- Red Sea Research Center, King Abdullah University of Science and Technology, Thuwal, Saudi Arabia
Ultraviolet-B (UVB) radiation is a global stressor that has profound impacts on freshwater and marine ecosystems. However, an analysis of the patterns of sensitivity to UVB radiation across aquatic photosynthetic organisms has not yet been published. Here, we performed a meta-analysis on results reported in 214 studies compiled from the published literature to quantify and compare the magnitude of responses of aquatic photosynthetic organisms to changes in UVB radiation. The meta-analysis was conducted on observations of marine (n = 893) and freshwater macroalgae (n = 126) and of marine (n = 1,087) and freshwater (n = 2,889) microalgae (total n = 4,995). Most of these studies (85%) analyzed the performance of organisms exposed to natural solar radiation when UVB was partially or totally reduced compared with the organismal performance under the full solar radiation spectrum, whereas the remaining 15% of the studies examined the responses of organisms to elevated UVB radiation mostly using artificial lamps. We found that marine photosynthetic organisms tend to be more sensitive than freshwater photosynthetic organisms to UVB radiation; responses to either decreased or increased UVB radiation vary among taxa; the mortality rate is the most sensitive of the trait responses to elevated UVB radiation, followed by changes in cellular and molecular traits; the sensitivity of microalgae to UVB radiation is dependent on size, with small-celled microalgae more sensitive than large-celled microalgae to UVB radiation. Thick macroalgae morphotypes were the less sensitive to UVB, but this effect could not be separated from phylogenetic differences. The high sensitivity of marine species, particularly the smallest photosynthetic organisms, to increased UVB radiation suggests that the oligotrophic ocean, a habitat comprising 70% of the world's oceans with high UVB penetration and dominated by picoautotrophs, is extremely vulnerable to changes in UVB radiation.
Introduction
The increased use of chlorofluorocarbons (CFCs) as refrigerants and propellants led to an alarming depletion of stratospheric ozone in the 1980s (Molina and Rowland, 1974) and to a corresponding increase in ultraviolet-B (UVB) radiation reaching the Earth's surface (Madronich et al., 1998). Although the Montreal Protocol led to a dramatic reduction in the emissions of CFCs (UNEP, Environmental Effects Assessment Panel, 2010), the ozone layer will likely not recover to 1980 levels within the coming decades (Weatherhead and Andersen, 2006). Thus, increased UVB radiation will continue to reach the biosphere over the coming years (Weatherhead and Andersen, 2006), although the healing of Antarctic ozone layer is occurring since 2000 (Solomon et al., 2016).
Increased UVB radiation has profound impacts on terrestrial and aquatic organisms (Caldwell et al., 1998; Häder et al., 2007). Bancroft et al. (2007) reported an overall negative effect of UVB on both survival and growth across species differing in life history, trophic position, and habitat. Similarly, a meta-analysis of experimental results showed that the mortality rates of marine biota increased rapidly in response to increased UVB radiation, with protists, corals, crustaceans, fish eggs, and larvae the most sensitive in marine ecosystems to increased UVB radiation (Llabrés et al., 2013).
Some of the mobile organisms may avoid exposure to UVB radiation by seeking shelter at depth and/or in shaded habitats. In contrast, the exposure of photosynthetic organisms to UVB radiation is dependent on light requirement and habitat. For example, obligate upper intertidal and supralittoral species (e.g., green macroalgae, Prasiola crispa) require exposure to air and are consequently exposed to high PAR and UVR (Holzinger et al., 2006). On the other hand, deep-water species (e.g., Eisenia galapagensis, Laminaria brasiliensis) require minimal PAR and consequently do not encounter high PAR and UVR in their habitat (Graham et al., 2007). Depending on their habitat and depth distribution, photosynthetic organisms have developed strong protection mechanisms and/or reparatory systems to avert damage. Because UVB was believed to be absorbed rapidly in aquatic ecosystems, the impacts of UVB radiation were believed to be stronger on terrestrial photosynthetic organisms than on marine photosynthetic organisms (Rozema et al., 2002). However, UVB penetrates to considerable depths in freshwater and marine ecosystems (Llabrés and Agustí, 2006; Tedetti et al., 2007), thereby impacting marine photosynthetic organisms as well, although the penetration of UVB varies with latitude, season and water turbidity (Hanelt et al., 2001; Wiencke et al., 2006).
Indeed, a large number of studies have addressed the effects of UVB on aquatic photosynthetic organisms including micro- and macro-algae both in freshwater and marine ecosystems (e.g., Häder et al., 2007, 2011). Photosynthetic organisms are more resistant than non-photosynthetic organisms to UVB (Llabrés et al., 2013; Agustí et al., 2015), suggesting that photoprotective and reparatory systems designed to counter UVB exposure operate efficiently in photosynthetic organisms. However, UVB radiation causes negative effects on the photosynthetic performance of micro- and macro-algae by reducing their photosystem II efficiency (e.g., Jiang and Qiu, 2005; Jin et al., 2013; Zhang et al., 2013), electron transport rate (e.g., Figueroa et al., 2014; Zhu et al., 2015) as well as photosynthetic carbon fixation rate (e.g., Villafañe et al., 2007; Helbling et al., 2008; Li et al., 2012). Photosynthetic organisms have thus evolved a range of strategies to mitigate UVB damage including increasing non-photochemical quenching (NPQ) (e.g., Jin et al., 2013), UVB-screening pigments (e.g., Klisch et al., 2002), UVB-absorbing/blocking secondary metabolites (e.g., phlorotannin compounds) and DNA repair systems as photolyase activity against UVB-induced DNA damage (e.g., van de Poll et al., 2001; Roleda et al., 2004). Despite these strategies, large variability in responses is found across photosynthetic taxa because the variety of damage induced by UVB is also large, with nucleic acids, proteins and lipids the primary targets of UVB (Buma et al., 2003), all of which can interfere with various cellular processes and metabolisms. For instance, one study reported that nitrogenase activity was completely inactivated under UVB exposure whereas nitrate reductase activity was doubled when compared with the same activities in the control in an N2-fixing cyanobacterium, suggesting diverse effects of UVB on nitrogen metabolisms (Kumar et al., 1996). At the organismal level, damage to specific proteins of photoreceptor and motor organelles may result in a variety of effects on motile behaviors (Zündorf and Häder, 1991). Moroz et al. (1999) reported that increased UVB decreased the number of gliding cells in the diatom Nitzschia lineariz whereas increased UVB exposure increased the number of immobile and oscillating cells.
The responses of macroalgae to UVB radiation may be dependent on life-history stages. Comparison between different early life-history stages of various kelp species of macroalgae from the Northern Hemisphere showed that spores are more susceptible to UVR damage compared with their corresponding juvenile sporophytic and gametophytic life stages (Dring et al., 1996; Roleda et al., 2007 and references therein). Roleda et al. (2009) also reported that the early life stages, e.g., propagules of a green macroalgae, Urospora penicilliformis, are more susceptible to UVB radiation than the adult life stages. In addition, Swanson and Druehl (2000) reported that kelp species of macroalgae with different meiospore sizes respond differently to UVR. The meiospore size difference was correlated with the depth distribution of adult plants, with the largest meiospores origination from shallow-dwelling adult kelp exposed to high UVR levels. Meiospores from adults growing in high UVR environments displayed greater germination and survival rates than the progeny of adult kelp occupying lower UVR environments. The responses of macroalgae to UVB radiation may be also dependent on thallus thickness and absorption spectra characteristics of the pigments (Roleda et al., 2006). Increasing thallus thickness minimizes UVR damage because the longer pathlength for UVR absorption across the thallus leads to outer cell layers shading inner cells (Franklin and Foster, 1997). Likewise, the vulnerability of microalgae to UVR damage may be dependent on cell size, which determines light absorption properties (Agustí, 1991). Photosynthetic carbon fixation of small phytoplankton species was reported to be much more sensitive to UVB radiation than that of large species in a coastal ecosystem (Li and Gao, 2013), and a recent laboratory study also revealed that the UVB sensitivity of diatoms decreased with increasing cell size (Wu et al., 2015). Small cells are also vulnerable to the accumulation of cyclobutane pyrimidine dimers but photosynthesis is less inhibited by UVB radiation, which may be caused by faster photo-repair in small cells (Buma et al., 2003). However, other factors such as bio-optical characteristics (Figueroa et al., 1997) and intraspecific differences (Halac et al., 2014) may outweigh the effects of cell size. As a consequence, there is currently no agreement on the generality of the relationship between cell size and UVB sensitivity across freshwater and marine microalgae.
The responses of aquatic photosynthetic organisms to UVB also depend on the dose of harmful radiation to which an individual organism is exposed. UVB dose is affected by latitude, altitude and the optical characteristics (i.e., UVB transmittance) of the water body. UVB radiation underwater is primarily absorbed by dissolved organic carbon (DOC) including chromophoric dissolved organic matter (CDOM) (Kirk, 1994) and particles. Since the concentrations of DOC and suspended particles that play key roles in absorbing UVB are much higher in freshwater ecosystems than in marine ecosystems, lower levels of UVB in the water column are expected in freshwater habitats. Thus, the impacts of UVB radiation on photosynthetic organisms are expected be the highest in marine ecosystems and alpine lakes (which have the lowest concentrations of DOC and suspended particles among freshwater habitats; Bancroft et al., 2007).
However, the analysis of the patterns of sensitivity to UVB radiation across aquatic photosynthetic organisms has not yet been published. We hypothesize that differences in responses of photosynthetic organisms to UVB radiation are associated with changes in molecular or metabolic pathways associated to taxa, as well as the differences in cell size of photosynthetic individuals. We also hypothesize that these differences may also be due to major habitat differences between freshwater and marine ecosystems.
Here, we compare the responses of photosynthetic organisms both in freshwater and marine ecosystems to UVB radiation. Specifically, we examine the variability in these responses across phyla and response traits, as well as the variability in responses due to cell size for unicellular algae and form-functional categories for macroalgae, to UVB radiation. We do so through a quantitative meta-analysis of the results of published studies on the responses of aquatic photosynthetic organisms to experimentally increased and reduced UVB. Not all traits that may affect the vulnerability of aquatic photosynthetic organisms to UVB radiation could be considered, because the number of reports providing information on traits such as life-history stages, habit, and thickness of macroalgae was too low to support a quantitative analysis.
Materials and Methods
Data Selection
We compiled data from experimental studies testing the responses of aquatic photosynthetic organisms to UVB radiation from the published literature. Our analysis focused on micro- and macro-algae due to their comparable and relatively large datasets, as data on responses of aquatic angiosperms are sparse. The resulting dataset of marine photosynthetic organisms included previous compilations [Agustí et al. (2015) and Llabrés et al. (2013), microalgae: n = 454, macroalgae: n = 432] and additional data published from April 2013 to July 2015 (microalgae: n = 633, macroalgae: n = 461) by a search on Web of Science and Google Scholar for the relevant keywords: UV radiation, aquatic and marine. Agustí et al. (2015) and Llabrés et al. (2013) reported data only for marine photosynthetic organisms, so the dataset on freshwater photosynthetic organisms, was produced entirely from reports retrieved from searches in the Web of Science and Google Scholar from 1950s to July 2015. The search used the keywords: UV radiation, aquatic, freshwater, and marine. Studies were selected when they met the following criteria outlined by Llabrés et al. (2013): (1) include assessments of the performance of aquatic microalgae and macroalgae under ambient irradiance levels, (2) report a treatment corresponding to the ambient solar radiation (i.e., control), and (3) include additional treatments by either removing or enhancing UVB radiation relative to ambient levels [e.g., control: PAR + ultraviolet-A (UVA) + UVB, treatment: PAR+UVA]. Papers not distinguishing the effects of UVA from that UVB were not included (e.g., PAR, and PAR + UVR). For the studies examined responses within time courses, we either collected the data at the end point (e.g., cellular/molecular traits) or derived, using the time trend, a rate (e.g., growth and mortality rate). Responses in the literatures were referred, by the authors, to either UVB doses, which consider exposure, or UVB intensity. Because our analysis is based, to allow for comparisons, on effect sizes, and relative change in UVB radiation, we followed the choice of the author so the relative change in UVB radiation reflect either change in doses or intensity as per the choice of the original study. Our search resulted in 214 papers reporting experimental results meeting these requirements. The authors are solely responsible for the accuracy of the dataset.
Data Analysis
We determined if the microalgae or macroalgae assessed in these papers had been classified at the phylum level to differentiate sensitivities to UVB and if the cell volume of the particular microalgae species had been reported. When microalgae cell volume was not reported, we derived the cell volume of the species from other published reports. The taxonomic classification followed AlgaeBase (www.algaebase.org). As thallus thickness was not reported for macroalgal species, we tested whether the response size of macroalgae to changes in UVB radiation differed among form-functional groups, which are related to thallus thickness (Duarte et al., 1995). Macroalgae were classified into the following morpho-functional groups, as described by Steneck and Dethier (1994): articulated calcareous, corticated, filamentous, foliose, foliose corticated, and leathery. Functional-form classifications, unlike taxonomic classifications, are not absolute and involve some degree of subjectivity in assigning a species to a category, as species display plasticity in growth dependent on growth conditions. Hence, we conferred our classification with that independently provided by two experts (D. Krause-Jensen, Aarhus University, Denmark, and Beatriz Martinez-Daranas, Univ. of La Habana, Cuba), and used the consensus classification for the statistical test. The wide range of response traits assessed in the literature were classified into the categories described by Llabrés et al. (2013), including: (1) Cellular/molecular, including a range of properties such as chlorophyll a, total lipids, carbohydrate, glutathione, and cyclobutane-pyrimidine dimers (CPDs) concentrations; (2) metabolic responses included photosynthetic rates (i.e., photosynthetic carbon fixation rate and photosynthetic oxygen evolution rate) and parameters (e.g., maximum photosystem II efficiency and non-photochemical quenching, NPQ); (3) growth; (4) mortality; and (5) motility, such as gliding and oscillating.
The response traits were standardized to allow comparisons across organisms. Responses across experiments assessing different traits were compared through the log-effect size, calculated as the natural log of the ratio of the response value in the control over that in the experimental treatment. We differentiated between the effect size of response traits that indicated stress (e.g., mortality rates, DNA damage, calculated as the ratio of the value in the treatment relative to the control) and that of response traits that signaled improved organism performance (e.g., growth, calculated as the ratio of the response value in the control over that in the experimental treatment) following Llabrés et al. (2013). A Ln effect size >0 signaled adverse effects on the organisms and a Ln effect size <0 signaled improved organism performance (Llabrés et al., 2013).
We calculated UVB relative as the ratio of UVBtreatment to UVBcontrol, as described by Llabrés et al. (2013). UVB relative values higher and lower than 1 indicated experimental treatments in which UVB was respectively increased or reduced relative to the irradiance received by the organism in situ. The experiments described in the papers were classified into two broad categories: (1) experiments that UVB was partially or totally removed under natural solar radiation, which dominated the data set (85.6% of the experiments) and (2) experiments that assessed responses to increased UVB radiation in experimental lab and in situ experiments with artificially supplemented UVB, which comprised 14.4% of the experiments in the data set.
We used one-way analysis of variance (ANOVA) to test for significant differences (p = 0.05) in the Ln effect size between two comparisons (e.g., freshwater microalgae vs. marine microalgae). We also used general linear models to test for significant differences in the Ln effect size between phyla or response traits in the sensitivity of organisms to UVB. We describe the relationships between responses from the Ln effect size and UVB dose and the UVB relative. The slope of the fitted regression equation between the Ln effect size and the UVB relative ratio indicates the sensitivity of organism examined to UVB radiation. A slope of 1 indicates that the relative biological response to UVB radiation is proportional to the relative change in UVB, whereas slopes significantly greater than and less than 1 indicate that the biological response respectively amplifies or buffers the relative change in UVB. The interactions between UVB relative levels and the habitat (marine or freshwater), the phylum of the organism (e.g., Bacillariophyta, Chlorophyta, Cyanobacteria), and cell size (for unicellular algae) were also tested using general linear models.
Results
The assembled data set included 4,995 observations of responses of aquatic photosynthetic organisms to UVB radiation, including 1980 observations of responses of marine photosynthetic organisms and 3,015 observations of responses of freshwater photosynthetic organisms (Table 1). The experiments on marine photosynthetic organisms reported 1,087 observations on microalgae and 893 observations on macroalgae, whereas the experiments on freshwater photosynthetic organisms reported 2,889 observations on microalgae and 126 observations on macroalgae (Table 1). The phylum Bacillariophyta was the subject of most of the experiments on marine microalgae (56%), whereas cyanobacteria dominated the experiments conducted on freshwater microalgae (79%) (Table S1 in Data Sheet 2). Most experiments on marine macroalgae focused on Rhodophyta (59%); most experiments on freshwater macroalgae targeted Charophyta (59%; Table 2). The overwhelming majority of the experiments showed that increased UVB radiation decreased the organism's performance. Indeed, only 3.06% of the experiments showed improved performance with increased UVB and just 5.78% of the experiments showed reduced performance with reduced UVB. These results demonstrated the expected dominance of the adverse effects of UVB radiation on the performance of aquatic photosynthetic organisms.
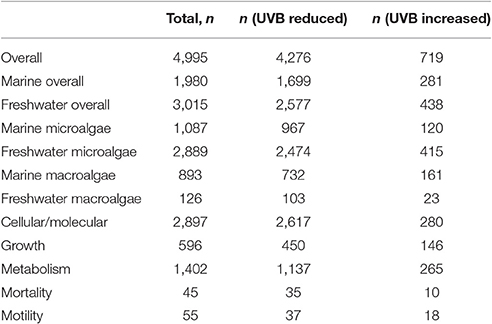
Table 1. Numbers of observations of the responses of freshwater and marine photosynthetic organisms to experimental changes in UVB radiation.
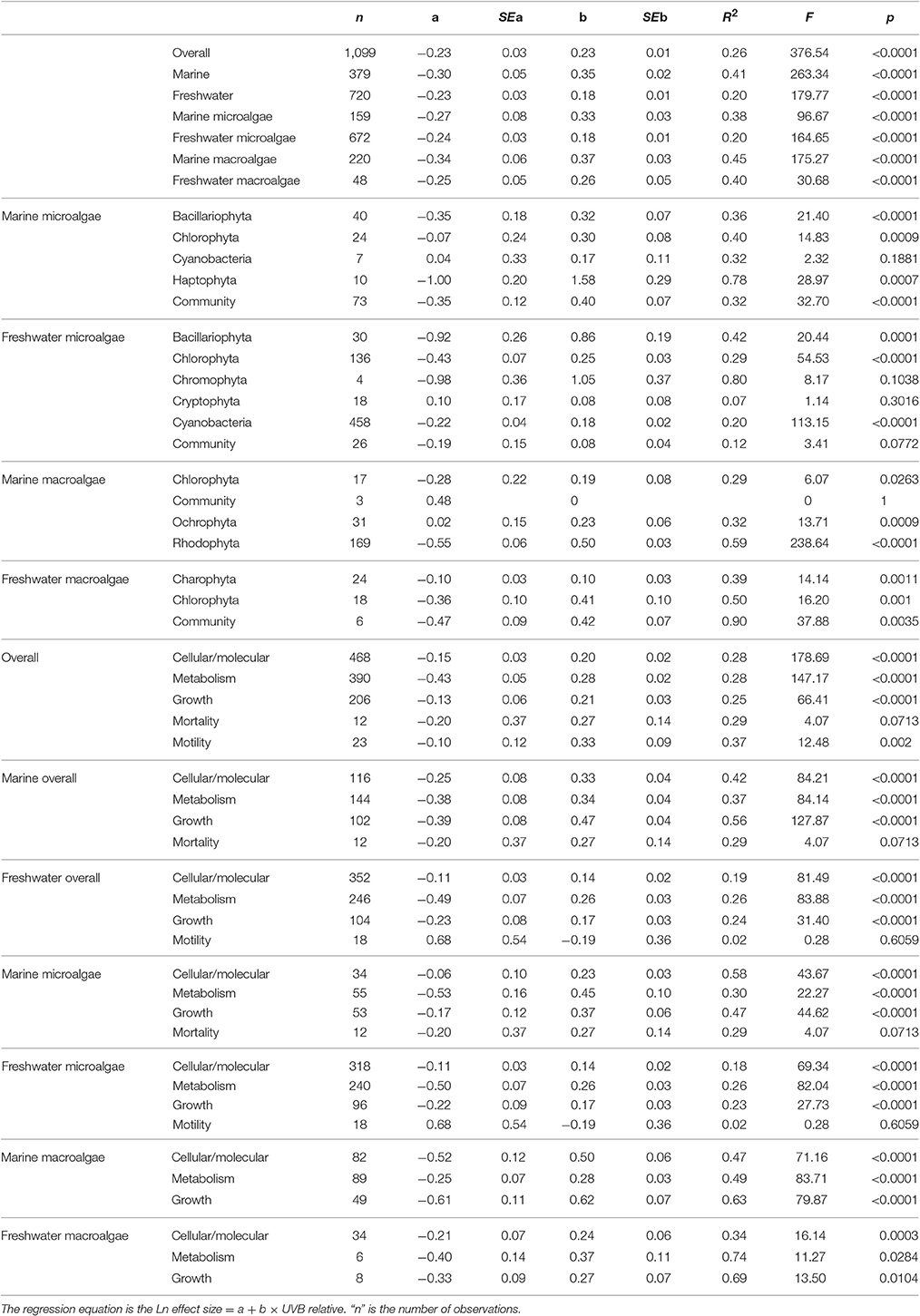
Table 2. Summary of meta-analysis results derived from the data set of experimental responses of freshwater and marine photosynthetic organisms to increased or reduced UVB levels.
Assessment of the Ln effect size in response to a change in UVB radiation indicated that both freshwater and marine photosynthetic organisms improved their performance when UVB was reduced; there were no statistically significant differences between their mean Ln effect size [freshwater: mean (±SE) Ln effect size = −0.537 ± 0.015; marine: mean (±SE) Ln effect size = −0.536 ± 0.012; one-way ANOVA, F = 0.00, p = 0.966; Figure 1]. However, closer inspection of the data revealed that marine microalgae exhibited the greatest improvement in their performance when UVB was reduced (Ln effect size = −0.672 ± 0.021, 22.7% higher than that of freshwater microalgae; one-way ANOVA, F = 25.69, p < 0.0001; Figure 1). Similarly, the improvement in the performance of marine macroalgae when UVB was reduced was 40.2% higher than that of the performance of freshwater macroalgae (one-way ANOVA, F = 4.88, p = 0.027; Figure 1). Both freshwater and marine photosynthetic organisms exhibited damage when UVB was elevated above ambient levels, with the greatest damage observed in marine microalgae (Ln effect size = 0.572 ± 0.037, which was 72.3% higher than that of freshwater microalgae; one-way ANOVA, F = 32.15, p < 0.0001; Figure 1b). In general, marine photosynthetic organisms were more sensitive (by 73.2%) to elevated UVB radiation than were freshwater photosynthetic organisms (one-way ANOVA, F = 55.41, p < 0.0001; Figure 1).
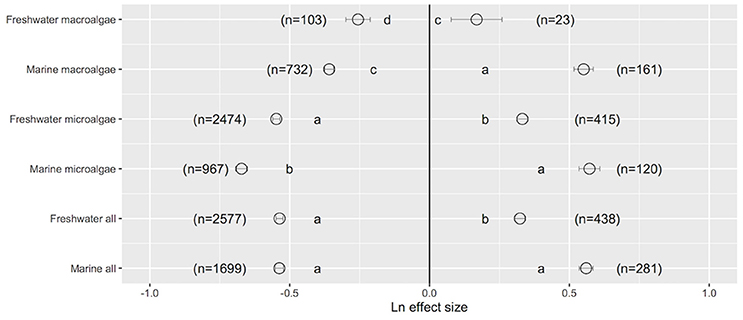
Figure 1. The mean ± SE Ln effect size quantifying the response of freshwater (FW) and marine (Mar) photosynthetic organisms to experimentally reduced or increased UVB radiation (relative to control). A Ln effect size <0 indicates an improvement in the trait and a Ln effect size >0 indicates a deterioration in performance. “n” is the number of observations.
Rhodophyta showed the greatest improvement in their performance when UVB was reduced (Ln effect size = −0.411 ± 0.079; Figure 2a). Charophyta was more resistance than Chlorophyta to increased UVB radiation (Charophyta: Ln effect size = 0.078 ± 0.059; Chlorophyta: Ln effect size = 0.273 ± 0.072; Figure 2a). The responses to increased UVB were generally consistent across marine macroalgae groups (Figure 2b). Diatoms exhibited the largest positive responses to reduced UVB radiation among all aquatic photosynthetic organisms (Ln effect size ~ −0.80), while whole communities exhibited the smallest positive responses (Ln effect size ~ −0.36; Figures 2c,d). Marine green microalgae experienced the greatest impact from increased UVB radiation among all aquatic photosynthetic organisms (Ln effect size ~ 0.79; Figure 2d).
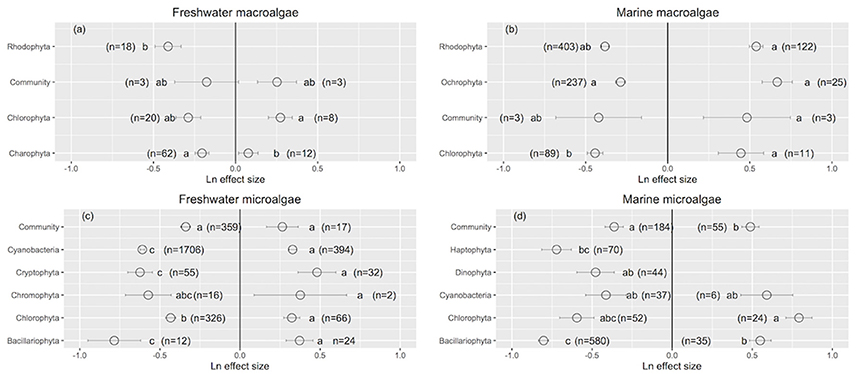
Figure 2. The mean ± SE Ln effect size for different taxonomic groups in response to experimentally reduced or increased UVB radiation for freshwater macroalgae (a), marine macroalgae (b), freshwater microalgae (c), and marine microalgae (d). A Ln effect size <0 indicates an improvement in the trait and a Ln effect size >0 indicates a deterioration in performance. “n” is the number of observations.
Cellular/molecular traits changed the most and growth rates changed the least when UVB radiation was reduced (Cellular/molecular: Ln effect size −0.599 ± 0.012 for overall dataset, −0.611 ± 0.024 for marine, and −0.594 ± 0.013 for freshwater photosynthetic organisms, Figures 3a–c, 4), although they did not differ to other response traits in some cases. Mortality changed the most in response to increased UVB radiation in marine photosynthetic organisms (0.677 ± 0.135, Figure 3b); no data were available assessing the change in mortality among freshwater photosynthetic freshwater organisms. Chlorophyll a concentrations changed the least in response to either increased or reduced UVB radiation (Figures 5a–c, 6), whereas photosynthetic parameters were more responsive, greatly deteriorating under increased UVB radiation and dramatically improving under reduced UVB radiation (Figures 5a–c, 6).
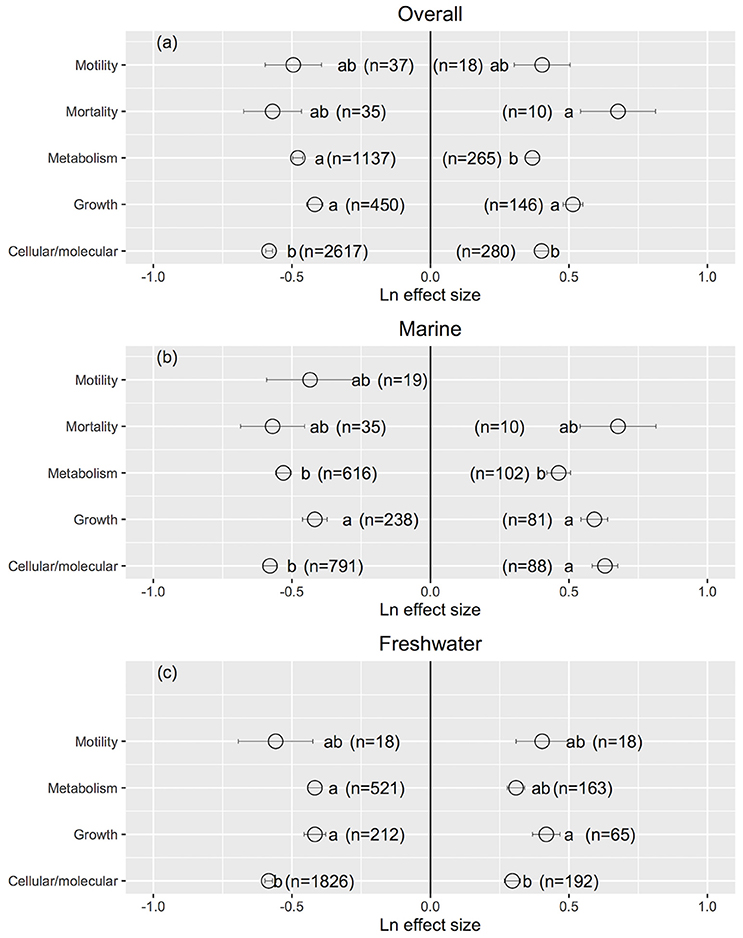
Figure 3. The mean ± SE Ln effect size for different response traits in response to experimentally reduced or increased UVB radiation for overall (a), marine (b) and freshwater photosynthetic organisms (c). A Ln effect size <0 indicates an improvement in the trait and a Ln effect size >0 indicates a deterioration in performance. “n” is the number of observations.
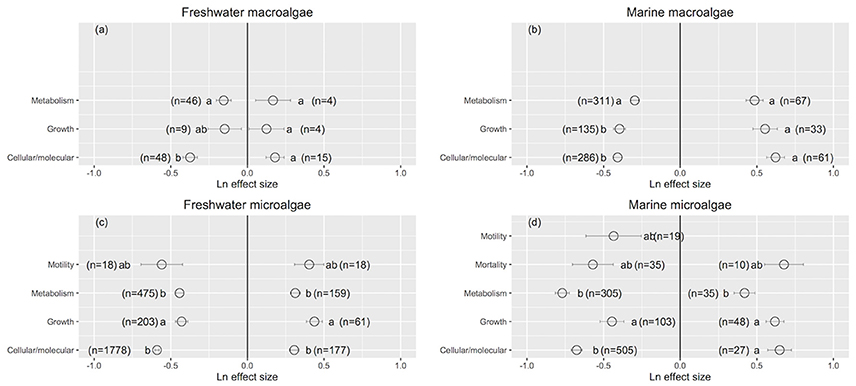
Figure 4. The mean ± SE Ln effect size for response traits in response to experimentally reduced or increased UVB radiation for freshwater macroalgae (a), marine macroalgae (b), freshwater microalgae (c), and marine microalgae (d). A Ln effect size <0 indicates an improvement in the trait and a Ln effect size >0 indicates a deterioration in performance. “n” is the number of observations.
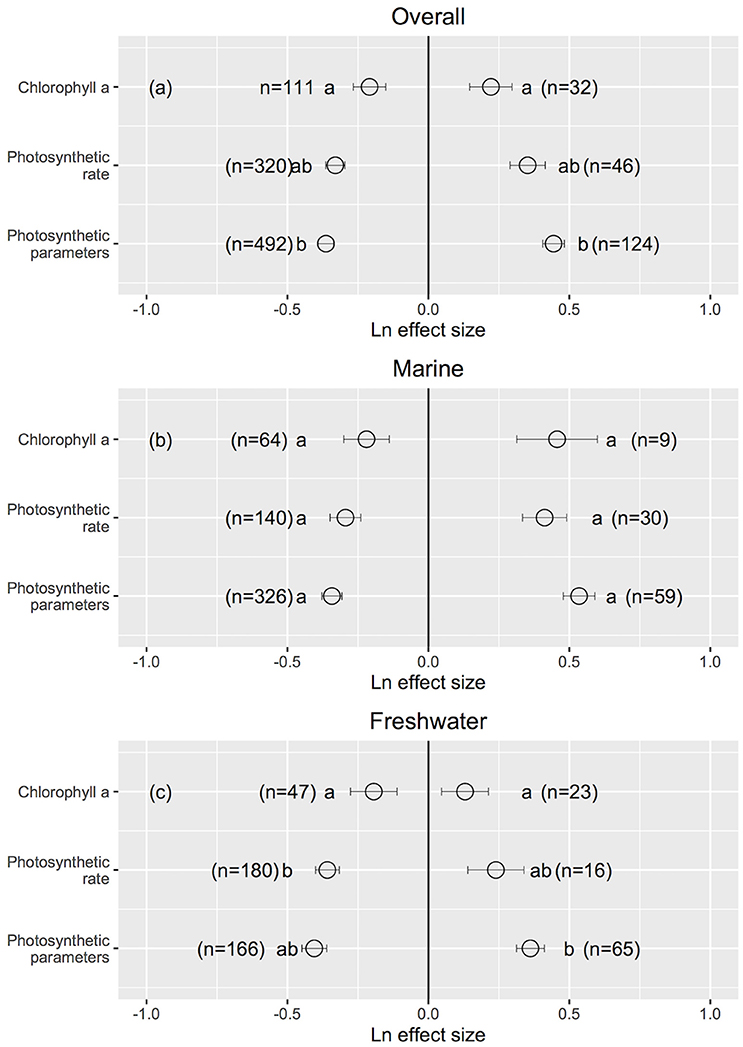
Figure 5. The mean ± SE Ln effect size for response traits of chlorophyll a, photosynthetic rate and photosynthetic parameters in response to experimentally reduced or increased UVB radiation for overall (a), marine (b) and freshwater photosynthetic organisms (c). A Ln effect size <0 indicates an improvement in the trait and a Ln effect size >0 indicates a deterioration in performance. “n” is the number of observations.
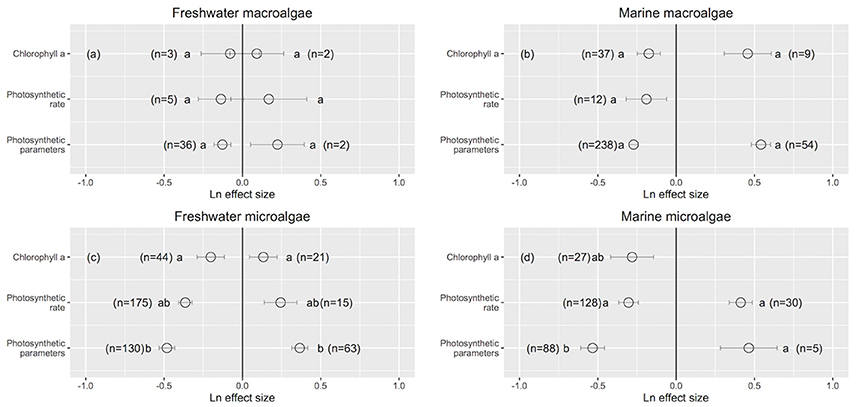
Figure 6. The mean ± SE Ln effect size for response traits of chlorophyll a, photosynthetic rate and photosynthetic parameters in response to experimentally reduced or increased UVB radiation for freshwater macroalgae (a), marine macroalgae (b), freshwater microalgae (c), and marine microalgae (d). A Ln effect size <0 indicates an improvement in the trait and a Ln effect size >0 indicates a deterioration in performance. “n” is the number of observations.
The response traits were, in general, relative insensitively to changes in UVB radiation [slope (Ln effect size vs. UVB relative) <1; Figures 7, 8], indicating that response traits tend to buffer, rather than to amplify changes in UVB radiation. However, marine photosynthetic organisms exhibited much higher sensitivity to UVB radiation than did freshwater organisms (marine: slope = 0.351 ± 0.021; freshwater: slope = 0.181 ± 0.013; ANOVA, t-test, p < 0.0001; Table 2; Figure 5a), as reflected in a significant interaction between the UVB relative ratio and habitat (marine/freshwater; ANOVA, t-test, p < 0.0001; Table 3, Figure 7a). Specifically, marine microalgae exhibited a similar sensitivity to UVB radiation across phyla (Figure 7a, Table 3). However, freshwater Bacillariophyta (diatoms) exhibited greater sensitivity to increasing UVB radiation (ANOVA, t-test, p = 0.05; Table 3; Figure 7c) than did other freshwater microalgae taxa. Red algae (Rhodophyta) were the most sensitive among the marine macroalgae phyla to UVB radiation with the slope of the Ln effect size in relation to the UVB relative ratio = 0.507 ± 0.023 (Figure 7d), although this result may be influenced by the fewer number of experiments on other taxa in which UVB radiation was increased (Figures 2a,b).
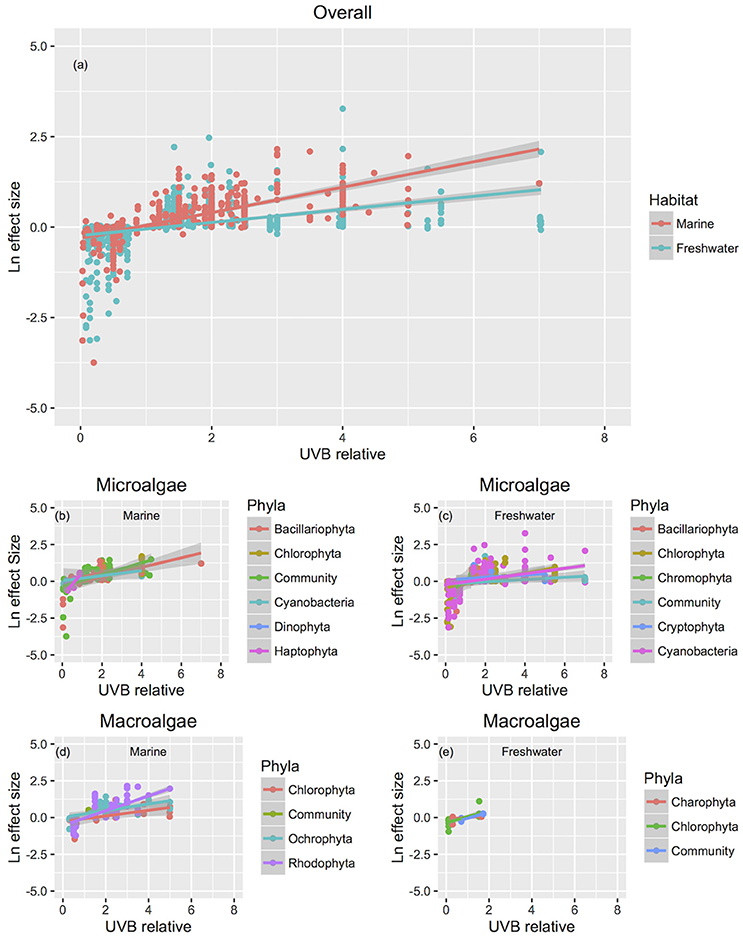
Figure 7. The relationship between biological responses and the relative change in UVB radiation with reference to different taxonomic groups. The relationship between the responses to experimental UVB manipulation, as indicated by the Ln effect size, and the change in UVB radiation relative to the ambient levels experienced by the photosynthetic organisms. Symbol colors identify different habitats (a) or taxonomic groups (b–e), and line colors represent the fitted regression equations. For the studies that UVB was totally removed, UVB relative was coded as 0.01, to allow log transformation of this variable. Each point represents an independent observation.
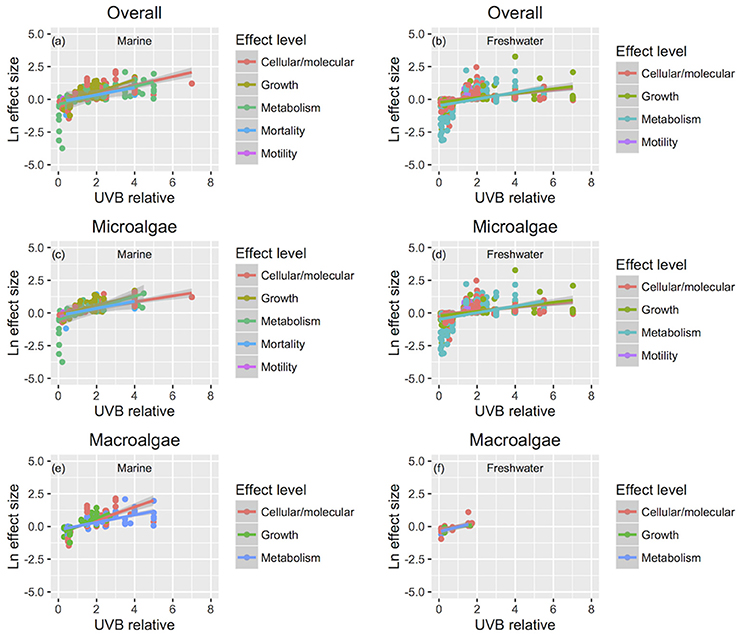
Figure 8. The relationship between changes in response traits and the change in UVB radiation. The relationship between the responses to experimental UVB manipulation, as indicated by the Ln effect size, and the change in UVB radiation relative to the ambient levels experienced by the photosynthetic organisms. Symbol colors identify different response traits for overall (a,b), microalgae (c,d), and macroalgae (e,f), and line colors identify the fitted regression equations. For the studies that UVB was totally removed, UVB relative was coded as 0.01, to allow log transformation of this variable. Each point represents an independent observation.
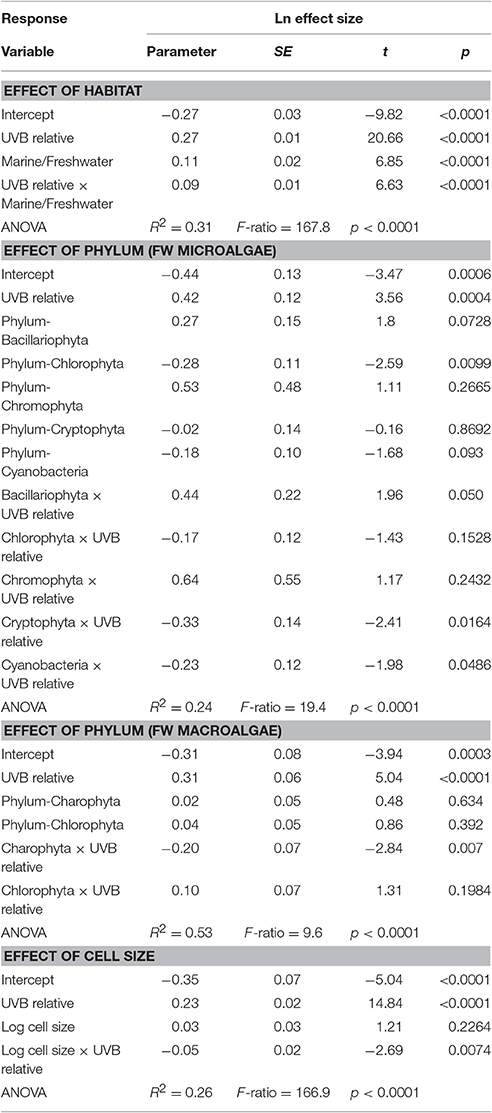
Table 3. General linear models showing the effect of the interaction between relative UVB radiation and habitat and between taxonomic groups and cell size.
The sensitivity of different response traits to changes in UVB radiation was doubled for marine photosynthetic organisms (slope ~ 0.35) compared with freshwater photosynthetic (slope ~ 0.18) organisms (including microalgae and macroalgae; Figures 8a,b). Furthermore, no significant interactions were found between any response trait and the UVB relative ratio, except for the growth of marine macroalgae, where the Ln effect size was higher under a higher UVB relative ratio than it was for other traits (ANOVA, t-test, p = 0.0148; Table 4; Figure 8e).
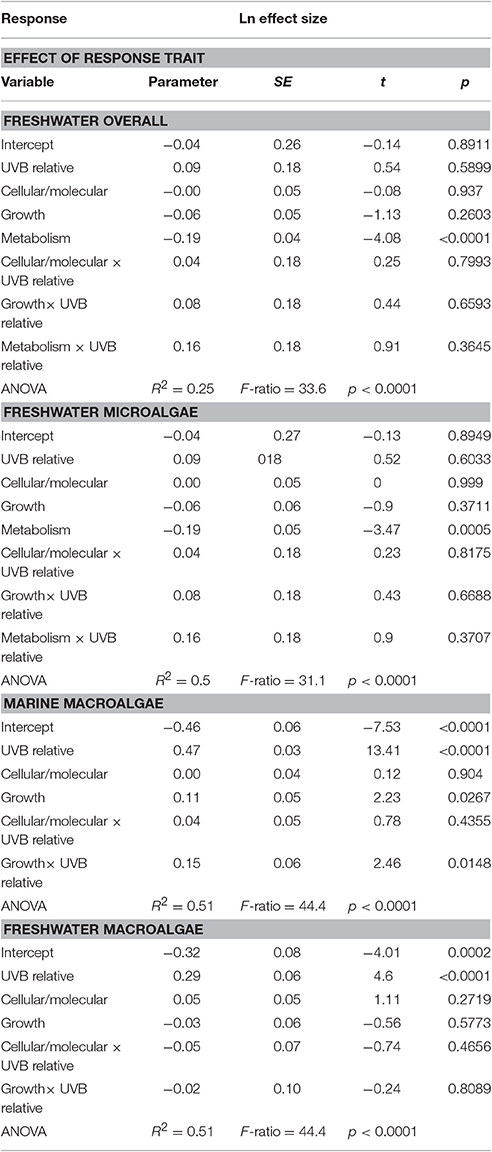
Table 4. General linear models showing the effect of the interaction between the relative UVB radiation and the response trait.
The morphology of macroalgae influenced their responses to ultraviolet radiation. When the UVB was reduced, foliose macroalgae (44% of Chlorophyta, 49% of Rhodophyta and 7% of Ochrophyta) exhibited a relatively higher improvement in their performance (foliose: Ln effect size −0.449 ± 0.035 for overall dataset; Figure 9a). Articulated calcareous macroalgae (100% of Rhodophyta) showed a relatively lower response to reduced UVB radiation, especially for marine algae (articulated calcareous: Ln effect size −0.174 ± 0.062; Figure 9b). No differences were observed in responses to reduced UVB radiation between the two freshwater morpho-functional groups (Figure 9c). In addition, no significant differences in the responses to increased UVB radiation were detected among different morpho-functional groups either in marine or freshwater ecosystem. This result is robust against the particularly functional-form classification chosen, as similar results were obtained when the macroalgae were classified according to Steneck and Watling (1982) and Littler and Littler (1980). However, the functional forms of marine macroalgae were not independent, in this data set, of the phylogenetic membership (R2 = 0.32, χ2-test, p < 0.0001), as all articulated calcareous algae and most corticated algae were red algae, most leathery algae were brown algae, and most filamentous algae were green algae, so the attribution of differences in response to UVB radiation among marine macroalgae to functional form maybe confounded by phylogenetic differences.
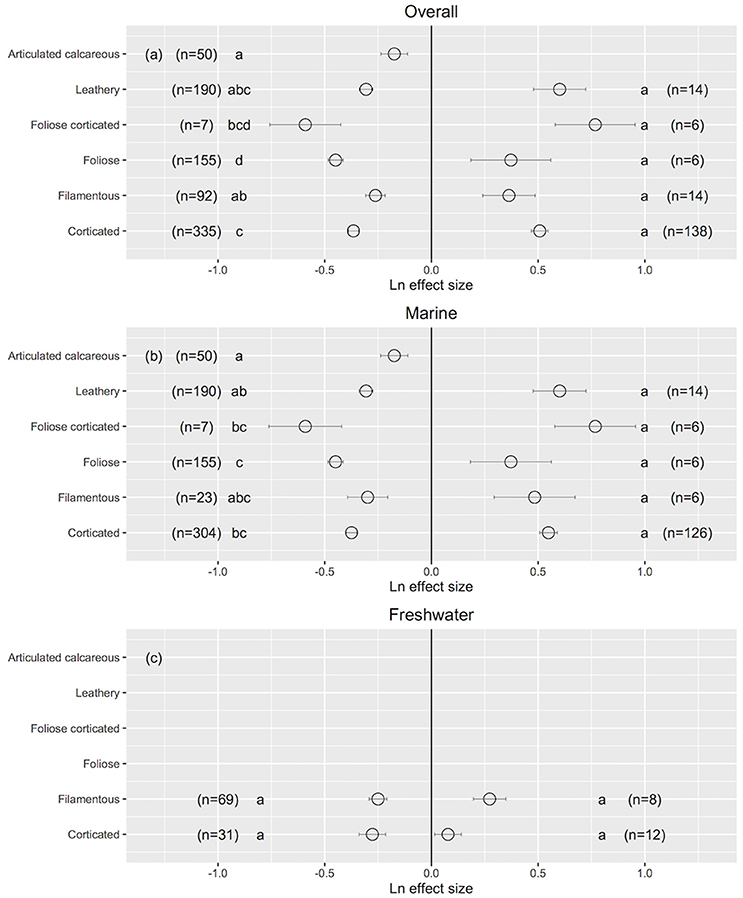
Figure 9. The mean ± SE Ln effect size for different morpho-functional groups in response to experimentally reduced or increased UVB radiation for overall (a), marine (b) and freshwater macroalgae (c). A Ln effect size <0 indicates an improvement in the trait and a Ln effect size >0 indicates a deterioration in performance. “n” is the number of observations.
The results confirmed our hypothesis that the response of microalgae to UVB radiation is dependent on cell size (Figure 10). Small-celled microalgae exhibited a much greater sensitivity to changes in UVB radiation than did large-celled microalgae (Figure 10). Indeed, the significant interaction of the log10 of the cell volume and the relative change in UVB radiation suggests that small-celled microalgae are more sensitive to changes in UVB radiation than are large-celled microalgae (ANOVA, p = 0.0074; Table 3; Figure 10).
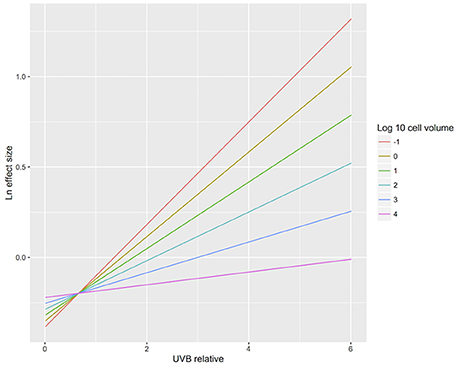
Figure 10. The relationship between biological responses and the change in UVB radiation for microalgae of different cell sizes. The relationship between the responses to experimental UVB manipulation, as indicated by the Ln effect size, and the change in UVB radiation relative to the ambient levels experienced by the photosynthetic organisms. Colour lines represent the fitted general linear models for microalgae across a range of cell sizes (log10 cell volume (μm3) = −1, 0, 1, 2, 3, and 4).
Discussion
It has been documented that photosynthetic organisms are, on average, more resistant to UVB radiation than other marine organisms in general (Llabrés et al., 2013). This meta-analysis further found that (1) marine photosynthetic organisms tend to be more sensitive than freshwater photosynthetic organisms to UVB radiation (Figures 1, 7); (2) responses to either decreased or increased UVB radiation vary among taxa (Figure 2); (3) the mortality rate is the most sensitive of the trait responses to elevated UVB radiation, followed by changes in cellular and molecular traits (Figure 3); and (4) as hypothesized, the sensitivity of microalgae to UVB radiation is dependent on size, with small-celled microalgae more sensitive than large-celled microalgae to UVB radiation (Figure 10).
UVB radiation that causes significant mortality among photosynthetic plankton has been reported to penetrate as deep as 60 m in the subtropical Atlantic Ocean (Llabrés and Agustí, 2006). However, the penetration of UVB in freshwater is generally much shallower than in marine waters because the concentrations of DOC and suspended particles, which attenuate UVB penetration in the water column, are typically much higher in freshwater than in marine water (Kirk, 1994). As a consequence, Bancroft et al. (2007) hypothesized that marine photosynthetic organisms experience greater risk of exposure to damaging UVB radiation than do freshwater photosynthetic organisms. Evidence of differential responses of marine and freshwater photosynthetic organisms to UVB radiation has not yet been reported. Indeed, Bancroft et al. (2007) did not find evidence of such differing responses. Although our meta-analysis results show that there were no significant differences between freshwater and marine photosynthetic organisms when UVB was reduced, they do show that marine photosynthetic organisms exhibited stronger sensitivity than freshwater photosynthetic organisms to increased UVB radiation (Figure 1). The discrepancy between Bancroft et al. (2007) results and our results is likely attributable to the vast difference in the scope of the two meta-analyses. We investigated 4995 responses to changes in UVB radiation, whereas the analysis of Bancroft et al. (2007) was based on six observations. Moreover, Bancroft et al. (2007) analyzed primary and secondary producers, whereas we studied micro- and macroalgae. Given our results, we expect that increased UVB radiation due to depletion of the stratospheric ozone layer (SOL) exerts a larger impact on marine primary producers than on freshwater primary producers. The impact on marine primary producers is likely to have been increased by the fact that the oligotrophic ocean, characterized by deep penetration of high UVB doses (Llabrés and Agustí, 2006; Tedetti et al., 2007) encompasses 70% of the surfaces of world's oceans and, thereby, a large fraction of the biosphere. However, ecosystem buffers may include many other factors (such as species-specific resistance, photo-adaptation capacities, nutrient levels, species habit), which were not assessed here, and these may add to the uncertainty in evaluating the impacts of elevated UVB radiation on marine ecosystems.
The Montreal Protocol largely halted further damage to the SOL, with a recovery of the SOL expected in the coming decades. Furthermore, it was reported that the healing of the Antarctic ozone layer is occurring nowadays since 2000 (Solomon et al., 2016). The relationship between the reduction in UVB radiation and the performance of primary producers might, thus, help to forecast the expected response of marine primary producers in a future scenario of ozone recovery (Bais et al., 2011). Although our comprehensive analysis showed that photosynthetic organisms in marine ecosystems are more sensitive than those in freshwater ecosystems to increased UVB, the photosynthetic organisms in alpine lakes, which were characterized by high UVB penetration and transparency, should be at higher risk of exposure to damaging UVB radiation than photosynthetic organisms in lowland freshwater ecosystems or in coastal waters of marine ecosystems.
UVB radiation affects pigment synthesis in aquatic photosynthetic organisms, stimulating the production of UVB-screening pigments (Klisch et al., 2002). However, the concentration of chlorophyll a was less sensitive to changes in UVB radiation than were photosynthetic traits (Figure 6). This finding is in contrast to reports that elevated UVB radiation reduces the chlorophyll a content of photosynthetic organisms through photo-bleaching (Agrawal, 1992, 1996) or that, in contrast, UVB radiation leads to increased chlorophyll a concentration in other organisms (Grobe and Murphy, 1998; Zhang et al., 2013). However, the underlying mechanism for these changes has not been documented (Grobe and Murphy, 1998). Phytoplankton have developed several mechanisms to cope with a wide range of light densities as well as different spectra of irradiance, including massive changes in the cellular content of both light-harvesting and photo-protective pigments, adjustments in their ultrastructures (Berner et al., 1989; Fisher et al., 1998) and regulation of the absorption cross section of photosystem II (σPSII) (e.g., Six et al., 2007). Specifically, photosynthetic parameters are sensitive to changes in UVB radiation, suggesting that downstream photosynthesis processes (e.g., photosynthetic electron transport) are more vulnerable than upstream processes to UVB radiation (e.g., light capture by chlorophyll a). Several studies report declines in photosynthetic activity (e.g., a decline in the maximum photosystem II efficiency) with increased UVB radiation (Rai and Mallick, 1998; Sinha et al., 2008; Zhang et al., 2013). However, it has also been documented that photosynthetic activity varies independently from the photosystem II content (Jeans et al., 2014), which is in agreement with our findings.
Cell size has long been proposed as a determinant of sensitivity to ultraviolet radiation (UVR) in phytoplankton. Larger cells are thought to be more resistant to UVR-induced damage due to their smaller surface-area-to-volume ratio, i.e., a. longer path length to the nucleus, which makes them more resistant to accumulation of cyclobutane pyrimidine dimers (CPD) and other forms of damage due to molecular self-shading (Garcia-Pichel, 1994). However, there are also a number of exceptions have been reported (Laurion and Vincent, 1998; Ferreyra et al., 2006). For example, Laurion and Vincent (1998) found that the smallest-celled phytoplankton are relatively resistant to UVR, and this may due to the fact that smaller cells are more vulnerable to DNA damage but more resistant to photosystem damage (Villafañe et al., 2003), or to the fact that smaller cells have faster biosynthetic rates (Peters, 1983). Furthermore, taxon-specific factors for mitigating UVR photodamage have the potential to compensate for size-related vulnerability (Laurion and Vincent, 1998). Our finding that UVB sensitivity in microalgae is dependent on size is consistent with previous reports of the size dependence of the optical properties of microalgae (Agustí, 1991), suggesting higher specific absorption of UVB radiation by small algae, and a recent report that the UV sensitivity of diatoms was negatively correlated with cell size (Wu et al., 2015). Our results also show that the differences in sensitivity between small-celled and large-celled phytoplankton are less important at low UVB irradiances, but that they increased with irradiance as the effect size of UVB radiation increased. Picophytoplankton (cell diameter <2 μm) live both in freshwaters and marine waters, but they dominate the oligotrophic ocean (Agawin et al., 2000). The greater sensitivity of small-celled phytoplankton to UVB radiation is, therefore, consistent with the reported sensitivity of Prochlorococcus and Synechococcus to UV radiation in the oligotrophic ocean, resulting in high cell mortality (Llabrés and Agustí, 2006; Agustí and Llabrés, 2007). Moreover, our results suggest that primary producers may have been heavily impacted by increased UVB radiation due to the erosion of the SOL in the oligotrophic ocean, consistent with evidence of a recent trend toward oligotrophication of the subtropical and tropical ocean (Behrenfeld et al., 2006; Boyce et al., 2010; Henson et al., 2010; Steinacher et al., 2010; Hofmann et al., 2011). Warming of the ocean is predicted to lead to a further expansion of picophytoplankton (Morán et al., 2010; Flombaum et al., 2013; Chen et al., 2014; Nagelkerken and Connell, 2015), suggesting an increase in the sensitivity of marine primary producers to UVB radiation. On the other hand, the predicted recovery of the SOL, with the associated decline in UVB radiation, may lead to improved performance of picophytoplankton in the future, but this improvement may be offset by a deeper penetration of UVB radiation in a warmer, more oligotrophic ocean.
Although the relationships between thickness or spore size and UVB levels in macroalgae were not determined in our analysis due to a limited number of studies reporting these, previous studies showed that these factors also affect their responses to UVB radiation. As translucence or opacity of thallus influences optical properties, such as reflection, attenuation, scattering, absorption, or transmittance of UVB radiation (Caldwell et al., 1983), UVB can, therefore, be attenuated by cell walls of the epidermal tissue effectively reducing UVB effect. For instance, macroalgae with thick thallus offer a longer pathlength to solar radiation resulting in outer cell layers shading inner ones (Roleda et al., 2006). Other protection mechanism described for macroalgae include the development of phlorotannin-containing paraphysis cells towering above the sporangial layer and physodes inside the meiospore-containing sporangium, which protect the soral tissue from UVR exposure (Gruber et al., 2011; Holzinger et al., 2011). Our results showed that foliose macroalgae exhibited the greatest improvement in their performance when UVB is reduced. Gómez and Huovinen (2011) described that foliose forms were able to acclimate rapidly to changing light. Therefore, their performance would be expected to improve rapidly when UVB was reduced, as showed in the present study. Freshwater macroalgae showed limited differentiation in morpho-functional groups, restricted to two groups in our data set, and phyla classification was more informative on their responses to UVB. For marine macroalgae, filamentous algae (e.g., Cladophora), which are typically thinner than the foliose algae (e.g., Porphyra), showed significantly smaller responses to reduced UVB radiation. Leathery algae (e.g., Laminaria), which are much thicker than the filamentous algae, showed comparable responses when UVB was reduced. Our data suggest that morpho-functional grouping account for some of the variation in the responses of marine macroalgae to UVB radiation, but that their responses may be buffered or amplified by many other factors besides functional form. Other factors (e.g., life-history stages, Roleda et al., 2007 and references therein; meiospore size, Swanson and Druehl, 2000) may also affect the responses of macroalgae to UVB radiation, but could not be assessed in present study because of limited number of studies addressing them. Moreover, the covariation between functional forms of marine macroalgae and their phylogenetic membership in our data set implies that the attribution of differences in response to UVB radiation among marine macroalgae to functional form maybe confounded by phylogenetic differences, as functional form classes differ in thallus thickness, which affects light absorption, but phylogenetic differences are associated with relevant differences, such as pigmentary compliments, which may also affect resistance to UVB radiation.
Our analysis provides evidence that sensitivity to UVB radiation is dependent on the phylum in microalgae. The lower sensitivity to UVB radiation of Chlorophyta compared with Chromophyta has been proposed to be due to the larger minimal cross-cell or cross-colony/cenobium distance of Chlorophyta, which can provide shading to the internal structures (Xiong et al., 1996). However, the greater sensitivity of Chlorophyta compared with Bacillariophyta to increased UVB radiation identified here remains unexplained, likely conditioned by the number of experiments (few) and the complex array of UVB damage that targets multiple processes and elicites a large number of protection mechanisms (Häder et al., 2015). Because UVB sensitivity differs among different taxa, it likely plays a crucial role in shaping phytoplankton community composition. Xenopoulos and Frost (2003) found that phytoplankton assemblages incubated under photosynthetic active radiation (PAR) had significantly higher richness, diversity and evenness than those incubated full spectrum irradiance, suggesting possible selection of UVR-tolerant taxa. Consequently, the full phytoplankton community should be more resistant to UVB than a single species or a taxon would be due to the selected effects of UVB. Our results showed that the full community exhibited the smallest responses to UVB (Figure 2). Reports that algal species originally isolated from high-UV radiation locations (Larson et al., 1990; Rautenberger et al., 2013) and marine organisms from the Southern Hemisphere that are subject to higher UVB radiation (Agustí et al., 2015) are particularly resistant to UVB radiation indicate that the history of UV radiation experienced by different taxonomic groups may also account for their diverse responses to UVB radiation. Furthermore, sensitivity to UVB will also imply other aspects than those analyzed in this meta-analysis, as it is expected some responses should be not related to phylum but show large variability among species within the same phylum (e.g., Xiong et al., 1996), therefore, we suggest that more studies on both micro- and macroalgae sensitivity to UVB should be conducted, and on the underlying mechanisms resulting in different responses are worthy of further investigation as well.
Our study confirmed the expected dominance of adverse effects of UVB radiation on the performance of aquatic photosynthetic organisms. However, a small number of observations showed a positive response to enhanced UVB (3.06% of the experiments showed improved performance with increased UVB and 5.78% of the experiments showed reduced performance with reduced UVB). Similarly, Flores-Moya et al. (1999) reported that in the marine alga Dictyota dichotoma, recovery of photoinhibition is delayed if the natural UVB radiation range is removed from the solar-spectrum. This was later confirmed in several macroalgae species (e.g Chara fibrosa), which were collected in shallow waters and have adapted to high UVB radiation environment (Hanelt et al., 2006; Hanelt and Roleda, 2009). A positive effect of UVB radiation has been reported for phytoplankton, where some taxa were most abundant in treatments of intermediate fluxes of UVB radiation (Thomson et al., 2008). Mechanistically, UVB may support repair or photoprotective processes (Sicora et al., 2003) or function as a photoreceptor signal (Hanelt and Roleda, 2009), leading to enhanced recovery of photoinhibition induced by high levels of solar radiation would be better under full solar radiation. Further studies are required to examine the molecular/physiological mechanisms of this photo-physiological function of UVB radiation.
The ocean is undergoing multiple changes including acidification, warming and deoxygenation (Gattuso et al., 2015), which also experiencing elevated UVB levels (Duarte et al., 2009). A vast number of studies have demonstrated that these other stressors could interact antagonistically or synergistically with UVB to influence aquatic biota (e.g., UVB and ocean acidification: Li et al., 2012; Jin et al., 2013; UVB and warming: Banaszak and Lesser, 2009; Xu et al., 2011; UVB and pollutants: Pelletier et al., 2006; UVB and nutrients: Li et al., 2015). Hence, the power of the analysis reported here, focused on the response of aquatic photosynthetic organisms to UVB radiation, is limited by current uncertainties owing to the nature and strength of interactions with other stressors. We, therefore, propose that more comprehensive analysis aiming at the interactive effects of multiple stressors with UVB radiation should be conducted for a better understanding of the consequences stressors for aquatic ecosystem. Our analysis already points to the great vulnerability of marine photosynthetic organisms to UVB radiation, with particularly important consequences for the vast span of oligotrophic ocean dominated by highly UVB-sensitive picophytoplankton.
Author Contributions
SA, CD, and PJ conceived and designed the research. PJ, SA, and CD acquired the data and all authors analyzed and interpreted the data. PJ drafted the work and all authors critically revised the work for intellectual content. All authors approved the final version to be published and agreed to accountable for all aspects of the work.
Funding
This research was funded by King Abdullah University of Science and Technology (KAUST) through the baseline funding to SA.
Conflict of Interest Statement
The authors declare that the research was conducted in the absence of any commercial or financial relationships that could be construed as a potential conflict of interest.
Acknowledgments
We thank Dorte Krause-Jensen (Aarhus University, Denmark) and Beatriz Martinez-Daranas (Univ. of La Habana, Cuba) for advice and help with the taxonomy and functional form classification of macroalgae.
References
Agawin, N. S., Duarte, C. M., and Agustí, S. (2000). Nutrient and temperature control of the contribution of picoplankton to phytoplankton biomass and production. Limnol. Oceanogr. 45, 591–600. doi: 10.4319/lo.2000.45.8.1891a
Agrawal, S. (1992). Effects of supplemental UV-B radiation on photosynthetic pigment, protein and glutathione contents in green algae. Environ. Exp. Bot. 32, 137–143.
Agrawal, S. (1996). Effect of enhanced ultraviolet-B radiation on growth, pigmentation, nitrogen metabolism and polyamine contents in a cyanobacterium Nostoc muscorum. Biotronics 25, 1–9.
Agustí, S. (1991). Allometric scaling of light absorption and scattering by phytoplankton cells. Can. J. Fish. Aquat. Sci. 48, 763–767.
Agustí, S., and Llabrés, M. (2007). Solar radiation-induced mortality of marine pico-phytoplankton in the oligotrophic ocean. Photochem. Photobiol. 83, 793–801. doi: 10.1111/j.1751-1097.2007.00144.x
Agustí, S., Llabrés, M., Carreja, B., Fernández, M., and Duarte, C. M. (2015). Contrasting sensitivity of marine biota to UV-B radiation between Southern and Northern hemispheres. Estuar. Coast. 38, 1126–1133. doi: 10.1007/s12237-014-9790-9
Bais, A., Tourpali, K., Kazantzidis, A., Akiyoshi, H., Bekki, S., Braesicke, P., et al. (2011). Projections of UV radiation changes in the 21st century: impact of ozone recovery and cloud effects. Atmos. Chem. Phys. 11, 7533–7545. doi: 10.5194/acp-11-7533-2011
Banaszak, A. T., and Lesser, M. P. (2009). Effects of solar ultraviolet radiation on coral reef organisms. Photochem. Photobiol. Sci. 8, 1276–1294. doi: 10.1039/b902763g
Bancroft, B. A., Baker, N. J., and Blaustein, A. R. (2007). Effects of UVB radiation on marine and freshwater organisms: a synthesis through meta-analysis. Ecol. Lett. 10, 332–345. doi: 10.1111/j.1461-0248.2007.01022.x
Behrenfeld, M. J., O'Malley, R. T., Siegel, D. A., McClain, C. R., Sarmiento, J. L., Feldman, G. C., et al. (2006). Climate-driven trends in contemporary ocean productivity. Nature 444, 752–755. doi: 10.1038/nature05317
Berner, T., Dubinsky, Z., Wyman, K., and Falkowski, P. G. (1989). Photoadaptation and the “package” effect in Dunaliella tertiolecta (Chlorophyceae). J. Phycol. 25, 70–78.
Boyce, D. G., Lewis, M. R., and Worm, B. (2010). Global phytoplankton decline over the past century. Nature 466, 591–596. doi: 10.1038/nature09268
Buma, A. G., Boelen, P., and Jeffrey, W. H. (2003). “UVR-induced DNA damage in aquatic organisms” in UV Effects in Aquatic Organisms and Ecosystems, eds E. W. Helbling and H. Zagarese (Cambridge: Royal Society of Chemistry), 291–327.
Caldwell, M. M., Björn, L. O., Bornman, J. F., Flint, S. D., Kulandaivelu, G., Teramura, A. H., et al. (1998). Effects of increased solar ultraviolet radiation on terrestrial ecosystems. J. Photochem. Photobiol. B 46, 40–52. doi: 10.1016/S1011-1344(98)00184-5
Caldwell, M. M., Robberecht, R., and Flint, S. D. (1983). Internal filters: prospects for UV acclimation in higher plants. Physiol. Plant 58, 445–450.
Chen, B., Liu, H., Huang, B., and Wang, J. (2014). Temperature effects on the growth rate of marine picoplankton. Mar. Ecol. Prog. Ser. 505, 37–47. doi: 10.3354/meps10773
Dring, M. J., Makarov, V., Schoschina, E., Lorenz, M., and Lüning, K. (1996). Influence of ultraviolet-radiation on chlorophyll fluorescence and growth in different life-history stages of three species of Laminaria (Phaeophyta). Mar. Biol. 126, 183–191.
Duarte, C. M., Conley, D. J., Carstensen, J., and Sánchez-Camacho, M. (2009). Return to Neverland: shifting baselines affect eutrophication restoration targets. Estuar. Coast. 32, 29–36. doi: 10.1007/s12237-008-9111-2
Duarte, C. M., Sand-Jensen, K., Nielsen, S. L., Enríquez, S., and Agustí, S. (1995). Comparative functional plant ecology: rationale and potentials. Trends. Ecol. Evol. 10, 418–421. doi: 10.1016/S0169-5347(00)89163-6
Ferreyra, G. A., Mostajir, B., Schloss, I. R., Chatila, K., Ferrario, M. E., Sargian, P., et al. (2006). Ultraviolet-B radiation effects on the structure and function of lower trophic levels of the marine planktonic food web. Photochem. Photobiol. 82, 887–897. doi: 10.1562/2006-02-23-RA-810
Figueroa, F. L., Domínguez-González, B., and Korbee, N. (2014). Vulnerability and acclimation to increased UVB radiation in three intertidal macroalgae of different morpho-functional groups. Mar. Environ. Res. 97, 30–38. doi: 10.1016/j.marenvres.2014.01.009
Figueroa, F. L., Mercado, J., Jiménez, C., Salles, S., Aguilera, J., Sánchez-Saavedra, M. P., et al. (1997). Relationship between bio-optical characteristics and photoinhibition of phytoplankton. Aquat. Bot. 59, 237–251.
Fisher, T., Berner, T., Iluz, D., and Dubinsky, Z. (1998). The kinetics of the photoacclimation response of Nannochloropsis sp.(Eustigmatophyceae): a study of changes in ultrastructure and PSU density. J. Phycol. 34, 818–824. doi: 10.1046/j.1529-8817.1998.340818.x
Flombaum, P., Gallegos, J. L., Gordillo, R. A., Rincón, J., Zabala, L. L., Jiao, N., et al. (2013). Present and future global distributions of the marine Cyanobacteria Prochlorococcus and Synechococcus. Proc. Natl. Acad. Sci. U.S.A. 110, 9824–9829. doi: 10.1073/pnas.1307701110
Flores-Moya, A., Hanelt, D., Figueroa, F. L., Altamirano, M., Viñegla, B., Altamirano, M., et al. (1999). Involvement of solar UV-B radiation in recovery of inhibited photosynthesis in brown alga Dictyota dichotoma (Hudson) Lamouroux. J. Photochem. Photobiol. B Biol. 49, 129–135.
Franklin, L. A., and Foster, R. M. (1997). The changing irradiance environment: consequences for marine macrophyte physiology, productivity and ecology. Eur. J. Phycol. 32, 207–232.
Garcia-Pichel, F. (1994). A model for internal self-shading in planktonic organisms and its implications for the usefulness of ultraviolet sunscreens. Limnol. Oceanogr. 39, 1704–1717.
Gattuso, J.-P., Magnan, A., Billé, R., Cheung, W., Howes, E., Joos, F., et al. (2015). Contrasting futures for ocean and society from different anthropogenic CO2 emissions scenarios. Science 349:aac4722. doi: 10.1126/science.aac4722
Gómez, I., and Huovinen, P. (2011). Morpho-functional patterns and zonation of South Chilean seaweeds: the importance of photosynthesis and bio-optic traits. Mar. Ecol. Prog. Ser. 422, 77–91. doi: 10.3354/meps08937
Graham, M. H., Kinlan, B. P., Druehl, L. D., Garske, L. E., and Banks, S. (2007). Deep-water kelp refugia as potential hotspots of tropical marine diversity and productivity. Proc Natl Acad Sci. U.S.A. 104, 16576–16580. doi: 10.1073/pnas.0704778104
Grobe, C. W., and Murphy, T. M. (1998). Solar ultraviolet-B radiation effects on growth and pigment composition of the intertidal alga Ulva expansa (Setch.) S. & G. (Chlorophyta). J. Exp. Mar. Biol. Ecol. 225, 39–51. doi: 10.1016/S0022-0981(97)00210-4
Gruber, A., Roleda, M. Y., Barstch, I., Hanelt, D., and Wiencke, C. (2011). Sporogenesis under ultraviolet radiation in Laminaria digitata (Phaeophyceae) reveals protection of photosentive meiospores within soral tissue: physiological and anatomical evidence. J. Phycol. 47, 603–614. doi: 10.1111/j.1529-8817.2011.00998.x
Häder, D.-P., Helbling, E., Williamson, C., and Worrest, R. (2011). Effects of UV radiation on aquatic ecosystems and interactions with climate change. Photochem. Photobiol. Sci. 10, 242–260. doi: 10.1039/C0PP90036B
Häder, D. P., Kumar, H., Smith, R., and Worrest, R. (2007). Effects of solar UV radiation on aquatic ecosystems and interactions with climate change. Photochem. Photobiol. Sci. 6, 267–285. doi: 10.1039/b700020k
Häder, D. P., Williamson, C. E., Wängberg, S. Å., Rautio, M., Rose, K. C., Gao, K., et al. (2015). Effects of UV radiation on aquatic ecosystems and interactions with other environmental factors. Photochem. Photobiol. Sci. 14, 108–126. doi: 10.1039/C4PP90035A
Halac, S., Villafañe, V., Gonçalves, R., and Helbling, E. W. (2014). Photochemical responses of three marine phytoplankton species exposed to ultraviolet radiation and increased temperature: role of photoprotective mechanisms. J. Photochem. Photobiol. B 141, 217–227. doi: 10.1016/j.jphotobiol.2014.09.022
Hanelt, D., Hawes, I., and Rae, R. (2006). Reduction of UV-B radiation causes an enhancement of photoinhibition in high light stressed aquatic plants from New Zealand lakes. J. Photochem. Photobiol. B 84, 89–102. doi: 10.1016/j.jphotobiol.2006.01.013
Hanelt, D., and Roleda, M. Y. (2009). UVB radiation may ameliorate photoinhibition in specific shallow-water tropical marine macrophytes. Aquat. Bot. 91, 6–12. doi: 10.1016/j.aquabot.2008.12.005
Hanelt, D., Tüg, H., Bischof, K., Groß, C., Lippert, H., Sawall, T., et al. (2001). Light regime in an Arctic fiord: a study related to stratospheric ozone depletion as a basis for determination of UV effects on algal growth. Mar. Biol. 138, 649–658. doi: 10.1007/s002270000481
Helbling, E. W., Buma, A. G., van de Poll, W., Zenoff, M. V. F., and Villafañe, V. E. (2008). UVR-induced photosynthetic inhibition dominates over DNA damage in marine dinoflagellates exposed to fluctuating solar radiation regimes. J. Exp. Mar. Biol. Ecol. 365, 96–102. doi: 10.1016/j.jembe.2008.07.044
Henson, S. A., Sarmiento, J. L., Dunne, J. P., Bopp, L., Lima, I. D., Doney, S. C., et al. (2010). Detection of anthropogenic climate change in satellite records of ocean chlorophyll and productivity. Biogeosciences 7, 621–640. doi: 10.5194/bg-7-621-2010
Hofmann, M., Worm, B., Rahmstorf, S., and Schellnhuber, H. (2011). Declining ocean chlorophyll under unabated anthropogenic CO2 emissions. Environ. Res. Lett. 6:034035. doi: 10.1088/1748-9326/6/3/034035
Holzinger, A., Karsten, U., Lütz, C., and Wiencke, C. (2006). Ultrastructure and photosynthesis in the supralittoral green macroalga Prasiola crispa from Spitsbergen (Norway) under UV exposure. Phycologia 45, 168–177. doi: 10.2216/05-20.1
Holzinger, A., Lütz, C., and Karsten, U. (2011). Desiccation stress causes structural and ultra-structural alterations in the aeroterrestrial green alga Klebsormidium crenulatum (Klebsormidiophyceae, Streptophyta) isolated from an alpine soil crust. J. Phycol. 47, 591–602. doi: 10.1111/j.1529-8817.2011.00980.x
Jeans, J., Szabó, M., Campbell, D., Larkum, A., Ralph, P., and Hill, R. (2014). Thermal bleaching induced changes in photosystem II function not reflected by changes in photosystem II protein content of Stylophora pistillata. Coral Reefs 33, 131–139. doi: 10.1007/s00338-013-1091-4
Jiang, H., and Qiu, B. (2005). Photosynthetic adaptation of a bloom-forming cyanobacterium Microcystis aeruginosa (Cyanophyceae) to prolonged UV-B exposure. J. Phycol. 41, 983–992. doi: 10.1111/j.1529-8817.2005.00126.x
Jin, P., Gao, K., Villafañe, V. E., Campbell, D. A., and Helbling, W. (2013). Ocean acidification alters the photosynthetic responses of a coccolithophorid to fluctuating UV and visible radiation. Plant Physiol. 162, 2084–2094. doi: 10.1104/pp.113.219543
Kirk, J. T. O. (1994). Light and Photosynthesis in Aquatic Ecosystems. Cambridge: Cambridge University Press.
Klisch, M., Sinha, R., and Hfider, D.-P. (2002). UV-absorbing compounds in algae. Curr. Top. Plant Biol. 3, 113–120.
Kumar, A., Sinha, R. P., and Häder, D.-P. (1996). Effect of UV-B on enzymes of nitrogen metabolism in the cyanobacterium Nostoc calcicola. J. Plant Physiol. 148, 86–91. doi: 10.1016/S0176-1617(96)80298-7
Larson, R., Garrison, W., and Carlson, R. (1990). Differential responses of alpine and non-alpine Aquilegia species to increased ultraviolet-B radiation. Plant Cell Physiol. 13, 983–987. doi: 10.1111/j.1365-3040.1990.tb01990.x
Laurion, I., and Vincent, W. F. (1998). Cell size versus taxonomic composition as determinants of UV-sensitivity in natural phytoplankton communities. Limnol. Oceanogr. 43, 1774–1779.
Li, G., and Gao, K. (2013). Cell size-dependent effects of solar UV radiation on primary production in coastal waters of the South China Sea. Estuar. Coast. 36, 728–736. doi: 10.1007/s12237-013-9591-6
Li, W., Gao, K., and Beardall, J. (2015). Nitrate limitation and ocean acidification interact with UV-B to reduce photosynthetic performance in the diatom Phaeodactylum tricornutum. Biogeosciences 12, 2383–2393. doi: 10.5194/bg-12-2383-2015
Li, Y., Gao, K., Villafañe, V., and Helbling, E. (2012). Ocean acidification mediates photosynthetic response to UV radiation and temperature increase in the diatom Phaeodactylum tricornutum. Biogeosciences 9, 3931–3942. doi: 10.5194/bg-9-3931-2012
Littler, M., and Littler, D. (1980). The evolution of thallus form and survival strategies in benthic marine macroalgae: field and laboratory tests of a functional form model. Am. Nat. 116, 25–44.
Llabrés, M., and Agustí, S. (2006). Picophytoplankton cell death induced by UV radiation: evidence for oceanic Atlantic communities. Limnol. Oceanogr. 51, 21–29. doi: 10.4319/lo.2006.51.1.0021
Llabrés, M., Agustí, S., Fernández, M., Canepa, A., Maurin, F., Vidal, F., et al. (2013). Impact of elevated UVB radiation on marine biota: a meta-analysis. Global Ecol. Biogeogr. 22, 131–144. doi: 10.1111/j.1466-8238.2012.00784.x
Madronich, S., McKenzie, R. L., Björn, L. O., and Caldwell, M. M. (1998). Changes in biologically active ultraviolet radiation reaching the Earth's surface. J. Photochem. Photobiol. B 46, 5–19. doi: 10.1016/S1011-1344(98)00182-1
Molina, M. J., and Rowland, F. S. (1974). Stratospheric sink for chlorofluoromethanes: chlorine atom-catalysed destruction of ozone. Nature 249, 810–812.
Morán, X. A. G., López-Urrutia, Á., Calvo-Díaz, A., and Li, W. K. (2010). Increasing importance of small phytoplankton in a warmer ocean. Global Change Biol. 16, 1137–1144. doi: 10.1111/j.1365-2486.2009.01960.x
Moroz, A. L., Ehrman, J. M., Clair, T. A., Gordon, R. J., and Kaczmarska, I. (1999). The impact of ultraviolet-B radiation on the motility of the freshwater epipelic diatom Nitzschia lineariz. Global Change Biol. 5, 191–199. doi: 10.1046/j.1365-2486.1999.00225.x
Nagelkerken, I., and Connell, S. D. (2015). Global alteration of ocean ecosystem functioning due to increasing human CO2 emissions. Proc. Natl. Acad. Sci. U.S.A.112, 13272–13277. doi: 10.1073/pnas.1510856112
Pelletier, E., Sargian, P., Payet, J., and Demers, S. (2006). Ecotoxicological effects of combined UVB and organic contaminants in coastal waters: a review. Photochem. Photobiol. 82, 981–993. doi: 10.1562/2005-09-18-RA-688
Peters, R. H. (1983). The Ecological Implications of Body Size. Cambridge: Cambridge University Press.
Rai, L., and Mallick, N. (1998). Algal responses to enhanced ultraviolet-B radiation. Proc. Indian Natl. Sci. Acad. B 64, 125–146.
Rautenberger, R., Wiencke, C., and Bischof, K. (2013). Acclimation to UV radiation and antioxidative defence in the endemic Antarctic brown macroalga Desmarestia anceps along a depth gradient. Polar Biol. 36, 1779–1789. doi: 10.1007/s00300-013-1397-2
Roleda, M., van de Poll, W. H., Hanelt, D., and Wiencke, C. (2004). PAR and UVBR effects on photosynthesis, viability, growth and DNA in different life stages of two coexisting Gigartinales: implications for recruitment and zonation pattern. Mar. Ecol. Prog. Ser. 281, 37–50. doi: 10.3354/meps281037
Roleda, M. Y., Campana, G., Wiencke, C., Hanelt, D., Quartino, M. L., and Wulff, A. (2009). Sensitivity of Antarctic Urospora penicilliformis (Ulotrichales, Chlorophyta) to ultraviolet radiation is life stage dependent. J. Phycol. 45, 600–609. doi: 10.1111/j.1529-8817.2009.00691.x
Roleda, M. Y., Wiencke, C., and Hanelt, D. (2006). Thallus morphology and optical characteristics affect growth and DNA damage by UV radiation in juvenile Arctic Laminaria sporophytes. Planta 223, 407–417. doi: 10.1007/s00425-005-0092-0
Roleda, M. Y., Wiencke, C., Hanelt, D., and Bischof, K. (2007). Sensitivity of the early life stages of macroalgae from the northern hemisphere to ultraviolet radiation. Photochem. Photobiol. 83, 851–862. doi: 10.1562/2006-08-17-IR-1005
Rozema, J., Björn, L. O., Bornman, J., Gaberščik, A., Häder, D.-P., Trošt, T., et al. (2002). The role of UV-B radiation in aquatic and terrestrial ecosystems-an experimental and functional analysis of the evolution of UV-absorbing compounds. J. Photochem. Photobiol. B 66, 2–12. doi: 10.1016/S1011-1344(01)00269-X
Sicora, C., Máté, Z., and Vass, I. (2003). The interaction of visible and UV-B light during photodamage and repair of Photosystem II. Photosynth. Res. 75, 127–137. doi: 10.1023/A:1022852631339
Sinha, R., Rastogi, R., Ambasht, N., and Häder, D. (2008). Life of wetland cyanobacteria under enhancing solar UV-B radiation. Proc. Indian Natl. Sci. Acad. B 78, 53–65.
Six, C., Finkel, Z. V., Irwin, A. J., and Campbell, D. A. (2007). Light variability illuminates niche-partitioning among marine picocyanobacteria. PLoS ONE 2:e1341. doi: 10.1371/journal.pone.0001341
Solomon, S., Ivy, D. J., Kinnison, D., Mills, M. J., Neely, R. R., and Schmidt, A. (2016). Emergence of healing in the Antarctic ozone layer. Science 353, 269–274. doi: 10.1126/science.aae00612016
Steinacher, M., Joos, F., Frolicher, T., Bopp, L., Cadule, P., Cocco, V., et al. (2010). Projected 21st century decrease in marine productivity: a multi-model analysis. Biogeosciences 7, 979–1005. doi: 10.5194/bg-7-979-2010
Steneck, R. S., and Dethier, M. N. (1994). A functional group approach to the structure of algal-dominated communities. Oikos. 69, 476–498.
Steneck, R. S., and Watling, L. (1982). Feeding capabilities and limitation of herbivorous molluscs: a functional group approach. Mar. Biol. 68, 299–319.
Swanson, A. K., and Druehl, L. D. (2000). Differential meiospore size and tolerance of ultraviolet light stress within and among kelp species along a depth gradient. Mar Biol. 136, 657–664. doi: 10.1007/s002270050725
Tedetti, M., Sempéré, R., Vasilkov, A., Charrière, B., Nérini, D., Miller, W. L., et al. (2007). High penetration of ultraviolet radiation in the south east Pacific waters. Geophys. Res. Lett. 34, L126101–L126105. doi: 10.1029/2007GL029823
Thomson, P. G., Davidson, A. T., and Cadman, N. (2008). Temporal changes in effects of ambient UV radiation on natural communities of Antarctic marine protists. Aquat. Microb. Ecol. 52, 131–147. doi: 10.3354/ame01201
UNEP, Environmental Effects Assessment Panel (2010). Environmental effects of ozone depletion and its interactions with climate change: progress report, 2009. Photochem. Photobiol. Sci. 9, 275–294.
van de Poll, W. H., Eggert, A., Buma, A. G. J., and Breeman, A. M. (2001). Effects of UV-B induced DNA damage and photoinhibition on growth of temperate marine red macrophytes: habitat related differences in UV-B tolerance. J. Phycol. 37, 30–37. doi: 10.1046/j.1529-8817.2001.037001030.x
Villafañe, V. E., Gao, K., Li, P., Li, G., and Hebling, E. W. (2007). Vertical mixing within the epilimnion modulates UVR-induced photoinhibition in tropical freshwater phytoplankton from southern China. Freshwat. Biol. 52, 1260–1270. doi: 10.1111/j.1365-2427.2007.01762.x
Villafañe, V. E., Sundbäck, K., Figueroa, F. L., and Hebling, E. W. (2003). “Photosynthesis in the aquatic environment as affected by UVR”, in UV Effects in Aquatic Organisms and Ecosystems, eds E. W. Helbling and H. Zagarese (Cambridge: Royal Society of Chemistry), 357–398.
Weatherhead, E. C., and Andersen, S. B. (2006). The search for signs of recovery of the ozone layer. Nature 441, 39–45. doi: 10.1038/nature04746
Wiencke, C., Roleda, M. Y., Gruber, A., Clayton, M. N., and Bischof, K. (2006). Susceptibility of zoospores to UV radiation determines upper depth distribution limit of Arctic kelps: evidence through field experiments. J. Ecol. 94, 455–463. doi: 10.1111/j.1365-2745.2006.01102.x
Wu, Y., Li, Z., Du, W., and Gao, K. (2015). Physiological response of marine centric diatoms to ultraviolet radiation, with special reference to cell size. J. Photochem. Photobiol. B 153, 1–6. doi: 10.1016/j.jphotobiol.2015.08.035
Xenopoulos, M. A., and Frost, P. C. (2003). UV radiation, phosphorus, and their combined effects on the taxonomic composition of phytoplankton in a boreal lake. J. Phycol. 39, 291–302. doi: 10.1046/j.1529-8817.2003.02125.x
Xiong, F., Lederer, F., Lukavský, J., and Nedbal, L. (1996). Screening of freshwater algae (Chlorophyta, Chromophyta) for ultraviolet-B sensitivity of the photosynthetic apparatus. J. Plant Physiol. 148, 42–48. doi: 10.1016/S0176-1617(96)80292-6
Xu, K., Gao, K., Villafañe, V. E., and Helbling, E. W. (2011). Photosynthetic responses of Emiliania huxleyi to UV radiation and elevated temperature: roles of calcified coccoliths. Biogeosciences 8, 1441–1452. doi: 10.5194/bg-8-1441-2011
Zhang, Y., Jiang, H. B., and Qiu, B. S. (2013). Effects of UVB radiation on competition between the bloom-forming cyanobacterium Microcystis aeruginosa and the Chlorophyceae Chlamydomonas microsphaera. J. Phycol. 49, 318–328. doi: 10.1111/jpy.12038
Zhu, L., Xiao, H., Wang, Y., Jian, X., Zhang, Z., Zhang, H., et al. (2015). Physiological responses of macroalga Gracilaria lemaneiformis (Rhodophyta) to UV-B radiation exposure. Chin. J. Oceanol. Limnol. 33, 389–399. doi: 10.1007/s00343-015-4073-2
Keywords: UVB, macroalgae, microalgae, photosynthesis, meta-analysis, cell size
Citation: Jin P, Duarte CM and Agustí S (2017) Contrasting Responses of Marine and Freshwater Photosynthetic Organisms to UVB Radiation: A Meta-Analysis. Front. Mar. Sci. 4:45. doi: 10.3389/fmars.2017.00045
Received: 11 September 2016; Accepted: 07 February 2017;
Published: 17 February 2017.
Edited by:
Christopher Edward Cornwall, University of Western Australia, AustraliaReviewed by:
Tiffany Ann Stephens, University of British Columbia, CanadaKen G. Ryan, Victoria University of Wellington, New Zealand
Copyright © 2017 Jin, Duarte and Agustí. This is an open-access article distributed under the terms of the Creative Commons Attribution License (CC BY). The use, distribution or reproduction in other forums is permitted, provided the original author(s) or licensor are credited and that the original publication in this journal is cited, in accordance with accepted academic practice. No use, distribution or reproduction is permitted which does not comply with these terms.
*Correspondence: Peng Jin, peng.jin@kaust.edu.sa