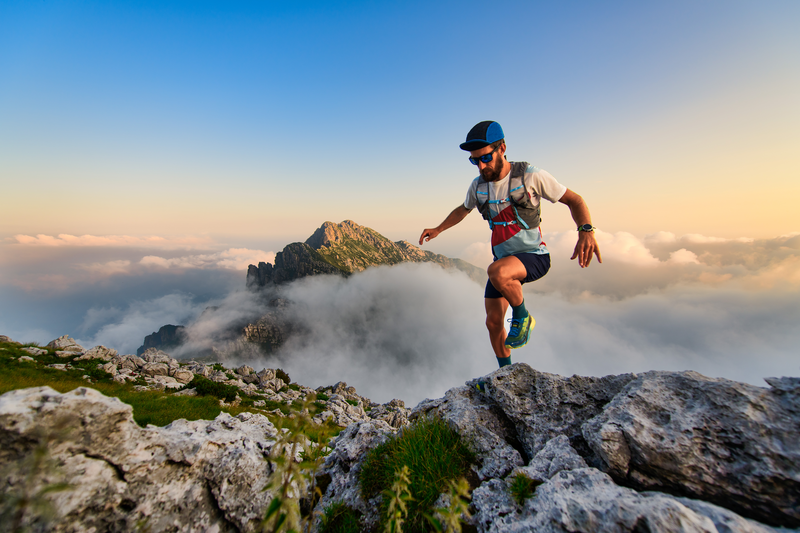
94% of researchers rate our articles as excellent or good
Learn more about the work of our research integrity team to safeguard the quality of each article we publish.
Find out more
ORIGINAL RESEARCH article
Front. Mar. Sci. , 07 February 2018
Sec. Marine Evolutionary Biology, Biogeography and Species Diversity
Volume 5 - 2018 | https://doi.org/10.3389/fmars.2018.00029
Mesophotic reef-building coral communities (~30–120 m depth) remain largely unexplored, despite representing roughly three-quarters of the overall depth range at which tropical coral reef ecosystems occur. Although many coral species are restricted to shallow depths, several species occur across large depth ranges, including lower mesophotic depths. Yet, it remains unclear how such species can persist under extreme low-light conditions and how the different symbiotic partners associated with these corals contribute to facilitate such broad depth ranges. We assessed holobiont genetic diversity of the Caribbean coral Agaricia undata over depth in three localities of Colombia: San Andres Island (between 37 and 85 m), Cartagena (between 17 and 45 m) and “Parque Nacional Natural Corales de Profundidad” (between 77 and 87 m). We used a population genomics approach (NextRAD) for the coral host, and amplicon sequencing for the associated Symbiodinium (non-coding region of the plastid psbA minicircle) and prokaryotic (V4 region of the 16S rRNA gene) symbiont community. For the coral host, genetic structuring was only observed across geographic regions, but not between depths. Bayesian clustering and discriminant analysis of principal components revealed genetic structuring between the three regions, but not between shallow (<30 m), upper (≥30 and ≤60 m) and lower mesophotic (>60 m) depths. This pattern was confirmed when evaluating pairwise differentiation (FST) between populations, with much higher values between regions (0.0467–0.1034) compared to between depths [within location; −0.0075–(−0.0007)]. Symbiotic partners, including seven types of zooxanthellae and 325 prokaryotic OTUs, did not exhibit partitioning across depths. All samples hosted Symbiodinium clade C3 and the type C3psbA_e was present in all depths. Alpha microbial diversity was not significantly different between zones (upper vs. lower), which community composition between coral colonies was similar in the two zones (ANOSIM, R = −0.079, P > 0.05). The coral microbiome was dominated by Uncultured Betaproteobacteria in the order EC94 (16%), Unknown-Bacteria (15%), family Cenarchaeaceae (12 %), Burkholderiaceae (10%), and Hahellaceae (10%). The constant coral-holobiont composition along the studied depth range suggests that identity of the symbionts is not responsible for the coral's broad depth range and adaptation to low light environments.
Symbiotic relationships in aquatic environments are widespread and play important roles for the health, adaptation, and evolution of the host organisms (Grossart et al., 2013). The most recognized example of symbioses in marine ecosystems is the association between the coral polyps and photosynthetic algae belonging to the genus Symbiodinium (Stat et al., 2008). The importance of the coral-Symbiodinium symbiosis is exceptional, since the success of corals (the foundation species of the most biodiverse marine ecosystem) in oligotrophic tropical waters largely contribute to this symbiosis. The 95% of the host's energy requirements is supplied by Symbiodinium (Jones, 2013; Smith et al., 2017). Furthermore, corals also associate with prokaryotes (Bacteria and Archaea), a symbiosis that can provide important resources to the host through biogeochemical cycling of carbon, nitrogen, and sulfur (Wild et al., 2004; Raina et al., 2013; Radecker et al., 2015). Prokaryotes can also contribute to the defense mechanisms of the coral through the provision of antibiotic compounds (Rohwer et al., 2002). The species composition of these microbiota can vary among colonies of the same host species, a fact that has been related to environmental differences (Klaus et al., 2007; Kahng et al., 2014; Ziegler et al., 2017), health status (Kimes et al., 2010) and biological traits (Bongaerts et al., 2013; Glasl et al., 2017).
Coral reef ecosystems comprise of diverse communities of scleractinian corals and can occur in excess of 100 meters depth (Goreau and Wells, 1967). However, for practical reasons most of scientific research has been restricted to the shallow reefs (<30 m), representing only a small fraction of the whole depth distribution (Bridge et al., 2012). Over the past decade, deeper reef zones (>30 m), or mesophotic coral ecosystems (MCEs), have drawn increased attention encouraged by technological developments and coral reefs conservation amidst worldwide concern on their fate (Kahng et al., 2014). In particular, closed-circuit rebreathers have opened up the opportunity for manned, scientific exploration of this so-called “twilight zone” (Pyle, 1998), an intermediate zone between shallow waters and deep-sea (Olson and Kellogg, 2010). This ecosystem represents a good subject to study changes the coral-symbiont species composition along a depth range given that MCEs can be divided in the “upper mesophotic zone” (30–60 m depth), which represents a transitory region from shallow reefs, and the “lower mesophotic zone” (≥60 m) where more distinct assemblages can be found (Bongaerts et al., 2010).
Coral species that occur over large depth ranges are known as “depth-generalists” (Kahng et al., 2010), and can be facilitated by adaptive traits to optimize light capture across the associated irradiance gradient over depth. Examples of such traits are changes in colonial morphology (e.g., from dome to flat shapes), pigment composition (e.g., more light-harvesting pigment and less photoprotective pigment concentration in low light) and Symbiodinium type (e.g., cladal or subcladal level type) (Bongaerts et al., 2015a; Ziegler et al., 2015). “Depth-generalists” species, like Stephanocoenia intersepta and Madracis pharensis, have also a higher flexibility in their prokaryotic community compared to “depth-specialist” species (exclusive of mesophotic reefs) (Glasl et al., 2017). In contrast, depth-specialist species like Agaricia grahamae and Madracis formosa have been associated to specialist photosynthetic endosymbionts (Bongaerts et al., 2015b) and have been reported to have a unvarying prokaryotic community (Glasl et al., 2017).
Holobiont studies on mesophotic reefs are sparse. Host connectivity between shallow and mesophotic reefs has been the main focus in the last years as well as host- Symbiodinium relationships (Bongaerts et al., 2015b, 2017; Meirelles et al., 2015; Silva-Lima et al., 2015; Turner et al., 2017). Studies in Bacteria and Archaea have gained interest due to their contribution to the acclimatization and adaptation to environmental change, where this association can complement the metabolic needs of the coral host (Olson and Kellogg, 2010; Ainsworth et al., 2015). For some coral, a shift in Symbiodinium type is important for enabling a wide depth distribution (Frade et al., 2008; Lesser et al., 2010). But more important, photobiological flexibility of both host and symbionts can be a key for persistence in contrasting light regimes (Cooper et al., 2011). Recent reviews of mesophotic reefs document a clear geographic bias, with studies concentrated in the Atlantic region and Indo-Pacific (Kahng et al., 2014; Turner et al., 2017). Studies are needed fulfilling gaps in geographical regions as well as holobiont approaches contributing to understand host-symbiont relationships (Turner et al., 2017).
In the Southern Caribbean, Agaricia undata (Ellis and Solander, 1786) is a dominant coral species between 60 and 100 m depth (Bongaerts et al., 2010, 2015b), and provides an important habitat for a diverse array of organisms, such as fish (Hoeksema et al., 2017) and crabs (van Tienderen and van der Meij, 2016). Similarly, on the oceanic reefs of Colombia, this species is abundant at lower mesophotic depths (≥60 m). However, near the coastline of mainland Colombia, where the water turbidity is high, it can be found as shallow as ~20 m and it is common at 35–45 m. Here, we explore the diversity and association patterns between host genetics, symbiont (Symbiodinium and prokaryotic community) composition and depth/geographic (i.e., coastal vs. oceanic reefs of Colombia's Caribbean). We studied three localities in Colombia: one oceanic reef (San Andres Island-SAI, 37–85 m depth) and two reefs near the continental shelf of Colombian Caribbean (Cartagena-CTG, 17–45 m; and Parque Nacional Natural Corales de profundidad-PNN, 77–87 m). We evaluated host genetic differentiation across populations using genome-wide single nucleotide polymorphisms (SNPs) and assessed symbiotic communities using targeted amplicon sequencing of the psbAncr for Symbiodinium and 16S ribosomal RNA gene for prokaryotes.
To understand coral variation across depths, we sampled colonies at three major reef formations in Colombia: San Andrés Island, Cartagena Bay and “Parque Nacional Natural Corales de Profundidad.” San Andrés Island is an Oligocene-Miocene atoll located at 700 km off the continental coast of Colombia (Diaz and García-llano, 2010). The coral reef system in Cartagena is one of the most complex siliciclastic coral reefs (Sánchez, 1999). Our collection sites were in the southwestern part of Cartagena, which is an area characterized by high sediment loads from the Dique's Canal at the mouth of the Magdalena river (Diaz et al., 2000; Cáceres and Sánchez Muñoz, 2015). This reef formation is characterized by a narrow terrace cut by a steep slope that exhibits an exuberant coral growth that goes beyond 40 m in depth. Our third sampling location is in the “Parque Nacional Natural Corales de Profundidad.” A National Park located 32 Km off the coast of Barú, Cartagena; and it is one of the marine protected areas of Colombia that exclusively is monitoring deep-sea ecosystems. This park includes the remains of an old barrier reef system drifting at the edge of the continental shelf, today with seldom-coral assemblages and almost 100% rodolith cover (MADS, 2013). In Cartagena, water discharge and sedimentation load from the Magdalena River has an impact in the costal ecosystems in the area (Restrepo et al., 2006). For example, the light penetration in this locality is lower than in San Andres or PNN Corales de Profundidad. Therefore, the light penetration at 40 m in Cartagena could be the same as the one at 70–80 m in San Andres.
In total, 103 colonies of A. undata were sampled between 17 and 87 m using SCUBA or mixed-gas closed-circuit rebreather (CCR) diving in 2015 and 2016 (Figure 1 and Table 1). San Andrés island was sampled in April and June 2015 and March 2016, Cartagena in July and November 2015 and PNN Corales de Profundidad in December 2015. Since, we only had 20–30 min of bottom time with Trimix (10/60, oxygen/helium) for about 3 h of total diving (including decompression stops) the sampling numbers although low, in comparison with shallow-water sampling, included the entire depth-range of the species at each locality. In addition, this species can have high coral cover locally but, to avoid resampling clones, we restricted sampling to colonies at least 5 m apart. Four samples from another phylogenetic study focusing on the genus Agaricia from Curaçao were included as an outgroup (Bongaerts et al., 2015b). A dry voucher for each colony is available at the Museo de Historia Natural ANDES (ANDES-IM 4681–4784). Research and collection of specimens were approved by the National Environmental Licensing Authority (ANLA, Spanish acronym): Collection Framework Agreement granted to Universidad de los Andes through resolution 1177 of October 9, 2014 - IBD 0359.
Figure 1. Map of the sampled locations in Colombian Caribbean. (1) Map of San Andrés Island showing the three localities where colonies were sampled. (2) Caribbean coast of Colombia showing the two localities (A) Parque Nacional Natural Corales de Profundidad (B) Cartagena Bay.
Table 1. Agaricia undata samples collected from different locations in the Caribbean region of Colombia.
The coral host was genotyped for 48 colonies (not including failed samples) using the nextRAD genotyping-by-sequencing method (Nextera- fragmented, reductively amplified DNA; SNPsaurus LLC). Genomic DNA was extracted using a salt-extraction protocol, including several centrifugation steps to reduce endosymbiont contamination (following conditions outlined in Bongaerts et al., 2017). Quality filtering, sequence clustering and SNP calling were carried out using the PyRAD pipeline (Eaton, 2014), and custom scripts as outlined in Bongaerts et al. (2017). The samples were grouped in four different categories: Cartagena-Shallow (CTGS; between 17and 30 m), Cartagena-Upper (CTGU; between 35 and 45 m), San Andres-Upper (SAIU; between 37 and 60 m), San Andres-Lower (SAIL; between 65 and 85 m) and Curaçao (CUR; 60 and 80 m), reflecting shallow (≤30 m), upper mesophotic (30–60 m) and lower mesophotic depths (>60 m).
Candidate loci under selection were evaluated using both LOSITAN (Beaumont and Nichols, 1996; Antao et al., 2008) and BayeScan 2.1 (Foll and Gaggiotti, 2008), with only those identified by both retained as true outliers. LOSITAN was run for 50,000 iterations, a 0.95% CI and FDR of 0.2. “Neutral” mean Fst and “Force mean Fst” were used to increase the reliability of the analysis. In BayeScan outliers were identified using default settings and a Bayes-factor cut-off of 0.01. Putative “neutral” (i.e., non-outlier) SNPs were defined as the subset of the dataset after eliminating outlier SNPs from both programs. Observed (Ho) and expected (He) heterozygosity, and the inbreeding coefficient (Fis) for each population were calculated using the “hierfstat” R package (Goudet, 2005). Pairwise FST values were calculated to determine the level of differentiation among population. Additionally, discriminant analysis of principal components (DAPC) and principal component analysis (PCA) using the “adegenet” R package (Jombart and Ahmed, 2011) were performed. Genetic clustering was assessed using a multi-threading wrapper (“structure_mp”; Bongaerts et al., 2017) for STRUCTURE (Pritchard et al., 2000), which assesses distinct subsamples of the dataset (each with a single SNP per nextRAD locus). Number of clusters were estimated based on MedMeaK, MaxMeaK, Med-MedK, and MaxMedK (threshold of 0.5; Puechmaille, 2016). All analyses were carried for datasets with all and “neutral” loci. Raw Illumina sequence data of A. undata are available at NCBI SRA database under accession number PRJNA385083 http://www.ncbi.nlm.nih.gov/bioproject/385083.
To extract overall holobiont DNA (including Symbiodinium and prokaryotes), we used the CTAB DNA extraction protocol for the whole 0.5 × 0.5 cm2 coral subsample (Coffroth et al., 1992). Symbiodinum clades were identified with the non-coding region of the plastid psbA minicircle (Lajeunesse and Thornhill, 2011). For the PCR amplification of the psbAncr we used the following primer: 7.4-For 5′GCATGAAAGAAATGCACACAACTTCCC-3′ and 7.8-Rev 5′-GGTTCTCTTATTCCATCAATATCTACTG-3′ (Lajeunesse and Thornhill, 2011). PCR was performed in a 15 μl reaction containing 1x PCR buffer, 1.5 mM MgCl2, 1 μl of 10x bovine serum albumin (BSA 20 mg/ul), 0.16 mM of each dNTP, 0.52 mM of each primer, 1 U Taq DNA polymerase and 1 μl of DNA (30 ng). PCR conditions were 94°C for 2 min followed by 35 cycles of 94°C for 90 s, 52°C for 90 s and 72°C for 60 s, and a 5 min extension at 72°C. Resulting PCR products were cleaned with FastAP Thermosensitive Alkaline Phosphatase (Thermo Scientific™) and sequenced using the BigDye Terminator v3.1 Cycle Sequencing Kit (Applied Biosystems) and run on an AB13730xl DNA Analyzer (Applied Biosystems). PCR products were sequenced in one direction with the primer 7.8-Rev.
To understand relationships among taxa across depth gradients, we reconstructed phylogenetic relationships using Bayesian inference with BEAST v1.8.2 (Drummond et al., 2012) and MrBayes v3.2.2 (Ronquist et al., 2012). Maximum Likelihood trees were estimated using RaxML (Stamatakis, 2006). Both methods resulted in the same tree topology, thus only the Bayesian tree is reported here. Evolutionary models were assessed using Jmodeltest 2.1.2 (Guindon and Gascuel, 2003; Darriba et al., 2012), which resulted in the HKY+ gamma according to the BIC calculations. FigTree 1.3.1 (Rambaut and Drummond, 2010) was used for tree visualization and edition. In order to identify phylospecies or clades, we applied the generalized mixed Yule-coalescent model in our Bayesian ultrametric trees using the R package bGMYC (Reid and Carstens, 2012). Additionally, we calculated the pairwise genetic distance in the datasets using MEGA6 (Tamura et al., 2013) and the coalescent Poisson Tree Process (PTP) method (Zhang et al., 2013). bGMYC analysis was based on 100 trees from the post burn-in generations obtained from the BEAST analysis. For each of the 100 trees, the Markov-chain Monte Carlo sampler was run for 50,000 generations, discarding the first 40,000 generations as burn-in and sampling every 100 generations. The upper threshold, defined as number of tips on the tree, was set to 39; and a cut-off value was set as 0.5 to delimit lineages. The coalescent Poisson Tree Process (PTP) method (Zhang et al., 2013) we used the web interface http://species.h-its.org with Bayesian tree as input, 100,000 MCMC generations, thinning every 100 generation and a burning fraction of 0.1. Since no identical sequences to A. undata Symbiodinium types were found in GenBank, sequences were named as follow: Symbiodinium clade, followed by psbA (gene used) and a randomly letter to distinguish the different types of dinoflagellates. GenBank Accession Numbers KY886412- KY886450.
To reveal prokaryote diversity, we amplified the hypervariable V4 region of the 16S rRNA gene using the 515F/806R PCR primers as originally described for the Earth Microbiome Project (EMP) (http://www.earthmicrobiome.org/emp-standard-protocols/16s/). We scored prokaryote diversity in a subset of 12 samples (not including failed samples, i.e., clones or not included in analysis due to low read coverage) of A. undata from San Andrés Island (37–80 m) the locality with the widest depth range. Barcoded amplicons were pooled in equimolar quantities and sequenced on an Illumina MiSeq platform with 250 pb paired-end read libraries. After sequencing, reads were demultiplexed, depleted of barcodes/primers, and paired-ends were merged using QIIME (Caporaso et al., 2010b) and Usearch v8.1 (Edgar, 2010). Sequences resulting in less than 248 base pairs and nucleotide errors above 0.5 were removed. Operational taxonomic units (OTUs) were defined at 97% similarity using UPARSE pipeline (Edgar, 2013), which also de-noised the sequences removing known singletons. In addition, UCHIME (Edgar et al., 2011) was used to check for chimeras using May 2016 Gold database (Reddy et al., 2015). Subsequently, OTUs were aligned using PyNAST (Caporaso et al., 2010a) and taxonomically assigned by RDP classifier against Greengenes database (DeSantis et al., 2006; Cole et al., 2014). After quality filtering, we retained 364,598 reads from a total of 756,201 (52% discarded). Coverage was uneven across samples and we used a random subsampling of 5,000 reads to make statistical comparisons.
The prokaryotic community was defined by the presence of any given phylotype in at least 3 samples. Diversity indices (Shannon-Weaver Index, Inverse Simpson index, CHAO-1) were estimated and comparisons among sites and depths made through a Mann-Whitney test. Prokaryote community composition was visualized using non-metric multidimensional scaling (NMDS) ordination based on Bray-Curtis similarity matrices. The Analysis of similarity was applied to test if the variation within zones was less than between (upper: 30–≤60 m vs. lower: 60–80 m) (ANOSIM; 1,000 permutations). OTUs and family level contributions between depths were estimated by similarity percentages (SIMPER). All statistical tests and graphs were performed in PAST (Hammer et al., 2001). All analyses were made at family level and OUT 97% and no differences were found. Family level is shown for statistical test (ANOSIM and SIMPER) and at OTU 97% for diversity indices. When we were unable to assign an OTU to a family level we kept the lowest classification possible either Order, Class or Kingdom. Demultiplexed 16S rRNA gene raw reads are available in the NCBI SRA database under accession number PRJNA382610 http://www.ncbi.nlm.nih.gov/bioproject/382610.
Targeted sequenced of nextRAD loci resulted in an average of 4.5 million (range: ~1 M−12 M) 150 bp Illumina reads for each of the individuals (n = 48) with successfully sequenced libraries. After filtering for read and base quality, overall representation (min. 80% of individuals genotyped), and non-coral contamination (sensu, Bongaerts et al., 2017), a total of 6,933 bi-allelic SNPs were retained. Evaluation of pairwise genetic distances between all individuals, revealed eight sets of clones (with 94.5–99% match) within the population. Expected heterozygosity (He) varied across all five populations and ranged between 0.1690 and 0.2004 (across all loci) and 0.1589 and 0.1990 (putative “neutral” loci) with all populations showing comparable levels of genetic diversity (Table 2). Bayesian clustering and discriminant analysis of principal components revealed genetic structuring between the three regions (i.e., Cartagena, San Andrés, and Curaçao), but not between shallow (<30 m), upper (≥30 and ≤60 m) and lower mesophotic (>60 m) depths (Figure 2). This pattern was confirmed when evaluating pairwise differentiation (FST; fixation index) between populations, with much higher values between regions (0.0467–0.1034) compared to between depths [within location; −0.0075–(−0.0007)] (Table 3). Sixteen SNPs were identified as under selection by both LOSITAN (604 outliers) and BayeScan (16), 15 SNPs showing distinct (near-) fixed allele frequencies between regions: one for San Andrés, eleven for Cartagena and three for Curaçao. Pairwise differentiation (FST) between shallow and upper mesophotic depths was lower in Cartagena (CTGS-CTGU: −0.0075) than upper and lower mesophotic depths in San Andrés (SAIU-SAIL: −0.0007).
Table 2. observed heterozygosity (Ho), expected heterozygosity (He) and inbreeding coefficient (Fis) (±standard deviations) for all-inclusive and neutral loci.
Figure 2. Genetic structure across locations and depths for the coral host Agaricia undata. (A) STRUCTURE diagrams (K = 3) for A. undata inferred from the all-inclusive loci (top) and “neutral” (bottom) data sets. (B) Principal component analysis (PCA) inferred from the overall data set, where individual samples are represented by dots and color code corresponding to the locations: CTGS (Cartagena shallow), dark green; CTGU (Cartagena upper), light green; SAIU (San Andrés upper), yellow; SAIL (San Andrés lower), red; and CUR (Curaçao), dark blue.
Amplicon sequencing of the psbA non-coding region of Symbiodinium algae resulted in a total of 470 pb, which clustered into 7–9 types depending on the species delimitation method (Figure 3A). Seven Symbiodinium types were captured in both methods and deemed as species (sensu Lajeunesse and Thornhill, 2011). All samples hosted clade C3 as previous report for other Agaricia species (Lajeunesse, 2002). Within each locality no depth zonation was observed in A. undata colonies. C3psbA_c was the only type of Symbiodinium that was found in all localities. In San Andres Island (n = 46) the most common Symbiodinium type was C3psbA_a across all depths, the type C3psbA_C was also found in both zones and only one colony in the lower zone have the type C3psbA_b. In Cartagena (n = 31), the most common type was C3psbA_e present in all depths, followed by C3psbA_f present also in the two zones and two colonies in the shallow zone with the types C3psba_C and C3psbA_g. In PNN “Corales de profundidad” (n = 2) the only two colonies we found have types C3psbA_C and C3psbA_d (Figure 3B, Supplementary Table 1).
Figure 3. Genetic diversity and distribution of Symbiodinium OTUs in Agaricia undata across depths and locations. (A) Bayesian MCMC phylogenetic tree of Symbiodinium zooxanthellae OTUs based on non-coding region of the plastid minicircle psbAncr. Colored bars to the right of the phylogeny represent OTUs grouping based on genetic distance thresholds (3%), bGMYC and bPTP with their corresponding nomenclature. Bootstrap values are based on Bayesian analyses, with only probabilities over 60% shown (B) Pie charts summarizing diversity and distribution of zooxanthellae OTUs across depth range per locality, which colors correspond to the clades defined in (A).
Amplicon sequencing of the V4 region of the 16S rRNA gene targeting prokaryotes resulted in a total of 364,598 paired-end Illumina reads (250 bp). The prokaryote community richness of A. undata samples were 325 OTUs (per sample ranged from 28 to 217 OTUs). Alpha diversity was not significantly different between zones (upper vs. lower) (t-test p > 0.05; Table 4; Supplementary Table 2). Additionally, the prokaryote community composition between coral colonies was similar in the two zones [Figure 4, ANOSIM, R = −0.079, P > 0.05 (family level) and ANOSIM, R = −0.2114, P > 0.05 (OTU 97% level)]. The coral microbiome was dominated by Uncultured Betaproteobacteria in the order EC94 (16%), Unknown-Bacteria (15%), family Cenarchaeaceae (12%), Burkholderiaceae (10%), and Hahellaceae (10%). Other families that were also abundant included Vibrionaceae (4%), Oxalobacteraceae (3%), and Rhodobacteraceae (3%). The families Burkholderiaceae and Alcanivoracaceae were only found in the upper zone while the family Spirochaetaceae, unknown families of the class PAUC37f and Gemm-4 was exclusive of the lower mesophotic zone. Similarity percentage analysis (SIMPER) revealed that main families responsible for the differentiation between NMDS groups (Figure 4B) were EC94, unknown-bacteria, Hahellaceae, Cenarchaeaceae, Vibrionaceae, and Oxalobacteraceae (Figure 4A; Table 5). Furthermore, three groups of colonies of A. undata, and two outlier samples, showed differences at family level (Figure 4B; ANOSIM, R = 0.96, P < 0.05).
Figure 4. Bacterial community composition associated to Agaricia undata from San Andres island. (A) Heatmap of the relative abundance of 16S rRNA gene amplicon sequences taxonomically classified to family level or lower classification ordered in relation with their percentage of contribution to dissimilarities between groups according to SIMPER analysis. The dendrogram is based on Bray-Curtis dissimilarities. (B) nMDS ordinations based on Bray-Curtis relative abundance dissimilarities of prokaryotic families shows a partitioning microbiome by groups, but not depth. Higher taxonomic ranks, e.g., k, kingdom; c, class; o, order; were used when we were unable to assign to family level (f), e.g., unassigned Bacteria.
Despite the large bathymetric range of the coral A. undata, from shallow depths (17 m) to the mesophotic zone (87 m), which extends well beyond the photosynthetic compensatory point (1% light level, ~77 m; Lesser et al., 2009), we did not observe genetic differentiation within the sampled depth ranges (17–45 m and 37–87 m) at each of the locations in the coral host, or the associated Symbiodinium and prokaryote communities. The ecological success of this species across these depth ranges does therefore not seem to be the result of genetic adaptation of the coral host, or due to the hosting of distinct specialized symbiont communities over depth. Further research should explore whether this is due to broad acclimatory potential (i.e., physiological plasticity) of this species, or whether differences in low-light environments (i.e., turbid shallower depths or oceanic lower mesophotic depths) are too minor to warrant further adaptive or acclimatory specialization.
Our genome-wide SNPs analysis indicated that A. undata populations lack a clear pattern of genetic differentiation across the studied depth ranges of 17–45 m (Cartagena Bay) and 37–85 m (San Andres Island). This contrasts to another assessment of vertical connectivity in Bermuda, where strong genetic differentiation was observed between shallow and upper mesophotic depths (12 vs. 40 m) for the related brooding coral species Agaricia fragilis (Bongaerts et al., 2017). Although other species for which vertical connectivity has been assessed in Bermuda, Montastraea cavernosa (4–58 m depth) and Stephanocoenia intersepta (12–40 m depth) (Serrano et al., 2014; Bongaerts et al., 2017), both broadcasting species, lacked genetic differentiation over depth. We observed genome-wide divergence between regions, which confirms expectations of geographic differentiation due to the distance of ~700 km between the oceanic island and the coast of Colombia. A small number of outlier loci were observed to be highly divergent between regions, indicating that local selection may have further contributed to the observed genome-wide divergence among localities (perhaps related to inshore vs. offshore environments).
Although A. undata associated with different Symbiodinium at the different geographic locations, no partitioning over depth was observed (Figure 2B). Phylogeographic patterns have been found in other studies, including a correspondence between population genetic structure of Symbiodinium and their hosts (Howells et al., 2009; Thornhill et al., 2009, 2014; Andras et al., 2013). Since Atlantic brooding corals including agaricids have very stable symbioses with Symbiodinium (Thornhill et al., 2006), these differences in zooxanthellae communities may respond to locally adapted genotypes of Symbiodinium rather than to limited connectivity among populations. The fact that one type was found in all locations suggests high dispersal potential of Symbiodinium in A. undata across the Colombian Caribbean or ancestral populations. Differences between San Andrés and Cartagena could be explained by environmental differences, with high sediment loads from rivers in Cartagena (Grajales and Sanchez, 2016) decreasing light and temperature levels at this location with contrasting oceanic conditions at San Andrés.
The differences in the Symbiodinium associated below 30 m to several Agaricia species, including A. undata, have been observed previously (Bongaerts et al., 2013, 2015a,b, 2017; Lucas et al., 2016). The relatively small depth range assessed for Cartagena (17–45 m) could explain why no differences were found in our study in that locality. Although, in other studies with a smaller range, other authors found depth differences, e.g., Agaricia fragilis (12–40 m), Galaxea astreata (10–55 m), Orbicella faveolata (5–25 m), O. franksi (10–25 m) (Bongaerts et al., 2011, 2015a, 2017). It has been proposed that the broad bathymetric range of Symbiodinium types may be explained by their ability to photoacclimate or be physiologically distinct (e.g., populations of Symbiodinium type C1 adapted to distinct thermal environments) (Howells et al., 2011). Also, Symbiodinium has the capacity to change the abundance of photosynthetic pigments and symbiont cell densities, which can increase over depth (Fricke et al., 1987). Nonetheless, future studies are needed to examine if the Symbiodinium types we found in A. undata have a greater potential for photosynthesis in lower light levels or if the Symbiodinium types have the capacity to acclimatize, e.g., Symbidinium cell densities or photoprotective to light-harvesting pigments ratio, as it has been reported by Ziegler et al. (2015) in MCEs. Colonies of A. undata hosted only a single Symbiodinium type C3 (based on the absence of peak for dubious bases, or multiple peaks in a single position). In the Brazilian endemic corals Mussismilia spp. three different strains were detected (A4, B19, and C3), where only one strain (B19) was found in mesophotic samples and multiple strains were found in the same M. braziliensis coral colony (Silva-Lima et al., 2015).
With respect to symbiotic phototrophs related to A. undata, it is important to mention that a portion of the samples (58%) had a close association with the endolithic algae Ostreobium (Gonzalez et al., submitted). It is of further interest to test if the contribution from this green alga could add in the acclimation and photophysiology of Symbiodium as it has been observed in shallower corals (Fine and Loya, 2002).
Microbial community composition was also unvarying in Bacteria and Archaea across depth zones in A. undata. We divided the samples in two groups: “upper mesophotic” and “lower mesophotic” according to the depth at which colonies were collected. Although we did not observe differences between these depths, there are differences at family level between three groups of colonies of A. undata and two outlier samples (Figure 4B). These groups did not show differences between depth, temperature (all groups have samples from upper and lower), reef sampling nor collection date. We hypothesized that differences in prokaryotic community in these groups could be explained by the vertical transmission of symbionts as it has been observed in other brooder corals such as Stylophora pistillata (Neave et al., 2017) and Mussismilia hispida (Leite et al., 2017). Our results where similar to previous studies in a “depth-specialists” species (Agaricia grahamae) were a unvarying prokaryotic community was associated with this coral with a narrow depth distribution (55–85 m) (Glasl et al., 2017).
There were 325 OTUs present in A. undata microbiome, though just some were important in contributing to dissimilarity among groups (Table 5). A single β-proteobacteria OTU from the order EC94 contributed to 21% to average measure of dissimilarity among groups of different depths. These OTUs with unknown function have been found to dominate in shallow and deep sea sponges (Jackson et al., 2013; Steinert et al., 2016). An unknown group of OTUs (4) classified as Bacteria that could not be further identified contributed to 16% to average measure of dissimilarity. The family Hahellaceae had 11% of the contribution. This family involves different functional characteristics such as nitrate reduction, chemotactic activity and production of antimicrobial compounds (Lawler et al., 2016) and includes common coral-associate taxa such as Endozoicomonas (Bayer et al., 2013; Neave et al., 2016, 2017). OTUs belonging to the genera Cenarchaeum and Nitrosopumilus, as well as other two unidentified genera of the family Cenarchaeaceae, were also present in A. undata samples (91% in one of the outlier samples of 70 m). These genera are involved in the metabolic cycle that transform ammonia to nitrite that can be transferable to Symbiodinium (Lins-de-Barros et al., 2010). Burkholderia were only present in colonies collected less than 60 m and prevalent in one of the samples (88%). Species of this genus can fixed nitrogen and degrading aromatic compounds and can be part of the coral core microbiome or be associated with a fungus on the coral (Bayer et al., 2013; Ainsworth et al., 2015; Leite et al., 2017).
In conclusion, this study revealed a lack of coral host differentiation between depths, with a remarkably consistent associated symbiont community (Symbiodinium and prokaryotes). The mechanism by which this species adjusts to distinct environments is still unknown, and further physiological assessments are required to understand this species' acclimatory potential across its distribution range.
FG-Z and JS: conceived the study; FG-Z and JS: collected the samples; FG-Z, CR-P, BA-O, and GW: conducted the laboratory work; FG-Z, PB, CR-P, and AR: performed the data analyses. FG-Z wrote the manuscript with contributions from JS, PB, CR-P, and AR. All authors edited the manuscript before submission.
This work was funded by COLCIENCIAS (Grant No. 120465944147) and Universidad de los Andes, which provided valuable resources to FG-Z through “Proyecto Semilla” (Convocatoria 2015-2) and to the research program “Completando la teoría del origen de las especies de Darwin: especiación ecológica en octocorales, desde niños a doctores” from Vicerrectoria de Investigaciones - Universidad de los Andes.
The authors declare that the research was conducted in the absence of any commercial or financial relationships that could be construed as a potential conflict of interest.
We would like to thank CORALINA (Convenios No. 13, 2014 and No. 21, 2015), particularly to N. Bolaños and E. Castro, and PNN “Corales de Profundidad” for their support in field logistics and aid with collection permits; to several colleagues that supported sample collection activities: J. Andrade, F. García, O. Ruiz, D. Seguro, L. Dueñas, M. Forero, L. Gutierrez, M. Gómez, M. Marrugo, and A. Henao; to P. Etter and E. Johnson of SNPsaurus for their advice regarding sequencing and library preparations; to G. Dantas and his group at the Washington University School of Medicine for their assistance in metagenomics and to the HPC group of Universidad de los Andes for their support with the bioinformatic tools. To reviewers for helpful comments on the manuscript.
Supplementary materialThe Supplementary Material for this article can be found online at: https://www.frontiersin.org/articles/10.3389/fmars.2018.00029/full#supplementary-material
Supplementary Table 1. Symbiodinium type for 79 colonies of Agaricia undata.
Supplementary Table 2. OTU level taxonomy assignments and abundance.
Ainsworth, T. D., Krause, L., Bridge, T., Torda, G., Raina, J.-B., Zakrzewski, M., et al. (2015). The coral core microbiome identifies rare bacterial taxa as ubiquitous endosymbionts. ISME J. 9, 2261–2274. doi: 10.1038/ismej.2015.39
Andras, J. P., Rypien, K. L., and Harvell, C. D. (2013). Range-wide population genetic structure of the Caribbean sea fan coral, Gorgonia ventalina. Mol. Ecol. 22, 56–73. doi: 10.1111/mec.12104
Antao, T., Lopes, A., Lopes, R. J., Beja-Pereira, A., and Luikart, G. (2008). LOSITAN: a workbench to detect molecular adaptation based on a F st -outlier method. BMC Bioinformatics 9:323. doi: 10.1186/1471-2105-9-323
Bayer, T., Neave, M. J., Alsheikh-Hussain, A., Aranda, M., Yum, L. K., Mincer, T., et al. (2013). The microbiome of the red sea coral stylophora pistillata is dominated by tissue-associated endozoicomonas bacteria. Appl. Environ. Microbiol. 79, 4759–4762. doi: 10.1128/AEM.00695-13
Beaumont, M. A., and Nichols, R. A. (1996). Evaluating loci for use in the genetic analysis of population structure. Proc. R. Soc. London Ser. B Biol. Sci. 263, 1619–1626. doi: 10.1098/rspb.1996.0237
Bongaerts, P., Carmichael, M., Hay, K. B., Tonk, L., Frade, P. R., and Hoegh-guldberg, O. (2015a). Prevalent endosymbiont zonation shapes the depth distributions of scleractinian coral species Subject. R. Soc. Open Sci. 2:140297. doi: 10.1098/rsos.140297
Bongaerts, P., Frade, P. R., Hay, K. B., Englebert, N., Latijnhouwers, K. R. W., Bak, R. P. M., et al. (2015b). Deep down on a Caribbean reef: lower mesophotic depths harbor a specialized coral-endosymbiont community. Sci. Rep. 5:7652. doi: 10.1038/srep07652
Bongaerts, P., Frade, P. R., Ogier, J. J., Hay, K. B., Bleijswijk, J., Van, and Englebert, N. (2013). Sharing the slope: depth partitioning of agariciid corals and associated Symbiodinium across shallow and mesophotic habitats (2-60 m) on a Caribbean reef. BMC Evol. Biol. 13:205. doi: 10.1186/1471-2148-13-205
Bongaerts, P., Ridgway, T., Sampayo, E. M., and Hoegh-Guldberg, O. (2010). Assessing the “deep reef refugia” hypothesis: Focus on Caribbean reefs. Coral Reefs 29, 309–327. doi: 10.1007/s00338-009-0581-x
Bongaerts, P., Riginos, C., Brunner, R., Englebert, N., Smith, S. R., and Hoegh-guldberg, O. (2017). Deep reefs are not universal refuges: reseeding potential varies among coral species. Sci. Adv. 3:e1602373. doi: 10.1126/sciadv.1602373
Bongaerts, P., Sampayo, E. M., Bridge, T. C. L., Ridgway, T., Vermeulen, F., Englebert, N., et al. (2011). Symbiodinium diversity in mesophotic coral communities on the Great Barrier Reef: a first assessment. Mar. Ecol. Prog. Ser. 439, 117–126. doi: 10.3354/meps09315
Bridge, T. C. L., Fabricius, K. E., Bongaerts, P., Wallace, C. C., Muir, P. R., Done, T. J., et al. (2012). Diversity of scleractinia and octocorallia in the mesophotic zone of the Great Barrier Reef, Australia. Coral Reefs 31, 179–189. doi: 10.1007/s00338-011-0828-1
Cáceres, S. M., and Sánchez Muñoz, J. A. (2015). Growth strategies of an abundant reef-building coral in the southern Caribbean (Undaria tenuifolia). Rev. la Acad. Colomb. Cienc. Exact. Fis. Nat. 39, 348-357. doi: 10.18257/raccefyn.195
Caporaso, J. G., Bittinger, K., Bushman, F. D., Desantis, T. Z., Andersen, G. L., and Knight, R. (2010a). PyNAST: a flexible tool for aligning sequences to a template alignment. Bioinformatics 26, 266–267. doi: 10.1093/bioinformatics/btp636
Caporaso, J. G., Kuczynski, J., Stombaugh, J., Bittinger, K., Bushman, F. D., Costello, E. K., et al. (2010b). correspondence QIIME allows analysis of high- throughput community sequencing data Intensity normalization improves color calling in SOLiD sequencing. Nat. Methods 7, 335–336. doi: 10.1038/nmeth.f.303
Coffroth, M. A., Lasker, H. R., Diamond, M. E., Bruenn, J. A., and Bermingham, E. (1992). DNA fingerprints of a gorgonian coral: a method for detecting clonal structure in a vegetative species. Mar. Biol. 114, 317–325. doi: 10.1007/BF00349534
Cole, J. R., Wang, Q., Fish, J. A., Chai, B., McGarrell, D. M., Sun, Y., et al. (2014). Ribosomal database project: data and tools for high throughput rRNA analysis. Nucleic Acids Res. 42, 633–642. doi: 10.1093/nar/gkt1244
Cooper, T. F., Ulstrup, K. E., Dandan, S. S., Heyward, A. J., Kühl, M., Muirhead, A., et al. (2011). Niche specialization of reef-building corals in the mesophotic zone: metabolic trade-offs between divergent Symbiodinium types. Proc. R. Soc. B Biol. 278, 1840–1850. doi: 10.1098/rspb.2010.2321
Darriba, D., Taboada, G. L., Doallo, R. R., and Posada, D. (2012). jModelTest 2: more models, new heuristics and parallel computing. Nat. Method 9:772. doi: 10.1038/nmeth.2109
DeSantis, T. Z., Hugenholtz, P., Larsen, N., Rojas, M., Brodie, E. L., Keller, K., et al. (2006). Greengenes, a chimera-checked 16S rRNA gene database and workbench compatible with ARB. Appl. Environ. Microbiol. 72, 5069–5072. doi: 10.1128/AEM.03006-05
Diaz, J. M., Diaz-Pulido, G., and Sanchez, J. (2000). Distribution and structure of the southernmost Caribbean coral reefs: Golfo de Uraba, Colombia. Sci. Mar. 64, 327–336. doi: 10.3989/scimar.2000.64n3327
Diaz, J. M., and García-llano, C. F. (2010). Moluscos Del Mioceno Y Del Pleistoceno De La Isla De San Andrés (Mar Caribe, Colombia) y consideraciones paleobiogeograficas. Rev. Acad. Colomb. Cienc. 34, 105–116.
Drummond, A. J., Suchard, M. A., Xie, D., and Rambaut, A. (2012). Bayesian phylogenetics with BEAUti and the BEAST 1.7. Mol. Biol. Evol. 29, 1969–1973. doi: 10.1093/molbev/mss075
Eaton, D. A. R. (2014). PyRAD: assembly of de novo RADseq loci for phylogenetic analyses. Bioinformatics 30, 1844–1849. doi: 10.1093/bioinformatics/btu121
Edgar, R. C. (2010). Search and clustering orders of magnitude faster than BLAST. Bioinformatics 26, 2460–2461. doi: 10.1093/bioinformatics/btq461
Edgar, R. C. (2013). UPARSE: highly accurate OTU sequences from microbial amplicon reads. Nat. Methods 10, 996–998. doi: 10.1038/nmeth.2604
Edgar, R. C., Haas, B. J., Clemente, J. C., Quince, C., and Knight, R. (2011). UCHIME improves sensitivity and speed of chimera detection. Bioinformatics 27, 2194–2200. doi: 10.1093/bioinformatics/btr381
Ellis, J., and Solander, D. (1786). “The Natural History of many curious and uncommon Zoophytes, collected from various parts of the Globe,” in Systematically Arranged and Described by the Late Daniel Solander, Vol. 4 (London: Benjamin White & Son), 1–206, 1–63. Available online at: http://www.biodiversitylibrary.org/item/131537#page/11/mode/1up
Fine, M., and Loya, Y. (2002). Endolithic algae: an alternative source of photoassimilates during coral bleaching. Proc. Biol. Sci. 269, 1205–1210. doi: 10.1098/rspb.2002.1983
Foll, M., and Gaggiotti, O. (2008). A genome-scan method to identify selected loci appropriate for both dominant and codominant markers: a Bayesian perspective. Genetics 180, 977–993. doi: 10.1534/genetics.108.092221
Frade, P. R., Bongaerts, P., Winkelhagen, A. J. S., Tonk, L., and Bak, R. P. M. (2008). In situ photobiology of corals over large depth ranges: a multivariate analysis on the roles of environment, host, and algal symbiont. Limnol. Oceanogr. 53, 2711–2723. doi: 10.4319/lo.2008.53.6.2711
Fricke, H. W., Vareschi, E., and Schlichter, D. (1987). Photoecology of the coral Leptoseris fragilis in the Red Sea twilight zone (an experimental study by submersible). Oecologia 73, 371–381. doi: 10.1007/BF00385253
Glasl, B., Bongaerts, P., and Hoegh-guldberg, N. H. E. O. (2017). Microbiome variation in corals with distinct depth distribution ranges across a shallow – mesophotic gradient (15 – 85 m). Coral Reefs 36, 447–452. doi: 10.1007/s00338-016-1517-x
Goreau, T. F., and Wells, J. W. (1967). The shallow-water scleractinia of Jamaica: revised list of species and their vertical distribution range. Bull. Mar. Sci. 17, 442–453.
Goudet, J. (2005). HIERFSTAT, a package for R to compute and test hierarchical F -statistics. Mol. Ecol. Notes 2, 184–186. doi: 10.1111/j.1471-8286.2004.00828.x
Grajales, A., and Sanchez, J. A. (2016). Holobiont assemblages of dominant coral species (Symbiodinium types and coral species) shape Caribbean reef community structure Holobiont assemblages of dominant coral species (Symbiodinium types and coral species). Rev. Acad. Colomb. Cienc. Ex. Fis. Nat. 40, 300–311. doi: 10.18257/raccefyn.294
Grossart, H. P., Riemann, L., and Tang, K. W. (2013). Molecular and functional ecology of aquatic microbial symbionts. Front. Microbiol. 4:59. doi: 10.3389/fmicb.2013.00059
Guindon, S., and Gascuel, O. (2003). A simple, fast, and accurate algorithm to estimate large phylogenies by maximum likelihood. Syst. Biol. 52, 696–704. doi: 10.1080/10635150390235520
Hammer, Ø., Harper, D. A. T., and Ryan, P. D. (2001). Paleontological statistics software package for education and data analysis. Palaeontol. Electron. 4, 9–18. Available online at: http://palaeo-electronica.org/2001_1/past/issue1_01.htm
Hoeksema, B. W., Bongaerts, P., and Baldwin, C. C. (2017). High coral cover at lower mesophotic depths: a dense Agaricia community at the leeward side of Curaçao, Dutch Caribbean. Mar. Biodivers. 47, 67–70. doi: 10.1007/s12526-015-0431-8
Howells, E. J., Beltran, V. H., Larsen, N. W., Bay, L. K., Willis, B. L., and van Oppen, M. J. H. (2011). Coral thermal tolerance shaped by local adaptation of photosymbionts. Nat. Clim. Chang. 2, 116–120. doi: 10.1038/nclimate1330
Howells, E. J., Van Oppen, M. J. H., and Willis, B. L. (2009). High genetic differentiation and cross-shelf patterns of genetic diversity among Great Barrier Reef populations of Symbiodinium. Coral Reefs 28, 215–225. doi: 10.1007/s00338-008-0450-z
Jackson, S. A., Flemer, B., McCann, A., Kennedy, J., Morrissey, J. P., Gara, F. O., et al. (2013). Archaea appear to dominate the microbiome of inflatella pellicula deep sea sponges. PLoS ONE 8:e84438. doi: 10.1371/journal.pone.0084438
Jombart, T., and Ahmed, I. (2011). adegenet 1.3-1: new tools for the analysis of genome-wide SNP data. Bioinformatics 27, 3070–3071. doi: 10.1093/bioinformatics/btr521
Jones, G. (2013). Coral animals combat stress with sulphur. Nature 502, 634–635. doi: 10.1038/nature12698
Kahng, S., Copus, J., and Wagner, D. (2014). Recent advances in the ecology of mesophotic coral ecosystems (MCEs). Curr. Opin. Environ. Sustain. 7, 72–81. doi: 10.1016/j.cosust.2013.11.019
Kahng, S. E., Garcia-Sais, J. R., Spalding, H. L., Brokovich, E., Wagner, D., Weil, E., et al. (2010). Community ecology of mesophotic coral reef ecosystems. Coral Reefs 29, 255–275. doi: 10.1007/s00338-010-0593-6
Kimes, N. E., Van Nostrand, J. D., Weil, E., Zhou, J., and Morris, P. J. (2010). Microbial functional structure of Montastraea faveolata, an important Caribbean reef-building coral, differs between healthy and yellow-band diseased colonies. Environ. Microbiol. 12, 541–556. doi: 10.1111/j.1462-2920.2009.02113.x
Klaus, J. S., Janse, I., Heikoop, J. M., Sanford, R. A., and Fouke, B. W. (2007). Coral microbial communities, zooxanthellae and mucus along gradients of seawater depth and coastal pollution. Environ. Microbiol. 9, 1291–1305. doi: 10.1111/j.1462-2920.2007.01249.x
Lajeunesse, T. C. (2002). Diversity and community structure of symbiotic dinoflagellates from Caribbean coral reefs. Mar. Biol. 141, 387–400. doi: 10.1007/s00227-002-0829-2
Lajeunesse, T. C., and Thornhill, D. J. (2011). Improved resolution of reef-coral endosymbiont (symbiodinium) species diversity, ecology, and evolution through psbA Non-Coding Region Genotyping. PLoS ONE 6:e29013. doi: 10.1371/journal.pone.0029013
Lawler, S. N., Kellogg, C. A., France, S. C., Clostio, R. W., Brooke, S. D., and Ross, S. W. (2016). Coral-associated bacterial diversity is conserved across two deep-sea anthothela species. Front. Microbiol. 7:458. doi: 10.3389/fmicb.2016.00458
Leite, D. C. A., Leão, P., Garrido, A. G., Lins, U., Santos, H. F., Pires, D. O., et al. (2017). Broadcast spawning coral mussismilia hispida can vertically transfer its associated bacterial core. Front. Microbiol. 8:176. doi: 10.3389/fmicb.2017.00176
Lesser, M. P., Marc, S., Michael, S., Michiko, O., Gates, R. D., and Andrea, G. (2010). Photoacclimatization by the coral Montastraea cavernosa in the mesophotic zone: light, food, and genetics. Ecology 91, 990–1003. doi: 10.1890/09-0313.1
Lesser, M. P., Slattery, M., and Leichter, J. J. (2009). Ecology of mesophotic coral reefs. J. Exp. Mar. Biol. Ecol. 375, 1–8. doi: 10.1016/j.jembe.2009.05.009
Lins-de-Barros, M. M., Vieira, R. P., Cardoso, A. M., Monteiro, V. A., Turque, A. S., Silveira, C. B., et al. (2010). Archaea, bacteria, and algal plastids associated with the reef-building corals siderastrea stellata and mussismilia hispida from Búzios, South Atlantic Ocean, Brazil. Microb. Ecol. 59, 523–532. doi: 10.1007/s00248-009-9612-y
Lucas, M. Q., Stat, M., Smith, M. C., Weil, E., and Schizas, N. V. (2016). Symbiodinium (internal transcribed spacer 2) diversity in the coral host Agaricia lamarcki (Cnidaria: Scleractinia) between shallow and mesophotic reefs in the Northern Caribbean (20-70 m). Mar. Ecol. 37, 1079–1087. doi: 10.1111/maec.12367
MADS (2013). Resolucion No. 0339 12 abril 2013 “Por Medio de la Cual se Reserva, Delimita, Alindera y Declara el Parque Nacional Natural Corales de Profundidad.”
Meirelles, P. M., Amado-Filho, G. M., Pereira-Filho, G. H., Pinheiro, H. T., De Moura, R. L., Joyeux, J. C., et al. (2015). Baseline assessment of mesophotic reefs of the Vitória-Trindade Seamount Chain based on water quality, microbial diversity, benthic cover and fish biomass data. PLoS ONE 10:e0130084. doi: 10.1371/journal.pone.0130084
Neave, M. J., Apprill, A., Ferrier-Pagès, C., and Voolstra, C. R. (2016). Diversity and function of prevalent symbiotic marine bacteria in the genus Endozoicomonas. Appl. Microbiol. Biotechnol. 100, 8315–8324. doi: 10.1007/s00253-016-7777-0
Neave, M. J., Rachmawati, R., Xun, L., Michell, C. T., Bourne, D. G., Apprill, A., et al. (2017). Differential specificity between closely related corals and abundant Endozoicomonas endosymbionts across global scales. ISME J. 11, 186–200. doi: 10.1038/ismej.2016.95
Olson, J. B., and Kellogg, C. A. (2010). Microbial ecology of corals, sponges, and algae in mesophotic coral environments. FEMS Microbiol. Ecol. 73, 17–30. doi: 10.1111/j.1574-6941.2010.00862.x
Pritchard, J. K., Stephens, M., and Donnelly, P. (2000). Inference of population structure using multilocus genotype data. Genetics 155, 945–959.
Puechmaille, S. J. (2016). The program structure does not reliably recover the correct population structure when sampling is uneven: subsampling and new estimators alleviate the problem. Mol. Ecol. Resour. 16, 608–627. doi: 10.1111/1755-0998.12512
Pyle, R. L. (1998). “Use of advanced mixed-gas diving technology to explore the coral reef ‘twilight zone’,” in Ocean Pulse, eds J. T. Tanacredi and J. Loret (Boston, MA: Springer), 71–88.
Radecker, N., Pogoreutz, C., Voolstra, C. R., Wiedenmann, J., and Wild, C. (2015). Nitrogen cycling in corals: the key to understanding holobiont functioning? Trends Microbiol. 23, 490–497. doi: 10.1016/j.tim.2015.03.008
Raina, J.-B., Tapiolas, D. M., Foret, S., Lutz, A., Abrego, D., Ceh, J., et al. (2013). DMSP biosynthesis by an animal and its role in coral thermal stress response. Nature 502, 677–680. doi: 10.1038/nature12677
Rambaut, A., and Drummond, A. J. (2010). FigTree v1. 3.1. Institute of Evolutionary Biology. University of Edinburgh.
Reddy, T. B. K., Thomas, A. D., Stamatis, D., Bertsch, J., Isbandi, M., Jansson, J., et al. (2015). The Genomes OnLine Database (GOLD) v.5: a metadata management system based on a four level (meta)genome project classification. Nucleic Acids Res. 43, D1099–D1106. doi: 10.1093/nar/gku950
Reid, N. M., and Carstens, B. C. (2012). Phylogenetic estimation error can decrease the accuracy of species delimitation: a Bayesian implementation of the general mixed Yule-coalescent model. BMC Evol. Biol. 12:196. doi: 10.1186/1471-2148-12-196
Restrepo, J. D., Zapata, P., Díaz, J. M., Garzón-Ferreira, J., and García, C. B. (2006). Fluvial fluxes into the Caribbean Sea and their impact on coastal ecosystems: the Magdalena River, Colombia. Glob. Planet. Change 50, 33–49. doi: 10.1016/j.gloplacha.2005.09.002
Rohwer, F., Seguritan, V., Azam, F., and Knowlton, N. (2002). Diversity and distribution of coral-associated bacteria. Mar. Ecol. Prog. Ser. 243, 1–10. doi: 10.3354/meps243001
Ronquist, F., Teslenko, M., Van Der Mark, P., Ayres, D. L., Darling, A., Höhna, S., et al. (2012). Mrbayes 3.2: efficient bayesian phylogenetic inference and model choice across a large model space. Syst. Biol. 61, 539–542. doi: 10.1093/sysbio/sys029
Sánchez, J. A. (1999). Black coral-octocoral distribution patterns on Imelda Bank, a deep-water reef, Colombia, Caribbean sea. Bull. Mar. Sci. 65, 215–225.
Serrano, X., Baums, I. B., Reilly, K. O., Smith, T. B., Jones, R. J., and Shearer, T. L. (2014). Geographic differences in vertical connectivity in the Caribbean coral Montastraea cavernosa despite high levels of horizontal connectivity at shallow depths. Mol. Ecol. 23, 4226–4240. doi: 10.1111/mec.12861
Silva-Lima, A. W., Walter, J. M., Garcia, G. D., Ramires, N., Ank, G., Meirelles, P. M., et al. (2015). Multiple symbiodinium strains are hosted by the brazilian endemic corals Mussismilia spp. Microb. Ecol. 70, 301–310. doi: 10.1007/s00248-015-0573-z
Smith, E. G., Angelo, C. D., Sharon, Y., Tchernov, D., and Wiedenmann, J. (2017). Acclimatization of symbiotic corals to mesophotic light environments through wavelength transformation by fluorescent protein pigments. Proc. R. Soc. B 284:20170320. doi: 10.1098/rspb.2017.0320
Stamatakis, A. (2006). RAxML-VI-HPC: maximum likelihood-based phylogenetic analyses with thousands of taxa and mixed models. Bioinformatics 22, 2688–2690. doi: 10.1093/bioinformatics/btl446
Stat, M., Morris, E., and Gates, R. D. (2008). Functional diversity in coral – dinoflagellate symbiosis. Proc. Natl. Acad. Sci. U.S.A. 105, 9256–9261. doi: 10.1073/pnas.0801328105
Steinert, G., Taylor, M. W., Deines, P., Simister, R. L., de Voogd, N. J., Hoggard, M., et al. (2016). In four shallow and mesophotic tropical reef sponges from Guam the microbial community largely depends on host identity. PeerJ. 4:e1936. doi: 10.7717/peerj.1936
Tamura, K., Stecher, G., Peterson, D., Filipski, A., and Kumar, S. (2013). MEGA6: molecular evolutionary genetics analysis version 6.0. Mol. Biol. Evol. 30, 2725–2729. doi: 10.1093/molbev/mst197
Thornhill, D. J., Fitt, W. K., and Schmidt, G. W. (2006). Highly stable symbioses among western Atlantic brooding corals. Coral Reefs 25, 515–519. doi: 10.1007/s00338-006-0157-y
Thornhill, D. J., Lewis, A. M., Wham, D. C., and LaJeunesse, T. C. (2014). Host-specialist lineages dominate the adaptive radiation of reef coral endosymbionts. Evolution 68, 352–367. doi: 10.1111/evo.12270
Thornhill, D. J., Xiang, Y., Fitt, W. K., and Santos, S. R. (2009). Reef endemism, host specificity and temporal stability in populations of symbiotic dinoflagellates from two ecologically dominant Caribbean corals. PLoS ONE 4:e6262. doi: 10.1371/journal.pone.0006262
Turner, J. A., Babcock, R. C., Hovey, R., and Kendrick, G. A. (2017). Deep thinking: a systematic review of mesophotic coral ecosystems. ICES J. Mar. Sci. 74, 1–12. doi: 10.1093/icesjms/fsx085
van Tienderen, K. M., and van der Meij, S. E. T. (2016). Occurrence patterns of coral-dwelling gall crabs (Cryptochiridae) over depth intervals in the Caribbean. PeerJ 4:e1794. doi: 10.7717/peerj.1794
Wild, C., Huettel, M., Klueter, A., Kremb, S. G., Rasheed, M. Y. M., and Jorgensen, B. B. (2004). Coral mucus functions as an energy carrier and particle trap in the reef ecosystem. Nature 428, 66–70. doi: 10.1038/nature02344
Zhang, J., Kapli, P., Pavlidis, P., and Stamatakis, A. (2013). A general species delimitation method with applications to phylogenetic placements. Bioinformatics 29, 2869–2876. doi: 10.1093/bioinformatics/btt499
Ziegler, M., Roder, C. M., Büchel, C., and Voolstra, C. R. (2015). Mesophotic coral depth acclimatization is a function of host-specific symbiont physiology. Front. Mar. Sci. 2:4. doi: 10.3389/fmars.2015.00004
Keywords: mesophotic coral ecosystems, holobiont, Agaricia undata, endosymbiont, coral reefs, Symbiodinium
Citation: Gonzalez-Zapata FL, Bongaerts P, Ramírez-Portilla C, Adu-Oppong B, Walljasper G, Reyes A and Sanchez JA (2018) Holobiont Diversity in a Reef-Building Coral over Its Entire Depth Range in the Mesophotic Zone. Front. Mar. Sci. 5:29. doi: 10.3389/fmars.2018.00029
Received: 29 September 2017; Accepted: 22 January 2018;
Published: 07 February 2018.
Edited by:
Tito Monteiro da Cruz Lotufo, University of São Paulo, BrazilReviewed by:
Detmer Sipkema, Wageningen University and Research, NetherlandsCopyright © 2018 Gonzalez-Zapata, Bongaerts, Ramírez-Portilla, Adu-Oppong, Walljasper, Reyes and Sanchez. This is an open-access article distributed under the terms of the Creative Commons Attribution License (CC BY). The use, distribution or reproduction in other forums is permitted, provided the original author(s) and the copyright owner are credited and that the original publication in this journal is cited, in accordance with accepted academic practice. No use, distribution or reproduction is permitted which does not comply with these terms.
*Correspondence: Juan A. Sanchez, anVhbnNhbmNAdW5pYW5kZXMuZWR1LmNv
Disclaimer: All claims expressed in this article are solely those of the authors and do not necessarily represent those of their affiliated organizations, or those of the publisher, the editors and the reviewers. Any product that may be evaluated in this article or claim that may be made by its manufacturer is not guaranteed or endorsed by the publisher.
Research integrity at Frontiers
Learn more about the work of our research integrity team to safeguard the quality of each article we publish.