- Illinois Natural History Survey, Prairie Research Institute, University of Illinois at Urbana-Champaign, Champaign, IL, United States
The blooming of global trade and travel and the intensification of global changes over the past decades are thought to be important drivers of accelerated range expansions of vector-borne pathogens (VBPs), with the potential to cause severe disease outbreaks around the world. As a bridge between hosts and pathogens, mosquitoes play a central role in the transmission of VBPs. With modern oversea/air transportation facilitating the introduction of different mosquito species into novel regions, there is concern that this may escalate the introduction and subsequent spread of introduced VBPs in those regions. Despite these potential impacts, there is still a lack of comprehensive understanding of the ecology of invasive mosquitoes and the consequences they have for VBP introductions and transmission intensity. Here we review common introduction pathways, limiting factors for the establishment and spread of invasive mosquito species and explore their role in the transmission of VBPs in invaded regions. We also highlight the major challenges in invasive mosquito surveillance and control and identify key research questions for advancing future control strategies and practices. This body of knowledge may contribute to the prevention of mosquito introductions, as well as risk assessment and risk management of VBPs.
Introduction
Vector-borne pathogens (VBPs) are disease agents (e.g., viruses, parasites or bacteria) of human and animal illness transmitted by arthropod vectors including flies, fleas, midges, mites, ticks and most deadly, mosquitoes (1). Over the past decades, the emergence or re-emergence of VBPs has accelerated, posing a heavy burden on public health and biodiversity conservation (2–5). According to the World Health Organization, for example, the annual number of estimated cases of dengue and malaria were up to 390 and 249 million, respectively, accounting for a considerable portion of the global infectious disease burden (6, 7). Blooming global trade and travel has most likely facilitated the movement of VBPs via infected humans, livestock, or vectors. As a result, many VBPs are expanding their distribution ranges, some even breaking through the intercontinental barriers and threatening new regions remote from their native ranges (5). A notorious recent example likely involving infected travelers was Zika virus (8), whose endemic regions consist of Africa and with infections later sporadically occurring in Asia. Following introduction to Yap Island in the western Pacific in 2007 and then French Polynesia in the southern Pacific in 2013-2014, a large epidemic occurred in Brazil in 2015-2016 and the virus continued to spread through Latin America, the Caribbean, and parts of Europe (9, 10). In Brazil, studies have shown that subsequent lower levels of incidence following the large outbreaks were most likely caused by the build-up of population immunity (11). Although there was no major outbreak of Zika in the US, locally acquired cases were also reported in Southern Texas and Florida in 2016-2017 ( (12) and references therein). Another example is the transmission of West Nile virus (WNV). Originally discovered in Uganda in 1937, WNV gradually spread throughout Africa, Middle East and Europe over the course of 60 years, and it spread to the Western hemisphere starting from the outbreak of WNV infection in avian communities in New York 1999, followed by thousands of reported human cases, including hundreds of deaths in the US and Canada in 2002 and 2003 ( (13) and references therein). This is a prime example of an arbovirus that not only was introduced and led to major outbreaks, but then rapidly spread over the course of a 4-year period across the continental United States and has become established in local avian populations (14).
Given these examples of recent invasions of vector-borne pathogens, and the threat associated with potential novel invasive pathogens, understanding the drivers for local and regional expansion of VBPs is of top priority. Global trade and travel have been suggested as the main driver for the introduction of VBPs into novel regions while land use and social changes (e.g., poverty) are more likely drivers of local expansion of already-circulating VBPs (5). In addition, global climate change may also contribute to the range expansion or shifts of VBPs if increasing temperatures enable certain vectors to expand their distribution range by latitude or elevation (15). In this context, the introduction of VBPs in novel regions can be facilitated by modern transportation carrying infected hosts and/or vectors, while the circulation of both novel and endemic VBPs relies on host and vector population dynamics that are regulated by environmental changes (Figure 1). Ultimately, the transmission of VBPs from an infected host to a new one depends on the blood-sucking behavior of vectors, and mosquitoes serve as primary vectors of many VBPs, such as avian Plasmodium and WNV (16, 17). Some VBPs circulate between humans, but others mainly infect animals, with humans and livestock acting as incidental hosts.
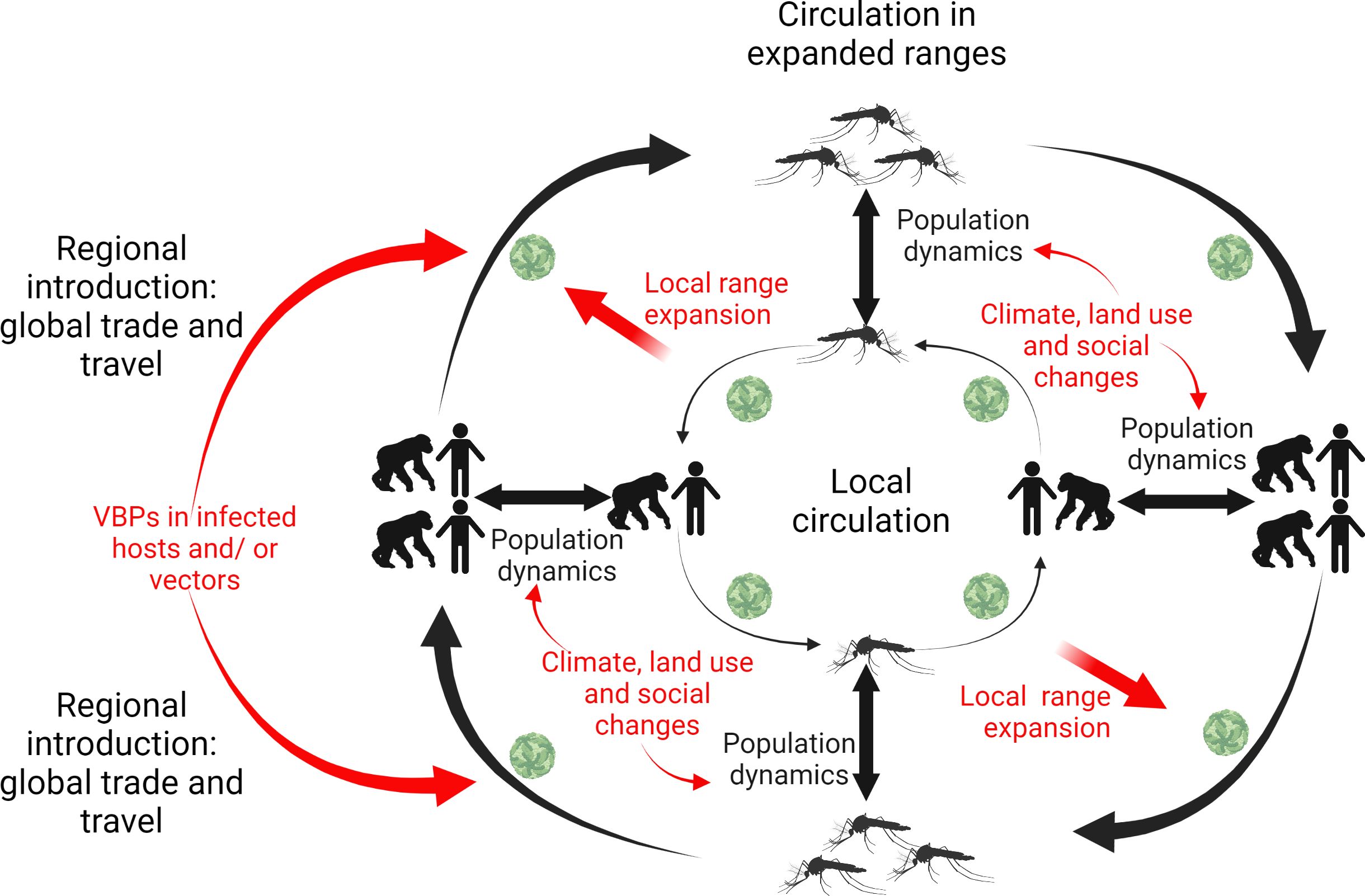
Figure 1. Drivers for the introduction and expansion of vector-borne pathogens (VBPs) at local and regional scales.
As a bridge between VBPs and their vertebrate hosts, mosquitoes play a central role in the transmission of VBPs. And those invasive mosquitoes become a research focus because they have the potential to become competent vectors of both novel and endemic VBPs and hence, significantly contribute to the expansion of VBPs. This review will focus on invasive mosquitoes, their introduction pathways, and the ecological factors that limit their establishment and spread. It will also examine the role these mosquitoes play in the transmission of VBPs during their introduction, establishment and spread, with particular attention to how the addition of an invasive mosquito can alter transmission intensity or pathways.
Invasive mosquito species
A common definition of invasive alien species are animals, plants or microorganisms that are introduced to a non-native ecosystem as a direct or indirect consequence of human actions, where they cause harm by threatening economic development, public health, food security or biodiversity (18–20). A number of mosquitoes that were introduced to new regions meet this definition and can be defined as invasive mosquitoes. For example, the southern house mosquito Culex quinquefasciatus, which is a native species of Africa, has been introduced to most of the world (see Table 1). The introduction of this species along with pathogens (such as avian malaria and poxvirus) they are able to spread to Hawaii has been regarded as the major driver for the decline and extinction of native avifauna (71). Anopheles stephensi, another invasive species whose native regions are Southern Asia and Arabian Peninsula, has been introduced to the Horn of Africa in recent years and has been tied to an increase in malaria cases in Djibouti following its introduction in 2012 (64). There is considerable concern about the range expansion of this malaria vector that relate to how its presence could alter the previously established transmission patterns. Particularly, this species is well adapted to urban environments and uses man-made containers as oviposition and larval development sites. This differs from the primary sub-Saharan African malaria vectors that tend to be associated with ephemeral larval habitats in rural environments. There is thus concern that the spread of this invasive mosquito could lead to a surge in urban malaria in the African continent. Further, it has been found that due to the use of man-made containers, this species appears to be capable of persisting and remaining active and abundant throughout the dry season, which poses the threat of year-round malaria (72). Its role in spreading drug-resistant malaria has recently been documented (73). Due to these threats, an initiative to mitigate the further spread of this vector has been initiated by the World Health Organization (74). We provide a table to summarize the native and invaded regions, primary hosts and vectors as well as the recent outbreak of VBPs associated with several major invasive mosquitoes from the genus of Aedes, Culex and Anopheles in Table 1.
Introduction pathways of invasive mosquitoes
The record of mosquito invasion dates back to the 16th century, when Ae. aegypti hitchhiked in the bilges of slave ships or in casks of drinking water from West Africa to the Americas (75, 76). Transoceanic ships were the major introduction pathway of invasive mosquitoes at that time. With the growth and development of the global economy and international transportation networks, modern trade and carriers have grown in importance as unintended pathways for mosquito invasion in new regions and even new continents. These pathways include two major trades via sea transportation (trades of used tires and Lucky bamboo), as well as air and ground transportation ((77) and references therein). In addition, natural dispersal of mosquitoes across terrestrial borders of adjacent countries may also be involved in range expansions (78).
Trade of used tires
The global trade of used tires has been considered as a major introduction pathway of invasive mosquitoes, especially container-inhabiting Aedes species. Used tires that contain stagnant water and organic matter (e.g., rotten leaves) can serve as ideal incubators for container-breeding mosquitoes like Aedes aegypti and Ae. albopictus. Juliano & Lounibos (21) found that mosquito species that produced desiccation-resistant eggs were more likely to become established in non-native environments than species that did not. This is a likely reason for the successful transport of viable eggs through the used tire trade for such species. It has been found that the darker the egg color of mosquito species is as a result of increased eggshell melanization, the more resistant to desiccation, which enables their eggs to survive for several months outside water (79). In the United States, the first established population of Ae. albopictus was documented in Houston, Texas in 1985, thought to be introduced from its native range in Asia via the transportation of used tires (80). Since then, this invasive species has continued to spread throughout the southern and eastern United States, with 40 states reporting occurrence of this species by 2016 (81). In Europe, trade and transportation of used tires has also been recognized as the pathway for the introduction of Ae. albopictus in Italy (82), France (83), Croatia (84), Belgium (85), the Netherlands (86), and Portugal (87). Similarly, Ae. japonicus and Ae. atropalpus were also introduced in France, Belgium, The Netherlands and Italy through the import of used tires ( (77) and references therein).
Trade of Lucky bamboo (Dracaena sp)
Lucky bamboo, an ornamental plant species, usually contains 5-8 cm of water during long-distance transport and can serve as a suitable development site for mosquito eggs and larvae. The importation of Lucky bamboo into Europe and the United States has caused repeated introductions of Ae. albopictus from its native range (88, 89). For example, the introductions of Ae. albopictus into southern California in 2001 and 2002 and the Netherlands in 2013 were reportedly associated with the shipment of Lucky bamboo from southern China (77, 90, 91).
Airplanes
Modern air transportation provides the potential of global transit in a single day for goods and passengers, possibly on some occasions including hitchhiking mosquitoes. Although no direct evidence for the introduction of invasive mosquitoes by air transport has been reported in any continent so far, airplanes have been identified as a highly likely pathway for the introduction of invasive mosquitoes in islands (92), and the recent establishment of Cx. quinquefasciatus in the Galápagos Islands was demonstrated to be ascribed to the regular air transport of mosquitoes from Ecuador (93). Similarly, 14 non-indigenous mosquito species were introduced to Guam following the second World War and this has been attributed to increased air traffic to the island (94). Further, the presence of invasive mosquitoes including Ae. aegypti, Ae. albopictus and Ae. koreicus at European airports have been reported (95, 96), and detections of Ae. aegypti at Australian airports have become increasingly common over recent years (97), indicating air transport of invasive mosquitoes is occurring and may prove a concern and/or require risk-based surveillance in more areas in the future (98, 99).
Ground vehicles
The initial detection of Ae. japonicus in northern Germany was in towns adjacent to a highway, suggesting that this species was likely introduced by ground vehicles (100). Although direct evidence is rare, it has been demonstrated that mosquitoes can hitchhike with ground vehicles to spread to new areas (101). Egizi et al (102) showed a correlation between genetic distance and distance along roads for Ae. japonicus in the northeastern United States, suggesting their local dispersal was related to road transportation. An analysis of gene flow for Ae. albopictus likewise pointed to the importance of highways and human-aided transport for long-distance dispersal of this species (103). Other recent studies reported that the introduction of Ae. albopictus into European countries, such as Germany and Spain, has been facilitated by ground vehicles (101, 104). Therefore, ground vehicles may play an important role in the introduction of invasive mosquitoes, especially for a short-distance introduction. Railway transportation has been linked to the movement of mosquitoes, particularly in the first half of the 20th century, including observations of Ae. notoscriptus and Ae. aegypti larvae breeding in fire buckets in railway stations, which were common at stations when steam locomotives were used, as well as frequent reports of passengers being bitten on trains (105).
Natural dispersal
Although the flight capacity for mosquitoes is very limited (106), natural active dispersal of invasive mosquitoes has been documented in some European countries (77). For example, the pathways for the introduction of Ae. japonicus from Austria to Italy and from Germany to Luxemburg have been identified as natural dispersal (78, 107). This pathway could be used by migratory mosquitoes to cross the borders of adjacent countries and over time allow them to expand their range. However, recent studies have also reported long-distance mosquito migration facilitated by wind, including the successful introduction of significant vector species (108, 109). For instance, the introduction of An. stephensi to Africa may have been driven by windborne dispersal over long distances (110).
Limiting factors for the invasion of mosquitoes
Temperature
Following introduction of propagules into a new environment, whether an invasive species will be able to establish and spread will determine on the suitability of the habitat in the new region. As such, temperature can be a major factor limiting the establishment and spread of invasive mosquitoes (111), and result in differences in distribution between invasive species, such as is seen for Ae. albopictus and Ae. japonicus in Europe (112). Low temperatures affect the survival of overwintering mosquito life stages and have been used to predict the northern distribution limits of invasive mosquitoes by comparison to the native range (113). Overwinter survival in species such as Ae. albopictus depends on photoperiod-induced egg diapause and cold tolerance of eggs, and species possessing these characteristics are therefore more likely to expand their range to temperate areas (114, 115). For example, Hawley et al (80) demonstrated that the eggs of invasive Ae. albopictus in the US had similar photoperiodic sensitivity and cold hardiness with those in their native range Northern Asia. These characteristics have been considered as an adaptation to cold environments in their native range, which may have laid the foundation for its invasion success in the temperate USA (114). In addition, this adaptation continues to evolve rapidly and divergently in the invaded ranges, with populations in the south reducing their diapause response, while populations at the northern range edge expressing enhanced diapause response, which may enable a continuing northward expansion (115–117).
Dryness
Dryness is another important climatic factor that affects the survival of eggs and hence, the invasion success of mosquitoes. Juliano and Lounibos (21) demonstrated that nearly half of invasive or non-native mosquito species have desiccation-resistant eggs and that this characteristic was strongly associated with becoming an introduced alien species. However, desiccation resistance was not significantly related to invasive status (i.e., invasive or non-invasive). This may indicate that dryness may strongly affect the establishment of an introduced mosquitoes, but other factors may play a greater role in limiting the spread of introduced mosquitoes (i.e., becoming invasive). The combination of desiccation tolerance and temperature can also affect the establishment of invasive species. For instance, in Florida Ae. albopictus had greater occupancy in cooler sites without a significant dry season, while Ae, aegypti showed greater occupancy in hotter sites with a dry season, highlighting that these factors jointly can affect the establishment and coexistence of invasive species (118).
Microhabitats
The presence of suitable microhabitats is important for the establishment of introduced mosquitoes. One way in which this can matter is if suitable microhabitats represent an ‘empty niche’ that can be filled by an introduced mosquito species, which could result in establishment while avoiding or limiting the extent of interspecific competition with native species. Many invasive and non-native mosquito species are able to develop in small natural and artificial containers (e.g., tree holes, man-made containers), which may indicate containers as a key for the successful introduction of non-native mosquitoes. However, the establishment and spread of introduced species was not significantly associated to their specific larval habitat types (i.e., container vs. non-container), rather success of invasive species was linked to their adaptation to non-natural microhabitats in urban, suburban, and domestic areas (21). This may indicate that human disturbance plays a role in the spread of introduced mosquitoes, e.g., by creating peridomestic underexploited habitats, or because invasion pathways for mosquitoes favor urbanized areas as a consequence of the associations with transportation nodes, and thereby anthropogenic environmental change could contribute to their invasiveness.
Interspecific competition
Following the introduction into new regions, interspecific competition between introduced and native species can take place if they occupy overlapping habitats. Interspecific competition can affect mosquito population dynamics through the negative effects of resource competition and mating interference and play a role in determining the outcome of an introduction (119). If introduced species are superior competitors, they may exclude resident species and become invasive in the new areas. On the contrary, an introduced species may not spread out because of the stronger competition from residents and become non-native in a limited range. Both lab and field experiments often showed that Ae. albopictus was superior in competition to Ae. aegypti (120, 121), as a result, the distribution of Ae. aegypti has declined with the expansion of Ae. albopictus in the continental US, whereas in certain tropical cities exclusion of Ae. albopictus by Ae. aegypti has been noted (119).
Predation and parasitism
Predation can have direct effect on population dynamics of introduced mosquitoes. Predators in the new areas for introduced mosquito species may help keep them from spreading out and becoming invasive. On the contrary, the absence of predators in the new areas for the introduced mosquitoes may facilitate the establishment and spread of the introduced species. This enemy release hypothesis while sometimes supported, is also frequently questioned (122). The extent to which it applies for different mosquito species is not entirely clear, but there are examples of both predation and parasitism affecting invasive mosquitoes. For example, the presence of Toxorhynchites rutilus, an efficient predator of Ae. aegypti, may have limited the invasion success of Ae. aegypti in wooded areas (123), while the absence of predators and competitors of Cx. quinquefasciatus in artificial container habitats have contributed to the invasion success of this species (124). Even when there are multiple exotic species coexisting, predation can severely lower their performance, especially the performance of the species that are less competitive, thus forming barriers for the spread of exotic species (125). Parasitism may have a similar effect on invasive species through pathogenic effects on development and performance (126). Parasitism by Ascogregarina taiwanensis, for example, has been demonstrated to reduce the competitive advantage of Ae. albopictus over a native species, Ae. triseriatus, which was thought to limit the establishment and spread of this invasive species (127, 128). However, such pathogenic effects are usually mild, depending on environmental conditions (126).
Potential roles in the transmission of VBPs
Invasive mosquitoes may pose a great threat to wildlife and human health, for instance through the introduction of invasive mosquitoes that are capable of transmitting exotic pathogens to native host populations lacking herd immunity. The introduction of invasive mosquitoes may play varying roles in the transmission of vector-borne pathogens, depending on their competence for transmitting native or novel pathogens and the ways in which their introduction shifts vectorial capacity of the local mosquito community for different pathogens (see Box 1 for an example). Some mosquito vectors and pathogens may be associated in their native ranges but are introduced separately into new regions, which is considered as the most common cause of disease outbreak in the invaded regions (21). In other cases, some mosquito vectors and pathogens are introduced simultaneously into new regions, or introduced mosquitoes are already capable of transmitting endemic pathogens.
Box 1. Role of Ae. japonicus japonicus as a disease vector in its native and invaded regions
The Asian bush or rock pool mosquito, Ae. japonicus japonicus (Theobald, 1901), originating from East Asia (northeastern Russian, Japan, Korea, Southern China), is a highly invasive species, which has spread throughout North American and later into Central Europe (32). Like the introduction of other invasive Aedes mosquitoes, transportation in used tires via international trade is presumably a major pathway for its invasion into novel regions. In 1993, the first record for its non-native range was reported in New Zealand, with larvae found in the used tires imported from Japan, although this introduction did not result in an established population (129, 130). In 1998, introduction of this species was first documented in Connecticut and then New York and New Jersey (131). Since then, Ae. japonicus japonicus has successfully established and was found in 33 states of the US by 2011 and southwestern Canada (31, 132). In 2000, this invasive species was first detected in France after which it spread to several central European countries including Belgium, Switzerland, Germany, Liechtenstein, Austria, The Netherlands, Croatia and Slovenia over the next decade (133).
In its native range, Ae. japonicus japonicus is not considered as an important disease vector (31). Only three arboviruses were tested on Ae. japonicus japonicus for its vectorial potential (Table 2). Japanese and Russian researchers demonstrated that this species was able to get infected both in the laboratory and field and transmit JEV in the laboratory (134, 135). It was also susceptible to ZIKV and GETV infection in the laboratory (147, 148). In its invaded areas, a number of arboviruses have been tested on Ae. japonicus japonicus (Table 2). It was susceptible to JEV, CHIKV and DENV infection in the laboratory, both infection and transmission in the laboratory for SLEV, EEEV, RVFV, UVSV, ZIKV, and infection and transmission in the laboratory and field infection for WNV and LACV (Table 2). However, no evidence from both its native and invaded regions has shown that it plays a major role in the field transmission of disease agents. Compared to its invaded regions, few experimental infections by arboviruses have been tested on Ae. japonicus japonicus in its native regions. As Ae. japonicus japonicus feeds on a variety of hosts and is capable of vectoring and/or transmitting an array of pathogens, it can be a potentially important bridge vector threatening humans, livestock and wildlife, especially in invaded novel regions where its enemies and competitors from its native region may be absent and it can pick up novel pathogens.
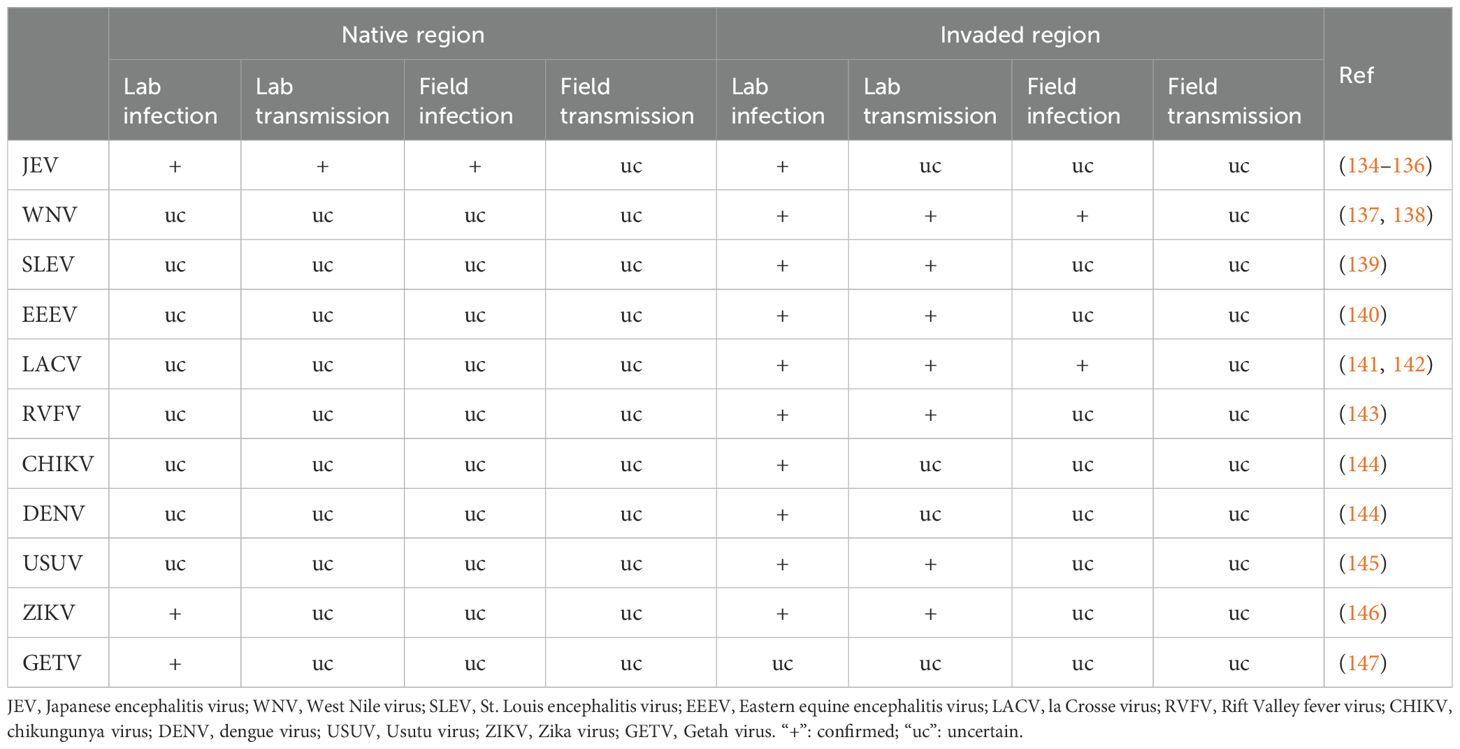
Table 2. Demonstrated role of Aedes japonicus japonicus in arbovirus transmission in native and invaded regions.
Separate introductions of novel vector and pathogens are likely to occur in introduction events as a consequence of mosquitoes and pathogens having different introduction pathways. For instance, the propagule pressure for mosquitoes could be greatest due to long distance travel of containers, and uninfected mosquitoes (e.g., transported in the egg stage) will arrive in a much greater volume than infected mosquitoes. At the same time, the pathogen might be introduced much less frequently through movement of the vector, compared to the movements of infected hosts such as humans, livestock, or migratory birds (5). Following establishment, introduced mosquitoes may acquire an association with separately introduced pathogens and create a new threat to susceptible hosts. For instance, the separate introductions of Cx. quinquefasciatus and avian Plasmodium in Hawaii have caused the outbreak of avian malaria and the decline of Hawaiian birds, particularly in those avian fauna at low elevations (149). Another example is the separate introduction of Culex pipiens complex and West Nile virus in North America where the pathogen was introduced long after the mosquito (150).
Simultaneous introductions of novel vectors and pathogens likely occurs much more rarely. However, it may still have the potential to cause large outbreaks of diseases, given native host populations are relatively naïve to novel pathogens. For example, the historical introductions of Ae. aegypti in North and South Americas have caused immediate outbreak of yellow fever and dengue, respectively, upon the arrival of slave ships from Africa that were presumably carrying yellow fever and dengue virus that were novel to these regions at that time (21).
Invasive mosquitoes can also be competent for existing native pathogens and modify local transmission cycles of vector-borne diseases. If the introduced vectors are highly competent, the disease transmission rates and risk to public health could be enhanced. But if the introduced vectors are less efficient or through competitive interactions lower the population densities or exclude existing primary vectors, the disease transmission rates could decrease. An example of this is Ae. aegypti which is an invasive species in Asia, originating in sub-Saharan Africa, and becomes a major vector of dengue in tropical Asia. Although it is possible that dengue originated in Africa, there is compelling evidence that dengue virus is native to Asia (151).
The introduction of invasive mosquitoes into local mosquito communities can significantly impact the transmission risk (e.g., potential exposure for humans) and intensity (e.g., the basic reproduction number of the pathogen) of VBPs in their invaded areas. Invasive species may compete with native mosquitoes for ecological niches, such as breeding sites and nectar, and disrupt the existing host-vector interaction networks. These changes can lead to alterations in native mosquito population densities and modify the rates at which pathogens are transmitted to native hosts and vectors. For instance, Box 2 provides a case study on how the invasive species Ae. albopictus and Ae. j. japonicus can alter the host-vector interaction network of the native species Ae. triseriatus in North America.
Box 2. Invasive mosquitoes alternative host-vector network in North America
The introduction of an invasive mosquito species can change transmission risk or intensity in a number of ways. Density-dependent competition can, as described above, lead to changes in the mosquito community with possible exclusion of certain native species, while if the niche differences are sufficiently large, coexistence can also occur. The exact outcome in terms of population densities of the various vector species relevant for the pathosystem in question will determine to a large extent what will occur. In the case of coexistence, the addition of another species to an assemblage of vectors can affect transmission in a number of ways. Increased vector diversity could take the form of temporal separation, and a result of this could potentially be that the effective transmission season of a given pathogen becomes extended (152). Another way in which transmission risk and intensity could shift with the addition of an invasive vector is through changes to the host-vector network. With different species having different preferences for host species, and different rates of blood feeding on those vertebrate hosts, it is possible that pathogens associated with a host that was initially not connected to other amplifying hosts or humans, now becomes so due to an invasive species. An example of such a network is provide in Figure 2.
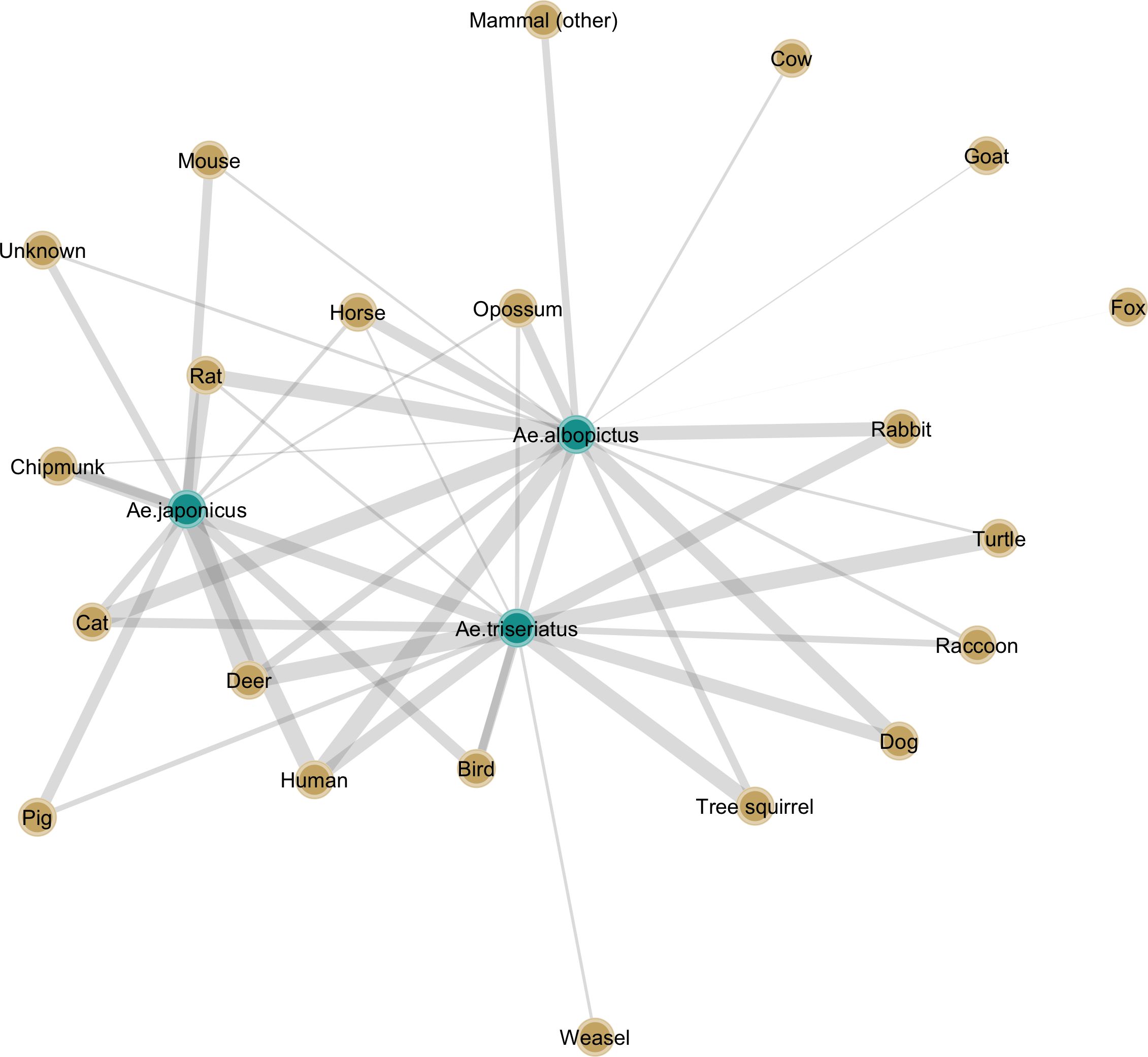
Figure 2. Bipartite graph highlighting the interactions between a native mosquito, Ae. triseriatus, and two invasive species, Ae. albopictus and Ae. j. japonicus, and vertebrate hosts used as blood meal sources. The connections between each vector and host species are weighted and represent average proportions of identified blood meals collated from studies in North America (153–167).
We reviewed the literature for studies in North America that investigated the blood feeding patterns of a native mosquito, Ae. triseriatus, considered the primary vector of La Crosse encephalitis virus, and two invasive species, Ae. albopictus and Ae. j. japonicus, both of which are competent vectors under laboratory conditions (168) and have been found to be infected in the field with this virus as well (169, 170) In areas where these three species co-occur, ignoring possible effects they may exert on each other’s population density, the range of host species fed upon is considerably more diverse than in areas with only the native species. In the case of LACV, where the primary hosts are considered chipmunks and squirrels, the invasive species could potentially become exposed. The role of rabbits is less clear, although there have been suggestions, they may play a role as well (157). The larger impact of these invasive species however could be that rather than increasing R0 in a meaningful way for a virus like LACV, they could however act as additional bridge vectors and increase the rate with which humans are bitten by infective mosquitoes. Theoretical models exploring these virus-vector-host networks, density-dependent interactions among vectors, and seasonal overlap, could shed light on exactly how VBP transmission would be altered by the introduction of one or more invasive species.
Challenges and future directions
Current surveillance and control programs for invasive mosquitoes and vector-borne pathogens (VBPs) have shown varying degrees of effectiveness across different regions (171, 172). This variability is influenced by key factors such as the scope and enforcement of national and intergovernmental regulatory policies enacted for preventing the introduction, establishment, and spread of alien species, public investment in vector control and surveillance capacity, as well as efforts to reduce the vectorial capacities of invasive mosquitoes. For instance, the increased movement of goods and tourists poses significant challenges for controlling the entry of alien mosquitoes and VBPs at points of entry (5). Integrated Vector Management (IVM) remains the most effective strategy for mosquito control (173). IVM combines chemical, biological, and physical control methods and typically requires the use of insecticides and significant resources, including trained technicians. However, resource limitations, environmental concerns, and the development of insecticide resistance can hinder the effectiveness of IVM (174). Additionally, the ecological mechanisms underlying the establishment and spread of invasive mosquitoes in new regions remain largely unknown (54), making ecological control a significant challenge.
These challenges underscore the need to develop and implement newer, safer, more effective, and sustainable tools for invasive mosquito control. To address these challenges and the process of mosquito invasion, we propose several priority research questions for future study and control of invasive mosquitoes and VBPs: 1) Integrating advanced techniques (e.g., artificial intelligence (AI), quantitative risk assessments) for predicting future invasion risks, identifying high risk routes of entry, and enhancing the detection sensitivity and control of imported mosquito adults and eggs at entry points; 2) innovating surveillance techniques (e.g., high-throughput molecular tools and rapid detection kits) for mosquito and VBP identification and screening; 3) unravelling the ecological mechanisms behind the success of invasive mosquitoes in invaded regions, from establishment to spread, particularly their interactions with native mosquito species, vertebrate hosts, and how they change vector-host-pathogen networks; 4) Incorporating advanced tools such as AI, sterile insect techniques, and gene drive into IVM to effectively monitor, suppress, or replace invasive mosquito populations and reduce their vectorial capacities; 5) Enhancing control options for invasive mosquitoes by exploiting a wider range of their life cycle and behaviors, such as novel baitstations based on plant-derived volatiles; 6) Enhancing community engagement and education, as well as public integration in mosquito surveillance and control programs, such as through citizen science initiatives aimed at early detection of novel vector introduction and spread.
Conclusions
Mosquitoes can be introduced into novel regions by the transportations of used tires and lucky bamboo in global trade and by hitchhiking airplanes and ground vehicles in global travel. Local ecological factors including temperature, dryness, competition, predation, and parasitism may limit the establishment and spread of the introduced mosquitoes in novel regions. Invasive mosquitoes can play a critical role in the transmission of VBPs in their introduced regions, depending on whether they are introduced separately or simultaneously with already vectoring VBPs, or whether they can acquire the competence for novel pathogens in the invaded regions over generations. Management and control effort should be targeted at cutting off their introduction pathways as the frontline, limiting their establishment and spread using ecological measures and reducing their contact with local hosts and pathogens.
Author contributions
JY: Conceptualization, Data curation, Investigation, Validation, Visualization, Writing – original draft, Writing – review & editing. AM: Data curation, Validation, Writing – review & editing. CS: Conceptualization, Funding acquisition, Visualization, Writing – original draft, Writing – review & editing.
Funding
The author(s) declare that financial support was received for the research, authorship, and/or publication of this article. This work was supported by the State of Illinois Used Tire Management and Emergency Public Health funds.
Conflict of interest
The authors declare that the research was conducted in the absence of any commercial or financial relationships that could be construed as a potential conflict of interest.
Generative AI statement
The author(s) declare that no Generative AI was used in the creation of this manuscript.
Publisher’s note
All claims expressed in this article are solely those of the authors and do not necessarily represent those of their affiliated organizations, or those of the publisher, the editors and the reviewers. Any product that may be evaluated in this article, or claim that may be made by its manufacturer, is not guaranteed or endorsed by the publisher.
References
1. Little SE. “Vector-Borne Diseases.,” In: Bowman DD, editor. Georgis’ Parasitology for Veterinarians-E-Book. Elsevier Health Sciences (2014).
2. Daszak P, Cunningham AA, Hyatt AD. Emerging infectious diseases of wildlife–threats to biodiversity and human health. Science. (2000) 287:443. doi: 10.1126/science.287.5452.443
3. Harrus S, Baneth G. Drivers for the emergence and re-emergence of vector-borne protozoal and bacterial diseases. Int J Parasitol. (2005) 35:1309–18. doi: 10.1016/j.ijpara.2005.06.005
4. Jones KE, Patel NG, Levy MA, Storeygard A, Balk D, Gittleman JL, et al. Global trends in emerging infectious diseases. Nature. (2008) 451:990–3. doi: 10.1038/nature06536
5. Kilpatrick AM, Randolph SE. Drivers, dynamics, and control of emerging vector-borne zoonotic diseases. Lancet. (2012) 380:1946–55. doi: 10.1016/S0140-6736(12)61151-9
6. World Health Organization. Dengue and Severe Dengue (2024). Available online at: https://www.who.int/news-room/fact-sheets/detail/dengue-and-severe-dengue (accessed August 15, 2024).
7. World Health Organization. Malaria (2023). Available online at: https://www.who.int/news-room/fact-sheets/detail/malaria (accessed August 15, 2024).
8. Grubaugh ND, Saraf S, Gangavarapu K, Watts A, Tan AL, Oidtman RJ, et al. Travel surveillance and genomics uncover a hidden zika outbreak during the waning epidemic. Cell. (2019) 178:1057–71.e11. doi: 10.1016/j.cell.2019.07.018
9. Baud D, Gubler DJ, Schaub B, Lanteri MC, Musso D. An update on zika virus infection. Lancet. (2017) 390:2099–109. doi: 10.1016/S0140-6736(17)31450-2
10. Brady OJ, Hay SI. The first local cases of zika virus in Europe. Lancet. (2019) 394:1991–2. doi: 10.1016/S0140-6736(19)32790-4
11. Netto EM, Moreira-Soto A, Pedroso C, Höser C, Funk S, Kucharski AJ, et al. High zika virus seroprevalence in salvador, northeastern Brazil limits the potential for further outbreaks. MBio. (2017) 8:e01390–17. doi: 10.1128/mbio.01390-17
12. Castro LA, Fox SJ, Chen X, Liu K, Bellan SE, Dimitrov NB, et al. Assessing real-time zika risk in the United States. BMC Infect Dis. (2017) 17:1–9. doi: 10.1186/s12879-017-2394-9
13. Ronca SE, Ruff JC, Murray KO. A 20-year historical review of west Nile virus since its initial emergence in North America: has west Nile virus become a neglected tropical disease? PloS Negl Trop Dis. (2021) 15:e0009190. doi: 10.1371/journal.pntd.0009190
14. Petersen LR, Hayes EB. West Nile virus in the Americas. Med Clinics North America. (2008) 92:1307–22. doi: 10.1016/j.mcna.2008.07.004
15. Iwamura T, Guzman-Holst A, Murray KA. Accelerating Invasion Potential of Disease Vector Aedes aEgypti under Climate Change. Nat Commun. (2020) 11:1–10. doi: 10.1038/s41467-020-16010-4
16. Farajollahi A, Fonseca DM, Kramer LD, Marm Kilpatrick A. Bird biting” Mosquitoes and human disease: A review of the role of culex pipiens complex mosquitoes in epidemiology. Infect Genet Evol. (2011) 11:1577–85. doi: 10.1016/j.meegid.2011.08.013
17. Foster WA, Walker ED. Mosquitoes (Culicidae). Med Vet Entomol. (2019), 261–325. doi: 10.1016/B978-0-12-814043-7.00015-7
18. Early R, Bradley BA, Dukes JS, Lawler JJ, Olden JD, Blumenthal DM, et al. Global threats from invasive alien species in the twenty-first century and national response capacities. Nat Commun. (2016) 7:12485. doi: 10.1038/ncomms12485
19. Mazza G, Tricarico E. Invasive species and human health: CAB International (2018). doi: 10.1079/9781786390981.0000
20. Pyšek P, Hulme PE, Simberloff D, Bacher S, Blackburn TM, Carlton JT, et al. Scientists’ Warning on invasive alien species. Biol Rev. (2020) 95:1511–34. doi: 10.1111/brv.12627
21. Juliano SA, Philip Lounibos L. Ecology of invasive mosquitoes: effects on resident species and on human health. Ecol Lett. (2005) 8:558–74. doi: 10.1111/j.1461-0248.2005.00755.x
22. Bonizzoni M, Gasperi G, Chen X, James AA. The invasive mosquito species aedes albopictus: current knowledge and future perspectives. Trends Parasitol. (2013) 29:460–8. doi: 10.1016/j.pt.2013.07.003
23. van den Hurk AF, Nicholson J, Beebe NW, Davis J, Muzari OM, Russell RC, et al. Ten Years of the Tiger: Aedes albopictus Presence in Australia since Its Discovery in the Torres Strait in 2005. One Health. (2016) 2:19–24. doi: 10.1016/j.onehlt.2016.02.001
24. Medlock J, Hansford K, Versteirt V, Cull B, Kampen H, Fontenille D, et al. An entomological review of invasive mosquitoes in Europe. Bull Entomol Res. (2015) 105:637. doi: 10.1017/S0007485315000103
25. Berry IM, Eyase F, Pollett S, Konongoi SL, Joyce MG, Figueroa K, et al. Global outbreaks and origins of a chikungunya virus variant carrying mutations which may increase fitness for aedes aEgypti: revelations from the 2016 Mandera, Kenya outbreak. Am J Trop Med Hyg. (2019) 100:1249. doi: 10.4269/ajtmh.18-0980
26. Brady OJ, Hay SI. The global expansion of dengue: how aedes aEgypti mosquitoes enabled the first pandemic arbovirus. Annu Rev Entomol. (2020) 65:191–208. doi: 10.1146/annurev-ento-011019-024918
27. Hsan K, Hossain MM, Sarwar MS, Wilder-Smith A, Gozal D. Unprecedented rise in dengue outbreaks in Bangladesh. Lancet Infect Dis. (2019) 19:1287. doi: 10.1016/S1473-3099(19)30616-4
28. Okada K, Morita R, Egawa K, Hirai Y, Kaida A, Shirano M, et al. Dengue virus type 1 infection in traveler returning from Tanzania to Japan, 2019. Emerg Infect Dis. (2019) 25:1782. doi: 10.3201/eid2509.190814
29. Jaenisch T, Junghanss T, Wills B, Brady OJ, Eckerle I, Farlow A, et al. Dengue expansion in Africa—Not recognized or not happening? Emerg Infect Dis. (2014) 20:e140487. doi: 10.3201/eid2010.140487
30. Situation Report No 18 - Dengue Epidemiological Situation in the Region of the Americas - Epidemiological Week 18, 2024. Pan American Health Organization (2024).
31. Schaffner F, Medlock J, Van Bortel W. Public health significance of invasive mosquitoes in Europe. Clin Microbiol Infect. (2013) 19:685–92. doi: 10.1111/1469-0691.12189
32. Kampen H, Werner D. Out of the Bush: The Asian Bush Mosquito Aedes japonicus japonicus (Theobald, 1901)(Diptera, Culicidae) Becomes Invasive. Parasites Vectors. (2014) 7:1–10. doi: 10.1186/1756-3305-7-59
33. Kaufman MG, Fonseca DM. Invasion Biology of Aedes japonicus japonicus (Diptera: Culicidae). Annu Rev Entomol. (2014) 59:31–49. doi: 10.1146/annurev-ento-011613-162012
34. Alfano N, Tagliapietra V, Rosso F, Manica M, Arnoldi D, Pindo M, et al. Changes in Microbiota across Developmental Stages of Aedes koreicus, an Invasive Mosquito Vector in Europe: Indications for Microbiota-Based Control Strategies. Front Microbiol. (2019) 10:2832. doi: 10.3389/fmicb.2019.02832
35. Ciocchetta S, Prow NA, Darbro JM, Frentiu FD, Savino S, Montarsi F, et al. The new European invader aedes (Finlaya) koreicus: A potential vector of chikungunya virus. Pathog Global Health. (2018) 112:107–14. doi: 10.1080/20477724.2018.1464780
36. Montarsi F, Ciocchetta S, Devine G, Ravagnan S, Mutinelli F, di Regalbono AF, et al. Development of Dirofilaria Immitis within the Mosquito Aedes (Finlaya) koreicus, a New Invasive Species for Europe. Parasites Vectors. (2015) 8:177. doi: 10.1186/s13071-015-0800-y
37. Medlock JM, Hansford KM, Schaffner F, Versteirt V, Hendrickx G, Zeller H, et al. A review of the invasive mosquitoes in Europe: ecology, public health risks, and control options. Vector-Borne Zoon Dis. (2012) 12:435–47. doi: 10.1089/vbz.2011.0814
38. Metzger ME, Wekesa JW, Kluh S, Fujioka KK, Saviskas R, Arugay A, et al. Detection and establishment of aedes notoscriptus (Diptera: culicidae) mosquitoes in southern California, United States. J Med Entomol. (2022) 59:67–77. doi: 10.1093/jme/tjab165
39. Kay BH, Boyd AM, Ryan PA. 2007 and Hall R. A. Mosquito feeding patterns and natural infection of vertebrates with Ross River and Barmah Forest viruses in Brisbane, Australia. Am J Trop Med Hyg. (2007) 76:417–23. doi: 10.4269/ajtmh.2007.76.417
40. Paris V, Rane RV, Mee PT, Lynch SE, Hoffmann AA, Schmidt TL, et al. Urban population structure and dispersal of an Australian mosquito (Aedes notoscriptus) involved in disease transmission. Heredity. (2023) 130:99–108. doi: 10.1038/s41437-022-00584-4
42. Tanaka K, Mizusawa K, Saugstad ES. A revision of the adult and larval mosquitoes of Japan (Including the Ryukyu archipelago and the Ogasawara islands) and Korea (Diptera: culicidae). Army Med Lab Pacific Apo San Francisco. (1979) vii+-987.
43. Peach DA, Almond M, Pol JC. Modeled Distributions of Aedes japonicus japonicus and Aedes Togoi (Diptera: Culicidae) in the United States, Canada, and Northern Latin America. J Vector Ecol. (2019) 44:119–29. doi: 10.1111/jvec.2019.44.issue-1
44. Arnell JH. Mosquito studies (Diptera, culicidae) xxxiii. A revision of the scapularis group of aedes. Contrib Am Entomol Instit. (1976) 13:1–144.
45. Campbell LP, Burkett-Cadena ND, Miqueli E, Unlu I, Sloyer KE, Medina J, et al. Potential distribution of aedes (Ochlerotatus) scapularis (Diptera: culicidae): A vector mosquito new to the Florida peninsula. Insects. (2021) 12:213. doi: 10.3390/insects12030213
46. Reeves LE, Medina J, Miqueli E, Sloyer KE, Petrie W, Vasquez C, et al. Establishment of aedes (Ochlerotatus) scapularis (Diptera: culicidae) in Mainland Florida, with notes on the ochlerotatus group in the United States. J Med Entomol. (2021) 58:717–29. doi: 10.1093/jme/tjaa250
47. Alarcón-Elbal PM, Rodríguez-Sosa MA, Newman BC, Sutton WB. The first record of aedes vittatus (Diptera: culicidae) in the Dominican republic: public health implications of a potential invasive mosquito species in the Americas. J Med Entomol. (2020) 57:2016–21. doi: 10.1093/jme/tjaa128
48. Pagac BB, Spring AR, Stawicki JR, Dinh TL, Lura T, Kavanaugh MD, et al. Incursion and establishment of the Old World arbovirus vector Aedes (Fredwardsius) vittatus (Bigot, 1861) in the Americas. Acta Trop. (2021) 213:105739. doi: 10.1016/j.actatropica.2020.105739
49. Mondal R, Pemola Devi N, Bhattacharya S. Seasonal prevalence and host preference of some medically important aedes species of Doon valley, India. J Commun Dis. (2021) 53:96–103. doi: 10.24321/0019.5138.202144
50. Petersen V, Santana M, Karina-Costa M, Nachbar JJ, Martin-Martin I, Adelman ZN, et al. Aedes (Ochlerotatus) scapularis, Aedes japonicus japonicus, and Aedes (Fredwardsius) vittatus (Diptera: Culicidae): Three Neglected Mosquitoes with Potential Global Health Risks. Insects. (2024) 15:600. doi: 10.3390/insects15080600
51. Diagne MM, Ndione MHD, Gaye A, Barry MA, Diallo D, Diallo A, et al. Yellow fever outbreak in eastern Senegal, 2020-2021. Viruses. (2021) 13:1475. doi: 10.3390/v13081475
52. Diallo D, Sall AA, Diagne CT, Faye O, Faye O, Ba Y. Zika virus emergence in mosquitoes in southeastern Senegal, 2011. PloS One. (2014) 9:e109442. doi: 10.1371/journal.pone.0109442
53. Service MW. Studies on the biology and taxonomy of Aedes (Stegomyia) vittatus (Bigot) (Diptera: Culicidae) in Northern Nigeria. Trans R Entomol Soc London. (1970) 122:101–43. doi: 10.1111/j.1365-2311.1970.tb00529.x
54. Wilke AB, Benelli G, Beier JC. Beyond frontiers: on invasive alien mosquito species in America and Europe. PloS Negl Trop Dis. (2020) 14:e0007864. doi: 10.1371/journal.pntd.0007864
55. Connelly CR, Alto BW, O’Meara GF. The spread of culex coronator (Diptera: culicidae) throughout Florida. J Vector Ecol. (2016) 41:194–8. doi: 10.1111/jvec.12213
56. Mackay AJ, Kramer WL, Meece JK, Brumfield RT, Foil LD. Host feeding patterns of culex mosquitoes (Diptera: culicidae) in east baton rouge Parish, Louisiana. J Med Entomol. (2014) 47:238–48. doi: 10.1093/jmedent/47.2.238
57. Bartholomay LC, Waterhouse RM, Mayhew GF, Campbell CL, Michel K, Zou Z, et al. Pathogenomics of culex quinquefasciatus and meta-analysis of infection responses to diverse pathogens. Science. (2010) 330:88–90. doi: 10.1126/science.1193162
58. van den Hurk AF, Hall-Mendelin S, Jansen CC, Higgs S. Zika virus and culex quinquefasciatus mosquitoes: A tenuous link. Lancet Infect Dis. (2017) 17:1014–6. doi: 10.1016/S1473-3099(17)30518-2
59. Longbottom J, Browne AJ, Pigott DM, Sinka ME, Golding N, Hay SI, et al. Mapping the spatial distribution of the Japanese encephalitis vector, Culex tritaeniorhynchus Giles, 1901 (Diptera: Culicidae) within areas of Japanese encephalitis risk. Parasites Vectors. (2017) 10:148. doi: 10.1186/s13071-017-2086-8
60. Pennington NE, Phelps CA. Identification of the host range of culex tritaeniorhynchus mosquitoes on Okinawa, Ryukyu islands. J Med Entomol. (1968) 5:483–7. doi: 10.1093/jmedent/5.4.483
61. Williams CR, Webb CE, Higgs S, van den Hurk AF. Japanese encephalitis virus emergence in Australia: Public health importance and implications for future surveillance. Vector Borne Zoon Dis. (2022) 22:529–34. doi: 10.1089/vbz.2022.0037
62. Hiwat H, Bretas G. Ecology of anopheles darlingi root with respect to vector importance: A review. Parasites Vectors. (2011) 4:177. doi: 10.1186/1756-3305-4-177
63. Takken W, Verhulst NO. Host preferences of blood-feeding mosquitoes. Annu Rev Entomol. (2013) 58:433–53. doi: 10.1146/annurev-ento-120811-153618
64. Faulde MK, Rueda LM, Khaireh BA. First record of the Asian malaria vector anopheles stephensi and its possible role in the resurgence of malaria in Djibouti, horn of Africa. Acta Tropica. (2014) 139:39–43. doi: 10.1016/j.actatropica.2014.06.016
65. Balkew M, Mumba P, Dengela D, Yohannes G, Getachew D, Yared S, et al. Geographical distribution of anopheles stephensi in eastern Ethiopia. Parasites Vectors. (2020) 13:1–8. doi: 10.1186/s13071-020-3904-y
66. World Health Organization. Vector Alert: Anopheles stephensi Invasion and Spread: Horn of Africa, the Republic of the Sudan and Surrounding Geographical Areas, and Sri Lanka: Information Note (2019). Available online at: https://iris.who.int/handle/10665/326595 (accessed August 15, 2024).
67. Sinka M, Pironon S, Massey N, Longbottom J, Hemingway J, Moyes C, et al. A New Malaria Vector in Africa: Predicting the Expansion Range of Anopheles stephensi and Identifying the Urban Populations at Risk. Proc Natl Acad Sci. (2020) 117:24900–8. doi: 10.1073/pnas.2003976117
68. Sinka ME, Bangs MJ, Manguin S, Chareonviriyaphap T, Patil AP, Temperley WH, et al. The dominant anopheles vectors of human malaria in the Asia-Pacific region: occurrence data, distribution maps and bionomic précis. Parasites Vectors. (2011) 4:1–46. doi: 10.1186/1756-3305-4-89
69. Tadesse FG, Ashine T, Teka H, Esayas E, Messenger LA, Chali W, et al. Anopheles stephensi Mosquitoes as Vectors of Plasmodium vivax and falciparum, Horn of Africa, 2019. Emerg Infect Dis. (2021) 27:603–7. doi: 10.3201/eid2702.200019
70. Afrane YA, Abdulai A, Mohammed AR, Akuamoah-Boateng Y, Owusu-Asenso CM, Sraku IK, et al. Detection of invasive Anopheles stephensi mosquitoes through molecular surveillance, Ghana. Emerg Infect Dis. (2024) 30:605. doi: 10.3201/eid3003.231638
71. Atkinson CT, LaPointe DA. Introduced avian diseases, climate change, and the future of Hawaiian honeycreepers. J Avian Med Surg. (2009) 23:53–63. doi: 10.1647/2008-059.1
72. Balkew M, Mumba P, Yohannes G, Abiy E, Getachew D, Yared S, et al. An update on the distribution, bionomics, and insecticide susceptibility of anopheles stephensi in Ethiopia, 2018–2020. Malaria J. (2021) 20:263. doi: 10.1186/s12936-021-03801-3
73. Emiru T, Getachew D, Murphy M, Sedda L, Ejigu LA, Bulto MG, et al. Evidence for a role of anopheles stephensi in the spread of drug-and diagnosis-resistant malaria in Africa. Nat Med. (2023) 29:3203–11. doi: 10.1038/s41591-023-02641-9
74. World Health Organization. WHO Initiative to Stop the Spread of Anopheles stephensi in Africa (2023). Available online at: https://www.who.int/publications/i/item/who-ucn-gmp-2022.06(accessed August 15, 2024).
75. Lounibos LP. Invasions by insect vectors of human disease. Annu Rev Entomol. (2002) 47:233–66. doi: 10.1146/annurev.ento.47.091201.145206
76. Bryant JE, Holmes EC, Barrett ADT. Out of Africa: A molecular perspective on the introduction of yellow fever virus into the Americas. PloS Pathog. (2007) 3:e75. doi: 10.1371/journal.ppat.0030075
77. Ibáñez-Justicia A. Pathways for introduction and dispersal of invasive aedes mosquito species in Europe: A review. J Eur Mosq Control Assoc. (2020) 38:1–10.
78. Seidel B, Montarsi F, Huemer HP, Indra A, Capelli G, Allerberger F, et al. First Record of the Asian Bush Mosquito, Aedes japonicus japonicus, in Italy: Invasion from an Established Austrian Population. Parasites Vectors. (2016) 9:1–4. doi: 10.1186/s13071-016-1566-6
79. Farnesi LC, Vargas HC, Valle D, Rezende GL. Darker eggs of mosquitoes resist more to dry conditions: melanin enhances serosal cuticle contribution in egg resistance to desiccation in aedes, anopheles and culex vectors. PloS Negl Trop Dis. (2017) 11:e0006063. doi: 10.1371/journal.pntd.0006063
80. Hawley WA, Reiter P, Copeland RS, Pumpuni CB, Craig GB. Aedes albopictus in North America: probable introduction in used tires from northern Asia. Science. (1987) 236:1114–6. doi: 10.1126/science.3576225
81. Hahn MB, Eisen RJ, Eisen L, Boegler KA, Moore CG, McAllister J, et al. Reported Distribution of Aedes (Stegomyia) aEgypti and Aedes (Stegomyia) albopictus in the United States, 1995-2016 (Diptera: Culicidae). J Med Entomol. (2016) 53:1169–75. doi: 10.1093/jme/tjw072
82. Dalla Pozza G, Majori G. First record of aedes albopictus establishment in Italy. J Am Mosq Control Assoc. (1992) 8:318–20.
83. Schaffner F, Karch S. First report of aedes albopictus (Skuse, 1984) in metropolitan France. Comptes rendus l’Academie Des Sci Serie III Sci la vie. (2000) 4:373–5. doi: 10.1016/s0764-4469(00)00143-8
84. Petrić D, Pajović I, Ignjatović-Ćupina A, Zgomba M. Aedes albopictus (Skuse, 1894), new mosquito species (Diptera: culicidae) in entomofauna of Yugoslavia. Biljni Lekar. (2001) 29:547–8.
85. Scholte E, Schaffner F. Waiting for the Tiger: Establishment and Spread of the Aedes albopictus Mosquito in Europe. In: Takken W, Knols B, editors. Emerging Pests and Vector-Borne Diseases in Europe. Wageningen, Netherlands: Wageningen Academic Publishers (2007).
86. Scholte E, Den Hartog W, Dik M, Schoelitsz B, Brooks M, Schaffner F, et al. Introduction and control of three invasive mosquito species in the Netherlands, July-October 2010. Eurosurveillance. (2010) 15:19710. doi: 10.2807/ese.15.45.19710-en
87. Osório HC, Zé-Zé L, Neto M, Silva S, Marques F, Silva AS, et al. Detection of the invasive mosquito species aedes (Stegomyia) albopictus (Diptera: culicidae) in Portugal. Int J Environ Res Public Health. (2018) 15:820. doi: 10.3390/ijerph15040820
88. Scholte EJ, Dijkstra E, Blok H, De Vries A, Takken W, Hofhuis A, et al. Accidental Importation of the Mosquito Aedes albopictus into the Netherlands: A Survey of Mosquito Distribution and the Presence of Dengue Virus. Med Vet Entomol. (2008) 22:352–8. doi: 10.1111/j.1365-2915.2008.00763.x
89. Metzger ME, Hardstone Yoshimizu M, Padgett KA, Hu R, Kramer VL. Detection and Establishment of Aedes aEgypti and Aedes albopictus (Diptera: Culicidae) Mosquitoes in California, 2011–2015. J Med Entomol. (2017) 54:533–43. doi: 10.1093/jme/tjw237
90. Linthicum KJ, Kramer VL, Madon MB, Fujioka K. Introduction and potential establishment of aedes albopictus in California in 2001. J Am Mosq Control Assoc. (2003) 19:301–8.
91. Madon MB, Hazelrigg JE, Shaw MW, Kluh S, Mulla MS. Has aedes albopictus established in California? J Am Mosq Control Assoc. (2003) 19:297–300.
92. Kilpatrick AM, Daszak P, Goodman SJ, Rogg H, Kramer LD, Cedeno V, et al. Predicting pathogen introduction: west Nile virus spread to Galapagos. Conserv Biol. (2006) 20:1224–31. doi: 10.1111/j.1523-1739.2006.00423.x
93. Bataille A, Cunningham AA, Cedeno V, Cruz M, Eastwood G, Fonseca DM, et al. Evidence for regular ongoing introductions of mosquito disease vectors into the Galápagos islands. Proc R Soc B: Biol Sci. (2009) 276:3769–75. doi: 10.1098/rspb.2009.0998
94. Ward R. Mosquito Fauna of Guam. Case History of an Introduced Fauna. In: Laird M, editor. Commerce and the spread of pests and disease vectors (1984). p. 143–62.
95. Ballardini M, Ferretti S, Chiaranz G, Pautasso A, Riina MV, Triglia G, et al. First report of the invasive mosquito aedes koreicus (Diptera: culicidae) and of its establishment in Liguria, Northwest Italy. Parasites Vectors. (2019) 12:1–13. doi: 10.1186/s13071-019-3589-2
96. Ibáñez-Justicia A, Smitz N, Den Hartog W, van de Vossenberg B, De Wolf K, Deblauwe I, et al. Detection of exotic mosquito species (Diptera: culicidae) at international airports in Europe. Int J Environ Res Public Health. (2020) 17:3450. doi: 10.3390/ijerph17103450
97. Schmidt TL, van Rooyen AR, Chung J, Endersby-Harshman NM, Griffin PC, Sly A, et al. Tracking Genetic Invasions: Genome-Wide Single Nucleotide Polymorphisms Reveal the Source of Pyrethroid-Resistant Aedes aEgypti (Yellow Fever Mosquito) Incursions at International Ports. Evolution Appl. (2019) 12:1136–46. doi: 10.1111/eva.2019.12.issue-6
98. Brown EB, Adkin A, Fooks AR, Stephenson B, Medlock JM, Snary EL. Assessing the risks of west Nile virus–infected mosquitoes from transatlantic aircraft: implications for disease emergence in the United Kingdom. Vector-Borne Zoon Dis. (2012) 12:310–20. doi: 10.1089/vbz.2010.0176
99. Grout A, Russell RC. Aircraft disinsection: what is the usefulness as a public health measure? J Travel Med. (2021) 28:taaa124. doi: 10.1093/jtm/taaa124
100. Werner D, Kampen H. The Further Spread of Aedes japonicus japonicus (Diptera, Culicidae) Towards Northern Germany. Parasitol Res. (2013) 112:3665–8. doi: 10.1007/s00436-013-3564-3
101. Eritja R, Palmer JR, Roiz D, Sanpera-Calbet I, Bartumeus F. Direct evidence of adult aedes albopictus dispersal by car. Sci Rep. (2017) 7:1–15. doi: 10.1038/s41598-017-12652-5
102. Egizi A, Kiser J, Abadam C, Fonseca DM. The Hitchhiker’s guide to becoming invasive: exotic mosquitoes spread across a US state by human transport not autonomous flight. Mol Ecol. (2016) 25:3033–47. doi: 10.1111/mec.2016.25.issue-13
103. Medley KA, Jenkins DG, Hoffman EA. Human-aided and natural dispersal drive gene flow across the range of an invasive mosquito. Mol Ecol. (2015) 24:284–95. doi: 10.1111/mec.2015.24.issue-2
104. Becker N, Schön S, Klein A-M, Ferstl I, Kizgin A, Tannich E, et al. First mass development of aedes albopictus (Diptera: culicidae)—Its surveillance and control in Germany. Parasitol Res. (2017) 116:847–58. doi: 10.1007/s00436-016-5356-z
105. Service M. Mosquito (Diptera: culicidae) dispersal—the long and short of it. J Med Entomol. (1997) 34:579–88. doi: 10.1093/jmedent/34.6.579
106. Verdonschot PF, Besse-Lototskaya AA. Flight distance of mosquitoes (Culicidae): A metadata analysis to support the management of barrier zones around rewetted and newly constructed wetlands. Limnologica. (2014) 45:69–79. doi: 10.1016/j.limno.2013.11.002
107. Schaffner F, Ries C. First evidence and distribution of the invasive alien mosquito aedes japonicus (Theobald, 1901) in Luxembourg. Bull Soc Nat luxemb. (2019) 121:169–83.
108. Atieli HE, Zhou G, Zhong D, Wang X, Lee MC, Yaro AS, et al. Wind-assisted high-altitude dispersal of mosquitoes and other insects in East Africa. J Med Entomol. (2023) 60:698–707. doi: 10.1093/jme/tjad033
109. Arai S, Kuwata R, Higa Y, Maekawa Y, Tsuda Y, Roychoudhury S, et al. Two hidden taxa in the Japanese encephalitis vector mosquito, Culex tritaeniorhynchus, and the potential for long-distance migration from overseas to Japan. PloS Negl Trop Dis. (2022) 16:e0010543. doi: 10.1371/journal.pntd.0010543
110. Lehmann T, Bamou R, Chapman JW, Reynolds DR, Armbruster PA, Dao A, et al. Urban malaria may be spreading via the wind—here’s why that’s important. Proc Natl Acad Sci. (2023) 120:e2301666120. doi: 10.1073/pnas.2301666120
111. Lahondère C, Bonizzoni M. Thermal biology of invasive aedes mosquitoes in the context of climate change. Curr Opin Insect Sci. (2022) 51:100920. doi: 10.1016/j.cois.2022.100920
112. Cunze S, Koch LK, Kochmann J, Klimpel S. Aedes albopictus and Aedes japonicus-Two Invasive Mosquito Species with Different Temperature Niches in Europe. Parasites Vectors. (2016) 9:1–12. doi: 10.1186/s13071-016-1853-2
113. Nawrocki S, Hawley W. Estimation of the northern limits of distribution of aedes albopictus in North America. J Am Mosq Control Assoc. (1987) 3:314–7.
114. Sherpa S, Blum MG, Després L. Cold adaptation in the Asian tiger mosquito’s native range precedes its invasion success in temperate regions. Evolution. (2019) 73:1793–808. doi: 10.1111/evo.13801
115. Medley KA, Westby KM, Jenkins DG. Rapid local adaptation to northern winters in the invasive Asian tiger mosquito aedes albopictus: A moving target. J Appl Ecol. (2019) 56:2518–27. doi: 10.1111/1365-2664.13480
116. Lounibos L, Escher R, Lourenço-de-Oliveira R. Asymmetric evolution of photoperiodic diapause in temperate and tropical invasive populations of aedes albopictus (Diptera: culicidae). Ann Entomol Soc America. (2003) 96:512–8. doi: 10.1603/0013-8746(2003)096[0512:AEOPDI]2.0.CO;2
117. Urbanski J, Mogi M, O’Donnell D, DeCotiis M, Toma T, Armbruster P. Rapid adaptive evolution of photoperiodic response during invasion and range expansion across a climatic gradient. Am Nat. (2012) 179:490–500. doi: 10.1086/664709
118. Juliano SA, O’Meara GF, Morrill JR, Cutwa MM. Desiccation and thermal tolerance of eggs and the coexistence of competing mosquitoes. Oecologia. (2002) 130:458–69. doi: 10.1007/s004420100811
119. Lounibos LP, Juliano SA. Where vectors collide: the importance of mechanisms shaping the realized niche for modeling ranges of invasive aedes mosquitoes. Biol Invasions. (2018) 20:1913–29. doi: 10.1007/s10530-018-1674-7
120. Barrera R. Competition and resistance to starvation in larvae of container-inhabiting aedes mosquitoes. Ecol Entomol. (1996) 21:117–27. doi: 10.1111/j.1365-2311.1996.tb01178.x
121. Juliano SA, Lounibos LP, O’Meara GF. A Field Test for Competitive Effects of Aedes albopictus on Ae. aEgypti in South Florida: Differences between Sites of Coexistence and Exclusion? Oecologia. (2004) 139:583–93. doi: 10.1007/s00442-004-1532-4
122. Heger T, Jeschke JM. The enemy release hypothesis as a hierarchy of hypotheses. Oikos. (2014) 123:741–50. doi: 10.1111/j.1600-0706.2013.01263.x
123. Grill CP, Juliano SA. Predicting species interactions based on behaviour: predation and competition in container-dwelling mosquitoes. J Anim Ecol. (1996) 65:63–76. doi: 10.2307/5700
124. Weinstein P, Laird M, Browne GN. Exotic and Endemic Mosquitoes in New Zealand as Potential Arbovirus Vectors. Ministry of Health, Wellington, New Zealand: Occasional Papers (1997).
125. Freed T, Leisnham P. Roles of spatial partitioning, competition, and predation in the North American invasion of an exotic mosquito. Oecologia. (2014) 175:601–11. doi: 10.1007/s00442-014-2909-7
126. Pereira TN, Prophiro JS, da Silva GL, de Oliveira JG, da Silva OS. Ascogregarina (Apicomplexa: Lecudinidae): An Overview of Its Distribution and Pathogenicity on Aedes aEgypti and Ae. albopictus Development. Biotemas. (2018) 31:1–13. doi: 10.5007/2175-7925.2018v31n3p1
127. Aliabadi BW, Juliano SA. Escape from gregarine parasites affects the competitive interactions of an invasive mosquito. Biol Invasions. (2002) 4:283–97. doi: 10.1023/A:1020933705556
128. Stump E, Childs LM, Walker M. Parasitism of Aedes albopictus by Ascogregarina Taiwanensis Lowers Its Competitive Ability against Aedes triseriatus. Parasites Vectors. (2021) 14:1–15. doi: 10.1186/s13071-021-04581-0
129. Laird M, Calder L, Thornton RC, Syme R, Holder P, Mogi M. Japanese Aedes albopictus among Four Mosquito Species Reaching New Zealand in Used Tires. J Am Mosq Control Association-Mosquito News. (1994) 10:14–23.
130. Derraik JG. Exotic mosquitoes in New Zealand: A review of species intercepted, their pathways and ports of entry. Aust New Z J Public Health. (2004) 28:433–44. doi: 10.1111/j.1467-842X.2004.tb00025.x
131. Peyton E, Campbell SR, Candeletti TM, Romanowski M, Crans WJ. Aedes (Finlaya) japonicus japonicus (Theobald), a New Introduction into the United States. J Am Mosq Control Assoc. (1999) 15:238–41.
132. Jackson M, Belton P, McMahon S, Hart M, McCann S, Azevedo D, et al. The first record of aedes (Hulecoeteomyia) japonicus (Diptera: culicidae) and its establishment in western Canada. J Med Entomol. (2016) 53:241–4. doi: 10.1093/jme/tjv164
133. Koban MB, Kampen H, Scheuch DE, Frueh L, Kuhlisch C, Janssen N, et al. The Asian Bush Mosquito Aedes japonicus japonicus (Diptera: Culicidae) in Europe, 17 Years after Its First Detection, with a Focus on Monitoring Methods. Parasites Vectors. (2019) 12:1–13. doi: 10.1186/s13071-019-3349-3
134. Takashima I, Rosen L. Horizontal and vertical transmission of Japanese encephalitis virus by aedes japonicus (Diptera: culicidae). J Med Entomol. (1989) 26:454–8. doi: 10.1093/jmedent/26.5.454
135. Chagin K, Kondratiev P. Vectors of autumnal (Japanese) encephalitis in primor’ye region and measures for controlling them. Meditsinskaia Parazitologiia i Parazitarnye Bolezni. (1943) 12:34–44.
136. Huber K, Jansen S, Leggewie M, Badusche M, Schmidt-Chanasit J, Becker N, et al. Aedes japonicus japonicus (Diptera: Culicidae) from Germany Have Vector Competence for Japan Encephalitis Virus but Are Refractory to Infection with West Nile Virus. Parasitol Res. (2014) 113:3195–9. doi: 10.1007/s00436-014-3983-9
137. Turell MJ, O’Guinn ML, Dohm DJ, Jones JW. Vector competence of North American mosquitoes (Diptera: culicidae) for west Nile virus. J Med Entomol. (2001) 38:130–4. doi: 10.1603/0022-2585-38.2.130
138. DeCarlo CH, Campbell SR, Bigler LL, Mohammed HO. Aedes japonicus and west Nile virus in New York. J Am Mosq Control Assoc. (2020) 36:261–3. doi: 10.2987/20-6958.1
139. Sardelis MR, Turell MJ, Andre RG. Experimental transmission of St. Louis encephalitis virus by ochlerotatus J. japonicus. J Am Mosq Control Assoc. (2003) 19:159–62.
140. Sardelis MR, Dohm DJ, Pagac B, Andre RG, Turell MJ. Experimental transmission of eastern equine encephalitis virus by ochlerotatus J. japonicus (Diptera: culicidae). J Med Entomol. (2002) 39:480–4. doi: 10.1603/0022-2585-39.3.480
141. Sardelis MR, Turell MJ, Andre RG. Laboratory transmission of la crosse virus by ochlerotatus J. japonicus (Diptera: culicidae). J Med Entomol. (2002) 39:635–9. doi: 10.1603/0022-2585-39.4.635
142. Westby KM, Fritzen C, Paulsen D, Poindexter S, Moncayo AC. La Crosse Encephalitis Virus Infection in Field-Collected Aedes albopictus, Aedes japonicus, and Aedes triseriatus in Tennessee. J Am Mosq Control Assoc. (2015) 31:233–41. doi: 10.2987/moco-31-03-233-241.1
143. Turell M, Byrd B, Harrison B. Potential for Populations of Aedes j. japonicus to Transmit Rift Valley Fever Virus in the USA. J Am Mosq Control Assoc. (2013) 29:133–7. doi: 10.2987/12-6316r.1
144. Schaffner F, Vazeille M, Kaufmann C, Failloux A-B, Mathis A. Vector competence of aedes japonicus for chikungunya and dengue viruses. Eur Mosq Bull. (2011) 29):141–2. doi: 10.5167/uzh-53052
145. Abbo SR, Visser TM, Wang H, Göertz GP, Fros JJ, Abma-Henkens MH, et al. The invasive Asian bush mosquito aedes japonicus found in the Netherlands can experimentally transmit zika virus and usutu virus. PloS Negl Trop Dis. (2020) 14:e0008217. doi: 10.1371/journal.pntd.0008217
146. Jansen S, Heitmann A, Lühken R, Jöst H, Helms M, Vapalahti O, et al. Experimental Transmission of Zika Virus by Aedes japonicus japonicus from Southwestern Germany. Emerg Microbes Infect. (2018) 7:1–6. doi: 10.1038/s41426-018-0195-x
147. Takashima I, Hashimoto N. Getah virus in several species of mosquitoes. Trans R Soc Trop Med Hyg. (1985) 79:546–50. doi: 10.1016/0035-9203(85)90091-4
148. Uchida L, Shibuya M, Morales-Vargas RE, Hagiwara K, Muramatsu Y. Zika virus potential vectors among aedes mosquitoes from Hokkaido, northern Japan: implications for potential emergence of zika disease. Pathogens. (2021) 10:938. doi: 10.3390/pathogens10080938
149. Samuel MD, Woodworth BL, Atkinson CT, Hart PJ, LaPointe DA. Avian malaria in Hawaiian forest birds: infection and population impacts across species and elevations. Ecosphere. (2015) 6:1–21. doi: 10.1890/ES14-00393.1
150. Fonseca DM, Keyghobadi N, Malcolm CA, Mehmet C, Schaffner F, Mogi M, et al. Emerging vectors in the culex pipiens complex. Science. (2004) 303:1535–8. doi: 10.1126/science.1094247
151. Vasilakis N, Weaver SC. The history and evolution of human dengue emergence. Adv Virus Res. (2008) 72:1–76. doi: 10.1016/S0065-3527(08)00401-6
152. Park AW, Cleveland CA, Dallas TA, Corn JL. Vector species richness increases haemorrhagic disease prevalence through functional diversity modulating the duration of seasonal transmission. Parasitology. (2016) 143:874–9. doi: 10.1017/S0031182015000578
153. Shahhosseini N, Frederick C, Racine T, Kobinger GP, Wong G. Modeling host-feeding preference and molecular systematics of mosquitoes in different ecological niches in Canada. Acta Tropica. (2021) 213:105734. doi: 10.1016/j.actatropica.2020.105734
154. Nasci RS. Differences in Host Choice between the Sibling Species of Treehole Mosquitoes Aedes triseriatus and Aedes hendersoni. Am J Trop Med Hyg. (1982) 31:411–5. doi: 10.4269/ajtmh.1982.31.411
155. Irby WS, Apperson CS. Hosts of mosquitoes in the coastal plain of North Carolina. J Med Entomol. (1988) 25:85–93. doi: 10.1093/jmedent/25.2.85
156. Molaei G, Andreadis TG, Armstrong PM, Diuk-Wasser M. Host-feeding patterns of potential mosquito vectors in Connecticut, USA: molecular analysis of bloodmeals from 23 species of aedes, anopheles, culex, coquillettidia, psorophora, and uranotaenia. J Med Entomol. (2008) 45:1143–51. doi: 10.1093/jmedent/45.6.1143
157. Szumlas DE, Apperson CS, Powell EE, Hartig P, Francy DB, Karabotsos N. Relative abundance and species composition of mosquito populations (Diptera: culicidae) in a la crosse virus-endemic area in western North Carolina. J Med Entomol. (1996) 33:598–607. doi: 10.1093/jmedent/33.4.598
158. Richards SL, Ponnusamy L, Unnasch TR, Hassan HK, Apperson CS. Host-feeding patterns of aedes albopictus (Diptera: culicidae) in relation to availability of human and domestic animals in suburban landscapes of central North Carolina. J Med Entomol. (2006) 43:543–51. doi: 10.1093/jmedent/43.3.543
159. Niebylski ML, Savage HM, Nasci RS, Craig GB Jr. Blood hosts of aedes albopictus in the United States. J Am Mosq Control Assoc. (1994) 10:447–50.
160. Savage H, Niebylski M, Smith G, Mitchell C, Craig G. Host-Feeding Patterns of Aedes albopictus (Diptera: Culicidae) at a Temperate North American Site. J Med Entomol. (1993) 30:27–34. doi: 10.1093/jmedent/30.1.27
161. Goodman H, Egizi A, Fonseca DM, Leisnham PT, LaDeau SL. Primary blood-hosts of mosquitoes are influenced by social and ecological conditions in a complex urban landscape. Parasites Vectors. (2018) 11:1–10. doi: 10.1186/s13071-018-2779-7
162. Gingrich JB, Williams GM. Host-feeding patterns of suspected West Nile virus mosquito vectors in Delaware, 2001-2002. J Am Mosq Control Assoc. (2005) 21:194–200. doi: 10.2987/8756-971X(2005)21[194:HPOSWN]2.0.CO;2
163. Faraji A, Egizi A, Fonseca DM, Unlu I, Crepeau T, Healy SP. Comparative host feeding patterns of the Asian tiger mosquito, aedes albopictus, in urban and suburban Northeastern USA and implications for disease transmission. PloS Negl Trop Dis. (2014) 8:e3037. doi: 10.1371/journal.pntd.0003037
164. Fikrig K, Martin E, Dang S, St Fleur K, Goldsmith H, Qu S, et al. The effects of host availability and fitness on aedes albopictus blood feeding patterns in New York. Am J Trop Med Hyg. (2022) 106:320. doi: 10.4269/ajtmh.21-0157
165. Apperson CS, Hassan HK, Harrison BA, Savage HM, Aspen SE, Farajollahi A, et al. Host feeding patterns of established and potential mosquito vectors of west Nile virus in the eastern United States. Vector-Borne Zoon Dis. (2004) 4:71–82. doi: 10.1089/153036604773083013
166. Molaei G, Farajollahi A, Scott JJ, Gaugler R, Andreadis TG. Human Bloodfeeding by the Recently Introduced Mosquito, Aedes japonicus japonicus, and Public Health Implications. J Am Mosq Control Assoc. (2009) 25:210–4. doi: 10.2987/09-0012.1
167. Little EA, Harriott OT, Akaratovic KI, Kiser JP, Abadam CF, Shepard JJ, et al. Host interactions of aedes albopictus, an invasive vector of arboviruses, in Virginia, USA. PloS Negl Trop Dis. (2021) 15:e0009173. doi: 10.1371/journal.pntd.0009173
168. Bara JJ, Parker AT, Muturi EJ. Comparative Susceptibility of Ochlerotatus japonicus, Ochlerotatus triseriatus, Aedes albopictus, and Aedes aEgypti (Diptera: Culicidae) to La Crosse Virus. J Med Entomol. (2016) 53:1415–21. doi: 10.1093/jme/tjw097
169. Gerhardt RR, Gottfried KL, Apperson CS, Davis BS, Erwin PC, Smith AB, et al. First isolation of La Crosse virus from naturally infected. Aedes albopictus Emerg Infect Dis. (2001) 7:807–11. doi: 10.3201/eid0705.017506
170. Harris MC, Dotseth EJ, Jackson BT, Zink SD, Marek PE, Kramer LD, et al. La Crosse Virus in Aedes japonicus japonicus mosquitoes in the Appalachian Region, United States. Emerg Infect Dis. (2015) 21:646–9. doi: 10.3201/eid2104.140734
171. Onstwedder C, Lock-Wah-Hoon J, Van Dorp S, Braks M, Van Asten L, Zheng Y, et al. Comparing vector-borne disease surveillance and response in Beijing and the Netherlands. Ann Global Health. (2022) 88:1–14. doi: 10.5334/aogh.3672
172. Aryaprema VS, Steck MR, Peper ST, Farooq M, Xue R-D, Qualls WA. Initial survey of mosquito control programs with set action thresholds and associated surveillance characteristics. J Am Mosq Control Assoc. (2022) 38:29–39. doi: 10.2987/21-7050
173. Connelly CR, Gerding JA, Jennings SM, Ruiz A, Barrera R, Partridge S, et al. Continuation of mosquito surveillance and control during public health emergencies and natural disasters. MMWR Morbid Mortal Week Rep. (2020) 69:938–40. doi: 10.15585/mmwr.mm6928a6
Keywords: mosquito-borne diseases, mosquito invasion, vector surveillance and control, alien species introduction, pathogen transmission
Citation: Yan J, Mackay AJ and Stone CM (2024) Dynamics of invasive mosquitoes: introduction pathways, limiting factors, and their potential role in vector-borne pathogen transmission. Front. Trop. Dis. 5:1503120. doi: 10.3389/fitd.2024.1503120
Received: 28 September 2024; Accepted: 20 November 2024;
Published: 12 December 2024.
Edited by:
Estela Gonzalez, Animal and Plant Health Agency, United KingdomReviewed by:
Zubaidah Ya’cob, University of Malaya, MalaysiaCharles Mbogo, Kenya Medical Research Institute (KEMRI), Kenya
Copyright © 2024 Yan, Mackay and Stone. This is an open-access article distributed under the terms of the Creative Commons Attribution License (CC BY). The use, distribution or reproduction in other forums is permitted, provided the original author(s) and the copyright owner(s) are credited and that the original publication in this journal is cited, in accordance with accepted academic practice. No use, distribution or reproduction is permitted which does not comply with these terms.
*Correspondence: Jiayue Yan, amlheXVlQGlsbGlub2lzLmVkdQ==