- 1Applied Ecology and Phycology, Institute of Biological Sciences, University of Rostock, Rostock, Germany
- 2Animal Physiology, Institute of Biological Sciences, University of Rostock, Rostock, Germany
Wooden groin systems on the southwestern Baltic Sea coast are a traditional and important coastal-protection facility, but have been regularly infested and destroyed by the wood-boring bivalve Teredo navalis since the early 1990s. The occurrence of T. navalis was presumed to be limited mainly by the prevailing low salinities. Recently, a possible range expansion of this invasive species to the more eastern parts of the Baltic Sea has been discussed. T. navalis larval settlement was therefore monitored at the distribution boundary of the species in the Baltic Sea over a period of 4 years. At 7 stations along the prevailing salinity gradient on the Mecklenburg-western Pomeranian coast, larval traps were installed at regular time intervals, while at the same time water temperature and salinity were measured continuously every hour. Correlations between measured abiotic parameters and borehole abundance of T. navalis were tested. For the German Baltic Sea coast, no range expansion of T. navalis was confirmed. The salinity and temperatures at the groin systems varied among the study years, and significant correlations between T. navalis borehole abundance and salinity as well as temperature were found. Higher summer temperatures favor the T. navalis borehole abundance on the Mecklenburg-western Pomeranian coast, and may slightly shift the distribution border of this species toward lower salinities.
Introduction
Wooden groins have been an important and common coastal-protection measure in the Mecklenburg-western Pomeranian section of the Baltic Sea coast for 150 years. Traditionally, local pinewood was used until the beginning of the 1990s. At that time, the invasive shipworm Teredo navalis Linnaeus 1758 (Bivalvia: Teredinidae) established stable reproductive populations in the southern Baltic Sea, and since then has regularly destroyed the wooden coastal and harbor infrastructure (Sordyl et al., 1998). However, it remains unknown which factors led to the permanent establishment of this shipworm species on the Mecklenburg-western Pomeranian Baltic Sea coast. Periodic mass outbreaks of T. navalis in the Baltic Sea were reported previously from Denmark, Sweden, and Germany, during the 1930s and 1950s, each lasting for only a few years (Bavendamm and Schmidt, 1948; Nakel, 1954; Hahn, 1956; Schütz, 1961). However, since the 1990s pinewood groins have had to be renewed regularly at intervals of 5–10 years, compared to 30–40 years previously (Michael Bugenhagen, StALU-MM: Staatliches Amt für Landwirtschaft und Umwelt Mittleres Mecklenburg—Governmental Agency for Agriculture and Environment Central Mecklenburg-western Pomerania, personal communication). Information on the resulting maintenance costs is sparse; Wichmann (2005) estimated expenses due to T. navalis infestations of about 50 million Euros in the Baltic Sea between 1993 and 2005. On the Mecklenburg-western Pomeranian coast, groins are today constructed mainly of tropical hardwoods, which are less attractive to shipworms. Even though the wood used is FSC-certified, the long transport distances may leave a negative ecological footprint. Furthermore, T. navalis is threatening wooden shipwrecks and other maritime-related structures of high archeological value (Björdal Gjelstrup et al., 2012). So far, most of these historical items are protected from shipworm attack because they are located in low-salinity waters that cannot be tolerated by this species. Recently, however, contradictory reports have appeared as to whether T. navalis is spreading into previously uninhabited areas, even into low-salinity waters (Hoppe, 2002; Tuente et al., 2002; Gollasch et al., 2009; Paalvast and van der Velde, 2011b; Borges, 2014; Appelqvist et al., 2015a).
Shipworms, members of the bivalve family Teredinidae, efficiently consume most kinds of wood in coastal habitats worldwide (Borges, 2014, and references therein). They bore into the wood physically by movements of their reduced shells, and digest the resulting wood particles with the aid of bacterial endosymbionts that produce cellulolytic enzymes and provide fixed nitrogen (Carpenter and Culliney, 1975; Distel et al., 2002). In European coastal waters, at least 9 shipworm species have been reported (Borges, 2014), although species identities are uncertain and molecular genetic characterization is incomplete (Borges et al., 2012; Weigelt et al., 2016). Based on a new COI (cytochrome c oxidase I) primer pair for identification by DNA barcoding, Weigelt et al. (2016) showed that T. navalis is presently the only teredinid species on the southwestern Baltic Sea coast. According to Borges et al. (2014) this species is one of the most destructive wood-borers worldwide.
In the Baltic Sea, the earliest known description of T. navalis dates to 1835, from Kiel Bay (Meyer and Möbius, 1865), and no scientific evidence exists that places T. navalis in the North Sea before 1730. The species, therefore, is classified as invasive in these waters (Leppäkoski and Olenin, 2000; Gollasch and Nehring, 2006). The distribution of T. navalis as a marine species depends to a large extent on the prevailing salinity conditions. The minimum salinity required for the survival of adult T. navalis is 5–6 (Blum, 1922; Nair and Saraswathy, 1971; Hoagland, 1986), and a maximum of 35 (Nair and Saraswathy, 1971) to 39 (Borges et al., 2014) is tolerated. Individuals show activity at salinities between 7 and 35 (Hoagland and Crocket, 1982; Spicer and Strömberg, 2003; Borges et al., 2014) and successful reproduction is presumed to occur above a salinity of 10 (Schütz, 1961; Soldatova, 1961; Sordyl et al., 1998; Tuente et al., 2002). Larvae survive salinities as low as 5 (Hoagland, 1986). Beside salinity conditions, however, temperature seems to play an important role for the destructive success of shipworms (Kristensen, 1969; Eckelbarger and Reish, 1972). Kristensen (1969), for example, found indications that high temperatures could compensate for low salinities within certain thresholds. The optimum temperature for growth and reproduction is between 15 and 25°C (Roch, 1932; Grave, 1942; Kristensen, 1979). Below 10°C metabolic activity decelerates and stops at temperatures below 5°C (Roch, 1932).
T. navalis is a protandric hermaphrodite species with planktonic veliger larvae. About 5 days after fertilization, the larvae are released into the water and remain there between 15 (Culliney, 1975) and 35 days (Schütz, 1961; Nair and Saraswathy, 1971), probably depending on the availability of wood. After settlement, the larvae metamorphose and bore into the wood quickly. Seven to 8 weeks after metamorphosis, at a length of 3–5 cm, the individuals can be sexually mature (Grave, 1942). Thus, under favorable conditions, more than one reproductive cycle per year is possible. This rapid sexual maturity of T. navalis and the very high reproductive potential, with several million larvae per year (Sordyl et al., 1998), allow wide dispersal by currents and efficient discovery of available wooden substrates.
The Baltic Sea is a semi-enclosed sea connected to the North Sea by two narrow and shallow straits, the Belt Sea and the Sound (Mohrholz et al., 2015). In the investigated area of the Baltic Sea a maximum tidal height of about 10 cm is estimated (Medvedev et al., 2016). However, changes of water level fluctuations driven by internal waves and air-pressure changes can be much higher and reach a maximum of 1.5 m annually in the Baltic Sea (Jerling, 1999). The average prevailing surface current along the southern Baltic Sea shoreline flows from west to east (http://www.stalu-mv.de/mm/Themen/Küstenschutz/Regelwerk-Küstenschutz-Mecklenburg-Vorpommern/). This aquatic ecosystem has horizontal and vertical salinity gradients. The salinity drops from 30–25 in the western parts (Belt Sea), to 8–15 in the southern parts (German Baltic Sea coastline) and even to fresh water in the northernmost part (Gulf of Bothnia). Inflows of saline waters from the North Sea occur only sporadically and sustain the brackish character of Baltic waters (Mohrholz et al., 2015). This limited water exchange with high-salinity waters from the Atlantic has a crucial influence on the environmental conditions in the Baltic Sea (Elken and Matthäus, 2008). Mainly, the salinity and oxygen supply affect the benthic community in the brackish-water system of the Baltic (Rönnberg and Bonsdorff, 2004). Due to the decreasing salinity, the number of marine species declines significantly in the southern Baltic and in the inner coastal waters (Zettler and Röhner, 2004; Bonsdorff, 2006). In the Mecklenburg-western Pomeranian part of the Baltic Sea coast, there is a horizontal salinity gradient between about 12 in the westernmost part and 7–8 around the island of Rügen. Since 1993, T. navalis has been permanently present in this area (Sordyl et al., 1998). For a long time it was assumed that the Darß Sill, with a salinity of around 10, was the easternmost distribution border for T. navalis in the Baltic Sea. In 1998, however, Sordyl et al. (1998) provided evidence for permanent T. navalis infestations east of the Darß Sill, at Zingst and on the western coast of the island of Hiddensee. They, however, did not detect T. navalis infestations farther east on the islands of Rügen and Usedom. Furthermore, Sordyl et al. (1998) found that the population was successfully reproducing, which had not been expected before due to the prevailing low salinities.
The mean Baltic Sea surface temperature from July to September has increased by 1.4°C between 1985 and 2000 (MacKenzie and Schiedeck, 2007). As mentioned above, the survival and distribution of T. navalis is mostly affected by salinity-temperature interactions (Kristensen, 1969; Borges, 2014). The rise in water temperature since 2000 has further increased by 0.6°C per decade; this trend will continue (Siegel and Gerth, 2016), and might thereby shift the eastern distribution border of this species farther. Since 1998, however, no investigation of the distribution of T. navalis on the Mecklenburg-western Pomeranian coast has been carried out and information on a potential range expansion, for example, to the island of Rügen, is lacking.
The particular abiotic conditions in the Baltic Sea, with a salinity gradient from west to east, provide a good opportunity to study T. navalis at its distribution border. Accordingly, for the first time in 17 years we systematically surveyed the occurrence of T. navalis in groin systems on the Mecklenburg-western Pomeranian coast over a period of 4 years, from 2012 to 2015. Temperature and salinity conditions in the shallow water areas investigated were simultaneously recorded, with high temporal resolution, to evaluate a potential expansion of the salinity and temperature range required by T. navalis. We also studied the start and duration of spat fall in relation to the spring temperature increase and summer temperatures. In 2014, a major intrusion of saline water from the North Sea to the Baltic occurred (Mohrholz et al., 2015). This provided an opportunity to investigate if these sporadic events improve the salinity conditions for T. navalis in the Mecklenburg-western Pomeranian part of the Baltic Sea.
Materials and Methods
Study Area and Larval Sampling
Along the Mecklenburg-western Pomeranian coastline of the Baltic Sea, seven locations were sampled between 2012 and 2015, from Boltenhagen (N53°59.877′, E11°11.722′) to the west and Glowe (N54°34.219′, E13°27.571′) on the island of Rügen to the east (Figure 1). Locations were chosen to assess the abundance of T. navalis boreholes and the time of larval settlement along the salinity gradient prevailing along this section of the Baltic Sea coast. The sampling sites mainly have a soft sandy bottom, partly interspersed with gravel and stones.
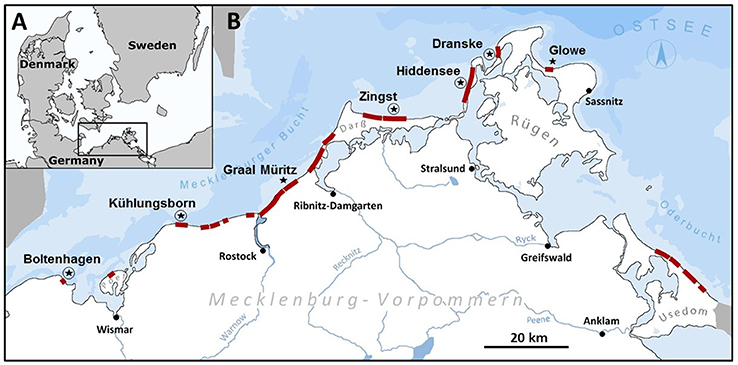
Figure 1. Sampling area: (A) Position of the sampling area on the southern Baltic Sea coast. (B) Sampling area: Red bars indicate groin systems in the sampling area. Stars mark sampling locations with only test panels, and stars with circles indicate sampling locations where test panels and data loggers were installed in combination.
Panels of unplaned pine wood (Pinus sylvestris, 10 × 20 × 2.5 cm), suitable as larval traps for teredinids (Borges et al., 2014), were attached to wooden pilings of the groin system by scuba divers, using cable ties. The panels were provided with a hole in each corner, at about 2.5 cm distance from the edges of the panel. Two cable ties (0.9 × 150 cm) (Figure 2) were drawn from the back side of the panel through one hole each, laid in parallel over the front surface of the panel, drawn through the opposite hole, and fixed to a groin piling. The cable ties covered 25 cm2 of the total panel surface of 200 cm2. Depending on the sampling site, the panels were placed at a depth between 1 and 3 m, about 0.5 m above the sea floor and distributed on three different rows of pilings in the respective groin system, with a distance of at least 50–70 m between the panels.
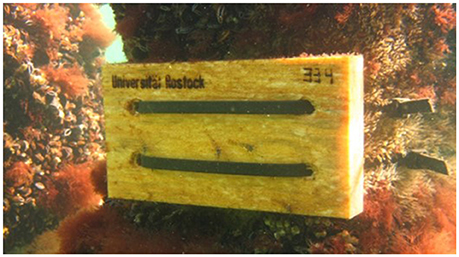
Figure 2. Larval trap: Representative pinewood panel attached to a groin in the shallow water of the southern Baltic Sea coast.
The total number of boreholes during one summer was quantified by exposing three larval traps per site each year from May to September or October. In total 84 panels were exposed for this purpose. To determine the time of larval settlement on the wood, three additional panels per site were exposed and replaced monthly between May and September or October, respectively. Between October and May, three panels per site were exposed to check for larvae arriving later in the year than October. This means that 504 panels were exposed and recovered to determine the time of larval settlement, giving a total number of 588 examined panels in the present investigation. After the defined exposure times, panels were recovered by scuba divers, gently cleaned of fouling organisms, blotted dry with tissue paper and air-dried for about 1 h. Next, the total number of boreholes on the panel front side, oriented toward the open sea water (~200 cm2), was counted using a stereomicroscope. On the panel front, sections were marked with a pencil. Each section was examined for boreholes, using a magnification of 6.7–10×. A typical borehole had a diameter of 400–700 μm. In 2013 and 2014, the density of boreholes at several sites was extremely high, making it impossible to count each hole separately. In these cases, the panel front was divided by a raster of 2.25 cm2. From this raster, 15 squares were randomly chosen and the boreholes in it were counted. The resulting number was extrapolated to the total surface of the sea water-exposed panel front.
According to Weigelt et al. (2016), T. navalis is the only teredinid species on the Mecklenburg-western Pomeranian Baltic Sea coast. In the present study, species identification of voucher specimens from all sampling locations was conducted by DNA barcoding as described by Weigelt et al. (2016). T. navalis was confirmed to be the only shipworm species in this area.
Abiotic Parameters
Salinity and temperature were measured hourly at three sites in 2012 (Boltenhagen, Zingst, Dranske) (Figure 1), at four sites in 2013 and 2015 (Boltenhagen, Kühlungsborn, Zingst, Dranske) and at five sites in 2014 (Hiddensee additionally). Data were obtained by autonomous Conductivity-Temperature Data Loggers (HOBO—U24-002, Onset Ltd., Cape Cod, Massachusetts, USA), attached to the groins by cable ties next to the wooden panels, about 0.5 m above the sea floor. According to the manufacturer, the specific-conductance accuracy for salinity measurements is about 3%, and that for temperature measurements ± 0.1°C. Although the data loggers were cleaned of fouling organisms every 4 weeks during the summer months, recolonization of the salinity sensor sometimes occurred rapidly and led to partial loss of data. All results were examined visually for consistency, and conspicuous data points that clearly indicated the influence of fouling were not used for further analysis. Temperature measurements were not affected by fouling.
Statistical Analysis
Abiotic data for the statistical analysis were collected at the Boltenhagen, Kühlungsborn, Zingst, Hiddensee, and Dranske stations. Borehole abundance data include all stations investigated. All statistical analyses were carried out with the statistical software R version 3.3.0 (R Development Core Team, 2009). Daily mean water temperature and salinity were calculated from the hourly data. To reveal significant differences in the parameters summer temperature (21 June to 20 September), salinity and T. navalis borehole abundance among sampling stations and years, a one-way Anova followed by the post-hoc Tukey HSD test was applied.
Significant correlations between borehole abundance at different sites and in different years and the environmental parameters water temperature and salinity were assessed by the Spearman's rank correlation coefficient. To rate the significance of the correlation, the p-value of the F-statistics was used. The following parameters were tested for significant correlation with borehole abundance: mean annual salinity, mean summer salinity and mean summer temperature, number of days with mean salinity below 8, number of days with mean salinity above 10 and above 12, number of days with mean temperature above 18°C, above 20°C and above 22°C. These parameters were chosen to reveal possible responses of T. navalis to certain salinity thresholds and to summer temperature peaks. Salinity of 8 is close to the assumed lower activity threshold of T. navalis (Hoagland and Crocket, 1982; Spicer and Strömberg, 2003), 10 and 12 are slightly above. Daily mean water temperatures above 20°C or even 22°C are not reached very often in the Baltic Sea during the course of the study, however higher temperature may favor the success of T. navalis in low salinity waters (Kristensen, 1969; Eckelbarger and Reish, 1972).
Results
Salinity and Temperature
Salinity conditions and water temperatures measured during the 4 years of investigation at different sites of the Mecklenburg-Western Pomeranian coast are shown in Figures 3, 4, and in Table 1. The stations follow the salinity gradient in the Baltic Sea and are significantly different in their mean salinity over the 4 years (ANOVA, p < 0.0005). The mean salinity over 4 years is plotted exemplarily for the stations Boltenhagen in the west and Dranske in the east of the sampling area (Figure 3). While at Boltenhagen, the salinity was mostly above 10 and reached values up to 17 during the winter months, at Dranske salinity ranged between 8 and 10 most of the time, but also periods with salinity below 8 were registered. The annual mean values for salinity (Table 1) showed highest values at Boltenhagen and Kühlungsborn with a mean yearly salinity between 12 and 14 and about 90% of the data points above a salinity of 10. Farther east, at Zingst, the mean summer salinity mainly ranged between 9 and 10. More than 30% of the daily mean salinities measured at Zingst during summer showed values above 10 in all of the years. Nevertheless, the number of days above a salinity of 10 is variable between years and even days with a mean salinity below 8 were observed (11%). At Dranske the frequency of mean daily salinities below 8 (5–11% of measured summer days) was similar to those at Zingst. However, the percentage of days with measured salinities above 10 was lower than at the more western location Zingst and the annual mean salinity at Dranske varied between 8 and 9. At the sampling stations Boltenhagen, Kühlungsborn, and Zingst the salinity did not change with the saltwater intrusion in 2014 (Table 1). During the investigated 4 years no relevant daily fluctuations in salinity were observed.
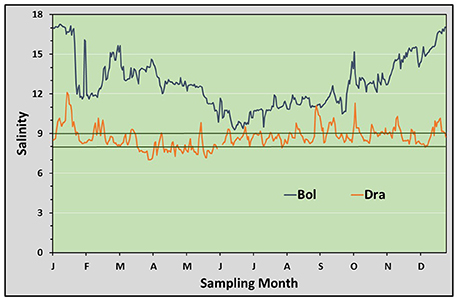
Figure 3. Salinity: Mean salinities based on data collected from 2012 to 2015. The westernmost (Boltenhagen) and the easternmost (Dranske) sampling sites are shown. The limiting lower salinity for Teredo navalis (between 8 and 9) is indicated by dark-green lines.
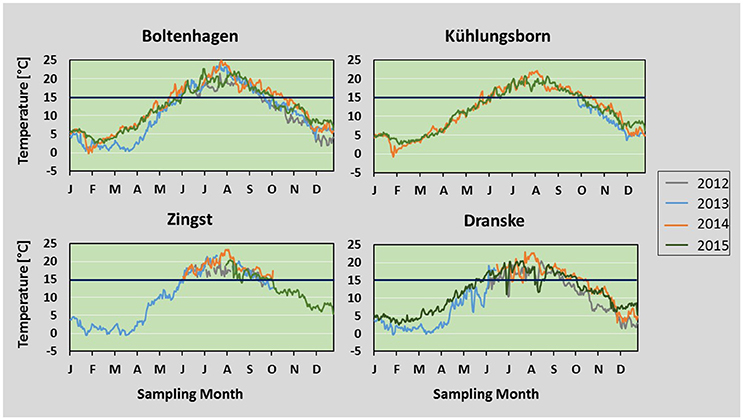
Figure 4. Temperature: Daily mean temperatures measured from 2012 to 2015 at Boltenhagen, Kühlungsborn, Zingst, and Dranske. Values are based on hourly measurement intervals. The dark-blue line indicates the presumed lower threshold of 15°C for the start of Teredo navalis reproduction.
During all of the investigated years temperatures above 15°C were reached between late May and early June and dropped below this value between mid of September and mid of October. The most striking differences between the years studied were the low water temperatures in winter 2012/13 lasting until mid of April 2013 and the long lasting high water temperatures in summer 2014 (Figure 4). The mean water temperature between 21th of December and 20th of March in 2012/13 was 2.7°C compared to 5.5°C in 2013/14 and 5.8°C in 2014/15. Despite this low mean water temperature, there were only few days between December 2012 and April 2013 with an mean daily temperature below 0°C (Boltenhagen 1; Zingst 14). During winter 2013/14 at 2 days mean temperatures below 0°C were measured, while in 2014/15 temperatures below 0°C were not reached at all.
The summer mean temperatures at Boltenhagen were highest in all years (19.3°C, four-year mean), while at Dranske they were always lowest (17.6°C, 4-year mean) (Table 1). Also during summer months, water temperatures at Dranske dropped below 15°C for short intervals (Figure 4), which might be due to upwelling events of colder bottom water in this region. At Boltenhagen the highest maximum values of summer water temperatures were measured, often reaching daily mean values of 22°C (Table 1). The summer mean temperatures including all stations differed significantly from each other, except Kühlungsborn and Zingst (ANOVA, p < 0.0005). In 2014, mean water temperatures were the highest at all stations. Furthermore, water temperatures above 15°C continued until October 20, which was 4 weeks longer compared to 2012 and 2013 and 2 weeks longer than in 2015. Also the summer means in the 4 years differed from each other: 2012 and 2015 were significantly colder than 2013 and 2014 (ANOVA, p < 0.0005).
Borehole Abundance
T. navalis boreholes in submerged pine wood panels were observed at all stations between Boltenhagen and Zingst during each of the 4 years (Figure 5). Farther east, at Hiddensee, Dranske, and Glowe, boreholes were found less frequently or were absent. At Glowe, no larval boring activity was observed on summer-exposed panels. However, in panels exposed at Glowe from late September or October to spring of the following year, several boreholes were found, indicating a late arrival of larvae (Figure 6). At both of the easternmost sampling sites, Dranske and Glowe, boreholes were always empty or with dead larvae, indicating unsuccessful recruitment. The borehole abundance was significantly lower in Dranske and Glowe compared to the other stations (ANOVA, p < 0.05).
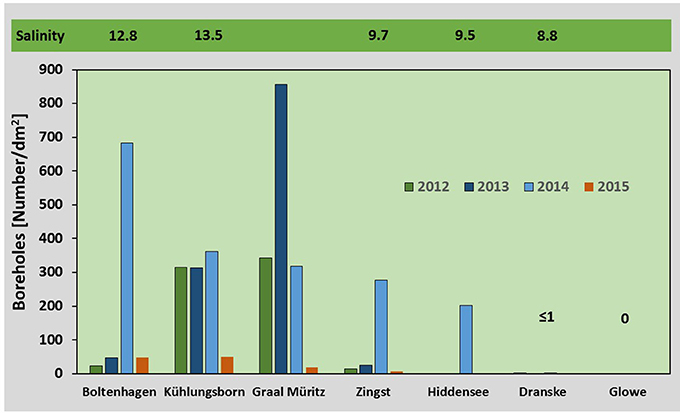
Figure 5. Larvae abundance: Bars indicate the median (n = 3) number of boreholes counted per dm2 on test panels at the different sampling sites in the years 2012–2015. Panels were exposed from May to October. The mean salinity based on measurements from 2012 to 2015 (hourly data) is given above the bars.
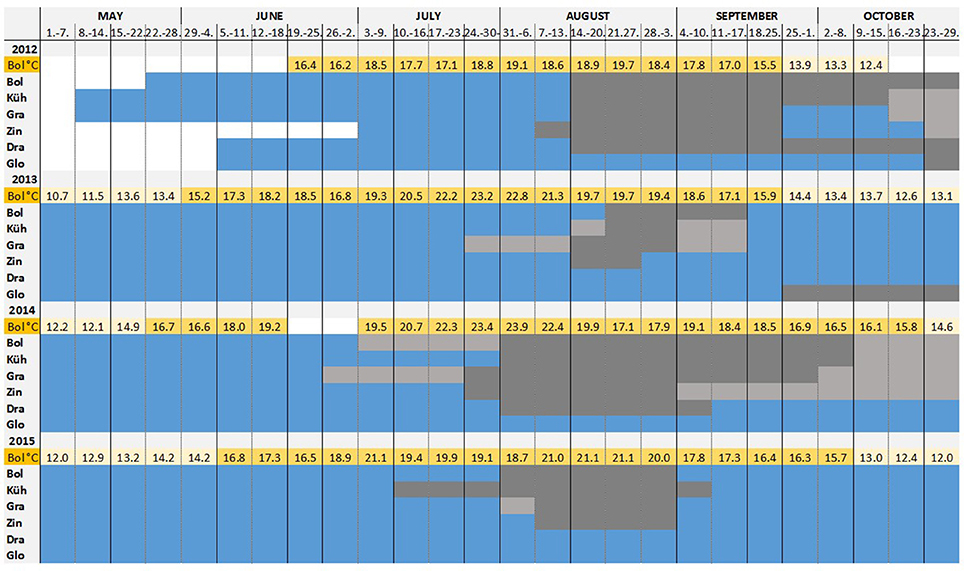
Figure 6. Time of larval settlement: During each month in summer, a test panel was collected and replaced by a new one. Periods of high, low and no Teredo navalis recruitment are shown. The mean temperature per week measured at Boltenhagen is indicated above the bars, showing the temperature variation during the summers of 2012–2015. Temperatures above 15°C are highlighted. The period of low recruitment is defined as the period when less than 10 percent of total larvae of the respective year and sampling location settled. no recruitment
main recruitment period
low recruitment period
no data.
The number of boreholes of T. navalis on the surface of the exposed pine wood varied considerably among locations and years, with the highest number of boreholes counted in 2014 (Median: 357 boreholes dm−2 between Boltenhagen and Zingst) and the lowest in 2015 (Median: 40 boreholes dm−2 between Boltenhagen and Zingst). The borehole abundance in 2015 differed significantly from the abundance in 2014 (ANOVA, p < 0.05). The highest abundance was found at Kühlungsborn and Graal Müritz during summer 2012 und 2013, while at Boltenhagen and Zingst it was comparatively low in these years. This pattern changed in 2014, showing a maximum at Boltenhagen in the west, and lower numbers at Kühlungsborn, Graal Müritz, and Zingst (Figure 5).
Influence of Temperature and Salinity on T. navalis Borehole Abundance
Significant correlations between the borehole abundance of T. navalis and seawater temperature or salinity revealed by Spearman's rank correlation test are shown in Table 2. The tests including the data from the Boltenhagen, Kühlungsborn, Zingst, and Dranske stations over all study years indicate significant correlations between borehole abundance and summer mean temperatures, as well as between borehole abundance and summer mean salinity. Salinity and temperature are not co-correlated in our data set. On the other hand, in the tests concerning data from single stations, significant correlations between borehole abundance and temperature were found only for Boltenhagen and Zingst. No correlations between borehole abundance and salinity were found by the single-station tests. Since a combined effect of temperature and salinity on T. navalis distribution seems probable in the Baltic Sea, an ANOVA was also applied, but did not yield any significant information on the variance.
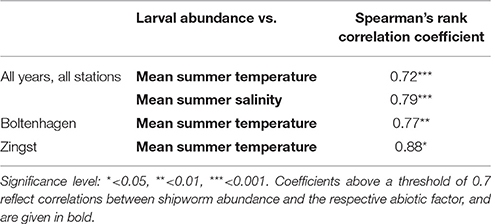
Table 2. Spearman's rank correlation coefficient for potential influence of seawater temperature and salinity on the abundance of Teredo navalis on the Mecklenburg-western Pomeranian coast.
Time of Larval Settlement
From the monthly sampling of larval traps, a peak period of settlement per year and sampling site was defined (Figure 6). The main settling period was considered as the time when the number of boreholes was higher than 10% of the total boreholes of the respective year and sampling location, and it was distinguished from the periods when only single larvae attached to the wood. This period of intensive wood attack by T. navalis differed only slightly among the sampling locations, but differed more among years. The earliest boreholes were found on the panels by the end of June (2014). The main larval settlement started in the middle to end of August (2012, 2013), and in mid-July (2015) to the beginning of August (2014, 2015). The main settlement period in 2013 and 2014 was relatively short compared to 2012 and 2015. In 2013 it lasted until mid-September, over a total period of 2–4 weeks, depending on the site (Figure 6). In 2015 newly settled larvae were found on the panels until the beginning of September, a period of 4–5 weeks. At Kühlungsborn, larvae were settling even over a period of 9 weeks. During 2012 and 2015, an intensive T. navalis attack continued until October. Individual larvae were found on the panels until the end of October in both years.
At Glowe, no larvae settled on the exposed panels during summer of the study years. In late September 2013 and late October 2014, however, larvae bored into the panels at Glowe, but did not survive. Similar observations that larvae could not settle successfully after September were made at the other locations investigated.
The main settling period of T. navalis larvae began 5–12 weeks after the water reached a mean weekly temperature above 15°C (Figure 6), a threshold for larvae release into the water in other regions (Loosanoff and Davis, 1963; Culliney, 1975).
Discussion
For several years, the possibility of a range expansion by T. navalis has been debated in the literature. Some authors have provided evidence that this species is or will be moving into formerly uninhabited areas, due to changes in hydrographic conditions caused partly by climate change (Hoppe, 2002; Tuente et al., 2002; Gollasch et al., 2009; Paalvast and van der Velde, 2011b; Borges, 2014). Other authors found that this is not the case for certain geographic areas such as the western coast of Sweden (Appelqvist et al., 2015a,b). For the southwestern Baltic Sea, Borges et al. (2014) postulated a range expansion of T. navalis by adaptation to salinities as low as 7. Adaptation of this species to lower salinities, however, was not confirmed experimentally. The present distribution border of T. navalis for the southwestern coast of the Baltic Sea is, according to the results of the present study, located east of the island of Hiddensee at salinities between 8 and 9. This coincides with the findings of Sordyl et al. (1998), implying that no range expansion has taken place during the last 17 years. However, from our results it is reasonable to assume that the actual distribution border is not strict, since slight changes in salinity or temperature may allow T. navalis to settle and reproduce farther east.
T. navalis was regularly found in high numbers between Boltenhagen in the west and Zingst in the east on the Mecklenburg-western Pomeranian coast (Figure 1). At Zingst, the annual mean salinity ranges between 9 and 10.8 (Table 1). Farther east at the island of Rügen, only sporadically low numbers of boreholes were found, even though the annual mean salinity at Dranske was only slightly lower in 2014 and 2015 compared to Zingst. The four-year mean salinity was higher at Zingst (9.7) than at Dranske (8.8). For the survival and growth of T. navalis, however, not the mean yearly salinities per se might be important, but rather the length of the time intervals above a certain salinity threshold. At Zingst, for example, the percentage of summer days with mean salinities above 10 was higher (43%) compared to Dranske (12%) in all years (Table 1). At Hiddensee the salinities were above 10 on 31% of the days measured, although only a few data points were collected from this station.
As mentioned above, Borges et al. (2014) found T. navalis settling on exposed wooden panels at a salinity of 7 in the Mecklenburg-western Pomeranian part of the Baltic Sea. The site described by Borges et al. (2014) (N 54°24.599′, E 12°36.6′), however, is located in the inner coastal waters of the Baltic Sea west of Zingst, in the Bodstedter Bodden which is part of the Darss-Zingst-Bodden-Chain (Schiewer et al., 1994). The salinity at the sampling location given by Borges et al. (2014) is based on information from a global hybrid data set, compiled primarily from the environmental dataset in BIO-ORACLE (www.oracle.ugent.be/). These values were not confirmed by daily long-term measurements (1979–2004) in the Bodstedter Bodden provided by Schumann et al. (2006), which show a median salinity of 5.4. Borges et al. (2014) described the sampling location as a wreck site in the mouth of the river Prerowstrom. In view of the low salinity in the Bodstedter Bodden and the absence of wrecks in this area, we find it highly unlikely that T. navalis was found in this part of the inner coastal waters of the Baltic. We suppose that the panels of Borges et al. (2014) were exposed ca. 2 km north of the Bodstedter Bodden at the former mouth of the Prerowstrom, which entered the open Baltic Sea until it was closed by sediments after a strong storm in 1872. At this location, several wrecks are located (among others the Darß Kogge), and prevailing salinities are similar to those measured at Zingst, making the presence of T. navalis highly likely there.
Dranske and Glowe, located on the island of Rügen, are the easternmost sampling sites and therefore those with the lowest salinity investigated in the present study. During the regular sampling described above, several boreholes were found at Dranske in three of the 4 years, even though the abundance was comparatively low, with one to four boreholes per test panel (≤1 dm−2). At Glowe, farther east than Dranske (Figure 1), boreholes were detected only in 2012 and 2013, with abundance levels similar to that at Dranske. Even though boreholes were present and salinities were mainly above 8, at least at Dranske, no living larvae or adult specimens were ever observed. During a T. navalis survey conducted in 2016, however, adult shipworms were unexpectedly found in older wooden panels exposed in 2013 and 2014. Specimens were up to 20 cm long (shipworm length, not tube length) and an abundance of three to five individuals per panel (about 2 dm−2) could be documented (own results, not shown here). According to the size of the individuals, they probably had already settled in 2013 or 2014, due to slow growth in low-salinity waters. Considering the salinity conditions, Dranske is located at the distribution border of T. navalis in the southwestern part of the Baltic Sea. Thus, even slight changes in the abiotic conditions can make this particular habitat favorable or unfavorable for the wood-borer.
The mean annual salinity at Dranske varied between 8.1 in 2013 and 9.5 in 2015, showing no clear relationship to borehole abundance in the respective year. The mean temperature in summer 2014, however, was 1.8 and 1.3°C, respectively, higher than in 2012 and 2015. The warm summer temperatures in 2014 lasted until October 21, thus 2–4 weeks longer than during the other years investigated. During the summers of 2012, 2013, and 2015, water temperatures at Dranske abruptly dropped below 15°C from time to time. Such rapid decreases were not observed in summer 2014 (Figure 4). Since reproduction, survival, and metamorphosis of T. navalis larvae are temperature-dependent (Nair and Saraswathy, 1971; Hoagland, 1986), we suggest that the higher and more stable temperatures in 2014 favored the establishment of T. navalis larvae and the growth of juveniles, although in terms of salinity, this species is at its lower physiological limit. For other teredinids (Lyrodus pedicellatus Quatrefages 1849) the importance of combined temperature-salinity effects was asserted by Eckelbarger and Reish (1972). Kristensen (1969), working in Danish waters, found indications that T. navalis attacks were most likely to take place when summer mean temperatures were above 19°C, and such attacks were strongest when high water temperatures coincided with increased salinities. Applying a climate-envelope model, Appelqvist et al. (2015a) found for the western Baltic Sea that favorable conditions for T. navalis were controlled by temperature whenever salinity was above 8. This result is supported by the present study through field investigations of T. navalis abundance combined with direct measurements of temperature and salinity conditions at the sampling locations. However, from the present data, this effect cannot be asserted statistically. The decreasing salinity from west to east along the Mecklenburg-western Pomeranian coast is confounded with decreasing temperature, making the chosen sampling spots inappropriate to test this hypothesis. Further investigation at selected sampling locations as well as laboratory experiments under controlled temperature and salinity conditions would be necessary.
Optimum temperatures for reproduction of T. navalis are above 15°C (Roch, 1932; Kristensen, 1979). Larvae are released at the straight-hinge veliger stage, about 5 days after fertilization (Culliney, 1975) and remain in the water column for another 15–35 days before settlement (Schütz, 1961; Nair and Saraswathy, 1971; Culliney, 1975). On the Mecklenburg-western Pomeranian coast, temperatures above 15°C were reached in early June in all of the years investigated. According to these observations and to the literature cited above, larvae would have been expected to start settling on the exposed panels between late June and early July. This was not the case in the present study, with few exceptions (see Figure 6). The settling period started 5–12 weeks after the optimum temperature of 15°C was reached. There was thus no obvious relationship between the threshold temperature persumed to trigger larval release and the settling period of T. navalis. Similarly, Paalvast and van der Velde (2011a) found T. navalis breeding from August to September in the Port of Rotterdam area, even though favorable conditions for reproduction were reached in April or May. Furthermore, Kristensen (1979) found T. navalis larvae settling in the Danish Isefjord from mid-August to the end of September. In contrast, on the Swedish west coast, larvae settled from the end of June to the beginning of July (Appelqvist and Havenhand, 2016), thus mainly several weeks earlier than on the Mecklenburg-western Pomeranian coast. Appelqvist and Havenhand (2016) established a significant correlation between the date at which the mean sea surface temperature reached 16°C and the start date of intensive recruitment. The results from the present study, as well as from Kristensen (1979) and Paalvast and van der Velde (2011a) may indicate that this temperature threshold is not relevant for all regions, or that abiotic factors other than temperature influence the start of larval settlement. These factors might be lower salinities, for example, compared to the Swedish west coast, or the availability of food or wood. Furthermore, prevailing wind direction and intensity influence coastal currents and may determine the settling success of released larvae. These influences, as well as the prevailing period of T. navalis larvae in the coastal waters of the southwestern Baltic Sea, were not analyzed in the present study. Therefore, no clear trend was found for the settling time of T. navalis larvae on the Mecklenburg-western Pomeranian coast during 2012 and 2015. However, different authors (Ryabchikov and Nikolaeva, 1963; Kristensen, 1979) did find that peak settling coincided with the end of a period of high temperatures. This relationship could not be confirmed by the present investigation, since sampling intervals of 4 weeks were too long for this purpose. Nevertheless, a tendency to intensive settlement toward the end of a period of warm temperatures can be hypothesized from the results, and should be investigated in more detail in future studies.
Compared to investigations on T. navalis from other coastal regions, the borehole abundance on the panels exposed at the Mecklenburg-western Pomeranian coast seems very high. Maximum numbers of boreholes in the study years were between 990 (2013) and 90 (2015) boreholes per dm2. Kristensen (1979) found a maximum of 27 juvenile T. navalis per dm2 in Danish waters, and Norman (1977a,b) and Appelqvist et al. (2015b) between 100 and 200 individuals per dm2, respectively, on the Swedish west coast. However, in these studies juvenile individuals were counted using x-ray radiography, in contrast to the present study, in which boreholes were counted. The latter method leads to an overestimation of larval abundance, since not every borehole was inhabited by a successfully metamorphosed living shipworm. Control counts comparing the number of living individuals with the number of boreholes showed that about 70% of the larvae arriving at a wooden panel successfully settled (own results, not shown here). Even taking this into account, the number of boreholes in the southwestern Baltic Sea is generally high compared to other regions, also in years with low borehole abundance at the study sites. Also, Zettler et al. (2000) observed that benthic marine species occur in higher abundances in the Mecklenburg Bight, close to their distribution border, than in more marine regions, although the individuals remain smaller due to low salinity. This might be due to a potentially large catchment area for larvae in this part of the Baltic Sea coast, including the German, Danish, and Swedish coasts (Appelqvist et al., 2015a), or to reduced competition for space due to lower biodiversity in low-salinity waters.
The sporadic inflows of high-salinity waters from the North Sea to the Baltic Sea strongly influence the environmental conditions in the Baltic (Elken and Matthäus, 2008), as mentioned in the introduction. One of the major intrusion events of the last century occurred in 1993 (Dahlin et al., 1993; Mohrholz et al., 2015), and coincided with the return and permanent establishment of T. navalis along the southwestern Baltic coast (Sordyl et al., 1998). This led to the assumption of several authors, that the salinity increase in the Baltic positively influenced the establishment of T. navalis (Sordyl et al., 1998; Nehring, 2005). During the present study the first major saltwater intrusion since 2003 (Mohrholz et al., 2015) was observed. We recorded a sharp, although temporary rise of monthly mean salinities in January 2014 at the Boltenhagen, Kühlungsborn, and Dranske stations. At Boltenhagen and Kühlungsborn the salinity was 18.5 and hence more than 3 units higher than the highest mean value for January measured during the 4 years of the present investigation. At Dranske the rise was more than 2 units up to a salinity of 10.4 (own results, not shown here). In February, salinity returned to levels frequently measured during the respective time of the year. These findings coincide with those of Schumann et al. (2006), who also found that a salinity increase related to salt-water intrusion lasted for only a few weeks, mainly January and February. In 2015 after the major salt-water intrusion, total larval densities were much lower than in other years. At Dranske und Glowe no larvae at all were found in 2015. The major salt-water intrusion in 1993 also occurred during winter, minimizing possible effects on the introduction of T. navalis to the Baltic Sea. We therefore conclude that the very sporadic major salt-water intrusions from the North Sea have little or no effect on T. navalis in the Baltic Sea, particularly during winter when temperature is too low for the physiological requirements of this wood-borer.
To prevent further spreading of the invasive T. navalis along the coastline, Appelqvist et al. (2015a) suggested to remove wooden structures as potential stepping stones. On the Mecklenburg-western Pomeranian coast, the groin systems deployed at short intervals along the shore indeed represent ideal man-made stepping stones for the distribution of T. navalis from higher-salinity waters toward the lower-salinity waters farther east. However, it must be noted that the use of wood as a natural building material in the Baltic Sea should be favored for coastal protection and other maritime purposes, and hence is highly recommended by HELCOM (Rec 16-3 Helcom, http://helcom.fi/helcom-at-work/recommendations).
The present investigation did not provide definitive results regarding the factors that might control the settlement of T. navalis larvae along the Mecklenburg-western Pomeranian coast. This is partly due to the high variances in borehole abundance. At Boltenhagen, for example, small numbers of boreholes, compared to Kühlungsborn and Graal Müritz, were found in the years 2012, 2013, and 2015, although Boltenhagen should have more favorable conditions for T. navalis larvae in terms of salinity and temperature. In 2014, the borehole abundance was much higher at Boltenhagen than in Kühlungsborn and Graal Müritz. In 2014, the number of settling larvae was increased at Zingst and Hiddensee compared to the other study years. At Zingst and Hiddensee the increased borehole density is probably due to the high summer temperature in 2014. The variability at Boltenhagen, however, cannot fully be explained by the abiotic parameters measured. Rather, variability in surface currents, perhaps due to prevailing wind directions in the respective year, may have caused the high larval abundance in 2014. Detailed information on water currents along the Mecklenburg-western Pomeranian coast in the study years would be essential but were not measured in this study. Furthermore, the varying salinity and temperature conditions and the parallel decrease of salinity and temperature toward the east make interpretations difficult. However, the present study was conducted as a first step toward an understanding of the species' occurrence at the limit of its distribution. For detailed analyses of combined salinity-temperature effects, experiments under controlled conditions (laboratory or mesocosm) would be preferable, where salinity and temperature combinations should be chosen according to the results of the present study.
Author Contributions
HL has to a great extend performed the field and laboratory work, has analyzed the data and written the first draft of the manuscript. RW has taken part in the field and the laboratory work, has contributed to figure drafts and has edited the manuscript. RK has essentially contributed to field and laboratory work. RB has been involved in project development and has contributed to the manuscript. UK has significantly been involved in project development and realization and has essentially edited the manuscript. All authors have approved the final manuscript.
Conflict of Interest Statement
The authors declare that the research was conducted in the absence of any commercial or financial relationships that could be construed as a potential conflict of interest.
Acknowledgments
This project was financed by the Federal Agency for Agriculture and Environment, Coastal Protection Department (STALU MM, Rostock) with strong support by Michael Bugenhagen. We are grateful to Henrike Pfefferkorn, Johannes Höhn, Juliane Müller, and Julia Regnery for their technical help in fieldwork and diving.
References
Appelqvist, C., Al-Hamdani, Z. K., Jonsson, P. R., and Havenhand, J. N. (2015a). Climate envelope modeling and dispersal simulations show little risk of range extension of the Shipworm, Teredo navalis (L.), in the Baltic sea. PLoS ONE 10:e0119217 doi: 10.1371/journal.pone.0119217
Appelqvist, C., and Havenhand, J. N. (2016). A phenological shift in the time of recruitment of the shipworm, Teredo navalis L., mirrors marine climate change. Ecol. Evol. 6, 3862–3870. doi: 10.1002/ece3.2126
Appelqvist, C., Havenhand, J. N., and Toth, G. B. (2015b). Distribution and abundance of teredinid recruits along the Swedish coast – are shipworms invading the Baltic Sea? J. Mar. Biol. Assoc. 95, 783–790. doi: 10.1017/S0025315414001830
Bavendamm, W., and Schmidt, H. (1948). Die Holzbohrmuscheln oder Teredinidae: Ihre Lebensweise, Schädlichkeit und Bekämpfung. Merkbl. des Reichsinst.für Forst- und Holzwirtschaft. Hamburg: Kröger.
Björdal Gjelstrup, C., Gregory, D., Manders, M., Al-Hamdani, Z., Appelqvist, C., Haverhand, J., et al. (2012) Strategies for Protection of Wooden Underwater Cultural Heritage in the Baltic Sea Against Marine Borers. The EU Project 'WreckProtect'. Conservation Management of Archaeological Sites 14 (1–4).
Blum, H. F. (1922). On the effect of low salinity on Teredo navalis. Univ. Calif. Publ. Zool. 22, 350–368.
Bonsdorff, E. (2006). Zoobenthic diversity-gradients in the Baltic Sea: continuous post-glacial succession in a stressed ecosystem. J. Exp. Mar. Biol. Ecol. 330, 383–391. doi: 10.1016/j.jembe.2005.12.041
Borges, L. M. S. (2014). Biodegradation of wood exposed in the marine environment: evaluation of the hazard posed by marine wood-borers in fifteen European sites. Int. Biodeterior. Biodegrad. 96, 97–104. doi: 10.1016/j.ibiod.2014.10.003
Borges, L. M., Merckelbach, L. M., Sampaio, Í., and Cragg, S. M. (2014). Diversity, environmental requirements, and biogeography of bivalve wood-borers (Teredinidae) in European coastal waters. Front. Zool. 11:13. doi: 10.1186/1742-9994-11-13
Borges, L. M. S., Sivrikaya, H., Le Roux, A., Shipway, J. R., Cragg, S. M., and Costa, F. O. (2012). Investigating the taxonomy and systematics of marine wood borers (Bivalvia. Teredinidae) combining evidence from morphology, DNA barcodes and nuclear locus sequences. Invertebr. Syst. 26, 572–582. doi: 10.1071/IS12028
Carpenter, E. J., and Culliney, J. L. (1975). Nitrogen fixation in marine shipworms. Science 187, 551–552. doi: 10.1126/science.187.4176.551
Culliney, J. L. (1975). Comparative larval development of the shipworms Bankia gouldi and Teredo navalis. Mar. Biol. 29, 245–251. doi: 10.1007/BF00391850
Dahlin, H., Fonselius, S., and Sjöberg, B. (1993). “The changes of the Hydrographic conditions in the Baltic proper due to 1993 major inflow to the Baltic Sea,” in ICES Statutory Meeting. (Dublin, ICES C.M, 1993/C), 58.
Distel, D. L., Morrill, W., MacLaren-Toussaint, N., Franks, D., and Waterbury, J. (2002). Teredinibacter turnerae gen. nov., sp. nov., a dinitrogen-fixing, cellulolytic, endosymbiotic gamma-proteobacterium isolated from the gills of wood-boring molluscs (Bivalvia, Teredinidae). Int. J. Syst. Evol. Microbiol. 52(Pt 6), 2261–2269. doi: 10.1099/00207713-52-6-2261
Eckelbarger, K. J., and Reish, D. (1972). Effects of varyingtemperatures and salinities on settlement, growth and reproduction of the wood-boring pelecypod Lyrodus pedicellatus. Bull. South. Calif. Acad. Sci. 1972, 116–127.
Elken, J., and Matthäus, W. (2008). “Physical system description,” in Assessment of Climate Change for the Baltic Sea Basin. Series: Regional climate studies, eds The BACC Author Team (von Storch, H.) (Berlin; Heidelberg: Springer-Verlag), 379–398.
Gollasch, S., Haydar, D., Minchin, D., Wolff, W. J., and Reise, K. (2009). “Introduced aquatic species of the North Sea coast and adjacent brackish waters,” in Biological Invasions in Marine Ecosystems, eds G. Rilov and J. A. Crooks (Berlin; Heidelberg: Springer Verlag), 507–528.
Gollasch, S., and Nehring, S. (2006). National checklist for aquatic alien species in Germany. Aquat. Invas. 1, 245–269. doi: 10.3391/ai.2006.1.4.8
Grave, B. H. (1942). The sexual cycle of the shipworm, Teredo navalis. Biol. Bull. 82, 438–445. doi: 10.2307/1537989
Hoagland, K. E. (1986). Effects of temperature, salinity, and substratum on larvae of the shipworm Teredo bartschi Clapp and Teredo navalis Linnaeus (Bivalvia: Teredinidae). Am. Malacol. Bull. 4, 89–99.
Hoagland, K. E., and Crocket, L. (1982). Ecological Studies of Wood-Boring Bivalves in the Vicinity of the Oyster Creek Nuclear Generating Station. Progress report, NUREG/CR-−2727.
Hoppe, K. (2002). “Teredo navalis – the cryptogenic shipworm,” in Invasive Aquatic Species of Europe, Distribution, Impacts and Management, eds E. Leppäkoski, S. Gollasch, and S. Olenin (Dordrecht: Springer), 116–119. doi: 10.1007/978-94-015-9956-6_12
Jerling, L. (1999). “Sea shores,” in Swedish Plant Geography - Acta Phytogeographica Suecica, Vol. 84, eds H. Rydin, P. Snoeijs, and M. Dieckmann (Uppsala: Opulus Press AB), 116–119.
Kristensen, E. (1969). Attacks by Teredo navalis L. in inner Danish waters in relation to environmental factors. Videnskabelige Medd. Dan. Naturhistorisk Forening 132, 199–210.
Kristensen, E. (1979). Observations on growth and life cycle of the shipworm Teredo navalis in Isefjord, Denmark. Ophelia 18, 235–242. doi: 10.1080/00785326.1979.10425501
Leppäkoski, E., and Olenin, S. (2000). Non-native species and rates of spread: lessons from the brackish Baltic Sea. Biol. Invas. 2, 151–163. doi: 10.1023/A:1010052809567
Loosanoff, V. L., and Davis, H. C. (1963). Rearing of bivalve mollusks. Adv. Mar. Biol. 1, 1–136. doi: 10.1016/S0065-2881(08)60257-6
MacKenzie, B. R., and Schiedeck, D. (2007). Daily ocean monitoring since the 1860s shows record warming of northern European seas. Glob. Chang. Biol. 13, 405–420. doi: 10.1111/j.1365-2486.2007.01360.x
Medvedev, I. P., Rabinovich, A. B., and Kulikov, E. A. (2016). Tides in three enclosed basins. The baltic, black, and Caspian Seas. Front. Mar. Sci. 3:46. doi: 10.3389/fmars.2016.00046
Meyer, H. A., and Möbius, K. (1865). Fauna der Kieler Bucht, Bd. I: Die Hinterkiemer Oder Opisthobranchia. Leipzig: Verlag Wilhelm Engelmann.
Mohrholz, V., Naumann, M., Nausch, G., Krüger, S., and Gräwe, U. (2015). Fresh oxygen for the Baltic Sea — An exceptional saline inflow after a decade of stagnation. J. Mar. Syst. 148, 152–166. doi: 10.1016/j.jmarsys.2015.03.005
Nair, N. B., and Saraswathy, M. (1971). The biology of wood boring teredinid molluscs. Adv. Mar. Biol. 9, 335–509. doi: 10.1016/S0065-2881(08)60345-4
Nakel, E. (1954). Über die Holzbohrmuschel und ihre Bekämpfung. Wasserwirtschaft - Wassertechnik 4, 141–146.
Nehring, S. (2005). Re-Occurrence and bioindication status of the netted dog whelk nassarius reticulatus (Linnaeus, 1758) in the Western Baltic Sea (Mecklenburg Bight, Germany). Russ. J. Mar. Biol. 31, 14–20. doi: 10.1007/s11179-005-0038-1
Norman, E. (1977a). “The time of settlement on the Swedish west coast of the wood-boring molluscs Teredo navalis, Psiloteredo megotara and Xylophaga dorsalis,” in Material und Organismen Beiheft 3 (Berlin: Duncker & Humblot), 531–542.
Norman, E. (1977b). The geographical distribution and the growth of the wood-boring molluscs Teredo navalis L., Psiloteredo megotara (Hanley) and Xylophaga dorsalis (Turton) on the Swedish West Coast. Ophelia 16, 233–250. doi: 10.1080/00785326.1977.10425473
Paalvast, P., and van der Velde, G. (2011a). Distribution, settlement, and growth of first-year individuals of the shipworm Teredo navalis L. (Bivalvia: Teredinidae) in the Port of Rotterdam area, the Netherlands. Int. Biodeterior. Biodegradation 65, 379–388. doi: 10.1016/j.ibiod.2010.11.016
Paalvast, P., and van der Velde, G. (2011b). New threats of an old enemy: the distribution of the shipworm Teredo navalis L. (Bivalvia: Teredinidae) related to climate change in the Port of Rotterdam area, the Netherlands. Mar. Pollut. Bull. 62, 1822–1829. doi: 10.1016/j.marpolbul.2011.05.009
R Development Core Team, R. (2009). R: A Language and Environment for Statistical Computing. Vienna.
Roch, F. (1932). Einige Beobachtungen zur Ökologie und Physiologie von Teredo navalis L. Arkiv Zool. 24, 1–17.
Rönnberg, C., and Bonsdorff, E. (2004). Baltic Sea eutrophication: area-specific ecological consequences. Hydrobiologia 514, 227–241. doi: 10.1023/B:HYDR.0000019238.84989.7f
Ryabchikov, P. L., and Nikolaeva, G. G. (1963). Settlement on wood of the larvae of Teredo navalis L. (Teredinidae, Mollusca) in the Black Sea. Trudy Inst. Okeanol. 70, 179–185.
Schiewer, U., Schlungbaum, G., and Arndt, E. A. (1994). Monographie der Darss-Zingster Boddenkette. Rostoc. Meeresbiol. Beitr. 2, 1–240.
Schumann, R., Baudler, H., Glass, Ä., Dümcke, K., and Karsten, U. (2006) Long-term observations on salinity dynamics in a tideless shallow coastal lagoon of the Southern Baltic Sea coast their biological relevance. J. Mar. Syst. 60, 330–344. doi: 10.1016/j.jmarsys.2006.02.007
Schütz, L. (1961). Verbreitung und Verbreitungsmöglichkeiten der Bohrmuschel Teredo navalis L. und ihr Vordringen in den NO-Kanal bei Kiel. Kieler Meeresforschung 17, 228–236.
Siegel, H., and Gerth, M. (2016). Sea Surface Temperature in the Baltic Sea in 2015. HELCOM Baltic Sea Environment Fact Sheet 2016. Available online at: http://www.helcom.fi/baltic-sea-trends/environment-fact-sheets/
Soldatova, I. N. (1961). Effects of various salinities on the bivalve mollusc Teredo navalis L. Trudy Instit. Okeanol. 49, 162–179.
Sordyl, H., Bönsch, R., Gercken, J., Gosselck, F., Kreuzberg, M., and Schulze, H. (1998). Verbreitung und Reproduktion des Schiffsbohrwurms Teredo navalis L. an der Küste Mecklenburg-Vorpommerns. Deutsche Gewässerkundliche Mitteilungen 42, 1–8.
Spicer, J. I., and Strömberg, J. O. (2003). Metabolic responses to low salinity of the shipworm Teredo navalis (L.). Sarsia 88, 302–305. doi: 10.1080/003648203103342902
Tuente, U., Piepenburg, D., and Spindler, M. (2002). Occurrence and settlement of the common shipworm Teredo navalis (Bivalvia: Teredinidae) in Bremerhaven harbours, northern Germany. Helgol. Mar. Res. 56, 87–94. doi: 10.1007/s10152-002-0101-7
Weigelt, R., Lippert, H., Borges, L. M. S., Appelqvist, C., Karsten, U., and Bastrop, R. (2016). First time DNA barcoding of the common shipworm Teredo navalis Linnaeus 1758 (Mollusca: Bivalvia: Teredinidae): Molecular-taxonomic investigation and identification of a widespread wood-borer. J. Exp. Mar. Biol. Ecol. 475, 154–162. doi: 10.1016/j.jembe.2015.11.008
Wichmann, G. (2005). “Stowaways,” in Schadenspiegel - Risk Factor of Water – Special Feature Issue. WRO (2010). (Hamburg: World Ocean Review, maribus GmbH), 112.
Keywords: marine wood-borers, shipworm, Baltic sea, temperature, salinity
Citation: Lippert H, Weigelt R, Glaser K, Krauss R, Bastrop R and Karsten U (2017) Teredo navalis in the Baltic Sea: Larval Dynamics of an Invasive Wood-Boring Bivalve at the Edge of Its Distribution. Front. Mar. Sci. 4:331. doi: 10.3389/fmars.2017.00331
Received: 20 June 2017; Accepted: 05 October 2017;
Published: 20 October 2017.
Edited by:
Christos Dimitrios Arvanitidis, Hellenic Centre for Marine Research, GreeceReviewed by:
Paola Del Negro, National Institute of Oceanography and Experimental Geophysics, ItalyDavide Francesco Tagliapietra, Istituto di Scienze Marine (CNR), Italy
Copyright © 2017 Lippert, Weigelt, Glaser, Krauss, Bastrop and Karsten. This is an open-access article distributed under the terms of the Creative Commons Attribution License (CC BY). The use, distribution or reproduction in other forums is permitted, provided the original author(s) or licensor are credited and that the original publication in this journal is cited, in accordance with accepted academic practice. No use, distribution or reproduction is permitted which does not comply with these terms.
*Correspondence: Heike Lippert, heike.lippert@uni-rostock.de