- 1Kepley Biosystems Incorporated, Greensboro, NC, United States
- 2High Point University, High Point, NC, United States
- 3Department of Biology, Columbia University, New York, NY, United States
- 4Joint School of Nanoscience and Nanoengineering, Greensboro, NC, United States
- 5ClienTell® Consulting, LLC, The Valley, Anguilla
Every year the Atlantic horseshoe crab (Limulus polyphemus) arrives on shore to spawn, a sight once taken for granted. However, in addition to the gradual climate changes impacting all ecosystems, commercial demand from the widespread application of Atlantic horseshoe crab blood in industrial endotoxin testing and steady use as eel and whelk bait has brought the future of this enduring species into question. In response, regulations have been adopted to enhance the traceability and record keeping of horseshoe crab harvest, which has historically been difficult to track. However, these regulations do not restrict or limit LAL harvest in any significant manner. Still, sometimes-lethal biomedical bleeding process and associated behavioral changes pose a risk to horseshoe crab viability after bleeding and once returned to the waters. As a result, regulators and environmentalists are concerned that current trends and overfishing of this marine arthropod will significantly impact the surrounding ecosystem. This review examines their role and recent trends in the biomedical industry that are impacting these ancient creatures and the derivative effect on shorebirds, while considering emerging alternatives where feasible, as well as ways to ensure sustainable and pragmatic harvesting strategies. Ultimately, healthy populations of horseshoe crabs are vital to restoring and maintaining ecosystems while balancing the need for medical and research applications entirely dependent on these unique creatures.
Introduction
The American horseshoe crab (Limulus polyphemus) is a valuable keystone species distributed across the Atlantic Coast of the United States and the Gulf of Mexico (Botton and Haskin, 1984; Botton and Ropes, 1989; Walls et al., 2002; Botton, 2009; Sekiguchi and Shuster, 2009). Horseshoe crabs play a key role in the eel and whelk fishing industry and an unparalleled, integral part in ensuring environmental safety and that of nearly every drug and medical device in use today (van Holde and Miller, 1995; Loveland et al., 1996).
This ancient aquatic arthropod, more closely related to scorpions and spiders than to crabs (Størmer, 1952), belongs to its own distinct class, Merostomata (Woodward, 1866), literally meaning “legs attached to mouth.” The name “horseshoe crab” is derived from the Limulus polyphemus' most recognizable features, its extended prosoma (or cephalothorax), a large shell that resembles a horseshoe (Figure 1). Commonly referred to as a “living fossil,” the horseshoe crab has been able to survive nearly unchanged for an estimated 200 million years (Walls et al., 2002; Kin and Blazejowski, 2014)–prior to recent population dynamics in the face of growing commercial demand. Concerted conservation and research efforts are vital for this species' future wellbeing. Improved or alternative biomedical harvest practices, environmental protection considerations and the suggested partnership of multiple stake-holding organizations are examined further, as discussed herein.
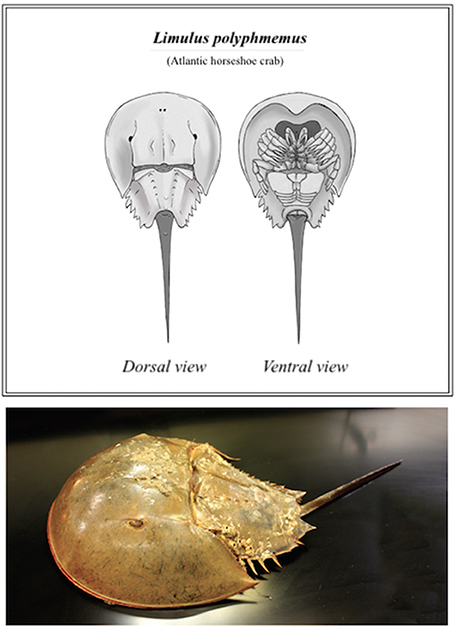
Figure 1. Basic anatomy of the horseshoe crab (L. polyphemus) (Top); and photograph of a mature female (Bottom).
Factors Affecting Horseshoe Crab Populations
Interest in the horseshoe crab has grown over last half century due to the distinctive nature of its blood and popular publications articulating the species link to migratory shorebirds. The animal's main commercial value is based on a substance found within its light blue blood (van Holde and Miller, 1995). Possessing an open circulatory system with no adaptive immune response, the horseshoe crab has survived through the ages by an “innate immunity” based on granular amebocytes, which comprise 99% of its hemocytes (Figure 2) (Shuster, 1978; Iwanaga et al., 1998; Medzhitov and Janeway, 2000). When these granular amebocytes come into contact with an endotoxin or 1,3ß-D-glucan (present in the cell walls of Gram-negative bacteria and fungi, respectively), a cascade of defense molecules is released, triggering coagulation and neutralization of the pathogens. The resulting clot effectively immobilizes the threat and prevents an infection from progressing beyond the wound (Isakova and Armstrong, 2003). While most recent research on horseshoe crabs has been focused on amebocytes and endotoxin detection (http://www.ncbi.nlm.nih.gov/pubmed/), some earlier studies have also yielded insights into human eyesight adaptation, the effect of circadian rhythms on vision, and the process by which sensory information is encoded (Hartline and McDonald, 1947; Barlow et al., 1977).
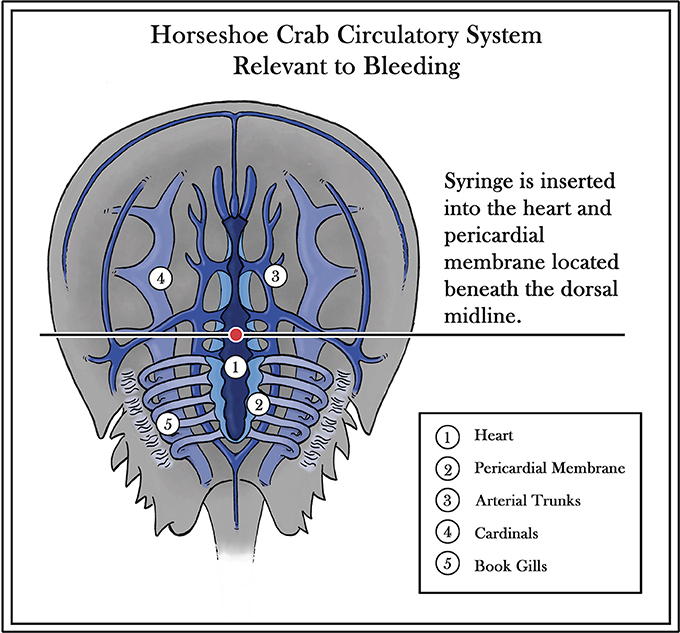
Figure 2. Diagram of horseshoe crab circulatory system (Diagram modified from Patten, 1912; copyright permission, reuse or modifications of Figure 2 are not required for this image as it is in the Public Domain and holds no copyright).
The unique ability of amebocytes to produce an instantaneous, visible reaction to endotoxins, in particular, has driven commercial demand from pharmaceutical and biomedical companies to confirm drug and medical device safety (Mikkelsen, 1988; Novitsky, 2009) using the Limulus amebocyte lysate (LAL) test, which has become the method of choice for endotoxin detection (Novitsky, 2009; Gauvry, 2015). These LAL test applications include quality assurance for: intravenous drugs; biologicals (e.g., clotting factors, insulin, and vaccines); recombinant drugs; and implantable medical devices (e.g., heart valves and orthopedic devices) (Novitsky, 2009). Environmental applications have also increased demand for the LAL test to ensure air quality and detect endotoxin concentrations in fresh water, sea water, and surrounding sediment (Novitsky, 2009).
Such vital benefits are nonetheless dependent on a crude LAL test manufacturing process; whereby the horseshoe crabs are captured, bled, and the collected blood is centrifuged to concentrate the amebocytes. Water is then added to the packed amebocytes, causing them to lyse and release coagulation proteins; thus, the “lysate” nomenclature.
Historically, horseshoe crabs have also been used apart from the extraction of blood for safety testing. They were once harvested for fertilizer and livestock feed; but this widespread practice ended in the 1920s, as the stock of horseshoe crabs began to decline and the public nuisance of the strong odor hastened the adoption of more competitive, alternative fertilizers (Walls et al., 2002). Thereafter, the use of horseshoe crabs as bait in commercial fishing became popular in the 1990s. Horseshoe crabs, particularly egg-bearing female crabs, proved to be an excellent bait for use in eel and whelk pots (Loveland et al., 1996). However, as the increasing biomedical industry requirements of horseshoe crab blood and the species link to migratory shorebirds viability were realized, new oversight agencies were established to mediate the risks from over-harvesting, and restrictions were placed on the number of horseshoe crabs collected for bait in order to regulate populations. These agencies further generated programs for stock management, developed state quota regulations, and established best practices for biomedical harvesting. In 2015, 583,208 horseshoe crabs were harvested as bait for eel and whelk (Atlantic States Marine Fisheries Commission, 2016), a significant reduction from the millions that were once harvested (Atlantic States Marine Fisheries Commission, 2013).
Impact of Amebocyte Harvesting on Horseshoe Crab Behavior and Physiology
The Atlantic States Marine Fisheries Commission (ASMFC) reported that in 2015, 559,903 horseshoe crabs were transported to biomedical facilities for the production of LAL (Atlantic States Marine Fisheries Commission, 2016). The raw materials for the preferred LAL test require careful extraction of blood from horseshoe crabs. Established methods entail introduction of a hypodermic needle placed directly into the exposed pericardial membrane of the horseshoe crab to draw from 50 to 400 mL of blood, depending on the sex and maturity of the horseshoe crab (Figure 2). The plasma is centrifuged, and LPS-free reagents, such as Na2EDTA or 3% NaCl, are added to help prevent clotting after extraction; this can occur as a result of the unintended introduction of endotoxins or other external factors, including undue stress during extraction and exposure to extreme temperatures. Careful handling of the horseshoe crab during bleeding, while maintaining the crab and blood at low and consistent temperatures, can help to prevent such coagulation issues (Armstrong and Conrad, 2008).
The harvest and collection procedure for bleeding horseshoe crabs may appear straightforward, but there are significant risks posed to the crabs at various stages of the process, ranging from transportation and crab storage, to the blood drawing itself. Horseshoe crab mortality rates following such harvesting range from 10 to 30%; however, these figures do not account for any further trauma and/or detrimental behavioral changes once the animals are returned to the ocean, nor the derivative population impact from the disruption of horseshoe crab spawning (Walls and Berkson, 2003; Anderson et al., 2013).
In fact, blood loss may not be the leading cause of death, but rather compounding factors, such as capture, handling and transportation. Biomedical harvest usually entails horseshoe crab collection from the bottom of the shallow seabed with dragging trawls and stacked on the bed of a boat before transferring them into plastic storage containers or bins for extended periods of time. During this process, crabs are crushed under the weight of other stacked crabs resulting in broken telsons and cracked shells, or accidentally impaled by the telsons of neighboring crabs. Each of these parameters must be evaluated when assessing overall mortality, rather than limiting the assessment to estimations. Prolonged survey and evaluation of recently bled horseshoe crabs could also provide more reliable extrapolation of morbidity if extended beyond the established 6-week assessment period.
A study evaluating these factors recorded no such sequelae in horseshoe crabs that were removed from the water only for the bleeding process and immediately returned, without being exposed to the impact of transport and storage (Hurton and Berkson, 2006). These “low stressed” crabs were observed to recover from removal of up to 40% of their estimated blood volume. However, when conventional horseshoe crab capture, transport, storage and handling procedures at biomedical bleeding facilities were simulated, the time spent out of the water and extent of exposure to elevated temperatures appeared to play a role in increased mortality. In controlled laboratory simulations, crabs exposed to capture, transport and holding stressors without bleeding, compared to those exposed to both bleeding and other external influences, yielded 2.6 vs. 8.3% mortality rates; while crabs losing the greatest percentage of blood along with capture, transport and storage exhibited the highest mortality rates overall. When both groups of crabs had 40% of their blood volume extracted, only 6% of non-stressed crabs perished vs. 15.4% of stressed crabs. (Hurton and Berkson, 2006). Thus, improved harvest practices have the potential to reduce mortality rates during biomedical harvest by more than half.
The stress from removing horseshoe crabs from the water during harvest may prove especially lethal. The horseshoe crab breathes through a set of gills and transports oxygen via hemocyanin (Towle and Henry, 2003). The primary function of the gills is to supply oxygen, not to remove CO2. Because carbon dioxide is water-soluble, it is easily removed when the animal is in an aquatic environment. However, when a crab is removed from the water, it is not able to efficiently remove CO2, and regulation of PCO2 results in abnormal hemolymph pH levels (Henry and Wheatly, 1992; Towle and Henry, 2003). While these animals can tolerate low oxygen environments based on various physiologic adaptations, such as a sharp decrease in heart rate and increased affinity of oxygen to hemocyanin (Towle and Henry, 2003); after removal from the water for only 5 min, they can develop severe hypoxia and metabolic acidosis. After 24 h of transportation out of water, horseshoe crabs have been shown to exhibit significantly diminished PO2 levels and extreme respiratory acidosis (Allender et al., 2010).
A review on the effects of hypoxic conditions on multiple marine organisms demonstrated that survival times are reduced by an average of 74% when an animal experiences hypoxia (Vaquer-Sunyer and Duarte, 2011). Accordingly, hypoxia has been associated with decreased stamina in hermit crabs (Mowles et al., 2009) and with altered fish migration patterns and distance (Ultsch, 1989). Assuming similar side effects are likely in horseshoe crabs, oxygen deprivation and the resultant disturbance in homeostasis has the potential to disrupt normal functions, such as spawning, even after the horseshoe crabs are returned to their natural habitat.
Exposing horseshoe crabs to high temperatures during capture and/or transportation has also been shown to negatively impact both blood quality and overall health (Coates et al., 2012). In a study to determine horseshoe crab response to varying temperatures, crabs held in the highest temperature (23°C) lost the most body weight and were among the only ones to expire. Hemocyanin and amebocyte concentrations were inversely proportional to temperature, with crabs held in the highest temperatures yielding the lowest concentrations. During the study, horseshoe crabs held in 18°C water yielded a 43.9% decrease in hemocyanin concentration, while those held in 23°C water showed a 69.3% decrease (Coates et al., 2012). Although the density of amebocytes decreased across all temperatures studied, the greatest decrease also occurred at the highest temperatures, with those held at 23°C yielding a decrease of 71.7%; which was also accompanied by notable morphological changes in the amebocytes.
Other, more nuanced behavioral changes brought about by the bleeding process have also been documented. Behavioral changes in horseshoe crabs have been observed for up to 2 weeks after harvesting (Anderson et al., 2013). The horseshoe crabs showed: slower walking; a 33–66% reduction in overall activity; and decreased expression of tidal rhythms, which dictate movement and spawning activity. Harvesting, in particular, may reduce spawning activity of females; which is especially problematic, since horseshoe crab harvest often takes place while spawning, when the crabs are easily accessible on the beach (Leschen and Correia, 2010). Upon habitat reintroduction, females have demonstrated markedly lethargic behavior and failed to spawn entirely (Anderson et al., 2013). This negative impact on the horseshoe crab population is further compounded by the high mortality rate of 30% following the bleeding of female horseshoe crabs regardless of pre- or post-spawning phases (Leschen and Correia, 2010); whereas, bleeding male horseshoe crabs has demonstrated a mortality rate of 8% (Walls and Berkson, 2003). In 2013, the reported mortality rate of horseshoe crabs harvested for solely biomedical purposes was 15%. However, when the number of crabs harvested, bled, sold by biomedical companies for bait, and counted against state bait quotas was factored in, the mortality rate jumped to 26% (Atlantic States Marine Fisheries Commission, 2013).
While research organizations continue to investigate the industry practices and associated effects of the horseshoe crab biomedical bleeding process, such studies have been largely dismissed or regarded as not following industry established Best Management Practices (BMP) in 2011 (Atlantic States Marine Fisheries Commission, 2014). Furthermore, some regulatory agencies have also asserted that such efforts would only be scientifically valid if all protocols were independently reviewed and approved by an advisory panel (Atlantic States Marine Fisheries Commission, 2010a). Notably, the current elected panel is predominantly comprised of stakeholders representing the agencies that control horseshoe crab biomedical assay commerce (Atlantic States Marine Fisheries Commission, 2013), suggesting that effective management strategies may be compromised by conflicting economic and environmental considerations.
Declining Populations of Horseshoe Crabs
Atlantic States Marine Fisheries Commission reports on horseshoe crab harvest mortality date back to 2004. From 2004 to 2012, the number of crabs delivered to biomedical bleeding facilities increased from 343,126 to 611,827, or by about 78%; while total mortality correspondingly increased by 75% (Atlantic States Marine Fisheries Commission, 2013). The percentage of horseshoe crabs that died prior to being bled more than doubled from 2008 to 2012 (Atlantic States Marine Fisheries Commission, 2013), which may be attributed to deleterious harvest and transportation practices. The maximum harvest mortality limit of 57,500 set by the ASMFC (based on the 15% mortality allowance) has been exceeded at times by more than 20,000 horseshoe crabs every year since 2007 (Atlantic States Marine Fisheries Commission, 1998, 2013). More recently, ASMFC data has estimated the mortality of horseshoe crabs harvested for the biomedical industry to be 70,000 (with a range of 23,000–140,000; Atlantic States Marine Fisheries Commission, 2016).
The cumulative effects of horseshoe crab harvest have also been well documented. An especially compelling example has been observed near Cape Cod at Mashnee Dike, where the spawning horseshoe crab count dwindled from around 3,000 to 148, representing a 95.3% decline over a 15-year period (1984–1999). Mashnee Dike was brought under the protection of the United States Army Corps of Engineers, and no external development in that area has been permitted since that time. As researchers have observed availability of a consistent food supply for the crabs (Widener and Barlow, 1999), human predation appears to be the primary cause of this collapse. Albeit not as severe, a Long Island-based study monitoring 68 sites showed horseshoe crab populations decreased just over 10%, or roughly one percent per year from 2003 to 2014 (Tanacredi and Portilla, 2015).
Reports from Delaware Bay and a few additional sites have cited modest horseshoe crab recoveries, but such examples have been the exception and seem to have been more than offset by shifting commercial activity to other geographic regions (Smith et al., 2009). Stricter horseshoe crab regulations around the Delaware Bay/New Jersey coastlines have led to increased harvesting in New England, where continued population declines were noted in a 2009 survey (Atlantic States Marine Fisheries Commission, 2013). As a result, regional management plans require coordination to ensure that horseshoe crabs are protected throughout their purview (Berkson et al., 2009).
The increased demands of the U.S. population, which is growing by 2.6 million people each year, and rapidly growing medical device and vaccine industries (Gauvry, 2015; Central Intelligence Agency, 2016) may not bode well for horseshoe crab populations. Based on current rates of horseshoe crab mortality and related population trends, over the next two decades, demand for the LAL test is likely to reach unsustainable levels. While horseshoe crab populations have moderately stabilized in some regions of the Atlantic, increases have also not been observed, which may be a result of negative behavioral or reproduction changes once the animals are returned to the ocean (Anderson et al., 2013) as well as deteriorating coastlines.
Global endotoxin detection is also dependent upon the TAL (Tachypleus amebocyte lysate) test produced in China, which is derived from the amebocytes of Tachypleus tridentatus and Tachypleus giga, Asian horseshoe crab species. Because these horseshoe crabs are often secondarily sold for human consumption or for the production of chitin after biomedical bleeding, resulting in a 100% mortality rate, population decline of these two species is a serious concern (Gauvry, 2015). While specific survey data are not available as in the U.S., decreased harvest quantities suggest an 83% drop in abundance (Gauvry, 2015). Unless China begins to regulate the harvest of T. tridentatus and T. giga, declining availability of the TAL test would be expected to increase demand for the LAL test throughout Asia (Gauvry, 2015).
Environmental Considerations
Sustaining the horseshoe crab population is also ecologically essential, as they play key roles as: bioturbators; hosts to a variety of epibionts on their shells; controllers of the population of many benthic invertebrates; and as a food source for a multitude of marine animals (Figure 3) (Botton and Haskin, 1984; Botton and Ropes, 1989; Walls et al., 2002; Botton, 2009). Barnacles, slipper limpets and blue mussels frequently live on the shells of horseshoe crabs, although the relationship is mostly neutral (Botton, 2009). Although they consume a broad, omnivorous diet, adult horseshoe crabs are important predators of benthic invertebrates, such as bivalves, polychaetes, crustaceans and gastropods, with a particular preference for thin-shelled bivalves, like small surf clams and blue mussels (Botton and Haskin, 1984; Botton and Ropes, 1989).
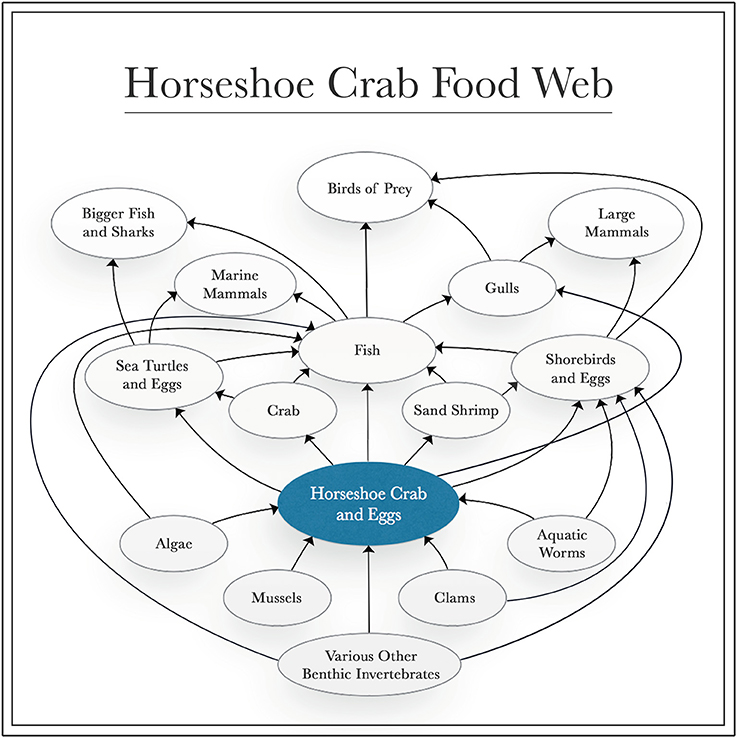
Figure 3. Diagram of feeding interactions of the horseshoe crab. Directions of arrows indicate impacts of each group on others through feeding interactions.
Shorebirds (e.g., red knots and ruddy turnstones), sand shrimp and fish (e.g., American eel, Atlantic silverside, catfish, devil ray, mullet, northern kingfish, silver perch, summer flounder, striped bass, swordfish, weakfish, white perch and winter flounder) consume horseshoe crab eggs and larvae (Warwell, 1897; Perry, 1931; Price, 1962; Spraker and Austin, 1997; Walls et al., 2002). In turn, crabs (e.g., blue, fiddler, green and hermit crabs) and pufferfish eat juvenile horseshoe crabs (Walls et al., 2002; Botton, 2009).
Mature horseshoe crabs are not significantly threatened by natural predators due to their large size and thick shell, but some have been identified (Walls and Berkson, 2003). For example, large American alligators have been observed eating adult horseshoe crabs in the Indian River Lagoon in Florida on several occasions, and leopard sharks have occasionally consumed them, as well (Reid and Bonde, 1990; Walls et al., 2002). Whenever adult horseshoe crabs are upturned and stranded on the beach, herring and black-backed gulls typically eat them, removing the gills and legs in order to access eggs and internal organs (Botton and Loveland, 1993; Walls et al., 2002). However, loggerhead turtles (listed as threatened by the U.S. Endangered Species Act) are the most common predator of mature horseshoe crabs, which have comprised a significant portion of these turtles' stomach contents when found in the lower Chesapeake Bay (Keinath, 2003).
That said, in the mid-1980s the diet of loggerhead turtles in Virginia was dominated by horseshoe crabs, before transitioning to blue crabs in the 1980s, and more recently to finfish (Seney and Musick, 2007). These shifts are believed to have been caused by the decline in horseshoe and blue crab populations. The drop in these two populations may also correlate to the overall decrease in the number of sea turtles in the Chesapeake Bay over the past few decades (Botton, 2009). In one survey, the sea turtle density in the lower Delaware Bay was comparable to the density of sea turtles in the lower Chesapeake Bay (Spotila et al., 2007), indicating the possibility that loggerheads in this area also feed on horseshoe crabs (Botton, 2009). While few such observations have been published to conclude whether many other species of sea turtles also consume horseshoe crabs, one of the most endangered, Kemp's Ridley turtles, have been observed eating them (Servis et al., 2015).
Shorebirds as Bellwethers
The spawning of horseshoe crabs in the Delaware Bay occurs between May and June, with 70% occurring during the first two spring tides in May (Smith and Michels, 2006). The migration of many shorebirds, such as the red knot (Calidris canutus), semipalmated sandpiper (Calidris pusilla), ruddy turnstone (Arenaria interpres), and sanderling (Calidris alba), correspond to horseshoe crab spawning (Clark et al., 1993). The rufa subspecies of the red knot (Calidris canutus rufa), for example, is a shorebird with one of the longest migrations in the animal kingdom, traveling up to 19,000 miles from its wintering regions in the southeastern U.S., northeastern Gulf of Mexico, northern Brazil, or the southern tip of South America to its breeding ground in the Canadian Arctic (U.S. Fish and Wildlife Service, 2015).
Arguably the most important site in the red knot migration is the final stop in the Delaware Bay, where shorebirds feed on horseshoe crab eggs and rely heavily on the nutrition they receive to survive the final stretch of their journey to the frigid, unpredictable Canadian or Arctic tundra, which is often barren of further sustenance when they arrive (Baker et al., 2004; Mizrahi and Peters, 2009). Once in the Delaware Bay, red knots and other shorebirds feed predominantly on the eggs laid by spawning horseshoe crabs while they are available (Tsipoura and Burger, 1999; Mizrahi and Peters, 2009; McGowan et al., 2011; Smith et al., 2013). It is unlikely that other food sources found at shorebird stopover sites would be as widely available or as nutritionally dense as horseshoe crab eggs (Mizrahi and Peters, 2009).
Specifically, the high fatty acid content of these eggs makes them the ideal food source for migrating shorebirds that need to rapidly gain weight in order to ensure robust fitness, and consequently survival, on their journeys (Mizrahi and Peters, 2009). Survival and successful reproduction of the many migratory shorebirds that stop to feed at Delaware Bay are strongly linked to horseshoe crab reproduction. It is unlikely that these birds would be able to adjust their migration schedule over time (Baker et al., 2004; Mizrahi and Peters, 2009). From 1980 to 2014, red knot populations decreased by as much as 75% in some areas, largely due to the lack of horseshoe crab eggs in Delaware Bay (U.S. Fish and Wildlife Service, 2014).
Stable isotope tracking to analyze the diets of shorebirds revealed that free-ranging shorebirds possessed 15N signatures identical to those of shorebirds raised in captivity and fed a diet solely comprised of horseshoe crab eggs (Haramis et al., 2007). An estimated 107 billion horseshoe crab eggs are necessary to support 423,000 shorebirds flying into Delaware Bay to feed before continuing on to their breeding grounds (U.S. Fish and Wildlife Service Shorebird Technical Committee, 2003). For example, when preparing for migration, sanderlings consume an average of 8,300 horseshoe crab eggs per day; ruddy turnstones, a daily average of 13,300 (with a peak daily consumption of 19,360 eggs); and red knots consume an average of 18,350 (with a peak consumption of 23,940 eggs/day) (Castro et al., 1989; Haramis et al., 2007).
Ensuring a consistent and sustainable supply of horseshoe crab eggs is of particular concern with respect to the red knot, which has been listed as a threatened species and may be one of most studied proxies for tracking horseshoe crab populations and viability (U.S. Fish and Wildlife Service, 2014). The current red knot population is estimated to require 15.4 billion eggs to obtain sufficient energy levels for migration, which is equal to the number of eggs laid by about 170,000 female horseshoe crabs (U.S. Fish and Wildlife Service Shorebird Technical Committee, 2003). To be healthy enough to complete their migration, red knots need to double their body mass (usually arriving at 90–120 g and departing at 180–220 g) before the entire flock departs Delaware Bay at month's end (Baker et al., 2004). In total, 1,890 kilojoules (kJ) of stored energy is necessary to successfully complete the 2,400-kilometer flight from Delaware Bay to the Arctic (Baker et al., 2004).
From 1997 to 2002, the number of red knots that reached their target weight decreased by 70%, possibly due to late arrival in the Delaware Bay, compounded by a shortage of horseshoe crab eggs. Average body mass upon departure showed significant decline from 1997 to 2002, going from 182.8 grams (±22.6 g) to 162.3 g (±24.5 g) (Baker et al., 2004). During these same 5 years, tagged survivors that made the journey back to Delaware Bay and were recaptured at least once were heavier than birds not seen again. Between May 2000 and May 2001, the number of returning red knots decreased by 47% (Baker et al., 2004). The trends in declining body mass and population of red knots in Delaware Bay have correlated to an increase in the harvest of horseshoe crabs. Beginning in 1990 and peaking in 1998, horseshoe crabs were used largely as bait for eel and whelk fisheries, further impacting the availability of eggs for shorebird consumption (Walls et al., 2002).
The size of the population of red knots in Tierra del Fuego, Argentina, also rapidly declined from 51,000 in 2000 to 27,000 in 2002 (Morrison et al., 2004). In January 2003, an aerial survey of red knot sites along the Patagonian coast known to be abundant in the 1980s, located only 560 red knots. Likewise, a survey in December 2003 of northern Brazil indicated an abnormally small population of birds, suggesting evidence of red knot mortality, rather than just redistribution (Baker et al., 2004).
The shorebirds' considerable nutritional requirements might appear to be decimating the horseshoe crab population. Although mortality in the early stages of life is a major impediment to horseshoe crab population growth (Sweka et al., 2007), shorebird predation on horseshoe crab eggs has not been found to reduce the size of the horseshoe crab population. Eggs brought to the surface by wave action (Nordstrom et al., 2006) or other spawning horseshoe crabs (Sweka et al., 2007) dry out and die if not consumed (Botton, 2009). In fact, the eggs most accessible to the birds in the upper 5 cm of the beach comprise about 10% or less of the total density of buried eggs (Smith, 2007; Botton, 2009).
Further, red knots and other shorebirds have long been of interest to recreational birdwatchers, and efforts in recent years have been made to ensure that their foraging goes undisturbed. A combination of specified viewing locations and enforcement of policies, such as keeping unleashed dogs from roaming the beaches, have greatly reduced interruptions of these pivotal refueling stops during their migration (Burger et al., 2004).
Conservation Efforts
Regulatory efforts are underway to address dwindling horseshoe crab numbers. The Horseshoe Crab Management Board of the ASMFC approved the Horseshoe Crab Fishery Management Plan (FMP) in October 1998, which provided initial management of horseshoe crabs in and around Delaware Bay. However, conservation efforts in Delaware ultimately led to increased biomedical and bait harvesting in other areas, offsetting the prospects for overall horseshoe crab population growth (Atlantic States Marine Fisheries Commission, 2013). Later addenda to the Horseshoe Crab FMP specified annual state-by-state landing quotas across the east coast and contributed to the establishment of the Carl N. Schuster Jr. Horseshoe Crab Reserve, a 1,500 square mile harvest-free zone (Atlantic States Marine Fisheries Commission, 2000). Historically, the most comprehensive data on horseshoe crab abundance has been based on the Benthic Trawl Survey conducted by Virginia Polytechnic Institute (VPI), but the survey faces inconsistent funding circumstances. Other studies including the Delaware Trawl Survey, the New Jersey Delaware Bay Trawl Survey, and the New Jersey Ocean Trawl Survey have been established and helped intermittent funding limitations (Smith et al., 2016). Funding for future years of the studies is undergoing evaluation.
Addendum IV of the FMP delayed harvest in Maryland and Virginia and restricted bait harvest in Delaware and New Jersey to 100,000 male-only crabs. It was approved in May 2006; and the addendum was extended through October 2013 (Atlantic States Marine Fisheries Commission, 2006) with the addition of seasonal harvest restrictions on all horseshoe crabs from January to June and on female horseshoe crabs from June to December in Delaware and New Jersey (Atlantic States Marine Fisheries Commission, 2010b).
An Adaptive Resource Management (ARM) framework, designed to account for populations of both red knots and horseshoe crabs when implementing regulations, was established by Addendum VII in 2012. The ARM framework uses models considering red knot stopovers in Delaware Bay to determine optimal horseshoe crab harvest; has shaped several initiatives that aid in the protection of both species; and is the basis for ongoing assessments to optimize management plans (Atlantic States Marine Fisheries Commission, 2012).
As a result of these efforts, the aggregate harvest of horseshoe crabs declined 70% from 1998 to 2006, with the greatest reductions occurring in Delaware Bay states (Smith et al., 2009). The focus for conservation has also gradually shifted specifically to the spawning locations of the horseshoe crabs. As most crabs bury their eggs approximately 15 cm from the surface and above the high tide line toward the shore (Weber and Carter, 2009), protection of coastlines in which the horseshoe crabs spawn has been vital in working to restore their numbers to previous levels (Berkson et al., 2009).
Actions have also been taken in the fishing industry to reduce harvesting of horseshoe crabs for use as bait. These include alternative baits (Ferrari and Targett, 2003; Fisher and Fisher, 2006; Atlantic States Marine Fisheries Commission, 2015) and using bait bags with improved efficiency that require as little as one-tenth of traditional quantities per bag (Atlantic States Marine Fisheries Commission, 2017). However, tensions persist due to the demand for horseshoe crab bait by both the eel and whelk fishing industries (Atlantic States Marine Fisheries Commission, 2013). Furthermore, in 2015, conservation groups listed the red knot as endangered in the U.S., which would result in increased protection for horseshoe crabs. Conversely, members of the fishing industry have also challenged quotas due to an apparent continuation in the decline of the red knot population, despite a 2-year ban on horseshoe crab harvest in 2006 and 2007 (Moore, 2008).
Commercial Considerations
Given mixed results from these conservation efforts and impact from unabated LAL testing demands and utilization as a bait in the fishing industry, more sustainable approaches to horseshoe crab management and harvesting practices are urgently needed for medical and environmental applications.
Before adoption of the LAL test, most research facilities, pharmaceutical and medical device companies used the United States Pharmacopeia (USP) rabbit pyrogen test to determine the presence of endotoxins (Pharmacopeial Forum, 1983). However, the method took significantly longer to obtain results, notwithstanding the inherent variability and ethical issues with the use of live rabbits. The World Health Organization (WHO) now recognizes several bacterial endotoxin test (BET) methods using amebocyte-derived LAL from the horseshoe crab, including measuring turbidity or chromophore release from the BET reaction; however, the preferred method is based on amebocyte lysate clotting upon exposure to endotoxins or β-glucans (World Health Organization, 2011). Notably, β-glucans can also be selectively “ignored” by removing the G factor responsible for the β-glucans clotting reaction.
To date, the LAL test has been the test of choice, despite a more recently uncertain supply of horseshoe crab blood. Fortunately, it has been possible to increase the hemolymph extraction volume from L. polyphemus, as more accurate techniques for measuring blood volume have been discovered. It was initially estimated that the blood volume of a horseshoe crab was 10% of its total body weight; however, more recent findings have shown that blood volume is actually closer to 25% of the animal's total weight (Hurton et al., 2005).
New endotoxin tests have been developed and may have the potential to replace or supplement the LAL test; and thus, reduce or eliminate the demand for wild horseshoe crab capture. The recombinant factor C (rFC) test, for example, uses a cloned rFC reagent extracted from the DNA of the Singapore horseshoe crab and thereby eliminates the need for repetitive bleeding (Ding et al., 1995). Like the LAL test, the rFC test triggers a pathway to coagulation when endotoxins come into contact with Factor C. The rFC molecule has multiple potential endotoxin binding sites, and as such, the rFC assay has been shown to be more sensitive and specific than the LAL test (Ding and Ho, 2001; Thorne et al., 2010). However, the rFC test is currently considered an “alternative assay” as outlined in the Pyrogen and Endotoxins Testing; Questions and Answers, released by the FDA in 2012 (U. S. Department of Health and Human Services, 2012), which also stipulates that manufacturers must provide method validation in compliance with requirements outlined in by United States Pharmacopeia (USP) section on Bacterial Endotoxin Testing (USP, Chapter 85).
Another “alternative assay” to the LAL test is the Monocyte Activation Test (MAT) (U. S. Department of Health and Human Services, 2012), the MAT uses the monocytes of humans to mimic febrile reactions and thus requires no horseshoe crab byproducts, in contrast to the LAL and rFC assays (Stang et al., 2014). The MAT has been used reliably to resolve discrepancies between LAL test results; however, it has been shown to be ineffective in the presence of cytotoxic agents (Dobrovolskaia et al., 2014; Stang et al., 2014). The standard MAT procedure also lacks the sensitivity to detect the required amount of pyrogens on medical surfaces (which is also a limitation of the LAL assay). While the MAT has been optimized to detect such pyrogens, including the ability to ensure sensitivity by incubating test materials in the MAT, the modified version can take up to 20 h and is therefore too time-consuming for practical application in most settings (Stang et al., 2014).
Although the rFC and MAT methods produce results comparable to the LAL test (Alwis and Milton, 2006; Thorne et al., 2010; Hermanns et al., 2011) while conserving the horseshoe crab and surrounding ecosystems, the widespread adoption of these alternative tests may prove to be extremely challenging. The industry has been reluctant to transition to newer methods due to the complex validation procedure and subsequent redesign of the manufacturing processes that would necessarily accompany the change to procedures that have been established and followed for approximately 40 years (Cohen, 1979; U. S. Department of Health and Human Services, 2012).
In fact, revising the current system to improve efficiencies in horseshoe crab use may be more viable in the near term. Rather than adopting alternative tests, some biomedical companies have opted to make existing tests more sustainable. For example, LAL assays with specially designed cartridges have been developed to reliably screen for endotoxins, while also using one-twentieth of the raw horseshoe crab material required by conventional LAL tests (Wainwright, 2013).
Another alternative would be the use of a line of amebocytes that could be cultured in vitro. Research in this arena has yielded promising but inconsistent results (Joshi et al., 2002; Hurton et al., 2005); whereas, mounting pressures on the harvest of horseshoe crabs may yet help justify continued efforts and investment into this approach.
Alternatives to Current Horseshoe Crab Harvesting Practices
As more may be learned from further study, ranching of horseshoe crabs could be considered to help replenish populations. An instructive 56-day study of horseshoe crabs in captivity revealed decreases in body weight and deteriorating health, as reflected in various biological markers, including hemocyanin and amebocyte concentrations, which declined significantly (Coates et al., 2012). Although these changes occurred at all temperatures over time, horseshoe crabs held in higher temperatures (23°C) experienced the most significant decreases in these key metrics. To achieve the lowest horseshoe crab mortality and highest blood quality during biomedical bleeding, a more systematic understanding of the nuances of the optimal horseshoe crab environment, feeding and care would be required to pursue this alternative. Alternatively, if horseshoe crabs were allowed to reach maturity in the wild and transferred to native and protected estuary habitats with periodic monitoring, the species vitality could be improved and a better chance of survival might be achieved, as well as facilitate more controlled bleeding operations and schedules.
Notwithstanding the deleterious effects of wild capture and transport, the mechanism of blood harvest via a needle puncture to the arthrodial membrane could also cause unintended damage to the horseshoe crab circulatory system. No studies to date have systematically examined the effects of the puncture wound itself; however, anatomy of the area whereby the cardiac rhythm is controlled by ganglia suggests the potential for such punctures to interfere with normal function (Watson and Groome, 1989). Given a better understanding of the bleeding process, more advanced protocols could assess the potential for using indwelling catheters or alternative extraction sites.
Within this same paradigm, additional research focused on the optimization of bleeding volume and intervals could assess the potential to decrease horseshoe crab mortality and increase amebocyte yield. If bleeding horseshoe crabs in a controlled protocol (e.g., a temperature-controlled environment with the immediate return to their habitat) might correspond to human benefits from blood donation (Salonen et al., 1998), horseshoe crabs could potentially be bled more frequently with less trauma, while removing a smaller volume of blood per drawing. Also analogous to human plasmapheresis, the crab blood could be separated from the amebocytes and reinfused, or be replaced with a blood volume expander; this could alleviate hypovolemia while reducing stress and should allow for more rapid recovery.
Another important improvement to the bleeding process would be to minimize or prevent horseshoe crab hypoxia caused by extended periods outside the water. This could be accomplished by transporting the crabs in compatible tanks; employing wet covers, or towels, etc. (Novitsky, 2015). Less stressful transportation might also be achieved with temperature-controlled containers and/or by locating bleeding facilities closer to the harvest sites.
Further, using a formula to estimate the total hemolymph volume could help ensure that safer amounts of blood are extracted on an individual basis, rather than applying a broad standard to all crabs (Hurton et al., 2005). Well enforced restrictions on female horseshoe crab bleeding would also help mitigate any resulting behavioral changes; foster future spawning; and help stabilize egg production for migrating bird sustenance. Establishing optimal female to male bleeding ratios to manage commercial pressures associated with a greater yield from females would also help ensure necessary breeding ratios toward the species' long-term viability.
Improving the survival rate of horseshoe crab larvae into adulthood would likewise contribute toward replenishing the horseshoe crab population. With a characteristically high mortality rate the early stages of life for numerous marine species, approximately 0.001% of crabs survive through the first year (Carmichael et al., 2003; Sweka et al., 2007). Researchers have had some success from collecting horseshoe crab eggs, rearing them in a laboratory, and releasing the crabs as juveniles. However, this approach has not been conducted on a large scale, and any objections to egg collection that might interfere with shorebird feeding would need to be addressed before advancing this notion to a broader initiative (Mishra, 2009; Schreibman and Zarnoch, 2009).
Finally, various steps could be employed to reduce fishing industry demands on wild horseshoe crab populations, such as alternative and/or synthetic baits for whelk and eel, which could be used in lieu of horseshoe crabs. Such alternatives utilizing reduced quantities of horseshoe crab have been researched and field-tested with encouraging results (Ferrari and Targett, 2003; Fisher and Fisher, 2006).
As horseshoe crabs harvested for bait have outnumbered biomedical counts in recent years (Atlantic States Marine Fisheries Commission, 2016), a reduction in bait harvest is vital for conservation of horseshoe crab populations. Yet, because biomedical harvesting does not typically result in immediate mortality, the full impact might be underestimated and unaccounted for once fatigued, traumatized, and sometimes dying horseshoe crabs are returned to their habitat. Given these factors, both bait and biomedical demands appear to pose unsustainable challenges to horseshoe crab populations. However, in the absence of a widely accepted alternative to the LAL test, and as it remains vital to global medicine, there are promising approaches that could be employed to lessen the impact and reduce the ultimate mortality from biomedical harvesting.
Conclusions
The unique characteristics of horseshoe crabs underpinning their irrefutable importance to medicine, environmental safety, and their role as a keystone species highlight an urgent and compelling need for conservation and sustainable practices. To date, horseshoe crab conservation has been largely unidimensional, with many of the regulations applying only to the commercial fishing industry (Berkson, 2009). However, ensuring the wellbeing of this enigmatic species—and those whose survival depends on it—requires a multi-faceted approach that combines informed and fair regulation; responsible and more innovative harvesting and bleeding practices; and a commitment to continued research in pursuit of viable alternatives to avert collapse, while working toward ultimately eliminating the demand for harvesting wild horseshoe crabs, entirely.
Moving forward, effective horseshoe crab management must also extend beyond traditional approaches (e.g., stock abundance, recruitment, and growth rates) and begin to incorporate interventional ecosystem strategies. New and improved operational protocols should be established scientifically and implemented universally. In October 2011, early steps were taken to establish a blueprint for Best Management Practices for the collection, bleeding and releasing of horseshoe crabs (Atlantic States Marine Fisheries Commission, 2011, 2013). The document was generated by the Horseshoe Crab Biomedical ad hoc Working Group and was comprised of experts from each of the key biomedical bleeding organizations. The early draft of the document showed promise, but it has yet to be updated based on further research, nor has it progressed beyond recommendations to enforcement. The ability to assess the value of a document has also been undermined by unpublished industry reporting, whereby horseshoe crab mortality is neither reported publicly nor tabulated empirically: it is merely assumed that 15% of the harvested crabs perish (Atlantic States Marine Fisheries Commisson, 2009).
While horseshoe crab populations have modestly stabilized in some regions (Smith et al., 2017), the International Union for Conservation of Nature (IUCN) has predicted declines of at least 30% over the next three generations (~40 years) (Smith et al., 2016). In sharp contrast, the global demand for vaccines, pharmaceuticals and medical devices over approximately the same period will require an increasing supply of LAL. These dynamics pose significant uncertainties as to whether current harvesting levels can be sustained, much less meet projected demands. With particularly rapid development in vaccine production, global pharmaceutical and the U.S. medical device markets have already been trending toward 6–8% and 25% annual growth, respectively.
In addition to these projections, these challenges intensify if the Asian species continues to decline at its current rate (Gauvry, 2015). Utilized for analogous testing by Asian and Pacific-based pharmaceutical and medical device manufacturers in TAL assays, a shortfall in Asian horseshoe crabs could lead to a spike in the global demand for LAL or force adoption of costly, alternative testing methods. Dependent on these two species of horseshoe crabs that appear to be facing significant decline, the growth in vaccine production is especially problematic; whereby, a large percentage of endotoxin detection is also performed using TAL for vaccines destined for emerging markets (Gauvry, 2015). Despite recent, isolated recoveries, the IUCN forecast nonetheless suggests that the U.S. indigenous horseshoe crab biomass could not withstand the growth of the LAL market, much less absorb a shift from current TAL shares.
Thoughtful and conservative approaches are needed, but require a fair understanding of the threats. Regulators are faced with the paradox of managing a species to protect and maintain dependent shorebird populations; facilitate multibillion dollar eel, whelk and conch fisheries; and support the growing global dependence on an essential medical safety resource (LAL). These drivers are both environmental and economic. Indeed, progress and effective management will only be achieved once Best Management Practices are universally adopted and implemented; as public reporting is instituted; and as empirical data are gathered and tracked over time to inform industry and environmental regulatory oversight so as to ensure the viability of this ancient and essential species.
Author Contributions
AD, TB, JK-G, and WA conceived of the presented idea. JK-G, WA, KD, RT-K, TB, and MG developed the theory and performed primary research reviews. AD, KD, CK, and TB verified the materials and methods. TB encouraged AD, JK-G, KD, and WA to investigate the biomedical medial industry impact on horseshoe crabs. AD supervised the findings of this work. All authors prepared analysis of the primary research and contributed to the final review manuscript.
Conflict of Interest Statement
The authors have partnered with the Georgia Department of Natural Resources to support future scientific and management initiatives in effort that ensure that the Georgia Atlantic horseshoe crab populations remain vibrant and healthy through potential husbandry and bleeding protocols. Authors AD, JK-G, TB, and LR are employed by company Kepley BioSystems, Inc. CK is a full-time professor at the Joint School of Nanoscience and Nanoengineering and works three summer months with the company Kepley BioSystems, Inc. WA was a NSF research undergraduate employed by company Kepley BioSystems Inc. MG was employed by ClienTell® Consulting, LLC.
The other authors declare that the research was conducted in the absence of any commercial or financial relationships that could be construed as a potential conflict of interest.
Acknowledgments
The authors would like to acknowledge the following funding sources: National Science Foundation's Research Experience for Undergraduates (REU) program (Grant # 1555752), North Carolina Biotechnology Center Industrial Intern Partnership (2017-IIP-4202), and North Carolina Sea Grant (2017-R/MG-1712).
Abbreviations
15N, Nitrogen-15; ARM, Adaptive Resource Management; ASMFC, Atlantic States Marine Fisheries Council; BET, Bacterial Endotoxin Test; BMP, Best Management Practices; FMP, Fishery Management Plan; LAL, Limulus Amebocyte Lysate; LPS, Lipopolysaccharide; MAT, Monocyte Activation Test; PCO2, Partial Pressure of Carbon Dioxide; PO2, Partial Pressure of Oxygen; rFC, Recombinant Factor C; TAL, Tachypleus Amebocyte Lysate; USP, United States Pharmacopeia; WHO, World Health Organization.
References
Allender, M. C., Schumacher, J., George, R., Milam, J., and Odoi, A. (2010). The effects of short- and long-term hypoxia on hemolymph gas values in the American horseshoe crab (Limulus polyphemus) using a point-of-care analyzer. J. Zoo Wildl. Med. 41, 193–200. doi: 10.1638/2008-0175R2.1
Alwis, K. U., and Milton, D. K. (2006). Recombinant factor C assay for measuring endotoxin in house dust: comparison with LAL, and (1 –> 3)-beta-D-glucans. Am. J. Ind. Med. 49, 296–300. doi: 10.1002/ajim.20264
Anderson, R. L., Watson, W. H., and Chabot, C. C. (2013). Sublethal behavioral and physiological effects of the biomedical bleeding process on the American horseshoe crab, Limulus polyphemus. Biol. Bull. 225, 137–151. doi: 10.1086/BBLv225n3p137
Armstrong, P., and Conrad, M. (2008). Blood collection from the American horseshoe crab, Limulus polyphemus. J. Vis. Exp. 20:958. doi: 10.3791/958
Atlantic States Marine Fisheries Commission (1998). Interstate Fishery Management Plan for Horseshoe Crab. Fishery Management Report No. 32 of the Atlantic States Marine Fisheries Commission (Washington, DC).
Atlantic States Marine Fisheries Commission (2000). Addendum I to the Fishery Management Plan for Horseshoe Crab. Fishery Management Report No. 32a of the Atlantic States Marine Fisheries Commission. Washington, DC.
Atlantic States Marine Fisheries Commission (2006). Addendum IV to the Fishery Management Plan for Horseshoe Crab. Fishery Management Report No. 32d of the Atlantic States Marine Fisheries Commission. Washington, DC.
Atlantic States Marine Fisheries Commisson (2009). Horseshoe Crab Stock Assessment for Peer Review. Stock Assessment Report No. 09-02 (Supplement A) (Washington, DC).
Atlantic States Marine Fisheries Commission (2010a). Horseshoe Crab Advisory Panel Report. Washington, DC.
Atlantic States Marine Fisheries Commission (2010b). Addendum VI to the Fishery Management Plan for Horseshoe Crab. Fishery Management Report No. 32f of the Atlantic States Marine Fisheries Commission. Washington, DC.
Atlantic States Marine Fisheries Commission (2011). Horseshoe Crab Biomedical ad-hoc Working Group Report. Washington, DC.
Atlantic States Marine Fisheries Commission (2012). Addendum VII to the Fishery Management Plan for Horseshoe Crab. Fishery Management Report No. 32g of the Atlantic States Marine Fisheries Commission. Washington, DC.
Atlantic States Marine Fisheries Commission (2013). Horseshoe Crab Stock Assessment Update. Arlington, VA.
Atlantic States Marine Fisheries Commission (2014). “Horseshoe crab technical committee meeting summary,” in Conference Call (Arlington, VA).
Atlantic States Marine Fisheries Commission (2015). 2015 Review of the Atlantic States Marine Fisheries Commission Fisheries Management Plan for Horseshoe Crab (Limulus polyphems) 2014 Fishing Year. Washington, DC.
Atlantic States Marine Fisheries Commission (2016). 2016 Review of the Atlantic States Marine Fisheries Commission Fisheries Management Plan for Horseshoe Crab (Limulus polyphems) 2015 Fishing Year. Washington, DC.
Atlantic States Marine Fisheries Commission (2017). “Horseshoe Crab”. Available online at: http://www.asmfc.org/species/horseshoe-crab
Baker, A. J., Gonzalez, P. M., Piersma, T., Niles, L. J., do Nascimento Ide, L., Atkinson, P. W., et al. (2004). Rapid population decline in red knots: fitness consequences of decreased refuelling rates and late arrival in Delaware Bay. Proc. Biol. Sci. 271, 875–882. doi: 10.1098/rspb.2003.2663
Barlow, R. B. Jr., Bolanowski, S. J. Jr., and Brachman, M. L. (1977). Efferent optic nerve fibers mediate circadian rhythms in the Limulus eye. Science 197, 86–89. doi: 10.1126/science.867057
Berkson, J. (2009). “An integrative approach to horseshoe crab multiple use and sustainability,” in Biology and Conservation of Horseshoe Crabs, eds T. J Tanacredi, M. L. Botton and D. R. Smith (New York, NY: Springer Science, U.S.), 387–398.
Berkson, J., Chen, C. P., Mishra, J., Shin, P., Spear, B., and Zaldivar-Rae, J. (2009). “A discussion of horseshoe crab management in five countries: Taiwan, India, China, United States, and Mexico,” in Biology and Conservation of Horseshoe Crabs, edS T. J Tanacredi, M. L. Botton and D. R Smith (New York, NY: Springer Science, U.S.), 465–475.
Botton, M. (2009). “The ecological importance of horseshoe crabs in estuarine and coastal communities: a review and speculative summary,” in Biology and Conservation of Horseshoe Crabs, eds T. J. Tanacredi, M. J. Botton, D. R. Smith (New York, NY: Springer Science, U.S.), 45–64.
Botton, M., and Haskin, H. (1984). Distribution and feeding of the horseshoe crab, Limulus polyphemus, on the continental shelf off New Jersey. Fish. Bull. 82, 383–389.
Botton, M., and Loveland, R. (1993). Predation by herring gulls and great black-backed gulls on horseshoe crabs. Wilson Bull. 105, 518–521.
Botton, M., and Ropes, J. (1989). Feeding ecology of horseshoe crabs on the continental shelf, New Jersey to North Carolina. Bull. Mar. Sci. 45, 637–647.
Burger, J., Jeitner, C., Clark, K., and Niles, L. (2004). The effect of human activities on migrating shorebirds: successful adaptive management. Environ. Conserv. 31, 283–288. doi: 10.1017/S0376892904001626
Carmichael, R. H., Rutecki, D., and Valiela, I. (2003). Abundance and population structure of the Atlantic horseshoe crab Limulus polyphemus in Pleasant Bay, Cape Cod. Mar. Ecol. Prog. Ser. 246, 225–239. doi: 10.3354/meps246225
Castro, G., Myers, J., and Place, A. (1989). Assimilation efficiency of sanderlings (Calidris alba) feeding on horseshoe crab (Limulus polyphemus) eggs. Physiol. Zool. 62, 716–731. doi: 10.1086/physzool.62.3.30157923
Central Intelligence Agency (2016). The World Factbook: United States. Available online at: https://www.cia.gov/library/publications/the-world-factbook/geos/us.html
Clark, K. E., Niles, L. J., and Burger, J. (1993). Abundance and distribution of migrant shorebirds in Delaware Bay. Condor 95, 694–705. doi: 10.2307/1369612
Coates, C., Bradford, E., Krome, C., and Nairn, J. (2012). Effect of temperature on biochemical and cellular properties of captive Limulus polyphemus. J. Aquac. 334–337, 30–38. doi: 10.1016/j.aquaculture.2011.12.029
Cohen, E. (1979). Report on the symposium on biomedical applications of Limulus polyphemus (horseshoe crab). Dev. Biol. 3, 365–371. doi: 10.1016/S0145-305X(79)80032-4
Ding, J. L., and Ho, B. (2001). A new era in pyrogen testing. Trends Biotechnol. 19, 277–281. doi: 10.1016/S0167-7799(01)01694-8
Ding, J. L., Navas, M. A., and Ho, B. (1995). Molecular cloning and sequence analysis of factor C cDNA from the Singapore horseshoe crab, Carcinoscorpius rotundicauda. Mol. Mar. Biol. Biotechnol. 4, 90–103.
Dobrovolskaia, M. A., Neun, B. W., Clogston, J. D., Grossman, J. H., and McNeil, S. E. (2014). Choice of method for endotoxin detection depends on nanoformulation. Nanomedicine 9, 1847–1856. doi: 10.2217/nnm.13.157
Ferrari, K. M., and Targett, N. M. (2003). Chemical attractants in horseshoe crab, Limulus polyphemus, eggs: the potential for an artificial bait. J. Chem. Ecol. 29, 477–496. doi: 10.1023/A:1022698431776
Fisher, R. A., and Fisher, D. L. (2006). The Use of Bait Bags to Reduce the Need for Horseshoe Crab as Bait in the Virginia Whelk Fishery. Gloucester Point, VA: Virginia Sea Grant.
Gauvry, G. (2015). “Current horseshoe crab harvesting practices cannot support global demand for TAL/LAL: the pharmaceutical and medical device industries' role in the sustainability of horseshoe crabs,” in Changing Global Perspectives on Horseshoe Crab Biology, Conservation and Management, eds M. L. Bottom, R. H. Carmichael, P. K. S. Shin, and S. G. Cheung (New York, NY: Springer Science, U.S.), 475–482.
Haramis, G., Link, W., Osenton, P., Carter, D., Weber, R., Clark, N., et al. (2007). Stable isotope and pen feeding trial studies confirm the value of horseshoe crab Limulus polyphemus eggs to spring migrant shorebirds in Delaware Bay. J. Avian Bio. 38, 367–376. doi: 10.1111/j.2006.0908-8857.03898.x
Hartline, H. K., and McDonald, P. (1947). Light and dark adaptation of single photoreceptor elements in the eye of Limulus. J. Cell. Comp. Physiol. 30, 225–253. doi: 10.1002/jcp.1030300303
Henry, R., and Wheatly, M. (1992). Interaction of respiration, ion regulation, and acid-base balance in the everyday life of aquatic crustaceans. Amer. Zool. 32, 407–416. doi: 10.1093/icb/32.3.407
Hermanns, J., Bache, C., Bjoern, B., Loeschner, B., Montag, T., and Spreitzer, I. (2011). “Alternatives to animal use for the LAL-assay,” in Paper Presented at the The 8th World Congress on Alternatives and Animal Use in the Life Science (Montreal, QC).
Hurton, L., and Berkson, J. (2006). Potential causes of mortality for horseshoe crabs (Limulus polyphemus) during the biomedical bleeding process. Fish. Bull. 104, 293–298.
Hurton, L., Berkson, J., and Smith, S. (2005). Estimation of total hemolymph volume in the horseshoe crab Limulus polyphemus. Mar. Freshwater. Behav. Physiol. 38, 139–147. doi: 10.1080/10236240500064354
Isakova, V., and Armstrong, P. B. (2003). Imprisonment in a death-row cell: the fates of microbes entrapped in the Limulus blood clot. Biol. Bull. 205, 203–204. doi: 10.2307/1543253
Iwanaga, S., Kawabata, S., and Muta, T. (1998). New types of clotting factors and defense molecules found in horseshoe crab hemolymph: their structures and functions. J. Biochem. 123, 1–15. doi: 10.1093/oxfordjournals.jbchem.a021894
Joshi, B., Chatterji, A., and Bhonde, R. (2002). Long term in vitro generation of amoebocytes from the Indian horseshoe crab Tachypleus gigas. In Vitro. Cell. Dev. Biol. Anim. 38, 255–257. doi: 10.1290/1071-2690(2002)038<0255:LTIVGO>2.0.CO;2
Keinath, J. (2003). “Predation of horseshoe crabs by loggerhead sea turtles,” in The American Horseshoe Crab, eds C. N Shuster, H. Brockman and R. B. Barlow (Cambridge, MA: Harvard Press), 152–153.
Kin, A., and Blazejowski, B. (2014). The horseshoe crab of the genus Limulus: living fossil or stabilomorph? PLoS ONE 9:9. doi: 10.1371/journal.pone.0108036
Leschen, A. S., and Correia, S. J. (2010). Mortality in female horseshoe crabs (Limulus polyphemus) from biomedical bleeding and handling: implications for fisheries management. Mar. Freshwater Behav. Physiol. 43 135–147. doi: 10.1080/10236241003786873
Loveland, R. E., Botton, M. L., and Shuster, C. N. (1996). “Life history of the American horseshoe crab (Limulus polyphemus) in Delaware Bay and its importance as a commercial resource,” in Presented at the Proceedings of the Horseshoe Crab Forum: Status of the Resource (Lewes, DE: University of Delaware Sea Grant College Program).
McGowan, C. P., Smith, D. R., Sweka, J. A., Martin, J., Nichols, J. D., Wong, R., et al. (2011). Multispecies modeling for adaptive management of horseshoe crabs and red knots in the Delaware Bay. Nat. Resour. Model. 24, 117–156. doi: 10.1111/j.1939-7445.2010.00085.x
Medzhitov, R., and Janeway, Jr. C. (2000). Innate immune recognition: mechanisms and pathways. Immunol. Rev. 173, 89–97. doi: 10.1034/j.1600-065X.2000.917309.x
Mishra, J. (2009). “Larval culture of Tachypleus gigas and its molting behavior under laboratory conditions,” in Biology and Conservation of Horseshoe Crabs, eds J. T. Tanacredi, M. L. Botton, and D. R. Smith (New York, NY: Springer Science), 513–520.
Mizrahi, D. S., and Peters, K. A. (2009). “Relationships between sandpipers and horseshoe crab in Delaware Bay: a synthesis,” in Biology and Conservation of Horseshoe Crabs, eds J. T. Tanacredi, M. L. Botton, and D. R. Smith (New York, NY: Springer Science), 65–87.
Morrison, R., Ross, R., and Nile, L. (2004). Declines in wintering populations of red knots in Southern South America. Condor 106, 60–70. doi: 10.1650/7372
Mowles, S. L., Cotton, P. A., and Briffa, M. (2009). Aerobic capacity influences giving-up decisions in fighting hermit crabs: does stamina constrain contests? Anim. Behav. 78, 735–740. doi: 10.1016/j.anbehav.2009.07.003
Nordstrom, K. F., Jackson, N. L., Smith, D. R., and Weber, R. G. (2006). Transport of horseshoe crab eggs by waves and swash on an estuarine beach: implications for foraging shorebirds. Estuar. Coast. Shelf Sci. 70, 438–448. doi: 10.1016/j.ecss.2006.06.027
Novitsky, T. J. (2009). “Biomedical applications of Limulus amebocyte lysate,” in Biology and Conservation of Horseshoe Crabs, eds J. T. Tanacredi, M. L. Botton, and D. R. Smith (New York, NY: Springer Science), 315–329.
Novitsky, T. J. (2015). “Biomedical implications for managing the Limulus polyphemus harvest along the Northeast Coast of the United States,” in Changing Global Perspectives on Horseshoe Crab Biology, Conservation, and Management, eds M. L. Botton, R. H. Carmichael, P. K. S. Shin, and S. G. Cheung (Cham: Springer), 489–495.
Patten, W. (1912). The Evolution of the Vertebrates and Their Kin. Philadelphia, PA: P. Blakiston's Son & Co.
Perry, L. M. (1931). Catfish feeding on the eggs of the horseshoe crab, Limulus polyphemus. Science 74:312. doi: 10.1126/science.74.1917.312
Pharmacopeial Forum (1983). “Characterization of rabbit colonies for the pyrogen test,” in Paper Presented at the Pharmacopeial Forum (Rockville, MD).
Price, K. S. (1962). Biology of the sand shrimp, Crangon septemspinosa, in the shore zone of the Delaware Bay region. Chesapeake Sci. 3, 244–255. doi: 10.2307/1350631
Reid, J., and Bonde, R. (1990). Alligator mississippiensis (American alligator) diet. Herpetol. Rev. 21:59
Salonen, J. T., Tuomainen, T. P., Salonen, R., Lakkta, T. A., and Nyyssonen, K. (1998). Donation of blood is associated with reduced risk of myocardial infarction. The Kuopio Ischaemic Heart Disease Risk Factor Study. Am. J. Epidemiol. 148, 445–451. doi: 10.1093/oxfordjournals.aje.a009669
Schreibman, M., and Zarnoch, C. (2009). “Aquaculture methods and early growth of juvenile horseshoe crabs (Limulus polyphemus),” in Biology and Conservation of Horseshoe Crabs, eds J. T. Tanacredi, M. L. Botton, and D. R. Smith (New York: Springer Science), 501–512.
Sekiguchi, K., and Shuster, C. N. (2009). “Limits on the global distribution of horseshoe crabs (Limulacea): lessons learned from two lifetimes of observations: asia and America,” in Biology and Conservation of Horseshoe Crabs, eds J. T. Tanacredi, M. L. Botton, and D. R. Smith (New York: Springer Science), 5–24.
Seney, E. E., and Musick, J. A. (2007). Historical diet analysis of loggerhead sea turtles (Caretta caretta) in Virginia. Copeia 2007, 478–489. doi: 10.1643/0045-8511(2007)7[478:HDAOLS]2.0.CO;2
Servis, J. A., Lovewell, G., and Tucker, A. D. (2015). Diet analysis of subadult kemp's ridley (Lepidochelys kempii) turtles from West-Central Florida. Chelonian Conserv. Biol. 14, 173–181. doi: 10.2744/CCB-1177.1
Shuster, C. N. (1978). The Circulatory System and Blood of the Horseshoe Crab (Limulus Polyphemus): A Review. Washington DC: U.S. Department of Federal Energy Regulatory Commission.
Smith, D. R. (2007). Effect of horseshoe crab spawning density on nest disturbance and exhumation of eggs: a simulation study. Estuar. Coast. 30, 287–295. doi: 10.1007/BF02700171
Smith, D. R., Beekey, M. A., Brockmann, H. J., King, T. L., Millard, M. J., and Zaldívar-Rae, J. A. (2016). Limulus Polyphemus. The International Union for Conservation of Nature and Natural Resources Red List of Threatened Species. Cambridge: IUCN Global Species Programme - Red List Unit.
Smith, D. R., Brockmann, J. H., Beekey, M., King, T. L., and Millard, M. J. (2017). Conservation status of the American horseshoe crab, (Limulus polyphemus): a regional assessment. Rev. Fish Biol. Fish. 27, 135–175. doi: 10.1007/s11160-016-9461-y
Smith, D. R., McGowan, C. P., Daily, J. P., Nichols, J. D., Sweka, J. A., and Lyons, J. E. (2013). Evaluating a multispecies adaptive management framework: must uncertainty impede effective decision making? J. Appl. Ecol. 50, 1431–1440. doi: 10.1111/1365-2664.12145
Smith, D. R., and Michels, S. F. (2006). Seeing the elephant: importance of spatial and temporal coverage in a large-scale volunteer-based program to monitor horseshoe crabs. Fisheries 31, 485–491. doi: 10.1577/1548-8446(2006)31[485:STE]2.0.CO;2
Smith, D. R., Millard, M. J., and Carmichael, R. H. (2009). “Comparative status and assessment of Limulus polyphemus with emphasis on the New England and Delaware Bay populations,” in Biology and Conservation of Horseshoe Crabs, eds J. T. Tanacredi, M. L. Botton, and D. R. Smith (New York, NY: Springer Science), 361–386.
Spotila, J., Plotkin, P., and Keinath, J. (2007). “Delaware Bay is an important foraging habitat for loggerhead tumrtles,” in Paper Presented at the 2007 Delaware Estuary Science Conference and Environmental Summit (Cape May, NJ).
Spraker, H., and Austin, H. M. (1997). Diel feeding periodicity of Atlantic Silverside, Menidia menidia, in the York River, Chesapeake Bay, Virginia. J. Elisha Mitchell Sci. Soc. 113, 171–182.
Stang, K., Fennrich, S., Krajewski, S., Stoppelkamp, S., Burgener, I. A., Wendel, H. P., et al. (2014). Highly sensitive pyrogen detection on medical devices by the monocyte activation test. J. Mater. Sci. Mater. Med. 25, 1065–1075. doi: 10.1007/s10856-013-5136-6
Sweka, J. A., Smith, D. R., and Millard, M. J. (2007). An age-structured population model for horseshoe crabs in the Delaware Bay area to assess harvest and egg availability for shorebirds. Estuar. Coast. 30, 277–286. doi: 10.1007/BF02700170
Tanacredi, J. T., and Portilla, S. (2015). “Habitat inventory trend analysis of Limulus polyphemus populations on Long Island, USA: From the tip of Brooklyn to the tip of Montauk, 2003–2014,” in Changing Global Perspectives on Horseshoe Crab Biology, Conservation, and Management, eds M. L. Botton, R. H. Carmichael, P. K. S. Shin, and S. G. Cheung (Cham: Springer), 229–236.
Thorne, P. S., Perry, S. S., Saito, R., O'Shaughnessy, P. T., Mehaffy, J., Metwali, N., et al. (2010). Evaluation of the Limulus amebocyte lysate and recombinant factor C assays for assessment of airborne endotoxin. Appl. Environ. Microbiol. 76, 4988–4995. doi: 10.1128/AEM.00527-10
Towle, D. W., and Henry, R. P. (2003). “Coping with environmental changes: physiological challenges” in The American Horseshoe Crab, eds C. N. Shuster, R. B. Barlow, and H. J Brockmann (Cambridge, MA: Harvard University Press), 224–244.
Tsipoura, N., and Burger, J. (1999). Shorebird diet during spring migration stopover on Delaware Bay. Condor 101, 635–644. doi: 10.2307/1370193
Ultsch, G. (1989). Ecology and physiology of hibernation and overwintering among freshwater fishes, turtles, and snakes. Biol. Rev. 64, 435–515. doi: 10.1111/j.1469-185X.1989.tb00683.x
U.S. Fish and Wildlife Service Shorebird Technical Committee (2003). Delaware Bay Shorebird-Horseshoe Crab Assessment Report and Peer Review. Arlington, VA: U.S. Fish and Wildlife Service Shorebird Technical Committee.
U. S. Department of Health and Human Services (2012). Guidance for Industry Pyrogen and Endotoxins Testing: Questions and Answers. Washington, DC: U.S. Food and Drug Administration.
U.S. Fish and Wildlife Service (2014). Service Protects Red Knot as Threatened Under the Endangered Species Act. Falls Church, VA: Division of Public Affairs.
U.S. Fish and Wildlife Service (2015). Status of the Species-Red Knot (Calidris canutus rufa). Washington, DC: Department of Interior.
van Holde, K. E., and Miller, K. I. (1995). Hemocyanins. Adv. Protein Chem. 47, 1–81. doi: 10.1016/S0065-3233(08)60545-8
Vaquer-Sunyer, R., and Duarte, C. (2011). Temperature effects on oxygen thresholds for hypoxia in marine benthic organisms. Global Change Biol. 17, 1788–1797. doi: 10.1111/j.1365-2486.2010.02343.x
Wainwright, N. (2013). Ever Had an Injection? Thank a Horseshoe Crab. Available online at: http://eureka.criver.com/ever-had-an-injection-thank-a-horseshoe-crab/
Walls, E., and Berkson, J. (2003). Effects of blood extraction on horseshoe crabs (Limulus polyphemus). Fish. Bull. 101, 457–459.
Walls, E., Berkson, J., and Smith, S. (2002). The horseshoe crab, Limulus polyphemus: 200 million years of existence, 100 years of study. Rev. Fish. Sci. 10, 39–73. doi: 10.1080/20026491051677
Watson, W. H. III., and Groome, J. R. (1989). Modulation of the Limulus heart. Am. Zool. 29, 1287–1303. doi: 10.1093/icb/29.4.1287
Weber, R., and Carter, D. (2009). “Distribution and development of Limulus egg clusters on intertidal beaches in Delaware Bay,” in Biology and Conservation of Horseshoe Crabs, eds J. T. Tanacredi, M. L. Botton, and D. R. Smith (New York, NY: Springer Science), 249–266.
Widener, J. W., and Barlow, R. B. (1999). Decline of a horseshoe crab population on Cape Cod. Biol. Bull. 197, 300–302. doi: 10.2307/1542664
Keywords: biomedical industry, ecological status, horseshoe crab, Limulus amebocyte lysate assay, Limulus polyphemus, migrating shorebirds, red knot, ocean ecology
Citation: Krisfalusi-Gannon J, Ali W, Dellinger K, Robertson L, Brady TE, Goddard MKM, Tinker-Kulberg R, Kepley CL and Dellinger AL (2018) The Role of Horseshoe Crabs in the Biomedical Industry and Recent Trends Impacting Species Sustainability. Front. Mar. Sci. 5:185. doi: 10.3389/fmars.2018.00185
Received: 15 November 2017; Accepted: 08 May 2018;
Published: 05 June 2018.
Edited by:
Elvira S. Poloczanska, Alfred Wegener Institut Helmholtz Zentrum für Polar und Meeresforschung, GermanyReviewed by:
David Smith, United States Geological Survey, United StatesDonald F. Boesch, University of Maryland, United States
Copyright © 2018 Krisfalusi-Gannon, Ali, Dellinger, Robertson, Brady, Goddard, Tinker-Kulberg, Kepley and Dellinger. This is an open-access article distributed under the terms of the Creative Commons Attribution License (CC BY). The use, distribution or reproduction in other forums is permitted, provided the original author(s) and the copyright owner are credited and that the original publication in this journal is cited, in accordance with accepted academic practice. No use, distribution or reproduction is permitted which does not comply with these terms.
*Correspondence: Anthony L. Dellinger, adellinger@kepleybiosystems.com