- 1Murdoch University Cetacean Research Unit, School of Veterinary and Life Sciences, Murdoch University, Perth, WA, Australia
- 2Department of Applied Ecology, North Carolina State University, Raleigh, NC, USA
- 3Marine Science Program, Department of Parks and Wildlife, Perth, WA, Australia
- 4Centre for Marine Futures, School of Animal Biology and Oceans Institute, The University of Western Australia, Crawley, WA, Australia
- 5Evolution and Ecology Research Centre, School of Biological, Earth and Environmental Sciences, University of New South Wales, Sydney, NSW, Australia
- 6Marine Evolution and Ecology, Groningen Institute for Evolutionary Life Sciences, University of Groningen, Groningen, Netherlands
Inherent difficulties in determining the sex of free-ranging, sexually monomorphic species often prevents a sex-specific focus on estimating abundance, movement patterns and survival rates. This study provides insights into sex-specific population parameters of Indo-Pacific bottlenose dolphins (Tursiops aduncus). Systematic, boat-based photo-identification surveys (n = 417) were conducted year-round from 2007 to 2013 in coastal and estuarine waters off Bunbury, Western Australia. Pollock's Robust Design was used to quantify population parameters for three datasets: (i) adults and juveniles combined, (ii) adult females and, (iii) adult males. For all datasets, abundance estimates varied seasonally, with general highs during summer and/or autumn, and lows during winter. Dolphins had seasonally structured temporary emigration rates with similar trends between sexes. The derived return rate (1-γ') of temporary emigrants into the study area was highest from winter to spring, indicating that dolphins had a high probability of return into the study area during spring. We suggest that the return of dolphins into the study area and increase in abundance is influenced by the breeding season (summer/autumn). Prey availability is likely a main driver responsible for the movement of dolphins out of the study area during winter. Seasonal apparent survival rates were constant and high (0.98–0.99) for all datasets. High apparent survival rates suggest there is no permanent emigration from the study area. Our sex-specific modeling approach offers a comprehensive interpretation of the population dynamics of a top predator in a coastal and estuarine environment and acts as a model for future sex-based population studies on sexually monomorphic species.
Introduction
Information on population abundance is ecologically important and integral to conservation and management of wildlife (Wilson et al., 1999; Hammond et al., 2002). Population abundance is often estimated through distance sampling (Buckland et al., 2001) and capture-recapture methods (Williams et al., 2002). The advantage of capture-recapture methods is that they allow for the estimation of other important biological parameters, such as temporary emigration and survival (Kendall and Bjorkland, 2001). Capture-recapture models are most informative when integrated with supplementary information on individuals' sex or age class, thereby allowing for a more holistic interpretation of model outputs (Lebreton et al., 1992; Crespin et al., 2008; Pradel et al., 2008). A sex-based approach to estimating population parameters is important as males and females often vary in their behavioral (social and mating strategies) and ecological (space and habitat use) characteristics (Clutton-Brock, 1989; Whitehead, 1990; van Toor et al., 2011; Sprogis et al., 2016). Hence, sex-based variability in demographic and population parameters can influence population dynamics, population viability, gene-flow, and social behavior (Ruckstuhl and Clutton-Brock, 2005).
Variability between sexes in demographic and population parameters can lead to conservation and management implications, as one sex may be more susceptible to threats than the other (e.g., Crespo et al., 1997; Van Dam et al., 2008; Schofield et al., 2013; Baird et al., 2015). For example, the wandering albatross (Diomedea exulans) exhibits sex-specific differences in foraging areas, which results in a sex-biased human induced mortality (Weimerskirch et al., 1997; Xavier and Croxall, 2005). Specifically, female albatross forage further from the breeding colony than males and, as a consequence, females interact with long-line fisheries more often, resulting in higher levels of bycatch (Xavier et al., 2004). This sex-dependent mortality results in lower survival rates of females, and a subsequent decline in breeding pairs (Xavier and Croxall, 2005). Documentation of whether males and females differ in their abundance, movement patterns and survival rates within a population leads to more comprehensive assessments of potential sex-biased threats and, ultimately, to better-informed conservation measures (Catry et al., 2005; Ruckstuhl and Clutton-Brock, 2005). For many species, however, identifying the sex of free-ranging individuals is often difficult, particularly for sexually monomorphic species (Nichols et al., 2004; Pradel et al., 2008), but also for sexually dimorphic species (Gowans et al., 2000).
Capture-recapture techniques for estimating various population parameters include physical tagging (Baker, 2004), genetic tagging (Palsbøll et al., 1997) or photographic identification (photo-ID) of individuals through their natural markings (Würsig and Jefferson, 1990; Hammond, 2009), including pigmentation patterns (Arzoumanian et al., 2005), pelage marks (Hastings et al., 2008), scars (Gilkinson et al., 2007), and whisker patterns (Anderson et al., 2010). Photo-ID has been widely used across taxonomic groups, such as terrestrial and marine mammals (Kelly, 2001; Langtimm et al., 2004), reptiles (Schofield et al., 2008; Gardiner et al., 2014) and fish (Couturier et al., 2014; Kanive et al., 2015). Photo-ID is considered a largely non-invasive method by which animals are initially “captured” in photographs, and subsequently matched through photographic “recaptures” to create an individual's capture history.
Photo-ID is one of the most commonly used capture-recapture methods for estimating the abundance of cetaceans (Bigg et al., 1990; Hammond, 1990; Würsig and Jefferson, 1990). Natural markings on cetaceans include nicks and notches along the trailing edge of the dorsal fin in bottlenose dolphins (Tursiops spp.; Würsig and Würsig, 1977), color patterns on the ventral surface of the fluke in humpback whales (Megaptera novaeangliae; Katona et al., 1979) and callosity patterns in right whales (Eubalaena australis; Payne, 1986). Photo-ID is most effective when applied to populations with a high proportion of distinctively marked individuals (Hammond, 2009).
Sex-specific population dynamic studies on cetaceans are limited as determining the sex of free ranging individuals is challenging (Morteo et al., 2014; Brown et al., 2016). Some cetacean species are sexually dimorphic in size, but this is not obvious in the field (e.g., the common bottlenose dolpin, Tursiops truncatus, Tolley et al., 1995), or are sexually monomorphic in size, shape and appearance (e.g., the Indo-Pacific bottlenose dolphin, T. aduncus, Hale et al., 2000; Kemper, 2004). The sex of an individual can be documented through several techniques, including molecular sexing (Gilson et al., 1998); laser photogrammetry (Rowe and Dawson, 2009); underwater photography (Webster et al., 2009); opportunistic observations of the genital area (Morteo et al., 2014); or, for females, repeated close association with a calf (Mann, 2000). However, sex determination of a sufficient proportion of individuals within a population to allow for sex-specific capture-recapture analyses requires long-term field effort (Rowe and Dawson, 2009).
Much of our understanding of sex-specific patterns in bottlenose dolphin (Tursiops spp.) social structure and movement ecology arise from long-term studies on T. truncatus in Sarasota Bay, USA (Wells, 2014), and T. aduncus in Shark Bay, Australia (Connor et al., 2000). Bottlenose dolphins exhibit sex-specific bonds, where their fission-fusion societies favor complex social structuring, typically consisting of mother-calf, adult-male, and mixed-sex juvenile groups (Wells et al., 1987; Smolker et al., 1992). Like most mammals, adult female, and adult male bottlenose dolphins have different strategies to optimize their reproductive output and survival. Adult female dolphins invest care into their offspring and their distribution is influenced by ecological parameters that optimize calf survival, such as the distribution of resources (i.e., prey and protected habitat) and threats (i.e., predators and the mating strategies of males; Connor et al., 2000). Male dolphins do not invest in care of their offspring, and their reproductive output is limited by the number of females they successfully monopolize (Connor et al., 1996). As bottlenose dolphins are polygynous, the distribution of males is heavily driven by the spatial and temporal distribution of receptive females (Emlen and Oring, 1977; Connor and Krützen, 2015). To our knowledge, there are currently no peer-reviewed sex-specific abundance estimates or temporary emigration rates reported for bottlenose dolphins. A sex-specific approach would provide an opportunity to explore ecological determinants driving any sex-based trends.
A population of T. aduncus with seasonal changes in abundance, reside in the coastal and estuarine waters off Bunbury, Western Australia (Smith et al., 2013). There are consistently fewer dolphins present during winter and higher numbers during summer and autumn. The increase in dolphin abundance during summer/autumn coincides with the peak-breeding season, and may be explained by an influx of adult males for breeding purposes (Smith et al., 2013). Here, adult males have larger home ranges than females, suggesting differences in movement patterns between the sexes (Sprogis et al., 2016). The aim of this study was to quantify sex-specific abundance, movement patterns and survival rates of T. aduncus across austral seasons to test the hypothesis that there is an influx of males into the study area during summer or autumn months. A sex-specific capture-recapture approach was implemented following Pollock's Robust Design (herein Robust Design; Pollock, 1982), using photo-ID capture histories.
Methods
Study Site
Data were collected year-round from March 2007 to April 2013 off Bunbury (115°63′ E, −33°32′ S), south-western Australia (Figure 1). The Bunbury region typically experiences a Mediterranean climate with cool, wet winters and warm, dry summers. The study site encompassed 120 km2, extending 2 km from shore and 50 km along the coast, and consisted of three transect routes: Buffalo Beach, Back Beach and the Inner waters (Figure 1). The Inner waters consisted of the Leschenault Inlet and Estuary, Inner and Outer Harbor, Koombana Bay and the lower reaches of the Collie River (Figure 1). Water depth ranged from < 1 m in the estuary to ~15 m offshore.
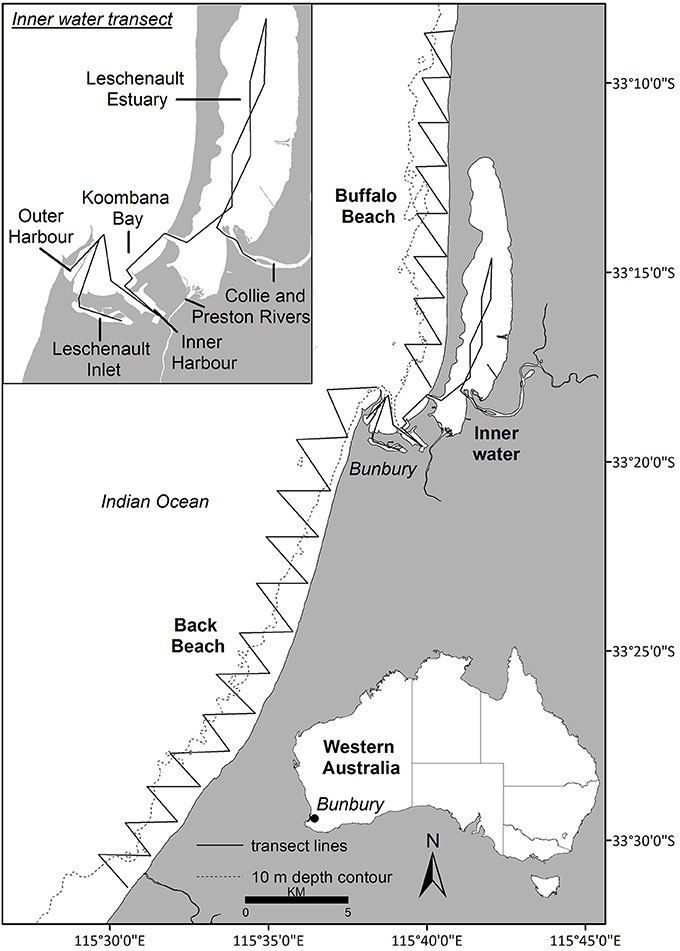
Figure 1. The study site (Bunbury, Western Australia; area = 120 km2) was divided into three transects: Buffalo Beach, Back Beach and the Inner water transect (see insert).
Data Collection
Photo-ID data were obtained during systematic boat-based surveys carried out across austral seasons: summer (December–February), autumn (March–May), winter (June–August), and spring (September–November). A 5 m research vessel with an 80 hp engine was navigated along pre-determined transect routes (Figure 1) at 10 kts. A survey was defined as the traverse of a full transect, with each dolphin group encounter termed a sighting. Surveys were undertaken in weather conditions with Beaufort sea states ≤ 3, and with two to five observers (median of four). While on transect, observers scanned for dolphins out to approximately 250 m on either side of the vessel.
During each sighting, an experienced researcher with a Nikon D300s camera and a 300 or 400 mm lens aimed to capture a photograph of every dolphin's dorsal fin for identification purposes (Würsig and Würsig, 1977). For each group encounter, Global Positioning System (GPS) location, time, group composition, and group size were recorded. A group was defined as one or more dolphins within 100 m of any other member involved in the same or similar behavioral activities (Irvine et al., 1981; Wells et al., 1987; Brager, 1999).
Sex of adult dolphins was determined through molecular analyses from biopsy samples collected as part of an associated research project (Manlik et al., unpublished data1), and/or for adult females, repeated, and consistent observations (>three times) in the presence of a dependent calf (Mann et al., 2000). We classified individuals into three age categories: adult, juvenile and calf. Following published work on bottlenose dolphins (e.g., Mann et al., 2000; Ansmann et al., 2013), adults were >2 m (Hale et al., 2000). It is noted that T. aduncus in Australia are smaller than other Tursiops spp. in other locations and that sizes vary between locations (Connor et al., 2000; Hale et al., 2000). Juveniles were small-bodied compared to adults (approximately two-thirds of adult size) and were not seen consistently beside an adult (Mann et al., 2000). Calves were small (less than two-thirds of adult size) and were consistent with calf length at birth for Tursiops spp. in other populations, and maintained repeated close proximity to their mothers (Whitehead and Mann, 2000).
Data Processing: Photographic-Identification and Photo Grading
Photographic images of each dorsal fin were used to identify dolphins by unique and permanent nicks and notches (Würsig and Würsig, 1977). Recaptures were matched to a fin catalog using ACDSee 12 software (©2010 ACD Systems International Inc.), following the protocols from the Sarasota Dolphin Research Program (i.e., Bassos-Hull et al., 2013). To help ensure correct identification, matching of “recapture” photos of each dolphin was carried out independently by a minimum of two observers.
Photo-ID data from 2007 to 2009, published in Smith et al. (2013), were incorporated into this study and reanalysed by implementing a photo-grading system, consistent with that applied to the 2010–2013 data. Four independent reviewers graded photographs following the protocols from Rosel et al. (2011; Supplementary Material 1). As such, photographs of each individual documented from 2007 to 2013 were graded for image quality and fin distinctiveness in order to minimize heterogeneity in capture probabilities (Stevick et al., 2001; Urian et al., 2015). Image quality affects the likelihood of recognizing an individual, thereby affecting the probability of capture. Fin distinctiveness is also important as some individuals are more recognizable than others, which can result in higher probability of capture of more recognizable individuals (Hammond, 1986). Thus, only good and excellent photographs (Q1 and Q2) and highly or moderately distinctive (D1 and D2) dorsal fins were used in analyses (Supplementary Material 1; Rosel et al., 2011). From these images, capture histories of individual dolphins were created and the subsequent capture-recapture analyses were carried out.
Datasets Used in Analyses
Analyses were run on three different data sets:
(a) Adults and juveniles combined (including individuals for which sex had not been determined; excluding calves as they were dependent);
(b) Adult females; and
(c) Adult males.
Analyses were also conducted on an additional dataset, which included all known adults in the population, including those of unknown sex (see Supplementary Material 2). In the adult male model, 13 adults were included as male despite not having been confirmed as a male either visually or through molecular analyses. This was justified based on strong and long-term associations with adult males of confirmed sex (see Supplementary Material 3).
Robust Design Structure and Model Assumptions
The Robust Design was implemented to estimate abundance, apparent survival and temporary emigration rates (Pollock, 1982; Kendall and Nichols, 1995; Kendall et al., 1997). One advantage of the Robust Design is that it accommodates for temporary emigration of individuals, which is not accounted for in closed (does not allow for emigration) or open models (only allows for permanent emigration; Kendall and Nichols, 1995). The Robust Design incorporates both open and closed population models and is structured to have open sampling events (termed “primary periods”), within which are multiple closed events (termed “secondary periods”). Between primary periods, the population is assumed to be open, allowing for births, deaths and permanent or temporary emigration and immigration. Within primary periods, the population is assumed closed, not allowing for change of any kind. In this study, population was defined as the number of individuals frequenting the study area (Williams et al., 2002). Primary periods were based on austral seasons (i.e., four primary periods per year), while the secondary periods were based on the number of days it took to complete the three transects within the study area (Figure 1). Only dolphin sightings that were collected “on-effort” were included in analyses. One sampling regime goal was to complete secondary periods within the shortest time possible (weather dependent) to satisfy the assumption of instantaneous sampling (see below) where possible.
Robust Design capture-recapture models make the following assumptions: (1) marks are unique, permanent and correctly reported; and for all individuals (including unmarked individuals) there are (2) homogenous capture probabilities between individuals within a sampling event; (3) homogeneous capture and recapture probabilities, i.e., no trap response and no heterogeneity; (4) homogenous survival probabilities; (5) instantaneous sampling for secondary periods; (6) the population is closed within primary periods, and (7) captures are independent between individuals (Pollock, 1982; Pollock et al., 1990; Williams et al., 2002).
To minimize violation of the above assumptions, the following steps were taken: (1) to ensure correct identification, unique and permanent nicks and notches were used to identify dolphins, and images were double-checked by two researchers; (2) to reduce heterogeneity in capture probabilities from fin distinctiveness, only graded photographs of good and excellent quality photographs (Q1 and Q2) and distinctive (D1 and D2) dorsal fins were used in analyses (see Supplementary Material 4 for more details on this assumption); (3) capture and recapture was assumed to be homogenous, as animals were not likely to exhibit behavioral responses while photographs were taken because dolphins were not physically captured or handled (see Parra et al., 2006; Nicholson et al., 2012); (4) to ensure homogenous survival probabilities, sex-specific datasets used adults only in analyses, as survival rate can vary by age (see apparent survival below); (5) to reach instantaneous sampling, secondary periods were completed within the shortest time period possible; (6) to achieve closure, primary periods were structured as seasons rather than years, however this assumption will not be completely satisfied as dolphins in this population temporarily emigrate out of the study area (Smith et al., 2013) and some individuals have larger home ranges than the study area (Sprogis et al., 2016); (7) the model assumption that captures are independent between individuals is most likely violated as dolphin populations are socially driven and the probability of capturing an individual may be increased by capturing its close associates (Connor et al., 2000; Nicholson et al., 2012). This does not bias the estimates, however, but it will inflate the corresponding standard errors (Williams et al., 2002).
Robust Design Analyses
Robust Design analyses were carried out using the program MARK (White and Burnham, 1999). Parameter estimations included: the abundance of animals that are in the study area for each primary period (N), the probability of temporary emigration (γ,” γ'), the probability of apparent survival (ϕ), the probability of first capture (p), and the probability of recapture (c).
Temporary emigration is the probability of being temporarily out of the study area if the individual was present (γ”) or absent (γ') in the previous primary period. Three models of temporary emigration were considered. The first was random temporary emigration, where both temporary emigration parameters were equal (γ” = γ') and there was no underlying sequential structure of movement (Kendall et al., 1997). The second was Markovian emigration, derived from the Markov process, where the probability of an individual being a temporary emigrant in time i was dependent on whether the individual was present/absent in the study area in time i-1 (γ” ≠ γ'; Kendall et al., 1997). Markovian emigration results in a temporally-structured process underlying the movements of individuals (Kendall and Bjorkland, 2001). The influence of temporary emigration for both random and Markovian models was examined as constant over time (.), time varying over primary periods (t) or varying over austral season (season). Constraints were placed on time-varying (ϕ(t)) random and Markovian models (i.e., γ”k = γ”k−1, y'k = γ'k−1), so that all parameters could be identified (Kendall, 2011). The random and Markovian models were tested against the third model; the null model of no temporary emigration (γ” = γ' = 0; Kendall, 2011; Kendall et al., 2012). The return rate (1-γ') of temporary emigrants into the study area from the super-population was also derived. The super-population is defined as the total number of individuals that were present in the study site during the study (Crosbie and Manly, 1985).
Apparent survival (ϕ) rates represent the product of true survival and permanent emigration (i.e., 1-permanent emigration). Survival probabilities can vary by sex and age class (Baker et al., 2010; Stanton and Mann, 2012). However, it is an assumption of the Robust Design that there is equal probability of apparent survival across all individuals (Burnham et al., 1987; Lebreton et al., 1992). Therefore, the sex-specific adult datasets satisfied this model assumption, as only dolphins that remained in the adult age-class throughout the duration of the study were used. However, the adult and juvenile dataset may have violated this assumption. Seasonal apparent survival rates from primary period t to primary period t + 1 were modeled as constant over time (ϕ(.)) and varying by time (ϕ(t)).
Capture (p) and recapture (c) probabilities were assumed to be equal (p = c) as photo-ID methods do not require physical handling of animals and, thus, captures are presumed to not affect recaptures (Boyd et al., 2010). Capture probability was modeled as time varying over primary periods (p = c(t)), since environmental conditions were not constant over the duration of the study. Furthermore, capture probability was modeled as constant within primary periods (p = c(t,.)) or varying by secondary periods within primary periods (p = c(t, s); See Supplementary Material 4 for results and discussion on capture probability).
Overall, a combination of each parameter varying with time, season and constant were used to build models, i.e., 21 different models were run for each dataset: eight time-varying Markovian; seven seasonally dependent Markovian; four random temporary emigration and two no temporary emigration models. The Akaike Information Criterion (AICc) was used for model selection, as this criterion adjusts for small sample sizes. Models with the lowest AICc score were deemed to be most parsimonious (Burnham and Anderson, 2002, 2004).
Estimating the Proportion of Marked Individuals for Abundance Estimates
Abundance estimates relate to the marked (D1 and D2) proportion of the population (). To estimate the total abundance of the population ), estimates were adjusted to account for the proportion of individuals that were unmarked (D3). Sightings in which all individuals were identified from excellent and good quality photographs (Q1 and Q2) were pooled to calculate mark rate (). Mark rate is the total of marked individuals, divided by the total number of individuals, i.e., including unmarked individuals (excluding calves):
Mark rate () was not calculated for the sex-specific models, as the sex of all individuals (marked or unmarked) in a group was rarely known. The adult and juvenile combined mark rate was not applied to the adult sex-specific models, as this could diffuse any adult trends. Juveniles are typically not well marked as dolphins accumulate marks with age, hence, a juvenile mark rate would bias the mark rate of adults downwards (Evans and Hammond, 2004; Urian et al., 2015). Therefore, was reported for the sex-specific models, and not total).
For the most parsimonious model for the combined adult and juvenile dataset, mark rate was calculated by year, where was used to adjust the estimated abundance of the population to report :
Standard errors for the total population size were calculated using the “delta method” (Seber, 1982; Williams et al., 2002):
where is the standard error of the marked population, and n is the total number of individuals from which was estimated (i.e., the cumulative number by year). Log-normal 95% confidence intervals were calculated with upper and lower bounds following Burnham et al. (1987):
Results
Sampling Structure and Survey Effort
In total, 25 primary periods and 139 secondary periods were included in our models (Supplementary Material 5). Secondary periods consisted of 417 surveys (Table 1), including 1310 dolphin group sightings. The time taken to complete secondary periods was weather dependent and ranged from 1 to 21 days ( SE). The mean number of days between consecutive secondary periods ( SE) and between primary periods ( SE) varied due to weather dependent data collection.
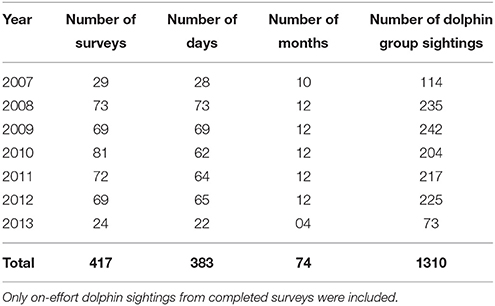
Table 1. Summary of annual survey effort covering the three transects, from March 2007 to April 2013.
Summary Statistics for Model Datasets
In the combined adult and juvenile dataset, a total of 229 dolphins were highly (D1) or moderately (D2) distinctive. Toward the end of the study, only a few new distinctive dolphins were identified (Figure 2). During each secondary period, the number of dolphins that were identified ranged from 6 to 72 ( SE; Supplementary Material 6). The sighting frequency across the duration of the study for individual adult and juvenile dolphins ranged from 1 to 82 ( SE; Figure 3).
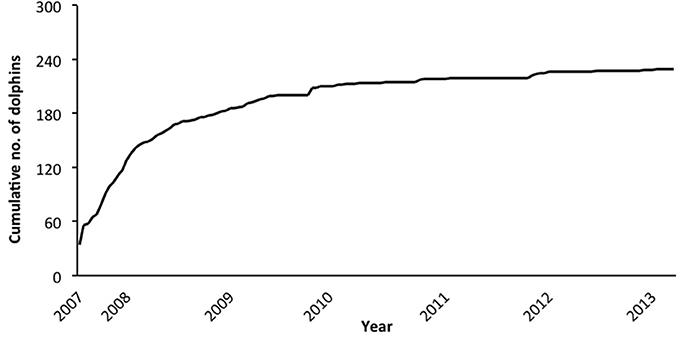
Figure 2. Cumulative discovery curve of the number of adult and juvenile dolphins combined (from excellent and good quality photographs, and highly and moderately distinctive dorsal fins), by secondary period (n = 139) from March 2007 to April 2013.
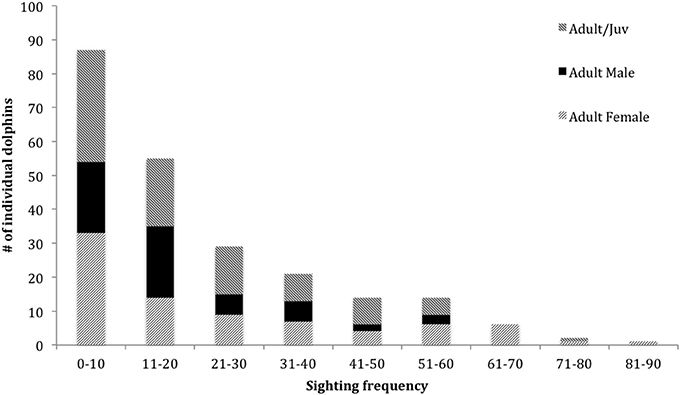
Figure 3. Sighting frequency of dolphins (from excellent and good quality photographs, and highly and moderately distinctive dorsal fins) from March 2007 to April 2013 for adult and juveniles combined (n = 229, 66 males, 97 females, 66 unknown sex), and adult female and adult male datasets (n = 81 and 59, respectively).
In the adult female dataset, there were 81 highly or moderately distinctive dolphins. Of these, 77 were inferred to be female as they had a dependent calf at some stage throughout the study period. Of these 77 individuals, 36 were also confirmed to be females through molecular analyses. Four females were sexed through molecular analyses only (including one stranded dolphin). During each secondary period, the number of female dolphins identified ranged from 3 to 36 ( 13.65 ± 0.48 SE; Supplementary Material 6). The sighting frequency of adult females ranged from 1 to 82 ( SE; Figure 3) across the duration of the entire study. Across all seasons, adult females were sighted more often than adult males (Table 2).

Table 2. The mean number of sightings ±SE for adult female (n = 81) and adult male (n = 59) dolphins pooled by season.
In the adult male dataset, there were 59 highly (D1) or moderately (D2) distinctive dolphins. Of these, 46 were of known sex based on molecular analyses and 13 through documentation of strong bonds in association (see Supplementary Material 3). The number of male dolphins identified during each secondary period ranged from 0 to 23 ( SE; Supplementary Material 6). The sighting frequency of adult males ranged from 1 to 52 ( SE; Figure 3) across the duration of the entire study.
Robust Design Abundance Estimates
The best-fitting model, based on the lowest AICc score, for the combined adult and juvenile dataset was ϕ(.)γ”(t)≠γ'(t)p = c(t,s) with constant survival, time varying Markovian temporary emigration and time varying capture probabilities within primary periods (Table 3; Supplementary Material 7). By contrast, the best-fitting models for the adult female and adult male datasets had seasonally dependent Markovian temporary emigration, ϕ(.)γ”(season) ≠ γ'(season)p = c(t,s) (Table 3; Supplementary Material 7).
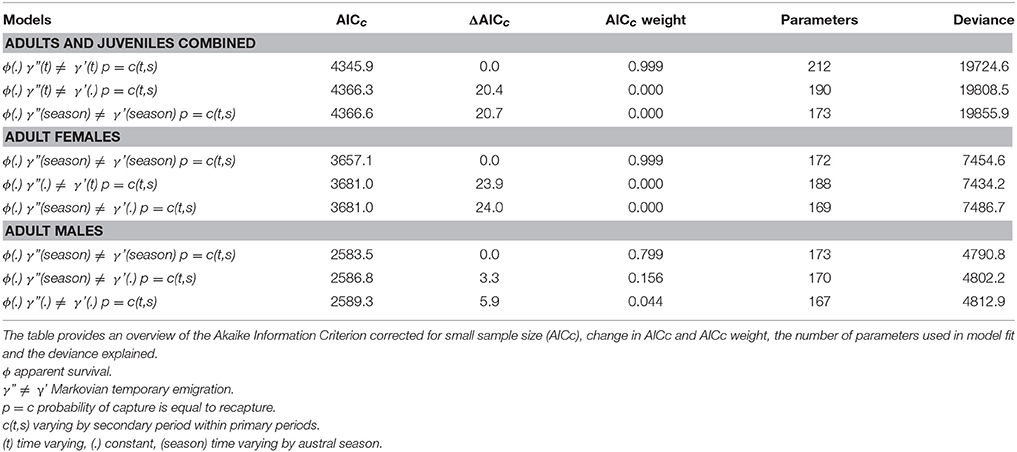
Table 3. The top three Robust Design models, in rank order of AICc scores, for adults and juveniles combined, adult females, and adult males.
Mark rate for the combined adult and juvenile dataset varied by year, with a low of 0.80 in 2010 and a high of 0.90 in 2008 (Supplementary Material 8). The total number of adults and juveniles combined () varied by season, with an unprecedented low in winter 2009 (76.23 ± 7.32 SE; 95% CI 67.99-85.48), and high in summer 2010 (184.78 ± 4.46 SE; 95% CI 170.97–199.70; Figure 4; Supplementary Material 8).
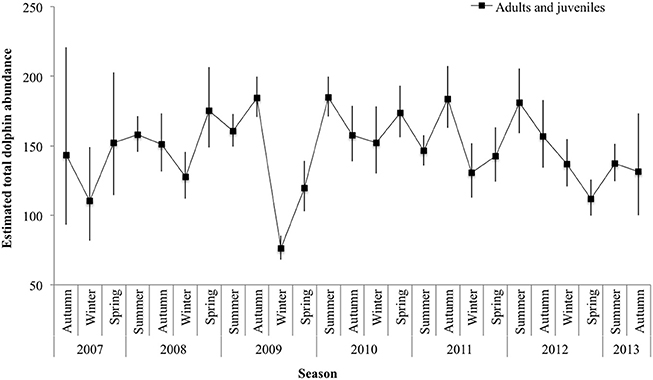
Figure 4. Seasonal abundance estimates () for adult and juvenile dolphins combined. Lines between data points of the seasonal mean have been used for illustrative purposes only; continuity of values is not implied. Vertical lines show 95% confidence intervals.
The estimated number of marked () adult females and males varied between sexes and by season. Abundance estimates for both sexes were, on average, lowest during winter and spring and highest during summer and autumn. For adult females, estimates were consistent among years for spring, apart from an outlier in 2012 with a low of 26.57 (±1.24 SE; 95% CI 26.04–33.58). There was also a low in winter 2009 (27.79 ± 2.99 SE; 95% CI 24.97–38.84). The highest estimate was in autumn 2009 (59.56 ± 2.38 SE; 95% CI 57.08–67.70; Figure 5).
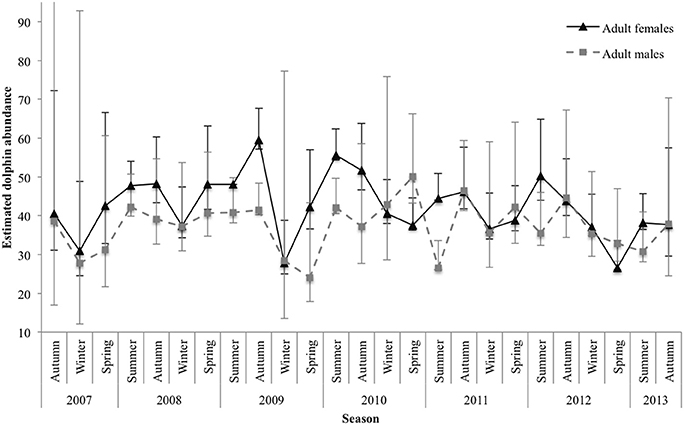
Figure 5. Seasonal abundance estimates for marked () adult female and adult male dolphins. Lines between data points of the seasonal mean have been used for illustrative purposes only; continuity of values is not implied. Vertical lines represent 95% confidence intervals. The upper interval for the initial estimate of adult males was truncated (upper 95% CI 142.42) for display purposes.
For adult males, abundance estimates were quite consistent for autumn; however, they were variable among years for the remaining seasons. Spring had the largest variance in estimated abundance among years, with a low in 2009 ( SE; 95% CI 17.88–43.26) and a high in 2010 ( SE; 95% CI 43.24–66.24; Figure 5).
Temporary Emigration Patterns
Markovian models performed better than both random temporary emigration and no-movement models (Table 4). Movement for the adult and juvenile dataset had time-varying temporary emigration, whereas adult females and adult males had seasonally dependent temporary emigration. Values for temporary emigration in and out of the study area were similar across datasets. The probability of a dolphin being out of the study area if the individual was absent (γ') in the previous primary period was higher than if the individual was present (γ”; Table 4). This implied that dolphins temporarily emigrated out of the study area, but subsequently returned.
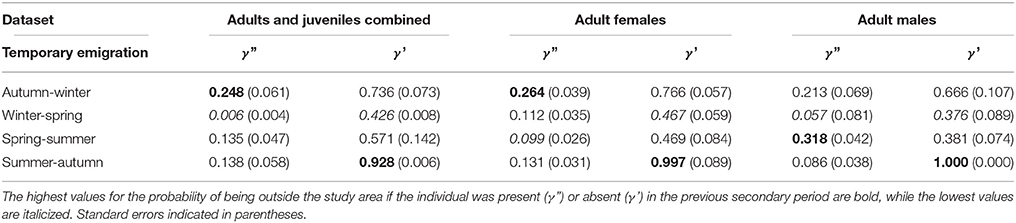
Table 4. Temporary emigration for three datasets: adults and juveniles combined (mean of time varying values), adult females, and adult males.
For γ', there was a peak from summer to autumn across all datasets (Table 4). Adult male γ' values were lower than adult female values across seasons, apart from summer to autumn. The derived return rate (1-γ') of temporary emigrants into the study area from the super-population was highest from winter to spring for all datasets (adult and juvenile = 0.57, adult females = 0.53, adult males = 0.62), indicating that dolphins had a high probability of return into the study area during spring.
For γ,” there was variability in the lows and highs among datasets (Table 4). For the adult and juvenile population, on average γ” was lowest from winter to spring (Table 4). Further, γ” had a peak from autumn to winter in 2009 of 0.57 (±0.05 SE), implying that dolphins present in the study area were temporary emigrants during winter, corresponding with an unprecedented decline in abundance (Figure 5). For adult females, γ” was generally low from spring to summer (0.099) and high from autumn to winter (0.264). For adult males, γ” was low from winter to spring (0.057) and high from spring to summer (0.318). Adult male γ” values were lower than those for adult females across seasons, except from spring to summer.
Survival Rates
Apparent survival rates were close to 1 at 0.99 (±0.002 SE, 95% CI 0.98–0.99), 0.98 (±0.004 SE, 95% CI 0.97–0.99), and 0.99 (±0.003 SE, 95% CI 0.98–0.10) for adults and juveniles combined, adult females and adult males, respectively. These values strongly suggests there is no permanent emigration in this population and true survival is very high.
Discussion
Abundance Estimates and Temporary Emigration Rates Varied Among Seasons
We documented seasonal fluctuations in dolphin abundance, with estimates generally lower in winter and higher in summer or autumn. The results derived from datasets using 3 years (2007–2009, Smith et al., 2013) and 6 years (March 2007–March 2013, this study), showed similar seasonal trends with comparable values. Smith et al. (2013) reported abundance estimates for adult and juveniles combined, and reported a low in dolphin abundance during winter 2007 of 63 (95% CI 59–73) and a high in autumn 2009 of 139 (95% CI 134–148). This study, documented a low of adult and juveniles in winter 2009 of 76 (95% CI 68–85) and a high in summer 2010 of 185 (95% CI 171–200). Variations in abundance among seasons and years could be a reflection of both intrinsic (dolphin biology and social dynamics) and extrinsic factors (environmental factors and prey availability).
Seasonal differences in abundance have also been reported in several T. truncatus populations. For example, across Florida, there is an increase in abundance during summer in Sarasota (Fazioli et al., 2006), an increase during winter in the Indian River Lagoon (Durden et al., 2011), and an increase in spring and autumn in St. Joseph Bay (Balmer et al., 2008). Conversely, there are no seasonal differences evident for T. spp. in Doubtful Sound, New Zealand (Williams et al., 1993), San Diego, USA (Defran and Weller, 1999) and Perth, Western Australia (Chabanne et al., 2012). Thus, the large degree of interpopulation variation in seasonal abundance patterns within species highlights the importance of seasonal sampling.
Temporary emigration for adult and juvenile, adult female and adult male dolphins followed a structured pattern of movement (Markovian). These results are consistent with findings by Smith et al. (2013), who reported seasonally structured movement patterns. Furthermore, the probability of an individual dolphin being out of the study area if the individual was absent in the previous primary period was higher than if the individual was present. This implies that dolphins temporarily emigrated out of the study area and subsequently returned. The derived return rate (1-γ') of temporary emigrants from the super-population was highest from winter to spring, indicating that dolphins had a high probability of return into the study area during spring. Some dolphins were most likely temporary emigrants to the study area (120 km2) due to having larger home ranges (i.e. adult male home ranges reported up to 180 km2; Sprogis et al., 2016) and/or because individual home ranges did not overlap entirely with our study area (Pollock et al., 1990). Therefore, it is likely that several dolphins moved in and out of the study area from the super-population, highlighting the need for temporary emigration to be considered during capture-recapture modeling.
Biological Factors Affecting Fluctuations in Seasonal Abundance
The seasonally dependent abundances can be partly explained by the social dynamics of bottlenose dolphins, including their breeding patterns and mating strategies. Bottlenose dolphins have a seasonal breeding pattern, are seasonally polyestrous and have a 12-month gestation period (Connor et al., 2000; Mann et al., 2000). In Bunbury, the peak breeding and calving season is late summer/early autumn (Smith et al., 2016), coinciding with higher dolphin numbers and sighting frequencies, and a return of dolphins into the study area. Compared with females, males form stronger, and more stable bonds (Supplementary Material 3). Such bonds between males are formed to cooperatively gain access to females in order to increase mating success in some populations (Connor and Krützen, 2015). Smith et al. (2013) suggested that the higher abundance of dolphins during summer and autumn may be a result of an influx of adult males for breeding purposes. However, our results do not support this hypothesis. We documented an increase in abundance for adults of both sexes during summer and autumn, with no relative increase in the abundance of males compared to females. There were differences in abundance estimates between sexes that varied across seasons and years, however, confidence intervals were generally overlapping. Furthermore, sighting frequencies of males and females were both relatively high during summer and autumn. However, interestingly, sighting frequencies and capture probabilities for males were lower than for females (see Supplementary Material 4 for discussion on capture probabilities). Therefore, if there was an influx of males, we would have expected to see higher temporary emigration rates of males into the study area compared to females, however, the values were similar for the two sexes. Overall, it is apparent that the higher abundance estimates during summer/autumn are due to an influx of both males and females, which are likely driven by their breeding patterns and prey availability (see below).
Environmental Factors Affecting Fluctuations in Seasonal Abundance
Dolphin abundance was generally lower during winter compared to other seasons. Consistent with T. truncatus studies in the USA, similar movements out of study areas occur in winter, most likely in response to fluctuations in prey availability (e.g., Maze and Würsig, 1999; Fazioli et al., 2006; Speakman et al., 2010). Elsewhere, seasonal dolphin movement patterns are also typically linked to prey availability (e.g., Similä et al., 1996; Degrati et al., 2012, 2013). In Bunbury, “potential dolphin prey” in the Leschenault Estuary, Koombana Bay, and near-shore coastal waters has been studied for seasonal abundance, biomass and calorific content (McCluskey et al., accepted). Using three types of fishing gear (seine nets, traps, and gill nets), McCluskey et al. (accepted) documented a decrease in abundance and biomass of prey during winters compared to summers. Additionally, dolphins have been observed >10 km offshore during winter, and not during summer (Sprogis, 2015). Therefore, it is likely that some dolphins temporarily move out of the study area during winter in search of adequate prey resources.
Dolphin movements offshore during winter may be associated with the Leeuwin Current, which flows off the Western Australian coast and transports low salinity waters pole-wards (Godfrey and Ridgway, 1985). There are strong seasonal differences in the Leeuwin Current, in which stronger winter currents flood the continental shelf (Pearce and Phillips, 1988; Cresswell and Griffin, 2004). During this time, primary productivity is enhanced through the entrainment of nutrient rich shelf waters and the formation of eddies (Hanson et al., 2005; Koslow et al., 2008). Ultimately, the strength of the current influences the recruitment and distribution of fish species (Lenanton et al., 1991; Caputi et al., 1996). Further investigation into offshore dolphin movements and prey availability are necessary to examine the extent of dolphin movements, and if resource use (e.g., diet and habitat use) differs between sexes (i.e., Rossman et al., 2015).
Survival Rates Were High and Constant
The most parsimonious models estimated constant and high apparent survival rates for adult and juveniles combined, adult females and adult males. High survival rates suggest low mortality and no permanent emigration of individuals. Similarly, other T. aduncus populations have low permanent emigration (e.g., Nicholson et al., 2012), most likely as a result of natal site philopatry (Krützen et al., 2004; Tsai and Mann, 2013). If permanent emigration approaches zero, apparent survival can be represented as true survival. In Bunbury, high survival rates and little permanent emigration are also supported by individual capture histories that spanned the duration of the study.
High and constant survival rates are common for long-lived species with slow growth rates and low fecundity (k-selected species; Gaillard et al., 1998), for example in other cetacean species (e.g., Ramp et al., 2010; Cantor et al., 2012; Tyne et al., 2014). Consistent with this general trend and the findings from our study, relatively high survival rates have also been shown in other T. aduncus populations (e.g., Reisinger and Karczmarski, 2010; Mansur et al., 2012; Webster et al., 2014). Our research provides an example for estimating sex-specific survival rates, which is especially important to determine for threatened populations (e.g., Baker et al., 2013) and for populations with sex-biased threats (e.g., Baird et al., 2015).
Concluding Remarks
This study provides insights into sex-specific population parameters of Indo-Pacific bottlenose dolphins in a coastal and estuarine environment. The results highlight that (i) abundance estimates were seasonally dependent and there was an increase of both sexes into the study area during summer and autumn, (ii) temporary emigration rates were seasonal and showed similar patterns between the sexes, (iii) survival was constant and high for both males and females, suggesting little or no permanent emigration from the study area. We suggest the peak breeding season (summer/autumn) motivates the return of dolphins into the study area, influencing the increase in abundance. Prey availability is likely a main reason dolphins move out of the study area during winter/spring (also see McCluskey et al., accepted). Our sex-specific modeling approach allowed for a holistic interpretation of the population dynamics of an apex marine predator in a coastal and estuarine environment.
Ethical Standards
This study was carried out with approval from the Murdoch University Animal Ethics committee (W2009/06, W2342/10) and was licensed by the Department of Parks and Wildlife (SF005811, SF007986, and SF008624). Biopsy sampling for molecular analyses were carried out as a part of broader study, with data collected in accordance to the Murdoch University Animal Ethics Committee approval (W2076/07; W2307/10; W2342/10), and collected under research permits (SF005997; SF006538; SF007046; SF007596; SF008480; SF009119) licensed by Department of Parks and Wildlife.
Author Contributions
KS, LB, and KP conceived the study. HR and KS implemented the fieldwork, data organization and data processing. SA collected biopsy samples as a part of a broader study. AK and OM processed biopsy samples and determined the sex of biopsied dolphins. JT assisted with data file processing. KS ran the abundance models and analyses. KS led the writing, with input from LB and KP. All authors critically reviewed the manuscript with final proofs.
Funding
We thank the funding partners for financial support from the South West Marine Research Program (SWMRP); Bemax Cable Sands, BHP Billiton Worsley Alumina Ltd, the Bunbury Dolphin Discovery Centre, Bunbury Port Authority, City of Bunbury, Cristal Mining, the Western Australian Department of Parks and Wildlife, Iluka, Millard Marine, Naturaliste Charters, Newmont Boddington Gold, South West Development Commission and WA Plantation Resources. KS was supported throughout her PhD by an Australian Postgraduate Award, Murdoch University Research Excellence Scholarship and a SWMRP Scholarship.
Conflict of Interest Statement
The authors declare that the research was conducted in the absence of any commercial or financial relationships that could be construed as a potential conflict of interest.
Acknowledgments
We thank numerous volunteers and research assistants who helped with fieldwork and data processing. Thank you to our research associates for collecting field data and/or data management; M. Cannon, D. Chabanne, V. Buchanan, K. Nicholson, and B. Goguelat. We are grateful to M. Krützen, D. McElligott, and A. Sellas, who obtained some of the biopsies for genetic analyses. We thank C. Daniel for sexing results from molecular analyses. We are grateful to R. Wells, G. Parra, R. Constantine, B. Würsig, K. Nicholson, and A. Friedlaender and G. Schofield, whose comments greatly improved this manuscript.
Supplementary Material
The Supplementary Material for this article can be found online at: http://journal.frontiersin.org/article/10.3389/fmars.2016.00012
Footnotes
1. ^Manlik, Oliver, et al., Evolution and Ecology Research Centre, University of New South Wales, Kensington, Australia, unpublished data.
References
Anderson, C. J. R., Lobo, N. D. V., Roth, J. D., and Waterman, J. M. (2010). Computer-aided photo-identification system with an application to polar bears based on whisker spot patterns. J. Mammal. 91, 1350–1359. doi: 10.1644/09-MAMM-A-425.1
Ansmann, I. C., Lanyon, J. M., Seddon, J. M., and Parra, G. J. (2013). Monitoring dolphins in an urban marine system: total and effective population size estimates of Indo-Pacific bottlenose dolphins in Moreton Bay, Australia. PLoS ONE 8:e65239. doi: 10.1371/journal.pone.0065239
Arzoumanian, Z., Holmberg, J., and Norman, B. (2005). An astronomical pattern-matching algorithm for computer-aided identification of whale sharks Rhincodon typus. J. Appl. Ecol. 42, 999–1011. doi: 10.1111/j.1365-2664.2005.01117.x
Baird, R. W., Mahaffy, S. D., Gorgone, A. M., Cullins, T., McSweeney, D. J., Oleson, E. M., et al. (2015). False killer whales and fisheries interactions in Hawaiian waters: evidence for sex bias and variation among populations and social groups. Mar. Mamm. Sci. 31, 579–590. doi: 10.1111/mms.12177
Baker, C. S., Hamner, R. M., Cooke, J., Heimeier, D., Vant, M., Steel, D., et al. (2013). Low abundance and probable decline of the critically endangered Maui's dolphin estimated by genotype capture-recapture. Anim. Conserv. 16, 224–233. doi: 10.1111/j.1469-1795.2012.00590.x
Baker, J. D. (2004). Evaluation of closed capture-recapture methods to estimate abundance of Hawaiian monk seals. Ecol. Appl. 14, 987–998. doi: 10.1890/03-5121
Baker, J. D., Westgate, A., and Eguchi, T. (2010). “Vital rates and population dynamics,” in Marine Mammal Ecology and Conservation: A Handbook of Techniques, eds I. L. Boyd, W. D. Bowen, and S. J. Iverson (Oxford: Oxford University Press), 119–143.
Balmer, B. C., Wells, R. S., Nowacer, S. M., Nowacek, D. P., Schwacke, L. H., McLellan, W. A., et al. (2008). Seasonal abundance and distribution patterns of common bottlenose dolphins (Tursiops truncatus) near St. Joseph Bay, Florida, USA. J. Cetac. Res. Manag. 10, 157–167.
Bassos-Hull, K., Perrtree, R. M., Shepard, C. C., Schilling, S., Barleycorn, A. A., Allen, J. B., et al. (2013). Long-term site fidelity and seasonal abundance estimates of common bottlenose dolphins (Tursiops truncatus) along the southwest coast of Florida and responses to natural perturbations. J. Cetac. Res. Manag. 13, 19–30.
Bigg, M. A., Olesiuk, P. F., Ellis, G. M., Ford, J. K. B., and Balcomb, K. C. I. (1990). “Social organization and genealogy of resident killer whales Orcinus orca in the coastal waters of British Columbia canada and Washington state USA,” in Report of the International Whaling Commission Special Issue, eds P. S. Hammond, S. A. Mizroch, and G. P. Donovan (Cambridge: The International Whaling Commission), 383–399.
Boyd, I. L., Don Bowen, W., and Iverson, S. J. (2010). Marine Mammal Ecology and Conservation. New York, NY: Oxford University Press.
Brager, S. (1999). Association patterns in three populations of Hector's Dolphin, Cephalorhynchus Hectori. Can. J. Zool. 77, 13–18. doi: 10.1139/cjz-77-1-13
Brown, A. M., Bejder, L., Parra, G. J., Cagnazzi, D., Hunt, T., Smith, J. L., et al. (2016). “Sexual dimorphism and geographic variation in dorsal fin features of Australian humpback dolphins, Sousa sahulensis,” in Advances in Marine Biology, eds A. J. Thomas and E. C. Barbara (Academic Press), 273–314. doi: 10.1016/bs.amb.2015.08.002
Buckland, S. T., Anderson, D. R., Burnham, K. P., Laake, J. L., Borchers, D. L., and Thomas, L. (2001). Introduction to Distance Sampling: Estimating Abundance of Biological Populations. Oxford: Oxford University Press.
Burnham, K. P., and Anderson, D. R. (2002). Model Selection and Multi-Model Inference: a Practical Information-Theoretic Approach. New York, NY: Springer.
Burnham, K. P., and Anderson, D. R. (2004). Multimodel inference - understanding AIC and BIC in model selection. Sociol. Methods Res. 33, 261–304. doi: 10.1177/0049124104268644
Burnham, K. P., Anderson, D. R., White, G. C., Brownie, C., and Pollock, K. H. (1987). Design and Analysis Methods for Fish Survival Experiments Based on Release-Recapture. Bethesda, MD: Amer Fisheries Society.
Cantor, M., Wedekin, L. L., Daura-Jorge, F. G., Rossi-Santos, M. R., and Simoes-Lopes, P. C. (2012). Assessing population parameters and trends of Guiana dolphins (Sotalia guianensis): an eight-year mark-recapture study. Mar. Mamm. Sci. 28, 63–83. doi: 10.1111/j.1748-7692.2010.00456.x
Caputi, N., Fletcher, W. J., Pearce, A., and Chubb, C. F. (1996). Effect of the Leeuwin Current on the recruitment of fish and invertebrates along the Western Australian coast. Marine Freshw. Res. 47, 147–155. doi: 10.1071/MF9960147
Catry, P., Phillips, R. A., and Croxall, J. P. (2005). “Sexual segregation in birds: patterns, processes and implications for conservation,” in Sexual Segregation in Vertebrates: Ecology of the Two Sexes, eds K. E. Ruckstuhl and P. Neuhaus. (New York, NY: Cambridge University Press), 351–378.
Chabanne, D., Finn, H., Salgado-Kent, C., and Bejder, L. (2012). Identification of a resident community of bottlenose dolphins (Tursiops spp.) in the Swan-Canning Estuary, Western Australia, using behavioural information. Pacific Conserv. Biol. 18, 247–262.
Clutton-Brock, T. H. (1989). Mammalian mating systems. Proc. R. Soc. B Biol. Sci. 236, 339–372. doi: 10.1098/rspb.1989.0027
Connor, R. C., and Krützen, M. (2015). Male dolphin alliances in Shark Bay: changing perspectives in a 30-year study. Anim. Behav. 103, 223–235. doi: 10.1016/j.anbehav.2015.02.019
Connor, R. C., Richards, A. F., Smolker, R. A., and Mann, J. (1996). Patterns of female attractiveness in Indian Ocean bottlenose dolphins. Behaviour 133, 37–69. doi: 10.1163/156853996X00026
Connor, R. C., Wells, R. S., Mann, J., and Read, A. J. (2000). “The bottlenose dolphin: social relationships in a fission-fusion society,” in Cetacean Societies: Field Sudies of Dolphins and Whales, eds. J. Mann, R. C. Connor, P. L. Tyack, and H. Whitehead (Chicago, IL: The University of Chicago Press), 91–126.
Couturier, L. I. E., Dudgeon, C. L., Pollock, K. H., Jaine, F. R. A., Bennett, M. B., Townsend, K. A., et al. (2014). Population dynamics of the reef manta ray Manta alfredi in eastern Australia. Coral Reefs 33, 329–342. doi: 10.1007/s00338-014-1126-5
Crespin, L., Choquet, R., Lima, M., Merritt, J., and Pradel, R. (2008). Is heterogeneity of catchability in capture-recapture studies a mere sampling artifact or a biologically relevant feature of the population? Popul. Ecol. 50, 247–256. doi: 10.1007/s10144-008-0090-8
Crespo, E. A., Pedraza, S. N., Dans, S. L., Alonso, M. K., Reyes, L. M., Garcia, N. A., et al. (1997). Direct and indirect effects of the highseas fisheries on the marine mammal populations in the northern and central Patagonian coast. J. Northw. Atlant. Fish. Sci. 189–207. doi: 10.2960/J.v22.a15
Cresswell, G. R., and Griffin, D. A. (2004). The Leeuwin Current, eddies and sub-Antarctic waters off south-western Australia. Mar. Freshw. Res. 55, 267–276. doi: 10.1071/MF03115
Crosbie, S. F., and Manly, B. F. J. (1985). Parsimonious modelling of capture-mark-recapture Studies. Biometrics 41, 385–398. doi: 10.2307/2530864
Defran, R. H., and Weller, D. W. (1999). Occurrence, distribution, site fidelity, and school size of bottlenose dolphins (Tursiops truncatus) off San Diego, California. Mar. Mamm. Sci. 15, 366–380. doi: 10.1111/j.1748-7692.1999.tb00807.x
Degrati, M., Dans, S. L., Garaffo, G. V., Cabreira, A. G., Castro Machado, F., and Crespo, E. A. (2013). Sequential foraging of dusky dolphins with an inspection of their prey distribution. Mar. Mamm. Sci. 29, 691–704. doi: 10.1111/j.1748-7692.2012.00615.x
Degrati, M., Dans, S. L., Garaffo, G. V., and Crespo, E. A. (2012). Diving for food: a switch of foraging strategy of dusky dolphins in Argentina. J. Ethol. 30, 361–367. doi: 10.1007/s10164-012-0333-1
Durden, W. N., Stolen, E. D., and Stolen, M. K. (2011). Abundance, distribution, and group composition of Indian River Lagoon bottlenose dolphins (Tursiops truncatus). Aquat. Mam. 37, 175–186. doi: 10.1578/AM.37.2.2011.175
Emlen, S. T., and Oring, L. W. (1977). Ecology, sexual selection, and evolution of mating systems. Science 197, 215–223. doi: 10.1126/science.327542
Evans, P. G. H., and Hammond, P. S. (2004). Monitoring cetaceans in European waters. Mamm. Rev. 34, 131–156. doi: 10.1046/j.0305-1838.2003.00027.x
Fazioli, K. L., Hofmann, S., and Wells, R. S. (2006). Use of gulf of Mexico coastal waters by distinct assemblages of bottlenose dolphins (Tursiops truncatus). Aquat. Mam. 32, 212–222. doi: 10.1578/AM.32.2.2006.212
Gaillard, J. M., Festa-Bianchet, M., and Yoccoz, N. G. (1998). Population dynamics of large herbivores: variable recruitment with constant adult survival. Trends Ecol. Evol. 13, 58–63. doi: 10.1016/S0169-5347(97)01237-8
Gardiner, R. Z., Doran, E., Strickland, K., Carpenter-Bundhoo, L., and Frère, C. (2014). A face in the crowd: a non-invasive and cost effective photo-identification methodology to understand the fine scale movement of eastern water dragons. PLoS ONE 9:e96992. doi: 10.1371/journal.pone.0096992
Gilkinson, A. K., Pearson, H. C., Weltz, F., and Davis, R. W. (2007). Photo-identification of sea otters using nose scars. J. Wildl. Manag. 71, 2045–2051. doi: 10.2193/2006-410
Gilson, A., Syvanen, M., Levine, K., and Banks, J. (1998). Deer gender determination by polymerase chain reaction: validation study and application to tissues, bloodstains, and hair forensic samples from California. Calif. Fish Game 84, 159–169.
Godfrey, J. S., and Ridgway, K. R. (1985). The large-scale environment of the poleward-flowing Leeuwin Current, Western Australia - longshore steric height gradients, wind stresses and geostrophic flow. J. Phys. Oceanogr. 15, 481–495.
Gowans, S., Dalebout, M. L., Hooker, S. K., and Whitehead, H. (2000). Reliability of photographic and molecular techniques for sexing northern bottlenose whales (Hyperoodon ampullatus). Can. J. Zool. 78, 1224–1229. doi: 10.1139/z00-062
Hale, P. T., Barreto, A. S., and Ross, G. J. B. (2000). Comparative morphology and distribution of the aduncus and truncatus forms of bottlenose dolphin Tursiops in the Indian and Western Pacific Oceans. Aquat. Mam. 26, 101–110.
Hammond, P. S. (1986). Estimating the size of natural marked whale populations. Int. Whal. Comm. 253–282.
Hammond, P. S. (1990). Capturing whales on film - estimating cetacean population parameters from individual recognition data. Mamm. Rev. 20, 17–22. doi: 10.1111/j.1365-2907.1990.tb00099.x
Hammond, P. S. (2009). “Mark–recapture,” in Encyclopedia of Marine Mammals, 2nd Edn., eds J. G. M. Thewissen, W. F. Perrin, and B. Würsig. (London: Academic Press), 705–709.
Hammond, P. S., Berggren, P., Benke, H., Borchers, D. L., Collet, A., Heide-Jorgensen, M. P., et al. (2002). Abundance of harbour porpoise and other cetaceans in the North Sea and adjacent waters. J. Appl. Ecol. 39, 361–376. doi: 10.1046/j.1365-2664.2002.00713.x
Hanson, C. E., Pattiaratchi, C. B., and Waite, A. M. (2005). Seasonal production regimes off south-western Australia: influence of the Capes and Leeuwin Currents on phytoplankton dynamics. Mari. Freshw. Res. 56, 1011–1026. doi: 10.1071/MF04288
Hastings, K. K., Hiby, L. A., and Small, R. J. (2008). Evaluation of a computer-assisted photograph-matching system to monitor naturally marked harbor seals at Tugidak Island, Alaska. J. Mammal. 89, 1201–1211. doi: 10.1644/07-MAMM-A-151.1
Irvine, A. B., Scott, M. D., Wells, R. S., and Kaufmann, J. H. (1981). Movements and activities of the Atlantic bottlenose dolphin (Tursiops truncatus) near Sarasota Florida USA. Fish. Bull. 79, 671–688.
Kanive, P. E., Rotella, J. J., Jorgensen, S. J., Chapple, T. K., Anderson, S. D., Klimley, A. P., et al. (2015). Estimating apparent survival of sub-adult and adult white sharks (Carcharodon carcharias) in central California using mark-recapture methods. Front. Mar. Sci. 2:19. doi: 10.3389/fmars.2015.00019
Katona, S., Baxter, B., Brazier, O., Kraus, S., Perkins, J., and Whitehead, H. (1979). Identification of humpback whales by fluke photographs. Behav. Mar. Anim. 3, 33–44. doi: 10.1007/978-1-4684-2985-5_2
Kelly, M. J. (2001). Computer-aided photograph matching in studies using individual identification: An example from Serengeti cheetahs. J. Mammal. 82, 440–449. doi: 10.1644/1545-1542(2001)082 < 0440:CAPMIS>2.0.CO;2
Kemper, C. M. (2004). Osteological variation and taxonomic affinities of bottlenose dolphins, Tursiops spp., from South Australia. Aust. J. Zool. 52, 29–48. doi: 10.1071/ZO03026
Kendall, W. L. (2011). “The robust design,” in Program MARK: A Gentle Introduction, ed E. W. Cooch, 1–50. Available online at: http://www.phidot.org/software/mark/docs/book/
Kendall, W. L., and Bjorkland, R. (2001). Using open robust design models to estimate temporary emigration from capture-recapture data. Biometrics 57, 1113–1122. doi: 10.1111/j.0006-341X.2001.01113.x
Kendall, W. L., and Nichols, J. D. (1995). On the use of secondary capture-recapture samples to estimate temporary emigration and breeding proportions. J. Appl. Stat. 22, 751–762. doi: 10.1080/02664769524595
Kendall, W. L., Nichols, J. D., and Hines, J. E. (1997). Estimating temporary emigration using capture-recapture data with Pollock's robust design. Ecology 78, 563–578.
Kendall, W. L., White, G. C., Hines, J. E., Langtimm, C. A., and Yoshizaki, J. (2012). Estimating parameters of hidden Markov models based on marked individuals: use of robust design data. Ecology 93, 913–920. doi: 10.1890/11-1538.1
Koslow, J. A., Pesant, S., Feng, M., Pearce, A., Fearns, P., Moore, T., et al. (2008). The effect of the Leeuwin Current on phytoplankton biomass and production off Southwestern Australia. J. Geophys. Res. 113:C07050. doi: 10.1029/2007JC004102
Krützen, M., Sherwin, W. B., Berggren, P., and Gales, N. (2004). Population structure in an inshore cetacean revealed by microsatellite and mtDNA analysis: Bottlenose dolphins (Tursiops sp.) in Shark Bay, Western Australia. Mar. Mamm. Sci. 20, 28–47. doi: 10.1111/j.1748-7692.2004.tb01139.x
Langtimm, C. A., Beck, C. A., Edwards, H. H., Fick-Child, K. J., Ackerman, B. B., Barton, S. L., et al. (2004). Survival estimates for Florida manatees from the photo-identification of individuals. Mar. Mamm. Sci. 20, 438–463. doi: 10.1111/j.1748-7692.2004.tb01171.x
Lebreton, J. D., Burnham, K. P., Clobert, J., and Anderson, D. R. (1992). Modeling survival and testing biological hypotheses using marked animals - a unified approach with case-studies. Ecol. Monogr. 62, 67–118. doi: 10.2307/2937171
Lenanton, R. C., Joll, L., Penn, J., and Jones, K. (1991). The influence of the Leeuwin Current on coastal fisheries of Western Australia. J. R. Soc. West. Aust. 74, 101–114.
Mann, J. (2000). “Unraveling the dynamics of social life: Long term studies and observational methods,” in Cetacean societies: Field studies of dolphins and whales, eds J. Mann, R. C. Connor, P. L. Tyack, and H. Whitehead (Chicago, IL: The University of Chicago Press), 45–64.
Mann, J., Connor, R. C., Barre, L. M., and Heithaus, M. R. (2000). Female reproductive success in bottlenose dolphins (Tursiops sp.): life history, habitat, provisioning, and group-size effects. Behav. Ecol. 11, 210–219. doi: 10.1093/beheco/11.2.210
Mansur, R. M., Strindberg, S., and Smith, B. D. (2012). Mark-resight abundance and survival estimation of Indo-Pacific bottlenose dolphins, Tursiops aduncus, in the Swatch-of-No-Ground, Bangladesh. Mar. Mamm. Sci. 28, 561–578. doi: 10.1111/j.1748-7692.2011.00520.x
Maze, K. S., and Würsig, B. (1999). Bottlenose dolphins of San Luis Pass, Texas: occurrence patterns, site-fidelity, and habitat use. Aquat. Mammals 25, 91–103.
McCluskey, S. M., Bejder, L., and Loneragan, N. R. (accepted). Assessing potential dolphin prey availability calorific value in an estuarine coastal environment. Front. Mar. Sci.
Morteo, E., Rocha-Olivares, A., and Abarca-Arenas, L. G. (2014). Sexual segregation of coastal bottlenose dolphins (Tursiops truncatus) in the southwestern Gulf of Mexico. Aquat. Mammals 40, 375–385. doi: 10.1578/AM.40.4.2014.375
Nichols, J. D., Kendall, W. L., Hines, J. E., and Spendelow, J. A. (2004). Estimation of sex-specific survival from capture-recapture data when sex is not always known. Ecology 85, 3192–3201. doi: 10.1890/03-0578
Nicholson, K., Bejder, L., Allen, S. J., Krützen, M., and Pollock, K. H. (2012). Abundance, survival and temporary emigration of bottlenose dolphins (Tursiops sp.) off Useless Loop in the western gulf of Shark Bay, Western Australia. Mar. Freshw. Res. 63, 1059–1068. doi: 10.1071/MF12210
Palsbøll, P. J., Allen, J., Bérubé, M., Clapham, P. J., Feddersen, T. P., Hammond, P. S., et al. (1997). Genetic tagging of humpback whales. Nature 388, 767–769. doi: 10.1038/42005
Parra, G. J., Corkeron, P. J., and Marsh, H. (2006). Population sizes, site fidelity and residence patterns of Australian snubfin and Indo-Pacific humpback dolphins: implications for conservation. Biol. Conserv. 129, 167–180. doi: 10.1016/j.biocon.2005.10.031
Payne, R. (1986). Long term behavioural studies of the southern right whale (Eubalaena australis). Rep. Int. Whaling Comm. 161–167.
Pearce, A. F., and Phillips, B. F. (1988). Enso events, the Leeuwin current, and larval recruitment of the western rock lobster. J. Du Conseil 45, 13–21. doi: 10.1093/icesjms/45.1.13
Pollock, K. (1982). A capture-recapture design robust to unequal probability of capture. J. Wildl. Manag. 46, 752–757. doi: 10.2307/3808568
Pollock, K. H., Nichols, J. D., Brownie, C., and Hines, J. E. (1990). Statistical-inference for capture-recapture experiments. Wildl. Monogr. 1–97.
Pradel, R., Maurin-Bernier, L., Gimenez, O., Genovart, M., Choquet, R., and Oro, D. (2008). Estimation of sex-specific survival with uncertainty in sex assessment. Can. J. Stat. Rev. Can. De Stat. 36, 29–42. doi: 10.1002/cjs.5550360105
Ramp, C., Berube, M., Palsboll, P., Hagen, W., and Sears, R. (2010). Sex-specific survival in the humpback whale Megaptera novaeangliae in the Gulf of St. Lawrence, Canada. Mar. Ecol. Progr. Ser. 400, 267–276. doi: 10.3354/meps08426
Reisinger, R. R., and Karczmarski, L. (2010). Population size estimate of Indo-Pacific bottlenose dolphins in the Algoa Bay region, South Africa. Mar. Mamm. Sci. 26, 86–97. doi: 10.1111/j.1748-7692.2009.00324.x
Rosel, P. E., Mullin, K. D., Garrison, L., Schwacke, L., Adams, J., Balmer, B., et al. (2011). Photo-Identification Capture-Mark-Recapture Techniques for Estimating Abundance of Bay, Sound and Estuary Populations of Bottlenose Dolphins along the U.S. East Coast and Gulf of Mexico: A Workshop Report. Lafayette, LA: NOAA Technical Memorandum NMFS-SEFSC-621. 30.
Rossman, S., McCabe, E. B., Barros, N. B., Gandhi, H., Ostrom, P. H., Stricker, C. A., et al. (2015). Foraging habits in a generalist predator: sex and age influence habitat selection and resource use among bottlenose dolphins (Tursiops truncatus). Mar. Mamm. Sci. 31, 155–168. doi: 10.1111/mms.12143
Rowe, L. E., and Dawson, S. M. (2009). Determining the sex of bottlenose dolphins from Doubtful Sound using dorsal fin photographs. Mar. Mamm. Sci. 25, 19–34. doi: 10.1111/j.1748-7692.2008.00235.x
Ruckstuhl, K. E., and Clutton-Brock, T. H. (2005). “Sexual segregation and the ecology of the two sexes,” in Sexual Segregation in Vertebrates: Ecology of the Two Sexes, eds K. E. Ruckstuhl and P. Neuhaus. (New York, NY: Cambridge University Press), 3–7.
Schofield, G., Dimadi, A., Fossette, S., Katselidis, K. A., Koutsoubas, D., Lilley, M. K. S., et al. (2013). Satellite tracking large numbers of individuals to infer population level dispersal and core areas for the protection of an endangered species. Divers. Distrib. 19, 834–844. doi: 10.1111/ddi.12077
Schofield, G., Katselidis, K. A., Dimopoulos, P., and Pantis, J. D. (2008). Investigating the viability of photo-identification as an objective tool to study endangered sea turtle populations. J. Exp. Mar. Biol. Ecol. 360, 103–108. doi: 10.1016/j.jembe.2008.04.005
Seber, G. A. F. (1982). The Estimation of Animal Abundance, and Related Parameters. New York, NY: MacMillan.
Similä, T., Holst, J. C., and Christensen, I. (1996). Occurrence and diet of killer whales in northern Norway: seasonal patterns relative to the distribution and abundance of Norwegian spring-spawning herring. Can. J. Fish. Aquat. Sci. 53, 769–779. doi: 10.1139/f95-253
Smith, H., Frère, C., Kobryn, H., and Bejder, L. (2016). Dolphin sociality, distribution and calving as important behavioural patterns informing management. Anim. Conserv. doi: 10.1111/acv.12263
Smith, H. C., Pollock, K., Waples, K., Bradley, S., and Bejder, L. (2013). Use of the robust design to estimate seasonal abundance and demographic parameters of a coastal bottlenose dolphin (Tursiops aduncus) population. PLoS ONE 8:e76574. doi: 10.1371/annotation/369119db-d9ca-4473-9390-89ee0c2a532f
Smolker, R. A., Richards, A. F., Connor, R. C., and Pepper, J. W. (1992). Sex-differences in patterns of assoctaion among Indian Ocean bottlenose dolphins. Behaviour 123, 38–69. doi: 10.1163/156853992X00101
Speakman, T. R., Lane, S. M., Schwacke, L. H., Fair, P. A., and Zolman, E. S. (2010). Mark-recapture estimates of seasonal abundance and survivorship for bottlenose dolphins (Tursiops truncatus) near Charleston, South Carolina, USA. J. Cetac. Res. Manag. 11, 153–162.
Sprogis, K. R. (2015). Sex-Specific Patterns in Abundance, Home Ranges and Habitat Use of Indo-Pacific Bottlenose Dolphins (Tursiops aduncus) in South-Western Australia. Ph.D., Murdoch University.
Sprogis, K. R., Raudino, H. C., Rankin, R., MacLeod, C. D., and Bejder, L. (2016). Home range size of adult Indo-Pacific bottlenose dolphins (Tursiops aduncus) in a coastal and estuarine system is habitat and sex-specific. Mar. Mamm. Sci. 32, 287–308. doi: 10.1111/mms.12260
Stanton, M. A., and Mann, J. (2012). Early social networks predict survival in wild bottlenose dolphins. PLoS ONE 7:e47508. doi: 10.1371/journal.pone.0047508
Stevick, P. T., Palsboll, P. J., Smith, T. D., Bravington, M. V., and Hammond, P. S. (2001). Errors in identification using natural markings: rates, sources, and effects on capture-recapture estimates of abundance. Can. J. Fish. Aquat. Sci. 58, 1861–1870. doi: 10.1139/cjfas-58-9-1861
Tolley, K. A., Read, A. J., Wells, R. S., Urian, K. W., Scott, M. D., Irvine, A. B., et al. (1995). Sexual dimorphism in wild bottlenosed dolphins (Tursiops truncatus) from Sarasota, Florida. J. Mammal. 76, 1190–1198. doi: 10.2307/1382611
Tsai, Y. J. J., and Mann, J. (2013). Dispersal, philopatry, and the role of fission-fusion dynamics in bottlenose dolphins. Mar. Mamm. Sci. 29, 261–279. doi: 10.1111/j.1748-7692.2011.00559.x
Tyne, J. A., Pollock, K. H., Johnston, D. W., and Bejder, L. (2014). Abundance and survival rates of the Hawai'i Island associated spinner dolphin (Stenella longirostris) stock. PLoS ONE 9:e86132. doi: 10.1371/journal.pone.0086132
Urian, K., Gorgone, A., Read, A., Balmer, B., Wells, R. S., Berggren, P., et al. (2015). Recommendations for photo-identification methods used in capture-recapture models with cetaceans. Mar. Mamm. Sci. 31, 298–321. doi: 10.1111/mms.12141
Van Dam, R. P., Diez, C. E., Balazs, G. H., Colón, L. A. C., McMillan, W. O., and Schroeder, B. (2008). Sex-specific migration patterns of hawksbill turtles breeding at Mona Island, Puerto Rico. Endanger. Species Res. 4, 85–94. doi: 10.3354/esr00044
van Toor, M. L., Jaberg, C., and Safi, K. (2011). Integrating sex-specific habitat use for conservation using habitat suitability models. Anim. Conserv. 14, 512–520. doi: 10.1111/j.1469-1795.2011.00454.x
Webster, I., Cockcroft, V. G., and Cadinouche, A. (2014). Abundance of the Indo-Pacific bottlenose dolphin Tursiops aduncus off south-west Mauritius. Afr. J. Mar. Sci. 36, 293–301. doi: 10.2989/1814232X.2014.946448
Webster, T. A., Dawson, S. M., and Slooten, E. (2009). Evidence of sex segregation in Hector's dolphin (Cephalorhynchus hectori). Aquat. Mammals 35, 212–219. doi: 10.1578/AM.35.2.2009.212
Weimerskirch, H., Cherel, Y., Cuenot-Chaillet, F., and Ridoux, V. (1997). Alternative foraging strategies and resource allocation by male and female Wandering Albatrosses. Ecology 78, 2051–2063. doi: 10.1890/0012-9658(1997)078[2051:AFSARA]2.0.CO;2
Wells, R. S. (2014). “Social structure and life history of bottlenose dolphins near Sarasota Bay, Florida: insights from four decades and five generations,” in Primates and Cetaceans: Field Research and Conservation of Complex Mammalian Societies, Primatology Monographs, eds J. Yamagiwa and L. Karczmarski (Tokyo: Springer), 149–172.
Wells, R. S., Scott, M. D., and Irvine, A. B. (1987). “The social structure of free-ranging bottlenose dolphins,” in Current Mammalogy, ed H. H. Genoways (New York, NY; London: Plenum Press), 247–305.
White, G. C., and Burnham, K. P. (1999). Program MARK: survival estimation from populations of marked animals. Bird Study 46, 120–139. doi: 10.1080/00063659909477239
Whitehead, H. (1990). Rules for roving males. J. Theor. Biol. 145, 355–368. doi: 10.1016/S0022-5193(05)80115-8
Whitehead, H., and Mann, J. (2000). “Female reproductive strategies of cetaceans - Life histories and calf care,” in Cetacean societies: Field Studies of Dolphins and Whales, eds J. Mann, R. C. Connor, P. L. Tyack, and H. Whitehead (Chicago, IL: The University of Chicago Press), 219–246.
Williams, B. K., Nichols, J. D., and Conroy, M. J. (2002). Analysis and Management of Animal Populations. San Diego, CA: Academic Press.
Williams, J. A., Dawson, S. M., and Slooten, E. (1993). The abundance and distribution of bottlenosed dolphins (Tursiops truncatus) in Doubtful Sound, New Zealand. Can. J. Zool. Rev. Can. De Zool. 71, 2080–2088. doi: 10.1139/z93-293
Wilson, B., Hammond, P. S., and Thompson, P. M. (1999). Estimating size and assessing trends in a coastal bottlenose dolphin population. Ecol. Appl. 9, 288–300. doi: 10.1890/1051-0761(1999)009[0288:ESAATI]2.0.CO;2
Würsig, B., and Jefferson, T. A. (1990). Methods of photo-identification for small cetaceans. Rep. Int. Whaling Comm. 43–52.
Würsig, B., and Würsig, M. (1977). The photographic determination of group size, composition, and stability of coastal porpoises (Tursiops truncatus). Science 198, 755–756. doi: 10.1126/science.198.4318.755
Xavier, J. C., and Croxall, J. P. (2005). “Sexual differences in foraging behaviour and diets: a case study of wandering albatrosses,” in Sexual Segregation in Vertebrates: Ecology of the Two Sexes, eds K. E. Ruckstuhl and P. Neuhaus (New York, NY: Cambridge University Press), 74–91.
Keywords: capture-recapture, capture probability, Robust Design, seasonal movements, sexually monomorphic, Western Australia
Citation: Sprogis KR, Pollock KH, Raudino HC, Allen SJ, Kopps AM, Manlik O, Tyne JA and Bejder L (2016) Sex-Specific Patterns in Abundance, Temporary Emigration and Survival of Indo-Pacific Bottlenose Dolphins (Tursiops aduncus) in Coastal and Estuarine Waters. Front. Mar. Sci. 3:12. doi: 10.3389/fmars.2016.00012
Received: 04 November 2015; Accepted: 25 January 2016;
Published: 16 February 2016.
Edited by:
Graeme Clive Hays, Deakin University, AustraliaReviewed by:
Ari Friedlaender, Oregon State University, USAGail Schofield, Deakin University, Australia
Copyright © 2016 Sprogis, Pollock, Raudino, Allen, Kopps, Manlik, Tyne and Bejder. This is an open-access article distributed under the terms of the Creative Commons Attribution License (CC BY). The use, distribution or reproduction in other forums is permitted, provided the original author(s) or licensor are credited and that the original publication in this journal is cited, in accordance with accepted academic practice. No use, distribution or reproduction is permitted which does not comply with these terms.
*Correspondence: Kate R. Sprogis, k.sprogis@murdoch.edu.au
†nee Smith