- 1Department of Ecology and Evolutionary Biology, University of Toronto, Toronto, ON, Canada
- 2Laboratory Services Branch, Ontario Ministry of the Environment and Climate Change, Toronto, ON, Canada
- 3Environment Department, University of York, York, United Kingdom
Expanded polystyrene (EPS) products and their associated chemicals (e.g., styrenes) are widespread in the marine environment. As a consequence, bans on their use for single-use packaging materials are being proposed in several municipalities. To better understand how science can inform decision-making, we looked at the available scientific literature about contamination and effects and conducted experiments to measure chemical leachate from polystyrene products and toxicity from the leachate. We conducted leaching experiments with common food matrices (water, soup broth, gravy, black coffee and coffee with cream and sugar) at relevant temperatures (70 and 95°C) that are consumed in or with several polystyrene products (coffee cup lids, polystyrene stir sticks, polystyrene spoons, EPS cups, EPS bowls, and EPS takeout containers). We analyzed each sample for styrene, ethylbenzene, toluene, benzene, meta- and para- xylene, isopropylbenzene, and isopropyltoluene—chemicals associated with polystyrene products. To determine whether the leachates are toxic, we conducted chronic toxicity tests, measuring survival and reproductive output in Ceriodaphnia dubia. Toxicity tests included nine treatments: seven concentrations of ethylbenzene, EPS cup leachate and a negative control. Overall, we found that temperature has a significant effect on leaching. We only detected leachates in trials conducted at higher temperature −95°C. Ethylbenzene was the only target analyte with final concentrations above the method limit of detection, and was present in the greatest concentrations in EPS and with soup broth. Measurable concentrations of ethylbenzene in the leachate ranged from 1.3 to 3.4 μg/L. In toxicity tests, the calculated LC50 for ethylbenzene was 14 mg/L and the calculated LC20 was 210 μg/L. For the treatment exposed to the EPS cup leachate, mortality was 40%—four times greater than the negative control. Finally, there was no significant difference (p = 0.17) between reproductive output for any treatment with ethylbenzene, but there was a significant reduction (p = 0.01) in reproductive output for the treatment exposed to the EPS leachate compared to the negative control. Thus, although the target analyte ethylbenzene was not toxic at concentrations detected in the leachate, significant adverse effects were detected in the whole EPS cup leachate sample.
Introduction
Plastic debris has become an issue of concern for marine and freshwater habitats globally (Kershaw and Rochman, 2015; Löhr et al., 2017). Plastic items of many types, whole and fragmented, are found on beaches (Browne et al., 2015), floating on the surfaces of oceans (van Sebille et al., 2015) and lakes (Eriksen et al., 2013), in the deep sea (Woodall et al., 2014), and in a great diversity of wildlife (Gall and Thompson, 2015). There are many solutions that have been proposed for reducing plastic emissions into the environment. Some of these solutions are at the local scale (Xanthos and Walker, 2017), while others aim to tackle the problem internationally (Borrelle et al., 2017; Löhr et al., 2017).
In general, there is no one-size-fits-all solution for mitigating plastic debris, and thus many solutions working in tandem are likely necessary. These can include innovation of more sustainable plastic products, new and improved waste management infrastructure, a global fund to help pay for development of new infrastructure and sustainable technologies, educational campaigns, clean-ups, and product bans (Borrelle et al., 2017). Single-use plastic product bans have become a popular solution, as single-use items are some of the most commonly found plastic litter items on beaches (e.g., bottle caps, plastic bags, plastic bottles, expanded polystyrene (EPS) takeout containers, straws) (Ocean Conservancy, 2017). For some single-use plastic items (e.g., plastic bags and microbeads in personal care products), bans are consistently being proposed and passed around the world (Xanthos and Walker, 2017). EPS (often referred to by the general public as Styrofoam™) is another item that is now on the table for a ban in several municipalities (http://www.surfrider.org/pages/polystyrene-ordinances). To better understand how scientific evidence could inform such legislation, we looked at the available scientific literature to review the evidence about contamination and effect. We also performed experiments of our own to measure chemical leachate from polystyrene products that come into contact with food and to measure the toxicity of the leachate.
In regards to contamination, EPS is commonly reported as one of the top items of debris recovered from shorelines and beaches worldwide (Garrity and Levings, 1993; Bravo et al., 2009; Lee et al., 2013; Ocean Conservancy, 2017), including in Antarctica (Convey et al., 2002). It has also been found on the surface of the open ocean (Morét-Ferguson et al., 2010) and on the seafloor (Keller et al., 2010). Widespread contamination has resulted in EPS being found in the gut contents of marine invertebrate and vertebrate wildlife (Boerger et al., 2010; Schuyler et al., 2014; Jang et al., 2016). In addition to physical EPS material, styrenes, the monomeric building blocks of the polymer, are found in ocean water and sediments globally (Kwon et al., 2015, 2017). Because polystyrene plastic is thought to be one of the only sources of styrenes to the environment, the contamination is expected to be from polystyrene weathering and leaching in the oceans (Kwon et al., 2017). Furthermore, in some parts of the world EPS has been cited as a source of other chemicals to the environment (Rani et al., 2015; Jang et al., 2017) and wildlife (Jang et al., 2016). In Asia, hexabromocyclododecanes (HBCDs) have been detected in EPS buoys and other consumer products (Rani et al., 2014). This contamination is thought to originate from the recycling of EPS materials with added flame retardants into other materials, namely materials that do not come into contact with food. Still, HBCDs were detected in some EPS products used for food packaging (Rani et al., 2014). These findings could have consequences to humans when they use the products and/or wildlife if the EPS products become marine debris and leach HBCD. The same research group found that sediments near aquaculture farms using EPS buoys have relatively higher concentrations of HBCD compared to other sites (Al-Odaini et al., 2015) and mussels living on EPS buoys have EPS fragments and greater concentrations of HBCD in their tissues than mussels that live on other materials (Jang et al., 2016). These studies suggest that HBCD from EPS can leach into environmental matrices, including wildlife. In general, there is no doubt that polystyrene and its associated chemicals contaminate oceans (Kwon et al., 2015; Jang et al., 2016).
There is concern that polystyrene may be more harmful than other plastic types because it is composed of relatively hazardous chemicals (Lithner et al., 2011). Because polystyrene microspheres are one of the only types of microplastics available from scientific companies, several studies have conducted laboratory toxicity tests with polystyrene. These laboratory studies suggest polystyrene microspheres can impact organisms. Here, only studies using more environmentally relevant concentrations are highlighted. Laboratory studies demonstrate that polystyrene microplastic can impact feeding behavior (Besseling et al., 2012; Cole et al., 2015), cause weight loss (Besseling et al., 2012), and affect reproduction (Cole et al., 2015; Sussarellu et al., 2016) in invertebrate species. These studies used microplastic particles, and thus whether these effects are from the physical plastic particle or chemical leachate is not known. Other studies measured effects using only chemicals related to polystyrene. A study testing toxicity of leachate from several plastic materials at room temperature found no toxicity from the treatment using a polystyrene cup (Bejgarn et al., 2015). In Daphnia magna, LC50 values for 48 h toxicity tests are reported as 23 mg/L for styrene, 75 mg/L for ethylbenzene, 200 mg/L for benzene and 310 mg/L for toluene (LeBlanc, 1980). Acute toxicity tests using fathead minnow determined LC50s of 10 mg/L for styrenes (Cushman et al., 1997). For styrenes, these concentrations are several orders of magnitude greater than those found in nature (Kwon et al., 2017).
The leaching of styrenes and other associated chemicals is one of the reasons why humans are more concerned with polystyrene relative to other plastic types. Under certain conditions, EPS leaches styrene and benzene, chemicals that have known toxic properties (Gibbs and Mulligan, 1997; Erickson, 2011; Andersen et al., 2017; Niaz et al., 2017). There is concern that EPS may cause harm if it leaches chemicals into the environment and/or into our food (Sanagi et al., 2008; Rani et al., 2014). The World Health Organization (WHO) lists the maximum permissible limit at 20 parts per billion (ppb) for styrene (World Health Organization, 2004). The amount that styrene leaches from polystyrene into food and drinks varies in the literature (from about 1 to 300 ppb), and several studies perform leaching experiments with different conditions, using various foods and/or solvents (Tawfik and Huyghebaert, 1998), varying time periods, and varying temperatures (Ahmad and Bajahlan, 2007; Sanagi et al., 2008). To try to understand exposure concentrations that may be realistic to human exposure, we chose to do our own leaching trials.
Our primary objectives were to better understand how chemicals leach from polystyrene products that come into contact with food and whether there is toxicity from the leachate. We conducted leaching experiments with common food matrices that are consumed in polystyrene packaging at relevant temperatures to test the hypothesis that polystyrene products leach styrenes and related chemicals (i.e., ethylbenzene, toluene, benzene, meta- and para- xylene, isopropylbenzene, and isopropyltoluene) (Ahmad and Bajahlan, 2007) into food consumed by humans. To test the hypothesis that such leachates may be toxic, we conducted toxicity experiments, measuring mortality and reproductive output in a standardized test species, Ceriodaphnia dubia. Aside from being a standardized test species, C. dubia also plays an important role in the food webs of freshwater habitats globally.
Materials and Methods
Leaching Experiments
Leaching experiments were conducted with several products made from polystyrene, three of which were EPS and three of which were non-expanded. Polystyrene products included coffee cup lids, stir sticks, spoons, EPS cups, EPS bowls, and EPS takeout containers. All products were either purchased from local grocery stores in Toronto, Ontario or donated from local coffee shops and restaurants. Where the material of the product was uncertain, a HORIBA XploRA Raman spectrometer was used to confirm polymer type.
Liquids and foods were chosen to be similar to what would be expected to be used for each product. This included leaching tests with water, instant coffee, instant coffee with cream (10% lipid) and sugar, instant chicken soup broth, and instant gravy. Treatments included coffee in a paper cup with a polystyrene lid, coffee with cream and sugar in a clean glass beaker with a polystyrene stir stick, soup broth in a clean glass beaker with a polystyrene spoon, water, coffee and coffee with cream and sugar in an EPS cup, soup broth in an EPS bowl, and instant gravy in an EPS takeout container. All treatments used 250 mL of liquid except for the paper cup with the polystyrene lid (200 mL of coffee), the EPS takeout container (50 mL of gravy), and the EPS cup with water (200 mL). Leaching trials lasted 30 min—roughly the length of time we might expect a person to have food or drink in a polystyrene product. For the paper cup with the polystyrene lid, the cup was tipped every 2 min to simulate drinking and allow the liquid to come in contact with the lid.
For leaching experiments, we ran three separate trials using temperatures that are realistic to hot food and drink −70 and 95°C (Brown and Diller, 2008; Table 1). For Trial 1, all food and liquid matrices were prepared with 70°C water and were in contact with polystyrene products for 30 min. All liquid and food matrices were prepared, added to the polystyrene product and allowed to sit uncovered (except for the polystyrene lid) for 30 min. Each treatment was run in triplicate (n = 3; See Table 1 for more detail). For Trial 2, all treatments were identical to Trial 1, except for one treatment where soup broth was prepared at 95°C for an EPS bowl and another treatment where an EPS bowl was microwaved for 3 min, reaching 95°C, and then allowed to sit outside the microwave uncovered for a subsequent 27 min (Table 1). Each treatment was run individually (n = 1). For Trial 3, all treatments were leached at 95°C for 30 min and covered with a petri dish. To simulate a “worst-case” scenario, an EPS cup was torn into pieces and placed in a glass flask with water that was kept at 95°C for the full 30 min by boiling it on a hot plate (Table 1). Each treatment was run in triplicate (n = 3). Over the 30 min period, 70°C liquids cooled to roughly 30 and 95°C liquids to 55°C. Directly after the 30 min leaching period, the leachate from each sample was transferred to a clean glass vial with no headspace and stored overnight at 4°C.
The following day, leachates were prepared and analyzed for seven volatile compounds (styrene, benzene, toluene, ethylbenzene, meta- and para- xylene, isopropylbenzene, and isopropyltoluene) using gas chromatography coupled with mass spectrometry (GC-MS). For Trial 1, all samples were analyzed using Headspace coupled to a GC-MS. For Trials 2 and 3, all samples were analyzed using Purge and Trap with GC-MS.
Chemical standards used for analysis were purchased from Sigma Aldrich. All samples were spiked with 5 μL of surrogate standard (Fluorobenzene, d8-toluene, bromofluorobenzene).
To analyze all samples in Trial 1, we used a Tekmar HT3 Headspace sampler coupled to an Agilent 7890A gas chromatograph with an Agilent 5975C (MSD) mass spectrometer with ultrapure grade (helium) carrier gas. 10 mL of sample was introduced to the Tekmar HT3 and a 2 mL sample from the headspace was injected into a J&W DB-VRX 20 m × 0.18 mm × 1.0 μm film column in split mode (50:1). The oven program started at 35°C, held for 4 min, increased by 14°C per minute until 100°C, increased by 20°C per minute until 220°C and then held for 2.72 min. The Agilent 5975 (MSD) was operating in full scan mode (mass range 34–350). Target analytes were quantified using the extracted ion and confirmed using retention times and the ratio of confirmation ions. Concentrations were determined using external calibration with surrogate standards. The limit of detection for this analysis was 25 ng/mL.
To analyze all samples in Trials 2 and 3, a Tekmar Atomx Purge and Trap system with a Vocarb 3000 coupled with a Thermo Trace gas chromatograph and DSQII mass spectrometer with ultrapure grade (helium) carrier gas was used. 20 mL of sample was purged directly in soil mode on the Atomx Purge and Trap concentrator and subsequently injected into a J&W DB-VRX 20 m × 0.18 mm × 1.0 μm film column in split mode (60:1). The oven program was the same as described above for Trial 1. The Thermo DSQII (MSD) was operating in full scan mode (mass range 34–350). Target analytes were quantified using the extracted ion and confirmed using retention time. The ratio of confirmation ions and concentrations were determined using external calibration with surrogate standards. The limit of detection for purge and trap analysis was approximately 1.25 ng/mL.
All glassware was cleaned and baked at 250°C for 12 h prior to use. Laboratory blanks were prepared for each sample matrix (e.g., hot water, coffee, and broth) using a clean glass beaker and no polystyrene product. Target analytes detected in laboratory blanks were not subtracted from the concentrations detected in all samples. See Tables S1 and S2 for concentrations of all target analytes in the laboratory blanks from Trial 2 and 3, respectively. Concentrations in laboratory blanks for Trial 1 are not listed because all samples were below the limit of detection. Spiked matrix blanks were also extracted and run with each sequence of samples to determine recovery. In spiked matrix blank samples, recoveries of the seven target analytes ranged from 29 to 120% across all matrices for headspace-GC-MS and 67–154% across all matrices for purge and trap with GC-MS (see Tables S3–S5 for detailed recoveries).
Leachate Toxicity Tests With C. dubia
Testing was conducted following the Environment Canada and Climate Change standard method for assessing survival and reproduction in the freshwater cladoceran C. dubia (EPS 1/RM/21; ECCC, 2007). Test solutions included different concentrations of ethylbenzene and leachate from the same EPS cups used in the leaching experiments described above.
Ethylbenzene was purchased from BDH Ltd. (99% purity) and used to make stock solutions. Stock solutions for EPS cups were prepared by placing 20 torn cups in 5 L of laboratory dilution water (dechlorinated City of Toronto tap water) in a stainless-steel stockpot and boiling for 30 min. Leachate was prepared on day 0 (test initiation), and stored in amber glass bottles with minimal headspace for use in water changes on each day of toxicity testing. Stock solutions of ethylbenzene were prepared each day of the test by spiking 6 uL into 1 L of lab dilution water and used for dilutions to make test concentrations. Because the solubility of ethylbenzene in water is 0.015 g/100 mL (20°C), no carrier solvent was used. Stock solutions were stored in glass vials with minimal headspace and used for dilutions to make test concentrations. Nominal test concentrations for ethylbenzene included 5.2, 2.6, 1.3, 0.7, 0.32, 0.16, and 0.08 mg/L. For the 5.2 mg/L ethylbenzene solution and the leachate from the EPS cup, actual concentrations were measured in solution at the start (day 0) and at day 8 using the same methods as above for leachates in Trials 2 and 3 (i.e., using purge and trap with GC-MS), except in water mode with a 10-mL purge. Because this method is slightly more sensitive, the limit of detection is 0.2 ug/L. At day 8, solutions were measured at the start and end of the 24 h period (i.e., to measure the decayed concentration). Measured concentrations of ethylbenzene in the 5.2 mg/L stock solution were 2.3 mg/L at day 0 and 4.8 mg/L at day 8. We note that the concentration on day 0 was much lower than expected. On this day only, it took a few hours before putting the test animals in the solution. On all other days, this only took a few minutes. Because the measured concentration at day 8 was what we expected, we are fairly confident that exposure concentrations in the toxicity test were similar to what we expected on all other days of the procedure. The measured concentration for the decayed solution was 0.2 mg/L, decaying as much as 96% over the 24 h period between renewal of the test solution. This is likely due to the volatility of ethylbenzene, which helps explain our lower concentration of stock solution on day 0. Measured concentrations in the leachate of the EPS cup were consistently below the limit of detection for toluene, meta- and para- xylene, isopropylbenzene, and isopropyltoluene. For styrene, concentrations in the starting solution were 0.6 μg/L at day 0 and 0.8 μg/L at day 8. The measured concentration of styrene in the decayed solution was below detection. For benzene, concentrations were 0.2 μg/L (at the detection limit) at day 0 and below the detection limit at day 8. The measured concentration of benzene in the decayed solution was also below the detection limit. For ethylbenzene, concentrations were 2.4 μg/L at day 0 and 2.1 μg/L at day 8. The measured concentration of ethylbenzene in the decayed solution was below the detection limit.
C. dubia were a single genetic stock cultured at the Ontario Ministry of the Environment and Climate Change. C. dubia are cultured at 25 ± 2°C temperature under a 16 h light/8 h dark photoperiod. Individuals are fed daily 0.5 mL unicellular green algae (Pseudokirchneriella subcapitata) and 0.01 mL of YCT (yeast/cerophyll/trout chow mix) (ECCC, 2007). Organisms used for testing met the culture health criteria of no ephipia, brood mortality did not exceed 20%, and produced broods of at least 15 neonates per female in the 7 days prior to test initiation. Water used in culturing and testing was City of Toronto tap water dechlorinated by activated carbon beds, and spiked with Selenium (3 μg/L) (Winner, 1989).
For each of the nine treatments (i.e., seven concentrations of ethylbenzene, EPS cup leachate, and a negative control) there were ten replicates (n = 10). Animals were exposed for 8 days. Each individual replicate consisted of a test volume of 15 mL and one female daphnid. Solutions were renewed daily. C. dubia were fed daily during the test following the same diet and ration as above. Water quality parameters pH, conductivity, dissolved oxygen (DO), and temperature were measured daily. In all treatments except for the EPS leachate, pH ranged from 8.2 to 8.5, conductivity from 270 to 353 μS/cm, DO from 7.6 to 9 mg/L and temperature from 21.5 to 22.8°C. In the EPS leachate, pH ranged from 8.1 to 9.9, conductivity from 229 to 305 μS/cm, DO from 4.6 to 8.5 mg/L and temperature from 21.7 to 22.6°C. Animals were acclimated to the experimental system for a 24 h period before commencement of the experiment. Each day, mortality of the first-generation individual daphnid and the number of live neonates produced each day were recorded. Overall, mortality, total brood size per individual and time to first brood were measured. For the test to be valid, we required 80% survival and at least 15 young per female on average for control animals over the 8-day test period.
Test results were analyzed statistically to determine the LC50 and LC20 for ethylbenzene and to test the hypothesis that ethylbenzene and EPS cup leachate would alter total brood size. LC50 and LC20 values and their 95% confidence limits were determined using the Probit Analysis method and calculated in a Probit Analysis calculator developed by Dr. Alpha Raj (Finney, 1952). Using GMAV (EICC, University of Sydney), a 1-factor ANOVA tested for differences in total brood size among ethylbenzene treatments (n = 10, α = 0.05) with fixed factor treatment (eight levels: 5.2, 2.6, 1.3, 0.7, 0.32, 0.16, 0.08, and 0 mg/L). We assured that our data was normally distributed via histograms. We did not run statistical tests for normality because ANOVAs are not very sensitive to moderate deviations from normality (Underwood, 1997). A Cochran's C-test (1951) showed homogeneity of variances (α = 0.05). A 2-tailed equal variances t-test analyzed differences in total brood size between the control and the EPS cup leachate treatments (n = 10, α = 0.05) using SYSTAT 12 (SYSTAT Software, Chicago, IL).
Results
Leachates From Polystyrene Products
For the leaching experiments in Trial 1 (Table 1), all products were exposed to food matrices at 70°C, uncovered, for 30 min. After the leaching experiments, all matrices were analyzed via headspace-GC-MS. For all seven target analytes, concentrations were below the limit of detection (25 μg/L). Because this limit of detection is relatively high, we decided to repeat the experiments and analyze the leachates using a more sensitive instrument with a lower detection limit (1.25 μg/L).
For the leaching experiments in Trials 2 and 3 (Table 1), we analyzed all samples using purge and trap with GC-MS. Experiments in Trial 2 were run with no replication to see if any of the target analytes could be detected. Because of the sensitivity of this instrument, we omitted samples with cream or gravy (i.e., relatively high lipid content) to keep the instrument from becoming too contaminated. In addition to running each polystyrene product with 70°C coffee or soup broth, we included two samples with soup broth at higher temperature in an EPS bowl. One sample was microwaved in the EPS bowl for 3 min to 95°C and allowed to sit out for 27 more minutes. The other was boiled to 95°C and the hot broth was poured into the EPS bowl and allowed to sit for 30 min. Across all samples run at 70°C, all target analytes were below the limit of detection or at trace levels that were similar to the concentration in the blank (see Table S1 for all data from Trial 2). For the two samples run at 95°C, ethylbenzene was the only target analyte above the limit of detection and that was not detected in the blanks. Ethylbenzene concentrations were similar between the two hot samples, with concentrations of 3.2 μg/L in the microwaved EPS bowl and 3.4 μg/L in the non-microwaved EPS bowl. This suggests that the higher heat is the cause of the higher concentrations of ethylbenzene in the leachate.
Trial 3 was conducted to repeat our test in Trial 2 with replication (n = 3) and to conduct all leaching trials at a higher temperature −95°C (Table 1). All of the same treatments in Trial 2, with the exception of the microwaved EPS bowl, were replicated in Trial 3 at 95°C. In addition, we added one more treatment with EPS in water maintained at 95°C for the full 30 min by boiling it on a hot plate. For this treatment one EPS cup per replicate was torn into pieces and placed in the flask of boiling water for the full 30 min. Again, some target analytes were detected at trace levels in some samples, but were similar to the concentration in the blank (see Table S2 for all data from Trial 3). Similar to Trial 2, ethylbenzene was the only target analyte that was above the limit of detection and not detected in the blanks. Ethylbenzene was detected in all three replicates of boiling water with EPS at concentrations of 1.5, 1.6, and 1.5 μg/L, of coffee with EPS at 1.3, 1.4, and 1.4 μg/L, and of broth with EPS at 1.6, 1.8, and 2.6 μg/L. In general, EPS leaches more than the other polystyrene products tested and soup broth induces greater leaching than hot coffee or water.
Toxicity in C. dubia
Across all treatments, there was no obvious response curve. This may be due to the high volatility of ethylbenzene. Higher concentrations did not always lead to a greater response. Total mortality ranged from 10 to 70% (Table 2; see Table S6 for mortality data). There was no difference in mortality between the control and the lowest two concentrations of ethylbenzene (0.16 and 0.08 mg/L), with all three having 10% mortality. One ethylbenzene treatment, 0.65 mg/L, had 20% mortality. Mortality in the EPS cup leachate and in the 0.325, 1.3, and 5.2 mg/L ethylbenzene treatments suffered 40% mortality—four times more than the control and two times above the acceptability criteria in this chronic test. The highest mortality was in the 2.6 mg/L ethylbenzene treatment, at 70% mortality. For ethylbenzene, the calculated LC50 was 14 mg/L (95% confidence interval 3.5–61 mg/L) and the calculated LC20 was 0.21 mg/L (95% confidence interval 0.05–0.9 mg/L).
Across all treatments, the average time to first brood ranged from 4.2 to 5.9 days (Table 2; see Table S7 for all reproductive data). Time to first brood ranged from 4.2 to 4.9 days for all treatments, except the 0.325 mg/L and EPS cup leachate treatments. For these two treatments, time to first brood was 5.7 ± 1.4 and 5.9 ± 1.2 days respectively—roughly an entire day later than the control treatment (4.8 ± 1 days).
Across all treatments, average total brood size ranged from 5 to 15 offspring. For total number of offspring, there was no significant difference between ethylbenzene treatments (p = 0.17; Figure 1). There was a significant difference in total number of offspring between the EPS cup leachate and the control treatments (p = 0.01), with the total brood size of C. dubia exposed to EPS cup leachate being significantly smaller than of C. dubia in the control treatment (Figure 2). Total average brood size for C. dubia in the control treatment was 15 ± 9 offspring, whereas total average brood size for C. dubia in the EPS cup leachate treatment was 5 ± 5 offspring.
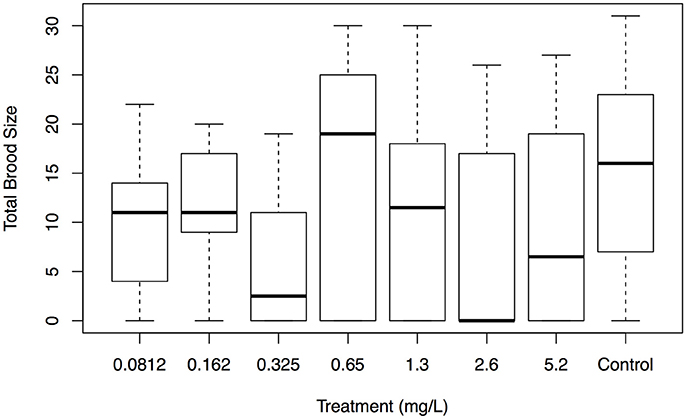
Figure 1. Total brood size of C. dubia exposed to different concentrations of ethylbenzene and the negative control. The box and whisker plot displays a five-number summary of the total brood size data for each treatment with ethylbenzene from lowest to highest concentration (mg/L) with the negative control on the right. The bar in the middle of each box represents the median, the top, and bottom of the box the lower and upper quartiles (25 and 75%) and the whiskers the minimum and maximum values.
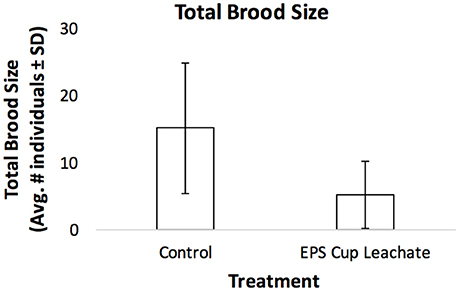
Figure 2. Total brood size of C. dubia exposed to the EPS cup leachate and the negative control. Each bar represents the mean total brood size of each treatment and the error bars represent the standard deviation.
Discussion
Here, we tested whether polystyrene products leach chemicals into food and drink matrices under realistic exposure scenarios and whether their leachates led to toxicity in freshwater zooplankton.
Low Levels of Volatile Compounds Leach From Polystyrene Products During Use
We only detected chemical leachates in trials conducted at 95°C, and the only chemical that was confidently detected in leachates was ethylbenzene. Ethylbenzene was present in concentrations ranging from 1.3 to 3.4 μg/L. In leaching trials, the highest concentrations were in the soup broth. In general, this suggests that temperature has a significant effect on the amount that chemicals will leach from polystyrene products, a trend that has been demonstrated in other studies (Tawfik and Huyghebaert, 1998; Ahmad and Bajahlan, 2007; Sanagi et al., 2008). It also suggests that matrices with lipids (chicken soup broth) cause greater leaching or better retain volatile leachates than matrices without lipids (water and coffee). This trend has also been found in a previous study (Tawfik and Huyghebaert, 1998). In addition, our results suggest that EPS leaches more than non-expanded polystyrene products, such as polystyrene cutlery and coffee cup lids.
Here, we aimed to conduct leaching experiments under scenarios that are realistic to how each product is used for eating and drinking. Temperatures used in this study ranged from 70 to 95°C (Brown and Diller, 2008), and products were not exposed to leachate for more than 30 min. Under these conditions, leachate concentrations for styrene and ethylbenzene were below the limits accepted by the WHO: 20 ppb for styrene and 300 ppb for ethylbenzene (World Health Organization, 2004). Concentrations of ethylbenzene in our experiments were two orders of magnitude lower than the limit deemed acceptable by World Health Organization (2004). Other studies that use realistic leaching conditions have found concentrations that do raise concerns for human health. Sanagi et al. (2008) found styrene concentrations ranging from 45 to 293 ppb in water under leaching conditions of 24–80°C for 30 min in a polystyrene cup. Tawfik and Huyghebaert (1998) found styrene concentrations of 24 ppb in whole milk under conditions at 40°C for 24 h and in ice-cream under conditions at −10°C for 30 days in polystyrene cups.
In this study, we targeted a suite of volatile chemicals that have been demonstrated to be associated with polystyrene and/or EPS in previous studies. As with any chemical analysis, there may be other chemicals present in these polystyrene products that we did not target. For example, Rani et al. (2014) detected flame retardants in polystyrene products at concentrations ranging from 24 to 199 ng/g (Rani et al., 2014).
Toxicity of the Leachate From EPS Food Containers
Because ethylbenzene was the only chemical detected at quantifiable levels in our leaching experiments, we focused on ethylbenzene for our toxicity tests. In addition, because EPS seemed to leach more than other products, we included a treatment that consisted of the whole leachate from an EPS cup. This treatment was included to determine whether there may be any toxicity due to chemicals we did not target for analysis.
For toxicity tests using several concentrations of ethylbenzene, higher concentrations did not always lead to greater effects (Table 2). This may have been due to the fact that ethylbenzene is a volatile chemical, and thus concentrations in the vials were variable based on the fast decay rates we observed. Here, the calculated LC50 was 14 mg/L and the calculated LC20 was 210 μg/L. These concentrations are several orders of magnitude greater than the ethylbenzene measured in our leaching trials. We also did not observe a significant difference in reproductive output among all treatments with ethylbenzene. These results suggest that the leachates from all of our leaching trials are not toxic. However, the results from the treatment with the EPS cup suggest otherwise.
The mortality observed in the treatment exposed to the EPS cup leachate was 40%, which is four times greater than the negative control. Moreover, the time to first brood was >1 day later than the control and we observed a significant reduction in reproductive output. The average total brood in the EPS treatment was three times less than that of the control. Such reproductive effects have the potential to lead to population level effects. Similar effects, demonstrating reduced reproduction in oysters (Sussarellu et al., 2016) and marine species of zooplankton (Cole et al., 2015) exposed to polystyrene, have also been observed.
Although we observed significant toxicity in C. dubia that were exposed to the EPS leachate, we do not know what led to the observed effects. One possible explanation is the high pH measured in the test solution at various time points. Another possible explanation is a chemical or combination of chemicals that we did not target in our analyses. Our results highlight the importance of measuring toxicity from the whole sample vs. simply measuring toxicity with one targeted chemical at a time. A whole sample provides a more holistic outlook on what types of effects we might observe in the real-world. Future studies should aim to conduct whole leachate toxicity tests using more products, under different scenarios and measuring more diverse effects. Different scenarios might include comparing leachates under different temperatures and in marine vs. freshwater.
Implications for Policy
When planning legislation many factors need to be considered and all informed by scientific evidence. It is important to consider implications for human health, wildlife, and sustainability. Here, we focused on implications for human health by measuring leaching and implications for wildlife by measuring toxicity in a freshwater invertebrate. In regards to human health, the results from our leaching experiments do not suggest that polystyrene is unsafe for humans. However, our results contradict those from other studies which do measure chemical leachates above safe limits (Tawfik and Huyghebaert, 1998; Sanagi et al., 2008). Thus, more evidence is necessary. For wildlife, our results, and those of others (Cole et al., 2015; Sussarellu et al., 2016), suggest that increasing accumulations of polystyrene in marine and freshwater environments could lead to population-level effects in invertebrate species. In regard to sustainability, data should be compiled from cradle-to-grave to determine how sustainability metrics for polystyrene and EPS compare to other material types.
Author Contributions
CR, CT, and RR designed the leaching experiments. CR, DP, and KS designed the toxicity experiments. CT conducted the leaching experiments. KS, DP, and HD conducted the toxicity experiments. Chemistry was run and analyzed by RR, CT, JD, and GS. Data were statistically analyzed by CR. The initial draft of the manuscript was written by CR and CT. All authors contributed to all drafts of the manuscript.
Conflict of Interest Statement
The authors declare that the research was conducted in the absence of any commercial or financial relationships that could be construed as a potential conflict of interest.
Acknowledgments
A grant from 5Gyres supported this work. An NSERC USRA was awarded to CT and Don Jackson during the time of the experiment. We thank Xianming Zhang for aiding with chemical analysis and E. Reiner and P. Helm for advice regarding the experimental design, all from the Ontario Ministry of the Environment and Climate Change. We thank E. Guo for assistance in the laboratory.
Supplementary Material
The Supplementary Material for this article can be found online at: https://www.frontiersin.org/articles/10.3389/fmars.2018.00071/full#supplementary-material
References
Ahmad, M., and Bajahlan, A. S. (2007). Leaching of styrene and other aromatic compounds in drinking water from PS bottles. J. Environ. Sci. 19, 421–426. doi: 10.1016/S1001-0742(07)60070-9
Al-Odaini, N. A., Shim, W. J., Han, G. M., Jang, M., and Hong, S. H. (2015). Enrichment of hexabromocyclododecanes in coastal sediments near aquaculture areas and a wastewater treatment plant in a semi-enclosed bay in South Korea. Sci. Tot. Environ. 505, 290–298. doi: 10.1016/j.scitotenv.2014.10.019
Andersen, M. E., Cruzan, G., Black, M. B., Pendse, S. N., Dodd, D., Bus, J. S., et al. (2017). Assessing molecular initiating events (MIEs), key events (KEs) and modulating factors (MFs) for styrene responses in mouse lungs using whole genome gene expression profiling following 1-day and multi-week exposures. Toxicol. Appl. Pharmacol. 335, 28–40. doi: 10.1016/j.taap.2017.09.015
Bejgarn, S., MacLeod, M., Bogdal, C., and Breitholtz, M. (2015). Toxicity of leachate from weathering plastics: an exploratory screening study with Nitocra spinipes. Chemosphere 132, 114–119. doi: 10.1016/j.chemosphere.2015.03.010
Besseling, E., Wegner, A., Foekema, E. M., van den Heuvel-Greve, M. J., and Koelmans, A. A. (2012). Effects of microplastic on fitness and PCB bioaccumulation by the lugworm Arenicola marina (L.). Environ. Sci. Technol. 47, 593–600. doi: 10.1021/es302763x
Boerger, C. M., Lattin, G. L., Moore, S. L., and Moore, C. J. (2010). Plastic ingestion by planktivorous fishes in the North Pacific Central Gyre. Mar. Pollut. Bull. 60, 2275–2278. doi: 10.1016/j.marpolbul.2010.08.007
Borrelle, S. B., Rochman, C. M., Liboiron, M., Bond, A. L., Lusher, A., Bradshaw, H., et al. (2017). Opinion: why we need an international agreement on marine plastic pollution. Proc. Natl. Acad. Sci. U.S.A. 114, 9994–9997. doi: 10.1073/pnas.1714450114
Bravo, M., de los Ángeles Gallardo, M., Luna-Jorquera, G., Núñez, P., Vásquez, N., and Thiel, M. (2009). Anthropogenic debris on beaches in the SE Pacific (Chile): results from a national survey supported by volunteers. Mar. Pollut. Bull. 58, 1718–1726. doi: 10.1016/j.marpolbul.2009.06.017
Brown, F., and Diller, K. R. (2008). Calculating the optimum temperature for serving hot beverages. Burns 34, 648–654. doi: 10.1016/j.burns.2007.09.012
Browne, M. A., Chapman, M. G., Thompson, R. C., Amaral Zettler, L. A., Jambeck, J., and Mallos, N. J. (2015). Spatial and temporal patterns of stranded intertidal marine debris: is there a picture of global change? Environ. Sci. Technol. 49, 7082–7094. doi: 10.1021/es5060572
Cole, M., Lindeque, P., Fileman, E., Halsband, C., and Galloway, T. S. (2015). The impact of polystyrene microplastics on feeding, function and fecundity in the marine copepod Calanus helgolandicus. Environ. Sci. Technol. 49, 1130–1137. doi: 10.1021/es504525u
Convey, P., Barnes, D., and Morton, A. (2002). Debris accumulation on oceanic island shores of the Scotia Arc, Antarctica. Polar Biol. 25, 612–617. doi: 10.1007/s00300-002-0391-x
Cushman, J. R., Rausina, G. A., Cruzan, G., Gilbert, J., Williams, E., and Harrass, M. C. (1997). Ecotoxicity hazard assessment of styrene. Ecotox. Environ. Saf. 37, 173–180. doi: 10.1006/eesa.1997.1540
ECCC (2007). Biological Test Method: Test of Reproduction and Survival Using the Cladoceran Ceriodaphnia dubia. Report EPS/RM/21, 2nd Edition. ECCC.
Erickson, B. E. (2011). Formaldehyde, styrene cancer warning. Chem. Eng. News 89:11. doi: 10.1021/cen-v089n025.p011
Eriksen, M., Mason, S., Wilson, S., Box, C., Zellers, A., Edwards, W., et al. (2013). Microplastic pollution in the surface waters of the Laurentian Great Lakes. Mar. Pollut. Bull. 77, 177–182. doi: 10.1016/j.marpolbul.2013.10.007
Gall, S. C., and Thompson, R. C. (2015). The impact of debris on marine life. Mar. Pollut. Bull. 92, 170–179. doi: 10.1016/j.marpolbul.2014.12.041
Garrity, S. D., and Levings, S. C. (1993). Marine debris along the Caribbean coast of Panama. Mar. Pollut. Bull. 26, 317–324. doi: 10.1016/0025-326X(93)90574-4
Gibbs, B. F., and Mulligan, C. N. (1997). Styrene toxicity: an ecotoxicological assessment. Ecotox. Environ. Saf. 38, 181–194. doi: 10.1006/eesa.1997.1526
Jang, M., Shim, W. J., Han, G. M., Rani, M., Song, Y. K., and Hong, S. H. (2016). Styrofoam debris as a source of hazardous additives for marine organisms. Environ. Sci. Technol. 50, 4951–4960. doi: 10.1021/acs.est.5b05485
Jang, M., Shim, W. J., Han, G. M., Rani, M., Song, Y. K., and Hong, S. H. (2017). Widespread detection of a brominated flame retardant, hexabromocyclododecane, in expanded polystyrene marine debris and microplastics from South Korea and the Asia-Pacific coastal region. Environ. Pollut. 231, 785–794. doi: 10.1016/j.envpol.2017.08.066
Keller, A. A., Fruh, E. L., Johnson, M. M., Simon, V., and McGourty, C. (2010). Distribution and abundance of anthropogenic marine debris along the shelf and slope of the US West Coast. Mar. Pollut. Bull. 60, 692–700. doi: 10.1016/j.marpolbul.2009.12.006
Kershaw, P. J., and Rochman, C. M. (2015). Sources, Fate and Effects of Microplastics in the Marine Environment: Part 2 of a Global Assessment. Reports and studies-IMO/FAO/Unesco-IOC/WMO/IAEA/UN/UNEP Joint Group of Experts on the Scientific Aspects of Marine Environmental Protection (GESAMP) eng no. 93.
Kwon, B. G., Amamiya, K., Sato, H., Chung, S. Y., Kodera, Y., Kim, S. K., et al. (2017). Monitoring of styrene oligomers as indicators of polystyrene plastic pollution in the North-West Pacific Ocean. Chemosphere 180, 500–505. doi: 10.1016/j.chemosphere.2017.04.060
Kwon, B. G., Koizumi, K., Chung, S. Y., Kodera, Y., Kim, J. O., and Saido, K. (2015). Global styrene oligomers monitoring as new chemical contamination from polystyrene plastic marine pollution. J. Hazard. Mater. 300, 359–367. doi: 10.1016/j.jhazmat.2015.07.039
LeBlanc, G. A. (1980). Acute toxicity of priority pollutants to water flea (Daphnia magna). Bull. Environ. Con. Toxicol. 24, 684–691. doi: 10.1007/BF01608174
Lee, J., Hong, S., Song, Y. K., Hong, S. H., Jang, Y. C., Jang, M., et al. (2013). Relationships among the abundances of plastic debris in different size classes on beaches in South Korea. Mar. Pollut. Bull. 77, 349–354. doi: 10.1016/j.marpolbul.2013.08.013
Lithner, D., Larsson, A., and Dave, G. (2011). Environmental and health hazard ranking and assessment of plastic polymers based on chemical composition. Sci. Total Environ. 409, 3309–3324. doi: 10.1016/j.scitotenv.2011.04.038
Löhr, A., Savelli, H., Beunen, R., Kalz, M., Ragas, A., and Van Belleghem, F. (2017). Solutions for global marine litter pollution. Curr. Opin. Environ. Sust. 28, 90–99. doi: 10.1016/j.cosust.2017.08.009
Morét-Ferguson, S., Law, K. L., Proskurowski, G., Murphy, E. K., Peacock, E. E., and Reddy, C. M. (2010). The size, mass, and composition of plastic debris in the western North Atlantic Ocean. Mar. Pollut. Bull. 60, 1873–1878. doi: 10.1016/j.marpolbul.2010.07.020
Niaz, K., Hassan, F. I., Mabqool, F., Khan, F., Momtaz, S., Baeeri, M., et al. (2017). Effect of styrene exposure on plasma parameters, molecular mechanisms and gene expression in rat model islet cells. Environ. Toxicol. Pharmacol. 54, 62–73. doi: 10.1016/j.etap.2017.06.020
Ocean Conservancy (2017). International Coastal Cleanup Report. Available online at: https://oceanconservancy.org/blog/2017/06/05/results-international-coastal-cleanup/
Rani, M., Shim, W. J., Han, G. M., Jang, M., Al-Odaini, N. A., Song, Y. K., et al. (2015). Qualitative analysis of additives in plastic marine debris and its new products. Arch. Environ. Con. Toxicol. 69, 352–366. doi: 10.1007/s00244-015-0224-x
Rani, M., Shim, W. J., Han, G. M., Jang, M., Song, Y. K., and Hong, S. H. (2014). Hexabromocyclododecane in polystyrene based consumer products: an evidence of unregulated use. Chemosphere 110, 111–119. doi: 10.1016/j.chemosphere.2014.02.022
Sanagi, M. M., Ling, S. L., Nasir, Z., Ibrahim, W. A. W., and Abu Naim, A. (2008). Determination of residual volatile organic compounds migrated from polystyrene food packaging into food simulant by headspace solid phase microextraction–gas chromatography. Malays. J. Anal. Sci. 12, 542–551.
Schuyler, Q., Hardesty, B. D., Wilcox, C., and Townsend, K. (2014). Global analysis of anthropogenic debris ingestion by sea turtles. Conser. Biol. 28, 129–139. doi: 10.1111/cobi.12126
Sussarellu, R., Suquet, M., Thomas, Y., Lambert, C., Fabioux, C., Pernet, M. E., et al. (2016). Oyster reproduction is affected by exposure to polystyrene microplastics. Proc. Natl. Acad. Sci. U.S.A. 113, 2430–2435. doi: 10.1073/pnas.1519019113
Tawfik, M. S., and Huyghebaert, A. (1998). Polystyrene cups and containers: styrene migration. Food Addit. Contam. 15, 592–599. doi: 10.1080/02652039809374686
Underwood, A. J. (1997). Experiments in Ecology: Their Logical Design and Interpretation Using Analysis of Variance. Cambridge, UK: Cambridge University Press.
van Sebille, E., Wilcox, C., Lebreton, L., Maximenko, N., Hardesty, B. D., Van Franeker, J. A., et al. (2015). A global inventory of small floating plastic debris. Environ. Res. Lett. 10:124006. doi: 10.1088/1748-9326/10/12/124006
Winner, R. W. (1989). Multigeneration life-span tests of the nutritional adequacy of several diets and culture waters for Ceriodaphnia dubia. Environ. Toxicol. Chem. 8, 513–520. doi: 10.1002/etc.5620080608
Woodall, L. C., Sanchez-Vidal, A., Canals, M., Paterson, G. L., Coppock, R., Sleight, V., et al. (2014). The deep sea is a major sink for microplastic debris. R. Soc. Open Sci. 1:140317. doi: 10.1098/rsos.140317
World Health Organization (2004). Guideline for Drinking-Water Quality[M], 3rd Edn. World Health Organization.
Keywords: polystyrene, leachate, toxicity, plastic debris, ethylbenzene
Citation: Thaysen C, Stevack K, Ruffolo R, Poirier D, De Frond H, DeVera J, Sheng G and Rochman CM (2018) Leachate From Expanded Polystyrene Cups Is Toxic to Aquatic Invertebrates (Ceriodaphnia dubia). Front. Mar. Sci. 5:71. doi: 10.3389/fmars.2018.00071
Received: 06 December 2017; Accepted: 14 February 2018;
Published: 28 February 2018.
Edited by:
Francois Galgani, French Research Institute for Exploitation of the Sea (ifremer), FranceReviewed by:
Kamal Niaz, Università degli Studi di Teramo, ItalyDenis Moledo De Souza Abessa, Universidade Estadual Paulista Júlio de Mesquita Filho (UNESP), Brazil
Copyright © 2018 Thaysen, Stevack, Ruffolo, Poirier, De Frond, DeVera, Sheng and Rochman. This is an open-access article distributed under the terms of the Creative Commons Attribution License (CC BY). The use, distribution or reproduction in other forums is permitted, provided the original author(s) and the copyright owner are credited and that the original publication in this journal is cited, in accordance with accepted academic practice. No use, distribution or reproduction is permitted which does not comply with these terms.
*Correspondence: Chelsea M. Rochman, chelsea.rochman@utoronto.ca