- 1Universidad de Córdoba, Instituto de Investigaciones Biológicas del Trópico, Monteria, Colombia
- 2Universidad de Córdoba, Facultad de Ciencias de la Salud, Departamento de Bacteriologia, Monteria, Colombia
- 3Universidad Cooperativa de Colombia, Facultad de Medicina, Villavicencio, Colombia
- 4Programa de Gerencia de la Calidad y Auditoria en Salud, Corporación Universitaria del Caribe, Sincelejo, Colombia
Introduction: Vector-borne diseases are a public health problem in tropical countries, especially Malaria, Leishmaniasis, Chagas, and the arboviral diseases Dengue, Chikungunya, and Zika. Tropical diseases represent a significant challenge to health systems in developing countries.
Objective: This study aimed to explore the association between climatic variables and primary vector-borne diseases in Colombia between 2011-2021.
Methods: A retrospective analysis of the epidemiological data of Malaria, Leishmania, Chagas, Dengue, Chikungunya, and Zika and the climatic data recorded in 2011-2021 was carried out. The cases were obtained from SIVIGILA of the Colombian Ministry of Health. The climatic data were obtained from WorldClim.
Results: Leishmania, Malaria, and Chagas occur in the departments of Caquetá (located in the Amazon rainforest), Chocó (known for its high rainfall), and Casanare (a region with a high prevalence of Chagas disease). There was no statistical correlation between the number of cases and climatic variables, except for malaria cases in which precipitation had a Pearson correlation value of 0.415. The results predicted an increase of 1.469 malaria cases for every unit increase in precipitation. The cases of dengue, Zika, and chikungunya had similar behavior, with the majority occurring in the departments of Valle del Cauca, Antioquia, Norte de Santander, and Bolívar.
Conclusions: Except for Malaria, the distribution of cases did not directly correlate with temperature and precipitation. Temperature and precipitation did not explain the epidemiological profile of the studied diseases.
Introduction
Infectious diseases transmitted by vectors are not just a local concern but a global public health problem. Arboviruses, such as dengue, chikungunya, and zika, as well as leishmaniasis, malaria, and Chagas, are considered relevant, causing high morbidity and mortality worldwide. These diseases, which are prevalent in tropical and subtropical areas, demand continuous epidemiological surveillance to curb their spread (1).
Parasitic diseases caused by Leishmania sp., Plasmodium sp., and Trypanosoma sp. are transmitted by sandflies, Anopheles, and triatomine, respectively (2). Leishmaniasis is a complex disease that can manifest in various ways, mainly in three clinical forms: cutaneous, mucocutaneous, and visceral. Each has different clinical characteristics and can vary in severity, some of which can be severe (3). Malaria, with symptoms of intermittent fever, chills, nausea, and fatigue, can lead to severe kidney and brain complications, and even death (4). Chagas disease has the potential to cause fever, fatigue, headache, body pain, and severe cardiac and gastrointestinal problems, and is endemic in Colombia and many countries with tropical climates (3).
In contrast, dengue, chikungunya, and zika are transmitted mainly to humans through the bite of female mosquitoes of the Aedes genus (5). The clinical manifestations of these arboviruses are similar at the beginning of the clinical presentation, and patients may present with fever, headache, rash, myalgia, and arthralgia (6). At the same time, these diseases are characterized by complications, such as microcephaly in newborns due to ZIKV (7, 8), chronic post-chikungunya joint disease, and severe dengue. They can also generate alterations such as shock due to plasma extravasation, respiratory difficulty, profuse bleeding, or severe organ involvement (9).
Owing to climate change, the vectors transmitting these diseases have extended their geographic distribution area to almost all continents (10). Indigenous cases of dengue, chikungunya, zika, and malaria have been reported in the United States (11–14). This is a clear indication of the urgent need for action. Temperature increase, a direct result of climate change, has significantly favored the life cycle of mosquitoes and the replication rate of the virus (15, 16). Temperatures between 26°C and 28°C increase the kinetics of development and survival in all stages of the genus Aedes (17). In addition, the eggs hatched at higher temperatures, which may indicate a possible adaptive physiological response.
Additionally, the impact of temperature on the behavior of the disease vectors is noteworthy. For instance, slightly higher temperatures of 28°C stimulate vectors to bite more frequently, thereby increasing the risk of disease transmission (17). This is particularly true for mosquitoes of the genus Anopheles, because extreme temperatures, whether low or high, can disrupt their development and survival (18). Similarly, sandflies exposed to 18°C experienced a high mortality rate among their larvae and pupae (19). Conversely, triatomines thrive at temperatures above 28°C, which favors their life cycles (20).
Conversely, rainfall increased the number of vector reproduction sites and the survival of adults. Temporary “pools” become favorable habitats for the development of mosquito larvae, whereas moist and decomposing organic matter is practical for the development of sandflies (15). Following this train of thought in this line of thought, climate change is one of the most relevant global challenges, mainly in developing countries, where the burden of infectious diseases is usually significantly higher in countries with limited medical care, water, drinking water, and adequate housing, among others.
The present work aimed to study climatic variables and their relationship with vector-borne disease cases in Colombia from 2011 to 2021.
Materials and methods
Type of study and data collection
This comprehensive retrospective descriptive study examined cases of dengue, chikungunya, cika, malaria, leishmaniasis, and chagas in Colombia from 2011 to 2021. Cases were obtained from the 21. Climate data were obtained from the WorldClim global meteorological and climate databases (22).
Obtention data and depuration analysis
The historical reports of diseases between 2011 and 2021 were initially downloaded through the comprehensive SIVIGILA platform, ensuring a wide range of data sources. Reports for the study diseases (dengue, Zika, chikungunya, leishmaniasis, Chagas, and malaria) were extracted from these records, providing a comprehensive overview of the diseases. No discrimination was made based on clinical manifestations of the disease. The database was built with the following aspects: name and code of municipality/population center, disease, number of cases, and year. The cases were then summarized as monthly cases, and the data were concatenated according to the code of each municipality to obtain a summary of the cases monthly from 2011 to 2021. This last database was subjected to eliminating incomplete records and duplicate data, and standardizing the geographic location to guarantee precise spatial alignment. In parallel, climate data were obtained from the WorldClim global weather and climate databases. The extracted variables included monthly temperature and precipitation averages from 2011 to 2021, at a spatial resolution of 30 s. These variables were selected because of their roles in vector biology and disease transmission dynamics. Precipitation and temperature data were extracted on a municipality/population center basis using QGIS version 3.28. The data were then concatenated, and a combination of the datasets was generated using RStudio. Spatially, data are associated with geographic coordinates. Temporally, the datasets were harmonized by month to account for seasonal trends.
Correlation and distribution analysis
The data analysis was conducted with utmost precision and thoroughness. The geographical distribution of the number of cases for each disease was meticulously shown in a heat map created in QGIS. The cases were assigned to municipalities and nearby geographical points to facilitate differentiation between the concentration points and a high incidence of diseases. Statistical analyses were performed using R Studio. The correlation of the cases with the climatic variables, temperature, and precipitation was performed using Pearson’s correlation, which is a robust statistical method. The relationship between the dependent variable (cases) and independent variables was described using multiple linear regression, providing a comprehensive understanding of the data.
Sociodemographic analysis of disease
Based on the analysis, diseases were consolidated and analyzed according to demographic variables, such as age, sex, and geographic location, using descriptive statistics. Data discrimination was performed according to disease classification. For this analysis, we considered the availability of reports provided by SIVIGILA.
Results
Distribution of dengue, chikungunya, and Zika cases
Between 2011 and 2021, more than 800,000 cases of Dengue, Chikungunya, and Zika were recorded in Colombia. Dengue is characterized by epidemic cycles that occur approximately every three years. 2019 was the most epidemic year, with the most cases historically reported, with around 122,998 cases. The DENV-2 serotype is the most prevalent strain in Colombia’s history of epidemics. Dengue is Colombia’s primary communicable disease and the most significant concern (6).
Chikungunya was introduced in Colombia at the end of 2014, and in 2021, more than 70,000 cases were reported. The year 2015 was the year of the epidemic with approximately 43,787 cases. Zika was introduced in Colombia at the end of 2015; in 2016, 66,960 cases were reported, which corresponded to the epidemic year. It is worth noting that in recent years, Zika and chikungunya have shown a significant decrease in cases, according to SIVIGILA reports, indicating progress in disease control.
Figure 1 shows the geographical distribution of dengue, chikungunya, and Zika diseases during the study period. Historically, dengue has been concentrated in the southwest of the country, particularly in the Valle del Cauca, followed by Antioquia, Norte de Santander, and Bolívar for Dengue, Zika, and Chikungunya. This regional concentration provides a clear picture of the impact of the disease in different parts of the country.
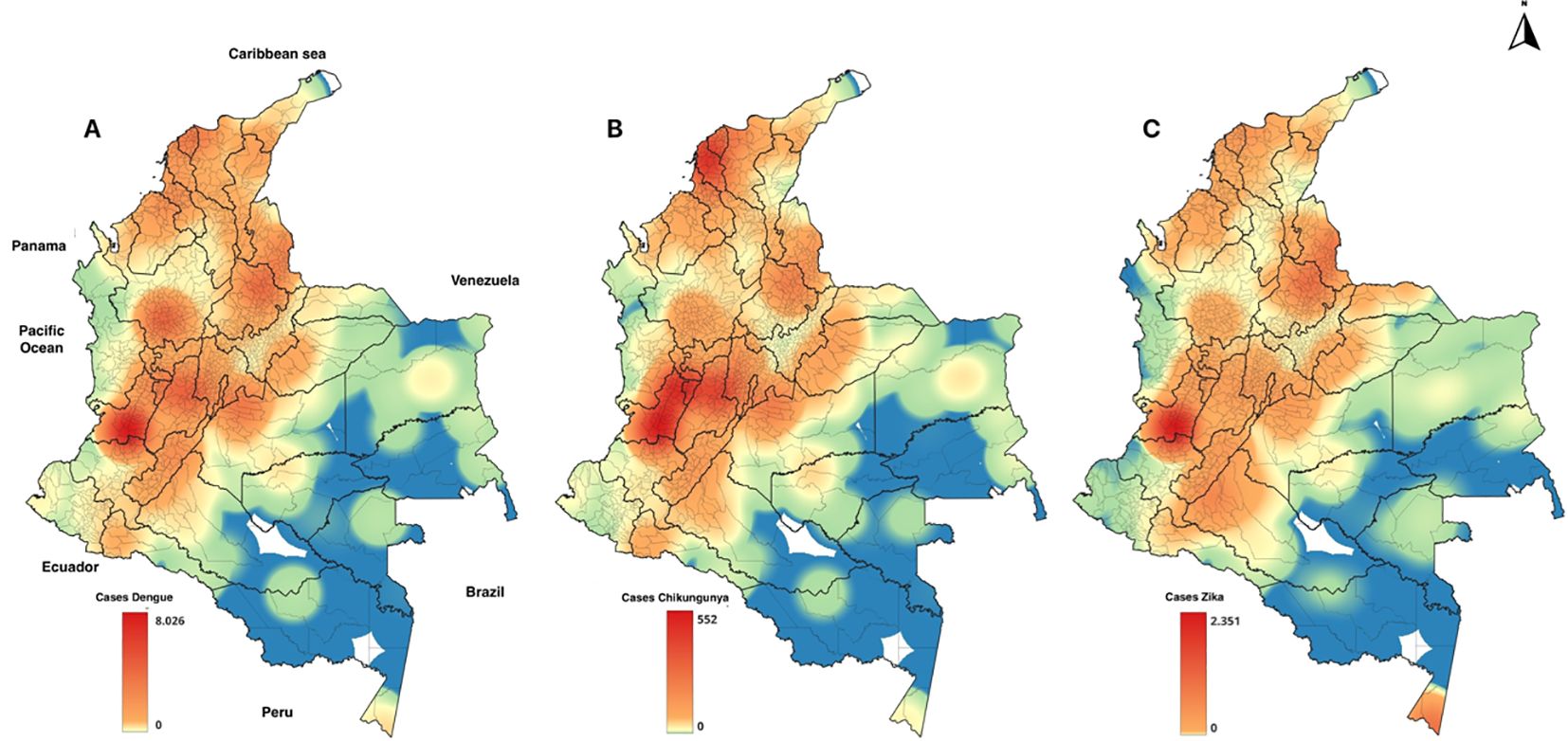
Figure 1. Geographic distribution of the main arboviruses in Colombia, 2011-2021. (A) Dengue (B) Chikungunya (C) Zika.
Distribution of malaria, leishmaniasis, and chagas disease
The distribution of these diseases is challenging. Between 2011 and 2021, approximately 662,012 cases were reported, 51% of which were caused by P. vivax. The data revealed a pattern of fluctuating disease incidence, underscoring the need for continuous monitoring and action. From 2011 to 2014, the malaria incidence decreased from 133.7 cases to 88.7 cases per 100,000 people. However, in 2015 and 2016, the malaria incidence increased, reaching 177.7 cases per 100,000 people at risk. The incidence remained relatively stable in the following years, with values ranging between 114 and 128.5 cases on the same basis as people at risk. Historically, the departments that have reported a more significant number of cases compared to other regions are Chocó, Antioquia, Córdoba, Nariño, Norte de Santander, Vichada, Guainía, Guaviare, and Amazonas (Figure 2).
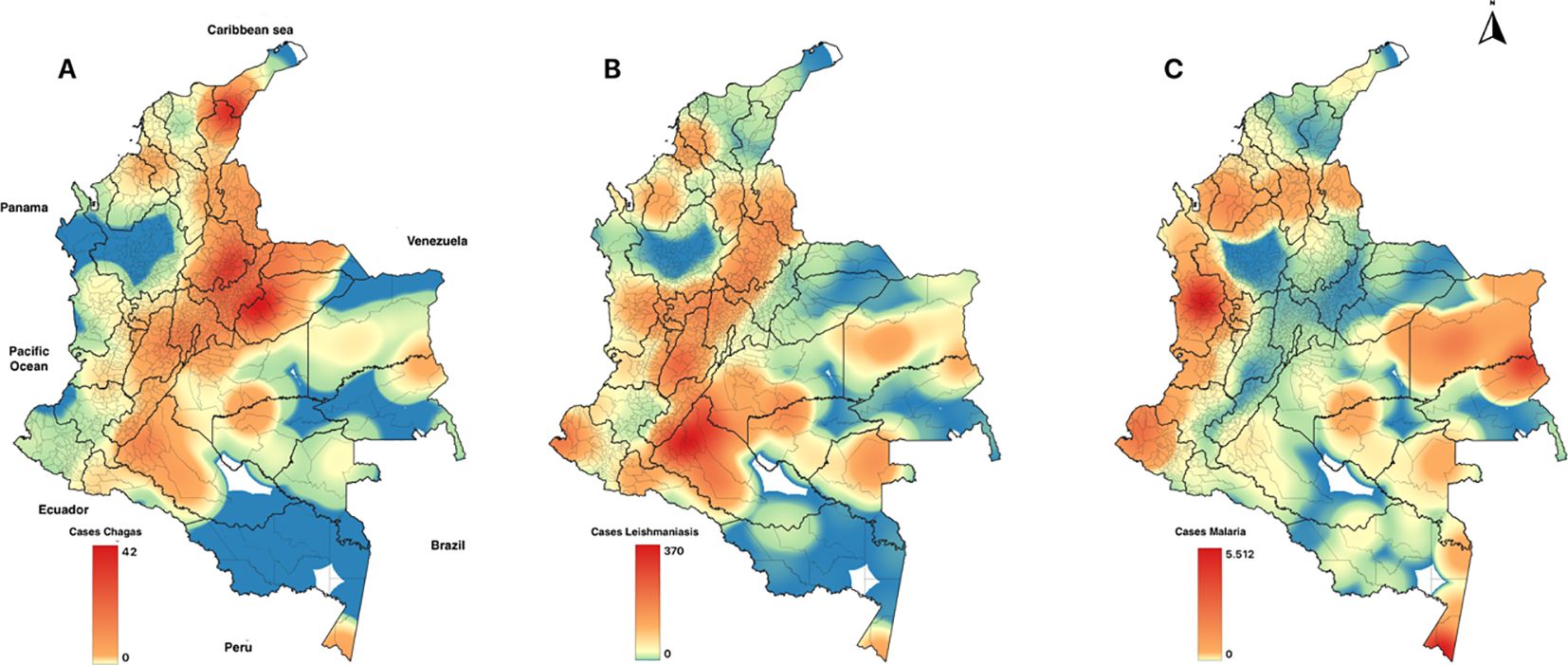
Figure 2. Geographic distribution of the primary parasitic diseases, 2011-2021. (A) Chagas, (B) Leishmania, (C) Malaria.
Regarding the different forms of leishmaniasis (cutaneous, mucocutaneous, and visceral), Colombia reported 117,541 cases between 2011 and 2021. Of the reported cases, 95% were cutaneous leishmaniasis, followed by mucocutaneous (4%) and visceral (1%) leishmaniasis. The number of annual cases increased between 2011 and 2017. As of 2017, these values have remained stable until 2021. Most of these cases were reported in men, particularly in the 20-29 age group, where men accounted for 44.51% of the total cases. Other important age groups were 30-39 years (14.2%) and 10-19 years (10.9%), with a consistently higher incidence among men across all age groups. This demographic breakdown highlights the urgent need for immediate and targeted interventions, especially for adult males who engage in outdoor activities in endemic areas, to curb the spread of the disease.
Colombia’s data on Chagas disease shows improved surveillance and diagnosis. Between 2012 and 2021, 3,785 cases have been reported. Notably, no cases were reported in 2011, indicating a potential gap between surveillance and diagnosis. However, from 2012 to 2020, the incidence of Chagas decreased annually, with figures varying between 0.2 and 1.0 cases per 100,000 inhabitants. Geographically, the cases were mainly concentrated in the departments of La Guajira, Cesar, Santander, and Casanare, bordering Venezuela. This geographical concentration provides a clear picture of the affected areas, aiding targeted interventions. It also served as a testament to the impact of our work on public health.
Precipitation and temperature of arboviruses and parasitosis in Colombia
Our study, as depicted in the geographical distribution of arboviruses (Figure 1), revealed a consistent pattern in the distribution of diseases. We have chosen to focus our reports on the Valle del Cauca department, which has an average temperature of 24.5°C and precipitation of 147 mm annually. This focus allowed us to delve deeper into the local factors that influence these diseases. However, our findings, as shown in the behavior of the cases against precipitation (Figure 3) and Pearson correlation analysis (Table 1), suggest that the climatic variables studied do not play a significant role in the behavior of dengue, Zika, and chikungunya in Colombia.
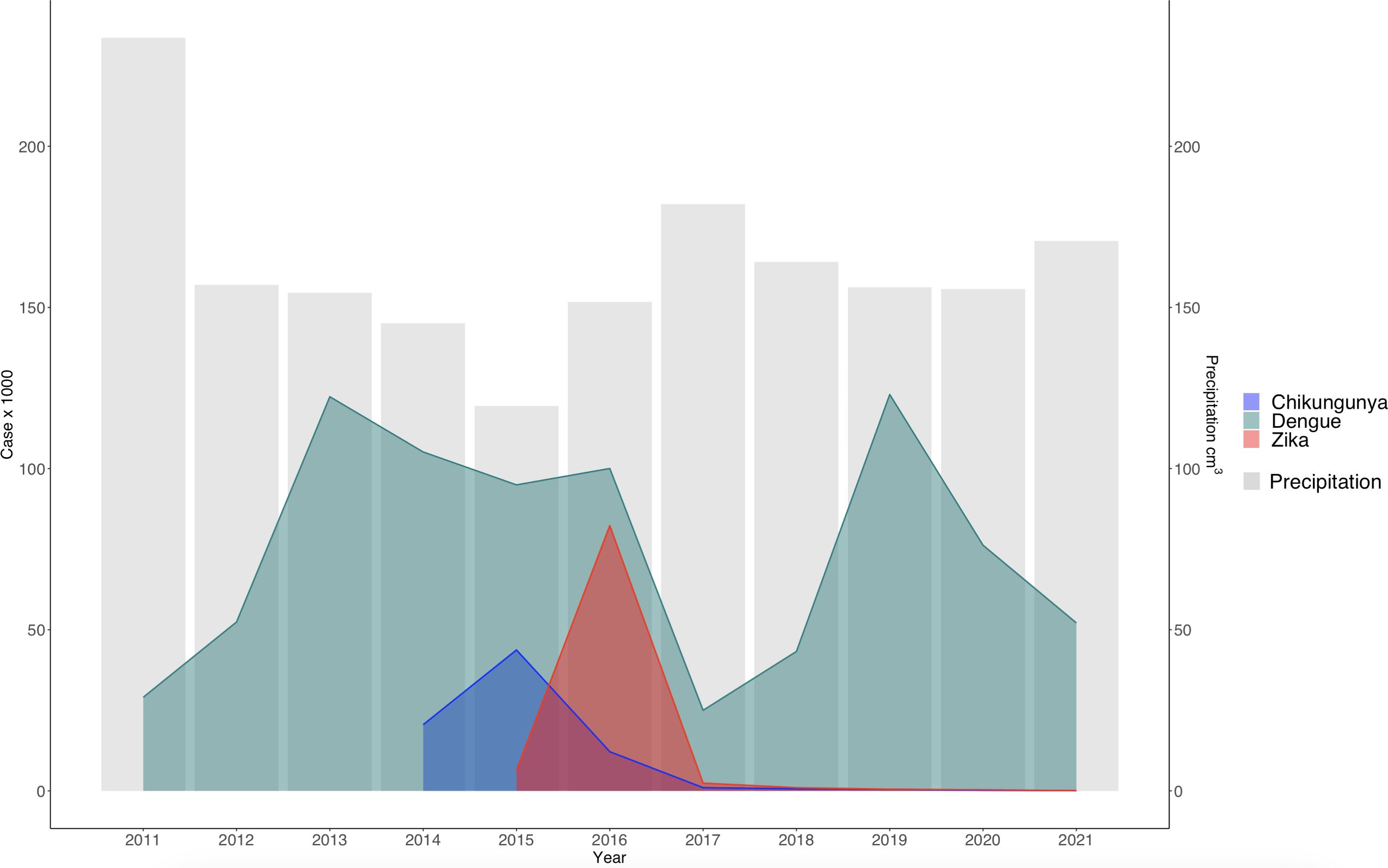
Figure 3. Temporal distribution of dengue, zika, and chikungunya cases versus annual precipitation in Colombia. The bars correspond to average annual precipitation (S2 = 79.6 cm3).
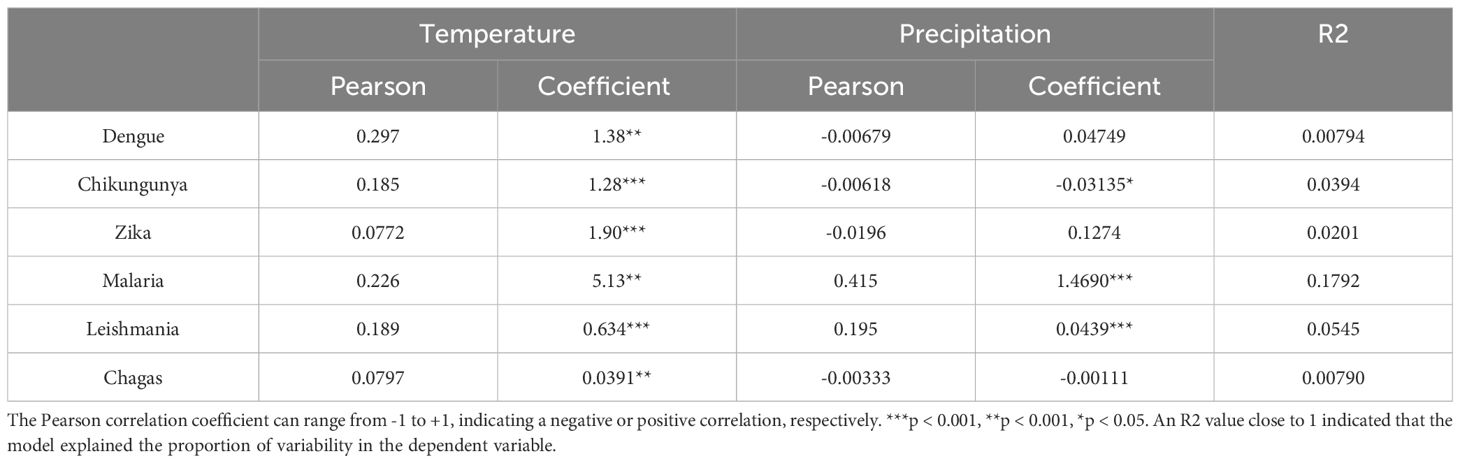
Table 1. Pearson correlation analysis and multiple linear regression for dengue, chikungunya, Zika, malaria, Leishmania, and Chagas cases in Colombia.
Similar to arboviruses, analyses indicated that temperature and precipitation do not directly influence the incidence of leishmaniasis and Chagas disease (Table 1; Figure 4). However, correlation analysis indicated that precipitation influenced the increase in malaria cases (Table 1). This could be because malaria cases occur mainly in regions with high rainfall, such as the Choco department (Figure 2).
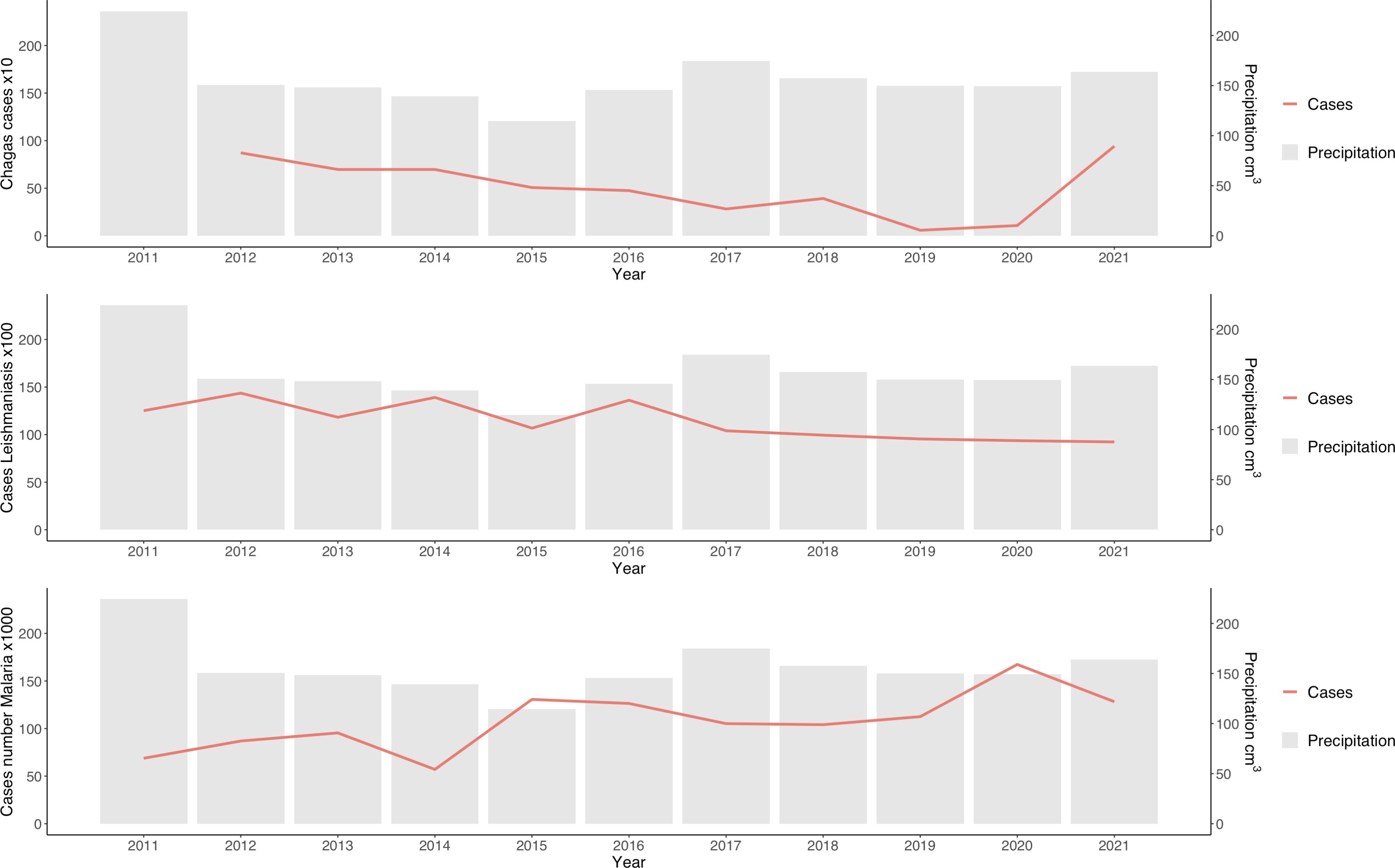
Figure 4. Temporal distribution of leishmaniasis, malaria, and Chagas disease versus annual precipitation in Colombia. The bars correspond to the average annual precipitation (S2 = 79.6 cm3).
Discussion
The research findings revealed that the dengue, chikungunya, and zika outbreaks were concentrated in Valle del Cauca, Antioquia, Atlántico, and Norte de Santander. These geographical patterns were consistent with the results obtained by Castrillón et al. (23). The data suggest that the disease behavior has remained relatively consistent since the initial records, with some variations over the years due to the vector’s geographic expansion (24). However, these departments exhibit distinct ecosystem variations that set them apart. The results also indicated that the climatic variables studied could not directly correlate with the increase in arboviruses in Colombia. For the chikungunya and zika outbreaks, it is believed that the strains were introduced from different countries and entered the Colombian Caribbean (25).
Other authors have reported that the increase in cases of arboviruses could be influenced by the Niño-Southern Oscillation (ENSO) index (26). However, it is essential to highlight that the influence of ENSO on the dynamics of arboviruses is not stationary, as it varies according to time and space. For instance, during El Niño years, increased rainfall and warmer temperatures can create more breeding sites for mosquitoes, leading to a higher risk of arbovirus transmission. In Colombia, regions with high population densities, such as the Pacific and Andean regions, play a crucial role in the dynamic relationship between arboviruses and this index at a national scale. Other authors have stated that the strongest correlation between climate and dengue is observed when combining variables such as time speed, relative humidity, temperature, precipitation, and humidity (27). Therefore, when evaluating climatic variables independently, the increase or decrease in the cases of these diseases at a national scale could not be explained.
Although the variables evaluated in the present work showed that they do not directly influence the increase in cases, some studies indicate that temperature is a determinant in the survival of mosquitoes and their vectorial capacity (28). This would explain the number of cases of arboviruses in Colombian cities with temperatures below 35°C. In response to temperature changes, the genus Aedes adapted by hibernating in shaded areas during daylight hours in hot environments (29). The impact of temperature on the behavior of Aedes mosquitoes is a crucial factor that must be urgently understood. In areas with temperatures above 30°C, the ability of Ae. aegypti to fly decreases, which affects nutrition and host finding (28). In contrast, studies have shown that Ae. albopictus in environments with an average temperature of 27°C increases the transmission efficiency of ZIKV by 57% (30).
Therefore, it is essential to consider the biological cycles of the vectors in future research. The population dynamics of these vectors must be considered to elucidate the behavior and distribution of these diseases. A combination of biological and non-biological factors can determine the transmission patterns of these diseases. In South American countries, the risk of tropical diseases is not solely determined by environmental factors, such as temperature. Human factors, such as violence, migration, and armed conflict, also play an essential role, as do viruses, which increase the capacity of vectors (31). It is essential to consider non-biological factors such as the sociodemographic conditions of the populations and their proximity to the ecological niches of the vectors. These elements are often decisive in determining the disease incidence. Within a city, there may be areas with a higher risk of infection, mainly near bodies of water, that promote mosquito population growth (32).
Regarding the geographic distribution of malaria, it was observed that the departments with the highest prevalence of this disease presented favorable climatic conditions for developing Anopheles mosquitoes. However, these regions also present distinctive ecosystem variations that influence vector dynamics. The unique ecosystem variations in different regions, such as the tropical forests and high humidity in Choco (33) and the savannas and dry forests in Cordoba (34), add a layer of complexity to the understanding of malaria distribution. Stratification of climatic data by department is essential to clarify these relationships. Effective surveillance and control systems in each department, such as prioritization of malaria cases and diagnosis in Chocó by health entities (35), could also contribute. Furthermore, the resistance of P. falciparum to drugs such as chloroquine and amodiaquine further complicates efforts to reduce the number of cases in this region (36).
Furthermore, mining has become an influential factor in the number of malaria cases in the Chocó department (37). Colombia, specifically the Pacific region, is an area where gold extraction points are concentrated. The mobility of unexposed populations to endemic areas in search of work contributes to around 3% of malaria cases (38). In these areas, people work outdoors and are exposed to mosquito bites daily for long periods. Furthermore, these areas often lack adequate healthcare and disease prevention measures (38).
As in previous studies (39), the analyses indicated an association between rainfall and the incidence of malaria. Precipitation has emerged as one of the factors affecting malaria cases (35). The incorporation of precipitation into models has even improved prediction accuracy (40). In this study, the analysis indicates that for every 1 cm³ increase in precipitation, an increase of 1.47 malaria cases is estimated, with a coefficient of 1.469 (p < 0.001). Although rainfall appears to be a key factor in the increase in malaria cases, other associated factors need to be included.
Although precipitation can account for the increase in malaria cases, it is crucial to gauge the model’s reach using the coefficient of determination. R2 yielded a value of 0.1792, indicating that precipitation could elucidate the variability in 18% of the cases. The remaining percentage could be due to other factors, such as vector exposure risk, sociodemographic conditions, and government public health actions (40). However, the impact of climate change on vector distribution may be predictable, and the relationship between temperature and the efficiency of vector-borne pathogen transmission is a complex puzzle, sometimes yielding seemingly contradictory inferences (39).
Conclusion
The study period saw Outbreaks of dengue, chikungunya, and Zika were primarily concentrated in the departments of Valle del Cauca, Antioquia, Atlántico, and Norte de Santander, respectively. Parasitic infections, on the other hand, exhibited more diverse behavior. With the exception of malaria, the distribution of cases did not directly correlate with temperature and precipitation. Climatic variations alone cannot explain the epidemiological patterns of the analyzed diseases. The data from this study point to the need to better understand the factors involved in the transmission of the diseases studied, beyond climatic factors.
Limitations of the study
The primary focus of this study was the association of dengue, Zika, chikungunya, chagas, malaria, and leishmania with climatic variables, such as temperature and precipitation. Although this focus is crucial, it is important to note that it may not fully capture the complexity of the factors that influence the dynamics of these tropical diseases. Sociodemographic aspects, population mobility, and vector resistance, for instance, were not within the study’s scope. However, the findings of this study still hold significant value and impact in understanding the epidemiological dynamics of these diseases in Colombia.
Author contributions
BG: Writing – original draft, Writing – review & editing. DE: Conceptualization, Data curation, Formal analysis, Investigation, Methodology, Supervision, Writing – original draft, Writing – review & editing. LS: Writing – review & editing. YE: Data curation, Investigation, Methodology, Writing – original draft, Writing – review & editing. YG: Conceptualization, Data curation, Formal analysis, Investigation, Methodology, Software, Supervision, Visualization, Writing – original draft, Writing – review & editing. YL: Conceptualization, Data curation, Formal analysis, Investigation, Methodology, Software, Supervision, Writing – original draft, Writing – review & editing. MY: Investigation, Resources, Writing – review & editing. GA: Writing – review & editing. SM: Writing – review & editing.
Funding
The author(s) declare that no financial support was received for the research, authorship, and/or publication of this article.
Acknowledgments
To the University of Cordoba, Colombia. The present manuscript is part of a postgraduate microbiology course.
Conflict of interest
The authors declare that the research was conducted in the absence of any commercial or financial relationships that could be construed as a potential conflict of interest.
Publisher’s note
All claims expressed in this article are solely those of the authors and do not necessarily represent those of their affiliated organizations, or those of the publisher, the editors and the reviewers. Any product that may be evaluated in this article, or claim that may be made by its manufacturer, is not guaranteed or endorsed by the publisher.
References
1. Wilson AL, Courtenay O, Kelly-Hope LA, Scott TW, Takken W, Torr SJ, et al. The importance of vector control for the control and elimination of vector-borne diseases. PLoS Negl Trop Dis. (2020) 14:1–31. doi: 10.1371/journal.pntd.0007831
2. Schaub GA, Vogel P, Balczun C. Parasite-vector interactions. Mol Parasitology: Protozoan Parasites their Molecules. (2016), 431–89. doi: 10.1007/978-3-7091-1416-2_14/COVER
3. Brindha J, Balamurali MM, Chanda K. An overview on the therapeutics of neglected infectious diseases—Leishmaniasis and Chagas diseases. Front Chem. (2021) 9:622286/BIBTEX. doi: 10.3389/FCHEM.2021.622286/BIBTEX
4. Kotepui M, Kotepui KU, De Jesus Milanez G, Masangkay FR. Plasmodium spp. mixed infection leading to severe malaria: a systematic review and meta-analysis. Sci Rep. (2020) 10:1–12. doi: 10.1038/s41598-020-68082-3
5. Castillo-Macías A, Salinas-Carmona MC, Torres-López E. Immunology of viral infections with a high impact in Mexico: Dengue, Chikungunya, and Zika. Medicina Universitaria. (2017) 19:198–207. doi: 10.1016/J.RMU.2017.09.001
6. Adams LE, Martin SW, Lindsey NP, Lehman JA, Rivera A, Kolsin J, et al. Epidemiology of dengue, Chikungunya, and Zika virus disease in U.S. States and territories 2017. Am J Trop Med Hyg. (2019) 101:884–90. doi: 10.4269/AJTMH.19-0309
7. Mattar S, Ojeda C, Arboleda J, Arrieta G, Bosch I, Botia I, et al. Case report: microcephaly associated with Zika virus infection, Colombia. BMC Infect Dis. (2017) 17:1–4. doi: 10.1186/S12879-017-2522-6
8. Méndez N, Oviedo-Pastrana M, Mattar S, Caicedo-Castro I, Arrieta G. Zika virus disease, microcephaly and Guillain-Barré syndrome in Colombia: epidemiological situation during 21 months of the Zika virus outbreak 2015-2017. Arch Public Health. (2017) 75. doi: 10.1186/S13690-017-0233-5
9. Martinez JD, de la Garza JAC, Cuellar-Barboza A. Going viral 2019: Zika, Chikungunya, and dengue. Dermatol Clin. (2019) 37:95–105. doi: 10.1016/j.det.2018.07.008
10. Judson SD, Rabinowitz PM. Zoonoses and global epidemics. Curr Opin Infect Dis. (2021) 34:385–92. doi: 10.1097/QCO.0000000000000749
11. Centers for Disease Control and Prevention. Locally acquired Malaria cases identified in the United States (2023). Available online at: https://emergency.cdc.gov/han/2023/han00494.asp (Accessed September 29, 2024).
12. Centers for Disease Control and Prevention. Zika cases in the United States (2024). Available online at: https://www.cdc.gov/zika/zika-cases-us/index.html:~:text=There%20is%20no%20current%20local,reported%20in%20Alaska%20and%20Hawaii (Accessed September 29, 2024).
13. Centers for Disease Control and Prevention. Chikungunya cases in the United States (2024). Available online at: https://www.cdc.gov/chikungunya/data-maps/chikungunya-us.html (Accessed September 29, 2024).
14. Centers for Disease Control and Prevention. Data and Statistics on Dengue in the United States (2024). Available online at: https://www.cdc.gov/dengue/data-research/facts-stats/index.html (Accessed September 29, 2024).
15. Reiter P. Climate change and mosquito-borne disease. Environ Health Perspect. (2001) 109:141–61. doi: 10.1289/EHP.01109S1141
16. Martin V, Chevalier V, Ceccato P, Anyamba A, De Simone L, Lubroth J, et al. The impact of climate change on the epidemiology and control of Rift Valley fever. Rev Sci tech. Off Int Epiz. (2008) 27:413–26. doi: 10.20506/rst.27.2.1802
17. Mikery Pacheco OF, Moo Llanes DA, Rebollar Téllez EA, Castillo Vera A. Influencia del cambio climático sobre la transmisión de leishmaniasis en Latinoamérica y el estatus de la investigación en México. Biomédica. (2023) 24:42–50. doi: 10.32776/revbiomed.v34i1.1006
18. Agyekum TP, Botwe PK, Arko-Mensah J, Issah I, Acquah AA, Hogarh JN, et al. A systematic review of the effects of temperature on anopheles mosquito development and survival: implications for malaria control in a future warmer climate. (2021) 18. doi: 10.3390/ijerph18147255
19. Ribeiro LM, Oshiro ET, de Souza DA, Fernandes MF, dos Santos KM, Mateus NLF, et al. Biological aspects of immature stage of Nyssomyia whitmani (Antunes and Coutinho) (Diptera, Psychodidae, Phlebotominae) in laboratory conditions. Rev Bras Entomol. (2015) 59:100–3. doi: 10.1016/J.RBE.2015.02.012
20. Badel J, Rodríguez L, Parra G. Análisis espacio-temporal de las condiciones biofísicas y ecológicasde Triatoma dimidiata (Hemiptera: Reduviidae: Triatominae)en la region nororiental de los Andes de Colombia. Biomédica. (2017) 37:106–23. doi: 10.7705/BIOMEDICA.V34I2.3496
21. Sistema de Vigilancia en Salud Pública (SIVIGILA). Boletín Epidemiológico. Available online at: https://www.ins.gov.co/buscador-eventos/Paginas/Vista-Boletin-Epidemilogico.aspx (Accessed February 10, 2024).
22. Fick SE, Hijmans RJ. WorldClim 2: new 1-km spatial resolution climate surfaces for global land areas. Int J Climatology. (2017) 37:4302–15. doi: 10.1002/JOC.5086
23. Castrillón JC, Castaño JC, Urcuqui S. Dengue en Colombia: diez años de evolución. Rev Chil infectología. (2015) 32:142–9. doi: 10.4067/S0716-10182015000300002
24. Rico-Mendoza A, Porras-Ramírez A, Chang A, Encinales L, Lynch R. Co-circulation of dengue, chikungunya, and Zika viruses in Colombia from 2008 to 2018. Rev Panam Salud Publica. (2019) 43. doi: 10.26633/RPSP.2019.49
25. Villero-Wolf Y, Mattar S, Puerta-González A, Arrieta G, Muskus C, Hoyos R, et al. Genomic epidemiology of Chikungunya virus in Colombia reveals genetic variability of strains and multiple geographic introductions in outbrea. Sci Rep. (2019) 9:1–11. doi: 10.1038/s41598-019-45981-8
26. Muñoz E, Poveda G, Arbeláez MP, Vélez ID. Spatiotemporal dynamics of dengue in Colombia in relation to the combined effects of local climate and ENSO. Acta Trop. (2021) 224:106136. doi: 10.1016/J.ACTATROPICA.2021.106136
27. Cassab A, Morales V, Mattar S. Climatic factors and cases of Dengue in Monteria, Colombia: 2003-2008. Rev Salud Pública. (2011) 13:115–28. doi: 10.1590/S0124-00642011000100010
28. Adachi A, Johnson N, Huang Y-JS, Kirk D, Failloux A-B, Bellone R. The role of temperature in shaping mosquito-borne viruses transmission. Front Microbiol. (2020) 11:584846. doi: 10.3389/fmicb.2020.584846
29. Ebi KL, Nealon J. Dengue in a changing climate. Environ Res. (2016) 151:115–23. doi: 10.1016/j.envres.2016.07.026
30. Onyango MG, Bialosuknia SM, Payne AF, Mathias N, Kuo L, Vigneron A, et al. Increased temperatures reduce the vectorial capacity of Aedes mosquitoes for Zika virus. Emerg Microbes Infect. (2020) 9:67–77. doi: 10.1080/22221751.2019.1707125
31. Rückert C, Ebel GD. How do virus–mosquito interactions lead to viral emergence? Trends Parasitol. (2018) 34:310–21. doi: 10.1016/j.pt.2017.12.004
32. Crocker W, Maute K, Webb C, French K. Mosquito assemblages associated with urban water bodies; implications for pest and public health threats. Faculty Science Med Health - Papers: Part A. (2017) 162:115. doi: 10.1016/j.landurbplan.2017.02.006
33. Rodríguez JCP, Uribe GÁ., Araújo RM, Narváez PC, Valencia SH. Epidemiology and control of malaria in Colombia. Mem Inst Oswaldo Cruz. (2011) 106:114–22. doi: 10.1590/S0074-02762011000900015
34. Gutiérrez LA, Gómez GF, González JJ, Castro MI, Luckhart S, Conn JE, et al. Microgeographic genetic variation of the malaria vector anopheles darlingi root (Diptera: Culicidae) from Córdoba and Antioquia, Colombia. Am J Trop Med Hyg. (2010) 83:38. doi: 10.4269/AJTMH.2010.09-0381
35. Olivera MJ, Guerra AP, Cortes LJ, Horth RZ, Padilla J, Novoa J, et al. Artemether-Lumefantrine Efficacy for the Treatment of Uncomplicated Plasmodium falciparum Malaria in Choco, Colombia after 8 Years as First-Line Treatment. Am J Trop Med Hyg. (2020) 102:1056–63. doi: 10.4269/ajtmh.19-0954
36. Osorio L. El control de la malaria en la costa Pacífica Colombiana. Biomédica. (2006) 26:313–6. doi: 10.7705/BIOMEDICA.V26I3.350
37. Shanks GD, Wongsrichanalai C. Mining-associated malaria epidemics. Am J Trop Med Hyg. (2022) 106:33. doi: 10.4269/AJTMH.21-0747
38. Recht J, Siqueira AM, Monteiro WM, Herrera SM, Herrera S, Lacerda MVG. Malaria in Brazil, Colombia, Peru and Venezuela: current challenges in malaria control and elimination. Malaria J. (2017) 16:1–18. doi: 10.1186/S12936-017-1925-6
39. Krefis AC, Schwarz NG, Krüger A, Fobil J, Nkrumah B, Acquah S, et al. Modeling the relationship between precipitation and malaria incidence in children from a Holoendemic area in Ghana. Am J Trop Med Hyg. (2011) 84:285. doi: 10.4269/AJTMH.2011.10-0381
Keywords: public health, epidemiology, arbovirus, climate change, tropical medicine
Citation: Gastelbondo-Pastrana B, Echeverri-De la Hoz D, Sanchez L, García Y, Espitia-Delgado Y, Lopez Y, Yasnot-Acosta MF, Arrieta G and Mattar S (2024) Climatic variables and their relationship with vector-borne disease cases in Colombia, 2011-2021. Front. Trop. Dis 5:1481991. doi: 10.3389/fitd.2024.1481991
Received: 17 August 2024; Accepted: 18 October 2024;
Published: 14 November 2024.
Edited by:
Hassen Mamo, Addis Ababa University, EthiopiaReviewed by:
Lara Ferrero Gómez, Universidade Jean Piaget de Cabo, Cabo VerdeCharles Mbogo, Kenya Medical Research Institute (KEMRI), Kenya
Copyright © 2024 Gastelbondo-Pastrana, Echeverri-De la Hoz, Sanchez, García, Espitia-Delgado, Lopez, Yasnot-Acosta, Arrieta and Mattar. This is an open-access article distributed under the terms of the Creative Commons Attribution License (CC BY). The use, distribution or reproduction in other forums is permitted, provided the original author(s) and the copyright owner(s) are credited and that the original publication in this journal is cited, in accordance with accepted academic practice. No use, distribution or reproduction is permitted which does not comply with these terms.
*Correspondence: Salim Mattar, c21hdHRhckBjb3JyZW8udW5pY29yZG9iYS5lZHUuY28=