- 1SECALIM, UMR 1014, ONIRIS, Université Bretagne Loire, Nantes, France
- 2USC Institut National de la Recherche Agronomique Sensométrie et Chimiométrie, ONIRIS, Université Bretagne Loire, Nantes, France
- 3Laboratoire Ecosystèmes Microbiens et Molécules Marines pour les Biotechnologies, IFREMER, Nantes, France
Optimization of Carnobacterium divergens V41 growth and bacteriocin activity in a culture medium deprived of animal protein, needs for food bioprotection, was performed by using a statistical approach. In a screening experiment, 12 factors (pH, temperature, carbohydrates, NaCl, yeast extract, soy peptone, sodium acetate, ammonium citrate, magnesium sulfate, manganese sulfate, ascorbic acid and thiamine) were tested for their influence on the maximal growth and bacteriocin activity using a two-level incomplete factorial design with 192 experiments performed in microtiter plate wells. Based on results, a basic medium was developed and three variables (pH, temperature and carbohydrates concentration) were selected for a scale-up study in bioreactor. A 23 complete factorial design was performed, allowing the estimation of linear effects of factors and all the first order interactions. The best conditions for the cell production were obtained with a temperature of 15°C and a carbohydrates concentration of 20 g/l whatever the pH (in the range 6.5–8), and the best conditions for bacteriocin activity were obtained at 15°C and pH 6.5 whatever the carbohydrates concentration (in the range 2–20 g/l). The predicted final count of C. divergens V41 and the bacteriocin activity under the optimized conditions (15°C, pH 6.5, 20 g/l carbohydrates) were 2.4 × 1010 CFU/ml and 819200 AU/ml respectively. C. divergens V41 cells cultivated in the optimized conditions were able to grow in cold-smoked salmon and totally inhibited the growth of Listeria monocytogenes (< 50 CFU g−1) during 5 weeks of vacuum storage at 4 and 8°C.
Introduction
Microbiological quality of food, especially ready-to-eat food, is a constant concern in the food industry. Lightly preserved fish products such as cold-smoked salmon (CSS) are classified as high risk products toward micro-organisms and particularly Listeria monocytogenes (Huss et al., 2000; Sumner and Ross, 2002; EFSA 2015). Indeed, (i) there is no critical control point during the process, (ii) those products have extended shelf-life during which growth of some psychrotrophic micro-organisms is possible, and (iii) they are consumed without further cooking. The preservative hurdles used in such products are salting, drying, smoking, vacuum-packaging, and chilled-storage, in association with good hygienic and manufacturing practices in industry. These hurdle could be combined with lactic acid bacteria (LAB) used as an extra hurdle to prevent the growth of spoiling and pathogenic bacteria. LAB are able to grow in cold-smoked fish products (Leroi et al., 1998; Gonzalez-Rodriguez et al., 2002; Mejlholm and Dalgaard, 2007) and some of them show an effective microbial antagonism due to competition mechanisms, production of organic acids, and/or production of bacteriocins (Rodgers, 2001; Ross et al., 2002; Pilet and Leroi, 2011), and in particular in seafood products (Leroi et al., 1996; Duffes et al., 1999a; Katla et al., 2001; Tahiri et al., 2009; Leroi, 2010; Leroi et al., 2015). In a former study at laboratory level, we have shown that a bacteriocin-producing strain of LAB, Carnobacterium divergens V41, co-inoculated with several strains of L. monocytogenes in CSS (inoculation level 105 and 20 CFU/l respectively), was able to prevent growth of Listeria during the 4 weeks of vacuum storage at 4 and 8°C (< 50 CFU/g) (Brillet et al., 2004). The inhibitory effect was attributed to the production of a class IIa bacteriocin, divercin V41 (Métivier et al., 1998) and this was confirmed by the use of a divercin- mutant which did not inhibit L. monocytogenes in CSS (Richard et al., 2003). Safety and acceptability criteria were provided (Brillet et al., 2005) and the species C. divergens has been included in a revised authoritative list of microorganisms published in 2002 as a result of a joint project between the International Dairy Federation and the European Food and Feed Cultures Association (Bourdichon et al., 2012). In order to implement the biopreservation strategy in food industry, it is necessary to optimize the production of Carnobacterium as a starter culture and to check its subsequent growth and inhibition activity in the product after inoculation. Although, in recent investigations specific environmental conditions including the ones found in various food-stuffs have been studied to determine their effect upon the bacteriocins production (Leal-Sanchez et al., 2002; Messens et al., 2003; Neysens et al., 2003; Mataragas et al., 2004; Drosinos et al., 2005; Schobitz et al., 2006; Polak-Berecka et al., 2010; Akkoç et al., 2011), little is known about growth and bacteriocins production of C. divergens (Connil et al., 2002). In most studies, optimization is performed in laboratory culture media such as MRS (De Man et al., 1960) but some studies also report cultivation tests on several raw materials, residues or by-products of low- (or zero-) cost for the production of bacteriocins (Todorov and Dicks, 2005, 2007a; Metsoviti et al., 2011; Garsa et al., 2014; Bali et al., 2016). Those media may contain meat extracts or animal sourced materials. In the hypothesis that residues of medium could be found in the protective culture that will be added to a food product, the composition of the media used for the starter production should be considered. In France, the use of cattle materials (like animals' flours) is totally prohibited in the feed for animals intended for human consumption (Règlement (CE) n°999/2001)1 as well as in dietary supplements and cosmetics in the United States (FDA, 2016). To avoid the risk of bovine spongiform encephalopathy infectivity the optimization of C. divergens V41 growth was performed in a medium where vegetal proteins replaced meat protein. Furthermore, it was important to test the effect of culture conditions of C. divergens on its subsequent activity in food matrix.
A first screening of the effect of 12 factors on C. divergens V41 growth was performed in microtiter plate wells. Experiments were organized following a two-level incomplete factorial design containing 192 experiments. Optimization was then performed in 2-l bioreactors. Nine of the previous factors were fixed at the level providing the best growth. A complete factorial design was used to model the effect of three selected factors (carbohydrates concentration, pH, and temperature). Finally, challenge tests using cells produced in optimized conditions were investigated in CSS in co-culture with L. monocytogenes.
Materials and Methods
Bacterial Strains
C. divergens V41 was isolated from salmon intestine and has been previously characterized by Pilet et al. (1995). This strain produces the divercin V41 which is a class IIa bacteriocin (Métivier et al., 1998), active against L. monocytogenes both in model medium and in CSS (Duffes et al., 1999a,b; Richard et al., 2003; Brillet et al., 2004). L. monocytogenes RF76, RF107, RF114, RF119, RF129, and RF148 isolated from CSS industry were kindly provided by ASEPT (Laval, France). All strains were stored at −80°C with 20% (v/v) sterile glycerol in Elliker broth (BK 054, Biokar Diagnostics, Beauvais, France) for C. divergens V41 and Brain Heart broth (BK015, Biokar Diagnostics) for L. monocytogenes.
Culture Media and Inoculum Preparation
The broth used to cultivate C. divergens V41 before inoculation in microtiter plate wells and bioreactors was made without protein of animal origin, and contained soy trypton (10 g/l, Organotechnie S.A., La Courneuve, France), yeast extract (5 g/l, Biokar Diagnostics), D-glucose (10 g/l), and NaCl (4 g/l). This medium was named SYGNa broth (Soy, Yeast, Glucose, and NaCl). For each experiment, the Carnobacterium strain was sub-cultured twice successively in 10 and 100 ml of SYGNa broth for 24 h at 30°C. L. monocytogenes RF76 used in the agar diffusion method was sub-cultured twice successfully in 10 ml of Elliker broth for 24 h at 30°C, and L. monocytogenes RF107, RF114, RF119, RF129, and RF148 used in the CSS experiment were sub-cultured twice successfully in 10 ml of Brain Heart broth with 3% (w/v) NaCl for 24 h at 15°C.
Experimental Designs
First, a screening experimental design was performed. Twelve factors having potential influence on growth have been chosen according to the literature (Connil et al., 2002; Messens et al., 2003; Neysens et al., 2003) and composition of selective LAB culture media such as Elliker (Elliker et al., 1956) or MRS (De Man et al., 1960): pH, temperature, carbohydrates (mix of equal weight of glucose, lactose, and saccharose), NaCl, yeast extract, soy peptone, sodium acetate, ammonium citrate, magnesium sulfate, manganese sulfate, ascorbic acid and thiamine concentrations. The effects of these factors were estimated by performing a two-level incomplete factorial design (128 experiments), with duplicates of 64 experiments. The factor levels, coded as values of −1 and +1, were as follows: pH 6.5 (−1) and 8.0 (+1), temperature 15°C (−1) and 30°C (+1), carbohydrates 2 g/l (−1) and 20 g/l (+1), NaCl 10 g/l (−1) and 60 g/l (+1), yeast extract 5 g/l (−1) and 15 g/l (+1), soy peptone 1 g/l (−1) and 10 g/l (+1), sodium acetate 0 g/l (−1) and 5 g/l (+1), ammonium citrate 0 g/l (−1) and 4 g/l (+1), magnesium sulfate 0 g/l (−1) and 0.4 g/l (+1), manganese sulfate 0 g/l (−1) and 0.08 g/l (+1), ascorbic acid 0 g/l (−1) and 1 g/l (+1), and thiamine 0 g/l (−1) and 0.05 g/l (+1).
The design (Table 1) is a D-optimal design (Box and Draper, 1987) allowing the estimation of the main effect of each factor and most of the first order interactions without aliasing. The screening experimental design to estimate the effect of each factor was chosen according to different considerations. Very often in literature, screening of numerous factors is performed with experimental designs containing reduced number of experiments (k+3 at maximum, k being the number of factors studied). Those designs, called Hadamard matrices (Plackett and Burmann, 1946) allow the estimation of the main effect of the k factors but they are all aliased with first (and higher) order interactions, thus leading to possible confusions when selecting the most influent factors. In our study, the main effects of the 12 factors and their first order interactions were estimated without aliasing. A 212−5 (128 experiments) fractional factorial design was necessary to estimate these effects. However, preparing 128 different culture media at the same time was unrealistic and experiments were performed in two trials. A D-optimal design was generated using the Federov modified method (procedure OPTEX of the SAS/QC software, Version 6, First Edition, Cary, NC): the determinant of the information matrix is maximized iteratively under the constraint of the number of experiments. Sixty four runs combining 11 factors (corresponding to 64 different culture media) were performed at 30°C in a first block of experiments and the same runs were then performed at 15°C in a second block. This design provided much more information than a classical fractional 212−6 (64) factorial design. A microtiter plate contains 96 wells, allowing replicates of 32 experiments in each block.
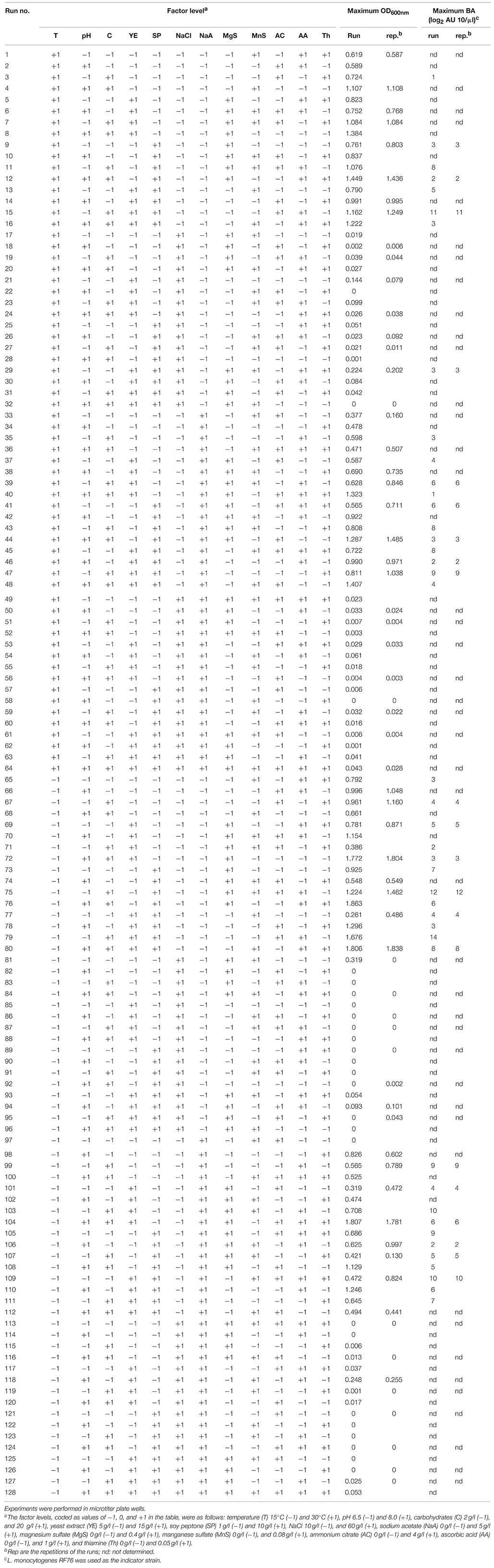
Table 1. Screening experimental design and factor levels (coded values) to estimate the main effect of each factor (and most of the order one interactions without aliasing) on the growth of C. divergens V41 (ODmax) and its maximal bacteriocin activity (BAmax).
Three variables (pH, temperature, and carbohydrates concentration) showing an interesting effect on the growth and/or on the production of bacteriocin by C. divergens V41 in microtiter plate wells were selected for study in bioreactor. In general, each component previously tested showing a positive effect on the responses was retained for the basic composition of the culture medium. The composition of the broth is detailed in the results part of this article. A 23 complete factorial design was performed, completed with three replicates of the center point of the domain (Table 2). All experiments were randomly run. The factor levels, coded as values of −1, 0 and +1, were as follows: pH 6.5 (−1), 7.25 (0) and 8.0 (+1), temperature 15°C (−1), 22.5 (0) and 30°C (+1), carbohydrates 2 g/l (−1), 11 (0), and 20 g/l (+1).
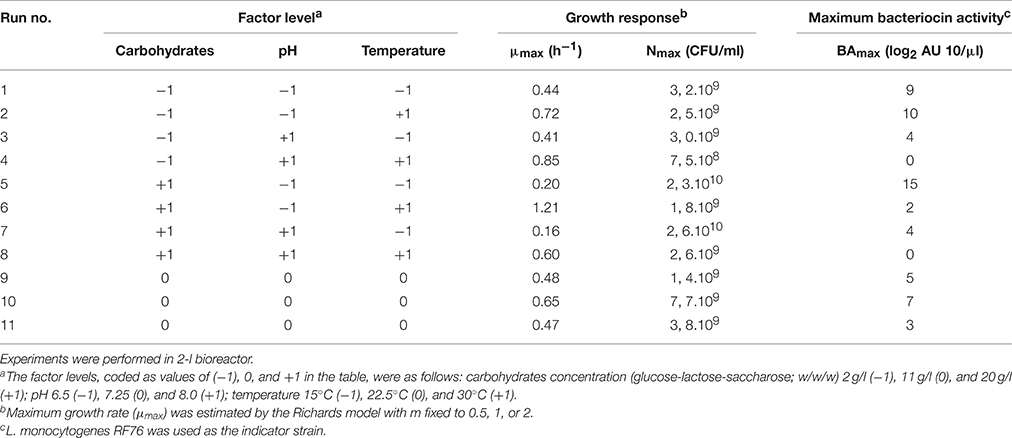
Table 2. Experimental design and factor levels (coded values) used to model maximum growth rate (μmax), maximum bacterial count (Nmax), and bacteriocin activity (BAmax) of C. divergens V41 as a function of carbohydrates concentration, pH and temperature.
Experimental Conditions
For the screening design, experiments were performed in microtiter plate containing 250 μl of medium per well (200 μl of culture medium and 50 μl of inoculum). Each compound (carbohydrates, soy peptone, sodium acetate, ammonium citrate, magnesium sulfate, manganese sulfate, ascorbic acid and thiamine) was dissolved individually in a buffer solution Na2HPO4/NaH2PO4 (0.1 M) at pH 6.5 and 8.0. For each level (+1 or −1) a 12.5-fold concentrated stock solution was prepared. NaCl being insoluble when concentrated 12.5 times was prepared with yeast extract, allowing a 6.25-fold concentration. Because of solubility problem in phosphate buffer, magnesium sulfate, and manganese sulfate solutions were prepared in distilled water adjusted at pH 6.5 and 8.0 with HCl/NaOH. Five milliliters of each of the eight individual solutions and 10 ml of NaCl + yeast extract solution were mixed according to the composition of the 64 culture media required for the experiments. The volume was adjusted to 50 ml when necessary with the appropriate buffer solution. The pH of each culture medium was verified (± 0.02 pH unit) and media were sterile filtered (0.22 μm) and kept at 4°C before used. Sixty four experiments were performed respectively at 15°C and at 30°C in two successive blocks of experiments, in a microtiter plate containing 200 μl of medium per well. For each temperature, 32 experiments were repeated. The culture media were inoculated with 50 μl of an appropriate dilution of a pre-culture of C. divergens V41 to obtain an initial inoculation concentration of 106 CFU/ml.
Experiments in bioreactors were performed in a 2-l SGI vessel (SGI, Toulouse, France) containing 940 ml of the optimized culture broth (see Section Screening experimental design) sterilized by autoclaving 15 min at 121°C in bioreactor. This medium contained (g/l): NaCl (10), yeast extract (15), soy peptone (10), MgSO4 (0.4), and ascorbic acid (1). Next, 4, 22, or 40 ml of filter-sterilized carbohydrates solution (glucose-lactose-saccharose; w/w/w; 500 g/l) were added to bring the final carbohydrates concentration to 2, 11, and 20 g/l respectively. When required, the volume was adjusted to 980 ml with sterile distilled water. The culture medium was brought to the final volume (1 l) by addition of 20 ml of the C. divergens V41 inoculum. The subculture obtained in SYGNa broth as described above was appropriately diluted to obtain an initial concentration of 107 CFU/ml in each bioreactor. Cultures were run under agitation (200 rpm) at 15, 22.5, or 30°C when appropriate and the pH was adjusted to 6.5, 7.25, or 8.0 by automatic addition of NaOH (6N).
Analysis of the Samples
For the screening design, bacterial growth was estimated by optical density (OD) monitoring at 600 nm, after shaking, with a microplate reader (ELX808iu Ultra Microplate Reader, Biotek Instruments, St-Quentin Yvelines, France). Measurements were done every hour during 48 h at 15°C and every half hour during 24 h at 30°C and data were collected on a computer (Excel software, version 10, Microsoft, France); the response retained was the maximal OD600nm (ODmax) calculated as the difference between the maximum OD600nm observed within 24–48 h of culture and minimum OD600nm. For the wells where a growth was observed, experiments were repeated in a new set and divercin V41 was quantified in the supernatant by the agar diffusion method (Brillet et al., 2004), using L. monocytogenes RF76 as target strain. The measures were done at the beginning of the stationary phase and at the end of the experiment (24 h at 30°C, or 48 h at 15°C), and the maximal bacteriocin activity detected in the supernatants was retained. Results were expressed as base 2 logarithm of arbitrary activity units per 10 μl (log2 AU/10 μl), AU/10 μl corresponding to the last dilution of the culture showing an inhibition zone of L. monocytogenes when spotting 10 μl of supernatant in a plate containing L. monocytogenes. This logarithmic transformation was used to reduce variance due to the wide range of activity values obtained. This answer had also a biological significance, corresponding to n, 1/2n being the dilution factor of the last spot showing an inhibition zone.
For experiments in bioreactors, samples were aseptically withdrawn at convenient time intervals. The OD600nm was measured every hour and growth curves were fitted with the Richards model (Zwietering et al., 1990) with parameter m fixed to 0.5, 1, or 2 as suggested by Dalgaard and Koutsoumanis (2001). This model allows neperian μmax to be estimated accurately from absorbance growth curve for wide range of growth conditions and gives better estimation than the Gompertz, exponential or Logistic models (Dalgaard and Koutsoumanis, 2001). Cultivable cell number and bacteriocin activity were determined after inoculation and at the beginning and the end of the stationary phase. Cultivable cells were determined by spread plating serial dilutions of the samples onto Elliker with 1.5% agar plates and incubating at 30°C for 48 h. Bacteriocin activity was semi quantified by the same method described for experiments in microtiter plate wells. μmax estimated from the Richards fitting, maximum bacterial count (Nmax) estimated by the plating technique and maximum bacteriocin activity (BAmax log2 AU 10 μ/l) were retained as responses.
Experiments in Sterile CSS Blocks
According to results from the experimental design in bioreactor, C. divergens V41 was cultivated again in three different conditions: (1) carbohydrates 20 g/l, pH = 8.0, 30°C; (2): carbohydrates 20 g/l, pH = 6.5, 15°C and condition 3: carbohydrates 2 g/l, pH = 6.5, 15°C. The inoculum size of C. divergens V41 and the regulation setting were the same as describe above. When the stationary growth phase was reached, the cells were centrifuged then washed in physiological saline solution (0.1% (w/v) tryptone (Biokar) and 0.85% (w/v) NaCl) just before used. Appropriate dilutions of these cultures were co-inoculated with a set of five L. monocytogenes strains (RF107, RF114, RF119, RF129, and RF148) in parts of 30 g of sterile CSS blocks (Joffraud et al., 1998). CSS blocks were vacuum-packed and stored for 36 days (9 days at 4°C followed by 27 days at 8°C). The initial desired levels of L. monocytogenes and C. divergens V41 in the salmon flesh were 20 and 105 CFU g−1 respectively. A control for L. monocytogenes was prepared by inoculating CSS blocks with L. monocytogenes alone (the Carnobacterium subculture being replaced by sterile physiological saline solution). Microbial analysis (LAB and Listeria spp.) was carried out weekly as described by Brillet et al. (2004). Each experiment was repeated three times.
Statistical Analysis
Results of the screening design and the design in bioreactor were analyzed by the Experimental Design Module of the Statgraphics software (Statgraphics Plus, version 4, Manugistics, Rockville, Maryland, USA). For microbial data in CSS, results are expressed as mean of three independent measures ±95% Confidence Interval (CI = 1.96 × ). Means were compared by the least significance difference (LSD) test at the 0.05 level of probability (Statgraphics Plus).
Results
Screening Experimental Design
The 192 growth curves performed in microtiter plate wells have been plotted (data not shown) and ODmax was retained as response (Table 1). The incomplete factorial design allowed the estimation without aliasing of the main effects of the 12 factors and most of the first order interactions. Nine factors had a significant effect (P < 0.05) on the response ODmax whereas three factors (ammonium citrate, thiamine and temperature) did not show significant effects on the response. Among the nine factors cited, NaCl had by far the main influence on the growth (-0.86). That means that when NaCl concentration varies from the level −1 (1%) to +1 (6%), ODmax estimated can be decreased by 0.86 OD unit. As an indication, the average ODmax, estimated when all the factors are at level 0, was 0.46. The effect of the eight other factors, positive or negative, was significant but weakest (ranging between 0.06 and 0.16). Carbohydrates (+0.16), pH (+0.15), ascorbic acid (+0.11), yeast extract (+0.10), soy peptone (+0.07), and magnesium sulfate (+0.06) had a positive effect, indicating that the response increased when these factors reached their maximum level into the limits of the coded values used in this study. The estimated effects of NaCl (−0.86), sodium acetate (−0.15), and manganese sulfate (−0.12) concentrations were negative. Five first order interactions had also a significant but slight effect on the response. The interactions of NaCl with carbohydrates (−0.19), pH (−0.16), or ascorbic acid (−0.08) or the interaction of temperature with ascorbic acid (−0.10) had a negative estimated effect, whereas the interaction of sodium acetate with NaCl (+0.13) had a positive effect on the response. Those results indicate that the inhibiting effect of NaCl on growth is reinforced at high value of carbohydrate, pH and ascorbic acid, but lowered in presence of sodium acetate.
For the wells where a growth was observed, runs were repeated in a new set of experiments and bacteriocin activity was measured at the beginning of the stationary phase (known from the first set of experiments) and at the end of the experiment (24 h at 30°C, 48 h at 15°C). The maximal bacteriocin activity detected in the supernatants was retained as response (Table 1). For the wells where no growth was observed (ODmax < 0.01 in 52 wells among 192), bacteriocin was supposed to be not produced. This hypothesis has been previously validated in two wells showing no OD600nm increase. Seven factors were found to have significant effects (P < 0.05) on the bacteriocin activity expressed as log2 AU 10/μl. The estimated effects of soy peptone (+1.61), ascorbic acid (+1.24), carbohydrates (+1.09), yeast extract (+0.70), and sodium acetate (+0.28) were positive, indicating that the response BAmax increased when these factors reached the level coded by the value (+1). The estimated effects of NaCl (−3.53), pH (−1.99), temperature (−0.84), and manganese sulfate (−0.51) were negative, indicating that the response decreased when the value of these factors increased. Thiamine, ammonium citrate and magnesium sulfate concentrations did not show significant effects on the response. Once again, NaCl was the factor with the highest effect on the response, followed by pH. The average BAmax, estimated when all the factors were at level 0, was 1.83. When NaCl concentration varies from the level +1 (6%) to −1 (1%), BAmax estimated increased by 3.53 log2 AU 10/μl. When pH varies from 8 to 6.5, BAmax estimated increased by 1.99 log2 AU 10/μl. The effect of the seven other factors, positive or negative, was significant but weakest, ranging from 0.28 to 1.61. Seven first order interactions had also a significant but slight effect on the response. The interactions of NaCl with carbohydrates (−1.21) or ascorbic acid (−1.11), the interaction of pH with soy peptone (−0.74) and the interaction of temperature with ascorbic acid (−0.59) had a negative estimated effect, whereas the interaction of NaCl with pH (+1.86), temperature (+0.94) or manganese sulfate (+0.59) had a positive effect on the response BAmax.
The purpose of the screening experimental design in microtiter plate wells was to obtain experimental data which served as an initial approach to optimize growth in larger volume, establishing which factors had significant effects on both responses. In the purpose of an application of C. divergens V41 in CSS at pilot scale, it is necessary to produce high quantity of living cells and so to validate in bioreactor results obtain in microtiter plate wells. The two factors having the main positive effects on growth (carbohydrates concentration (2–20 g/l) and pH (6.5–8.0) were retained for further experiments. The other components previously tested showing a significant positive effect on the responses (ascorbic acid, yeast extract, soy peptone, and magnesium sulfate) were retained for the basic composition of the culture medium. The concentration chosen corresponded to the level coded by the value (+1). Concentrations of the components showing a negative or a non-significant effect (NaCl, sodium acetate, manganese sulfate, ammonium citrate, and thiamine) were fixed at the level coded by the value (−1). For all those factors, the levels chosen for the basic medium also corresponded to the levels in favor of a high production of bacteriocin. The factor temperature (15–30°C) was tested again because of its influence on the bacteriocin activity. Finally, the composition of the basic culture medium used in bioreactor was as follows (g/l): NaCl (10), yeast extract (15), soy peptone (10), MgSO4 (0.4), and ascorbic acid (1).
Optimization of C. divergens V41 Growth and Bacteriocin Activity in a Bioreactor
The different responses measured in the 11 bioreactors (μmax, Nmax, and BAmax) are presented in Table 2. The μmax (neperian) ranged between 0.16 and 1.21 h−1, corresponding to generation times of 4.33 and 0.57 h respectively. The Nmax ranged from 7.5 × 108 to 2.6 1010 CFU g−1 and BAmax between 0 and 15 log2 AU 10/μl. Results from the complete two-level factorial design performed in bioreactor allowed to estimate the linear effects of the three factors carbohydrate concentration, pH and temperature and their first order interactions on the different responses. An additional experiment in the center of the domain, done in triplicate, allowed to estimate experimental error and to validate the linear model a priori postulated. Results are shown in Table 3. Temperature was the only factor having a significant effect (+0.54; P < 0.05) on μmax and no significant interaction between factors was detected. The μmax increased proportionally (linearly) to the temperature increase, by 0.54 h−1 when temperature raised from 15 to 30°C. As an example, Figure 1A shows μmax as a function of temperature and carbohydrate, pH being fixed at level −1 (6.5). A lack of fit test was designed to determine whether the linear model chosen was adequate to describe the observed data, or whether a more complicated model should be used. The test was performed by comparing the variability of the current model residuals to the variability between observations at replicate settings of the factors. The P-value for lack-of-fit was 0.21 (greater than 0.05), indicating the model was adequate for the observed data at the 95.0% confidence level. The R-Squared statistic indicates that the model as fitted explains 90.0% of the variability in μmax.
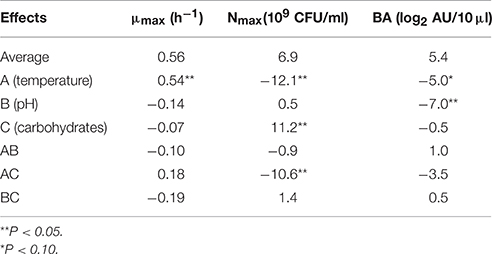
Table 3. Effects of temperature, pH and carbohydrates and their interactions on the responses maximum growth rate (μmax), maximum bacterial count (Nmax), and bacteriocin activity (BA; L. monocytogenes RF76 was used as the indicator strain).
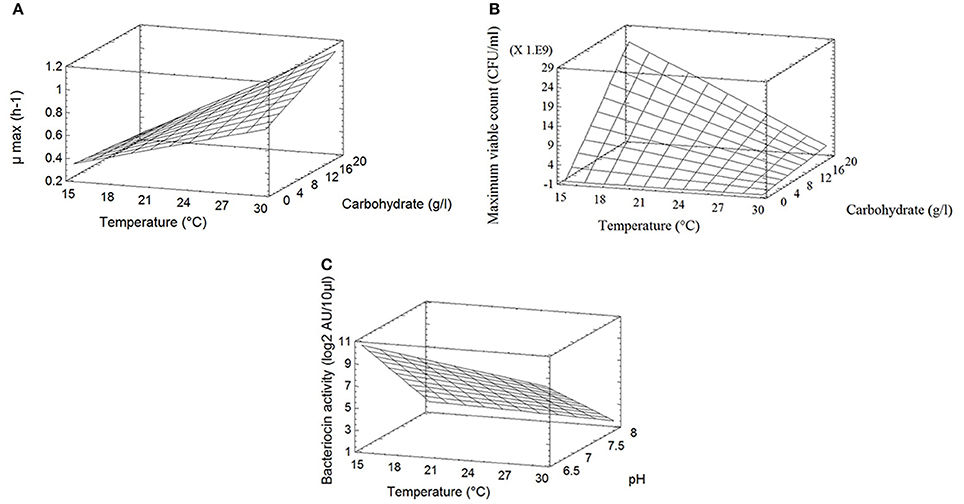
Figure 1. (A) Response surface of the maximum growth rate μmax (h−1) of C. divergens V41 as a function of temperature and carbohydrates concentration at pH 6.5. (B) Response surface of the maximum bacterial count (CFU/ml) of C. divergens V41 as a function of temperature and carbohydrates concentration at pH 6.5. (C) Response surface of the bacteriocin production (log2 AU 10/μl) by C. divergens V41 as a function of temperature and pH with a carbohydrates concentration of 2 g/l. L. monocytogenes RF76 was used as the indicator strain.
Temperature and carbohydrates concentration, and their interaction, showed significant effects (respectively −12.1 × 109, +11.2 × 109; −10.6 × 109; P < 0.05) on the response Nmax, whereas pH had no effect (Table 3). The maximum bacterial count as a function of temperature and carbohydrate is shown on Figure 1B, pH being fixed at level −1 (6.5). Contrary to what has been observed for μmax, the effect of temperature is negative, indicating that the maximum bacterial count increased when the temperature decreased in the limit of the experimental design. The effect of carbohydrates concentration is positive. Due to an important negative interaction between temperature and carbohydrates concentrations, the negative effect of temperature is reinforced at high carbohydrates concentrations, and the positive effect of carbohydrate concentration is increased at low temperatures. The lack of fit test (P = 0.41) indicated that the linear model could be validated at the 95.0% confidence level. The R-Squared statistic indicates that the model as fitted explains 94.0% of the variability in maximum bacterial counts.
Concerning the bacteriocin activity, pH was the factor which had the main effect on the response, expressed as log2 AU 10/μl (−7.0; P < 0.05). This effect was negative, i.e., bacteriocin activity decreased when pH increased. Temperature had a slight significant effect on the response (−5.0; P < 0.10) whereas carbohydrates concentration had no significant effect. No interaction between factors was observed. The linear model was once again validated by the three experiments performed at the center of the domain and R2 was 0.84. As an example, the bacteriocin activity as a function of temperature and pH is shown on Figure 1C, carbohydrates being fixed at 2 g/l. The average BAmax, estimated when all the factors are at level 0, was 5.4 log2 AU 10/μl, corresponding to 4222 AU/ml. When the pH varies from the level +1 (8.0) to −1 (6.5), BAmax estimated can be increased by 7.0 log2 AU 10/μl i.e., the bacteriocin activity in AU per milliliter is multiplied by 27 when pH varies from 8.0 to 6.5. As an example, predicted BAmax is equal to 373 AU/ml at pH 8 (the other factors being fixed at the level 0), and to 47771 AU/ml at pH 6.5.
According to these results, the culture conditions to maximize the final bacterial cultivable count in bioreactor in the broth without animal protein are 20 g/l of carbohydrates and a temperature of 15°C, pH within the range 6.5–8.0 having low effect on this response. With those conditions (carbohydrate 20 g/l, temperature 15°C, pH 8.0), a final predicted bacterial count of 2.4 × 1010 CFU/ml can be reached. On the other hand, at 15°C, μmax is much lower than at 30°C (0.16 and 0.60 h−1 respectively) and consequently, the maximum bacterial count is reached after 38–40 h of fermentation vs. 18–20 h at 30°C.
The optimal conditions for bacteriocin activity in the broth without animal protein were pH 6.5 with a temperature of 15°C, carbohydrates concentration within the range 2–20 g/l having no significant effect on the response. In those conditions (pH 6.5, temperature 15°C, carbohydrate 20 g/l), a production of 13 log2 AU 10/μl was predicted, corresponding to 819200 AU/ml.
Growth and Inhibition Capacities of C. divergens V41 in CSS After Growing in Optimized Media
To evaluate the influence of the culture conditions on the subsequent capacity of C. divergens V41 to grow in CSS and inhibit L. monocytogenes, C. divergens V41 was cultivated in bioreactor either in the optimal conditions of growth and divercin V41 production (conditions 1: carbohydrates 20 g/l, pH = 6.5, 15°C,) or in culture medium and conditions nearer from the CSS matrix (conditions 2: carbohydrates 2 g/l, pH 6.5, 15°C). In conditions 1 and 2, a final bacterial concentration of 2.4 × 1010 and 2.0 × 109 CFU/ml were predicted by the model. As a potential application of the strain in a biopreservation strategy, C. divergens V41 cultivated in those two different conditions was inoculated in sterile CSS blocks in co-culture with L. monocytogenes. The salt and total phenol concentrations of CSS blocks used in these experiments were respectively 4.3% (w/w, total phase) corresponding to 6.3% (w/w, water phase) and 1.25 mg/100 g. Dry matter and total fat content were 35.9% (w/w) and 7.6% (w/w) respectively. Figure 2 represents the growth curves of C. divergens V41 and L. monocytogenes in CSS during the 5 weeks of vacuum storage at chilled temperature. C. divergens V41 inoculated after pre-cultivating under the two different conditions were able to colonize CSS blocks. The inoculation levels in CSS blocks were 3.7 ± 1.7 × 105 and 2.3 ± 0.6 × 105 CFU/g respectively for condition 1 and 2 (no statistical difference). A very slight growth was observed during the chilled storage, growth curves being generally similar but final count was slightly higher for C. divergens V41 pre-cultivated in conditions 1 than for C. divergens V41 in conditions 2 (1.8 ± 0.9 × 106 and 6.1 ± 1.5 × 105 CFU/g respectively). L. monocytogenes inoculated alone grew easily in CSS blocks from 4.0 ± 0.9 × 101 to 0.8 ± 1.2 × 104 CFU/g. When the pathogenic strains were in co-culture with C. divergens V41 pre-cultivated in conditions 1 and 2, the growth of L. monocytogenes was totally inhibited all over the storage, the final count reaching 3.5 ± 0.5 × 101 and 2.2 ± 0.9 × 101 CFU/g respectively (no statistical difference).
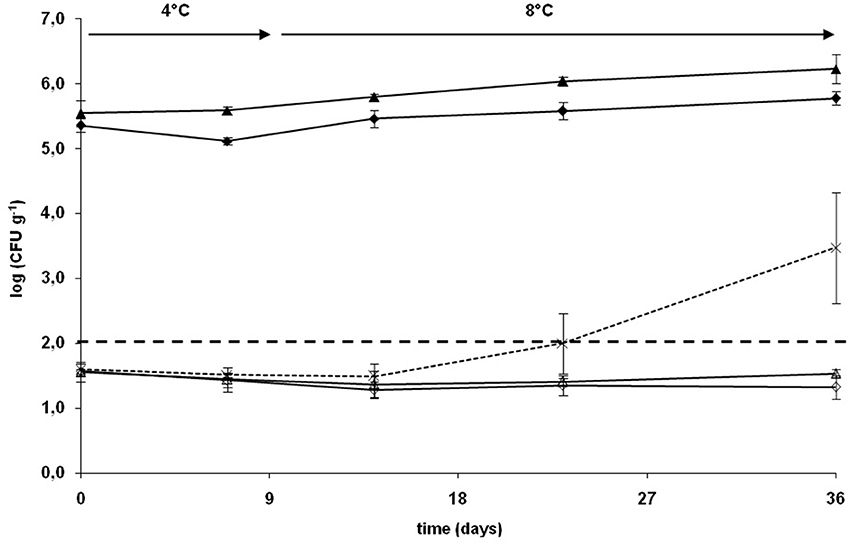
Figure 2. Growth of a mix of five strains of L. monocytogenes alone (×, dotted lines) and with C. divergens V41 pre-cultured in bioreactor in conditions 1 (15°C, pH 6.5, 20 g/l carbohydrates, ▵ full line) and 2 (15°C, pH 6.5, 2 g/l carbohydrates, full line ♢) in sterile CSS blocks during vacuum storage at 4 and 8°C. Growth of C. divergens V41 in presence of L. monocytogenes after pre-culture in bioreactor in conditions 1 (full line▴) and conditions 2 (full line ♦). Each growth curve represents the mean of three enumerations (bars: 95% confidence interval).
Discussion
After analyzing the screening experiments, the NaCl concentration showed the greatest effect on the growth and the bacteriocin activity. The level (+1) corresponding to 6% NaCl (w/v) in the medium was chosen because it corresponded to the NaCl concentration found in CSS (water phase) in Europe (Cardinal et al., 2004). The effect of NaCl was negative into the limits of the values used in this study. Although, the Carnobacteria are halotolerant (Laursen et al., 2005), a high concentration of NaCl partially inhibited the growth, as already shown by Connil et al. (2002) in sterile CSS extract. The pH factor had a positive effect on the growth. It is reported that Carnobacteria can grow easily at alkaline pH (Holzapfel, 1992). On the contrary, pH had a significant negative effect on the bacteriocin activity, showing a slight acidic pH such as 6.5 is necessary to obtain a high bacteriocin activity. A different pH optimum for growth and for bacteriocin activity has already been observed for a bacteriocin-producing strain of Lactobacillus curvatus (Messens et al., 2003). Concerning the main components of the culture medium which had a positive effect on the growth, carbohydrates, soy peptone and ascorbic acid are energetic and constitutive substrates. The ascorbic acid decreases the redox potential which is favorable for the growth of facultative anaerobic micro-organisms such as LAB. Manganese sulfate as a stimulator of certain enzymes in LAB (Archibald, 1986) has been shown to stimulate growth of Carnobacteria (Baird et al., 1989) and a high concentration is used by Wasney et al. (2001) to develop a selective culture medium for this genus. The addition of manganese sulfate in a modified M17 broth led to an impressive increase in bacteriocin production by a Lactobacillus acidophilus strain (Abo-Amer, 2011), and the exclusion of manganese sulfate in MRS broth had also a negative effect on the bacteriocin production of some Lb. plantarum and Lb. fermentum strains (von Mollendorff et al., 2009). In our study, manganese sulfate did not show interesting effects on the growth and bacteriocin activity, probably due to the presence of other components (vitamins, cofactors…) in the soy peptone and yeast extract present in the medium even at level −1 (1 and 5 g/l respectively). On few Lactobacillus species cultured in supplemented MRS broth, it was shown that thiamine may increase the bacteriocin production (Todorov and Dicks, 2007b), however, most of the time the thiamine has no significant effect on bacteriocin production (Todorov and Dicks, 2006), neither on the growth (Polak-Berecka et al., 2010), as observed in our experiment.
The experimental design in bioreactors was performed to verify the results obtained in the screening experiment. Indeed, the conditions in bioreactors are probably different from those in microtiter plate wells, especially agitation conditions and pH regulation. The maximum bacterial count was mainly correlated to temperature and carbohydrate concentration, pH showing no significant effect. Nmax was observed at 20 g/l carbohydrate and 15°C. The simplified fitted model (non-significant parameters have been left out) was Nmax (CFU/ml) = 6.9 × 109 − 6,0 × 109 × (T°)c +5.6 × 109 × (carbohydrate)c − 5.3 × 109 × (T°)c × (carbohydrate)c where (T°)c and (carbohydrate)c were the coded values of the factors. The maximum carbohydrate concentration tested was 20 g/l as for some LAB (Leuconostoc mesenteroides), a decline of μmax as glucose concentration increased from 20 g/l to 30 or 45 g/l was observed, leading to a final biomass at 30 or 45 g/l glucose lower than that with 20 g/l (Drosinos et al., 2005). A mix of glucose, lactose, and saccharose was used as the incidence on growth/bacteriocin may vary according to carbohydrate source (Todorov et al., 2000; Drosinos et al., 2005). The negative effect of temperature on Nmax was coherent with previous results observed in Elliker broth by Duffes et al. (1999b). On the other hand, an increasing temperature significantly increased μmax. The fitted model was μmax (h−1) = 0.56+0.27 × (T°)c. In conclusion, at 15°C and 20 g/l of carbohydrate, a final biomass of 2.4 × 1010 CFU/ml can be obtained in 38–40 h, whereas at 30°C, 1.2 × 109 CFU/ml can be reached in 18–20 h.
The bacteriocin activity was mainly dependent on pH and temperature, maximum BA being obtained at low pH and low temperature, confirming results in microtiter plate wells. Many studies have shown that highest bacteriocin titres usually are obtained at pH and temperatures values lower than the optimum ones for growth (De Vuyst et al., 1996; Krier et al., 1998; Aasen et al., 2000; Abo-Amer, 2011). In this study, the optimum for bacteriocin activity and μmax where 15 and 30°C, respectively. Duffes et al. (1999b) also have shown that C. divergens V41 produced more divercin V41 at 20°C than at 30°C, in Elliker medium. However, the maximum cell density is obtained at 15°C, and this probably explain the accumulation of bacteriocin at this temperature. Carbohydrate concentration has low effect on bacteriocin activity, which is surprising as this concentration limits the number of C. divergens. Aktypis et al. (2007) have shown that 5 g/l glucose seemed to be insufficient to sustain an adequate growth of Streptococcus thermophilus ACA-DC 0040, resulting in a limited termophilin production. In the optimum condition, (pH 6.5, temperature 15°C, carbohydrate 20 g/l), an activity of 13 log2 AU 10/μl was predicted, corresponding to 819,200 AU/ml. This production is much higher than semi-optimized production obtained by Métivier et al. (1999). They obtained 4050 AU/ml after 40 h of culture in 2-l or 10-l tween-80 deficient MRS bioreactors, 20°C and pH regulated at 6.5. However, results are difficult to compare as the indicating L. monocytogenes strain used by Métivier et al. (1999) was different from the strain used in the present study. The experiments in CSS were performed to verify if the culture conditions of the strain had an effect on its subsequent growth and inhibition capacity in CSS. C. divergens V41 grew in CSS and maintain L. monocytogenes under 100 CFU/g during 5 weeks of vacuum storage at chilled and abuse temperature, whatever the culture conditions in bioreactor. Brillet et al. (2004) also observed a total inhibition of L. monocytogenes in presence of C. divergens V41 precultured in Elliker medium at 20°C, pH 6.5. A third culture condition has been tested (data not shown) (conditions 3: carbohydrates 20 g/l, pH 8, 30°C) that allowed to obtain a satisfying Nmax in a shorter time (1.6 × 109 CFU/ml in 18–20 h). However, we did not reach the desired inoculation level in CSS (1 log lower than expected) so the results could not be exploited.
In conclusion, for a biopreservation strategy, C. divergens V41 biomass can be produced in bioreactor in a medium deprived of protein of animal origin. The best culture conditions to obtain both a maximum biomass and an effective subsequent implantation and inhibition of L. monocytogenes in CSS are 15°C, pH 6.5, 20 g/l carbohydrates. In those conditions, a maximum biomass of living cells of 2.4 × 1010 CFU/ml is obtained in 38–40 h. When inoculated in CSS at an initial level of 105 CFU/g, the strain is able to guarantee that the count of L. monocytogenes, if not exceeding 40 CFU/g just after production, which is by far higher than the contamination level currently found in the CSS industry (Jorgensen and Huss, 1998), will never overpass the 100 CFU/g maximum tolerable limit till the sell-by date in many European countries.
Author Contributions
AB: Ph.D. student, in charge of experimentations, data treatments and contribution to the writing. PC: Conception of the experimental design. MP, FL and HP: Co-Director of the Ph.D. student. FL: Writing of the article.
Funding
This study was supported by a public grant from the French Agence Nationale de la Recherche within the context of ERANET COFASP program (reference ANR-14-COFA-0001, project SAFEFISHDISH).
Conflict of Interest Statement
The authors declare that the research was conducted in the absence of any commercial or financial relationships that could be construed as a potential conflict of interest.
Acknowledgments
We thank Frédérique Chevalier for her technical support and Carol Robins for the English language editing of the manuscript.
Footnotes
1. ^Règlement (CE) n°999/2001 du parlement européen et du conseil du 22 mai 2001 fixant les règles pour la prévention, le contrôle et l'éradication de certaines encéphalopathies spongiformes transmissibles. Journal Officiel L 147 du 31 Mai 2001. Available online at: http://eur-lex.europa.eu/LexUriServ/LexUriServ.do?uri=CONSLEG:2001R0999:20110318:FR:PDF (Access 25-07-2016).
References
Aasen, I. M., Moretro, T., Katla, T., Axelsson, L., and Storro, I. (2000). Influence of complex nutrients, temperature and pH on bacteriocin production by Lactobacillus sakei CCUG 42687. Appl. Microbiol. Biotechnol. 53, 159–166. doi: 10.1007/s002530050003
Abo-Amer, A. (2011). Optimization of bacteriocin production by Lactobacillus acidophilus AA11, a strain isolated from Egyptian cheese. Ann. Microbiol. 61, 445–452. doi: 10.1007/s13213-010-0157-6
Akkoç, N., Ghamat, A., and Akçelik, M. (2011). Optimisation of bacteriocin production of Lactococcus lactis subsp. lactis MA23, a strain isolated from Boza. Int. J. Dairy Technol. 64, 425–432. doi: 10.1111/j.1471-0307.2011.00671.x
Aktypis, A., Tychowski, M., Kalantzopoulos, G., and Aggelis, G. (2007). Studies on bacteriocin (thermophilin T) production by Streptococcus thermophilus ACA-DC 0040 in batch and fed-batch fermentation modes. Antonie van Leeuwenhoek 92, 207–220. doi: 10.1007/s10482-007-9148-4
Archibald, F. (1986). Manganese: its acquisition by and function in the lactic acid bacteria. Crit. Rev. Microbiol. 13, 63–109. doi: 10.3109/10408418609108735
Baird, R. M., Corry, J. E. L., Curtis, G. D. W., Mossel, D. A. A., and Skovgaard, N. P. (1989). Cresol Red Thallium Acetate Sucrose (CTAS) Agar. Int. J. Food Microbiol. 9, 129–131. doi: 10.1016/0168-1605(89)90058-5
Bali, V., Panesar, P. S., and Bera, M. B. (2016). Trends in utilization of agro-industrial byproducts for production of bacteriocins and their biopreservative applications. Critic Rev. Biotechnol. 36, 204–214. doi: 10.3109/07388551.2014.947916
Bourdichon, F., Casaregola, S., Farrokh, C., Frisvad, J. C., Gerds, M. L., Hammes, W. P., et al. (2012). Food fermentations: microorganisms with technological beneficial use. Int. J. Food Microbiol. 154, 87–97. doi: 10.1016/j.ijfoodmicro.2011.12.030
Box, G. E. P., and Draper, N. R. (1987). Empirical Model-Building and Response Surfaces. New York, NY: John Wiley and Sons.
Brillet, A., Pilet, M. F., Prevost, H., Bouttefroy, A., and Leroi, F. (2004). Biodiversity of Listeria monocytogenes sensitivity to bacteriocin-producing Carnobacterium strains and application in sterile cold-smoked salmon. J. Appl. Microbiol. 97, 1029–1037. doi: 10.1111/j.1365-2672.2004.02383.x
Brillet, A., Pilet, M. F., Prevost, H., and Leroi, F. (2005). Effect of inoculation of Carnobacterium divergens V41, a biopreservative strain against Listeria monocytogenes risk, on the microbiological, chemical and sensory quality of cold-smoked salmon. Int. J. Food Microbiol. 104, 309–324. doi: 10.1016/j.ijfoodmicro.2005.03.012
Cardinal, M., Gunnlaugsdottir, H., Bjoernevik, M., Ouisse, A., Vallet, J. L., and Leroi, F. (2004). Sensory characteristics of cold-smoked Atlantic salmon (Salmo salar) from European market and relationships with chemical, physical and microbiological measurements. Food Res. Int. 37, 181–193. doi: 10.1016/j.foodres.2003.12.006
Connil, N., Plissoneau, L., Onno, B., Pilet, M. F., Prevost, H., and Dousset, X. (2002). Growth of Carnobacterium divergens V41 and production of biogenic amines and divercin V41 in sterile cold-smoked salmon extract at varying temperatures, NaCl levels, and glucose concentrations. J. Food Prot. 65, 333–338.
Dalgaard, P., and Koutsoumanis, K. (2001). Comparison of maximum specific growth rates and lag times estimated from absorbance and viable count data by different mathematical models. J. Microbiol. Methods 43, 183–196. doi: 10.1016/S0167-7012(00)00219-0
De Man, J. D., Rogosa, M., and Sharpe, M. E. (1960). A Medium for the Cultivation of Lactobacilli. J. Appl. Bacteriol. 23, 130–135. doi: 10.1111/j.1365-2672.1960.tb00188.x
De Vuyst, L., Callewaert, R., and Crabbe, K. (1996). Primary metabolite kinetics of bacteriocin biosynthesis by Lactobacillus amylovorus and evidence for stimulation of bacteriocin under unfavourable growth conditions. Microbiology 142, 817–827. doi: 10.1099/00221287-142-4-817
Drosinos, E. H., Mataragas, M., Nasis, P., Galiotou, M., and Metaxopoulos, J. (2005). Growth and bacteriocin production kinetics of Leuconostoc mesenteroides E131. J. Appl. Microbiol. 99, 1314–1323. doi: 10.1111/j.1365-2672.2005.02735.x
Duffes, F., Corre, C., Leroi, F., Dousset, X., and Boyaval, P. (1999a). Inhibition of Listeria monocytogenes by in situ produced and semipurified bacteriocins of Carnobacterium spp. on vacuum-packed, refrigerated cold-smoked salmon. J. Food Prot. 62, 1394–1403
Duffes, F., Leroi, F., Boyaval, P., and Dousset, X. (1999b). Inhibition of Listeria monocytogenes by Carnobacterium spp. strains in a simulated cold smoked fish system stored at 4 degrees C. Int. J. Food Microbiol. 47, 33–42. doi: 10.1016/S0168-1605(98)00206-2
EFSA (2015). The European Union Summary Report on Trends and Sources of Zoonoses, Zoonotic Agents and Food-borne Outbreaks in 2013-3547. Available online at: http://www.efsa.europa.eu/sites/default/files/scientific_output/files/main_documents/3991.pdf (Accessed: July 9, 2015)
Elliker, P. R., Anderson, A. W., and Hannesson, G. (1956). An agar culture medium for lactic acid Streptococci and Lactobacilli. J. Dairy Sci. 39, 1611–1612. doi: 10.3168/jds.S0022-0302(56)94896-2
FDA (2016). Use of materials derived from cattle in human food and cosmetics. Fed. Regist. 81, 14718–14732.
Garsa, A. K., Kumariya, R., Sood, S. K., Kumar, A., and Kapila, S. (2014). Bacteriocin production and different strategies for their recovery and purification. Probiot. Antimicrob. Proteins. 6, 47–58. doi: 10.1007/s12602-013-9153-z
Gonzalez-Rodriguez, M. N., Sanz, J. J., Santos, J. A., Otero, A., and Garcia-Lopez, M. L. (2002). Numbers and types of microorganisms in vacuum-packed cold-smoked freshwater fish at the retail level. Int. J. Food Microbiol. 77, 161–168. doi: 10.1016/S0168-1605(02)00048-X
Holzapfel, W. H. (1992). Culture media for non-sporulating Gram-positive food spoilage bacteria. Int. J. Food Microbiol. 17, 113–133. doi: 10.1016/0168-1605(92)90110-O
Huss, H. H., Reilly, A., and Ben Embarek, P. K. (2000). Prevention and control of Hazards in seafood. Food Control 11, 149–156. doi: 10.1016/S0956-7135(99)00087-0
Joffraud, J. J., Leroi, F., and Chevalier, F. (1998). Development of a sterile cold-smoked fish model. J. Appl. Microbiol. 85, 991–998. doi: 10.1111/j.1365-2672.1998.tb05263.x
Jorgensen, L. V., and Huss, H. H. (1998). Prevalence and growth of Listeria monocytogenes in naturally contaminated seafood. Int. J. Food Microbiol. 42, 127–131. doi: 10.1016/S0168-1605(98)00071-3
Katla, T., Moretro, T., Aasen, I. M., Holck, A., Axelsson, L., and Naterstad, K. (2001). Inhibition of Listeria monocytogenes in cold smoked salmon by addition of sakacin P and/or live Lactobacillus sakei cultures. Food Microbiol. 18, 431–439. doi: 10.1016/j.ijfoodmicro.2009.04.004
Krier, F., Revol-Junelles, A. M., and Germain, P. (1998). Influence of temperature and pH production of two bacteriocins by Leuconostoc mesenteroides subsp. mesenteroides FR52 during batch fermentation. Appl. Microbiol. Biotechnol. 50, 359–363. doi: 10.1007/s002530051304
Laursen, B. G., Bay, L., Cleenwerck, I., Vancanneyt, M., Swings, J., Dalgaard, P., et al. (2005). Carnobacterium divergens and Carnobacterium maltaromicum as spoilers or protective cultures in meat and seafood: phenotypic and genotypic characterisation. Syst. Appl. Microbiol. 28, 151–164. doi: 10.1016/j.syapm.2004.12.001
Leal-Sanchez, M. V., Jimenez-Diaz, R., Maldonado-Barragan, A., Garrido-Fernandez, A., and Ruiz-Barba, J. L. (2002). Optimization of bacteriocin production by batch fermentation of Lactobacillus plantarum LPCO10. Appl. Environ. Microbiol. 68, 4465–4471. doi: 10.1128/AEM.68.9.4465-4471.2002
Leroi, F. (2010). Occurrence and role of lactic acid bacteria in seafood products. Food Microbiol. 27, 698–709. doi: 10.1016/j.fm.2010.05.016
Leroi, F., Arbey, N., Joffraud, J. J., and Chevalier, F. (1996). Effect of inoculation with lactic acid bacteria on extending the shelf life of vacuum-packed cold smoked salmon. Int. J. Food Sci. Technol. 31, 497–504. doi: 10.1046/j.1365-2621.1996.00366.x
Leroi, F., Cornet, J., Chevalier, F., Cardinal, M., Coeuret, G., Chaillou, S., et al. (2015). Selection of bioprotective cultures for preventing cold-smoked salmon spoilage. Int. J. Food Microbiol. 213, 79–87. doi: 10.1016/j.ijfoodmicro.2015.05.005
Leroi, F., Joffraud, J. J., Chevalier, F., and Cardinal, M. (1998). Study of the microbial ecology of cold-smoked salmon during storage at 8 degrees C. Int. J. Food Microbiol. 39, 111–121. doi: 10.1016/S0168-1605(97)00126-8
Mataragas, M., Drosinos, E. H., Tsakalidou, E., and Metaxopoulos, J. (2004). Influence of nutrients on growth and bacteriocin production by Leuconostoc mesenteroides L124 and Lactobacillus curvatus L442. Antonie Van Leeuwenhoek 85, 191–198. doi: 10.1023/B:ANTO.0000020291.01957.a2
Mejlholm, O., and Dalgaard, P. (2007). Modeling and predicting the growth of lactic acid bacteria in lightly preserved seafood and their inhibiting effect on Listeria monocytogenes. J. Food Prot. 70, 2485–2497.
Messens, W., Verluyten, J., Leroy, F., and De Vuyst, L. (2003). Modelling growth and bacteriocin production by Lactobacillus curvatus LTH 1174 in response to temperature and pH values used for European sausage fermentation processes. Int. J. Food Microbiol. 81, 41–52. doi: 10.1016/S0168-1605(02)00168-X
Métivier, A., Boyaval, P., Duffes, F., Dousset, X., Compoint, J. P., and Marion, D. (1999). Triton X-114 phase partitioning for the isolation of a pediocin-like bacteriocin from Carnobacterium divergens. Lett. Appl. Microbiol. 30, 42–46. doi: 10.1046/j.1472-765x.2000.00655.x
Métivier, A., Pilet, M. F., Dousset, X., Sorokine, O., Anglade, P., Zagorec, M., et al. (1998). Divercin V41, a new bacteriocin with two disulphide bonds produced by Carnobacterium divergens V41: primary structure and genomic organization. Microbiology 144, 2837–2844. doi: 10.1099/00221287-144-10-2837
Metsoviti, M., Paramithiotis, S., Drosinos, E. H., Skandamis, P. N., Galiotou-Panayotou, M., and Papanikolaou, S. (2011). Biotechnological valorization of low-cost sugar-based media for bacteriocin production by Leuconostoc mesenteroides E131. N. Biotechnol. 28, 600–609. doi: 10.1016/j.nbt.2011.03.004
Neysens, P., Messens, W., and De Vuyst, L. (2003). Effect of sodium chloride on growth and bacteriocin production by Lactobacillus amylovorus DCE 471. Int. J. Food Microbiol. 88, 29–39. doi: 10.1016/S0168-1605(03)00079-5
Pilet, M. F., Dousset, X., Barré, R., Novel, G., Desmazeaud, M., and Piard, J. C. (1995). Evidence for two bacteriocins produced by Carnobacterium piscicola and Carnobacterium divergens isolated from fish and active against Listeria monocytogenes. J. Food Prot. 58, 256–262.
Pilet, M. F., and Leroi, F. (2011). “Applications of protective cultures, bacteriocins and bacteriophages in seafood products,” in Protective Cultures, Antimicrobial Metabolites and Bacteriophages for Food and Beverage Biopreservation, ed L. Christophe (Cambridge: Woodhead Publishing Series in Food Science, Technology and Nutrition), 324–347.
Plackett, R. L., and Burmann, J. P. (1946). The design of optimum multifactorial experiments. Biometrika 33, 305–332. doi: 10.1093/biomet/33.4.305
Polak-Berecka, M., Wasko, A., Kordowska-Wiater, M., Podlesny, M., Targonski, Z., and Kubik-Komar, A. (2010). Optimization of medium composition for enhancing growth of Lactobacillus rhamnosus PEN using response surface methodology. Pol. J. Microbiol. 59, 113–118.
Richard, C., Brillet, A., Pilet, M. F., Prevost, H., and Drider, D. (2003). Evidence on inhibition of Listeria monocytogenes by divercin V41 action. Lett. Appl. Microbiol. 36, 288–292. doi: 10.1046/j.1472-765X.2003.01310.x
Rodgers, S. (2001). Preserving non-fermented refrigerated foods with microbial cultures - a review. Trends Food Sci. Tech. 12, 276–284. doi: 10.1016/S0924-2244(01)00093-0
Ross, R. P., Morgan, S., and Hill, C. (2002). Preservation and fermentation : past, present and future. Int. J. Food Microbiol. 79, 3–16. doi: 10.1016/S0168-1605(02)00174-5
Schobitz, R. P., Borquez, P. A., Costa, M. E., Ciampi, L. R., and Brito, C. S. (2006). Bacteriocin like substance production by Carnobacterium piscicola in a continuous system with three culture broths. Study of antagonism against Listeria monocytogenes on vacuum packaged salmon. Braz. J. Microbiol. 37, 52–57. doi: 10.1590/S1517-83822006000100010
Sumner, J., and Ross, T. (2002). A semi-quantitative seafood safety risk assessment. Int. J. Food Microbiol. 77, 55–59. doi: 10.1016/S0168-1605(02)00062-4
Tahiri, I., Desbiens, M., Kheadr, E., Lacroix, C., and Fliss, I. (2009). Comparison of different application strategies of divergicin M35 for inactivation of Listeria monocytogenes in cold-smoked wild salmon. Food Microbiol. 26, 783–793. doi: 10.1016/j.fm.2009.05.003
Todorov, S. D., and Dicks, L. M. T. (2005). Optimization of bacteriocin ST311LD production by Enterococcus faecium ST311LD, isolated from spoiled black olives. J. Microbiol. 43, 370–374.
Todorov, S. D., and Dicks, L. M. T. (2006). Medium components effecting bacteriocin production by two strains of Lactobacillus plantarum ST414BZ and ST664BZ isolated from boza. Biologia 61, 269–274. doi: 10.2478/s11756-006-0049-1
Todorov, S. D., and Dicks, L. M. T. (2007a). Bacteriocin production by Lactobacillus pentosus ST712BZ isolated from Boza. Braz. J. Microbiol. 38, 166–172. doi: 10.1590/S1517-83822007000100034
Todorov, S. D., and Dicks, L. M. T. (2007b). Partial characterisation of two bacteriocins produced by Lactobacillus paracasei subsp. paracasei ST242BZ and ST284BZ and the effect of medium components on their production. Ann. Microbiol. 57, 363–368. doi: 10.1007/BF03175074
Todorov, S. D., Gotcheva, B., Dousset, X., Onno, B., and Ivanova, I. (2000). Influence of growth medium on bacteriocin production in Lactobacillus plantarum ST31. Biotechnol. Biotechnol. Equip. 14, 50–55. doi: 10.1080/13102818.2000.10819062
von Mollendorff, J. W., Todorov, S. D., and Dicks, L. M. T. (2009). Optimization of growth medium for production of bacteriocins produced by Lactobacillus plantarum JW3BZ and JW6BZ, and Lactobacillus fermentum JW11BZ and JW15BZ isolated from boza. TJS 7, 22–33. Available online at: http://www.uni-sz.bg/tsj/Vol7No1_2009/Todorov1.pdf
Wasney, M. A., Holley, R. A., and Jayas, D. S. (2001). Cresol Red Thallium Acetate Sucrose Inulin (CTSI) agar for the selective recovery of Carnobacterium spp. Int. J. Food Microbiol. 64, 167–174. doi: 10.1016/S0168-1605(00)00464-5
Keywords: optimization, Carnobacterium, growth, bacteriocin, experimental design, bioreactor, cold-smoked salmon
Citation: Brillet-Viel A, Pilet M-F, Courcoux P, Prévost H and Leroi F (2016) Optimization of Growth and Bacteriocin Activity of the Food Bioprotective Carnobacterium divergens V41 in an Animal Origin Protein Free Medium. Front. Mar. Sci. 3:128. doi: 10.3389/fmars.2016.00128
Received: 23 March 2016; Accepted: 07 July 2016;
Published: 03 August 2016.
Edited by:
Ioannis S. Boziaris, University of Thessaly, GreeceReviewed by:
Taoufik Ghrairi, University Tunis-ElManar, TunisiaEleftherios Drosinos, Agricultural University of Athens, Greece
Copyright © 2016 Brillet-Viel, Pilet, Courcoux, Prévost and Leroi. This is an open-access article distributed under the terms of the Creative Commons Attribution License (CC BY). The use, distribution or reproduction in other forums is permitted, provided the original author(s) or licensor are credited and that the original publication in this journal is cited, in accordance with accepted academic practice. No use, distribution or reproduction is permitted which does not comply with these terms.
*Correspondence: Françoise Leroi, Zmxlcm9pQGlmcmVtZXIuZnI=