- 1Mote Marine Laboratory, Sarasota, FL, United States
- 2Marine Operations Department, Florida Institute for Human and Machine Cognition, Pensacola, FL, United States
Cephalopods' visually driven, dynamic, and diverse skin display makes them a key animal model in sensory ethology and camouflage research. Development of novel methods is critically important in order to monitor and objectively quantify cephalopod behavior. In this work, the development of Cephalopod Experimental Projected Habitat (CEPH) is described. This newly developed experimental design bridges computational and ethological sciences, providing a visually controlled arena which requires limited physical space and minimal previous technical background. Created from relatively inexpensive and readily available materials, the experimental apparatus utilizes reflected light which closely resembles natural settings. Preliminary results suggest the experimental design reproducibly challenges marine organisms with visually dynamic surroundings, including videos of prey and predator. This new approach should offer new avenues for marine organism sensory research and may serve researchers from various fields.
Introduction
Living under intense predation pressure has driven cephalopods (octopus, squid, and cuttlefish) to develop an impressive and rapid dynamic camouflaging capability, taking avail of their complex skin structure to defend themselves against predators, approach prey, and communicate (Moynihan, 1985; Packard, 1988; Hanlon and Messenger, 1998; Borrelli et al., 2006; Hanlon et al., 2009; Mäthger et al., 2009).
Cephalopods multilayered and sophisticated skin structure consists of a dense network of pigmented muscle-driven chromatophore cells, which are neurally stimulated to actuate and affect local skin coloring (reviewed in Hanlon and Messenger, 1998). When camouflaging, these visually-oriented mollusks alter their appearance to match their surroundings which, providing researchers a glimpse into the animal's apparent visual perspective (Messenger, 1977; Muntz and Gwyther, 1988; Hanlon and Messenger, 1998; Chiao and Hanlon, 2001b; Barbosa et al., 2007; Hanlon et al., 2009; Josef et al., 2012, 2017; Schwarz, 2015). Theoretically, by manipulating the visual environment it might be possible to characterize and test the background effect and eliciting these various displays.
Initially researchers used static printed backgrounds to elicit and study camouflaging behaviors (Chiao and Hanlon, 2001a,b; Grable et al., 2002; Barbosa et al., 2004; Chiao et al., 2005; Shohet et al., 2006). Background patterns were statically or permanently mounted to the bottom of the experimental tank, offering limited alternatives in any given scenario. Although much was learned about cephalopod's behavior using these important static backgrounds, the rapid and dynamic camouflage capability of these animals called for an agile and more responsive experimental design in which the animal might even passively or interactively affect its own surroundings.
More recently, computational technologies have been utilized in hopes of objectively quantifying and categorizing cephalopods skin patterns (Barbosa et al., 2008; Hanlon et al., 2009; Chiao et al., 2010; Zylinski et al., 2010; Josef et al., 2012, 2017; Orenstein et al., 2016) and other marine organisms behavior under gradient sensory cues (Berdahl et al., 2013). Modulating the visual surroundings while concurrently studying an organism's behavior provides a subjective and interactive modality which opened new avenues for behavioral, physiological and psychological research. This approach also provided a platform for addressing old research questions and asking new ones.
In a previous study, a first evidence that video playback is a valuable tool for studying cephalopod behaviors was demonstrated by Pronk et al. (2010), showing that gloomy octopus (Octopus tetricus) responds to video playback of prey items. Nonetheless, the technique was developed to specifically induce preying behavior and was not design to create an immersive experience, nor elicited a camouflaging response. In a more recent work, Jaffe et al. (2011) identified the need for a dynamic testing system. They designed a similar system, named the Sub-Sea Holodeck (SSH), which provided an immersive environment for studying camouflaging cephalopods. The system described in their important work provided a crucial baseline and preliminary results, confirming the system's applicability to cephalopod research. While the holodeck array is a most impressive piece of engineering, it mandated multiscale design and fabrication.
As a cephalopod behavior scientist I believed a simpler, more cost-effective design would be of great value. Building upon Jaffe et al. (2011) inspiring work, I developed the Cephalopod Experimental Projected Habitat (CEPH) system, which is comprehensively detailed below. While this responsive artificial lighting and projecting apparatus does not simulate ocean light conditions, it does create dynamic visual stimuli of either artificial or natural scenes. Although the system described herein was originally designed to study cephalopods camouflage behavior, its flexibility and non-specificity offers new opportunities to expand such research to other marine organisms. I believe that this apparatus will allow new multidisciplinary and novel questions to be answered both within and outside of the marine behavioral study field.
In the following work, I bring CEPH's novel experimental design as well as some of the preliminary data demonstrating its reliability.
Materials and Methods
In this section, I describe the system setup and configuration of the experimental lab, also illustrated in a diagram (Figure 1). The lab has been specifically designed to hold a large experimental tank in which I conduct all experiments. The room's walls and ceiling are painted matte black to minimize any possible reflections and eliminate any obvious visual landmarks. A Viewsonic DLP short-throw projector (model PJD5553LWS) is suspended from the ceiling, facing straight down and projects directly onto the bottom. A high-resolution Basler acA1920-50gc GigE camera (capable of 50 fps) is also suspended from the ceiling and is used to capture all activity within the tank. The projector and camera are both connected to a high performance Windows-based desktop machine which is located in the lab. This device runs the customized Matlab-based software described below. Experimental room temperature was maintained at 22 Celsius with relative humidity under 45% at all times, providing adequate conditions and protecting all electronic components from corrosion and failure. Although cephalopods does not require ethical authorization under the Institutional Animal Care and Use Committee (IACUC), the experiments carried out in this study complied with the European legislation for animal experiments and with EU directive 2010/63 on the protection of animals used for scientific purposes (Smith et al., 2013; Fiorito et al., 2014).
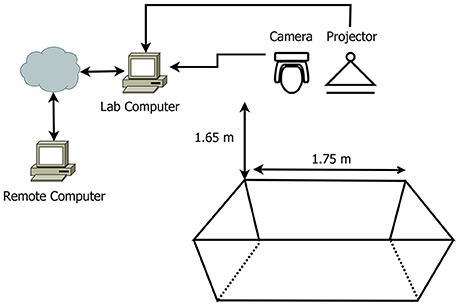
Figure 1. An illustration of the experimental design. Room and tank dimensions dictated the position of the camera and projector.
Experimental Tank
The fiberglass experimental tank measures 1.2 m2 on the bottom and 1.75 m2 at the top with. The sides of the tank angle outwards 20° from the bottom (Figure 2A). The tank is painted matte white, providing an adequate projection background. This design allows for images and/or video to be projected both onto the bottom and the sides of the tank, creating an immersive, and dynamic environment (Figures 2B–E). With the current system configuration, each projected pixel formed a 1 mm2 at the bottom of the tank, so that creating known size visual objects is relatively easy. Also, the experimental tank is plumbed in such a way that it can be filled and drained between experiments, eliminating chemical and physical traces from one session to another. It may be configured either as an open loop system in which clean salt water is added or as a closed loop system in which water recirculates while maintaining any desired water temperature.
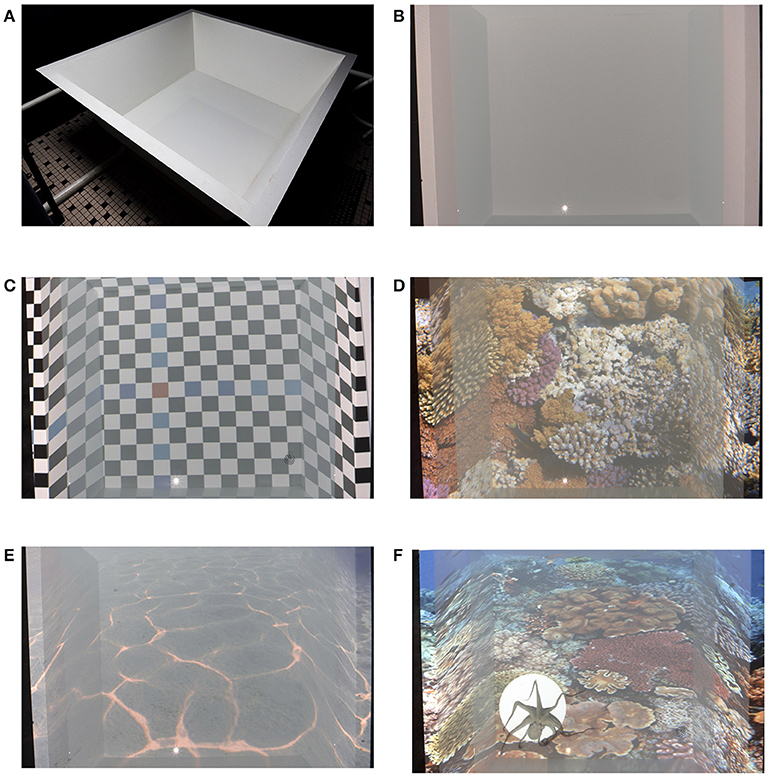
Figure 2. The experimental tank and some example of its capabilities. (A) side-view of the matt white square experimental tank with its slanted sides surrounded by the black matt room (B) The white tank as pictured from above by the ceiling camera (C) Artificial pattern projected onto the tank. This pattern also helped in aligning and positioning of the projected images (D) Coral reef projection as an example for complex natural habitat, with various visual cues (E) A natural uniform substrate (F) An octopus located in a projected reef arena, excluded from the projection and tracked as it freely swims in the tank.
Although the Matlab software runs on a computer sitting within the lab, it is remotely controlled from a nearby office, eliminating any external effects of human presence throughout the behavioral experiments. The software used in the system is described in more detail below.
Software Description
Using Matlab, I developed a graphical user interface (GUI) to ease the use of the Matlab acquisition and post analysis code. All codes and GUI are available as Supplementary Material—and are presented here to be used in future studies (with the adequate credit/reference).
The Matlab functionality may be divided into three major sections:
(1) Acquisition: with the frame covering the entire tank, the full resolution capabilities of the camera (1,936 × 1,216 pixels) was used to acquire RGB videos at a 25 fps rate. Each video was then segmented into 3 min fragments making it easier to save, load and post-analyze. Although much of the video analysis is done in real-time, all videos are recorded as uncompressed AVI files.
(2) Tracking: While the animals were swimming in the tank, the real-time streaming videos were analyzed by using image subtraction motion-based object tracking to, continuously acquire the animal's position.
(3) Manipulation: Images projected onto the tank were manipulated according to the animal's position. This manipulation can take many forms depending on the research question in hand. As an example, for camouflage studies the system needs to project the background such that colored pixels are replaced with white pixels wherever the animal is located. As the animal moves around the tank, this “exclusion zone” must track and move with the animal (Figure 2F). This feature is important so that The octopus' camouflaging behavior can be seen, captured, and quantified—without having the animal's body pattern manipulated by the projected image (Figure 3D and Video S1). Other possible manipulations could include (but are not limited to): manipulating the color, size, movement velocity, or other characteristics of prey or predator projected into the tank; displaying bi-chromatic images on each side of the animal; providing a constant visual stimulus to each eye; or displaying variable habitats allowing the platform to serve other organisms and/or other research questions.
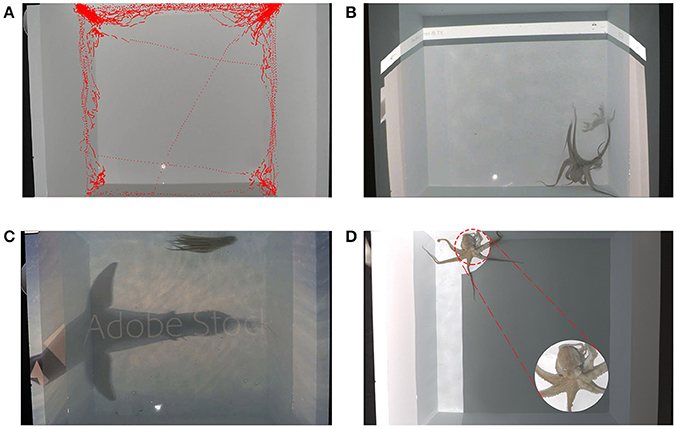
Figure 3. Some preliminary and validation results of the method. (A) Spatial tracking of one of the octopuses. Tracking was done in a uniform white tank (no patterns) for 15 min. (B) One of the octopuses approaching a projected stone crab. The predation behavior is fully documented in the Video S2. (C) An escape response to a swimming shark silhouette, also available on Video S4. (D) An octopus presenting a unilateral display in response to a black and white projected arena also available in Video S3.
Test Animals
Seven wild caught naive adult octopuses (Octopus vulgaris), 4 females and 3 males with average weight of 0.66±0.09 kg were used to test and validate the system functionality. Animals were separately maintained in a specially designed glass tanks (60 × 40 × 45 cm), sea water maintained at 20° at all times while the room was illuminated in natural spectrum 13:11 day:night light cycle and were fed with live shrimps, live Florida stone crabs (Menippe mercenaria) and frozen clams. Animals were introduced one at time into the experimental tank and were left to settle for at least 10 min before any experiments were performed. Daily, before the octopuses were fed, a live crab (attached to a nylon string) was introduced into their holding tank, timing the latency between the crab entering the water and the octopus motion toward the prey. At this point, the crab was pooled out preventing the octopus to catch it. These measurements provided us with reliable estimate for the latency of an octopus to a live crab in captivity. The same procedure was performed when introducing the video recorded carbs onto the CEPH substrate.
Preliminary Results
In the following section, I describe some of the preliminary results of our system. I believe these early data provide insight into the potential offered by this system.
Automated Spatial Tracking
The acquisition and tracking features of the code offers an opportunity to track any animal inside the tank (octopuses in our case), learning more about their spatial orientations, spatial learning, mapping etc. (Figure 3A). In the following example it became clear that as in earlier experiments using rats (Avni et al., 2008; Yaski et al., 2011), when first introduced into a new environment all seven octopuses tend to remain in the perimeter. Although the results and significance are not in the scope of this paper, this observation suggests many more options that can be addressed given this code's capabilities. The described apparatus is an effective way to understand the spatial and navigational decisions of visually oriented marine organisms such as light regimes as navigation cues in reef fish, the effect of constant anthropogenic light on marine organisms spatial awareness, the use of visual landmarks in navigation, or spatial memory and many more.
Octopus Respond to Prey
With six out of the seven octopuses introduced in the tank, projecting a recorded video of a stone crab, M. mercenaria (a natural and favored prey of Octopus vulgaris in the gulf of Mexico) drew the octopus's attention and elicited predation behavior. Once the crab's video displayed, octopuses immediately moved toward the projected crab and tried to catch it, engulfing it with its arms and web (Video S2). The crab's two-dimensional features, lack of olfactory signals and other stimuli coming from a real crab did not effect the octopus predatory reaction. It was clear that the octopus were highly interested in the projected crab, creating a positive control (Figure 3B). The average latency of the octopuses to a live Florida stone crab was 2.3 ∓ 1.3 s. while the latency of the octopuses to a projected crab was 2.9 ∓ 1.5 s. These results support the reliability of the projected visual stimuli.
Octopus Respond to Predator
To confirm that the octopus recognized and identified the projected image in the prey experiment, a video of a predator (shark) was projected into the tank. In four out of the seven octopuses, the video evoked an escape response, supporting the assumption that the projected video recordings were actually perceived as expected (Figure 3C).
Octopus Unilateral Display
Providing a dichromatic pattern on each side of a static/moving octopuses, elicited a unilateral display, suggesting that spatial and directional stimuli elicit the expected skin coloration and behavioral response (Video S3 and Figure 3D).
Conclusions
The CEPH offers versatile, variable, and reliable and dynamically changing underwater visual surroundings. Building and experimenting with the CEPH system, some limitations, and advantages were noticeable. Pros: The light regime, intensity, and the visual input are easily displayed, modified and potentially allow addressing a versatile set of question. Another point is that a light coming from the water surface and reflected from the tank back to the animal's eye resembles the light field in real scenario vs. the Plasma/LCD display alternatives. Moreover, the simple and user-friendly set-up allowed non-qualified staff, students and interns to assist, design, and execute their idea with ease. Furthermore, the entire system may be remotely controlled which allows researchers to design and conduct their experiments from anywhere in the world (with the exception of animal maintenance and handling). Cons: As mentioned, the CEPH room had to be painted matt black, which could be of issue in different laboratories and locations. Also, the GigE camera we used requires a significant amount of light to obtain a clear and high-resolution image, which might be problematic when studying light sensitive organisms. In our case, octopuses had to be continuously and carefully monitored due to the infamous octopus escaping behavior and the open-lid arrangement of the tank.
Given the octopus's response to prey and predators, I believe that the CEPH's validity as an experimental testing environment has been verified. Obviously, responses to projected visual stimuli can only be intuitive; nonetheless, the animals' responses to the presence of prey (Stone crab) and predator (Shark) strongly suggest that the animals truly perceive what is expect them to see. Further, the fact that the octopuses matched the background intensity (Unilateral display) further supports the adequacy of using these visual stimuli in a variety of behavioral experiments including camouflage and communication. By recreating and isolating the effect of visual cues on marine organisms, the CEPH offers unprecedented opportunity to study the effect of artificial and natural light fields at various spatio-temporal resolutions on the behavior, navigation and ecology of visually oriented organisms (e.g., the natural flickering light on the seabed, Video S5). Creatively, by adding measured and known sensory stimuli researchers may even use this system to study sensory cue integration in a controlled environment.
I believe that the CEPH system's simplicity, affordability and the ease of assembly makes it an important tool available for use in multiple future experimental arenas, hopefully expanding our understanding of animals' perception and behavior.
Author Contributions
NJ contributed in conceiving the idea, planed and built it. Also responsible of data collection, manuscript writing and media preparation.
Funding
Mote Marine Laboratory and Aquarium fully funded this work.
Conflict of Interest Statement
The author declares that the research was conducted in the absence of any commercial or financial relationships that could be construed as a potential conflict of interest.
The reviewer EM and handling Editor declared their shared affiliation.
Acknowledgments
I would like to thank Mote's postdoctoral fellowship and the Selby foundation for their generous support, allowing me to conduct this research, Dr. Michael Crosby, Dr. Ken Ford, Dr. Ernie Estevez, and David Fries for their support and good advice, the entire Mote's technical team in general with a special appreciations to Derek Templeton, Paul Barricklow, Randy Grams, and John Fowler, for their valuable help in constructing the life support system and the described experimental settings. Also, I would like to greatly thank all of my lab's volunteers and interns with a special thanks to Mr. Andy Dietsch, Mrs. Vicki Porter-Fink, and Dr. Aaron Fink, for their assistance in running experiments, maintenance, reviewing the manuscript and their helpful suggestions along the way.
Supplementary Material
The Supplementary Material for this article can be found online at: https://www.frontiersin.org/articles/10.3389/fmars.2018.00073/full#supplementary-material
References
Avni, R., Tzvaigrach, Y., and Eilam, D. (2008). Exploration and navigation in the blind mole rat (Spalax ehrenbergi): global calibration as a primer of spatial representation. J. Exp. Biol. 211, 2817–2826. doi: 10.1242/jeb.019927
Barbosa, A., Florio, C. F., Chiao, C. C., and Hanlon, R. T. (2004). Visual background features that elicit mottled body patterns in Cuttlefish, Sepia officinalis. Biol. Bull. 207:154. doi: 10.1086/BBLv207n2p154
Barbosa, A., Mäthger, L. M., Buresch, K. C., Kelly, J., Chubb, C., Chiao, C. C., et al. (2008). Cuttlefish camouflage: the effects of substrate contrast and size in evoking uniform, mottle or disruptive body patterns. Vision Res. 48, 1242–1253. doi: 10.1016/j.visres.2008.02.011
Barbosa, A., Mäthger, L. M., Chubb, C., Florio, C., Chiao, C. C., and Hanlon, R. T. (2007). Disruptive coloration in cuttlefish: a visual perception mechanism that regulates ontogenetic adjustment of skin patterning. J. Exp. Biol. 210, 1139–1147. doi: 10.1242/jeb.02741
Berdahl, A., Torney, C. J., Ioannou, C. C., Faria, J. J., and Couzin, I. D. (2013). Emergent sensing of complex environments by mobile animal groups. Science 339, 574–576. doi: 10.1126/science.1225883
Borrelli, L., Gherardi, F., and Fiorito, G. (2006). A Catalogue of Body Patterning in Cephalopoda. Firenze: Firenze University Press.
Chiao, C. C., Chubb, C., Buresch, K. C., Barbosa, A., Allen, J. J., Mäthger, L. M., et al. (2010). Mottle camouflage patterns in cuttlefish: quantitative characterization and visual background stimuli that evoke them. J. Exp. Biol. 213, 187–199. doi: 10.1242/jeb.030247
Chiao, C. C., and Hanlon, R. T. (2001a). Cuttlefish camouflage: visual perception of size, contrast and number of white squares on artificial checkerboard substrata initiates disruptive coloration. J. Exp. Biol. 204, 2119–2125.
Chiao, C. C., and Hanlon, R. T. (2001b). Cuttlefish cue visually on area—not shape or aspect ratio—of light objects in the substrate to produce disruptive body patterns for camouflage. Biol. Bull. 201, 269–270. doi: 10.2307/1543359
Chiao, C. C., Kelman, E. J., and Hanlon, R. T. (2005). Disruptive body patterning of cuttlefish (Sepia officinalis) requires visual information regarding edges and contrast of objects in natural substrate backgrounds. Biol. Bull. 208, 7–11. doi: 10.2307/3593095
Fiorito, G., Affuso, A., Anderson, D. B., Basil, J., Bonnaud, L., Botta, G., et al. (2014). Cephalopods in neuroscience: regulations, research and the 3Rs. Invertebrate Neurosci. 14, 13–36. doi: 10.1007/s10158-013-0165-x
Grable, M. M., Shashar, N., Gilles, N. L., Chiao, C. C., and Hanlon, R. T. (2002). Cuttlefish body patterns as a behavioral assay to determine polarization perception. Biol. Bull. 203, 232–234. doi: 10.2307/1543414
Hanlon, R. T., Chiao, C. C., Mäthger, L. M., Barbosa, A., Buresch, K. C., and Chubb, C. (2009). Cephalopod dynamic camouflage: bridging the continuum between background matching and disruptive coloration. Philos. Trans. R. Soc. Lond. B Biol. Sci. 364, 429–437. doi: 10.1098/rstb.2008.0270
Hanlon, R. T., and Messenger, J. B. (1998). Cephalopod Behaviour. Cambridge: Cambridge University Press.
Jaffe, J. S., Laxton, B., and Zylinski, S. (2011). “The sub sea holodeck: A 14-megapixel immersive virtual environment for studying cephalopod camouflage behavior,” in OCEANS, 2011 IEEE-Spain (Santander: IEEE), 1–6.
Josef, N., Amodio, P., Fiorito, G., and Shashar, N. (2012). Camouflaging in a complex environment–octopuses use specific features of their surroundings for background matching. PLoS ONE 7:e37579. doi: 10.1371/journal.pone.0037579
Josef, N., Berenshtein, I., Rousseau, M., Scata, G., Fiorito, G., and Shashar, N. (2017). Size matters: observed and modeled camouflage response of European Cuttlefish (Sepia officinalis) to different substrate patch sizes during movement. Front. Physiol. 7:671. doi: 10.3389/fphys.2016.00671
Mäthger, L. M., Shashar, N., and Hanlon, R. T. (2009). Do cephalopods communicate using polarized light reflections from their skin? J. Exp. Biol. 212, 2133–2140. doi: 10.1242/jeb.020800
Moynihan, M. (1985). Communication and Non-communication in Cephalopods. Bloomington, IL: Indiana University Press.
Muntz, W. R. A., and Gwyther, J. (1988). Visual discrimination of distance by octopuses. J. Exp. Biol. 140, 345–353.
Orenstein, E. C., Haag, J. M., Gagnon, Y. L., and Jaffe, J. S. (2016). Automated classification of camouflaging cuttlefish. Methods Oceanogr. 15, 21–34. doi: 10.1016/j.mio.2016.04.005
Packard, A. (1988). The skin of Cephalopods (Coleoids): general and special adaptations. Mollusca 11:30. doi: 10.1016/B978-0-12-751411-6.50010-2
Pronk, R., Wilson, D. R., and Harcourt, R. (2010). Video playback demonstrates episodic personality in the gloomy octopus. J. Exp. Biol. 213(Pt 7), 1035–1041. doi: 10.1242/jeb.040675
Shohet, A. J., Baddeley, R. J., Anderson, J. C., Kelman, E. J., and Osorio, D. (2006). Cuttlefish responses to visual orientation of substrates, water flow and a model of motion camouflage. J. Exp. Biol. 209(Pt 23), 4717–4723. doi: 10.1242/jeb.02580
Smith, J. A., Andrews, P. L. R., Hawkins, P., Louhimies, S., Ponte, G., and Dickel, L. (2013). Cephalopod research and EU Directive 2010/63/EU: requirements, impacts and ethical review. J. Exp. Mar. Biol. Ecol. 447, 31–45. doi: 10.1016/j.jembe.2013.02.009
Yaski, O., Portugali, J., and Eilam, D. (2011). Arena geometry and path shape: when rats travel in straight or in circuitous paths? Behav. Brain Res. 225, 449–454. doi: 10.1016/j.bbr.2011.07.055
Keywords: dynamic camouflage, Octopus vulgaris, animal vision, light in the ocean, benthic, animal behavior
Citation: Josef N (2018) Cephalopod Experimental Projected Habitat (CEPH): Virtual Reality for Underwater Organisms. Front. Mar. Sci. 5:73. doi: 10.3389/fmars.2018.00073
Received: 03 December 2017; Accepted: 16 February 2018;
Published: 06 March 2018.
Edited by:
Antonio Trincone, Istituto di Chimica Biomolecolare (CNR), ItalyReviewed by:
Graziano Fiorito, Stazione Zoologica Anton Dohrn, ItalyErnesto Mollo, Consiglio Nazionale Delle Ricerche (CNR), Italy
Copyright © 2018 Josef. This is an open-access article distributed under the terms of the Creative Commons Attribution License (CC BY). The use, distribution or reproduction in other forums is permitted, provided the original author(s) and the copyright owner are credited and that the original publication in this journal is cited, in accordance with accepted academic practice. No use, distribution or reproduction is permitted which does not comply with these terms.
*Correspondence: Noam Josef, YmlnYmx1ZTI4MTBAZ21haWwuY29t