- 1Programa de Pós-Graduação em Ecologia, Instituto de Biologia, Universidade Estadual de Campinas, Campinas, São Paulo, Brazil
- 2Departamento de Biologia Animal, Instituto de Biologia, Universidade Estadual de Campinas, Campinas, São Paulo, Brazil
Macroevolutionary studies using the fossil record have provided valuable information about the evolutionary history of mammals, helping us to understand some of the processes underlying shifts in diversification dynamics. Yet, most studies on mammal diversification have focused on the Northern Hemisphere. The general view that the quality of the fossil record of South American clades is too limited has precluded continental-level macroevolutionary studies in the continent. However, to adequately evaluate how much we can learn from the South American fossil record, we need to understand how the limitations of the fossil record affect the uncertainty of macroevolutionary estimates. Here, we investigated the spatiotemporal distribution of fossil occurrences from eleven South American mammalian clades and used a Bayesian approach that accounts for the incompleteness of the fossil record to analyze how estimates of times of origination and extinction, and origination and extinction rates are affected by the quality of the fossil record. We show that the main shortcoming of the South American mammalian fossil record is not its overall quality but its spatiotemporal unevenness. Most early South American and early immigrant clades have lower preservation rates than late immigrant clades. Accordingly, uncertainty in root age and estimates of times of origination and extinction is larger for earlier South American clades. Despite the limitations of the fossil record, we were still able to identify significant rate shifts throughout the diversification of most South American clades that may be explained by environmental changes. Yet, we also find discrepancies with macroevolutionary patterns inferred from phylogenies, which suggest that some of the patterns we detect with fossils might reflect regional macroevolutionary trends or be driven by lineages with higher preservation. Contrasting the results obtained using different approaches, such as rate estimates from fossils and molecular data, to find where they converge and diverge, may help to delineate the spatial scale and phylogenetic scope of observed macroevolutionary patterns. Our work contributes to a better understanding of the limitations and opportunities in the research about the evolution of South American mammals
Introduction
The fossil record is the only direct evidence of the past biota and is essential in studies aiming to uncover long-term variations in biodiversity or to reconstruct the diversification dynamics of specific clades (Alroy, 1996; Foote, 2001; Quental and Marshall, 2010; Marshall, 2017). Yet, fossils offer a fragmentary view of the past (Raup, 1972; Kidwell and Holland, 2002; Vilhena and Smith, 2013), as not all traces of changes in biodiversity through time are preserved as fossils (Foote and Raup, 1996; Foote, 2000; Hagen et al., 2018). The fossil record is subject to different types of biases, such as variation in preservation potential, differential sampling across environments and geographical regions, and uneven representativeness of time periods in the geological record (Kidwell and Holland, 2002; Vilhena and Smith, 2013; Holland, 2016; Benson et al., 2021). All of those sources of biases affect the completeness of the fossil record, and influence our ability to understand macroevolutionary patterns and the processes underlying those patterns.
Origination and extinction rates can be estimated from dated fossil occurrences to assess diversification dynamics (Foote, 2000). Yet, because the first and last appearances of a taxon in the fossil record are very unlikely to accurately represent its origination and extinction (Signor-lipps effect; Signor and Lipps, 1982; Foote and Miller, 2006), statistical approaches that correct estimates of origination and extinction times according to fossil recovery rate, the rate at which fossils representing a taxon are sampled between the first and last appearance, need to be used to account for uncertainty (e.g., Alroy, 2000; Silvestro et al., 2014a, b; Mitchell and Rabosky, 2017; Silvestro et al., 2019a). Fossil recovery rate depends on the abundance of that taxon throughout its lifetime, preservation potential, which depends on traits such as body size, and the presence of hard parts, and sampling (Schopf, 1975). The higher the recovery rate, the smaller the uncertainty associated with origination and extinction times, but even groups with high quality fossil records are subject to some degree of uncertainty in origination and extinction estimates.
When compared to other groups, mammals have an excellent fossil record (Quental and Marshall, 2013), and macroevolutionary studies were able to reveal long-term patterns in mammalian diversification, such as trends in body size evolution (Alroy, 1998, 1999; Sanisidro et al., 2023), and the role of climate (Vrba, 1992; Janis, 2003; Barnosky et al., 2003; Cantalapiedra et al., 2021; Porto et al., 2023) and biotic interactions (Silvestro et al., 2015; Cantalapiedra et al., 2017; Pires et al., 2017; Nascimento et al., 2024) in shaping the evolutionary history of different mammalian clades. However, most macroevolutionary studies of mammal clades are focused on organisms from the Northern Hemisphere due to the incompleteness of the fossil record in some areas of the planet (Žliobaitė and Fortelius, 2022) and to historical differences in sampling effort across continents (Raja et al., 2022). This is particularly true for South American mammals (Kohn et al., 2015; Gomes Rodrigues et al., 2017; Solórzano and Núñez-Flores, 2021). There is a general understanding that the quality of the fossil record of South American clades is too limited, either in terms of spatial and temporal coverage, or the accuracy of fossil dating, to allow macroevolutionary inferences (Patterson and Pascual, 1968; Prevosti et al., 2013; Bennett et al., 2018; Carrillo et al., 2020). Despite those limitations, the South American fossil record of mammals has allowed paleontologists and geologists to reconstruct large scale phenomena that shaped the South American mammal fauna.
Throughout the Cenozoic, South America was relatively isolated from other continents [Simpson (1980), but see Pascual (2006)]. During this period of isolation, endemic mammalian groups diversified, such as the ungulates Notoungulata and Litopterna, which have no surviving members, the elusive Xenarthra, whose largest taxa like ground sloths and glyptodons did not survive the Pleistocene megafaunal extinction, and a unique and diverse fauna of metatherians that included the extinct carnivores within Sparassodonta and the Didelphimorphia, which still bears high diversity of extant taxa (Patterson and Pascual, 1968; Simpson, 1980; Webb, 1985; Pascual, 2006). Sporadic dispersal events introduced the ancestors of early immigrant clades into South America, such as the Caviomorph rodents and New World monkeys (Platyrrhini) (Pascual, 2006; Oliveira et al., 2009; Antoine et al., 2011; Croft, 2012; Goin et al., 2012). The relative isolation of South America eventually ended with the Great American Biotic Interchange (GABI) as the gradual closing of the Panama Isthmus consolidated the land bridge that allowed several waves of migration between South and North American faunas (Simpson, 1980; Marshall et al., 1982; Stehli and Webb, 1985; Woodburne et al., 2006; Woodburne, 2010). Among the new migrants from the North were several orders of placental mammals, such as Carnivora, Artiodactyla, and Perissodactyla, which established themselves alongside the native fauna (Marshall et al., 1982; Webb, 1991). After the GABI, there appears to be a significant increase in the diversification of mammalian lineages of North American origin in South America (Webb and Marshall, 1982; Webb, 2006; Carrillo et al., 2020). This led to a higher richness of species with North American origin relative to that of mammals originating in South America in the recent fauna. Even though such evolutionary and ecological dynamics were inferred based on the mammalian South American fossil record, several uncertainties about the diversification of those groups persist.
While the mammalian South American fossil record is the most abundant among the continents in the southern hemisphere (Goin et al., 2012), it has been sampled unevenly in space and time (Croft, 2012; Carrillo et al., 2015, 2020). The tropics are especially underrepresented in the fossil record, with an overrepresentation of high latitude sites (Patterson and Pascual, 1968; Croft, 2012; Goin et al., 2012; Carrillo et al., 2015; Bacon et al., 2015). Since the fossil record comes primarily from lowland sedimentary areas (Holland, 2016; Holland et al., 2022), changes in geomorphology may have also altered the preservation potential of fossils over time, aggravating biases in the fossil record. The Andes started rising around 40 million years ago, with rapid changes later on (1 to 4 million years ago) (Garzione et al., 2008), which would have contributed to fluctuations in the chances of accommodation of sediment and consequently, of fossil preservation (Croft, 2012). The rise of the Andes, alongside the establishment of the Circumpolar and Humboldt oceanic currents, also increased temperate and arid conditions by the Pliocene-Pleistocene transition (Patterson and Pascual, 1968; Hinojosa, 2005; Goin et al., 2012; Toledo et al., 2015), further altering climatic conditions and impacting fossil preservation potential.
To comprehend how the variation in the completeness of the fossil record affects our knowledge about the evolution of mammals in South America, we need to quantify the impact of those biases in macroevolutionary inferences about South American mammalian clades. In the present study, we first examine the spatiotemporal distribution of fossil occurrences from eleven mammalian clades (Artiodactyla, Carnivora, Caviomorpha, Cingulata, Didelphimorphia, Folivora, Litopterna, Notoungulata, Perissodactyla, Primates, and, Sparassodonta) present in South America from the early Cenozoic to late Quaternary. Next, we analyzed how the quality of the fossil record of each clade affected estimates of origination and extinction times and origination and extinction rates using a Bayesian framework that accounts for time variation in fossil recovery rates (Silvestro et al., 2014a, b; Silvestro et al., 2019a). Our work contributes to understanding how much we can learn about the evolutionary dynamics of South American mammals despite the various limitations of the fossil record.
Methods
Data acquisition and curation
We obtained fossil occurrence data for eleven mammalian clades from South America (Artiodactyla, Carnivora, Caviomorpha, Cingulata, Didelphimorphia, Folivora, Litopterna, Notoungulata, Perissodactyla, Primates, and Sparassodonta) from the Paleobiology Database (PBDB, https://paleobiodb.org/). We included only occurrences identified at the species level. This criterion was adopted due to the excellent preservation of cenozoic mammals in comparison to other groups (Quental and Marshall, 2013) and because our focus was to evaluate how much we can infer about the evolution of South American mammalian clades utilizing fossil occurrences that would allow for a higher taxonomic resolution. We further excluded occurrences in which taxonomy presented uncertainty, i.e., where species or genera were marked with qualifiers such as sp., cf., aff., and ‘?’. The database was curated to verify the validity of the species included and correct synonyms or, in any other way, invalid specific names based on an extensive literature review.
Diversification analysis
We analyzed the macroevolutionary dynamics of the clades using the Bayesian approach implemented in PyRate (Silvestro et al., 2014a, b; Silvestro et al., 2019a). PyRate estimates the times of speciation and extinction for each species, while accounting for uncertainties related to sampling and preservation. PyRate includes three models of preservation: the Homogeneous Poisson process (HPP), Nonhomogeneous Poisson process (NHPP) and Time-variable Poisson process (TPP). For each analyzed clade, we selected the best model of preservation using a Maximum-Likelihood Test (Silvestro et al., 2019a). We combined the selected model with a Gamma model to account for rate heterogeneity across lineages.
In its current implementation, PyRate uses a reversible-jump Monte Carlo Markov Chain (RJMCM) to jointly estimate the times of origination and extinction for each species, from which it estimates the speciation and extinction rates, as well as the preservation rates for the clade (Silvestro et al., 2019a). We ran the RJMCM for 10.000.000 generations or until convergence was achieved. We used Tracer v1.7 (Rambaut et al., 2018) to verify convergence and effective sample sizes (ESS) of the RJMCMC runs, considering that ESS ≥ 200 were indicative of convergence. To account for the dating uncertainties associated with each occurrence, we ran 30 replicates, randomly sampling the age of each occurrence within its range (Silvestro et al., 2014a). All replicates were combined to generate the estimated values of each parameter. The estimated ages of speciation and extinction were used to generate Rate Through Time (RTT) plots and Lineage Through Time (LTT) plots indicating the number of lineages existing at each time point.
Characterizing uncertainty
To measure uncertainty, we calculated the range of the credible interval of the posterior density, using the ci function from the bayestestR v0.14.0 package (Makowski et al., 2019). We measured uncertainty for estimates of root age, times of extinction and speciation, and the net diversification rate of all clades (Zenil-Ferguson and Liow, 2024). By doing this, we are not trying to quantify biases of the fossil record, but to evaluate how the variation in the occurrence of fossils across South America over time impacts macroevolutionary inference.
Results
South American mammalian fossil record
We analyzed a dataset of 5239 fossil occurrences from 1407 species across South America (Figure 1). Caviomorpha (1354 occurrences and 375 species), Notoungulata (1168 occurrences and 306 species), and Cingulata (784 occurrences and 175 species) comprised most of the occurrences and species from the dataset. Perissodactyla had the fewest records (106) and lowest species richness (19) out of all clades. Even though those clades comprise the majority of the occurrences, individual taxa within those and other Early South American clades (Litopterna, Notoungulata, Cingulata, Folivora, and Sparassadonta), and early immigrant clades (Primates and Caviomorpha), are not well represented in the fossil record with the lowest number of records per species (mean of 3.6). Late immigrant clades (Artiodacyla, Carnivora, and Perissodactyla) had the highest number of records per species (mean of 4.7).
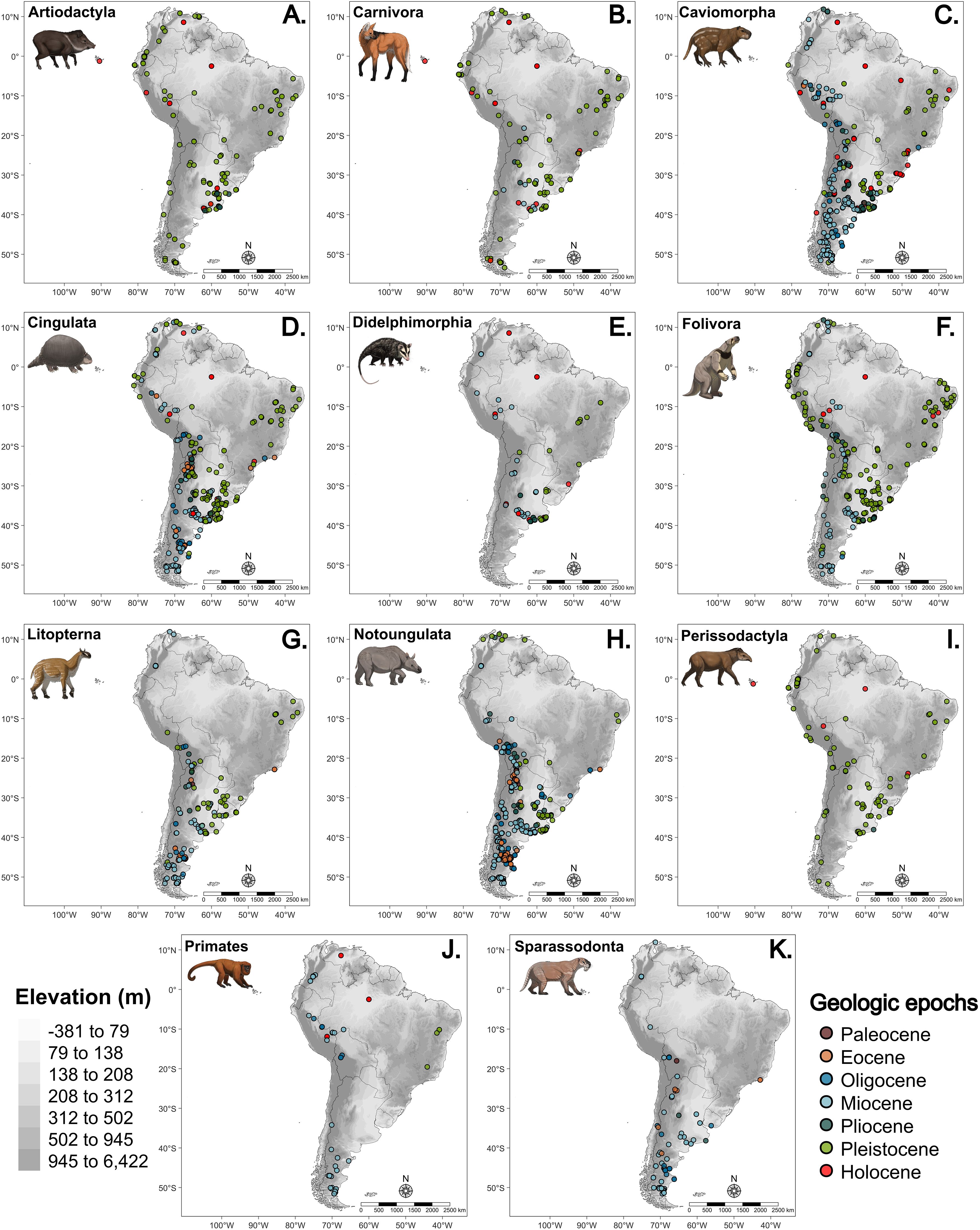
Figure 1. Distribution of fossil occurrences of each South American clade analyzed: (A) Artiodactyla, (B) Carnivora, (C) Caviomorpha, (D) Cingulata, (E) Didelphimorphia, (F) Folivora, (G) Litopterna, (H) Notoungulata, (I) Perissodactyla, (J) Primates, and (K) Sparassodonta. Points are colored according to the estimated geological period of each record. The gray palette represents the current elevation. Art by Felipe C. Coelho.
Younger records comprise the majority of fossil records of mammals in South America. Almost 80% of all occurrences are from the Miocene onwards, from 23.03 million years ago (Ma) to the Holocene, with close to 60% of those from the Miocene. The Paleocene is the least represented era, with only 21 records.
The mammalian fossil record of South America is heavily biased toward the southernmost latitudes (Figure 1, Supplementary Figure S1), and most of the records are located below 1000m (Figure 1, Supplementary Figure S2). All early South American clades, except Folivora, and Caviomorpha have over 70% of records in subtropical or temperate regions (around 20°S or below). Among late immigrant clades Artiodactyla (58%) and Perissodactyla (63%) also have more than half of their records below the tropical region. In contrast, Carnivora had the same proportion of records in subtropical and tropical regions (above 20°S). Primates were the only clade with the highest proportion of records in tropical areas. Most clades showed a significant negative correlation between the number of records and latitude (Supplementary Figure S3), with Folivora (r = -0.88, p = 0.047) and Cingulata (r = -0.83, p = 0.004) having the strongest correlation. Only Carnivora showed a significant positive correlation between the number of records and latitude (r = 0.31, p = 0.002). Most records (72%) are concentrated below 700m, and only Folivora displayed a significant negative correlation between the number of records and elevation (Supplementary Figure S4). Those patterns are also prevalent in most records from each geological era (Supplementary Figures S5, S6).
Fossil preservation and uncertainty
Preservation rates of all analyzed clades increased towards the present (Figure 2). Earlier South American clades, like Litopterna, Notoungulata, and Sparassodonta, had the lowest preservation rates, with lower rates before the Miocene that increased over the Pliocene. Early immigrants, Caviomorpha and Primates, alongside Didelphimorphia, had consistently low preservation rates, with the former having no supported shifts in preservation over time. The preservation rates of late immigrants were considerably higher than those of earlier clades (Figure 2).
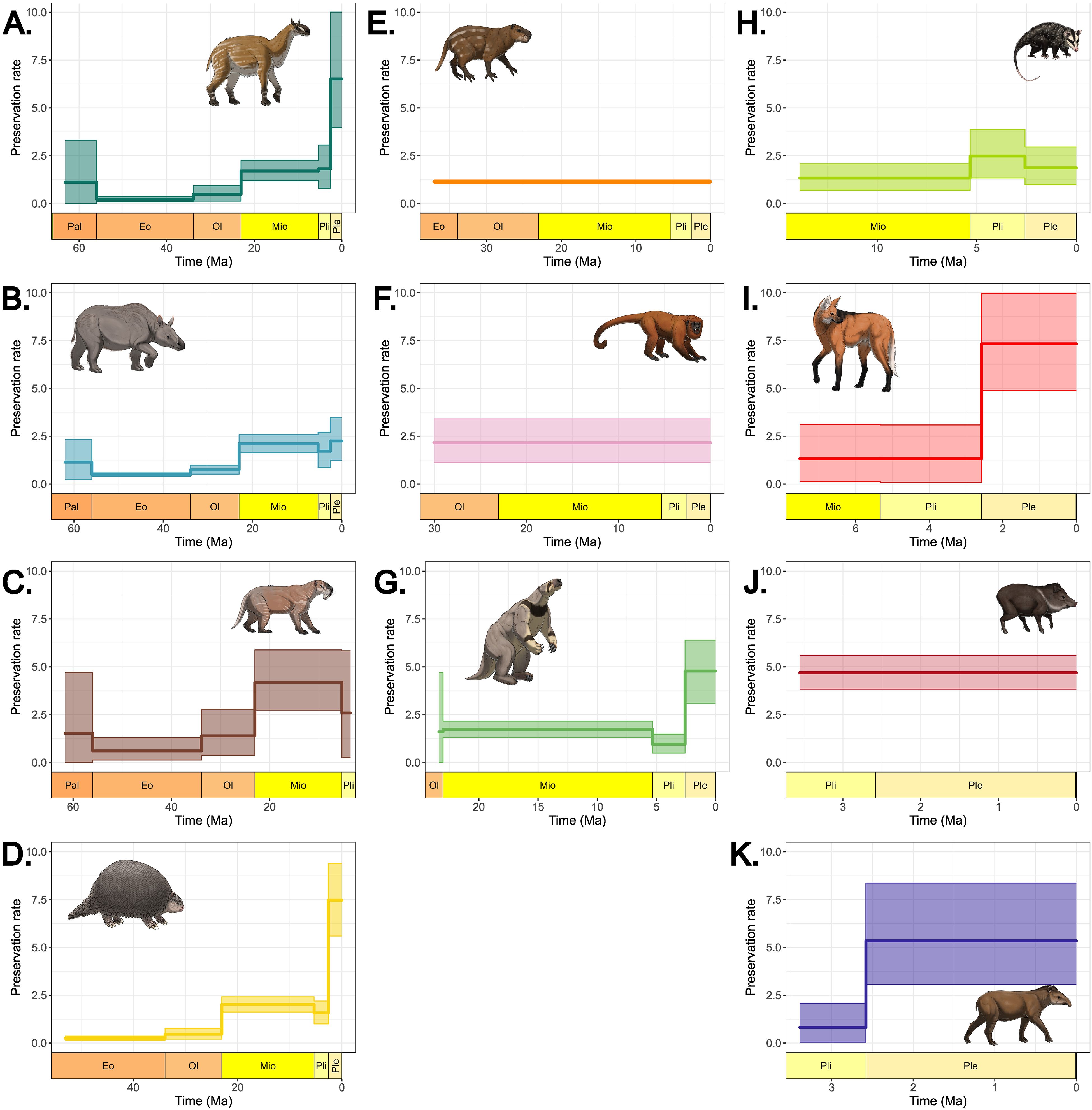
Figure 2. Preservation rates through time computed by PyRate based on of fossil occurrences of taxa within each South American clade analyzed: (A) Litopterna, (B) Notoungulata, (C) Sparassodonta, (D) Cingulata, (E) Caviomorpha, (F) Primates, (G) Folivora, (H) Didelphimorphia, (I) Carnivora, (J) Artiodactyla, and (K) Perissodactyla. Art by Felipe C. Coelho.
Those low preservation rates contribute to an increase in the uncertainty in the estimated root age of the earliest clades. For instance, the 95% Highest Posterior Density (HPD) of the root age of the earliest South American clades, Sparassodonta, Litopterna, and Notoungulata, is defined between 65 and 60 Ma, with a mean variance of 2.75 (Figure 3). The uncertainty in root age can also be seen by the multiple peaks in the posterior densities for root age estimates of some clades, such as Notoungulata, Sparassodonta, Primates, Folivora, and Didelphimorphia. For instance, the origin (migration) of South American primates is estimated at between 30 and 40 Ma to South America, with considerably high uncertainty in the location of the mode of the posterior density (Figure 3). Only Cingulata and Caviomorpha show unimodal posteriors, indicating less uncertainty in the clade’s origination (or immigration) time. The arrival of late immigrant clades is also consistently estimated to have occurred between 8 and 9 Ma for Carnivora and Artiodactyla, and between 4 and 6 Ma for Perissodactyla, with the uncertainty being much more constrained in those cases (mean variance of 0.74).
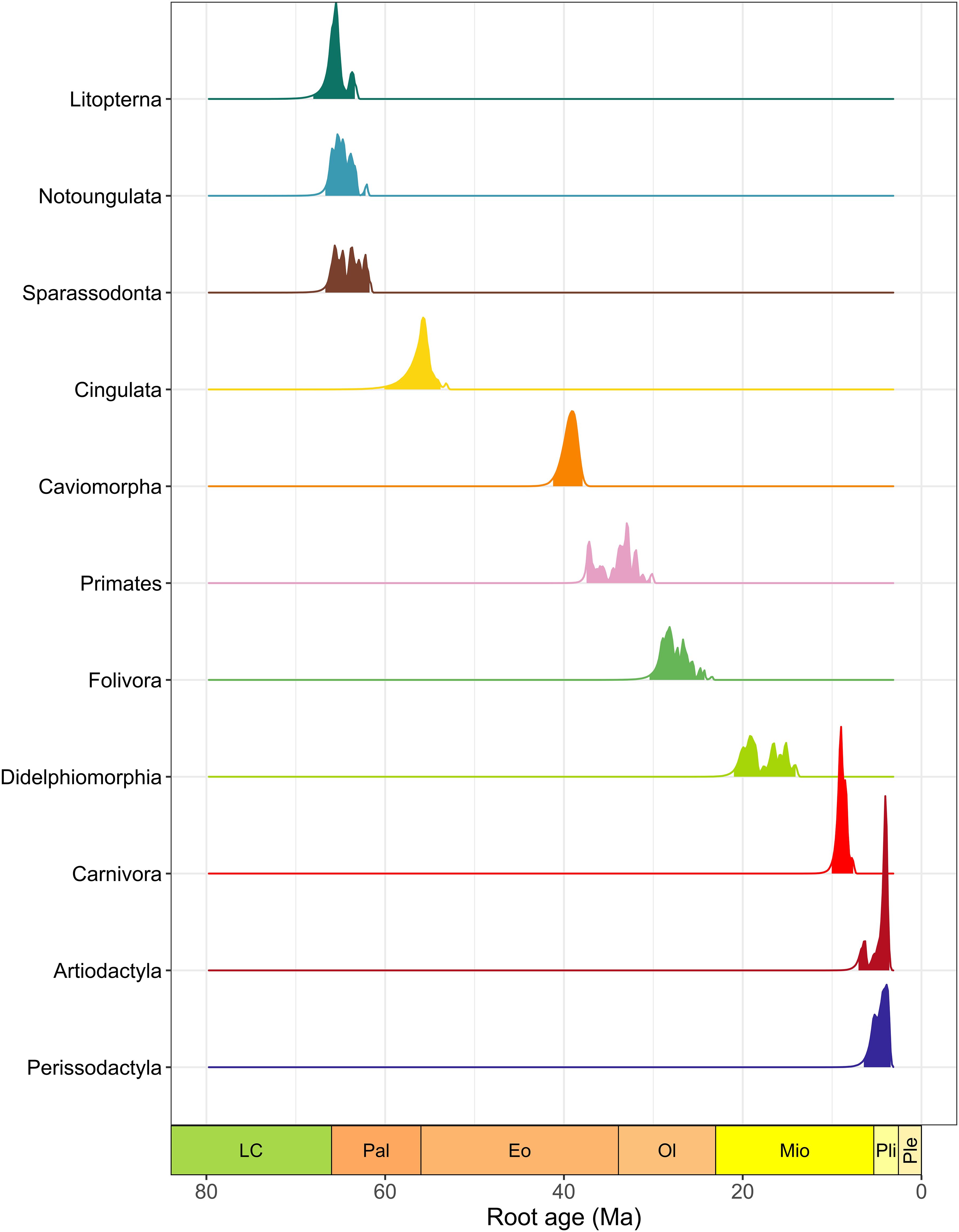
Figure 3. Root age estimates for each South American clade analyzed. Curves represent the Posterior Density Distribution for each clade. Colored areas in the curves represent the 95% Higher Posterior Distribution (HPD) of estimates.
We also detect high variation in the estimates of origination and extinction times for taxa within most clades (Figure 4). Uncertainty in speciation and extinction times, measured by the range of the credible interval, were often higher than 5 Myr for taxa of early originating clades such as Litopterna, Notoungulata, Sparrassodonta, and Cingulata, with values over 10 Myr in some cases. For late arriving clades, estimate uncertainty was more constrained, from 1.47 to 1.66 Myr in the time of speciation and 0.37 to 0.82 Myr in the time of extinction (Figure 4). Of all clades, Perissodactyla had the lowest level of uncertainty in estimates of times of speciation and extinction.
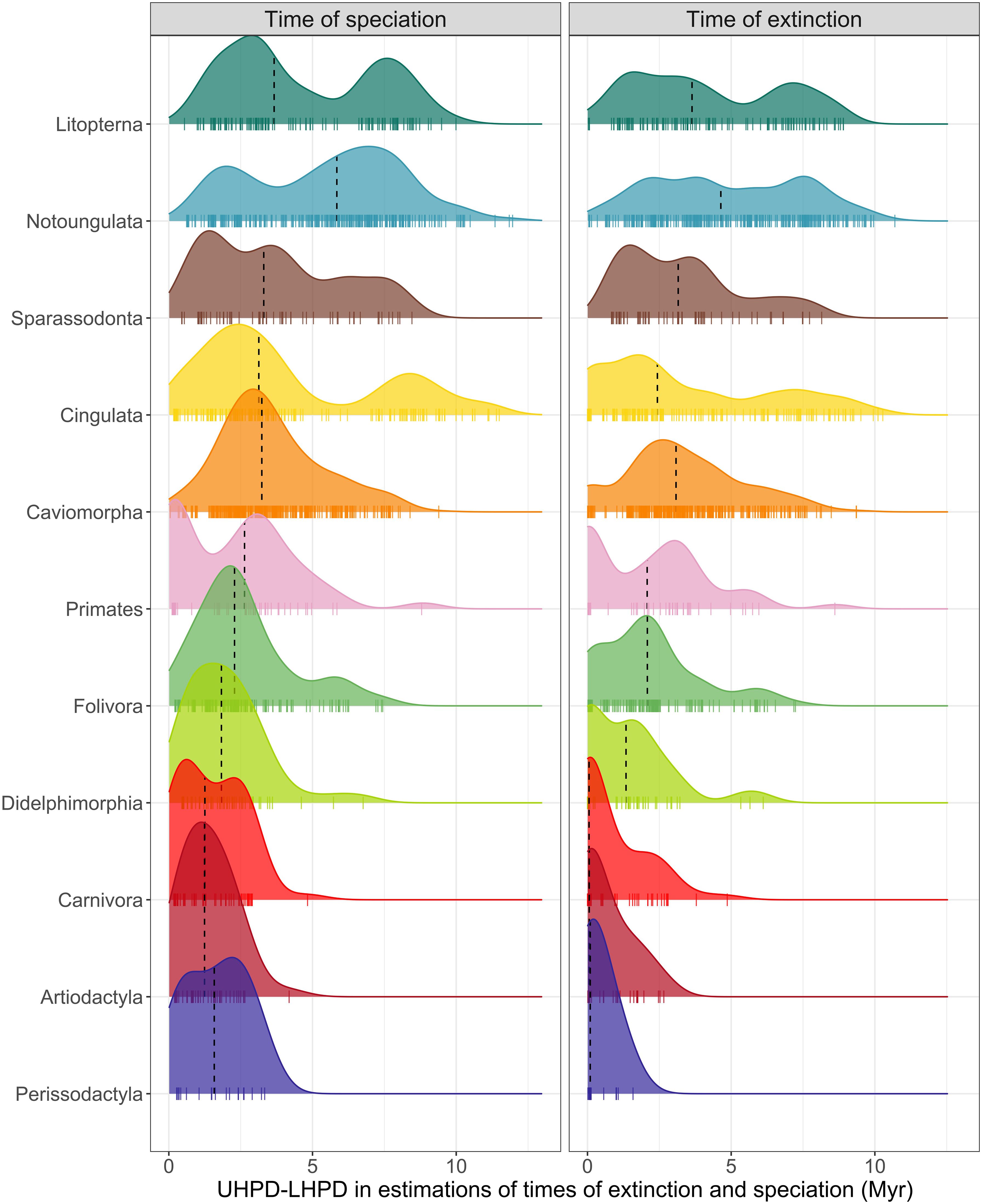
Figure 4. Level of uncertainty, measured as the range of the 95% Higher Posterior Distribution (HPD), in the estimates of times of speciation and extinction for all taxa within each South American clade analyzed. The black dashed line in the density plots represents the median. Small tick marks represent individual values.
Diversification dynamics
Despite the uncertainty in the estimates of times of speciation and extinction, fossil occurrences allowed the identification of significant rate shifts throughout the diversification of most South American clades. The estimates of speciation rates suggest high speciation at the origin of Litopterna and Notoungulata during the Paleocene-Eocene transition, followed by a decrease around 55 Ma (Supplementary Figure S7). Speciation rose again for both clades as well as for Sparasodonta and Cingulata at the Oligocene-Miocene, around 23 Ma. For those clades, however, the rise in speciation was followed by an increase in extinction (Supplementary Figure S8), signaling high species turnover and producing negative diversification rates during the early Miocene (Figure 5). Despite brief moments of positive diversification between 20 and 18 Ma, negative rates persisted for Litopterna, Notoungulata, and Sparassodonta, indicating a steady decline until the extinction of those clades. Folivora and Cingulata underwent a rise in speciation around 20 Ma during the Early Miocene. For Cingulata this increase was partially compensated by an increase in extinction. Extinction rate then remained constant for both clades until a peak during the Early Pleistocene that yielded negative diversification rates when most lineages in both clades became extinct. Although the credible interval for rate estimates is wide for early South American clades, there is statistical support for those shifts for all clades (Supplementary Figure S9).
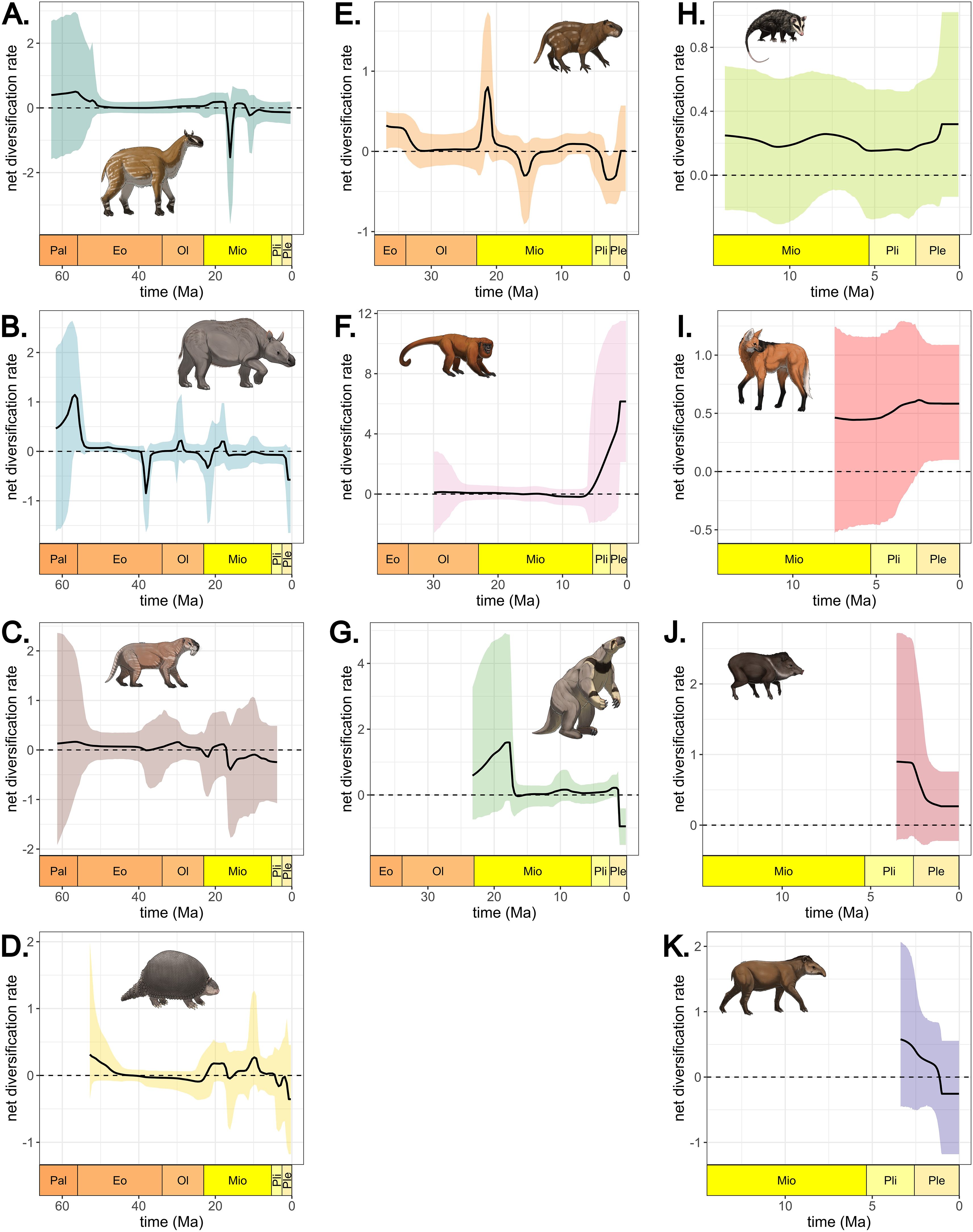
Figure 5. Net diversification rates through time for each South American clade analyzed: (A) Litopterna, (B) Notoungulata, (C) Sparassodonta, (D) Cingulata, (E) Caviomorpha, (F) Primates, (G) Folivora, (H) Didelphimorphia, (I) Carnivora, (J) Artiodactyla, and (K) Perissodactyla. Net diversification rate equals speciation minus extinction rates. Art by Felipe C. Coelho.
The two early migrating clades, Caviomorpha and Primates show disparate diversification trajectories according to our analyses (Figure 5). Contrary to the other clades which entered a period of negative diversification during the Oligocene-Miocene, Caviomorpha underwent a rise in speciation and a decline in extinction at that point (Supplementary Figures S7, S8), which produced a short burst in diversification followed by a drop during the middle Miocene. Extinction rose again in the Pliocene, producing a decline in diversification that was later compensated by an increase in speciation (Supplementary Figures S9, S10). Although primates also immigrated relatively early, with the estimated root age for Platyrrhines at least as early as 30 Ma, our analysis suggests that primates only underwent a burst in speciation around 5 Ma, with no shifts in extinction rates (Supplementary Figure S10), resulting in a late spike in diversification (Figure 5).
None of the late immigrant clades show shifts in speciation rates (Supplementary Figure S11), but speciation was consistently larger than extinction (Supplementary Figures S7, S8), generating positive net diversification throughout most of the history of those clades in South America (Figure 5). The exception is Perissodactyla, which underwent a peak in extinction between 1.5 Ma and 1 Ma, showing a consistent decline in diversification towards the end of the Pliocene.
Uncertainty in diversification rates for early South American endemic clades was especially high closer to their estimated root age, varying from -2 to 2 lineages/Myr for Sparassodonta, for instance. Uncertainty is also high during most rate shifts. The highest level of uncertainty was in magnitude of the rate shift we detect in Primates, varying from below one to 12 lineages/Myr. This indicates that although we can identify when shifts were more likely, there is a high level of uncertainty on the magnitude of some of those shifts.
Discussion
Here, we examined the fossil occurrences of Cenozoic mammals available in PBDB to understand the status of the South American mammalian fossil record and evaluate the limitations its temporal and spatial heterogeneity imposes for macroevolutionary studies. Overall we found that the gaps in fossil occurrences affect the precision of estimates for earlier clades and limit the spatial scope of inferences, but can also reveal macroevolutionary patterns that may be informative about the evolution of the South American mammalian fauna as long as they are interpreted with caution.
Almost all South American endemic clades display a significant negative correlation between number of records and latitude, a pattern already observed in earlier studies (Patterson and Pascual, 1968; Croft, 2012; Goin et al., 2012; Carrillo et al., 2015; Carrillo et al., 2020). We show that this is even more prevalent for older lineages (e.g., Marshall et al., 1982; Rougier et al., 2009) since all records from the Paleocene and most from the Eocene, Oligocene, Miocene, and Pliocene were found around 20°S and below. Fossil occurrences from the Pleistocene and Holocene are more evenly distributed across latitudes, and these periods encompass most records from the tropics. This spatial pattern is likely related to the decrease in fossil preservation potential related to climatic conditions but also depends on the availability of sedimentary outcrops and sampling effort (Foote and Miller, 2006).
The lack of fossil occurrences across South American tropical areas generates an underrepresentation of early lineages of clades that presumably diversified in the tropical region. Didelphimorphia has around 70% of fossil occurrences in temperate areas, yet the highest richness and phylogenetic diversity today is observed in areas of tropical South American forests (Figueiredo and Grelle, 2018). Several studies, using nuclear genes (Jansa et al., 2014), mitochondrial genomes and nuclear loci (Mitchell et al., 2014), and biogeographical reconstruction (Castro et al., 2021) indicate that the origin and early diversification of Didelphidae occurred in humid forests. This also seems to apply for Caviomorpha, which has about 80% of its fossil occurrences below 20°S, but may have diversified in tropical latitudes. Fossil discoveries from the Amazonian rainforest dated to the middle Eocene are interpreted as an indication that the clade only dispersed to higher latitudes after diversifying in low latitudes (Antoine et al., 2011). However, the spatiotemporal distribution of the fossil record for such clades does not necessarily mirror this complex evolutionary trajectory nor the diversification of tropical lineages. Even for more recent periods, tropical lineages are still underrepresented and, considering that current diversity is considerably greater in the tropics for many lineages, that displays how the variation in preservation and sampling across temperate and tropical areas might induce a misinterpretation of earlier diversity spatial patterns and diversification dynamics. The expectation for the distribution of the fossil occurrences of Caviomorpha and Didelphimorphia, and other South American clades that seem to have diversified in the tropics, is that fossils of tropical lineages would be more frequent than those of temperate ones, which is not the case. An important implication of those patterns is that most of the estimates of diversification rates may represent the dynamics of lineages of southernmost latitudes within each clade and may not be general for the entire clade. Whether or not the lineages with a predominantly tropical distribution followed the same trends cannot be answered with analysis based on the current data.
We also tested the relationship between the number of records and elevation for all clades, and only Folivora displayed a significant negative correlation. However, the majority of South American fossils were found below 1000m. Based on current diversity distribution patterns, a higher frequency of fossil occurrences in lowlands is expected, since species richness often decreases with altitude (McCain and Grytnes, 2010). Yet, preservation probability is also negatively affected by altitude because of erosion and geological processes (Holland et al., 2022) and the paucity of fossils in higher altitudes could limit the knowledge about the lineages that evolved in higher elevations, besides impacting our understanding about macroevolutionary phenomena such as radiations driven by mountain uplifts (Perrigo et al., 2020). Considering how the Andes uplift could have also affected the preservation potential of fossils (Croft, 2012), this underscores the importance of yet another source of uncertainty related to altitude in one of the most diverse and notorious mammalian fossil records known today. Consequently, diversity patterns and their effects on the diversification history of mammals are subject to be misrepresented due to the spatial patchiness of the fossil record on a regional scale (Benson et al., 2021).
We can detect the effects of the spatial unevenness of the fossil record when contrasting the patterns we observed for the entire South America with the results of previous studies that focused on specific regions. For example, our diversification rate estimates for notoungulates reveal steep increases and decreases in net diversification rate that differ from those found in a recent analysis on the diversification of Notoungulata focusing solely on the southern region of South America (Solórzano et al., 2024). We observed increased speciation and extinction rates during the Paleocene, which are absent in Solórzano et al. (2024). Additionally, the first steep decrease and increase in diversification rates occurred close to 10 and 5 Myr earlier, respectively, and with lower values than those observed by Solórzano et al. (2024). Including records from the entire continent, especially from earlier periods, may have pushed back and decreased the magnitude of estimated rate shifts in their diversification rates. However, we still found roughly similar patterns for later periods, like the early Miocene peak in diversification driven by increased speciation. Considering the gap in the record from tropical regions and high altitudes (Solórzano and Núñez-Flores, 2021), spatially restricting the analysis may be the best practice. The extent to which those patterns apply to tropical lineages, however, is difficult to assess.
A similar problem applies to all South American clades but may be more relevant for those with worse representation across their range. Sparassodonta, for instance, had only 30 records from tropical regions. With so few records from tropical areas, inferring changes in the diversification history of the clade for the entire continent is not advisable. The patterns we detect are close to those obtained by Pino et al. (2022) and Tarquini et al. (2022) but differ from those obtained by Croft et al. (2018). Analyzing Sparassodonta diversity within a single region, the latter study found an increase in diversity during the Middle Miocene, whereas our analyses suggest that the clade was already in decline at that point. Such disparities may signal that the clade diversification underwent different dynamics in different regions and reinforce that extrapolating observed macroevolutionary patterns across scales can be misleading.
Of all clades analyzed in this study, New World monkeys (Primates: Platyrrhine) presented the largest divergence in our analysis when compared to previous work, most likely as a consequence of the limited fossil record (Žliobaitė and Fortelius, 2022). Additionally, the taxonomic classification of platyrrhine fossils has been discussed among scholars for decades, which increases the uncertainty in macroevolutionary inference based solely on the fossil record. Platyrrhine had the highest uncertainty in the estimated root age, suggesting it to be between 38 and 31 Ma, with estimates around 33 Ma for most replicates. Interestingly, this estimate and the level of uncertainty coincide with estimates using phylogenetic approaches (Beck et al., 2023). After the discovery of a fossil from the late Eocene (Bond et al., 2015), Silvestro et al. (2019b) combined molecular and paleontological evidence and suggested platyrrhine origination at 43 Ma (95% CI: 37.64–50.77 Ma). This has significant implications for understanding the evolutionary history of the group, since platyrrhines could have originated in Africa, dispersed to South America, and then became extinct in the continent of origin (Silvestro et al., 2019b; Bond et al., 2015). The main contrast with previous work, however, is that here we identify a late burst in Platyrrhini diversification, whereas earlier work using molecular data (Perez et al., 2013) suggested an early radiation of platyrrhines with a recent slowdown in diversification (Aristide et al., 2015). This divergence may be a product of the biases in the fossil record. Primates, especially small bodied species, have low fossil recovery rates, which is also associated with their habitat preferences and arboreality. If the group underwent an earlier radiation in the tropics, the analysis of the fossil record may not be able to detect it, even though the signal may be assessed from molecular data. Additionally, there is a lack of platyrrhine intermediate forms in the fossil record, which hinders the understanding of the relationship between groups and their evolutionary history (Schrago, 2007). In that sense, the patterns we uncover here might not be representative of the entire clade, but influenced by those groups with greater representativeness in the record. This highlights an important aspect of macroevolutionary analysis using fossils: certain lineages within a clade might disproportionately affect observed patterns as a function of variation in preservation potential, demanding a caution when interpreting patterns and trends.
Another temporal incongruence emerges when contrasting the fossil and phylogenetically informed evolution of sloths (Folivora). Although the estimated root age based on fossil occurrences suggests the clade originated and radiated during the late Oligocene, recent phylogenetic studies point towards a much earlier origin in the late Eocene (Tejada et al., 2024). The most likely reason for this discrepancy is the fossil gap in tropical regions; whereas the fossil record suggests the clade originated in Patagonia, biogeographical reconstructions based on molecular evidence indicate an earlier origin that could have occurred in the Amazon, although fossil evidence in the tropics is limited to a few fragments (Tejada et al., 2024). Incongruences between macroevolutionary hypotheses supported by fossils and molecular techniques can point to limitations of either type of evidence. Recent advances in the assembly of molecular (e.g., Voloch et al., 2013; Welker et al., 2015; Westbury et al., 2017; Presslee et al., 2019) and morphological (e.g., Forasiepi, 2009; Chimento and Agnolin, 2020; Perini et al., 2022; Casali et al., 2022; Püschel et al., 2024) phylogenies will be important to validate estimated patterns as well as to detect unsettled questions in the evolution of South American clades.
Despite the limitations imposed by the gaps in the South American fossil record, it is still possible to detect macroevolutionary patterns that seem to be explained by climatic and geological phenomena. For all earlier clades, we identify more complex diversification dynamics, with greater variation in speciation and extinction rates, during early and middle Miocene. The early Miocene is marked by climatic oscillations (Zachos et al., 1997), with corresponding shifts in the composition of vegetation in South America, when grass-dominated open habitat begins to expand (Barreda and Palazzesi, 2007; Strömberg et al., 2013). Those changes in climate and vegetation seem to correspond with increases in both speciation and extinction rates in the contemporary clades, generating high species turnover and marking the onset of the declining phase of Notoungulata, Litopterna, and Sparassadonta, while seemingly favoring the diversification of Cingulata, Folivora, and Caviomorpha. Temperatures rise during the middle Miocene, with warm, humid conditions peaking between 17 and 15 Ma, the Middle Miocene Climatic Optimum (Croft et al., 2016). Those changing conditions seem to coincide with the largest drops in the diversification of Litopterna, Sparassodonta, and Caviomorpha, but precede a peak in the diversification of Cingulata. After the Miocene Climatic Optimum, global climate underwent a steady phase of cooling and aridification, shaping the current climatic latitudinal gradients and biogeographical patterns (Herbert et al., 2016). The uplift of the Eastern part of the Andes between early and late Miocene and marine transgressions in Patagonia (Boschman, 2021) might also have contributed to the observed shifts in diversification, by changing available habitat and creating greater spatial heterogeneity. Although the climate changes during the late Miocene are often associated with major faunal disruptions in other continents (Fortelius et al., 2006), we found no detectable shifts in diversification dynamics of any South American clade during this period. Similarly, although increased aridity during the Pliocene has been related to regional faunal turnovers (Vizcaíno et al., 2004), we only detect extinction peaks during this period in Cingulata and Caviomorpha. This might suggest that the environmental shifts during the early and middle Miocene were more important for reshaping terrestrial mammal diversity in South America than other periods.
The high diversification rates of late immigrant clades suggest continuous radiation since their arrival to South America, but those radiations are not synchronous, supporting a complex history of immigration during the formation of the Panama isthmus (Bacon et al., 2015). Interestingly, while immigrant clades diversified, many of the clades of South American origin were already in a declining phase, which might signal that, instead of outcompeting the incumbent lineages, arriving lineages benefited from the ecological opportunities generated by extinction (Prevosti et al., 2013; Carrillo et al., 2020).
One important caveat is that temporal variation in sampling may also affect some of the temporal patterns we observe in the South American fossil record. The increase in Miocene fossil occurrences for some clades, for instance, may be associated with more adequate preservation conditions caused by climatic or geological phenomena, such as marine transgressions (Cuitiño et al., 2015; 2017), or higher sampling effort in certain formations, such as Ituzaingó Formation in Argentina (Cione et al., 2000). Presumably, this is addressed by the estimated preservation rate, which is accounted for when computing speciation and extinction rates, but large discrepancies in sampling may still create spurious patterns if the number of taxa or occurrences is too low for some temporal windows. It has already been shown that using small datasets tends to increase Bayesian credible intervals in birth-death models (Silvestro et al., 2014b) and may present challenges in accurately estimating rate shifts using PyRate (Černý et al., 2022). One possible alternative is to use a lower taxonomic resolution (eg. genus instead of species level) for the analysis, which increases the number of occurrences and may reduce problems associated with geographical representativeness, but then the results may not be comparable with those of studies analyzing species origination and extinction. Although quantitative approaches that account for preservation, like PyRate are helpful to devise hypotheses about the drivers of diversification trends, a more definite answer about the processes shaping macroevolutionary patterns for South American mammals still requires a more equitable representation of the mammalian fossil record across the continent.
Conclusion
Even though South American mammals are known to have a unique evolutionary history, macroevolutionary studies have historically focused on clades from the Northern Hemisphere. The lack of fossil records from tropical and intertropical regions limits our knowledge about the general diversification patterns in the continent, and macroevolutionary inference might need to be restricted to temperate areas where fossil preservation potential, the number of fossiliferous outcrops, and sampling is higher. Although we detect that variation in the completeness of the fossil record does impact the uncertainty in the estimates of times of speciation and extinction and diversification rates, we were still able to identify diversification patterns that may signal true shifts in diversification rather than effects of changes in preservation. The main shortcoming of the South American fossil record is not its overall quality, but its spatiotemporal unevenness. The patterns we detect reflect the dynamics occurring at southernmost latitudes and may not be representative of the entire continent or entire clades. Discrepancies between estimates using fossils and phylogenetic analysis may stem from the fact that molecular data may better capture the history of tropical lineages. With little information on the fossil record of tropical mammals, we still have a large knowledge gap on the diversification of South American mammals, but we are optimistic that combined approaches that account for those uncertainties may help to shed light on the evolutionary history of South American mammals.
Data availability statement
The datasets presented in this study can be found in online repositories. The names of the repository/repositories and accession number(s) can be found below: The inputs used to conduct the study are openly available at https://doi.org/10.5281/zenodo.13974828, an open-source online data repository hosted at Zenodo.
Ethics statement
Ethical approval was not required for the study involving animals in accordance with the local legislation and institutional requirements because the data used for this study comes from the fossil record, openly available at the Paleobiology Database (PBDB).
Author contributions
PU: Data curation, Formal Analysis, Investigation, Visualization, Writing – original draft, Writing – review & editing. JN: Data curation, Investigation, Writing – review & editing. MP: Conceptualization, Methodology, Writing – review & editing, Project administration, Supervision.
Funding
The author(s) declare that financial support was received for the research, authorship, and/or publication of this article. PU is funded by Fundação de Amparo à Pesquisa do Estado de São Paulo (FAPESP) via grant #2023/15709-7. JN is funded by FAPESP via grant #2021/04220-1. MP is funded by FAPESP via grant #2023/03965-9 and Conselho Nacional de Desenvolvimento Científico e Tecnológico (CNPq) via grant #313059/2022-5.
Acknowledgments
We are grateful to Fundação de Amparo à Pesquisa do Estado de São Paulo (FAPESP) and Conselho Nacional de Desenvolvimento Científico e Tecnológico (CNPq) for the concession of funds that enabled this study. We also acknowledge the reviewers and the editors of the Research Topic: Emergence of Diversity at Different Scales of Space, Time, and Organizational Complexity within Mammalia.
Conflict of interest
The authors declare that the research was conducted without any commercial or financial relationships that could be construed as a potential conflict of interest.
Generative AI statement
The author(s) declare that no Generative AI was used in the creation of this manuscript.
Publisher’s note
All claims expressed in this article are solely those of the authors and do not necessarily represent those of their affiliated organizations, or those of the publisher, the editors and the reviewers. Any product that may be evaluated in this article, or claim that may be made by its manufacturer, is not guaranteed or endorsed by the publisher.
Supplementary material
The Supplementary Material for this article can be found online at: https://www.frontiersin.org/articles/10.3389/fmamm.2024.1518039/full#supplementary-material
References
Alroy J. (1996). Constant extinction, constrained diversification, and uncoordinated stasis in North American mammals. Palaeogeography Palaeoclimatology Palaeoecol. 127, 285–311. doi: 10.1016/S0031-0182(96)00100-9
Alroy J. (1998). Cope’s rule and the dynamics of body mass evolution in North American fossil mammals. Science 280, 731–734. doi: 10.1126/science.280.5364.731
Alroy J. (1999). The fossil record of North American mammals: evidence for a Paleocene evolutionary radiation. Systematic Biol. 48, 107–118. doi: 10.1080/106351599260472
Alroy J. (2000). New methods for quantifying macroevolutionary patterns and processes. Paleobiology 26, 707–733. doi: 10.1666/0094-8373(2000)026<0707:NMFQMP>2.0.CO;2
Antoine P.-O., Croft D., Billet G., Ganerød M., Jaramillo C., Martin T., et al. (2011). Middle Eocene rodents from Peruvian Amazonia reveal the pattern and timing of caviomorph origins and biogeography. Proc. Biol. sciences/The R. Soc. 279, 1319–1326. doi: 10.1098/rspb.2011.1732
Aristide L., Rosenberger A. L., Tejedor M. F., Perez S. I. (2015). Modeling lineage and phenotypic diversification in the New World monkey (Platyrrhini, Primates) radiation. Mol. Phylogenet. Evol. 82, 375–385. doi: 10.1016/j.ympev.2013.11.008
Bacon C. D., Silvestro D., Jaramillo C., Smith B. T., Chakrabarty P., Antonelli A. (2015). Biological evidence supports an early and complex emergence of the Isthmus of Panama. Proc. Natl. Acad. Sci. 112, 6110–6115. doi: 10.1073/pnas.1423853112
Barnosky A. D., Hadly E. A., Bell C. J. (2003). Mammalian response to global warming on varied temporal scales. J. Mammalogy 84, 354–368. doi: 10.1644/1545-1542(2003)084<0354:MRTGWO>2.0.CO;2
Barreda V., Palazzesi L. (2007). Patagonian vegetation turnovers during the Paleogene-Early Neogene: origin of arid-adapted floras. Bot. Rev. 73, 31–50. doi: 10.1663/0006-8101(2007)73[31:PVTDTP]2.0.CO;2
Beck R. M. D., de Vries D., Janiak M. C., Goodhead I. B., Boubli J. P. (2023). Total evidence phylogeny of platyrrhine primates and a comparison of undated and tip-dating approaches. J. Hum. Evol. 174, 103293. doi: 10.1016/j.jhevol.2022.103293
Bennett C. V., Upchurch P., Goin F. J., Goswami A. (2018). Deep time diversity of metatherian mammals: implications for evolutionary history and fossil-record quality. Paleobiology 44, 171–198. doi: 10.1017/pab.2017.34
Benson R. B. J., Butler R., Close R. A., Saupe E., Rabosky D. L. (2021). Biodiversity across space and time in the fossil record. Curr. Biol. 31, R1225–R1236. doi: 10.1016/j.cub.2021.07.071
Bond M., Tejedor M. F., Campbell K. E., Chornogubsky L., Novo N., Goin F. (2015). Eocene primates of South America and the African origins of New World monkeys. Nature 520, 538–541. doi: 10.1038/nature14120
Boschman L. M. (2021). Andean mountain building since the Late Cretaceous: a paleoelevation reconstruction. Earth-Science Rev. 220, 103640. doi: 10.1016/j.earscirev.2021.103640
Cantalapiedra J. L., Prado J. L., Hernández Fernández M., Alberdi M. T. (2017). Decoupled ecomorphological evolution and diversification in Neogene-Quaternary horses. Science 355, 627–630. doi: 10.1126/science.aag1772
Cantalapiedra J. L., Sanisidro Ó., Zhang H., Alberdi M. T., Prado J. L., Blanco F., et al. (2021). The rise and fall of proboscidean ecological diversity. Nat. Ecol. Evol. 5, 1266–1272. doi: 10.1038/s41559-021-01498-w
Carrillo J. D., Faurby S., Silvestro D., Zizka A., Jaramillo C., Bacon C. D., et al. (2020). Disproportionate extinction of South American mammals drove the asymmetry of the Great American Biotic Interchange. Proc. Natl. Acad. Sci. 117, 26281–26287. doi: 10.1073/pnas.2009397117
Carrillo J. D., Forasiepi A., Jaramillo C., Sánchez-Villagra M. R. (2015). Neotropical mammal diversity and the Great American Biotic Interchange: spatial and temporal variation in South America’s fossil record. Front. Genet. 5. doi: 10.3389/fgene.2014.00451
Casali D. M., Boscaini A., Gaudin T. J., Perini F. A. (2022). Reassessing the phylogeny and divergence times of sloths (Mammalia: Pilosa: Folivora), exploring alternative morphological partitioning and dating models. Zoological J. Linn. Soc. 196, 1505–1551. doi: 10.1093/zoolinnean/zlac041
Castro M. C., Dahur M. J., Ferreira G. S. (2021). Amazonia as the origin and diversification area of Didelphidae (Mammalia: Metatheria), and a review of the fossil record of the clade. J. Mamm. Evol. 28, 583–598. doi: 10.1007/s10914-021-09548-7
Černý D., Madzia D., Slater G. J. (2022). Empirical and methodological challenges to the model-based Inference of diversification rates in extinct clades. Systematic Biol. 71, 153–171. doi: 10.1093/sysbio/syab045
Chimento N. R., Agnolin F. L. (2020). Phylogenetic tree of Litopterna and Perissodactyla indicates a complex early history of hoofed mammals. Sci. Rep. 10, 13280. doi: 10.1038/s41598-020-70287-5
Cione A., Azpelicueta M., Bond M., Carlini A., Casciotta J., Cozzuol M., et al. (2000). Miocene vertebrates from Entre Ríos province, eastern Argentina. Serie Correlación Geológica 14, 191–237.
Croft D. A. (2012). “Punctuated isolation: the making and mixing of South America’s mammals,” in Bones, Clones and Biomes: The History and Geography of Recent Neotropical Mammals. Eds. Patterson B. D., Costa L. P. (Chicago: The University of Chicago Press), 9–19.
Croft D. A., Carlini A. A., Ciancio M. R., Brandoni D., Drew N. E., Engelman R. K., et al. (2016). New mammal faunal data from Cerdas, Bolivia, a middle-latitude Neotropical site that chronicles the end of the Middle Miocene Climatic Optimum in South America. J. Vertebrate Paleontology 36, e1163574. doi: 10.1080/02724634.2016.1163574
Croft D. A., Engelman R. K., Dolgushina T., Wesley G. (2018). Diversity and disparity of sparassodonts (Metatheria) reveal non-analogue nature of ancient South American mammalian carnivore guilds. Proc. R. Soc. B: Biol. Sci. 285, 20172012. doi: 10.1098/rspb.2017.2012
Cuitiño J. I., Dozo M. T., del Río C. J., Buono M. R., Palazzesi L., Fuentes S., et al. (2017). “Miocene marine transgressions: paleoenvironments and paleobiodiversity,” in Late Cenozoic of Península Valdés, Patagonia, Argentina: An Interdisciplinary Approach. Eds. Bouza P., Bilmes A. (Springer International Publishing, Cham), 47–84. doi: 10.1007/978-3-319-48508-9_3
Cuitiño J. I., Ventura Santos R., Alonso Muruaga P. J., Scasso R. A. (2015). Sr-stratigraphy and sedimentary evolution of early Miocene marine foreland deposits in the northern Austral (Magallanes) Basin, Argentina. Andean Geology 42, 364–385. doi: 10.5027/andgeoV42n3-a05
Figueiredo M. S. L., Grelle C. E. V. (2018). Phylogenetic diversity as a key to understand mechanisms of New World marsupials diversification (Didelphimorphia: Didelphidae). Oecologia Australis 22, 168–178. doi: 10.4257/oeco.2018.2202.06
Foote M. (2000). Origination and extinction components of taxonomic diversity: General problems. Paleobiology 26, 74–102. doi: 10.1017/S0094837300026890
Foote M. (2001). Inferring temporal patterns of preservation, origination, and extinction from taxonomic survivorship analysis. Paleobiology 27, 602–630. doi: 10.1666/0094-8373(2001)027<0602:ITPOPO>2.0.CO;2
Foote M., Raup D. M. (1996). Fossil preservation and the stratigraphic ranges of taxa. Paleobiology 22, 121–140. doi: 10.1017/S0094837300016134
Forasiepi A. M. (2009). Osteology of Arctodictis sinclairi (Mammalia, Metatheria, Sparassodonta) and phylogeny of Cenozoic metatherian carnivores from South America. Monografías del Museo Argentino Cienc. Naturales “Bernardino Rivadavia”, 6, 1–174.
Fortelius M., Eronen J., Liu L., Pushkina D., Tesakov A., Vislobokova I., et al. (2006). Late Miocene and Pliocene large land mammals and climatic changes in Eurasia. Palaeogeography Palaeoclimatology Palaeoecol. 238, 219–227. doi: 10.1016/j.palaeo.2006.03.042
Garzione C. N., Hoke G. D., Libarkin J. C., Withers S., MacFadden B., Eiler J., et al. (2008). Rise of the Andes. Science 320, 1304–1307. doi: 10.1126/science.1148615
Goin F. J., Gelfo J. N., Chornogubsky L., Woodburne M. O., Martin T. (2012). “Origins, radiations, and distribution of South American mammals,” in Bones, Clones and Biomes: The History and Geography of Recent Neotropical Mammals. Eds. Patterson B. D., Costa L. P. (Chicago: The University of Chicago Press), 20–49.
Gomes Rodrigues H., Herrel A., Billet G. (2017). Ontogenetic and life history trait changes associated with convergent ecological specializations in extinct ungulate mammals. Proc. Natl. Acad. Sci. 114, 1069–1074. doi: 10.1073/pnas.1614029114
Hagen O., Andermann T., Quental T. B., Antonelli A., Silvestro D. (2018). Estimating Age-Dependent Extinction: Contrasting Evidence from Fossils and Phylogenies. Systematic Biology 67, 458–474. doi: 10.1093/sysbio/syx082
Herbert T. D., Lawrence K. T., Tzanova A., Peterson L. C., Caballero-Gill R., Kelly C. S. (2016). Late Miocene global cooling and the rise of modern ecosystems. Nat. Geosci 9, 843–847. doi: 10.1038/ngeo2813
Hinojosa L. F. (2005). Cambios climáticos y vegetacionales inferidos a partir de paleofloras cenozoicas del sur de Sudamérica. Rev. geológica Chile 32, 95–115. doi: 10.4067/S0716-02082005000100006
Holland S. M. (2016). The non-uniformity of fossil preservation. Philos. Trans. R. Soc. B: Biol. Sci. 371, 20150130. doi: 10.1098/rstb.2015.0130
Holland S. M., Loughney K. M., Cone M. (2022). Preferential preservation of low-elevation biotas in the nonmarine fossil record. Geology 51, 111–114. doi: 10.1130/G50579.1
Janis C. (2003). “17 - Tectonics, climate change, and the evolution of mammalian ecosystems,” in Evolution on Planet Earth. Eds. Rothschild L. J., Lister A. M. (Academic Press, London), 319–338. doi: 10.1016/B978-012598655-7/50044-6
Jansa S. A., Barker F. K., Voss R. S. (2014). The early diversification history of didelphid marsupials: a window into South America’s “splendid isolation. Evolution 68, 684–695. doi: 10.1111/evo.12290
Kidwell S. M., Holland S. M. (2002). The quality of the fossil record: implications for evolutionary analyses. Annu. Rev. Ecology Evolution Systematics 33, 561–588. doi: 10.1146/annurev.ecolsys.33.030602.152151
Kohn M. J., Strömberg C. A. E., Madden R. H., Dunn R. E., Evans S., Palacios A., et al. (2015). Quasi-static Eocene–Oligocene climate in Patagonia promotes slow faunal evolution and mid-Cenozoic global cooling. Palaeogeography Palaeoclimatology Palaeoecol. 435, 24–37. doi: 10.1016/j.palaeo.2015.05.028
Makowski D., Ben-Shachar M. S., Lüdecke D. (2019). bayestestR: Describing effects and their uncertainty, existence and significance within the bayesian Framework. J. Open Source Software 4, 1–8. doi: 10.21105/joss.01541
Marshall C. R. (2017). Five palaeobiological laws needed to understand the evolution of the living biota. Nat. Ecol. Evol. 1, 1–6. doi: 10.1038/s41559-017-0165
Marshall L. G., Webb S. D., Sepkoski J. J., Raup D. M. (1982). Mammalian evolution and the great american interchange. Science 215, 1351–1357. doi: 10.1126/science.215.4538.1351
McCain C. M., Grytnes J.-A. (2010). “Elevational gradients in species richness,” in Encyclopedia of Life. (Chichester: John Wiley & Sons, Ltd). doi: 10.1002/9780470015902.a0022548
Mitchell K. J., Pratt R. C., Watson L. N., Gibb G. C., Llamas B., Kasper M., et al. (2014). Molecular phylogeny, biogeography, and habitat preference evolution of marsupials. Mol. Biol. Evol. 31, 2322–2330. doi: 10.1093/molbev/msu176
Mitchell J. S., Rabosky D. L. (2017). Bayesian model selection with BAMM: effects of the model prior on the inferred number of diversification shifts. Methods Ecol. Evol. 8, 37–46. doi: 10.1111/2041-210X.12626
Nascimento J. C. S., Blanco F., Domingo M. S., Cantalapiedra J. L., Pires M. M. (2024). The reorganization of predator–prey networks over 20 million years explains extinction patterns of mammalian carnivores. Ecol. Lett. 27, e14448. doi: 10.1111/ele.14448
Oliveira F. B., Molina E. C., Marroig G. (2009). “Paleogeography of the south Atlantic: a route for Primates and rodents into the New World?,” in South American Primates: Comparative Perspectives in the Study of Behavior, Ecology, and Conservation. Eds. Garber P. A., Estrada A., Bicca-Marques J. C., Heymann E. W., Strier K. B. (Springer, New York, NY), 55–68. doi: 10.1007/978-0-387-78705-3_3
Pascual R. (2006). Evolution and geography: the biogeographic history of South American land mammals. Ann. Missouri Botanical Garden 93, 209–230. doi: 10.3417/0026-6493(2006)93[209:EAGTBH]2.0.CO;2
Patterson B., Pascual R. (1968). The fossil mammal fauna of South America. Q. Rev. Biol. 43, 409–451. doi: 10.1086/405916
Perez S. I., Tejedor M. F., Novo N. M., Aristide L. (2013). Divergence times and the evolutionary radiation of New World monkeys (Platyrrhini, Primates): an analysis of fossil and molecular data. PloS One 8, e68029. doi: 10.1371/journal.pone.0068029
Perini F. A., Macrini T. E., Flynn J. J., Bamba K., Ni X., Croft D. A., et al. (2022). Comparative endocranial anatomy, encephalization, and phylogeny of Notoungulata (Placentalia, Mammalia). J. Mammal Evol. 29, 369–394. doi: 10.1007/s10914-021-09583-4
Perrigo A., Hoorn C., Antonelli A. (2020). Why mountains matter for biodiversity. J. Biogeography 47, 315–325. doi: 10.1111/jbi.13731
Pino K., Vallejos-Garrido P., Espinoza-Aravena N., Cooper R. B., Silvestro D., Hernández C. E., et al. (2022). Regional landscape change triggered by Andean uplift: the extinction of Sparassodonta (Mammalia, Metatheria) in South America. Global Planetary Change 210, 103758. doi: 10.1016/j.gloplacha.2022.103758
Pires M. M., Silvestro D., Quental T. B. (2017). Interactions within and between clades shaped the diversification of terrestrial carnivores. Evolution 71, 1855–1864. doi: 10.1111/evo.13269
Porto L. M. V., Etienne R. S., Maestri R. (2023). Evolutionary radiation in canids following continental colonizations. Evolution 77, 971–979. doi: 10.1093/evolut/qpad015
Presslee S., Slater G. J., Pujos F., Forasiepi A. M., Fischer R., Molloy K., et al. (2019). Palaeoproteomics resolves sloth relationships. Nat. Ecol. Evol. 3, 1121–1130. doi: 10.1038/s41559-019-0909-z
Prevosti F. J., Forasiepi A., Zimicz N. (2013). The evolution of the Cenozoic terrestrial mammalian predator guild in South America: competition or replacement? J. Mammal Evol. 20, 3–21. doi: 10.1007/s10914-011-9175-9
Püschel H. P., Shelley S. L., Williamson T. E., Perini F. A., Wible J. R., Brusatte S. L. (2024). A new dentition-based phylogeny of Litopterna (Mammalia: Placentalia) and ‘archaic’ South American ungulates. Zoological J. Linn. Soc. 202, zlae095. doi: 10.1093/zoolinnean/zlae095
Quental T. B., Marshall C. R. (2010). Diversity dynamics: molecular phylogenies need the fossil record. Trends Ecol. Evol. 25, 434–441. doi: 10.1016/j.tree.2010.05.002
Quental T. B., Marshall C. R. (2013). How the red queen drives terrestrial mammals to extinction. Science 341, 290–292. doi: 10.1126/science.1239431
Raja N. B., Dunne E. M., Matiwane A., Khan T. M., Nätscher P. S., Ghilardi A. M., et al. (2022). Colonial history and global economics distort our understanding of deep-time biodiversity. Nat. Ecol. Evol. 6, 145–154. doi: 10.1038/s41559-021-01608-8
Rambaut A., Drummond A. J., Xie D., Baele G., Suchard M. A. (2018). Posterior summarization in Bayesian phylogenetics using tracer 1.7. Syst. Biol. 67, 901–904. doi: 10.1093/sysbio/syy032
Raup D. M. (1972). Taxonomic diversity during the Phanerozoic. Science 177, 1065–1071. doi: 10.1126/science.177.4054.1065
Rougier G. W., Chornogubsky L., Casadio S., Arango N. P., Giallombardo A. (2009). Mammals from the Allen formation, late cretaceous, Argentina. Cretaceous Res. 30, 223–238. doi: 10.1016/j.cretres.2008.07.006
Sanisidro O., Mihlbachler M. C., Cantalapiedra J. L. (2023). A macroevolutionary pathway to megaherbivory. Science 380, 616–618. doi: 10.1126/science.ade1833
Schopf J. M. (1975). Modes of fossil preservation. Rev. Palaeobotany Palynology 20, 27–53. doi: 10.1016/0034-6667(75)90005-6
Schrago C. G. (2007). On the time scale of New World primate diversification. Am. J. Phys. Anthropology 132, 344–354. doi: 10.1002/ajpa.20459
Signor P., Lipps J. (1982). Sampling bias, gradual extinction patterns and catastrophes in the fossil record. Geological Society of America Special Paper. 190, 291–296. doi: 10.1130/SPE190-p291
Silvestro D., Antonelli A., Salamin N., Quental T. B. (2015). The role of clade competition in the diversification of North American canids. Proc. Natl. Acad. Sci. 112, 8684–8689. doi: 10.1073/pnas.1502803112
Silvestro D., Salamin N., Antonelli A., Meyer X. (2019a). Improved estimation of macroevolutionary rates from fossil data using a Bayesian framework. Paleobiology 45, 546–570. doi: 10.1017/pab.2019.23
Silvestro D., Salamin N., Schnitzler J. (2014a). PyRate: a new program to estimate speciation and extinction rates from incomplete fossil data. Methods Ecol. Evol. 5, 1126–1131. doi: 10.1111/2041-210X.12263
Silvestro D., Schnitzler J., Liow L. H., Antonelli A., Salamin N. (2014b). Bayesian estimation of speciation and extinction from incomplete fossil occurrence data. Systematic Biol. 63, 349–367. doi: 10.1093/sysbio/syu006
Silvestro D., Tejedor M. F., Serrano-Serrano M. L., Loiseau O., Rossier V., Rolland J., et al. (2019b). Early arrival and climatically-linked geographic expansion of New World monkeys from tiny African ancestors. Systematic Biol. 68, 78–92. doi: 10.1093/sysbio/syy046
Simpson G. G. (1980). Splendid Isolation: The Curious History of South American Mammals. New Haven: Yale University Press.
Solórzano A., Núñez-Flores M. (2021). Evolutionary trends of body size and hypsodonty in notoungulates and their probable drivers. Palaeogeography Palaeoclimatology Palaeoecol. 568, 110306. doi: 10.1016/j.palaeo.2021.110306
Solórzano A., Núñez-Flores M., Rodríguez-Serrano E. (2024). The rise and fall of notoungulates: how Andean uplift, available land area, competition, and depredation driven its diversification dynamics. Gondwana Res. 135, 116–132. doi: 10.1016/j.gr.2024.08.002
Stehli F. G., Webb S. D. (Eds.) (1985). The Great American Biotic Interchange (Boston, MA: Springer US). doi: 10.1007/978-1-4684-9181-4
Strömberg C. A. E., Dunn R. E., Madden R. H., Kohn M. J., Carlini A. A. (2013). Decoupling the spread of grasslands from the evolution of grazer-type herbivores in South America. Nat. Commun. 4, 1478. doi: 10.1038/ncomms2508
Tarquini S. D., Ladevèze S., Prevosti F. J. (2022). The multicausal twilight of South American native mammalian predators (Metatheria, Sparassodonta). Sci Rep 12, 1224. doi: 10.1038/s41598-022-05266-z
Tejada J. V., Antoine P.-O., Münch P., Billet G., Hautier L., Delsuc F., et al. (2024). Bayesian total-evidence dating revisits sloth phylogeny and biogeography: a cautionary tale on morphological clock analyses. Systematic Biol. 73, 125–139. doi: 10.1093/sysbio/syad069
Toledo N., Bargo M. S., Vizcaíno S. F., Iuliis G. D., Pujos F. (2015). Evolution of body size in anteaters and sloths (Xenarthra, Pilosa): phylogeny, metabolism, diet and substrate preferences. Earth and Environmental Science Transactions of The Royal Society of Edinburgh 106, 289–301. doi: 10.1017/S1755691016000177
Vilhena D. A., Smith A. B. (2013). Spatial bias in the marine fossil record. PloS One 8, e74470. doi: 10.1371/journal.pone.0074470
Vizcaíno S. F., Fariña R. A., Zárate M. A., Bargo M. S., Schultz P. (2004). Palaeoecological implications of the mid-Pliocene faunal turnover in the Pampean Region (Argentina). Palaeogeography Palaeoclimatology Palaeoecol. 213, 101–113. doi: 10.1016/j.palaeo.2004.07.004
Voloch C. M., Vilela J. F., Loss-Oliveira L., Schrago C. G. (2013). Phylogeny and chronology of the major lineages of New World hystricognath rodents: insights on the biogeography of the Eocene/Oligocene arrival of mammals in South America. BMC Res. Notes 6, 160. doi: 10.1186/1756-0500-6-160
Vrba E. S. (1992). Mammals as a key to evolutionary theory. J. Mammalogy 73, 1–28. doi: 10.2307/1381862
Webb S. D. (1985). “Late Cenozoic mammal dispersals between the Americas,” in The great American biotic interchange. (Boston: Springer), 357–386.
Webb S. D. (1991). Ecogeography and the great American interchange. Paleobiology 17, 266–280. doi: 10.1017/S0094837300010605
Webb S. D. (2006). The Great American Biotic Interchange: patterns and processes. Ann. Missouri Botanical Garden 93, 245–257. doi: 10.3417/0026-6493(2006)93[245:TGABIP]2.0.CO;2
Webb S. D., Marshall L. G. (1982). “Historical biogeography of recent South American land mammals” in Mammalian Biology in South America. Mares M. A., Genoways H. H.. eds. Spec. Pub., Pymatuning Lab. Ecol., Univ. of Pittsburgh. 6, 39–52.
Welker F., Collins M. J., Thomas J. A., Wadsley M., Brace S., Cappellini E., et al. (2015). Ancient proteins resolve the evolutionary history of Darwin’s South American ungulates. Nature 522, 81–84. doi: 10.1038/nature14249
Westbury M., Baleka S., Barlow A., Hartmann S., Paijmans J. L. A., Kramarz A., et al. (2017). A mitogenomic timetree for Darwin’s enigmatic South American mammal Macrauchenia patachonica. Nat. Commun. 8, 15951. doi: 10.1038/ncomms15951
Woodburne M. O. (2010). The reat American Biotic Interchange: dispersals, tectonics, climate, sea level and holding pens. J. Mammal Evol. 17, 245–264. doi: 10.1007/s10914-010-9144-8
Woodburne M., Cione A., Tonni E. (2006). “Central American provincialism and the great America biotic interchange” in Advances in Late Tertiary Vertebrate Paleontology in Mexico and the Great American Biotic Interchange. Eds. Carranza-Castañeda O., Lindsay E. H. (Mexico City: Universidad Nacional Autónoma de México), 71–101.
Zachos J. C., Flower B. P., Paul H. (1997). Orbitally paced climate oscillations across the Oligocene/Miocene boundary. Nature 388, 567–570. doi: 10.1038/41528
Zenil-Ferguson R., Liow L. H. (2024). Simpson’s tachytely or bradytely? The importance of quantifying rate uncertainty. Methods Ecol. Evol. doi: 10.1111/2041-210X.14442
Keywords: diversification, macroevolution, mammalia, fossil record bias, speciation, extinction
Citation: Ugarte PDdS, Nascimento JCS and Pires MM (2025) Spatiotemporal variability in the South American mammalian fossil record and its impact on macroevolutionary inference. Front. Mamm. Sci. 3:1518039. doi: 10.3389/fmamm.2024.1518039
Received: 27 October 2024; Accepted: 10 December 2024;
Published: 07 January 2025.
Edited by:
S. Ivan Perez, National Scientific and Technical Research Council (CONICET), ArgentinaReviewed by:
Leandro Aristide, National Scientific and Technical Research Council (CONICET), ArgentinaJose Alexandre Felizola Diniz-Filho, UFG, Brazil
Copyright © 2025 Ugarte, Nascimento and Pires. This is an open-access article distributed under the terms of the Creative Commons Attribution License (CC BY). The use, distribution or reproduction in other forums is permitted, provided the original author(s) and the copyright owner(s) are credited and that the original publication in this journal is cited, in accordance with accepted academic practice. No use, distribution or reproduction is permitted which does not comply with these terms.
*Correspondence: Mathias M. Pires, cGlyZXNtbUB1bmljYW1wLmJy