- 1Hellenic Centre for Marine Research, Institute of Oceanography, Athens, Greece
- 2Centro de Baleares, Instituto Español de Oceanografia, Palma de Mallorca, Spain
- 3Hellenic Centre for Marine Research, Institute of Oceanography, Heraklion, Greece
- 4Israel Oceanographic and Limnological Research, National Institute of Oceanography, Haifa, Israel
Atmospheric deposition is assumed to stimulate heterotrophic processes in highly oligotrophic marine systems, controlling the dynamics and trophic efficiency of planktonic food webs, and is expected to be influenced by climate change. In the course of an 8-day mesocosm experiment, we examined the channeling, of the Saharan dust (SD) and mixed aerosols (A) effects on microplankton up to the copepod trophic level, in the highly oligotrophic Eastern Mediterranean Sea. Based on mesocosms with SD and A treatments, we evaluated the feeding response of the dominant copepod Clausocalanus furcatus every other day. We hypothesized that increased food availability under atmospheric deposition would result in increased copepod ingestion rates, selectivity and production. Overall, no robust pattern of food selection was documented, and daily rations on the prey assemblage of all mesocosms were very low indicating severe food limitation of C. furcatus. Although increased food availability was not true, after few days ingestion of ciliates was maximized, followed by egg production, in both the SD and A treatments, indicating their importance in the diet of this copepod as well as a response of C. furcatus feeding performance. Our results help in understanding the trophic efficiency of marine food webs in ultra-oligotrophic environments under atmospheric deposition. We suggest that future mesocosm research in oligotrophic waters should consider more than one copepod species.
Introduction
Inorganic nutrients are among the key abiotic factors regulating primary producers and, ultimately, the trophic status of marine ecosystems. Dust deposition is recognized as a significant source of macro- and micro-nutrients to the surface ocean (Jickells et al., 2005; Mahowald et al., 2008) and is particularly important in areas with little input from other external sources (e.g., Jickells et al., 2005; Duce et al., 2008), as in the Eastern Mediterranean Sea (Herut et al., 2002; Krom et al., 2004). Recent efforts to comprehend the action of these contributions on ocean biogeochemistry, has dedicated on the influence on primary productivity, assumed their aptitude to generate new production (e.g., Ridame et al., 2014). However, recent studies, merging field and experimental work, indicated significant boost in heterotrophic bacterial abundance and respiration following up atmospheric deposition in oligotrophic systems (Lekunberri et al., 2010; Romero et al., 2011; Pulido-Villena et al., 2014). Additionally, heterotrophic processes were found to be further activated by dust pulses when compared to autotrophic processes with increasing degree of oligotrophy, the dominant response being controlled by the nutrients competition between bacteria and phytoplankton (Maranon et al., 2010).
Normally the dominance of copepods is a well-established pattern in extremely oligotrophic systems (Nuwer et al., 2008; Villar-Argaiz et al., 2012). Even if many factors have been identified to qualitatively affect zooplankton succession (temperature, predation rates, etc.), food availability has been identified as a key regulatory aspect of the zooplankton growth (Sterner and Elser, 2002). Marine copepods may reveal a considerable selective feeding response, selecting the most appropriate prey based on size (Mullin, 1963; Frost, 1972), motility (Atkinson, 1995; Broglio et al., 2001), and nutritional value (Cowles et al., 1988; Isari et al., 2013). Copepods usually choose microzooplankton prey over smaller phytoplankton (Calbet and Saiz, 2005; Saiz and Calbet, 2011), therefore microzooplankton may be an essential linkage between phytoplankton and metazoans (Stoecker and Capuzzo, 1990; Schmoker et al., 2013). Finally, in an increase of total food availability, feeding rates of copepods are expected to be increased and prey selection to be more intense (DeMott, 1989, 1995), ultimately leading to a positive influence of copepod production.
The present paper was aimed at elucidating how the impact of Saharan dust and mixed aerosols (polluted and desert origin) on microplankton may be channeled to marine copepods. The Mediterranean Sea might receive high amounts of atmospheric particles, of both natural (Saharan) and anthropogenic origin, over broad areas (e.g., Guerzoni et al., 1999; Pulido-Villena et al., 2014). These atmospheric depositions possibly represent the major input of external nutrients entering offshore surface waters (Herut et al., 2002; Bartoli et al., 2005; Guieu et al., 2010). A mesocosm experiment was designed to test the influence of atmospheric deposition on the plankton community of the Eastern Mediterranean. This extremely oligotrophic ecosystem, described as phosphorus limitated (Krom et al., 1991), or nitrogen and phosphorous co-limitated (Tanaka et al., 2011; Ternon et al., 2011), is ideal for testing the atmospheric deposition hypothesis. We hypothesized that increased food availability under atmospheric deposition would result in increased copepod ingestion rates, selectivity and production.
For the estimation of copepod vital rates three deposition treatments were used in parallel. Based on incubation experiments during the evolution of an initially triplicate plankton assemblage, we estimated the Clausocalanus furcatus response [based on feeding rates, prey preferences, daily ration (DR) and egg production], which dominated in the mesocosm plankton community. Although this copepod is known for its extensive distribution and biological importance, there is insufficient information on its feeding response under natural conditions (Paffenhofer et al., 2006; Cornils et al., 2007b). The present paper encompasses the first results on the Clausocalanus furcatus feeding ecology, triggered by atmospheric deposition events. This may help in explaining its response under specific nutrient regimes and in obtaining vision on the functioning of trophic webs in ultra-oligotrophic environments.
Methods
Mesocosm Set up and experimental Design
The influence of atmospheric deposition on copepods was investigated as a part of the ATMOMED mesocosm experiment carried out from 10 to 18 May 2012 at the CRETACOSMOS facility of the Hellenic Centre for Marine Research in Crete, Greece (www.cretacosmos.eu). The facility consists of a 350 m−3 land-based concrete pond, 5 m deep, supplied with continuous seawater flow-through in order to maintain ambient surface water temperature. The experiment was carried out using surface (~10 m depth) seawater that was collected using a rotary submersible pump placed on board the R/VPhilia from allocation 5 nautical miles north of Heraklion port in the Cretan Sea (35o 24.975N, 25o 14.441E). The collected seawater was equally distributed by gravity into nine food-grade polyethylene mesocosm bags to ensure the homogeneity of the collected seawater between bags. The mesocosms were mounted on aluminum frames (1.12 m diameter) attached to the pool's walls. Each mesocosm had a total volume of 3 m−3. The mesocosms were gently mixed throughout the experiment, using an airlift pump to avoid stratification. They were covered with a two-layer lid in order to protect them from natural atmospheric aerosol depositions during the experiment and mimic the light conditions at a 10 m water depth.
The mesocosm set up involved two distinct deposition treatments [Sahara Dust (SD) and mixed aerosols (A)] and a control (C) all triplicated in the bags. A more detailed description of the mesocosm set up and experimental design is provided by Tsagaraki et al. (this issue) and Herut et al. (2016). For the copepod feeding and production experiments, we used all three treatments (C, SD, and A). The water was sampled by all three replicates of each treatment, which was then mixed to produce one water mass for each treatment.
Zooplankton Sampling/analysis
Zooplankton abundance (individuals m−3) and copepod community composition were determined at the start (24 h before Day 0) and at the end (Day 18) of the mesocosm experiment. The initial zooplankton sampling was performed, when the water for the mesocosms was collected, using a modified WP2 45 μm net. Samples were preserved in 4% buffered formalin and then inspected under a stereoscopic microscope. At the end of the experiment, the water of each replicate treatment was filtered over a 45 μm net and zooplankton samples were treated as described above. A total of 12 samples were analyzed: three from the field and nine from the nine bags at the end of the experiment.
Clausocalanus furcatus Experiments
Copepod feeding and production were assessed on four dates (11, 12, 15, 17, May 2012), in each of the three mesocosm treatments. Four experiments were conducted, Exp1 on 11/5, Exp2 on 12/5, Exp3 on 15/5, and Exp4 on 17/5.
For the feeding experiments, adult females of the dominant copepod species Clausocalanus furcatus were used. The copepods were collected from the same area as the original water for the mesocosms, and pre-conditioned for 24 h in water from the corresponding treatment and under the respective ambient conditions. Water for the incubations was taken from each replicate enclosure early in the morning and mixed (three to one) according to the respective treatment. Then it was gently inversely filtered through a 100 mm mesh to exclude any zooplankton, while minimizing effects on delicate organisms such as ciliates (Broglio et al., 2004). For each mesocosm treatment, nine bottles (1.3 L polycarbonate) were prepared amended with 1 μM NH4Cl, 0.07 μM Na2HPO4, and 0.5 μM Na2SiO3. This nutrient addition was used to ensure that nutrients were not limiting in any treatment (Calbet et al., 2012) and to avoid selectively increased phytoplankton growth in the copepod-amended bottles due to excretion. Adult copepods (ca. 10–12 females) were added to three of the nine bottles, whereas the other six served as initial (three) and control (three) bottles. The experimental bottles were incubated for ca. 24 h, hanged at 0.5 m depth from a floating wheel rotating at ca. 1 r.p.m and propelled by a submerged water pump. This approach assured that food conditions were homogeneous in all bottles (Calbet et al., 2012).
The content of the bottles was collected by reverse filtration over a 200 mm mesh, at the beginning for the initial ones and after 24 h for the rest ones. Then samples for chlorophyll a (Chl a) and microplankton analysis were taken. For the control and copepod bottles, the remaining content was carefully emptied over a 200 μm mesh, and the copepod number and condition was checked. Total mortality was almost 0. Aliquots of 500 mL were filtered onto 0.2, μm polycarbonate filters to estimate chlorophyll a concentration. We considered that microplankton included ciliates and dinoflagellates, assuming all dinoflagellates as potentially heterotrophic, as in Loder et al. (2011). Microplankton samples were preserved in 2% acidic Lugol, settled in sedimentation chambers (100 mL) and counted using an inverted microscope at 200–400 magnification. For each microplankton category considered, cell size was measured and then converted into biovolume using simple geometric formulae (Hillebrand et al., 1999). Biovolume conversion to carbon was done according to the equations given in Menden-Deuer and Lessard (Menden-Deuer and Lessard, 2000) for dinoflagellates. Separate conversion factors were applied to aloricate (Putt and Stoecker, 1989) and loricate (Verity and Langdon, 1984) ciliates. Chl a concentrations were converted to carbon biomass using a conversion factor of 50 (C:Chl a = 50).
For the estimation of the egg production 25–30 healthy-looking, actively swimming females without an egg-sac, were sorted using a stereomicroscope. Three to four females were placed in each of six 620 ml glass jars (replicates) containing well-mixed 60 μm filtered water collected from each treatment (for details see below). The females were incubated at the ambient sea temperature (20°C) and photoperiod for 24 h after which the spawned eggs were counted and female lengths and egg diameters were measured. Overall mortality was 0.
Feeding rates were determined according to the equations of Frost (Frost, 1972). The carbon content of C. furcatus was estimated from length measurements applying the length-body carbon regression equation for Paracalanus spp. by Uye (1991). Then daily rations (DRs) in % body carbon ingested per day were calculated. Copepod feeding preference of ciliates and dinoflagellates size fractions (small vs. large size cells) was assessed by the Chesson's selectivity index a (Chesson, 1983):
where ri is the frequency of the food type i in the diet, ni is the frequency of the food type i in the available food and m is the number of food types. This index fluctuates between 0 and 1 and neutral selection corresponds to ai = 1/n. Therefore, non-selective feeding is indicated by ai = 0.25 in the case of a four-option choice (i.e., the taxonomic group, size). Higher values than these indicate positive preference for food type i, whereas lower values indicate avoidance for food type i.
Results
Copepod Community
Copepods were the numerically dominant group both in the field at the onset of the experiment (87.6%) and in the three mesocosm treatments after the end of the experiment (C, SD, and A: 87.4–92.6), followed by pteropods (7–10.7%) and other zoolankton (0.2–4.1%). The composition of the copepod community is included in Table 1. Female Clausocalanus furcatus with the genus copepodites clearly dominated (field: 54%; C: 30%; SD: 35%, A: 32%), while copepodites of the cyclopoid Oithona spp., the copepod nauplii, and the harpacticoid Microsetella spp. followed in numbers. The copepod number in C at the end of the experiment (113 ind. m−3) was higher than those of SD and A (96 and 98 ind. m−3). The abundance of the genus Clausocalanus was almost triple in the field, when compared to the mesocosms (Table 1).
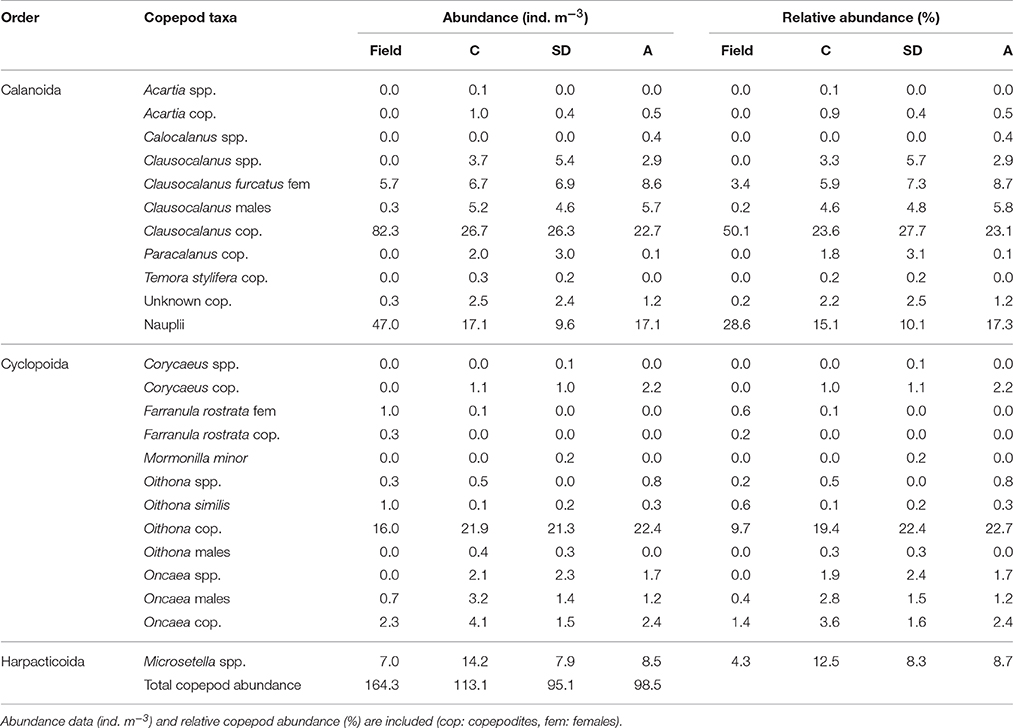
Table 1. Copepods identified at the beginning of the experiment (in the field) and at the end of the experiment (in the mesocosm treatments C, SD, and A).
Clausocalanus furcatus Egg Production
Clausocalanus furcatus production (specific egg production, SEP) was extremely low, ranging from 0 to 0.67% body C day−1 in the C treatment, from 0 to 6.32% body C day−1 in the SD treatment and from 0 to 7.53% body C day−1 in the A treatment. The two maxima 6.32 and 7.53% body C day−1, corresponding to 5.6 and 6.7 eggs ind−1 day−1 respectively, were recorded in Exp3.
Potential Prey of C. furcatus
The evolution of the Chl a values in the 3 mesocosms during the experiment is presented in Figure 1A. The SD and A treatments revealed a gradual decrease from the first to the last day. In general Chl a showed very low values in all treatments due to the oligotrophy of the area. The Chl a concentrations in SD and A were, on average across all samplings, higher than in C mesocosm. Dinoflagellates (DF) showed a gradual decrease from Exp1 to Exp3 in both SD and A treatments. Ciliates (CIL) exhibited an increase from Exp1 to Exp3 in C and A treatments, and an overall increase after Exp1 in SD mesocosm (Figure 1A). Two-way ANOVA tests showed no significant differences among the treatments comparing with the initial prey concentrations
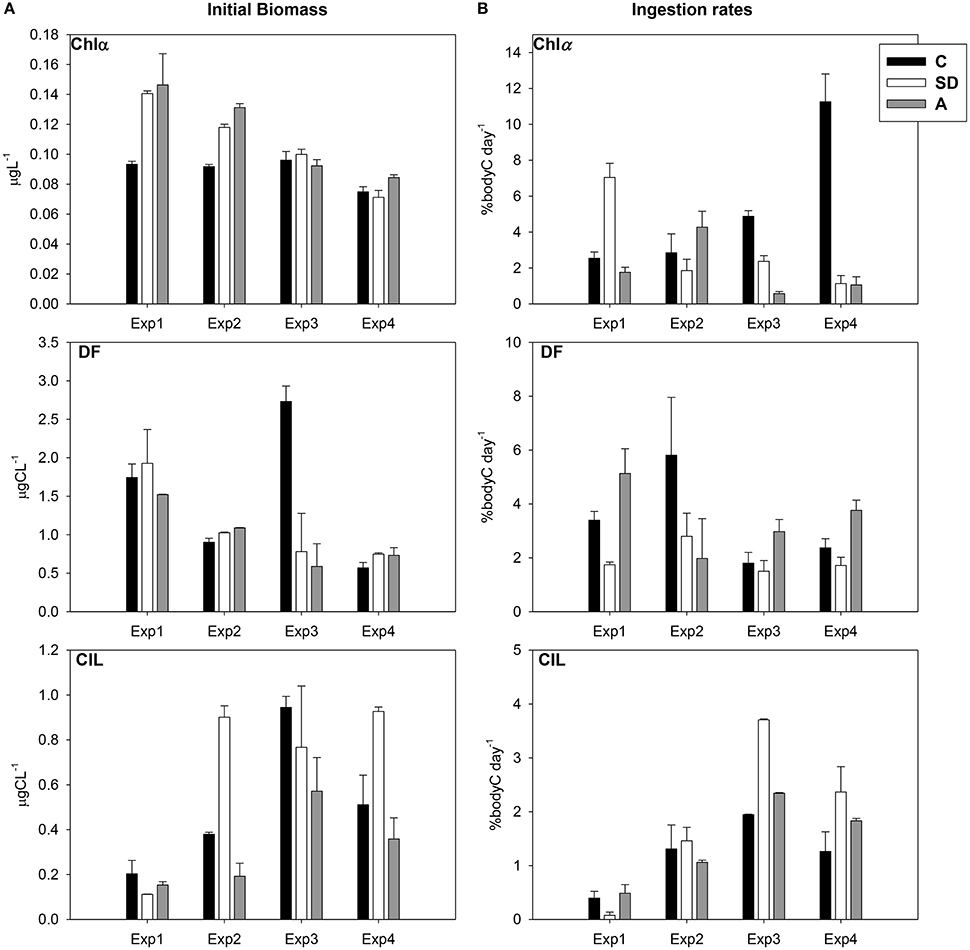
Figure 1. (A) Temporal variation of chlorophyll a (Chl a, μg l−1), dinoflagellates (DF, μg C l−1), and ciliates (CIL, μg C l−1) in the mesocosm treatments for the days of the feeding experiments (values represent initial samples of the feeding experiments) (B) Temporal variation of ingestion rates (% body C d−1) on Chl a, DF and CIL.
Total available carbon exhibited a gradual decrease from Exp1 to Exp4 in both SD and A treatments (Figure 2A). However, this decrease in control was interrupted by a maximum in Exp3. DF was the major food component contributing from 47 to 95% (Figure 2A). The carbon development pattern was comparable in SD and A treatments, with decreasing DF and increasing CIL. In almost all cases, but one, DF had the higher contribution to the total available carbon. However, DF/CIL equaled in Exp3 in the SD and A treatments, comprising 50.5/49.5 and 50.7/49.3 respectively.
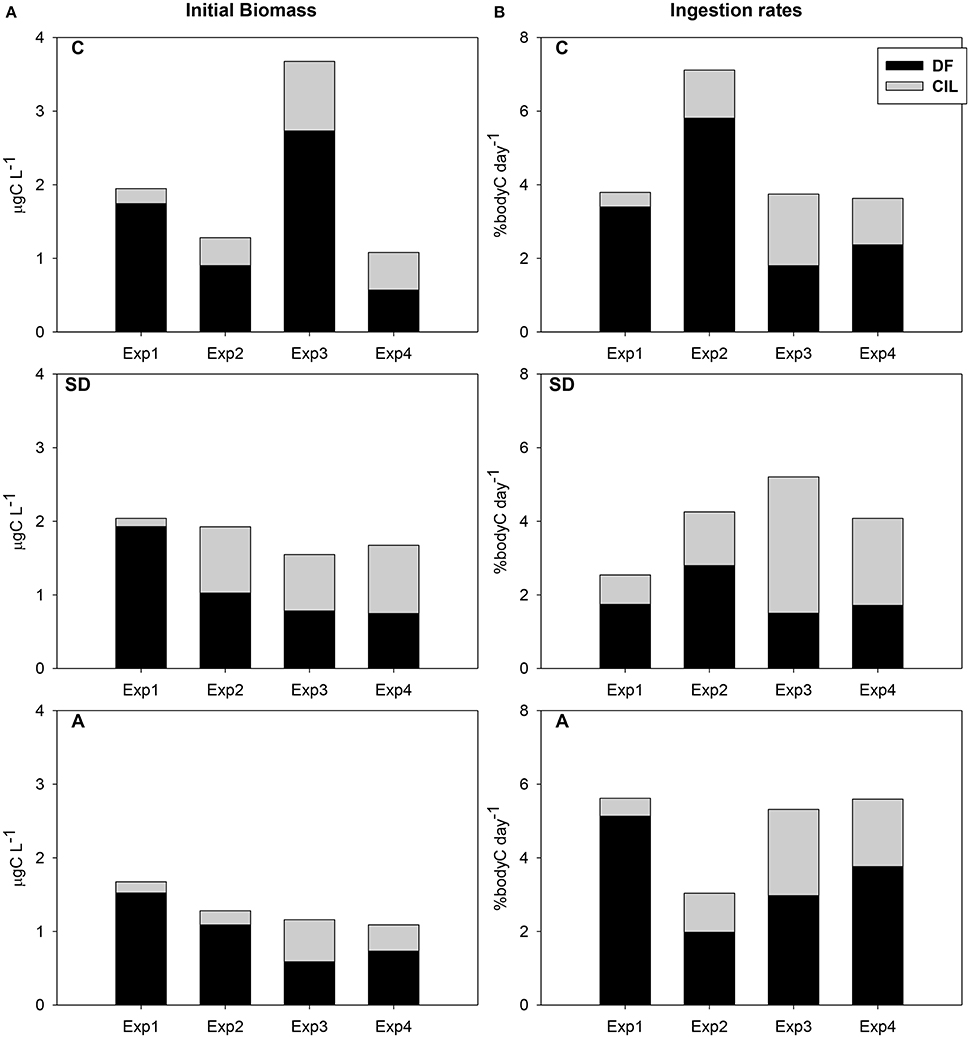
Figure 2. (A) Temporal variation of the contribution to the available heterotroph carbon (DF and CIL) in the mesocosm treatments during the experiments (values represent initial samples of the feeding experiments) (B) Contribution of DF and CIL to the DR of C. furcatus in the mesocosm treatments.
Clausocalanus furcatus Feeding Response
Vital feeding was found in all cases on DF and CIL, whereas clearance rates on Chl a were lower (Figure 3). Clearance rates on both DF and CIL were more or less similar (DF: 22–97 ml cop−1 d−1; CIL: 14–152 ml cop−1 d−1). Clearance rates on CIL attained some higher values, with the highest ones to be detected in Exp3 in the A and SD treatments. In contrast, in SD mesocosms DF were cleared at significantly lower rate than the other two treatments (p < 0.05). Finally, Chl a was cleared at much lower rates (2–54 ml cop−1 d−1).
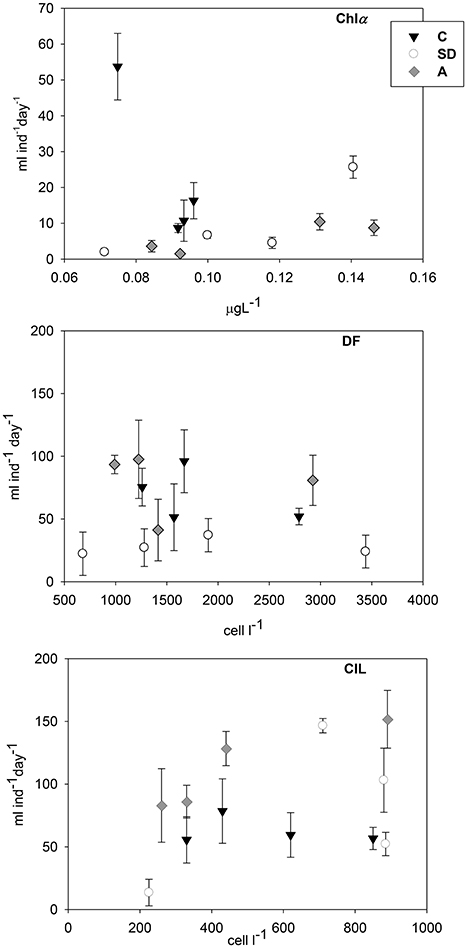
Figure 3. Copepod clearance rates (ml cop−1 d−1) on chlorophyll a (Chl a), dinoflagellates (DF), and ciliates (CIL) in the three mesocosm treatments. Error bars indicate the standard error.
An increasing trend was evident concerning Chl a ingestion rates in the C incubations, opposing to the decreasing trend in the SD ones (Figure 1B). DF ingestion rates did not exhibit any distinct pattern and the highest values were observed in the C and A treatments in Exp2 and Exp1, respectively. However, CIL ingestion rates showed an increasing trend from Exp1 to Exp3 in all treatments, with the highest values for both DF and CIL to be detected in Exp3 (Figure 1B). No statistically significant differences were found.
Taking into account the total consumed carbon (Figure 2B), no clear pattern was identified among treatments, but an increase from Exp1 to Exp3 in the SD treatment and from Exp2 to Exp4 in the A treatment. Average consumed carbon was 4.6, 3.8, and 4.9 % body C d−1 in the C, SD, and A mesocosms respectively, ranging in total from 1.2 to 7.1% body C d−1. No positive relation was observed between DR and prey availability. The highest ingestion rate in the C mesocosm was recorded in Exp2, Concerning SD and A treatments, total ingestion rates were very low (<6% body C d−1). However, regarding CIL contribution to the total DR, an increase was evident from Exp1 to Exp3 in both the SD and A treatments (Figure 2B). No statistically significant differences were found.
The results of Chesson's selectivity index (Chesson, 1983) are presented in Figure 4. In the C treatment, C. furcatus exhibited the highest selectivity for DF, mostly for those <20 μm. Under SD and A conditions, selectivity patterns confirmed the DF preference. However, in the SD treatment, the overall preference was stronger for the >20 μm DF, opposing to the <20 μm DF selectivity which was stronger in the A treatment. The highest selectivity, for <20 μm DF, was observed on the third day.
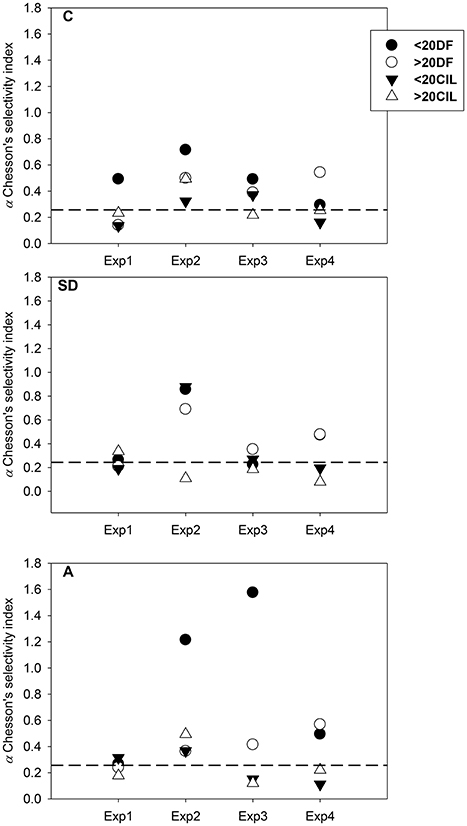
Figure 4. Chesson's selectivity index values calculated for (circles) dinoflagellates >20 μm over <20 μm, (triangles) ciliates >20 μm over <20 μm. The dashed horizontal line represents a level of a, where no selectivity is detected (a: 0.25). SD, Saharan dust; A, mixed aerosols; C, control.
Discussion
Clausocalanus furcatus, a small clausocalanid, is one of the dominant calanoid copepods in warm waters globally (e.g., Mazzocchi and Ribera d'Alcalà, 1995; Cornils et al., 2007a; Miyashita et al., 2009; Schnack-Schiel et al., 2010; Siokou-Frangou et al., 2010). This copepod occurs in the epipelagic zone all over the world (Frost and Fleminger, 1968), also in the Mediterranean region (e.g., Siokou-Frangou et al., 2010), and usually dominates zooplankton communities in oligotrophic environments (Schulz, 1986; Mazzocchi and Ribera d'Alcalà, 1995; Webber and Roff, 1995; Cornils et al., 2007a). In a recent paper, investigating the niche separation of Clausocalanus species in the Atlantic Ocean, it was found that C. furcatus had a narrow thermal and salinity niche and its optimal conditions were warm, saline and very oligotrophic waters (Peralba et al., in press).
The maximum egg production of C. furcatus found in our work was almost half to that reported for the same species in the Gulf of Mexico (Bi and Benfield, 2006) and fall in the range reported for C. furcatus in the Gulf of Aqaba (Cornils et al., 2007a). In the Atlantic Ocean, the shortest interclutch period of C. furcatus was recorded in mesotrophic sites (around the NW African upwelling), where the potential food availability would be favorable for egg production (Peralba et al., in press). On the other hand, it has been observed that C. furcatus production rate in laboratory conditions, was higher at low food concentrations (Mazzocchi and Paffenhofer, 1998). Peralba et al. (in press), commenting on this issue, explained that the high diversity of a natural diet may be more profitable for the metabolic needs and would enhance higher egg production rates.
In the present work C. furcatus appeared to produce eggs at low available food, although in most cases could not. It has been reported that clausocalanoids are able to produce eggs at low food availability (Mazzocchi and Paffenhofer, 1998, 1999; Bi and Benfield, 2006; Cornils et al., 2007a). Indeed, Mazzocchi and Paffenhofer (1998) showed that in the lab, C. furcatus egg production was higher when food (dinoflagellates and diatoms) concentrations were low than when provided with high food concentrations, hence proposing an adaptive strategy of this copepod to oligotrophic environments. In general egg production of clausocalanids, especially those producing egg sacs, appears to be low when compared to other calanoids (Cornils et al., 2007a; review in Mauchline, 1998).
Comparisons with data available on C. furcatus feeding rates revealed that the ingestion rates of the present work stand within the lower part of the range reported for experiments with natural prey assemblage or with cultured monospecific diets. The low ciliate and dinoflagellate ingestion rates in our study are comparable to the laboratory findings of Mazzocchi and Paffenhofer (1998) with dinoflagellates as food. However, the latter rates enhanced with increasing cell concentrations (Cornils et al., 2007b). Mazzocchi and Paffenhofer (1999) found low ratios in spite of their observations of increased swimming movement, suggesting that nonstop movement might be energetically superior to discontinuing movement. In the NW Mediterranean ingestion rates for Clausocalanus spp. (10–40% body C d−1, Broglio et al., 2004) were 3 to 4 times higher than those of the present study. In general, under natural conditions, the ingestion rates can fluctuate within a broad range, as it has been reported for other medium to small sized copepods (e.g., Centropages typicus, 4–70%; Dagg and Grill, 1980).
In our work, clearance rates of C. furcatus on the different food types fall in the range reported for this copepod in other oligotrophic areas (Paffenhofer et al., 2006; Isari et al., 2014). Daily rations (DRs) on the available food of all treatments were low, either taking into account only ciliate or dinoflagellate food (maxima of 3.7% in SD and 5.8% in A, respectively) or as a total (maximum of 7.1% in C). These values are lower than DRs (10–34%) based on feeding rates of this copepod on monospecific dinoflagellate diets (Mazzocchi and Paffenhofer, 1998), and are either much lower or slightly higher than results for other clausocalanids fed on natural diets in subtropical oligotrophic waters (Paffenhofer et al., 2006: 38%; Cornils et al., 2007b: 2%). DRs of this study are, also, a bit lower than those of C. furcatus from an earlier mesocosm experiment, at the same area but not same season (Isari et al., 2014). Evaluating the adequacy of the DRs taking into account the daily metabolic requirements, we found that the daily basal metabolic demands at 20°C would account for 23.4% of its body weight, which would need a DR of 26% using an assimilation efficiency of 90% (Paffenhofer, 2006). This value is more than duplicated the DRs achieved in our feeding experiments, indicating serious food limitation of C. furcatus in the mesocosm experiments. It has been suggested that the basal metabolic demands of C. furcatus may be satisfied even under the mostly limited food availability in the subtropical open seas (Paffenhofer et al., 2006). Nevertheless, the strategy developed by this copepod to deal with the scarcity of food in the marine environment is not clear (Paffenhofer et al., 2006; Cornils et al., 2007b), and it is worth questioning in what way an ingestion hardly sufficient to meet the energy requirements in our experiment would sustain the egg production of this copepod in the mesocosms. Information about nanoplankton availability as potential food is lacking from our work. Nanoplankton has been found to be important in the diet of C. furcatus from an earlier mesocosm experiment, at the same area (Isari et al., 2014). Copepods, depending on the species and the feeding strategy, may fulfill their metabolic requirements by foraging on other non-living material as well (e.g., detrital material; Roman, 1984) or even utilizing prey patchiness (Tiselius, 1992; Saiz et al., 1993). However, it is impossible that such procedures may be triggered inside mesocosms.
Food availability has been considered as a major importance issue in determining copepod feeding strategy in the marine environment (Saiz and Calbet, 2007, 2011). Overall, in the present work, no robust pattern of food selection was observed, in agreement with the prediction of the optimal foraging theory, demonstrating that copepods may feed selectively when food is plentiful and non-selectively when food is limited (DeMott, 1989, 1995). In the oligotrophic Gulf of Aqaba, C. furcatus ingested preferably ciliates and dinoflagellates, whereas no preference was detected for diatoms and flagellates (Cornils et al., 2007b); nevertheless, selectivity indices, calculated according to Chesson (1983), revealed no preference of any food type. Therefore, it was assumed that the C. furcatus diet in the Gulf of Aqaba was diverse and depended mainly on food abundance rather than food type (Cornils et al., 2007b).
Selective predation on CIL is well documented in copepod feeding behavior (Calbet and Saiz, 2005; Saiz and Calbet, 2011), related with their high nutritional quality (Stoecker and Capuzzo, 1990), their optimal size range, and their motility pattern (Tiselius and Jonsson, 1990; Atkinson, 1995; Saiz and Kiørboe, 1995; Kiørboe et al., 1996). Preference for CIL has been pointed out for Clausocalanus spp. in NW Mediterranean (Batten et al., 2001; Broglio et al., 2004) and a selection for both CIL and DF, was indicated for C. furcatus in the Gulf of Aqaba (Cornils et al., 2007b). In addition to CIL, DF also comprise a substantial amount of the carbon consumed by copepods in oligotrophic seas and in many cases are positively selected, even though to a lower degree when compared to CIL (Saiz and Calbet, 2011). In this work, according to Chesson (1983), C. furcatus revealed preference for DF <20 μm and in a lesser degree for CIL. However, CIL ingestion was maximized in Exp3, in both the SD and A treatments, and concurred with the highest copepod spawning, indicating that CIL consumption might enhance copepod production. This can be likely explained not only by the conventional size-dependent selective feeding strategy but also by a selectivity based on nutritional criteria. It was found that C. furcatus retains an array of mechanical, chemical and dual-function sensors over its antenna (A1), by which the copepod can identify surroundings stimuli (Uttieri et al., 2008), thus improving its feeding success.
In general, the food types tested in all related studies involved only a small variation in the food concentration, which remained mostly in the ranges of oligotrophy (Isari and Saiz, 2011). Therefore, there is a lack of comprehensive information on the ability of C. furcatus for energy exploitation in environments without food limitation (Isari and Saiz, 2011), where they can also populate (e.g., Valdes et al., 2007).
Concluding on C. furcatus feeding response in the present study, based on (a) the lack of robust food selection patterns (b) the lack of statistically significant differences, (c) the DF <20 μm preference according to Chesson (1983), and (d) the maximum CIL ingestion along with the maximum egg production, in Exp3; it appears that C. furcatus “touches the threshold” between feeding selectively and feeding non-selectively, switching continuously, very efficiently, from one mode to the other. This flexibility can be the C. furcatus setup, for the optimum exploitation of all available food (including food patchiness events) in oligotrophic environments.
Conclusions
Our data showed that, although increased food availability was not true, the feeding performance of the dominant copepod C. furcatus did have a response to the atmospheric deposition, through the changes mediated in the microbial food web (Tsagaraki et al., this issue; Herut et al., 2016). This is of great importance for the coupling between lower and higher trophic levels, through a more efficient transfer of food toward top consumers. However, the impact of atmospheric deposition, on the allocation of matter and energy through the planktonic food wed, is critically dependent on the copepod community composition; even if Clausocalanus furcatus is the dominant copepod, definitely does not represent the complete copepod diversity concerning trophic pathways. Different copepods and copepod stages display variable behaviorally driven feeding activities and preferences (Castellani et al., 2008), and daily feeding behaviors (Calbet et al., 1999). Future mesocosm research of the impact of atmospheric depositions on marine system performance should consider, if not the whole copepod community, more copepod species, especially regarding ultra-oligotrophic waters, and when necessary, deploy technologically highly developed experimental set-ups (Riebesell et al., 2013), closely imitating the actual characteristics of natural environment.
Author Contributions
EC participation in experimental work, work planning, writing the ms. SZ participation in the field and experimental work, work planning, sample analysis, data processing. MF participation in the field and experimental work. MP sample analysis. IV sample analysis. PP work planning. TT participation in experimental work. BH work planning.
Funding
Hellenic Centre for Marine Research.
Conflict of Interest Statement
The authors declare that the research was conducted in the absence of any commercial or financial relationships that could be construed as a potential conflict of interest.
Acknowledgments
This work was financed by the EU-FP7 project (grant agreement no. 228224) “MESOAQUA: Network of leading MESOcosm facilities to advance the studies of future AQUAtic ecosystems from the Arctic to the Mediterranean” and by the project ADAMANT: Atmospheric deposition and Mediterranean sea water productivity (nr code/MIS: 383551), co-financed by the European Union (European Social Fund –ESF) and Greek national funds through the Operational Program “Education and Lifelong Learning” of the National Strategic Reference Framework (NSRF) (Research Funding Program: THALES).
References
Atkinson, A. (1995). Onmivory and feeding selectivity in five copepod species during spring in the Bellingshausen Sea, Antarctica. ICES J. Mar. Sci. 52, 385–396. doi: 10.1016/1054-3139(95)80054-9
Bartoli, G., Migon, C., and Losno, R. (2005). Atmospheric input of dissolved inorganic phosphorus and silicon to the coastal northwestern Mediterranean Sea: fluxes, variability and possible impact on phytoplankton dynamics. Deep Sea Res. I 52, 2005–2016. doi: 10.1016/j.dsr.2005.06.006
Batten, S. D., Fileman, E. S., and Halvorsen, E. (2001). The contribution of microzooplankton to the diet of mesozooplankton in an upwelling filament off the north west coast of Spain. Prog. Oceanogr. 51, 385–398. doi: 10.1016/S0079-6611(01)00076-3
Bi, H., and Benfield, M. (2006). Egg production rates and stage-specific development times of Clausocalanus furcatus (Copepoda, Calanoida) in the northern Gulf of Mexico. J. Plankton Res. 28, 1199–1216. doi: 10.1093/plankt/fbl050
Broglio, E., Johansson, M., and Jonsson, P. R. (2001). Trophic interaction between copepods and ciliates: effects of prey swimming behavior on predation risk. Mar. Ecol. Prog. Ser. 220, 179–186. doi: 10.3354/meps220179
Broglio, E., Saiz, E., Calbet, A., Trepat, I., and Alcaraz, M. (2004). Trophic impact and prey selection by crustacean zooplankton on the microbial communities of an oligotrophic coastal area (NW Mediterranean Sea). Aquat. Microb. Ecol. 35, 65–78. doi: 10.3354/ame035065
Calbet, A., Martınez, R. A., Isari, S., Zervoudaki, S., Nejstgaardc, J. C., Pitta, P., et al. (2012). Effects of light availability on mixotrophy and microzooplankton grazing in an oligotrophic plankton food web: evidences from a mesocosm study in Eastern Mediterranean waters. J. Exp. Mar. Biol. Ecol. 424–425, 66–77. doi: 10.1016/j.jembe.2012.05.005
Calbet, A., and Saiz, E. (2005). The ciliate-copepod link in marine ecosystems. Aquat. Microb. Ecol., 38, 157–167. doi: 10.3354/ame038157
Calbet, A., Saiz, E., Irigoien, X., Alcaraz, M., and Trepat, I. (1999). Food availability and diel feeding rhythms in the marine copepods Acartia grani and Centropages typicus. J. Plankton Res., 21, 1009–1015. doi: 10.1093/plankt/21.5.1009
Castellani, C., Irigoien, X., Mayor, D. J., Harris, R. P. and Wilson, D. (2008). Feeding of Calanus finmarchicus and Oithona similis on the microplankton assemblage in the Irminger Sea, North Atlantic. J. Plankton Res. 30, 1095–1116. doi: 10.1093/plankt/fbn074
Chesson, J. (1983). The estimation and analysis of preference and its relationhip to foraging models. Ecology, 64, 1297–1304. doi: 10.2307/1937838
Cornils, A., Niehoff, B., Richter, C., Al-Najjar, T., and Schnack-Schiel, S. B. (2007a). Seasonal abundance and reproduction of clausocalanid copepods in the northern Gulf of Aqaba (Red Sea). J. Plankton Res. 29, 57–70. doi: 10.1093/plankt/fbl057
Cornils, A., Schnack-Schiel, S. B., Boer, M., Graeve, M., Struck, U., Al-Najjar, T., et al. (2007b). Feeding of Clausocalanids (Calanoida, Copepoda) on naturally occurring particles in the northern Gulf of Aqaba (Red Sea). Mar. Biol., 151, 1261–1274. doi: 10.1007/s00227-006-0569-9
Cowles, T. J., Olson, R. J., and Chisholm, S. W. (1988). Food selection by copepods: discrimination on the basis of food quality. Mar. Biol. 100, 41–49. doi: 10.1007/BF00392953
Dagg, M. J., and Grill, D. W. (1980). Natural feeding rates of Centropages typicus females in the New York Bight. Limnol. Oceanogr. 25, 597–609. doi: 10.4319/lo.1980.25.4.0597
DeMott, W. R. (1989). Optimal foraging theory as a predictor of chemically mediated food selection by suspension-feeding copepods. Limnol. Oceanogr. 34, 140–154. doi: 10.4319/lo.1989.34.1.0140
DeMott, W. R. (1995). Optimal foraging by a suspension-feeding copepod: responses to short-term and seasonal variation in food resources. Oecologia 103, 230–240. doi: 10.1007/BF00329085
Duce, R. A., LaRoche, J., Altieri, K., Arrigo, K. R., Baker, A. R., Capone, D. G., et al. (2008). Impacts of atmospheric anthropogenic nitrogen on the open ocean. Science 320, 893–897. doi: 10.1126/science.1150369
Frost, B., and Fleminger, A. (1968). A revision of the genus Clausocalanus (Copepoda: Calanoida) with remarks on distributional patterns in diagnostic characters. Bull. Scripps Inst. Oceanogr. 12, 1–235.
Frost, B. W. (1972). Effects of size and concentration of food particles on the feeding behavior of the marine planktonic copepod Calanus pacificus. Limnol. Oceanogr. 17, 805–815. doi: 10.4319/lo.1972.17.6.0805
Guerzoni, S., Chester, R., Dulac, F., Herut, B., Loye-Pilot, M. D., Measures, C., et al. (1999). The role of atmospheric deposition in the biogeochemistry of the Mediterranean Sea. Prog. Oceanogr. 44, 147–190. doi: 10.1016/S0079-6611(99)00024-5
Guieu, C., Loye-Pilot, M. D., Benyaya, L., and Dufour, A. (2010). Spatial variability of atmospheric fluxes of metals (Al, Fe, Cd, Zn and Pb) and phosphorus over the whole Mediterranean from a one year monitoring experiment: biogeochemical implications. Mar. Chem. 120, 164–178. doi: 10.1016/j.marchem.2009.02.004
Herut, B., Collier, R., and Krom, M. D. (2002). The role of dust in supplying nitrogen and phosphorus to the Southeast Mediterranean. Limnol. Oceanogr. 47, 870–878. doi: 10.4319/lo.2002.47.3.0870
Herut, B., Rahav, E., Tsagaraki, T. M., Giannakourou, A., Tsiola, A., Psarra, S., et al. (2016). The impact of Saharan dust and polluted aerosols on biogeochemical processes in the East Mediterranean Sea, an overview of a mesocosm experimental approach. Front. Mar.Sci. 3:226. doi: 10.3389/fmars.2016.00226
Hillebrand, H., Durselen, C. D., Kirschtel, D., Pollingher, U., and Zohary, T. (1999). Biovolume calculation for pelagic and benthic microalgae. J. Phycol. 35, 403–424. doi: 10.1046/j.1529-8817.1999.3520403.x
Isari, S., Anto, M., and Saiz, E. (2013). Copepod foraging on the basis of food nutritional quality: can copepods really choose? PLoS ONE 8:e84742. doi: 10.1371/journal.pone.0084742
Isari, S., and Saiz, E. (2011). Feeding performance of the copepod Clausocalanus lividus (Frost and Fleminger, 1968). J. Plankton Res. 33, 715–728. doi: 10.1093/plankt/fbq149
Isari, S., Zervoudaki, S., Calbet, A., Saiz, E., Ptacnikova, R., Nejstgaard, J. C., et al. (2014). Light-induced changes on the feeding behaviour of the calanoid copepod Clausocalanus furcatus (Brady, 1883): evidence from a mesocosm study. J. Plankton Res. 36, 1233–1246. doi: 10.1093/plankt/fbu054
Jickells, T. D., An, Z. S., Andersen, K. K., Baker, A. R., Bergametti, G., Brooks, N., et al. (2005). Global iron connections between desert dust, ocean biogeochemistry, and climate. Science 308, 67–71. doi: 10.1126/science.1105959
Kiørboe, T., Saiz, E., and Viitasalo, M. (1996). Prey switching behaviour in the planktonic copepod Acartia tonsa. Mar. Ecol. Prog. Ser. 143, 65–75. doi: 10.3354/meps143065
Krom, M. D., Herut, B., and Mantoura, R. F. C. (2004). Nutrient budget for the Eastern Mediterranean: implications for phosphorus limitation. Limnol. Oceanogr. 49, 1582–1592. doi: 10.4319/lo.2004.49.5.1582
Krom, M. D., Kress, N., Brenner, S., and Gordon, L. I. (1991). Phosphorus limitation of primary productivity in the eastern Mediterranean Sea. Limnol. Oceanogr. 36, 424–432. doi: 10.4319/lo.1991.36.3.0424
Lekunberri, I., Lefort, T., Romero, E., Vazquez-Dominguez, E., Romera-Castillo, C., Marrase, C., et al. (2010). Effects of a dust deposition event on coastal marine microbial abundance and activity, bacterial community structure and ecosystem function. J. Plank. Res. 32, 381–396. doi: 10.1093/plankt/fbp137
Loder, M. G. J., Meunier, C., Wiltshire, K. H., Boersma, M., and Aberle, N. (2011).The role of ciliates, heterotrophic dinoflagellates and copepods in structuring spring plankton communities at Helgoland Roads, North Sea. Mar. Biol. 158, 1551–1580. doi: 10.1007/s00227-011-1670-2
Mahowald, N., Jickells, T. D., Baker, A. R., Artaxo, P., Benitez- Nelson, C. R., et al. (2008). Global distribution of atmospheric phosphorus sources, concentrations and deposition rates, and anthropogenic impacts. Global Biogeochem. Cy. 22, GB4026. doi: 10.1029/2008GB003240
Maranon, E., Fernandez, A., Mourino-Carballido, B., Martinez-Garcia, S., Teira, E., Cermeno, P., et al. (2010). Degree of oligotrophy controls the response of microbial plankton to Saharan dust. Limnol. Oceanogr. 55, 2339–2352. doi: 10.4319/lo.2010.55.6.2339
Mazzocchi, M. G., and Paffenhofer, G. A. (1998). First observations on the biology of Clausocalanus furcatus (Copepoda, Calanoida). J. Plankton Res. 20, 331–342. doi: 10.1093/plankt/20.2.331
Mazzocchi, M. G., and Paffenhofer, G. A. (1999). Swimming and feeding behaviour of the planktonic copepod Clausocalanus furcatus. J. Plankton Res. 21, 1501–1518. doi: 10.1093/plankt/21.8.1501
Mazzocchi, M. G., and Ribera d'Alcalà, M. (1995). Recurrent patterns in zooplankton structure and succession in a variable coastal environment. ICES J. Mar. Sci. 52, 679–691. doi: 10.1016/1054-3139(95)80081-6
Menden-Deuer, S., and Lessard, E. J. (2000). Carbon to volume relationships for dinoflagellates, diatoms, and other protist plankton. Limnol. Oceanogr. 45, 569–579. doi: 10.4319/lo.2000.45.3.0569
Miyashita, L. K., de Melo, J. M., and Lopes, R.M. (2009). Estuarine and oceanic influences on copepod abundance and production of a subtropical coastal area. J. Plankton Res. 31, 815–826. doi: 10.1093/plankt/fbp039
Mullin, M. M. (1963). Some factors affecting the feeding of marine copepods of the genus Calanus. Limnol. Oceanogr. 8, 239–250. doi: 10.4319/lo.1963.8.2.0239
Nuwer, M. L., Frost, B. W., and Armbrust, E. V. (2008). Population structure of the planktonic copepod Calanus pacificus in the North Pacific Ocean. Mar. Biol. 156, 107–115. doi: 10.1007/s00227-008-1068-y
Paffenhofer, G. A. (2006). Oxygen consumption in relation to motion of marine planktonic copepods. Mar. Ecol. Prog. Ser. 317, 187–192. doi: 10.3354/meps317187
Paffenhofer, G. A., Mazzocchi, M. G., and Tzeng, M. W. (2006). Living on the edge: feeding of subtropical open ocean copepods. Mar. Ecol. 27, 99–108. doi: 10.1111/j.1439-0485.2006.00086.x
Peralba, À., Mazzocchi, M. G., and Harris, R. P. (in press). Niche separation reproduction of Clausocalanus species (Copepoda, Calanoida) in the Atlantic Ocean. Prog. Oceanogr. doi: 10.1016/j.pocean.2016.08.002
Pulido-Villena, E., Baudoux, A. C., Obernosterer, I., Landa, M., Caparros, J., Catala, P., et al. (2014). Microbial food web dynamics in response to a Saharan dust event: results from a mesocosm study in the oligotrophic Mediterranean Sea. Biogeosciences 11, 5607–5619. doi: 10.5194/bg-11-5607-2014
Putt, M., and Stoecker, D. K. (1989). An experimentally determined carbon: volume ratio for marine oligotrichous ciliates from estuarine and coastal waters. Limnol. Oceanogr. 34, 1097–1103 doi: 10.4319/lo.1989.34.6.1097
Ridame, C., Dekaezemacker, J., Guieu, C., Bonnet, S., L'Helguen, S., and Malien, F. (2014). Phytoplanktonic response to contrasted Saharan dust deposition events during mesocosm experiments in LNLC environment, Biogeosciences Discuss. 11, 753–796, doi: 10.5194/bgd-11-753-2014.
Riebesell, U., Czerny, J., Von Brockel, K., Boxhammer, T., Budenbender, J., Deckelnick, M., et al. (2013). Technical Note: a mobile sea-going mesocosm system—new opportunities for ocean change research. Biogeosciences 10, 1835–1847. doi: 10.5194/bg-10-1835-2013
Roman, M. R. (1984). Utilization of detritus by the copepod, Acartia tonsa. Limnol. Oceanogr. 29, 949–959. doi: 10.4319/lo.1984.29.5.0949
Romero, E., Peters, F., Marrasé, C., Guadayol, O., Gasol, J. M., and Weinbauer, M. (2011). Coastal Mediterranean plankton stimulation dynamics through a dust storm event: an experimental simulation. Estuar. Coast. Shelf Sci. 93, 27–39. doi: 10.1016/j.ecss.2011.03.019
Saiz, E., and Calbet, A. (2007). Scaling of feeding in marine calanoid copepods. Limnol. Oceanogr. 52, 668–675. doi: 10.4319/lo.2007.52.2.0668
Saiz, E., and Calbet, A. (2011). Copepod feeding in the ocean: scaling patterns, composition of their diet and the bias of estimates due to microzooplankton grazing during incubations. Hydrobiologia 666, 181–196. doi: 10.1007/s10750-010-0421-6
Saiz, E., and Kiørboe, T. (1995). Predatory and suspension feeding of the copepod Acartia tonsa in turbulent environments. Mar. Ecol. Prog. Ser. 122, 147–158. doi: 10.3354/meps122147
Saiz, E., Tiselius, P., Jonsson, P. R., Verity, P., and Paffenhofer, G. A. (1993). Experimental records of the effects of food patchiness and predation on egg production of Acartia tonsa. Limnol. Oceanogr. 38, 280–289. doi: 10.4319/lo.1993.38.2.0280
Schmoker, C., Hernandez-Leon, S., and Calbet, A. (2013). Microzooplankton grazing in the oceans: impacts, data variability, knowledge gaps and future directions. J. Plankton Res. 35, 691–706. doi: 10.1093/plankt/fbt023
Schnack-Schiel, S. B., Mizdalski, E., and Cornils, A. (2010). Copepod abundance and species composition in the Eastern subtropical/tropical Atlantic. Deep Sea Res. II Topic. Stud. Oceanogr. 57, 2064–2075. doi: 10.1016/j.dsr2.2010.09.010
Schulz, K. (1986). Aspects of calanoid copepod distribution in the upper 200 m of the central and southern Sargasso Sea in spring 1979. Syllogeus 58, 459–466.
Siokou-Frangou, I., Christaki, U., Mazzocchi, M. G., Montresor, M., Ribera d'Alcalà, M., Vaqué, D., et al. (2010). Plankton in the open Mediterranean Sea: a review. Biogeosciences 7, 1–44. doi: 10.5194/bg-7-1543-2010
Sterner, R. W., and Elser, J. J. (2002). Ecological Stoichiometry: The Biology of Elements from Molecules to the Biosphere. Princeton, NJ: Princeton University Press.
Stoecker, D. K., and Capuzzo, J. M. (1990). Predation on protozoa: its importance to zooplankton. J. Plankton Res. 12, 891–908. doi: 10.1093/plankt/12.5.891
Tanaka, T., Thingstad, T. F., Christaki, U., Colombet, J., Cornet-Barthaux, V., Courties, C., et al. (2011). Lack of P-limitation of phytoplankton and heterotrophic prokaryotes in surface waters of three anticyclonic eddies in the stratified Mediterranean Sea. Biogeosciences 8, 525–538. doi: 10.5194/bg-8-525-2011
Ternon, E., Guieu, C., Ridame, C., L'Helguen, S., and Catala, P. (2011). Longitudinal variability of the biogeochemical role of Mediterranean aerosols in the Mediterranean Sea. Biogeosciences 8, 1067–1080 doi: 10.5194/bg-8-1067-2011
Tiselius, P. (1992). Behavior of Acartia tonsa in patchy food environments. Limnol. Oceanogr. 37, 1640–1651. doi: 10.4319/lo.1992.37.8.1640
Tiselius, P., and Jonsson, P. R. (1990). Foraging behaviour of six calanoid copepods: observations and hydrodynamic analysis. Mar. Ecol. Prog. Ser. 66, 23–33. doi: 10.3354/meps066023
Uttieri, M., Brown, E. R., Boxshall, G. A., and Mazzocchi, M. G. (2008). Morphology of antennular sensors in Clausocalanus furcatus (Copepoda: Calanoida). J. mar. biol. Ass. UK, 88, 535–541 doi: 10.1017/s0025315408000854
Uye, S. (1991). Length-weight relationships of important zooplankton from the Inland Sea of Japan. J. Oceanogr. Soc. Jpn. 38, 149–158. doi: 10.1007/BF02110286
Valdes, L., Lopez-Urrutia, A., Cabal, J., Alvarez-Ossorio, M., Bode, A., Miranda, A., et al. (2007). A decade of sampling in the Bay of Biscay: what are the zooplankton time series telling us? Prog. Oceanogr. 74, 98–114. doi: 10.1016/j.pocean.2007.04.016
Verity, P., and Langdon, C. (1984). Relationships between lorica volume, carbon, nitrogen and ATP content of tintinnids in Narragansett Bay. J. Plankton Res. 6, 859–868. doi: 10.1093/plankt/6.5.859
Villar-Argaiz, M., Bullejos, F. J., Medina-Sanchez, J. M., Ramos-Rodriguez, E., Delgado-Molina, J. A., and Carrillo, P. (2012). Disentangling food quantity and quality effects in zooplankton response to P-enrichment and UV radiation. Limnol.Oceanogr. 57, 235–250. doi: 10.4319/lo.2012.57.1.0235
Keywords: mesocosm experiments, dust, aerosols, copepods, feeding, Clausocalanus furcatus, Eastern Mediterranean
Citation: Christou ED, Zervoudaki S, Fernandez De Puelles ML, Protopapa M, Varkitzi I, Pitta P, Tsagaraki TM and Herut B (2017) Response of the Calanoid Copepod Clausocalanus furcatus, to Atmospheric Deposition Events: Outcomes from a Mesocosm Study. Front. Mar. Sci. 4:35. doi: 10.3389/fmars.2017.00035
Received: 30 September 2016; Accepted: 27 January 2017;
Published: 14 February 2017.
Edited by:
Xabier Irigoien, King Abdullah University of Science and Technology, Saudi ArabiaReviewed by:
Dacha Atienza, Spanish National Research Council, SpainHans Uwe Dahms, Kaohsiung Medical University, Taiwan
Copyright © 2017 Christou, Zervoudaki, Fernandez De Puelles, Protopapa, Varkitzi, Pitta, Tsagaraki and Herut. This is an open-access article distributed under the terms of the Creative Commons Attribution License (CC BY). The use, distribution or reproduction in other forums is permitted, provided the original author(s) or licensor are credited and that the original publication in this journal is cited, in accordance with accepted academic practice. No use, distribution or reproduction is permitted which does not comply with these terms.
*Correspondence: Epaminondas. D. Christou, ZWRjQGhjbXIuZ3I=