- 1Marine Geology, Geological Survey of Finland (GTK), Espoo, Finland
- 2Marine Research Center, Finnish Environment Institute (SYKE), Helsinki, Finland
The European Marine Strategy Framework Directive requires the development of suitable indicators for regular reporting on the environmental state and achievement of a good environmental status of EU's marine waters by 2020. The development of indicators for determining seafloor integrity and its possible disturbance by human activities have so far largely ignored the geological properties of seafloor. This paper presents a study of Vuosaari and Uusikaupunki-D offshore dumping sites in Finland, the northern Baltic Sea. Full coverage multibeam bathymetry and relative backscatter data, and a number of sediment cores were collected over the sites. The areas covered by dumped dredge spoil stand out in the multibeam images because of their irregular surface and elevated backscatter. The short gravity cores were studied for lithology, and in 1-cm slices for 137Cs activity, organic content, and grain size distribution. The dumped material is represented in the cores by the gravelly mud lithofacies with massive texture and angular coarse particles. The dumped material is coarser, less sorted and has higher kurtosis compared to natural sediment due to the admixing of blasted rock during the dredging activities, and limited sorting during fall through the water column upon dumping. Dispersed dredge spoil, which was suspended in the water column during the dumping activities or reworked from the dumped material mounds and redistributed along the seafloor soon thereafter, was deposited over a wide area as a thin layer that is not necessarily readily identifiable by visual inspection in the cores. Cesium activity helped distinguish the dumped material from the 137Cs-enriched natural sediments deposited after the 1986 Chernobyl disaster. Considering that the dumped material at many of the coring sites in the Vuosaari dumping area is covered by natural sediment, it probably is largely stable. In contrast, dumped material at the shallower Uusikaupunki-D site has slumped down to an adjacent channel and is likely being redistributed by near-bottom currents. Based on the findings of the study, a protocol for the assessment of the geological integrity of seafloor, its anthropogenic change due to dumping, and its potential recovery is proposed, as required by the Marine Strategy Framework Directive.
Introduction
Disposal of dredged material (dumping) is a notable issue in coastal zone management, which may represent a major anthropogenic disturbance to the structure and functioning of the seabed, albeit often at very local scales (e.g., Olenin, 1992; Essink, 1999; Bolam et al., 2006; Powilleit et al., 2006; Korpinen et al., 2012). Although open water disposal is often the primary alternative due to economic considerations, dumping effects should be evaluated in order to determine the potential impact on human health, marine ecosystems, and other legitimate uses of the sea. Guidelines and procedures have been established by intergovernmental bodies and international organizations (e.g., OSPAR, 2014; HELCOM, 2015; IMO, 2015) with an aim at reducing the adverse effects of dumping operations. Environmentally acceptable offshore dumping requires the careful evaluation of a number of issues, including the prior characterization of material to be dredged considering the prerequisite that the dredged material is clean or low contaminated, the selection of a suitable dumping site with regard to the stability of the dumped material mounds on the seafloor (depositional site) or its resuspension by currents and transportation to adjacent locations (dispersive site), and the monitoring of environmental effects caused by dumping during and after the operations.
The European Marine Strategy Framework Directive (MSFD) demands regular reporting on the environmental state and the achievement of a Good Environmental Status (GES) of the EU's marine waters by 2020. Eleven descriptors were constituted and associated criteria and indicators were defined to interpret the GES (European Commission, 2017). The Descriptor 6 (seafloor integrity) of GES is targeted to assess the degree to which the seafloor is adversely affected by human activities. Indicators for determining the seafloor integrity and its change are being developed (e.g., Rice et al., 2012; Berg et al., 2015; Teixeira et al., 2016); however, these efforts are focused on the biological (e.g., macrofauna) and hydrochemical (e.g., dissolved oxygen) aspects of the seafloor ecosystem. As yet, there is no consensus on assessment methods to estimate effects of anthropogenic pressures, as required by the recently adopted GES criteria (European Commission, 2017). Even though an approach has been proposed in the Baltic Sea (Korpinen et al., 2013; HELCOM, 2017), determination of the physical (geological) integrity of a seafloor, or its change, is still in an early phase. This lack of geological indicators for the seafloor physical integrity further reverberates in the current consideration of suitable ecosystem service indicators (Broszeit et al., 2017). In this contribution, geological integrity is understood as the integrity of geological properties of the seafloor surface sediment, with respect to its ability to provide a habitable substrate for the indigenous macrozoobenthic community.
Only few studies have considered the physical sedimentary impacts of offshore dumping, and the physical recovery of the seafloor substrate after the cessation of dumping activities. Stronkhorst et al. (2003) observed a recovery of sediment texture a year after the cessation of activities at a dumping site in the North Sea, near the port of Rotterdam. Wienberg and Hebbeln (2005) observed a recovery of seafloor dune morphology and redistribution of dumped material from a dumping site within few months in the Weser Estuary, North Sea. Du Four and Van Lancker (2008) observed a restoration of seafloor morphology and sediment texture within 1 year at a dumping site near the Zeebrugge harbor, also in the North Sea. Marmin et al. (2016) observed a partial recovery of seafloor morphology and the admixing of dumped fine sand with coarser natural sediments after 14 months at an experimental dumping site in the Bay of Seine, the English Channel. All these studies are from tidal high-energy sandy seafloor environments, where the dumped dredge spoil is intensively reworked and redistributed along the seafloor. The physical sedimentary impacts of dumping activities, as well as the process of the possible recovery of the seafloor substrate, are expected to be different in wave-dominated coastal areas with minimal tidal amplitude and soft muddy sediments. Indeed, Lepland et al. (2009) observed restricted spread of dumped mixture of mud, sand and gravel, and loading-induced compaction of underlying natural organic-rich mud at a dumping site in Oslofjord, Norway. Stockmann et al. (2009) and Tauber (2009) observed minimal change in the volume of experimentally dumped mounds of glacial till and mixed till, silt and sand, but modest smoothing of the surface roughness by reworking of fine-grained material to local depressions and the development of sandy lags on local elevations during 3 years in the Mecklenburg Bight, southern Baltic Sea.
The purpose of this study is to develop observation techniques and criteria for the assessment of the geological integrity of seafloor, its anthropogenic change due to dumping, and recovery as required by the MSFD. The assessment techniques are based on multibeam seafloor surveys as well as sedimentary 137Cs activity, organic content and grain size distribution parameters determined for sub-sample slices of sediment cores. Those data can be routinely collected in order to facilitate the establishment of robust and cost-effective monitoring programmes that are required to make future assessments of the status of the marine environment. Findings from the study are used for producing a graphical illustration of the assessment process. Grain-size data have been successfully used previously for inferring sediment sources and depositional sub-environments (e.g., Folk and Ward, 1957; Stevens et al., 1996; Bobertz and Harff, 2004; Kairyte and Stevens, 2015), as well as anthropogenic influence such as the redistribution of dumped dredge spoil on the seafloor (Okada et al., 2009; McLaren and Teear, 2014). This study focuses on two offshore dumping areas in Finland, Vuosaari in the south coast and Uusikaupunki-D in the west coast (Figures 1, 2), which provide test cases for discriminating dumped dredge spoil and naturally deposited sediments.
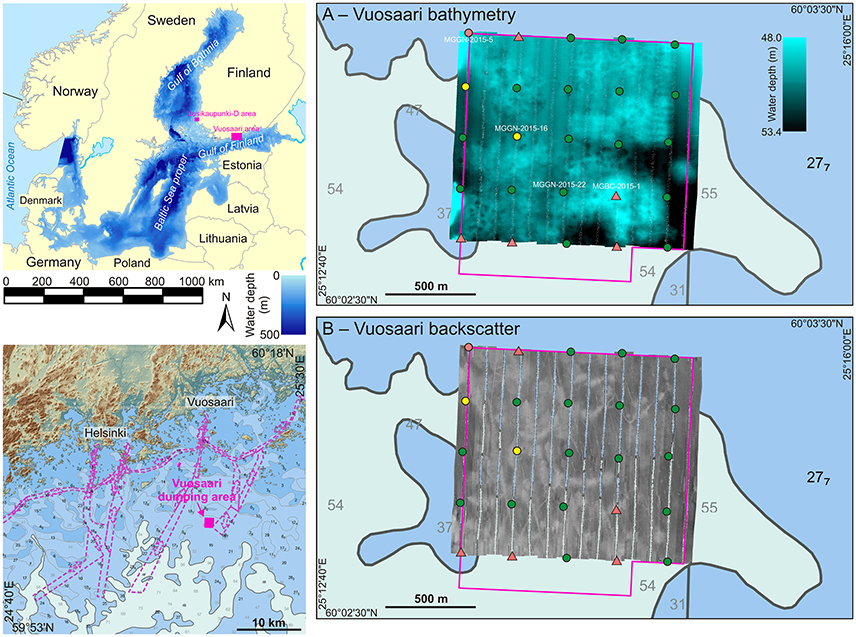
Figure 1. Maps of the Baltic Sea and the Helsinki sea area. (A) Multibeam bathymetric image over the Vuosaari dumping area. (B) Multibeam relative backscatter image over the Vuosaari dumping area. Magenta line indicates the permitted dumping area. Circle symbol: Gemax short gravity coring site. Triangle symbol: box coring site. Symbol colors: green, organic-rich mud (natural seafloor); red, dumped dredge spoil; yellow, mixture of organic-rich mud and dumped dredge spoil. Coordinate system ETRS-TM35FIN. Base map: ESRI Inc. (Redlands) data & maps of 2005. Bathymetric data: BALANCE project (www.helcom.fi). Nautical chart: S-57 Finnish Transport Agency 2017. Topographic map: National Land Survey of Finland digital elevation model 2 m 2017.
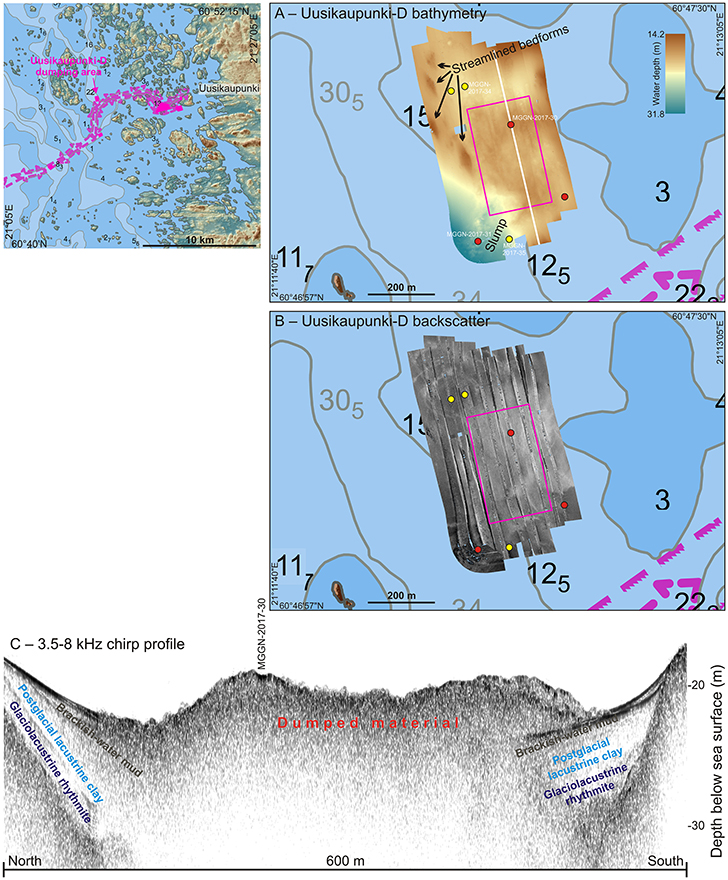
Figure 2. Maps of the Uusikaupunki sea area. (A) Multibeam bathymetric image over the Uusikaupunki-D dumping area. (B) Multibeam relative backscatter image over the Uusikaupunki-D dumping area. Magenta line indicates the permitted dumping area. White line indicates the CHIRP sub-bottom transect in (C). Circle symbol: Gemax short gravity coring site. Symbol colors: green, organic-rich mud (natural seafloor); red, dumped dredge spoil; yellow, mixture of organic-rich mud and dumped material. (C) 3.5–8 kHz CHIRP sub-bottom profile over the dumping area. The interpretation of seismo-acoustic units in the profile follows Virtasalo et al. (2007, 2014). Coordinate system ETRS-TM35FIN. Base map: ESRI Inc. (Redlands) data & maps of 2005. Bathymetric data: BALANCE project (www.helcom.fi). Nautical chart: S-57 Finnish Transport Agency 2017. Topographic map: National Land Survey of Finland digital elevation model 2 m 2017.
Study Area
The southern coastal sea areas (archipelagos) of Finland are characterized by a mosaic of numerous islands and small bays. These areas have been identified as seabed heterogeneity (i.e., geodiversity) hotspots in the Baltic Sea (Kaskela and Kotilainen, 2017). High primary productivity and sluggish near-bottom water exchange in these patchy sea areas frequently result in seasonal and longer-lasting hypoxia in local sub-basins (Virtasalo et al., 2005; Jokinen et al., 2015). The waters are annually covered by sea ice for 2–4 months, and the ice season usually ends in April (Seinä and Peltola, 1991). The sea is essentially non-tidal, but irregular water level fluctuations of up to +2.5 m occur due to variations in wind and air pressure.
The Finnish south coast was covered during the last (Weichselian) glacial maximum by the Fennoscandian continental ice-sheet, which retreated northwestwards ca. 13 thousand years ago (Stroeven et al., 2016). The ice-margin retreat was followed by the successive deposition of till, outwash, glaciolacustrine varved silt–sand and clay, postglacial lacustrine silty clay, and brackish-water organic-rich mud (Virtasalo et al., 2007, 2014). The glaciolacustrine and postglacial lacustrine sediments were deposited in deep freshwater as drapes with comparably uniform thicknesses. The superimposed brackish-water muds were deposited after the marine flooding and the establishment of brackish-water conditions with estuarine circulation in the Baltic Sea basin at ca. 7.6 thousand years ago (Eronen et al., 2001; Virtasalo et al., 2007). The brackish-water muds have lower consistency due to the increased organic-matter delivery from amplified primary production, and were thus deposited as asymmetric sediment drifts, influenced by near-bottom currents and wave action (Virtasalo et al., 2007).
The Vuosaari offshore dumping area came into use in 2003, when the construction of the Vuosaari harbor began near the city of Helsinki. The 2.3 km2 rectangular dumping area is located ca. 20 km south from the Finnish coastline, in the northern part of a local depression with water depths between 50 and 60 m (Figure 1). The site is below fairweather wave-base but above storm wave-base (Kohonen and Winterhalter, 1999). The natural seafloor, before the dumping activities, was covered by a thin (<1 m) surface layer of organic-rich brackish-water mud, which sharply overlies a 2–10 m thick layer of organic-bearing brackish-water mud with thin sand–silt stripes. In the downward order, these sediments are underlain by postglacial lacustrine silty clays, glaciolacustrine varved sand–silt and clay, and till. The crystalline bedrock surface is buried down to 60 m below the seafloor, but till and bedrock outcrops flank the dumping area in the east, west, and north (Rantataro, 1997).
The most active phase of dredging and dumping in Vuosaari was 2004–2006. The dumping ceased with the completion of the harbor construction works in 2008, when 6.14 million hopper m3 of dredge spoil had been dumped (Vatanen et al., 2012). The dredged sediments in the Vuosaari harbor area were characterized by a thin (<1 m) surface layer of organic-rich (LOI 5.7%) brackish-water mud that was underlain by a few meters of organic-bearing (LOI 2.8%) brackish-water mud. Till, boulders, and crystalline bedrock were exposed locally, and the dredged material also included blasted rock from the necessary demolitions. Contaminated sediments were stabilized in banks in the harbor area and not transported to the offshore dumping area. Environmental monitoring studies show that PCB contents were below detection limit and metal contents were at the background level except Cd that was slightly elevated in seafloor sediments at the dumping area in 2009 (Vatanen et al., 2012).
The Uusikaupunki-D offshore dumping area was in use in 2013–2014, when the shipping lane to the Uusikaupunki harbor was deepened from a draft of 10 to 12.5 m. The 0.062 km2 rectangular dumping area is located ca. 9 km west from the coastline, in a small depression with water depths between 22 and 25 m (Figure 2). The site is more or less at the fairweather wave-base. The natural seafloor in the depression before the activities was composed of a thin (<10 cm) surface layer of organic-rich brackish-water mud (LOI 6.6%), which sharply overlies compact sandy mud (Vatanen et al., 2014). The dumping area is surrounded from west, north, and east by ridges of slightly harder sediment with LOI between 2.8 and 3.6%. Altogether 0.563 million hopper m3 of dredge spoil, composed mainly of silt with minor till and blasted rock, were dumped to the area. A sediment trap study, carried out during the dumping operations, shows that suspended solids were transported to a maximum range of 600 m from the site (Vaittinen and Vartia, 2016). Turbidity peaks resulting from the dumping activities typically cleared within 45 min. Contaminated sediments in the dredged areas were stabilized in the Uusikaupunki harbor area, and not transported to the Uusikaupunki-D dumping area.
Materials and Methods
The fieldwork at the Vuosaari offshore dumping area was carried out during 2 weeks in August 2015, whereas the Uusikaupunki-D area was studied in June 2017 (Figures 1, 2). Multibeam seafloor surveys were run at 5 knots along predetermined transect lines using a 200 kHz Atlas Fansweep 20 multibeam echo sounder onboard r/v Geomari of the Geological Survey of Finland. Seismo-acoustic sub-bottom profiles were collected simultaneously using Meridata 28 kHz pinger and Massa TR-61A 3.5–8 kHz compressed high-intensity radar pulse (CHIRP) systems. The parallel survey lines were spaced at 100 m intervals in Vuosaari and at 50 m intervals in Uusikaupunki-D to permit full multibeam coverage, and orientated N-S on the basis of typical wind (wave) direction. Sound velocity and temperature profiles of the water column were measured during the survey using a Reson SVP 15T profiler. Multibeam bathymetric data were collected and processed with Hypack, and visualized with Fledermaus 7.4.4b software. A relative backscatter mosaic was produced using a GeoCoder algorithm. Sub-bottom profile data were processed and interpreted using Meridata MDPS software. The seismo-acoustic units and corresponding sediment types were interpreted following Virtasalo et al. (2007, 2014): glaciolacustrine rhythmite—a draped unit of fairly uniform thickness that is characterized by parallel internal reflectors of high amplitude; postglacial lacustrine clay—a draped unit of fairly uniform thickness that is characterized by parallel internal reflectors of low amplitude; brackish-water mud—an asymmetric drift unit of variable thickness that is characterized by downlapping and onlapping internal reflectors of low amplitude.
Sediment cores were collected in a predetermined 5 × 5 grid pattern (altogether 25 sites) in Vuosaari (Figure 1, Table 1), whereas in the smaller Uusikaupunki-D area, six sites were cored with an aim to capture the natural seafloor types present as well as the dumped material (Figure 2, Table 1). At each site, three cores were collected by two pulls using a twin barrel Gemax short gravity corer (core diameter 9 cm), which is designed for retrieving cores with undisturbed surfaces of soft sediments. One core of the first pull was cut in half lengthwise and cleaned for sedimentological description and digital photography, whereas the other core and a third core from the second pull were sectioned using a rotary device into 1 cm subsamples. After description and photography, the cleaned surface of the core MGGN-2017-34 from Uusikaupunki-D was sampled in a 28 × 5 × 2 cm plastic box for high-resolution digital X-ray imaging using a GE phoenix v|tome|x s micro-computed-tomography device supplied by GE phoenix (Wunstorf, Germany). At four sites in Vuosaari, where the substrate was too hard for the gravity corer to penetrate, a custom-made box corer, 20 × 20 cm in cross section, was used. One side of each box core was cleaned for sedimentological description and photography, after which the cores were sliced into 2 cm thick subsamples using a knife. All subsamples were stored refrigerated in plastic bags until laboratory analysis.
Subsample slices of the first-pull gravity cores were analyzed for 137Cs activity in order to determine the amount of sediment in each core that was deposited after the fallout from the 1986 Chernobyl nuclear disaster. The 137Cs activity of fresh subsamples was measured for 60 min using an EG&G Ortec ACE™-2K gamma spectrometer equipped with a four-inch NaI/TI detector at the Geological Survey of Finland (Ojala et al., 2017). The cores were analyzed starting from the uppermost 1-cm subsample slice and progressing downwards until near-zero (background) activity levels were measured in at least three consecutive subsamples. Weight loss on ignition (LOI) was analyzed for the same subsamples after the 137Cs analysis for the entire length of the gravity cores, and for the uppermost 2 cm subsamples of the box cores. LOI was determined by weighing subsamples after drying at 105°C for 16 h and weighing again after ignition at 550°C for 2 h (Bengtsson and Enell, 1986).
Grain size distribution was analyzed for selected 1-cm subsample slices of the second-pull gravity cores at the commercial laboratory Labtium Ltd. The uppermost (seafloor) subsample was selected for analysis in each core, as well as representative subsamples of each lithofacies present in the core (for lithofacies descriptions, see Results section). The subsamples were freeze dried prior to sieving by ISO 3110/1 test sieves. The <63 μm size fraction was further analyzed down to 0.6 μm using a Micromeritics Sedigraph III 5120 X-ray absorption sedimentation analyzer. The sieving results were merged with sedimentation data in Sedigraph software. Grain size distribution parameters (mean grain-size, sorting [graphic standard deviation], skewness, and kurtosis) were calculated according to the geometric Folk and Ward (1957) graphical measures implemented in GRADISTAT 4.0 software (Blott and Pye, 2001). Clay is defined as grains finer than 2 μm, whereas mud is clay and silt (<63 μm), sand is 63 μm to 2 mm, gravel is 2–64 mm, and boulder 64–2,048 mm (Blott and Pye, 2012).
Results
Vuosaari Offshore Dumping Area
Multibeam bathymetric image shows a gently undulating seafloor with water depths between 48 and 53 m over the 1,200 × 1,200 m survey area (Figure 1A). Numerous small irregular pits and mounds, a few meters to tens of meters in diameter, characterize the bottom topography. Relative backscatter displays a more complex picture of the seafloor with bright streaks and diffuse patches on a dark background (Figure 1B). The bright tone corresponds to relatively hard substrate that produces stronger acoustic backscatter, i.e. the presumed dumped material.
Of the 25 predefined coring sites, 20 were successfully sampled with the Gemax short gravity corer. Gemax did not penetrate successfully at the remaining five sites because of hard seafloor substrate, and the box corer was used instead for sampling. In the backscatter image, the box coring sites plot on the bright patches of hard seafloor (Figure 1B).
The four lithofacies that were identified in the studied sediment cores have been described in detail in previous studies in the area (Virtasalo et al., 2007, 2014). The key features of the lithofacies are recapitulated here in the upward stratigraphic order.
Postglacial lacustrine silty clay facies is represented by the lower half of the gravity core MGGN-2015-22 (Figure 3A). The facies is composed of poorly layered organic-bearing (<3.5% LOI) gray silty clay with black spots and mottling by iron monosulfides.
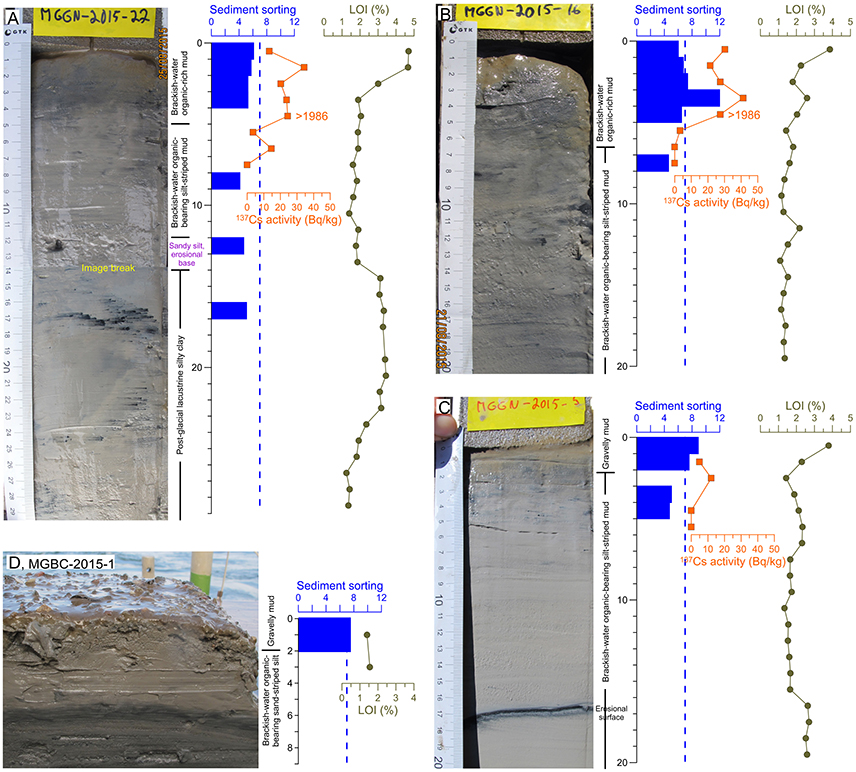
Figure 3. Digital images and the plots of grain size sorting, 137Cs activity and weight loss on ignition (LOI) of selected sediment cores from the Vuosaari dumping area. (A) Gravity core MGGN-2015-22. (B) Gravity core MGGN-2015-16. (C) Gravity core MGGN-2015-5. (D) Box core MGBC-2015-1. Dashed vertical line indicates the local threshold sorting value of 7 for dumped dredge spoil. The core locations are indicated in Figure 1A.
Brackish-water organic-bearing silt-striped mud is the most characteristic facies of the studied cores (Figure 3). The facies is gray with thin silt stripes and diffuse dark mottling by iron monosulfides, and small macrofauna burrows in particular in the upper part of the cores. The facies also contains clay balls and less frequent sand stripes. The contact to the underlying postglacial lacustrine silty clays in MGGN-2015-22 is erosional and with a 2 cm thick basal sandy silt layer that gradates upward to the brackish-water mud (Figure 3A). This basal sandy silt layer is a transgressive shelf sand sheet, which is a widespread stratigraphic feature in the region, resulting from the mid-Holocene marine flooding of the Baltic Sea Basin (Virtasalo et al., 2016).
Brackish-water organic-rich mud is the uppermost and loosest facies in most of the studied cores (Figures 3A,B). The facies is olive green and intensely mottled by small macrofauna burrows. The sediment is soft and with a high water content and LOI of c. 4% or higher. The basal contact to the brackish-water organic-bearing silt-striped mud is sharp to bioturbated. When at the core top, the facies has a ca. 1 cm thick fluffy brown oxidized surface layer that may locally have high sand content.
Gravelly mud facies is found at the top of the gravity core MGGN-2015-5, as well as in all of the box cores (Figures 3C,D). The gray-colored mud facies has a massive texture and contains angular to jagged gravel-sized particles and small boulders. The facies is relatively hard because of the coarse grains present, which probably is the reason for the poor success with gravity coring. The basal contact is sharp and with frequent load structures (Figure 3D). When at the core top, the facies often has a fluffy brown oxidized surface layer.
Cesium-137 activity in the sediment cores from the Vuosaari dumping area is low (<100 Bq/kg, Figure 3) compared to the present values exceeding 1,000 Bq/kg in the sediments that were deposited off the Finnish south coast soon after the 1986 Chernobyl nuclear disaster (e.g., Jokinen et al., 2015; Vallius, 2015). Furthermore, the 137Cs curves do not display well-developed activity peaks that would denote the year of fallout. Therefore, the 137Cs values above the pre-fallout values with the threshold of c. 20 Bq/kg are taken as indicative of sediments younger than 1986. Indeed, the overall comparably low 137Cs activity, together with the low consistency and low thickness (<6 cm) of the 137Cs-enriched sediment surface layer, suggest that the 137Cs-enriched sediments in the studied cores were deposited sometime after 1986, possibly during the past few years.
Sediments from the Vuosaari dumping area are composed of mixed grain sizes, as is shown by the poor to very poor sorting of the sub-samples, and there is a strong relationship between improved sorting and smaller grain size (Figure 5A). In particular, there is a very poorly sorted sub-population with values of larger than 7 and mean grain sizes exceeding 12 μm. Most of the sub-samples have a balanced grain size distribution (symmetrical skewness), but several sub-samples are dominated by coarse particles (coarse/negative skewed) and some by fine particles (fine/positive skewed; Figure 5B). Kurtosis of the most grain size distributions is platykurtic (negative), but there is a leptokurtic (positive) sub-population with primarily heavy-tailed distributions (DeCarlo, 1997). The predominantly symmetrical skewness with a wide range of values is indicative of fluctuating energy levels with intermittent erosion and deposition of particles from mixed sediment sources (Duane, 1964). The coarsest, worst sorted and leptokurtic subsamples are interpreted to be from dumped material; these grain size characteristics reflect admixing of coarse grains and little sorting during fall through the water column.
Uusikaupunki-D Offshore Dumping Area
Multibeam bathymetric image over the survey area shows a relatively flat seafloor with water depths between 18 and 21 m, except streamlined bedforms that rise up to 15 m water depth, and a NW-SE trending channel with water depths of more than 30 m in the southwest (Figure 2A). The seafloor is notably smooth, except the dumping area that has irregular topography at the meter scale. A slump in the southwest documents the flow of material to the channel. Relative backscatter image shows patchy texture with generally brighter tones over the dumping area and also to the north and east of the area, indicating a harder consistency of the dumped material compared to the natural seafloor, and that not all dumped material actually sit on the permitted dumping area (Figure 2B). The dumped material mounds are well distinguishable in the sub-bottom profiles based on the poor penetration of the seismo-acoustic signal and the irregular surface morphology (Figure 2C).
The two lithofacies present in the cores from the Uusikaupunki-D dumping area are similar to those in the Vuosaari area. Their key characteristics are as follows:
Brackish-water organic-rich mud is the uppermost and loosest facies in the studied cores (Figure 4). The facies is olive green and intensely mottled by small macrofauna burrows. The primary physical sedimentary structure is preserved locally in short thin-bedded intervals with smothered bed contacts. Diffuse dark patches of presumably iron monosulfide are present. The sediment is soft, and its organic content of ca. 3% is higher than in the underlying sediments but lower than in brackish-water organic-rich mud in the Vuosaari dumping area. The facies comprises the whole core MGGN-2017-35 (Figure 4B), where it is underlain by a compact sand layer at the 12 cm depth that was not penetrated by the Gemax corer. The facies is present in the basal part of MGGN-2017-34 below the depth of ca. 26 cm where it is sharply truncated from top, as well as in the uppermost 5 cm of that core (Figure 4A). When at the core top, the facies has a ca. 1 cm thick fluffy brown oxidized surface layer.
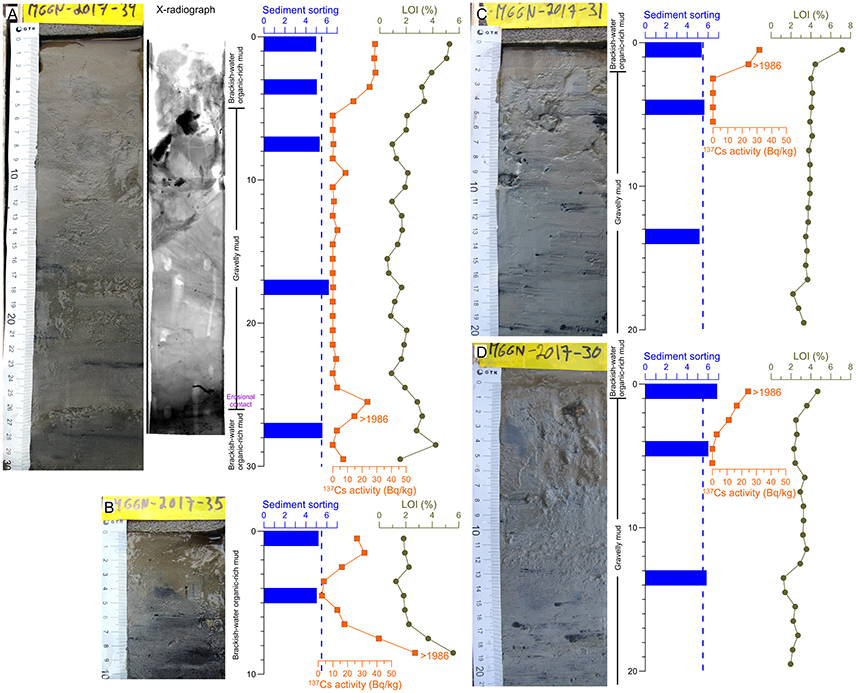
Figure 4. Digital images and the plots of grain size sorting, 137Cs activity and weight loss on ignition (LOI) of selected sediment cores from the Uusikaupunki-D dumping area. (A) Gravity core MGGN-2017-34. (B) Gravity core MGGN-2017-35. (C) Gravity core MGGN-2017-31. (D) Gravity core MGGN-2017-30. Dashed vertical line indicates the local threshold sorting value of 5.5 for dumped dredge spoil. The core locations are indicated in Figure 2A.
Gravelly mud facies is found in almost all of the studied cores. The gray-colored facies has a massive structure with local dark monosulfide spots and patches (Figure 4). The grain size is dominated by mud, but the presence of larger grains including angular to jagged gravel (Figure 6C) results in comparably hard consistency. The facies is present in MGGN-2017-34 between 26 and 5 cm, where it has an erosional contact to the underlying brackish-water mud (Figure 4A). X-radiograph of this interval shows that the sediment is strongly deformed and includes rotated sediment clasts. Dislocated sediment clasts are also present in MGGN-2017-31 (Figure 4C). When at the core top, the facies often has a fluffy brown oxidized surface layer.
Cesium-137 activity in the Uusikaupunki-D cores (<100 Bq/kg, Figure 4) is at the similar low level than in the Vuosaari dumping area. Together with the low consistency and low thickness (<6 cm) of the 137Cs-enriched sediment surface layer, this suggests that the 137Cs-enriched sediments in the cores were deposited during the past few years. Notably, 137Cs-enriched brackish-water organic-rich mud is erosionally overlain by gravelly mud at 26 cm in MGGN-2017-34 (Figure 4A). The underlying brackish-water mud layer is younger than 1986, and the gravelly mud layer was emplaced on it during dumping activities. The gravelly mud layer has since been buried by 5 cm of organic-rich brackish-water mud.
Sorting of the sediment subsamples is very poor, which is similar to the majority of subsamples from Vuosaari (Figure 6A). The relationship between improved sorting and smaller grain size is weaker than in the Vuosaari subsamples. The grain size distributions are symmetrical or fine skewed, except a couple of subsamples that are coarse skewed (Figure 6B). Kurtosis of the subsamples is mostly platykurtic. There is a very poorly sorted sub-population with values exceeding 5.5 and with kurtosis higher than 0.72; these subsamples are interpreted to be from dumped material, with their grain size distributions reflecting admixing of coarse grains and minimal sorting during fall through the water column.
Discussion
Two offshore dumping areas, Vuosaari in the south coast and Uusikaupunki-D in the west coast of Finland, have been studied with an aim to develop observation techniques and criteria for the assessment of the geological integrity of seafloor, its anthropogenic change due to dumping, and recovery as required by the MSFD. Full coverage multibeam bathymetry and relative backscatter data as well as a number of sediment cores have been analyzed over the dumping areas.
Sedimentary Environment
Adequate knowledge about sedimentary environment of an offshore dumping area is necessary for understanding the fate of the dumped dredge spoil, which can be resuspended by currents and transported to adjacent locations (dispersive site), or buried by natural sediment deposition (depositional site).
Based on the analysis of sediment cores, the Vuosaari dumping area is net depositional area, although the sediment deposition is slow and episodic. The local seafloor is composed of brackish-water silt-striped organic-bearing mud that is covered with a thin layer of soft brackish-water organic-rich mud (Figure 3). The silt stripes and less frequent sand stripes in the organic-bearing mud indicate that episodic strong near-bottom currents result in seafloor erosion and the deposition of thin silt and sand layers from traction transport. The poor sorting and the predominantly symmetrical skewness of both the organic-bearing and organic-rich brackish-water mud (Figure 5) further indicate importation of particles from mixed sediment sources (Duane, 1964; Stevens et al., 1996). These particles originate from till, glaciofluvial sand formations, glaciolacustrine varved silt–sand and clay, postglacial lacustrine silty clay and previously deposited brackish-water mud in the area (Rantataro, 1997; Virtasalo et al., 2007, 2014). This observation is in line with previous studies, which show that resuspension accounts for a considerable proportion of deposition in the regressive coastal sea areas of the northern Baltic Sea (Jokinen et al., 2015; Rasmus et al., 2015; Nuorteva and Kankaanpää, 2016).
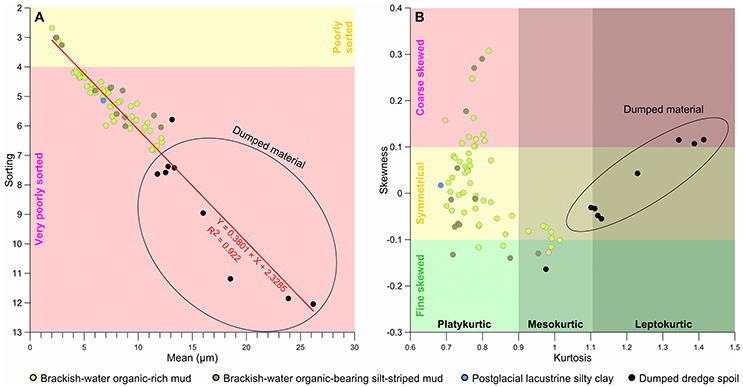
Figure 5. Plots for the total-sample size distributions of (A) sorting and mean, and (B) skewness and kurtosis from the Vuosaari dumping area. Sample classes follow lithofacies classification described in the text. The sorting, skewness, and kurtosis class limits follow geometric Folk and Ward (1957) graphical measures as modified by Blott and Pye (2001).
The comparably low 137Cs activity and the lack of well-developed peaks in the 137Cs curves from the Vuosaari gravity cores (Figure 3) suggest that the thin surficial organic-rich mud layer was deposited during the past few years, and that the contact to the underlying more compact silt-striped organic-bearing mud is likely to be unconformable. Recent studies show that 137Cs is still being redistributed and deposited in significant amounts in the Finnish coastal sea areas (Jokinen et al., 2015; Vallius, 2015). The exact cause of increased organic-content in the recently deposited mud is not known, but it may be related to the recent increase in organic sedimentation as a consequence of eutrophication (Bonsdorff et al., 1997; Weckström, 2006; Tuovinen et al., 2010).
The Uusikaupunki-D dumping area shows characteristics of both dispersive and depositional site. The presence of streamlined bedforms on the relatively shallow seafloor indicate strong reworking by waves and near-bottom currents (Figure 2A). The seafloor is covered with a patchy and comparably thin (<20 cm) layer of brackish-water organic-rich mud. The underlying, older and more compact sandy mud, postglacial lacustrine and glaciolacustrine sandy–silty clays, and till are exposed in the surrounding ridges and local elevations. The very poor sorting and mostly symmetrical skewness of the thin organic-rich brackish-water mud blanket indicate that it to a large part is composed of material reworked from previously deposited local sediments (Duane, 1964; Stevens et al., 1996). The low 137Cs activity and the lack of well-developed peaks in the 137Cs curves further indicate that the brackish-water mud blanket was deposited during the recent past and may to a large degree be semi-permanent. These findings are in agreement with a recent study by Vatanen et al. (2014), which shows that recent sediment deposition in the area is minor and restricted to shallow local depressions.
Dumped Material
At the Vuosaari offshore dumping area, the mounds of dumped dredge spoil, possibly enhanced by scouring around the individual mounds (Du Four and Van Lancker, 2008), result in irregular seafloor topography (Figure 1A). In addition, the dumped dredge spoil stands out bright among natural sediments in the multibeam backscatter image because of its harder consistency and irregular surface morphology (Figure 1B). In the studied sediment cores, the dumped material is represented by the gravelly mud facies with massive texture and angular to jagged coarse particles, and an unconformable contact to the underlying sediments (Figure 3). The dumped material in general is coarser, less sorted and of higher kurtosis compared to natural sediment (Figure 5), as a result of the admixing of coarser grains during the dredging operations, and limited sorting during fall through the water column upon dumping. Dumped dredge spoil also has lower 137Cs activity than natural seafloor sediments because most of the dredged sediments are older than the 1986 Chernobyl disaster. During the 7 years after the cessation of dumping activities, the dumped material at many of the coring sites has been covered by few centimeters of younger sediments, which shows that it is largely stable in the Vuosaari dumping area.
The Uusikaupunki-D dumping area is located in a small depression, which has been filled to the level of the surrounding till and hard mud ridges. The seafloor topography at the dumping area is irregular and the dumped material has brighter tone in the multibeam backscatter image compared to the surrounding natural seafloor, which has been smoothed and streamlined by current activity (Figure 2). The dumped material has a mixed texture with dislocated mud clasts, which is different from Vuosaari and probably results from the shorter fall through the shallow water column upon dumping (Figures 4A,C). The poor sorting and higher kurtosis of dumped material compared to natural sediment is similar to Vuosaari, and further indicates the admixing of coarser grains during dredging and minimal sorting during fall through the water column (Figure 6). Irregularities in the dumped material surface will likely be leveled off and the eroded material dispersed in the surrounding area by strong near-bottom currents (Tauber, 2009). Mean current velocities, measured 2 m above seafloor during 4 weeks in October 2014, occasionally exceeded 30 cm/s (Vaittinen and Vartia, 2016), which is well above the threshold for erosion of the muddy seafloor (Rasmus et al., 2015). A sediment core shows that the dumped material has been covered by 1 cm layer of natural sediment during the 3 years after the dumping activities, but the site would need to be revisited after a few years to determine the permanence of this very thin covering layer. In addition, dumped material has slumped down to the adjacent channel (Figure 2A), where it likely will be transported further by strong near-bottom currents.
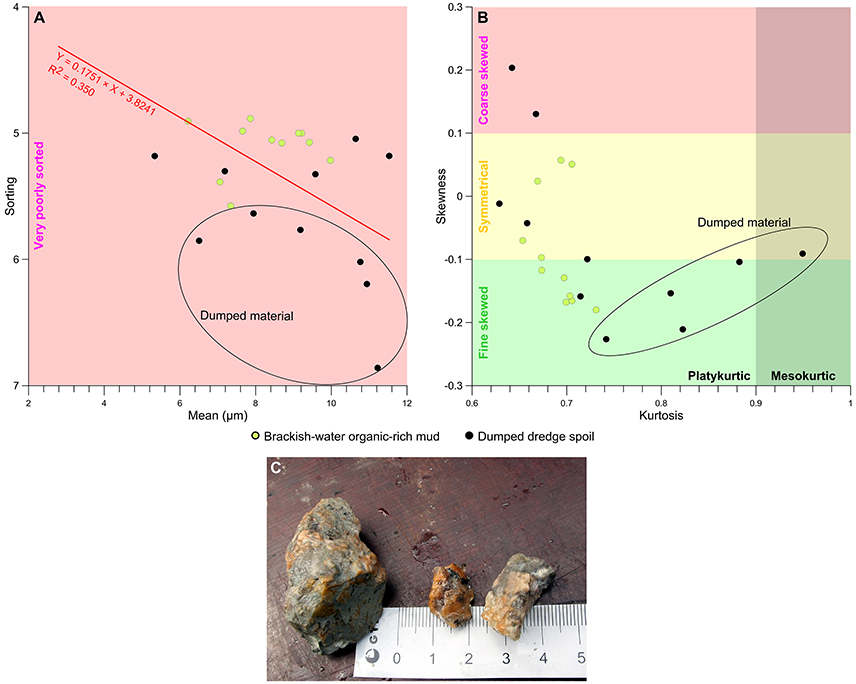
Figure 6. Plots for the total-sample size distributions of (A) sorting and mean, and (B) skewness and kurtosis, and (C) a picture of blasted rock fragments from the Uusikaupunki-D dumping area. Sample classes follow lithofacies classification described in the text. The sorting, skewness, and kurtosis class limits follow geometric Folk and Ward (1957) graphical measures as modified by Blott and Pye (2001).
The dumped material is not always readily identifiable to the naked eye. In the core MGGN-2015–16 from Vuosaari, a poorly-sorted thin layer of dumped material at 3–4 cm is difficult to tell apart from brackish-water sediments immediately above and below based on the visual inspection alone (Figure 3B). Similarly, in the core MGGN-2017-35 from Uusikaupunki-D, upward decreasing organic content and a nadir in 137Cs activity at 3–5 cm indicate a significant share of admixed old material (Figure 4B). The admixed dumped material probably was dispersed in the water column during the dumping activities, or resuspended from a dumped material mound and transported along the seafloor by near-bottom currents soon thereafter (Truitt, 1988; Du Four and Van Lancker, 2008; Marmin et al., 2016).
Assessment of Seafloor Geological Integrity
In Europe, the GES of seafloor integrity is assessed through the terms “physical loss of seabed” and “adverse effects from physical disturbance to seabed” (European Commission, 2017). These two stressors are defined by changes in the seabed substrate and morphology, and distinguished by recovery time; seabed that requires more than 10 years to recover is considered as “physically lost” in the GES assessment.
In order to address the requirement for the assessment of the physical loss and disturbance of seabed substrate and morphology, an assessment protocol for the geological integrity of seafloor substrate in the context of offshore dumping is proposed (Figure 7). The proposed assessment protocol is based on the successful discrimination of dumped dredge spoil and naturally deposited sediments by the observation techniques used in the two offshore dumping areas. In this approach, geological integrity is understood as the capability of seafloor to provide a habitable substrate for indigenous benthic communities. The proposed protocol includes two assessment methods. Seabed seismo-acoustic survey allows quick identification of dumped material mounds and their areal coverage on the seafloor, and repeated surveys permit the assessment of their possible reworking and burial (e.g., Stockmann et al., 2009; Tauber, 2009). Sediment core analysis permits ground-truthing of seismo-acoustic data, and detailed determination of the thickness and quality of the dumped material layer and its possible burial by natural sediment at the coring locations. The two methods ideally should be used in parallel with more weight given on the sediment core analysis.
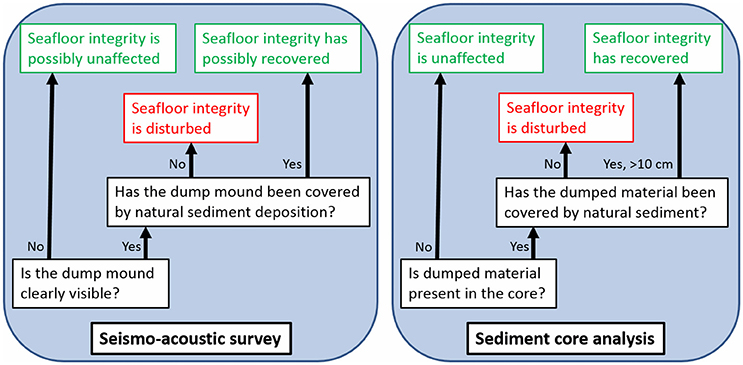
Figure 7. The proposed assessment protocol of the seafloor geological integrity. Seismo-acoustic surveys permit the estimation of the areal coverage of dumped material, whereas sediment core analysis is more informative about the degree of physical change in seafloor substrate and the state of possible recovery. These methods should preferably be used in parallel with more weight given on the sediment core data.
In the proposed assessment protocol (Figure 7), the burial of dumped dredge spoil by natural sediment is considered as a prerequisite for the recovery of the seafloor geological integrity. This is obviously possible at depositional dumping areas only, whereas at dispersive sites the dumped material will remain exposed and be redistributed along the seafloor, as is commonly the case with dumping sites in tidal high-energy sandy seafloor environments (e.g., Stronkhorst et al., 2003; Wienberg and Hebbeln, 2005; Du Four and Van Lancker, 2008; Marmin et al., 2016), and largely also in the Uusikaupunki-D dumping area. The required thickness of the natural sediment cover for the recovery will depend on the species specific requirements in the local macrobenthic community, and will differ considerably between locations. Already a relatively thin layer of natural sediment may make the seafloor appear as a natural substrate for macrofauna in the northern Baltic Sea, where the macrozoobenthic communities are dominated by small organisms with shallow burrowing depths due to considerable environmental stress caused by low salinity with further aggravation in areas of oxygen deficiency (Elmgren et al., 1984; Bonsdorff et al., 1996; Laine et al., 2007; Virtasalo et al., 2011). Therefore, the burial of dumped material by 10 cm of natural sediment is proposed as a rule-of-thumb criterion for the recovery of the seafloor geological integrity that is applicable over wide areas in the northern Baltic Sea.
In the case the dumped material is not buried by natural sediment (dispersive site), the new macrozoobenthic community that may establish on the site will differ from the undisturbed one, and a new steady state will appear. The magnitude of change in the macrozoobenthic community will depend on how closely the dumped material mounds resemble the natural seafloor in terms of e.g., grain size, organic content, and consistency (e.g., Bolam et al., 2006; Powilleit et al., 2006; Barrio Froján et al., 2011). The re-establishment of the macrozoobenthic community often takes a few years if analyzed by univariate indices but at least 5 years when analyzed by multivariate analyses of the species composition (Bolam et al., 2006; Barrio Froján et al., 2011). By the strictest (and likely unrealistic) interpretation of the “physical loss,” the geological integrity of seafloor in such a case would be lost forever unless restored by human intervention (European Commission, 2017). However, a seafloor that does provide a habitat to an indigenous macrozoobenthic community, although different from the earlier one, should hardly be considered “lost.” Therefore, the “physical loss” may require a practical definition, which could be based on macrozoobenthic or other biological condition of the site. This method could contain an estimate of the potential ecological value of such an artificial habitat. It should be noted that its ecological value is not necessarily less than that of a natural seafloor.
In addition to the seafloor geological properties, the re-establishment of a macrozoobenthic community is controlled by other abiotic factors such as oxygen saturation. The macrozoobenhic community at the Vuosaari offshore dumping area disappeared because of the dumping activities, but the accurate impact of dumping in the reduction is difficult to estimate due to the episodic hypoxia that had set in just before the dumping operations (Vatanen et al., 2012). In the surrounding areas 0.6–1.2 km from the site, the communities were only slightly affected. In 2009, a year after the cessation of dumping and under improved oxygen conditions, macrozoobenthic density increased to the level of the surrounding areas and the community included the amphipod Monoporeia affinis, the bivalve Macoma balthica, the priapulid Halicryptus spinulosus, and the invasive polychaete Marenzelleria spp. The shallower Uusikaupunki-D dumping area, in contrast, is generally well oxygenated, but no macrozoobenthic studies on the effects of dumping have been carried out there (Vatanen et al., 2014).
Sediment core analysis is the only means of gaining detailed information about the geological integrity of seafloor, its change due to human activities such as dumping, and its possible recovery. The analysis requires scrutiny, such as the analysis of 1 cm vertical subsamples of gravity cores with geochronological control that is carried out here (Figures 3, 4). A similar analysis is not possible when the sediment samples are collected by grab samplers, which are typically used for macrozoobenthic sampling and mix the sediment structure to a large extent (e.g., Olenin, 1992; Powilleit et al., 2006; Okada et al., 2009; McLaren and Teear, 2014; Marmin et al., 2016). The selection of the monitoring method can, however, be determined on the basis of the known characteristics of the site. In case the dumping history is known and the site is of dispersive type, then sediment core analysis may not be required and macrozoobenthic sampling may be sufficient. Such knowledge about the sites will considerably decrease monitoring costs.
Conclusions
Knowledge about reference conditions before significant human influence is needed to determine the seafloor physical (geological) integrity, and to set targets for achieving good environmental status of the EU's marine waters within the MSFD (European Commission, 2017). Techniques and concepts presented in this paper provide a means for discriminating uncontaminated dumped dredge spoil from sediments that were deposited naturally at a dumping area, both before the dumping activities and after them in the possible case that recovery has taken place. The analysis of grain size distribution parameters and lithofacies characteristics of sediment cores allows the identification of (1) dumped dredge spoil sensu stricto that rapidly and with minimal sorting fell through the water column during the dumping activities, and (2) dispersed dredge spoil that was suspended in the water column during the dumping activities, or reworked from the dumped material mounds and redistributed along the seafloor soon after the dumping activities, and deposited over a wider area as a thin layer that is not necessarily readily identifiable to the naked eye. Radiochronological techniques such as 137Cs dating help distinguish the dumped material in sediment cores.
Following the proposed assessment protocol (Figure 7), multibeam data indicate the presence of dumped material mounds at the Vuosaari dumping area, and sediment core analyses document shallow burial of the dumped material by natural sediment deposition at some of the coring locations. These findings show that slow recovery of the seafloor geological integrity is taking place at the area. However, the dumped material has not been buried by at minimum 10 cm of natural sediment, meaning that the seafloor has not yet fully recovered at any of the coring sites during the seven years after the dumping activities. It remains to be seen whether the seafloor is “adversely affected” or “physically lost” according to the GES assessment. The dump mounds are visible in multibeam data over the Uusikaupunki-D dumping area, as well. Sediment core analyses show that little natural sediment has deposited on the dumped material during the 3 years since the dumping activities ceased. Taking into the consideration the highly dynamic sedimentary environment of the Uusikaupunki-D dumping area, the seafloor would seem “physically lost.”
Anthropogenic disturbance studies should be accompanied by detailed geological investigation, in order to comprehensively assess the consequent physical changes in the structure and consistency of the seafloor substrate. The proposed assessment protocol for the seafloor geological integrity is meant to give a more accurate overview of the extent of disturbance and the possible physical recovery of the seafloor substrate after dumping operations. The protocol can be used to support studies of the re-establishment of macrozoobenthic communities.
Author Contributions
JV: responsible for the sample collection, analysis, and the results; SK: contributed to writing about EU directives and benthic ecology, and to the development of the assessment protocol; AK: support in sample collection and interpretation of results.
Conflict of Interest Statement
The authors declare that the research was conducted in the absence of any commercial or financial relationships that could be construed as a potential conflict of interest.
Acknowledgments
We acknowledge the crew of our r/v Geomari and those who assisted in the sediment sampling. Kimmo Alvi prepared the multibeam images. Jukka Kuva produced the X-radiograph in Figure 4A. This work has been partially supported by the Strategic Research Council at the Academy of Finland, project SmartSea (grant 292 985).
References
Barrio Froján, C. R. S., Cooper, K. M., Bremner, J., Defew, E. C., Wan Hussin, W. M. R., and Paterson, D. M. (2011). Assessing the recovery of functional diversity after sustained sediment screening at an aggregate dredging site in the North Sea. Estuar. Coast. Shelf Sci. 92, 358–366. doi: 10.1016/j.ecss.2011.01.006
Bengtsson, L., and Enell, M. (1986). “Chemical analysis,” in Handbook of Holocene Palaeoecology and Palaeohydrology, ed D. E. Berglund (Chichester, UK: Wiley), 423–451.
Berg, T., Fürhaupter, K., Teixeira, H., Uusitalo, L., and Zampoukas, N. (2015). The Marine Strategy Framework Directive and the ecosystem-based approach – pitfalls and solutions. Mar. Pollut. Bull. 96, 18–28. doi: 10.1016/j.marpolbul.2015.04.050
Blott, S. J., and Pye, K. (2001). GRADISTAT: a grain size distribution and statistics package for the analysis of unconsolidated sediments. Earth Surf. Process. Landforms 26, 1237–1248. doi: 10.1002/esp.261
Blott, S. J., and Pye, K. (2012). Particle size scales and classification of sediment types based on particle size distributions: review and recommended procedures. Sedimentology 59, 2071–2096. doi: 10.1111/j.1365-3091.2012.01335.x
Bobertz, B., and Harff, J. (2004). Sediment facies and hydrodynamic setting: a study in the south western Baltic Sea. Ocean Dyn. 54, 39–48. doi: 10.1007/s10236-003-0061-8
Bolam, S. G., Rees, H. L., Somerfield, P., Smith, R., Clarke, K. R., Warwick, R. M., et al. (2006). Ecological consequences of dredged material disposal in the marine environment: a holistic assessment of activities around the England and Wales coastline. Mar. Pollut. Bull. 52, 415–426. doi: 10.1016/j.marpolbul.2005.09.028
Bonsdorff, E., Blomqvist, E. M., Mattila, J., and Norkko, A. (1997). Long-term changes and coastal eutrophication. examples from the Åland Islands and the Archipelago sea, Northern Baltic sea. Oceanol. Acta 20, 319–329.
Bonsdorff, E., Diaz, R. J., Rosenberg, R., Norkko, A., and Cutter, G. R. Jr. (1996). Characterization of soft-bottom benthic habitats of the Åland Islands, northern Baltic Sea. Mar. Ecol. Prog. Ser. 142, 235–245. doi: 10.3354/meps142235
Broszeit, S., Beaumont, N. J., Uyarra, M., Heiskanen, A.-S., Frost, M., Somerfield, P. J., et al. (2017). Whan can indicators of good environmental status tell us about ecosystem services?: reducing efforts and increasing cost-effectiveness by reapplying biodiversity indicator data. Ecol. Indic. 81, 409–442. doi: 10.1016/j.ecolind.2017.05.057
DeCarlo, L. T. (1997). On the meaning and use of kurtosis. Psychol. Methods 2, 292–307. doi: 10.1037/1082-989X.2.3.292
Duane, D. B. (1964). Significance of skewness in recent sediments, western Pamlico Sound, North Carolina. J. Sediment. Petrol. 34, 864–874.
Du Four, I., and Van Lancker, V. (2008). Changes of sedimentological patterns and morphological features due to the disposal of dredge spoil and the regeneration after cessation of the disposal activities. Mar. Geol. 255, 15–29. doi: 10.1016/j.margeo.2008.04.011
Elmgren, R., Rosenberg, R., Andersin, A.-B., Evans, S., Kangas, P., Lassig, J., et al. (1984). Benthic macro- and meiofauna in the Gulf of Bothnia (northern Baltic). Finnish Mar. Res. 250, 3–18.
Eronen, M., Glückert, G., Hatakka, L., van de Plassche, O., van der Plicht, J., and Rantala, P. (2001). Rates of Holocene isostatic uplift and relative sealevel lowering of the Baltic in SW Finland based on studies of isolation contacts. Boreas 30, 17–30. doi: 10.1080/030094801300062248
Essink, K. (1999). Ecological effects of dumping of dredged sediments; options for management. J. Coast. Conserv. 5, 69–80. doi: 10.1007/BF02802741
European Commission (2017). Commission Decision (EU) 2017/848 of 17 May 2017 laying down criteria and methodological standards on good environmental status of marine waters and specifications and standardised methods for monitoring and assessment, and repealing Decision 2010/477/EU. Off. J. Eur. Union L125, 43–74.
Folk, R. L., and Ward, W. C. (1957). Brazos river bar: a study in the significance of grain size parameters. J. Sediment. Petrol. 27, 3–26. doi: 10.1306/74D70646-2B21-11D7-8648000102C1865D
HELCOM (2015). HELCOMGuidelines for Management of Dredged Material at Sea and HELCOMReporting Format for Management of Dredged Material at Sea. Helsinki: HELCOMBaltic Marine Environment Protection Commission.
HELCOM (2017). First version of the ‘State of the Baltic Sea’ report – June 2017 – to be Updated in 2018. Helsinki: HELCOM Baltic Marine Environment Protection Commission. Available online at: http://stateofthebalticsea.helcom.fi
IMO (2015). Guidelines for Low Cost, Low Technology Assessment of Dredged Material. London: International Maritime Organization.
Jokinen, S. A., Virtasalo, J. J., Kotilainen, A. T., and Saarinen, T. (2015). Varve microfabric record of seasonal sedimentation and bottom flow-modulated mud deposition in the coastal northern Baltic Sea. Mar. Geol. 366, 79–96. doi: 10.1016/j.margeo.2015.05.003
Kairyte, M., and Stevens, R. L. (2015). Composite methodology for interpreting sediment transport pathways from spatial trends in grain size: a case study of the Lithuanian coast. Sedimentology 62, 681–696. doi: 10.1111/sed.12156
Kaskela, A. M., and Kotilainen, A. T. (2017). Seabed geodiversity in a glaciated shelf area, the Baltic Sea. Geomorphology 295, 419–435. doi: 10.1016/j.geomorph.2017.07.014
Kohonen, T., and Winterhalter, B. (1999). Sediment erosion and deposition in the western part of the Gulf of Finland. Baltica 12, 53–56.
Korpinen, S., Meidinger, M., and Laamanen, M. (2013). Cumulative impacts on seabed habitats: an indicator for assessments of good environmental status. Mar. Pollut. Bull. 74, 311–319. doi: 10.1016/j.marpolbul.2013.06.036
Korpinen, S., Meski, L., Andersen, J. H., and Laamanen, M. (2012). Human pressures and their potential impact on the Baltic sea ecosystem. Ecol. Indic. 15, 105–114. doi: 10.1016/j.ecolind.2011.09.023
Laine, A. O., Andersin, A.-B., Leiniö, S., and Zuur, A. F. (2007). Stratification-induced hypoxia as a structuring factor of macrozoobenthos in the open Gulf of Finland (Baltic Sea). J. Sea Res. 57, 65–77. doi: 10.1016/j.seares.2006.08.003
Lepland, A., Bøe, R., Lepland, A., and Totland, O. (2009). Monitoring the volume and lateral spread of disposed sediments by acoustic methods, Oslo Harbor, Norway. J. Environ. Manage. 90, 3589–3598. doi: 10.1016/j.jenvman.2009.06.013
Marmin, S., Lesueur, P., Dauvin, J. C., Samson, S., Tournier, P., Gallicher Lavanne, A., et al. (2016). An experimental study on dredge spoil of estuarine sediments in the Bay of Seine (France): a morphosedimentary assessment. Cont. Shelf Res. 116, 89–102. doi: 10.1016/j.csr.2016.01.010
McLaren, P., and Teear, G. (2014). A Sediment Trend Analysis (STA®) in support of dredged material management in Lyttelton Harbour, Christchurch, New Zealand. J. Coast. Res. 30, 438–447. doi: 10.2112/JCOASTRES-D-13-00136.1
Nuorteva, J., and Kankaanpää, H. T. (2016). Relocation of soft mud deposits: an example from the Archipelago Sea, northern Baltic Sea. Mar. Geol. 380, 148–162. doi: 10.1016/j.margeo.2016.08.002
Ojala, A. E. K., Luoto, T. P., and Virtasalo, J. J. (2017). Establishing a high-resolution surface sediment chronology with multiple dating methods – testing 137Cs determination with Nurmijärvi clastic-biogenic varves. Quat. Geochronol. 37, 32–41. doi: 10.1016/j.quageo.2016.10.005
Okada, T., Larcombe, P., and Mason, C. (2009). Estimating the spatial distribution of dredged material disposed of at sea using particle-size distributions and metal concentrations. Mar. Pollut. Bull. 58, 1164–1177. doi: 10.1016/j.marpolbul.2009.03.023
Olenin, S. (1992). “Changes in a south-eastern Baltic soft-bottom community induced by dredged spoil dumping,” in Proceedings 12th Baltic Marine Biologist Symposium, eds E. Bjørrnestad, L. Hagerman, and K. Jensen (Fredensborg: Olsen & Olsen), 119–123.
OSPAR (2014). OSPARGuidelines for the Management of Dredged Material at Sea, Agreement 2014-06. London: OSPARCommission.
Powilleit, M., Kleine, J., and Leuchs, H. (2006). Impacts of experimental dredged material disposal on a shallow, sublittoral macrofauna community in Mecklenburg Bay (western Baltic Sea). Mar. Pollut. Bull. 52, 386–396. doi: 10.1016/j.marpolbul.2005.09.037
Rantataro, J. (1997). Itä-Tontun ja Soratontun Hiekka-Alueiden Sekä Eesti-Luodon Eteläpuolisen Läjitysalueen Akustis-Seismiset Tutkimukset. Espoo: Geological Survey of Finland.
Rasmus, K., Kiirikki, M., and Lindfors, A. (2015). Long-term field measurements of turbidity and current speed in the Gulf of Finland leading to an estimate of natural resuspension of bottom sediment. Boreal Environ. Res. 20, 735–747.
Rice, J., Arvanitidis, C., Borja, A., Frid, C., Hiddink, J. G., Krause, J., et al. (2012). Indicators for sea-floor integrity under the European Marine Strategy Framework Directive. Ecol. Indic. 12, 174–184. doi: 10.1016/j.ecolind.2011.03.021
Seinä, A., and Peltola, J. (1991). Duration of the ice season and statistics of fast ice thickness along the Finnish coast 1961–1990. Finnish Mar. Res. 258, 1–46.
Stevens, R. L., Bengtsson, H., and Lepland, A. (1996). Textural provinces and transport interpretations with fine-grained sediments in the Skagerrak. J. Sea Res. 35, 99–110. doi: 10.1016/S1385-1101(96)90739-X
Stockmann, K., Riethmüller, R., Heineke, M., and Gayer, G. (2009). On the morphological long-term development of dumped material in a low-energetic environment close to the German Baltic coast. J. Mar. Syst. 75, 409–420. doi: 10.1016/j.jmarsys.2007.04.010
Stroeven, A. P., Hättestrand, C., Kleman, J., Heyman, J., Fabel, D., Fredin, O., et al. (2016). Deglaciation of Fennoscandia. Quat. Sci. Rev. 147, 91–121. doi: 10.1016/j.quascirev.2015.09.016
Stronkhorst, J., Ariese, F., van Hattum, B., Postma, J. F., de Kluijver, M., Den Besten, P. J., et al. (2003). Environmental impact and recovery at two disposal sites for dredged material in the North Sea. Environ. Pollut. 124, 17–31. doi: 10.1016/S0269-7491(02)00430-X
Tauber, F. (2009). Sidescan sonar survey of a dumping site in the Mecklenburg Bight (south-western Baltic Sea). J. Mar. Syst. 75, 421–429. doi: 10.1016/j.jmarsys.2008.04.006
Teixeira, H., Berg, T., Uusitalo, L., Fürhaupter, K., Heiskanen, A.-S., Mazik, K., et al. (2016). A catalogue of marine biodiversity indicators. Front. Mar. Sci. 3:207. doi: 10.3389/fmars.2016.00207
Truitt, C. L. (1988). Dredged material behavior during open-water disposal. J. Coastal Res. 4, 389–397.
Tuovinen, N., Weckström, K., and Virtasalo, J. J. (2010). Assessment of recent eutrophication and climate influence in the Archipelago Sea based on the subfossil diatom record. J. Paleolimnol. 44, 95–108. doi: 10.1007/s10933-009-9390-z
Vaittinen, T., and Vartia, M. (2016). Sedimentation Study for Sea Banking During the Uusikaupunki Sea Route Deepening Project. Research Reports of the Finnish Transport Agency 22/2016.
Vallius, H. (2015). Sediment and carbon accumulation rates off the southern coast of Finland. Baltica 28, 81–88. doi: 10.5200/baltica.2015.28.08
Vatanen, S., Haikonen, A., and Kervinen, J. (2014). Uudenkaupungin Väylän ja Sataman Ruoppaus- Ja Läjityshankkeen vesistö- Ja Kalataloustarkkailu – Ennakkotarkkailu Sekä Rakentamisen Aikainen Tarkkailu Vuonna 2013. Klaukkala: Kala- ja vesitutkimus Oy.
Vatanen, S., Haikonen, A., and Piispanen, A. (2012). Vuosaaren Sataman Rakentamisen Aikaisen (2003–2008) Vesistö- Ja kalataloustarkkailun Yhteenvetoraportti. Klaukkala: Kala- ja vesitutkimus Oy.
Virtasalo, J. J., Bonsdorff, E., Moros, M., Kabel, K., Kotilainen, A. T., Ryabchuk, D., et al. (2011). Ichnological trends along an open-water transect across a large marginal-marine epicontinental basin, the modern Baltic Sea. Sediment. Geol. 241, 40–51. doi: 10.1016/j.sedgeo.2011.09.010
Virtasalo, J. J., Endler, M., Moros, M., Jokinen, S. A., Hämäläinen, J., and Kotilainen, A. T. (2016). Base of brackish-water mud as key regional stratigraphic marker of mid-Holocene marine flooding of the Baltic Sea Basin. Geo-Marine Letters 36, 445–456. doi: 10.1007/s00367-016-0464-4
Virtasalo, J. J., Hämäläinen, J., and Kotilainen, A. T. (2014). Toward a standard stratigraphical classification practice for the Baltic Sea sediments: the CUAL approach. Boreas 43, 924–938. doi: 10.1111/bor.12076
Virtasalo, J. J., Kohonen, T., Vuorinen, I., and Huttula, T. (2005). Sea bottom anoxia in the Archipelago Sea, northern Baltic Sea implications for phosphorus remineralization at the sediment surface. Mar. Geol. 224, 103–122. doi: 10.1016/j.margeo.2005.07.010
Virtasalo, J. J., Kotilainen, A. T., Räsänen, M. E., and Ojala, A. E. K. (2007). Late-glacial and post-glacial deposition in a large, low relief, epicontinental basin: the northern Baltic Sea. Sedimentology 54, 1323–1344. doi: 10.1111/j.1365-3091.2007.00883.x
Weckström, K. (2006). Assessing recent eutrophication in coastal waters of the Gulf of Finland (Baltic Sea) using subfossil diatoms. J. Paleolimnol. 35, 571–592. doi: 10.1007/s10933-005-5264-1
Keywords: impact study, seafloor integrity, dredged material dumping, sediment, grain size, multibeam echosounder, Baltic Sea, Finland
Citation: Virtasalo JJ, Korpinen S and Kotilainen AT (2018) Assessment of the Influence of Dredge Spoil Dumping on the Seafloor Geological Integrity. Front. Mar. Sci. 5:131. doi: 10.3389/fmars.2018.00131
Received: 30 January 2018; Accepted: 28 March 2018;
Published: 10 April 2018.
Edited by:
Ulrich Volkmar Bathmann, Leibniz Institute for Baltic Sea Research (LG), GermanyReviewed by:
Vanesa Magar, Centro de Investigación Científica y de Educación Superior de Ensenada, MexicoIan Townend, CoastalSEA, United Kingdom
Copyright © 2018 Virtasalo, Korpinen and Kotilainen. This is an open-access article distributed under the terms of the Creative Commons Attribution License (CC BY). The use, distribution or reproduction in other forums is permitted, provided the original author(s) and the copyright owner are credited and that the original publication in this journal is cited, in accordance with accepted academic practice. No use, distribution or reproduction is permitted which does not comply with these terms.
*Correspondence: Joonas J. Virtasalo, joonas.virtasalo@gtk.fi