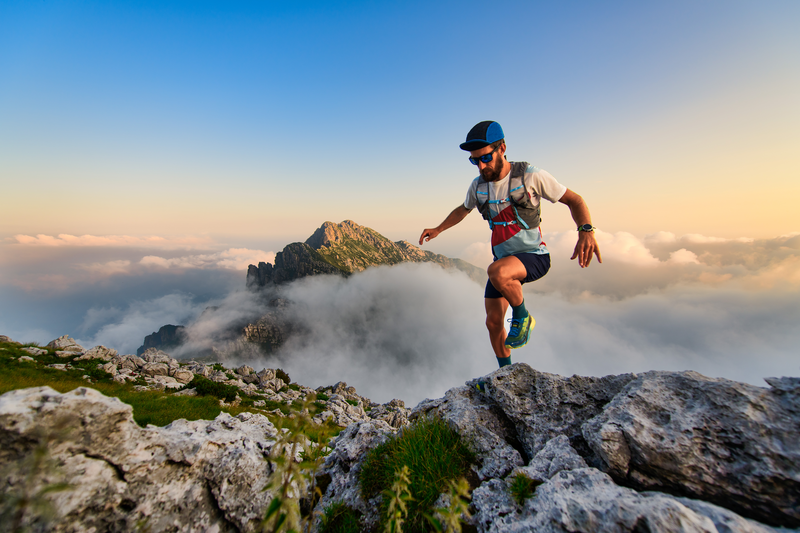
94% of researchers rate our articles as excellent or good
Learn more about the work of our research integrity team to safeguard the quality of each article we publish.
Find out more
REVIEW article
Front. Mar. Sci. , 27 October 2015
Sec. Global Change and the Future Ocean
Volume 2 - 2015 | https://doi.org/10.3389/fmars.2015.00083
This article is part of the Research Topic Ocean research priorities and prioritizing ocean research View all 5 articles
Fisheries bycatch is a threat to species of marine megafauna across the world's oceans. Work over the past several decades has greatly advanced our understanding of the species affected, the magnitude and the spatial extent of bycatch. In the same time period, there have been substantial advances in the development of mitigation strategies and best practices to reduce bycatch. In this paper, we take stock of bycatch knowledge and science to address the critical question “Where do we go from here?” First, we review the current state of global bycatch science, including bycatch rate estimation and biological effects of bycatch, and bycatch mitigation practices and gear. We then identify knowledge gaps as well as socio-cultural constraints that hamper effective knowledge transfer or implementation, and discuss emerging transdisciplinary approaches to address these issues. Finally, we discuss the need to consider bycatch in a changing ocean and socio-cultural context where species, ecosystems, and people are responding to multiple stressors and dynamic conditions. As the field of bycatch research moves into the twenty-first century, a new perspective is needed to develop responsive strategies that effectively address the shifting ecological, social, cultural, and economic contexts of the global bycatch seascape.
Global fisheries bycatch, the incidental catch of unused or unmanaged species (Davies et al., 2009), is a major threat to the world's oceans (Hall et al., 2000; Kelleher, 2005). Bycatch of long-lived marine megafauna has both direct and indirect ecological effects (Lewison et al., 2004), directly reducing megafauna populations (Heppell et al., 2000) and indirectly changing trophic dynamics of ocean systems (Estes et al., 2011; McCauley et al., 2015). Megafauna bycatch (hereafter referring to marine mammals, elasmobranchs, seabirds, and marine turtles) presents a global resource management challenge as many marine megafauna crisscross ocean basins, necessitating international action, coordination and, in some cases, policy instruments to address bycatch at these large scales.
Understanding the problems and developing effective bycatch reduction strategies is a complex, environmental challenge with scientific, socio-cultural, and socio-economic components. In recent years, bycatch research has broadened (see Figure 1 in Soykan et al., 2008), greatly advancing our knowledge of the species affected, the magnitude and the spatial extent of bycatch. Concurrently, there have been substantial advances in the development of mitigation strategies. These achievements represent significant progress, yet some critical knowledge gaps and the need for innovation remains. Megafauna bycatch continues to threaten populations, and for some already depleted species such as the vaquita (Phocoena sinus), Amsterdam albatross (Diomedea amsterdamensis), leatherback sea turtle (Dermochelys coriacea), and dugong (Dugong dugon), bycatch is the largest contributing threat to their risk of extinction (D'Agrosa et al., 2000; Marsh et al., 2002; Read, 2008; Rivalan et al., 2010; Wallace et al., 2013; CIRVA, 2014). Current efforts to reduce megafauna bycatch, particularly in developing nations, have called attention to the socio-cultural factors that may supercede the ecological concerns regarding dwindling megafauna populations (Teh et al., 2015). Addressing the complex ecological and human dimensions of megafauna bycatch requires multifaceted and comprehensive approaches, some of which have been developed and others which are still emerging. In this paper, we review the current state of global bycatch science, including bycatch rate estimation and biological effects of bycatch, and bycatch mitigation practices and gear. We then identify knowledge gaps, socio-cultural constraints, and the need to consider bycatch in a changing ocean and socio-cultural context where species, ecosystems, and people are responding to multiple stressors and dynamic conditions. For each of these issues, we discuss emerging transdisciplinary approaches that demonstrate how the future of bycatch science will need to develop to meet ongoing challenges.
Megafauna bycatch in many fisheries is a rare event that is difficult to detect unless fishing vessels have onboard scientific observers. Despite their low frequency of occurrence, these events are important to quantify because they can have negative consequences for marine megafauna, particularly for small populations. Yet this inherent “rarity” characteristic makes attaining robust estimations of bycatch rates a central challenge to bycatch research. Bycatch data can be documented in fishers' logbooks or by independent observer programs, but these are not available for many fisheries and regions, particularly for small-scale fisheries. When data are available, they typically cover a small fraction of total fishing effort (Lewison et al., 2004; Moore et al., 2009; Phillips, 2013). This reality is at odds with the need for relatively high sample sizes required to estimate megafauna bycatch rates with precision (Babcock et al., 2003; Amande et al., 2012). The rarity in bycatch events, which leads to issues of overdispersion and zero-inflated distributions, coupled with spatial correlation and variability in fishing locations, presents additional analytical challenges to accurate bycatch estimation (Sims et al., 2008; Barlow and Berkson, 2012). These data characteristics contribute to uncertainty in rate estimation, hinder accurate bycatch assessments, and ultimately impede development of effective mitigation strategies due to uncertainty.
In the two past decades, the bycatch research community has made large strides tackling these issues by developing approaches to address data gaps and improve quantitative analyses of existing bycatch data to address and incorporate uncertainty, in some cases in conjunction with incorporation of other oceanographic data. Development of bycatch estimators and using existing data to generate a range of estimates from worst to best case scenarios have been employed to estimate bycatch rates in the face of data deficiencies (Lewison and Crowder, 2003; Miller and Skalski, 2006). Bayesian methods have also recently been developed to predict unobserved bycatch events, estimating cumulative mortality and probabilities of mortality exceeding regulatory thresholds. (Martin et al., 2015; Moore and Curtis, in press). Many approaches have drawn on advances from other fields, highlighting the utility of interdisciplinary collaboration to solve today's complex ecological problems. For example, Bayesian hierarchical models developed from an epidemiological spatial model (Best et al., 2005) use data from surrounding areas to generate smoothed bycatch rates. This approach addresses statistical issues of analyzing spatial patterns from raw bycatch rates (i.e., overdispersion, spatial correlation, and fishing effort variability; Sims et al., 2008) and generates bycatch rate maps within and across taxa with increased stability and confidence. These and other advances in quantifying spatial patterns have enabled the identification of “bycatch hotspots,” i.e., areas of persistent bycatch events for one or multiple species of conservation concern to focus mitigation efforts (Lewison et al., 2009; Yeh et al., 2013). Characterization of bycatch spatial patterns and hotspots in conjunction with research on animal movement patterns has identified relationships between sea surface temperatures, chlorophyll, geostrophic currents, and other ocean, fishery or gear characteristics and bycatch (James et al., 2005; Polovina et al., 2006; Sims et al., 2008; Zydelis et al., 2011; Briscoe et al., 2014). For example, by identifying oceanographic conditions and habitat features associated with loggerhead and leatherback turtles off Hawaii, the NOAA-led program Turtlewatch creates weekly maps depicting ocean areas where bycatch of both species is more likely (Howell et al., 2008, 2015). These and other similar analyses have been critical in enhancing our understanding of target and non-target species' ecology, identifying particular taxa and areas in peril, evaluating population level effects, and linking specific risk factors to species of conservation concern.
Artisanal and other small-scale fisheries represent a particularly data-poor sector, yet compose a large majority of the world's fishers (Béné, 2006; Pauly, 2006) and can have significant negative impacts on marine megafauna (D'Agrosa et al., 2000; Peckham et al., 2007; Mangel et al., 2010). These fisheries, particularly in developing countries, are diffuse and seldom regulated, making the data collection methods employed for industrial fisheries infeasible. Alternatively, researchers have drawn on community-based social science interview and survey methods to begin to address this knowledge gap (Okada et al., 2005; Otero et al., 2005). Moore et al. (2010) developed a systematic interview protocol that has been tested, adapted and applied to study bycatch in multiple other countries (UNEP, 2010; Whitty et al., 2010; Kiszka, 2012). Applying interview techniques in this standardized way across broad spatial scales has great potential to address small-scale fisheries knowledge gaps by generating comparable data to estimate cumulative bycatch and identify patterns across regions, species, and gear types.
Another challenge of bycatch research has been to quantify the biological impacts of bycatch. Effects of bycatch occur at population, species, and ecosystem levels (e.g., population declines, creation of sink populations within species, altered food web interactions). Connecting specific fisheries or gear types to these consequences can be particularly important to support legal and other conservation initiatives, as well as linking recoveries to mitigation practices (Soykan et al., 2008). However, quantifying biological effects requires not only robust bycatch rate estimations and spatial patterns, but also knowledge of life history, demographics, population connectivity, and trophic and other ecological relationships. Like bycatch events themselves, the pelagic and migratory nature as well as variable spatial distribution of many megafauna makes comprehensive collection of such data logistically difficult, impeding studies of biological impacts. Additional factors such as time lags common in long-lived vertebrates and sublethal impacts (e.g., injuries or other negative fitness consequences from bycatch interactions) can complicate both the detection of population declines and linking them to bycatch. Despite these constraints, advances have significantly improved both our understanding of the biological effects of bycatch and how mitigation strategies can contribute to diminishing these impacts.
Bycatch researchers have utilized multidisciplinary and often creative approaches to assess population-level impacts in the face of uncertainty in demographic parameters and bycatch rates. Some have incorporated uncertainty by generating estimates across plausible ranges or scenarios for demographic and other unknown parameters to compare predicted population effects (Mangel, 1993; Lewison and Crowder, 2003; Zador et al., 2008), employing matrix models that incorporate stochasticity into population trajectories (Anderson et al., 2008), and utilizing model selection methodologies (Veran et al., 2007). Population-level bycatch research has identified important population and bycatch mortality thresholds for marine mammal, sea turtle, and seabird species affected by different fisheries (Dillingham and Fletcher, 2008; Moore et al., 2013), modeled after mortality thresholds developed for marine mammals, termed Potential Biological Removal (PBR, Wade, 1998). Similar thresholds have since been developed for seabirds and sea turtles (Dillingham, 2010; Dillingham and Fletcher, 2011; Curtis and Moore, 2013; Curtis et al., 2015a,b). These examples highlight the importance of evaluating and identifying population level effects and population thresholds, even when there is uncertainty in key parameters.
Early in the development of bycatch science, researchers established that differences in reproductive value and other contributions to population sustainability among life stages can be particularly important for long-lived, late maturing megafauna (Crowder et al., 1994), making it critical to account for these factors in evaluations of bycatch population effects. Demographic techniques such as elasticity analyses have been key in determining how bycatch mortality can influence population growth and distinguish particularly sensitive age classes, utilizing general life history characteristics when species-specific data is unavailable (Heppell et al., 2000). These approaches have been useful to estimate population impacts, forecast recovery due to mitigation efforts (e.g., implementation of sea turtle exclusion devices in trawl fisheries; Crowder et al., 1994), and address issues of data uncertainty in combination with other methods (e.g., combining Monte Carlo approaches that address population vital rate uncertainty with life tables to evaluate negative impacts of gillnet or trawling bycatch on cetaceans; Caswell et al., 1998; Dans et al., 2003). Once bycatch sensitive life stages have been identified, often stages with high reproductive values (Fisher, 1930; Keyfitz and Caswell, 2005), researchers can apply this knowledge to ascertain which fisheries and gear are likely to result in the bycatch that can have the largest negative consequences on population growth (Alfaro Shigueto et al., 2008; Wallace et al., 2008).
Most recently, researchers have begun integrating biological impact assessments across populations, species, fisheries and gear types, or regions (Wallace et al., 2010; Geijer and Read, 2013). For example, Wallace et al. (2013) developed bycatch impact scores that integrate information on bycatch rates, fishing effort, mortality rates, and body sizes to assess effects and risk for global sea turtle populations. While there is much work yet to be done, these synthetic approaches are providing critical insight for assessing cumulative impacts, identifying high risk fisheries, gear or locations, and prioritizing mitigation efforts (Lewison et al., 2014). Finally, there has been increasing recognition of the need to quantify indirect, ecological effects of bycatch, such as altered food web dynamics and ecosystem functions due to the loss of large predators (i.e., trophic down-grading; Pauly et al., 1998; Estes et al., 2011). Multispecies models incorporating biomass and abundance time series data across trophic levels have identified evidence of trophic impacts due to bycatch mortality (Cox et al., 2002), but much more research is needed to increase our understanding of the cascading ecological impacts of global bycatch.
A third component of successful advances in bycatch reduction is the development and successful implementation of mitigation strategies. Effective solutions are often complex because they must balance benefits of each element to reduce bycatch against the costs to fishers' livelihoods. Additionally, diversity among the regulatory, logistical and socio-cultural constraints among fisheries and regions creates strong context dependencies for the efficacy of different strategies. In the past decade, research and development of gear modifications, risk assessment and spatiotemporal limit frameworks, and real-time data and technology integration has advanced considerably. Combined with investment in building social networks and improving communication among scientists, managers, and fishers, these approaches have shown huge potential for addressing bycatch while maintaining fisheries' economic viability.
Increased understanding of the behavioral, physiological, and sensory ecology of affected megafauna paired with industry innovation has fueled development of deterrents and gear modifications to reduce bycatch interactions. For some drift and gillnet fisheries, deployment of visual or acoustic deterrents have been shown to substantially reduce seabird bycatch (Melvin et al., 1999; Maree et al., 2014), while acoustic alarms (a.k.a. pingers) have been demonstrated to decrease bycatch for multiple marine mammal species (Dawson et al., 1998; Barlow and Cameron, 2003; Carretta and Barlow, 2011; Bjorge et al., 2013; Mangel et al., 2013; Larsen and Eigaard, 2014). The use of turtle exclusion devices (TEDs) can be highly effective to reduce sea turtle bycatch in trawl fisheries (Crowder et al., 1994; Lewison et al., 2003). However, implementation of TEDs in the US also demonstrates the complexities of bycatch mitigation, as multiple social and economic factors have hampered fisher compliance with TED regulations (Jenkins, 2007; Campbell and Cornwell, 2008). In addition to deterrents, gear and fishing practice modifications have increased survival rates for animals that are caught and released. In particular, the implementation of circle hooks and alternate baits in longline fisheries has been shown across multiple studies to not only generally diminish bycatch of sea turtles as well as sharks and other non-target fishes, but also increase post-release survival (Watson et al., 2005; Kerstetter and Graves, 2006; Gilman et al., 2007). In 2009, the Food and Agriculture Organization of the United Nations released guidelines to reduce sea turtle mortality in fishing operations, which identifies best practices in bycatch mitigation as well as best practices for retrieving and removing gear from turtles captured (FAO et al., 2009).
In the past decade technological improvements in remote sensing and animal tracking technologies have been used to develop management tools that minimize bycatch interactions and ecological impacts. For example, time-area closures have traditionally been used to minimize bycatch by temporarily closing a given area to fishing during a time period of high risk for bycatch interaction (Lewison et al., 2003). However, closures set by static seasonal dates and geographical reference points may have limited efficacy because target and bycatch species' migratory, reproductive and other cycles are frequently tied to oceanic conditions (e.g., temperature, chlorophyll) that are spatially and temporally dynamic. Such mismatches between management structures and ocean conditions can lead to inadequate protection for sensitive species or unnecessary economic losses for fisheries. Newer approaches that use telemetry, satellite data to account for dynamic conditions and bycatch risk hold promise for bycatch reduction within a sustainable fisheries context (e.g., TurtleWatch; Howell et al., 2008, 2015).
Finally, there has been an emergence of strong socio-cultural and economic approaches to bycatch reduction. Social networks and community-based cooperatives or councils have been demonstrated to be a powerful tool for effective bycatch mitigation (Hall et al., 2007; Peckham and Maldonado-Diaz, 2012). Implementation of such frameworks can improve communication and knowledge transfer among scientists, managers, and fishers, and creates a culture where all groups actively contribute to the development, testing, feedback, and implementation of mitigation strategies. This enhances fishers' understanding of the rationale underlying fishery regulations, and incorporates local knowledge and community needs into sustainable management solutions. These networks have been particularly critical for small-scale fisheries where top down regulation or enforcement is not feasible, making social capital and community buy-in imperative for compliance and sustainability (Campbell and Cornwell, 2008; Alfaro-Shigueto et al., 2010; Peckham and Maldonado-Diaz, 2012; Piovano et al., 2012). More formal and structured social and traditional media initiatives, like the Marine Stewardship Council and the Monterey Bay Aquarium Seafood Watch also have been effective at helping consumers make informed decisions, potentially increasing market value and demand for sustainable, low-bycatch fisheries.
Even with the impressive advances in bycatch research and mitigation, there are substantial barriers or impediments to reducing bycatch. Our review of the current state of bycatch science points to six persistent gaps or barriers to bycatch reduction: lack of data and data sharing, uncertainty of population level effects of bycatch, limited understanding of the broader ecological effects of bycatch, challenges of how to address bycatch within dynamic ecological and social systems, the need to address the linked socio-ecological factors govern bycatch, and the importance of fostering stakeholder engagement in the development of sustainable bycatch reduction strategies and actions (Table 1). In this section, we explore emerging innovations and transdisciplinary approaches that offer opportunities to tackle these ongoing challenges to bycatch reduction.
Table 1. Critical knowledge gaps and socio-cultural, economic and other barriers remain that hinder effective bycatch mitigation. Emerging transdisciplinary approaches offer opportunities to address these challenges.
The creation of international collaborative working groups and organizations (e.g., Project GLoBAL, New England Aquarium, Consortium for Wildlife Bycatch Reduction) has greatly facilitated organization and synthesis of available bycatch data (Moore et al., 2009; Finkbeiner et al., 2011; Lewison et al., 2014). These efforts have helped identify patterns for many populations, species, regions, and fishing practices. Importantly, such large-scale collaborative efforts have yielded a synoptic characterization of global bycatch (Figure 1), creating a reference on the state of global bycatch knowledge, and serving as a resource for scientists and managers to identify areas of highest concern to focus conservation and mitigation efforts. Yet, this work has also highlighted the disproportionate reliance on data from industrial fishing in developed countries (McCluskey and Lewison, 2008; Lewison et al., 2011). There is still relatively little data on both megafauna bycatch and fishing effort in small and large-scale fisheries in certain regions (e.g., Indian Ocean, eastern Atlantic, southeast Asia, and central and western Pacific). This, in turn, prevents global comparative risk assessments to identify hotspots of concern. The application of alternative, context-dependent approaches such as systematic interview protocols (Moore et al., 2010) have begun to address this data gap, and their use can supplement traditional data collection. Importantly, these data are most powerful when collected a standardized, systematic manner, enabling global-scale assessments across locations, fisheries, and species. Moving forward, international collaborative working groups and organizations are poised to play an important role in facilitating collection of such standardized, robust fishery and bycatch data.
Figure 1. Global map of cumulative bycatch intensity across three species groups (seabirds, marine mammals, and sea turtles) and gear types (gillnet, longline, and trawl) from Lewison et al. (2014), reprinted with permission. Numbers represent the number of data records included within each data polygon considered.
Methodological gaps continue to hinder accurate assessment of bycatch rates and biological effects. Though there has been some recent research integrating bycatch rate estimation and biological effects assessments across fisheries, species and regions (Wallace et al., 2010; Geijer and Read, 2013), there is a great need to develop analytical techniques to expand these efforts. Particularly for migratory megafauna species that encounter multiple fisheries over large geographic distances, such assessments are imperative to understand species impacts as well as transfer effects from mitigation efforts. Recent approaches integrating evolutionary and ecological theory with species-specific knowledge to improve estimation of key parameters (e.g., rmax, λmax, generation times), and improvements in limit reference point estimators (LRPs) are advancing robust population risk assessment and estimation of sustainable bycatch limits, particularly for species with limited demographic information (Dillingham, 2010; Curtis and Moore, 2013; Moore et al., 2013; Curtis et al., 2015a,b; Dillingham et al., 2015). Building on the PBR model used for marine mammals, Dillingham and Fletcher (2011) established LRPs for seabirds using logistic population growth models, and calculated productivity from estimates of adult survival and allometry-derived age of first reproduction (Niel and Lebreton, 2005; Dillingham, 2010). Similarly, Curtis et al. (2015b) demonstrated how local abundance estimates from nest counts, vital rate information, satellite tag data, and fishery observer data can be used to identify thresholds for leatherback sea turtles. Advancements in these quantitative approaches have made them increasingly adaptable to a wide breadth of contexts, able to align with a range of management goals, available data types, and different species (Curtis et al., 2015a,b). Additionally, population effects and thresholds determined from these methods can be used to inform larger-scale ecosystem-based management approaches.
Although it is widely recognized that megafaunal decline due to bycatch and other stressors can have cascading, widespread ecological impacts, there are few approaches to detect or forecast these effects. Quantifying the indirect consequences of bycatch remains particularly challenging due to the multitude of anthropogenic stressors on global marine ecosystems. Recently, ecological risk assessments and ecosystem-based management frameworks have been employed in a moderate number of fisheries and locations (McLeod and Leslie, 2009; Hobday et al., 2011; Small et al., 2013; Gilman et al., 2014; Kirby and Ward, 2014), incorporating direct and indirect adverse effects on habitats, non-target species, and ecological functions into adaptive management strategies. Multispecies and fishery syntheses have also begun quantifying cumulative bycatch impacts (Figure 1) and identifying ecological transfer issues, whereby alterations in fishing practices decrease bycatch of one taxa or region but increase it for other species or taxa (Mukherjee, 2015). Further development and application of these approaches is critical to understand both the broader ecological context of bycatch effects and to design management strategies that effectively promote ecological sustainability.
Fisheries bycatch is now occurring in ocean systems experiencing anthropogenically driven climate change as well as natural climate variation (e.g., multidecadal oscillations) altering temperature, oxygen, biogeochemistry, primary productivity, ocean circulation, and food web dynamics (IPCC, 2014). Life history and other ecological differences between target and bycatch species are likely to result in divergent climate responses, altering ecological dynamics and potentially create novel bycatch interactions. To date there have been very few studies exploring these issues to understand under what contexts we may expect to see compensatory, additive, synergistic, or other effects in marine megafauna (but see Bunce et al., 2002; Rivalan et al., 2010; Hobday et al., 2015). Assessing climate change impacts on bycatch, in conjunction with other anthropogenic stressors such as exploitation, habitat degradation, and pollution, is critical to estimate and reduce cumulative impacts on marine megafauna and global oceans.
Human adaptation to climate change will also likely influence bycatch dynamics because fisheries and the marine ecosystems they rely on are inherently coupled social-ecological systems (Turner et al., 2010; Sumaila et al., 2011; Pinsky and Fogarty, 2012). As many fishery target species shift their distributions due to climate change, fisheries often lag behind due to logistical restraints, e.g., gear switching, inability to travel to fishing grounds, lack of landing sites, regulatory restrictions, social structure, or political boundaries (Coulthard, 2009; McCay et al., 2011). Limits to social adaptation also may contribute to altered or novel fishery-bycatch interactions and dynamics, further intensifying the need for transnational collaborative resource management.
Approaches are emerging to incorporate forecasted climate impacts and social adaptations into fishery management (Sumaila et al., 2011; Pinsky and Mantua, 2014), but have not yet extended into marine megafauna bycatch assessments or mitigation strategies. However, climate variation has been incorporated into a few mitigation efforts that may serve as templates for the comprehensive integration needed in future sustainable management planning. One example is the U.S. National Marine Fisheries Service's time-area closure of California's gillnet fishery during El Niño periods, aimed at protecting against loggerhead turtle bycatch forecasted to occur when turtles are expected to travel further north due to warmer waters. This measure was enacted in 2003, but was not triggered until 2014 (due to persistent high water temperatures, but not specifically associated with El Niño) and again in 2015, and it is unclear how this regulation will translate into the future if warmer temperatures become more common due to long-term, directional climate change even in absence of El Niño events. However, observing the implementation, efficacy, and adaptability of this and other similar measures to identify successes and areas needing improvement will offer insight for future climate-responsive management strategies.
Dynamic ocean management practices (DOM) that explicitly account for dynamic oceanographic and fishing conditions are increasingly gaining traction worldwide (Hobday et al., 2014; Lewison et al., 2015; Maxwell et al., 2015). DOM combines technological advances to utilize and share near real-time environmental and biological data with industry to promptly communicate conditions to stakeholders. This allows managers and fishers to rapidly adjust fishing practices, efforts, and locations in response to changing conditions. Thus, DOM offers one avenue to integrate species' responses to climate change and other stressors into mitigation strategies, and also holds great potential to minimize both bycatch mortality and economic losses for fisheries. Improved integration of science, technology, and stakeholder engagement into management policies is a next critical step that will facilitate effective DOM for bycatch reduction (Hobday et al., 2014). Statistical models integrating environmental and telemetry data are also being developed to forecast the presence of high-risk bycatch species presence, guiding dynamic spatiotemporal fisheries restriction decisions (e.g., bluefin tuna in eastern Australia; Hobday et al., 2010). Importantly, the development and effective application of these “dynamic tools” is reliant on robust input data, making continued collection of both environmental and biological data critical to success. Limited or outdated availability of data increase error, which can render the approaches less effective by negatively impacting species or unnecessarily closing fisheries, but also risks undermining stakeholder trust and engagement. Finally, in conjunction with these dynamic approaches, the further development of practices aimed at managing marine ecosystems for resiliency (Levin and Lubchenco, 2008; Fujita et al., 2013) has the capacity to cultivate transdisciplinary, adaptive frameworks needed for sustainable management of dynamic social-ecological systems into coming decades.
Beyond the individual dynamics of both ecological and social systems is the need to identify and resolve socio-cultural and economic factors that lead to bycatch within a coupled social-ecological context. Efficacy of bycatch solutions is recognized as highly context dependent; approaches that are successful for one fishery or region can fail completely in others due to a variety of factors such as political drivers, infrastructure, culture, economic and ecological conditions, or scale (Hall et al., 2007). For example, bycatch avoidance measures found to be highly effective in experimental testing can fall short when implemented in commercial fishing operations because of differences between controlled experimental and real-world conditions (Cox et al., 2007). Strategies that work for large-scale, highly regulated U.S. commercial fishing operations are not applicable to small-scale, diffuse artisanal fisheries with little top-down enforcement (Peckham et al., 2007; Brewer, 2013). Early identification of the linked eco-socio-cultural and economic context dependencies relevant to a given region and fishery are critical to the development of creative, stakeholder-engaged transdisciplinary solutions to overcome these constraints (Campbell and Cornwell, 2008; Peckham and Maldonado-Diaz, 2012).
Socio-cultural barriers can hamper both effective knowledge transfer and implementation of mitigation strategies. These include traditional socio-cultural factors among an ethnicity, community, or demographic that can alter perception and motivations (e.g., cultural values of conservation, family relationships and hierarchies, fishing knowledge, and beliefs; Chan et al., 2012; Teh et al., 2012, 2015), all related to the local culture of fishing. Many fishers around the globe share strong common values of independence and are historically not accustomed to strong regulation (Gislason, 2015). This can hinder compliance with restrictions limiting fishing effort (i.e., fishery closures) or requiring gear modification, particularly when enforcement, incentives, or investment in social capital are low (Cox et al., 2007; Peckham et al., 2007).
Socio-economic factors can also affect the efficacy of bycatch mitigation approaches. Artisanal fishing communities are frequently marginalized in many developing nations (Pauly, 1997), contributing to limited access to education and other resources, high poverty, overexploitation of marine resources, and few alternative livelihood options (Béné, 2003; Cinner, 2011). These conditions limit both the ability and willingness of fishers to alter fishing methods to mitigate bycatch. In particular, compliance in both small-scale and large commercial fisheries is challenging if mitigation techniques have, or are perceived to have, economic costs, such as target catch reduction, increased fuel or equipment costs, or opportunity costs (Cox et al., 2007; Campbell and Cornwell, 2008).
Integrated frameworks have been developed and applied in a variety of conservation contexts to identify socio-cultural and economic factors affecting ecosystem management (Chan et al., 2012; Schultz et al., 2015). Adaptations of these transdisciplinary frameworks are emerging to contextualize the enabling and limiting socio-ecological conditions affecting successful bycatch mitigation (Lewison et al., 2011; Teh et al., 2015). Importantly, many factors enabling bycatch conservation occur at local scales, underscoring the importance of social capital and community participation, particularly in small-scale fisheries. The continued application of these frameworks is a critical first step in assessment of social context dependencies of bycatch issues. Importantly, this must be followed by the development of mitigation strategies that address these factors, facilitate continued dialogue and feedback, and allow for adaptability of management policies to adjust to dynamic socio-cultural, economic, and environmental conditions.
The scale of mitigation policies can also greatly change socio-economic contexts. For commercial fisheries that compete internationally for import or export of their catch, regulations that restrict activities or increase costs within a country or region, e.g., within an Exclusive Economic Zone (EEZ) vs. international waters, can weaken their ability to compete if their international counterparts do not have the same restrictions. With increasing market globalization, bycatch reduction-related measures can lead to market transfer effects (Sarmiento, 2006; Rausser et al., 2009; Bartram et al., 2010). For example, Rausser et al. (2009) found that the 2001–2004 closure of the Hawaiian longline swordfish fishery, implemented to protect sea turtles, may have substantially increased sea turtle interactions with international, and often less regulated, fishing fleets that stepped in to meet U.S. swordfish market demands. Effective bycatch mitigation strategies need to consider, integrate and support the livelihood of associated fishing industries and communities. Effective mitigation measures must also consider the underlying economic and market forces that drive fishery landings. In this context, bycatch-driven fisheries closures can be just as unsustainable as the bycatch that motivated that closure. Similarly, bycatch-driven shifts in gear or fishing practices can lead to unintended consequences, solving a bycatch problem for one species in one area, but creating a new bycatch problem for another species or fishing location (Mukherjee, 2015). Long-term sustainable bycatch solutions must account for these and other behavioral, economic and social changes that can lead to negative, unintended consequences (Abbott and Haynie, 2012) and ultimately undermine sustainable fisheries worldwide.
Interwoven with the need to understand dynamic SES systems is the strong need for stakeholder engagement, feedback, and evaluation for all types of fisheries, management strategies, and regions. Stakeholder engagement not only strongly influences successful implementation and compliance of bycatch mitigation strategies, but also is key to finding creative solutions that will be successful under real conditions without threatening fishers' livelihoods (Cox et al., 2007; Campbell and Cornwell, 2008). In conjunction with identifying SES linkages, co-management and community cooperatives have proven to be instrumental for the successful implementation of sustainable fishing policies, particularly for small-scale fisheries (Peckham and Maldonado-Diaz, 2012). Such approaches facilitate continued dialogue among scientists, fishers, and managers to assess and adapt mitigation strategies to adjust to real vs. ideal implementation scenarios. Utilizing a framework of standards to perform systematic, robust assessment of mitigation technologies and practices, facilitate feedback, and drive adaptation has also been effective in fisheries with greater infrastructure capacity (Kirby and Ward, 2014). Solving global bycatch issues necessitates the continuation and expansion of these approaches to engage stakeholders and evaluate and adapt bycatch mitigation strategies across socio-ecological contexts.
There have been impressive advances in bycatch research and mitigation, but there are still substantial remaining and emerging barriers to reducing fisheries bycatch. Global bycatch, like other challenges to sustainable use, occurs in a changing ocean and socio-cultural context where species, ecosystems, and people are responding to multiple stressors and dynamic conditions. As the oceans continue to transform and the field of bycatch research moves into the twenty-first century, emerging dynamic, transdisciplinary approaches that integrate both the human and ecological dimensions of bycatch offer innovative opportunities to meet these challenges. We have identified focal areas where more effort is needed; the continued support by research and management communities of further development, testing and implementation of emerging strategies to meet these needs is essential. There is not a single panacea to solve fisheries bycatch in our complex, changing world. Rather, investing in a diverse, adaptive portfolio of these tools and approaches to best match ecological, sociocultural, and economic contexts offers the best path forward to address global bycatch and support sustainable fisheries into the future.
Support for LK was provided by the National Academies, National Research Council post-doctoral research fellowship. Support for RL was provided by NASA Earth Science Division/Applied Sciences Program's ROSES A.36 Ecological Forecasting. Funding for open access publication was provided by the University of California, Davis Shields Library open access program.
The authors declare that the research was conducted in the absence of any commercial or financial relationships that could be construed as a potential conflict of interest.
We would like to thank Dr. Jeffrey Moore and two reviewers for constructive comments on earlier versions of this manuscript.
Abbott, J. K., and Haynie, A. C. (2012). What are we protecting? Fisher behavior and the unintended consequences of spatial closures as a fishery management tool. Ecol. Appl. 22, 762–777. doi: 10.1890/11-1319.1
Alfaro Shigueto, J., Mangel, J., Seminoff, J., and Dutton, P. (2008). Demography of loggerhead turtles Caretta caretta in the southeastern Pacific Ocean: fisheries-based observations and implications for management. Endanger Species Res 5, 129–135. doi: 10.3354/esr00142
Alfaro-Shigueto, J., Mangela, J. C., Pajuelo, M., Dutton, P. A., Seminoff, J. A., and Godley, B. J. (2010). Where small can have a large impact: structure and characterization of small-scale fisheries in Peru. Fish. Res. 106, 8–17. doi: 10.1016/j.fishres.2010.06.004
Amande, M. J., Chassot, E., Chavance, P., Murua, H., Delgado de Molina, A., and Bez, N. (2012). Precision in bycatch estimates: the case of tuna purse-seine fisheries in the Indian Ocean. ICES J. Mar. Sci. 69, 1501–1510. doi: 10.1093/icesjms/fss106
Anderson, D. J., Huyvaert, K. P., Awkerman, J. A., Proaño, C. B., Milstead, W. B., Jiménez-Uzcátegui, G., et al. (2008). Population status of the critically endangered waved albatross Phoebastria irrorata, 1999 to 2007. Endanger Species Res. 5, 185–192. doi: 10.3354/esr00089
Babcock, E. A., Pikitch, E. K., and Hudson, C. G. (2003). How much Observer Coverage is Enough to Adequately Estimate Bycatch? Washington, DC; Miami, FL: Pew Institute for Ocean Science and Oceana.
Ban, N. C., Mills, M., Tam, J., Hicks, C. C., Klain, S., Stoeckl, N., et al. (2013). A social-ecological approach to conservation planning: embedding social considerations. Front. Ecol. Environ. 11, 194–202. doi: 10.1890/110205
Barlow, J., and Cameron, G. A. (2003). Field experiments show that acoustic pingers reduce marine mammal bycatch in the California drift gill net fishery. Mar. Mamm. Sci. 19, 265–283. doi: 10.1111/j.1748-7692.2003.tb01108.x
Barlow, P. F., and Berkson, J. (2012). Evaluating methods for estimating rare events with zero-heavy data: a simulation model estimating sea turtle bycatch in the pelagic longline fishery. Fish. B-NOAA 110, 344–360. Available online at: http://fishbull.noaa.gov/1103/barlow.pdf
Bartram, P. K., Kaneko, J. J., and Kucey-Nakamura, K. (2010). Sea turtle bycatch to fish catch ratios for differentiating Hawaii longline-caught seafood products. Mar. Policy 34, 145–149. doi: 10.1016/j.marpol.2009.05.006
Béné, C. (2003). When fishery rhymes with poverty: a first step beyond the old paradigm on poverty in small-scale fisheries. World Dev. 31, 949–975. doi: 10.1016/S.0305-750X.(03)00045-7
Béné, C. (2006). Small-Scale Fisheries: Assessing Their Contribution to Rural Livelihoods in Developing Countries. Rome: FAO Fisheries Circular. No. 1008. p. 46. Available online at: http://www.fao.org/3/a-j7551e.pdf
Best, N., Richardson, S., and Thomson, A. (2005). A comparison of Bayesian spatial models for disease mapping. Stat. Methods Med. Res. 14, 35–39. doi: 10.1191/0962280205sm388oa
Bjorge, A., Skern-Mauritzen, M., and Rossrnan, M. C. (2013). Estimated bycatch of harbour porpoise (Phocoena phocoena) in two coastal gillnet fisheries in Norway, 2006-2008. Mitigation Implications Conserv. Biol Conserv. 161, 164–173. doi: 10.1016/j.biocon.2013.03.009
Brewer, T. (2013). Dominant discourses, among fishers and middlemen, of the factors affecting coral reef fish distributions in Solomon Islands. Mar. Policy 37, 245–253. doi: 10.1016/j.marpol.2012.05.006
Briscoe, D., Hiatt, S., Lewison, R., and Hines, E. (2014). Modeling habitat and bycatch risk for dugongs in Sabah, Malaysia. Endanger Species Res. 24, 237–247. doi: 10.3354/esr00600
Bunce, A., Norman, F. I., Brothers, N., and Gales, R. (2002). Long-term trends in the Australasian gannet (Morus serrator) population in Australia: the effect of climate change and commercial fisheries. Mar. Biol. 141, 263–269. doi: 10.1007/s00227-002-0838-1
Campbell, L., and Cornwell, M. (2008). Human dimensions of bycatch reduction technology: current assumptions and directions for future research. Endanger Species Res. 5, 325–334. doi: 10.3354/esr00172
Carretta, J. V., and Barlow, J. (2011). Long-term effectiveness, failure rates, and “dinner bell” properties of acoustic pingers in a gillnet fishery. Mar. Technol. Soc. J. 45, 7–19. doi: 10.4031/MTSJ.45.5.3
Caswell, W., Brault, S., Read, A. J., and Smith, T. D. (1998). Harbor porpoise and fisheries: an uncertainty analysis of incidental mortality. Ecol. Appl. 8, 1226–1238. doi: 10.1890/1051-0761(1998)008[1226:HPAFAU]2.0.CO;2
Chan, K. M. A., Guerry, A. D., Balvanera, P., Klain, S., Satterfield, T., Basurto, X., et al. (2012). Where are cultural and social in ecosystem services? A framework for constructive engagement. Bioscience 62, 744–756. doi: 10.1525/bio.2012.62.8.7
Cinner, J. (2011). Social-ecological traps in reef fisheries. Glob. Environ. Change 21, 835–839. doi: 10.1016/j.gloenvcha.2011.04.012
CIRVA (2014). Report of the Sixth Meeting of the Comité Internacional para la Recuperación de la Vaquita. San Diego, CA.
Coulthard, S. (2009). “Adaptation and conflict within fisheries: insights for living with climate change,” in Adapting to Climate Change: Thresholds, Values, Governance, eds W. N. Adger, I. Lorenzoni, and K. L. O'brien (Cambridge: Cambridge University Press), 255–268.
Cox, S. P., Essington, T., Kitchell, J., Martell, S., Walters, C., Christofer Boggs, C., et al. (2002). Reconstructing ecosystem dynamics in the central Pacific Ocean, 1952–1998, II. A preliminary assessment of the trophic impacts of fishing and effects on tuna dynamics. Can. J. Fish. Aquat. Sci. 59, 1736–1747. doi: 10.1139/f02-138
Cox, T. M., Lewison, R. L., Zydelis, R., Crowder, L. B., Safina, C., and Read, A. J. (2007). Comparing effectiveness of experimental and implemented bycatch reduction measures: the ideal and the real. Conserv. Biol. 21, 1155–1164. doi: 10.1111/j.1523-1739.2007.00772.x
Crowder, L. B., Crouse, D. T., Heppell, S. S., and Martin, T. H. (1994). Predicting the impact of turtle excluder devices on loggerhead sea turtle populations. Ecol. Appl. 4, 437–445. doi: 10.2307/1941948
Curtis, K. A., and Moore, J. E. (2013). Calculating reference points for anthropogenic mortality of marine turtles. Aquat. Conserv. 23, 441–459. doi: 10.1002/aqc.2308
Curtis, K. A., Moore, J. E., and Benson, S. R. (2015b). Estimating limit reference points for Western Pacific leatherback turtles (Dermochelys coriacea) in the U. S. West Coast EEZ. PLoS ONE 10:e0136452. doi: 10.1371/journal.pone.0136452
Curtis, K., Moore, J. E., Boyd, C., Dillingham, P., Lewison, R., Taylor, B., et al. (2015a). Managing catch of marine megafauna: guidelines for setting limit reference points. Mar. Policy 61, 249–263. doi: 10.1016/j.marpol.2015.07.002
D'Agrosa, C., Lennert-Cody, C., and Vidal, O. (2000). Vaquita bycatch in Mexico's artisanal gillnet fishery: driving a small population to extinction. Conserv. Biol. 14, 110–119. doi: 10.1046/j.1523-1739.2000.98191.x
Dans, S. L., Alonso, M. K., Pedraza, S. N., and Crespo, E. A. (2003). Incidental catch of dolphins in trawling fisheries off Patagonia, Argentina: can populations persist? Ecol. Appl. 13, 754–762. doi: 10.1890/1051-0761(2003)013[0754:ICODIT]2.0.CO;2
Davies, R. W. D., Cripps, S. J., Nickson, A., and Porter, G. (2009). Defining and estimating global marine fisheries bycatch. Mar. Policy 33:661–672. doi: 10.1016/j.marpol.2009.01.003
Dawson, S. M., Read, A., and Slooten, E. (1998). Pingers, porpoises and power: uncertainties with using pingers to reduce bycatch of small cetaceans. Biol. Conserv. 84, 141–146. doi: 10.1016/S0006-3207(97)00127-4
Dillingham, P. W. (2010). Generation time and the maximum growth rate for populations with age-specific fecundities and unknown juvenile survival. Ecol. Model. 221, 895–899. doi: 10.1016/j.ecolmodel.2009.12.008
Dillingham, P. W., and Fletcher, D. (2008). Estimating the ability of birds to sustain additional human-caused mortalities using a simple decision rule and allometric relationships. Biol. Conserv. 141, 1783–1792. doi: 10.1016/j.biocon.2008.04.022
Dillingham, P. W., and Fletcher, D. (2011). Potential biological removal of albatrosses and petrels with minimal demographic information. Biol. Conserv. 144, 1885–1894. doi: 10.1016/j.biocon.2011.04.014
Dillingham, P., Moore, J., Fletcher, D., Corteìs, E., Curtis, K., James, K., et al. (2015). Improved estimation of intrinsic growth rmax for long-lived species: integrating matrix models and allometry. Ecol. Appl. doi: 10.1890/14-1990.1. [Epub ahead of print].
Estes, J. A., Terborgh, J., Brashares, J. S., Power, M. E., Berger, J., Bond, W. J., et al. (2011). Trophic downgrading of planet earth. Science 333, 301–306. doi: 10.1126/science.1205106
FAO (Food Agriculture Organization of the United Nations). (2009). Guidelines to Reduce Sea Turtle Mortality in Fishing Operations. Fisheries and Aquaculture Department, Rome. Available online at: http://www.fao.org/docrep/012/i0725e/i0725e.pdf
Finkbeiner, E., Wallace, B., Moore, J., Lewison, R., Crowder, L., and Read, A. (2011). Cumulative estimates of sea turtle bycatch and mortality in USA fisheries between 1990 and 2007. Biol. Conserv. 144, 2719–2727. doi: 10.1016/j.biocon.2011.07.033
Fujita, R., Moxley, J. H., Debey, H., Van Leuvan, T., Leumer, A., Honey, K., et al. (2013). Managing for a resilient ocean. Mar. Policy 38, 538–544. doi: 10.1016/j.marpol.2012.05.025
Geijer, C. K. A., and Read, A. J. (2013). Mitigation of marine mammal bycatch in U. S. fisheries since 1994. Biol. Conserv. 159, 54–60. doi: 10.1016/j.biocon.2012.11.009
Gilman, E., Kobayashi, D., Swenarton, T., Brothers, N., Dalzell, P., and Kinan-Kelly, I. (2007). Reducing sea turtle interactions in the Hawaii-based longline swordfish fishery. Biol. Conserv. 139, 19–28. doi: 10.1016/j.biocon.2007.06.002
Gilman, E., Owens, M., and Kraft, T. (2014). Ecological risk assessment of the Marshall Islands longline tuna fishery. Mar. Policy 44, 239–255. doi: 10.1016/j.marpol.2013.08.029
Gislason, G. (2015). “Bycatch management in fisheries: impacts and challenges,” in Fisheries Bycatch: Global Issues and Creative Solutions, eds G. H. Kruse, H.-C. An, J. DiCosimo, C. A. Eischens, G. S. Gislason, D. N. McBride, C. S. Rose, and C. E. Siddon (University of Alaska Fairbanks: Alaska Sea Grant), 25–39.
Hall, M. A., Alverson, D. L., and Metuzals, K. I. (2000). By-catch: problems and solutions. Mar. Pollut. Bull. 41, 204–219. doi: 10.1016/S0025-326X(00)00111-9
Hall, M., Nakano, H., Clarke, S., Thomas, S., Molloy, J., Peckham, S., et al. (2007). “Working with fishers to reduce bycatches,” in Bycatch Reduction in the World's Fisheries, eds S. Kennelly (Dordrecht: Springer-Verlag), 235–288.
Heppell, S. S., Caswell, H., and Crowder, L. B. (2000). Life histories and elasticity patterns: perturbation analysis for species with minimal demographic data. Ecology 81, 654–665. doi: 10.1890/0012-9658(2000)081[0654:LHAEPP]2.0.CO;2
Hobday, A. J., Bell, J. D., Cook, T. R., Gasalla, M. A., and Weng, K. C. (2015). Reconciling conflicts in pelagic fisheries under climate change. Deep Sea Res. II. 113, 291–300. doi: 10.1016/j.dsr2.2014.10.024
Hobday, A. J., Hartog, J. R., Timmis, T., and Fielding, J. (2010). Dynamic spatial zoning to manage southern bluefin tuna capture in a multi-species longline fishery. Fish Oceanogr. 19, 243–253. doi: 10.1111/j.1365-2419.2010.00540.x
Hobday, A. J., Maxwell, S. M., Forgie, J., Mcdonald, J., Darby, M., Seto, K., et al. (2014). Dynamic ocean management: integrating scientific and technological capacity with law, policy and management. Stanf. Environ. Law J. 33, 125–165. Available online at: https://journals.law.stanford.edu/sites/default/files/stanford-environmental-law-journal-selj/print/2014/03/i_hobday_final.pdf
Hobday, A. J., Smith, A. D. M., Stobutzki, I. C., Bulman, C., Daley, R., Dambacher, J. M., et al. (2011). Ecological risk assessment for the effects of fishing. Fish. Res. 108, 372–384. doi: 10.1016/j.fishres.2011.01.013
Howell, E. A., Hoover, A., Benson, S. R., Bailey, H., Polovina, J. J., Seminoff, J. A., et al. (2015). Enhancing the TurtleWatch product for leatherback sea turtles, a dynamic habitat model for ecosystem-based management. Fish Oceanogr. 24, 57–68. doi: 10.1111/fog.12092
Howell, E., Kobayashi, D., Parker, D., Balazs, G., and Polovina, J. (2008). TurtleWatch: a tool to aid in the by- catch reduction of loggerhead turtles Caretta caretta in the Hawaii-based pelagic longline fishery. Endanger Species Res. 5, 267–278. doi: 10.3354/esr00096
IPCC (2014). Climate Change 2014, Impacts, Adaptation and Vulnerability, Part A: Global and Sectoral Aspects. Working Group II contribution to the Fifth Assessment Report of the Intergovernmental Panel on Climate Change (Cambridge: Cambridge University Press).
James, M., Ottensmeyer, C., and Myers, R. (2005). Identification of high-use habitat and threats to leatherback sea turtles in northern waters: new directions for conservation. Ecol. Lett. 8, 195–201. doi: 10.1111/j.1461-0248.2004.00710.x
Jenkins, L. (2007). Bycatch: interactional expertise, dolphins and the US tuna fishery. Stud. Hist. Philos. Sci. 38, 698–712. doi: 10.1016/j.shpsa.2007.09.005
Kelleher, K. (2005). Discards in the World's Marine Fisheries: An Update. Technical Paper. No. 470. Rome: FAO Fisheries. p. 131.
Kerstetter, D. W., and Graves, J. E. (2006). Effects of circle versus J-style hooks on target and non-target species in a pelagic longline fishery. Fish. Res. 80, 239–250. doi: 10.1016/j.fishres.2006.03.032
Keyfitz, N., and Caswell, H. (2005). Applied Mathematical Demography. 3rd Edn. New York, NY: Springer.
Kirby, D. S., and Ward, P. (2014). Standards for the effective management of fisheries bycatch. Mar. Policy 44, 419–426. doi: 10.1016/j.marpol.2013.10.008
Kiszka, J. (2012). Bycatch Assessment of Vulnerable Megafauna in Coastal Artisanal Fisheries in the Southwest Indian Ocean. Final Report to South West Indian Ocean Fisheries Project (SWIOFP).
Larsen, F., and Eigaard, O. R. (2014). Acoustic alarms reduce bycatch of harbour porpoises in Danish North Sea gillnet fisheries. Fish. Res. 153, 108–112. doi: 10.1016/j.fishres.2014.01.010
Levin, S. A., and Lubchenco, J. (2008). Resilience, robustness, and marine ecosystem-based management. Bioscience 58, 27–32. doi: 10.1641/B580107
Lewison, R., and Crowder, L. (2003). Estimating fishery bycatch and effects on a vulnerable seabird population. Ecol. Appl. 13, 743–753. doi: 10.1890/1051-0761(2003)013[0743:EFBAEO]2.0.CO;2
Lewison, R., Crowder, L. B., and Shaver, D. J. (2003). The Impact of turtle excluder devices and fisheries closures on loggerhead and Kemp's ridley strandings in the western Gulf of Mexico. Conserv. Biol. 17, 1089–1097. doi: 10.1046/j.1523-1739.2003.02057.x
Lewison, R., Crowder, L., Read, A., and Freeman, S. (2004). Understanding impacts of fisheries bycatch on marine megafauna. Trends Ecol. Evol. 19, 598–604. doi: 10.1016/j.tree.2004.09.004
Lewison, R. L., Crowder, L. B., Wallace, B. P., Moore, J. E., Cox, T., Zydelis, R., et al. (2014). Global patterns of marine mammal, seabird, and sea turtle bycatch reveal taxa-specific and cumulative megafauna hotspots. PNAS. 111, 5271–5276. doi: 10.1073/pnas.1318960111
Lewison, R., Hobday, A., Maxwell, S., Hazen, E., Hartog, J., Dunn, D., et al. (2015). Dynamic ocean management: identifying the critical ingredients of dynamic approaches to ocean resource management. BioScience. 65, 486–498. doi: 10.1093/biosci/biv018
Lewison, R. L., Soykan, C. U., and Franklin, J. (2009). Mapping the bycatch seascape: multispecies and multi-scale spatial patterns of fisheries bycatch. Ecol. Appl. 19, 920–930. doi: 10.1890/08-0623.1
Lewison, R., Soykan, C., Cox, T., Peckham, H., Pilcher, N., Leboeuf, N., et al. (2011). Ingredients for addressing the challenges of fisheries bycatch. Bull. Mar. Sci. 87, 1–16. doi: 10.5343/bms.2010.1062
Mangel, J. C., Alfaro-Shigueto, J., Van Waerebeek, K., Caceres, C., Bearhop, S., Witt, M. J., et al. (2010). Small cetacean captures in Peruvian artisanal fisheries: high despite protective legislation. Biol. Conserv. 143, 136–143. doi: 10.1016/j.biocon.2009.09.017
Mangel, J. C., Alfaro-Shigueto, J., Witt, M. J., Hodgson, D. J., and Godley, B. J. (2013). Using pingers to reduce bycatch of small cetaceans in Peru's small-scale driftnet fishery. Oryx 47, 595–606. doi: 10.1017/S0030605312000658
Mangel, M. (1993). Effects of high-seas driftnet fisheries on the Northern right whale dolphin (Lissodelphis borealis). Ecol. Appl. 3, 221–229. doi: 10.2307/1941825
Maree, B. A., Wanless, R. M., Fairweather, T. P., Sullivan, B. J., and Yates, O. (2014). Significant reductions in mortality of threatened seabirds in a South African trawl fishery. Anim. Conserv. 17, 520–529. doi: 10.1111/acv.12126
Marsh, H., Penrose, H., Eros, C., and Hugues, J. (2002). Dugong Status Report and Action Plans for Countries and Territories. UNEP Early Warning and Assessment Report UNEP/DEWA/RS.02-1:1–161.
Martin, S. L., Stohs, S. M., and Moore, J. E. (2015). Bayesian inference and assessment for rare-event bycatch in marine fisheries: a drift gillnet fishery case study. Ecol. Appl. 25, 416–429. doi: 10.1890/14-0059.1
Maxwell, S. M., Hazen, E. L., Lewison, R. L., Dunn, D. C., Bailey, H., Bograd, S. J., et al. (2015). Dynamic ocean management: defining and conceptualizing real-time management of the ocean. Mar. Policy 58, 42–50. doi: 10.1016/j.marpol.2015.03.014
McCauley, D. J., Pinsky, M. L., Palumbi, S. R., Estes, J. A., Joyce, F. H., and Warner, R. R. (2015). Marine defaunation: animal loss in the global ocean. Science 347, 247. doi: 10.1126/science.1255641
McCay, B., Weisman, W., and Creed, C. (2011). “Coping with environmental change: systemic responses and the roles of property and community in three fisheries,” in World Fisheries: A Socio- Ecological Analysis, eds R. Ommer, R. Perry, K. Cochrane, and P. Cury (West Sussex: Blackwell Publishing), 381–400. doi: 10.1002/9781444392241.ch23
McCluskey, S., and Lewison, R. (2008). Quantifying fishing effort: a synthesis of current methods and their applications. Fish Fish. 9, 188–200. doi: 10.1111/j.1467-2979.2008.00283.x
McLeod, K., and Leslie, H. (2009). Ecosystem- Based Management for the Oceans. Washington, DC: Island Press.
Melvin, E. F., Parrish, J. K., and Conquest, L. L. (1999). Novel tools to reduce seabird bycatch in coastal gillnet fisheries. Conserv. Biol. 13, 1386–1397. doi: 10.1046/j.1523-1739.1999.98426.x
Miller, T. J., and Skalski, J. R. (2006). Estimation of seabird bycatch for North Pacific longline vessels using design- and model-based methods. Can. J. Fish. Aquat. Sci. 63, 1878–1889. doi: 10.1139/f06-075
Moore, J. E., and Curtis, K. A. (in press). “Developing control rules for threatened bycatch species,” in Management Science in Fisheries: An Introduction to Simulation Based Methods, eds C. T. T. Edwards D. J. Dankel (New York, NY: Taylor & Francis).
Moore, J. E., Cox, T. M., Lewison, R. L., Read, A. J., Bjorkland, R., Mcdonald, S. L., et al. (2010). An interview-based approach to assess marine mammal and sea turtle captures in artisanal fisheries. Biol. Conserv. 143, 795–805. doi: 10.1016/j.biocon.2009.12.023
Moore, J. E., Wallace, B. P., Lewison, R. L., Žydelis, R., Cox, T. M., and Crowder, L. B. (2009). A review of marine mammal, sea turtle and seabird bycatch in USA fisheries and the role of policy in shaping management. Mar. Policy 33, 435–451. doi: 10.1016/j.marpol.2008.09.003
Moore, J., Curtis, K., Lewison, R., Dillingham, P., Cope, J., Fordham, S., et al. (2013). Evaluating sustainability of fisheries bycatch mortality for marine megafauna: a review of conservation reference points for data-limited populations. Environ. Conserv. 40, 329–344. doi: 10.1017/s037689291300012x
Mukherjee, Z. (2015). An economic approach to understanding the international transfer of bycatch from unilateral bycatch reduction policies. Mar. Policy 51, 190–195. doi: 10.1016/j.marpol.2014.07.024
Niel, C., and Lebreton, J.-D. (2005). Using demographic invariants to detect overharvested bird populations from incomplete data. Conserv. Biol. 19, 826–835. doi: 10.1111/j.1523-1739.2005.00310.x
Okada, E., Agostinho, A., and Gomes, L. (2005). Spatial and temporal gradients in artisanal fisheries of a large Neotropical reservoir, the Itaipu Reservoir, Brazil. Can. J. Fish. Aquat. Sci. 62, 714–724. doi: 10.1139/f05-015
Otero, J., Rocha, F., Gonzalez, A., Gracia, J., and Guerra, A. (2005). Modelling artisanal coastal fisheries of Galicia (NW Spain) based on data obtained from fishers: the case of Octopus vulgaris. Sci. Mar. 69, 577–585. doi: 10.3989/scimar.2005.69n4577
Pauly, D. (1997). “Small-scale fisheries in the tropics: marginality, marginalization, and some implications for fisheries management,” in Global Trends: Fisheries Management, eds E. Pikitch, D. Huppert, and M. Sissenwine (Bethesda, MD: American Fisheries Society), 40–49.
Pauly, D. (2006). Major trends in small-scale marine fisheries, with emphasis on developing countries, and some implications for the social sciences. Maritime Stud. 4, 7–22. Available online at: http://www.marecentre.nl/mast/documents/Pauly_Mast2006vol_4no_2_new.pdf
Pauly, D., Christensen, V. V., Dalsgaard, J., Froese, R., and Torres, F. Jr. (1998). Fishing down marine food webs. Science 279, 860–863. doi: 10.1126/science.279.5352.860
Peckham, S., and Maldonado-Diaz, D. (2012). “Empowering small scale fishermen to be conservation heroes: a trinational fishermen's exchange to protect loggerhead turtles,” in Sea Turtles of the Eastern Pacific Ocean: Natural History, Conservation Challenges and Signs of Success, eds J. Seminoff and B. Wallace (Tuscon, AZ: University of Arizona Press), 279–301.
Peckham, S. H., Maldonado-Diaz, D., Walli, A., Ruiz, G., Crowder, L. B., and Nichols, W. J. (2007). Small-scale fisheries bycatch jeopardizes endangered Pacific loggerhead turtles. PLoS ONE 2:e1041. doi: 10.1371/journal.pone.0001041
Phillips, R. A. (2013). Requisite improvements to the estimation of seabird by-catch in pelagic longline fisheries. Anim. Conserv. 16, 157–158. doi: 10.1111/acv.12042
Pinsky, M. L., and Fogarty, M. (2012). Lagged social-ecological responses to climate and range shifts in fisheries. Clim. Change 115, 883–891. doi: 10.1007/s10584-012-0599-x
Pinsky, M., and Mantua, N. (2014). Emerging adaptation approaches for climate- ready fisheries management. Oceanography 27, 146–159. doi: 10.5670/oceanog.2014.93
Piovano, S., Basciano, G., and Swimmer, Y. (2012). Evaluation of a bycatch reduction technology by fishermen: a case study from Sicily. Mar. Policy 36, 272–277. doi: 10.1016/j.marpol.2011.06.004
Polovina, J., Uchida, I., Balazs, G., Howell, E., Parker, D., and Dutton, P. (2006). The Kuroshio Extension Bifurcation Region: a pelagic hotspot for juvenile loggerhead sea turtles. Deep Sea Res. II 53, 326–339. doi: 10.1016/j.dsr2.2006.01.006
Rausser, G., Hamilton, S., Kovach, M., and Stifter, R. (2009). Unintended consequences: the spillover effects of common property regulations. Mar. Policy 33, 24–39. doi: 10.1016/j.marpol.2008.03.020
Read, A. J. (2008). The looming crisis: interactions between marine mammals and fisheries. J. Mammal. 89, 541–548. doi: 10.1644/07-MAMM-S-315R1.1
Rivalan, P., Barbraud, C., Inchausti, P., and Weimerskirch, H. (2010). Combined impacts of longline fisheries and climate on the persistence of the Amsterdam Albatross Diomedia amsterdamensis. Ibis 152, 6–18. doi: 10.1111/j.1474-919X.2009.00977.x
Sarmiento, C. (2006). Transfer function estimation of trade leakages generated by court rulings in the Hawai'i longline fishery. Appl. Econ. 38, 183–190. doi: 10.1080/00036840500368078
Schultz, L., Folke, C., Österblom, H., and Olsson, P. (2015). Adaptive governance, ecosystem management, and natural capital. PNAS 112, 7369–7374. doi: 10.1073/pnas.1406493112
Sims, M., Cox, T., and Lewison, R. (2008). Modeling spatial patterns in fisheries bycatch: improving bycatch maps to aid fisheries management. Ecol. Appl. 18, 649–661. doi: 10.1890/07-0685.1
Small, C., Waugh, S., and Phillips, R. (2013). The justification, design and implementation of ecological risk assessments of the effects of fishing on seabirds. Mar. Policy 37, 192–199. doi: 10.1016/j.marpol.2012.05.001
Soykan, C., Moore, J., Zydelis, R., Crowder, L., Safina, C., and Lewison, R. (2008). Why study bycatch? An introduction to the Theme Section on fisheries bycatch. Endanger Species Res. 5, 91–102. doi: 10.3354/esr00175
Sumaila, U. R., Cheung, W. W. L., Lam, V. W. Y., Pauly, D., and Herrick, S. (2011). Climate change impacts on the biophysics and economics of world fisheries. Nat. Clim. Change 1, 449–456. doi: 10.1038/nclimate1301
Teh, L. S. L., Teh, L. C. L., Hines, E. L., Jumchompoo, C., and Lewison, R. L. (2015). Contextualising the coupled socio-ecological conditions of marine megafauna bycatch. Ocean Coast Manage. 116, 449–465. doi: 10.1016/j.ocecoaman.2015.08.019
Teh, L., Teh, L., and Meitner, M. (2012). Preferred resource spaces and fisher flexibility: implications for spatial management of small-scale fisheries. Hum. Ecol. 40, 213–226. doi: 10.1007/s10745-012-9464-9
Turner, W. R., Bradley, B. A., Estes, L. D., Hole, D. G., Oppenheimer, M., and Wilcove, D. S. (2010). Climate change: helping nature survive the human response. Conserv. Lett. 3, 304–312. doi: 10.1111/j.1755-263X.2010.00128.x
UNEP (2010). Standardised Dugong Catch/By-catch Questionnaire. UNEP/CMS Dugong MoU Abu Dhabi Office.
Veran, S., Gimenez, O., Flint, E., Kendall, W. L., Doherty, P. F., and Lebreton, J. D. (2007). Quantifying the impact of longline fisheries on adult survival in the black-footed albatross. J. Appl. Ecol. 44, 942–952. doi: 10.1111/j.1365-2664.2007.01346.x
Wade, P. R. (1998). Calculating limits to the allowable human-caused mortality of cetaceans and pinnipeds. Mar. Mamm. Sci. 14, 1–37. doi: 10.1111/j.1748-7692.1998.tb00688.x
Wallace, B. P., Lewison, R. L., Mcdonald, S. L., Mcdonald, R. K., Kot, C. Y., Kelez, S., et al. (2010). Global patterns of marine turtle bycatch. Conserv. Lett. 3, 131–142. doi: 10.1111/j.1755-263X.2010.00105.x
Wallace, B., Heppell, S., Lewison, R., Kelez, S., and Crowder, L. (2008). Impacts of fisheries bycatch on loggerhead turtles worldwide inferred from reproductive value analyses. J. Appl. Ecol. 45, 1076–1085. doi: 10.1111/j.1365-2664.2008.01507.x
Wallace, B., Kot, C., Dimatteo, A., Lee, T., Crowder, L., and Lewison, R. (2013). Impacts of fisheries bycatch on marine turtle popilations worldwide: toward conservation and research priorities. Ecosphere 4, 1–49. doi: 10.1890/ES12-00388.1
Watson, J. W., Epperly, S. P., Shah, A. K., and Foster, D. G. (2005). Fishing methods to reduce sea turtle mortality associated with pelagic longlines. Can. J. Fish. Aquat. Sci. 62, 965–981. doi: 10.1139/f05-004
Whitty, T., Davis, P., Poonian, C., and Leandre, I. (2010). “Rapid assessment of marine megafauna capture, fishing effort, and socioeconomic and cultural drivers of artisanal fisheries in northern Madagascar,” in Proceedings of the World Small Scale Fisheries Congress (Bangkok).
Yeh, Y., Huang, H., Dietrich, K., and Melvin, E. (2013). Estimates of seabird incidental catch by pelagic longline fisheries in the South Atlantic Ocean. Anim. Conserv. 16, 141–152. doi: 10.1111/j.1469-1795.2012.00588.x
Zador, S. G., Punt, A. E., and Parrish, J. K. (2008). Population impacts of endangered short-tailed albatross bycatch in the Alaskan trawl fishery. Biol. Conserv. 141, 872–882. doi: 10.1016/j.biocon.2008.01.001
Keywords: marine megafauna, bycatch, social-ecological systems, marine conservation, sustainable fisheries, global change
Citation: Komoroske LM and Lewison RL (2015) Addressing fisheries bycatch in a changing world. Front. Mar. Sci. 2:83. doi: 10.3389/fmars.2015.00083
Received: 12 August 2015; Accepted: 02 October 2015;
Published: 27 October 2015.
Edited by:
Paul Snelgrove, Memorial University of Newfoundland, CanadaReviewed by:
Konstantinos Tsagarakis, Hellenic Centre for Marine Research, GreeceCopyright © 2015 Komoroske and Lewison. This is an open-access article distributed under the terms of the Creative Commons Attribution License (CC BY). The use, distribution or reproduction in other forums is permitted, provided the original author(s) or licensor are credited and that the original publication in this journal is cited, in accordance with accepted academic practice. No use, distribution or reproduction is permitted which does not comply with these terms.
*Correspondence: Lisa M. Komoroske, bGlzYS5rb21vcm9za2VAbm9hYS5nb3Y=
Disclaimer: All claims expressed in this article are solely those of the authors and do not necessarily represent those of their affiliated organizations, or those of the publisher, the editors and the reviewers. Any product that may be evaluated in this article or claim that may be made by its manufacturer is not guaranteed or endorsed by the publisher.
Research integrity at Frontiers
Learn more about the work of our research integrity team to safeguard the quality of each article we publish.