- 1Laboratory of Immunology of Infectious and Parasitic Diseases and Obesity, Department of Parasitology, Microbiology, and Immunology, Federal University of Juiz de Fora, Juiz de Fora, Brazil
- 2Laboratory of Molecular Pathology of Cancer, Faculty of Health Sciences and Medicine, University of Brasilia, Brasilia, Brazil
Introduction: It is well known that dietary changes have a significant impact on the immune system, and modifications in lipid balance may contribute to disease progression in several cases. Malaria is still a major global health concern, and the development of the disease has already been linked to the host’s nutritional status, so it’s critical to understand how environmental factors, such as dietary variations, can influence the outcome of infection. We therefore investigated the effect of a short-term diet in a murine model of experimental cerebral malaria.
Methods: For this, male C57BL/6 mice were fed a high fat diet containing 60% of the calories from lipids for 5 days. Following this period, the animals were infected with Plasmodium berghei ANKA, and parasitemia, survival, and neurological scores were compared. Considering that one of the first elimination routes of the intracellular parasite is oxidative stress, the antioxidant N-acetylcysteine was administered to assess whether the protection would be reversed.
Results and discussion: Animals fed a hyperlipidic diet reacted the same way to infection even after NAC administration. Unlike the control group, which died after eight days of infection with roughly 7% parasitized red blood cells, the hyperlipidic diet group was resistant to infection, with no clinical signs and no increase in blood parasitemia. Several proinflammatory cytokines such as TNF-α IFN-γ and IL-6 were increased in the spleen of both infected groups, regardless of their diet. The provision of a high-fat diet to mice for as little as 5 days completely prevents Plasmodium berghei ANKA infection in C57BL/6 mice, while the treatment of an antioxidant failed to reverse the parasite protection.
1 Introduction
Malaria remains a significant public health problem in the world. Considered one of the most prominent global health parasitic diseases. Malaria is responsible for more than 627,000 deaths worldwide in 2020; in the same year, approximately 241 million cases were registered. Approximately 40% of the world’s population is at risk of transmitting the disease. According to individual characteristics of the host, such as age, immune status, and genetic factors, infection with Plasmodium falciparum can progress to severe malaria, characterized by sequestration and adhesion of infected erythrocytes in vital organs such as the lungs and brain, leading to inflammatory responses in these sites that can be fatal (1). The pathogenesis of cerebral malaria (CM) is still not completely understood, but high levels of circulating proinflammatory cytokines are associated with the pathogenesis of CM in murine models and human studies and increased mortality (1–3). At the same time, many studies show the role of proinflammatory cytokines in the resolution of the infection in both types of hostsl (1). A deficient proinflammatory response can lead to the persistence and replication of the parasites while exacerbating this response can lead to immunopathological responses such as CM. Inflammatory mediators are repeatedly implicated in disease severity, leading to the belief that severe malaria is, at least in part, an immune-mediated disease, making it an exciting target of immunological studies (4, 5). In mice, CM is characterized by a complex cascade of events, including the breakdown of the blood-brain barrier, sequestration of parasitized red blood cells, accumulation of white blood cells in the brain, and production of pro-inflammatory cytokines (6–8). One of the most critical steps in developing CM is the sequestration of parasitized erythrocytes. The fundamental role of the CD36 receptor in the interaction with Plasmodium berghei ANKA proteins and its involvement in this sequestration has already been demonstrated. In addition, this receptor is also involved in resistance to mortality during uncomplicated malaria (9).
CD8+ T cells are also fundamental in the pathogenesis of CM. In mice, the number of these cytotoxic cells that infiltrate the brain during infection is high, which contributes to changes in the permeability of the blood-brain barrier through perforin-dependent mechanisms (10, 11). CM caused by P. falciparum in humans shares many characteristics with that caused by P. berghei ANKA in mice and is used as a comparison due to its ability to sequester red blood cells in the microcirculation. Infecting susceptible mice causes the typical symptoms and signs of disease in humans.
The relationship between obesity and diseases characteristic of first-world countries, such as heart disease, cancer, type 2 diabetes, asthma, and hypertension, is already well described (12). However, the relationship between obesity and significant pathogens that affect developing countries is still poorly studied. Malaria is a disease that affects countries with low human development index (HDI) and harms social and economic stability (4). Thus, considering the progressive growth of obesity throughout the world, the high mortality and morbidity rates associated with this disease, and the impacts it causes on the immune system, studies that assess the relationship of this metabolic condition in the immune response in infectious diseases are sorely needed. There are still few studies relating obesity and parasitic infections, which affect thousands of people in developing countries. With globalization, changing habits, and easy access to fatty foods, low-income countries are now concerned not only with malnutrition but also with obesity and are facing infections in this context (13–15). Existing studies show that each infection, with its particularities, can be affected differently by obesity or a high-fat diet. In the case of short-term diet consumption, studies are even scarcer. However, it has already been demonstrated that the high-fat diet increased the susceptibility of mice to Giardia duodenalis and Listeria monocytogenes, highlighting the imbalance of the microbiota during giardiasis (16, 17).
Therefore, the present study aimed to evaluate the role of long term and short term high-fat diet consumption associated with cerebral malaria complications.
2 Materials and methods
2.1 Animals and diets
Male C57BL/6 mice (6-8 weeks old) were obtained from the Reproductive Biology Center of Federal University of Juiz de Fora (UFJF), Minas Gerais, Brazil. The animals were housed in cages in temperature-controlled rooms and received water ad libitum and received a high-fat diet (HFD) or standard chow diet (SCD) for 12 weeks (LT-HFD) or 5 days (ST-HFD). The high-fat diet was produced at the Immunology Laboratory to obtain a percentage of 60% of fat, maintaining the equivalent of proteins, vitamins, minerals, and fibers of the standard ratio of the controls (NUVILAB®).
To collect blood and organs for subsequent analysis, experimental mice were first anesthetized with 150 µL of a 60 mg/kg ketamine and 8 mg/kg xylazine solution in a 0.9% saline solution administered intraperitoneally, then euthanasia was then performed by cervical dislocation. All procedures were performed with the approval of the Animal Ethics Committee of the UFJF (reference number 019/2018).
2.2 Evaluation of body weight
Body weight was evaluated after 12 weeks of dietary consumption. The delta body weight was represented by weighing the diet offered at the beginning and end of each week. The difference between the offered diet and the remainder not consumed was considered as weekly consumption. The animals were weighed weekly on a semi-analytical scale to assess weight gain. On the day before euthanasia, the animals were weighed on a precision scale to obtain the final body weight. Obesity was assessed based on weight gain, perigonadal fat, and Lee index (cube root of body weight (g) divided by naso-anal length (cm)).
2.3 Experimental infection
After 12 weeks (LT-HFD) or 5 days (ST-HFD) of feeding on a high-fat or standard diet (SCD), mice were infected with Plasmodium berghei ANKA. To ensure the viability of the infection, two mice, previously infected with a frozen strain of P. berghei ANKA were euthanized by anesthesia, and their blood was used to infect experimental groups when parasitemia reached 7%. The animals were infected with intraperitoneal injection of 106 parasitized red blood cells, while the controls were injected with saline solution. Parasitemia was assessed by microscopy every 24 h after infection. To evaluate the impact of consumption high fat diet on the course of infection with P.berghei ANKAGFP or NK65, a strain that induces severe malaria in C57BL/6, they were monitored by microscopy daily until 30 days after infection and survival was monitored daily.
2.4 Parasitemia and evaluation of symptoms of CM
The parasitemia were monitored every 24 h by from caudal blood smears. After infection, smears were taken daily from the third day of infection to measure the parasitemia of the animals. Blood samples were obtained by making a small incision at the tip of the tail of the mice. Blood smears were then made and stained with a rapid panoptic staining kit. For the determination of parasitemia, the percentage of parasitized red blood cells out of a total of 1000 red blood cells counted under light microscopy at 100x magnification was considered. To perform an evaluation of symptoms of cerebral malaria, each mouse was subjected to the Rapid Murine Coma Behavior Scale (RMCBS) (Table 1) described by Carroll et al. (18), which is a tool for identifying subjects with cerebral malaria (CM). The scale consists of 10 behavioral parameters: gait, balance, motor performance, body position, limb strength, touch escape, pinna reflex, toe pinch, aggression, and grooming. Each parameter was scored from 0 to 2, with a total score of 20. In the present study, the method was employed to compare disease progression between obese mice and controls and to determine the appropriate time for euthanasia.
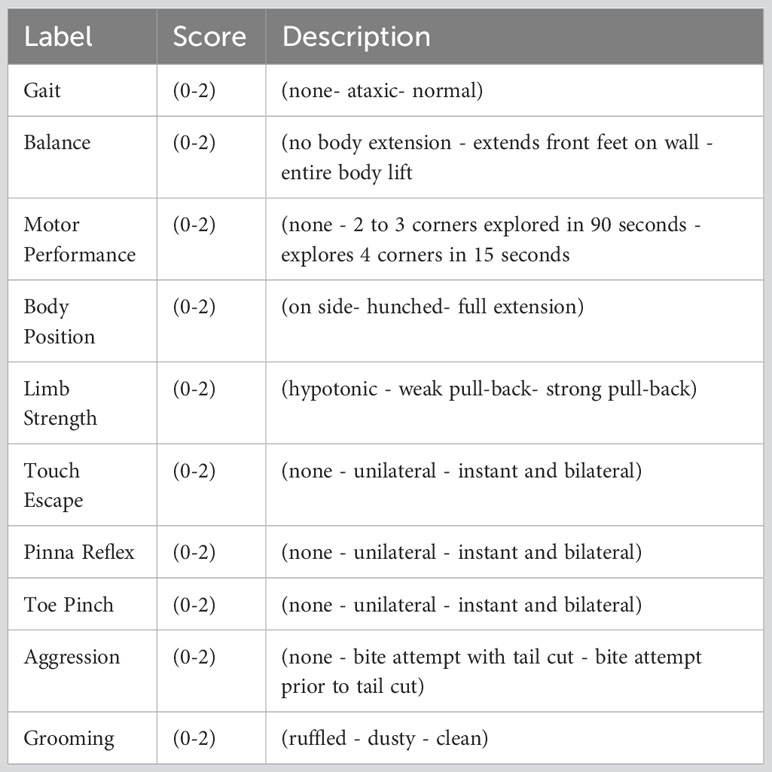
Table 1 Rapid murine coma and behavior scale (RMCBS) to evaluate mice clinical symptoms according to Carroll, R., 2010 (18).
2.5 Evaluation of cerebral vascular permeability
To compare the cerebral vascular permeability between the animals of the control group and the obese group, the integrity of the vascular endothelium of the brain of the infected animals was evaluated by the Evans blue staining method when the animals of the control group presented a score of the rapid scale of behavior and murine coma lower than 5. For this purpose, mice were anesthetized with 150uL of 60 mg/kg ketamine, 8mg/kg xylazine solution in 0.9% saline solution intraperitoneally. Evans blue 2% dye was diluted in 0.9% saline and injected via retro orbital route. After 50 minutes the mice were euthanized by excess anesthetic, perfused and the brains collected and photographed. The dye when injected into the bloodstream binds to plasma proteins, mainly albumin. If there is a change in the integrity of the vascular endothelial wall the dye leaks from the vascular lumen and attaches to the interstitial tissue.
2.6 Cytokine levels
The concentration of leptin in the serum was determined using ELISA (R&D Systems), following the manufacturer’s protocol recommendations. The optical density reading was taken using a microplate reader (SPECTRAMAX 190, Molecular Devices) at 450 nm. The concentrations obtained were calculated from the standard curve, which was generated using different concentrations of the recombinant antibody. The other cytokine levels were determined by flow cytometry using aliquots of murine brain and spleen macerated with cytokine extraction solution after the experimental period. The CBA Kit (Cytometric Bead Array Mouse Th1/Th2/Th17 Cytokine Kit - BD®) was used in this study to quantify the cytokines IL-6, IFN-γ, TNF-α, IL-2, IL-4, IL-10, IL-17A in the same sample according to the manufacturer’s instructions. The results were generated in graphs and tables using FlowJo software.
2.7 Flow cytometry
Single-cell suspensions of splenocytes, and adipose SVF were harvested and 106 cells per well plated to perform cell labeling with the following antibodies: anti-CD8, anti-CD3, anti CD25, anti FOXP3 and anti NK-1.1.Cells were fixed in 1% paraformaldehyde and acquired on an BD FACSCanto II TM cytometer and data analyzed using FlowJo v10 (LLC) software.
2.8 RNA, cDNA and RT-qPCR
Adipose Tissue were homogenized in Invitrogen™ TRIzol™ Reagent and then total RNA extracted using an optimized chloroform method (19). The mRNA was reverse transcribed into cDNA using High-Capacity RNA-to-cDNA™ Kit (Applied Biosystems™). Quantitative real-time PCR was performed using SYBR® Green Kit, Fold changes in the mice groups were analyzed using the 2 −ΔΔCT method. The β-actin gene was used as a housekeeping gene, as inner reference (ΔCt). The oligonucleotide primers used in qPCR experiments were: Pb18S rRNA- AAGCATTAAATAAAGCGAATACATCCTTAC; GGAGATTGGT TTTGACGTTTATGTG β-actina- CCACCATGTACCCAGGCATT ACGCAGCTCTCAGTAACAGT CD36- ATTCCCTTGGCAACCAACCA CGTGGCCCGGTTCTAATTCA.
2.9 Statistical analysis
Statistical analysis was conducted using GraphPad Prism 9.0 statistical software. The data were presented as means and standard error (Mean ± SEM), differences were compared using a one-way ANOVA test, survival analysis was performed using the Kaplan-Meier log-rank test and Student’s t-test was used for nominal variables. P-values <0.05 were considered significant.
3 Results
3.1 Long-term hyperlipidic diet effects on weight gain and leptin levels in mice
The weekly weight variation of the animals was evaluated and beginning in the fifth week a statistically significant weight difference was observed between the animals (Figure 1A). It was discovered that the amount of food consumed in grams by obese animals was lower indicating that the increase in diet was not due to hyperphagia but rather its composition (Figure 1B), a significant difference was found between Lee’s index of mice of LT-FHD and SDC (Figure 1C), the perigonadal adipose tissue of the obese animals was significantly heavier than that of the animals fed a standard diet (Figure 1D). Leptin is one of the hormones most strongly associated with obesity, and it has been demonstrated to be directly proportional to adiposity (20). Consistent with this, an increase in serum leptin concentration was observed in the adipose tissue of LT-HFD when compared to SDC (Figure 1E).
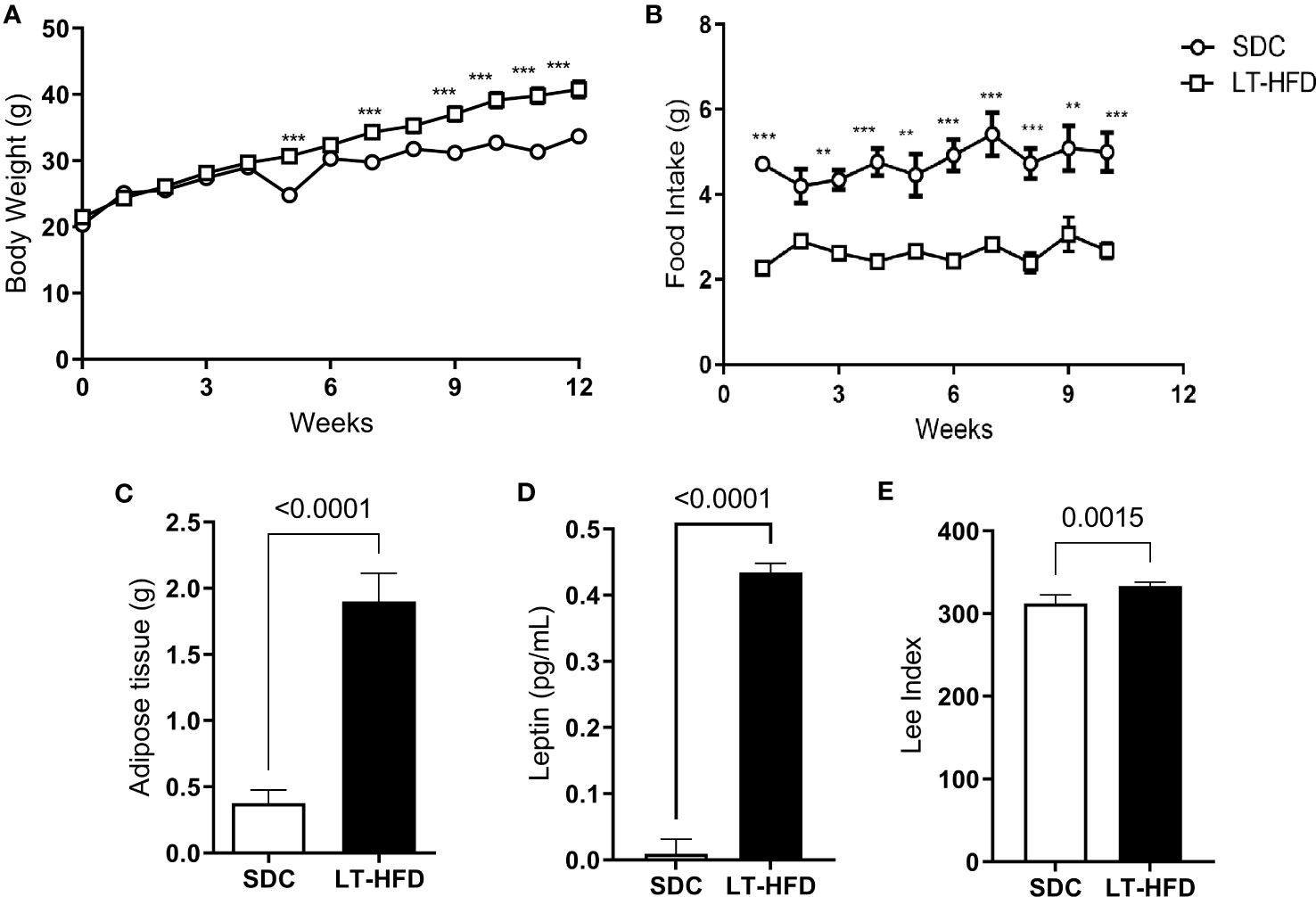
Figure 1 Evaluation of the model of obesity induction by a long-term consumption hyperlipidic diet in C57BL/6 mice over a period of 12 weeks. (A) Evolution of the animals’ weight for 12 weeks (SDC n = 20; LT-HFD n = 20). (B) Evolution of the animals’ feed intake (SDC n = 10; LT-HFD n = 10). (C) Weight of perigonadal adipose tissue after twelve weeks of diet (SDC n = 6; LT-HFD n = 10). (D) Serum leptin concentration in C57BL/6 mice subjected to standard and hyperlipidic diet after twelve weeks of treatment (SDC n = 4; LT-HFD n = 4). (E) Lee index (SDC n = 10; LT-HFD n = 8). Results are expressed as mean ± standard error of the mean. Groups were compared using Student’s unpaired t-test, adopting a significance level of 5% (p < 0.05). SDC, Standard chow diet; LT-HFD, Long term high fat diet. ** Significantly different from control (p < 0.005), *** Significantly different from control (p < 0.001).
3.2 A high-fat diet fed to mice long-term provides protection against Plasmodium berghei ANKA infection but not Plasmodium berghei NK65 infection
Mice were infected after 12 weeks of diet by different inoculum routes. In addition to intraperitoneal infection, retro orbital venous infection was also tested to confirm that protection against Plasmodium was not due to a physical barrier caused by excess adiposity in the peritoneal tissue (Figure 2A). The number of parasites circulating in the blood of mice fed chow diet was greater than in obese animals (Figure 2B) as can be observed with the micrographs (Figure 2C), this consistently ensured survival of obese animals, resulting in complete survival in the obese animals tested (Figure 2D).
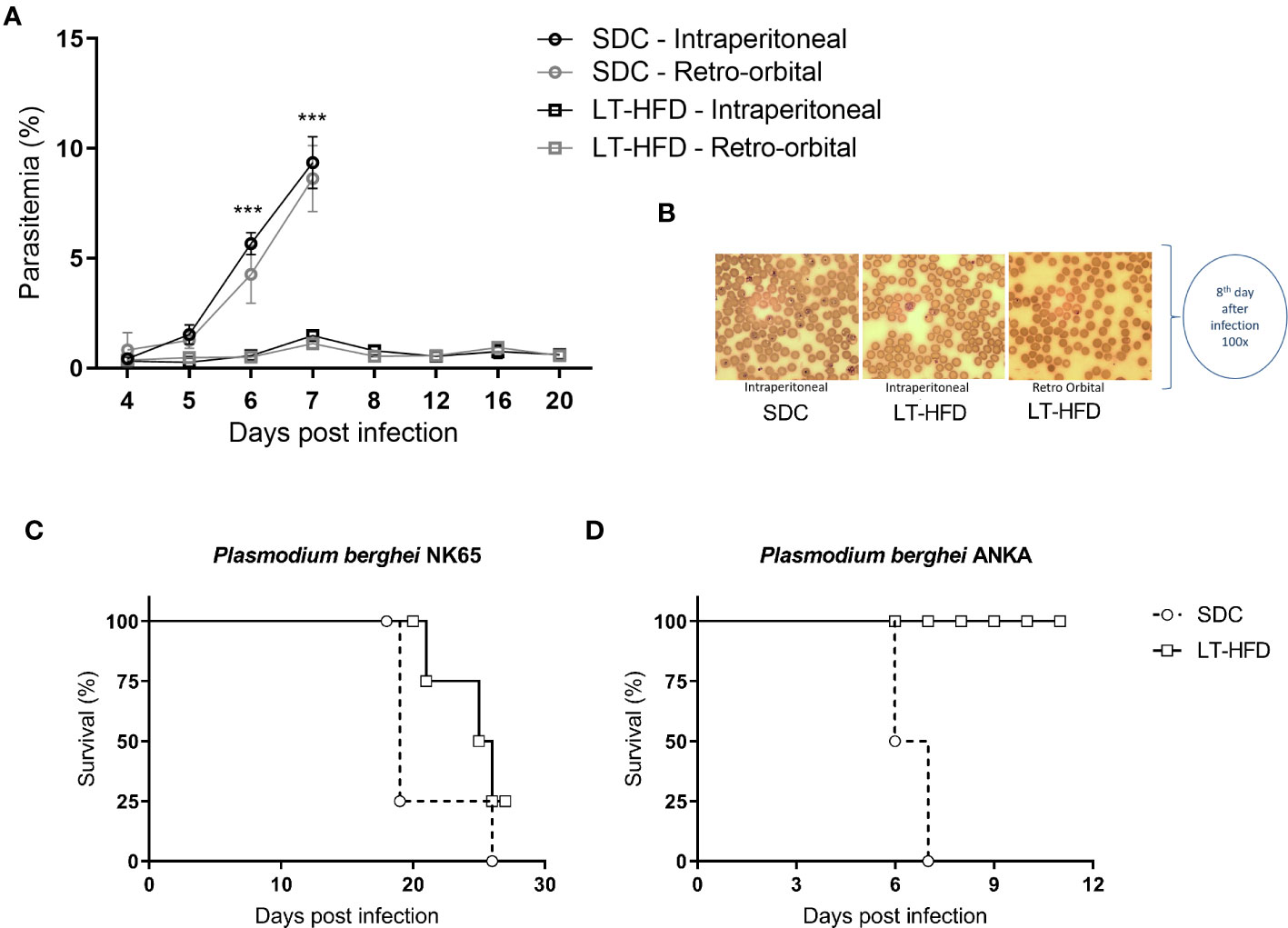
Figure 2 Parasitemia and development of cerebral malaria were studied in mice infected with different strains of P. berghei and subjected to either a standard or hyperlipidic diet. (A) Parasitemia was monitored in C57BL/6 mice infected with P. berghei via different routes and subjected to different diets over a period of 20 days. (B) Blood smears were taken from mice subjected to different diets and infected with P. berghei via different routes after 8 days of infection (100x). (C) The survival rate of C57BL/6 mice infected with P. berghei ANKA and subjected to different diets (SDC n=8, LT-HFD n=8) was recorded as a percentage. (D) The survival rate of C57BL/6 mice infected with P. berghei NK65 and subjected to different diets (SDC n=4, LT-HFD n=5) was also recorded. SDC denotes the standard chow diet, while LT-HFD denotes the long-term high-fat diet. *** Significantly different from control (p < 0.001).
Another aspect that can be observed is related to cerebral vascular permeability. Increased cerebral vascular permeability is one of the main features in the pathogenesis of cerebral malaria, commonly explained by the acute inflammatory process caused by sequestration of infected red blood cells in the cerebral microvasculature and adhesion of lymphocytes in the region (21). Therefore, both groups were submitted to this evaluation to assess the integrity of the blood-brain barrier (Figure 3). The Evans blue technique was used on the sixth day of infection in which animals of the control group showed critical clinical signs according to the RMCBS index, previously described. Following the parasitemia results, lower extravasation of the dye into the brain capillaries was demonstrated in LT-HFD group when compared to SDC infected with Plasmodium berghei ANKA. On the other hand, in the infected SDC group, there was greater vascular permeability, characteristic of the breakdown of the blood-brain barrier observed in this model of experimental cerebral malaria (ECM) (1).
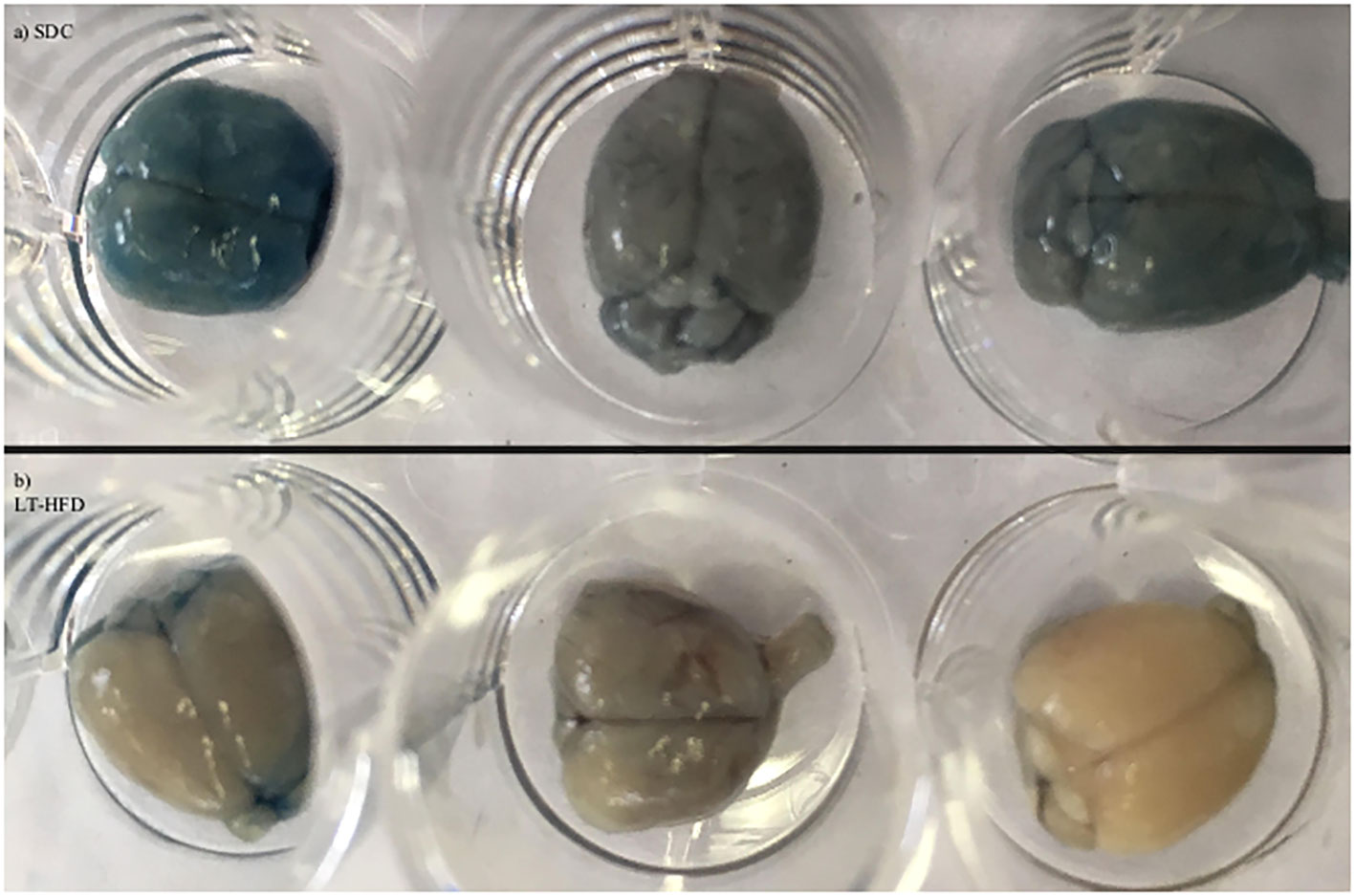
Figure 3 Assessment of cerebral vascular permeability by Blue Evans dye. Illustrative images of the brains of both groups of animals after Blue Evans staining assay on day 6 after Plasmodium berghei ANKA infection. SDC, standard chow diet (SCD); (LT-HFD) high-fat diet for 12 weeks (SDC n=3, LT-HFD n=3).
3.3 Infiltration of immune cells in the adipose tissue and spleen of infected animals is lower in those fed a hyperlipidic diet
Upon examination of the cellular profile of adipose tissue and spleen from Plasmodium berghei ANKA infected subjects, a lower percentage of cytotoxic cells was observed within both adipose tissue and spleen in animals that were simultaneously exposed to a high-fat diet and infection, as compared to the lean cohort. In addition, an analysis of Treg cell populations revealed a lower proportion of these cells only in the spleen of animals in the high-fat diet and infection group, with no significant differences observed in adipose tissue. Furthermore, no significant differences were observed in regulatory cells populations between the two groups.
3.4 Short-term consumption of a high-fat diet is effective in protecting against Plasmodium berghei ANKA -induced malaria
Since mice fed with a hiperlipidic diet for 12 weeks presented protection against Plasmodium berghei anka-induced malaria, we investigated whether the protection was due to obesity itself or the administered diet. The short-term consumption of the hyperlipidic diet led to a subtle but significant increase in the body weight (Figure 4A) of the ST-HFD animals and the mass of perigonadal adipose (Figure 4B) tissue. Surprisingly, animals fed a hyperlipidic diet for only five days demonstrated complete resistance to Plasmodium berghei ANKA infection, with the high-fat diet fed animals exhibiting close to zero circulating parasitemia (Figure 4C) and no neurological symptoms (Figure 4D).
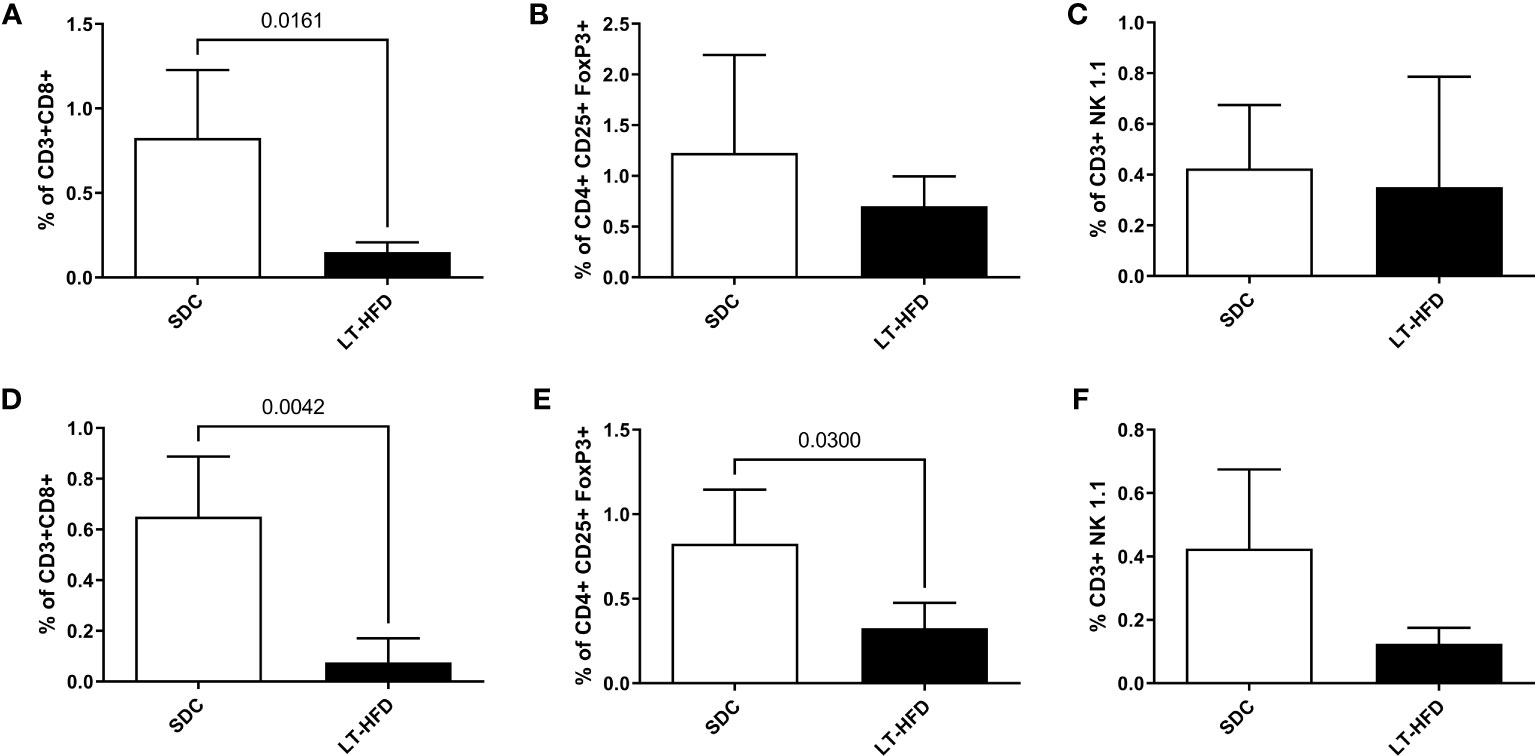
Figure 4 Different lymphocyte populations in perigonadal adipose tissue and spleen of C57BL/6 mice infected with P. berghei ANKA and treated with standard (n=4) or hyperlipidic (n=4) diet. Population of cytotoxic T lymphocytes - CD3+CD8+ (A), regulatory T lymphocytes - CD4+CD25+FoxP3+ (B) and natural killer cells - CD3+NK1.1 (C) in the perigonadal adipose tissue of infected C57BL/6 mice. Population of cytotoxic T lymphocytes - CD3+CD8+ (D), regulatory T lymphocytes CD4+CD25+FoxP3+ (E) and natural killer cells - NK CD3+NK1.1 (F) in the spleen of infected C57BL/6 mice. Results are expressed as mean ± standard error of the mean. Groups were compared using Student’s unpaired t-test, adopting a significance level of 5% (p < 0.05). SDC, Standard chow diet (n = 4); LT-HFD, Long term high fat diet (n = 4).
Plasmodium berghei ANKA infection in C57BL/6 mice is marked by the sequestration of parasites to crucial sites within the host. The adipose tissue is among the organs with a higher parasite burden (1). In this study, we aimed to investigate whether the increase in adipose tissue resulting from a hyperlipidic diet could influence parasitemia by acting as an attractive pole for parasites. To achieve this, we compared Pb18S gene expression in adipose tissue to determine parasite load in different groups (Figure 4E). Contrary to our initial hypothesis, we observed an increased parasite load only in SDC group, which corresponded to the peripheral parasitemia. In contrast, animals fed a hyperlipidic diet exhibited reduced parasite loads in adipose tissue, suggesting that the sequestration process of parasites to this region was also prevented.
Recent literature reports have described that hyperlipidic diet-induced obesity stimulates CD36 receptor expression in pre-adipocytes, leading to their differentiation into mature adipocytesy (22). As CD36 is a key receptor involved in the sequestration of infected erythrocytes to tissues, we evaluated its expression in adipose tissue. Our findings revealed a significant elevation in CD36 receptor expression in the eutrophic infected group, while animals fed a hyperlipidic diet exhibited a trend towards an increase in CD36 expression. However, the expression of this molecule did not reach the level observed in animals with the highest parasitemia (Figure 4F).
Considered as an environment of significant relevance in the immune system, the cytokine profile of adipose tissue in animals subjected to short-term hyperlipidic diet following Plasmodium berghei ANKA infection was evaluated via the Cytometric bead array (CBA) method. Surprisingly, the short-term hyperlipidic diet did not impact the increase in pro-inflammatory cytokines present in adipose tissue, as depicted in Figure 5. The cytokines IFN-γ (Figure 6A), TNF-α (Figure 6B), and IL-6 (Figure 6C) exhibited increased levels in eutrophic infected animals, as is characteristic of the immune response to malaria, and decreased in infected animals subjected to the HFD diet. While IL-2 (Figure 6E) did not show significant changes due to infection, nor did the anti-inflammatory cytokine IL-10 (Figure 6F), the cytokine IL-17 (Figure 6D) showed reduced expression in the infected control group compared to non-infected animals, probably due to the activation of the TH17 profile.
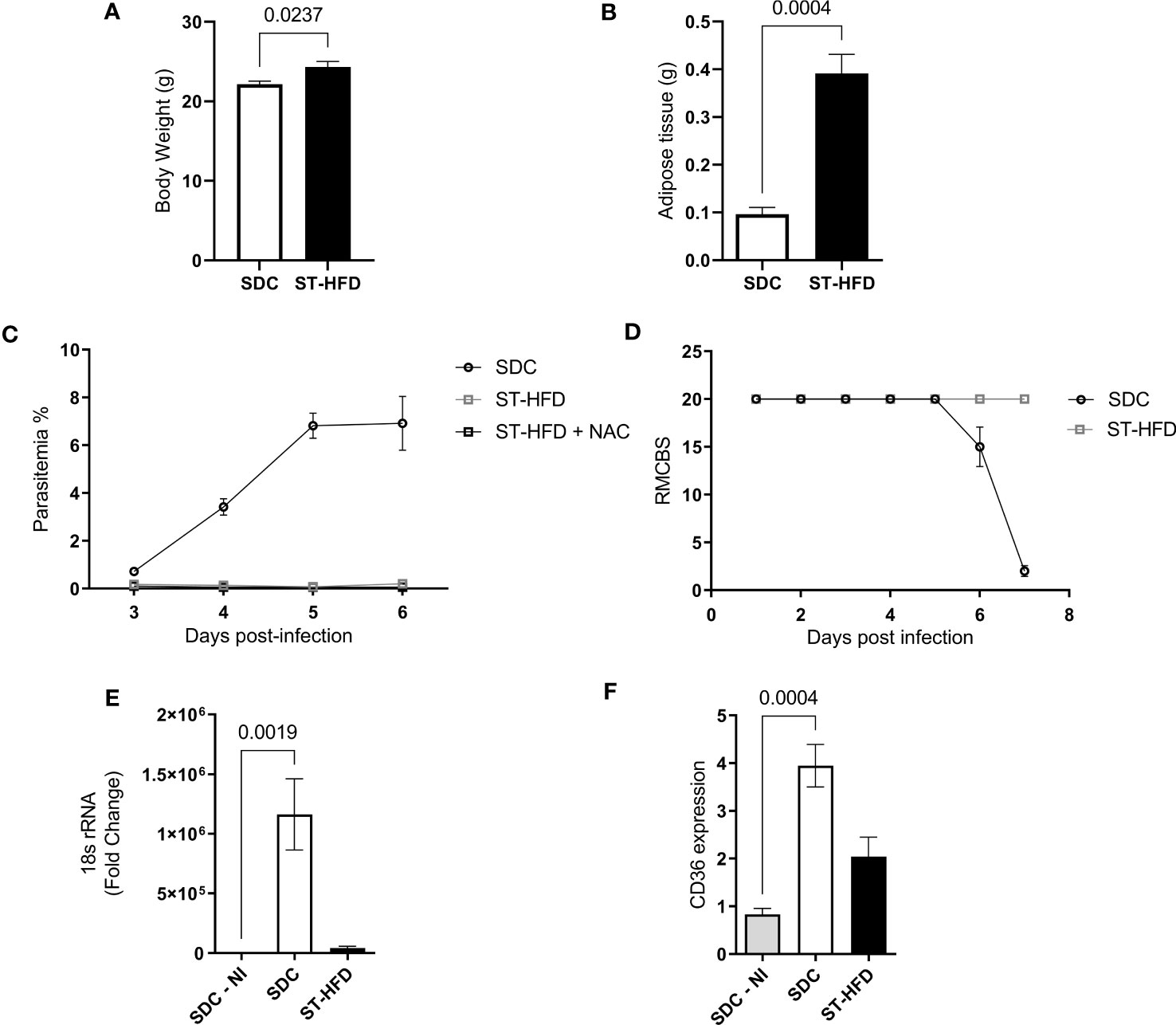
Figure 5 Synergistic effects of P. berghei ANKA infection and different diets on body weight (A), perigonadal adipose tissue weight (B), parasitemia (C), Rapid murine coma and behavior scale (RMCBS) (D), and gene expressions of Pb18s and CD36 in perigonadal adipose tissue. Animals were treated with standard or hyperlipidic diet and subsequently infected with P. berghei ANKA. After five days on the diet, a statistically significant difference in body weight (A) and perigonadal adipose tissue (B) was observed. Furthermore, animals treated with hyperlipidic diet alone, or associated with NAC, showed close to zero parasitemia (C). Data from RMCBS (D) show scores of neurological symptoms characteristic of the murine model of cerebral malaria. (E) Gene expression analyses of Pb18s and (F) CD36 demonstrate sequestration of parasitized hemacytes. Results are expressed as mean ± standard error of the mean. To compare body weight (SDC: n = 5; ST-HFD: n = 5) and adipose tissue (SDC: n = 4; ST-HFD: n = 5) in the different groups, unpaired Student’s t-test was used, adopting the significance level of 5% (p < 0.05). To compare the gene expression of Pb18s (SDC-NI: n = 4; SDC: n = 4; ST-HFD: n = 4) and CD36 (SDC-NI: n = 4; SDC: n = 3; ST-HFD: n = 3) genes in the perigonadal adipose tissue of the different groups, one-way ANOVA test was used, followed by Tukey’s post-test, adopting the significance level of 5% (p < 0.05). SDC - NI = non-infected mice treated with standard diet; SDC - infected mice treated with standard diet; ST-HFD = infected mice treated with hyperlipidic diet for five days.
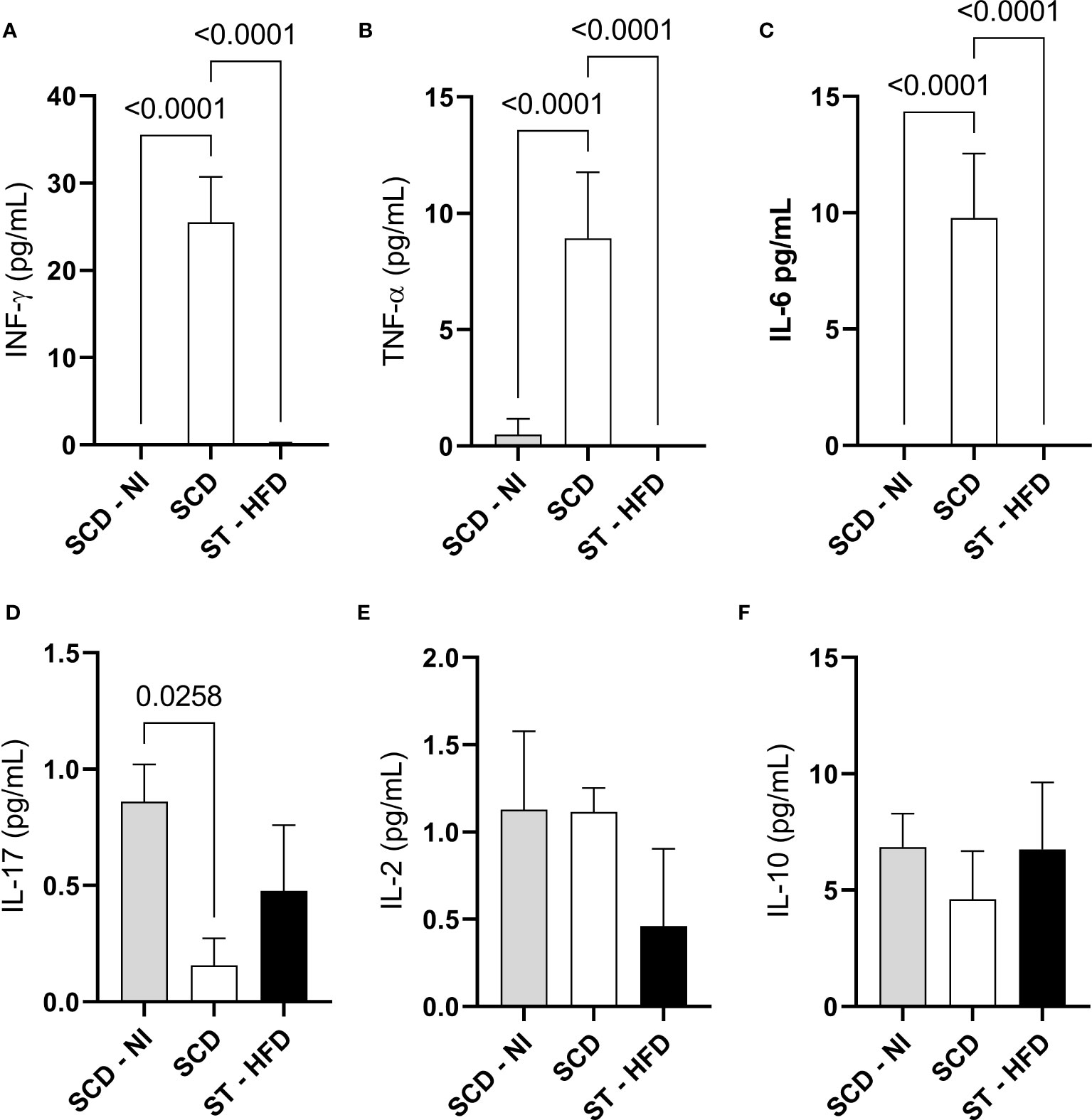
Figure 6 Effects of P. berghei ANKA infection on cytokine production in the perigonal adipose tissue of C57BL/6 mice submitted to different diets. After five days of standard or hyperlipidic diet, C57BL/6 mice were infected with P. berghei ANKA and six days after infection, the animals were euthanized, perigonadal adipose tissue collected and homogenized to investigate the secretion of the cytokines INF-γ (A), TNF-α (B), IL-6 (C), IL-17 (D), IL-2 (E) and IL-10 (F) by CBA. Results are expressed as mean ± standard error of the mean. The groups were compared using a one-way ANOVA test, followed by Tukey’s post-test, adopting a significance level of 5% (p < 0.05). SDC - NI = non-infected mice treated with standard diet (n = 4); SDC- infected mice treated with standard diet (n = 4); ST-HFD = infected mice treated with hyperlipidic diet for five days (n = 4).
Knowing that the erythrocytic stage is critical in malaria infection, the spleen, an organ of the immune system responsible for the removal of cells and particles present in the blood circulation, and in the activation of the innate and adaptive immune response, plays a key role during the immune response to these parasites (23). One of the clinical signs of malaria in humans is an enlarged spleen, splenomegaly, and patients diagnosed with severe malaria show an increase in this organ when compared to those infected with sepsis (20).
When comparing the spleen weight of the groups of infected animals, euthanized on the seventh day after infection, a significantly higher weight was observed in the animals of the ST-HFD group and, considering the parasitemia of the animals in the HFD group was approximately zero from the first day of infection, it becomes a surprising result (Figure 7A).
It was therefore possible to verify that, despite the absence of progression of parasitemia in the ST-HFD animals, cytokine production in the spleen was similar between the infected groups. Specifically, the major pro-inflammatory cytokines involved in malaria, IFN-γ (Figures 7B–H) and TNF-α, (Figure 7C) remained highly expressed in both infected groups. In addition, the presence of the cytokine IL-10 (Figure 7F) remained similar in both infected groups, unlike in the uninfected control group. There was a significant discrepancy between the presence of IL-4 (Figure 7E) in the spleen of STHFD and SDC groups.
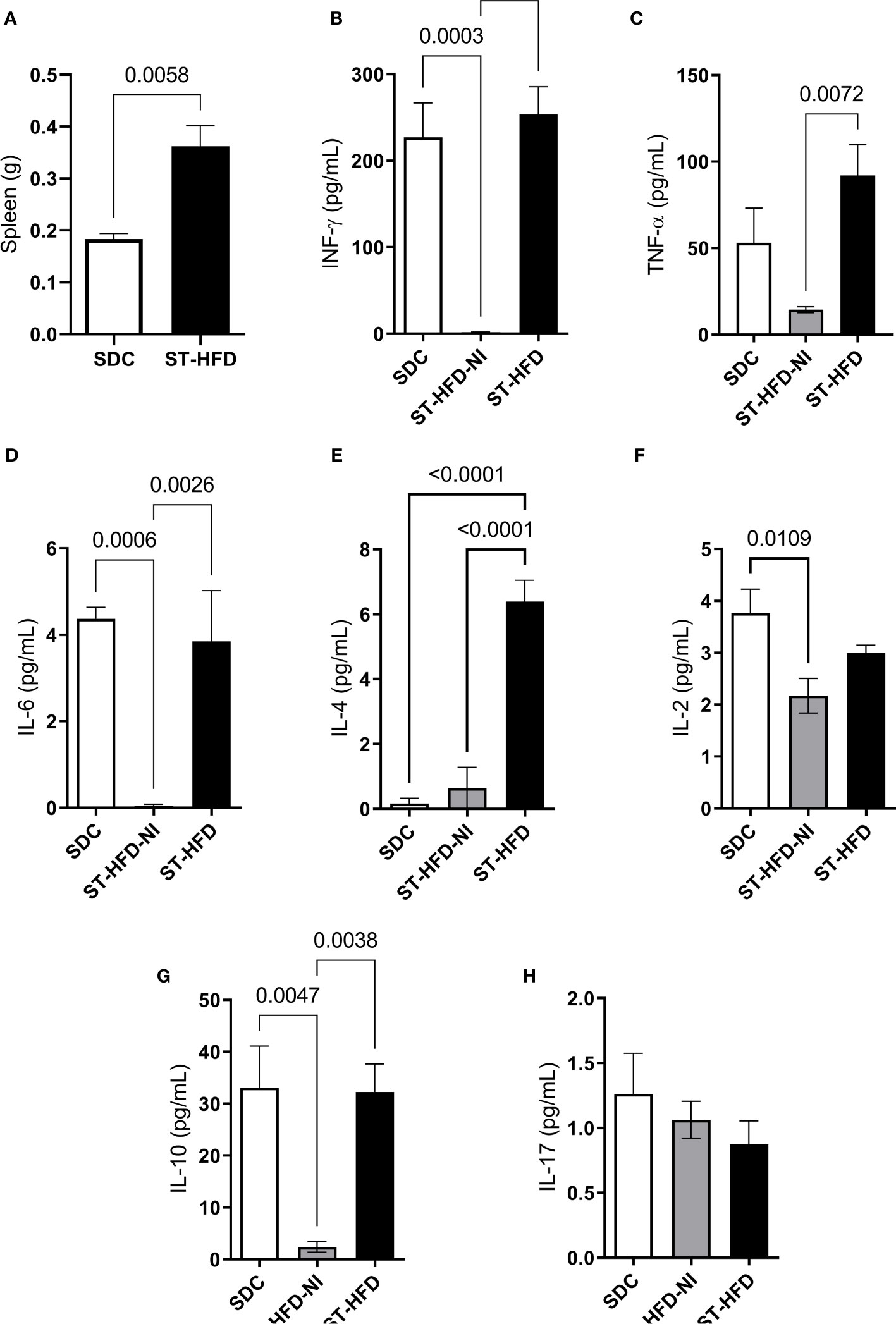
Figure 7 Effects of P. berghei ANKA infection on spleen weight and cytokine production in C57BL/6 mice submitted to different diets. After five days of standard or hyperlipidic diet, C57BL/6 mice were infected with P. berghei ANKA and six days after infection, animals were euthanized, spleen collected, weighed (A) and homogenized to investigate the secretion of cytokines INF-γ (B), TNF-α (C), IL-6 (D), IL-4 (E), IL-2 (F), IL-10 (G) and IL-17 (H) by CBA. Results are expressed as mean ± standard error of the mean. To compare the spleen weight in the different groups, Student’s unpaired t-test was used, adopting the significance level of 5% (p < 0.05). To compare the concentrations of the different cytokines secreted in the different groups, one-way ANOVA test was used, followed by Tukey’s post-test, adopting the significance level of 5% (p < 0.05). SDC - NI = non-infected mice treated with standard diet (n = 5); SDC - infected mice treated with standard diet (n = 5); ST-HFD = infected mice treated with hyperlipidic diet for five days (n = 5).
4 Discussion
The increase of obesity and overweight prevalence globally has numerous impacts on public health, and its influence on the immune system has been extensively studied (24). However, there are still gaps to be filled regarding the role of obesity during the development of infectious diseases. Although obesity and the consumption of hyperlipidic diets are commonly associated with worse prognosis for several sterile pathologies, such as cancer, diabetes, and arteriosclerosis (25, 26) their role in infectious diseases remains contentious. Nevertheless, obesity has exhibited a paradoxical role in specific scenarios, particularly in certain types of infections that require a delicate balance in the host-pathogen relationship (27, 28).
One of the most impactful and controllable factors in the development of obesity is food consumption. With urbanization, the world has undergone a major nutritional transition that has transformed dietary patterns. Due to the increased consumption of sugary, fatty, and ultra-processed foods, it has become essential to understand how this high-fat diet can modulate the immune system and alter the response to infections (16, 29, 30).
Prior research has demonstrated that the genetic background (31) of the host and its dietary patterns (32) are both factors that impact susceptibility to the development of cerebral malaria. In this current study, we investigated the protective effects of a high-fat diet, during short or long term, on animals challenged with Plasmodium berghei ANKA.
Initially, we evaluated the impact of consuming a long-term hyperlipidic diet on Plasmodium berghei ANKA infection. We have shown that animals fed a high-fat diet for 12 weeks exhibited obesity-like characteristics, including significant weight gain and the accumulation of perigonadal fat. The adipose tissue expansion is known to be involved in the inflammatory process and metabolic diseases that frequently accompany obesity (33). It is important to observe that obesity was caused by the high lipid content of the diet and not by hyperphagia, as animals fed the hyperlipidic diet consumed less food from the start, which suggests that the kind of macronutrient consumed has a significant impact on weight gain and obesity.
In a previous study utilizing the obesity model with ob/ob leptin-deficient animals, extended survival of the animals infected with Plasmodium berghei ANKA was observed. However, in this case, the parasitemia of these animals continued to rise, resulting in a malaria-like anemia. The author demonstrated that even after adjusting the infection doses, doubling the amount of sporozoites for obese mice, the disease did not progress to cerebral malaria (34). In contrast, we found high levels of circulating leptin, but it was still observed that diet-induced obesity had a protective effect against the development of cerebral malaria. Specifically, the group subjected to a high-fat diet exhibited a longer survival time and lower levels of circulating parasites during the infection as compared to the non-obese group. To ensure that protection occurred independently of physical barriers during parasite inoculation, two routes of infection were used - intraperitoneal and retro-orbital - resulting in the protection of obese animals in both routes.
On the other hand, upon infection with the nonneurotropic strain, Plasmodium berghei NK65, it was observed that the protective effects of obesity against malaria infection were not presen and survival rates did not differ between obese and non-obese animals infected with this strain of malaria. These findings suggest that the protective effects of diet and obesity against malaria may be specific to certain strains of the parasite. Furthermore, it is possible that Plasmodium berghei ANKA specific characteristics, such as tissue adherence, may be responsible for contributing to the protection provided by obesity.
Through an analysis of the cellular profile of the adipose tissue and spleen of infected animals, we have observed an increase in the infiltration of TCD4+, TCD8+, and Treg cells in the adipose tissue. Based on these findings, it is possible to suggest that in the presence of parasite sequestration in adipose tissue, the balance of immune cell populations may shift towards a Th1 type immune response, which may promote a more aggressive response to combat infection.
The consumption of a hyperlipidic diet alone for a period of five days was observed to provide the same level of protection against malaria achieved in animals on this diet for 12 weeks. Notably, this level of protection was observed without any accompanying increase in parasitemia or symptoms of experimental cerebral malaria. These findings lend support to the hypothesis that diet plays a critical role in protection against malaria, regardless of the degree of obesity.
Considering the characteristic sequestration of parasitized red blood cells in adipose tissue during Plasmodium berghei ANKA infection, it was hypothesized that protection against infection could potentially be mediated by the accumulation of the parasite in this tissue. However, subsequent analyses of parasitemia in that organ demonstrated that obese animals didn’t present sequestered infected red blood cells when compared with lean mice. Additionally, while CD36 expression in adipose tissue was modestly increased in animals fed a hyperlipidic diet, it was found to be lower than the expression observed in tissues from eutrophic animals that developed malaria following infection. Upon analysis of cytokine secretion from adipose tissue, it was observed that infected animals fed a standard diet exhibited a significant increase in proinflammatory cytokines. Conversely, this increase was not observed in animals that were fed a high-fat diet.
Comparing the organ weight of experimental animals, an increase in the spleen weight was observed in the ST-HFD as compared to the SDC. This was observed despite the ST-HFD infected group showing close to zero parasitemia from the beginning of the infection. Analysis of cytokines present in the spleen revealed a significant increase in pro-inflammatory cytokines, including IFN-γ, TNF-α, and IL-6 in animals fed the HFD when compared to non-infected animals. This indicates that although the HFD group apparently eliminated the parasite at the beginning of the infection, an inflammatory response was still observed in the spleen. Interestingly, a significant increase in the cytokine IL-4 was observed in the spleen of the infected HFD group animals as compared to the infected standard diet-fed animals. A recent article has shown that IL-4 treatment decreases the pathogenesis of cerebral malaria in animals infected with Plasmodium berghei ANKA, acting in different ways, including increasing the phagocytic potential of macrophages in the spleen, assisting in parasite elimination, and regulating immune response. It also reduces the infiltration of cytotoxic CD8 T cells in the brain (35).
Despite advances in the understanding of Plasmodium berghei ANKA infection, the relationship between microbiota alterations and this infection remains relatively understudied. Plasmodium berghei ANKA infection itself is able to impact and modulate the microbiota of mice (36). In addition, studies involving the microbiota, even when considering a short-term diet, analyze at least from 4 weeks of a high fat diet administration (37, 38).
In summary, our data suggest that a high-fat diet, whether short-term or long-term, may play a protective role for the host during Plasmodium berghei ANKA infection by preventing severe malaria symptoms, controlling parasitemia, and avoiding lethality. This model enhances our understanding of the role of dietary modulation as a modulator of the immune system’s response to infection. Understanding how these changes can modify the response to infection is relevant to comprehending the immune system’s functioning and may lead to new therapeutic approaches. Additionally, this research highlights the complex interactions among obesity, inflammation, and the immune response during infectious diseases, emphasizing the need for a comprehensive understanding of these interactions to develop effective interventions for infectious diseases in obese individuals.
Data availability statement
The raw data supporting the conclusions of this article will be made available by the authors, without undue reservation.
Ethics statement
All procedures were performed with the approval of the Animal Ethics Committee of the UFJF (reference number 019/2018).
Author contributions
SS, AG and JG: design, writing, and final content. SS, AG, AC, DG and JG: sample acquisition and analysis. SS, AG, AC and JG experimental analysis. SS and JG: project administration. SS, AG, AC, DG and JG: writing — original draft. All authors: writing—review and editing. All authors contributed to the article and approved the submitted version.
Funding
The authors received financial support from Brazilian National Council for Scientific and Technological Development (CNPq/479154/2012-0), Coordination for the Improvement of Higher Education Personnel (CAPES), and Minas Gerais State Research Foundation (FAPEMIG/RED-00313-16).
Conflict of interest
The authors declare that the research was conducted in the absence of any commercial or financial relationships that could be construed as a potential conflict of interest.
The author(s) JG declared that they were an editorial board member of Frontiers, at the time of submission. This had no impact on the peer review process and the final decision.
Publisher’s note
All claims expressed in this article are solely those of the authors and do not necessarily represent those of their affiliated organizations, or those of the publisher, the editors and the reviewers. Any product that may be evaluated in this article, or claim that may be made by its manufacturer, is not guaranteed or endorsed by the publisher.
References
1. Franke-Fayard B, Fonager J, Braks A, Khan SM, Janse CJ. Sequestration and tissue accumulation of human malaria parasites: can we learn anything from rodent models of malaria? PLoS Pathog (2010) 6(9):e1001032. doi: 10.1371/journal.ppat.1001032
2. Clark IA, Budd AC, Alleva LM, Cowden WB. Human malarial disease: A consequence of inflammatory cytokine release. Malaria J (2006) 5:85. doi: 10.1186/1475-2875-5-85
3. Dunst J, Kamena F, Matuschewski K. Cytokines and chemokines in cerebral malariaPathogenesis. Front Cell infection Microbiol (2017) 20:324.eCollection 2017(7). doi: 10.3389/fcimb.2017.00324
4. Gazzinelli RT, Kalantari P, Fitzgerald KA, Golenbock DT. Innate sensing of malaria parasites. Nat Rev Immunol (2014) 14(11):744–57. doi: 10.1038/nri3742
5. Stevenson MM, Riley EM. Innate immunity to malaria. Nat Rev Immunol (2004) 4:169–80. doi: 10.1038/nri1311
6. Combes V, Coltel N, Faille D, Wassmer SC, Grau GE. Cerebral malária: role of microparticles and platelets in alterations of the blood-brain barrier. Int J Parasitol (2006) 36(5):541–6. doi: 10.1016/j.ijpara.2006.02.005
7. Nacer A, Movila A, Sohet F, Giris NM, Gundra UM, Loke P, et al. Experimental cerebral malaria pathogenesis-hemodynamics at the blood brain barrier. PLoS Pathog (2014) 10(12):e1004528. doi: 10.1371/journal.ppat.1004528
8. Riley EM, Wahl S, Perkins DJ, Schofield L. Regulating immunity to malaria. Parasite Immunol (2006) 28(1–2):35–49. doi: 10.1111/j.1365-3024.2006.00775.x
9. Cabrera A, Neculai D, Kain KC. CD36 and malária: friends or foes? A decade of data provides some answers. Trends Parasitol (2014) 30(9):436–44. doi: 10.1016/j.pt.2014.07.006
10. Nitcheu J, Bonduelle O, Combadiere C, Tefit M, Seilhean D, Mazier D. Combadiere, B.,”Perforin-dependent brain-infiltrating cytotoxic CD8+T lymphocytes mediate experimental cerebral malaria pathogenesis. J Immunol (2003) 170(4):2221–8. doi: 10.4049/jimmunol.170.4.2221
11. Rénia L, Grau GE, Wassmer SC. CD8 + T cells and human cerebral malaria: a shifting episteme. J Clin Invest (2020) 130(3):1109–11. doi: 10.1172/JCI135510
12. Bluher M. Obesity: global epidemiology and pathogenesis. Nat Rev Endocrinol (2019) 15(5):288–98. doi: 10.1038/s41574-019-0176-8
13. Goryakin Y, Lobbstein T, James WPT, Suhrcke M. The impact of economic, political and social globalization on overweight and obesity on the 56 low- and middle-income countries. Soc Sci Med (2015) 133:67–76. doi: 10.1016/j.socscimed.2015.03.030
14. Mokhtar N, Elati J, Chabir R, Bour A, Elkari K, Schlossman NP, et al. Diet culture and obesity in northern Africa. J Nutr (2001) 131(3):887S–92S. doi: 10.1093/jn/131.3.887S
15. Prentice A. The emerging epidemic of obesity in developing countries. Int J Epidemiol (2006) 35(1):93–9. doi: 10.1093/ije/dyi272
16. Allain T, Fekete E, Sosnowski O, Desmonts de Lamache D, Motta JP, Leger D, et al. High-fat diet increases the severity of Giardia infection in association with low-grade inflammation and gut microbiota dysbiosis. Sci Rep (2021) 11(1):18842. doi: 10.1038/s41598-021-98262-8
17. Las Heras V, Clooney AG, Ryan FJ, Cabrera-Rubio R, Casey PG, Hueston CM, et al. Short-term consumption of a high-fat diet increases host susceptibility to Listeria monocytogenes infection. Microbiome (2019) 7(1):7. doi: 10.1186/s40168-019-0621-x
18. Carroll R, Wainwright MS, Kim KY, Kidambi T, Gómez ND, Taylor T, et al. A rapid murine coma and behavior scale for quantitative assessment of murine cerebral malaria. PloS One (2010) 5(10):e13124. doi: 10.1371/journal.pone.0013124
19. Toni LS, Garcia AM, Jeffrey DA, Jiang X, Stauffer BL, Miyamoto SD, et al. Optimization of phenol-chloroform RNA extraction. MethodsX (2018) 5:599–608. doi: 10.1016/j.mex.2018.05.011
20. Guthmiller JJ, Graham AC, Zander RA, Pope RL, Butler NS. IL-10 is essential for the generation of germinal center B cell responses and anti-plasmodium humoral immunity. J Immunol (Baltimore Md. : 1950) (2017) 198(2):617–22. doi: 10.4049/jimmunol.1601762
21. Van der Heyde HC, Nolan J, Combes V, Gramaglia I, Grau GE. A unified hypothesis for the genesis of cerebral malaria: sequestration, inflammation and hemostasis leading to microcirculatory dysfunction. Trends Parasitol (2006) 22(11):503–8. doi: 10.1016/j.pt.2006.09.002
22. Luo X, Li Y, Yang P, Chen Y, Wei L, Yu T, et al. Obesity induces preadipocyte CD36 expression promoting inflammation via the disruption of lysosomal calcium homeostasis and lysosome function. EBioMedicine (2020) 56:102797. doi: 10.1016/j.ebiom.2020.102797
23. Ghosh D, Stumhofer JS. The spleen: ‘Epicenter’ in malaria infection and immunity. J Leukocyte Biol (2021) 110(4):753–69. doi: 10.1002/JLB.4RI1020-713R
24. Kawai T, Autieri MV, Scalia R. Adipose tissue inflammation and metabolic dysfunction in obesity. Am J Physiol Cell Physiol (2020) 320(3):C375–91. doi: 10.1152/ajpcell.00379.2020
25. Vieira R, Souto SB, Sánchez-López E, Machado AL, Severino P, Jose S, et al. Sugar-lowering drugs for type 2 diabetes mellitus and metabolic syndrome-strategies for in vivo administration: part-II. J Clin Med (2019) 8(9):1332. doi: 10.3390/jcm8091332
26. Calle EE, Kaaks R. Overweight, obesity and cancer: epidemiological evidence and proposed mechanisms. Nat Rev Cancer (2004) 4(8):579–91. doi: 10.1038/nrc1408
27. Braun N, Gomes F, Schutz P. The obesity paradox” in disease-is the protective effect of obesity true? Swiss Med weekly (2015) 145:w14265. doi: 10.4414/smw.2015.14265
28. Schetz M, Jong AD, Deane AM, Druml W, Hemelaar P, Pelosi P, et al. Obesity in the critically ill: a narrative review. Intensive Care Med (2019) 45(6):757–69. doi: 10.1007/s00134-019-05594-1
29. Buettner R, Scholmerich J, Bollheimer LC. High-fat diets: modeling the metabolic disorders of human obesity in rodents. Obesity (2007) 15(4):798–808. doi: 10.1038/oby.2007.608
30. Popkin BM, Adair LS, Ng SW. Global nutrition and the pandemic of obesity in developing countries. Nutr Rev (2012) 70(1):3–21. doi: 10.1111/j.1753-4887.2011.00456.x
31. Cimperman CL, Pena C, Gokcek SM, Theall BP, Patel MV, Sharma A, et al. Cerebral malaria is regulated by host-mediated changes in plasmodium gene expression. MBio (2023) 14(2):e03391–22. doi: 10.1128/mbio.03391-22
32. Oliveira-Lima OC, Almeida NL, Almeida-Leite CM, Carvalho-Tavares J. Mice chronically fed a high-fat diet are resistant to malaria induced by plasmodium Berghei ANKA. Parasitol Res (2019) 118(10):2969–77. doi: 10.1007/s00436-019-06427-2
33. Bucci M, Karmi AC, Iozzo P, Fielding BA, Viljanen A, Badeau MR, et al. Enhanced fatty acid uptake in visceral adipose tissue is not reversed by weight loss in obese individuals with the metabolic syndrome. Diabetologia (2015) 58:158–64. doi: 10.1007/s00125-014-3402-x
34. Robert V, Bourgouin C, Depois D, Thouvenot C, Lombard MN, Grellier P. Malaria and obesity: obese mice are resistant to cerebral malaria. Malaria J (2008) 7:81. doi: 10.1186/1475-2875-7-81
35. Wu X, Thylur RP, Dayanand KK, Punnath K, Norbury CC, Gowda DC. IL-4 treatment mitigates experimental cerebral malaria by reducing parasitemia, dampening inflammation, and lessening the cytotoxicity of T cells. J Immunol (2021) 206(1):118–31. doi: 10.4049/jimmunol.2000779
36. Guan W, Yang S, Zhao Y, Cheng W, Song X, Yao Y, et al. Observation of the gut microbiota profile in C57BL/6 mice induced by plasmodium Berghei ANKA infection. Front Cell Infect Microbiol (2021) 11:680383. doi: 10.3389/fcimb.2021.680383
37. Wu ZH, Yang J, Chen L, Du C, Zhang Q, Zhao SS, et al. Short-term high-fat diet fuels colitis progression in mice associated with changes in blood metabolome and intestinal gene expression. Front Nutr (2022) 9:899829. doi: 10.3389/fnut.2022.899829
Keywords: malaria, plasmodium, obesity, infection, high fat diet, hiperlipidic
Citation: Soares SMA, Gualberto ACM, da Costa AC, Gonçalves DA and Gameiro J (2023) A high-fat diet protects C57BL/6 mice from Plasmodium berghei ANKA infection in an experimental malaria study. Front. Trop. Dis 4:1188902. doi: 10.3389/fitd.2023.1188902
Received: 20 March 2023; Accepted: 19 October 2023;
Published: 28 November 2023.
Edited by:
Carlos Brites, Federal University of Bahia (UFBA), BrazilReviewed by:
Rajnikant Dixit, National Institute of Malaria Research (ICMR), IndiaKleber Luz, Federal University of Rio Grande do Norte, Brazil
Copyright © 2023 Soares, Gualberto, da Costa, Gonçalves and Gameiro. This is an open-access article distributed under the terms of the Creative Commons Attribution License (CC BY). The use, distribution or reproduction in other forums is permitted, provided the original author(s) and the copyright owner(s) are credited and that the original publication in this journal is cited, in accordance with accepted academic practice. No use, distribution or reproduction is permitted which does not comply with these terms.
*Correspondence: Jacy Gameiro, amFjeS5nYW1laXJvQGljYi51ZmpmLmJy