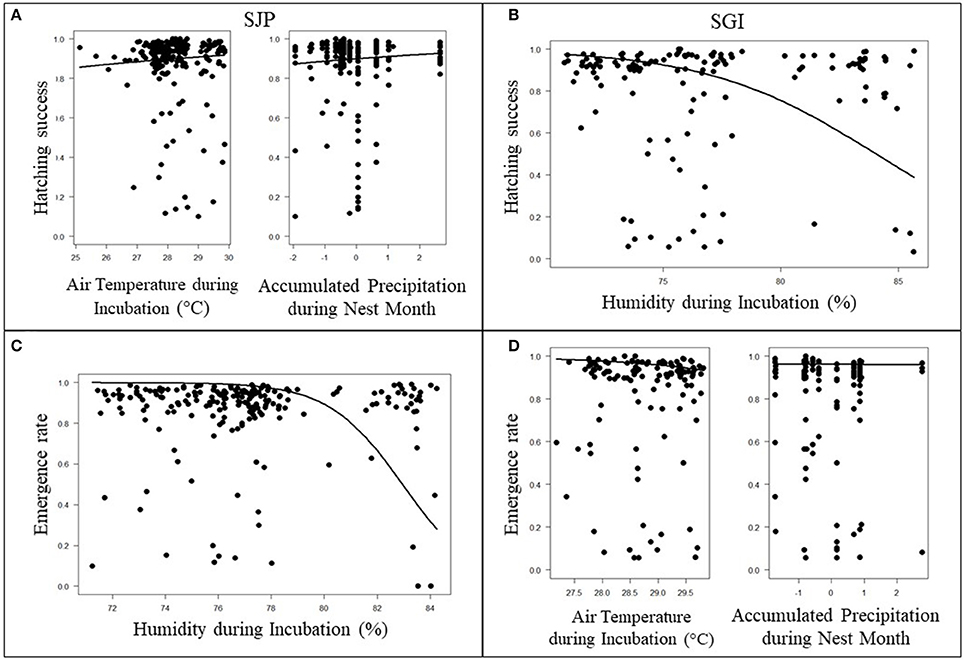
Figure 4. Effects of local climate on hatching success at (A) T.H. Stone Memorial St. Joseph Peninsula (SJP) and (B) St. George Island (SGI) and emergence rate at (C) SJP and (D) SGI are represented by the solid lines. Air temperatures during the incubation period and precipitation during the month the nest was deposited had a positive effect on hatching success at SJP (A). Humidity during the incubation period had a negative effect with hatching success at SGI (B) and emergence rate at SJP (C). Air temperatures and precipitation had a negative effect on emergence rate at SGI (D).
For hatching success at SGI, the lowest AICc with high significance was the average humidity throughout incubation (Figure 4B; Table S1 in Supplementary material). There was a strong negative and significant effect indicating higher humidity values decreasing hatchling success at SGI throughout the study period (p < 0.001; Figure 4B). The model with the lowest AICc and is significant for emergence rate at SGI was the average air temperature during incubation in combination with accumulated rainfall during the nest month (p < 0.001 for both parameters; Figure 4D, Table S1 in Supplementary material). Although the effect is small, it was significant and indicated that higher air temperatures and precipitation slightly decreases emergence rate at SGI throughout the study period (p < 0.001 for both parameters; Figure 4D).
Projections
Climate
At SJP by 2100, air temperatures are projected to increase throughout the nesting season by 1.3–4°C with August being the warmest month under RCP8.5 at 31.9°C (Figure 5A) and July being the warmest month under RCP4.5 at 30°C (Figure 5B). Depending on the scenario, precipitation is projected to decrease by 5.2–14 mm or increase by 2.1–36.8 mm by 2100 with the wettest month projected to be August under both RCP8.5 at 177.6 mm (Figure 5C) and RCP4.5 at 185 mm (Figure 5D). Depending on the scenario, humidity is projected to decrease by 0.1–1.5% or increase by 0.1–1.12% by 2100 with the most humid month projected to be September with 80.1% humidity under RCP8.5 (Figure 5E) and 80.6% under RCP4.5 (Figure 5F).
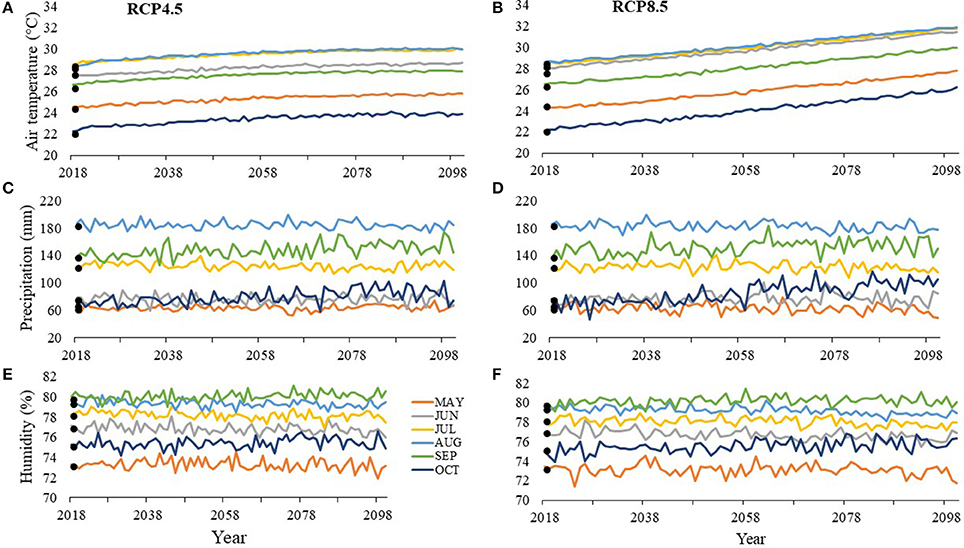
Figure 5. Average values between 2013 and 2016 (solid points) and projected values (lines) for (A, B) air temperature, (C, D) precipitation, and (E, F) humidity at T.H. Stone Memorial St. Joseph Peninsula (SJP) for months within the nesting and hatching season (May–October). RCP4.5 projections, where greenhouse gas concentrations stabilize, are on the left (A, C, E), while RCP8.5 projections, where greenhouse gas concentrations continuously increase, are on the right (B, D, F).
At SGI by 2100, air temperatures during the nesting season are projected to increase by 1.5–4.1°C with July being the warmest month under both RCP8.5 at 32.7°C (Figure 6A) and RCP4.5 at 30.78°C (Figure 6B). Depending on the scenario, precipitation is projected to decrease by 0.8–13.1 mm or increase by 7.3–33.9 mm with June being the wettest month under RCP8.5 at 160.1 mm by 2100 (Figure 6C) and August being the wettest month under RCP4.5 at 139.7 mm by 2100 (Figure 6D). Depending on the scenario, humidity is projected to decrease by 0.3–3.1% or increase by 0.1–0.8% by 2100 with the most humid month being September with 79% humidity under RCP8.5 (Figure 6E) and 80% under RCP4.5 (Figure 6F).
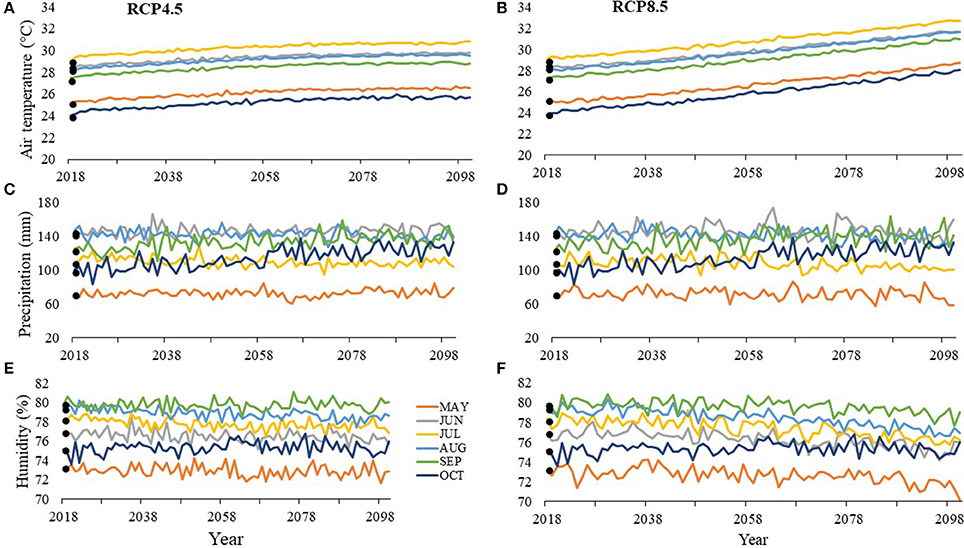
Figure 6. Average values between 2013 and 2016 (solid points) and projected values (lines) for (A, B) air temperature, (C, D) precipitation, and (E, F) humidity at St. George Island (SGI) for months within the nesting and hatching season (May–October). RCP4.5 projections, where greenhouse gas concentrations stabilize, are on the left (A, C, E), while RCP8.5 projections, where greenhouse gas concentrations continuously increase, are on the right (B, D, F).
Hatching Success Projections
Using the best fit models describing hatching success highlighted in Table S1 in Supplementary material and our climate projections during the nesting and hatching season (May–October), our models predicted the average yearly hatchling success for undisturbed nests (not affected by depredation and storm-related impacts) throughout the 21st century at SGI and SJP to increase. At SJP, hatching success was best described by the average air temperature during incubation in combination with accumulated precipitation during the month nests were laid. Hatching success at SJP is projected to increase, by 2100, from an average across the study period of 87.3 to 90.7% under RCP8.5 and to 88.3% under RCP4.5 (Figure 7A). Hatching success at SGI was best described by the average humidity during incubation. Hatching success at SGI is predicted to increase from a four-year average of 80.8 to 88.4% under RCP8.5 and to 86.1% under RCP4.5 (Figure 7B).
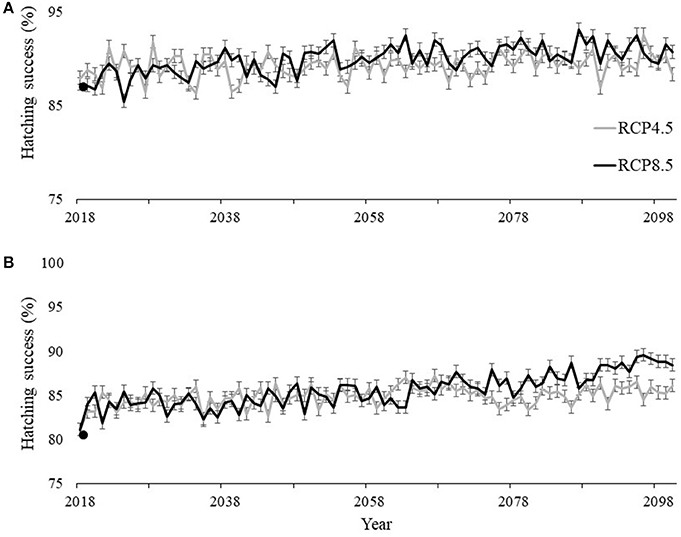
Figure 7. Historic average (solid points) and projected hatching success at (A) T.H. Stone Memorial St. Joseph Peninsula (SJP) and (B) St. George Island (SGI) under the extreme (black line) RCP8.5 scenario where greenhouse gas concentrations continuously increase and the more conservative (gray line) RCP4.5 scenario where greenhouse gas concentrations stabilize. Bars indicate 95% confidence intervals for each projected hatching success value up to 2100.
Discussion
In the context of climate change it is important to understand the complex relationships between climate variables and different species to be able to project how a species might be affected in the future (Duarte et al., 2012; Griffith et al., 2018; Rather et al., 2018). Here we provide new insights into how climatic variables affect an important marine megafauna. We show that humidity and air temperature during incubation in combination with accumulated precipitation during the month nests were laid, were found to be significant climatic drivers of loggerhead hatchling production at two high nesting density beaches within the NGM Recovery Unit. Average humidity during the incubation period had a strong negative effect on emergence rate at SJP and hatching success at SGI. These relationships may be a result of the high humidity levels in the region (73–80% throughout the nesting season), especially in August and September when hatchlings are toward the end of their incubation and emerging from nests. These humidity values are similar to those found at loggerhead nesting beaches in South Florida, such as Boca Raton (75–80%) (Lolavar and Wyneken, 2017). High humidity may make the environment too moist, potentially fostering fungal growth within nests decreasing hatching success due to infection (Sarmiento-Ramírez et al., 2010). Precipitation and air temperature had a positive effect at SJP, which could be a result of the nesting beach being too dry and/or too cool for successful incubation (Ackerman, 1997; Howard et al., 2014; Santidrián Tomillo et al., 2015). On the other hand, precipitation and air temperature had a negative effect on emergence rate at SGI, which is the warmer and wetter site. This negative relationship may be a result of extreme temperatures and precipitation causing pre-emergent mortality (Matsuzawa et al., 2002; Segura and Cajade, 2010). Here, we found hatchling production at both sites to be significantly influenced by both humidity and precipitation, highlighting the need to further understand the effects of moisture on the reproductive output of turtles at a regional level. Importantly, future studies exploring the effects of climatic variables on hatchling production should also consider in situ climatic data for nests so that more robust relationships can be obtained, to account for differences in nest location which may alter the effects of climatic conditions (e.g., vegetation cover, distance to high tide mark). Future studies can also benefit from further knowledge, of thermal thresholds for sea turtles and reaction norms of incubating eggs (Fuentes et al., 2017; Monsinjon et al., 2017; Girondot et al., 2018).
While recent advancements have been made to better understand how embryonic development and hatchling physiology are influenced by moisture content during incubation (Lolavar and Wyneken, 2017), less studied climate variables (e.g., SST, wind speed) may be interacting with the environment and also influencing reproductive output (Schofield et al., 2009; Katselidis et al., 2012; Best, 2017; Rivas et al., 2018). Although our study indicates that moisture and air temperature are the most significant climatic variables, models with sea surface temperature also had low AICc values. As climate change progresses, less studied variables will likely also change, thus influencing the spatial and temporal distributions of nesting and overall reproductive output (Young et al., 2011; Katselidis et al., 2012; Saba et al., 2016). Therefore, it is crucial to understand how the individual and synergistic effects of the local climate influence sea turtle reproductive output to more accurately predict potential future impacts and vulnerabilities and better inform management.
The beaches studied here experience a temperate climate regime and, thus, our projections show potential increases in their hatching success by 1–7.6% by 2100. Climate projections were not extrapolated but were in context of historical data. With most of the monthly projections predicting general decreases in humidity, within a tolerable range (70–81%), it is reasonable that our models projected increases in hatching success due to the negative relationship of humidity on hatching success in this region throughout the study period (2013–2016). However, the interaction between warming air temperatures and decreasing moisture levels may eventually make the incubating environment too dry and/or too warm for eggs to successfully incubate, potentially reducing developmental success and emergence as climate change progresses (Mortimer, 1990; Santidrián Tomillo et al., 2015; Almpanidou et al., 2016). Increases in temperature and declines in humidity and precipitation will likely reduce male production in the region, potentially causing a feminization of the hatchlings being produced. This may cause reduced fecundity or female infertility, a reduction in effective population size, and loss of genetic variation, with more extreme climate projections, causing the production of single sex cohorts (Wibbels, 2003; Mitchell et al., 2010; Fuller et al., 2013). Since temperatures in Northern Florida are cooler than other tropical beaches, and not close to lethal temperatures, short-term climatic changes will likely affect hatchling production in this region differently than in more tropical regions, which are already experiencing very warm and lethal temperatures (Hays et al., 2017; Bladow, 2018). Other temperate nesting grounds are also projected to experience increases in hatching success through the end of the 21st century due to the seasonality of these regions, making them more resilient to climate change than tropical beaches (Hawkes et al., 2007; Katselidis et al., 2012; Mazaris et al., 2013; Pike, 2014; Almpanidou et al., 2016). Indeed, tropical nesting beaches in the Caribbean, Central America, and Australasia are projected to experience decreases in hatchling production throughout the 21st century due to projected climate change (Pike, 2014; Santidrián Tomillo et al., 2015; Hays et al., 2017). The variability in how hatchling production from temperate and tropical nesting beaches may be affected by climatic changes highlights the need for focused research and conservation initiatives on temperate nesting grounds as they may become future havens of successful reproductive output (Katselidis et al., 2012; Almpanidou et al., 2016; Fuentes et al., 2016).
A potential adaptation to changes in climate by adult females is the shift of their nesting phenology to months of the year that become more suitable for incubation as climate change advances (Pike, 2009; Fuentes et al., 2011; Katselidis et al., 2012; Almpanidou et al., 2016). This shift in phenology has already been observed in central east Florida in response to warming temperatures (Weishampel et al., 2004), resulting in shorter nesting seasons with earlier nesting (Pike et al., 2006). Short nesting seasons are typical in temperate regions relative to tropical regions such as South Florida where the loggerhead nesting season begins in March. As climate change progresses, nesting beaches in North Florida may experience longer nesting seasons and potentially have higher nesting densities as turtles migrate poleward due to potentially less suitable nesting beaches at lower latitudes (Weishampel et al., 2004; Lamont and Houser, 2014; Fuentes et al., 2016). Leatherback and loggerhead sea turtles have already been observed migrating poleward in response to shifts in the 15°C isotherm (McMahon and Hays, 2006; Witt et al., 2010). However, nesting areas that have optimal climatic conditions for incubation may also be impacted by sea level rise, extreme weather events, increased inundation, erosion, and human development (Fuentes and Abbs, 2010; Fuentes et al., 2010a, 2016; Pike, 2013). Thus, there is importance in considering the synergetic and cumulative impacts of multiple threats to identify which beaches will be most resilient as climate change progresses (Fuentes et al., 2013).
Author Contributions
MF and NM conceived and designed the study NM, MF, SC, and KG performed the study, conducted analyses and wrote the paper. All authors gave final approval of the version to be published and agree to be accountable for all aspects of the work.
Conflict of Interest Statement
The authors declare that the research was conducted in the absence of any commercial or financial relationships that could be construed as a potential conflict of interest.
The reviewer JL and handling editor declared their shared affiliation at time of review.
Acknowledgments
We would like to thank all staff, volunteers, and interns who conducted sea turtle nest monitoring at both SGI State Park and T. H. Stone Memorial St. Joseph Peninsula (SJP) State Park during the 2013–2016 nesting seasons. We are grateful to Joshua Hodson and Mark Knapke, Park Manager for SGI and SJP (respectively), for authorizing the use of sea turtle nesting data for this study and to Beth Mongiovi, Florida Fish and Wildlife Research Institute, for her assistance with compiling the historical dataset. We would also like to thank Dr. Scott Burgess for his statistical guidance. Finally, we are thankful to the Leslie N. Wilson–Delores Auzenne Assistantship and the Earth, Ocean, and Atmospheric Science Winchester Fund for providing NM the funds needed to complete her graduate degree and thesis related to this manuscript.
Supplementary Material
The Supplementary Material for this article can be found online at: https://www.frontiersin.org/articles/10.3389/fmars.2018.00262/full#supplementary-material
Footnotes
References
Ackerman, R. A. (1997). “The nest environment and the embryonic development of sea turtles,” in The Biology of Sea Turtles, eds P. L. Lutz and J. A. Musick (Boca Raton, FL: CRC Press), 83–106.
Almpanidou, V., Schofield, G., Kallimanis, A. S., Türkozan, O., Hays, G. C., and Mazaris, A. D. (2016). Using climatic suitability thresholds to identify past, present and future population viability. Ecol. Indic. 71, 551–556. doi: 10.1016/j.ecolind.2016.07.038
Bates, D., Maechler, M., Bolker, B., Walker, S., Christensen, R. H. B., Singmann, H., et al. (2018). Package ‘lme4'. Linear Mixed-Effects Models using ‘Eigen' and S4. Madison, WI.
Bellard, C., Bertelsmeier, C., Leadley, P., Thuiller, W., and Courchamp, F. (2012). Impacts of climate change on the future of biodiversity. Ecol. Lett. 15, 365–377. doi: 10.1111/j.1461-0248.2011.01736.x
Best, Z. E. (2017). Environmental Factors Affecting Loggerhead Sea Turtle (Caretta caretta) Nesting, Hatching, and Incubation Patterns in Broward County, Florida. Master of Science. Thesis, Nova Southeastern University.
Bladow, R. (2018). “Sea turtle development in a changing climate: goldilocks temperatures are hard to find,” in Southeast Regional Sea Turtle Meeting, February, 2018 (Myrtle Beach, SC).
Booth, D. T. (2017). The influence of incubation temperature on sea turtle hatchling quality. Integr. Zool. 2017, 352–360. doi: 10.1111/1749-4877.12255
Donlon, C. J., Minnett, P. J., Gentemann, C., Nightingale, T. J., Barton, I. J., Ward, B., et al. (2002). Toward improved validation of satellite sea surface skin temperature measurements for climate research. J. Clim. 15, 353–369. doi: 10.1175/1520-0442(2002)015 < 0353:TIVOSS>2.0.CO;2
Duarte, H., Tejedo, M., Katzenberger, M., Marangoni, F., Baldo, D., Beltrán, J. F., et al. (2012). Can amphibians take the heat? Vulnerability to climate warming in subtropical and temperate larval amphibian communities. Glob. Change Biol. 18, 412–421. doi: 10.1111/j.1365-2486.2011.02518.x
Foley, A. M., Peck, S. A., and Harman, G. R. (2006). Effects of sand characteristics and innundation on the hatchling success of loggerhead sea turtle (Caretta caretta) clutches on low-relief mangrove islands in Southwest Florida. Chelonian Conserv. Biol. 5, 32–41. doi: 10.2744/1071-8443(2006)5[32:EOSCAI]2.0.CO;2
Fuentes, M. M. P. B., and Abbs, D. (2010). Effects of projected changes in tropical cyclone frequency on sea turtles. Mar. Ecol. Prog. Ser. 412, 283–292. doi: 10.3354/meps08678
Fuentes, M. M. P. B., Limpus, C. J., Hamann, M., and Dawson, J. (2010a). Potential impacts of projected sea-level rise on sea turtle rookeries. Aquat. Conserv. Mar. Freshw. Ecosystems 20, 132–139. doi: 10.1002/aqc.1088
Fuentes, M. M. P. B., Gredzens, C., Bateman, B. L., Boettcher, R., Ceriani, S. A., Godfrey, M. H., et al. (2016). Conservation hotspots for marine turtle nesting in the United States based on coastal development. Ecol. Appl. 26, 2706–2717. doi: 10.1002/eap.1386
Fuentes, M. M. P. B., Hamann, M., and Limpus, C. J. (2010b). Past, current and future thermal profiles of green turtle nesting grounds: implications from climate change. J. Exp. Mar. Biol. Ecol. 383, 56–64. doi: 10.1016/j.jembe.2009.11.003
Fuentes, M. M. P. B., Limpus, C. J., and Hamann, M. (2011). Vulnerability of sea turtle nesting grounds to climate change. Glob. Chang. Biol. 17, 140–153. doi: 10.1111/j.1365-2486.2010.02192.x
Fuentes, M. M. P. B., Maynard, J. A., Guinea, M., Bell, I. P., Werdell, P. J., and Hamann, M. (2009). Proxy indicators of sand temperature help project impacts of global warming on sea turtles in northern Australia. Endanger. Species Res. 9, 33–40. doi: 10.3354/esr00224
Fuentes, M. M. P. B., Monsinjon, J., Lopez, M., Lara, P., Santos, A., Dei Marcovaldi, M. A. G., et al. (2017). Sex ratio estimates for species with temperature-dependent sex determination differ according to the proxy used. Ecol. Modell. 365, 55–67. doi: 10.1016/j.ecolmodel.2017.09.022
Fuentes, M. M., Pike, D. A., Dimatteo, A., and Wallace, B. P. (2013). Resilience of marine turtle regional management units to climate change. Glob. Chang. Biol. 19, 1399–1406. doi: 10.1111/gcb.12138
Fuller, W., Godley, B., Hodgson, D., Reece, S., Witt, M., and Broderick, A. (2013). Importance of spatio-temporal data for predicting the effects of climate change on marine turtle sex ratios. Mar. Ecol. Prog. Ser. 488, 267–274. doi: 10.3354/meps10419
Gasparrini, A., Guo, Y., Sera, F., Vicedo-Cabrera, A. M., Huber, V., Tong, S., et al. (2017). Projections of temperature-related excess mortality under climate change scenarios. Lancet Planet. Health 1, e360–e367. doi: 10.1016/S2542-5196(17)30156-0
Gelaro, R., Mccarty, W., Suárez, M. J., Todling, R., Molod, A., Takacs, L., et al. (2017). The modern-era retrospective analysis for research and applications, version 2 (MERRA-2). J. Clim. 30, 5419–5454. doi: 10.1175/JCLI-D-16-0758.1
Girondot, M., and Kaska, Y. (2015). Nest temperatures in a loggerhead nesting beach in Turkey is more determined by sea surface than air temperature. J. Therm. Biol. 47, 13–18. doi: 10.1016/j.jtherbio.2014.10.008
Girondot, M., Monsinjon, J., and Guillon, J. M. (2018). Delimitation of the embryonic thermosensitive period for sex determination using an embryo growth model reveals a potential bias for sex ratio prediction in turtles. J. Therm. Biol. 73, 32–40. doi: 10.1016/j.jtherbio.2018.02.006
Griffith, G. P., Strutton, P. G., and Semmens, J. M. (2018). Climate change alters stability and species potential interactions in a large marine ecosystem. Glob Chang Biol. 24, e90–e100. doi: 10.1111/gcb.13891
Hamann, M., Fuentes, M. M., Ban, N., and Mocellin, V. (2013). “Climate change and marine turtles,” in The Biology of Sea Turtles, eds J. Wyneken, K. L. Lohmann, and J. A. Musick (Boca Ranton, FL: Taylor and Francis Group).
Hawkes, L. A., Broderick, A. C., Coyne, M. S., Godfrey, M. H., and Godley, B. J. (2007). Only some like it hot-quantifying the environmental niche of the loggerhead sea turtle. Divers. Distrib. 13, 447–457. doi: 10.1111/j.1472-4642.2007.00354.x
Hawkes, L. A., Broderick, A. C., Godfrey, M. H., and Godley, B. J. (2009). Climate change and marine turtles. Endanger. Species Res. 7, 137–154. doi: 10.3354/esr00198
Hays, G. C., Mazaris, A. D., Schofield, G., and Laloë, J. O. (2017). Population viability at extreme sex-ratio skews produced by temperature-dependent sex determination. Proc. Biol. Sci. 284:20162576. doi: 10.1098/rspb.2016.2576
Houghton, J. D. R., Myers, A. E., Lloyd, C., King, R. S., Isaacs, C., and Hays, G. C. (2007). Protracted rainfall decreases temperature within leatherback turtle (Dermochelys coriacea) clutches in Grenada, West Indies: ecological implications for a species displaying temperature dependent sex determination. J. Exp. Mar. Biol. Ecol. 345, 71–77. doi: 10.1016/j.jembe.2007.02.001
Howard, R., Bell, I., and Pike, D. A. (2014). Thermal tolerances of sea turtle embryos: current understanding and future directions. Endanger. Species Res. 26, 75–86. doi: 10.3354/esr00636
Howard, R., Bell, I., and Pike, D. A. (2015). Tropical flatback turtle (Natator depressus) embryos are resilient to the heat of climate change. J. Exp. Biol. 218, 3330–3335. doi: 10.1242/jeb.118778
Jensen, M. P., Allen, C. D., Eguchi, T., Bell, I. P., Lacasella, E. L., Hilton, W. A., et al. (2018). Environmental warming and feminization of one of the largest sea turtle populations in the world. Cur. Biol. 28, 154–159 doi: 10.1016/j.cub.2017.11.057
Katselidis, K. A., Schofield, G., Stamou, G., Dimopoulos, P., Pantis, J. D., and Katzner, T. (2012). Females first? past, present and future variability in offspring sex ratio at a temperate sea turtle breeding area. Anim. Conserv. 15, 508–518. doi: 10.1111/j.1469-1795.2012.00543.x
Laloë, J.-O., Cozens, J., Renom, B., Taxonera, A., and Hays, G. C. (2014). Effects of rising temperature on the viability of an important sea turtle rookery. Nat. Clim. Chang. 4, 513–518. doi: 10.1038/nclimate2236
Laloë, J.-O., Cozens, J., Renom, B., Taxonera, A., and Hays, G. C. (2017). Climate change and temperature-linked hatchling mortality at a globally important sea turtle nesting site. Glob. Chang. Biol. 2017, 1–10. doi: 10.1111/gcb.13765
Laloë, J.-O., and Hays, G. C. (2017). Can sea turtles cope with climate change? Austrl. Sci. 38, 17–19.
Lamont, M. M., and Fujisaki, I. (2014). Effects of ocean temperature on nesting phenology and fecundity of the loggerhead sea turtle (Caretta caretta). J. Herpetol. 48, 98–102. doi: 10.1670/12-217
Lamont, M. M., and Houser, C. (2014). Spatial distribution of loggerhead turtle (Caretta caretta) emergences along a highly dynamic beach in the northern Gulf of Mexico. J. Exp. Mar. Biol. Ecol. 453, 98–107. doi: 10.1016/j.jembe.2013.11.006
Lolavar, A., and Wyneken, J. (2015). Effect of rainfall on loggerhead turtle nest temperatures, sand temperatures and hatchling sex. Endanger. Species Res. 28, 235–247. doi: 10.3354/esr00684
Lolavar, A., and Wyneken, J. (2017). Experimental assessment of the effects of moisture on loggerhead sea turtle hatchling sex ratios. Zoology 123, 64–70. doi: 10.1016/j.zool.2017.06.007
Matsuzawa, Y., Sato, K., Sakamoto, W., and Bjorndal, K. (2002). Seasonal fluctuations in sand temperature: effects on the incubation period and mortality of loggerhead sea turtle (Caretta caretta) pre-emergent hatchlings in Minabe, Japan. Mar. Biol. 140, 639–646. doi: 10.1007/s00227-001-0724-2
Mazaris, A. D., Kallimanis, A. S., Pantis, J. D., and Hays, G. C. (2013). Phenological response of sea turtles to environmental variation across a species' northern range. Proc. R. Soci. B Biol. Sci. 280:20122397.doi: 10.1098/rspb.2012.2397
McMahon, C. R., and Hays, G. C. (2006). Thermal niche, large-scale movements and implications of climate change for a critically endangered marine vertebrate. Glob. Chang. Biol. 12, 1330–1338. doi: 10.1111/j.1365-2486.2006.01174.x
Meinshausen, M., Smith, S. J., Calvin, K., Daniel, J. S., Kainuma, M. L. T., Lamarque, J. F., et al. (2011). The RCP greenhouse gas concentrations and their extensions from 1765 to 2300. Clim. Change 109, 213–241. doi: 10.1007/s10584-011-0156-z
Mickelson, L. E., and Downie, J. R. (2010). Influence of incubation temperature on morphology and locomotion performance of leatherback (Dermochelys coriacea) hatchlings. Can. J. Zool. 88, 359–368. doi: 10.1139/Z10-007
Miller, J. D. (1985). “Embryology of marine turtles,” in Biology of the Reptilia, Vol. 14, eds C. Gans, F. Billett, and P. F. A. Maderson (New York, NY: Wiley Interscience), 271–328.
Mitchell, N. J., Allendorf, F. W., Keall, S. N., Daugherty, C. H., and Nelson, N. J. (2010). Demographic effects of temperature-dependent sex determination: will tuatara survive global warming? Glob. Chang. Biol. 16, 60–72. doi: 10.1111/j.1365-2486.2009.01964.x
Monsinjon, J., Jribi, I., Hamza, A., Ouerghi, A., Kaska, Y., and Girondot, M. (2017). Embryonic growth rate thermal reaction norm of mediterranean caretta caretta embryos from two different thermal habitats, Turkey and Libya. Chelonian Conserv. Biol. 16, 172–179. doi: 10.2744/CCB-1269.1
Mortimer, J. A. (1990). The influence of beach sand characteisitics on the nesting behavior and clutch survival of green turtles (Chelonia mydas). Copeia 1990, 802–817. doi: 10.2307/1446446
Mota, M. J. (2009). Beach Restoration in Florida: Effects on Sea Turtle Nesting and Hatchling Physiology. PhD. Dissertation, University of Florida.
Pike, D. A. (2009). Do green turtles modify their nesting seasons in response to environmental temperatures? Chelonian Conserv. Biol. 8, 43–47. doi: 10.2744/CCB-0726.1
Pike, D. A. (2013). Forecasting range expansion into ecological traps: climate-mediated shifts in sea turtle nesting beaches and human development. Glob. Chang. Biol. 19, 3082–3092. doi: 10.1111/gcb.12282
Pike, D. A. (2014). Forecasting the viability of sea turtle eggs in a warming world. Glob. Chang. Biol. 20, 7–15. doi: 10.1111/gcb.12397
Pike, D. A., Antworth, R. L., and Stiner, J. C. (2006). Earlier nesting contributes to shorter nesting seasons for the loggerhead seaturtle, Caretta caretta. J. Herpetol. 40, 91–94. doi: 10.1670/100-05N.1
Rather, R. N., Wani, A. A., Kashtwari, M., and Beigh, Z. A. (2018). Phenological shifts due to climate change and the associated conservation threats. Climate Change 4, 80–86.
Rivas, M. L., Spinola, M., Arrieta, H., and Faife-Cabrera, M. (2018). Effect of extreme climatic events resulting in prolonged precipitation on the reproductive output of sea turtles. Anim. Conserv. 2018, 1–9. doi: 10.1111/acv.12404
Root, T. L., Price, J. T., Hall, K. R., Schneider, S. H., Rosenzweig, C., and Pounds, A. J. (2003). Fingerprints of global warming on wild animals and plants. Nature 421, 57–60. doi: 10.1038/nature01333
Saba, V. S., Griffies, S. M., Anderson, W. G., Winton, M., Alexander, M. A., Delworth, T. L., et al. (2016). Enhanced warming of the Northwest Atlantic Ocean under climate change. J. Geophys.Res. Oceans 121, 118–132. doi: 10.1002/2015JC011346
Santidrián Tomillo, P., Saba, V. S., Blanco, G. S., Stock, C. A., Paladino, F. V., and Spotila, J. R. (2012). Climate driven egg and hatchling mortality threatens survival of eastern pacific leatherback turtles. PLoS ONE 7:e37602. doi: 10.1371/journal.pone.0037602
Santidrián Tomillo, P., Saba, V. S., Lombard, C. D., Valiulis, J. M., Robinson, N. J., Paladino, F. V., et al. (2015). Global analysis of the effect of local climate on the hatchling output of leatherback turtles. Sci. Rep. 5:16789. doi: 10.1038/srep16789
Sarmiento-Ramírez, J. M., Abella, E., Martín, M. P., Tellería, M. T., López-Jurado, L. F., Marco, A., et al. (2010). Fusarium solani is responsible for mass mortalities in nests of loggerhead sea turtle, Caretta caretta, in Boavista, Cape Verde. FEMS Microbiol. Lett. 312, 192–200. doi: 10.1111/j.1574-6968.2010.02116.x
Schofield, G., Bishop, C. M., Katselidis, K. A., Dimopoulos, P., Pantis, J. D., and Hays, G. C. (2009). Microhabitat selection by sea turtles in a dynamic thermal marine environment. J. Anim. Ecol. 78, 14–21. doi: 10.1111/j.1365-2656.2008.01454.x
Segura, L. N., and Cajade, R. (2010). The effects of sand temperature on pre-emergent green sea turtle hatchlings. Herpetol. Conserv. Biol. 5, 196–206.
Shamblin, B. M., Bolten, A. B., Bjorndal, K. A., Dutton, P. H., Nielsen, J. T., Abreu-Grobois, F. A., et al. (2012). Expanded mitochondrial control region sequences increase resolution of stock structure among North Atlantic loggerhead turtle rookeries. Mar. Ecol. Prog. Ser. 469, 145–160. doi: 10.3354/meps09980
Sifuentes-Romero, I., Tezak, B. M., Milton, S. L., and Wyneken, J. (2017). Hydric environmental effects on turtle development and sex ratio. Zoology 126, 89–97. doi: 10.1016/j.zool.2017.11.009
Wallace, B. P., Dimatteo, A. D., Hurley, B. J., Finkbeiner, E. M., Bolten, A. B., Chaloupka, M. Y., et al. (2010). Regional management units for marine turtles: a novel framework for prioritizing conservation and research across multiple scales. PLoS ONE 5:e15465. doi: 10.1371/journal.pone.0015465
Walther, G. R., Prost, E., Convey, P., Menzels, A., Parmesan, C., Beebee, T. J. C., et al. (2002). Ecological responses to recent climate change. Nature 416, 389–395. doi: 10.1038/416389a
Weishampel, J., Bagley, D. A., and Ehrhart, L. M. (2004). Earlier nesting by loggerhead sea turtles following sea surface warming. Glob. Chang. Biol. 10, 1424–1427. doi: 10.1111/j.1529-8817.2003.00817.x
Wibbels, T. (2003). “Critical approaches to sex determination in sea turtles,” in The Biology of Sea Turtles, Vol II. Marine Biology, eds P. Lutz, J. Musik, and J. Wynekan (CRC Press), 103–134.
Witt, M. J., Hawkes, L. A., Godfrey, M. H., Godley, B. J., and Broderick, A. C. (2010). Predicting the impacts of climate change on a globally distributed species: the case of the loggerhead turtle. J. Exp. Biol. 213, 901–911. doi: 10.1242/jeb.038133
Wyneken, J., and Lolavar, A. (2015). Loggerhead sea turtle environmental sex determination: implications of moisture and temperature for climate change based predictions for species survival. J. Exp. Zool. 324, 295–314. doi: 10.1002/jez.b.22620
Keywords: marine turtle, Caretta caretta, humidity, precipitation, temperate, reproductive output, emergence rate
Citation: Montero N, Ceriani SA, Graham K and Fuentes MMPB (2018) Influences of the Local Climate on Loggerhead Hatchling Production in North Florida: Implications From Climate Change. Front. Mar. Sci. 5:262. doi: 10.3389/fmars.2018.00262
Received: 26 May 2018; Accepted: 13 July 2018;
Published: 31 July 2018.
Edited by:
Graeme Clive Hays, Deakin University, AustraliaReviewed by:
Gail Schofield, Queen Mary University of London, United KingdomRichard Reina, Monash University, Australia
Jacques-Olivier LALOE, Deakin University, Warrnambool, Australia
Copyright © 2018 Montero, Ceriani, Graham and Fuentes. This is an open-access article distributed under the terms of the Creative Commons Attribution License (CC BY). The use, distribution or reproduction in other forums is permitted, provided the original author(s) and the copyright owner(s) are credited and that the original publication in this journal is cited, in accordance with accepted academic practice. No use, distribution or reproduction is permitted which does not comply with these terms.
*Correspondence: Mariana M. P. B. Fuentes, bWZ1ZW50ZXNAZnN1LmVkdQ==