- 1Bimini Biological Field Station Foundation, Bimini, Bahamas
- 2Department of Biological Sciences, Florida International University, Miami, FL, U.S.A
- 3Microwave Telemetry, Inc., Columbia, MD, U.S.A
- 4Leibniz-Institute of Freshwater Ecology and Inland Fisheries, Berlin, Germany
- 5Department of Fisheries and Wildlife, Michigan State University, East Lansing, MI, U.S.A
- 6Department of Biology and Institute of Environmental Science, Carleton University, Ottawa, ON, Canada
- 7Waterman Project, Geneva, Switzerland
- 8Florida State University Coastal and Marine Laboratory, St. Teresa, FL, U.S.A
- 9Rosenstiel School of Marine and Atmospheric Sciences, University of Miami, Miami, FL, U.S.A
A thorough understanding of movement patterns of a species is critical for designing effective conservation and management initiatives. However, generating such information for large marine vertebrates is challenging, as they typically move over long distances, live in concealing environments, are logistically difficult to capture and, as upper-trophic predators, are naturally low in abundance. Large-bodied, broadly distributed tropical shark typically restricted to coastal and shelf habitats, the great hammerhead shark Sphyrna mokarran epitomizes such challenges. Highly valued for its fins (in target and incidental fisheries), it suffers high bycatch mortality coupled with fecundity conservative life history, and as a result, is vulnerable to over-exploitation and population depletion. Although there are very little species-specific data available, the absence of recent catch records give cause to suspect substantial declines across its range. Here, we used biotelemetry techniques (acoustic and satellite), conventional tagging, laser-photogrammetry, and photo-identification to investigate the level of site fidelity/residency for great hammerheads to coastal areas in the Bahamas and U.S., and the extent of movements and connectivity of great hammerheads between the U.S. and Bahamas. Results revealed large-scale return migrations (3030 km), seasonal residency to local areas (some for 5 months), site fidelity (annual return to Bimini and Jupiter for many individuals) and numerous international movements. These findings enhance the understanding of movement ecology in great hammerhead sharks and have potential to contribute to improved conservation and management.
Introduction
Animal movement influences the distribution and abundance of organisms, as well as ecological processes operating at the population, community, and ecosystem level (Bestley et al., 2013). A thorough understanding of movement patterns of a species is critical for designing effective conservation and management initiatives (Lascelles et al., 2014; Heupel et al., 2015). However, incorporation of movement data into conservation strategies remains underutilized and a common challenge for researchers, as there is often a lack of knowledge of where, when, and why a species moves (Allen and Singh, 2016). These difficulties are exacerbated for large marine vertebrates that move long distances, live in concealing environments, are logistically difficult to capture and as upper-trophic predators, are naturally low in abundance (McClenachan et al., 2012; Dulvy et al., 2014). However, recent advances in remote monitoring devices, such as biotelemetry (satellite, radio and acoustic telemetry) and biologging (archival loggers) have revolutionized our capabilities for observation (Cooke et al., 2008), providing the tools to understand the causes and consequences of species' movement patterns and their underlying drivers, over ecologically meaningful spatial and temporal scales (Hussey et al., 2015). These data have resulted in conservation success stories; for example, strategies to reduce leatherback turtle (Dermochelys coriacea) fisheries interactions were developed from telemetry data (Shillinger et al., 2008). Using a behavioral representation of bluefin tuna (Thunnus thynnus) migration, Armsworth et al. (2010) determined the economic efficiency of a time-area closure to protect spawning fish. More recently, studies have highlighted the importance of movement data for identifying stock boundaries and to inform management of highly migratory species, as well as species of conservation concern (Block et al., 2011; Costa et al., 2012; Queiroz et al., 2016).
For animals that live in concealing environments, such as sharks, biotelemetry advances have been crucial in revealing common behaviors across species, such as philopatry, a broad concept describing the geospatial preference of animals for their homes (Mayr, 1963; Hueter et al., 2005; Chapman et al., 2015). This definition includes residential, non-dispersive behavior as well as more complex return-migrations in which individuals occupy a site for a defined period of time, leave it, and later return to it (Chapman et al., 2015). Philopatric behavior is ubiquitous for marine and terrestrial taxa including bony fishes, turtles, birds and mammals and can have important implications for structuring populations (Greenwood, 1980; Dittman and Quinn, 1996; Lohmann et al., 2013). Philopatry is particularly significant for migratory marine species that are inherently challenging to manage, as fidelity to well-defined habitats supports marine protected area (MPA) designations (Lascelles et al., 2014).
For sharks, a recent review found evidence of philopatric behavior in 31 species from six of the nine extant orders, with robust evidence of residency and site fidelity in many species, and emerging evidence of natal or regional philopatry by reproducing individuals (Chapman et al., 2015). Most revealing is that examples were taxonomically diverse, from highly mobile white sharks (Carcharodon carcharias), that made long-distance movements punctuated by periods of seasonal residency at a few aggregation sites (Bruce and Bradford, 2013), to the typically sedentary, benthic Port Jackson shark (Heterodontus portusjacksoni), that returned annually to parturition sites (Bass et al., 2016). Furthermore, philopatric behaviors were in some cases limited to different life stages; small juvenile sandbar sharks (Carcharhinus plumbeus) demonstrated seasonal climatic migrations of more than 200 km from their natal estuary during their first winter, returning to the natal estuary in subsequent summers for at least 3 years (Grubbs et al., 2007; Grubbs, 2010). More recently, a study on oceanic whitetips (Carcharhinus longimanus) at Cat Island, Bahamas, revealed the first evidence of philopatry and seasonal site fidelity in a highly mobile pelagic species (Howey-Jordan et al., 2013; Madigan et al., 2015). For lemon sharks (Negaprion brevirostris), this behavioral trait has even been extended to natal homing to specific nursery sites (Feldheim et al., 2014). Exhibiting such behaviors makes sharks, and indeed other marine species that behave similarly, vulnerable to spatially focused fishing, which can lead to disproportionate effects on different population components (Mucientes et al., 2009; Lascelles et al., 2014; Chapman et al., 2015). Unquestionably, effective shark fisheries management requires detailed, species-specific information to identify spatial hotspots to inform decisions, such as MPA and time-area closures (Lascelles et al., 2014).
The great hammerhead (Sphyrna mokarran) is a large, highly mobile, coastal-pelagic and semi-oceanic shark with a circumtropical distribution (Compagno, 1984; Cliff, 1995). This species inhabits deep waters, shallow lagoons and coral reefs. It is considered an upper-trophic level consumer (Cliff, 1995; Mourier et al., 2013), and due to its known long-range movements is likely an important mobile link species between ecosystems (Lundberg and Moberg, 2003; Heupel et al., 2015) playing an important part in the functioning, structure, and stability of these systems (Ferretti et al., 2010; Roff et al., 2016). Great hammerheads are a target or bycatch species in a variety of fisheries throughout their range, and as a result, substantial population declines are suspected to have occurred in many areas (Denham et al., 2007; Miller et al., 2014). Globally, the International Union for the Conservation of Nature (IUCN) Red List categorizes great hammerheads as Endangered (Denham et al., 2007). In 2013, they were added to the Convention on International Trade in Endangered Species (CITES) Appendix II, and in 2014 the Convention on the Conservation of Migratory Species of Wild Animals (CMS) Appendix II. These listings should improve international regulation and management, and promote sustainable and legal trade of hammerhead shark products.
In the western North Atlantic, great hammerheads occur from the Florida Keys to Virginia and across the Bahamas (Kohler et al., 1998; Miller et al., 2014). Through a long-term mark-recapture study, Kohler and Turner (2001) found the maximum distance traveled to be 1180 km with four years at liberty. Hammerschlag et al. (2011) highlighted an individual migration of at least 1200 km from the Florida Keys to 500 km off the coast of New Jersey, suggesting that this shark followed the warm waters of the Gulf Stream. More recently a study that deployed satellite devices on great hammerheads (n = 18) found their core habitat-use areas to be in the combined waters of Florida and U.S. exclusive economic zone (EEZ) (Graham et al., 2016). Documented movements into fronts within the Atlantic Ocean were further highlighted by Queiroz et al. (2016). Historically, across the Bahamas great hammerheads have been rarely encountered. Only two individuals were captured on shallow-water longlines set bi-monthly in Bimini from 2003 to 2008 (Kessel, 2010), and three across four seasons in Eleuthera (Brooks et al., 2012). Interestingly, a handful of observations have documented great hammerhead sharks' use of extreme shallow water (< 2 m) habitats in Andros Island and Eleuthera (Roemer et al., 2016), as well as two predation events in Bimini on a southern stingray (Dasyatis americana) and spotted eagle ray (Aetobatus narinari) (Strong et al., 1990; Chapman and Gruber, 2002). However, more recently, great hammerheads have been reliably encountered off the west of Bimini, in shallow sand bottom waters during the winter months (O'Connell and Leurs, 2016).
Previous studies suggest that great hammerheads are likely predictable in their horizontal movements, with core-habitat use areas in coastal regions and seasonal movements to temperate oceanic waters. However, despite these recent advances in our understanding there is an urgent need for further information on great hammerhead spatial hotspots and migratory corridors to improve their conservation and management. In the present study, we used biotelemetry techniques (acoustic and satellite), conventional tagging, laser-photogrammetry, and photo-identification to investigate: (a) the level of site fidelity/residency for great hammerhead sharks in coastal areas in the Bahamas and U.S. and (b) the extent of movements and connectivity of great hammerheads between the U.S. and Bahamas. Results provide new insights into the movement ecology of this endangered, large coastal shark that could be important for designing effective conservation action.
Materials and Methods
Study Sites
To examine philopatric behavior in the great hammerhead shark our team focused research efforts at two study sites; (1) Bimini, Bahamas (25°44′ N, 79°16 W)—a chain of small, mangrove-fringed islands located on the western edge of the Great Bahama Bank, 86 km due east of Miami, Florida. The two main islands South and North Bimini envelope a semi-enclosed shallow (< 2 m) lagoon (area, ~21 km2), with seagrass beds and sand flat habitats extending east onto the Great Bahamas Bank. On the west of the islands, fringing coral reefs, sand and seagrass slope to the deep pelagic zone and the Gulf Stream (a powerful, warm, western-bound current), separating the islands from mainland U.S. and, (2) Jupiter, Florida, U.S. (26°55′ N, 80°06 W)—located on the southeast Atlantic coast of Florida. The marine environment here is composed of the relatively narrow continental shelf (~6 km) dropping off steeply to the east into the deeper waters of the Gulf Stream. The shelf contains reef-lines at 20, 30 and 50 m depth contours, with an intermittent string of artificial reefs, running south to north (Banks et al., 2008; Kessel et al., 2014a).
At both sites, it was possible to capture and equip great hammerhead sharks with acoustic, satellite and conventional tags, however Bimini's excellent underwater conditions enabled our team to use additional methods, such as breath-hold diving to attach tags, photo-identification and laser photogrammetry to supplement tracking data (O'Connell and Leurs, 2016). Methods varied based on study site and available personnel and gear, and these, and the associated monitoring periods, are summarized in Table 1. Below, we include basic descriptions of the methods used and provide further details in the Supplementary Materials.
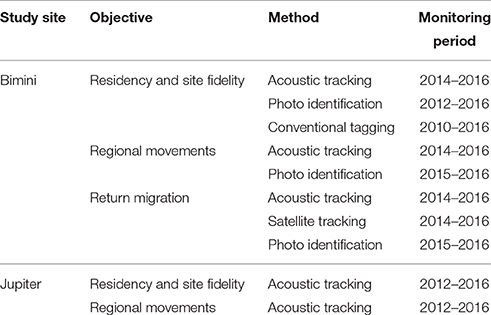
Table 1. Summary of methods used to examine the philopatric behavior of the great hammerhead shark, Sphyrna mokarran in Bimini, Bahamas and Jupiter Inlet, Florida, U.S.
Acoustic Tagging and Monitoring
Great hammerheads were either tagged externally with acoustic transmitters (V16-6H; battery life 1623 days, interval 90–150 s) via breath-hold techniques, or were implanted once captured via a free-floating method (see Kessel et al., 2014a and Supplementary Materials for description; Table 2). Length estimates were either made via measurement pre-caudal (LPC) and fork (LF) on the side of the research vessel, laser photogrammetry techniques (see Supplementary Materials) or to nearest 50 cm by the co-author who tagged the sharks (WW). Following Miller et al. (2014) all length estimates were converted to total length (LTL).
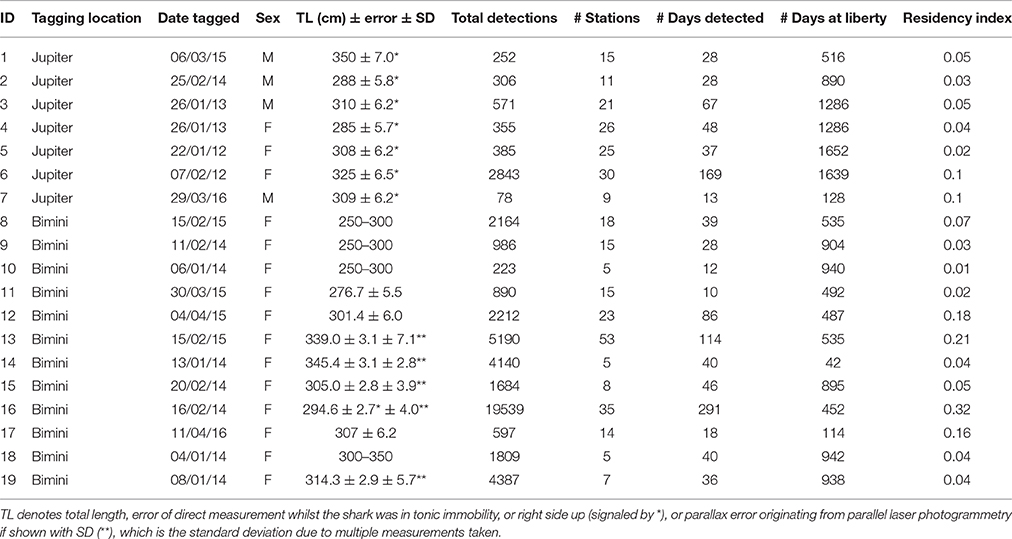
Table 2. Summary of great hammerhead sharks, Sphyrna mokarran (n = 19) monitored within the Atlantic Cooperative Telemetry (ACT) network in the western North Atlantic Ocean with acoustic transmitters.
In January 2006, an array of 17 acoustic receivers (VR2W; Vemco®) was deployed along the coast between Delray Beach (26° 28′ N, 80° 02′ W) and Hobe Sound, Florida (27° 03′ N, 80° 02′W; Figure 1) as part of a long-term study monitoring large coastal sharks (see Kessel et al., 2014b). In January 2014, 27 acoustic receivers (VR2W; Vemco®) were deployed in various habitats in Bimini, Bahamas (25° 44′ N, 79° 16′ W). This array was subsequently increased in February 2015 to 49 receivers, expanding coverage (Figure 1). Water temperature was continuously monitored by attaching HOBO® Pro V2 (Onset Computer Corporation) temperature loggers to each acoustic receiver in Jupiter, Florida and a subset of receivers in Bimini. Receivers were retrieved and downloaded in Jupiter annually and in Bimini every 6 months. Participation in a data-sharing network substantially increased the potential for detecting great hammerhead movements outside of the array. For details on this data-sharing network, receiver locations, detection range, and mooring types, see Supplementary Materials.
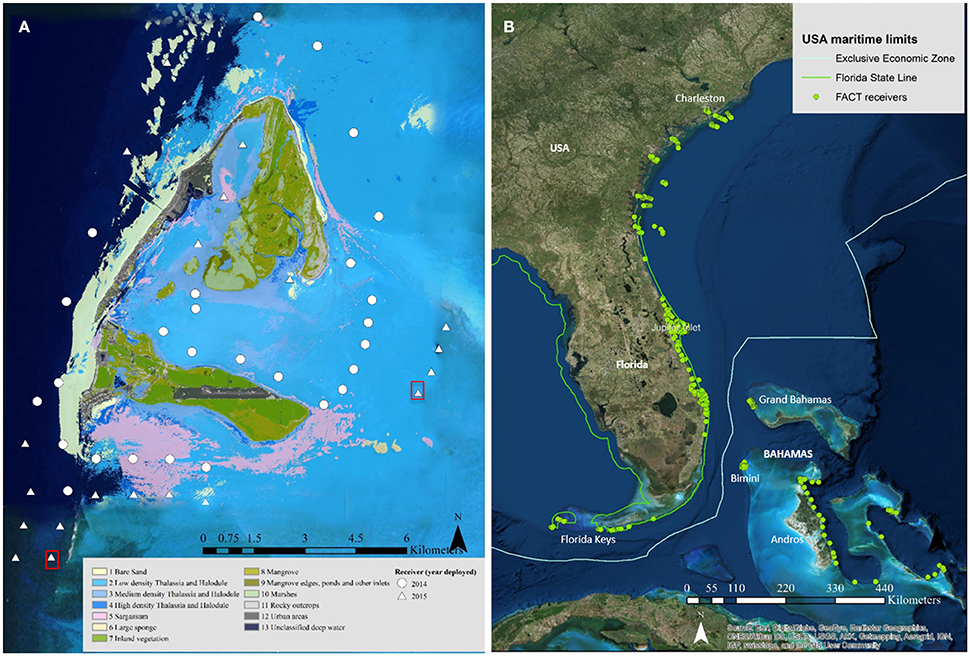
Figure 1. (A) The Bimini islands in the Bahamas. Symbols depict individual receiver stations deployed in 2014 (circles) and 2015 (triangles) in various habitats indicated by different colors (see figure legend on bottom). Red squares indicate receiver locations with sentinel tags deployed. Image credit: LAND INFO Worldwide Mapping, LLC, includes material Copyright © DigitalGlobe-Longmont, Colorado. All rights reserved, through a Enterprise Licence. (B) Atlantic Cooperative Telemetry (ACT) data share consortium with individual receiver stations (n = 762) spanning mainland USA (Florida, Georgia and South Carolina) and the Bahamas (e.g., Andros, Bimini, Cape Eleuthera and Grand Bahama). Source: Esri, DigitalGlobe, GeoEye, Earthstar Geographics, CNES/Airbus DS, USDA, USGS, AEX, Getmapping, Aerogrid, IGN, IGP, Swisstopo, and the GIS user community.
Archival Satellite Telemetry
Great hammerheads (n = 5) were tagged with two types of pop-up satellite archival tags (PSATs): X-Tags (Standard Rate [SR] and High Rate [HR]) and one Standard Archival Tag (HR) (Microwave Telemetry, Inc., Columbia, MD, USA). Tags with SR programming transmitted depth, temperature and light-level data at a resolution of > 15-min intervals to the Argos satellite constellation (Table 3). HR tags were programed to pop-off after 30 days and transmit 5-min records. SR tags also provided daily minimum and maximum depth and temperature records, determined from archived 2-min records. Although SR tags provided light-based location estimates, the two SR tags in this study were only at liberty for 3 days before they were shed, and thus, movements could not be resolved by light-based location estimation (error is at best ± 0.5° Longitude and ± 1° Latitude) (http://www.microwavetelemetry.com/fish/; Standard Rate X-Tag Data and Reports). Consequently, for all tag deployments, we have the deployment (start) location and the first Argos (end, Doppler) location, allowing for the approximation of individual net displacement. See Supplementary Materials for information regarding methods of attachment.
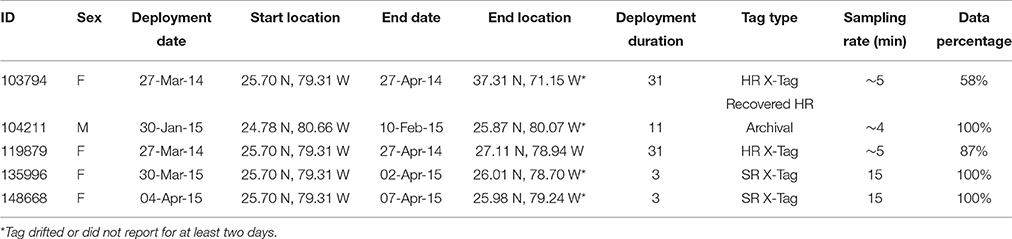
Table 3. Pop-up satellite archival tag (PSAT) summary information for great hammerhead sharks, Sphyrna mokarran tagged in Bimini, Bahamas (n = 4) and Florida, U.S. (n = 1).
Photo-Identification
Underwater visibility in Bimini was typically high allowing for quality images of great hammerheads during dives. Photographs were taken to facilitate individual identification using natural marks, fin morphology, wounds and pigmentation (Kohler and Turner, 2001; Domeier and Nasby-Lucas, 2007; Marshall and Pierce, 2012). Images supplemented external dart and acoustic tagging information, providing important re-identification methods if tags were shed or did not report. Profiles of individual great hammerheads were generated such that detailed tagging and photographic information would ensure sharks could be tracked throughout seasons and across years.
Data Analysis
Acoustic data were filtered to remove false-positive detections, which were defined as any single transmitter detection occurring alone within a 1-h period (see Kessel et al., 2014b). In addition, detections during the first 24 h post-tagging were discarded to eliminate potential bias resulting from the tagging event. Finally, double detections (n = 2105) by multiple receivers were removed from the full data set, yielding 49,670 detections. Prior to analysis, great hammerheads were omitted (16 of 35) if individuals were detected less than 10 days within the Atlantic Cooperative Telemetry (ACT) data-share consortium (Kessel et al., 2014b), taking into account the migratory behavior of the species (no detections occurred outside ACT network). Acoustic data obtained from two external tags that were observed removed was pooled with data from two internals tag (great hammerhead # 12 and 17, Table 1) as photo-identification confirmed the identity of the individuals. Detection data of the remaining individuals (n = 19) were analyzed to obtain, for all arrays combined (i.e., ACT network) the number of times an individual was detected, the number of receiver locations on which an individual was present, the number of days an individual was detected, and the number of days at liberty. A monthly, standardized residency index (RI) for each individual was calculated as the number of days within a month in which the animal was detected divided by the number of days at liberty each month in which it could have been detected (Bond et al., 2012). Movements were included for great hammerheads that crossed natural barriers (e.g., Gulf Stream or Northwest Providence channel) as well as when sharks crossed exclusive economic zones (EEZ) or state boundaries.
In 2016, we calculated a residency index for each individual to our provisioning site. This was the number of days within a month in which an animal was observed (via photo ID) divided by the number of days in which it could have been observed.
Archival Satellite Telemetry
One individual demonstrated a northern migration while occupying a relatively narrow depth and temperature range. In an effort to discern the northern migration route, we matched the tag recorded temperature with the temperature-at-depth World Ocean Atlas (WOA) 2013 dataset; the WOA April statistical mean dataset is compiled from multiple decades on a 0.25° grid (Locarnini et al., 2013). Considering only the period of time after the last acoustic detection at Bimini, daily mean tag-recorded depth and temperature were calculated. Next, the locations (latitude/longitude) with matching WOA and tag-recorded temperature-at-depth (within ±0.5°C) were overlaid on the April sea surface temperature (SST) field obtained from the WOA 2013 dataset, allowing for visual assessment of a potential migration route (see Supplementary Material). Each day, until the end of deployment, was considered independently.
Laser Photogrammetry
Video of 1080p resolution (30 frames per second) was used to record great hammerheads during dives and individual still frames were selected when the shark appeared to be perpendicular to the camera. Images were analyzed using ImageJ to count the number of pixels between the two laser marks. Pre-caudal length (cm) was then determined by measuring straight-line distance from the anterior edge of the cephalofoil to midway between the pre-caudal notches. Pre-caudal length was converted to total length using the formula: TL = (PCL + 9.16)/0.81 (Cliff, 1995). Individuals were measured on multiple occasions throughout the season and the mean of these was taken as the best length estimate.
Results
Tagging Summary
From 2000 to 2016 great hammerheads (n = 64; 48 female, 14 male, 2 unconfirmed) were caught or pole-tagged in Bimini, Bahamas ranging in size from 230 to 360 cm TL (301.9 ± 34.2, mean ± SD). From 2006 to 2016 great hammerheads (n = 44; 11 female, 27 male, 6 unconfirmed) were captured in Jupiter, Florida ranging in size from 195 to 400 cm TL (290.2 ± 38.5, mean ± SD). Acoustic transmitters (n = 31; 21 external and 10 internal) were deployed on great hammerheads between January 2012 and April 2016. Nineteen great hammerheads were detected on > 10 days, seven were tagged in Jupiter (3 female, 4 male) and 12 in Bimini (all female) (See Table 2 for further details). Most individuals were likely sexually mature based on Piercy et al. (2010) and Miller et al. (2014).
Site Fidelity and Residency
Across multiple years from 2012 to 2016, the majority of great hammerhead acoustic detections occurred synchronously between October and March, with individuals rarely detected April through November of respective years (Figure 2). Residency indices (RIs) within the overall U.S. and Bahamas receiver arrays ranged from 0.01 to 0.38 (0.08 ± 0.10) for the duration of the study period (Table 2).
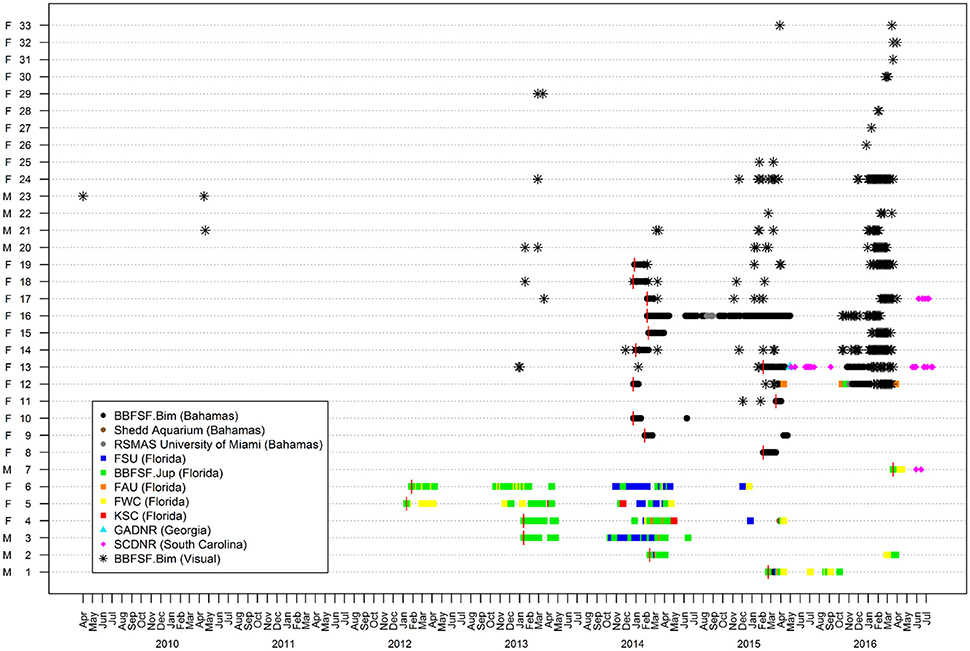
Figure 2. Presence/absence plot for great hammerhead sharks, Sphyrna mokarran (n = 33) between April 2012 and August 2016. Depicted are histories of weekly acoustic detections within Atlantic Cooperative Telemetry network of great hammerheads tagged in Jupiter, Florida (ID # 1–7) and Bimini, Bahamas (ID # 8–19), and re-sightings in Bimini through visual identification (ID # 11–33). Vertical red lines denote date of tagging.
Bimini, Bahamas
Great hammerheads monitored in Bimini displayed long-term site fidelity and seasonal residency (October–April) (Figure 2). Using a combination of photo ID, external dart tags and acoustic tracking we identified 26 individuals (Table 2 and see Figures 3A–E showing persistent fin notches, pigmentation and scars). Great hammerheads (n = 14; 4 male, 10 female) were confirmed to use Bimini across multiple years (Figure 4). Three females (# 13, 14 and 17) were identified on four consecutive seasons (2013–2016). One male (# 21) that was first tagged with a NMFS dart-tag on 2 May 2010, was re-sighted in 2014 and 2015, and captured in 2016. This represented a regular return use of Bimini across a 7-year period. Two females (# 18 and 19) returned for three consecutive years and another male (# 20) returned three of 4 years (2013–2016). Finally, two females (# 15 and 33) and males (# 22 and 23) were identified upon return for 2 years (Figure 4).
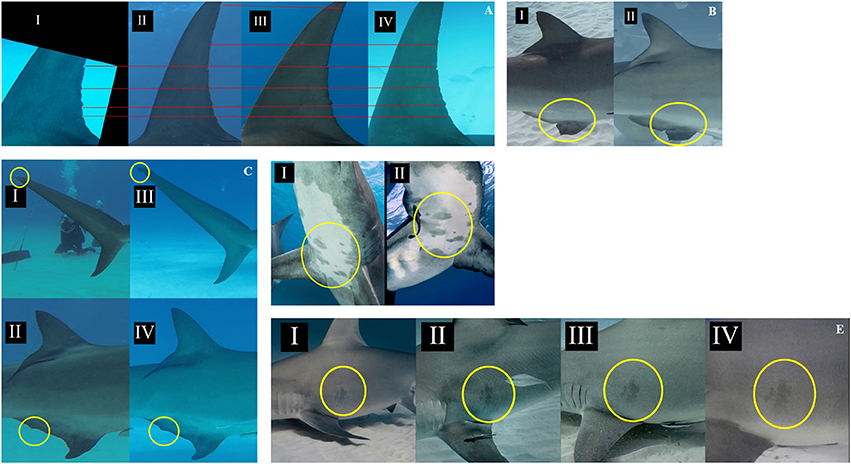
Figure 3. Depictions of several shark characteristics used for individual recognition across multiple years: (A) Dorsal fin trailing edge notches of great hammerhead shark, Sphyrna mokarran #17 I: Feb 2013, II: Mar 2014, III: Jan 2015, IV: Apr 2016, (B) Damaged anal fin of great hammerhead #20 I: Jan 2013, II: Feb 2016, (C) Disfigured upper lobe of caudal fin and anal fin of great hammerhead # 21 I and II: Mar 2014, III and IV: Jan 2016, (D) Ventral spot pattern of great hammerhead #12, I: Mar 2014, II: Jan 2016, (E) Pigmentation visible on great hammerhead #13 = I: Feb 2013, II: Jan 2014, III: Feb 2015, IV: Mar 2016.
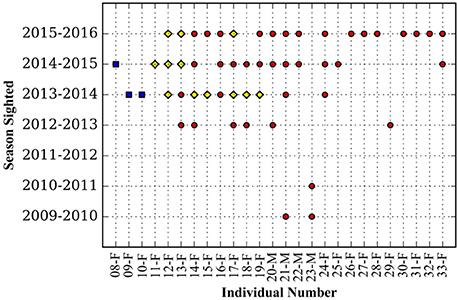
Figure 4. Seasonal presence in Bimini, Bahamas of individual great hammerhead sharks, Spyhrna mokarran obtained through a combination of visual identification (photo and external dart tag) (red circles) or photo identification and passive acoustic telemetry (yellow diamonds), or passive acoustic telemetry (blue squares).
Overall, great hammerheads were detected in Bimini throughout the year, with the exception of September. Monthly mean residency indices were highest during autumn (October–December) (0.42 ± 0.26) and winter (January–March) (0.68 ± 0.11), compared to spring (April–June) (0.28 ± 0.21) and summer (July–September) (0.15 ± 0.17). There was a negative correlation between mean monthly RI and water temperature (R2 = 0.70, P < 0.01; Figure 5A).
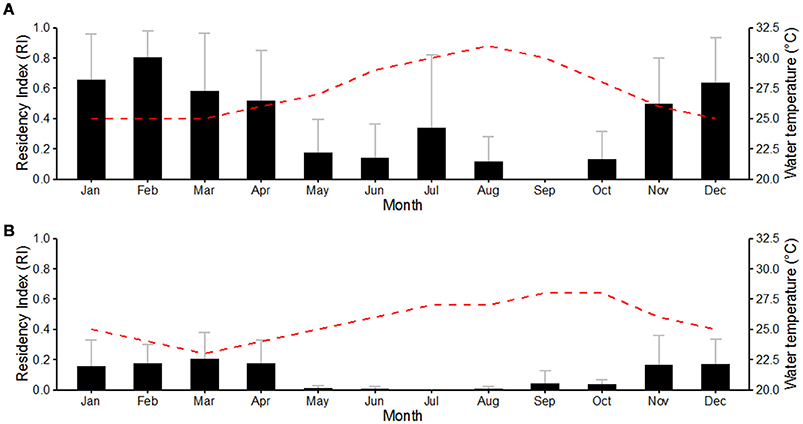
Figure 5. Effect of month on the mean residency index in Bimini, Bahamas (A) and Jupiter, Florida (B) of great hammerhead sharks Sphyrna mokarran, with mean monthly sea temperature. Bar charts depict mean monthly residency indices between March 2012 and January 2016. Gray error bars represent standard deviation. The red dashed line represents mean monthly water temperature recorded at the study sites.
In the 2015–2016 season, 82 observation days were conducted off South Bimini from 23 October to 12 April. Great hammerheads (n = 18) were identified via visual ID (Figure 3) (Female = 15, Male = 3) and were observed arriving throughout the season (e.g., 23 October 2015–March 31 2016). Some sharks were present nearly the entire season (e.g., great hammerheads # 14 and 13 with; 155 and 121 days, respectively), whereas others were sighted on a few days (e.g., great hammerheads # 28 and 32 for 2 days each) and then likely departed Bimini. Overall, mean provisioning site residency ranged from 0.01 to 0.6 (0.21 ± 0.20) with the highest value recorded during February (0.27 ± 0.26) and lowest in December (0.09 ± 0.17). There was a positive correlation between overall provisioning site index and the number of days between a shark's first and last sighting in Bimini (R2 = 0.74, P < 0.001).
Jupiter, U.S.
Great hammerheads displayed long-term site fidelity and seasonal residency off Jupiter Inlet, Florida (October–March) (Figure 2). One female great hammerhead (# 6) was detected in Florida (BBFSF-Jupiter and FWC arrays) on four consecutive seasons and two female great hammerheads (# 5 and # 4) were detected for three consecutive seasons (BBFSF-Jupiter and FWC). Further, a male great hammerhead (# 3) was detected for two consecutive seasons (BBFSF-Jupiter) and another (# 2) was detected (BBFSF-Jupiter) in winter 2014 and 2016. Although punctuated with occasional periods of absence, great hammerheads were frequently detected in the BBFSF-Jupiter and FWC arrays for continuous time periods (e.g., great hammerhead # 6 was detected regularly across 177 days in 2012–2013, and 172 days 2013–2014) indicative of seasonal residency to this coastal area (between Delray Beach [26° 28′ N, 80° 02′ W] to the south and Port St. Lucie [27° 14′ N, 80° 07′ W] to the north, stretching offshore ~7 km. Monthly mean RIs supported this pattern with the highest values during autumn (October–December) (0.12 ± 0.08) and winter (January–March) (0.18 ± 0.02), compared to spring (April–June) (0.07 ± 0.1) and summer (July–September) (0.02 ± 0.02). There was a negative correlation between mean monthly RI and water temperature (R2 = 0.51, P < 0.01; Figure 5B).
Regional Movements and Return Migrations
Four great hammerheads tagged in Bimini exhibited movements (three round-trips) to the Grand Bahamas and Florida coastline (Figure 6; Table 4). Two great hammerheads tagged in Jupiter, Florida made movements to Bimini and Andros (Figure 6; Table 4). Finally, four great hammerheads (Bimini, n = 3 and Jupiter, n = 1) made long-distance (> 500 km; two round-trip) movements departing April and May to South Carolina and Virginia (Figure 6; Table 4).
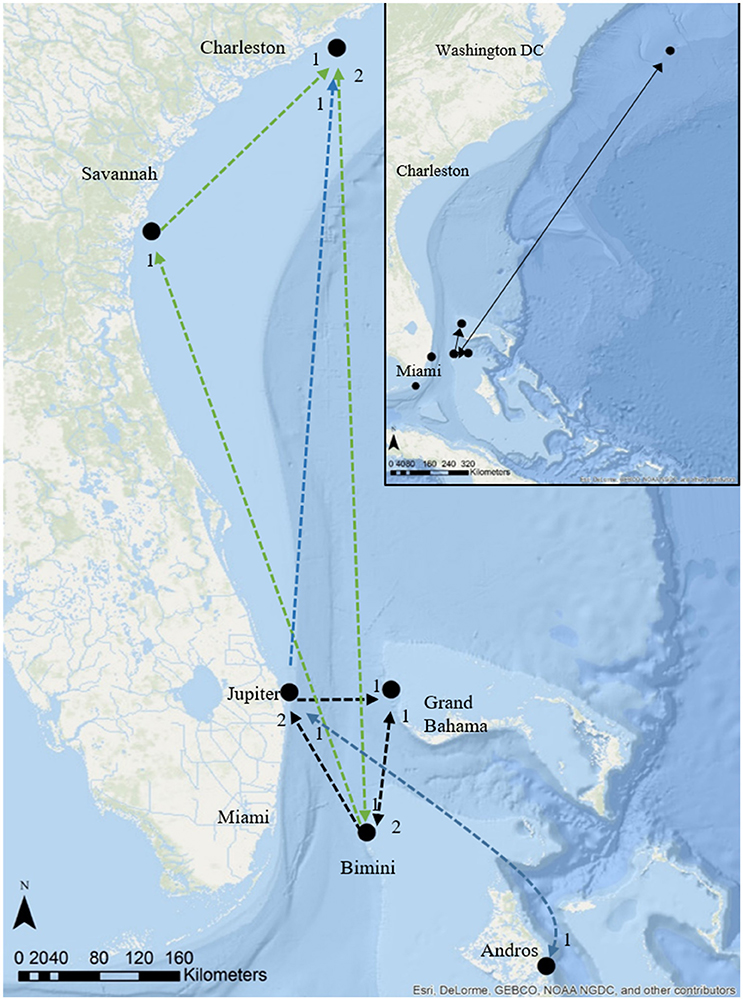
Figure 6. Regional movements and migrations of great hammerhead sharks, Sphyrna mokarran tracked using satellite telemetry and photo ID (insert), main map acoustic telemetry and tracks of great hammerhead # 12 (black dashed line) and great hammerhead # 13 (green dashed line) and multiple individuals (blue dashed line). Numbers below symbols denote # directed movements between locations. Source: Esri, Delorme, GEBCO, NOAA NGDC, and other contributors.
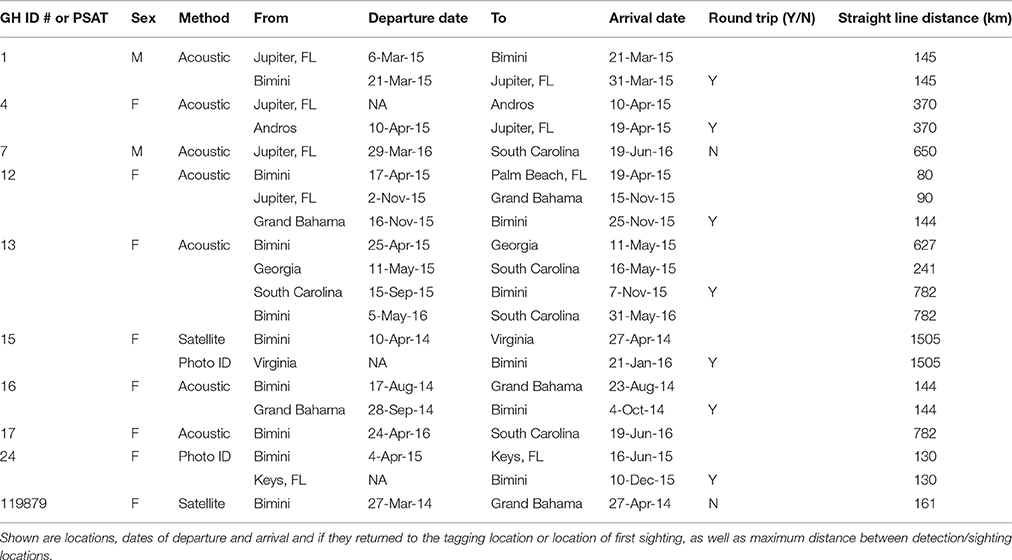
Table 4. Regional movements and displacements of a subset of great hammerhead sharks, Sphyrna mokarran in the western North Atlantic Ocean monitored with acoustic and satellite telemetry or through photo identification.
Highlighted Individual Movements
Great hammerhead # 12 was monitored for 2 weeks in Bimini January 2014 after which its external tag was observed detached. It was identified via external features on 15 November 2014 and subsequently observed in Bimini until 4 April 2015, when it was acoustically tagged internally and satellite tagged (PSAT ID 148668). It was then observed in Bimini for a further 8 days, 10–17 April 2015, and on 19 and 22 April 2015 was detected in Florida Atlantic University (FAU) array (Boca Raton) and FWC (Jupiter Inlet) arrays for 1 day each, respectively. Its movements during the summer were unknown until being detected on FAU array toward the end of October for 6 days, followed by 1 and 2 days in BBFSF Jupiter and Grand Bahamas (15 November), respectively. It returned to Bimini on 25 November 2015 and stayed for 67 days until 20 January 2016 (Figure 6, Table 4). On 6 April, 2016 it was last detected in FAU for 1 day.
Great hammerhead # 13 stayed in Bimini for 69 days after receiving an external tag on 15 February 2015. She was subsequently detected in Georgia on 11 May 2015 and South Carolina for 128 days from 16 May to 15 September 2015. She was again detected in Bimini on 7 November 2015 throughout winter 2016 for 179 days (Figure 6, Table 4), departing 5 May 2016. Finally, for the second consecutive year she was detected in South Carolina, arriving 31 May, latest record 3 August 2016.
Great hammerhead # 15 stayed in Bimini for 36 days after receiving an external tag on 21 February 2014. On 27 March 2014, a PSAT (ID 103794) was attached. For the next 2 weeks this shark was detected intermittently in Bimini until its last detection on 10 April 2014. The first Argos location was 1505 km northeast, ~500 km off the Virginia coastline on 27 April 2014. Assuming migration commenced as early as 10 April, this shark covered an average of 83.6 km per day (based on straight-line distance). During this time (between the last Bimini detection and the first Argos location), the locations with matching tag-recorded and WOA-derived temperature-at-depth values overlapped the warm waters of the Gulf Stream (Supplementary Material S2). On 21 January 2016, this shark returned to Bimini, as confirmed via dorsal fin identification (Figure 3A). It remained in Bimini until 23 March 2016 (Table 4).
Discussion
This study provides the first evidence of philopatric behavior (e.g., return-migrations, seasonal residency and long-term site fidelity) in the highly mobile great hammerhead shark (Sphyrna mokarran). Great hammerheads tagged in Bimini, Bahamas and Jupiter, Florida were recorded to make return-migrations from as far north as Virginia, U.S. (return trip ~3000 km), as well as movements to and from Jupiter and Key Largo in Florida, and Bahamian Islands: Andros, Bimini and Grand Bahama. These movements were typically made toward the end of the winter season, indicating they were of alimentary or reproductive, rather than climatic, migrations (Grubbs and Kraus, 2010). Great hammerheads at both of our study sites displayed long-term site fidelity, with some individuals (both sexes) observed or detected in four consecutive winter seasons. Further, many great hammerheads stayed during the winter (i.e., seasonal residency) and were either detected throughout the FACT array along the Florida coastline, as far north as Cape Canaveral, observed at the dive site, or detected in our acoustic array in Bimini. Such philopatric behavior is emerging as a common phenomenon structuring taxonomically diverse animal populations, with important implications for management (Greenwood, 1980; Chapman et al., 2015). For example, the predictable, seasonal, return-use of specific locations, areas or migratory routes enhances vulnerability to spatially focused fishing (Chapman et al., 2015; Queiroz et al., 2016). For great hammerheads, this is particularly concerning given their declining global populations, low and diffuse abundance, sensitivity to capture mortality and demand in the fin trade (Miller et al., 2014; Gulak et al., 2015).
Evidence for Philopatry
Site Fidelity and Residency
Mature male and female great hammerheads tagged in Bimini, Bahamas and Jupiter, Florida displayed long-term site fidelity, with some individuals recorded on up to four consecutive winter seasons. Such annual reuse of particular areas has long been documented across the animal kingdom, including sea turtles (Schofield et al., 2010), bats (Hillen et al., 2009) and birds (Blackburn and Cresswell, 2016), and is emerging as a widespread behavior for large-bodied coastal and oceanic sharks (Chapman et al., 2015). For example, tiger (Galeocerdo cuvier), lemon and oceanic whitetip sharks tracked in the same region exhibited, repeated migratory philopatry to overwintering sites (Howey-Jordan et al., 2013; Kessel et al., 2014a; Lea et al., 2015). For the latter two species, the authors concluded that enhanced feeding opportunities and thermal conditions were the likely driver of the aggregations. At our study sites, there was an absence of fresh mating wounds on female great hammerheads, or swollen claspers in males, which suggests, that the main purpose of site fidelity in great hammerheads is not mating, pupping or use of natal sites. Both our study sites are productive systems, prompting the hypothesis that great hammerheads return for feeding opportunities. The Bimini islands are the only mangrove habitat on the northwestern edge of the Great Bahama Bank (Jennings et al., 2012). They provide key nursery areas for diverse species, including various elasmobranchs (Jennings et al., 2012; Feldheim et al., 2014) that represent important dietary items for great hammerhead sharks (Miller et al., 2014). Indeed, the only published natural predation events by great hammerheads were documented in Bimini on southern stingray and eagle rays (Strong et al., 1990; Chapman and Gruber, 2002). Dasyatid stingrays dominated the diet of great hammerheads captured in South Africa, Australia as well as in Florida, U.S. (Dodrill, 1977; Stevens and Lyle, 1989; Cliff, 1995). Similarly, in the Jupiter region spawning bait fish, as well as, migration of blacktip sharks, Carcharhinus limbatus and abundance of other small sharks and batoids, likely provide important feeding grounds for great hammerheads (Hueter and Tyminski, 2007; Wiley and Simpfendorfer, 2007; Kajiura and Tellman, 2016).
At both study sites the highest monthly residency for great hammerheads was during the winter months. Preliminary analyses indicated that like other coastal species (Kessel et al., 2014a; Kajiura and Tellman, 2016; e.g., lemon and blacktip sharks) water temperature was a major driver of seasonal occurrence, however, future research should include other abiotic and biotic factors to better understand the causal relationship between environmental factors responsible for migratory behavior in this species (Schlaff et al., 2014). Like other coastal shark species (e.g., bull sharks, Espinoza et al., 2016), there was individual variation in great hammerhead seasonal residency. For example, in Bimini some great hammerheads arrived mid-season (i.e., February; great hammerheads # 28 and 32), only visiting for a handful of days, while others were detected on our acoustic array, or confirmed at the dive site weekly throughout the season (e.g., great hammerheads # 13 and 14). This variation did not appear to be influenced by sex or life stage, as all great hammerheads were likely mature and both sexes showed residency, as well as, transient site use. In Bimini, it is likely that regular provisioning, conducted from January through April since 2014 by diver operators, influenced the great hammerheads local behavior. Investigating this in detail is beyond the scope of the current study; however, early analyses from the 2015 to 2016 season showed that residency time in Bimini positively correlated with time spent at the provisioning site. Although we were unable to confirm that presence at the provisioning site each visit resulted in food consumption, it was clear from the number of sharks detected at this site's receiver, and those in close proximity, this activity was concentrating great hammerheads to the west of South Bimini. However, like tiger and white sharks that are regularly provisioned in Grand Bahama and Australia, respectively (Hammerschlag et al., 2012; Bruce and Bradford, 2013), great hammerheads continued to make long-distance migrations and departed at different times during the winter season.
Regional Movements and Return-Migrations
Three female great hammerheads (tagged in Bimini, Bahamas) and one male (tagged in Jupiter, Florida) made long-distance migrations (two of which were round trips spanning 1564 and 3010 km), in late spring to northwesterly locations in the U.S. (South Carolina and Virginia). Hammerschlag et al. (2011) documented the migration of a male great hammerhead from the Keys, Florida to 500 km off the coast of New Jersey, a distance of at least 1200 km. This shark presumably departed Florida in late march with locations transmitting mid-April off the coast of New Jersey, consistent with the timing of northern movements of female great hammerheads in Bimini and the male from Jupiter Hammerschlag et al. (2011) hypothesized that their great hammerhead likely followed the warm waters of the Gulf Stream in pursuit of prey fishes that make a similar migration. Great hammerheads are thought to be predators of blacktip sharks and have been documented via aerial surveys along the Florida coastline among mass aggregations of this species, suggesting this could be an important seasonal food source (Kajiura and Tellman, 2016). Interestingly, great hammerhead # 15, with its tag popping off in a similar location to the individual tagged in Hammerschlag et al. (2011), showed a distinct narrow temperature use (22.54 ± 0.34°C) after departing Bimini matching the water temperatures of the Gulf Stream. For aquatic ectotherms, seasonal rises in water temperature can act as an important cue in the timing of migration (e.g., salmon [Salmo salar] smolts Jonsson and Ruud-Hansen, 1985; squid [Loliginid forbesi] Sims et al., 2001). All three great hammerheads that exhibited long-distance migrations departed Bimini April to early May when the water temperature began to rise above 26°C. In the same region, water temperature was found to be a key predictor for the presence and absence of lemon and blacktip shark seasonal aggregations in Florida (Kessel et al., 2014a; Reyier et al., 2014; Kajiura and Tellman, 2016). Future studies on great hammerhead sharks should explore the timing, repeatability, and motivations for such long-distance movements, particularly as their travel routes cross various management and political boundaries.
Female great hammerhead #13 was detected off South Carolina, U.S. intermittently from June to September in the summer months for two consecutive years. It is possible she traveled north for parturition in one of those years. Great hammerheads in Bimini have been observed with distended abdomens, appearing to be gravid. Further examination of a pregnant great hammerhead (292 cm, TL) captured off South Bimini in winter contained 20 embryos (27.07 cm ± 0.99) indicating she was mid-term (Grubbs unpublished data). It is thought great hammerheads give birth late spring or early summer in the western North Atlantic (Clark and von Schmidt, 1965), although young of the year are captured (Hueter and Tyminski, 2007). However, recent work has confirmed the presence of neonate great hammerhead sharks in South Carolina estuaries (Barker et al. in review). Future studies should explore genetic differentiation by examining haplotype frequency of young of the year great hammerheads and adults sampled throughout the region. This will be important in determining if females are returning faithfully to parturition sites and whether there is an absence of female-mediated gene flow, which has consequences for stock management as discussed for other marine species, including sharks (Mourier and Planes, 2013; Ashe et al., 2015) cetaceans (Baker et al., 2013) and pinnipeds (Lopes et al., 2015).
Not all great hammerheads embarked on northerly migrations. Female great hammerhead # 24 was observed off Key Largo, Florida in June, and another female (# 16) was detected intermittently throughout the summer months in Bimini. Further, two male great hammerheads (# 1, # 3) were detected in June and August off Jupiter Inlet, Florida. Supporting these findings, aerial surveys conducted along the coast at Cape Canaveral documented large hammerhead (unidentified species), but most likely great hammerhead (> 300 cm) during the summer (Jennings, 1985). The idea that a portion of a population remains resident while the other migrate, known as partial migration, is a common phenomenon across the animal kingdom (Chapman et al., 2012). It has recently been suggested for some highly mobile shark species including tiger and bull sharks (Carcharhinus leucas) (Papastamatiou et al., 2013; Lea et al., 2015; Espinoza et al., 2016) with important implications for management and conservation. Further, Grubbs and Kraus (2010) demonstrated differential migration in the highly migratory and highly exploited sandbar shark, Carcharhinus plumbeus where partial migration is sex, age and maturity dependent. Juvenile sandbar sharks undergo alimentary return migrations to their natal region, but the duration (years) this migration is repeated is sex-dependent. As adults, pregnant females return to the natal region to give birth whereas non-pregnant females and adult males do not make this journey. Clearly further tagging efforts and monitoring will be required in order to explore this phenomenon in great hammerheads.
Conservation and Management Implications
Recently, in response to a petition to list great hammerheads under the U.S. Endangered Species Act, Miller et al. (2014) conducted a status review of the great hammerhead shark current and foreseeable extinction risk (defined as 50 years). Based on abundance trends, growth and productivity, spatial structure and diversity, they concluded their current extinction risk using various criteria ranged from “no risk” to “moderate risk.” Improvement in species identification and recent management (e.g., most declines were documented in the 1980 s; Jiao et al., 2011) resulted in a more positive outlook for future risk, with “no to low risk” scored. However, in their final remarks it was noted that much of the data were “severely lacking or flawed,” and they further emphasized that insufficient evidence was available on habitat characteristics, nursery grounds, with no documentation of female philopatry or specific migration routes. Importantly, since this report, a handful of studies have advanced our understanding. Using satellite telemetry Queiroz et al. (2016) found that the distribution of great hammerheads was generally restricted to coastal and shelf habitat, with sharks associating with sea surface temperature discontinuities and high productivity. Further, great hammerheads remained within relatively localized areas (e.g., Florida Keys and Daytona Beach) for extended periods of time, and made long-distance movements to and from predominant-use habitats. Graham et al. (2016), using data from the same sharks with an additional six animals, found that 17.87% of their core habitat use areas were within the protected Florida state waters (5.6 km off shore in the Atlantic and 16.7 km offshore in the Gulf of Mexico), which prohibit the harvest, possession, sale and exchange of great hammerheads (Florida Fish and Wildlife Conservation Commission, 2011).
Our results show that great hammerheads display strong philopatric behavior to overwintering sites in the U.S. and Bahamas. This finding is important as such information can be used to help define great hammerhead essential fish habitat (EFH), defined as, “those waters and substrate necessary to fish for spawning, breeding, feeding, or growth to maturity” (NOAA, 2016 Draft Amendment). Such designations have proven important to minimize, to the extent practical, the adverse effects of fishing and non-fishing activities in EFH for other marine species (NOAA, 2016 Draft Amendment). In addition, great hammerheads' distribution during winter months appeared to be contracted compared to the summer, where they can be found as far north as Virginia and south to the lower Florida Keys. This range contraction as well as expansion, coupled with fidelity to coastal areas during the winter could have important implications for management. Indeed, the results presented here generally support the great hammerhead EFH distribution designated by NOAA recently (NOAA, 2016 Draft Amendment). However, the current EFH map does not include areas north of Florida. Early indications from our telemetry results suggests that coastal regions off South Carolina and Georgia might be important parturition sites during the summer for great hammerheads. Furthermore, if female great hammerheads are returning to their birthplace, like some other species, then this could have implications for management, as female natal philopatry is one of the factors that could reduce gene flow across the Atlantic (Feldheim et al., 2014; Chapman et al., 2015).
Future analyses of great hammerhead captures and distribution of effort in the Directed Shark Bottom Longline Fishery and NMFS fishery independent longline surveys, could be combined with acoustic and satellite tracking data to identify areas and times where the highest number of interactions occur. Gulak et al. (2015) noted that reducing soak times to avoid capture was not economically viable, thus time-area closures were discussed as a possible option to improve management. The above analysis would enable the identification of potential time-area closures that could be most effective on reducing great hammerhead catches, while minimizing lost catches of other species and effort displacement. Further, detailed information on great hammerhead vertical habitat use, including temperature and depth use will be important in revealing interactions with diverse fisheries that use different gear types and deployment depths (Beverly et al., 2009).
Finally, great hammerheads were found to make regular movements across state boundaries, as well as between the U.S. and Bahamas EEZs. These findings further highlight the need for cooperation between jurisdictions to ensure great hammerheads receive necessary protection throughout their migrations. For marine migratory species coordinated actions by many nations, international organizations, multilateral environmental agreements and industry regulators will be required if their populations are to recover to healthy levels and be safeguarded into the future (Lascelles et al., 2014; Allen and Singh, 2016).
Author Contributions
TG: designed study, conducted field work, data analysis, and wrote paper. MV and CB: conducted field work, data analysis, and wrote paper. LH: designed study and data analysis. JF and JB: conducted field work and data analysis. SK and MB: designed study, conducted field work, and wrote paper. WW and RC: conducted field work. LJ and ET: data analysis and wrote paper. RDG and SG: conducted field work and wrote paper.
Conflict of Interest Statement
The authors declare that the research was conducted in the absence of any commercial or financial relationships that could be construed as a potential conflict of interest.
Acknowledgments
This work was supported in part by the Bimini Biological Field Station Foundation (BBFSF) and the Save Our Seas Foundation Keystone Grant, awarded to TG and SG. A special thank-you to the Swiss Shark Foundation (Hai Siftung) and the Guy Harvey Ocean Foundation for many years of funding support. We wish to acknowledge the Commonwealth of The Bahamas, Department of Fisheries for issuing permits that allow us to work in the islands of Bimini. A permit (no: MAF/LIA/22) to conduct scientific marine animal research was supplied by the Department of Marine Resources, Bahamas. Research was conducted in Florida State waters under FWC Special Activity License 16-0397 SRP and in United States Federal waters under NMFS Highly Migratory Species Exempted Fishing Permit SHK-EFP-16-05 and FSU IACUC Protocol 1411. BBFSF also wishes to thank Sundance, TwinVee, Mercury Engines and Davey Marine. A special thank you to Sean Williams, Grant Johnson, Katie Grudecki, Emily Marcus and Jim Barley for support, use of photos and for establishing the great hammerhead site in Bimini. We would also like to acknowledge Bill Parks, Cheryl Caroll, Capt. Mike Newman, Joann and John Fraser, Tony Grogan, Hannah Medd, Jeff Trotta and Scott Briegel for ongoing support in shark captures, laser photogrammetry equipment and receiver maintenance. Further, we are extremely grateful to Neal Watson Jr. for SCUBA equipment sponsorship and continued support in Bimini. Finally, we acknowledge all FACT and ACT members who provided us with hammerhead detections outside of our personal acoustic coverage.
Supplementary Material
The Supplementary Material for this article can be found online at: http://journal.frontiersin.org/article/10.3389/fmars.2017.00003/full#supplementary-material
References
Allen, A. M., and Singh, N. J. (2016). Linking movement ecology with wildlife management and conservation. Front. Ecol. Evol. 3:155. doi: 10.3389/fevo.2015.00155
Armsworth, P. R., Block, B. A., Eagle, J., and Roughgarden, J. E. (2010). The economic efficiency of a time–area closure to protect spawning bluefin tuna. J. Appl. Ecol. 47, 36–46. doi: 10.1111/j.1365-2664.2009.01738.x
Ashe, J. L., Feldheim, K. A., Fields, A. T., Reyier, E. A., Brooks, E. J., O'Connell, M. T., et al. (2015). Local population structure and context-dependent isolation by distance in a large coastal shark. Mar. Ecol. Prog. Ser. 520, 203–216. doi: 10.3354/meps11069
Baker, C. S., Steel, D., Calambokidis, J., Falcone, E., González-Peral, U., Barlow, J., et al. (2013). Strong maternal fidelity and natal philopatry shape genetic structure in North Pacific humpback whales. Mar. Ecol. Prog. Ser. 494, 291–306. doi: 10.3354/meps10508
Banks, K. W., Riegl, B. M., Richards, V. P., Walker, B. E., Helmle, K. P., Jordan, L. K. B., et al. (2008). “The reef tract of continental Southeast Florida (Miami-Dade, Broward, and Palm Beach Counties, USA),” in Coral Reefs of the USA, eds B. Riegl and R.E. Dodge (Dordrecht: Springer-Verlag), 125–172.
Bass, N., Mourier, J., Day, J., Knott, N., Brown, C., and Guttridge, T. L. (2016). Long-term migration patterns and bisexual philopatry in a benthic shark species. Mar. Freshwater Res. doi: 10.1071/MF16122. [Epub ahead of print].
Bestley, S., Jonsen, I. D., Hindell, M. A., Guinet, C., and Charrassin, J. B. (2013). Integrative modelling of animal movement: incorporating in situ habitat and behavioural information for a migratory marine predator. Proc. R. Soc. Lond. B Biol. Sci. 280:20122262. doi: 10.1098/rspb.2012.2262
Beverly, S., Curran, D., Musyl, M., and Molony, B. (2009). Effects of eliminating shallow hooks from tuna longline sets on target and non-target species in the Hawaii-based pelagic tuna fishery. Fish. Res. 96, 281−288. doi: 10.1016/j.fishres.2008.12.010
Blackburn, E., and Cresswell, W. (2016). High winter site fidelity in a long-distance migrant: implications for wintering ecology and survival estimates. J. Ornithol. 157, 93–108. doi: 10.1007/s10336-015-1252-z
Block, B. A., Jonsen, I. D., Jorgensen, S. J., Winship, A. J., Shaffer, S. A., Bograd, S. J., et al. (2011). Tracking apex marine predator movements in a dynamic ocean. Nature 475, 86–90. doi: 10.1038/nature10082
Bond, M. E., Babcock, E. A., Pikitch, E. K., Abercrombie, D. L., Lamb, N. F., and Chapman, D. D. (2012). Reef sharks exhibit site-fidelity and higher relative abundance in marine reserves on the Mesoamerican Barrier Reef. PLoS ONE 7:e32983. doi: 10.1371/journal.pone.0032983
Brooks, E. J., Mandelman, J. W., Sloman, K. A., Liss, S., Danylchuk, A. J., Cooke, S. J., et al. (2012). The physiological response of the Caribbean reef shark (Carcharhinus perezi) to longline capture. Comp. Biochem. Physiol. A Mol. Integr. Physiol. 162, 94–100. doi: 10.1016/j.cbpa.2011.04.012
Bruce, B. D., and Bradford, R. W. (2013). The effects of shark cage-diving operations on the behaviour and movements of white sharks, Carcharodon carcharias, at the Neptune Islands, South Australia. Mar. Biol. 160, 889–907. doi: 10.1007/s00227-012-2142-z
Chapman, B. B., Hulthén, K., Brodersen, J., Nilsson, P. A., Skov, C., Hansson, L. A., et al. (2012). Partial migration in fishes: causes and consequences. J. Fish Biol. 81, 456–478. doi: 10.1111/j.1095-8649.2012.03342.x
Chapman, D. D., Feldheim, K. A., Papastamatiou, Y. P., and Hueter, R. E. (2015). There and back again: a review of residency and return migrations in sharks, with implications for population structure and management. Ann. Rev. Mar. Sci. 7, 547–570. doi: 10.1146/annurev-marine-010814-015730
Chapman, D. D., and Gruber, S. H. (2002). A further observation of the prey-handling behavior of the great hammerhead shark, Sphyrna mokarran: predation upon the spotted eagle ray, Aetobatus narinari. Bull. Mar. Sci. 70, 947–952.
Clark, E., and von Schmidt, K. (1965). Sharks of the central gulf coast of Florida. Bull. Mar. Sci. 15, 13–83.
Cliff, G. (1995). Sharks caught in the protective gill nets off KwaZulu-Natal, South Africa. 8. The great hammerhead shark Sphyrna mokarran (Rüppell). S. Afr. J. Mar. Sci. 15, 105–114. doi: 10.2989/025776195784156331
Compagno, L. J. V. (1984). FAO species catalogue, Vol. 4, Sharks of the world. An annotated and illustrated catalogue of shark species known to date. Part 2. Carcharhiniformes. FAO Fish. Synop. 4(Pt 2), 251–655.
Cooke, S. J., Hinch, S. G., Farrell, A. P., Patterson, D. A., Miller-Saunders, K., Welch, D. W., et al. (2008). Developing a mechanistic understanding of fish migrations by linking telemetry with physiology, behavior, genomics and experimental biology: an interdisciplinary case study on adult Fraser River sockeye salmon. Fisheries 33, 321–339. doi: 10.1577/1548-8446-33.7.321
Costa, D. P., Breed, G. A., and Robinson, P. W. (2012). New insights into pelagic migrations: implications for ecology and conservation. Annu. Rev. Ecol. Evol. Syst. 43, 73–96. doi: 10.1146/annurev-ecolsys-102710-145045
Denham, J., Stevens, J., Simpfendorfer, C. A., Heupel, M. R., Cliff, G., Morgan, A., et al. (2007). Sphyrna Mokarran. The IUCN Red List of threatened species. Version 2014. Available online at: http://www.iucnredlist.org (accessed 20 June 2014).
Dittman, A., and Quinn, T. (1996). Homing in Pacific salmon: mechanisms and ecological basis. J. Exp. Biol. 199, 83–91.
Dodrill, J. W. (1977). A Hook and Line Survey of the Sharks Found within Five Hundred Meters of Shore Along Melbourne Beach, Brevard County, Florida. M.Sc. Thesis, Florida Institute of Technology. 304.
Domeier, M. L. Nasby-Lucas, N. (2007). Annual re-sightings of photographically identified white sharks (Carcharodon carcharias) at an eastern Pacific aggregation site (Guadalupe Island, Mexico). Mar. Biol. 150, 977–984. doi: 10.1007/s00227-006-0380-7
Dulvy, N. K., Fowler, S. L., Musick, J. A., Cavanagh, R. D., Kyne, P. M., Harrison, L. R., et al. (2014). Extinction risk and conservation of the world's sharks and rays. eLife 3:e00590. doi: 10.7554/eLife.00590
Espinoza, M., Heupel, M. R., Tobin, A. J., and Simpfendorfer, C. A. (2016). Evidence of partial migration in a large coastal predator: opportunistic foraging and reproduction as key drivers? PLoS ONE 11:e0147608. doi: 10.1371/journal.pone.0147608
Feldheim, K. A., Gruber, S. H., Dibattista, J. D., Babcock, E. A., Kessel, S. T., Hendry, A. P., et al. (2014). Two decades of genetic profiling yields first evidence of natal philopatry and long-term fidelity to parturition sites in sharks. Mol. Ecol. 23, 110–117. doi: 10.1111/mec.12583
Ferretti, F., Worm, B., Britten, G. L., Heithaus, M. R., and Lotze, H. K. (2010). Patterns and ecosystem consequences of shark declines in the ocean. Ecol. Lett. 13, 1055–1071. doi: 10.1111/j.1461-0248.2010.01489.x
Florida Fish Wildlife Conservation Commission (2011). Commercial Regulations for Shark. Florida Fish and Wildlife Conservation Commission. Available online at: http://myfwc.com/fishing/saltwater/commercial/shark/ (Accessed October 2012).
Graham, F., Rynne, P., Estevanez, M., Luo, J., Ault, J. S., and Hammerschlag, N. (2016). Use of marine protected areas and exclusive economic zones in the subtropical western North Atlantic Ocean by large highly mobile sharks. Divers. Distributions 22, 534–546. doi: 10.1111/ddi.12425
Greenwood, P. J. (1980). Mating systems, philopatry and dispersal in birds and mammals. Anim. Behav. 28, 1140–1162. doi: 10.1016/S0003-3472(80)80103-5
Grubbs, R. D. (2010). “Chapter 7 Ontogenetic shifts in movements and habitat use,” in Sharks and Their Relatives II: Biodiversity, Adaptive Physiology, and Conservation, eds J. F. Carrier, J. A. Musick, and M. R. Heithaus (New York, NY: CRC Press), 319–350.
Grubbs, R. D., and Kraus, R. T. (2010). “Migrations in fishes,” in Encyclopedia of Animal Behavior, Vol. 1, eds M. D. Breed and J. Moore (Oxford: Academic Press), 715–724.
Grubbs, R. D., Musick, J. A., Conrath, C. L., and Romine, J. G. (2007). “Long-term movements, migration, and temporal delineation of summer nurseries for juvenile sandbar sharks in the Chesapeake Bay region,” in Shark nursery grounds of the Gulf of Mexico and the East Coast waters of the United States, Vol. 50, eds C. T. McCandless, N. E. Kohler, and H. L. Pratt, Jr. (Bethesda, MD: American Fisheries Society Symposium), 87–108.
Gulak, S. J. B., de Ron Santiago, A. J., and Carlson, J. K. (2015). Hooking mortality of scalloped hammerhead Sphyrna lewini and great hammerhead Sphyrna mokarran sharks caught on bottom longlines. Afr. J. Mar. Sci. 37, 267–273. doi: 10.2989/1814232X.2015.1026842
Hammerschlag, N., Gallagher, A. J., Lazarre, D. M., and Slonim, C. (2011). Range extension of the Endangered great hammerhead shark Sphyrna mokarran in the Northwest Atlantic: preliminary data and significance for conservation. Endanger. Species Res. 13, 111–116. doi: 10.3354/esr00332
Hammerschlag, N., Gallagher, A. J., Wester, J., Luo, J., and Ault, J. S. (2012). Don't bite the hand that feeds: assessing ecological impacts of provisioning ecotourism on an apex marine predator. Funct. Ecol. 26, 567–576. doi: 10.1111/j.1365-2435.2012.01973.x
Heupel, M. R., Simpfendorfer, C. A., Espinoza, M., Smoothey, A. F., Tobin, A., and Peddemors, V. (2015). Conservation challenges of sharks with continental scale migrations. Front. Mar. Sci. 2:12. doi: 10.3389/fmars.2015.00012
Hillen, J., Kiefer, A., and Veith, M. (2009). Foraging site fidelity shapes the spatial organisation of a population of female western barbastelle bats. Biol. Conserv. 142, 817–823. doi: 10.1016/j.biocon.2008.12.017
Howey-Jordan, L. A., Brooks, E. J., Abercrombie, D. L., Jordan, L. K., Brooks, A., Williams, S., et al. (2013). Complex movements, philopatry and expanded depth range of a severely threatened pelagic shark, the oceanic whitetip (Carcharhinus longimanus) in the Western North Atlantic. PLoS ONE 8:e56588. doi: 10.1371/journal.pone.0056588
Hueter, R. E., Heupel, M. R., Heist, E. J., and Keeney, D. B. (2005). Evidence of philopatry in sharks and implications for the management of shark fisheries. J. Northwest Atl. Fishery Sci. 35, 239–247. doi: 10.2960/j.v35.m493
Hueter, R. E., and Tyminski, J. P. (2007). Species-specific distribution and habitat characteristics of shark nurseries in Gulf of Mexico waters off peninsular Florida and Texas. Am. Fish. Soc. Symp. 50, 193–223.
Hussey, N. E., Kessel, S. T., Aarestrup, K., Cooke, S. J., Cowley, P. D., Fisk, A. T., et al. (2015). Aquatic animal telemetry: a panoramic window into the underwater world. Science 348:1255642. doi: 10.1126/science.1255642
Jennings, D. E., DiBattista, J. D., Stump, K. L., Hussey, N. E., Franks, B. R., Grubbs, R. D., et al. (2012). Assessment of the aquatic biodiversity of a threatened coastal lagoon at Bimini, Bahamas. J. Coast. Conserv. 16, 405–428. doi: 10.1007/s11852-012-0211-6
Jennings, R. D. (1985). Seasonal abundance of hammerhead sharks off Cape Canaveral, Florida. Copeia 1985, 223–225. doi: 10.2307/1444814
Jiao, Y., Cortés, E., Andrews, K., and Guo, F. (2011). Poor-data and data-poor species stock assessment using a Bayesian hierarchical approach. Ecol. Appl. 21, 2691–2708. doi: 10.1890/10-0526.1
Jonsson, B., and Ruud-Hansen, J. (1985). Water temperature as the primary influence on timing of seaward migration of Atlantic salmon (Salmo salar) smolts. Can. J. Fish. Aquat. Sci. 42, 593–595. doi: 10.1139/f85-076
Kajiura, S. M., and Tellman, S. L. (2016). Quantification of massive seasonal aggregations of blacktip sharks (Carcharhinus limbatus) in Southeast Florida. PLoS ONE 11:e0150911. doi: 10.1371/journal.pone.0150911
Kessel, S. T. (2010). Investigation into the Behaviour and Population Dynamics of the Lemon Shark (Negaprion brevirostris). Doctoral dissertation, Cardiff University.
Kessel, S. T., Chapman, D. D., Franks, B. R., Gedamke, T., Gruber, S. H., Newman, J. M., et al. (2014a). Predictable temperature-regulated residency, movement and migration in a large, highly mobile marine predator (Negaprion brevirostris). Mar. Ecol. Prog. Ser. 514, 175–190. doi: 10.3354/meps10966
Kessel, S. T., Cooke, S. J., Heupel, M. R., Hussey, N. E., Simpfendorfer, C. A., Vagle, S., et al. (2014b). A review of detection range testing in aquatic passive acoustic telemetry studies. Rev. Fish Biol. Fish. 24, 199–218. doi: 10.1007/s11160-013-9328-4
Kohler, N. E., Casey, J. G., and Turner, P. A. (1998). NMFS cooperative shark tagging program, 1962-93: an atlas of shark tag and recapture data. Mar. Fish. Rev. 60, 1–87.
Kohler, N. E., and Turner, P.A. (2001). “Shark tagging: a review of conventional methods and studies,” in The Behavior and Sensory Biology of Elasmobranch Fishes: An Anthology in Memory of Donald Richard Nelson, eds T. C. Tricas and S. H. Gruber (Springer Netherlands), 191–224.
Lascelles, B., Notarbartolo Di Sciara, G., Agardy, T., Cuttelod, A., Eckert, S., Glowka, L., et al. (2014). Migratory marine species: their status, threats and conservation management needs. Aquat. Conserv. Mar. Freshwater Ecosyst. 24, 111–127. doi: 10.1002/aqc.2512
Lea, J. S., Wetherbee, B. M., Queiroz, N., Burnie, N., Aming, C., Sousa, L. L., et al. (2015). Repeated, long-distance migrations by a philopatric predator targeting highly contrasting ecosystems. Sci. Rep. 5:11202. doi: 10.1038/srep11202
Locarnini, R. A., Mishonov, A. V., Antonov, J. I., Boyer, T. P., Garcia, H. E., Baranova, O. K., et al. (2013). “World Ocean Atlas 2013,” in Temperature, Vol. 1, eds S. Levitus and A. Mishonov (Silver Spring, MD: NOAA Atlas NESDIS), 73.
Lohmann, K. J., Lohmann, C. M., Brothers, J. R., and Putman, N. F. (2013). Natal homing and imprinting in sea turtles. Biol. Sea Turtles 3, 59–77. doi: 10.1201/b13895-4
Lopes, F., Hoffman, J. I., Valiati, V. H., Bonatto, S. L., Wolf, J. B., Trillmich, F., et al. (2015). Fine-scale matrilineal population structure in the Galapagos fur seal and its implications for conservation management. Conserv. Genet. 16, 1099–1113. doi: 10.1007/s10592-015-0725-1
Lundberg, J., and Moberg, F. (2003). Mobile link organisms and ecosystem functioning: implications for ecosystem resilience and management. Ecosystems 6, 0087–0098. doi: 10.1007/s10021-002-0150-4
Madigan, D. J., Brooks, E. J., Bond, M. E., Gelsleichter, J., Howey, L. A., and Abercrombie, D. L. (2015).. Diet shift and site-fidelity of oceanic whitetip sharks Carcharhinus longimanus along the Great Bahama Bank. Mar. Ecol. Prog. Ser. 529, 185–197. doi: 10.3354/meps11302
Marshall, A. D., and Pierce, S. J. (2012). The use and abuse of photographic identification in sharks and rays. J. Fish Biol. 80, 1361–1379. doi: 10.1111/j.1095-8649.2012.03244.x
McClenachan, L., Cooper, A. B., Carpenter, K. E., and Dulvy, N. K. (2012). Extinction risk and bottlenecks in the conservation of charismatic marine species. Conserv. Lett. 5, 73–80. doi: 10.1111/j.1755-263X.2011.00206.x
Miller, M. H., Carlson, J., Hogan, L., and Kobayashi, D. (2014). Status Review Report: Great Hammerhead Shark (Sphyrna mokarran). Final Report to National Marine Fisheries Service, Office of Protected Resources. Silver Spring (MD). June 2014. 116
Mourier, J., and Planes, S. (2013). Direct genetic evidence for reproductive philopatry and associated fine-scale migrations in female blacktip reef sharks (Carcharhinus melanopterus) in French Polynesia. Mol. Ecol. 22, 201–214. doi: 10.1111/mec.12103
Mourier, J., Planes, S., and Buray, N. (2013). Trophic interactions at the top of the coral reef food chain. Coral Reefs 32, 285. doi: 10.1007/s00338-012-0976-y
Mucientes, G. R., Queiroz, N., Sousa, L. L., Tarroso, P., and Sims, D. W. (2009). Sexual segregation of pelagic sharks and the potential threat from fisheries. Biol. Lett. 5, 156–159. doi: 10.1098/rsbl.2008.0761
NOAA (2016). Amendment 10 to the 2006 Consolidated Atlantic Highly Migratory Species Fishery Management Plan: Essential Fish Habitat. St. Petersburg, FL: National Marine Fisheries Service.
O'Connell, C. P., and Leurs, G. (2016). A minimally invasive technique to assess several life-history characteristics of the endangered great hammerhead shark Sphyrna mokarran. J. Fish Biol. 88, 1257–1264. doi: 10.1111/jfb.12900
Papastamatiou, Y. P., Meyer, C. G., Carvalho, F., Dale, J. J., Hutchinson, M. R., and Holland, K. N. (2013). Telemetry and random-walk models reveal complex patterns of partial migration in a large marine predator. Ecology 94, 2595–2606. doi: 10.1890/12-2014.1
Piercy, A. N., Carlson, J. K., and Passerotti, M. S. (2010). Age and growth of the great hammerhead shark, Sphyrna mokarran, in the north-western Atlantic Ocean and Gulf of Mexico. Mar. Freshwater Res. 61, 992–998. doi: 10.1071/MF09227
Queiroz, N., Humphries, N. E., Mucientes, G., Hammerschlag, N., Lima, F. P., Scales, K. L., et al. (2016). Ocean-wide tracking of pelagic sharks reveals extent of overlap with longline fishing hotspots. Proce. Natl. Acad. Sci. U.S.A. 113, 1582–1587. doi: 10.1073/pnas.1510090113
Reyier, E. A., Franks, B. R., Chapman, D. D., Scheidt, D. M., Stolen, E. D., and Gruber, S. H. (2014). Regional-scale migrations and habitat use of juvenile lemon sharks (Negaprion brevirostris) in the US South Atlantic. PLoS ONE 9:e88470. doi: 10.1371/journal.pone.0088470
Roemer, R. P., Gallagher, A. J., and Hammerschlag, N. (2016). Shallow water tidal flat use and associated specialized foraging behavior of the great hammerhead shark (Sphyrna mokarran). Mar. Freshwater Behav. Physiol. 49, 235–249. doi: 10.1080/10236244.2016.1168089
Roff, G., Doropoulos, C., Rogers, A., Bozec, Y. M., Krueck, N. C., Aurellado, E., et al. (2016). The ecological role of sharks on coral reefs. Trends Ecol. Evol. 31, 395–407. doi: 10.1016/j.tree.2016.02.014
Schlaff, A. M., Heupel, M. R., and Simpfendorfer, C. A. (2014). Influence of environmental factors on shark and ray movement, behaviour and habitat use: a review. Rev. Fish Biol. Fish 24, 1089–1103. doi: 10.1007/s11160-014-9364-8
Schofield, G., Hobson, V. J., Fossette, S., Lilley, M. K. S., Katselidis, K. A., and Hays, G. C. (2010). Biodiversity Research: fidelity to foraging sites, consistency of migration routes and habitat modulation of home range by sea turtles. Diversity and Distributions, 16, 840–853. doi: 10.1111/j.1472-4642.2010.00694.x
Shillinger, G. L., Palacios, D. M., Bailey, H., Bograd, S. J., Swithenbank, A. M., Gaspar, P., et al. (2008). Persistent leatherback turtle migrations present opportunities for conservation. PLoS Biol. 6:e171. doi: 10.1371/journal.pbio.0060171
Sims, D. W., Genner, M. J., Southward, A. J., and Hawkins, S. J. (2001). Timing of squid migration reflects North Atlantic climate variability. Proc. Biol. Sci. 268, 2607–2611. doi: 10.1098/rspb.2001.1847
Stevens, J. D., and Lyle, J. M. (1989). Biology of three hammerhead sharks (Eusphyra blochii, Sphyrna mokarran and S. lewini) from northern Australia. Mar. Freshwater Res. 40, 129–146. doi: 10.1071/MF9890129
Strong, W. R., Snelson, F. F., and Gruber, S. H. (1990). Hammerhead shark predation on stingrays: an observation of prey handling by Sphyrna mokarran. Copeia 1990, 836–840. doi: 10.2307/1446449
Keywords: Sphyrnidae, movement ecology, site fidelity, seasonal residency, migration, aquatic telemetry
Citation: Guttridge TL, Van Zinnicq Bergmann MPM, Bolte C, Howey LA, Finger JS, Kessel ST, Brooks JL, Winram W, Bond ME, Jordan LKB, Cashman RC, Tolentino ER, Grubbs RD and Gruber SH (2017) Philopatry and Regional Connectivity of the Great Hammerhead Shark, Sphyrna mokarran in the U.S. and Bahamas. Front. Mar. Sci. 4:3. doi: 10.3389/fmars.2017.00003
Received: 10 September 2016; Accepted: 05 January 2017;
Published: 20 January 2017.
Edited by:
Michael Paul Jensen, Southwest Fisheries Science Center, USAReviewed by:
Antonios D. Mazaris, Aristotle University of Thessaloniki, GreeceXimena Velez-Zuazo, Smithsonian Institution, USA
Copyright © 2017 Guttridge, Van Zinnicq Bergmann, Bolte, Howey, Finger, Kessel, Brooks, Winram, Bond, Jordan, Cashman, Tolentino, Grubbs and Gruber. This is an open-access article distributed under the terms of the Creative Commons Attribution License (CC BY). The use, distribution or reproduction in other forums is permitted, provided the original author(s) or licensor are credited and that the original publication in this journal is cited, in accordance with accepted academic practice. No use, distribution or reproduction is permitted which does not comply with these terms.
*Correspondence: Tristan L. Guttridge, dHJpc3Rhbi5ndXR0cmlkZ2VAYmltaW5pc2hhcmtsYWIuY29t