- 1Veterans Affairs Northern California Health Care System, Martinez, CA, United States
- 2Department of Psychology, University of California, Berkeley, Berkeley, CA, United States
Introduction: The specific role that Broca's area plays in speech and language has been hotly debated in the literature. Some research has pointed to a specific role in particular aspects of speech production, while other findings have suggested additional roles in aspects of language comprehension. In the current study, we had the opportunity to take a broad approach by analyzing lesion and behavioral data from a large cohort of left hemisphere stroke patients. In this brief report, our objective was to identify which speech-language measures show a significant association with Broca's area, specifically pars opercularis and pars triangularis.
Methods: Lesion site and neuropsychological data from 173 chronic left hemisphere stroke patients were analyzed in the current study. Univariate lesion-symptom mapping (LSM) with rigorous correction was used to identify brain regions associated with individual test performance on a large battery of speech and language tasks. Multivariate LSM analyses were conducted in subsequent runs to confirm findings.
Results: The LSM results identified many predictable left hemisphere gray and white matter regions significantly associated with the speech-language data, but Broca's area was not implicated in performance on any speech or language measure. Regions adjacent to Broca's area, however, in left central opercular, precentral, and insular cortices were associated with speech production and motor speech performance.
Discussion: The current study failed to identify a single speech or language measure in our comprehensive test battery that was dependent on Broca's area. This finding could not be attributed to a lack of power, as Broca's area had among the highest power values and substantial lesion coverage. Interrogation of data at the individual patient level revealed the likely source of this null finding: Patients with lesions involving Broca's area varied widely in their speech-language performance, with profiles ranging from non-aphasic to Broca's to global aphasia. Given previous studies in acute stroke patients and healthy participants implicating Broca's area in speech-language, the current findings suggest that Broca's area plays a more supplementary than critical role and can be compensated by surrounding brain regions in chronic stroke.
Introduction
Much confusion and debate has swirled around the precise function of Broca's area in human speech and language. Broca's area is defined here as pars opercularis and pars triangularis in the left hemisphere, also known as Brodmann's cytoarchitectonic Areas (BA) 44 and 45, respectively, which make up the posterior portion of the left inferior frontal gyrus. In the latter half of the 19th century, Broca (1861) originally associated this region with speech articulation based on his patients with lesions in this region who had difficulty with speech production. Later interpretations began to discuss Broca's area as a syntactic or grammatical region, due in part to the “agrammatic” speech of patients with lesions in this region and patients' difficulty comprehending certain types of complex sentence constructions (Caramazza and Zurif, 1976; Santi and Grodzinsky, 2007). Other studies, however, challenged the notion that Broca's area was associated with grammaticality/syntax based on a number of findings: (1) Patients with lesions outside Broca's area also exhibit similar grammatical deficits (Caplan et al., 1996); (2) agrammatic patients like those with Broca's aphasia can accurately make grammaticality judgments, even if they do not always produce grammatical language (Linebarger et al., 1983); and (3) cross-linguistic studies show that patients with Broca's aphasia do not exhibit the same kind of agrammatic production if grammatical markers carry meaning (Bates et al., 1991).
One potential issue that has caused confusion in previous interpretations of the literature is that Broca's aphasia (the clinical syndrome characterized by reduced, agrammatic output and relatively intact comprehension) is sometimes used as a proxy for Broca's area without confirming lesion site. Indeed, Broca's aphasia stems from rather large left hemisphere lesions that extend beyond simply left BA 44 and 45, and sometimes Broca's area is not even lesioned in these patients (Mohr et al., 1978; Alexander et al., 1990; Dronkers and Baldo, 2011). Other confusion has arisen from studies that describe lesion findings and activations in healthy participants in the left inferior frontal gyrus (LIFG) in relation to speech-language performance, and then use the label “LIFG” synonymously with “Broca's area,” despite distinct (though overlapping) geographies.
Given all the complexity and mix of interpretations around Broca's area, we chose to let the data do the talking in the current study. To do this, we submitted all speech-language and imaging data from our relatively large chronic stroke patient group to a series of lesion-symptom mapping (LSM) analyses. Our objective was to identify which speech and language measures in our large test battery were significantly associated with Broca's area. Broca's area was defined as pars opercularis and pars triangularis, and both these regions were independently interrogated in the analyses, as previous studies have attributed distinct functions to these sub-regions (e.g., Goucha and Friederici, 2015).
Methods
Participants
Behavioral and brain imaging data from 173 chronic left hemisphere stroke patients (32 female) were retrospectively analyzed for the current study. Individuals were recruited primarily via clinician/colleague referrals at a VA Medical Center, as well as local community stroke groups and word-of-mouth. Inclusion criteria included: History of a single, chronic left hemisphere stroke (≥3months post-stroke at time of testing); concurrent brain imaging; native English speaker (prior to age 5); pre-morbidly right-handed (based on Edinburgh Handedness Inventory); no other neurologic history; no visual agnosia; no severe psychiatric history (e.g., bipolar disorder, schizophrenia); normal/corrected-to-normal vision and hearing; and completion of ≥75% of behavioral tests in the test battery. Stroke etiology (ischemic vs. hemorrhagic) was not available on all study participants due to the retrospective nature of the study, but etiology was predominantly ischemic (82%) in the 83 patients whose etiology was noted in the medical record.
Patients' mean age was 62 years (range 31–86, SD = 11), mean education was 14 years (range 5–22, SD = 3), and mean time post-stroke was 44 months (range 3–271, SD = 52). Ethnic background in the sample included White (155), Black (16), and Asian American (2). The sample was almost uniformly monolingual English speakers, with three individuals raised in a bilingual household (two Spanish and one Japanese).
Based on the Western Aphasia Battery (WAB and WAB-revised; Kertesz, 1982, 2007), the sample included 37 patients with Broca's aphasia, 15 patients with Wernicke's aphasia, 11 patients with conduction aphasia, 57 patients with anomic aphasia, six patients with global aphasia, two patients with transcortical sensory aphasia, one patient with transcortical motor aphasia, one with isolation aphasia, one unclassifiable, and 42 patients who scored within-normal limits (WNL). WNL status is based on the WAB manual cut-off score of 93.7/100; WNL includes both individuals with mild residual speech/language deficits and individuals who were never aphasic. All aphasia profile diagnoses were based on WAB testing in the chronic phase of stroke at time of testing and did not reflect earlier aphasia diagnoses that patients may have carried.
All patients provided informed consent. The study was approved by the VA Northern California Institutional Review Board and completed in accordance with the Helsinki Declaration.
Materials and procedures
Behavioral test battery
Licensed speech-language pathologists and trained neuropsychologists administered a large battery of speech and language tasks that required 2–3 study sessions to complete (see Table 2). The primary speech-language tasks were subtests of the WAB, which includes measures of speech fluency (rating from 0 to 10), speech production (sentence completion, responsive speech, category fluency), object naming, repetition (single words, phrases, and sentences), auditory comprehension (single word, yes/no questions, and complex sequential commands), reading, and writing. Participants were also administered a standard Motor Speech Exam (Wertz and Rosenbek, 1992) by a trained speech-language pathologist who rated severity of dysarthria and apraxia of speech. Additional speech/language measures included the Boston Naming Test (BNT15; Kaplan et al., 2001; Abeare et al., 2022), the Curtis-Yamada Comprehensive Language Evaluation [CYCLE, auditory comprehension of simple (e.g., simple declarative) and complex (e.g., object relative) sentences; Curtiss, 1988], the Redfern Repetition test (word, non-word, and sentence repetition; unpublished test), and letter (FAS) and category (animals) fluency (Benton et al., 1983).
Brain imaging and lesion reconstructions
Neuroimaging data were collected when lesion site and size were stabilized (at least 3 months post-stroke). Patients' lesions were reconstructed from 3D MRI T1 scans, or 3D CT scans when MRI was contraindicated. Imaging was acquired close to the time of the first test session. For 23 patients, anatomical scans were obtained on a 3T Siemens Verio scanner (Syngo MR B17) with a 12-channel phased-array head coil. The 3T T1 MPRage anatomical image was acquired for each subject (TR/TE/FA = 2,200 ms/1.62 ms/9°, FOV = 256 × 192 × 256 mm, 1 × 1 × 1 mm3 voxels, inversion = 900 ms, bandwidth = 343 Hz/voxel, and GRAPPA factor = 2), along with a T2 image (TR/TE/FA = 3,000 ms/409 ms/120°, FOV = 256 × 192 × 256 mm, 1 × 1 × 1 mm3 voxels, bandwidth = 751 Hz/voxel) and a FLAIR image (TR/TE/FA = 6,000 ms/388 ms/120°, FOV = 250 × 250 × 192 mm, 0.488 × 0.488 × 1 mm3 voxels, inversion = 2,100 ms, bandwidth = 781 Hz/voxel). For 62 patients, high-resolution T1-weighted 3D MRI scans were obtained on a 1.5 T Phillips Eclipse scanner. T1-weighted images were acquired with a Spoiled Gradient Recall (SPGR) coronal sequence (TR/TE/FA = 15 ms/4.47 ms/35°, FOV = 256 × 240 × 256, 0.94 × 1.3 × 0.94 mm3 voxels).
Patient lesions were outlined directly on the patient's T1 digital MRI image using MRIcron (Rorden and Brett, 2000) and then registered with the MNI template using the standard non-linear spatial normalization procedure from SPM (Statistical Parametric Mapping, Wellcome Trust Centre for Neuroimaging). A cost function masking procedure was used to avoid distortions due to the presence of the lesion (Brett et al., 2001). T2 and FLAIR images were yoked to the T1 images in MRIcron to verify the extent of the lesion.
When digital images were not available, lesions were drawn from hard-copy MRI (n = 81) or CT films (n = 7). MRI images were collected on the 1.5 T scanner described above, and the CT scanner was a Siemens Somatom Emotion 16 CT scanner with 3 × 3 × 3 mm3 imaging. A board-certified neurologist blind to patient clinical presentation and study hypotheses outlined lesions onto a standardized, 11-slice template [based on the atlas by DeArmond et al. (1989)]. Reliability with this technique has been previously demonstrated (Knight et al., 1988; Friedrich et al., 1998). SPM was then utilized to digitize and non-linearly transform all brain templates into MNI space (Collins et al., 1994). Transformations were completed using 50 control point pairs to match anatomical features on the two templates. Matlab imaging toolbox functions (cpselect, cp2tform, and imtransform) were then used to align slices using a local weighted mean transformation.
Across all reconstruction methods, the resulting lesion masks were compared back to the original raw data in native space to ensure as faithful as possible a representation of the structural lesion. Minor manual adjustments were made in the lesion mask if the automated warping techniques produced minor discrepancies (e.g., distortions due to oversized ventricles). This review process was conducted by three of the authors (BC, JB, ND) who have decades' experience in imaging/lesion reconstruction.
An overlay map of patients' lesions is shown in Figure 1. As shown, the extent of lesion coverage in the analyses included much of the left cerebral hemisphere and underlying white matter, predominantly in the middle cerebral artery distribution. The average lesion volume for the sample was 108 cc (range 0.04–421 cc; SD = 93). Most pertinent, Broca's area had significant lesion coverage (57% of patients had lesions involving pars opercularis and 52% had lesions involving pars triangularis) and high statistical power (see Table 1). Of those patients with lesions involving pars opercularis, the median volume of pars opercularis lesioned was 98%; of those patients with lesions involving pars triangularis, the median volume of pars triangularis lesioned was 93%.
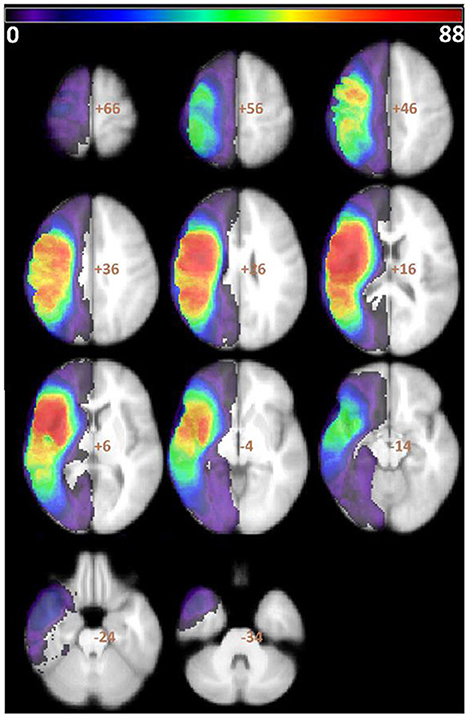
Figure 1. Overlay of all patients' lesions. Color bar indicates number of patients with lesions overlapping in each region. VLSM analyses were conducted in all voxels with at least 5% of the patient sample, which is represented here by all colors except purple.
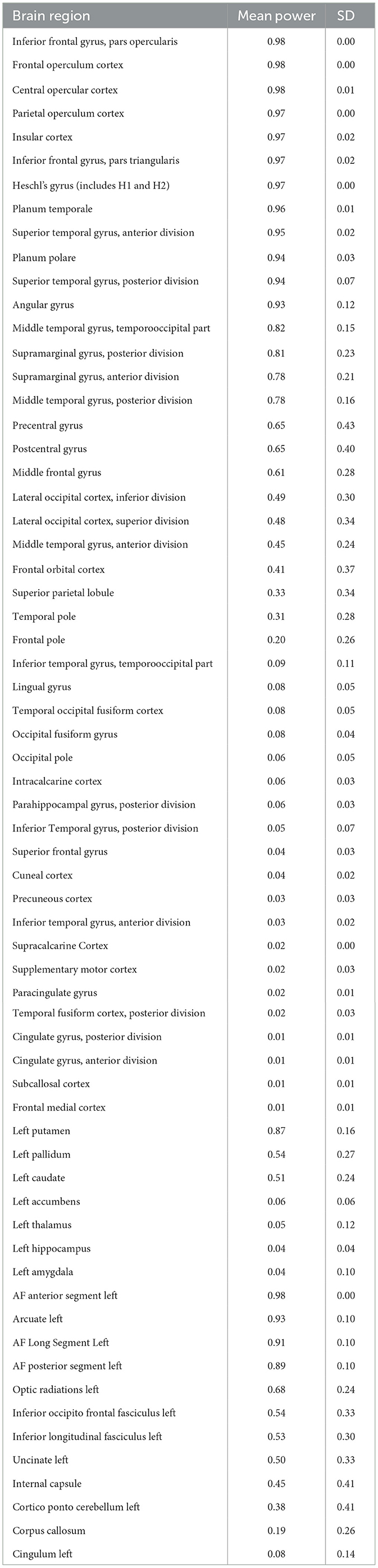
Table 1. Lesion mask-based voxelwise power for each left hemisphere brain region (voxelwise p < 0.05 as target; SD = voxelwise standard deviation).
Lesion-symptom mapping
We conducted a series of LSM analyses to relate lesion sites in the left hemisphere to each of the distinct speech-language performance scores. Here, we report findings from univariate LSM, which were largely in agreement with the multivariate results (see Supplementary material for multivariate results). This whole-brain univariate LSM approach was undertaken, rather than an ROI approach with Broca's area alone, so that we could evaluate the involvement of both Broca's area as well as surrounding regions. Univariate LSM analyses entail conducting a series of t-tests at every voxel to identify voxels in which patients with a lesion in that voxel perform worse on a task than patients without a lesion in that voxel (total of 52,490 voxels). Only voxels lesioned in a minimum of 5% of the sample were used in the LSM analyses to minimize spurious results based on lopsided t-tests with only a few patients in one group (Ivanova et al., 2021; Baldo et al., 2022). Lesion size was included as a covariate in all LSM analyses; age, gender, education and log(months post-stroke) were covaried during an earlier stage of data processing. Permutation testing was used to control for family-wise error, as traditional types of corrections like Bonferroni have been shown to be inappropriate for lesion imaging data (see Ivanova et al., 2021; Baldo et al., 2022). Permutation testing involves re-shuffling behavioral data and behavior-linked covariates across the lesion data (here, 3,000 permutations), in order to establish the distribution of the test statistic by calculating t-values at all voxels for each permutation.
Significance testing was based on the t-nu = 125 threshold, which reflects the 125th-largest voxelwise t-value, where 125 corresponds to 1 cm3 with 2 mm-sided voxels (see Mirman et al., 2018). This cutoff threshold was used to provide a greater opportunity to detect significant voxels [compared to traditional maximum t-threshold maps; see simulations in Ivanova et al. (2021)]. The Harvard-Oxford 50%+ atlas (Desikan et al., 2006) and Catani atlas (Catani and De Schotten, 2008) were used to identify gray and white matter regions corresponding to significant voxels identified in the LSM analyses.
Power calculations were conducted to ensure sufficient statistical power to detect differences in individual left hemisphere brain regions (see Table 1). Power was based on an unpaired Welch test using the Dixon-Massey formula between patient groups with and without a lesion in a particular voxel, in the context of an ideal behavioral function separating the two groups (i.e., mean difference of 1, standard deviation of 1). Importantly, Broca's area (pars opercularis and triangularis) had some of the highest statistical power at 0.97–0.98, thus ensuring that there was a theoretical behavioral function that could readily identify significant voxels in Broca's area.
Results
The LSM results for all speech-language measures are shown in Table 2, including both cortical and white matter regions in the left hemisphere. As can be seen, Broca's area (pars opercularis and triangularis) was not significantly implicated in any speech or language measure in our battery, even in tasks involving speech production. Central opercular, precentral, and insular cortices, which are adjacent to Broca's area, were significantly associated with speech fluency on the WAB and motor speech ratings. Since our lesion coverage and statistical power in Broca's area were very high, this null finding in Broca's area was not explained by a lack of power in this region.

Table 2. LSM results of significant left hemisphere gray and white matter regions associated with individual speech-language measures.
To further evaluate this null result in Broca's area, we also computed a more liberal cluster-size permutation-based LSM with a fixed t threshold set to p < 0.01, which provided much larger significant clusters and correspondingly smaller t-values (t > 2.33). We still did not find any voxels in Broca's area associated with the speech and language measures (see Supplementary material).
To understand this null finding in Broca's area, we reviewed individual level data and found that patients whose lesions involved Broca's area had highly disparate speech-language scores and aphasia profiles. Patients spanned a wide range of aphasia severity from “within normal limits” (mild or no aphasia) to anomic to conduction aphasia to Broca's and global aphasia. This wide range of speech-language profiles in patients with Broca's area lesions is consistent with the lack of association between Broca's area and speech-language performance.
Discussion
The current study retrospectively analyzed data from a large database of behavioral and imaging data in chronic stroke patients to investigate which speech-language measures are critically dependent on Broca's area (pars opercularis and triangularis). Data were subjected to a series of lesion-symptom mapping (LSM) analyses that identified left hemisphere gray and white matter correlates of speech-language performance. To our surprise, Broca's area was not identified as a significant correlate of any speech-language measure in the battery, including speech production/fluency. This finding could not be explained by lack of statistical power or lesion coverage in Broca's area, as both were very high. Also, robust associations were observed between speech-language measures and regions adjacent to Broca's area, such as left insular cortex. When we looked at individual level data, it was clear that the lack of association between Broca's area and specific speech-language measures was due to the fact that patients with lesions involving Broca's area were extremely heterogeneous with respect to their performance across measures. This heterogeneity was reflected in their aphasia subtypes which ranged from non-aphasic to Broca's aphasia to global aphasia.
Although surprising, the current findings are consistent with a number of previous studies that have shown that lesions in Broca's area alone do not lead to lasting speech-language deficits (Alexander, 2000). Rather, chronic Broca's aphasia has been shown to arise only from much larger left peri-Sylvian regions, as was the case for patients in this chronic stroke cohort (Dronkers et al., 2007; Dronkers and Baldo, 2011). Previous studies with acute/post-acute patients, however, have reported a more critical role for Broca's area in earlier stages post-stroke (e.g., Davis et al., 2008). This acute vs. chronic contrast, along with fMRI findings in healthy participants (e.g., Papoutsi et al., 2009), leads to interesting theories about the role that Broca's area plays in the healthy brain. The current findings suggest that, unlike some critical left posterior language zones that cannot be compensated for, Broca's area speech-language functions can be supported by adjacent left hemisphere speech-language regions in its absence in the chronic phase of stroke aphasia.
In the current study, we did find that left central opercular, precentral, and insular cortices adjacent to Broca's area were associated with speech fluency/production, and thus these regions may be more critical. This is consistent with findings such as those from direct cortical surface recording that find that Broca's area plays a supplementary role to motor cortex and is not directly critical for speech-language production (e.g., Flinker et al., 2015). We also observed an association between speech fluency/production and the AF long segment and anterior segment, which are closely connected with the pars opercularis and triangularis, respectively. Interestingly, the only other measure associated with the combination of these AF segments was another production task, writing. The AF long segment alone was also significantly associated with a number of language comprehension measures in the current study.
The current results were based on univariate LSM analyses, and given recent recommendations (e.g., Ivanova et al., 2021), we also ran all analyses using multivariate LSM as well (see Supplementary material). Consistent with the univariate results presented above, we did not observe any significant associations with Broca's area using a partial least squares (PLS) model. A multivariate singular value decomposition (SVD) plus Lasso regression model also showed no relationship between Broca's area and speech production/fluency or motor speech, although there was a modest association of Broca's area with single word comprehension and the FAS task. The observed association with FAS is consistent with our previous findings showing a double dissociation of letter vs. category fluency with left lateral prefrontal cortex and left mid-posterior temporal cortex, respectively (Baldo et al., 2006, 2010) and also consistent with other findings suggesting a role of the left inferior frontal gyrus in selection (Thompson-Schill et al., 1998; Novick et al., 2005; Thompson-Schill, 2005). It is also consistent with studies suggesting a role for Broca's area (and surrounding regions) in processing phonological information (Opitz et al., 2003).
Given that the current findings regarding Broca's area were essentially a null result, it is significant that we utilized a statistical threshold of nu = 125 in the LSM analyses recommended by Mirman et al. (2018), which has been shown to produce reliable findings with a good balance of Type 1 and 2 error in LSM simulations (Ivanova et al., 2021). Also critical when considering a null result, statistical power was robust enough to identify significant voxels in regions neighboring Broca's area, which provides further confidence in the reported null findings. Nonetheless, caution is always warranted in interpreting null findings. Thus, we ran an additional LSM analysis using a liberal cluster-based analysis with a very modest fixed t threshold, and Broca's area still did not emerge for any of the speech-language measures.
Although the battery of speech-language measures used in the current study was quite comprehensive, it was by no means exhaustive. More specific or experimental speech-language measures may be more sensitive to Broca's area dysfunction. However, given the very heterogeneous speech-language performance of patients in the current study who had Broca's area lesions, such a measure would likely be tapping into something much more subtle that does not track with speech-language presentation.
An important limitation in the current study is that speech-language functioning was assessed in chronic stroke patients, when neuroplastic compensatory changes have already taken place (Hartwigsen and Saur, 2019). Thus, the current findings can only speak to brain regions that are critical for speech-language functions that are not amenable to compensation. That is, it is still possible that Broca's area is normally recruited in the healthy brain for speech-language functions, but the current data show that it is apparently not critical for such functions in chronic aphasia outcome.
The spatial resolution and lesion reconstruction methods in the current study allowed us to evaluate speech-language correlates of pars opercularis and triangularis independently; however, some studies have begun to identify distinct subregions within these areas that are involved in different speech-language and cognitive abilities. For example, Clos et al. (2013) suggested that pars opercularis alone has subregions associated with speech/action production, working memory, semantics, and cognitive control. The current findings could not test such distinctions at this fine-grained level, as patients' lesions in the current study almost always involved the entire pars triangularis or opercularis.
It should also be noted that the relatively large patient sample in the current study required decades to acquire, and thus some of the lesion reconstructions were necessarily based on lower resolution CT and MRI films which did not allow us to measure and take into consideration white matter burden (see Bonkhoff et al., 2022 for impact of white matter lesions on outcome). We also combined lesion data from different stroke etiologies, and etiology was not always available in the medical record. However, regardless of original lesion data source or etiology, lesion reconstructions were compared to the original films for accuracy and minor manual adjustments were made if needed. Improvements in lesion reconstruction methodology are ongoing and will likely be conducted by fully automated algorithms in the near future and will be based on ever-increasingly higher spatial resolution scans that can more faithfully capture the extent and degree of lesion burden in both gray and white matter regions (Gryska et al., 2021).
Another limitation of the current study was that the sample was predominantly white, male (due to a large number of US Veteran participants), and highly educated; these factors limit generalizability to other patient samples.
Last, the current study focused on data from speech-language measures and thus cannot speak to the role of Broca's area in other cognitive processes. Some studies have suggested that Broca's area is not particular to speech-language functions at all, but rather plays a critical role in aspects of cognition more generally, which in turn can indirectly impact speech and language. Such domain-general theories have posited that Broca's area is critical for working memory (e.g., Chein et al., 2002), action processing (e.g., Papitto et al., 2020), attention/executive functioning (Novick et al., 2005), and hierarchical processing (e.g., Zaccarella and Friederici, 2017). Prior work from our group in this same stroke patient cohort has used LSM to identify neural correlates of several of these cognitive abilities, including working memory and executive functioning. In those studies, some involvement of Broca's area was noted for spatial working memory (backward span; Paulraj et al., 2018) and executive functioning (Wisconsin Card Sorting test; Baldo et al., 2005). Taken together, such cognitive findings support a role for Broca's area in strategic retrieval/manipulation (see Novick et al., 2005).
Conclusion
In this brief report, we had the opportunity to explore a large database of speech-language and neuroimaging data in chronic stroke patients to address the question: Which speech-language functions are critically associated with Broca's area? Surprisingly, Broca's area (pars opercularis and triangularis) did not emerge as a neural correlate of any of the speech-language measures in our battery, which encompassed a range of both speech production and comprehension tasks. Continuing work is needed to more conclusively discern the distinct role of Broca's area and its surrounding regions in both language and cognition.
Data availability statement
The datasets presented in this article are not readily available because of privacy limitations for VA patients. Requests to access the datasets should be directed to anVsaWFuYS5iYWxkbyYjeDAwMDQwO3ZhLmdvdg==.
Ethics statement
The studies involving humans were approved by VA Northern California Institutional Review Board. The studies were conducted in accordance with the local legislation and institutional requirements. The participants provided their written informed consent to participate in this study.
Author contributions
TH: Conceptualization, Data curation, Formal analysis, Investigation, Methodology, Software, Validation, Visualization, Writing – review & editing, Writing – original draft. KS: Funding acquisition, Investigation, Resources, Supervision, Writing – review & editing. BC: Data curation, Project administration, Validation, Writing – review & editing. SL: Investigation, Project administration, Validation, Writing – original draft, Writing – review & editing. MS: Data curation, Investigation, Project administration, Writing – review & editing. CL: Conceptualization, Data curation, Investigation, Project administration, Writing – review & editing. ND: Conceptualization, Data curation, Formal analysis, Funding acquisition, Investigation, Methodology, Resources, Supervision, Writing – review & editing. JB: Conceptualization, Data curation, Funding acquisition, Investigation, Methodology, Project administration, Resources, Supervision, Validation, Writing – original draft, Writing – review & editing.
Funding
The author(s) declare that financial support was received for the research, authorship, and/or publication of this article. This research was supported by the Department of Veterans Affairs Office of Research & Development CX001879 and NIH R21DC021042. The contents reported/presented within do not represent the views of the Department of Veterans Affairs or the United States Government.
Acknowledgments
We would like to thank the research participants who took part in this study.
Conflict of interest
The authors declare that the research was conducted in the absence of any commercial or financial relationships that could be construed as a potential conflict of interest.
Publisher's note
All claims expressed in this article are solely those of the authors and do not necessarily represent those of their affiliated organizations, or those of the publisher, the editors and the reviewers. Any product that may be evaluated in this article, or claim that may be made by its manufacturer, is not guaranteed or endorsed by the publisher.
Supplementary material
The Supplementary Material for this article can be found online at: https://www.frontiersin.org/articles/10.3389/flang.2024.1398616/full#supplementary-material
Supplementary Data Sheet 1. Findings with Multivariate LSM analysis using SVD.
Supplementary Data Sheet 2. Findings with Cluster size permutation-based LSM, p < 0.01.
Supplementary Data Sheet 3. Findings with Multivariate LSM using PLS.
References
Abeare, K., Cutler, L., An, K. Y., Razvi, P., Holcomb, M., and Erdodi, L. A. (2022). BNT−15: Revised performance validity cutoffs and proposed clinical classification ranges. Cogn. Behav. Neurol. 35, 155–168. doi: 10.1097/WNN.0000000000000304
Alexander, M. P. (2000). Aphasia I: Clinical and Anatomic Issues. Patient-Based Approaches to Cognitive Neuroscience. Cambridge: MIT Press, 165–181.
Alexander, M. P., Naeser, M. A., and Palumbo, C. (1990). Broca's area aphasias: aphasia after lesions including the frontal operculum. Neurology 40, 353–353. doi: 10.1212/WNL.40.2.353
Baldo, J. V., Dronkers, N. F., Wilkins, D., Ludy, C., Raskin, P., and Kim, J. (2005). Is problem solving dependent on language? Brain Lang. 92, 240–250. doi: 10.1016/j.bandl.2004.06.103
Baldo, J. V., Ivanova, M., Herron, T. J., Wilson, S. M., and Dronkers, N. F. (2022). “Voxel-based lesion symptom mapping,” in Lesion to Symptom Mapping: Principles and Tools, eds. D. Pustina, and D. Mirman (New York, NY: Springer).
Baldo, J. V., Schwartz, S., Wilkins, D., and Dronkers, N. F. (2006). Role of frontal versus temporal cortex in verbal fluency as revealed by voxel-based lesion symptom mapping. J. Int. Neuropsychol. Soc. 12, 896–900. doi: 10.1017/S1355617706061078
Baldo, J. V., Schwartz, S., Wilkins, D. P., and Dronkers, N. F. (2010). Double dissociation of letter and category fluency following left frontal and temporal lobe lesions. Aphasiology 24, 1593–1604. doi: 10.1080/02687038.2010.489260
Bates, E., Wulfeck, B., and MacWhinney, B. (1991). Cross-linguistic research in aphasia: an overview. Brain Lang. 41, 123–148. doi: 10.1016/0093-934X(91)90149-U
Benton, A. L., Hamsher, K., and Sivan, A. B. (1983). Controlled Oral Word Association Test (COWAT). Multilingual Aphasia Examination, 3rd Edn. Iowa City, IA: AJA Associates.
Bonkhoff, A. K., Hong, S., Bretzner, M., Schirmer, M. D., Regenhardt, R. W., Arsava, E. M., et al. (2022). Association of stroke lesion pattern and white matter hyperintensity burden with stroke severity and outcome. Neurology 99, e1364–e1379. doi: 10.1212/WNL.0000000000200926
Brett, M., Leff, A. P., Rorden, C., and Ashburner, J. (2001). Spatial normalization of brain images with focal lesions using cost function masking. Neuroimage 14, 486–500. doi: 10.1006/nimg.2001.0845
Broca, P. (1861). Remarks on the seat of the faculty of articulated language, following an observation of aphemia (loss of speech). Bull. Soc. Anatomique 6, 330–357.
Caplan, D., Hildebrandt, N., and Makris, N. (1996). Location of lesions in stroke patients with deficits in syntactic processing in sentence comprehension. Brain 119, 933–949. doi: 10.1093/brain/119.3.933
Caramazza, A., and Zurif, E. B. (1976). Dissociation of algorithmic and heuristic processes in language comprehension: evidence from aphasia. Brain Lang. 3, 572–582. doi: 10.1016/0093-934X(76)90048-1
Catani, M., and De Schotten, M. T. (2008). A diffusion tensor imaging tractography atlas for virtual in vivo dissections. Cortex 44, 1105–1132. doi: 10.1016/j.cortex.2008.05.004
Chein, J. M., Fissell, K., Jacobs, S., and Fiez, J. A. (2002). Functional heterogeneity within Broca's area during verbal working memory. Physiol. Behav. 77, 635–639. doi: 10.1016/S0031-9384(02)00899-5
Clos, M., Amunts, K., Laird, A. R., Fox, P. T., and Eickhoff, S. B. (2013). Tackling the multifunctional nature of Broca's region meta-analytically: co-activation-based parcellation of area 44. Neuroimage 83, 174–188. doi: 10.1016/j.neuroimage.2013.06.041
Collins, D. L., Neelin, P., Peter, T. M., and Evans, A. C. (1994). Automatic 3D intersubject registration of MR volumetric data in standardized Talairach space. J. Comput. Assist. Tomogr. 18, 192–205.
Curtiss, S. (1988). The Curtiss–Yamada comprehensive language evaluation (CYCLE). Unpublished test. Los Angeles, CA: University of California.
Davis, C., Kleinman, J. T., Newhart, M., Gingis, L., Pawlak, M., and Hillis, A. E. (2008). Speech and language functions that require a functioning Broca's area. Brain Lang. 105, 50–58. doi: 10.1016/j.bandl.2008.01.012
DeArmond, S. J., Fusco, M. M., and Dewey, M. M. (1989). Structure of the Human Brain: A Photographic Atlas. Oxford University Press.
Desikan, R. S., Ségonne, F., Fischl, B., Quinn, B. T., Dickerson, B. C., Blacker, D., et al. (2006). An automated labeling system for subdividing the human cerebral cortex on MRI scans into gyral based regions of interest. Neuroimage 31, 968–980. doi: 10.1016/j.neuroimage.2006.01.021
Dronkers, N. F., and Baldo, J. V. (2011). “Broca's area,” in The Cambridge Encyclopedia of the Language Sciences, ed. P. C. Hogan (Cambridge: Cambridge University Press), 139–142.
Dronkers, N. F., Plaisant, O., Iba-Zizen, M. T., and Cabanis, E. A. (2007). Paul Broca's historic cases: high resolution MR imaging of the brains of Leborgne and Lelong. Brain 130, 1432–1441. doi: 10.1093/brain/awm042
Flinker, A., Korzeniewska, A., Shestyuk, A. Y., Franaszczuk, P. J., Dronkers, N. F., Knight, R. T., et al. (2015). Redefining the role of Broca's area in speech. Proc. Nat. Acad. Sci. U. S. A> 112, 2871–2875. doi: 10.1073/pnas.1414491112
Friedrich, F. J., Egly, R., Rafal, R. D., and Beck, D. (1998). Spatial attention deficits in humans: a comparison of superior parietal and temporal-parietal junction lesions. Neuropsychology 12, 193–207. doi: 10.1037//0894-4105.12.2.193
Goucha, T., and Friederici, A. D. (2015). The language skeleton after dissecting meaning: a functional segregation within Broca's area. Neuroimage 114, 294–302. doi: 10.1016/j.neuroimage.2015.04.011
Gryska, E., Schneiderman, J., Björkman-Burtscher, I., and Heckemann, R. A. (2021). Automatic brain lesion segmentation on standard magnetic resonance images: a scoping review. BMJ Open 11:e042660. doi: 10.1136/bmjopen-2020-042660
Hartwigsen, G., and Saur, D. (2019). Neuroimaging of stroke recovery from aphasia–insights into plasticity of the human language network. Neuroimage 190, 14–31. doi: 10.1016/j.neuroimage.2017.11.056
Ivanova, M. V., Herron, T. J., Dronkers, N. F., and Baldo, J. V. (2021). An empirical comparison of univariate versus multivariate methods for the analysis of brain–behavior mapping. Hum. Brain Mapp. 42, 1070–1101. doi: 10.1002/hbm.25278
Kaplan, E., Goodglass, H., and Weintraub, S. (2001). Boston Naming Test. Austin: The Clinical Neuropsychologist.
Knight, R. T., Scabini, D., Woods, D. L., and Clayworth, C. (1988). The effects of lesions of superior temporal gyrus and inferior parietal lobe on temporal and vertex components of the human AEP. Electroencephalogr. Clin. Neurophysiol. 70, 499–509. doi: 10.1016/0013-4694(88)90148-4
Linebarger, M. C., Schwartz, M. F., and Saffran, E. M. (1983). Sensitivity to grammatical structure in so-called agrammatic aphasics. Cognition 13, 361–392. doi: 10.1016/0010-0277(83)90015-X
Mirman, D., Landrigan, J., Kokolis, S., Verillo, S., Ferrara, C., and Pustina, D. (2018). Corrections for multiple comparisons in voxel-based lesion-symptom mapping. Neuropsychologia 115, 112–123. doi: 10.1016/j.neuropsychologia.2017.08.025
Mohr, J. P., Pessin, M. S., Finkelstein, S., Funkenstein, H. H., Duncan, G. W., and Davis, K. R. (1978). Broca aphasia: pathologic and clinical. Neurology 28, 311–311. doi: 10.1212/WNL.28.4.311
Novick, J. M., Trueswell, J. C., and Thompson-Schill, S. L. (2005). Cognitive control and parsing: Reexamining the role of Broca's area in sentence comprehension. Cogn. Affect. Behav. Neurosci. 5, 263–281. doi: 10.3758/CABN.5.3.263
Opitz, B., Müller, K., and Friederici, A. D. (2003). Phonological processing during language production: fMRI evidence for a shared production-comprehension network. Cogn. Brain Res. 16, 285–296. doi: 10.1016/S0926-6410(02)00284-7
Papitto, G., Friederici, A. D., and Zaccarella, E. (2020). The topographical organization of motor processing: An ALE meta-analysis on six action domains and the relevance of Broca's region. Neuroimage 206:116321. doi: 10.1016/j.neuroimage.2019.116321
Papoutsi, M., de Zwart, J. A., Jansma, J. M., Pickering, M. J., Bednar, J. A., and Horwitz, B. (2009). From phonemes to articulatory codes: an fMRI study of the role of Broca's area in speech production. Cereb. Cortex 19, 2156–2165. doi: 10.1093/cercor/bhn239
Paulraj, S., Schendel, K., Curran, B., Dronkers, N., and Baldo, J. (2018). Role of the left hemisphere in visuospatial working memory. J. Neurolinguist. 48, 133–141. doi: 10.1016/j.jneuroling.2018.04.006
Rorden, C., and Brett, M. (2000). Stereotaxic display of brain lesions. Behav. Neurol. 12, 191–200. doi: 10.1155/2000/421719
Santi, A., and Grodzinsky, Y. (2007). Working memory and syntax interact in Broca's area. Neuroimage 37, 8–17. doi: 10.1016/j.neuroimage.2007.04.047
Thompson-Schill, S. L. (2005). “Dissecting the language organ: a new look at the role of Broca's area in language processing” in Twenty-First Century Psycholinguistics, ed. A. Cutler (Lawrence Erlbaum), 173–190.
Thompson-Schill, S. L., Swick, D., Farah, M. J., D'Esposito, M., Kan, I. P., and Knight, R. T. (1998). Verb generation in patients with focal frontal lesions: a neuropsychological test of neuroimaging findings. Proc. Nat. Acad. Sci. U. S. A. 95, 15855–15860. doi: 10.1073/pnas.95.26.15855
Wertz, R. T., and Rosenbek, J. C. (1992). Where the ear fits: a perceptual evaluation of motor speech disorders. Semin. Speech Lang. 13, 39–54. doi: 10.1055/s-2008-1064185
Keywords: speech, language, Broca's area, aphasia, Broca's aphasia
Citation: Herron TJ, Schendel K, Curran BC, Lwi SJ, Spinelli MG, Ludy C, Dronkers NF and Baldo JV (2024) Is Broca's area critical for speech and language? Evidence from lesion-symptom mapping in chronic aphasia. Front. Lang. Sci. 3:1398616. doi: 10.3389/flang.2024.1398616
Received: 10 March 2024; Accepted: 10 June 2024;
Published: 23 July 2024.
Edited by:
Stephanie Kathleen Ries, San Diego State University, United StatesReviewed by:
Anna Seydell-Greenwald, Georgetown University Medical Center, United StatesKyle Jasmin, University of London, United Kingdom
Copyright © 2024 Herron, Schendel, Curran, Lwi, Spinelli, Ludy, Dronkers and Baldo. This is an open-access article distributed under the terms of the Creative Commons Attribution License (CC BY). The use, distribution or reproduction in other forums is permitted, provided the original author(s) and the copyright owner(s) are credited and that the original publication in this journal is cited, in accordance with accepted academic practice. No use, distribution or reproduction is permitted which does not comply with these terms.
*Correspondence: Juliana V. Baldo, anVsaWFuYS5iYWxkbyYjeDAwMDQwO3ZhLmdvdg==