- Laboratório de Ecologia Bentônica, Programa de Pós-Graduação em Ecologia e Biomonitoramento, CIENAM, Instituto de Biologia, Universidade Federal da Bahia, Salvador, Brazil
Predator-prey dynamics can affect assemblage structure and ecosystem processes representing a central theory in ecology. In coral reef ecosystems, recent evidences have suggested that sponge assemblages in regions with high diversity, like the Caribbean, are controlled by reef fishes (i.e., top-down control); however, this has been poorly studied in low diversity coral reefs. This study investigated the influence of fish predators on sponge assemblage structure in South Atlantic coral reefs, systems with high endemism and relatively low hermatypic coral diversity. We investigates (i) whether sponge cover is negatively correlated to spongivorous fish density, (ii) potential spongivory effects on competitive interactions between sponges and hermatypic corals, and (iii) foraging preferences of spongivorous fishes. Benthic cover and spongivorous fish density were assessed by photo sampling and visual census, respectively. We did not observe a negative correlation of the total density of spongivorous fish with total sponge cover. However, a significant negative correlation between density of fish species Pomacanthus arcuatus and cover of sponge species Scopalina ruetzleri was found. Spongivorous fish consumed preferentially the sponges Desmapsamma anchorata, Niphates erecta, Aplysina cauliformis, and S. ruetzleri, the first two species considered palatable and the second two with chemically defense mechanism. An increase to angelfish density was not related with the number of coral-sponge encounters. Thus, the effects of spongivorous fishes on sponges cover and competitive interactions with hermatypic corals is weaker in Southwestern Atlantic than previously reported in Caribbean coral reefs. We discuss how local human impacts (e.g., fishing and nutrients input) can influence the observed patterns.
Introduction
Predation is an important ecological process that impacts energy and matter flows in food chains. Food web dynamics has been proposed as one of the most important theories in ecology (Fretwell, 1987) and as the main regulatory mechanism of biological assemblage structure at local levels (Paine, 1966).
In marine systems, it is well-known that predator-prey interactions can affect the structure of biological assemblages and ecosystem functions (Hixon, 1991; Bellwood et al., 2004b). Furthermore, predation may reduce competitive exclusion by decreasing abundances of superior competitors (Paine, 1966; Hay, 1991). Competition for space is often an important regulator process in marine hard bottoms, as coral and rocky reefs, and influences patterns of abundance and diversity of biological assemblages (Connell et al., 2004; Chadwick and Morrow, 2011).
Within reef environments, generally sponges are better competitors for space than corals (Aerts, 1998; Pawlik, 2011), mostly due to their defense strategies (Wulff, 2006), as secondary metabolites, and overgrow ability (Aerts, 1998; Meurer et al., 2010). Sponges can negatively affect the recruitment and growth of hermatypic corals, as well as other important coral reefs processes (e.g., substrate construction) (Chadwick and Morrow, 2011). The effects of sponge competition on corals can be indirectly reduced by fish predation on sponges (i.e., fish spongivory) which may reduce competitive encounters between both taxa (Hill, 1998; Loh et al., 2015). Since Paine's work (Paine, 1966), it is widely accepted that the indirect effects of predation can have an influence on community structure, identifying and examining these effects is an important task in understanding community dynamics (Menge, 1995).
Sponges are frequently diverse and abundant in coral reef benthic communities, thus being a good model to evaluate predator-prey interactions (Dunlap and Pawlik, 1996; Ruzicka and Gleason, 2009). Sponges are key to ecosystem functioning because they are highly efficient filters, removing microbes and organic matter from water, transferring nutrient from pelagic to benthic habitats (Bell and Barnes, 2000; Wulff, 2001; de Goeij et al., 2013). Sponges contribute to complex balance between calcium carbonate loss or accretion, essential in the dynamics of coral reef growth (Wulff Buss and Buss, 1979; Wulff, 2001; Bell, 2008) and are an important food source for many marine organisms, especially for fishes (Randall and Hartman, 1968; Wulff, 1994).
Spongivory is mainly performed by angelfishes (Pomacanthidae family) of genera Pomacanthus and Holacanthus (Randall and Hartman, 1968; Carballo and Camacho, 2010), which are the most common and conspicuous sponge-feeding reef fishes around the world (e.g., Bellwood et al., 2004a). Different approaches have been used to investigate the potential effect of predation by reef fishes, for instance by using stomach content analysis (Randall and Hartman, 1968; Andréa et al., 2007; Reis et al., 2013) and fish feeding activity observations (Longo and Floeter, 2012; Pratchett et al., 2014). Although there is information about fish predation pressure on sponge assemblages (Pawlik et al., 2018), little is known about the spongivorous fish foraging behavior. Foraging activity is an essential aspect of habitat use by fishes and a predominant daily activity.
Evidences in high diversity coral reefs, such as Caribbean reefs, showed that top-down regulation by fish play a decisive role on growth of different sponge species, consequently affecting benthic assemblages (Dunlap and Pawlik, 1996; Pawlik, 1997, 1998; Pawlik et al., 2013, 2018). Brazilian coral reefs have peculiar features as relatively low coral diversity, high sedimentation rates and elevated turbidity due to abundant river flow into shore. These conditions have been considered marginal for coral optimal growth, so these reefs are known as marginal coral reefs (Leão et al., 2003; Suggett et al., 2012; Loiola et al., 2013). A small number of studies have conducted spongivorous fish stomach content analysis in rock (Andréa et al., 2007; Batista et al., 2012) or coral reefs (Reis et al., 2013) in Brazil and spongivorous feeding behavior was not previously investigated.
Here, we evaluate whether spongivory performed by angelfishes can influence the sponge assemblage structure and indirectly influence competitive encounter number between sponges and hermatypic corals (i.e., each contact found between different colonies, or encounter closer than 5 cm) in marginal coral reefs. To achieve this, we tested whether (1) sponge cover would be negatively related to angelfish density, (2) whether competitive encounter number between sponges and hermatypic corals would be negatively related to angelfish density and (3) sponge preferences by angelfishes during foraging activities. With (1) we investigated if top-down control would directly be acting, with (2) we considered a potential indirect effect of top-down control and with (3) we explored potential specific relationships between spongivorous fish and sponges.
Materials and Methods
Study Area
The study was conducted at Todos os Santos Bay (TSB), located in eastern coast of Brazil (Figure 1), a region with high endemism of coral species in the South Atlantic Ocean (Leão et al., 2003) and considered priority for conservation (Vila-Nova et al., 2014; Cruz et al., 2015). Coral reefs in TSB are located both in entrance (the outer reefs, occupying an area of 17.7 km2) and in its interior (the inner reefs, 13 km2) (Figure 1). The sponge assemblage are specially well-developed in inner reefs where are subject to several types of human interference (e.g., nutrient input, fishing) (Cruz et al., 2009). These interferences can contribute to changes in sponge assemblages by reducing of fish predators and increasing nutrients through discharge of domestic and industrial effluents. These reefs, at depths of ~2–13 m, consists of reef patches 20–60 m in length surrounded by fine sediments. Ten reef sites haphazardly selected in TSB (Supplementary Table 1), were sampled between January and March 2016 (Figure 1).
Benthic and Angelfishes Assemblages Surveys
We characterized sponge and coral assemblages using photo-quadrats (0.25 × 0.25 m). Two SCUBA divers haphazardly took 100 high resolution digital images (CANON G12 model, 3,648 × 2,736 pixels) on each of the 10 reef sites using a PVC frame at 40 cm distance from the substrate. Photo-quadrats (e.g., Supplementary Figure 1) were taken around 2 m of each other, resulting to a total sampled area of 6.25 m2 for each reef site. Benthic cover (%) were estimated through 20 randomly distributed points per photo-quadrat using Coral Counting Point with Excel Extensions Software (CPCe) (Kohler and Gill, 2006). These quadrats (n = 100) were used to sample the sponge assemblage and other benthic invertebrates at each site. Identification of sponges and benthic groups was performed in situ for each photo-quadrat. Whenever identification in the field was not possible, the specimen was collected, fixed in 80% ethanol and identified in the laboratory following available literature (Hajdu et al., 2011). The number of sponge-coral encounter (i.e., all sponges in contact with scleractinian corals and calcified hydroids colonies) was quantified in the photographs. We considered a natural encounter when one sponge was in contact with, or closer than 5 cm to, a coral colony (Chadwick and Morrow, 2011). Encounters were quantified in the entire area of each photo-quadrat and photos of each reef were pooled for analyses.
Densities of angelfish species Pomacanthus arcuatus, P. paru, and Holacanthus ciliaris were estimated by trained divers along ten 20 × 2 m transects (400 m2 per reef site) following fish monitoring protocol of the Atlantic and Gulf Rapid Reef Assessment (AGRRA) (Kramer and Lang, 2003).
Fish Foraging Activity
Focal animal method was used to measure angelfishes foraging activity (i.e., feeding rates and food preferences) (Bonaldo et al., 2006; Pereira et al., 2016). For each focal animal, angelfish specimen was followed and observed during 2 min intervals by SCUBA divers at 3 m distant from the fish, during this time we counted number of bites identifying sponge species. All occurrences were recorded on clipboards.
To avoid observation of the same specimens, each observation was conducted at least 5 m distant (Nunes et al., 2013). A total of 36 specimens of P. arcuatus, 12 P. paru, and 11 H. ciliaris were observed across all the reef sites, excepting Ilha de Maré where visibility was lower than 3 m. All observations were conducted between 09:00 and 15:00 h. We sampled spongivorous fish behavior in similar conditions of temperature and luminosity.
Data Analysis
To evaluate the relationship between angelfish density and (i) sponge cover and (ii) number of sponge-coral encounters, Spearman correlation coefficients were calculated with 95% confidence intervals and α = 0.05. For (i) we used means of angelfish density and means of sponge percentage cover on each reef and, for (ii) we used polled data (from all photo-quadrats of each reef) to test whether encounter would be negatively related to mean angelfish density and also used reefs as replicates. To evaluate the species-specific relationship of each angelfish species (three species, Pomacanthus paru, P. arcuatus, and Holacantus ciliares) mean density and their interactions with 8 sponge species mean cover (five sponge species selected by fishes observed in focal animal method and three most abundant found in the benthic data survey), multiple regressions using the stepwise model selection method were conducted with an α of 0.01, using each reef as a replicate. These analyses were performed using the R software (R Core Team, 2013): the packages pspearman and My.stepwise were used for Spearman correlation and stepwise multiple regression (Savicky, 2015; Hu, 2017), respectively.
The Ivlev's electivity index was used to verify angelfish preference or rejection by sponges that were bitten during foraging activity (focal animal). The electivity index was calculated, with pooled data from all reefs, as Ei = (ri – ni)/(ri + ni) where Ei is the value of electivity for the sponge species i; ri is the percentage of bites on the sponge i and ni is the sponge cover i across all locations. The electivity index varies from −1 to +1, where values near −1 indicate low preference or rejection while values near +1 indicate high preference for a particular sponge (Krebs, 1989). To generate a 95% confidence interval around the observed Ei, we used non-parametric bootstrapping procedures, in which fish samples were pooled for each reef and used as sample units (10,000 randomizations). The confidence limits were determined using the percentile method (Manly, 1997).
We used permutational multivariate analysis of variance (PERMANOVA, Anderson, 2001) to explore potential differences of fish and sponge assemblages between reef sites, based on the untransformed data with Bray-Curtis dissimilarities, using 9,999 random permutations. A pair-wise test was used to analyze which reef differs from each other. To visualize the spatial structure of both assemblages we used nonmetric multidimensional scaling (nMDS). These analyses were performed using the software PRIMER 6 (Clarke and Warwick, 2001).
Results
Benthic and Angelfish Assemblages
Overall sponge cover were 9.96 ± 0.76% (mean for all sites ± standard error), while hermatypic corals were 11.30 ± 2.25%, zoanthids 12.76 ± 6.34%, and turf algae 38.15 ± 3.93%. The Ilha de Maré reef site had the highest sponge cover 15.13 ± 0.51%, whereas Poste 1 had the lowest 7.3 ± 0.16% (Figure 2).
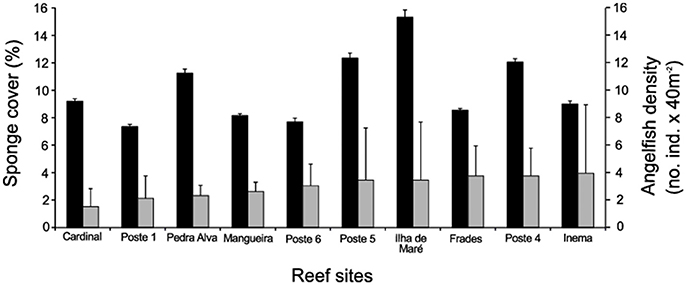
Figure 2. Mean (+s.e.) sponge cover (black bars) and mean angelfish density (gray bars) of 10 reef sites in Todos os Santos Bay.
The top five most abundant sponge species were Desmapsamma anchorata (3.8 ± 0.79%), Neopetrosia sp. (0.84 ± 0.22%), Clathria venosa (0.83 ± 0.16%), Aplysina cauliformis (0.78 ± 0.1%) and Ircinia strobilina (0.67 ± 0.24%) (Supplementary Figure 2A; Supplementary Table 2). The angelfish density was 2.96 ± 0.24 (fishes mean for all sites/40 m2 ± standard error). The highest angelfish density were observed at Inema (3.9 ± 4.82), Poste 4 (3.7 ± 2.0) and Frades Sul (3.7 ± 2.16) while Cardinal were the lowest (1.5 ± 1.2) (Supplementary Figure 2B). The most abundant species was P. arcuatus (1.92 ± 2.9) followed by H. ciliaris (6.0 ± 1.89) and P. paru (4.4 ± 1.39) (Supplementary Figure 2B).
Overall, in assemblage level, there was no significant relationship between angelfish density and sponge cover (Figure 3A). However, the species-specific relationship among P. arcuatus density and S. ruetzleri cover was significant (Figure 3B). PERMANOVA showed significant differences in the structure of sponge (p < 0.001) and angelfish assemblages (p < 0.001) between sites (Supplementary Tables 3–5; Supplementary Figure 3) showing a lot of spatial variability in the abundance of both groups along Todos os Santos Bay.
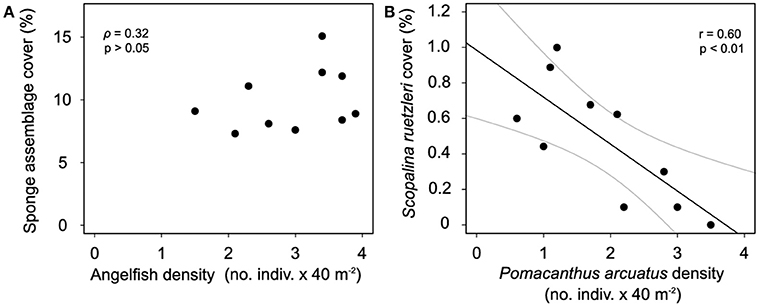
Figure 3. (A) Relation between mean percentage of sponge cover and mean angelfish density (ρ = 0.32, p > 0.05) on each reef and (B) relation between Scopalina ruetzleri and Pomacanthus arcuatus on each reef with best fit-line showing negative correlation (S. ruetzleri cover = 1.02–0.28 P. arcuatus density). Gray lines indicate 95% confidence intervals.
Foraging Activity
P. arcuatus had the highest bites rates (10.1 bites mean per 2 min ± 2.2, n = 36), followed by H. ciliaris (4.66 ± 2.19, n = 12) and P. paru (1.55 ± 0.69, n = 11). The electivity index showed that all three angelfish species selected D. anchorata during foraging activities (Figure 4). S. ruetzleri was selected by both P. arcuatus and H. ciliaris. In addition, P. arcuatus also selected other two sponge species Niphates erecta and A. cauliformis.
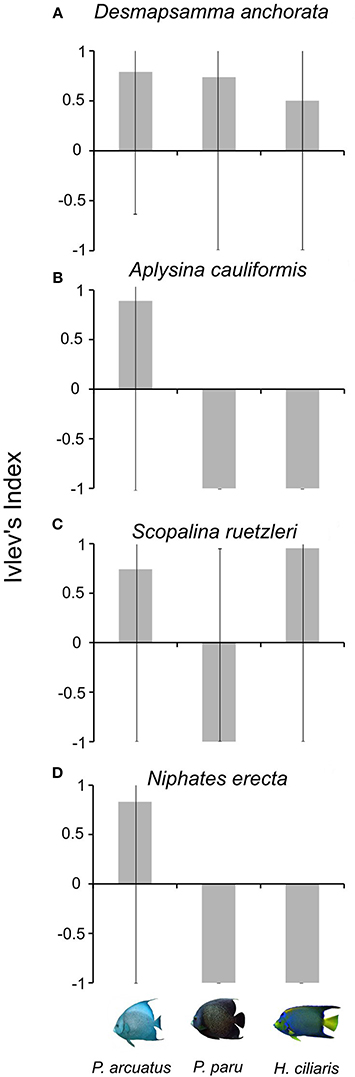
Figure 4. Ivlev's electivity index results of the spongivourous fish Pomacanthus arcuatus, Pomacanthus paru, and Holacanthus ciliaris for sponges (A–Desmapsamma anchorata, B–Aplysina cauliformes, C–Scopalina ruetzleri, D–Niphates erecta) bitten by at least one spongivourous fish. Lines indicate 95% confidence intervals.
Sponge-Coral Encounters
A total of 109 sponge-coral encounters were observed. The average sponge-coral encounters TSB was 12.6 ± 1.6 and the top three most abundant sponge species had the higher number of encounters with corals were D. anchorata (29%, n = 32), A. cauliformis (15%, n = 16) and C. venosa (12%, n = 13). The most frequent coral species encountered with sponges was Montastraea cavernosa (Supplementary Table 6). There was no significant relationship between sponge-coral encounters and angelfish density (ρ = 0.05; p = 0.8; Figure 5).
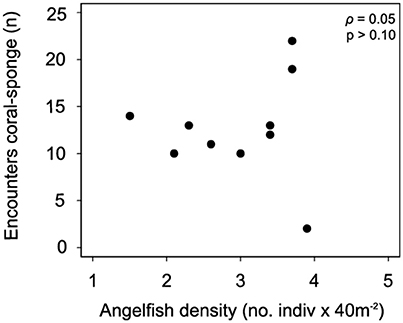
Figure 5. Relationship between total number of sponge-coral encounters and mean angelfish (spongivorous) density (ρ = 0.05; p = 0.8).
Discussion
Top-down or bottom-up control of sponge assemblages in coral reefs is an important contemporary discussion (e.g., Pawlik et al., 2018). An evidence for top-down control in TSB reefs would be if sponge cover in sites with higher angelfish density were smaller than in sites with low angelfish (spongivorous) densities. Concomitantly, there would be more competition between hermatypic corals and sponges in sites with smaller densities of angelfish. The results did not indicate a general negative relationship between abundances of angelfish and sponges and there was no significant relationship between coral-sponge encounters and angelfishes density. However, a significant species-specific negative relationship between P. arcuatus density and S. ruetzleri cover was observed. Additionally, both angelfish P. arcuatus and H. ciliaris selected S. ruetzleri during feeding activities. Thus, our results do not suggest a general top-down control of sponge assemblages but potential important species-specific relationships.
In Caribbean reefs sponge assemblages have been found to be primarily controlled by predatory reef fishes (Pawlik et al., 2013) and by hawksbill turtles (Pawlik et al., 2018). The biodiversity and abundance of spongivorous fishes and sponges in Caribbean reefs are considerably higher than in Southwestern Atlantic reefs (Roberts et al., 2002; van Soest et al., 2012). High predator species number and abundance could explain regional differences in ecosystem processes (Hooper et al., 2005; Floeter et al., 2008). For instance, biodiversity strongly influences ecosystem processes by altering pathways of energy and material flows due to functions performed by different groups of organisms at different systems (Hooper et al., 2005).
Local stressors such as sedimentation, nutrient inputs and overfishing also may contribute to reduce top-down effects decreasing fish predator density or favoring growing of certain sponges (Hughes, 1994; Roberts, 1995; Fabricius, 2005; Bell et al., 2015). High natural sedimentation rates intensified by human activities, can affect benthic sessile groups like sponges (Bell et al., 2015) and hermatypic corals (Fabricius, 2005). Nevertheless, some sponges can tolerate it, and in some cases thrive in turbid environments (Cerrano et al., 2007; de Voogd, 2012; Schönberg, 2015). The most abundant sponge species in TSB, D. anchorata, presented two important characteristics. First, it is able to incorporate free sediment particles that may give structural reinforcement to their body (Hajdu et al., 2011; Schönberg, 2016). Second, it can establish interactions with several organisms, mainly growing over other organisms with erect growth such the octocoral Carijoa riisei (Calcinai et al., 2004) and the branching coral Millepora alcicornis (McLean and Yoshioka, 2008). In fact, we observed contact between D. anchorata and M. alcicornis and M. cavernosa in the present study, potentially allowing D. anchorata to avoid sediment accumulation (McLean and Yoshioka, 2008; Schönberg, 2016). Sediment-incorporation by the sponge can also reduce the necessity of spicule formation (i.e., spiculogenesis) saving energy that can be allocated to other biological demands such as growth, reproduction and competition (Schönberg, 2016). In addition, D. achorata morphology can also occur as a branching form, avoiding sediment accumulation, with relatively high growth rates, that may accelerate the recovery of predation and competition damages (Wulff, 2006). These adaptations to high sediment conditions (e.g., branching morphology, fast growth rates and rapid regeneration) can explain the increased cover of D. anchorata in TSB reefs. The hypothesis of superior competitive abilities due to tolerance of natural and/or anthropogenic sedimentation can also be important to explain higher abundance of other sponge species. However, manipulative experiments are necessary to understand the role of competition in shaping sponge assemblages in TSB.
The relative high sponge cover found in TSB may be associated with availability of particulate food as picoplankton (Lesser, 2006; Lesser and Slattery, 2013) and dissolved organic carbon (DOC), both important resources used by sponges (de Goeij et al., 2013; Poppell et al., 2014; Pawlik et al., 2015, 2018). Local aspects of TSB such as natural and anthropic nutrient inputs and picoplankton availability may favor or control sponge assemblages. However, studies that suggested bottom-up control on sponge assemblages do not analyzed DOC as available resource (Lesser, 2006; Lesser and Slattery, 2013), not helping to sustain the argument (see review in Pawlik et al., 2015). Thus, in order to investigate what regulates sponge assemblages, futures studies must also evaluate nutritional aspects. From our results, considering the current status of TSB (e.g., anthropogenic impacts), the bottom-up control appears to have major influence on sponge assemblages in the area.
We observed a large variability in the structure of sponges and angelfishes between reefs. Unlikely these spatial differences are only caused by natural variables. But, we believe that these patterns are strongly influenced by multiple human activities (e.g., fishing, sedimentation, effluents) which also change in space and influence reef environmental quality in this bay (e.g., Cruz et al., 2014). For instance, several coral reefs in TSB have historically undergone anthropic impacts such as contamination by organic and inorganic pollution from domestic influxes and industrial effluents (e.g., Souza Santos et al., 2000; Barros et al., 2012; Hatje and Barros, 2012). High levels of nutrients can increase cover and abundance of some sponge species (Zea et al., 1994), although it has been shown as a factor that reduces sponge species diversity (Muricy, 1989). C. venosa, frequently found in TSB, can increase its abundance in sites with large organic contamination while S. ruetzleri, with relatively lower abundances in TSB, was suggested as sensible to organic pollution (Alcolado and Herrera, 1987).
A possible evidence that top-down control was operating in TSB was that S. ruetzleri had negative relationship with P. arcuatus abundance, showing a specific top-down control. Surprisingly, our results of electivity index showed that S. ruetzleri, previously considered chemically defended (Chanas and Pawlik, 1996), was selected by both P. arcuatus and H. ciliaris. The sponge D. anchorata was selected by all spongivorous fish, probably due to intense use (i.e., high bite rates) and high availability on coral reefs studied, being easily find by fish.
Marine fishes have been affected by many anthropogenic stressors such as overfishing and pollution (Pauly et al., 2002; Pereira et al., 2014) which can cause a decline on species richness and abundance (e.g., McKinley and Johnston, 2010). Overfishing is generally regarded as the major human activity impacting fish assemblages. In Brazil, several studies suggested that a large number of edible and ornamental fish, have been exploited, leaving significant changes in structure of fish assemblages (Costa et al., 2003; Gasparini et al., 2005; Floeter et al., 2006). For instance, angelfishes (Pomacanthus and Holacanthus genus) are often target of ornamental fishing in Brazil (Gasparini et al., 2005) and there are regulations for exploitation (Sampaio and Nottingham, 2008), however, the enforcement of such laws are usually not efficient.
Overfishing on Caribbean coral reefs increases the incidence of sponge-coral interactions, especially with sponges overgrowing corals (Loh et al., 2015), but we did not find a significant correlation between the number of encounters of sponge-coral and spongivorous fish density. Generally, D. anchorata was frequently observed in contact with corals (especially with M. cavernosa) in TSB. This sponge showed highest relative frequency of contact with corals also in Caribbean coral reefs, frequently overgrowing corals (Aerts and Van Soest, 1997).
The depletion of consumer fishes by overfishing may indirectly result in elevate prey abundance modifying the ecosystem structure and function according to trophic cascade theory (Pinnegar et al., 2000; Dulvy et al., 2004). Therefore, the top-down control of spongivory on sponge assemblages could also be weak on coral reefs at other Southwestern Atlantic reef sites. In TSB, coral reefs are under intense ornamental fishing and trade (Sampaio, 2006) and combined with organic matter influxes and other factors can result in dominances of non-reef build groups, as sponges (McClanahan et al., 2002; Maliao et al., 2008; Cruz et al., 2014). Furthermore, there is an urgent need to carefully evaluate regulations of fishing and implementation of management plans for TSB, as in many parts of the world.
The interactions between fishes and sponges and indirect effects on hermatypic corals could be a suitable model to evaluate coral reef integrity and functioning. We believe that the inclusion of fish-sponge-coral interactions to coral reef monitoring protocols will bring important insights of coral reef functioning and will allow the detection of annual or decadal changes. Nevertheless, ongoing coral reef monitoring programs are essential but will be of little use if the data are not available to support management actions and support investigations of different reef processes.
Ethics Statement
All applicable international, national, and/or institutional guidelines for the care and use of animals were followed.
Author Contributions
FL, RM, and JN hypotheses building, sample design, sampling, data analyses, writing. FB hypotheses building, sample design, data analyses, writing, financial support, supervision.
Funding
This work was supported by FAPESB (Baías da Bahia, PET0035/2012). FL and RM were supported by CAPES scholarships, JN (PDJ 437804/2016-0) and FB (PQ 306332/2014-0; 304907/2017-0) by CNPq.
Conflict of Interest Statement
The authors declare that the research was conducted in the absence of any commercial or financial relationships that could be construed as a potential conflict of interest.
Acknowledgments
We acknowledge I. F. Buda Andrade, T. Albuquerque, and R. Guimarães for help in the field. We thank G. Muricy, A. Leduc, and A. Reis for comments on earlier drafts of the manuscript, A. Tagliafico for manuscript review, C. Menegola for taxonomic expertise, Y. Costa and E. Mariano-Neto for the statistical advices, map formatting, and discussions. Thanks to all LEB (Laboratório de Ecologia Bentõnica) colleagues for the stimulating discussions.
Supplementary Material
The Supplementary Material for this article can be found online at: https://www.frontiersin.org/articles/10.3389/fmars.2018.00256/full#supplementary-material
Supplementary Figure 1. Examples of photo quadrats taken on different reefs of Todos os Santos Bay. Superior left: Frades Sul (Aplysina fistularis); Inferior left: Cardinal Ectyoplasia ferox); Superior right: Mangueira (Desmapsamma anchorata); Inferior right: Pedra Alva (Neopetrosia sp.).
Supplementary Figure 2. (A) Mean (+s.e.) of the five most abundant sponge species at each reef and (B) mean (+s.e.) density of the three spongivorous fish at each reef.
Supplementary Figure 3. nMDS showing similarities between reefs based on (A) sponge assemblages (% cover) and (B) spongivorous fish assemblages (densities).
Supplementary Table 1. Sampling sites coordinates and depth range. The fieldwork was conducted between January and March of 2016.
Supplementary Table 2. Mean percentage cover of the different taxa sampled by photo-quadrats in ten reefs of Todos os Santos Bay (MA, Mangueiras; IM, Ilha de Maré; P1, Poste 1; CA, Cardinal; IN, Inema; P4, Poste 4; P5, Poste 5; P6, Poste 6; PA, Pedra Alva; FS, Frades Sul).
Supplementary Table 3. Summary of PERMANOVA for sponge and fish assemblages based on Bray-Curtis dissimilarity with α = 0.05.
Supplementary Table 4. Par-wise testing for differences among reefs with sponge assemblages, based on Bray–Curtis dissimilarity with α = 0.05.
Supplementary Table 5. Par-wise testing for differences among reefs with angelfish assemblages, based on Bray–Curtis dissimilarity with α = 0.05.
Supplementary Table 6. Number of interactions among sponge species and hermatypic coral species.
References
Aerts, L. A. M. (1998). Sponge/coral interactions in Caribbean reefs: analysis of overgrowth patterns in relation to species identity and cover. Mar. Ecol. Prog. Ser. 175, 241–249. doi: 10.3354/meps175241
Aerts, L. A. M., and Van Soest, R. W. M. (1997). Quantification of sponge/coral interactions in a physically stressed reef community, NE Colombia. Mar. Ecol. Prog. Ser. 148, 125–134. doi: 10.3354/meps148125
Alcolado, P. M., and Herrera, A. (1987). Efectos De La Contaminación Sobre Las Comunidades De Esponjas En El Litoral De La Habana. Cuba: Instituto de Oceanologia, Academia de Ciencias de Cuba.
Anderson, M. J. (2001). A new method for non-parametric multivariate analysis of variance. Austral. Ecol. 26, 32–46. doi: 10.1111/j.1442-9993.2001.01070.pp.x
Andréa, B. R., Batista, D., Sampaio, C. L. S., and Muricy, G. (2007). “Spongivory by juvenile angelfish (Pomacanthidae) in Salvador, Bahia State, Brazil,” in Porifera Research: Biodiversity, Innovation and Sustainability, eds M. R. Custódio, G. Lôbo-Hajdu, E. Hajdu, and G. Muricy (Rio De Janeiro: UFRJ), 131–137.
Barros, F., de Carvalho, G. C., Costa, Y., and Hatje, V. (2012). Subtidal benthic macroinfaunal assemblages in tropical estuaries: generality amongst highly variable gradients. Mar. Environ. Res. 81, 43–52. doi: 10.1016/j.marenvres.2012.08.006
Batista, D., Muricy, G. R. S., Andréa, B. R., and Villaça, R. C. (2012). High intraspecific variation in the diet of the french angelfish Pomacanthus paru in the south-western Atlantic. Braz. J. Oceanogr. 60, 449–454. doi: 10.1590/S1679-87592012000300015
Bell, J. J. (2008). The functional roles of marine sponges. Estuar. Coast. Shelf. Sci. 79, 341–353. doi: 10.1016/j.ecss.2008.05.002
Bell, J. J., and Barnes, D. K. A. (2000). “A sponge diversity centre within a marine ‘Island',” in Island, Ocean and Deep-Sea Biology, eds M. B. Jones, J. M. N. Azevedo, A. I. Neto, A. C. Costa, and A. M. Frias Martins (Dordrecht: Kluwer Academic Publishers), 55–64.
Bell, J. J., McGrath, E., Biggerstaff, A., Bates, T., Bennett, H., Marlow, J., et al. (2015). Sediment impacts on marine sponges. Mar. Pollut. Bull. 94, 5–13. doi: 10.1016/j.marpolbul.2015.03.030
Bellwood, D. R., Hughes, T. P., Folke, C., and Nyström, M. (2004a). Confronting the coral reef crisis. Nature 429, 827–833. doi: 10.1038/nature02691
Bellwood, D. R., van Herwerden, L., and Konow, N. (2004b). Evolution and biogeography of marine angelfishes (Pisces: Pomacanthidae). Mol. Phylogenet. Evol. 33, 140–155. doi: 10.1016/j.ympev.2004.04.015
Bonaldo, R. M., Krajewski, J. P., Sazima, C., and Sazima, I. (2006). Foraging activity and resource use by three parrotfish species at Fernando de Noronha Archipelago, tropical West Atlantic. Mar. Biol. 149, 423–433. doi: 10.1007/s00227-005-0233-9
Calcinai, B., Bavestrello, G., and Cerrano, C. (2004). Dispersal and association of two alien species in the Indonesian coral reefs: the octocoral Carijoa riisei and the demosponge Desmapsamma anchorata. J. Mar. Biol. Assoc. U.K. 84, 937–941. doi: 10.1017/S0025315404010227h
Carballo, J. L., and Camacho, M. L. (2010). A qualitative assessment of sponge-feeding organisms from the Mexican Pacific coast. Open Mar. Biol. J. 4, 39–46. doi: 10.2174/1874450801004010039
Cerrano, C., Calcinai, B., Di Camillo, C. G., Valisano, L., and Bavestrello, G. (2007). “How and why do sponges incorporate foreign material? Strategies in Porifera” in Porifera Research: Biodiversity, Innovation And Sustainability, eds M. R. Custódio, G. Lôbo-Hajdu, E. Hajdu, and G. Muricy (Rio De Janeiro: UFRJ), 239–246.
Chadwick, N. E., and Morrow, K. M. (2011). “Competition among sessile organisms on coral reefs,” in Coral Reefs: An Ecosystem In Transition, eds Z. Dubinsky, N. Stambler (Dordrecht: Springer), 347–371.
Chanas, B., and Pawlik, J. (1996). Does the skeleton of a sponge provide a defense against predatory reef fish. Oecology 107, 225–231. doi: 10.1007/BF00327906
Clarke, K. R., and Warwick, R. M. (2001). Change in Marine Communities: An Approach to Statistical Analysis and Interpretation, 2nd Edn. Plymouth: PRIMER-E.
Connell, J. H., Hughes, T. P., Wallace, C. C., Tanner, J. E., Harms, K. E., and Kerr, A. M. (2004). A long-term study of competition and diversity of corals. Ecol. Monogr. 74, 179–210. doi: 10.1890/02-4043
Costa, P. A. S., da Costa Braga, A., and da Rocha, L. O. F. (2003). Reef fisheries in Porto Seguro, eastern Brazilian coast. Fish Res. 60, 577–583. doi: 10.1016/S0165-7836(02)00145-5
Cruz, I. C. S., Kikuchi, R. K. P., and Leão, Z. M. A. N. (2009). Characterization of coral reefs from Todos os Santos Bay protected area for management purpose, Bahia, Brazil. J. Integr. Coast Z. Manag. 9,16–36. doi: 10.5894/rgci150
Cruz, I. C. S., Kikuchi, R. K. P., Leão, Z. M. A. N., and Done, T. J. (2014). Reef quality criteria for marine reserve selection: an example from eastern Brazil. Aquat. Conserv. Mar. Freshw. Ecosyst. 25, 223–234. doi: 10.1002/aqc.2417
Cruz, I. C., Loiola, M., Albuquerque, T., Reis, R., Nunes, J. A. C. C., Reimer, J. D., et al. (2015). Effect of phase shift from corals to Zoantharia on reef fish assemblages. PLoS ONE 10:e0116944. doi: 10.1371/journal.pone.0116944
de Goeij, J. M., van Oevelen, D., Vermeij, M. J. A., Osinga, R., Middelburg, J. J., de Goeij, A. F. P., et al. (2013). Surviving in a marine desert: the sponge loop retains resources within coral reefs. Science 342, 108–110. doi: 10.1126/science.1241981
de Voogd, N. J. (2012). On sand-bearing myxillid sponges, with a description of Psammochela tutiae sp. nov. (Poecilosclerida, Myxillina) from the northern Moluccas, Indonesia. Zootaxa 3155, 21–28. doi: 10.5281/zenodo.208938
Dulvy, N. K., Freckleton, R. P., and Polunin, N. V. C. (2004). Coral reef cascades and the indirect effects of predator removal by exploitation. Ecol. Lett. 7, 410–416. doi: 10.1111/j.1461-0248.2004.00593.x
Dunlap, M., and Pawlik, J. R. (1996). Video-monitored predation by Caribbean reef fishes on an array of mangrove and reef sponges. Mar. Biol. 126, 117–123. doi: 10.1007/BF00571383
Fabricius, K. E. (2005). Effects of terrestrial runoff on the ecology of corals and coral reefs: review and synthesis. Mar. Pollut. Bull. 50, 125–146 doi: 10.1016/j.marpolbul.2004.11.028
Floeter, S. R., Halpern, B. S., and Ferreira, C. E. L. (2006). Effects of fishing and protection on Brazilian reef fishes. Biol. Conserv. 128, 391–402. doi: 10.1016/j.biocon.2005.10.005
Floeter, S. R., Rocha, L. A., Robertson, D. R., Joyeux, J. C., Smith-Vaniz, W. F., Wirtz, P., et al. (2008). Atlantic reef fish biogeography and evolution. J. Biogeogr. 35, 22–47. doi: 10.1111/j.1365-2699.2007.01790.x
Fretwell, S. D. (1987). Food chain dynamics: the central theory of ecology? Oikos 50, 291–301. doi: 10.2307/3565489
Gasparini, J. L., Floeter, S. R., Ferreira, C. E. L., and Sazima, I. (2005). Marine ornamental trade in Brazil. Biodivers. Conserv. 14, 2883–2899. doi: 10.1007/s10531-004-0222-1
Hajdu, E., Peixinho, S., and Fernandez, J. C. C. (2011). Esponjas Marinhas da Bahia: Guia de Campo e Laboratório. Rio de Janeiro: Museu Nacional.
Hatje, V., and Barros, F. (2012). Overview of the 20th century impact of trace metal contamination in the estuaries of Todos os Santos Bay: past, present and future scenarios. Mar. Pollut. Bull. 64, 2603–2614. doi: 10.1016/j.marpolbul.2012.07.009
Hay, M. E. (1991). “Fish-seaweed interactions on coral reefs: effects of herbivorous fishes and adaptations of their prey,” in The Ecology of Fishes on Coral Reef, ed P. F. Sale (San Diego, CA: Academic Press), 96–119.
Hill, M. S. (1998). Spongivory on Caribbean reefs releases corals from competition with sponges. Oecologia 117, 143–150. doi: 10.1007/s004420050642
Hixon, M. A. (1991). “Predation as a process structuring coral reef fish communities,” in The Ecology of Fishes on Coral Reefs, ed P. F. Sale (San Diego, CA: Academic Press), 475–508.
Hooper, D. U., Chapin, F. S., Ewel, J. J., Hector, A., Inchausti, P., Lavorel, S., et al. (2005). Effects of biodiversity on ecosystem functioning: a consensus of current knowledge. Ecol. Monogr. 75, 3–35. doi: 10.1890/04-0922
Hu, F. C. (2017). Stepwise Variable Selection Procedures for Regression Analysis. Package ‘My.stepwise'. Available online at: http://www.R-project.org/
Hughes, T. P. (1994). Catastrophes, phase shifts, and large-scale degradation of a Caribbean coral reef. Sci. Pap. Ed. 265, 1547–1551. doi: 10.1126/science.265.5178.1547
Kohler, K. E., and Gill, S. M. (2006). Coral point count with excel extensions (CPCe): a visual basic program for the determination of coral and substrate coverage using random point count methodology. Comput. Geosci. 32, 1259–1269. doi: 10.1016/j.cageo.2005.11.009
Kramer, P. R., and Lang, J. C. (2003). Appendix one: The Atlantic and Gulf Rapid Reef Assessment(AGRRA) protocols: former version 2. 2. Atoll. Res. Bull. 496, 611–624. doi: 10.5479/si.00775630.496-34.611
Leão, Z. M. A. N., Kikuchi, R. K. P., and Testa, V. (2003). Corals and coral reefs of Brazil. Lat. Am. Coral Reefs 1, 9–52.
Lesser, M. P. (2006). Benthic–pelagic coupling on coral reefs: feeding and growth of Caribbean sponges. J. Exp. Mar. Biol. Ecol. 328, 277–288. doi: 10.1016/j.jembe.2005.07.010
Lesser, M. P., and Slattery, M. (2013). Ecology of Caribbean sponges: are top-down or bottom-up processes more important? PLoS ONE 8:e79799. doi: 10.1371/journal.pone.0079799
Loh, T. L., McMurray, S. E., Henkel, T. P., Vicente, J., and Pawlik, J. R. (2015). Indirect effects of overfishing on Caribbean reefs: sponges overgrow reef-building corals. PeerJ 3:e901. doi: 10.7717/peerj.901
Loiola, M., Oliveira, M. D. M., and Kikuchi, R. K. P. (2013). Tolerance of Brazilian brain coral Mussismilia braziliensis to sediment and organic matter inputs. Mar. Pollut. Bull. 77, 55–62. doi: 10.1016/j.marpolbul.2013.10.033
Longo, G. O., and Floeter, S. R. (2012). Comparison of remote video and diver's direct observations to quantify reef fishes on benthos in coral and rocky reefs. J. Fish. Biol. 81, 1773–1780. doi: 10.1111/j.1095-8649.2012.03441.x
Maliao, R. J., Turingan, R. G., and Lin, J. (2008). Phase-shift in coral reef communities in the Florida Keys National Marine Sanctuary (FKNMS), USA. Mar. Biol. 154, 841–853. doi: 10.1007/s00227-008-0977-0
Manly, B. F. J. (1997). Randomization, Bootstrap and Monte Carlo Methods in Biology. London: Chapman & Hall.
McClanahan, T., Polunin, N., and Done, T. (2002). Ecological states and the resilience of coral reefs. Conserv. Ecol. 6:18. doi: 10.5751/ES-00461-060218
McKinley, A., and Johnston, E. L. (2010). Impacts of contaminant sources on marine fish abundance and species richness: a review and meta-analysis of evidence from the field. Mar. Ecol. Prog. Ser. 420, 175–191. doi: 10.3354/meps08856
McLean, E. L., and Yoshioka, P. M. (2008). Substratum effects on the growth and survivorship of the sponge Desmapsamma anchorata. Caribb. J. Sci. 44, 83–89. doi: 10.18475/cjos.v44i1.a9
Menge, B. A. (1995). Indirect effects in marine rocky intertidal interaction webs: patterns and importance. Ecol. Monogr. 65, 21–74. doi: 10.2307/2937158
Meurer, B. C., Lages, N. S., Pereira, O. A., and Palhano, S. (2010). First record of native species of sponge overgrowing invasive corals Tubastraea coccinea and Tubastraea tagusensis in Brazil. Mar. Biod. Rec. 3:e62. doi: 10.1017/S1755267210000527
Muricy, G. (1989). Sponges as pollution-biomonitors at Arraial do Cabo, Southeastern Brazil. Rev. Bras. Biol. 49, 347–354.
Nunes, J. D. A. C. C., Sampaio, C. L. S., and Barros, F. (2013). How wave exposure, group size and habitat complexity influence foraging and population densities in fishes of the genus Halichoeres (Perciformes: Labridae) on tropical rocky shores. Mar. Biol. 160, 2383–2394. doi: 10.1007/s00227-013-2233-5
Paine, R. T. (1966). Food web complexity and species diversity. Am. Nat. 100, 65–75. doi: 10.1086/282400
Pauly, D., Christensen, V., Guénette, S., Pitcher, T. J., Sumaila, R., Walters, C. J., et al. (2002). Towards sustainability in world fisheries. Nature 418, 689–695. doi: 10.1038/nature01017
Pawlik, J. R. (1997). “Fish predation on Caribbean reef sponges: an emerging perspective of chemical defenses,” in Proceedings of 8th International Coral Reef Symposium, eds H. A. Lessios and I. G. Macintyre (Panama City: Smithsonian Tropical Research Institute), 1255–1258.
Pawlik, J. R. (1998). Coral reef sponges: do predatory fishes affect their distribution? Limnol. Oceanogr. 43, 1396–1399 doi: 10.4319/lo.1998.43.6.1396
Pawlik, J. R. (2011). The chemical ecology of sponges on Caribbean reefs: natural products shape natural systems. Bioscience 61, 888–898. doi: 10.1525/bio.2011.61.11.8
Pawlik, J. R., Loh, T. L., and McMurray, S. E. (2018). A review of bottom-up vs. top-down control of sponges on Caribbean fore-reefs: what's old, what's new, and future directions. PeerJ 6:e4343. doi: 10.7717/peerj.4343
Pawlik, J. R., Loh, T. L., McMurray, S. E., and Finelli, C. M. (2013). Sponge communities on Caribbean coral reefs are structured by factors that are top-down, not bottom-up. PLoS ONE 8:e62573. doi: 10.1371/journal.pone.0062573
Pawlik, J. R., McMurray, S. E., Erwin, P., and Zea, S. (2015). A review of evidence for food limitation of sponges on Caribbean reefs. Mar. Ecol. Prog. Ser. 519, 265–283. doi: 10.3354/meps11093
Pereira, P. H. C., Moraes, R. L., dos Santos, M. V. B., Lippi, D. L., Feitosa, J. L. L., and Pedrosa, M. (2014). The influence of multiple factors upon reef fish abundance and species richness in a tropical coral complex. Ichthyol. Res. 61, 375–384. doi: 10.1007/s10228-014-0409-8
Pereira, P. H., Santos, M., Lippi, D. L., and Silva, P. (2016). Ontogenetic foraging activity and feeding selectivity of the Brazilian endemic parrotfish. Scarus zelindae. PeerJ 4:e2536. doi: 10.7287/peerj.preprints.2369v1
Pinnegar, J. K., Polunin, N. V. C., Francour, P., Badalamenti, F., Chemello, R., Harmelin-Vivien, M. L., et al. (2000). Trophic cascades in benthic marine ecosystems: lessons for fisheries and protected-area management. Environ. Conserv. 27, 179–200. doi: 10.1017/S0376892900000205
Poppell, E., Weisz, J., Spicer, L., Massaro, A., and Hill, M. (2014). Sponge heterotrophic capacity and bacterial community structure in high-and low-microbial abundance sponges. Mar. Ecol. 35, 414–424. doi: 10.1111/maec.12098
Pratchett, M. S., Hoey, A. S., Cvitanovic, C., Hobbs, J. P. A., and Fulton, C. J. (2014). Abundance, diversity, and feeding behaviour of coral reef butterflyfish at Lord Howe Island. Ecol. Evol. 4, 3612–3625. doi: 10.1002/ece3.1208
Randall, J. E., and Hartman, W. D. (1968). Sponge-feeding fishes of the West Indies. Mar. Biol. 1, 216–225. doi: 10.1007/BF00347115
R Core Team (2013). R: A Language and Environment for Statistical Computing. Vienna: R Foundation for Statistical Computing. Available online at: http://www.R-project.org/
Reis, F., Moraes, F., Batista, D., and Villaça, R. (2013). Diet of the queen angelfish Holacanthus ciliaris (Pomacanthidae) in São Pedro e São Paulo Archipelago, Brazil. J. Mar. Biol. Assoc. U. K. 93, 453–460. doi: 10.1017/S0025315412001099
Roberts, C. M. (1995). Effects of fishing on the ecosystem structure of coral reefs. Conserv. Biol. 9, 988–995. doi: 10.1046/j.1523-1739.1995.9051332.x-i1
Roberts, C. M., McClean, C. J., Veron, J. E. N., Hawkins, J. P., Allen, G. R., McAllister, D. E., et al. (2002). Marine biodiversity hotspots and conservation priorities for tropical reefs. Science 295, 1280–1284. doi: 10.1126/science.1067728
Ruzicka, R., and Gleason, D. F. (2009). Sponge community structure and anti-predator defenses on temperate reefs of the South Atlantic Bight. J. Exp. Mar. Bio. Ecol. 380, 36–46. doi: 10.1016/j.jembe.2009.08.011
Sampaio, C. L. S. (2006). Monitoramento da Atividade de Coleta de Organismos Ornamentais Marinhos na Cidade de Salvador, Bahia, Brasil. Ph.D. thesis, Universidade Federal da Paraíba, Paraíba.
Sampaio, C. L. S., and Nottingham, M. C. (2008). Guia Para Identificação de Peixes Ornamentais Brasileiros: Espécies Marinhas. Brasília: Ibama.
Savicky, P. (2015). Spearman's Rank Correlation Test. Package ‘pspearman’. Available onlione at: http://www.R-project.org/
Schönberg, C. H. L. (2015). Self-cleaning surfaces in sponges. Mar. Biodivers. 45, 623–624. doi: 10.1007/s12526-014-0302-8
Schönberg, C. H. L. (2016). Happy relationships between marine sponges and sediments–a review and some observations from Australia. J. Mar. Biol. Assoc. U. K. 96, 493–514. doi: 10.1017/S0025315415001411
Souza Santos, V. L. C., Raymundo, C. C., and Tavares, T. (2000). Isomers of the dodecylbenzene in marine sediments from the Todos os Santos Bay, Bahia, Brazil. Aquat. Ecosyst. Health Manag. 3, 479–484. doi: 10.1016/S1463-4988(00)00050-6
Suggett, D. J., Kikuchi, R. K. P., Oliveira, M. D. M., Spanó, S., Carvalho, R., and Smith, D. J. (2012). Photobiology of corals from Brazil's near-shore marginal reefs of Abrolhos. Mar. Biol. 159, 1461–1473. doi: 10.1007/s00227-012-1925-6
van Soest, R. W., Boury-Esnault, N., Vacelet, J., Dohrmann, M., Erpenbeck, D., Santodomingo, N., et al. (2012). Global diversity of sponges (Porifera). PLoS ONE 7:e35105. doi: 10.1371/journal.pone.0035105
Vila-Nova, D. A., Ferreira, C. E. L., Barbosa, F. G., and Floeter, S. R. (2014). Reef fish hotspots as surrogates for marine conservation in the Brazilian coast. Ocean Coast Manag. 102, 88–93. doi: 10.1016/j.ocecoaman.2014.09.005
Wulff, J. (2001). Assessing and monitoring coral reef sponges: why and how? Bull. Mar. Sci. 69, 831–846.
Wulff, J. L. (1994). “Sponge feeding by Caribbean angelfishes, trunkfishes, and filefishes,” in Sponges Time Space, eds R. W. M. van Soest, T. M. G. van Kempen, J. C. Braekman (Rotterdam: Balkema), 265–271.
Wulff, J. L. (2006). Ecological interactions of marine sponges. Can. J. Zool. 84, 146–166. doi: 10.1139/z06-019
Wulff, J. L., and Buss, L. W. (1979). Do sponges help hold coral reefs together? Nature 281, 474–475. doi: 10.1038/281474a0
Keywords: predation, angelfishes, porifera, sponge-coral interaction, top-down control, Todos os Santos Bay, reef ecology
Citation: Lorders FL, Miranda RJ, Nunes JACC and Barros F (2018) Spongivory by Fishes on Southwestern Atlantic Coral Reefs: No Evidence of Top-Down Control on Sponge Assemblages. Front. Mar. Sci. 5:256. doi: 10.3389/fmars.2018.00256
Received: 24 January 2018; Accepted: 09 July 2018;
Published: 07 August 2018.
Edited by:
Mario Barletta, Departamento de Oceanografia da Universidade Federal de Pernambuco (UFPE), BrazilReviewed by:
Thanos Dailianis, Hellenic Centre for Marine Research, GreeceJosé Lino Vieira De Oliveira Costa, Universidade de Lisboa, Portugal
Copyright © 2018 Lorders, Miranda, Nunes and Barros. This is an open-access article distributed under the terms of the Creative Commons Attribution License (CC BY). The use, distribution or reproduction in other forums is permitted, provided the original author(s) and the copyright owner(s) are credited and that the original publication in this journal is cited, in accordance with accepted academic practice. No use, distribution or reproduction is permitted which does not comply with these terms.
*Correspondence: Francisco Barros, ZnJhbmNpc2NvYmFycm9zLnVmYmFAZ21haWwuY29t