- 1Ecology Department, Leibniz Centre for Tropical Marine Research (ZMT), Bremen, Germany
- 2Faculty of Biology & Chemistry (FB2), University of Bremen, Bremen, Germany
- 3Department of Biogeochemistry and Geology, Leibniz Centre for Tropical Marine Research (ZMT), Bremen, Germany
- 4Center for Marine, Coastal and Small Islands Research, Hasanuddin University, Makassar, Indonesia
- 5Theoretical Ecology and Modelling Department, Leibniz Centre for Tropical Marine Research (ZMT), Bremen, Germany
The Spermonde Archipelago is a complex of ~70 mostly populated islands off Southwest Sulawesi, Indonesia, in the center of the Coral Triangle. The reefs in this area are exposed to a high level of anthropogenic disturbances. Previous studies have shown that variation in the benthos is strongly linked to water quality and distance from the mainland. However, little is known about the fish assemblages of the region and if their community structure also follows a relationship with benthic structure and distance from shore. In this study, we used eight islands of the archipelago, varying in distance from 1 to 55 km relative to the mainland, and 3 years of surveys, to describe benthic and fish assemblages and to examine the spatial and temporal influence of benthic composition on the structure of the fish assemblages. Cluster analysis indicated that distinct groups of fish were associated with distance, while few species were present across the entire range of sites. Relating fish communities to benthic composition using a multivariate generalized linear model confirmed that fish groups relate to structural complexity (rugosity) or differing benthic groups; either algae, reef builders (coral and crustose coralline algae) or invertebrates and rubble. From these relationships we can identify sets of fish species that may be lost given continued degradation of the Spermonde reefs. Lastly, the incorporation of water quality, benthic and fish indices indicates that local coral reefs responded positively after an acute disturbance in 2013 with increases in reef builders and fish diversity over relatively short (1 year) time frames. This study contributes an important, missing component (fish community structure) to the growing literature on the Spermonde Archipelago, a system that features environmental pressures common in the greater Southeast Asian region.
Introduction
The distribution of fishes among coral reefs has been central to numerous studies throughout coastal and oceanic areas of the world (Bellwood and Hughes, 2001; Wismer et al., 2009; Kulbicki et al., 2013), including areas of research testing theories in biodiversity (Connolly et al., 2003) and assessing ecosystem function (Stuart-Smith et al., 2013), ecosystem services (Worm et al., 2006), the impacts of global and local disturbances (Wilson et al., 2006) and importantly, defining the baseline status of communities with respect to the presence of species and their abundances. The links between ecosystem variation and coral reef fish assemblages have been studied across a wide range of spatial scales (Bell and Galzin, 1984; Bellwood and Hughes, 2001; Alvarez-Filip et al., 2009; Wismer et al., 2009; Chong-Seng et al., 2012) and short and long temporal scales (Pandolfi et al., 2003; McClanahan et al., 2007; Babcock et al., 2010; Alvarez-Filip et al., 2015; Plass-Johnson et al., 2016b). It is well-known that the structure of coral reef fish assemblages and biodiversity are strongly linked to ecosystem health and functioning (Wismer et al., 2009; Chong-Seng et al., 2012). Although the relationship between coral reef fish communities and the habitat is complex, live coral cover, and diversity (Chabanet et al., 1997) and structural complexity (rugosity) have been found to be the most important determinants (Gratwicke and Speight, 2005; Alvarez-Filip et al., 2009; Graham and Nash, 2013). The sensitivity of coral reef fishes to changes in the habitat potentially make them important conveyors of local and regional ecosystem change (Graham et al., 2006; Wilson et al., 2010; Pratchett et al., 2011).
The Coral Triangle is the most diverse marine region in the world and accounts for 75% of all known coral species and more than 3,000 fish species (Veron et al., 2009; Burke et al., 2012). Marine resources of the Coral Triangle support ~130 million people locally, with tens of millions more when considering exports (Burke et al., 2011). Within this area, localized, human-derived pressure on coral reefs is considered to be of greater immediate concern than large-scale global pressures. A recent report has rated 85% of the reefs within the Coral Triangle as threatened, with overfishing, including destructive techniques, development and watershed pollution as the most pervasive and damaging threats (Burke et al., 2012). Although studies that examine processes that might drive ecosystem regime shifts are important, studies of their consequences on species' distributions and how long these effects last are critical to evaluate the potential impacts of global and local disturbances.
The extent and concentration of nutrient enrichment in coastal waters directly influence primary and secondary production, which in turn has substantial effects on the growth of scleractinian corals (Fabricius, 2011). In the Spermonde Archipelago (Southwest Sulawesi, Indonesia), long-term exposure to a pronounced on-to-offshore gradient in water quality from mainland Sulawesi has been a strong determinant in structuring coral reef benthic communities (Becking et al., 2006; Plass-Johnson et al., 2016a) and corals in particular (Sawall et al., 2013; Polónia et al., 2015). Nearshore sites have low coral diversity (Cleary et al., 2005) and structural complexity (Plass-Johnson et al., 2016b) and high abundances of turf algae (Plass-Johnson et al., 2016a). However, benthic communities are also structured by acute impacts such as dynamite fishing (Pet-Soede and Erdmann, 1998b; Sawall et al., 2013) and outbreaks of crown-of-thorns starfish (CoTS; Acanthaster cf. solaris, Vogler et al., 2008; Baird et al., 2013). A CoTS outbreak occurred in 2013, causing extensive loss of live coral at some sites (Plass-Johnson et al., 2015b). While the on-to-offshore gradient in coral cover is driven by the capacity of individual species to adapt in the long term to changing water conditions (Sawall et al., 2011), the short-term effect of acute disturbances will be determined by new recruitment and growth. Given the strong dependence of coral reef fishes on their coral habitat, spatial and temporal changes in the benthic habitat are expected to be reflected in the fish assemblages.
Although the benthic communities of the Spermonde Archipelago have received much attention (Edinger et al., 2000; Cleary et al., 2005; Becking et al., 2006; de Voogd et al., 2006; van der Ent et al., 2016; Kegler et al., 2017), relatively little work has focused on the consequences of benthic habitat disturbances for fish assemblages. The little data available have shown variation in fish species diversity following an on-to-offshore gradient in water quality and benthic communities, while their functional assemblage becomes more variable across very short time spans (hours) with an increase in disturbance (Plass-Johnson et al., 2016b). Nevertheless, the important function of herbivory is maintained across the archipelago, breaking down only in the most extreme environments (Plass-Johnson et al., 2015a). This is likely driven by the capacity of species to vary their trophic niche (Plass-Johnson et al., 2013, 2018). Changes in the fish assemblages are most likely linked to the degree of habitat degradation, where some fish species may have the capacity to persist because their trait composition allows adaptation while others will decrease in abundance due to their strong association with the habitat.
The general objective of this study was to fill a knowledge-gap concerning the fish communities of the Spermonde Archipelago by providing general patterns in fish communities, changes in their composition relative to an environmental gradient, and also provide data on species' distributions and relative abundances. Specific objectives were to (i) describe the coral reef fish assemblages in detail across an on-to-offshore gradient in the Spermonde Archipelago for 2012 through 2014; (ii) relate fish abundances to general proxies of habitat conditions; (iii) examine inter-annual stability of fish species assemblages across a spatial gradient across years that include strong, acute habitat disturbances; and lastly (iv) provide a general interpretation of the relative state of the reefs based on environmental and community indices. To accomplish this we surveyed fish and benthic assemblages and water quality at eight islands across the continental shelf off southwest Sulawesi, Indonesia, across 3 years. We examined within-year changes in fish assemblages, providing both quantitative and qualitative comparisons. Secondly, we conducted a time-series analysis to compare assemblages among years to examine inter-annual variation in abundances. These data provide a detailed report of the fish communities and how these communities react to relevant environmental drivers in the Spermonde Archipelago, thereby providing a baseline for future research, but we also interpret the relative state of the reefs based on less-specific environmental and community indices.
Methods and Materials
Study Site
This study was conducted across 3 consecutive years during the annual dry season in the Spermonde Archipelago, Indonesia. The first sampling campaign occurred between the 20th of September and the 11th of October, 2012. The second sampling was between the September 22nd and October 1st, 2013, and the third sampling was between the 16th and 29th of November, 2014. Consistently utilizing the dry season standardized sampling among years and also presented the most stable environment to guarantee successful sampling campaigns and allowing for a balanced experimental design. To relate patterns in fish communities to their habitat under consideration of the previously identified on-to-offshore gradients in water quality and benthos, eight islands with increasing distance from the city of Makassar were chosen (Figure 1). In order of increasing distance from shore, with distance in brackets, the islands comprised Laelae (LL; 05°08S, 119°23E, 1 km), Samalona (SA; 05°07S, 119°20E, 7 km), Barrang Lompo (BL; 05°02S, 119°19E, 11 km), Bonetambung (BO; 05°01S, 119°16E, 14 km), Badi (BA; 04°57S, 119°16E, 19 km), Lumulumu (LU; 04°58S, 119°12E, 22 km), Karang Kassi (KS; 04°53S, 119°09E, 27 km), and Kapoposang (KP; 04°41S, 118°57E, 55 km; Figure 1).
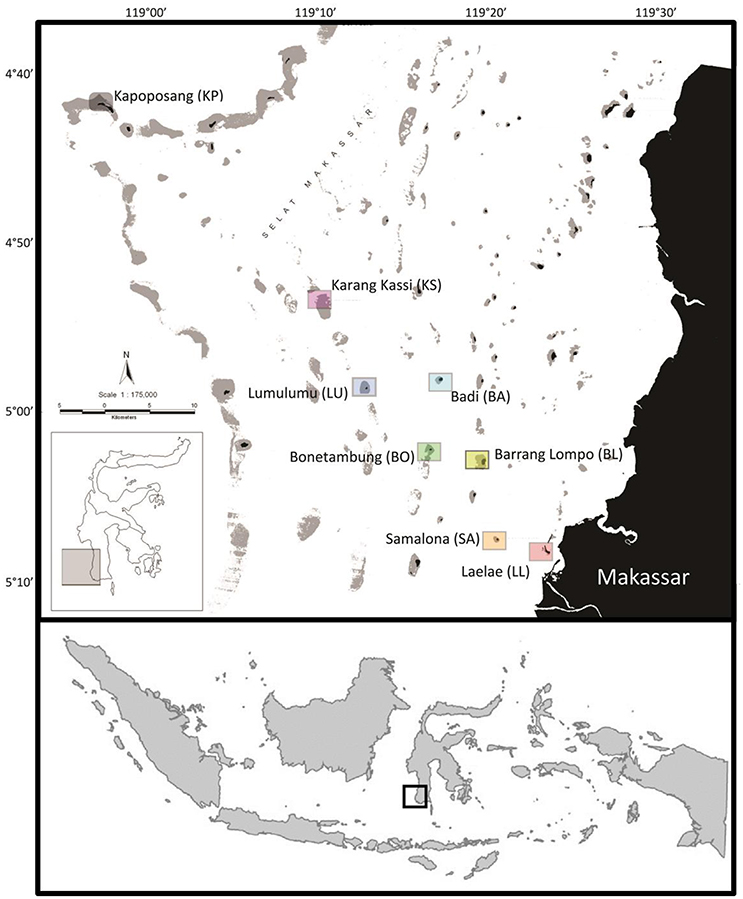
Figure 1. Map of the Spermonde Archipelago, Indonesia indicating the sampling sites. The lowest box indicates southwest Sulawesi relative to Indonesia and the middle inset indicates the location of the Spermonde Archipelago off southwest Sulawesi.
All islands are located on the continental shelf and, apart from KP, experience similar oceanographic conditions with respect to wave exposure and currents. KP was the only site which differed; located on the edge of the continental shelf, this site is exposed to deep oceanic waters, and stronger waves and currents. The northwest corner of each island (except KP) was used to standardize the sampling sites among reefs. The western sides of the islands generally feature a well-developed, carbonate fore-reef and a sandy back-reef and flat. The reef crest was shallow (~3 m) and the slope reaches down to 15 m. The last study site, KP, was located on the reef edge above the outer continental shelf wall (Figure 1). Work at KP was conducted at the northeast side of the island at the edge of the carbonate shelf because this area displays the highest level of reef accretion. Although KP differs from the other seven sites in habitat structure and wave exposure, fish communities at the other sites are subsets of the species at KP, with a small proportion of species present at each island due to adaptation to degraded habitats (Allen and Erdmann, 2012). Given KP's distance from the mainland, it receives no land-based effluents and minimal fishing pressure. Thus, the benthic and fish communities there represent a relatively healthy state of reef habitat allowing for comparative analyses. This site, however, should not be interpreted as a control because the presence of some fish species is determined by unique ecological niches created by the site's specific oceanographic conditions.
At each site, three 50 m permanent transects were installed along the reef and parallel to the shore, where all fish, benthic, and water environmental data were collected (see Teichberg et al., 2018 for details on water environmental data). Transects were standardized at 2 m below the reef crest, which for each site fell between 4 and 5 m depth at low tide. Transects were separated by at least 10 m, and the beginning and end of each transect were marked with steel rebar to provide attachment points for the transect tapes. All work, including fish and benthic surveys, were conducted during multiple dives throughout 1 day at each site. Fish surveys were always conducted by the same observer (JPJ) with one dive per transect. Benthic data was collected by a second diver following the first diver so as not to interfere with fish behavior. Work was started at a standardized time each day (08:00 h); weather was always sunny and dry with minimal wave energy.
Fish Surveys
Visual surveys of fish species were conducted within 2.5 m on either side of the permanent transects. The fish survey method followed Hill and Wilkinson (2004; fish belt transects), however this was modified to count both mobile and small species during the same survey. This was justified because mobile species had limited contribution to the communities allowing for greater focus on small species. Estimations of small schooling species were performed by identifying the area needed for 10 individuals of the same size, and this was multiplied to fit the entire school. This may have implications for abundance estimates within a size range if school aggregations separated based on size classes. If size groupings were observed, abundance estimates were modified to include only the space where those sizes were observable. All individuals of fish species >3 cm were counted and their size estimated to the nearest cm. Cryptic and transient, pelagic species were not recorded because accurate counts and identification could not be guaranteed in the former or, in the case of the latter, they have limited association with the reef as habitat. The exclusion of these species may result in over- or under-reporting structural changes at the community level in the fishes. Subsequent analyses identified the important functional group of herbivores to see their contribution to the biomass of the fish assemblages. Identification of species and diets followed Hill and Wilkinson (2012), Green and Bellwood (2009), and FishBase.org (Froese and Pauly, 2016). Biomass of fishes was calculated from individual size observations using length-weight relationships obtained from Kulbicki et al. (2005).
Fish Community Indices
For each transect, a number of community indices were calculated. These included: the total number of species, total abundance, total fish community biomass, herbivore biomass, the slope of the log-linear size spectrum of the total fish community (henceforth termed “slope”) and its y-intercept rescaled to the mid-point (henceforth termed “intercept”) (Daan et al., 2005; Graham et al., 2005). The slope is calculated by fitting a linear regression through the data points of the community-wide size frequency distribution, and intercept is the y-intercept of this slope when the y-axis is rescaled to the mid-point of the community-wide data. Slope and y-intercept are indicators of fishing where an increasingly negative slope across the size–spectrum results from the loss of large bodied fishes and a lower y-intercept results from a net reduction in abundances (Graham et al., 2005). Green and Bellwood (2009) was used to define herbivores. Each community index was analyzed with permutational analysis of variance (PERMANOVA) with year as a categorical factor and distance as a covariate. The full model, including the interaction, was assessed to check the assumption of homogeneity of slopes. When the interaction was significant, the effect was considered with regard to year. When the interaction was non-significant, it was removed from the model and the model was rerun.
Spatial and Temporal Analysis
To examine the spatial and temporal variation within fish communities at the species level, separate hierarchical cluster analyses were performed on fish assemblages for each year using the Bray-Curtis dissimilarity coefficient as the distance measure on fourth-root transformed data to reduce the influence of highly abundant species (Clarke et al., 2014). Rare fish species were removed from the analysis if their abundance was <0.1% of the total abundance across species within a year. Rare fish species can perform some of the most important ecological functions on coral reefs (Bellwood et al., 2003; Mouillot et al., 2013; Plass-Johnson et al., 2014) but their removal was considered appropriate because at very low abundances, encounter of species involves a stochastic element. Furthermore, our study concerns spatial and temporal patterns of communities, and the presence of rare species was considered to unduly influence clustering patterns (Busby et al., 2014). A hierarchical agglomerative cluster analysis was used with a flexible beta clustering method with β = −0.25. Significant clusters were identified via similarity profile analysis (SIMPROF) (Clarke et al., 2008) with α = 0.05. A similar clustering analysis was performed on sites to identify differences in fish communities among sites.
Differences in species composition across all years were examined with PERMANOVA using year and site as factors. As with the cluster analysis, PERMANOVAs were performed with the Bray-Curtis dissimilarity coefficient on fourth-root transformed abundances. When the overall test was significant, pairwise multiple comparison tests were conducted. Where significant differences were found (p < 0.05), a similarity percentages (SIMPER) (Clarke, 1993) analysis was used to identify the discriminating species. PERMANOVAs, cluster analyses and SIMPROF tests were all performed in PRIMER+ PERMANOVA v. 7.
Benthic-Fish Relationship
To determine whether fish communities were influenced by health of the benthic community, benthic community assessments reported in Teichberg et al. (2018) were recategorized into the following biotic groups: reef builders (crustose coralline algae [CCA] and live scleractinian coral), other invertebrates (defined as all non-scleractinian organisms), algae (macroalgae and turf algae), and cyanobacteria. A composite indicator of benthic community health was then derived by conducting a principal component analysis (PCA) of the benthic data. These broad benthic groupings based on Smith et al. (2016) were used because they represent temporal trajectories in reef health where increases in reef builders indicate carbonate accretion while fleshy algae indicate net loss of carbonate on a reef. As described in Teichberg et al. (2018), benthic communities were quantified with 50 photographic quadrats per 50 m transect. Photographs were taken at 1 m (standardized with a measuring pole) above the substratum, every 2 m along each transect. At every second meter, a photograph was taken on both sides of the transect tape, with a section of the tape within the picture, to identify total area of the picture. Analysis of the pictures was conducted with Coral Point Count with Excel extensions (CPCE; Kohler and Gill, 2006). Fifty randomized points (based on results from power analysis) were analyzed per photograph and classified into the biotic groups. To complement this, surface structure was also identified when benthic cover was any biotic group except live scleractinian coral. Surface structure groups comprised sand, rubble and pavement, with the latter two groups largely dominated by differing morphological forms of dead coral. Lastly, by combining live scleractinian coral with CCA, information is lost about the important contribution of structural complexity to the habitats. Therefore, rugosity was included as an additional measurement of the benthic habitat, and this was assessed with the linear distance-fitted chain method (Risk, 1972). The chain length used was 20 m, and measurement was conducted once per transect, starting at the first 10 m point.
To determine the relationship among benthic and fish communities, all benthic data were first analyzed with PCA (performed in PRIMER+ PERMANOVA v. 7) on square-root transformed data from which PC1 and PC2 were then used in multivariate generalized linear models (manyglm) from the mvabund package in R (Wang et al., 2012). Manyglm fits a separate, univariate, generalized linear model to the recorded abundance of each taxon and relates each abundance to a common set of explanatory variables to create a multivariate analysis across taxa. The function uses resampling-based hypothesis testing to make community-level and taxon-specific inferences, returning a multivariate analysis testing which factors or environmental variables are associated with the multivariate abundances (i.e., with the community of species as a whole). Manyglm was applied to each year and group of fish, i.e., y~Benthic PC1 + Benthic PC2, where y is a multispecies fish group identified by cluster analysis within a year, with a negative binomial distribution to determine the benthic groups that were most related to the fish abundances. A new model was fitted for each species group, resulting in a total of 22 models. Model fits were assessed by examining residual plots. Additionally, the relationships of individual species with the benthic PCs were also examined to see which species are contributing to patterns at the community level, i.e., y~Benthic PC1 + Benthic PC2, where y is a species within a year. All analyses were conducted at the level of transect.
Status of Spermonde Reefs (2012–2014)
To relate the state of reefs to each other, and assess how their status changed throughout the 3 years of sampling, PCAs were performed on the normalized fish community indices, and on water quality variables (data reported in Teichberg et al., 2018 and taken during the same sampling campaign). The first PCs from the benthic data (see section Benthic-Fish Relationship), fish indices, and water quality variables were then plotted in a bubble plot to visualize their relationships among sites and years. Fish indices were standardized prior to analyses because they involve highly differing values.
Results
Fish Community Indices
Overall, the total number of fish species was >100 across all sites, with the highest species number of 125 occurring in 2012 compared to 117 and 111 in 2014 and 2013, respectively. These totals consisted of many rare species (<0.1%), where only 53, 39, and 41% of all species contributed ≥0.1% of total abundance in 2012, 2013, and 2014, respectively, and were included for further analyses (see Supplementary Table 1 for full list of species recorded).
Among the fish indices, number of species, total and herbivore biomass, slope, and intercept increased in values with increasing distance from shore (Table 1). All PERMANOVAs, including the covariate interaction, of the fish indices, except for abundance, found distance to be significant (Table 1). The interaction was significant for the number of species and total biomass, showing that trends differed among years. In the reduced models, distance was significant for herbivore biomass, intercept and slope, and sampling year did not have a significant influence on any of the indices (Table 1).
Spatial and Temporal Patterns in Species Assemblages
Cluster analyses indicated that species assemblages and site groups (groups of sites that are similar in their fish community composition) were unique in 2013 compared to 2012 and 2014 (Figures 2–4 and Supplementary Figure 1). This was supported by the PERMANOVA [site × year: Pseudo-F(1, 62) = 2.811, p < 0.001], where fish assemblages in 2013 differed from 2012 [Pseudo-F(1, 41) = 1.502, p = 0.012] and 2014 [Pseudo-F(1, 41) = 1.889, p = 0.002]. Fish assemblages in 2012 and 2014 were not significantly different from each other [Pseudo-F(1, 41) = 3.531, p = 0.15]. The taxa contributing most to differences between 2012 and 2013 as indicated through the SIMPER analysis were Pomacentrus aurifrons, Pomacentrus alexanderae, Pomacentrus adelus, Chrysiptera rollandi, and Scarus flavipectoralis (Figures 2, 3). These species contributed a total of 30% of the difference between years. Much of the difference (31%) between 2013 and 2014 was attributed to the same species and additionally to Pomacentrus burroughi and Cirrhilabrus ryukyuensis (Figures 3, 4).
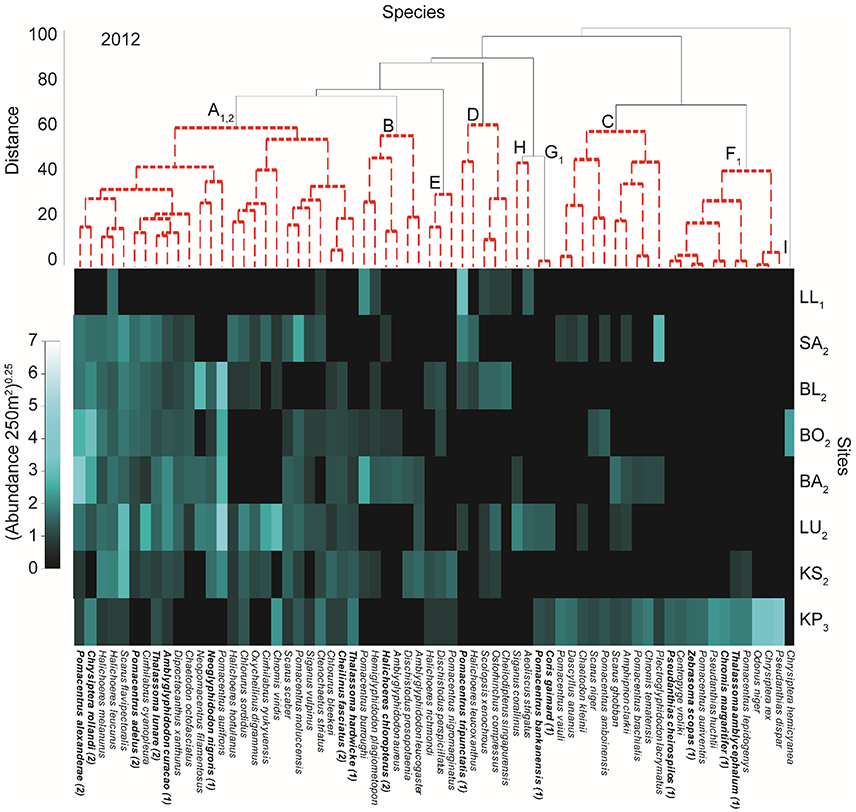
Figure 2. Shade plot of relative species abundances in 2012. Results of cluster analysis showing significant groups (SIMPROF test) of species (capital lettering on species dendrogram and indicated with red dotted line) and sites (numbered subscript on site acronyms and identified in Supplementary Figure 1) with relative abundance of fish indicated in the shade plot. Sites are ordered from top to bottom increasing in distance from the mainland (Figure 1). Subscript on species groups indicates a significant relationship with PCs of the benthic PCA (Supplementary Figure 3) as evaluated by multivariate GLM (Supplementary Table 2). Bolded species names indicate a specie that is related to at least one of the two (indicated in parentheses after specie name) benthic PCs. Full results for individual species can be found in Supplementary Table 1.
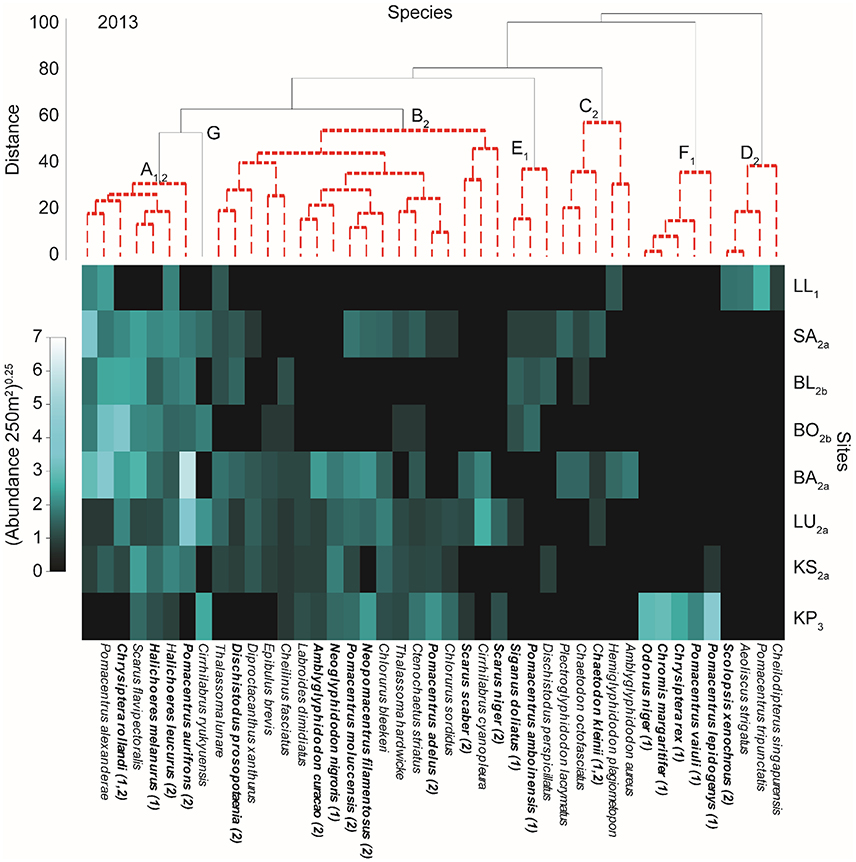
Figure 3. Shade plot of relative species abundances in 2013. Results of cluster analysis showing significant groups (SIMPROF test) of species (capital lettering on species dendrogram and indicated with red dotted line) and sites (numbered subscript on site acronyms and identified in Supplementary Figure 1) with relative abundance of fish indicated in the shade plot. Sites are ordered from top to bottom increasing in distance from the mainland (Figure 1). Subscript on species groups indicates a significant relationship with PCs of the benthic PCA (Supplementary Figure 3) as evaluated by multivariate GLM (Supplementary Table 2). Bolded species names indicate a specie that is related to at least one of the two (indicated in parentheses after specie name) benthic PCs. Full results for individual species can be found in Supplementary Table 1.
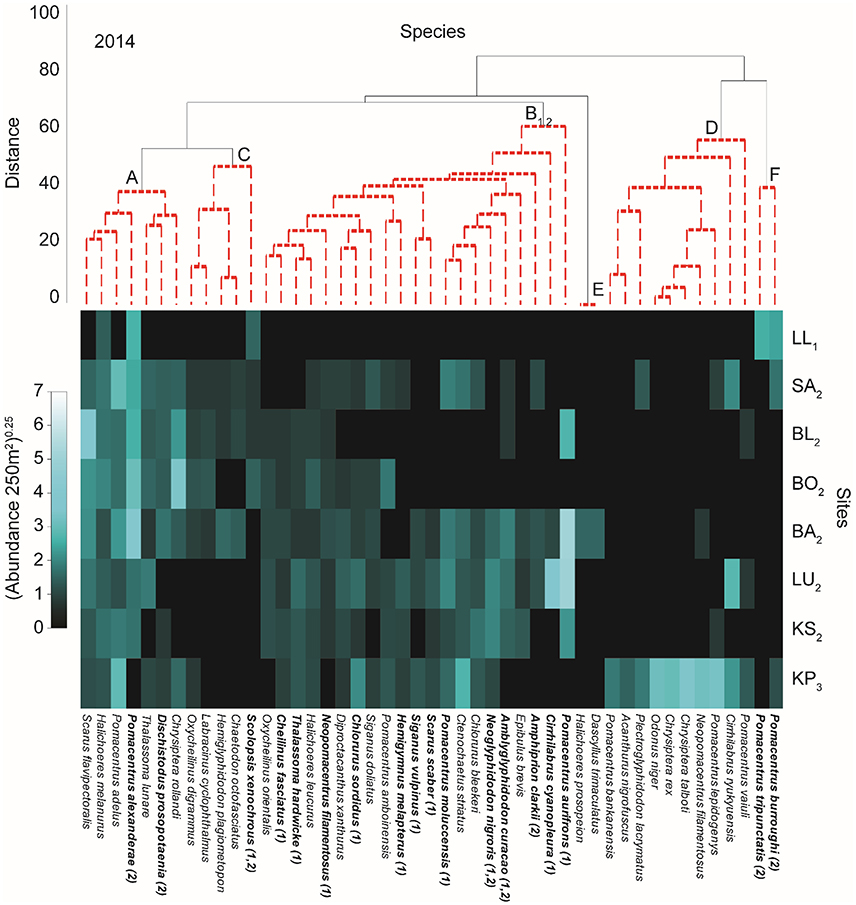
Figure 4. Shade plot of relative species abundances in 2014. Results of cluster analysis showing significant groups (SIMPROF test) of species (capital lettering on species dendrogram and indicated with red dotted line) and sites (numbered subscript on site acronyms and identified in Supplementary Figure 1) with relative abundance of fish indicated in the shade plot. Sites are ordered from top to bottom increasing in distance from the mainland (Figure 1). Subscript on species groups indicates a significant relationship with PCs of the benthic PCA (Supplementary Figure 3) as evaluated by multivariate GLM (Supplementary Table 2). Bolded species names indicate a specie that is related to at least one of the two (indicated in parentheses after specie name) benthic PCs. Full results for individual species can be found in Supplementary Table 1.
From the cluster analyses it appears that some species groups and station groups were related to distance from shore (Figures 2–4). In 2012, the assemblage structure consisted of nine species groups (A–I; groups of species that display similar distribution in their abundances across the sites) and three site groups (1–3) (Figure 2 and Supplementary Figure 1). The six middle sites grouped together (site group 2), with the two end sites, LL (site group 1) and KP (site group 3), forming a separate category each (Figure 2 and Supplementary Figure 1). Moderate to high abundances of species in group A12 (henceforth subscript indicates survey year [i.e., 2012: 12; 2013: 13; 2014: 14] for species groups) characterized the middle sites (group 2), with Pomacentrus alexanderae, Chrysiptera rollandi, and Scarus flavipectoralis having very high abundances at many sites (Figure 2). Species groups D12, H12, and B12 contained species robust to highly degraded habitats as they were characteristic of LL (group 1), with Pomacentrus tripunctatus displaying very high abundances. The species of groups D12, H12, and B12 were not unique to LL, and many of them were also present in low abundances among middle sites (site group 2; Figure 2). KP was characterized by species groups C12 and F12. Species of group F12 were mostly restricted to KP, while some of the species in group C12 were also found in low abundances at some of the middle sites (Figure 2). Pseudanthias dispar, Chrysiptera rex, and Odonus niger (group F12) had the highest abundances at KP.
Cluster analysis and ANOSIM indicated that site groupings in 2013 separated into four groups (1, 2a, 2b, 3) and the assemblage structure consisted of seven species groups (A–G; Figure 3 and Supplementary Figure 1). While LL (site group 1) and KP (site group 3) again each constituted a distinct category, site group 2 from 2012 split into two groups (2a and 2b) in 2013. The new site group 2b consisted of the third and fourth farthest sites from the mainland (Figures 2, 3 and Supplementary Figure 1). A second group among the middle sites in 2013 consisted of the second and fifth through seventh (site group 2a; in distance from shore) sites (Figure 3 and Supplementary Figure 1). Similar to 2012, species group A13 was found across the middle sites (site groups 2a and 2b); however, the number of species in that group was much reduced (Figure 3). Species with high abundances in species group A13 were Pomacentrus aurifrons, Pomacentrus burroughi, and Pomacentrus alexanderae. Site group 2b was much reduced in the number of species compared to site group 2 in 2012 (Figures 2, 3). Site group 2a shared many of the species from 2012. Pomacentrus moluccensis, Neopomacentrus filamentosus, Neoglyphidodon nigroris, and Amblyglyphidodon curacao all had high abundances at site group 2a. Species groups C13, E13, and G13 occurred, with varying abundances, across both groups of middle sites. KP was also characterized by many of the same species as in 2012, and these were exclusive to this site (Figure 3). In contrast to the previous year, one group of species (group D13; Pomacentrus tripunctatis, Cheilodipterus singapurensis, Aeoliscus strigatus, and Scolopsis xenochrous) was unique to LL.
In 2014 there were five species groups (A14–F14) and three site groups (1–3; Figure 4 and Supplementary Figure 1). The site groupings were the same as 2012 (Figures 2, 4). Species group A14, with very high abundances of Pomacentrus alexanderae and Pomacentrus adelus, was present across all site groups. The species group B14 was present at all sites but with markedly low abundances for LL. Pomacentrus aurifrons was particularly high in abundance for this species group. KP again was characterized by a group of species with high abundance mostly restricted to this site (species group D14). Species group F14 comprised of a few species that were found in high abundances at LL (Figure 4).
Benthic-Fish Relationships
Across years and sites the dominant benthic biotic group was algae (Table 2), with a large proportion of this being turf algae (see Teichberg et al., 2018 for more detailed description of benthic groups). The cover of algae was generally lowest at KP, the site furthest from the mainland, and increased at each site closer to shore. The exception was the site closest to shore, LL, where algal cover was relatively low (Table 2). Reef builders displayed an opposing trend, with offshore sites having highest cover and nearshore sites the lowest (Table 2). In 2013, BO (the fourth site along the gradient) had the lowest cover of reef builders (3%); however, this rebounded to 13% in 2014 (Teichberg et al., 2018). Likewise, in 2012 and 2013 LU (the sixth site along the gradient) had ~35% cover of reef builders, but this increased drastically in 2014 to 71% (Table 2) due to growth of crustose coralline algae.
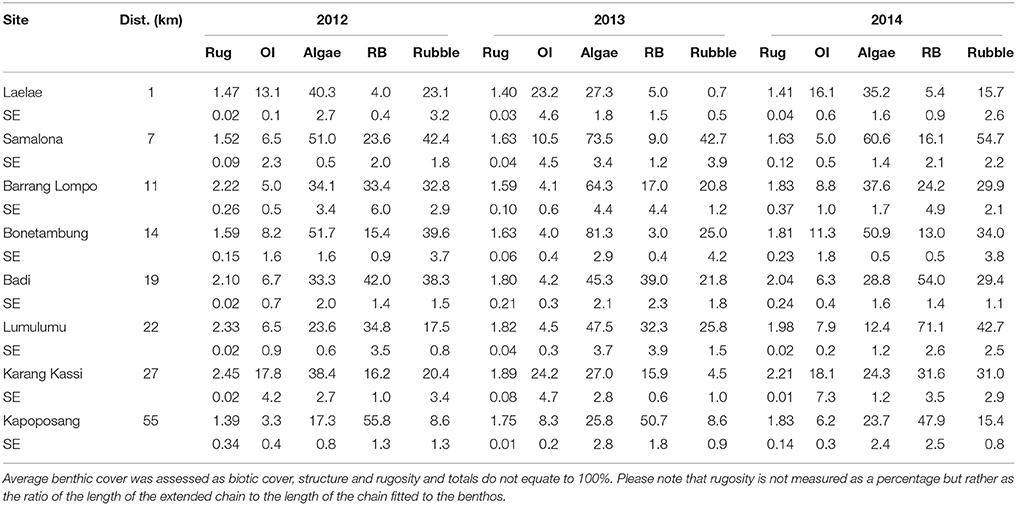
Table 2. Average (% ±SE) cover of benthic functional groups [OI, other invertebrates (sponges, tunicates, etc.), Algae (macroalgae + turf algae), RB, reef builders (live coral + CCA), Rubble] and structural complexity (Rug = rugosity).
The PCA on benthic communities found that much of the information was retained on the first two axes (70.4%, henceforth termed PC1benthic and PC2benthic; Supplementary Figures 2, 3). PC1benthic was largely determined by algae, reef builders and rugosity, while PC2benthic was affected by other invertebrates, rubble and reef builders (Supplementary Figure 2). In general, there was a pattern, moving left to right along PC1benthic, that corresponded with a shift from algae to an increase in reef builder cover (Figure 5). Therefore PC1benthic can be seen as a surrogate for benthic condition as defined by Smith et al. (2016), while PC2benthic is characterized by other invertebrates and rubble.
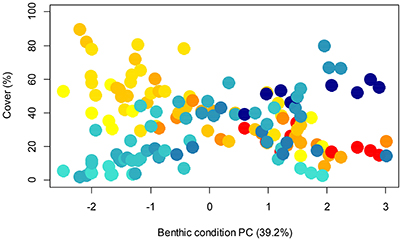
Figure 5. Relationship between the benthic condition PC (benthic PC1), reef builders (blue) and algae (yellow-red) showing all transects with greater distance from shore indicated by darker colors. This shows the opposing relationship of the two benthic groups with benthic PC1.
Using PC1benthic and PC2benthic for the manyglm analysis on fish abundances for each year provides evidence that benthic condition affected some fish groups, although a few were not significantly related to the benthos. For 2012, species group A12 was affected by both PC1benthic and PC2benthic (Figure 2, see subscripts top panel, Supplementary Table 2). Species groups G12 and F12 were related to PC1benthic alone (Table 2). In 2013 species group A13 was also related to both PCsbenthic (Figure 3, Supplementary Table 2), E13 and F13 were related to PC1benthic and B13, C13 and D13 were related to PC2benthic (Figure 3, Supplementary Table 2). In 2014 only species group B14 was significantly related to any benthic PC, being linked to both PC1benthic and PC2benthic (Figure 4, Supplementary Table 2).
Among individual species only two species, Amblyglyphidodon curacao and Neoglyphidodon nigroris, were related to at least one benthic PC for each year of the analyses (Figures 2–4 and Supplementary Table 1). Nine species (Cheilinus fasciatus, Chromis margaritifer, Dischistodus prosopotaenia, Pomacentrus adelus, Pomacentrus alexanderae, Pomacentrus aurifrons, Scolopsis xenochrous, and Thalassoma hardwicke) were related to the same benthic PC in two different years, and 23 species showed a significant relationship to one benthic PC in one of the years. Of all the species displaying significant relationships with the benthic PCs (36), the majority (28) were related to PC1benthic. Interestingly, species with very high abundances at KP only displayed significant relationships to PC1benthic, never to PC2benthic, while abundant species at LL (Scolopsis xenochrous and Pomacentrus tripunctatis) only displayed significant relationships to PC2benthic.
As shown in Teichberg et al. (2018), water quality parameters correlated with distance from shore and similarly did the benthic community (PC1benthic, algae, reef builder, rugosity). In a bi-plot defined by PC1benthic and by water quality (PC1water), the islands followed an order determined by distance to shore so that pronounced regions (nearshore, mid shelf, outer shelf) could be identified (Figure 6). When combined with fish indices, the pattern confirms greater complexity of fish communities along the distance gradient, however with obvious yearly variations (Figures 2–4). Water quality (PC1water) discriminated among nearshore reefs (LL, SL but also for BO and BA in 2012), whereas benthic condition (PC1benthic) discriminated sites along the entire gradient. The clustering was more spread for nearshore reefs (and partly mid shelf islands), indicating a higher variability and e.g. reaction to local biotic disturbances (CoTS outbreak). Thus, linking water quality with benthic community data analyses reveled a clear pattern for fish health indicators increasing with distance from shore.
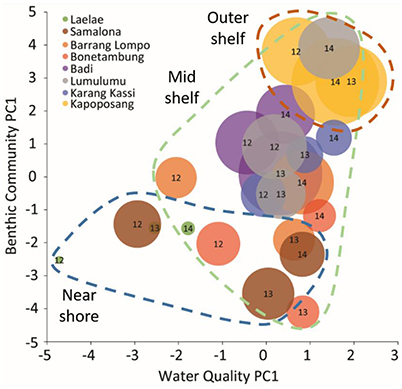
Figure 6. Relationship of PC1s from independent benthic community (Figure 5), water quality (Supplementary Figure 4), and fish indices (Supplementary Figure 5) PCAs. A higher score on the benthic PC1 indicates communities with relatively high cover (%) of reef builders while low values indicate high cover of algae (Figure 5). A high value on the water quality PC1 indicates better water quality (i.e., low dissolved organic carbon and chlorophyll a and high oxygen). The size of the bubbles represents a relatively higher score on the fish PC1 indicating higher values in most fish community indices or, a healthier fish community. The numbers within the bubbles represent the year of sampling (2012: 12, 2013: 13, 2014: 14). Dashed lines indicate groups of sites relative to distance from shore (blue = nearshore, green = mid-shelf, and red = outer-shelf) providing visual interpretation. These groups are loosely based on Renema and Troelstra (2001), however Samalona was grouped with Laelae because values were similar, while Kapoposang was defined as the sole outer shelf site because its fish communities composition is distinct.
Discussion
We predicted that the coral reef fish communities of the Spermonde Archipelago would be sensitive to acute perturbations, and we anticipated that there would be differences in assemblage structures related to the on-to-offshore gradient displayed in the benthic habitat. Indeed there was a significant change in fish assemblage structure (i.e., species composition and their abundances) in 2013 coinciding with the outbreak of the live coral predator, CoTS (Plass-Johnson et al., 2015b). Additionally, within a year, there were significant differences in fish communities among groups of sites related to their distance from shore and in some instances, this variation was determined by a limited set of species.
When comparing differences in fish communities between 2013 and the other 2 years (2012 vs. 2013, 2013 vs. 2014), five species contributed to differences for both year pairings (Pomacentrus aurifrons, Pomacentrus alexanderae, Pomacentrus adelus, Chrysiptera rollandi, and Scarus flavipectoralis).
Moreover, in this study we could identify groups of fish species that displayed a relationship to the on-to-offshore variation in benthic structure, while other groups displayed more localized or stochastic patterns. In all 3 years, species group A was generally abundant across all sites. The species associated with species group A were generally dominated by the families Pomacentridae (damselfishes) and Labridae (wrasses), and this species group was significantly related to all benthic components (PC1benthic and PC2benthic) in 2012 and 2013. This relationship was not evident in 2014, suggesting that the species composition and abundances of this group, which changed after strong acute disturbance, was not strongly related to the habitat. In 2013, the separation of site group 2a from site group 2b was largely driven by the loss of rare species (in that year, 43 species fell above the cut-off, as opposed to 66 in the previous year). The two sites comprising this new group were the most impacted by a loss of critical, live coral habitat due to the CoTS outbreak (Plass-Johnson et al., 2015b).
The communities from the farthest site from shore were never associated with other sites, underlining the unique fish assemblage at KP [2012 and 2013 = species group F(12, 13), 2014 = species group D14]. In 2012 and 2013 this species group was related to the PC1benthic and likewise, some of the species, a mix of anthias, acanthurids, pomacentrids, labrids, and balistids, are associated with seaward reefs with high coral cover (Allen and Erdmann, 2012) while also sharing many of the most dominant species (e.g., Ctenochaetus striatus, Chlorurus bleekeri, Chrysiptera rollandi, and Thalassoma lunare) with the other sites. Separation of the nearshore site, LL (group site 1), was most likely driven by the relatively low number of species and their abundances found there. This was the only site that had a proportionally high number of species from the labrids, a group that is generally robust to conditions of low coral cover and high sedimentation (Osenberg et al., 2004) due to its reliance on infauna as sources of nutrition (Kramer et al., 2015).
Relationships between individual species and the benthos may help to understand the consequences for reef fish assemblages under continued or intensified degradation of the reef system. While fish species related to PC1benthic are likely to be affected by processes that reduce live coral cover, species related to PC2benthic respond rather to other processes that increase non-coral species (increases in sponges and algae), and those species displaying no significant relationship appear to be less dependent on the benthic components included in our assessment, or react in complex and non-linear ways. Therefore, the species related to benthic PCs are most likely to respond to benthic recovery, while those unrelated are most likely to persist in the face of habitat degradation, or alternatively, respond in an unpredictable way. Because of their close relationship with attributes associated with healthy coral reefs, species with a positive correlation to PC1benthic may act as potential sentinels for ecosystem monitoring.
Differences in fishing intensity among sites may result in differential effects on the reef fish community structure. Information on the spatial distribution of fishing activity was not available for the present study. However, a previous study by Pet-Soede et al. (2001) did not detect effects of fishing intensity on the spatial structure of reef fish communities across Spermonde, and effects of fishing are thus assumed to act mostly via alteration of the benthic habitat. It should be noted that although we used broad indicators of relative benthic health (PCA axes) to help interpret the effects of changing habitat health on fish communities, this study does not discern among the many potential contributors to habitat modification/degradation. In the Spermonde Archipelago, blast fishing (Pet-Soede and Erdmann, 1998a) and boat anchoring (Edinger et al., 1998) are noted as significant contributors to benthic degradation. Less-studied but noted additional impacts include cyanide fishing, localized sewage output from the islands and boat groundings (Edinger et al., 1998). These can result in the mechanical alteration of the seafloor and/or directly affect the fish communities, contributing to an increased rate of mortality among these organisms. Given that our analysis only considered spatial and temporal change in the state of benthic organisms, this study cannot link specific impact types to their effect on the fish communities, but community indices can provide an indication of drivers on fish communities (e.g., slope of the log-linear size spectrum; see section Fish Community Indices).
All fish community indices, besides abundance, were significantly affected by distance from shore. Fish communities farther from shore had more larger fishes but also more species and biomass. The important functional group of herbivores was also greater in biomass with greater distance from shore. For two indices, number of species and total biomass, the effect of distance differed among years. While number of species showed a general increase across the shelf in 2012 and 2014, that trend was less pronounced in 2013. In contrast, the increase in biomass away from shore became more pronounced over the 3 years. A similar trend was apparent for herbivore biomass. At LL and LU, the community size spectra displayed steep slopes, reflecting a lack of larger individuals, while the assemblages at SA, BL, and KP were more equally distributed among size classes. A decrease of the size spectra slope may also result from a reduction of juvenile fishes following habitat degradation (Wilson et al., 2010). The lack of a consistent effect of sampling year on slope indicates that benthic communities at the different islands did not show a consistent trajectory over the years, which is in line with the results of the benthic PCA. Low intercept values reflect a low community biomass (Trenkel and Rochet, 2003), and indeed the intercept and total biomass values showed a similar pattern across the sites and years. Community biomass was particularly low at those sites with high fishing pressure (LL, BL, and KA). Biomass values at several islands were below 200 kg/ha, indicating severe overfishing with potential negative consequences for key ecosystem processes (McClanahan et al., 2011). At KP, biomass values in the last 2 years approached those estimated for unfished reefs (MacNeil et al., 2015). Together, the different community indices show a relatively coherent picture of a gradient in the fish assemblage from on-to-offshore across the shelf. Yet, they also reflect the patchy nature of the environment in the Spermonde Archipelago, with some of the reefs along the gradient displaying degradation of the benthos and fishing impacts from local anthropogenic factors and the outbreak of CoTS in 2013.
Overall, the combined results from the multi-year investigations (Figure 6) confirm the on-to-offshore gradient is reflected in both the water quality and benthic community composition, and correlate with the derived fish community health indicators. Interestingly water quality is more important to discriminate among nearshore sites. This is consistent with Gilby et al. (2016), who indicate that water quality outweighs the influence of marine protected areas in an Australian bay system. Additionally, the marked on-to-offshore gradient in water quality corresponds clearly with the benthic communities of the reef system. In a gradient analysis of Thousands Island in Jakarta Bay, Cleary et al. (2015) identified a similar gradient in water quality and benthic community but also emphasize changes in species composition and their abundances among sites.
By covering 3 subsequent years our study allows to observe the eminent variability between nearshore and mid-shelf sites, and among years as a reaction to recent disruptions, thus emphasizing the need to conduct long-term monitoring to distinguish between specific impacts. This study illustrates the potential of reefs in the Spermonde Archipelago to react positively after what seems to be acute disturbances. Although a previous study identified a CoTS outbreak in 2013, we are not able to identify this as the primary source of change in the benthic community. Destructive fishing practices and/or anchoring could also contribute to the observed patterns in the benthic and fish communities. To analyze the potential for recovery, and the overall ecological trajectory as a response to changes in the environment and resource use, long term monitoring is needed (Glaser et al., 2018). Here, those species identified as sensitive to changes in reef builders may be used for identifying changes in the reef ecosystem. Through the analysis of the on-to-offshore gradient and multi-year character, this study fills an intermediate gap about variation in fish communities and thus contributes important, fundamental knowledge on the fish community structure in the Spermonde Archipelago; a baseline that could contribute to future monitoring work. This is especially important in a system that receives severe direct and indirect human pressure common to many reef systems of South-East Asian islands.
Author Contributions
All authors listed have made a substantial, direct and intellectual contribution to the work, and approved it for publication.
Funding
This study was funded by the German Federal Ministry of Education and Research (BMBF; Grant No. 03F0643A) under the bilateral German Indonesian project, Science for the Protection of Indonesian Coastal Ecosystems (SPICE) III. SCAF acknowledges additional funding from the German Federal Ministry of Education and Research, BMBF (REPICORE project, grant number: 01LN1303A).
Conflict of Interest Statement
The authors declare that the research was conducted in the absence of any commercial or financial relationships that could be construed as a potential conflict of interest.
The reviewer MC and handling Editor declared their shared affiliation.
Acknowledgments
We want to thank the University of Hasanuddin for access to the Barrang Lompo research station. Also, P. Thiriet for statistical help, N. Abu for logistical help, and N. Hertz for help with the data. We would like to also thank the Indonesian Ministry of Science and Technology (Kementerian Riset dan Teknologi) for permitting our work in the Spermonde Archipelago (Permit numbers: 353/SIP/FRP/SM/IX/2012 and 544/FRP/SM/X/2014).
Supplementary Material
The Supplementary Material for this article can be found online at: https://www.frontiersin.org/articles/10.3389/fmars.2018.00036/full#supplementary-material
References
Allen, G., and Erdmann, M. (2012). Reef Fishes of the East Indies. Perth, WA: Tropical Reef Research.
Alvarez-Filip, L., Dulvy, N. K., Gill, J. A., Côté, I. M., and Watkinson, A. R. (2009). Flattening of Caribbean coral reefs: region-wide declines in architectural complexity. Proc. R. Soc. B Biol. Sci. 276, 3019–3025. doi: 10.1098/rspb.2009.0339
Alvarez-Filip, L., Paddack, M. J., Collen, B., Robertson, D. R., and Côté, I. M. (2015). Simplification of Caribbean reef-fish assemblages over decades of coral reef degradation. PLoS ONE 10:e0126004. doi: 10.1371/journal.pone.0126004
Babcock, R. C., Shears, N. T., Alcala, A. C., Barrett, N. S., Edgar, G. J., Lafferty, K. D., et al. (2010). Decadal trends in marine reserves reveal differential rates of change in direct and indirect effects. Proc. Natl. Acad. Sci. U.S.A. 107, 18256–18261. doi: 10.1073/pnas.0908012107
Baird, A. H., Pratchett, M. S., Hoey, A. S., Herdiana, Y., and Campbell, S. J. (2013). Acanthaster planci is a major cause of coral mortality in Indonesia. Coral Reefs 32, 803–812. doi: 10.1007/s00338-013-1025-1
Becking, L., Cleary, D., de Voogd, N., Renema, W., de Beer, M., van Soest, R. W. M., et al. (2006). Beta diversity of tropical marine benthic assemblages in the Spermonde Archipelago, Indonesia. Mar. Ecol. 27, 67–88. doi: 10.1111/j.1439-0485.2005.00051.x
Bell, J. D., and Galzin, R. (1984). Influence of live coral cover on coral-reef fish communities. Mar. Ecol. Prog. Ser. 15, 265–274. doi: 10.3354/meps015265
Bellwood, D. R., Hoey, A. S., and Choat, J. H. (2003). Limited functional redundancy in high diversity systems: resilience and ecosystem function on coral reefs. Ecol. Lett. 6, 281–285. doi: 10.1046/j.1461-0248.2003.00432.x
Bellwood, D. R., and Hughes, T. P. (2001). Regional-scale assembly rules and biodiversity of coral reefs. Science 292, 1532–1535. doi: 10.1126/science.1058635
Burke, L., Reytar, K., Spalding, K., and Perry, A. (2012). Reefs at Risk Revisited in the Coral Triangle. Rome. Available online at: https://www.wri.org
Burke, L., Reytar, K., Spalding, M., and Perry, A. (2011). Reefs at Risk: Revisited. Washington, DC: World Resource Institute. Available online at: https://www.wri.org
Busby, M. S., Duffy-Anderson, J. T., Mier, K. L., and De Forest, L. G. (2014). Spatial and temporal patterns in summer ichthyoplankton assemblages on the eastern Bering Sea shelf 1996-2007. Fish. Oceanogr. 23, 270–287. doi: 10.1111/fog.12062
Chabanet, P., Ralambondrainy, H., Amanieu, M., Faure, G., and Galzin, R. (1997). Relationships between coral reef substrata and fish. Coral Reefs 16, 93–102. doi: 10.1007/s003380050063
Chong-Seng, K. M., Mannering, T. D., Pratchett, M. S., Bellwood, D. R., and Graham, N. A. (2012). The influence of coral reef benthic condition on associated fish assemblages. PLoS ONE 7:e42167. doi: 10.1371/journal.pone.0042167
Clarke, K., Gorley, R., Somerfield, P., and Warwick, R. (2014). Change in Marine Communities: An Approach to Statistical Analysis and Interpretation, 3rd Edn. Plymouth: PRIMER-E.
Clarke, K. R. (1993). Non-parametric multivariate analyses of changes in community structure. Aust. J. Ecol. 18, 117–143. doi: 10.1111/j.1442-9993.1993.tb00438.x
Clarke, K. R., Somerfield, P. J., and Gorley, R. N. (2008). Testing of null hypotheses in exploratory community analyses: similarity profiles and biota-environment linkage. J. Exp. Mar. Biol. Ecol. 366, 56–69. doi: 10.1016/j.jembe.2008.07.009
Cleary, D. F. R., Becking, L. E., de Voogd, N. J., Renema, W., de Beer, M., van Soest, R. W. M., et al. (2005). Variation in the diversity and composition of benthic taxa as a function of distance offshore, depth and exposure in the Spermonde Archipelago, Indonesia. Estuar. Coast. Shelf Sci. 65, 557–570. doi: 10.1016/j.ecss.2005.06.025
Cleary, D. F., Polónia, A. R. M., Renema, W., Hoeksema, B. W., Rachello-Dolmen, P. G., Moolenbeek, R. G., et al. (2015). Variation in the composition of corals, fishes, sponges, echinoderms, ascidians, molluscs, foraminifera and macroalgae across a pronounced in-to-offshore environmental gradient in the Jakarta Bay-Thousand Islands coral reef complex. Mar. Pollut. Bull. 110, 701–717. doi: 10.1016/j.marpolbul.2016.04.042
Connolly, S., Bellwood, D., and Hughes, T. (2003). Indo-Pacific biodiversity of coral reefs: deviations from a mid-domain model. Ecology 84, 2178–2190. doi: 10.1890/02-0254
Daan, N., Gislason, H., Pope, J. G., and Rice, J. C. (2005). Changes in the North Sea fish community: evidence of indirect effects of fishing? ICES J. Mar. Sci. 62, 177–188. doi: 10.1016/j.icesjms.2004.08.020
de Voogd, N., Cleary, D., Hoeksema, B., Noor, A., and van Soest, R. (2006). Sponge beta diversity in the Spermonde Archipelago, SW Sulawesi, Indonesia. Mar. Ecol. Prog. Ser. 309, 131–142. doi: 10.3354/meps309131
Edinger, E., Jompa, J., Limmon, G., Widjatmoko, W., and Risk, M. (1998). Reef degradation and coral biodiversity in Indonesia: effects of land-based pollution, destructive fishing practices and changes over time. Mar. Pollut. Bull. 36, 617–630. doi: 10.1016/S0025-326X(98)00047-2
Edinger, E., Kolasa, J., and Risk, M. (2000). Biogeographic variation in coral species diversity on coral reefs in three regions of Indonesia. Divers. Distrib. 6, 113–127. doi: 10.1046/j.1472-4642.2000.00076.x
Fabricius, K. E. (2011). “Factors determining the resilience of coral reefs to eutrophication: a review and conceptual model,” in Coral Reefs: An Ecosystem in Transition, eds Z. Dubinsky and N. Stambler (Dordrecht: Springer), 493–506.
Froese, R., and Pauly, D. (2016). FishBase. Available online at: www.fishbase.org
Gilby, B. L., Tibbetts, I. R., Olds, A. D., Maxwell, P. S., and Stevens, T. (2016). Seascape context and predators override water quality effects on inshore coral reef fish communities. Coral Reefs 35, 979–990. doi: 10.1007/s00338-016-1449-5
Glaser, M., Plass-Johnson, J. G., Ferse, S. C. A., Neil, M., Satari, D. Y., Teichberg, M., et al. (2018). Breaking resilience for a sustainable future: thoughts for the anthropocene. Front. Mar. Sci. 5:34. doi: 10.3389/fmars.2018.00034
Graham, N. A. J., and Nash, K. L. (2013). The importance of structural complexity in coral reef ecosystems. Coral Reefs 32, 315–326. doi: 10.1007/s00338-012-0984-y
Graham, N. A., Wilson, S. K., Jennings, S., Polunin, N. V., Bijoux, J. P., and Robinson, J. (2006). Dynamic fragility of oceanic coral reef ecosystems. Proc. Natl. Acad. Sci. U.S.A. 103, 8425–8429. doi: 10.1073/pnas.0600693103
Graham, N., Dulvy, N., Jennings, S., and Polunin, N. (2005). Size-spectra as indicators of the effects of fishing on coral reef fish assemblages. Coral Reefs 24, 118–124. doi: 10.1007/s00338-004-0466-y
Gratwicke, B., and Speight, M. R. (2005). The relationship between fish species richness, abundance and habitat complexity in a range of shallow tropical marine habitats. J. Fish Biol. 66, 650–667. doi: 10.1111/j.0022-1112.2005.00629.x
Green, A. L., and Bellwood, D. R. (2009). Monitoring Functional Groups of Herbivorous Reef Fishes as Indicators of Coral Reef Resilience: A Practical Guide for Coral Reef Managers in the Asia Pacific Region. Available online at: http://eprints.jcu.edu.au/10959/ (Accessed June 18, 2012).
Hill, J., and Wilkinson, C. R. (2004). Methods for Ecological Monitoring of Coral Reefs. Townsville, QLD: Australian Instititue of Marine Science.
Kegler, H. F., Lukman, M., Teichberg, M., Plass-Johnson, J., Hassenrück, C., Wild, C., et al. (2017). Bacterial community composition and potential driving factors in different reef habitats of the spermonde archipelago, Indonesia. Front. Microbiol. 8:662. doi: 10.3389/fmicb.2017.00662
Kohler, K., and Gill, S. (2006). Coral point count with excel extensions (CPCe): a visual basic program for the determination of coral and substrate coverage using random point count methodology. Comput. Geosci. 32, 1259–1269. doi: 10.1016/j.cageo.2005.11.009
Kramer, M. J., Bellwood, O., Fulton, C. J., and Bellwood, D. R. (2015). Refining the invertivore: diversity and specialisation in fish predation on coral reef crustaceans. Mar. Biol. 162, 1779–1786. doi: 10.1007/s00227-015-2710-0
Kulbicki, M., Guillemot, N., and Amand, M. (2005). A general approach to length-weight relationships for New Caledonian lagoon fishes. Cybium 29, 235–252.
Kulbicki, M., Parravicini, V., Bellwood, D. R., Arias-Gonzàlez, E., Chabanet, P., Floeter, S. R., et al. (2013). Global biogeography of reef fishes: a hierarchical quantitative delineation of regions. PLoS ONE 8:e81847. doi: 10.1371/journal.pone.0081847
MacNeil, M. A., Graham, N. A., Cinner, J. E., Wilson, S. K., Williams, I. D., Maina, J., et al. (2015). Recovery potential of the world's coral reef fishes. Nature 520, 341–344. doi: 10.1038/nature14358
McClanahan, T., Graham, N., Maina, J., Chabanet, P., Bruggemann, J., and Polunin, N. (2007). Influence of instantaneous variation on estimates of coral reef fish populations and communities. Mar. Ecol. Prog. Ser. 340, 221–234. doi: 10.3354/meps340221
McClanahan, T. R., Graham, N. A., MacNeil, M. A., Muthiga, N. A., Cinner, J. E., Bruggemann, J. H., et al. (2011). Critical thresholds and tangible targets for ecosystem-based management of coral reef fisheries. Proc. Natl. Acad. Sci. U.S.A. 108, 17230–17233. doi: 10.1073/pnas.1106861108
Mouillot, D., Bellwood, D. R., Baraloto, C., Chave, J., Galzin, R., Harmelin-Vivien, M., et al. (2013). Rare species support vulnerable functions in high-diversity ecosystems. PLoS Biol. 11:e1001569. doi: 10.1371/journal.pbio.1001569
Osenberg, C. W., Shima, J. S., and Mary, C. M. S. T. (2004). “Habitat degredation and settlement behavior: effects on fish settlement, survival, and recruitment,” in Proceedings of the 10th International Coral Reef Symposium (Okinawa: Japanese Coral Reef Society, Tokyo), 257–263.
Pandolfi, J. M., Bradbury, R. H., Sala, E., Hughes, T. P., Bjorndal, K. A., Cooke, R. G., et al. (2003). Global trajectories of the long-term decline of coral reef ecosystems. Science 301, 955–958. doi: 10.1126/science.1085706
Pet-Soede, L., and Erdmann, M. (1998a). An overview and comparison of destructive fishing practices in Indonesia. SPC Live Reef Fish. Inf. Bull. 4, 28–36.
Pet-Soede, L., and Erdmann, M. (1998b). Blast Fishing in Southwest Sulawesi, Indonesia. Naga: ICLARM Q. Available online at: http://aquaticcommons.org/9430/ (Accessed February 6, 2014).
Pet-Soede, L., Van Densen, W. L. T., Pet, J. S., and Machiels, M. A. M. (2001). Impact of Indonesian coral reef fisheries on fish community structure and the resultant catch composition. Fish. Res. 51, 35–51. doi: 10.1016/S0165-7836(00)00236-8
Plass-Johnson, J. G., Bednarz, V. N., Hill, J. M., Jompa, J., Ferse, S. C. A., and Teichberg, M. (2018). Contrasting responses in the niches of two coral reef herbivores along a gradient of habitat disturbance in the Spermonde Archipelago, Indonesia. Front. Mar. Sci. 5:32. doi: 10.3389/fmars.2018.00032
Plass-Johnson, J. G., Ferse, S. C., Jompa, J., Wild, C., and Teichberg, M. (2015a). Fish herbivory as key ecological function in a heavily degraded coral reef system. Limnol. Oceanogr. 60, 1382–1391. doi: 10.1002/lno.10105
Plass-Johnson, J. G., Ferse, S. C., Wild, C., and Teichberg, M. (2014). Observation of macroalgal browsing in juvenile humphead parrotfish, Bolbometopon muricatum, in the Spermonde Archipelago, Indonesia. Bull. Mar. Sci. 90, 763–764. doi: 10.5343/bms.2014.1006
Plass-Johnson, J. G., Heiden, J. P., Abu, N., Lukman, M., and Teichberg, M. (2016a). Experimental analysis of the effects of consumer exclusion on recruitment and succession of a coral reef system along a water quality gradient in the Spermonde Archipelago, Indonesia. Coral Reefs 35, 229–243. doi: 10.1007/s00338-015-1369-9
Plass-Johnson, J. G., McQuaid, C. D., and Hill, J. M. (2013). Stable isotope analysis indicates a lack of inter- and intra-specific dietary redundancy among ecologically important coral reef fishes. Coral Reefs 32, 429–440. doi: 10.1007/s00338-012-0988-7
Plass-Johnson, J. G., Schwieder, H., Heiden, J. P., Weiand, L., Wild, C., Jompa, J., et al. (2015b). A recent outbreak of crown-of-thorns starfish (Acanthaster planci) in the Spermonde Archipelago, Indonesia. Reg. Environ. Chang. 15, 1157–1162. doi: 10.1007/s10113-015-0821-2
Plass-Johnson, J. G., Taylor, M. H., Husain, A. A., Teichberg, M. C., and Ferse, S. C. A. (2016b). Non-random variability in functional composition of coral reef fish communities along an environmental gradient. PLoS ONE 11:e0154014. doi: 10.1371/journal.pone.0154014
Polónia, A. R. M., Cleary, D. F. R., de Voogd, N. J., Renema, W., Hoeksema, B. W., Martins, A., et al. (2015). Habitat and water quality variables as predictors of community composition in an Indonesian coral reef: a multi-taxon study in the Spermonde Archipelago. Sci. Total Environ. 537, 139–151. doi: 10.1016/j.scitotenv.2015.07.102
Pratchett, M. S., Hoey, A. S., Wilson, S. K., Messmer, V., and Graham, N. A. J. (2011). Changes in biodiversity and functioning of reef fish assemblages following coral bleaching and coral loss. Diversity 3, 424–452. doi: 10.3390/d3030424
Renema, W., and Troelstra, S. R. (2001). Larger foraminifera distribution on a mesotrophic carbonate shelf in SW Sulawesi (Indonesia). Palaeogeogr. Palaeoclimatol. Palaeoecol. 175, 125–146. doi: 10.1016/S0031-0182(01)00389-3
Risk, M. (1972). Fish diversity on a coral reef in the Virgin Islands. Atoll Res. Bull. 153, 1–6. doi: 10.5479/si.00775630.153.1
Sawall, Y., Jompa, J., Litaay, M., Maddusila, A., and Richter, C. (2013). Coral recruitment and potential recovery of eutrophied and blast fishing impacted reefs in Spermonde Archipelago, Indonesia. Mar. Pollut. Bull. 74, 374–382. doi: 10.1016/j.marpolbul.2013.06.022
Sawall, Y., Teichberg, M. C., Seemann, J., Litaay, M., Jompa, J., and Richter, C. (2011). Nutritional status and metabolism of the coral Stylophora subseriata along a eutrophication gradient in Spermonde Archipelago (Indonesia). Coral Reefs 30, 841–853. doi: 10.1007/s00338-011-0764-0
Smith, J. E., Brainard, R., Carter, A., Grillo, S., Edwards, C., Harris, J., et al. (2016). Re-evaluating the health of coral reef communities: baselines and evidence for human impacts across the central Pacific. Proc. R. Soc. B Biol. Sci. 283, 20151985. doi: 10.1098/rspb.2015.1985
Stuart-Smith, R. D., Bates, A. E., Lefcheck, J. S., Duffy, J. E., Baker, S. C., Thomson, R. J., et al. (2013). Integrating abundance and functional traits reveals new global hotspots of fish diversity. Nature 501, 539–542. doi: 10.1038/nature12529
Teichberg, M., Wild, C., Bednarz, V. N., Kegler, H. F., Lukman, M., Gärdes, A. A., et al. (2018) Spatio-temporal patterns in coral reef communities of the spermonde archipelago, 2012–2014, I: comprehensive reef monitoring of water benthic indicators reflect changes in reef health. Front. Mar. Sci. 5:33. doi: 10.3389/fmars.2018.00033
Trenkel, V. M., and Rochet, M. (2003). Performance of indicators derived from abundance estimates for detecting the impact of fishing on a fish community. Can. J. Fish. Aquat. Sci. 60, 67–85. doi: 10.1139/f02-163
van der Ent, E., Hoeksema, B. W., and de Voogd, N. J. (2016). Abundance and genetic variation of the coral-killing cyanobacteriosponge Terpios hoshinota in the Spermonde Archipelago, SW Sulawesi, Indonesia. J. Mar. Biol. Assoc. 96, 453–463. doi: 10.1017/S002531541500034X
Veron, J. E. N., Devantier, L. M., Turak, E., Green, A. L., Kininmonth, S., Stafford-Smith, M., et al. (2009). Delineating the Coral Triangle. Galaxea, J. Coral Reef Stud. 11, 91–100. doi: 10.3755/galaxea.11.91
Vogler, C., Benzie, J., Lessios, H., Barber, P., and Wörheide, G. (2008). A threat to coral reefs multiplied? Four species of crown-of-thorns starfish. Biol. Lett. 4, 696–699. doi: 10.1098/rsbl.2008.0454
Wang, Y., Naumann, U., Wright, S. T., and Warton, D. I. (2012). Mvabund- an R package for model-based analysis of multivariate abundance data. Methods Ecol. Evol. 3, 471–474. doi: 10.1111/j.2041-210X.2012.00190.x
Wilson, S., Graham, N., Pratchett, M., Jones, G., and Polunin, N. (2006). Multiple disturbances and the global degradation of coral reefs: are reef fishes at risk or resilient? Glob. Chang. Biol. 12, 2220–2234. doi: 10.1111/j.1365-2486.2006.01252.x
Wilson, S. K., Fisher, R., Pratchett, M. S., Graham, N. A., Dulvy, N. K., Turner, R. A., et al. (2010). Habitat degradation and fishing effects on the size structure of coral reef fish communities. Ecol. Appl. 20, 442–451. doi: 10.1890/08-2205.1
Wismer, S., Hoey, A. S., and Bellwood, D. R. (2009). Cross-shelf benthic community structure on the Great Barrier Reef: relationships between macroalgal cover and herbivore biomass. Mar. Ecol. Prog. Ser. 376, 45–54. doi: 10.3354/meps07790
Keywords: Acanthaster spp., environmental gradient, Coral Triangle, Indonesia, coral reefs, fish-habitat association, community composition
Citation: Plass-Johnson JG, Teichberg M, Bednarz VN, Gärdes A, Heiden JP, Lukman M, Miñarro S, Kegler H, Weiand L, Wild C, Reuter H and Ferse SCA (2018) Spatio-Temporal Patterns in the Coral Reef Communities of the Spermonde Archipelago, 2012–2014, II: Fish Assemblages Display Structured Variation Related to Benthic Condition. Front. Mar. Sci. 5:36. doi: 10.3389/fmars.2018.00036
Received: 13 February 2017; Accepted: 24 January 2018;
Published: 16 February 2018.
Edited by:
Angel Borja, Centro Tecnológico Experto en Innovación Marina y Alimentaria (AZTI), SpainReviewed by:
José Lino Vieira De Oliveira Costa, Universidade de Lisboa, PortugalMaria C. Uyarra, Centro Tecnológico Experto en Innovación Marina y Alimentaria (AZTI), Spain
Copyright © 2018 Plass-Johnson, Teichberg, Bednarz, Gärdes, Heiden, Lukman, Miñarro, Kegler, Weiand, Wild, Reuter and Ferse. This is an open-access article distributed under the terms of the Creative Commons Attribution License (CC BY). The use, distribution or reproduction in other forums is permitted, provided the original author(s) and the copyright owner are credited and that the original publication in this journal is cited, in accordance with accepted academic practice. No use, distribution or reproduction is permitted which does not comply with these terms.
*Correspondence: Jeremiah G. Plass-Johnson, jplass@uni-bremen.de
†Present Address: Jeremiah G. Plass-Johnson, Centre for Ocean Life, National Institute of Aquatic Resources (DTU-Aqua), Technical University of Denmark, Lyngby, Denmark;
Vanessa N. Bednarz, Marine Department, Centre Scientifique de Monaco, Principality of Monaco, Monaco, Monaco;
Jasmin P. Heiden, Section Biogeoscience, Department EcoTrace, Alfred Wegener Institute for Polar and Marine Research, Bremerhaven, Germany;
Sara Miñarro, Institute of Environmental Science and Technology (ICTA), Universitat Autònoma de Barcelona, Bellaterra, Spain