Corrigendum: Long-term Shifts in Coral Communities On Shallow to Deep Reef Slopes of Curaçao and Bonaire: Are There Any Winners?
- 1Wageningen University and Marine Research, Den Helder, Netherlands
- 2NIOZ Royal Netherlands Institute for Sea Research and Utrecht University, Texel, Netherlands
- 3Carmabi Foundation, Willemstad, Curaçao
Tropical coral reefs are among the most biologically diverse and economically important ecosystems on earth. Nevertheless, we found dramatic changes in coral communities on the reef slopes of Curaçao and Bonaire since 1973. Cover and abundance declined for virtually all coral species. The data show a shift from communities dominated by framework building species (e.g., Orbicella spp.) to communities consisting of small opportunistic, phenotypically plastic, species, including few remaining structural colonies. Madracis mirabilis, Porites astreoides, Diploria strigosa, and Agaricia lamarcki are at present modest winners in the coral assemblage, although overall cover declined also for these species. Increased frequency and intensity of events inducing coral mortality and ongoing reduction in suitable hard substratum, provided by the remnants of large colony building species, could reduce the chance of these species to remain winners in the longer run. The observed loss in coral cover and the shift from larger structural to smaller opportunistic species reduced reef carbonate production by 67% and therewith, in combination with a trend toward smaller coral colonies, reef complexity. Alarmingly, reefs at upper-mesophotic depths (30–40 m) did not escape the general degradation of the coral community. The negative effects are larger around densely populated areas where local stressors are adding to degradation caused, for instance, by region wide mass bleaching. Without proper conservation and management this already dramatic degradation will continue and turn more and more coral species into losers.
Introduction
Tropical reefs are among the most biologically rich ecosystems on earth providing income for millions of people through fisheries, tourism and coastal protection (Moberg and Rönnbäck, 2003). On tropical reefs scleractinian corals are largely responsible for the architectural complexity due to the deposition of calcium carbonate and thereby provide habitat, food, and refuge for many taxonomic groups. Over the past decades corals reefs have been degrading worldwide at unprecedented rates (Gardner et al., 2003; Bak et al., 2005; Pandolfi and Jackson, 2006). Deteriorating coastal water quality (Rogers, 1990; Williams et al., 2002; Vega-Thurber et al., 2014), overexploitation of herbivores (Jackson et al., 2001) and anthropogenic induced climate change (Glynn, 1996; Aronson et al., 2002a; Wilkinson and Souter, 2008) are generally associated with this ubiquitous decline in coral cover (Jackson et al., 2014). These stressors negatively impact coral resilience, impeding the ability of corals to cope with disease, bleaching or physical disturbance, which in turn affects their competitive position with respect to other reef organisms such as macroalgae or sponges (Vega-Thurber et al., 2014; Zaneveld et al., 2016). Aforementioned developments are suggested to cause shifts in coral communities from assemblages dominated by framework building corals to a dominance of more persistent and opportunistic non-framework building species (e.g., Green et al., 2008; Alvarez-Filip et al., 2011a; Darling et al., 2012; Perry et al., 2015).
Whether coral species are likely to become winners or losers in the changing reef environment depends strongly on their specific life-history traits (Knowlton, 2001; Van Woesik et al., 2011). In an attempt to predict what species are likely to be lost and what species may be more persistent, several studies have divided species over groups based on shared characteristics such as: way of propagation, growth rate and thermo-tolerance (e.g., Edinger and Risk, 2000; Murdoch, 2007). Recently, Darling et al. (2012) divided 147 coral species from tropical reefs all over the world over four main groups: competitive, weedy or ruderal, stress-tolerant and generalist. This grouping was based on 11 functional traits. In the early 1970s coral reefs were still generally dominated by stress-tolerant, competitive and generalist species. It is hypothesized, however, that coral communities are shifting toward species assemblages comprising mainly of phenotypically plastic weedy species and some persistent framework building (stress-tolerant, generalist) species (Darling et al., 2012; Grottoli et al., 2014; McClanahan et al., 2014; Perry et al., 2015). Since the frequency and intensity of events inducing coral mortality (e.g., bleaching and hurricanes) is predicted to increase, recovery and adaptation will become progressively more difficult for all coral species (Hoegh-Guldberg, 1999; Van Woesik et al., 2011; McClanahan et al., 2014). As a consequence, the contribution of corals in benthic reef communities will likely decrease even further and the most stress susceptible coral species may disappear entirely at many reefs (Knowlton, 2001; Hoegh-Guldberg et al., 2007). Predicting trajectories of coral community change, however, is extremely complex due to the numerous factors involved (Hughes and Tanner, 2000; Done et al., 2010). Additionally, shifts in coral communities may occur slowly and are therefore often difficult to distinguish (Pauly, 1995). There appears to be a consistent lack of long-term temporal quantitative data documenting the trajectories of different species in the coral community.
Here, we provide data on the coral community dynamics at reefs on the southern Caribbean islands of Curaçao and Bonaire. Over the course of the studied time series human populations in the Caribbean increased from 27 million in 1973 to approximately 43 million in 2015 (United Nations, Department of Economic and Social Affairs, Population, Division, 2015). As a consequence, the Caribbean basin experiences chronic decline of water quality and overexploitation of fish (Siung-Chang, 1997; Jackson et al., 2014). Due to climate change and increasing sea water temperature, Caribbean corals have been exposed to mass bleaching events in 1998 (Aronson et al., 2002a), 2005 (Wilkinson and Souter, 2008; Eakin et al., 2010) and 2010 (Alemu and Clement, 2014). Furthermore, White Band Disease greatly reduced the cover of Acropora spp. from the late seventies to the mid-eighties, which negatively impacted rates of calcification and architectural complexity of the shallow reef (Gladfelter, 1982; Aronson and Precht, 2001). Additionally, herbivory was greatly reduced due to the mass-mortality of the Diadema antillarum sea-urchin in 1983 (Bak et al., 1984), formerly important in controlling the densities of competitive algae (De Ruyter Van Steveninck and Bak, 1986).
In comparison to other tropical regions (e.g., the Pacific), the Caribbean has relatively few coral species (Veron, 2014). As a consequence, certain ecologically relevant traits of specific species may be lost more rapidly in the Caribbean compared to other regions (Nyström, 2006). In many Caribbean sites the cover of corals providing complex 3D reef structure, mostly Orbicella spp. (= Montastraea, sensu Budd et al., 2012) and Acropora spp. was already strongly reduced over the past decades (Van Duyl, 1985; Aronson and Precht, 2001; Bruckner and Bruckner, 2006). The calcification balance on many of these reefs may currently have shifted toward net erosion which will result in the reduction of architectural complexity (Alvarez-Filip et al., 2009; Kennedy et al., 2013; Perry et al., 2013). This may drastically alter reef functioning and lead to loss of biodiversity as well as a reduction in important ecosystem services for coastal human population such as wave protection and food security (Moberg and Rönnbäck, 2003; Alvarez-Filip et al., 2009, 2011b; Graham and Nash, 2013; Newman et al., 2015).
On degrading Caribbean reefs, phenotypically plastic corals such as Madracis spp., Porites spp., Siderastrea spp. or Agaricia spp., are expected to replace species controlling reef structure like Acropora spp. and Orbicella spp. (Pandolfi and Jackson, 2006) and become dominant in coral communities (Jackson, 2001; Aronson et al., 2002b; Green et al., 2008; Perry et al., 2015). These species are, however, often small and generally not capable of fulfilling the important ecological functions provided by large framework building species (Bellwood et al., 2004; Nyström, 2006; Alvarez-Filip et al., 2013; Perry et al., 2013). Moreover, it may be expected that in the long run opportunistic weedy species (Darling et al., 2012) will also disappear since no coral species appears to be effectively insensitive to anthropogenic impact (McClanahan et al., 2014). We aim, on the basis of images from 18 time series collected at five reef sites in Bonaire and Curaçao, and covering up to 40 years, to (1) determine temporal and spatial changes in dominant coral species, (2) link observed trajectories of the coral community to the life-history strategies of large framework building species and opportunistic weedy species, (3) estimate the relative loss of carbonate production due to changes in coral species composition and cover over time, and finally (4) discriminate between the winners and losers on these Caribbean coral reefs.
Materials and Methods
Site Description and Procedure for Image Collection
The spatio-temporal dynamics of coral reef communities at four southern Caribbean sites were tracked based on photographic records spanning a period of more than 40 years (Bak and Nieuwland, 1995; Bak et al., 2005). The survey sites are located at the leeward side of the islands of Curaçao: Carmabi Buoy One (sites I and II) and Carmabi Buoy Two (site III); and Bonaire: Karpata (site IV) (Figure 1, Table 1). The leeward fringing reef on both islands is characterized by a shallow reef terrace that transitions into a seaward slope with a drop off that varies from less than 20° to vertical (Bak, 1975; Van Duyl, 1985). At each site four permanent quadrats (9 m2) were set out and marked in 1973 on the fore-reef slope at 10, 20, 30, and 40 m depth, one quadrat at each depth. The 10 m quadrats were too deep to cover the Acropora palmata and Acropora cervicornis zones, which are both situated, if present, on the shallow reef terrace at less than 10 m depth (Bak, 1977). Surveys were conducted with variable intervals between 1973 and 2014 and comprised of collecting overview images complemented by a series of detail recordings. In addition to the four main sites a fifth site, Awa Blancu (AB), located at the far south-eastern side of Curaçao, was included with a quadrat positioned at 10 m (since 1983) and 20 m (since 1992) depth. AB is located relatively far upstream of any urban areas and therefore suffers relatively less local anthropogenic disturbance (Gast et al., 1999; Lapointe and Mallin, 2011) (Figure 1). This series was used as a relatively pristine reference to the shallow fore-reef slope (10–20 m) of sites I-IV which lie downstream of densely populated areas and close to outlet bays (Van Duyl, 1985). Per series, a maximum of 9 years was analyzed (Table 1).
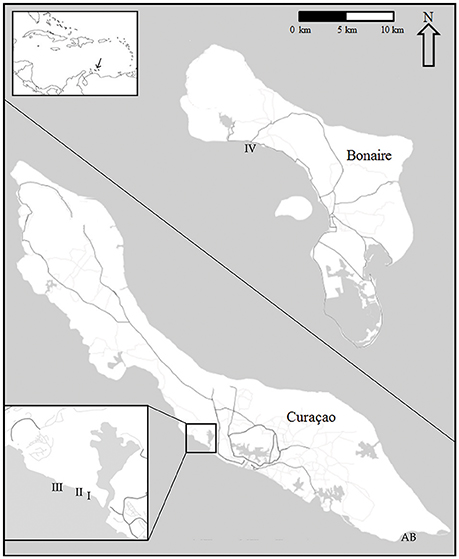
Figure 1. Quadrat locations at Curaçao (I-III, AB) and Bonaire (IV). Including their position in the wider Caribbean sea (top left). Enlarged section at the lower left corner shows in detail the position of sites I-III on Curaçao.
Coral Community Characteristics
All coral colonies that were present in a quadrat were recorded. A coral was considered an individual colony when the tissue was distinctly separated in space from the tissue of any neighboring corals. When a colony was located on the edge of the quadrat only the area within the actual 9 m2 quadrat was included in the survey. Fitted image overlays including all coral colonies were created in Photoshop CC 2015 (Adobe) and Scion Image v4.0.3.2. Actual live surface area (cm2) and colony outline (cm) were extracted from the overlay images and used as general metrics for coral colony size. The ratio between colony outline and live surface area of each colony was used as a measure for colony shape (shape index). Larger round colonies have a relatively low shape index. Partial mortality, particularly in larger colonies (Type II, sensu Meesters et al., 1996), or exposure to competing organisms leads to a relatively larger outline compared to the surface area, resulting in a higher shape index. Colonies were identified to species level, but because the different Orbicella species (O. annularis, O. faveolata, O. franksi) could not be separated reliably in retrospect, they were grouped into the Orbicella complex. For the same reason there was no further distinction made between the species Madracis pharensis, M. formosa, M. senaria, and M. decactis, which were grouped together under the Madracis taxonomic spp. complex. As a base-line, the classification system proposed by Darling et al. (2012) was used to a priori subdivide the coral species. The class “stress-tolerant,” however, proved at times confusing and was replaced by “large framework building.” This class included the entire Orbicella species complex, in contrast to Darling et al. (2012) who categorized O. faveolata and O. franksi as “generalists.” “Weedy” species are here referred to as opportunistic.
Statistical Analyses
The original data consists of a matrix where each row represents a single coral colony in a certain quadrat at a certain year and columns display surface area, outline, shape and specific site characteristics of each colony. This set was used to acquire the total number of colonies in each quadrat. Subsequently, the total planar cover per species was calculated for each quadrat and expressed as a percentage of the total quadrat area (9 m2). Before statistical multivariate analysis the influence of less dominant species was increased by applying a square-root transformation. Non-metric Multi-Dimensional Scaling (nMDS) was used as a tool to display community development over time at each depth averaged over sites I-IV and also for AB at 10 and 20 m. To test for a directional development of the community composition over time a seriation routine (Clarke and Warwick, 1994) was conducted in the multivariate software program Primer-e v7.0.9 (RELATE function with M = 9999 simulations). This procedure allows testing of similarity of a community distance (sub)matrix to a model matrix based on the Spearman rank correlation coefficient (Kendall, 1948; Clarke and Warwick, 1994) and provides a measure (Rho) for linearity in temporal community development. A Rho-value close to 1 is indicative of a linear development over time. Similarly, a second stage routine was applied to submatrices of specific sites per depth to reveal consistencies or variations in temporal patterns of development in community composition among sites (Clarke et al., 2006). A positive correlation in the pattern of development of two time series will result in a high Rho-value. Temporal variation in colony size distributions were determined based on univariate measures of colony size, namely, mean size, skewness and kurtosis (Mardia, 1970), because changes in size frequency can be related to shifts in coral communities (Bak and Meesters, 1998). The difference in mean colony size at sites I-IV over time (of all colonies) was assessed by two-way ANOVA for each depth based on fourth-root transformed data, with year (start and end) and site as categorical variables. Assumptions for ANOVA were confirmed visually (Zuur et al., 2007). Subsequently Post Hoc (Tukey HSD with Bonferroni correction) tests were applied to compare colony size at the start and end in each individual quadrat. Sites were also compared with reference site AB using a different starting year (since 1983 and 1992 for 10 and 20 m resp.). Various diversity indices were computed for each quadrat and year: Shannon-Wiener diversity index (Shannon and Weaver, 1949), Simpson's diversity index (Simpson, 1949), Pielou's evenness (Pielou, 1969) and total number of species. Statistical testing of other community characteristics (e.g., no. of colonies, no. of species, diversity indices, skewness, kurtosis) was inappropriate because this would result in only one replicate value per quadrat. With the exception of the RELATE and Second stage routines all analyses were performed in the R programming environment v3.2.3 (R Core Team, 2014). Within R the following packages were used: vegan (Oksanen et al., 2016) and moments (Komsta and Novomestky, 2015).
Carbonate Production
The relative contribution of the coral community to reef carbonate production in kg CaCO3 m−2 y−1 in a quadrat at each measured point in time was estimated based on total planar cover of each species. Species-specific or nearest equivalent species accretion rates were adapted from the ReefBudget method as proposed by Perry et al. (2012), who acquired the various rates from the available literature. Since our data on coral cover was extracted from 2D-images the obtained estimates will be an underestimation compared to the ReefBudget method where 3D surface is included. Moreover, the ReefBudget method provides calcification rates for shallow reefs between 5 m and 10 m depth. In order to compensate for the decrease in carbonate production with increasing depth a correction was applied to rates of carbonate production in quadrats at greater depth (Equation 1).
Where Gm is the carbonate production at maximum light intensity at the surface, k the extinction coefficient (0.05, Bosscher and Schlager, 1992) and m the depth at which the quadrat is located.
Results
Consistencies and Variability in Trajectories of Community Change
The coral community at the four main sites I-IV has since 1973 developed in a constant uni-directional degrading way (Figure 2, Table 2), which is illustrated in the nMDS plot by the generally linear arrangement of points (years) along the y-axis (Figure 2). This suggests a directional trend in community development gradually moving away from (upwards in Figure 2) the initial community compositions at the start of the time series, irrespective of depth. The first axis indicates a clear effect of depth caused by strong variations in community composition across the depth gradient. The trajectories at greater depths (30 and 40 m) were very similar (Figure 2). At AB, change in community development was less pronounced compared to sites I-IV (Figure 2, Table 2), particularly at 20 m depth. The line of 10 m at the reference site AB was arranged relatively close to the average for sites I-IV at 10 m, suggesting similarity in community composition. At AB 10 m the community in 2014 resembled the community of 1997–2002 at sites I-IV, implying a time lag in reef degradation. A longer time lag was observed for 20 (Figure 2). Also, the community at AB 20 m appears to be more different from sites I-IV (Figure 2). At 20 m (recorded since 1992) the high, albeit not significant, Rho-value (0.926, p = 0.087) indicated linear development in coral community composition, but AB covered a shorter time period and less surveys were included. High Rho-values and non-significant p-values appear to be contradicting, but are likely the result of the low number of time points. In such a case more emphasis may be put on the Rho-value (Clarke and Warwick, 1994), but these values should be interpreted with caution.
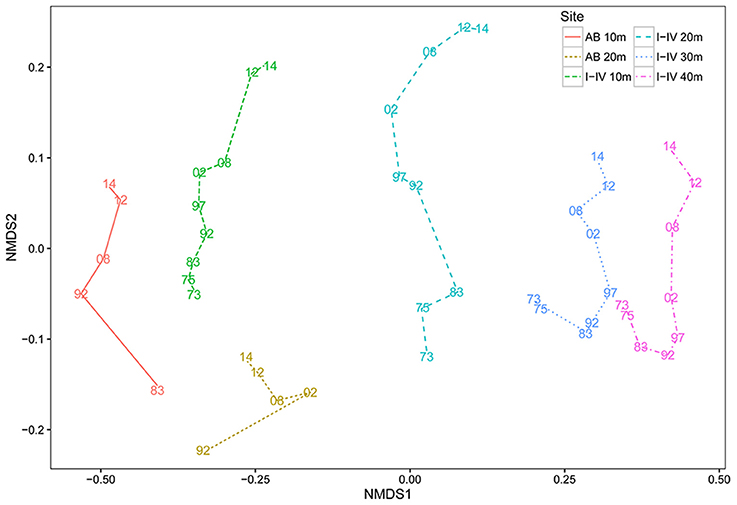
Figure 2. Coral community dynamics on Curaçao and Bonaire. Non-metric Multi-Dimensional Scaling ordinations (nMDS) displaying mean community development over time of sites I-IV, separated by depth (I-IV-10 m, I-IV-20 m, I-IV-30 m, I-IV-40 m) and the reference site Awa Blancu 10 and 20 m (AB-10 m and AB-20 m). Each color-line type combination corresponds to a specific site-depth combination. Lines represent the degree and direction of community change between two surveys. All surveys are labeled as the last two numbers of the year a survey was conducted. The closer the distance between two points, the more similar in composition they are.
Overall, the total area covered by hard corals (Figure 3A) declined on average from 33.8% in 1973 to 10.1% in 2014 over the main sites (I-IV), resp. 95% confidence intervals: 25.7–42.9 and 6.9–14.5. The observed decline was highly comparable among depths but was greatest at 20 m depth, showing a decrease of 78.9% from 43% in 1973 to 9.1% cover in 2014 (Figure 3A). In comparison coral cover at 10, 30, and 40 m depth in 1973 was 42, 33, and 23% and declined by 68.9, 63.5, and 58.6% respectively to 13, 12, and 9.5% cover in 2014. This indicates a pronounced reduction in coral cover along the entire depth gradient studied. In comparison, coral cover at AB declined by 31% at 10 m and 49% at 20 m depth since 1983 and 1992, resp. (Figure 3A, Table 3). The mean percentage of change in cover at these two depths at sites I-IV, over the same time-span as AB, was much larger with 71% (10 m) and 76% (20 m), resp. 95% confidence intervals: 24–96 and 73–78.
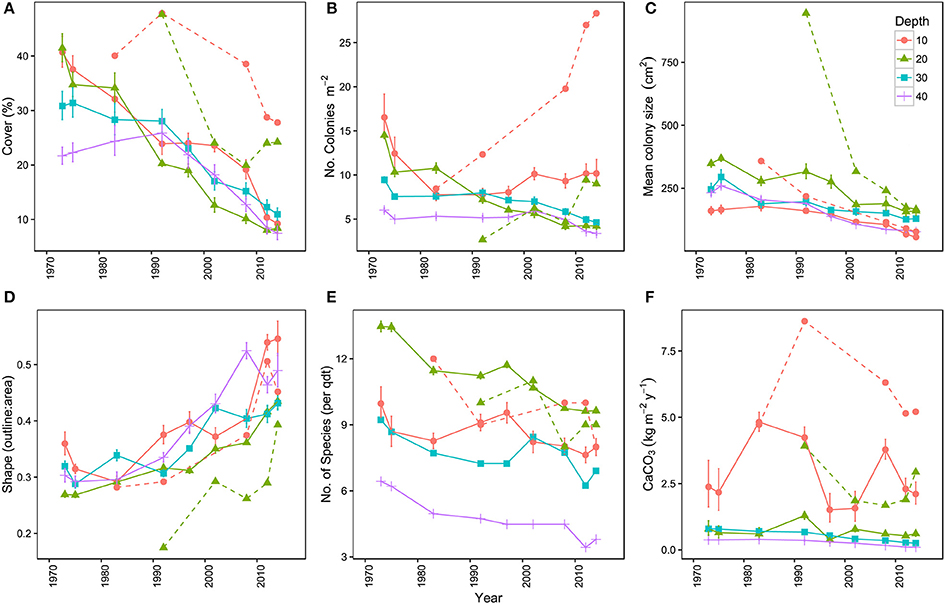
Figure 3. Temporal trends in the coral communities on Curaçao and Bonaire. (A) mean percentage of the 9 m2 quadrat covered by hard coral, (B) mean number of coral colonies per m2, (C) mean colony size (cm2), (D) mean colony shape index represented by the outline:area ratio of corals, (E) mean number of hard coral species in the quadrats and (F) mean carbonate production (kg m−2 y−1) of the coral community. Points represent the mean of the four main locations (I–IV) at a surveyed point in time. Each color represents a specific depth. Dashed lines represent the reference site Awa Blancu (AB) at 10 and 20 m depth. All means are provided with their respective 95% confidence intervals.
Analogous with the decline in coral cover the total number of coral colonies decreased in the majority of the quadrats, particularly between 1973 and 1983 (Figure 3B, Table 3). At 10 m depth the number of colonies initially decreased almost exponentially in the first decade, but subsequently the number stagnated and finally started increasing again after the late 1990s (Figure 3B). This pattern was largely driven by the dynamic M. mirabilis, which has been a dominant species at 10 m depth (Figure 4), but through time proved to be highly variable in the number of colonies and cover. By 2014, M. mirabilis had increased in density at sites III and IV, and also at AB (steep increase in Figure 3B), while an obvious decrease was observed at site I. After 1983 the number of colonies on mesophotic depths (30 and 40 m) remained relatively constant until the late 1990s, after which a gradual decline was observed.
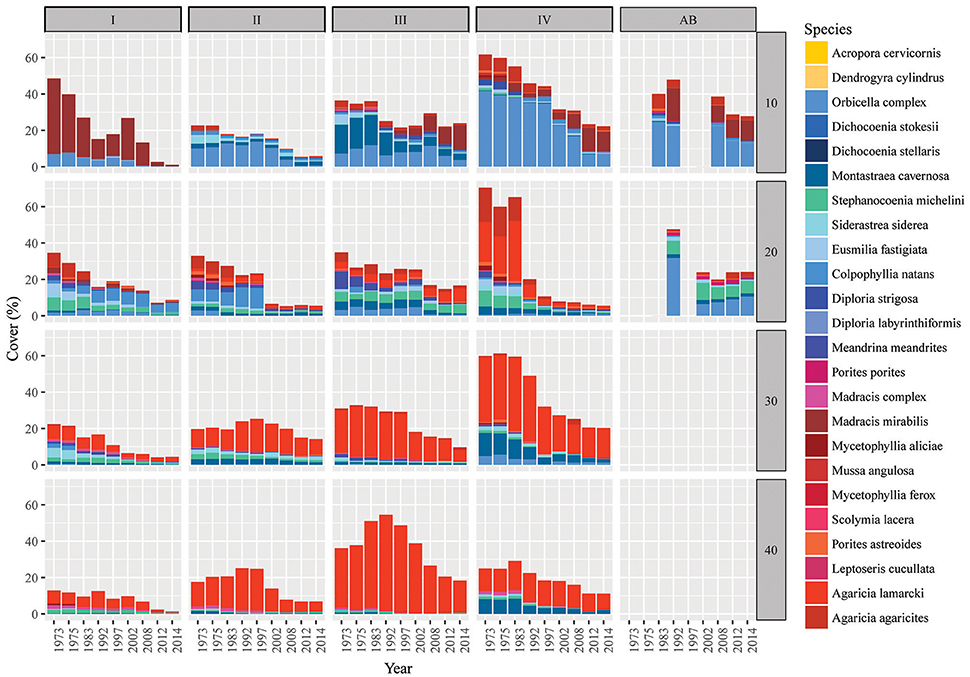
Figure 4. Spatio-temporal dynamics in coral community composition on Curaçao and Bonaire. Bars represent the coral cover at surveyed points in time in the 9 m2 quadrats. Bars are subdivided (stacked) by species. Competitive species are given in shades of yellow (virtually absent), framework building species in blue and opportunistic species in red/purple. Sites are listed as I-IV (main sites) and AB (reference site Awa Blancu) indicated along the top and the depths (10, 20, 30, and 40 m) given on the right side of the panel. Detailed characteristics per quadrat can be found in Tables S2, S3.
In addition to the decline in the number of colonies (Figure 3B), the community at sites I-IV has generally shifted to a relative dominance by smaller colonies in 2014 compared to 1973 (Figure 3C, Table 3). At 10 m, 20 m and 40 m a significant difference was observed between years [F(1) = 78, p < 0.001, F(1) = 22, p < 0.001 and F(1) = 7, p < 0.01, resp.] and sites [F(3) = 97, p < 0.001, F(3) = 17, p < 0.001 and F(3) = 8, p < 0.001, resp.]. At 30 m a significant difference was only found between sites [F(3) = 12, p < 0.001]. A Post Hoc test comparing sites including AB showed significant larger colonies at AB in 1992 at 20 m depth (all p < 0.001), but by 2014 a dramatic decrease resulted in values that are comparable to those at sites I-IV (Figure 3C, Table 3). At 10 m depth, only site I differed significantly from AB in 1983 and 2014 (p < 0.05). Reduced mean colony size over time is in part a consequence of the loss of large colony building species such as Orbicella spp. and a still relatively high number of small colonies of, for instance, M. mirabilis (Figure 5). Both the Orbicella complex (dominant on the shallow fore-reef) and A. lamarcki (dominant on the deep fore-reef) showed a shift toward smaller colonies (Figure 5). This shift is also shown by an increased positive skewness for many quadrats, including AB, in 2014 compared to the beginning of the time series (Table 3). In contrast to the two other dominant species, the opportunistic M. mirabilis does not show a clear shift in colony size, but more a general loss in the number of colonies (Figure 5). Coral colony “shape” (outline:area) appears to represent a shift from larger round colonies to smaller and more complexly shaped colonies (Figure 3D). This is likely a consequence of increased occurrence of partial colony mortality. Partial mortality may result in a relatively large increase in outline compared to a minor decrease in total surface area.
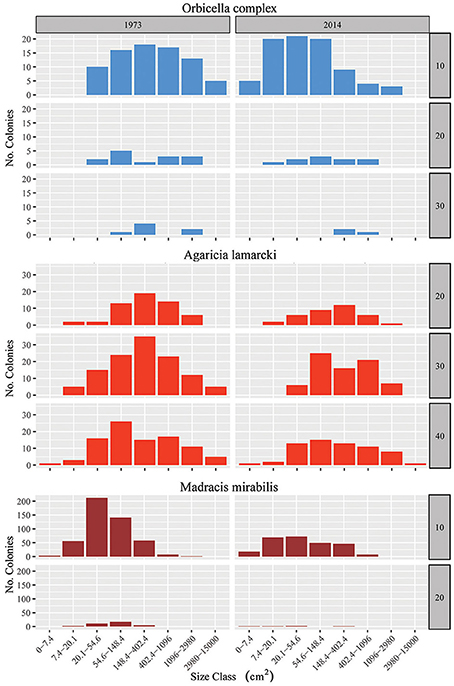
Figure 5. Colony size frequency distribution for the three most prominent species: Orbicella spp., Agaricia lamarcki and Madracis mirabilis. Total number of colonies in 9 m2 quadrats in 1973 (start time series) and 2014 (end) summed over the four main sites (I–IV) on a log scale (8 size classes), at different depths (when the species was present). Scale size varies for the different species. Species color codes correspond to the colors given in Fig. 4. For supporting data, also on the other species, see Figure S1 and Tables S2, S3.
Trends in Species Composition
Over the course of the period surveyed (1973–2014) a total of 30 scleractinian coral species were recorded in the quadrats. After combining the various Madracis and Orbicella species into two groups 24 species/complexes remained (Table 4). The number of species declined in the majority of the quadrats between 1973 and 2014 (Figure 3E, Table 3). In 2014 opportunistic species accounted for 62% of the total coral cover, compared to 53% in 1973 at sites I-IV. At AB, framework building species kept dominating the coral community at both 10 and 20 m, accounting for 52% and 85%, respectively, of the total coral cover in 2014 (Table S3). The cover of competitive species (Acropora spp. and Dendrogyra cylindrus) in the quadrats was negligible. Highest coral diversity was generally found around 20 m depth and lowest at 40 m depth (Table 3, Figure 3E). A relatively low, but significant, correlation (Rho) was found among multiple sites, including AB, at the shallow (10–20 m) fore-reef (Table S1). This observation coincides with a clear variation in species composition and trajectories of coral community development between these sites, particularly regarding the dominance of M. mirabilis, Orbicella spp. or Montastraea cavernosa (Figure 4). In comparison, the species composition at 30 and 40 m depth was highly comparable among sites, with an overall dominance of Agaricia lamarcki (Figure 4), which resulted in strong correlations between all sites (Table S1).
In 1973 the coral community on the shallow fore-reef (10 m and 20 m) at sites I-IV was overall dominated by Orbicella spp. (28%), A. agaricites (20%) and M. mirabilis (17%) (Table S2). By 2014, M. mirabilis had become the dominant species (28%), followed by Orbicella complex (16%) and A. lamarcki (12%). The latter confirms the absence of obvious shifts in species composition but instead a general decline in cover of all species. It does, however, also show the increased relative importance of M. mirabilis with respect to other species at the most shallow quadrats over the more recent years (Figure 4). At 10 m depth evenness increased in most quadrats in 2014 compared to 1973 (Table 3) which is indicative of a more equal contribution of the species present to total coral cover. This is largely a consequence of the decline in cover of the both initially dominant M. mirabilis and Orbicella complex (Figure 3). On the deep fore-reef (30 and 40 m) the coral community was dominated by A. lamarcki throughout the studied period (63% in 1973, 77% in 2014) resulting in generally low evenness scores (Table 3). In 1973, Montastraea cavernosa (13%), Siderastrea siderea (6%), and Stephanocoenia michelini (6%) followed A. lamarcki in cover. These same species were still dominating the community in 2014: M. cavernosa (8%), S. michelini (6%) and S. siderea (3%), but all had declined in total cover.
Some species appear relatively persistent, and include both framework building species (Colpophyllia natans, S. michelini, Meandrina meandrites, Diploria strigosa) and opportunistic species (M. mirabilis, A. lamarcki, Madracis complex, Porites astreoides). These species showed either an increase in cover, a decrease of less than 10% or they had newly emerged in multiple quadrats. An increase in the cover of certain species over time was almost exclusively observed on the shallow reef and no species consistently increased in all quadrats in which it was initially present (Table S2). Species that were dominant in 1973 but declined consistently in the majority of the quadrats included: Orbicella complex, Eusmilia fastigiata, M. cavernosa, Mycetophyllia spp., S. siderea and A. agaricites, M. complex, M. meandrites, S. michelini (Table S2).
Comparable to the main quadrats (I-IV), the Orbicella spp. and M. cavernosa declined strongly at both depths at AB. At 10 m there was a clear increase in cover of M. mirabilis and a less distinct increase in M. meandrites. All other species showed a considerable decline. At 20 m, with the exception of Porites porites, S. siderea and S. michelini, all species declined in cover. A particularly strong decline occurred in the Orbicella complex (71%) and C. natans (100%). Nevertheless, Orbicella spp. still covered 12% of the total quadrat at both depths in 2014.
Temporal Change in Coral Calcium Carbonate Production
The consistent decline in coral cover inherently caused a reduction in the estimated rates of coral carbonate production in 2014 compared to 1973 (Figure 3F). The estimated mean contribution of corals to carbonate production (kg CaCO3 m−2 y−1) over all quadrats declined by 72% from a mean of 2.5 kg m−2 y−1 in 1973 to 0.8 kg m−2 y−1 in in 2014, respective 95% confidence intervals: 0.7–4.3 and 0.02–1.6. The three species that accounted for the highest cover also contributed most prominently to the estimated coral calcification: M. mirabilis, Orbicella complex and A. lamarcki in both 1973 and 2014. In 1973, coral carbonate production was highest at 10 m depth (Figure 3F, Table 3), with a mean of 6.7 kg m−2 y−1, largely due to the high cover of the two rapidly calcifying species M. mirabilis (rate: 29 kg m−2 y−1) and Orbicella spp. (rate: 14 kg m−2 y−1) (Table 3). Particularly the 97% decline in Orbicella complex cover has resulted in a considerable decrease in calcification by 2014. Total coral calcification decreased with depth (Figure 3F) and at different depths a decline occurred over time (Table 3). The reduction in coral cover at 20 m depth at AB resulted in a decline in estimated coral calcification from 3.7 kg m−2 y−1 (1983) to 2.9 kg m−2 y−1 (2014), which is still high compared to sites I-IV (Table 3). Due to the vast increase in M. mirabilis at AB the total estimated coral calcification at 10 m depth had increased here slightly since 1983 (Table 3).
Discussion
Since the early 1970s the coral communities of Curaçao and Bonaire have changed significantly in coral cover and species composition. Total cover has declined and colonies have generally become smaller on both the shallow (10–20 m) and upper-mesophotic reef (30–40 m). In particular, initially dominant framework building species such as Orbicella spp., M. cavernosa and S. siderea, lost significant cover, a trend that occurs throughout the wider Caribbean region (Bruckner and Bruckner, 2006; Edmunds and Elahi, 2007; Jackson et al., 2014). Concurrently, the relative cover of some phenotypically plastic so-called weedy species (sensu Knowlton, 2001) moderately increased since 1973. The observed shift also took place at the reference site Awa Blancu (AB) which is located far upstream and relatively distant from anthropogenic disturbances along the coast of Curaçao (Figure 1), but less distinct.
Overall, reef degradation was less pronounced at Awa Blancu (Figure 2). A noticeable difference with the shallow reef of sites I-IV was that Orbicella, over time, remained the dominant genus at AB with still relatively high cover by 2014 (13% and 11% of the total quadrat at 10 and 20 m respectively). Moreover, the coral communities at AB remained dominated by framework building species throughout the times series. This is in contrast to sites I-IV, where opportunistic species have become dominant. The latter suggests that coral communities along the leeward coast near urban areas and outlet bays (I-IV) receive additional stress on top of stress factors acting on a larger scale (i.e., regional or worldwide), at least down to 20 m depth. Since the start of our time series both Curaçao and Bonaire have experienced significant human population expansion (50% increase on Bonaire since 2001) as well as increased tourism (CBS Curaçao 2015, http://www.cbs.cw; CBS Dutch Caribbean 2015 www.rijksdienstcn.com). Both have consequently led to extensive development of the coastal area. Factors associated with coastal development such as pollution, turbidity and sedimentation negatively impact coral recruitment and survival by smothering corals or reducing the ability of corals to cope with diseases, bleaching or spatial competition (e.g., Meesters et al., 1992; Nugues and Roberts, 2003; Fabricius, 2005; Jackson et al., 2014; Vega-Thurber et al., 2014). Similar to many tropical regions, sewage water on Curaçao and Bonaire is generally insufficiently treated or even largely untreated before it is discharged (Buth and Ras, 1992; Lapointe and Mallin, 2011). Indeed, along the coast of Curaçao clearly higher nutrient concentrations have been found at sites I-III compared to Fuik bay, which is located near Awa Blancu (Gast et al., 1999; Govers et al., 2014). Elevated nutrient levels have also been found at Karpata (IV) on Bonaire during measurements in 2012 and 2013 (Slijkerman et al., 2014). The observed spatial variation in coral community degradation may therefore reflect the impact of persevering stress factors associated with coastal urbanization around more densely populated areas. These results suggest that implementation of thorough local conservation and management strategies, such as the implementation of properly working sewage treatment plants, will benefit local reef health and retard reef degradation. In addition, stronger recreational diving regulation may be useful since approximately 75% of the stay-over tourists on Bonaire are divers (IVM report 2013; http://www.rijksdienstcn.com), undoubtedly having a local impact on the reef (e.g., Hawkins et al., 1999; Lamb et al., 2014). Comparable data are lacking for Curaçao. It is clear that supporting data on specific effects of the various local stressors will be essential for proper implementation of conservation strategies, but at present such data is very limited or even absent.
The observed general decline in cover and abundance of corals not only occurred among reef building species, but included also most opportunistic species (e.g., P. porites, Mycetophyllia spp., A. agaricites and Madracis spp.). A few species, in particular M. mirabilis, A. lamarcki and P. astreoides, however, appear to be more resilient and their decrease in cover and abundance was less distinct (Figure 4, Table S2). This is confirmed by observations throughout the Caribbean (Green et al., 2008; Anderson et al., 2014; Perry et al., 2015). Nevertheless, these species seem to decline when the overall coral cover in the quadrat was substantially reduced. Like many coral species, M. mirabilis can not easily settle on loose substratum such as sand or rubble (Bak and Criens, 1981; Nagelkerken et al., 2000). Presumably, M. mirabilis relies on substratum provided by other species after mortality to settle or expand. Indeed, we found an increase in cover of M. mirabilis in quadrats where large colony building species (e.g., Orbicella complex) used to be dominant but died and left available hard substratum. Contrastingly, in quadrats where larger species were not initially present the cover of M. mirabilis remained low or even declined (e.g., site I, 10 m). This implies that survival of this species is all but certain. According to predictions (e.g., Darling et al., 2012), several larger framework building species such as S. michelini and M. meandrites indeed appear to be relatively persistent (stress-tolerant sensu Darling et al., 2012) and were not readily lost over time. Though their average cover declined in all quadrats, they continued to contribute to total quadrat cover by 2014 (Figure 3). D. strigosa even newly appeared in several shallow quadrats and seems relatively stress-tolerant (Figure 3, Table S2). If, however, the observed declining trend continues, it is likely that even these species will eventually disappear. Also, it remains to be seen whether the apparently opportunistic weedy species will be sufficiently tolerant to cope with the generally expected increase in frequency and severity of catastrophic events (Van Woesik et al., 2011; McClanahan et al., 2014). Historic records (e.g., van Woesik et al., 2012) show that even opportunistic species can become regionally extinct, as a consequence of large-scale climate disturbances. At the present rate of decline (Figure 3A) total coral cover on the reef slopes of Curaçao and Bonaire is expected to have dropped below 1% by 2030.
Evidently, the decline in colony size and shift toward dominance of smaller colonies is largely caused by a decline in abundance of species that potentially form large colonies such as the Orbicella spp. (Figure 5). This was most apparent on AB at 20 m which was originally dominated by many large Orbicella colonies (Table S3). The observed shift in colony size of Orbicella, A. lamarcki and most other species is largely a result of partial mortality of large colonies. Coral growth or tissue regeneration may be impeded by increased spatial competition between corals and other benthic organisms such as algae or benthic cyanobacterial mats (Hughes, 1994). Meesters et al. (1997a) describe two types of lesion in coral tissue: the so-called type I, where the lesion is completely enclosed by tissue; and type II, most frequently observed, where the lesion is located at the edge of the colony. The observed increase in the ratio of colony outline and live surface area of coral colonies (Table 3) could be an indication of the incapability of the coral to reclaim substratum after such a type II lesion (Meesters et al., 1997b). Partial mortality will generally result in a larger loss of surface area than colony outline, thus leading to a higher shape-ratio. A possible effect on colony size distribution caused by increased recruitment can be neglected since no new recruits were present in 2014. M. mirabilis may at present be the sole species to show recruitment within the quadrats. Fragmentation, however, makes it difficult to distinguish actual new M. mirabilis recruits. Nevertheless, the relative increase in abundance of opportunistic species in the past decade has contributed to a higher density of small colonies (Knowlton, 2001; Darling et al., 2012).
An important consequence of reduced coral cover is the loss of reef structural complexity and associated loss of biodiversity, but also coastal protection and human food security (e.g., Alvarez-Filip et al., 2009; Newman et al., 2015). In many of the studied quadrats, coral cover has approached or dropped below the 10% level. This point has been suggested as the threshold after which carbonate accretion will be surpassed by erosion and architectural complexity is being lost (Perry et al., 2013). Following this rationale, nine out of the 16 main quadrats would currently be in an eroding state (Table 3). Indeed, the estimated carbonate production has decreased considerably since 1973 in virtually all quadrats, largely attributed to the general reduction of coral cover and in particular the loss of large calcifying species such as Orbicella spp. (Perry et al., 2015). The relative decline in carbonate production was highly comparable across depths, with the exception of 10 m. At 10 m depth the degree by which carbonate production changed over time was generally lower and largely driven by the temporal dynamics in cover of the rapidly calcifying M. mirabilis. High M. mirabilis cover may compensate some of the reduction in production but it cannot be considered an important framework builder able to compensate for the loss of structural complexity provided by large calcifying species (such as Orbicella spp. and Acropora spp.) (Alvarez-Filip et al., 2011a). Moreover, opportunistic rapidly calcifying species such as M. mirabilis are typically absent at greater depths.
Diversity indices may reflect a gradient of decreased disturbance with greater depth. According to the intermediate disturbance theory highest community diversity is found at intermediate disturbance (Connell, 1978). Over the depth range studied, highest diversity occurred at 20 m depth both in 1973 and 2014, which could be indicative of intermediate disturbance level. At 10 m depth, high disturbance likely impacted all but the most resistant species such as M. mirabilis or P. astreoides, resulting in a low diversity. Both natural (e.g., hurricanes) and anthropogenic disturbances (e.g., eutrophication and run-off) generally have the highest impact on the more shallow reef. Particularly the anthropogenic stress has intensified over the past decades likely putting more stress on the most shallow reef zones (<20 m). The generally low diversity of coral communities at greater depth (>20 m), in particular at 40 m, might indicate that these reefs experience lower levels of disturbance (e.g., declined water quality, reduced herbivory and hurricanes). Following the intermediate disturbance theory, reefs with low disturbance are characterized by dominant competitors (Connell, 1978). Indeed, the deeper reefs of Curaçao and Bonaire were dominated by A. lamarcki consistently between 1973 and 2014. In addition, low light intensities at greater depth likely support a generally lower diversity of species. Although, the reefs at greater depths may initially have experienced less disturbance (Bak et al., 2005) an obvious degrading trend was nevertheless observed, in particular since approximately 1990. A decline in cover and abundance of coral, including A. lamarcki may suggest that stress on these reefs has recently been increasing. Lapointe and Mallin (2011), for instance, found evidence for land-based sewage inputs reaching the deeper reef (18 m) near urban areas on Curaçao. Nutrient measurements on reefs below 18 m are virtually absent, but eutrophication may be reaching greater depths as well. The observed deep community dynamics of the recent years may, however, not be linked (anymore) to the absence of disturbance, but simply be the outcome of one strong competitor (A. lamarcki) and a generally limited pool of species at greater depths. The absence of weedy species such as M. mirabilis here may impede a shift to a more opportunistic coral community as seen on the shallow reef. Such species are simply unable to settle at these depths due to factors such as low light intensity. As yet no new opportunistic candidate has settled and with A. lamarcki, still dominant though steadily declining as well, the prospect for recovery of mesophotic coral communities on reefs appears to be very limited.
In conclusion, we found that coral communities of Curaçao and Bonaire have seen dramatic changes since 1973. Overall coral communities were heavily degraded by 2014. Phenotypically plastic and opportunistic species have increased and M. mirabilis, A. lamarcki, P. astreoides and D. strigosa may at present be considered very modest winners among the Caribbean coral assembly. These species, however, are unlikely to remain winners in a compromised habitat where suitable substratum for settlement is lost and disruptive events may become more frequent and severe, nor will they be able to provide the same structural complexity as the species from the near past (e.g. Orbicella spp.). The reefs at mesophotic depths (30–40 m) do not escape the general degradation of the coral community. Degradation was less evident away from urban areas. This suggests that local management, such as the provision of proper sewage treatment and run-off prevention, can have a positive effect on the general health and survival of coral reef communities. Although, the upsurge of some opportunistic species may provide a glimmer of hope for coral communities proper conservation and management will be essential to keep coral species from turning collectively into losers.
Author Contributions
Data collection: RB, EM, and GN; Data analysis: DD, EM, RB, and GN; Interpretation of the data: DD, EM, FV, and RB; Drafting work: DD; Critical revision: EM, FV, RB, and GN; Final approval: EM and FV; Agreement to be accountable for all aspects of the work: DD, EM, FV, RB, and GN.
Conflict of Interest Statement
The authors declare that the research was conducted in the absence of any commercial or financial relationships that could be construed as a potential conflict of interest.
Acknowledgments
We thank Alice Webb from The Royal Netherlands Institute for Sea Research (NIOZ) for assistance with data organization and analyses. Furthermore, we are thankful for continuous the support received by the CARMABI research institute on Curaçao and STINAPA on Bonaire. The present research project was conducted by Wageningen University and Marine Research, formerly IMARES-WUR, at the request of and with funding from the Ministry of Economic Affairs for the purposes of Policy Support Research Theme “Analyse fotomateriaal koraalrif/fase 1” (project no. BO-11-019.02-038). The Royal Netherlands Institute for Sea Research (NIOZ) provided funding of exploitation costs and in kind support. Finally, we would like to extend our gratitude to both reviewers for their constructive review of the manuscript.
Supplementary Material
The Supplementary Material for this article can be found online at: http://journal.frontiersin.org/article/10.3389/fmars.2016.00247/full#supplementary-material
References
Alemu, I. J. B., and Clement, Y. (2014). Mass coral bleaching in 2010 in the southern Caribbean. PLoS ONE 9:e83829. doi: 10.1371/journal.pone.0083829
Alvarez-Filip, L., Carricart-Ganivet, J. P., Horta-Puga, G., and Iglesias-Prieto, R. (2013). Shifts in coral-assemblage composition do not ensure persistence of reef functionality. Sci. Rep. 3:3486. doi: 10.1038/srep03486
Alvarez-Filip, L., Dulvy, N. K., Côté, I. M., Watkinson, A. R., and Gill, J. A. (2011a). Coral identity underpins architectural complexity on Caribbean reefs. Ecol. Appl. 21, 2223–2231. doi: 10.1890/10-1563.1
Alvarez-Filip, L., Dulvy, N. K., Gill, J. A., Côté, I. M., and Watkinson, A. R. (2009). Flattening of Caribbean coral reefs: region-wide declines in architectural complexity. Proc. R. Soc. B. 276, 3019–3025. doi: 10.1098/rspb.2009.0339
Alvarez-Filip, L., Gill, J. A., Dulvy, N. K., Perry, A. L., Watkinson, A. R., and Côté, I. M. (2011b). Drivers of region-wide declines in architectural complexity on Caribbean reefs. Coral Reefs 30, 1051–1060. doi: 10.1007/s00338-011-0795-6
Anderson, R., Morrall, C., Jossart, J., Nimrod, S., Bolda, E., Musser, K., et al. (2014). Marine Protected Area monitoring in the nearshore waters of Grenada, Eastern Caribbean: benthic cover and fish populations. Rev. Biol. Trop. 62, 273–286. doi: 10.15517/rbt.v62i0.15922
Aronson, R. B., Macintyre, I. G., Precht, W. F., Murdoch, T. J. T., and Wapnick, C. M. (2002b). The expanding scale of species turnover events on coral reefs in Belize. Ecol. Monogr. 72, 233–249. doi: 10.1890/0012-9615(2002)072[0233:TESOST]2.0.CO;2
Aronson, R. B., and Precht, W. F. (2001). White-band disease and the changing face of Caribbean coral reefs. Hydrobiologia 460, 25–38. doi: 10.1023/A:1013103928980
Aronson, R. B., Precht, W. F., Toscano, M., and Koltes, K. H. (2002a). The 1998 bleaching event and its aftermath on a coral reef in Belize. Mar. Biol. 141, 435–447. doi: 10.1007/s00227-002-0842-5
Bak, R. P. M. (1975). Ecological aspects of the distribution of reef corals in the Netherlands Antilles. Bijdr. Dierkd. 45, 181–190
Bak, R. P. M. (1977). Coral reefs and their zonation in Netherlands Antilles: modern and ancient reefs. AAPG Stud. Geol. 4, 3–16
Bak, R. P. M., Carpay, M. J. E., and De Ruyter Van Steveninck, E. D. (1984). Densities of the sea urchin Diadema antillarum before and after mass mortalities on the coral reefs on Curaçao. Mar. Ecol. Prog. Ser. 17, 105–108. doi: 10.3354/meps017105
Bak, R. P. M., and Criens, S. R. (1981). “Survival after fragmentation of colonies of Madracis mirabilis, Acropora palmate, and A. cervicornis (Scleractinia) and the subsequent impact of a coral disease,” in Proceedings of the 4th International Coral Reef Symposium, Vol. 2 (Manila), 221–227.
Bak, R. P. M., and Meesters, E. H. W. G. (1998). Coral population structure: the hidden information of colony size-frequency distributions. Mar. Ecol. Prog. Ser. 162, 301–306. doi: 10.3354/meps162301
Bak, R. P. M., and Nieuwland, G. (1995). Long-term change in coral communities along depth gradients over leeward reefs in the Netherlands Antilles. Bull. Mar. Sci. 56, 609–619
Bak, R. P. M., Nieuwland, G., and Meesters, E. H. W. G. (2005). Coral reef crisis in deep and shallow reefs: 30 years of constancy and change in reefs of Curaçao and Bonaire. Coral Reefs 24, 475–479. doi: 10.1007/s00338-005-0009-1
Bellwood, D. R., Hughes, T. P., Folke, C., and Nyström, M. (2004). Confronting the coral reef crisis. Nature 429, 827–833. doi: 10.1038/nature02691
Bosscher, H., and Schlager, W. (1992). Computer simulation of reef growth. Sedimentology 39, 503–512. doi: 10.1111/j.1365-3091.1992.tb02130.x
Bruckner, A. W., and Bruckner, R. J. (2006). The recent decline of Montastraea annularis (complex) coral populations in western Curaçao: a cause for concern? Rev. Biol. Trop. 54, 45–58
Budd, A. F., Fukami, H., Smith, N. D., and Knowlton, N. (2012). Taxonomic classification of the reef coral family Mussidae (Cnidaria: Anthozoa: Scleractinia). Zool. J. Linnean Soc. 166, 465–529. doi: 10.1111/j.1096-3642.2012.00855.x
Buth, L., and Ras, J. (1992). Inventory of the Land-based Sources of Marine Pollution Netherlands Antilles. Department of Public Works of Curaçao and Environmental Service Curaçao; prepared for CEPPOL Program, IOC-UNEP CAR/RCU. 29
Clarke, K. R., and Warwick, R. M. (1994). An Approach to Statistical Analysis and Interpretation, 2nd Edn. Plymouth: PRIMER-E.
Clarke, R. K., Somerfield, P. J., Airoldi, L., and Warwick, R. M. (2006). Exploring interactions by second-stage community analyses. J. Exp. Mar. Biol. Ecol. 338, 179–192. doi: 10.1016/j.jembe.2006.06.019
Connell, J. H. (1978). Diversity in tropical rain forests and coral reefs. Science 199, 1302–1310. doi: 10.1126/science.199.4335.1302
Darling, E. S., Alvarez-Filip, L., Oliver, T. A., McClanahan, T. R., Côté, I. M., and Bellwood, D. (2012). Evaluating life-history strategies of reef corals from species traits. Ecol. Lett. 15:1378–1386. doi: 10.1111/j.1461-0248.2012.01861.x
De Ruyter Van Steveninck, E. D. D., and Bak, R. P. M. (1986). Changes in abundance of coral-reef bottom components related to mass mortality of the sea-urchin Diadema antillarum. Mar. Ecol. Prog. Ser. 34, 87–94. doi: 10.3354/meps034087
Done, T. J., DeVantier, L. M., Turak, E., Fisk, D. A., Wakeford, M., and Van Woesik, R. (2010). Coral growth on three reefs: development of recovery benchmarks using a space for time approach. Coral Reefs 29, 815–833. doi: 10.1007/s00338-010-0637-y
Eakin, C. M., Morgan, J. A., Heron, S. F., Smith, T. B., Liu, G., Alvarez-Filip, L., et al. (2010). Caribbean corals in crisis: record thermal stress, bleaching, and mortality in 2005. PLoS ONE 5:e13969. doi: 10.1371/journal.pone.0013969
Edinger, E. N., and Risk, M. J. (2000). Reef classification by coral morphology predicts coral reef conservation value. Biol. Conserv. 92, 1–13. doi: 10.1016/S0006-3207(99)00067-1
Edmunds, P. J., and Elahi, R. (2007). The demographics of a 15-year decline in cover of the Caribbean reef coral Montastraea annularis. Ecol. Monogr. 77, 3–18. doi: 10.1890/05-1081
Fabricius, K. E. (2005). Effects of terrestrial runoff on the ecology of corals and coral reefs: review and synthesis. Mar. Pollut. Bull. 50, 125–146. doi: 10.1016/j.marpolbul.2004.11.028
Gardner, T. A., Côté, I. M., Gill, J. A., Grant, A., and Watkinson, A. R. (2003). Long-term region-wide declines in Caribbean corals. Science 301, 958–960. doi: 10.1126/science.1086050
Gast, G. J., Jonkers, P. J., Van Duyl, F. C., and Bak, R. P. M. (1999). Bacteria, flagellates and nutrients in island fringing coral reef waters: influence of the ocean, the reef and eutrophication. Bull. Mar. Sci. 65, 523–538.
Gladfelter, W. B. (1982). White-band disease in Acropora palmata: implications for the structure and growth of shallow reefs. Bull. Mar. Sci. 32, 639–643.
Glynn, P. W. (1996). Coral reef bleaching: facts, hypotheses and implications. Glob. Chang. Biol. 2, 495–509. doi: 10.1111/j.1365-2486.1996.tb00063.x
Govers, L. L., Lamers, L. P. M., Bouma, T. J., de Brouwer, J. H. F., and van Katwijk, M. M. (2014). Eutrophication threatens Caribbean seagrasses–An example from Curaçao and Bonaire. Mar. Pollut. Bull. 89, 481–486. doi: 10.1016/j.marpolbul.2014.09.003
Graham, N. A. J., and Nash, K. L. (2013). The importance of structural complexity in coral reef ecosystems. Coral Reefs 32, 315–326. doi: 10.1007/s00338-012-0984-y
Green, D. H., Edmunds, P. J., and Carpenter, R. C. (2008). Increasing relative abundance of Porites astreoides on Caribbean reefs mediated by an overall decline in coral cover. Mar. Ecol. Prog. Ser. 359, 1–10. doi: 10.3354/meps07454
Grottoli, A. G., Warner, M. E., Levas, S. J., Aschaffenburg, M. D., Schoepf, V., McGinley, M., et al. (2014). The cumulative impact of annual coral bleaching can turn some coral species winners into losers. Glob. Chang. Biol. 20, 3823–3833. doi: 10.1111/gcb.12658
Hawkins, J. P., Roberts, C. M., Van'T Hof, T., De Meyer, K., Tratalos, J., and Aldam, C. (1999). Effects of recreational scuba diving on Caribbean coral and fish communities. Conserv. Biol. 13, 888–897. doi: 10.1046/j.1523-1739.1999.97447.x
Hoegh-Guldberg, O. (1999). Climate change, coral bleaching and the future of the world's coral reefs. Mar. Freshwater Res. 50, 839–866. doi: 10.1071/MF99078
Hoegh-Guldberg, O., Mumby, P. J., Hooten, A. J., Steneck, R. S., Greenfield, P., Gomez, E., et al. (2007). Coral reefs under rapid climate change and ocean acidification. Science 318, 1737–1742. doi: 10.1126/science.1152509
Hughes, T. P. (1994). Catastrophes, phase shifts, and large-scale degradation of a Caribbean coral reef. Science 265, 1547–1551. doi: 10.1126/science.265.5178.1547
Hughes, T. P., and Tanner, J. E. (2000). Recruitment failure, life histories, and long-term decline of Caribbean corals. Ecology 81, 2250–2263. doi: 10.1890/0012-9658(2000)081[2250:RFLHAL]2.0.CO;2
Jackson, J. B. C. (2001). What was natural in the coastal oceans? Proc. R. Soc. B. 98, 5411–5418. doi: 10.1073/pnas.091092898
Jackson, J. B. C., Donovan, M., Cramer, K., and Lam, V. (eds.). (2014). Status and Trends of Caribbean Coral Reefs 1970–2012. Washington, DC: Global Coral Reef Monitoring Network, International Union for the Conservation of Nature Global Marine and Polar Program.
Jackson, J. B. C., Kirby, M. X., Berger, W. H., Bjorndal, K. A., Botsford, L. W., Bourque, B. J., et al. (2001). Historical overfishing and the recent collapse of coastal ecosystems. Science 293, 629–638. doi: 10.1126/science.1059199
Kennedy, E. V., Perry, C. T., Halloran, P. R., Iglesias-Prieto, R., Schönberg, C. H. L., Wisshak, M., et al. (2013). Avoiding coral reef functional collapse requires local and global action. Curr. Biol. 23, 912–918. doi: 10.1016/j.cub.2013.04.020
Knowlton, N. (2001). The future of coral reefs. Proc. Natl. Acad. Sci. U.S.A. 98, 5419–5425. doi: 10.1073/pnas.091092998
Komsta, L., and Novomestky, F. (2015). Moments, Cumulants, Skewness, Kurtosis and Related Tests. R package Version 0.14.
Lamb, J. B., True, J. D., Piromvaragorn, S., and Willis, B. L. (2014). Scuba diving damage and intensity of tourist activities increases coral disease prevalence. Biol. Conserv. 178, 88–96. doi: 10.1016/j.biocon.2014.06.027
Lapointe, B. E., and Mallin, M. A. (2011). Nutrient Enrichment and Eutrophication on Fringing Coral Reefs of Bonaire and Curaçao, Netherlands Antilles. Report to the United Nations Environment Programme for the NACRI Coral Reef Monitoring Program, Harbor Branch Oceanographic Institute, Ft Pierce Fl, 42.
Mardia, K. V. (1970). Measures of multivariate skewness and kurtosis with applications. Biometrika 57, 519–530. doi: 10.1093/biomet/57.3.519
McClanahan, T. R., Graham, N. A. J., and Darling, E. S. (2014). Coral reefs in a crystal ball: predicting the future from the vulnerability of corals and reef fishes to multiple stressors. Curr. Opin. Env. Sust. 7, 59–64. doi: 10.1016/j.cosust.2013.11.028
Meesters, E. H. W. G., Bos, A., and Gast, G. J. (1992). “Effects of sedimentation and lesion position on coral tissue regeneration,” Proceedings of the 7th International Coral Reef Symposium, 2, 681–688.
Meesters, E. H. W. G., Pauchli, W., and Bak, R. P. M. (1997b). Predicting regeneration of physical damage on a reef-building coral by regeneration capacity and lesion shape. Mar. Ecol. Prog. Ser. 146, 91–99. doi: 10.3354/meps146091
Meesters, E. H. W. G., Wesseling, I., and Bak, R. P. M. (1996). Partial mortality in three species of reef-building corals and the relation with colony morphology. Bull. Mar. Sci. 58, 838–852.
Meesters, E. H. W. G., Wesseling, I., and Bak, R. P. M. (1997a). Coral colony tissue damage in six species of reef-building corals: partial mortality in relation with depth and surface area. Neth. J. Sea Res. 37, 131–144. doi: 10.1016/S1385-1101(96)00004-4
Moberg, F., and Rönnbäck, P. (2003). Ecosystem services of the tropical seascape: interactions, substitutions and restoration. Ocean Coast. Manage. 46, 27–46. doi: 10.1016/S0964-5691(02)00119-9
Murdoch, T. J. T. (2007). A Functional Group Approach for Predicting the Composition of Hard Coral Assemblages in Florida and Bermuda. Ph.D. dissertation, Department of Marine Science, University of South Alabama.
Nagelkerken, I., Bouma, S., Van Den Akker, S., and Bak, R. P. M. (2000). Growth and survival of unattached Madracis mirabilis fragments transplanted to different reef sites, and the implication for reef rehabilitation. Bull Mar Sci 66, 497–505.
Newman, S. P., Meesters, E. H. W. G., Dryden, C. S., Williams, S. M., Sanchez, C., Mumby, P. J., et al. (2015). Reef flattening effects on total richness and species responses in the Caribbean. J. Anim. Ecol. 84, 1678–1689. doi: 10.1111/1365-2656.12429
Nugues, M. M., and Roberts, C. M. (2003). Partial mortality in massive reef corals as an indicator of sediment stress on coral reefs. Mar. Pollut. Bull. 46, 314–323. doi: 10.1016/S0025-326X(02)00402-2
Nyström, M. (2006). Redundancy and response diversity of functional groups: implications for the resilience of coral reefs. Ambio 35, 30–35. doi: 10.1579/0044-7447-35.1.30
Oksanen, J., Blanchet, F. G., Kindt, R., Legendre, P., Minchin, P. R., O'Hara, R. B., et al. (2016). Vegan: Community Ecology Package. R Package Version 2.3–5.
Pandolfi, J. M., and Jackson, J. B. C. (2006). Ecological persistence interrupted in Caribbean coral reefs. Ecol. Lett. 9, 818–826. doi: 10.1111/j.1461-0248.2006.00933.x
Pauly, D. (1995). Anecdotes and the shifting baseline syndrome of fisheries. Trends Ecol. Evol. (Amst). 10, 430. doi: 10.1016/S0169-5347(00)89171-5
Perry, C., Edinger, E., Kench, P., Murphy, G., Smithers, S., Steneck, R., et al. (2012). Estimating rates of biologically driven coral reef framework production and erosion: a new census-based carbonate budget methodology and applications to the reefs of Bonaire. Coral Reefs 31, 853–886. doi: 10.1007/s00338-012-0901-4
Perry, C. T., Murphy, G. N., Kench, P. S., Smithers, S. G., Edinger, E. N., Steneck, R. S., et al. (2013). Caribbean-wide decline in carbonate production threatens coral reef growth. Nat. Commun. 4, 1402. doi: 10.1038/ncomms2409
Perry, C. T., Steneck, R. S., Murphy, G. N., Kench, P. S., Edinger, E. N., Smithers, S. G., et al. (2015). Regional–scale dominance of non–framework building corals on Caribbean reefs affects carbonate production and future reef growth. Glob. Chang. Biol. 21, 1153–1164. doi: 10.1111/gcb.12792
Rogers, C. S. (1990). Responses of coral reefs and reef organisms to sedimentation. Mar. Ecol. Prog. Ser. 62, 185–202. doi: 10.3354/meps062185
Shannon, C. E., and Weaver, W. (1949). The Mathematical Theory of Communication. Urbana, IL: University of Illinois Press.
Siung-Chang, A. (1997). A review of marine pollution issues in the Caribbean. Environ. Geochem. Health 19, 45–55. doi: 10.1023/A:1018438119034
Slijkerman, D. M. E., de León, R., and de Vries, P. (2014). A baseline water quality assessment of the coastal reefs of Bonaire, Southern Caribbean. Mar. Pollut. Bull. 86, 523–529. doi: 10.1016/j.marpolbul.2014.06.054
United Nations, Department of Economic Social Affairs, Population, Division. (2015). World Population Prospects: The 2015 Revision, Key Findings and Advance Tables. ESA/P/WP.241
Van Duyl, F. C. (1985). Atlas of the Living Reefs of Curaçao and Bonaire (Netherlands Antilles). Utrecht: Foundation for Scientific Research in Surinam and the Netherlands Antilles.
van Woesik, R., Franklin, E. C., O'Leary, J., McClanahan, T. R., Klaus, J. S., and Budd, A. F. (2012). Hosts of the Plio-Pleistocene past reflect modern-day coral vulnerability. Proc. R. Soc. B. 279, 2448–2456. doi: 10.1098/rspb.2011.2621
Van Woesik, R., Sakai, K., Ganase, A., and Loya, Y. (2011). Revisiting the winners and the losers a decade after coral bleaching. Mar. Ecol. Prog. Ser. 434, 67–76. doi: 10.3354/meps09203
Vega-Thurber, R. L., Burkepile, D. E., Fuchs, C., Shantz, A. A., McMinds, R., and Zaneveld, J. R. (2014). Chronic nutrient enrichment increases prevalence and severity of coral disease and bleaching. Glob. Chang. Biol. 20, 544–554. doi: 10.1111/gcb.12450
Veron, J. E. N. (2014). Results of an update of the Corals of the World Information Base for the Listing Determination of 66 Coral Species under the Endangered Species Act. Report to the Western Pacific Regional Fishery Management Council, Honolulu.
Wilkinson, C. R., and Souter, D. N. (2008). Status of Caribbean Coral Reefs after Bleaching and Hurricanes in 2005. Global Coral Reef Monitoring Network, 148.
Williams, D. M. B., Roth, C. H., Reichelt, R., Ridd, P., Rayment, G. E., Larcombe, P., et al. (2002). The current level of scientific understanding on impacts of terrestrial run-off on the Great Barrier Reef World Heritage Area. Townsville, QLD: CRC Reef Research Centre.
Keywords: coral, coral community dynamics, reef degradation, carbonate production, Caribbean, anthropogenic stress
Citation: De Bakker DM, Meesters EH, Bak RPM, Nieuwland G and Van Duyl FC (2016) Long-term Shifts in Coral Communities On Shallow to Deep Reef Slopes of Curaçao and Bonaire: Are There Any Winners? Front. Mar. Sci. 3:247. doi: 10.3389/fmars.2016.00247
Received: 28 July 2016; Accepted: 11 November 2016;
Published: 25 November 2016.
Edited by:
Christian Wild, University of Bremen, GermanyReviewed by:
Susana Enríquez, National Autonomous University of Mexico, MexicoVerena Schoepf, University of Western Australia, Australia
Copyright © 2016 De Bakker, Meesters, Bak, Nieuwland and Van Duyl. This is an open-access article distributed under the terms of the Creative Commons Attribution License (CC BY). The use, distribution or reproduction in other forums is permitted, provided the original author(s) or licensor are credited and that the original publication in this journal is cited, in accordance with accepted academic practice. No use, distribution or reproduction is permitted which does not comply with these terms.
*Correspondence: Didier M. De Bakker, didier.debakker@wur.nl; didierdebakker@gmail.com
Fleur C. Van Duyl, fleur.van.duyl@nioz.nl