- 1Parks and Environmental Services, Marine Programs, Capital Regional District, Victoria, BC, Canada
- 2School of Earth and Ocean Sciences, University of Victoria, Victoria, BC, Canada
Concerns over the fate of pharmaceuticals and personal care products (PPCP) within the environment have been growing with the advent of more precise analytical methods. The discharge of municipal wastewater has been identified as a significant source of these contaminants, particularly where wastewater treatment is minimal. Here we present results from a comprehensive monitoring regime located around Victoria, BC, Canada. Samples were collected between 2009 and 2016 from a variety of sources including marine water, sediment and biota adjacent to two major untreated sewage outfalls, as well as the sewage itself. PPCP concentrations within the untreated sewage were high, and the sediment surrounding the outfalls showed corresponding contamination. However, this contamination dropped quickly with distance from the outfall such that by 800 m distance most PPCPs were below detection limits. Tissue samples of resident Northern Horse mussels (Modiolus modiolus) collected adjacent to one of the major sewage outfalls showed high single sample concentrations of the antimicrobial triclosan (317 ng g−1 dry weight), the antibiotic ciprofloxacin (176 ng g−1 dry weight), as well as the antidepressant sertraline (84.1 ng g−1 dry weight). Reference stations from around the region showed very low concentrations of contamination with almost all PPCP concentrations being below detection limits. Within the sewage, concentrations were largely stable overtime, with the exception of triclosan and triclocarban which both showed declines over the study period.
Introduction
The presence of detectable concentrations of pharmaceuticals and synthetic personal care products (PPCPs) within natural waters has been known for some time now (Daughton and Ternes, 1999; Fatta-Kassinos et al., 2011). However, the effects of these trace contaminants are less clear (Fatta-Kassinos et al., 2011; Vidal-Dorsch et al., 2012; Arpin-Pont et al., 2016) and impacts are only starting to emerge in the literature. For example, antibiotic resistant bacteria have been shown to evolve rapidly in areas subject to continual discharge of antibiotics, such as within wastewater treatment plants (WWTP) and even their immediate receiving environments (Rizzo et al., 2013; Everage et al., 2014; Manaia, 2014). Populations of fish exposed to even low concentrations of estrogenic compounds, have shown skewness in sex ratios and much higher incidents of intersex, among other problems (Vajda et al., 2008; Sumpter and Jobling, 2013).
Although there are many sources of PPCPs to the environment, WWTPs and sewage outfalls are the biggest source (Benotti and Brownawell, 2007; Gaw et al., 2014; Luo et al., 2014). Some PPCPs are able to resist degradation in traditional secondary treatment plants, however many PPCPs are decreased to some extent during wastewater treatment processes, either by degradation or sorption to settling particulate matter (Suárez et al., 2008). In jurisdictions with limited or no sewage treatment, such as in Victoria B.C. Canada, PPCP concentrations in effluent tend to be higher than effluent from facilities with higher levels of treatment which has been shown to effectively reduce the concentration of many PPCPs (Kasprzyk-Hordern et al., 2009; Lowe, 2011; Sun et al., 2016).
Because sewage contains a complex mixture of contaminants it is difficult to separate the ecological impacts of PPCPs from the many other toxic substances present in sewage. None-the-less, pharmaceuticals are unique among environmental contaminants as they are purposefully engineered to have impacts on patients (human and animal) at very low concentrations. The sub-lethal effects of these compounds individually or as a mixture are difficult to assess (Rodea-Palomares et al., 2016) and some impacts may be masked by the presence of other contaminants or cause subtle changes that are difficult to observe, but could have ecosystem wide impacts (Daughton and Ternes, 1999).
Study Site
Greater Victoria is located on the southern tip of Vancouver Island, and the surrounding marine environment is comparatively pristine (Ban et al., 2010). However, the city is unique among its North American and European counterparts for its lack of even primary sewage treatment (Environment and Climate Change Canada, 2012; European Environmental Agency, 2013; OECD, 2015). The city of nearly 350,000 inhabitants (Statistics Canada, 2012) fine screens (6 mm) its wastewater to remove large debris (CRD, 2015) before discharging it through two deep-sea outfalls into the cold fast moving waters of Juan de Fuca Strait (Krogh, 2017). The two pump stations are located at Macaulay (MAC) and Clover (CLO) Points (Figure 1) and each outfall extends over a kilometer offshore to approximately 65 m depth. The wastewater is dispersed through a series of diffuser ports along the final 150 m (CRD, 2015) of each outfall. Each outfall discharged an annual mean of 50,000 m3 d−1 per day with biochemical oxygen demand concentrations averaging 260 mg L−1 for MAC and 220 mg L−1 for CLO (CRD, 2015).
Currents at the outfall sites are dominated by mixed semi-diurnal tides with speeds up to 1 m s−1. The residual non-tidal currents in the bottom of the water column are toward the south-east at the MAC outfall (Krogh, 2017) and toward the east at the CLO outfall (Chandler, 1997). These residual currents are driven by the large estuarine circulation of the Fraser River (Krogh, 2017). Water temperatures are cool (8–12°C) and oxygen levels are high (200 μmol kg−1, Krogh, 2017). Of the two outfalls MAC has lower flows, but the effluent typically has higher concentrations of contaminants, and the receiving environment is generally considered to be more vulnerable to negative impacts as current speeds are lower than around CLO (Dinn et al., 2012). The seabed around MAC is made up of a mixture of sediment size classes ranging from fines to gravel while CLO is rocky (Markovic, 2003; CRD, 2015; Krogh, 2017). Past sediment quality assessments from MAC show that elevated concentrations of many sewage derived compounds extend out to 800 m in the dominant down current direction (south-east) (Dinn et al., 2012; CRD, 2015). The seabed is also littered with debris from when the seabed was used as the city's garbage dump between 1901 and 1955 (Ringuette, 2009).
Study Overview
The operation and environmental monitoring of CLO and MAC outfalls is the responsibility of the Capital Regional District (CRD). Beginning in 2004, the CRD began exploratory sampling for PPCPs within wastewater and the marine receiving environment in addition to their routine monitoring efforts (Verenitch et al., 2006; Lowe, 2011; Saunders et al., 2016). Since 2004, monitoring of PPCPs was intermittent, with more regular quarterly monitoring of select PPCPs within the effluent beginning in 2014. Monitoring of PPCPs in the marine receiving environment water, sediment and marine biota continued to be sporadic, but became more frequent and broader in scope post-2009. Table 1 outlines the observational scope of this study. To aid in the interpretation of results, we have omitted the majority of samples which had no detectable concentrations of PPCPs. The detection limit of each compound from each matrix is provided in Tables S1–S5.
Methods
Sample sites were selected based on a series of criteria including the co-location with historical sampling locations as well as ensuring the known gradient of contamination was captured. Several stations were clustered close to CLO and MAC sewage outfalls because these areas were known to have the highest concentrations of sewage derived contamination. Relatively clean reference stations located at Finnerty Cove (FC), Albert Head (AH), Parry Bay (PB), and Constance Bank (CB) were also sampled. Victoria Harbor samples (VH1 and VH2) were collected opportunistically and represent a highly anthropogenic environment. Although no municipal sewage is legally discharged into the harbor and it is presumed to not be significantly impacted by MAC and CLO effluent, it is possible that some sewage is being discharged from vessels, and illicit cross connections to the storm sewer may exist.
All sample collection equipment was pre-cleaned (acid/solvent/DI rinsed) before use and all collection bottles and jars were certified trace clean to EPA standards by SGS AXYS Analytical Services in Sidney, BC.
Wastewater Sample Collection
Wastewater flows have a strong diurnal pattern with daily maxima typically occurring in the morning (~550 L s−1), with a second peak in the evening and lowest flows overnight (~130 L s−1). From the start of 2014 to the end of 2016 wastewater samples were collected at both the MAC and CLO pump stations on a quarterly basis each year (January, April, July, and October) for PPCP analysis. Samples were collected post screening (6 mm) immediately prior to discharge as a 24-h composite (400 mL collected every 30 min for 24 h) using an automated, refrigerated Teledyne ISCO sampler (Lincoln, Nebraska USA). While continuously stirring the large composite, subsamples were taken in 1L amber glass bottles with Teflon lined lids and transported on ice to the laboratory for analysis (section Wastewater, Sediment and Tissue Lab Analysis).
In November 2009, daily wastewater samples were collected for five consecutive days from CLO and MAC using an identical sampling technique to that described above. All wastewater collection equipment including hoses, carboys, and lids bottles were polytetrafluoroethylene (PTFE, Teflon) lined.
Marine Sediment Sample Collection
Marine sediment from three stations (M100E, M200E, M800E, Figure 1) near the Macaulay outfall and a reference station in Parry Bay (PB, Figure 1) were sampled in September 2010 using a 0.1 m2 stainless steel Van Veen grab sampler. Stations to the east of Macaulay Point were sampled instead of the likely slightly more contaminated south-east, in order to be coordinated with other sediment sampling that was occurring in 2010. Several grabs were taken at each station and the top 10 cm of sediment from each grab sample was homogenized into a single composite sample for each station and stored in amber glass jars with Teflon lined lids for analysis. The number of grab samples integrated into the composite varied depending on grab volume and the amount of sediment required for analyses but always included at least three grabs. Samples were stored on ice for transport to the laboratory where they were stored frozen for up to 3 weeks prior to analysis (section Wastewater, Sediment and Tissue Lab Analysis) of PPCP list 1–5 compounds (Tables S1–S5). Following the same procedure as 2010, sediment samples were collected in September 2014 from AH1, AH2, M0, and PB1 and in January 2015 from FC1 and FC2 (Figure 1) and analyzed for list 3 (Table S3) compounds.
Marine Water
The water column extending approximately 100 m around each of the CLO an MAC sewage outfall diffusers is known as the initial dilution zone (IDZ). Four stations at the edge of each IDZ were sampled in October 2012 for List 1 PPCPs (Table S1) in the predicted down current direction (Hodgins, 2009). The down current direction was chosen in order to maximize the likelihood of sampling within the wastewater plumes, therefore capturing the highest contaminant concentrations.
Samples were collected at depths ranging from 5 meters below the surface to 5 meters from the bottom (60–65 m total water column depth) using an automated rosette equipped with 4L Niskin bottles. Subsamples of seawater were decanted from the Niskin bottles into pre-cleaned amber glass bottles and stored on ice for transport to the laboratory for analysis (section Wastewater, Sediment and Tissue Lab Analysis).
Benthic Tissue
Horse mussels (Modiolus modiolus) were collected from two stations near the CLO sewage outfall (C0 and C200E, Figure 1) and one reference station at Constance Bank (CB, Figure 1) in September 2015. Fifteen (15) mussels were collected using a 0.1 m2 stainless steel Van Veen grab sampler at each station.
Intertidal blue mussels (Mytilus edulis) were sampled opportunistically in Victoria Harbor off a house boat (VH1, Figure 1), off of a floating log boom (VH2, Figure 1) and from an intertidal rocky outcrop at Albert Head (AH3, Figure 1). All blue mussels were collected in October 2015 by plucking specimens off their respective substrate.
Benthic biota samples were collected at Finnerty Cove (FC1) and Albert Head (AH1) (Figure 1) using a 0.1 m2 stainless steel Van Veen grab sampler. Biota samples were screened from the sediment samples using stainless steel trays with a 1.0 mm mesh that was acetone and DI cleaned. During screening, individual organisms were removed with clean forceps and placed in amber glass jars.
Wastewater, Sediment and Tissue Lab Analysis
Laboratory analysis was carried out by SGS AXYS Analytical Services in Sidney, BC by AXYS method MLA-075 using LC-MS/MS techniques. Tables S1–S5 give a complete list of analytes. No corrections were applied to final analytical values.
All samples measured in water (wastewater and marine waters) were filtered using a 1.0 μm glass fiber filter prior to analysis and only the filtrate was analyzed. Prior to extraction, samples were adjusted to the required pH and fortified with isotopically labeled quantification standards.
Biota samples were prepared by SGS AXYS Analytical Services. Mussel samples were shucked and composited, and benthic biota samples were homogenized, in clean room conditions prior to extraction and analysis. Isotopically labeled quantification standards were also added to the extracts.
All sediment and tissue samples were frozen for up to several weeks before analysis.
Statistical analysis and figure generation was carried out using the R software package to calculate p-values (α = 0.05), slopes, intercepts, Pearson R2, and their accompanying standard errors. Plots show the 95% confidence interval about the estimated slopes.
Results
Post-2014 Quarterly Wastewater Sampling
Figures 2, 3 (as well as Table 2) show the results of quarterly wastewater sampling at CLO and MAC. Due to the lack of primary or secondary treatment, concentrations of PPCPs were generally higher than those observed in treated wastewater elsewhere (Weigel et al., 2004; Nakada et al., 2006; Vidal-Dorsch et al., 2012; Luo et al., 2014; Meador et al., 2016). This comes as little surprise as many PPCPs have been shown to be effectively reduced by wastewater treatment, either by absorption to sinking particles or biodegradation (Luo et al., 2014).
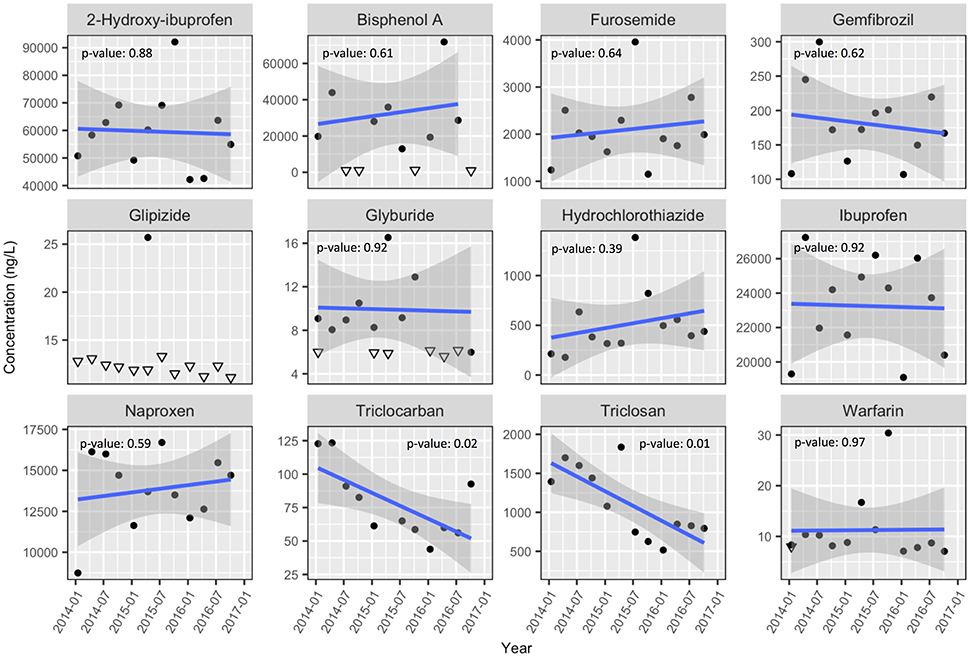
Figure 2. Filtered wastewater PPCP concentrations in MAC wastewater pre-discharge. Solid blue lines are linear fits (with p-value) with the gray banding representing 95% confidence intervals. Split lab replicates were averaged; non-detected values are marked with their sample specific detection limit as downward facing open triangles. Post-2014 data are presented separately from 2009 data due to changes in analytical methods. For full statistics see Table S7.
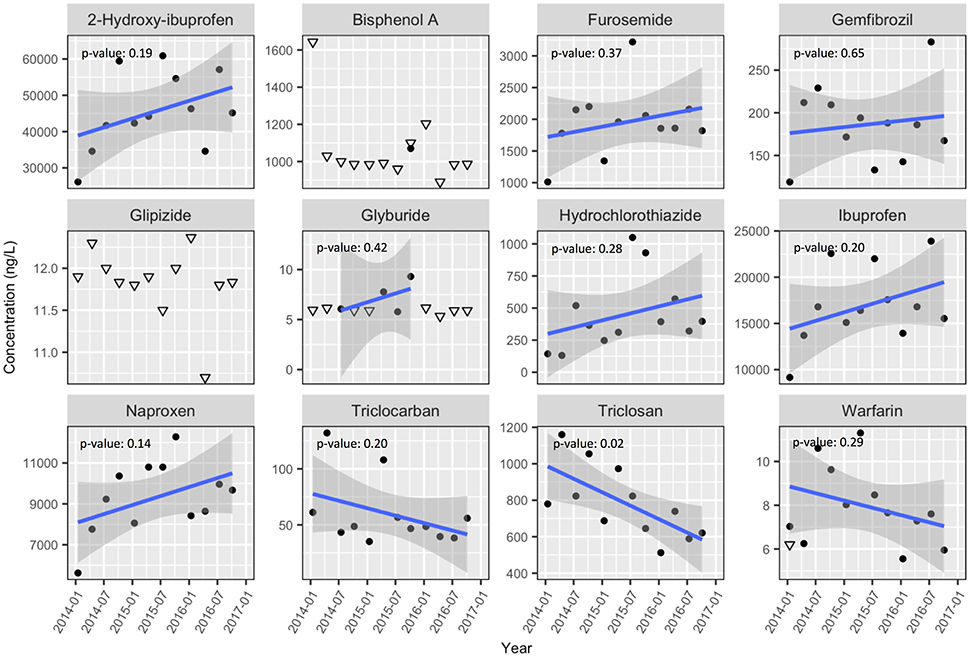
Figure 3. Same as Figure 2 but for CLO wastewater. Again, downward facing open triangles indicate the sample specific detection limit where no detection occurred. Post-2014 data are presented separately from 2009 data due to changes in analytical methods.
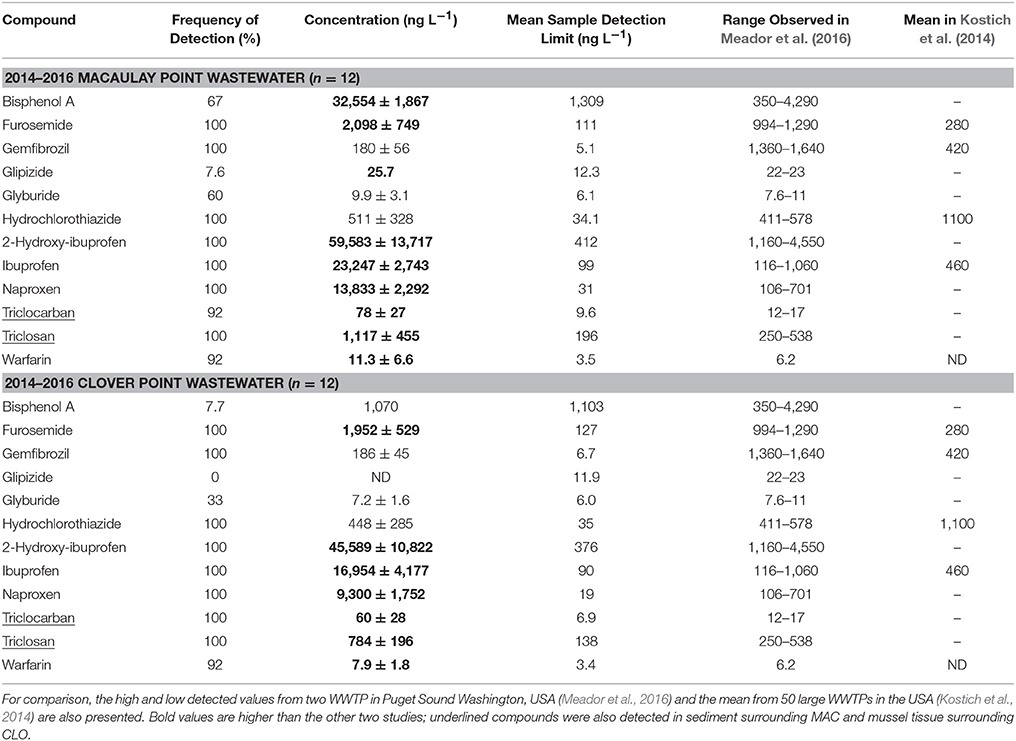
Table 2. Concentrations (mean ± standard deviation) of detected PPCPs in CLO and MAC wastewater observed between 2014 and 2016.
With only a few exceptions, the concentrations of detected PPCPs within the wastewater were stable over the period 2014–2016. Notable exceptions to this include statistically significant (p-value < 0.05) declines in triclosan concentrations at both MAC (p-value: 0.01, Figure 2) and CLO (p-value: 0.02, Figure 3), as well as triclocarban at MAC (p-value: 0.02). Both triclosan and triclocarban are antimicrobial agents used extensively in consumer soaps and other products (Food and Drug Administration, HHS, 2016). The observed declines could be due to the voluntary phase out of these compounds by manufacturers in the face of new regulations limiting their use in the United States (Food and Drug Administration, HHS, 2016), European Union and other countries (Environment and Climate Change Canada, 2016). These declines are further supported qualitatively by the 2009 results where triclosan and triclocarban concentrations at both CLO and MAC were even higher than those observed in 2014 (Table S8).
The industrial chemical bisphenol-A (BPA) was present in MAC effluent at concentrations of up to 73,800 ng L−1 (Figure 2). Concentrations this high exceed known chronic toxicity thresholds for a wide array of aquatic taxa (Flint et al., 2012) and far exceed those observed in other municipal wastewater effluents (Nakada et al., 2006; Vidal-Dorsch et al., 2012; Luo et al., 2014; Meador et al., 2016) including those collected from CLO (Figure 3, Table 2). The presence of BPA in MAC effluent was, however, highly variable with about 25% of all wastewater samples taken at MAC having BPA concentrations below detection limits (approximately 1,100 ng L−1). Exploratory sampling conducted in January 2016 of the regional landfill's leachate, which is periodically released into the MAC sewage system, found a BPA concentration of 638,000 ng L−1, high for municipal landfill leachate (Kurata et al., 2008). Given the relatively large volumes and periodic release of the landfill leachate into the MAC sewage system, it is considered probable that this is the source of the periodically high BPA concentrations measured at MAC. Similar observations of periodically high BPA in MAC effluent have been observed in the past (Lowe, 2011).
A few compounds appeared to experience subtle seasonal cycles in wastewater concentration (e.g. MAC naproxen, Figure 2). The MAC and CLO wastewater catchment areas are susceptible to significantly increased wastewater flow volumes during heavy rains (Lowe, 2011; CRD, 2015). As such, it was expected that dilution effects would be observed in PPCP concentrations during the winter rainy season. Correlations were made between the wastewater PPCP concentrations and collection day flow volumes (Figures S1, S3). Only naproxen concentrations at MAC showed a significant (p-value < 0.05) negative correlation with flow volume (p-value = 0.002, i.e., a dilution effect). Seasonal changes in wastewater temperature could also potentially impact PPCP concentrations, as temperature can impact microbial activity and therefore PPCP breakdown in the catchment system. Correlations between PPCP concentration and collection day temperature were also assessed (Figures S2, S4); significant (p-value < 0.05) positive correlations were observed at CLO for naproxen (p-value = 0.01), ibuprofen (p-value = 0.003), furosemide (p-value = 0.008) and 2-hydroxy-ibuprofen (p-value = 0.01). Wastewater sampling frequency, however, was probably insufficient to capture the full seasonal flow volume or temperature to concentration relationships (Ort et al., 2010a,b).
2009 Daily Wastewater Sampling
Tables 3, 4 show the partial results of daily sampling from November 2009 (for full results see Lowe, 2011). Results were truncated and presented separately from the 2014–2016 wastewater data because the 2009 data does not capture the full seasonal cycle and analytical methods changed slightly between 2009 and 2014 (Sean Campbell, SGS AXYS Analytical Services, personal communication; for a side-by-side comparison of results see Table S8). Only compounds that were also detected in 2014–2016 wastewater, the sediment surrounding MAC, or the horse mussels surrounding CLO are presented.
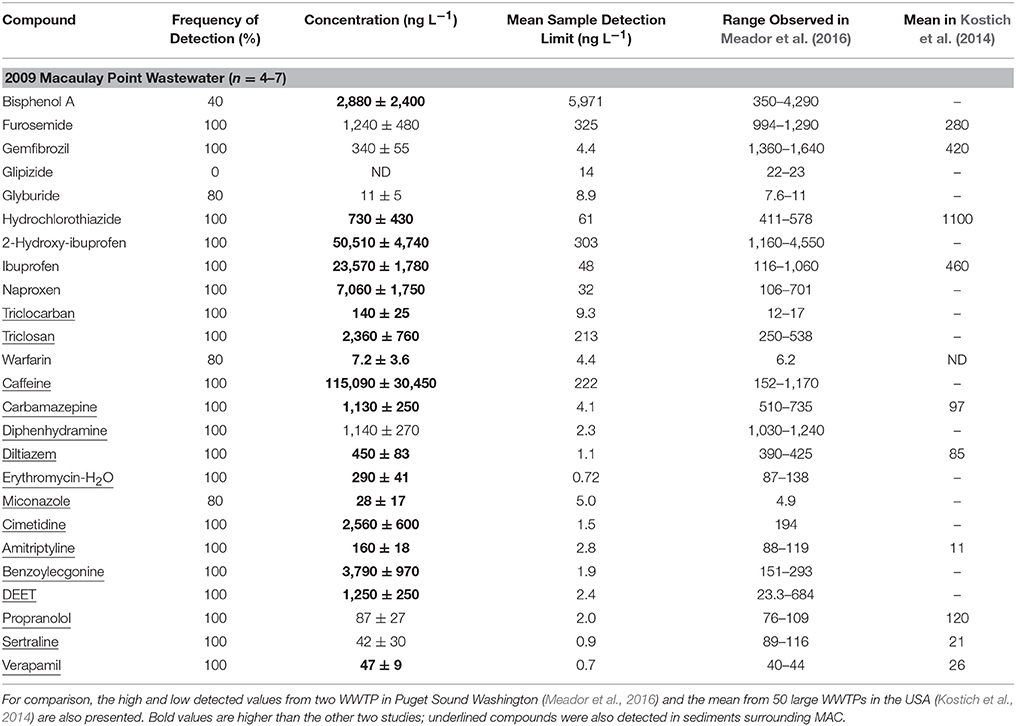
Table 3. Concentration (mean ± standard deviation) of detected PPCPs in MAC wastewater (full results see Lowe 2011) in November 2009.
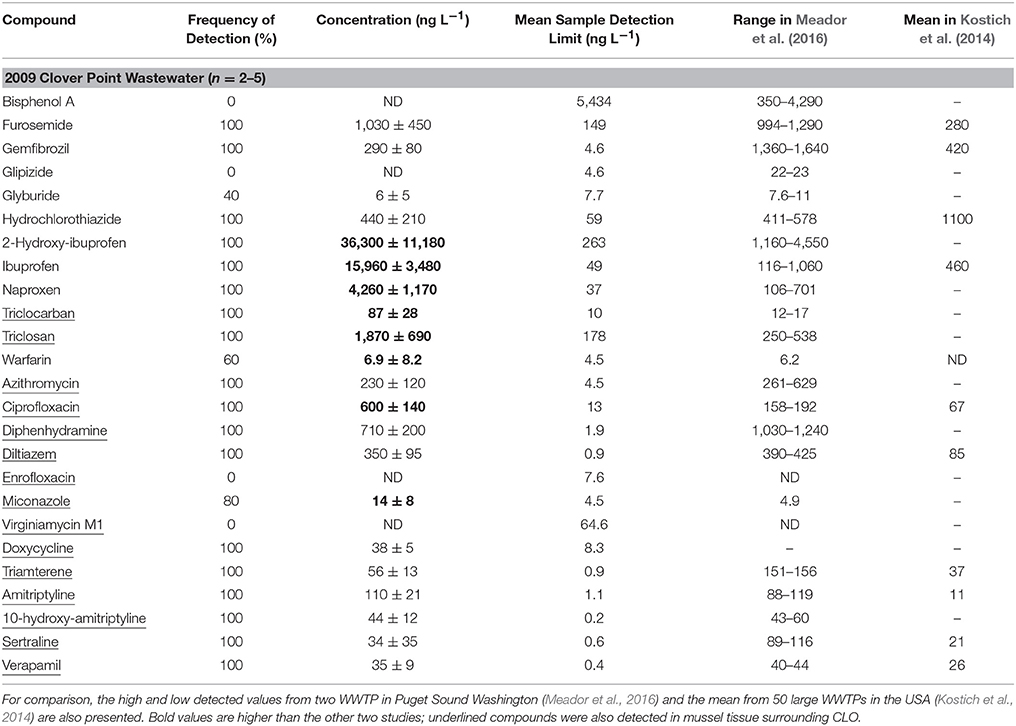
Table 4. Concentration (mean ± standard deviation) of detected PPCPs in CLO wastewater (full results see Lowe 2011) in November 2009.
Marine Water
Six samples of seawater, collected from depths ranging from near surface to near bottom were analyzed for 46 PPCPs (Table S1). From this set of samples, only caffeine (up to 37.4 ng L−1), acetaminophen (up to 44.7 ng L−1), and dehydronifedipine (up to 1.53 ng L−1) were detected in at least one of the samples. Although somewhat high for natural marine waters, concentrations of this magnitude or even higher (in the case of Jamaica Bay, NY) have been observed in other areas heavily influenced by human activity and wastewater (Benotti and Brownawell, 2007; Klosterhaus et al., 2013; Arpin-Pont et al., 2016; Meador et al., 2016; Cantwell et al., 2017).
Sediment
Figure 4 shows all detected compounds from the 2010 and 2014 sediment sampling (single samples at each location; no stations were sampled in both 2010 and 2014). Sediment contamination near the Macaulay outfall was high (Table 5) compared to other studies (Maruya et al., 2012; Klosterhaus et al., 2013; Long et al., 2013; Beretta et al., 2014). However, with the exception of DEET, concentrations of all detected PPCPs declined quickly with increasing distance from the outfall, and stations close to the outfall were all higher than at the PB reference station. Future monitoring will determine whether the DEET observation was an anomaly. In 2014, only List 3 compounds (Table S3) were analyzed from samples collected at M0, AH2, AH1, FC1, and FC2. From this round of sampling, only triclosan and triclocarban were detected at M0; all other 2014 results were below detection limits (for full results of the 2014 sediment see Table S10). Triclosan and triclocarban were also the only two compounds that exhibited statistically significant (p-value < 0.05) declining trends in wastewater concentrations over the 2014 to 2016 sampling period (section Post-2014 Quarterly Wastewater Sampling). Because sediment samples were only collected once at each station, we cannot tell whether the wastewater declines resulted in concurrent sediment concentration declines. Regardless, the spatial pattern of higher concentrations at M0 remains (Figure 4) even though the M0 stations were sampled after (September 2014) the other stations (September 2010). The CRD plans to include some PPCP analyses in sediment as part of the routine outfall monitoring program to assess changes in concentrations over space and time.
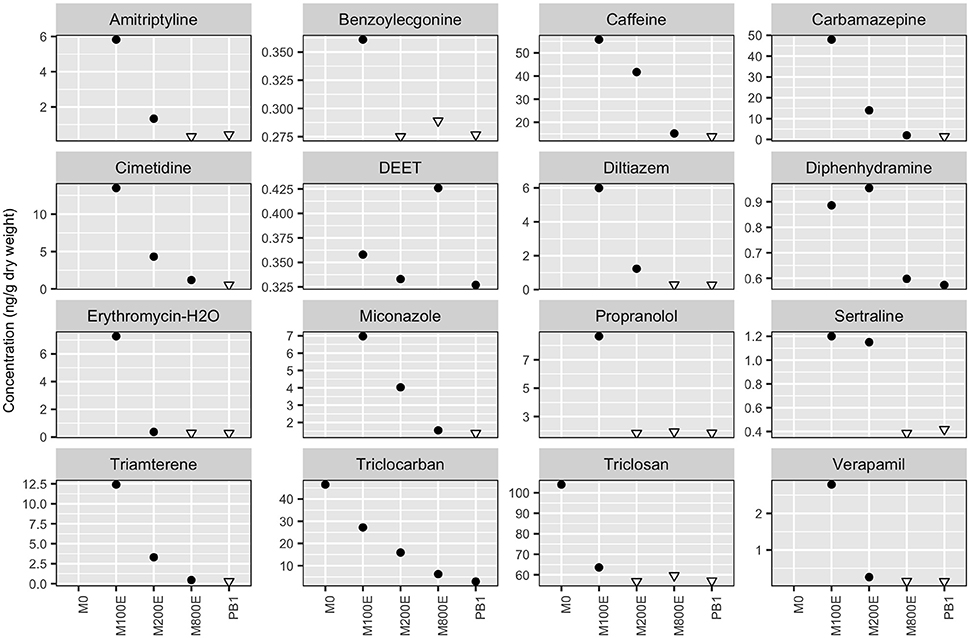
Figure 4. Detection (marked with filled circles) of PPCP's in the sediment surrounding the MAC outfall. Distance between the outfall and the station increase toward the right side of the plots. Only List 3 compounds were analyzed for at M0, from which only triclocarban and triclosan were detected. Open downward facing triangles indicate sample specific detection limits where no detection occurred.
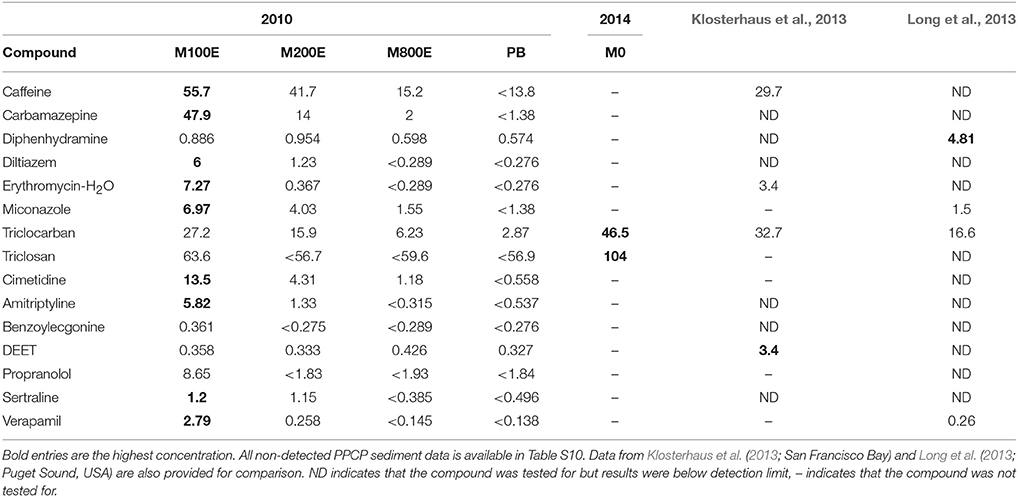
Table 5. Concentrations (ng g−1 dry weight) of detected PPCPs in sediments (single samples) near the MAC outfall.
The spatial pattern of PPCP sediment contamination generally matches that of PCBs, PBDEs and other compounds monitored as part of the CRD's routine sediment monitoring program (Golder Associates Ltd., 2008; Dinn et al., 2012). However, PPCP contamination does seems to fall faster with increasing distance than other compounds (such as metals and POPs); this is possibly a result of greater rates of environmental degradation. The most highly impacted areas around the outfall correspond closely with high benthic biota abundance and low diversity (CRD, 2015).
Clover Point Horse Mussels
Clover Point Horse Mussel tissue PPCP concentrations (single sample results; reported on a dry weight basis; Table 6) were higher than those observed in wild marine organisms elsewhere (Dodder et al., 2014; Arpin-Pont et al., 2016; Meador et al., 2016). However, most concentrations fell quickly with distance from the CLO outfall diffuser, similar to the MAC PPCP sediment patterns. Interestingly, the veterinary antibiotic virginiamycin M1 was not detected in CLO effluent (<64.6 ng L−1), but was detected in CLO mussel tissues with a contamination pattern opposite to the remaining PPCPs: the highest concentration of virginiamycin M1 was observed in the CB reference station samples (87.1 ng g−1 dry weight), while the lowest concentrations were measured near the CLO sewage outfall (67.4 ng g−1 dry weight). Sulfanilamide, an antibacterial agent, was only detected at the CB reference station (134 ng g−1), and not in CLO effluent or at the other two Horse Mussel stations. Although surprising, these results are similar to observations made in Puget Sound where virginiamycin M1 was not detected in wastewater effluent or seawater, but was present in both salmon and sculpin tissue at up to 34 ng g−1 wet weight (Meador et al., 2016). These results suggest that there may be in-situ sources of virginiamycin M1 within marine biota. Table S11 shows bioaccumulation factors from CLO effluent and the tissue chemistry from C0.
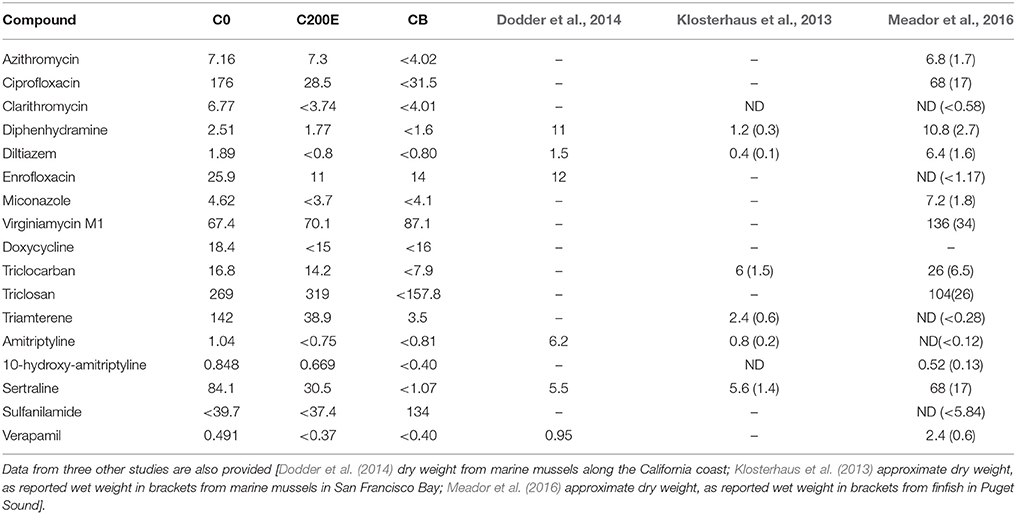
Table 6. Tissue concentrations (single sample results, ng g−1 dry weight) from Horse Mussels collected near the CLO outfall.
Homogenized Benthic Tissue and Blue Mussel Tissue
Blue mussel and biota samples collected from Victoria Harbor, Albert Head, and Finnerty Cove showed little PPCP contamination. Only naproxen (15.6 ng g−1 dry weight, AH1 biota, Figure 1) and triclocarban (10.2 g g−1, dry weight, VH1 blue mussels, Figure 1) were detected with all other List 3 compounds below detection limit (full results presented in Table S9). For VH1, these largely non-detect PPCP results stand in contrast to the heavy contamination of these harbor sediment with metals, PAHs and PCBs from over 100 years of industrial activity (UMA Engineering Morrow Environmental Consultants, 2007).
Conclusions
Without advanced wastewater treatment, PPCP concentrations within the MAC and CLO wastewaters are some of the highest reported for municipal sewage (Lishman et al., 2006; Meador et al., 2016). Seasonal patterns, due to flow volume dilution changes, in PPCP wastewater concentrations were only significant for naproxen at MAC. Additional seasonal patterns may be observed if wastewater sampling frequency was increased.
Due to the high energy of the marine environment, receiving environment contamination was highly variable. Very near the sewage outfalls (<200 m), environmental concentrations within sediment and resident Horse Mussel tissue were high, sometimes exceeding maximum concentrations observed elsewhere in the natural environment (Dodder et al., 2014; Arpin-Pont et al., 2016; Meador et al., 2016) but not necessarily those observed at heavily anthropogenic areas. However, within the IDZ of both outfalls, water column contamination was minimal with only three PPCPs being detected. Beyond 200 m from the outfalls, sediment PPCP concentrations were similar to those observed near other sewage treatment plant outfalls (Dodder et al., 2014; Meador et al., 2016). Likewise, nearly all reference stations (>5 km from the outfalls) had no detectable PPCPs within the sediment or benthic biota samples. Blue mussel tissue samples collected within Victoria Harbor had detectable concentrations of the antimicrobial triclocarban but no other List 3 (Table S3) compounds were detected.
The statistically significant declines in the concentrations of triclosan and triclocarban indicated that the phase out of these antimicrobial agents is occurring; continued monitoring will reveal if these declines continue. Between 2009, when triclosan and triclocarban were first measured at CLO and MAC, and the end of 2016 concentrations had fallen by approximately two-thirds.
Greater Victoria has started building a tertiary sewage treatment plant with planned operation by 2020. Once the plant is operational, PPCP concentrations within the treated effluent and within the initial dilution zones are expected to be significantly reduced. With time, this reduction of inputs is expected to result in reduced concentrations both within the sediments and biological tissue surrounding both outfalls. Monitoring the pace of this recovery could serve as a natural laboratory on the ultimate fate and environmental residence time of these compounds in marine sediments and biota. Upcoming monitoring efforts include the continuation of PPCP sampling within biota including fin-fish and crabs.
Author Contributions
JK: Primary author, crafted the main structure of the manuscript, conducted data analysis, constructed figures; SL: Primary collector of field samples, assisted in the gathering and organization of past data, provided input and edits to the manuscript; CL: Secondary collector of field samples, provided analysis of all 2009 data and gave significant input and edits to the manuscript.
Funding
All funding for this study was provided by the Capital Regional District's Marine Programs.
Conflict of Interest Statement
The authors declare that the research was conducted in the absence of any commercial or financial relationships that could be construed as a potential conflict of interest.
The reviewer MIUD and handling Editor declared their shared affiliation.
Acknowledgments
Vancouver Aquarium (Peter Ross, Carmen Morales and Marie Noel), Biologica (Tara Macdonald), Ecostat Research (Brenda Burd), and the three reviewers for their feedback and suggestions.
Supplementary Material
The Supplementary Material for this article can be found online at: https://www.frontiersin.org/articles/10.3389/fmars.2017.00415/full#supplementary-material
References
Arpin-Pont, L., Bueno, M. J., Gomez, E., and Fenet, H. (2016). Occurrence of PPCPs in the marine environment: a review. Environ. Sci. Pollut. Res. 23, 4978–4991. doi: 10.1007/s11356-014-3617-x
Ban, N. C., Alidina, H. M., and Ardron, J. A. (2010). Cumulative impact mapping: Advances, relevance and limitations to marine management and conservation, using Canada's Pacific waters as a case study. Mar. Policy 34, 876–886. doi: 10.1016/j.marpol.2010.01.010
Benotti, M. J., and Brownawell, B. J. (2007). Distributions of pharmaceuticals in an urban estuary during both dry- and wet-weather conditions. Environ. Sci. Technol. 41, 5795–5802. doi: 10.1021/es0629965
Beretta, M., Britto, V., Tavares, T. M., da Silva, S. M. T., and Pletsch, A. L. (2014). Occurrence of pharmaceutical and personal care products (PPCPs) in marine sediments in the todos os santos bay and the north coast of Salvador, Bahia, Brazil. J. Soils Sediments 14, 1278–1286. doi: 10.1007/s11368-014-0884-6
Cantwell, M. G., Katz, D. R., Sullivan, J. C., Ho, K., and Burgess, R. M. (2017). Temporal and spatial behavior of pharmaceuticals in Narragansett Bay, Rhode Island, United States. Environ. Toxicol. Chem. 36, 1846–1855. doi: 10.1002/etc.3710
CRD (2015). Macaulay and Clover Point Wastewater and Marine Environment Program 2014 Annual Report. Capital Regional District, Environmental Services Department, Victoria, BC.
Daughton, C. G., and Ternes, T. A. (1999). Pharmaceuticals and personal care products in the environment: agents of subtle change? Environ. Health Perspect. 107, 907–938. doi: 10.1289/ehp.99107s6907
Dinn, P. M., Johannessen, S. C., Macdonald, R. W., Lowe, C. J., and Whiticar, M. J. (2012). Effect of receiving environment on the transport and fate of polybrominated diphenyl ethers near two submarine municipal outfalls. Environ. Toxicol. Chem. 31, 566–573. doi: 10.1002/etc.1735
Dodder, N. G., Maruya, K. A., Lee Ferguson, P., Grace, R., Klosterhaus, S., La Guardia, M. J., et al. (2014). Occurrence of contaminants of emerging concern in mussels (Mytilus spp.) along the California coast and the influence of land use, storm water discharge, and treated wastewater effluent. Mar. Pollut. Bull. 81, 340–346. doi: 10.1016/j.marpolbul.2013.06.041
Environment and Climate Change Canada (2012). Municipal Wastewater Treatment Indicator. Available online at: https://www.ec.gc.ca/indicateurs-indicators/default.asp?lang=En&n=2647AF7D-1
Environment and Climate Change Canada (2016). Risk Management Approach for Phenol, 5-chloro-2-(2,4-dichlorophenoxy) (Triclosan) Chemical Abstracts Service Registry Number:3380-34-5. Available online at: http://www.ec.gc.ca/ese-ees/371A2F3C-9ACB-46E3-B7D6-33E2FC7F2DAD/TriclosanRMApproach-EN-2016-Nov-15-clean.pdf
European Environmental Agency (2013). European Environmental Agency Urban Waste Water Treatment. Available online at: https://www.eea.europa.eu/data-and-maps/indicators/urban-waste-water-treatment/urban-waste-water-treatment-assessment-3
Everage, T. J., Boopathy, R., Nathaniel, R., LaFleur, G., and Doucet, J. (2014). A survey of antibiotic-resistant bacteria in a sewage treatment plant in Thibodaux, Louisiana, USA. Int. Biodeterior. Biodegradation 95, 2–10. doi: 10.1016/j.ibiod.2014.05.028
Fatta-Kassinos, D., Meric, S., and Nikolaou, A. (2011). Pharmaceutical residues in environmental waters and wastewater: current state of knowledge and future research. Anal. Bioanal. Chem. 399, 251–275. doi: 10.1007/s00216-010-4300-9
Flint, S., Markle, T., Thompson, S., and Wallace, E. (2012). Bisphenol a exposure, effects, and policy: a wildlife perspective. J. Environ. Manage. 104, 19–34. doi: 10.1016/j.jenvman.2012.03.021
Food and Drug Administration, HHS. (2016). Safety and effectiveness of consumer response antiseptics- topical antimicrobial drug products for over-the-counter human use. Fed. Regist. 81, 61106–61130. Available online at: https://www.gpo.gov/fdsys/pkg/FR-2016-09-06/pdf/2016-21337.pdf
Gaw, S., Thomas, K. V., and Hutchinson, T. H. (2014). Sources, impacts and trends of pharmaceuticals in the marine and coastal environment. Philos. Trans. R. Soc. Lond. Series B Biol. Sci. 369:20130572. doi: 10.1098/rstb.2013.0572
Golder Associates Ltd. (2008). Final Report on 2007 Benthic Macroinvertebrate Analyses and Multi-year Trend Assessment for the Macaulay Point Outfall. Report No. 08-1421-0025. Golder Associates Ltd., Burnaby, BC.
Hodgins, D. (2009). The C3-UM and Sediment Modelling System and Their Implementation for the Capital Regional District. Salt Spring Island, BC: Seaconsult Marine Research.
Kasprzyk-Hordern, B., Dinsdale, R. M., and Guwy, A. J. (2009). The removal of pharmaceuticals, personal care products, endocrine disruptors and illicit drugs during wastewater treatment and its impact on the quality of receiving waters. Water Res. 43, 363–380. doi: 10.1016/j.watres.2008.10.047
Klosterhaus, S. L., Grace, R., Hamilton, M. C., and Yee, D. (2013). Method validation and reconnaissance of pharmaceuticals, personal care products, and alkylphenols in surface waters, sediments, and mussels in an urban estuary. Environ. Int. 54, 92–99. doi: 10.1016/j.envint.2013.01.009
Kostich, M. S., Batt, A. L., and Lazorchak, J. M. (2014). Concentrations of prioritized pharmaceuticals in effluents from 50 large wastewater treatment plants in the US and implications for risk estimation. Environ. Pollut. 184, 354–359. doi: 10.1016/j.envpol.2013.09.013
Krogh, J. (2017). Dissolved Oxygen and Inorganic Carbon Dynamics in a High-Energy Coastal Environment Near Victoria BC's Untreated Municipal Sewage Outfalls. Master's thesis. Victoria: University of Victoria. Available online at: http://hdl.handle.net/1828/8023
Kurata, Y., Ono, Y., and Ono, Y. (2008). Occurrence of phenols in leachates from municipal solid waste landfill sites in Japan. J. Mat. Cycles Waste Manage. 10, 144–152. doi: 10.1007/s10163-008-0200-x
Lishman, L., Smyth, S. A., Sarafin, K., Kleywegt, S., Toito, J., Peart, T., et al. (2006). Occurrence and reductions of pharmaceuticals and personal care products and estrogens by municipal wastewater treatment plants in Ontario, Canada. Sci. Total Environ. 367, 544–558. doi: 10.1016/j.scitotenv.2006.03.021
Long, E. R., Dutch, M., Weakland, S., Chandramouli, B., and Benskin, J. P. (2013). Quantification of pharmaceuticals, personal care products, and perfluoroalkyl substances in the marine sediments of Puget Sound, Washington, USA. Environ. Toxicol. Chem. 32, 1701–1710. doi: 10.1002/etc.2281
Lowe, C. J. (2011). Pharmaceuticals, Personal Care Products, Illicit Drugs and Their Metabolites in Screened Municipal Wastewaters. Master's thesis. Victoria: University of Victoria. Available online at: http://hdl.handle.net/1828/4964
Luo, Y., Guo, W., Ngo, H. H., Nghiem, L. D., Hai, F. I., Zhang, J., et al. (2014). A review on the occurrence of micropollutants in the aquatic environment and their fate and removal during wastewater treatment. Sci. Total Environ. 473–474, 619–641. doi: 10.1016/j.scitotenv.2013.12.065
Manaia, C. M. (2014). Antibiotic resistance in wastewater: origins, fate, and risks. Prävention Gesundheitsförderung 9, 180–184. doi: 10.1007/s11553-014-0452-3
Markovic, D. L. (2003). Untreated Municipal Sewage Discharge in Victoria Bight, British Columbia, Canada: An Investigation of Sediment Metal Contamination and Implications for Sustainable Development. Master's thesis. Victoria: Royal Roads University.
Maruya, K. A., Vidal-Dorsch, D. E., Bay, S. M., Kwon, J. W., Xia, K., and Armbrust, K. L. (2012). Organic contaminants of emerging concern in sediments and flatfish collected near outfalls discharging treated wastewater effluent to the Southern California Bight. Environ. Toxicol. Chem. 31, 2683–2688. doi: 10.1002/etc.2003
Meador, J. P., Yeh, A., Young, G., and Gallagher, E. P. (2016). Contaminants of emerging concern in a large temperate estuary. Environ. Pollut. 213, 254–267. doi: 10.1016/j.envpol.2016.01.088
Nakada, N., Tanishima, T., Shinohara, H., Kiri, K., and Takada, H. (2006). Pharmaceutical chemicals and endocrine disrupters in municipal wastewater in Tokyo and their removal during activated sludge treatment. Water Res. 40, 3297–3303. doi: 10.1016/j.watres.2006.06.039
Ort, C., Lawrence, M. G., Reungoat, J., and Mueller, J. F. (2010a). Sampling for PPCPs in wastewater systems: comparison of different sampling modes and optimization strategies. Environ. Sci. Technol. 44, 6289–6296. doi: 10.1021/es100778d
Ort, C., Lawrence, M. G., Rieckermann, J., and Joss, A. (2010b). Sampling for pharmaceuticals and personal care products (PPCPs) and illicit drugs in wastewater systems: are your conclusions valid? a critical review. Environ. Sci. Technol. 44, 6024–6035. doi: 10.1021/es100779n
Ringuette, J. (2009). City of Gardens was once a City of Garbage, Beacon Hill Park History. Available online at: http://www.beaconhillparkhistory.org/articles/122_garbage.htm
Rizzo, L., Manaia, C., Merlin, C., Schwartz, T., Dagot, C., Ploy, M. C., et al. (2013). Urban wastewater treatment plants as hotspots for antibiotic resistant bacteria and genes spread into the environment: a review. Sci. Total Environ. 447, 345–360. doi: 10.1016/j.scitotenv.2013.01.032
Rodea-Palomares, I., Gonzalez-Pleiter, M., Gonzalo, S., Rosal, R., Leganes, F., Sabater, S., et al. (2016). Hidden drivers of low-dose pharmaceutical pollutant mixtures revealed by the novel GSA-QHTS screening method. Sci. Adv. 2:e1601272. doi: 10.1126/sciadv.1601272
Saunders, L. J., Mazumder, A., and Lowe, C. J. (2016). Pharmaceutical concentrations in screened municipal wastewaters in Victoria, British Columbia: a comparison with prescription rates and predicted concentrations. Environ. Toxicol. Chem. 35, 919–929. doi: 10.1002/etc.3241
Statistics Canada (2012). Focus on Geography Series, 2011 Census. Statistics Canada Catalogue no. 98-310-XWE2011004. Ottawa, ON: Statistics Canada.
Suárez, S., Carballa, M., Omil, F., and Lema, J. M. (2008). How are pharmaceutical and personal care products (PPCPs) removed from urban wastewaters? Rev. Environ. Sci. Biotechnol. 7, 125–138. doi: 10.1007/s11157-008-9130-2
Sumpter, J. P., and Jobling, S. (2013). The occurrence, causes, and consequences of estrogens in the aquatic environment. Environ. Toxicol. Chem. 32, 249–251. doi: 10.1002/etc.2084
Sun, Q., Li, M., Ma, C., Chen, X., Xie, X., and Yu, C. P. (2016). Seasonal and spatial variations of PPCP occurrence, removal and mass loading in three wastewater treatment plants located in different urbanization areas in Xiamen, China. Environ. Pollut. 208, 371–381. doi: 10.1016/j.envpol.2015.10.003
UMA Engineering and Morrow Environmental Consultants. (2007). Ecological and Human Health Risk Assessment of Transport Canada Administered & Controlled Harbour Floor in Upper Victoria Harbour. Report prepared for Transport Canada Victoria & Esquimalt Harbours Environmental Program.
Vajda, A. M., Barber, L. B., Gray, J. L., Lopez, E. M., Woodling, J. D., and Norris, D. O. (2008). Reproductive disruption in fish downstream of an estrogenic wastewater effluent reproductive disruption in fish downstream from an estrogenic wastewater effluent. Environ. Sci. Technol. 42, 3407–3414. doi: 10.1021/es0720661
Verenitch, S. S., Lowe, C. J., and Mazumder, A. (2006). Determination of acidic drugs and caffeine in municipal wastewaters and receiving waters by gas chromatography-ion trap tandem mass spectrometry. J. Chromatogr. A 1116, 193–203. doi: 10.1016/j.chroma.2006.03.005
Vidal-Dorsch, D. E., Bay, S. M., Maruya, K., Snyder, S. A., Trenholm, R. A., and Vanderford, B. J. (2012). Contaminants of emerging concern in municipal wastewater effluents and marine receiving water. Environ. Toxicol. Chem. 31, 2674–2682. doi: 10.1002/etc.2004
Weigel, S., Berger, U., Jensen, E., Kallenborn, R., Thoresen, H., and Hühnerfuss, H. (2004). Determination of selected pharmaceuticals and caffeine in sewage and seawater from Tromsø/Norway with emphasis on ibuprofen and its metabolites. Chemosphere 56, 583–592. doi: 10.1016/j.chemosphere.2004.04.015
Keywords: PPCP, sewage, sediment, benthic biota, Victoria BC, mussels
Citation: Krogh J, Lyons S and Lowe CJ (2017) Pharmaceuticals and Personal Care Products in Municipal Wastewater and the Marine Receiving Environment Near Victoria Canada. Front. Mar. Sci. 4:415. doi: 10.3389/fmars.2017.00415
Received: 02 October 2017; Accepted: 01 December 2017;
Published: 18 December 2017.
Edited by:
Juan Jose Alava, University of British Columbia, CanadaReviewed by:
Victoria Otton, Simon Fraser University, CanadaMiguel Ignacio Uyaguari-Diaz, University of British Columbia, Canada
Monica F. Costa, Universidade Federal de Pernambuco, Brazil
Copyright © 2017 Krogh, Lyons and Lowe. This is an open-access article distributed under the terms of the Creative Commons Attribution License (CC BY). The use, distribution or reproduction in other forums is permitted, provided the original author(s) or licensor are credited and that the original publication in this journal is cited, in accordance with accepted academic practice. No use, distribution or reproduction is permitted which does not comply with these terms.
*Correspondence: Christopher J. Lowe, cjlowe@uvic.ca; clowe@crd.bc.ca