- 1Laboratorio de Eco-Fisiología Animal, Instituto de Investigaciones sobre los Recursos Naturales, Universidad Michoacana de San Nicolás de Hidalgo, Morelia, Mexico
- 2Departamento de Biología Celular y Fisiología, Instituto de Investigaciones Biomédicas Sede I, Universidad Nacional Autónoma de México, Ciudad de Mexico, Mexico
Egg translocation and incubation in man-made nests (MMN) are common conservation practices through marine turtle hatcheries worldwide. These measures have been associated with reduced hatching rates, altered hatchling sex ratio, fetal dysmorphic anatomical features, and feeble hatchlings health. Previous studies have shown that MMN and natural nests (NN) provide different incubatory conditions. Therefore, incubatory challenges imposed by MMN conditions on fetal development could induce stress responses affecting hatchlings functional morphology later on life. There is no evidence of incubatory stress associated with conservation measures in turtle fetuses or hatchlings. Thus, in this paper we tested the hypothesis that MMN incubation exposes turtle fetuses to stressing conditions. Given that the hypothalamic-pituitary-interrenal axis begins functioning by day 11 of incubation in reptiles, our experiments explored the effects of incubatory conditions, rather than those associated with translocation, on fetal stress responses. We showed that Lepidochelys olivacea hatchlings incubated in MMN displayed reduced body weight, hypertrophic inter-renal glands, testicular hypotrophy and hypotrophic dorso-medial cortical pyramidal neurons, when compared with hatchlings emerging from NN. Furthermore, MMN hatchlings had higher serum levels of corticosterone at emergence, and displayed an attenuated acute stress response after traversing the beach. Therefore, the relocation of nests to protect them could negatively impact the health and survival of sea turtles. Thus, this action should only be undertaken when no alternative is available.
Introduction
Sea turtle populations have been declining through the last century (Sarti et al., 2007; Seminoff and Shanker, 2008). Several conservation strategies aimed at reversing these trends are currently in practice (Eckert et al., 1999). The creation of hatcheries where turtle eggs are translocated from natural nests (NN) and incubated in man-made nests (MMN) is one of the main strategies currently used. Hatcheries protect turtle eggs from poaching, predation, and beach erosion, factor all that could reduce marine turtles' reproductive success (Seminoff et al., 2003; Abreu-Grobois and Plotkin, 2008). However, egg translocation to and incubation in MMN is associated with reduced hatching and emergence success (Limpus et al., 1979; Parmenter, 1980; Eckert and Eckert, 1990; McElroy et al., 2015; Ahles and Milton, 2016), weak hatchling health (Maulany et al., 2012), misbalance sex ratio (Pintus et al., 2009), and increased frequency of fetal dysmorphic anatomical features (Sönmez et al., 2011). Although the precise mechanism underlying these negative outcomes is unclear, it is possible that mechanical, humidity, thermal and microbiological conditions (Chan et al., 1985; Booth and Astill, 2001; Arzola-González, 2007; Tuttle and Rostal, 2010; DeGregorio and Southwood, 2011; Patino-Martinez et al., 2012) in MMN could induce fetal incubatory stress responses.
Stress is a physiological response triggered by stimuli that challenge body homeostasis (Chrousos and Gold, 1992). During the stress response, the hypothalamic-pituitary-adrenal (HPA) axis releases catecholamines and gluco-corticoids into the blood stream. Metabolic adjustments (e.g., increased glucose serum availability) follow the rise of these hormones (Chrousos and Gold, 1992; Tsigos and Chrousos, 2002). Under acute circumstances, all these adjustments subside in a matter of minutes to few hours (McEwen, 2000; Tsigos and Chrousos, 2002), and the organism recovers its homeostatic state (McEwen, 2000). However, when the organism is chronically challenged, it develops sustained, chronic allostatic stress responses, which predisposes the organism to develop different functional and morphological disorders (Avitsur and Sheridan, 2009). This is especially true when exposure to stressing conditions occurs during prenatal development (Kapoor and Matthews, 2008).
Hence, in the present study, we evaluated whether L. olivacea hatchlings produced by eggs translocated and incubated in MMN had functional and morphological signs of stress at emergence, suggesting their exposure to stressing conditions during fetal life. Given that the hypothalamic-pituitary-interrenal (HPI) axis in turtles begins functioning between days 11 and 15 of incubation (Kuntz, 1912; Pearson et al., 1983; Milano, 1991; Jenkins and Porter, 2004), it is probable that our results predominantly reflect the effects of MMN incubatory conditions, rather than effects associated with egg translocation.
Materials and Methods
Study Site and Nesting Conditions
L. olivacea hatchlings were collected and sampled during the 2013 reproductive season. NN hatchlings had an average of 47.66 ± 1.52 incubation days by the time of emergence. MMN hatchlings had an average of 53.5 ± 2.42 incubation days by the time of emergence. All nests were built through the beach “Barra de Pichi” in Lázaro Cárdenas, Michoacán, México (Supplementary Figure 1); MMN hatchlings emerged in the hatchery “La Tortuga” (17°58′23.86″ N; 102°19′24.63″ W; 7 feet altitude; Google earth image @2016 Digital globe @Google; Supplementary Figure 2). Egg translocation and reburial in MMN were performed by hatchery staff and followed protocols recommended by the Mexican official norm (NOM-162-SEMARNAT-2011, http://dof.gob.mx/nota_detalle_popup.php?codigo=5233078). Briefly, hatchery personnel patrolled the beach at night to identify nesting turtles. Once the nesting female was spotted, staff carefully removed the sand and collected the entire clutch after oviposition was completed and the turtle had left the nest; eggs were placed in plastic bags and transported to the hatchery. The complete clutch was reburied with wet sand followed by dry sand. At all times, efforts were made to avoid egg rotation and major mechanical perturbations. Egg translocation and reburial took no more than 2 h. Natural incubation occurred, on the other hand, near the hatchery, 80 m away from the tide line (Supplementary Figure 2). Staff personnel patrolled the beach to locate nesting females. The staff circle-fenced the nesting site using a wire-made meshwork, after the females had left the nest.
Hatchlings Collection
All hatchlings used for the histological and/or the biochemical analyses at emergence or at sea arrival were collected from the same nests.
Seventeen hatchlings from NN (n = 3) and 17 hatchlings from MMN (n = 3) were captured for conducting the anatomical evaluation of the brain, gonads and inter-renal glands, and corticosterone measurements in serum samples at emergence (Supplementary Table 1). During specimen collection, hatchlings were randomly taken, one by one, at 5-min intervals, as they surfaced. Hatchlings were weighed on a roman tubular precision scale, measured using a digital Vernier (snout-vent and carapace lengths) and decapitated. The blood was individually collected in microfuge tubes. Then, the heart was exposed by surgically removing the pectoral scutes; additional blood samples were withdrawn by direct heart puncturing. In the meantime, a second researcher dissected the brains and placed them into light protected vials containing the Golgi's solution (see below). Samples were kept at ambient temperature until further processing. Finally, the rest of the plastron was removed and the inter-renal glands and the gonads were dissected from the abdominal cavity and placed in vials containing buffered paraformaldehyde (4%) and kept at ambient temperature until reaching the laboratory where they were stored at 4°C.
To estimate corticosterone serum levels at sea arrival, we captured 18 hatchlings from NN (n = 3) and 10 hatchlings from MMN (n = 2) (Supplementary Table 1). Hatchlings used for estimating corticosterone serum levels at sea arrival were also taken one by one, transferred to a common departing site located 20 m away from the shoreline, but close to the nest they emerged. Once placed in the “start” site, they were set free to crawl toward the sea. As soon as they reached the ocean shore, they were re-captured, weighted, measured, decapitated and bled as previously described.
Animal handling, sacrifice, and sampling protocols were all approved by the SEMARNAT's Animal Rights Committee, under the License Number SGPA/DGVS/05459/13; Supplementary Presentation 1, and in full accordance to the Mexican Official Norm, NOM-033-ZOO-1995 for humanitarian sacrifice of domestic and wild animals. The comparisons were made by evaluators blind to the experimental conditions.
Corticosterone Serum Levels
Serum samples were obtained after centrifuging the coagulated blood during 15 min at 2,000 rpm and ambient temperature using a portable microfuge (Eppendorf). The samples were stored in liquid nitrogen and, once in the laboratory, were kept frozen at −80°C until further processing. Costicosterone levels were measured using an enzyme-linked immunoabsorbent assay (ALPCO, 55-CORMS-E01), following the manufacturer's instructions. The intra-assay variation coefficient was 2.8–8.3% and the assay sensitivity was 4.1 ng/mL.
Histological Procedures
Inter-renal glands and gonads were weighted with an analytical micro-balance (Mettler Toledo, MX, Max 5.1 g d = 1 μg), embedded in paraffin through conventional methods and sliced (5 μm) using a microtome. Sections were mounted onto gelatin-coated slides, de-waxed, hydrated, and stained with hematoxylin-eosin (Merck). Finally, slides were dehydrated, xylene-cleared and cover-slipped using Cytoseal 60 (Richard Alan Scientific).
The brains, on the other hand, were rapidly dissected, weighed and processed immediately for Golgi impregnation following the manufacturer's instructions (Golgi Staining Kit II Neurotechniques Inc). The brains were cut through the coronal plane (200 μm) using a cryostat. Sections were mounted onto gelatin-coated slides, air-dried, xylene-cleared, and cover-slipped.
Quantitative Organ Cyto-Morphology
Inter-renal Glands
Twelve longitudinal sections (5 μm) per turtle were randomly sampled along each inter-renal gland. Steroid-producing cells per animal (Ray and Maiti, 2001) were counted in all sections and their nuclear area estimated in digital photomicrographs taken at x1,000 (Leica DM3000); cell counts and nuclear tracing were performed manually using the NIH ImageJ software. Cell density was estimated by dividing the number of cell nuclei on the total area sampled.
Testes
As shown in Supplementary Table 1, histological evaluation of the gonads showed that most of the hatchlings captured and sampled happened to be males. Our observation does not necessarily mean that there is a male predominance in all nests analyzed given that in our study (1) hatchling sampling number per nest was small, (2) we did not estimate sex ratio per nest, (3) hatchlings capturing was arbitrary, and (4) capturing only lasted approximately an hour. In addition, several factors may explain male predominance in our samples, including that female hatchlings pip at a slower pace than male ones (Mahmoud et al., 2005), that marine turtles nest emergence is asynchronous (Houghton and Hays, 2001), and that nest emergence is dependent on the development of a time sense by the hatchlings (Salmon and Raising, 2014); this varies among individuals. Therefore, our hypothesis was evaluated in males because they were more numerous.
Two longitudinal slices (5 μm) were taken approximately from the middle section of the gonad and were randomly and digitally photographed at x400 using a Leica DM3000 microscope. The circularity index of the seminiferous cords was estimated with the aid of ImageJ. The 50 cords with the highest circularity index were selected. The average circularity index for hatchlings emerging from NN was 0.990 ± 0.01, and for hatchlings emerging from MMN was 0.991 ± 0.01. The average testicular epithelial cell number per seminiferous cord was then manually counted in both groups of hatchlings using x400 digital images and the ImageJ software.
Neurons
Dorso-medial pyramidal neurons (n = 10 per turtle) were manually drawn with the aid of a camera Lucida adapted to a bright field Leica microscope. Neuron drawings were then scanned and digitized. Dendritic complexity was evaluated using Sholl's analyses (Shankaranarayana Rao et al., 2001); concentric rings were separated from each other by 10 μm intervals. The number of intersections between dendritic processes and concentric rings was counted. In addition, the area of neuronal cell bodies was estimated by digitally creating a mask of each soma that later was automatically measured with the aid of ImageJ.
Statistical Analyses
Data of all variables in both animal groups were normally distributed and had variance homogeneity, with the exception of the number of dendritic intersections (Kolmogorov–Smirnov tests, α > 0.05).
Nested analysis of variance (ANOVA) were conducted to compare all variables (with the exception of serum levels of corticosterone and number of dendritic intersections) among treatments, and to evaluate variability among groups within treatments. For conducting these analyses, we used a generalized lineal model (GLM) that nested hatchlings groups within treatments.
To compare serum corticosterone levels at different times (emergence/sea arrival) and between treatments, a nested ANOVA was conducted nesting groups within sampling condition (at emergence and at sea arrival) or treatments. Additionally, a Fisher LSD multiple comparison post hoc tests with 95% (α ≤ 0.05) confidence was carried out to identify significant differences among groups.
Finally, we also used a nested ANOVA to compare the number of dendritic intersections between hatchling groups. The number of dendritic intersections was then nested within groups and treatments, using a generalized non-lineal model (GNLM) with a Poisson's distribution and a Log function. Statistica 7 software was used to perform all analyses. Means and standard errors are presented.
Results
Macroscopic Measures
MMN hatchlings displayed significantly lower body weights (15.84 + 0.43 g) than NN hatchlings [17.02 ± 0.17 g. Nested ANOVA F(1, 30) = 6.341, *p = 0.017; Supplementary Figure 3A]. In contrast, other parameters were similar, including:
a) Body length [Nested ANOVA F(1, 30) = 1.97, p = 0.171. MMN 66.76 ± 0.42 and NN 67.48 ± 0.59 cm. Supplementary Figure 3B],
b) Carapace length [MMN, 42.35 ± 0.40 and NN 42.76 ± 0.34 cm] and width [MMN 35.06 ± 0.25 and NN 34.38 ± 0.37 cm],
c) Plastron length [MMN 32.71 ± 0.36 and NN 33.02 ± 0.32 cm] and width [MMN 29.40 ± 0.41 and NN 29.16 ± 0.55 cm],
d) Brain weight [MMN, 11.20 ± 0.55 and NN, 10.61 ± 0.51 mg],
e) Interrenal gland weight [MMN 6.5 ± 0.92 and NN, 7.83 ± 0.77 mg].
In addition, MMN hatchlings had significantly lighter testicles (1.95 ± 0.19 mg) than NN hatchlings [2.79 ± 0.36 mg. Nested ANOVA F(1, 18) = 4.59, *p = 0.046].
Glucocorticoid-Producing Cells
Qualitative observations showed that MMN hatchlings display glucocorticoid producing cells with hypertrophied cytoplasm (compare Figures 1A,B). This explained why these cells' nuclear density was significantly reduced in MMN hatchlings (1,582 ± 69.10 cells/mm2) with respect to NN turtles [1993.69 ± 136.88 cells/mm2; Nested ANOVA F(1, 28) = 7.77, *p = 0.009, Figure 1C]. In addition, glucocorticoid producing cells had significantly larger nuclei (33.42 ± 1.43 μm2) than those observed in NN hatchlings [29.50 ± 0.87 μm2, ANOVA F(1, 28) = 11.14, *p = 0.002, compare Figure 1A and Figure 1B; also Figure 1D].
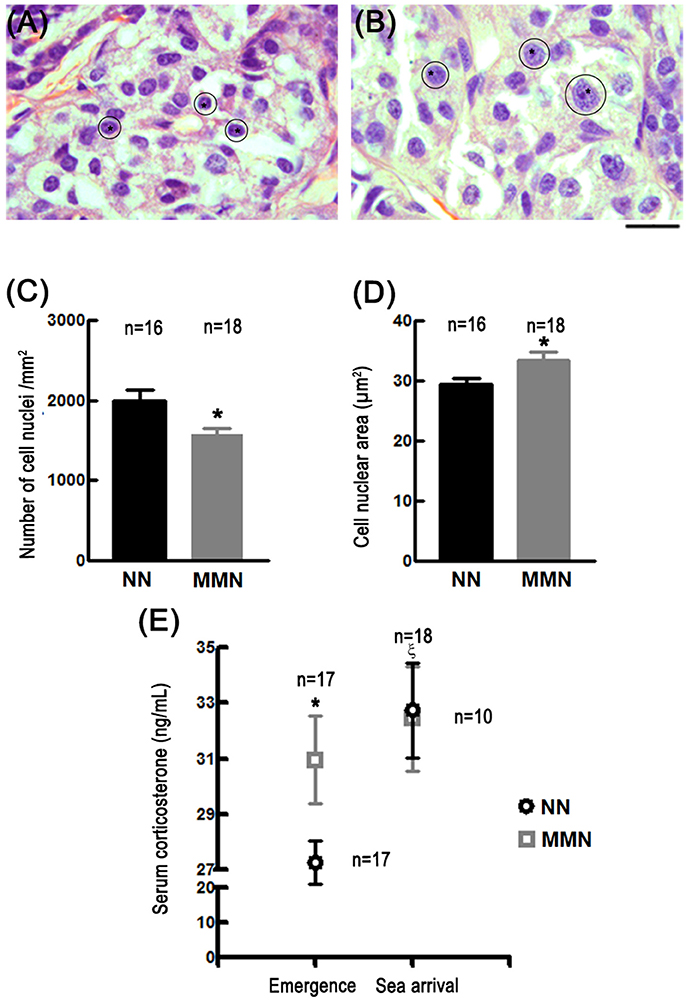
Figure 1. Photomicrographs of histological sections stained with hematoxylin-eosin showing glucocorticoid-producing cells in the interrenal gland of hatchlings that emerged from NN (A) or MMN (B). Notice the enlarged cell nuclei (asterisks and outlines) and hypertrophied cytoplasm in MMN hatchlings. Since lipids were extracted during histological processing, the cytoplasm is observed as a white space surrounding the cell nuclei. Scale bar = 20 μm. Bar graphs that show the nuclear cell density [C; Nested ANOVA F(1, 28) = 7.77, *p = 0.009] and cell nuclear area [D; Nested ANOVA F(1, 28) = 11.14, *p = 0.002] of glucocorticoid-producing cells in NN and MMN hatchlings. (E) Graph showing corticosterone serum levels in hatchlings from NN or MMN at nest emergence and at sea arrival [Nested ANOVA F(2, 51) = 5.58, p = 0.006]. At emergence, serum basal levels were significantly higher in MMN than in NN hatchlings (Fisher LSD means comparison test, *p = 0.044); no differences were observed between groups at sea arrival. At sea arrival, the magnitude of the evoked release of corticosterone was significantly lower MMN than in NN hatchlings (intragroup comparisons; Fisher LSD means comparison test, ξp = 0.003).
Corticosterone Serum Levels
At emergence, corticosterone serum levels in MMN hatchlings were significantly higher (30.96 ± 1.57 ng/mL) than those estimated in NN hatchlings (27.27 ± 0.79 ng/mL, Fisher LSD means comparison test, *p = 0.044, Figure 1E).
HPA axis over-activation may be inferred by exposing already stressed organisms to additional stressful challenges (Jones and Bell, 2004). Under these circumstances, a discrete increase in corticosterone must be observed in chronically stressed organisms since they already have augmented basal levels of serum corticosterone. This is precisely what happened when MMN hatchlings were subjected to a stressful challenge. Indeed, at the sea arrival, the increase in corticosterone serum levels was significantly reduced in MMN hatchlings (32.43 + 1.44 ng/mL, Fisher LSD means comparison test, p = 0.479, Figure 1E) as compared to their NN counterparts (32.75 + 1.87 ng/mL, Fisher LSD means comparison test, ξp = 0.03, Figure 1E).
Testicular Cytology
Qualitative observations showed that MMN hatchlings have reduced numbers of epithelial cells in the seminiferous cords and a poorly developed testicular stroma, as compared to NN hatchlings (Figures 2A,B). Quantitative analyses confirm this impression [MMN: 7.22 ± 0.25 cells and NN: 8.39 ± 0.41 cells per seminiferous cord, nested ANOVA F(1, 23) = 4.844, *p = 0.038, Figure 2C].
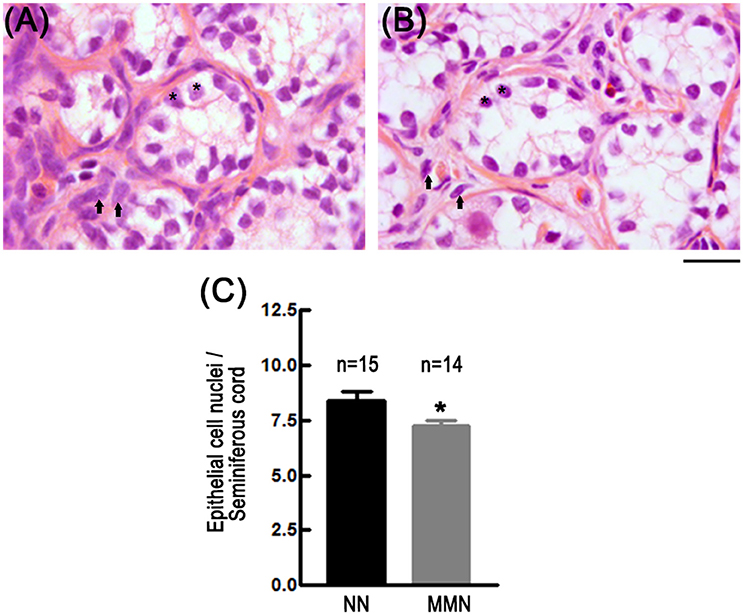
Figure 2. Photomicrographs of histological sections showing epithelial cells of seminiferous cords in NN (A) and MMN (B) hatchlings. Notice that testicles of MMN hatchlings have hypotrophic epithelial (*) and interstitial cells (arrows). The connective tissue surrounding seminiferous tubules is more compact in NN than in MMN hatchlings. Hematoxylin-Eosin staining. Scale bar = 20 μm. (C) Bar graph that depicts epithelial cell number per seminiferous cord in NN and MMN hatchlings [Nested ANOVA F(1, 23) = 4.84, *p = 0.038].
Morphology of Pyramidal Neurons
Neurons in the dorso-medial cortex of MMN hatchlings showed a reduced cell area [Nested ANOVA F(1, 13) = 11.998, *p = 0.004, Figures 3A,B] and decreased dendritic arbor complexity [Nested ANOVA Wald = 539.900, *p < 0.001, Figure 3C] when compared to those observed in NN hatchlings.
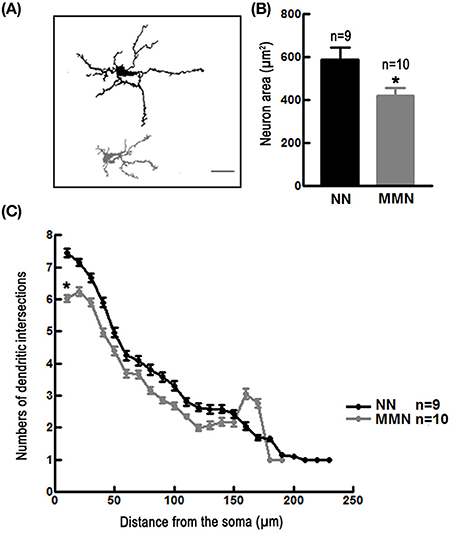
Figure 3. (A) Representative camera Lucida drawings of Golgi-impregnated pyramidal neurons located in the dorso-medial cortex of an NN (upper panel) and of an MMN (lower panel) hatchling. Scale bar = 100 μm. (B) Bar graph depicting the average total area of pyramidal neurons located in the dorso-medial cortex of hatchlings that emerged from NN or MMN [Nested ANOVA F(1, 13) = 11.99, *p = 0.004]. (C) Graph depicting the average number of dendritic intersections relative to the distance from the geometric center of the cell soma of pyramidal neurons of the dorso-medial cortex in NN and MMN hatchlings [Nested ANOVA Wald = 539.90, p < 0.001].
Discussion
Incubation in MMN is frequently associated with reduced emergence rate, misbalanced sex ratio, weaker hatchling health, and increased frequency of congenital malformations. It is possible that MMN exposes turtles to an incubatory environment that induces stress in developing fetuses. It has been reported that MMN humidity, temperature (e.g., Reece et al., 2002; Arzola-González, 2007) possibly and microbiological (Bézy et al., 2014) conditions differ from those recorded for NN. In this study, we conducted preliminary experiments that explored whether MMN incubatory conditions induce stress in fetal turtles. Our results support this hypothesis. At emergence, MMN L. olivacea hatchlings displayed, lower body weight, hypertrophied glucocorticoid producing cells, hypotrophic testicles, and hypotrophic dorso-medial cortical pyramidal neurons, as well as increased basal corticosterone serum levels. In addition, after crawling through the beach, MMN hatchlings showed an attenuated rise of corticosterone levels. In conjunction, these results suggest that MMN hatchlings developed a stress response during incubation. Because HPI axis begins functioning by day 11 of incubation, it is possible that the functional morphological changes observed in MMN hatchlings predominantly reflect stress induced by incubatory conditions, rather than by translocation. We cannot rule out, however, the possibility that egg translocation could have conditioned HPI axis development through epigenetic mechanisms before the 11th day of incubation. This issue could be addressed by removing eggs from sham NN, holding them out for the time taken to relocate a nest, and finally placing them back into the same sham NN to complete their incubation. If, under these circumstances, hatchlings produced by sham NN had similar functional morphological attributes to those observed in NN hatchlings, it may be concluded that incubatory environment stresses turtle embryonic/fetal development. If, on the other hand, sham NNs and MMNs hatchlings were similar to one another, but differ from NN hatchlings, then the differences among groups could be attributed to translocation. Future studies must be performed to assess both possibilities.
A frequent finding reported in mammals is that gestational stress decreases fetal growth rate (Brunton and Russell, 2010; Brunton, 2013), a circumstance that leads to reduced body weight at birth. In mammals, stress-associated fetal growth retardation commonly results from prenatal undernourishment due to placental insufficiency, a process mediated by epigenetic mechanisms (Leigh, 2010). Turtles are not placental animals, and most of the energy used by fetuses to grow is allocated in the yolk (Hewavisenthi and Parmenter, 2002; Venkatesan et al., 2005). In fact yolk mass, with some contribution of egg mass as an estimation mainly of hydrated albumin mass, correlate positively with hatchlings weight at emergence (Wallace et al., 2006). Therefore, it is possible that the reduced body weight observed in MMN hatchlings at emergence may result from stress-related loss of yolk and egg/albumin mass. However, we did not measure yolk mass at emergence and/or egg mass at laying. Our results do not include egg mass because we wanted to leave NN fully undisturbed to reduce as much as possible the potential sources of stress. Hence, future experiments must evaluate whether MMN incubation decreases yolk and egg size and/or if it shifts their biochemical composition. It will be also useful to study expression and methylation patterns of genes that are involved in modulating fetal growth (e.g., hepatocyte growth factor; Kawashima-Ohya et al., 2011). In spite of these limitations, the differential effects (and the absence of them; e.g., carapace length) that MMN incubatory conditions had on various morphological parameters and on corticosterone serum levels still support the general inference of this work, namely, MNN promotes stress in turtle fetuses.
In mammals, individuals subjected to prenatal stress may develop altered behavior (e.g., aggressiveness, depression; Kapoor and Matthews, 2008; Leigh, 2010), reproductive dysfunction (e.g., infertility; Kapoor and Matthews, 2008), metabolic syndrome (Antti-Jussi et al., 2010), immune depression (Martin, 2009), and even degenerative diseases that affect various organ systems (e.g., cardiovascular) at some point of their lives (Engler, 1995). Prenatal stress also reprograms and imprints gamete genome (Chehreie et al., 2013), thus passing diseased states to the progeny through transgenerational epigenetic inheritance (Jablonka and Raz, 2009; Leigh, 2010; Daxinger and Whitelaw, 2012; Heard and Martienssen, 2014). It is thus possible that worldwide hatcheries release numerous specimens that may be carrying heritable, non-adaptive traits. Although in this work, we did not provide evidence in favor of this possibility, the fact that MMN hatchlings showed testicular hypotrophy and a decreased number of epithelial cells in the seminiferous cords support this possibility. In this regard, one may think that relocation not into a designated hatchery, but to sites closer to dunes may prevent stress-associated, gamete genome reprogramming and imprinting. It is known, nonetheless, that incubatory conditions differ significantly at different location of the same beach (e.g., Bézy et al., 2014). In spite of uncertainties, and based upon our results, it is probable that some of the current conservation practices may have a negative impact on adult marine turtle survival and reproductive success in the long term. Thus, this action should only be undertaken when no alternative is available.
Lastly, the present observations are also significant with regard to other conservation activities conducted by the attending personnel, at least in Mexican hatcheries. Staff -retain hatchlings soon after emergence for several hours (Van de Merwe et al., 2013). This practice provides the opportunity for hatchery managers to raise money by charging a fee to attend hatchling release events. Unfortunately, it also furthers post-emergence stress during the frenzy stage (Wyneken and Salmon, 1992), a critical period in which hatchlings try to avoid predation both on land and in the sea (Pilcher and Enderby, 2001; Burgess et al., 2006; Ischer et al., 2009; Van de Merwe et al., 2013). Based on our results, we recommend that hatchery managers be advised to stop this potentially harmful practice.
Author Contributions
MH-V, FB-P, AB-S, and HS-C: carried-out experiments and analyzed data; MH-V, EM-H, GG-O, and AF-F: interpreted results and wrote the manuscript; EM-H, GG-O, and AF-F: designed experiments and obtained funding; All authors reviewed and approved the final version manuscript.
Funding
This work was supported by grants from Consejo Nacional de Ciencia y Tecnologí-a (CONACYT no. 180762 to EM-H and no. 258747 to AF-F). Additional funding was provided by grants from Coordinación de la Investigación Científica to EM-H and AF-F. MH-V and FB-P are Ph.D. and M.S. fellows from CONACYT (CVU 257690 and 738354).
Conflict of Interest Statement
The authors declare that the research was conducted in the absence of any commercial or financial relationships that could be construed as a potential conflict of interest.
Acknowledgments
Authors thank to Dr. Jesús Ramírez, Biol. Hugo Olivera Rodríguez, Biol. Edel Pineda López and Saúl González de la Luz for valuable technical assistance.
Supplementary Material
The Supplementary Material for this article can be found online at: https://www.frontiersin.org/articles/10.3389/fmars.2017.00400/full#supplementary-material
References
Abreu-Grobois, A., and Plotkin, P. (IUCN SSC Marine Turtle Specialist Group) (2008). Lepidochelys olivacea. The IUCN Red List of Threatened Species 2008:e.T11534A3292503. doi: 10.2305/IUCN.UK.2008.RLTS.T11534A3292503.en
Ahles, N., and Milton, S. L. (2016). Mid-incubation relocation and embryonic survival in Loggerhead sea turtle eggs. J. Wildl. Manage. 80, 430–437. doi: 10.1002/jwmg.1023
Pyykkönen A, J., Räikkönen, K., Tuomi, T., Eriksson, J. G., Groop, L., and Isomaa, B. (2010). Stressful life events and the metabolic syndrome. Diabetes Care 33, 378–384. doi: 10.2337/dc09-1027
Arzola-González, J. F. (2007). Humedad y temperatura en nidos naturales y artificiales de tortuga golfina Lepidochelys olivacea (Eschssholtz 1829). Rev. Biol. Mar. Oceanogr. 42, 377–383. doi: 10.4067/S0718-19572007000300017
Avitsur, R., and Sheridan, J. F. (2009). Neonatal stress modulates sickness behavior. Brain. Behav. Immun. 23, 977–985. doi: 10.1016/j.bbi.2009.05.056
Bézy, S. V., Roldán, A. V., and Craig, J. P. (2014). Olive ridley sea turtle hatching success as a function of microbial abundance and the microenvironment of in situ nest sand at Ostional, Costa Rica. J. Mar. Biol. 2014:351921. doi: 10.1155/2014/351921
Booth, D. T., and Astill, K. (2001). Temperature variation within and between nests of the green sea turtle, Chelonia mydas (Chelonia: Cheloniidae) on Heron Island, Great Barrier Reef. Aust. J. Zool. 49, 71–84. doi: 10.1071/ZO00059
Brunton, P. J. (2013). Effects of maternal exposure to social stress during pregnancy: consequences for mother and offspring. Reproduction 146, 175–189. doi: 10.1530/REP-13-0258
Brunton, P. J., and Russell, J. A. (2010). Prenatal social stress in the rat programmer neuroendocrine and behavioral responses to stress in the adult offspring: sex specific effects. J. Neuroendocrinol. 22, 258–271. doi: 10.1111/j.1365-2826.2010.01969.x
Burgess, E. A., Booth, D. T., and Lanyon, J. M. (2006). Swimming performance of hatchling green turtles is affected by incubation temperature. Coral Reefs 25, 341–349. doi: 10.1007/s00338-006-0116-7
Chan, E. H., Salleh, H. D., and Liew, H. C. (1985). Effects of handling on hatchability of eggs of the leatherback turtle, Dermochelys coriacea (L.). Pertanika 8, 265–271.
Chehreie, S., Rabzia, A., and Farhadi-Mesterkhani, M. (2013). Maternal water deprivation affects the spermatogenesis of the offspring rats. Int. J. Morphol. 31, 156–161. doi: 10.4067/S0717-95022013000100026
Chrousos, P. G., and Gold, P. W. (1992). The concepts of stress and stress system disorders, overview of physical and behavioral homeostasis. JAMA 256, 1244–1252. doi: 10.1001/jama.1992.03480090092034
Daxinger, L., and Whitelaw, E. (2012). Understanding transgenerational epigenetic inheritance via the gametes in mammals. Nat. Rev. Genet. 13, 153–162. doi: 10.1038/nrg3188
DeGregorio, B. A., and Southwood, A. W. (2011). Incubation temperatures and metabolic heating of relocated and in situ longgerhead sea turtle (Caretta caretta) nests at a Northern Rookery. Chelonian Conserv. Biol. 10, 54–61. doi: 10.2744/CCB-0880.1
Eckert, K. L., Bjorndal, K. A., Abreu-Grobois, F. A., and Donnelly, M. (1999). Research and Management Techniques for the Conservation of Sea Turtles. SSC/IUCN Marine Turtle Specialist Group Publication No 4. Pennsylvania, PA: Blanchard Press.
Eckert, K. L., and Eckert, S. A. (1990). Embryo mortality and hatch success in “in situ” and translocated leatherback sea turtle (Dermochelys coriacea) eggs. Biol. Cons. 53, 37–46. doi: 10.1016/0006-3207(90)90061-S
Engler, M. B. (1995). Assessment of the cardiovascular effects of stress. J. Cardiovasc. Nurs. 10, 51–63. doi: 10.1097/00005082-199510000-00005
Heard, E., and Martienssen, R. A. (2014). Transgenerational epigenetic inheritance: myths and mechanisms. Cell 157, 95–109. doi: 10.1016/j.cell.2014.02.045.NI
Hewavisenthi, S., and Parmenter, C. J. (2002). Egg components and utilization of yolk lipid during development of the flatback turtle Natatur depressus. J. Herpetol. 36, 43–50. doi: 10.1670/0022-1511(2002)036[0043:ECAUOY]2.0.CO;2
Houghton, J. D., and Hays, G. C. (2001). Asynchronous emergence by loggerhead turtle (Caretta caretta) hatchlings. Naturwissenschaften 88, 133–136. doi: 10.1007/s001140100212
Ischer, T., Ireland, K., and Booth, D. T. (2009). Locomotion performance of green turtle hatchlings from the Heron Island Rookery, Great Barrier Reef. Mar. Biol. 156, 1399–1409. doi: 10.1007/s00227-009-1180-7123
Jablonka, E., and Raz, G. (2009). Transgenerational epigenetic inheritance: prevalence, mechanisms, and implications for the study of heredity and evolution. Q. Rev. Biol. 84, 131–176. doi: 10.1086/598822
Jenkins, S. A., and Porter, T. E. (2004). Ontogeny of the hypothalamo–pituitary–adrenocortical axis in the chicken embryo: a review. Domest. Anim. Endocrinol. 26, 267–275. doi: 10.1016/j.domaniend.2004.01.001
Jones, M. S., and Bell, K. (2004). Plasma corticosterone concentrations in males of the skink Egernia whitii during acute and chronic confinement, and over a diel period. Comp. Biochem. Physiol. A 137, 105–113. doi: 10.1016/S1095-6433(03)00267-8
Kapoor, A., and Matthews, S. G. (2008). Prenatal stress modifies behavior and hypothalamic-pituitary-adrenal function in female guinea pig offspring: effects of timing of prenatal stress and stage of reproductive cycle. Endocrinology 149, 6406–6415. doi: 10.1210/en.2008-0347
Kawashima-Ohya, Y., Narita, Y., Nagashima, H., Usuda, R., and Kuratani, S. (2011). Hepatocyte growth factor is crucial for development of the carapace in turtles. Evol. Dev. 13, 260–268. doi: 10.1111/j.1525-142X.2011.00474.x
Kuntz, A. (1912). The development of the adrenal in the turtle. Dev. Dyn. 13, 71–89. doi: 10.1002/aja.1000130105
Leigh, H. (2010). “How does stress work? The role of memes in epigenesist,” in Genes, Memes, Culture, and Mental Illness, Toward an Integrative Model, ed H. Leigh (San Francisco, CA: Springer Press), 11–22.
Limpus, C. J., Baker, V., and Miller, J. D. (1979). Movement induced mortality of loggerhead eggs. Herpetologica 35, 335–338.
Mahmoud, I. Y., Alkidini, A. Y., Ba-Omar, T. A., Al-Siyabi, S., Al-Bahry, S. N., Elshafie, A. Q., et al. (2005). Emergence pattern of the green turtle, Chelonia mydas, hatchlings under laboratory and natural conditions. Zool. Middle East 35, 19–28. doi: 10.1080/09397140.2005.10638099
Martin, L. B. (2009). Stress and immunity in wild vertebrates: timing is everything. Gen. Comp. Endocrinol. 163, 70–76. doi: 10.1016/j.ygcen.2009.03.008
Maulany, R. I., Booth, D. T., and Baxter, G. S. (2012). The effect of incubation temperature on hatchling quality in the olive ridley turtle, Lepidochelys olivacea, from Alas Purwo National Park, East Java, Indonesia: implications for hatchery management. Mar. Biol. 159, 2651–2661. doi: 10.1007/s00227-012-2022-6
McElroy, M., Dodd, M. G., and Catleberry, S. B. (2015). Effects of common loggerhead sea turtle nest management methods on hatching and emergence success at Sapelo Island, Georgia, USA. Chelonian Conserv. Biol. 14, 49–55. doi: 10.2744/ccab-14-01-49-55.1
McEwen, S. B. (2000). The neurobiology of stress: from serendipity to clinical relevance. Brain Res. 886, 172–189. doi: 10.1016/S0006-8993(00)02950-4
Milano, E. G. (1991). Development of the adrenal gland in amniotes: a comparison between chelonians and birds. Boll. Zool. 58, 205–209. doi: 10.1080/11250009109355753
Parmenter, C. J. (1980). Incubation of the eggs of the green sea turtle, Chelonia mydas, in Torres Strait, Australia: the effect of movement on hatchability. Aust. Wildl. Res. 7, 487–491. doi: 10.1071/WR9800487
Patino-Martinez, J., Marco, A., Qui-oñes, L., Abella, E., Abad, R. M., and Diéguez-Uribeondo, J. (2012). How do hatcheries influence embryonic development of sea turtle eggs? Experimental analysis and isolation of microorganisms in Latherback turtle eggs. J. Exp. Zool. 317, 47–54. doi: 10.1002/jez.719
Pearson, A. K., Wurst, G. Z., and Cadle, J. E. (1983). Ontogeny and immunocytochemical differentiation of the pituitary gland in a sea turtle, Caretta caretta. Anat. Embryol. 167, 13–37. doi: 10.1007/BF00304598
Pilcher, N. J., and Enderby, S. (2001). Effects of prolonged retention in hatcheries on green turtle (Chelonia mydas) hatchling swimming speed and survival. J. Herpetol. 35, 633–638. doi: 10.2307/1565902
Pintus, K. J., Godley, B. J., McGowan, A., and Broderick, A. C. (2009). Impact of clutch relocation on green turtle offspring. J. Wildl. Manage 73, 1151–1157. doi: 10.2193/2008-103
Ray, P. P., and Maiti, B. R. (2001). Adrenomedullary hormonal and glycemic responses to high ambient temperature in the soft-shelled turtle, Lissemys punctata punctata. Gen. Comp. Endocrinol. 22, 17–22. doi: 10.1006/gcen.2001.7606
Reece, S. E., Broderick, A. C., Godley, B. J., and West, S. A. (2002). The effects of incubation environment, sex and pedigree on the hatchling phenotype in a natural population of loggerhead turtles. Evol. Ecol. Res. 4, 737–748. Available online at: http://citeseerx.ist.psu.edu/viewdoc/download?doi=10.1.1.571.9060&rep=rep1&type=pdf
Salmon, M., and Raising, M. (2014). Emergence rhythms of hatchling marine turtles: is a time sense involved? Chelonian Conserv. Biol. 13, 282–285. doi: 10.2744/CCB-1121.1
Sarti, S. L., Barragán, R. A., García, M. D., García, N., Huerta, P., and Vargas, F. (2007). Conservation and biology of the Leatherback turtle in the Mexican Pacific. Chelonian Conserv. Biol. 6, 70–78. doi: 10.2744/1071-8443(2007)6[70:CABOTL]2.0.CO;2
Seminoff, J. A., Jones, T. T., Reséndiz, P. A., Wallace, J. N., and Chaloupka, M. Y. (2003). Monitoring green turtles (Chelonia mydas) at a coastal foraging area in Baja California, Mexico: multiple indices describe population status. J. Mar. Biol. 83, 1355–1362. doi: 10.1017/S0025315403008816
Seminoff, J. A., and Shanker, K. (2008). Marine turtles and IUCN red listing: a review of the process, the pitfalls, and novel assessment approaches. J. Exp. Mar. Biol. Ecol. 356, 52–68. doi: 10.1016/j.jembe.2007.12.007
Shankaranarayana Rao, B. S., Govindaiah, Laxmi, T. R., Meti, B. L., and Raju, T. R. (2001). Subicular lesions cause dendritic atrophy in CA1 and CA3 pyramidal neurons of the rat hippocampus. Neuroscience 102, 319–327. doi: 10.1016/S0306-4522(00)00462-0
Sönmez, B., Cemal, T., and Sükran, Y. Ö. (2011). The effect of relocation on the morphology of Green Turtle, Chelonia mydas (Linnaeus, 1758), hatchlings on Samandag beach, Turkey. Zool. Middle East 52, 29–38. doi: 10.1080/09397140.2011.10638476
Tsigos, C., and Chrousos, P. G. (2002). Hypothalamic-pituitary-adrenal axis, neuroendocrine factors and stress. J. Psichosom. Res. 53, 865–871. doi: 10.1016/S0022-3999(02)00429-4
Tuttle, J., and Rostal, D. (2010). Effects of nest relocation on nest temperature and embryonic development of Loggerhead sea turtles (Caretta caretta). Chelonian Conserv. Biol. 9, 1–7. doi: 10.2744/CCB-0769.1
Van de Merwe, J. P., Ibrahim, K., and Whittier, J. M. (2013). Post-emergence handling of green turtle hatchlings: improving hatchery management worldwide. Anim. Conserv. 16, 316–323. doi: 10.1111/j.14691795.2012.00603.x
Venkatesan, S., Kannan, P., Rajagopalan, M., and Vivekanandan, E. (2005). Embryonic energetics in the egg of the green turtle Chelonia mydas. J. Mar. Biol. Ass. India 47, 193–197. Available online at: http://eprints.cmfri.org.in/2060/
Wallace, B. P., Sotherland, P. R., Tomillo, P. S., Bouchard, S. S., Reina, R. D., Spotila, J. R., et al. (2006). Egg components, egg size, and hatchling size in leatherback turtle. Comp. Biochem. Physiol. A Mol. Integr. Physiol. 145, 524–532. doi: 10.1016/j.cbpa.2006.08.040
Keywords: hypothalamic-pituitary-adrenal axis, fetal development, artificial nesting, Lázaro Cárdenas Michoacán, reproductive success, hatcheries, conservation, early-life stress
Citation: Herrera-Vargas MA, Meléndez-Herrera E, Gutiérrez-Ospina G, Bucio-Piña FE, Báez-Saldaña A, Siliceo-Cantero HH and Fuentes-Farías AL (2017) Hatchlings of the Marine Turtle Lepidochelys olivacea Display Signs of Prenatal Stress at Emergence after Being Incubated in Man-Made Nests: A Preliminary Report. Front. Mar. Sci. 4:400. doi: 10.3389/fmars.2017.00400
Received: 30 June 2017; Accepted: 24 November 2017;
Published: 08 December 2017.
Edited by:
Wen-Cheng Wang, National Taiwan Normal University, TaiwanReviewed by:
David Terrington Booth, The University of Queensland, AustraliaGail Schofield, Deakin University, Australia
Mariana M. P. B. Fuentes, Florida State University, United States
Copyright © 2017 Herrera-Vargas, Meléndez-Herrera, Gutiérrez-Ospina, Bucio-Piña, Báez-Saldaña, Siliceo-Cantero and Fuentes-Farías. This is an open-access article distributed under the terms of the Creative Commons Attribution License (CC BY). The use, distribution or reproduction in other forums is permitted, provided the original author(s) or licensor are credited and that the original publication in this journal is cited, in accordance with accepted academic practice. No use, distribution or reproduction is permitted which does not comply with these terms.
*Correspondence: Esperanza Meléndez-Herrera, esperanzamelendezherrera@hotmail.com
Alma L. Fuentes-Farías, almafuentes70@hotmail.com