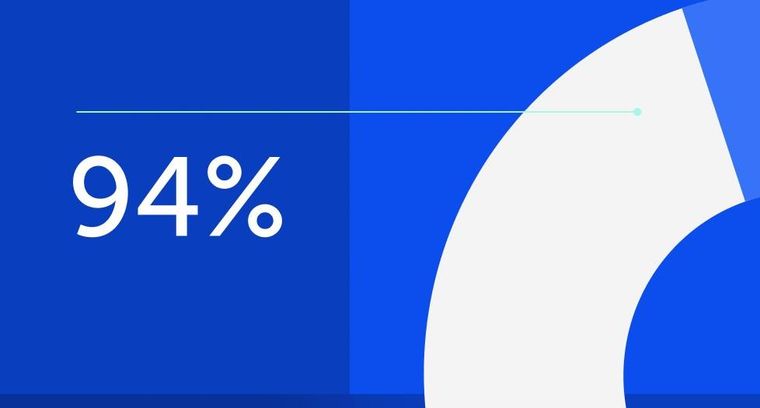
94% of researchers rate our articles as excellent or good
Learn more about the work of our research integrity team to safeguard the quality of each article we publish.
Find out more
ORIGINAL RESEARCH article
Front. Lang. Sci., 27 May 2024
Sec. Neurobiology of Language
Volume 3 - 2024 | https://doi.org/10.3389/flang.2024.1398125
This article is part of the Research TopicRevisiting a 150-year-old conundrum on the role of Broca’s area in language processing: Embracing expected and unexpected resultsView all 4 articles
Introduction: Cognitive control processes have been extensively studied in spoken word production, however, relevant investigations of written word production are scarce. Using data from a group of post-stroke individuals we studied, for the first time, the neural substrates of cognitive control in written word production. We addressed three questions: Are control mechanisms: (1) shared by language and non-language domains; (2) shared by lexical and segmental levels of word production within the word production system; (3) related to both interference and facilitation effect types?
Methods: To address these questions, for each participant we calculated cognitive control indices that reflected the interference and facilitation effects observed in written Blocked Cyclic Naming (written language production) and Simon (visuo-spatial processing) tasks. These behavioral cognitive control indices were studied both on their own, as well as in relation to the distribution of structural (gray matter) lesions.
Results: For Question 1, we provide strong evidence of domain-specific control mechanisms used in written word production, as, among other findings, distinct regions within Broca's Area were associated with control in written word production vs. control in visuo-spatial processing. For Question 2, our results provide no strong evidence of shared control mechanisms for lexical and segmental levels of written word production, while they highlight the role of BA45 in instantiating control mechanisms that are specific to the two levels. For Question 3, we found evidence that BA45 supports distinct mechanisms associated with facilitation and interference, while orbital frontal cortex supports control process(es) associated with both.
Discussion: These findings significantly advance our understanding of the cognitive control mechanisms involved in written language production, as well as of the role of Broca's Area in cognitive control and language production more generally.
The specific contribution of Broca's Area (BA44/45) to language processing is still a matter of on-going debate. Broca's original proposal was that this area supported speech production and that damage to the region was associated with deficits affecting articulatory planning (Broca, 1861; Mohr et al., 1978; Berker et al., 1986). Since then, Broca's Area has also been implicated in multiple core language functions, including syntactic processing (Grodzinsky, 2000; Kaan and Swaab, 2002; Ben-Shachar et al., 2003), phonological processing (Paulesu et al., 1993; Buckner et al., 1995; Zatorre et al., 1996), and semantic processing (Kapur et al., 1994; Martin et al., 1995). In other words, Broca's Area has been implicated both in lower-level language processes (e.g., speech articulation) as well as higher-level language ones (e.g., syntactic processing). While the region could support multiple language processes, it is also possible that it supports a function shared by different language processes, or that this region instantiates multiple functionally distinct components, some of which are language specific, and others than are shared across domains, including language (Fedorenko and Blank, 2020). One hypothesis is that cognitive control might constitute such a shared cognitive process/system (Goghari and MacDonald, 2009). In the research we report on here, we specifically investigated the neurocognitive control mechanisms involved in written word production, with a particular focus on evaluating the possible role of substrates within left frontal cortex, including BA44/45. The investigation of these issues in individuals with post-stroke language deficits provides the opportunity to not only evaluate relevant behavioral effects that can be amplified by brain damage, but to also examine the differential distributions of lesions across the relevant neural substrates.
Broadly defined, cognitive control refers to the ability to detect and resolve cognitive conflict and the ability to maintain active relevant representations and bias/facilitate the activation needed to achieve task goals. This is a function that is assumed to be necessary across multiple cognitive domains and modalities (Botvinick et al., 2001; Miller and Cohen, 2001). In the context of language, cognitive control is thought to be needed when multiple linguistic options are available and selection among them is necessary. For example, in sentence processing when multiple syntactic structures are available to express an idea to be communicated (e.g., Novick et al., 2005, 2014; Patra et al., 2023) or in word production when multiple word candidates are available to express semantic meanings (e.g., Damian et al., 2001; Crowther and Martin, 2014; Schnur, 2014; Nozari et al., 2016). The focus of this study is on written word production. In that context, it has been proposed that a number of mechanisms are involved in optimizing the selection of the intended word target and its constituent letters.
We will not adopt a specific proposal of cognitive control in word production; however, the following represent the types of mechanisms and processes that are often included in these discussions. It has been proposed that in the course of word production the activation levels of the relevant items (i.e., the level of conflict among activated items) is assessed relative to a difference criterion, according to which an item is selected only if its activation differs from that of the other activated items by a certain amount (Nozari and Hepner, 2019b). To achieve this, conflict-monitoring mechanisms operate over selection during word production (Botvinick et al., 2001; Nozari et al., 2011; Nozari and Hepner, 2019b). These monitoring mechanisms can, in turn, engage other mechanisms that may be external to the representational mapping system to help the process of meeting the difference criterion (Oppenheim et al., 2010; Nozari and Hepner, 2019b) and, hence, selection. These additional mechanisms include working memory to maintain active the specific task goals and other relevant task information (Belke, 2008; Crowther and Martin, 2014), top-down biasing mechanisms which, given the task goals and knowledge of the target items, operate so as to bias (i.e., send additional activation to) the anticipated target (Belke, 2008), and inhibitory control mechanisms that serve to suppress competing items by inhibiting or reducing their activity (McClelland and Rumelhart, 1981; Berg and Schade, 1992; Miyake et al., 2000; Nozari, 2019). The nature and timing of the interactions amongst these mechanisms to optimize production are complex and the subject of active ongoing research and debate (Costa, 2019; Nozari and Hepner, 2019a,b; Oppenheim and Balatsou, 2019; Roelofs, 2020; Oppenheim and Nozari, 2021; Oppenheim, 2024). It is beyond the scope of this paper to review this work, however, we will refer to the possible specific contributions of these mechanisms to written word production in the following sections.
It has been found that, for both language and non-language domains, prefrontal cortices may be broadly involved in the selection of the relevant item(s) among competing alternatives, which are themselves stored in other, usually posterior, areas of the brain (Cohen and Servan-Schreiber, 1992; Miller and Cohen, 2001). This is accomplished via white matter connectivity between anterior and posterior brain regions (e.g., Harvey and Schnur, 2015).
With regard to language processing specifically, there is a great deal of converging evidence supporting the hypothesis that Broca's Area, and other left Inferior Frontal Gyrus (LIFG) regions, such as BA47, are involved in cognitive control in the context of high-conflict situations during both word production and comprehension (Thompson-Schill et al., 1997, 1998; Devlin et al., 2003; Zhang et al., 2004; Badre et al., 2005; Moss et al., 2005; de Zubicaray et al., 2006; Schnur et al., 2009). For example, in a seminal study, Thompson-Schill et al. (1997) showed the involvement of LIFG in cognitive control during lexical semantic processing. In this study, a group of healthy adults performed three tasks during fMRI scanning, One task was a production task in which participants were asked to generate verbs in response to visually presented nouns that either induced low competition (e.g., KITE, which evokes the verb fly) or high competition (e.g., WHEEL, which evokes several different verbs, such as turn, spin or roll). The other two tasks were comprehension tasks. All three semantic retrieval tasks showed effects of cognitive control demands in overlapping regions in the LIFG, specifically in BA44, with higher brain activity for the high cognitive control demand conditions compared to the low cognitive control demand conditions.
The LIFG has also been associated specifically with control in response selection in a number of domains. For example, there is evidence that the LIFG supports the selection of information from the phonological system (e.g., Marian et al., 2014; Attout et al., 2022), as well as the selection of motor responses for spoken production (Tremblay and Small, 2011). In terms of non-linguistic processes, the LIFG has been linked to cognitive control in a large range of functions such as in retrieval from long-term (Sohn et al., 2003), and short-term memory (Zhang et al., 2004), and in non-verbal, body movement (Di Russo et al., 2006), etc. However, the extent to which these frontal regions instantiate the same or different mechanisms across language and non-language domains is still a matter of intense debate (Fedorenko et al., 2013; Kan et al., 2013; Caplan, 2014; Fedorenko, 2014). Different sources of evidence, including neurostimulation (specifically, Transcranial Magnetic Stimulation; TMS) studies in healthy individuals support the hypothesis of at least some domain-specificity of cognitive control mechanisms. For example, it has been found that when participants had to perform lexical and perceptual selection tasks, stimulation over the anterior LIFG increased reaction times for the lexical, but not the perceptual non-lexical tasks (Devlin et al., 2003; Whitney et al., 2011). On the other hand, some fMRI activation studies have shown overlap in areas involved in control during response selection for both syntactic comprehension and perceptual processing tasks (January et al., 2009; Ye and Zhou, 2009), providing support for domain-general cognitive control.
There is also a question regarding the degree of specificity of control processes within the language domain. For example, in word production, which is the focus of the current investigation, there are selection demands at the different levels of processing, which may or may not have their own selection mechanisms. Most models of single word production (across both the spoken and the written modalities) assume semantic, lexical and segmental levels of representation and processing (Figure 1) (e.g., Dell, 1986; Roelofs, 1992; Levelt et al., 1999; Rapp and Goldrick, 2000). During word production, there is a “meaning-driven” processing stage (Figure 1—Stage 1), during which the activation of a set of relevant semantic features or nodes serves as the basis for activation at the lexical level, including activation of the target lexical item. The second stage is “form-based” (Figure 1—Stage 2), during which activation from the lexical level serves as the basis for activation at the segmental level. The segments correspond to the word's constituent graphemes and phonemes, for written and spoken word production, respectively.
Figure 1. A representation of the “two-stage” model of single word naming, with three levels of representation: Semantic, Lexical and Segmental. Stage 1 involves the mapping from the Semantic to the Lexical level (i.e., meaning-driven stage), while Stage 2 represents the mapping from the Lexical to the Segmental level (i.e., form-based stage). For purposes of illustration, the units at the Segmental level are orthographic units (i.e., letters) but they would be phonological units (i.e., phonemes) in spoken production. Also, for simplicity of illustration, not all relevant segments of the four lexical items are represented. Note also that there is debate regarding whether or not the lexical level corresponds to amodal representations (lemmas; Levelt et al., 1999) and/or modality-specific phonological and orthographic lexemes (Caramazza, 1997).
The handful of studies that have specifically investigated the cognitive control processes involved in selection at lexical and segmental levels of processing have examined spoken word production. These studies have reported evidence that more anterior parts of the LIFG support selection at the lexical level, and more posterior parts support selection at the segmental (i.e., phonological) level (Schnur et al., 2009; Klaus and Hartwigsen, 2019). In other words, the evidence to date suggests that cognitive control mechanisms within the word production system may be level-specific, at least in the spoken modality. No studies have investigated how the LIFG supports cognitive control in written word production.
As mentioned, the need for cognitive control depends on the degree of conflict within a system. Behaviorally, high conflict levels result in slower response times and higher errors rates, referred to as interference effects. Conflict in word production has been extensively studied using the Blocked Cyclic Naming (BCN) paradigm. In the BCN paradigm, sets of pictures are named multiple times (e.g., a set of five pictures is presented once and then it is repeated with the items presented in different orders for some number of cycles—with each repetition of the set corresponding to a cycle). The critical manipulation is the “naming context” in which these sets of pictures are presented, where context refers to the number of features that are shared by the items in a set. In homogeneous or related contexts, one or more features is shared across items, whereas in heterogenous or unrelated contexts, items do not share features. This paradigm has been used to study control mechanisms at the lexical level via the naming of sets of items that share a semantic category (e.g., Brown, 1981; Belke et al., 2005; Schnur et al., 2009) (e.g., naming horse, in the context of zebra, lion, giraffe) compared to naming items in the context of unrelated items (naming horse in the context of bed, car, glass). Control mechanisms have also been studied at the segmental level via the naming of items that share phonological or orthographic segments (e.g., pig in the context of fig, pin and tin, compared to pig in the context of glass, bed, mat) (e.g., Meyer, 1991; Damian, 2003; Schnur et al., 2009; Breining et al., 2016; Wang et al., 2018; Breining and Rapp, 2019).
The basic finding from BCN is that items that are repeatedly named in related contexts are named more slowly and/or with more errors, compared to items named in unrelated contexts. This effect is attributed to the exacerbation of the competition/inhibition between items with shared features that is caused by their repetition. According to spreading activation principles, activation spreads more between related than unrelated items. Therefore, when naming an item in a related context, activation spreads to related items (including the other items in the related set) at every naming trial. With multiple repetitions of related items, the activation levels of all related items increase, creating greater selection difficulty. Some models of word production, propose that, under these conditions, control mechanisms detect conflict and work to facilitate selection. For example, one proposal is that a booster mechanism is triggered to help distinguish among the active response options and eventually select the target item for production (Dell et al., 1997). In terms of neural substrates, this selection mechanism has been proposed to be instantiated in the LIFG (Oppenheim et al., 2010). Thus, BCN can be used to manipulate and examine various aspects of the naming context in order to better understand the control mechanisms that are deployed.
It is worth noting, however, that interference is not the only type of effect observed with the BCN paradigm. Indeed, in addition to the interference effects that have formed the focus of most investigations, facilitation effects are also commonly reported in the BCN paradigm specifically from the first to the second presentation (i.e., cycle) of items (Belke et al., 2005; Damian and Als, 2005; Schnur et al., 2006; Navarrete et al., 2012). The basis for these facilitation effects is not well understood.
A pervasive finding across a wide range of paradigms is that naming an item after processing a related (or identical) item leads to faster responses for the later item (Sperber et al., 1979; Huttenlocher and Kubicek, 1983; Hartsuiker et al., 2005). One explanation of this facilitation effect is that it is the result of what is referred to as “priming” of the later item (the target) by the earlier one (the prime). As a consequence of the spreading activation principles discussed above, the more the prime and the target share features, the greater the pre-activation of the target by the prime and, therefore, the faster the naming of the target. Given that the BCN paradigm involves the repetition of items that are highly related, we might expect that, in addition to the interference effects described above, there should also be facilitatory priming effects. Additionally, Belke (2008) proposed an account of facilitation effects in BCN that does not rely on spreading activation. According to Belke (2008), the facilitation is the result of a top-down modulation mechanism which, given the task goals and the knowledge of the items involved, operates so as to bias (i.e., send additional activation) to the anticipated target. However, after the second cycle, when activation has significantly increased for all related items, this top-down mechanism may not be as effective, and a net effect of interference is observed. While facilitation effects are widely observed in studies that use BCN, very little work has been done to understand the mechanisms that give rise to the seemingly contradictory effects of facilitation and interference during word naming.
Key questions include whether or not the same or different processing mechanisms give rise to the facilitation and interference effects that are observed and if they arise from shared or distinct neural substrates. While there is evidence that the LIFG plays an important role in this top-down “boosting” mechanism (Belke and Stielow, 2013; Belke, 2017; Nozari and Pinet, 2020), very few studies have directly examined this issue in the context of the BCN paradigm (e.g., de Zubicaray et al., 2006; Riès et al., 2015).
Despite the long line of research on word production and Broca's Area, as the brief review above reveals, key issues remain unresolved. Furthermore, almost all studies investigating cognitive control in word production and its relationship to Broca's Area have focused on spoken production, with sparse research directed at understanding the control mechanisms that support selection in written production.
The current study focuses on written word production and addresses important gaps in our understanding of cognitive control and how it relates to Broca's Area by investigating three questions: (1) Are control mechanisms shared by language and non-language domains or are they domain-specific? (2) Are control mechanisms within the word production system shared by lexical and segmental levels of word production, or are they level-specific? and, (3) Do interference and facilitation effect types arise from the same control mechanisms, or are these mechanisms effect-type specific? In this study of individuals with post-stroke aphasia we will bring to bear both behavioral and neuroimaging data on these three questions. The behavioral language data consist of interference and facilitation effects observed in written BCN. For question (1), given that writing is a highly visuo-spatial task, we also examine data from a non-verbal visuo-spatial processing task. The neuroimaging data consist of measures of gray matter lesion location and extent.
The empirical logic is similar for all three questions, since all three questions are associated with the same two classes of hypotheses about cognitive control mechanisms: shared mechanisms hypotheses, and independent mechanisms hypotheses. In terms of behavioral evidence, shared mechanisms hypotheses predict correlated behavioral effects, while independent mechanisms hypotheses predict an absence of correlation. With regard to domain specificity (Question 1), the prediction of the shared mechanisms hypothesis is that any interference effects that are observed should be correlated across language and visuo-spatial tasks. With regard to level specificity (Question 2), the shared mechanisms hypothesis predicts that lexical and segmental facilitation and/or interference effects observed in BCN should be correlated. And, finally, with regard to effect-type specificity (Question 3), the shared mechanisms hypothesis predicts that facilitation and interferences effects should be correlated at each level. The independent mechanisms hypotheses predict an absence of the respective correlations for each of the three questions. To evaluate these predictions, for each participant, we computed the magnitude of interference and/or facilitation effects for language and non-language tasks. Within the language domain, we examined effects arising at different levels of written word processing by manipulating lexical and segmental similarity in the BCN paradigm.
In terms of the neural data, the basic prediction of the shared mechanisms hypotheses is that the relevant behavioral effects should be associated with the same neural substrates, while the independent mechanisms hypotheses predict that the relevant behavioral effects should be associated with distinct neural substrates. With regard to domain specificity (Question 1), the shared mechanisms hypothesis predicts an association between neural substrates and the behavioral effects indexing the different processing domains (i.e., interference effects in language and spatial tasks). With regard to level specificity (Question 2), the shared mechanisms hypothesis predicts that there will be an association between neural substrates and the behavioral effects indexing different levels of word production (i.e., interference and/or facilitation effects at lexical and segmental levels). Finally, with regard to effect-type specificity (Question 3), the shared mechanisms hypothesis predicts an association between neural substrates and the behavioral effects for facilitation and interference effect types overall (across lexical and segmental levels). In contrast, independent mechanisms hypotheses predict dissociations between the relevant behavioral effects and lesioned substrates for each question of interest. To evaluate these predictions, we examined 73 parcels within the left hemisphere that corresponded to those regions for which at least four individuals in our participant group had substantial damage. We carried out regression analyses to evaluate the strength of the association between extent of damage and magnitude of the relevant behavioral effects.
Briefly, on the basis of the analysis of the behavioral effects (Analysis 1) and of the relationships between the distribution of neural damage and interference and facilitation effects across language and non-language domains (Analyses 2 and 3), we found evidence of the role of left frontal regions, including Broca's Area, in instantiating domain-specific control mechanisms involved in written word production but not visuo-spatial processing. These domain-specific mechanisms recruited distinct and nonoverlapping regions of frontal cortex. With regard to written word production, the rostral part of BA45 in Broca's Area was found to support both level-specific and effect-type specific mechanisms, while the Orbital gyrus was found to support both interference and facilitation effects in written word production.
We considered participants from previous aphasia/dysgraphia studies in our lab and those currently enrolled in a local aphasia center for participation in this study. To determine the eligibility of those who expressed interest, we administered a screening test and/or we consulted their previous testing results. Exclusion criteria were: (a) Below normal semantic processing, to rule out the possibility of naming difficulties stemming from impairments in the semantic system; (b) Difficulty with peripheral motor processes in writing and speaking; (c) Severe comprehension deficits that may affect the ability to understand task instructions. The final set of participants consisted of 19 individuals (10 female) at the chronic (minimum 3 years) post-stroke stage. Mean age was 63.3 years (SD = 13.3). Appendix A reports demographic data for the stroke participants. Prior to their stroke, 15 individuals were right-handed and four were left-handed. For writing, n = 12 used their premorbidly preferred hand, the others used the opposite hand. Their performance on a range of tasks assessing their language and cognitive skills is reported in Appendix A. Overall, the testing indicates good performance on the picture-based comprehension test (Pyramids and Palm Trees; Howard and Patterson, 1992) and difficulty in one or more of the language tasks.
In order to assess whether the experimental tasks/paradigms that we used generated the expected behavioral effects of interest, we also included a small group of neurologically healthy control individuals. The healthy control group consisted of nine individuals (six female), age-matched to the stroke cohort (mean age = 61.1, SD = 7.3), with no history of psychiatric or neurological disorders. All healthy control individuals were right-handed. All participants provided consent for research using procedures consistent with the Declaration of Helsinki and approved by the Johns Hopkins University Institutional Review Board.
Cognitive control demands in written word production were assessed using a version of the BCN paradigm in which, as described earlier, small sets of pictures are named repeatedly. The parameter choices of the experimental paradigm (i.e., number of items per set, cycles per block and sets per condition, as well as stimuli presentation parameters) were based on the work of Nozari et al. (2016). The BCN paradigm usually involves multiple sets of 5 or 6 items, repeated in cycles. However, similar to Nozari et al. (2016), the current study used pairs of items, rather than sets, to avoid effects of working memory demands on performance. The effectiveness of this paradigm was evaluated by Nozari et al. (2016) who replicated basic BCN effects reported in previous studies.
Given that the goal of the task was to specifically investigate the cognitive control mechanisms involved in written picture naming, we wanted to minimize any effects related to deficits arising from lexical or segmental representation/mapping processes themselves. Therefore, for each stroke participant we selected 24 items that they could accurately name and spell. To identify these items, each participant performed a pretest. The items used in the pretest consisted of a set of 18 lexically (semantically) related pairs and a set of 18 segmentally related pairs. Lexically related items were related in meaning (e.g., dog/cat)1 while segmentally related items shared orthographic and phonological rhymes (e.g., bear/pear). During testing, items were pseudo-randomly shuffled so that no two consecutive items were segmentally or lexically related.2 Participants were first familiarized with the names of the items so that they would name the pictures using the “intended” names. During the familiarization phase, participants saw each line drawing along with its label, the experimenter read the name and the participant repeated the name. After familiarizing the participant with all line drawings and their names, the same line drawings were presented to the participant for both written and oral naming. This process was repeated twice to ensure that the 24 items (six pairs of lexically and six pairs of segmentally related items) we selected for each participant were items that the participant consistently named and spelled correctly (see Appendix B, for the stimuli administered to one of the participants). The healthy individuals were tested on the same lists of items used with the stroke participants. To ensure that they could correctly spell all the items in the list they were assigned to, they were asked to spell to dictation the relevant items prior to the experimental session.
The task included three experimental conditions: lexically related, segmentally related and unrelated. The lexical and segmental relatedness conditions each included six pairs of words for a total of 12 pairs of words. Unrelated pairs were constructed by pseudo-randomly shuffling the words in the related pairs such that the two words in the new, unrelated pair had minimal semantic similarity and no segmental overlap. Thus, overall, each participant was tested on a total of 24 pairs of words (12 related and 12 unrelated).
The same black and white line-drawings used for the pretest were used as experimental stimuli. In total, each participant was presented with 24 different pictures, corresponding to 24 words they could consistently name and spell correctly based on the pre-testing. Pictures were presented on a computer monitor in different blocks. An example of a block is depicted in Figure 2. Each block only included one pair of items (similar to Nozari et al., 2016), and each pair of items was presented five times within the block for a total of 10 trials per block (i.e., five presentations of each item in a pair). The order of trial presentation within a block was pseudorandomized so that the same picture never appeared more than twice consecutively.3 Since each pair of items was presented in a different block, there were six blocks of lexically related items, six blocks of segmentally related items and 12 blocks of unrelated items. The order of the blocks was pseudo-randomized such that the same type of condition was never immediately repeated. In total, there were 240 trials [10 trials per block × (six segmental relatedness blocks + six lexical relatedness blocks + 12 unrelated blocks)].
Figure 2. Example of a lexically related block in the current study. The two items presented in this block are DOG and CAT. Each item was presented five times (i.e., five cycles), for a total of 10 trials.
Participants wrote their responses on a Wacom Intuos4 Medium graphic tablet (model: PTK-440) using an inking tablet pen. Each response was written on a separate piece of paper, measuring 1.5 × 2 inches, that was attached on the writing surface of the tablet before the beginning of each trial. By using an inking tablet pen we recorded both their written responses (on paper and digitally) as well as information about the location and the pressure of the pen on the writing surface, separately for each trial. Information about the location and the pressure of the pen on the writing surface was recorded using in-house developed scripts in Matlab (version: 9.11.0; MATLAB, 2021). This information was then used to calculate reaction times (RTs). RTs were measured from stimulus presentation on the computer monitor (picture of the item to be named) to the time the writing surface was touched to begin the written response. Before the beginning of the experiment, participants were familiarized with the task with a practice block (i.e., 10 trials) with two unrelated items that were not included in the experimental set of 24 items. At the end of the practice block each participant was asked if they had any questions before starting the main experiment.
During the experiment, before each block, participants were re-familiarized with the items that would be presented in the upcoming block, to ensure that the intended label would be used, similar to Nozari et al. (2016). At the beginning of each trial, participants were asked to place the pen on a marked starting point on a non-responsive edge of the writing tablet approximately 1 inch to the right or the left of the writing surface, depending on the hand they were using to write with. Having participants always start writing from the same point increased accuracy of the reaction time measurements across trials. Each trial began with a “beep” at the same time that a fixation cross was presented at the center of the screen for 3 s, followed by the presentation of the stimulus picture. Stimulus presentation times varied across participants and were decided based on their writing speed as assessed in a short pre-test during the familiarization phase. For healthy controls it was 9 s for all participants; for the stroke participants it ranged from 9 to 20 s. On every trial, after writing their response, participants were asked to return the hand holding the pen to the “starting” point to be ready for the next trial.
To examine whether or not cognitive control mechanisms are domain-specific, we also administered a visuo-spatial task with significant cognitive control demands—the Simon task (Simon and Rudell, 1967). In the Simon paradigm, a visual stimulus (e.g., a blue or red square) is presented on the right or left side of a computer monitor and participants are instructed to respond with a key-press with their left hand to one stimulus and with their right hand to the other. Participants are asked to attend to a specific stimulus feature (e.g., color), independently of stimulus location (left or right of screen). For example, they may be asked to respond with the right hand to a blue stimulus and with the left to a red one, regardless of location. Cognitive control demands can be considered analogous to those in word naming in that, in both cases, multiple responses are available and potentially competing (left and right button presses in the Simon task vs. multiple words and segments in word naming) while the correct one must be selected for production. In the Simon task, the response conflict arises because the spatial representation of the stimulus (left or right of screen) is in conflict with the spatial representation of the response rule (left or right key).
The Simon task has been reliably shown to produce strong visual-motor compatibility/interference effects, such that individuals are faster and more accurate on congruent trials (i.e., trials in which the stimulus is presented on the same side of the screen as the response key for the trial) vs. incongruent trials (i.e., trials in which the stimulus is presented on the opposite side of the screen relative to the response key for the trial; Figure 3). Only a handful of studies with post-stroke individuals have investigated performance on the Simon task, but all of them have shown the expected pattern of interference (i.e., longer RTs and/or more errors in incongruent trials vs. congruent trials) (Martin et al., 2012; Nozari, 2019; Obermeyer et al., 2020).
Figure 3. Representation of Congruent and Incongruent trials in the Simon task. If the task instructions are to press the left button when a blue square appears and the right button when a red square appears, then congruent trials are those in which a blue square appears on the left and a red square appears on the right. Incongruent trials are those in which a red square appears on the left and a blue square appears on the right.
The experiment began with 12 practice trials, at the end of which each participant was asked if they had any questions before starting the main experiment. In total, there were 120 trials: 60 congruent trials and 60 incongruent trials. Within each congruency condition, half of the trials included a blue square and the other half a red square. Trials were presented in a pseudo-randomized order, such that no more than two consecutive trials were identical (i.e., with respect to congruency and color identity).
The experiment was presented on a laptop Dell Latitude 5520 computer with a 15.6-in. monitor. The sequence of events and data collection were controlled using the OpenSesame program (version 3.3.12; Mathôt et al., 2012). Each trial began with a fixation cross (+) in the center of the screen, that remained visible for 800 ms and was followed by a 250-ms blank interval. At the end of this interval, a red or blue square appeared on the left or the right side of the screen and remained on the screen for 1,000 ms if there was no response. Participants were instructed to press the Z key when they saw a blue square and the M key when they saw a red square. The relevant keys were marked with stickers in the respective colors. The timing began with the onset of the stimulus, and a response terminated the stimulus presentation; there was then a 500-ms blank interval before the onset of the next trial. RTs (stimulus onset to response) were collected and responses were marked as correct or incorrect to assess performance accuracy.
Magnetization-prepared rapid acquisition with gradient echo (MPRAGE) structural scans and DTI scans were acquired on a 3T Philips Achieva MRI scanner with a 32-channel head coil at the FM Kirby Research Center for Functional Brain Imaging at the Johns Hopkins Kennedy Krieger Institute. For MPRAGE, we used a 3D inversion recovery sequence with the following parameters: TR/TE/TI = 8.1/3.7/842 ms, resolution = 1 × 1 × 1 mm3, FOV = 224 × 224 mm, 165 axial slices, flip angle = 8°, acquisition time = 4 min and 31 s.
For the stroke participants, MPRAGE scans were used to identify each individual's lesion. For each individual, lesion volumes were extracted in MRIcron by identifying areas with signal abnormalities, and then warped to MNI space with Statistical Parametric Mapping software (Friston et al., 2012). Figure 4 presents a lesion overlay map, depicting all participants' lesions in MNI space. As evident from the figure, the stroke participants demonstrated a range of lesions over the left hemisphere, with the greatest area of lesion overlap in the left inferior and middle frontal gyrus, the insula, the lateral precentral and postcentral gyri, the posterior portion of the superior temporal gyrus and the supramarginal gyrus.
Figure 4. (Panel A) A lesion overlay map, showing all stroke participants' lesions in MNI space. (Panel B) The same lesion overlay map showing the overlap with Broca's Area (BA44/45) in light blue. Colors of the lesion overlay map indicate the number of participants with a lesion in a given voxel.
Structural T1-weighted scans were used to assess the integrity of the relevant gray matter regions. For this study, we used the parcellation from the Human Brainnetome atlas (Fan et al., 2016), which divides the brain into 210 cortical regions. For each participant, the parcellation was transformed in their native space, to avoid gross distortions that are produced when normalizing lesioned brains into standard space (Gleichgerrcht et al., 2017). To achieve this, the enantiomorphic inverse-normalization process was followed, which allows for the least warping of the focally lesioned brain (Nachev et al., 2008), and hence, for minimum distortion. First, a lesion mask was manually drawn on the participant's T1-weighted image in MRIcron. Then, using the Clinical Toolbox (Rorden et al., 2012) in SPM12 (Friston et al., 2012), the lesion mask was used to substitute the lesion with healthy tissue from the contra-lesional hemisphere (Nachev et al., 2008). Finally, using the transformation parameters from the previous step, the mask with the 210 ROIs was inverse-normalized to each participant's T1-weighted scan.
To assess the integrity of the relevant gray matter regions,4 we used an in-house script using Python (Van Rossum and Drake, 2003) [utilizing functions from packages: “numpy” (Harris et al., 2020), “nilearn” (Abraham et al., 2014), and “matplotlib” (Hunter, 2007)], to calculate for each participant the percentage of each ROI that overlapped with the lesion, henceforth referred to as Lesion Percentage. Parcels for which fewer than four individuals had substantial damage (where substantial damage was defined as more than 10 lesioned voxels), were removed from the analysis. In total, 73 parcels in the left hemisphere met the selection criteria: 27 in the frontal lobe, 25 in the temporal lobe, 17 in the parietal lobe and 4 in the occipital lobe. These 73 parcels were used for the statistical analyses discussed below (see Appendix C for a list of the 73 parcels and their anatomical/cyto-architectonic descriptions).
Our statistical analysis can be divided into two sections. Section A consists of the analysis of the behavioral data from both the stroke and healthy control individuals to determine if the main behavioral effects of the stroke group were in the expected direction as those of the healthy control individuals. This analysis was further used to calculate, for each participant, the cognitive control indices of lexical and segmental interference and facilitation for the main set of analyses. Section B consists of the main set of three analyses that directly address the three questions of interest.
In order to determine if the main behavioral effects were in the expected direction as the healthy control individuals, we first ran an analysis with both stroke participants and healthy controls, separately for each task. All analyses were performed in R (R Core Team, 2013), using the “stats” package for the linear regression models and the “lme4” package for the linear mixed-effects models (LMEM) (v1.1-31; Bates et al., 2015). The variance inflation factor (VIF) was calculated for all models using the package “car” (v3.1-1; Fox and Monette, 1992; Fox, 2015).
As discussed, 24 unique items were included, and each of these unique items appeared both in the context (i.e., within the same block) of a related pair (either lexically related or segmentally related) and in the context of an unrelated pair. Each unique item only appeared in one of the two related conditions, so the data were organized into two subsets based on relatedness: Lexical and Segmental subsets. The Lexical subset included all the trials corresponding to Lexically related items, and the Segmental subset included all the trials corresponding to segmentally related items, regardless of context. In other words, each subset included trials from both related and unrelated contexts, so that we could investigate the effects of relatedness. For example, if CAT appeared both with DOG in a lexically related context, as well as with BUS in an unrelated context then all trials of CAT (both from the lexically related pair and the unrelated pair) were included in the Lexical subset.
The statistical analysis of the written BCN data was based on the data from cycles 1–4, although five cycles were administered. Analysis of the variability of response latencies across cycles showed that variability decreased from cycle 1 to cycle 4 and then significantly increased from cycle 4 to cycle 5 (F = 0.870, p = 0.046), presumably due to observed fatigue. This, along with the fact that a fifth cycle is rarely if ever included in the BCN task, motivated us to exclude cycle 5 from analysis. Two sets of models were used in our statistical analysis of the written BCN data, that investigated: interference effects across cycles 2–4 and Facilitation effects in cycles 1–2. To measure lexical and segmental interference we quantified the effect of Relatedness, comparing performance on Related trials vs. Unrelated trials. To investigate lexical and segmental facilitation, we quantified the effect of Cycle, comparing performance on Cycle 2 vs. Cycle 1. LMEMs were used to separately evaluate the Lexical and Segmental data. The analyses considered RTs5 because, while accuracy measurements were also collected, the errors rates across conditions and individuals low and did not allow us to statistically evaluate performance based on accuracy. RTs were measured from the onset of the target to the initiation of a response. Omissions, error responses in which participants did not correctly produce the target response, responses of RT < 250 ms and outliers of RT greater that 2.5 standard deviations from each condition's (lexical relatedness, segmental relatedness and unrelated) overall mean, calculated for each individual, were removed from further analysis. Errors and outliers accounted for 8% of the data.6 Across all analyses, reaction time data were log-transformed, contrasts for non-continuous variables were sum coded (i.e., the levels of the independent variable sum to 0, to compare each level to the overall mean) and continuous variables were mean-centered.
For the analysis of interference effects, the dependent variable consisted of all RTs from every trial in either the Lexical or the Segmental data subset, for every individual participant. Fixed effects were main effects of Relatedness (related vs. unrelated), Age and Gender, item Frequency,7 Length, Switch/Repeat, Block number, the interaction of these seven variables with Group (stroke vs. healthy), as well as main effects of Group, Hand Intactness and Session number. The Hand Intactness variable indicated whether or not the hand that the stroke participants used to write in the experiment was the hand that they also used for writing premorbidly. The Switch/Repeat variable indicated if, on each trial, the item was switched or repeated relative to the previous trial.8 Session number indicates if a given trial was administered in the first or second session, since some stroke participants completed the task in two different sessions. Block number indicated the order of the block on which a trial was administered within the session. Random effects were intercepts and slopes for Relatedness, Switch/Repeat and Block number by-subject. To determine the statistical significance of group-specific effects, follow-up analyses included models specific to each group. The structure of those models was identical to the models with both groups, except that the main effect of Group and its interactions with the other variables were removed.
For the analysis of facilitation effects, the structure of the models was identical to the models used for the interference effects except for replacing Relatedness with Cycle. In other words, relatedness was collapsed in the analysis of facilitation given that facilitation was measured by comparing RTs from Cycle 1 vs. Cycle 2.
For the Simon task, the statistical analysis of the data was similar to that of the Written BCN task. The major difference was that in the Simon task we exclusively investigated interference effects, which are consistently found across studies and different variations of this task. LMEMs were again used to analyze the RT data. RTs were measured from the onset of the target. Omissions, error responses, responses of RT < 100 ms and outliers of RT greater that 2.5 standard deviations from each condition's (congruent and incongruent) overall mean, calculated for each individual, were removed from the analysis of RTs. Errors and outliers accounted for 13.4% of the data. RT data were log-transformed, contrasts for non-continuous variables were sum coded (i.e., the levels of the predictor summed to zero) and continuous variables were mean-centered. RTs were used as the dependent variable. Fixed effects were main effects of Congruency (congruent vs. incongruent), subject's Age and Gender, the interaction of these three variables with Group (stroke vs. healthy),9 as well as main effects of Group and Hand Intactness. Random effects were intercepts and slopes for Congruency by-subject.
Three statistical analyses were performed to address the questions of interest (see below), and they all utilized cognitive control indices calculated based on the analysis of the main behavioral effects from the written BCN and the Simon tasks discussed above. We calculated five cognitive control indices for every individual:
1. Lexical interference.
2. Segmental interference.
3. Lexical facilitation.
4. Segmental facilitation.
5. Simon interference.
In this study we used the by-Subject random effect slopes to calculate cognitive control indices for each individual instead of the raw RTs, as they allow consideration of effects independently of the contribution of other variables (such as age and gender) since those variables were included in the LMEMs. The slopes were further adjusted to account for overall differences in speed across individuals, which can be quite prominent in the post-stroke population.10 These adjusted slopes more objectively reflect differences in selection effects across individuals compared to the slopes extracted from the LMEMs. For a detailed description of the calculation of the individual cognitive control indices, see Appendix D.11
A total of six correlations were performed -two to address each of the three questions of interest. Spearman correlation was used for this analysis. For Question 1, which is concerned with the domain-specificity of cognitive control mechanisms, we correlated the interference effects across the two cognitive domains, language and visuo-spatial processing: (i) Segmental Interference and Simon Interference, as well as (ii) Lexical Interference and Simon Interference. For Question 2, which is concerned with the level-specificity of selection mechanisms in written word production, we correlated the two interference and the two facilitation effects extracted from the written BCN task with one another: (iii) Lexical Interference and Segmental Interference as well as (iv) Lexical Facilitation and Segmental Facilitation. Finally, for Question 3, which is concerned with the effect-type specificity of the control mechanisms that give rise to the effects (interference vs. facilitation) observed in the BCN task, we correlated interference and facilitation effects for each level of processing: (v) Lexical Interference and Lexical Facilitation, and (vi) Segmental Interference and Segmental Facilitation. False Discovery Rate (FDR) correction for multiple comparisons was applied across the six correlations investigated.
The goal of Analysis 2 was to identify brain regions (parcels) that exhibited a relationship between their integrity and the behavioral cognitive control indices. The parcels identified in Analysis 2 were then further examined in Analysis 3. Simple linear regression models were used separately for each of the five cognitive control indices, for each of the 73 parcels of interest. For each model, the dependent variable was the parcel's Lesion Percentage, and the independent variable was the cognitive control index of interest, with Total Lesion size and Gender included as covariates. We evaluated the Variance Inflation Factor (VIF) of each of the models and found that VIFs for each of the independent variables in each model were at an acceptable level of < 3 (James et al., 2013). Typically, regression analyses evaluating the relationship between brain tissue integrity and behavioral measurements assign the behavioral measurements to the dependent variable, and the brain integrity measurements as an independent variable. However, in the current study, the structure of the regression models was reversed to be able to perform parts of Analysis 3 (see below), which required having multiple cognitive control indices in a given analysis simultaneously, and this could only be achieved if the cognitive control indices were used as independent variables.
The goal of Analysis 3 was to evaluate the specificity of the relationships between gray matter integrity and behavioral cognitive control indices that were identified in Analysis 2. To that end, different analyses were performed to evaluate specificity across Domains and specificity across Levels and Effect-types.
A. Domain specificity
To evaluate Domain Specificity, we carried out two analyses. (1) We evaluated the similarity of the neural distribution of the relationship between gray matter integrity and behavioral cognitive control effects for the two cognitive domains (written word production and visuo-spatial processing) across all parcels. Specifically, we correlated the significance values (p-values) for all parcels from Analysis 2 for Simon Interference and Lexical Interference, and also for Simon Interference and Segmental Interference. (2) We more stringently evaluated these relationships in Broca's Area, the main area of interest in the current study. We did so by evaluating the statistical significance of these effects specifically for the five parcels that form Broca's Area: BA44d, BA45c, BA45r, BA44op, BA44v, applying a correction for multiple comparisons.
B. Level and effect-type specificity
Analysis 2 identified 4 brain parcels that were associated with more than one cognitive control index for Level and Effect-Type. These associations could be considered to be evidence of shared mechanisms. However, it is possible that, instead of a shared mechanism, there are specific mechanisms that are actually supported by different subregions within a parcel that are damaged differently across the participants. One way to evaluate this possibility more stringently is to determine if the two control indices associated with a parcel each explain unique variance in the Lesion Percentage of the parcel.12 The analysis approach described in this section aimed to examine this possibility by evaluating if one of the processing levels associated with the parcel (e.g., Lexical level) accounted for unique variance in Lesion Percentage over and above the variance accounted for by the other processing level (e.g., Segmental level). An analogous approach was used to evaluate effect-type specificity.
To make these determinations, we carried out model comparisons that we refer to as Level Specificity and Effect-Type Specificity Analyses (note: comparable Domain Specificity analyses were not necessary, because there were no brain regions from Analysis 2 in which there was an association with Lesion Percentage and both language and non-language cognitive control indices).
Overall, five different models were used for the model comparisons (all VIFs were below 5). For all five models, Lesion Percentage of the parcel was the dependent variable, while the independent variables were Total Lesion and Gender, as well as the following key variables of interest:
1. Lexical model: lexical interference + lexical facilitation.
2. Segmental model: segmental interference + segmental facilitation.
3. Interference model: lexical interference + segmental interference.
4. Facilitation model: lexical facilitation + segmental facilitation.
5. Full model: lexical interference + lexical facilitation + segmental interference + segmental facilitation.
In general, model comparison evaluates if any additional variability is explained (i.e., change in R2) when variables of interest are added. In this context, for example, a comparison of the Lexical model (#1, above) with the Full Model (#5, above) can evaluate whether Segmental interference and/or facilitation explain any additional and unique variance above and beyond that explained by Lexical interference and/or facilitation. If so, then this would constitute evidence that the brain area supports mechanisms that are specific to Segmental and Lexical control. This approach (a) uses the change in R2 between the two models to quantify unique variance explained by each level and type of cognitive control effects, and (b) allows for the determination of the statistical significance of the change in R2.
For the Level Specificity analysis, since the goal was to determine if there were unique associations between Lesion Percentage and each of the control indices associated with the two processing levels (Segmental and Lexical), we performed model comparisons in parcels that, in Analysis 2, showed statistically significant effects for both the lexical and the segmental levels (regardless of whether the effects were for the interference or the facilitation indices, or both). Only one parcel identified in Analysis 2 was evaluated in the Level specificity analysis (see Table 1). Thus, two model comparisons were performed: (i) Lexical model vs. Full model, and (ii) Segmental model vs. Full model. For the Effect-Type Specificity analysis, since the goal was to evaluate if there were unique associations between Lesion Percentage and each of the two types of cognitive control effects, we performed model comparisons in the parcels that, in Analysis 2, showed statistically significant effects for both interference and the facilitation (regardless of whether the effects were for the lexical or the segmental levels, or both). In total, four parcels identified in Analysis 2 were evaluated in the Effect-Type specificity analysis (see Table 1). Again, two model comparisons were performed for each of the four parcels of interest: (i) Interference model vs. Full model, and (ii) Facilitation model vs. Full model.
Table 1. Parcels identified in Analysis 2 that showed statistically significant effects (p < 0.05) either for both Levels or for both Effect-types, and were therefore further investigated in Analysis 3 (Level and Effect-Type Specificity analyses) (Note that, on the basis of Analysis 2 results, BA45r was evaluated for both Levels and Effect-Types).
In this section we present the results of the statistical analyses that investigated if the main behavioral effects in the two experimental tasks (Simon and Written Blocked Cyclic Naming) were in the expected direction. This was important given that the calculation of the cognitive control indices used in the subsequent analyses (Section B) were based on the outputs of these analyses.
I Interference effects
Results of the two LMEMs for the Lexical and Segmental conditions across all cycles and across both groups are presented in detail in Appendix E. With respect to the main effect of interest, Relatedness was statistically significant for the Lexical condition (p < 0.001), with longer RTs for items named in the Related condition, compared to items in the Unrelated condition. For the Segmental condition, the effect was not statistically significant, but it was still in the same direction (see Figure 5). Importantly, the pattern was the same for both groups. Although the interaction of Relatedness X Group was not statistically significant, the stroke group exhibited numerically large interference effects, indicating disruption to control processes involved in written word production.
II Facilitation effects
Figure 5. Plot of the interaction effect between Relatedness and Group on Reaction Times (RTs) in milliseconds. Panels (A, B) show the effects for the Lexical and the Segmental conditions, respectively. Error bars indicate 95% confidence intervals.
Results of the two LMEMs for the Lexical and Segmental conditions are presented in detail in Appendix E. With respect to the main effect of interest, Cycle was statistically significant for both Lexical (p < 0.001) and Segmental (p = 0.003) conditions, with shorter RTs for items in Cycle 2 compared to items in Cycle 1 (see Figure 6). Again, the overall pattern was the same for both groups.
Figure 6. Blocked cyclic naming task: Plot of the interaction effect between Cycle and Group on Reaction Times (RTs) in milliseconds. Panels (A, B) show the facilitation effects across Cycle 1 and 2 for the Lexical and the Segmental conditions, respectively. Error bars indicate 95% confidence intervals.
Results of the LMEM for the Simon task are presented in detail in Appendix E. With respect to the main effect of interest, Congruency was statistically significant (p < 0.001), with longer RTs for incongruent trials compared to congruent trials (Figure 7), for both groups. Although the interaction of Relatedness X Group was not statistically significant, the stroke group exhibited numerically large interference effects, indicating disruption to control processes involved in response selection in this task.
Figure 7. Simon task: Plot of the interaction effect between Congruency and Group on Reaction Times (RTs) in milliseconds. Error bars indicate 95% confidence intervals.
Overall, the results of the analyses reported in this section confirm that the experimental paradigms we used for both the language and the visuo-spatial domains yield the predicted pattern of results at the group level. This validates the use of these task to calculate the individual cognitive control indices to be used in the subsequent analyses.
In this section we report the results of the main set of three analyses that directly addressed the three questions of interest. Analysis 1 addressed all three questions of interest by evaluating the correlation of the behavioral cognitive control indices that were relevant to each question. Analysis 2 aimed to identify brain regions that exhibited a relationship between their integrity and the behavioral cognitive control indices. Finally, Analysis 3 aimed to evaluate the specificity of the effects identified in Analysis 2. Note: the analyses reported in this section only involve stroke participants.
Figure 8 depicts the correlation values between the cognitive control indices for the comparisons of interest. With regard to Domain Specificity (Question 1), Simon interference did not show a statistically significant relationship with either Lexical (p = 0.65) or Segmental interference (p = 0.25). Regarding Level Specificity (Question 2), the correlation of effects between the two levels of processing was statistically significant, both for the Interference (p < 0.001) and Facilitation (p < 0.001) indices. The correlation of the effects between the two levels (e.g., Lexical and Segmental interference) was positive, indicating that the stroke participants who showed a larger effect at one level, showed a larger effect at the other. Finally, regarding Effect-type Specificity (Question 3), statistically significant correlations were found for the two types of cognitive control indices, both when evaluated using Lexical (p < 0.001) and Segmental (p = 0.001) level indices. The direction of these correlations was positive, indicating that the stroke participants who showed a larger effect of one effect-type, showed a larger effect of the other.
Figure 8. Correlation of cognitive control indices for the comparisons of interest, and the associated FDR corrected p-values (*p-value < 0.05).
For Question 1, the results are consistent with the predictions of the hypothesis of independent, domain-specific mechanisms for written word production and visuo-spatial processing. In contrast, for Questions 2 and 3, they are consistent with the predictions of the hypotheses of shared mechanisms across levels (lexical and segmental) and effect-types (interference and facilitation) in written word production.
Given that the conclusion of domain-specific mechanisms for written word production and visuo-spatial processing was based on a null effect (i.e., lack of a strong correlation between the behavioral cognitive control indices in the two domains), we also performed a post-hoc Bayesian analysis that evaluated the strength of the evidence for the null hypothesis that the correlation between the relevant behavioral indices is equal to 0, as opposed to the alternative hypothesis that the correlation between the relevant behavioral indices is >0. For details of the analysis see Appendix F. The results provided substantial evidence in support of the null hypothesis, namely that Simon Interference is not correlated with either Lexical (bayes factor = 5.85) or Segmental Interference (bayes factor = 7.04). The analysis provided no evidence in support of the alternative hypothesis for either Lexical (bayes factor = 0.17) or Segmental Interference (bayes factor = 0.14).
Figure 9 depicts and Table 2 reports the parcels that showed significant effects (p < 0.05; uncorrected) for each of the five cognitive control indices. Note that, for both interference and facilitation effects, positive values indicate that more brain damage is associated with higher levels of interference or facilitation, while negative values indicate that less brain damage is associated with higher levels of interference or facilitation.
Figure 9. Overlap map of the parcels that showed significant effects (p < 0.05; uncorrected) for each cognitive control index (note: because lexical interference overlaps entirely with lexical facilitation, no red areas are visible).
Table 2. The 17 parcels (of the total 73 examined) that showed a significant (*p < 0.05, uncorrected) effect in Analysis 2 for at least one of the five control indices.
As can be seen in Figure 9, the parcels that showed significant effects for the different cognitive control indices were in most cases adjacent to each other. There were two clusters of parcels: (1) the parcels associated with both Lexical Interference and Facilitation formed a cluster in the inferior frontal and orbital gyri, and (2) the parcels associated with Simon Interference formed one large cluster extending across inferior, middle frontal, and precentral gyri. There were just a few parcels that were not associated with these two major clusters including a parcel in the inferior parietal lobe associated with Lexical Facilitation, a parcel in the superior temporal gyrus associated with Segmental Interference, a parcel in the orbital gyrus associated with Segmental Facilitation and two parcels in the parahippocampal gyrus associated with Simon Interference.
In sum, this analysis revealed: (a) no overlap between the parcels that showed control effects for the domains of written word production and visuo-spatial processing and (b) the effects of levels and effect-type control overlapped in a number of frontal parcels. Analysis 3 aimed to examine these two sets of findings more closely.
A. Domain specificity
To evaluate Domain specificity, first, for the 73 parcels evaluated in Analysis 2, we correlated the significance values (p-values) for Simon Interference and Lexical Interference, and also for Simon Interference and Segmental Interference. We found that the p-values across parcels for the association of Simon interference with Lesion Percentage showed no statistically significant correlation with either the p-values of the association of Lexical (r = −0.09, p = 0.43) or Segmental (r = −0.15, p = 0.19) interference with Lesion Percentage. This finding provides further support for the absence of a relationship between the cognitive control mechanisms in the two domains.
Second, we focused specifically on the five parcels that make up Broca's Area. For these, we found that the dissociation identified in Analysis 2 between BA45r (which showed relationships only with the written word production control indices) and the rest of the Broca area parcels (which showed relationships only with the visuo-spatial domain control index) is present even after correction for multiple comparisons across the Broca's Area parcels (see Table 3).
B. Level and effect-type specificity
To better determine whether or not the parcels that showed effects of multiple Level and Effect-type control indices in Analysis 2 should be interpreted as providing evidence for shared control mechanism or of distinct mechanisms, we evaluated, for each parcel, whether the individual indices accounted for unique variance above and beyond that explained by the other level/effect-type. We did so by using model comparisons, as detailed in Section 3.2.3.B. Table 4 presents the unique variance explained (Unique-R2) and the associated p-values of the relevant model comparisons, separately for each parcel of interest, and separately for (A) Level specificity and (B) Effect-type specificity.
Level Specificity was examined for the single parcel (BA45r) that, in Analysis 2, showed statistically significant effects for both the lexical and the segmental levels (regardless of whether the effects involved interference or the facilitation indices, or both). Effect-Type Specificity was examined in the four parcels that, in Analysis 2, showed statistically significant effects for both the interference and the facilitation indices (regardless of whether the effects were for the lexical or the segmental levels, or both (see Table 1).
As can be seen in Tables 4A, B, the results of Analysis 3B show that it was only in BA45r that lesion patterns across participants explained statistically significant unique variance in the cognitive control indices for levels and effect-types. Figure 10 reports the distribution of explained variance among the variables tested for BA45r, separately for levels of processing (Figure 10A) and types of cognitive control indices (Figure 10B).
Figure 10. Distribution of variance explained by the variables tested for parcel BA45r, separately for (A) Level Specificity (Lexical vs. Segmental) and (B) Effect-type Specificity (Interference vs. Facilitation). “Shared” indicates the variance that is shared by the two variables of interest.
In this study, we used data from a group of individuals with post-stroke aphasia to study, for the first time, the neural substrates of cognitive control in written word production. To do so, we studied written word production using the blocked cyclic naming paradigm (BCN) which serves to amplify selection difficulty in written word production. Specifically, we investigated whether: (1) control mechanisms are specific to or shared by language and non-language cognitive domains; (2) control mechanisms within the word production system are specific to or shared by the lexical and segmental levels of word production; and, (3) interference and facilitation effect types arise from the same or different control mechanisms. Our key findings were as follows: (1) There are domain-specific control mechanisms used in written word production that are not shared with control mechanisms involved in visuo-spatial processing; (2) With regard to Broca's Area (BA 44/45), we found that distinct and nonoverlapping regions are associated with control in written word production vs. control in visuo-spatial processing (see beige vs. green parcels in Figure 11); (3) Rostral BA45 in Broca's Area (beige parcel in Figure 11) was found to support multiple types of cognitive control mechanisms that are specific to lexical and segmental levels of written word production, as well as mechanisms specifically associated with facilitation and interference; (4) We found evidence that orbital frontal cortex (pink parcels in Figure 11) supports control involved in both facilitation and interference that was strongly associated with lexical level control and less so with segmental control; (5) We did not find clear evidence of shared control mechanisms for lexical and segmental levels of written word production.
Figure 11. Parcels in the frontal lobe that exhibited significant control effects across analyses. In beige: BA45r, where Lexical, Segmental, Interference and Facilitation effects in written word production were each found to be associated with specific control mechanisms. In pink: BA11l, BA12/47l and BA12/47o, where Interference and Facilitation effects in written word production were associated with a shared control mechanism. In green: BA45c, BA44d, BA44v, and BA44op, where there was evidence of a control mechanism associated only with Interference on the Simon visuo-spatial compatibility task.
Before discussing the relevant evidence below, we would like to highlight an important aspect of the current study. This study was specifically designed to study mechanisms of cognitive control independently of mechanisms that support the representation of orthographic forms. In studies with healthy individuals because both representation and cognitive control processes are assumed to be intact, experimental manipulations designed to specifically “tax” cognitive control processes can be assumed to be largely effective. However, in studies with individuals with language impairments, this cannot be taken for granted. In the current study, in order to isolate effects related to cognitive control from effects related to orthographic representation, for each individual we identified words that they were able to consistently name correctly. By establishing that the representations of the items used in the experimental task were largely intact, we could more confidently argue that the experimental manipulations used were largely successful in specifically targeting cognitive control processes. Indeed, as shown from the analysis of the main behavioral effects (Section 4.1.1.), the group of stroke-aphasia individuals in this study showed the same pattern of effects as healthy controls. Specifically, both in the written BCN and the Simon tasks, stroke-aphasia individuals showed increased response times in the conditions with increased cognitive control demands (i.e., the related condition in the written BCN task, and the incongruent condition in the Simon task; see Figures 5, 6). On the basis of these results, we then proceeded to address the main questions of this study.
The first question was concerned with whether or not cognitive control in written word production is supported by domain-specific or domain-general mechanism(s). A domain-specific mechanism would be a mechanism that would specifically support cognitive control in written word production,13 while a domain-general mechanism would support cognitive control across different cognitive systems. In this study, we examined the relationship between cognitive control in written word production and cognitive control in the domain of visuo-spatial processing. As a reminder, to address this question, analysis was limited to behavioral interference effects only (i.e., not facilitation effects) as this is the effect type most often studied in both domains. We found that the evidence for independent control mechanisms for visuo-spatial processing and written word production was clear and compelling.
First, the results of the behavioral investigation (Analysis 1) showed no significant correlation between the interference effects observed in the two domains (p's > 0.2). In other words, individuals with difficulty exercising control and thus strong interference effects in one domain did not necessarily exhibit strong interference effects in the other domain. Second, our analysis of the neural data (Analysis 2) showed that, for both domains, cognitive control was associated with damage primarily in frontal areas, providing evidence for the role of executive mechanisms in the two experimental tasks used. However, as shown in Figure 11 (beige vs. green parcels), the spatial distribution of the effects was very distinct, with no overlap between them. Analysis 3A, statistically evaluating the overlap between the spatial distribution of the cognitive control effects in the two domains, showed that the distributions were not significantly correlated. Furthermore, when we specifically considered the five parcels that make up Broca's Area (BA44 and 45), we found a completely non-overlapping distribution of significant associations for the two domains, as reported in Table 3. Within Broca's Area, cognitive control for written word production was concentrated in the rostral part of BA45, while the control mechanisms associated with the Simon task were distributed across BA44 and the anterior portion of BA45. These findings complement previous results from neurostimulation studies, showing that TMS stimulation of the LIFG affected cognitive control processes in language tasks, but not in non-language, perceptual tasks (Devlin et al., 2003; Whitney et al., 2011).
While the bulk of the findings for both domains involved the frontal lobe, some relationships between lesion percentage and cognitive control were also found in non-overlapping areas outside the frontal lobe (see Figure 9). For written word production we found significant associations in the left superior temporal gyrus and inferior parietal lobule, while for the visuo-spatial domain, we found effects in the left parahippocampal gyrus.
Taken together, these findings provide strong evidence for domain-specific cognitive control mechanisms, at least with respect to written word production and visuo-spatial processing. The convergence of the behavioral and neural findings increases confidence in the conclusion of independent control mechanisms across domains. However, further investigations with a broader range of tasks and with data suitable for investigating both hemispheres are still needed before ruling out that there may also be domain-general cognitive control processes that contribute to written word production and to develop a more comprehensive understanding of these issues. This is especially necessary given claims in the literature that domain-general cognitive control mechanisms, like inhibitory control, are involved in word production (Shao et al., 2013), as well as in other language functions, like syntactic comprehension (January et al., 2009; Ye and Zhou, 2009), and that these mechanisms are supported by Broca's Area (January et al., 2009; Ye and Zhou, 2009; Novick et al., 2010) or the left dorsolateral prefrontal cortex more broadly (Duncan and Owen, 2000; Duncan, 2010), as well as the left parietal lobe (Nagel et al., 2008).
The second question concerned whether or not cognitive control in written word production is supported by level-specific or shared mechanism(s) at the two key levels of word production: lexical and segmental. Analyses of the behavioral data (Analysis 1) revealed statistically significant correlations between cognitive control effects across the two levels of processing. This would seem to indicate that cognitive control mechanisms are shared between levels of processing. However, alternative interpretations that do not assume a shared mechanism can also explain the association of effects in the behavioral data. For example, one alternative hypothesis is that cognitive control at the two levels of processing is, in fact, supported by distinct cognitive control mechanisms (i.e., the mechanisms are level-specific), but that these mechanisms are supported by neurally adjacent regions that tend to be similarly affected by the neurological damage, but which can be selectively damaged. The examination of the neural data could therefore be useful in distinguishing between these two possible interpretations of the observed association between the behavioral effects.
Analysis 2 provided evidence of both dissociations and associations between the neural substrates of selection mechanisms associated with the two levels of processing. Dissociations were observed in the orbitofrontal cortex such that BA11 and 12/47 were associated with Lexical control indices but not Segmental ones, while BA13 showed the reverse pattern. Only BA45r showed an association with control indices at both levels. Analysis 3B was carried out to specifically evaluate if either cognitive control index accounted for unique variance in the lesion distribution of BA45r. The results of this analysis showed that most of the variance that was explained by the combination of the Lexical and Segmental behavioral cognitive control effects was decomposed into unique associations of each of the two behavioral cognitive control effects with variance in the integrity of BA45r, providing further evidence for level-specificity.
It is worth noting that although Analysis 2 did not identify any parcels other than BA45r in which the extent of damage was associated with both Lexical and Segmental levels this is a null result that could have resulted due to low statistical power. In other words, it is possible that there might have been additional parcels in which there were associations between percentage lesion and lexical and segmental indices that we were unable to identify given our sample.
Overall, the results from our neural data analyses provide evidence for distinct mechanisms of cognitive control at lexical and segmental levels of processing in written word production. These were associated with BA45r and also with parcels within the orbital frontal cortex. These findings largely converge with those reported for spoken word production where evidence from studies with healthy individuals primarily supports the hypothesis of distinct cognitive control mechanisms. For example, Schnur et al. (2009), using the blocked cyclic naming paradigm to study spoken word production, provided evidence that the activation in the LIFG is associated with semantic interference, but not with phonological interference. Evidence from comprehension studies also points to a dissociation of cognitive control mechanisms for the two levels of processing (Devlin et al., 2003; Badre et al., 2005; Gough et al., 2005; Attout et al., 2022). In a TMS study, Gough et al. (2005) showed that, compared to no stimulation, stimulation over the anterior part of the LIFG selectively increased the response latencies for synonym judgements, but not for homophone judgments. The opposite pattern was observed when the posterior part of the LIFG was stimulated. The same dissociation was also reported in an fMRI study (Devlin et al., 2003), providing support for functionally distinct subdivisions of the LIFG that separately support a relationship with selection at semantic and phonological levels of processing. These studies have shown that more anterior regions of the LIFG support lexical level processing, and more posterior regions support segmental level processing. In the current investigation, however, we did not find clear support for this anterior/posterior distinction. We found evidence for distinct Lexical and Segmental control mechanisms within posterior BA45r. Also, both lexical and segmental control were associated with different parcels in anterior orbital frontal cortex (i.e., BA11, BA12/47, and BA13).
Finally, two parcels outside of the frontal lobe exhibited relationships with cognitive control in written word production. Segmental control was associated with the anterior part of the temporal gyrus (BA38). Associations of temporal regions with cognitive control in word production have been previously reported in the spoken modality (de Zubicaray et al., 2006; Noonan et al., 2013), and have been explained as the result of the operation of top-down processes originating from the prefrontal regions, manipulating the activation levels of representations stored in the temporal lobe (Miller, 2000). In addition, Lexical control effects were associated with damage to the inferior parietal lobe region, specifically, the angular gyrus (BA39), an area that has been previously implicated in semantic processing (Bonner et al., 2013; Price et al., 2015), as well as in semantic control more specifically (Noonan et al., 2013).
Finally, it is important to point out that, despite the strong evidence of distinct mechanisms of cognitive control at the two levels of processing in written word production, there can still be cognitive control mechanism/s that is/are shared between these two levels of processing. A shared mechanism would interact with diverse types of representations, including lexical and phonological ones. Even in the current set of findings we identified a portion of the variance in the integrity of BA45r that was shared between Lexical and Segmental behavioral cognitive control effects (see Figure 10). Thus, further investigations are needed to fully uncover the complex interplay between distinct and shared mechanisms of cognitive control across levels of processing in written word production.
The third question considered if the cognitive control mechanisms that support written word production are effect-type specific. In other words, if the same and/or different mechanisms give rise to the two types of behavioral effects observed in written word production—facilitation and interference.
The specific implementation of the BCN task used in this study, creates an environment in which multiple and/or prepotent responses are “in competition”14 and in which both facilitation and interference effects may be expected. Based on the various proposals in the literature we can speculate on how control might operate in this environment. Belke (2008, 2017) proposed that in BCN a top-down biasing mechanism uses knowledge of the task goals and the items involved (available after the 1st cycle) to send activation to the anticipated targets. This would account for the facilitation effects observed for the second cycle relative to the first. However, spreading activation within the word production system can be expected to especially increase activation among related items, eventually, after multiple cycles, increasing the difficulty of selecting the correct response for production. This conflict would be detected by the conflict monitor (Botvinick et al., 2001; Nozari et al., 2011; Nozari and Hepner, 2019b) which would act to resolve the conflict and reduce interference by enlisting mechanisms that would work to ensure selection of the target by boosting the target and/or suppressing its competitors. For example, Oppenheim et al. (2010) proposed a booster mechanism that repeatedly amplifies each item's activation until a winner can be selected among the items that are already activated. This may or may not be the same top-down biasing mechanism described just above (Belke, 2008, 2017) that operated prior to a signal from the conflict monitor. Also note that, in the context of the BCN task (especially with only two targets), facilitation of targets will soon begin to create interference as the targets serve as competitors within the next trial or two. In addition to the top-down biasing mechanism, selection may be assisted by an inhibitory control mechanism that would work to suppress activation of the competitors. Here again, in this task, the competitors that are inhibited will, within a trial or two, serve as the targets. As a result, in this specific task environment, both faciliatory and inhibitory mechanisms may serve to produce a net interference effect. In the course of their operation, the mechanisms involved in cognitive control produce the observable effects of facilitation and interference that we can use to draw inferences about whether or not these effects arise from the same or different mechanisms.
The high correlation of the behavioral interference and facilitation effects in Analysis 1 provides evidence for the hypothesis that shared cognitive control mechanisms give rise to facilitation and interference effects. Also consistent with this, Analysis 2 identified a cluster of orbital frontal parcels (BA12/47l, BA12/47o, and BA11) associated with both Facilitation and Interference (see pink parcels in Figure 11). Furthermore, the fact these effects did not dissociate in Analysis 3B, and that a portion of the variance in the integrity of BA45r was explained jointly by Interference and Facilitation control indices, is also consistent with the role of a common mechanism giving rise to both facilitation and interference. In contrast, however, Analysis 3B also found that, in BA45r, interference and facilitation indices each explained unique variance in lesion distribution. This latter finding constitutes evidence that separate mechanisms are associated with facilitation and interference effect. A further finding to consider is that throughout these regions the relationship between lesion extent and magnitude of facilitation and interference was negative, indicating that more damage was similarly associated with less facilitation and also less interference. In sum, the study finds evidence that the interference and facilitation effects that we observe (a) may arise both from shared and distinct mechanisms, and that (b) damage to these mechanisms (at least for the experimental task used) results in reduction of both facilitation and interference effects.
Given our relative lack of understanding of the functional architecture of cognitive control in word production and the complexity of the interactions amongst the mechanisms, it is especially difficult to make predictions regarding behavioral patterns and lesion-symptom mapping in an environment in which one or more of these mechanisms may be damaged. This necessarily challenges our ability to interpret the findings. Here, we speculatively suggest one possible interpretation. In terms of a shared mechanism, the same top-down biasing mechanism may be responsible for sending additional activation into the system early in the task (based on knowledge of task goals and items) generating facilitation. It may also be triggered later in response to detection of the conflict that is developing and increasing. At that point, it sends additional activation to the target which, in combination with spreading activation, actually leads to an increase in the activity of competitors and, therefore, to an increase interference. In this way, a single mechanism may be responsible for both facilitation and interference effects. With regard to the evidence of different mechanisms, it may be that the shared biasing mechanism is triggered at different points in time by different monitoring mechanisms. Much like in the attentional system, the early signal to engage the bias control mechanism might be considered to be “exogenous” (based on determining probabilities of upcoming words), while the later signal might be considered to be “endogenous” to the word production system (based on activation levels/competition). Different mechanisms may be responsible for monitoring these two situations. Finally, there is what could be considered to be the paradoxical finding that greater damage to these mechanisms is associated with smaller facilitation and interference effects. Damage to the biasing mechanism could certainly explain the reduction in facilitation. Furthermore, if it is the case that this mechanism (as described above) is eventually responsible for increasing interference, then damage to it could be associated with reduction in interference. This is a plausible scenario but, most certainly, prediction and interpretation in these complex systems will require further empirical work that will likely also benefit greatly from computation modeling.
As discussed in the Introduction, there is a plethora of evidence linking the LIFG, and Broca's Area specifically, to cognitive control across domains as well as to specific distinct functions/levels of processing within the language domain. However, Broca's Area is a rather large region, with cyto-architectonically and anatomically distinct subregions, and, referring to it as a single, homogeneous entity can be misleading. When addressing questions regarding the specificity of the cognitive control mechanisms supported by this region, it is important to use data and analytic approaches that are well-suited for these types of questions. Without directly comparing cognitive control across different domains and functions/levels of processing within the language system itself it is difficult to draw strong conclusions about the specificity of the mechanism/s that are supported by this region. Nonetheless, even in cases that follow this approach, the results have varied. Some studies have provided evidence that Broca's Area supports a general cognitive control mechanism (e.g., January et al., 2009; Ye and Zhou, 2009; Fedorenko et al., 2012), while other studies have provided evidence that it supports specific cognitive control mechanisms (e.g., Devlin et al., 2003; Badre et al., 2005; Gough et al., 2005; Whitney et al., 2011; Attout et al., 2022). This underscores that further investigation deploying a range of analytic approaches will be required to provide the evidence needed to clarify and reconcile these findings.
To examine the internal organization of Broca's Area, we used an atlas that separates BA44/45 in five different parcels. On that basis we identified a subregion of Broca's Area -BA45r- for which we identified unique relationships between the distribution of damage and behavioral indices of cognitive control at different levels of written word production, and we found that there was no relationship between the distribution of damage to this area and the cognitive control involved in visuo-spatial processing. Cognitive control in visuo-spatial processing was found, instead, to be associated with other subregions of Broca's Area. This complementary pattern of relationships between the Broca's Area parcels and the control indices in the two cognitive domains under investigation is in line with the rest of the findings we reported in this study, which support domain-specific cognitive control mechanisms. Given that previous investigations on spoken word production have shown cognitive control (e.g., semantic interference effects) associations throughout Broca's Area, future studies need to explore the extent to which this complementary pattern of relationships we report here is specific to the written modality and/or whether certain methodological parameters (e.g., the fact that we have subdivided BA44/45 into five parcels) have contributed to it. These findings highlight the need to deploy methods and analytic approaches that are specifically well-suited for evaluating the types of complex questions that need to be addressed in order to further our understanding of the role of Broca's Area in cognitive control and language processing more generally.
The results of our investigation showed that distinct cognitive control mechanisms support written word production and visuo-spatial processing. While this evidence supports the domain-specific hypothesis, further investigations using tasks that involve other cognitive domains are still needed to determine the full extent of the domain-specificity of the cognitive control mechanisms that contribute to written word production. Also, it will be important for future work to examine the relationship between cognitive control mechanisms used in written and spoken word production to understand whether these mechanisms are shared or independent across these two modalities of word production. Finally, due to the relatively small sample, we were limited to an ROI-based analysis of the neural data. This analysis might have been too coarse-grained to allow us to observe certain relationships between behavioral effects and neural integrity. Therefore, it would be useful for future studies with greater analytic power to examine these relationships in greater spatial detail.
A broader concern is that the interpretation of lesion-deficit associations individuals with brain damage can be challenging given that what we observe is the end-result of complex neuroplastic changes that occur subsequent to brain damage (Shallice, 1988). The possibility of these changes raises questions regarding the extent to which conclusions reached reflect the organization of the undamaged system. While research has consistently shown striking convergence of conclusions based on data from brain-damaged and neurologically healthy individuals, it is not a guaranteed outcome. For example, it is possible that damage to areas that under normal circumstances support a given control mechanism forces these individuals to use alternative, compensatory mechanisms that may otherwise not normally be used in an intact system. Thus, it could be that the damaged areas we found to be associated with cognitive control in the current study were recruited only because the areas that normally instantiate these functions were too damaged to show significant relationships with behavior. To address concerns such as these, neuroimaging studies with healthy individuals are needed to determine if there is convergence of findings across these populations.
This is the first investigation of the neural mechanisms that support cognitive control in written word production. The results strongly support the hypothesis of domain-specific mechanisms for cognitive control in written word production, relative to control mechanisms used for visuo-spatial processing more generally. With respect to the level-specificity of control mechanisms within the written word production system, we provided evidence of both shared and distinct mechanisms for Lexical and Segmental control. In line with previous studies in other domains, cognitive control mechanisms were shown to be primarily instantiated in left prefrontal regions with our results particularly highlighting the role of Broca's Area, specifically BA45, in cognitive control in written word production. Our experimental manipulations were able to isolate the contribution of this region to cognitive control independently of the representations of the word forms themselves, and our statistical analyses allowed us to identify evidence of unique associations with levels of written word production (lexical and segmental) as well as types of effects (interference and facilitation). These results emphasize the intricate neural instantiation of functions within Broca's Area, as well as its contribution to a wide range of language processing mechanisms.
The raw data supporting the conclusions of this article will be made available by the authors, without undue reservation.
The studies involving humans were approved by Johns Hopkins University Institutional Review Board. The studies were conducted in accordance with the local legislation and institutional requirements. The participants provided their written informed consent to participate in this study.
KN: Conceptualization, Data curation, Formal analysis, Investigation, Methodology, Project administration, Software, Writing – original draft, Writing – review & editing. RW: Formal analysis, Methodology, Software, Supervision, Writing – review & editing. BR: Conceptualization, Formal analysis, Methodology, Supervision, Writing – original draft, Writing – review & editing.
The author(s) declare that financial support was received for the research, authorship, and/or publication of this article. This work was supported by the Scholarship for Hellenes awarded to KN by the Onassis Foundation and funding support to BR from the Therapeutic Cognitive Neuroscience Fund, Cognitive Neurology/Neuropsychology Division, Department of Neurology, Johns Hopkins Medical Institutions, and anonymous donors.
We would like to thank our participants for their dedication and interest in our study, and the Snyder Center for Aphasia Life Enhancement (SCALE) Aphasia Program for their support in participant recruitment and facilitation of data collection. We would also like to thank Prof. Michael McCloskey for providing us with in-house developed scripts to record written responses from the graphic tablet. The input provided by Professors Bonnie Nozari and Susan Courtney with regard to various aspects of the project were invaluable.
The authors declare that the research was conducted in the absence of any commercial or financial relationships that could be construed as a potential conflict of interest.
All claims expressed in this article are solely those of the authors and do not necessarily represent those of their affiliated organizations, or those of the publisher, the editors and the reviewers. Any product that may be evaluated in this article, or claim that may be made by its manufacturer, is not guaranteed or endorsed by the publisher.
The Supplementary Material for this article can be found online at: https://www.frontiersin.org/articles/10.3389/flang.2024.1398125/full#supplementary-material
1. ^Lexically related items were semantically related based on taxonomic relationships (e.g., dog-cat) rather than thematic relationships (e.g., cow-milk), since only taxonomically related items have been consistently found to cause interference effects (Oppenheim and Nozari, 2021).
2. ^Two stroke participants (GWL and RBM) were pre-tested on an additional set of 30 items, since we were unable to find 24 items that they could correctly name and spell twice from the original set of 72 items.
3. ^In the classic design of the BCN task, a cycle has unique items, each presented once before the next cycle begins. However, given that in the present study only two items were used in each block and their order was pseudorandomized, cycles could not be structured in the traditional manner. Therefore, in the current study, cycle is synonymous to the presentation “number “of a given item (e.g., Cycle 2 of item X is the second presentation of item X, Cycle 3 is its third presentation, up to Cycle 5). This was modeled after Nozari et al. (2016).
4. ^While the main region of interest in the current study was the LIFG, we studied all regions with substantial damage across individuals.
5. ^The performance of individuals with aphasia on this task is more commonly assessed based on accuracy but given that we selected items that each stroke participant could name and spell correctly and was refamiliarized with before testing, the high accuracy values we observed were not surprising.
6. ^As a reference, the percentage of error rates reported in, for example, Schnur et al. (2006), which is the only other study that used the BCN paradigm in a group of individuals with aphasia, was 28%.
7. ^Item frequency was indexed using subtitle frequency from the English Lexicon Project (Balota et al., 2007).
8. ^This was particularly relevant in this task design as there were only two distinct items in each block.
9. ^The interaction of Age × Group was subsequently removed because when it was included the model did not converge.
10. ^We also calculated the individual effects without adjusting for overall differences in speed, and the correlation between the adjusted and non-adjusted cognitive control indices ranged between 0.93–0.98. This indicates that the speed adjustment did not wash out the main effects contributing to the behavioral cognitive control indices.
11. ^Simon effects have shown varying levels of reliability in the past, ranging from moderate (Paap and Sawi, 2016) to high (Soveri et al., 2018) test-retest reliability. In the current study, split-half reliability for the Simon Interference effects was 0.98.
12. ^A more direct way to evaluate this possibility would be with a more fine-grained brain parcellation. However, this was not possible, given the relatively small sample size of this study.
13. ^Or, language production more generally, but that was not evaluated with this dataset.
14. ^Whether there is direct competition between items such that that the activity of one influences the others (e.g., via lateral inhibition mechanisms) or if, instead, the competition arises by virtue of similar/overlapping activation functions (e.g., as in a horse race) is a matter of debate in the word production literature (see Oppenheim and Balatsou, 2019, for a review). We do not believe that this distinction influences the conclusions of this study.
Abraham, A., Pedregosa, F., Eickenberg, M., Gervais, P., Mueller, A., Kossaifi, J., et al. (2014). Machine learning for neuroimaging with scikit-learn. Front. Neuroinform. 8:14. doi: 10.3389/fninf.2014.00014
Attout, L., Grégoire, C., Querella, P., and Majerus, S. (2022). Neural evidence for a separation of semantic and phonological control processes. Neuropsychologia 176:108377. doi: 10.1016/j.neuropsychologia.2022.108377
Badre, D., Poldrack, R. A., Paré-Blagoev, E. J., Insler, R. Z., and Wagner, A. D. (2005). Dissociable controlled retrieval and generalized selection mechanisms in ventrolateral prefrontal cortex. Neuron 47, 907–918. doi: 10.1016/j.neuron.2005.07.023
Balota, D. A., Yap, M. J., Hutchison, K. A., Cortese, M. J., Kessler, B., Loftis, B., et al. (2007). The English lexicon project. Behav. Res. Methods 39, 445–459. doi: 10.3758/BF03193014
Bates, D., Maechler, M., Bolker, B., Walker, S., Christensen, R. H. B., Singmann, H., et al. (2015). Package ‘lme4'. convergence, 12, 2.
Belke, E. (2008). Effects of working memory load on lexical-semantic encoding in language production. Psychon. Bull. Rev 15, 357–363. doi: 10.3758/PBR.15.2.357
Belke, E. (2017). Effects of lesions to the left lateral prefrontal cortex on task-specific top-down biases and response strategies in blocked-cyclic naming. Cogn. Neuropsychol. 34, 26–32. doi: 10.1080/02643294.2017.1329200
Belke, E., Meyer, A. S., and Damian, M. F. (2005). Refractory effects in picture naming as assessed in a semantic blocking paradigm. Q. J. Exp. Psychol. A 58, 667–692. doi: 10.1080/02724980443000142
Belke, E., and Stielow, A. (2013). Cumulative and non-cumulative semantic interference in object naming: evidence from blocked and continuous manipulations of semantic context. Q. J. Exp. Psychol. 66, 2135–2160. doi: 10.1080/17470218.2013.775318
Ben-Shachar, M., Hendler, T., Kahn, I., Ben-Bashat, D., and Grodzinsky, Y. (2003). The neural reality of syntactic transformations: evidence from functional magnetic resonance imaging. Psychol. Sci. 14, 433–440. doi: 10.1111/1467-9280.01459
Berg, T., and Schade, U. (1992). The role of inhibition in a spreading-activation model of language production. I. The psycholinguistic perspective. J. Psycholinguist. Res. 21, 405–434. doi: 10.1007/BF01067522
Berker, E. A., Berker, A. H., and Smith, A. (1986). Translation of Broca's 1865 report. Localization of speech in the third left frontal convolution. Arch. Neurol. 43, 1065–1072. doi: 10.1001/archneur.1986.00520100069017
Bonner, M. F., Peelle, J. E., Cook, P. A., and Grossman, M. (2013). Heteromodal conceptual processing in the angular gyrus. Neuroimage 71, 175–186. doi: 10.1016/j.neuroimage.2013.01.006
Botvinick, M. M., Braver, T. S., Barch, D. M., Carter, C. S., and Cohen, J. D. (2001). Conflict monitoring and cognitive control. Psychol. Rev. 108:624. doi: 10.1037/0033-295X.108.3.624
Breining, B., Nozari, N., and Rapp, B. (2016). Does segmental overlap help or hurt? Evidence from blocked cyclic naming in spoken and written production. Psychon. Bull. Rev. 23, 500–506. doi: 10.3758/s13423-015-0900-x
Breining, B., and Rapp, B. (2019). Investigating the mechanisms of written word production: insights from the written blocked cyclic naming paradigm. Read. Writ. 32, 65–94. doi: 10.1007/s11145-017-9742-4
Broca, P. (1861). Remarks on the seat of the faculty of articulated language, following an observation of aphemia (loss of speech). Bull. Soc. Anat. 6, 330–357.
Brown, A. S. (1981). Inhibition in cued retrieval. J. Exp. Psychol. Hum. Learn. Mem. 7:204. doi: 10.1037//0278-7393.7.3.204
Buckner, R. L., Raichle, M. E., and Petersen, S. E. (1995). Dissociation of human prefrontal cortical areas across different speech production tasks and gender groups. J. Neurophysiol. 74, 2163–2173. doi: 10.1152/jn.1995.74.5.2163
Caplan, D. (2014). Commentary on “The role of domain-general cognitive control in language comprehension” by Fedorenko. Front. Psychol. 5:629. doi: 10.3389/fpsyg.2014.00629
Caramazza, A. (1997). How many levels of processing are there in lexical access? Cogn. Neuropsychol. 14, 177–208. doi: 10.1080/026432997381664
Cohen, J. D., and Servan-Schreiber, D. (1992). Context, cortex, and dopamine: a connectionist approach to behavior and biology in schizophrenia. Psychol. Rev. 99:45. doi: 10.1037/0033-295X.99.1.45
Costa, A. (2019). A flexible criterion of response selection: when is it needed? Comment on Nozari and Hepner (2018). Cogn. Neuropsychol. 36, 208–211. doi: 10.1080/02643294.2018.1532404
Crowther, J., and Martin, R. (2014). Lexical selection in the semantically blocked cyclic naming task: the role of cognitive control and learning. Front. Hum. Neurosci. 8:9. doi: 10.3389/fnhum.2014.00009
Damian, M. F. (2003). Articulatory duration in single-word speech production. J. Exp. Psychol. Hum. Learn. Mem. 29, 416–431. doi: 10.1037/0278-7393.29.3.416
Damian, M. F., and Als, L. C. (2005). Long-lasting semantic context effects in the spoken production of object names. J. Exp. Psychol. Learn. Mem. Cogn. 31, 1372–1384. doi: 10.1037/0278-7393.31.6.1372
Damian, M. F., Vigliocco, G., and Levelt, W. J. M. (2001). Effects of semantic context in the naming of pictures and words. Cognition 81, B77–B86. doi: 10.1016/S0010-0277(01)00135-4
de Zubicaray, G., McMahon, K., Eastburn, M., and Pringle, A. (2006). Top-down influences on lexical selection during spoken word production: a 4T fMRI investigation of refractory effects in picture naming. Hum. Brain Mapp. 27, 864–873. doi: 10.1002/hbm.20227
Dell, G. S. (1986). A spreading-activation theory of retrieval in sentence production. Psychol. Rev. 93, 283–321.
Dell, G. S., Schwartz, M. F., Martin, N., Saffran, E. M., and Gagnon, D. A. (1997). Lexical access in aphasic and nonaphasic speakers. Psychol. Rev. 104:801. doi: 10.1037/0033-295X.104.4.801
Devlin, J. T., Matthews, P. M., and Rushworth, M. F. (2003). Semantic processing in the left inferior prefrontal cortex: a combined functional magnetic resonance imaging and transcranial magnetic stimulation study. J. Cogn. Neurosci. 15, 71–84. doi: 10.1162/089892903321107837
Di Russo, F., Taddei, F., Apnile, T., and Spinelli, D. (2006). Neural correlates of fast stimulus discrimination and response selection in top-level fencers. Neurosci. Lett. 408, 113–118. doi: 10.1016/j.neulet.2006.08.085
Duncan, J. (2010). The multiple-demand (MD) system of the primate brain: mental programs for intelligent behaviour. Trends Cogn. Sci. 14, 172–179. doi: 10.1016/j.tics.2010.01.004
Duncan, J., and Owen, A. M. (2000). Common regions of the human frontal lobe recruited by diverse cognitive demands. Trends Neurosci. 23, 475–483. doi: 10.1016/S0166-2236(00)01633-7
Fan, L., Li, H., Zhuo, J., Zhang, Y., Wang, J., Chen, L., et al. (2016). The human brainnetome atlas: a new brain atlas based on connectional architecture. Cereb. Cortex 26, 3508–3526. doi: 10.1093/cercor/bhw157
Fedorenko, E. (2014). The role of domain-general cognitive control in language comprehension. Front. Psychol. 5:335. doi: 10.3389/fpsyg.2014.00335
Fedorenko, E., and Blank, I. A. (2020). Broca's area is not a natural kind. Trends Cogn. Sci. 24, 270–284. doi: 10.1016/j.tics.2020.01.001
Fedorenko, E., Duncan, J., and Kanwisher, N. (2012). Language-selective and domain-general regions lie side by side within Broca's Area. Curr. Biol. 22, 2059–2062. doi: 10.1016/j.cub.2012.09.011
Fedorenko, E., Duncan, J., and Kanwisher, N. (2013). Broad domain generality in focal regions of frontal and parietal cortex. Proc. Nat. Acad. Sci. 110, 16616–16621. doi: 10.1073/pnas.1315235110
Fox, J., and Monette, G. (1992). Generalized collinearity diagnostics. J. Am. Stat. Assoc. 87, 178–183.
Friston, K. J., Ashburner, J., Kiebel, S. J., Nichols, T. E., and Penny, W. D. (2012). Statistical Parametric Mapping: The Analysis of Functional Brain Images (Version 12) [Computer software]. Wellcome Trust Centre for Neuroimaging, University College London. https://www.fil.ion.ucl.ac.uk/spm/
Gleichgerrcht, E., Fridriksson, J., Rorden, C., and Bonilha, L. (2017). Connectome-based lesion-symptom mapping (CLSM): a novel approach to map neurological function. Neuroimage Clin. 16, 461–467. doi: 10.1016/j.nicl.2017.08.018
Goghari, V. M., and MacDonald, A. W. (2009). The neural basis of cognitive control: response selection and inhibition. Brain Cogn. 71, 72–83. doi: 10.1016/j.bandc.2009.04.004
Gough, P. M., Nobre, A. C., and Devlin, J. T. (2005). Dissociating linguistic processes in the left inferior frontal cortex with transcranial magnetic stimulation. J. Neurosci. 25, 8010–8016. doi: 10.1523/JNEUROSCI.2307-05.2005
Grodzinsky, Y. (2000). The neurology of syntax: language use without Broca's area. Behav. Brain Sci. 23, 1–21; discussion 21–71. doi: 10.1017/S0140525X00002399
Harris, C. R., Millman, K. J., van der Walt, S. J., Gommers, R., Virtanen, P., Cournapeau, D., et al. (2020). Array programming with NumPy. Nature 585, 357–362. doi: 10.1038/s41586-020-2649-2
Hartsuiker, R. J., Pickering, M. J., and De Jong, N. H. (2005). Semantic and phonological context effects in speech error repair. J. Exp. Psychol. Learn. Mem. Cogn. 31, 921–932. doi: 10.1037/0278-7393.31.5.921
Harvey, D. Y., and Schnur, T. T. (2015). Distinct loci of lexical and semantic access deficits in aphasia: evidence from voxel-based lesion-symptom mapping and diffusion tensor imaging. Cortex 67, 37–58. doi: 10.1016/j.cortex.2015.03.004
Howard, D., and Patterson, K. (1992). Pyramids and Palm Trees: A Test of Semantic Access from Pictures and Words. Bury St. Edmunds: Thames Valley Test Company.
Hunter, J. D. (2007). Matplotlib: a 2D graphics environment. Comput. Sci. Eng. 9, 90–95. doi: 10.1109/MCSE.2007.55
Huttenlocher, J., and Kubicek, L. F. (1983). The source of relatedness effects on naming latency. J. Exp. Psychol. Learn. Mem. Cogn. 9, 486–496.
James, G., Witten, D., Hastie, T., and Tibshirani, R. (2013). An Introduction to Statistical Learning, Vol. 112. Berlin: Springer. doi: 10.1007/978-1-4614-7138-7
January, D., Trueswell, J. C., and Thompson-Schill, S. L. (2009). Co-localization of Stroop and syntactic ambiguity resolution in Broca's area: implications for the neural basis of sentence processing. J. Cogn. Neurosci. 21, 2434–2444. doi: 10.1162/jocn.2008.21179
Kaan, E., and Swaab, T. Y. (2002). The brain circuitry of syntactic comprehension. Trends Cogn. Sci. 6, 350–356. doi: 10.1016/S1364-6613(02)01947-2
Kan, I. P., Teubner-Rhodes, S., Drummey, A. B., Nutile, L., Krupa, L., Novick, J. M., et al. (2013). To adapt or not to adapt: the question of domain-general cognitive control. Cognition 129, 637–651. doi: 10.1016/j.cognition.2013.09.001
Kapur, S., Craik, F. I., Tulving, E., Wilson, A. A., Houle, S., Brown, G. M., et al. (1994). Neuroanatomical correlates of encoding in episodic memory: levels of processing effect. Proc. Nat. Acad. Sci. 91, 2008–2011. doi: 10.1073/pnas.91.6.2008
Klaus, J., and Hartwigsen, G. (2019). Dissociating semantic and phonological contributions of the left inferior frontal gyrus to language production. Hum. Brain Mapp. 40, 3279–3287. doi: 10.1002/hbm.24597
Levelt, W. J., Roelofs, A., and Meyer, A. S. (1999). A theory of lexical access in speech production. Behav. Brain Sci. 22, 1–38. doi: 10.1017/S0140525X99001776
Marian, V., Chabal, S., Bartolotti, J., Bradley, K., and Hernandez, A. E. (2014). Differential recruitment of executive control regions during phonological competition in monolinguals and bilinguals. Brain Lang. 139, 108–117. doi: 10.1016/j.bandl.2014.10.005
Martin, A., Haxby, J. V., Lalonde, F. M., Wiggs, C. L., and Ungerleider, L. G. (1995). Discrete cortical regions associated with knowledge of color and knowledge of action. Science 270, 102–105. doi: 10.1126/science.270.5233.102
Martin, N., Kohen, F., Kalinyak-Fliszar, M., Soveri, A., and Laine, M. (2012). Effects of working memory load on processing of sounds and meanings of words in aphasia. Aphasiology 26, 462–493. doi: 10.1080/02687038.2011.619516
Mathôt, S., Schreij, D., and Theeuwes, J. (2012). OpenSesame: an open-source, graphical experiment builder for the social sciences. Behav. Res. Methods 44, 314–324. doi: 10.3758/s13428-011-0168-7
McClelland, J. L., and Rumelhart, D. E. (1981). An interactive activation model of context effects in letter perception: I. An account of basic findings. Psychol. Rev. 88:375. doi: 10.1037//0033-295X.88.5.375
Meyer, A. S. (1991). The time course of phonological encoding in language production: phonological encoding inside a syllable. J. Mem. Lang. 30, 69–89. doi: 10.1016/0749-596X(91)90011-8
Miller, E. K. (2000). The prefontral cortex and cognitive control. Nat. Rev. Neurosci. 1:1. doi: 10.1038/35036228
Miller, E. K., and Cohen, J. D. (2001). An integrative theory of prefrontal cortex function. Annu. Rev. Neurosci. 24, 167–202. doi: 10.1146/annurev.neuro.24.1.167
Miyake, A., Friedman, N. P., Emerson, M. J., Witzki, A. H., Howerter, A., Wager, T. D., et al. (2000). The unity and diversity of executive functions and their contributions to complex “frontal lobe” tasks: a latent variable analysis. Cogn. Psychol. 41, 49–100. doi: 10.1006/cogp.1999.0734
Mohr, J. P., Pessin, M. S., Finkelstein, S., Funkenstein, H. H., Duncan, G. W., Davis, K. R., et al. (1978). Broca aphasia: pathologic and clinical. Neurology 28, 311–311. doi: 10.1212/WNL.28.4.311
Moss, H. E., Abdallah, S., Fletcher, P., Bright, P., Pilgrim, L., Acres, K., et al. (2005). Selecting among competing alternatives: selection and retrieval in the left inferior frontal gyrus. Cereb. Cortex 15, 1723–1735. doi: 10.1093/cercor/bhi049
Nachev, P., Coulthard, E., Jäger, H. R., Kennard, C., and Husain, M. (2008). Enantiomorphic normalization of focally lesioned brains. Neuroimage 39, 1215–1226. doi: 10.1016/j.neuroimage.2007.10.002
Nagel, I. E., Schumacher, E. H., Goebel, R., and D'Esposito, M. (2008). Functional MRI investigation of verbal selection mechanisms in lateral prefrontal cortex. Neuroimage 43, 801–807. doi: 10.1016/j.neuroimage.2008.07.017
Navarrete, E., Del Prato, P., and Mahon, B. Z. (2012). Factors determining semantic facilitation and interference in the cyclic naming paradigm. Front. Psychol. 3:38. doi: 10.3389/fpsyg.2012.00038
Noonan, K. A., Jefferies, E., Visser, M., and Lambon Ralph, M. A. (2013). Going beyond inferior prefrontal involvement in semantic control: evidence for the additional contribution of dorsal angular gyrus and posterior middle temporal cortex. J. Cogn. Neurosci. 25, 1824–1850. doi: 10.1162/jocn_a_00442
Novick, J. M., Hussey, E., Teubner-Rhodes, S., Harbison, J. I., and Bunting, M. F. (2014). Clearing the garden-path: improving sentence processing through cognitive control training. Lang. Cogn. Neurosci. 29, 186–217. doi: 10.1080/01690965.2012.758297
Novick, J. M., Trueswell, J. C., and Thompson-Schill, S. L. (2005). Cognitive control and parsing: reexamining the role of Broca's area in sentence comprehension. Cogn. Affect. Behav. Neurosci. 5, 263–281. doi: 10.3758/CABN.5.3.263
Novick, J. M., Trueswell, J. C., and Thompson-Schill, S. L. (2010). Broca's area and language processing: evidence for the cognitive control connection. Lang. Linguist. Compass 4, 906–924. doi: 10.1111/j.1749-818X.2010.00244.x
Nozari, N. (2019). The dual origin of semantic errors in access deficit: activation vs. inhibition deficit. Cogn. Neuropsychol. 36, 31–53. doi: 10.1080/02643294.2019.1587397
Nozari, N., Dell, G. S., and Schwartz, M. F. (2011). Is comprehension necessary for error detection? A conflict-based account of monitoring in speech production. Cogn. Psychol. 63, 1–33. doi: 10.1016/j.cogpsych.2011.05.001
Nozari, N., Freund, M., Breining, B., Rapp, B., and Gordon, B. (2016). Cognitive control during selection and repair in word production. Lang. Cogn. Neurosci. 31, 886–903. doi: 10.1080/23273798.2016.1157194
Nozari, N., and Hepner, C. R. (2019a). To select or to wait? Response to the commentaries. Cogn. Neuropsychol. 36, 226–233. doi: 10.1080/02643294.2019.1632280
Nozari, N., and Hepner, C. R. (2019b). To select or to wait? The importance of criterion setting in debates of competitive lexical selection. Cogn. Neuropsychol. 36, 193–207. doi: 10.1080/02643294.2018.1476335
Nozari, N., and Pinet, S. (2020). A critical review of the behavioral, neuroimaging, and electrophysiological studies of co-activation of representations during word production. J. Neurolinguistics 53:100875. doi: 10.1016/j.jneuroling.2019.100875
Obermeyer, J., Schlesinger, J., and Martin, N. (2020). Evaluating the contribution of executive functions to language tasks in cognitively demanding contexts. Am. J. Speech Lang. Pathol. 29, 463–473. doi: 10.1044/2019_AJSLP-CAC48-18-0216
Oppenheim, G., and Nozari, N. (2021). “Behavioral interference or facilitation does not distinguish between competitive and noncompetitive accounts of lexical selection in word production,” in 43rd Annual Conference of the Cognitive Science Society: Comparative Cognition: Animal Minds. eScholarship Publishing (University of California). doi: 10.31234/osf.io/rjezp
Oppenheim, G. M. (2024). Lexical selection in language production. Available online at: https://files.osf.io/v1/resources/k2s3p/providers/osfstorage/65a709aba246ff0d09dd3de9?action=downloadanddirectandversion=1 (accessed March 8, 2024).
Oppenheim, G. M., and Balatsou, E. (2019). Lexical competition on demand. Cogn. Neuropsychol. 36, 216–219. doi: 10.1080/02643294.2019.1580189
Oppenheim, G. M., Dell, G. S., and Schwartz, M. F. (2010). The dark side of incremental learning: a model of cumulative semantic interference during lexical access in speech production. Cognition 114, 227–252. doi: 10.1016/j.cognition.2009.09.007
Paap, K. R., and Sawi, O. (2016). The role of test-retest reliability in measuring individual and group differences in executive functioning. J. Neurosci. Methods 274, 81–93. doi: 10.1016/j.jneumeth.2016.10.002
Patra, A., Kirkwood, J., Middleton, E. L., and Thothathiri, M. (2023). Variation in how cognitive control modulates sentence processing. R. Soc. Open Sci. 10:211969. doi: 10.1098/rsos.211969
Paulesu, E., Frith, C. D., and Frackowiak, R. S. J. (1993). The neural correlates of the verbal component of working memory. Nature 362:6418. doi: 10.1038/362342a0
Price, A. R., Bonner, M. F., Peelle, J. E., and Grossman, M. (2015). Converging evidence for the neuroanatomic basis of combinatorial semantics in the angular gyrus. J. Neurosci. 35, 3276–3284. doi: 10.1523/JNEUROSCI.3446-14.2015
R Core Team (2013). R: A Language and Environment for Statistical Computing. R Foundation for Statistical Computing. Available online at: http://www.R-project.org/
Rapp, B., and Goldrick, M. (2000). Discreteness and interactivity in spoken word production. Psychol. Rev. 107, 460–499. doi: 10.1037/0033-295X.107.3.460
Riès, S. K., Karzmark, C. R., Navarrete, E., Knight, R. T., and Dronkers, N. F. (2015). Specifying the role of the left prefrontal cortex in word selection. Brain Lang. 149, 135–147. doi: 10.1016/j.bandl.2015.07.007
Roelofs, A. (1992). A spreading-activation theory of lemma retrieval in speaking. Cognition 42, 107–142. doi: 10.1016/0010-0277(92)90041-F
Roelofs, A. (2020). Self-monitoring in speaking: in defense of a comprehension-based account. J. Cogn. 3:18. doi: 10.5334/joc.61
Rorden, C., Bonilha, L., Fridriksson, J., Bender, B., and Karnath, H. O. (2012). Age-specific CT and MRI templates for spatial normalization. Neuroimage 61, 957–965. doi: 10.1016/j.neuroimage.2012.03.020
Schnur, T. T. (2014). The persistence of cumulative semantic interference during naming. J. Mem. Lang. 75, 27–44. doi: 10.1016/j.jml.2014.04.006
Schnur, T. T., Schwartz, M. F., Brecher, A., and Hodgson, C. (2006). Semantic interference during blocked-cyclic naming: evidence from aphasia. J. Mem. Lang. 54, 199–227. doi: 10.1016/j.jml.2005.10.002
Schnur, T. T., Schwartz, M. F., Kimberg, D. Y., Hirshorn, E., Coslett, H. B., Thompson-Schill, S. L., et al. (2009). Localizing interference during naming: convergent neuroimaging and neuropsychological evidence for the function of Broca's area. Proc. Nat. Acad. Sci. 106, 322–327. doi: 10.1073/pnas.0805874106
Shallice, T. (1988). From Neuropsychology to Mental Structure. Cambridge, MA: Cambridge University Press. doi: 10.1017/CBO9780511526817
Shao, Z., Meyer, A. S., and Roelofs, A. (2013). Selective and nonselective inhibition of competitors in picture naming. Mem. Cogn. 41, 1200–1211. doi: 10.3758/s13421-013-0332-7
Simon, J. R., and Rudell, A. P. (1967). Auditory SR compatibility: the effect of an irrelevant cue on information processing. J. Appl. Psychol. 51, 300–304. doi: 10.1037/h0020586
Sohn, M.-H., Goode, A., Stenger, V. A., Carter, C. S., and Anderson, J. R. (2003). Competition and representation during memory retrieval: roles of the prefrontal cortex and the posterior parietal cortex. Proc. Nat. Acad. Sci. 100, 7412–7417. doi: 10.1073/pnas.0832374100
Soveri, A., Lehtonen, M., Karlsson, L. C., Lukasik, K., Antfolk, J., Laine, M., et al. (2018). Test–retest reliability of five frequently used executive tasks in healthy adults. Appl. Neuropsychol. Adult 25, 155–165. doi: 10.1080/23279095.2016.1263795
Sperber, R. D., McCauley, C., Ragain, R. D., and Weil, C. M. (1979). Semantic priming effects on picture and word processing. Mem. Cogn. 7, 339–345. doi: 10.3758/BF03196937
Thompson-Schill, S. L., D'Esposito, M., Aguirre, G. K., and Farah, M. J. (1997). Role of left inferior prefrontal cortex in retrieval of semantic knowledge: a reevaluation. Proc. Nat. Acad. Sci. 94, 14792–14797. doi: 10.1073/pnas.94.26.14792
Thompson-Schill, S. L., Swick, D., Farah, M. J., D'Esposito, M., Kan, I. P., Knight, R. T., et al. (1998). Verb generation in patients with focal frontal lesions: a neuropsychological test of neuroimaging findings. Proc. Nat. Acad. Sci. 95, 15855–15860. doi: 10.1073/pnas.95.26.15855
Tremblay, P., and Small, S. (2011). Motor response selection in overt sentence production: a functional MRI study. Front. Psychol. 2:253. doi: 10.3389/fpsyg.2011.00253
Van Rossum, G., and Drake, F. L. (2003). Python Language Reference Manual. Network Theory United Kingdom.
Wang, M., Shao, Z., Chen, Y., and Schiller, N. O. (2018). Neural correlates of spoken word production in semantic and phonological blocked cyclic naming. Lang. Cogn. Neurosci. 33, 575–586. doi: 10.1080/23273798.2017.1395467
Whitney, C., Kirk, M., O'Sullivan, J., Lambon Ralph, M. A., and Jefferies, E. (2011). The neural organization of semantic control: TMS evidence for a distributed network in left inferior frontal and posterior middle temporal gyrus. Cereb. Cortex 21, 1066–1075. doi: 10.1093/cercor/bhq180
Ye, Z., and Zhou, X. (2009). Conflict control during sentence comprehension: fMRI evidence. Neuroimage 48, 280–290. doi: 10.1016/j.neuroimage.2009.06.032
Zatorre, R. J., Meyer, E., Gjedde, A., and Evans, A. C. (1996). PET studies of phonetic processing of speech: review, replication, and reanalysis. Cereb. Cortex 6, 21–30. doi: 10.1093/cercor/6.1.21
Keywords: Broca's area, cognitive control, written word production, domain-specificity, interference, facilitation, blocked cyclic naming, Simon task
Citation: Neophytou K, Wiley RW and Rapp B (2024) Cognitive control in written word production. Front. Lang. Sci. 3:1398125. doi: 10.3389/flang.2024.1398125
Received: 08 March 2024; Accepted: 03 May 2024;
Published: 27 May 2024.
Edited by:
Anais Llorens, Université de Paris, FranceReviewed by:
Susan E. Teubner-Rhodes, Auburn University, United StatesCopyright © 2024 Neophytou, Wiley and Rapp. This is an open-access article distributed under the terms of the Creative Commons Attribution License (CC BY). The use, distribution or reproduction in other forums is permitted, provided the original author(s) and the copyright owner(s) are credited and that the original publication in this journal is cited, in accordance with accepted academic practice. No use, distribution or reproduction is permitted which does not comply with these terms.
*Correspondence: Kyriaki Neophytou, a25lb3BoeTFAamh1LmVkdQ==
Disclaimer: All claims expressed in this article are solely those of the authors and do not necessarily represent those of their affiliated organizations, or those of the publisher, the editors and the reviewers. Any product that may be evaluated in this article or claim that may be made by its manufacturer is not guaranteed or endorsed by the publisher.
Research integrity at Frontiers
Learn more about the work of our research integrity team to safeguard the quality of each article we publish.