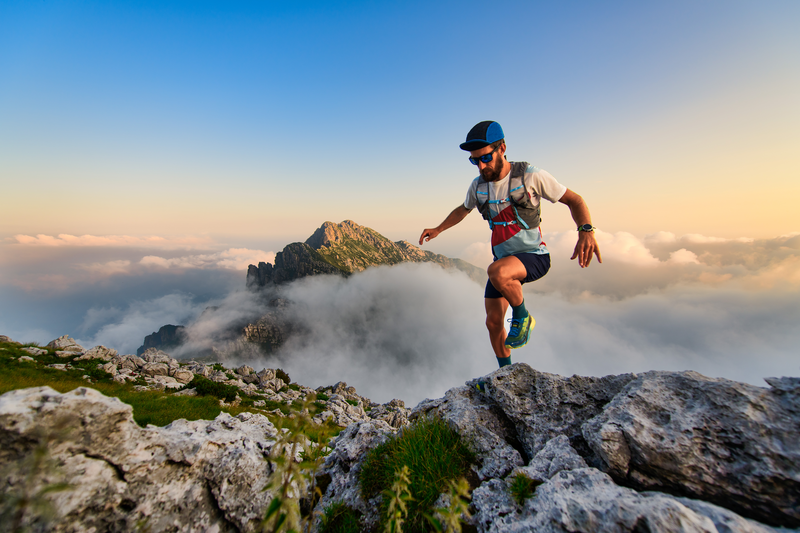
94% of researchers rate our articles as excellent or good
Learn more about the work of our research integrity team to safeguard the quality of each article we publish.
Find out more
ORIGINAL RESEARCH article
Front. Trop. Dis. , 26 January 2023
Sec. Vector Biology
Volume 4 - 2023 | https://doi.org/10.3389/fitd.2023.1126869
Management of insecticide resistance in vector control requires development and evaluation of active ingredients (AIs) with new modes of action. VECTRON™ T500 is a wettable powder formulation used for Indoor Residual Spraying (IRS) containing 50% of broflanilide as an AI. This study evaluated the efficacy of VECTRON™ T500 sprayed on blocks of different substrates (concrete, mud and plywood) against pyrethroid susceptible and resistant Anopheles gambiae sensu stricto (s.s.) strains, and wild An. arabiensis. It also assessed the efficacy of VECTRON™ T500 in experimental huts plastered with mud and concrete against wild free-flying An. arabiensis; and non-inferiority to a World Health Organization listed indoor residual spraying product Actellic® 300CS in terms of mortality in Moshi, Tanzania.Monthly cone bioassays on blocks and in experimental huts (against pyrethroid susceptible and resistant An. gambiae s.s.) were conducted over a 12-month period after spraying of VECTRON™ T500 and Actellic® CS300. Collections of wild free-flying An. arabiensis were also done in the sprayed huts. The main outcome for cone bioassays was mortality while for the wild hut trial collections, it was mortality and blood feeding inhibition. Grouped logistic regressions with random effects were used to analyse all dichotomous outcome variables from wild collections.The results showed residual efficacy of VECTRON™ T500 of at least 80% mortality was longest on concrete, followed by plywood and then mud substrates for all mosquito strains. Furthermore, VECTRON™ T500 significantly increased the likelihood of mortality (OR:> 1.37, P<0.001) in wild collections of An. arabiensis compared to Actellic® 300CS. Blood feeding was not significantly different in the wild collection of An. arabiensis between VECTRON™ T500 and Actellic® 300CS arms.These results show that VECTRON™ T500 is efficacious against pyrethroid-resistant An. gambiae s.s. and non-inferior to Actellic® 300CS. Therefore, it should be an important addition to the current arsenal of insecticides used for insecticide resistance management and vector control.
Vector control using indoor residual spraying (IRS) is among the crucial elements of achieving a 90% reduction in the global malaria burden by the year 2030 (1). It is estimated that IRS contributed to a 10% decline in malaria cases between 2000 and 2015 (2). In 2020, the number of people protected by IRS globally stood at 87 million (3). However, the growing physiological resistance to insecticides by malaria vectors, if left unchecked, threatens the effectiveness of IRS and could result in missing the target of reducing malaria burden as set out in the Global Technical Strategy (GTS) (1).
The Global Plan for Insecticide Resistance Management (GPIRM) has listed the development of innovative tools and new active ingredients (AIs) with different modes of action among the activities required to manage insecticide resistance in malaria vectors (4). This has recently seen the development of IRS products containing new classes of insecticides (5). The 27 IRS products currently prequalified by the World Health Organization Prequalification Unit Vector Control Product Assessment Team (WHO PQT/VCP) are from four insecticide classes, namely, pyrethroid, carbamate, organophosphate and neonicotinoid (6). Neonicotinoid is the most recent insecticide class repurposed for IRS which has been added to the WHO PQT/VCP prequalified list (5, 7). Current neonicotinoid IRS formulations contain clothianidin, either containing a single AI (SumiShield™ 50 WG and Klypson 500 WG) or containing a mixture with the pyrethroid deltamethrin (Fludora® Fusion and 2GARD) (6). Currently, 19 PQ-listed IRS products are pyrethroids but there are also two organophosphate products (Actellic® 300 CS and Actellic® EC; AI pirimiphos-methyl) and two carbamate products (Ficam® and FastM; AI bendiocarb) (6). From 2010 to 2020, resistance in at least one malaria vector to pyrethroid, carbamate and organophosphate insecticides was detected in 68%, 34% and 28% respectively in the sites that reported insecticide resistance monitoring data to the WHO (3). With this scenario, there is need to develop new AIs with different modes of action to which no resistance has been detected.
VECTRON™ T500, containing the meta-diamide AI broflanilide (tradename TENEBENAL™), is a new IRS product developed by Mitsui Chemicals Agro, Inc. (Tokyo, Japan) which has shown great promise in preliminary semi-field experimental hut trials against pyrethroid-resistant malaria vectors (8, 9). Broflanilide, N-[2-bromo-4-(perfluoropropan-2-yl)-6-(trifluoromethyl) phenyl]-2-fluoro-3-(N-methylbenzamido) benzamide] is a nervous system inhibitor targeting the γ-aminobutyric acid (GABA) receptor of sodium channels (10). It has a good safety profile and low mammalian toxicity with higher selectivity for insect resistance to dieldrin GABA receptors compared to mammalian GABA and glycine receptors (10, 11). Broflanilide is classified by the Insecticide Resistance Action Committee (IRAC) in class 30, GABA-gated chloride channel allosteric modulators (10, 12). Evaluation of an IRS product with new chemistry such as VECTRON™ T500 is important as it could be an additional tool for insecticide resistance management (IRM) in malaria vectors, and help in attaining the target of reducing malaria burden set out in the GTS (1). The WHO Global Malaria Programme has developed a procedure to decide whether a candidate IRS or insecticide treated net (ITN) product should be covered by an existing policy recommendation for vector control intervention class (13, 14). The procedure describes how entomological data generated through experimental huts can be used for this purpose. It involves showing that the entomological efficacy of a candidate IRS or ITN product is no worse than the standard of care product by more than a specified non-inferiority margin and superior to the negative control. For IRS, a new product must be non-inferior in terms of mosquito mortality (13). Here, the efficacy of VECTRON™ T500 was assessed in the laboratory, following the Organization for Economic Cooperation and Development principles on good laboratory practice (OECD-GLP) (15–18). Residual efficacy was determined against colonized pyrethroid susceptible and resistant Anopheles gambiae sensu stricto (s.s.) and wild An. arabiensis. Furthermore, VECTRON™ was evaluated in experimental huts for efficacy against colonized pyrethroid susceptible and resistant An. gambiae s.s. and wild free flying An. arabiensis; and non-inferiority to a WHO PQT/VCP listed indoor residual spraying product Actellic® 300CS in terms of mortality.
Data for the study were collected from December 2020 to December 2021. The laboratory study was conducted at Kilimanjaro Christian Medical University College, Pan-African Malaria Vector Research Consortium (KCMUCo-PAMVERC) insecticide test facility (ITF) in urban Moshi, Tanzania. The ITF has designated rooms for receiving test systems, testing, block spraying, treated block storage and chemical storage among others. The experimental hut study was conducted at KCMUCo-PAMVERC’s Harusini field site (3° 40’S, 37° 36’E) (8) in Lower Moshi, Tanzania. The site has eight East African-style experimental huts (19) adjacent to the Lower Moshi rice irrigation scheme. The main malaria vector in the locality is An. arabiensis which peaks in density during the rice growing periods from June to September and from November to March (8), and is partly zoophilic from feeding on cattle and humans (20, 21). Anopheles arabiensis in Lower Moshi is resistant against pyrethroids, which is driven by overexpression of cytochrome P450 monooxygenases (22, 23).
As part of the quality management system implemented by KCMUCo-PAMVERC, standard operating procedures (SOPs) have been implemented describing the procedures for laboratory and experimental hut studies. Among others, the SOPs describe procedures for mosquito rearing, and spray application using a Potter tower and backpack sprayer. Staff are also trained and assessed for their competency in following these SOPs.
The preparation of test (VECTRON™) and reference items (Actellic® 300CS), spraying of blocks, rearing of mosquitoes, and running of cone bioassays on blocks described below were performed following SOPs implemented at KCMUCo-PAMVERC to help ensure consistency and reproducibility in the conduct of the study.
VECTRON™ T500 was applied on each of the block substrate at a concentration of 100 mg AI/m2 with Actellic® 300CS applied at a target concentration of 1000 mg AI/m2 as a comparator. The VECTRON™ T500 (batch number 19I-3965) and Actellic® 300CS (batch number BSN9A2383) samples used in this study were supplied with their respective certificate of analysis which validated their use in this study. Water was used for spraying the negative control blocks. The blocks of substrates were prepared as follows:
i. Concrete blocks were made of a ratio of 2 parts cement, 4 parts sand and 1.2 parts water. The cement was bought locally in Moshi, Tanzania. Concrete blocks were left to cure for a minimum of 1 month before they were used in assays.
ii. Mud blocks were made using a ratio of 2 parts soil, 3 parts sand and 1.25 parts water. The soil was from the experimental hut study site at Harusini to minimize variation between the mud used in this laboratory study and experimental hut study. Mud blocks were left to dry for a minimum of 1 week before they were used in assays.
iii. Plywood sheets of 3-ply construction and 4mm thick were bought locally and cut into 8.5cm diameter circles by a carpenter in Moshi.
The ratios of material used above (i and ii) in the preparation of concrete and mud blocks have been shown to maximize the block integrity at KCMUCo-PAMVERC; and were previously by Oxborough et al. (24). The pH of all mud and concrete blocks was tested on the day they were sprayed with insecticides. Scrapings for pH testing were taken from the surface of blocks made on the same day but not to be used for assays. Only blocks with a pH below 8 were considered acceptable for spraying. This pH is within the range 6 – 10 judged to be suitable (25) and is also within the KCMUCo-PAMVERC Test Facility set range of 6 – 8. The pH can have an effect on the insecticide sprayed on a substrate surface (26)
Prior to spraying, the Potter tower and the nozzle arms were centralised and the even delivery of spray solution to blocks was verified using a 50% glycerol solution. Three plywood pieces in a sub-sectioned Petri dish were used for the centralisation. After verification of the Potter tower, the spray output calibration was carried out using tap water. Negative control blocks were sprayed first, followed by the VECTRON™ T500 blocks sprayed at a target concentration of 100 mg AI/m2 and the Actellic® 300CS blocks sprayed at a target concentration of 1000 mg AI/m2. The amount of spray solution (in grams, g) applied to the blocks was recorded and the percentage deviation from the target spray amount calculated as a quality control check for the spraying process. Only blocks that received a spray solution weight that was within ±10% of the target spray solution weight were used in subsequent bioassays. Four blocks were needed per treatment per substrate for this study, but additional spare blocks were also sprayed. Spare blocks were sprayed in case the integrity of the original blocks became compromised (cracked or broken) over time. Sprayed blocks were stored in the ITF Block Room post-spraying at 30 ± 2°C and 80 ± 10%RH (19) for 1 week prior to cone bioassay testing, and between test dates.
For each insecticide sprayed and for the negative water only control, two Whatman No. 1 filter papers were sprayed before and after all of the blocks for that treatment had been sprayed. Filter papers were allowed to dry for 1 hour in the ITF block room. They were subsequently wrapped in aluminium foil and stored in sealable plastic bags in the Chemical Storeroom fridge at 5 ± 3°C until ready for dispatch. Filter papers were labelled with a unique code, date sprayed and technician initials. The sprayed filter papers were sent to a GLP-compliant Test Facility, CEM Analytical Services Limited (CEMAS; Imperial House Oaklands Business Centre, Oaklands Park, Wokingham, Berkshire, RG41 2FD, UK), to be analysed for determination of insecticide concentration using High-Performance Liquid Chromatography (HPLC).
The test systems that were used for cone bioassays on treated surfaces were the susceptible An. gambiae s.s. Kisumu strain and the pyrethroid-resistant An. gambiae s.s. Muleba-Kis strain. Both insectary-reared strains are routinely characterised in terms of body weight, wing length, resistance status (phenotypic and genotypic) against three different insecticide classes (pyrethroids, carbamates and organophosphates) and species identification. Mosquitoes of the Kisumu strain show susceptibility to pyrethroids, carbamates and organophosphates; and do not express any knockdown resistance, kdr (L1014S and L1014F), or Ace-1 (G119S) mutations. The Muleba-Kis strain is resistant to pyrethroids based on kdr (L1014S) target site mutation and a metabolic mechanism associated with overexpression of the cytochrome P450-dependent monooxygenase, CYP6P3 (8, 27). The Muleba-kis strain does not express Ace-1 mutation, and is susceptible to carbamates and organophosphates (8).
Wild mosquitoes were collected as larvae from rice fields and reared in the Harusini insectary. For each test system 88 specimens from the first and last series of cone assays were packed individually in capsules for species identity confirmation and kdr target site (L1014S) mutation confirmation using molecular assays (28, 29); all other mosquitoes were disposed of.
Mosquitoes were held pre-exposure for at least one hour in paper cups, 5 – 10 mosquitoes per cup, at 27 ± 2°C and 75 ± 10% relative humidity (RH). The cone assays were conducted on the test bench under the same conditions. A calibrated data logger Tinytag® View 2 Data Logger (Gemini Data Loggers Ltd, Chichester, UK) was present on the work surface for recording conditions during pre-exposure and exposure periods. One hour before exposure, 10% glucose-soaked cotton wool was removed from the mosquito holding cups.
Assays were run at 27 ± 2°C and 75 ± 10% RH, during the day. About 10 ± 2 mosquitoes were exposed to the treated blocks for 30 minutes, with exposure time being monitored using a stopwatch, after which the mosquitoes were released into a mosquito cage, collected, and transferred into holding cups. Holding cups containing mosquitoes were taken to the temperature and humidity-controlled holding room (27 ± 2°C and 80 ± 10% RH) and provided with 10% glucose-soaked cotton wool. If the negative control mortality was ≤20% at 24 h post-exposure, then the assays were considered valid. The outcome measures include percentage knockdown after 60 minutes post-exposure and monthly percentage mortality at 24, 48 and 72 h over twelve months period post-spraying. At the end of the experiment, the blocks were disposed as hazardous waste as described by Msapalla et al. (30).
The preparation of huts, spraying of huts, running cone bioassays on walls, and wild collections in experimental huts were performed following SOPs implemented at KCMUCo-PAMVERC to help ensure consistency and reproducibility in the conduct of the study.
Experimental huts at the Harusini field site were refurbished and carefully cleaned. A layer of fresh concrete or mud, approximately 3 cm thick, was plastered over walls and ceilings. The mud was made of a soil to sand ratio of 4:7 and the concrete was made of a cement to sand ratio of 1:3. These ratios of materials maximize the integrity of the plaster on the walls and are part of the SOPs implemented at KCMUCo-PAMVERC. The concrete and mud surfaces were allowed to cure for at least 1 month before spraying. Prior to spraying, the pH of the mud and concrete was tested using wall scrapings with litmus paper being used to confirm that the pH was below 8.
The absence of insecticidal contamination of the new substrates was demonstrated using cone assays. In each experimental hut, 2 cones were attached to the unsprayed walls and 10 female insecticide susceptible mosquitoes (Kisumu strain) were exposed to the wall substrate for 30 minutes. Huts were deemed fit for spraying if the mean mosquito mortality was <20% at 24 h post-exposure.
All treatments were sprayed using a manual compression sprayer (CS-14, Micron Group, Hertfordshire, UK) onto the walls and ceilings of experimental huts. Spray tanks were fitted with a 1.5 bar control flow valve and an 8002E nozzle spraying at an application rate of 30 ml/m2. Spray tanks were calibrated on the day of spraying and the average flow rate calculated. Separate spray tanks were allocated to the negative control (water), test item (VECTRON™ T500) and comparator product (Actellic® 300CS). Prior to spraying, the eave gaps and doors were covered with a double layer of plastic sheeting covered with hessian to prevent contamination of the verandahs. VECTRON™ T500 was applied at a target concentration of 100 mg AI/m2 while Actellic® 300CS at 1000 mg AI/m2. A deviation from the target concentration of ±50% was acceptable following WHO guidelines on non-inferiority trials (13). Trial arms were randomly allocated to the huts (Additional file 1: Table S1) using randomizer software from www.random.org.
The negative control huts numbers 1 and 8 were sprayed with water only, using the spray tank labelled “control”. Huts number 6 and 7 (replicate 1) were sprayed next. The spray solution (VECTRON™ T500) volume was sufficient to cover spraying both huts. After spraying replicate 1 the tank was depressurized and the volume of the remaining insecticide solution in the spray tank was emptied into a jerry can designated for liquid insecticidal waste. The spray solution for huts 2 and 5 (replicate 2) was poured into the same spray tank and the procedure as described above was repeated. Lastly, hut numbers 3 and 4 (Actellic® 300CS) were sprayed using a different spray tank. Spraying was conducted over two days.
The volume of the spray solution was recorded before and after spraying each pair of huts, as a quality control check for the spraying process. The volume to be sprayed was calculated by multiplying the surface area of the huts by the application rate (30 ml/m2). As the spray tanks do not spray accurately when the volume in the tank is close to zero, 150 ml was added to the calculated volume, plus an additional 30% allowance for solution used during tank calibration, accidental spills and to mitigate against possible over-spraying. The residual spray solution was measured to estimate how much spray solution was applied in the huts and what the percentage deviation was from the target volume. The Quality Assurance Manager audited the preparation and spraying of the insecticides.
Samples of the spray solution were pipetted into 1.5 ml microfuge tubes (Eppendorf®; Eppendorf AG, Hamburg Germany) from the spray tanks, before and after spraying each pair of huts i.e., 8 samples of spray solution. Additionally, two 9 cmø filter papers per wall and ceiling (Whatman® no.1; Whatman International Ltd, Maidstone England) were mounted inside Petri dish lids in each hut prior to spraying: 80 filter papers in total. The position and unique ID of filter papers were recorded. After spraying filter papers were wrapped in aluminium foil and stored in the chemical storeroom fridge at 5 ± 3°C in the ITF until shipment. Together with the Eppendorf tubes, the sprayed filter papers were shipped to CEMAS for determination of insecticide concentration using HPLC.
The test systems that were used for WHO cone bioassays (19) on walls of the huts were the susceptible An. gambiae s.s. Kisumu strain and the pyrethroid-resistant An. gambiae s.s. Muleba-Kis strain. For both test systems, the cone assays were carried out one week ±4 days after spraying and thereafter at monthly intervals ±7 days. Two cones per wall and two on the ceiling were used per round of assays, with 10 ± 2 mosquitoes per cone. Cones randomly allocated to positions on the walls were attached using masking tape and the randomised location of each cone bioassay was marked. Prior to exposure, 10 ± 2 mosquitoes per cup were held for at least 1 h in the control experimental hut. Blood unfed, female mosquitoes (10 ± 2) were transferred into the cones by using mouth aspirator (a separate aspirator was used for each insecticide) and exposed for 30 minutes. A battery-powered aspirator was used to remove the mosquitoes from the cones so that the field staff are not aspirating directly from a treated surface. Post-exposure the mosquitoes were returned to the clean holding cup, provided with 10% glucose-soaked cotton wool and kept in the holding room at ambient temperature and 75 ± 15% RH. Assay environmental conditions were recorded for the pre-exposure, exposure and post-exposure (at 60 minutes, 24 h, 48 h and 72 h) periods using a Tinytag® View 2 Data Logger. Monthly average temperature and humidity were calculated for the pre-exposure, exposure and post-exposure periods. Assays were considered valid if the negative control mortality was ≤20% at 24 h post-exposure. Outcome measures included percentage knockdown 60 min after exposure and percentage mortality after 24, 48 and 72 h.
In the first round of collections 88 mosquitoes morphologically identified as An. gambiae sensu lato (s.l.) were subjected to species identification and presence/absence of kdr mutation (L1014S) using Taqman real-time PCR assays for Anopheles species (28) and kdr mutation (29), respectively. Due to the zoophilic tendency of An. arabiensis in Lower Moshi (20, 21), experimental huts were baited with one-year old non-lactating cows placed in wooden cow frames (31). Mosquito collections were made every morning from each of the experimental huts. To control for individual attractiveness to mosquitoes, cows were rotated daily between the huts so that each cow was allocated to each hut for an equal number of times. After each 8-day period, 1 day was used for cleaning and airing the huts.
Every day, all dead mosquitoes were collected from each hut location (verandah, exit trap, room floor) and live mosquitoes removed from verandah and window exit traps only. Live mosquitoes found within the room of the hut were left to exit ad libitum, as with a longer natural exposure to the IRS on the walls, they may still die in the room or exit and be collected the next day (either dead or alive). Live mosquitoes captured from the verandah and window exit traps of the experimental huts were placed into labelled holding cups, provided access to a 10% glucose solution and kept for 72 h at ambient temperature and 75 ± 15% RH. The outcome measures were individual mosquito statuses of blood feeding at collection and mortality at 24, 48 and 72 h holding. At the end of the experiment, the wall sprayed with insecticides were chipped-off and disposed as hazardous waste following the procedure describes by Msapalla et al. (30).
Statistical software used in the analysis of data generated during the conduct of the laboratory and experimental hut studies were validated in accordance with requirements of the OECD principles on GLP and in compliance with the relevant KCMUCo-PAMVERC SOPs.
Stata SE version 16.1 (StataCorp LCC, College Station, TX, USA) was used to process the laboratory and experimental hut collected data. All graphs for cone bioassay mortality on blocks and in experimental huts were created in Microsoft Excel Office 2019 (Microsoft Corporation, Washington USA). For each monthly cone bioassay, the IRS product was considered effective if 72 h mortality was ≥ 80% in accordance with the WHO threshold (13, 19). Control corrected (using Abbot’s formula (19)) and uncorrected mortality are reported. The Wilson 95% confidence intervals are reported on all graphs. Data from the experimental hut trial with wild free flying mosquitoes were analysed using grouped mixed effects multiple logistic regression with a Logit function. The independent variables in the regression were trial arm and substrate with random effects for the month of collection, cow and experimental hut. Following the WHO recommendation, the non-inferiority margin of mortality between Actellic® 300CS and VECTRON ™ T500 was defined as an odds ratio of 0.7 (13); and VECTRON ™ T500 was considered non-inferior if the lower 95% confidence interval of the odds ratio was greater than 0.7 (13). A power analysis was performed in R statistical software to check if the study had sufficient power to detect a non-inferiority margin of an odds ratio of 0.7 in mortality between wild mosquitoes collected in the Actellic® 300CS and VECTRON ™ T500 arms of the hut trial (32, 33). Statistical significance was considered at α = 0.05.
The target mass of the spray required was calculated by multiplying the surface area of the blocks by the application rate (40 ml/m2) and density of water (1 g/ml). The calculated percentage deviation of VECTRON ™ T500 and Actellic® 300CS sprayed on the blocks from the target amount are in Table 1. These data show that there was only a small deviation from the target spray deposition and actual spray deposition onto substrate blocks.
The calculated target spray solution concentration for both active ingredients, broflanilide and pirimiphos-methyl, was 3.33 mg/ml and 33.0 mg/ml respectively. The actual spray solution concentrations for these active ingredients, taken before and after applications to substrate blocks and analysed via HPLC, are shown in Table 2. The actual spray solution concentration for broflanilide before spraying was slightly below the target concentration and after spraying was above the target concentration. Similarly, the actual spray solution concentration for pirimiphos-methyl was lower than the target concentration, both before and after the treatment application.
Table 2 Quality control - Spray solution concentration by HPLC analysis of aliquots taken before and after treatment applications.
The results from the chemical analysis of filter papers suggest that both VECTRON™ T500 and Actellic® 300CS were underapplied to the substrate blocks (Table 3). However, except for two of the VECTRON™ T500 applications to filter papers, all the other applications were within a ±50% deviation from the target dose.
Table 3 Quality control – Insecticide application rates determined by HPLC analysis of extracts from filter papers treated during applications.
A total of 23,121 female mosquitoes were used in the bioassays comprising 9,031 An. gambiae s.s. Kisumu, 8,960 An. gambiae s.s. Muleba-Kis and 5,130 An. arabiensis. Cone bioassays with An. gambiae s.s. Kisumu showed mean knockdown of < 1% in the control, 15% in the Actellic® 300CS and 7% in the VECTRON™ T500 arms. Control mortality was ≤10% on all substrates after 24 h post exposure observation (Figure 1A). On the concrete substrate, the mortality threshold of 80% was achieved after 24 h observation post exposure in the VECTRON™ T500 arm and mortality was > 98% after 72 h throughout the 12 months post spraying period. On the plywood substrate, the 80% mortality threshold was achieved after 48 h observation in the VECTRON™ T500 arm throughout the 12 months post spraying period (Figure 1B). On mud substrate, 72 h mortality only dropped below 80% threshold in month 11 (Figure 1C). Mortality in the Actellic® 300CS arms was above the 80% threshold for three months on concrete and two months on mud and plywood substrates after 72 h observation post exposure (Figure 1C). The residual efficacies of both Actellic® 300CS and VECTRON™ T500 remained unchanged on all substrates after 72 h post-exposure mortality was corrected using Abbott’s formula ( Additional File1: Figure S1).
Figure 1 Mean mortality (uncorrected) in cone bioassays for pyrethroid susceptible An. gambiae s.s. Kisumu exposed to Actellic® 300CS and VECTRON™ T500 at 24 h (A), 48 h (B) and 72 h (C) post exposure observation. Error bars represent 95% Wilson confidence intervals. Crosses indicate that no cone bioassays were conducted.
Altogether, cone bioassays with An. gambiae s.s. Muleba-Kis showed mean knockdown of 0%, 3% and 1% in the control, Actellic® 300CS and VECTRON™ T500 arms, respectively. Mortality in the control arm was <10% on all substrates at 24 h post-exposure (Figure 2A). In the VECTRON™ T500 arm, mortality was above the 80% threshold on concrete and plywood substrates at 48 h post exposure (Figure 2B). On mud substrate, mortality was consistently over the 80% threshold at 72 h post exposure (Figures 2A–C) in the first 6 months post spraying period. Thereafter, fluctuation in mortality was observed (Figure 2C). Mortality was only consistently above 80% for two months on concrete and plywood substrates at 72 h post-exposure in the Actellic® 300CS before correction for control mortality (Figures 2A–C). For Actellic® 300CS on mud substrate in the first month, mortality was < 80%, but in the second month it was > 80%. Thereafter, mortality was consistently less than 80%. There was no change in residual efficacy of VECTRON™ T500 with mortality ≥ 80% throughout the 12-month period on concrete and plywood, and up to 8 months on mud after 72 h mortality was corrected using Abbott’s formula. However, for Actellic® 300CS, 72 h mortality after correction using Abbott’s formula on plywood substrate dropped below 80% in the second month ( Additional File1: Figure S2).
Figure 2 Mean mortality (uncorrected) in cone bioassays for pyrethroid resistant An. gambiae s.s. Muleba-Kis exposed to Actellic® 300CS and VECTRON™ T500 at 24 h (A), 48 h (B) and 72 h (C) post exposure observation. Error bars represent 95% Wilson confidence intervals. Crosses indicate that no cone bioassays were conducted.
Overall, mean knockdown in cone bioassays with An. arabiensis was 0%, 1% and <1% in the control, Actellic® 300CS and VECTRON™ T500 arms, respectively. Control mortality was <10% after 24 h on all substrates (Figure 3A). Mortality was above the 80% threshold after 72 h on the concrete substrate in the VECTRON™ T500 arm (Figure 3C) from the first to the seventh month after spraying. Mortality on mud and plywood substrates only met the 80% threshold for the first and the first two months, respectively, post spraying at 72 h post exposure in the VECTRON™ T500 arm. In the Actellic® 300CS arm, the 80% mortality threshold was only met on concrete substrate in the first month post spraying at 72 h post exposure (Figures 3A–C). The residual efficacies of both Actellic® 300CS and VECTRON™ T500 in terms of the number of months with mortality ≥ 80% remained unchanged after the 72 h post-exposure mortality was corrected using Abbott’s formula (Additional File1: Figure S3).
Figure 3 Mean mortality (uncorrected) in cone bioassays for wild F1 An. arabiensis exposed to Actellic® 300CS and VECTRON™ T500 at 24 h (A), 48 h (B) and 72 h (C) post exposure. Error bars represent 95% Wilson’s confidence intervals. Crosses indicate that no cone bioassays were conducted.
All wild mosquitoes reared from larvae were identified as An. arabiensis while all insectary reared mosquitoes were identified as An. gambiae s.s. using species identification molecular assays. Molecular analysis for kdr in An. gambiae s.s. Muleba-Kis samples collected from first series of cone bioassays showed 52% were homozygous resistant (RRe), 43% heterozygous resistant (RSe) and 5% homozygous susceptible (SSe); while those collected from last series of cone bioassays showed kdr gene frequency of 48% RRe, 42% RSe and 10% SSe. Anopheles gambiae s.s. Kisumu and An. arabiensis from both the first and last series of cone bioassays showed kdr 100% SSe.
The target volume of spray solution in each hut was 852.5 ml. The actual volume of spray solution applied and deviation from the targeted residual volume are shown in Table 4.
The calculated target spray solution concentration for both active ingredients, broflanilide and pirimiphos-methyl, was was 3.33 mg/ml and 33.0 mg/ml, respectively. The actual spray solution concentrations for these two treatments, determined by HLPC analysis of aliquots of spray solution taken before and after treatment applications and analysed via HPLC, are shown in Table 5. The actual spray solution concentration for broflanilide before spraying was slightly below the target concentration and after spraying was above the target concentration.
Table 5 Quality control - Spray solution concentration by HPLC analysis of aliquots taken before and after treatment applications.
The actual spray solution concentration for pirimiphos-methyl was lower than the target concentration, both before and after the treatment application.
The results from the chemical analysis of filter papers suggest that VECTRON™ T500 was underapplied. Actellic® 300CS applications were generally nearer to the target dose. However, all applications were within the WHO-indicated limit of ±50% deviation from the target dose (Table 6) showing that the treatments were correctly applied.
Table 6 Quality control – Insecticide application rates determined by HPLC analysis of extracts from filter papers treated during applications.
The highest monthly average temperature at 27.5°C was experienced in month 11 post spraying at the 60 minutes post exposure period while the lowest average monthly temperature was 20.8°C experienced in month 6 post spraying during the start of acclimation of test systems (Additional File: Figure S4A). Regarding the relative humidity during the cone bioassays on walls, the highest of 81.1% RH was during cone bioassays in huts at month 4 post spraying while the lowest was 63.7% RH at month 11 post spraying during 60 minutes post exposure period (Additional File: Figure S4B).
A total of 22,090 mosquitoes were used for in-situ cone bioassays comprising An. gambiae s.s. Kisumu strain (48.2%) and An. gambiae s.s. Muleba-Kis strain. A summary of the mosquito strain used for each substrate in the in-situ cone bioassays is given in Additional File 1: Table S2.
In total, in situ cone bioassays with An. gambiae s. s. Kisumu showed knockdown of <1% in all trial arms. Control mortality was <10% on all substrates after 72 h post exposure observation (Figures 4A–C). On the concrete substrate, the mortality threshold of 80% was achieved after 48 h observation post exposure in the VECTRON™ T500 arm (Figures 4A–B, throughout the 12 months post spraying period. On the mud substrate, mortality was above the 80% threshold after 72 h observation in the VECTRON™ T500 arm up to and including the fifth month post spraying period (Figure 4C). In the sixth month post spraying period, mortality was 71.3% on the mud substrate in the VECTRON™ T500 arm. Mortality in the Actellic® 300CS arm mortality dropped from 100% in the first month post spraying period to less than 80% by the second month on the concrete substrate after 72 h observation post exposure. On the mud substrate, mortality in the Actellic® 300CS arm was recorded to be above 80% only at week 1 after spraying (Figure 4C).
Figure 4 Mean mortality in cone bioassays for pyrethroid susceptible An. gambiae s.s. Kisumu exposed to Actellic® 300CS and VECTRON™ T500 at 24 h (A), 48 h (B) and 72 h (C) post exposure on concrete and mud substrates in experimental huts. Error bars represent 95% Wilson’s confidence intervals.
After considering control mortality at 72 h observation post exposure, residual efficacy at a cut off of 80% mortality remained unchanged in all treatment arms regardless of the substrate (Additional File1: Figure S5).
There was no knockdown of An. gambiae s.s. Muleba-Kis in any of the trial arms. Control mortality was less than 10% on all substrates after 72 h post exposure observation during the entire duration of the study (Figures 5A–C). Mortality of above 80% was achieved within 48 h post exposure the VECTRON™ T500 on the concrete substrate throughout the 12 months post spraying period. For VECTRON™ T500 on the mud substrate, 80% mortality was achieved after 72 h post exposure observation, and this lasted for 3 months after spraying (Figures 5A–C). By the twelfth month after spraying, mortality had dropped to less than 30% in the VECTRON™ T500 arm after 72 h post exposure observation (Figure 5C). In the Actellic® 300CS arm, mortality was only above 80% up to the first month on the concrete substrate. On mud substrate, mortality in the Actellic® 300CS arm was recorded to be above 80% at week two post spraying (Figure 5C). The residual efficacies of both Actellic® 300CS and VECTRON™ T500 in terms of the number of months with mortality ≥ 80% remained unchanged even after the 72 h post-exposure mortality was corrected using Abbott’s formula ( Additional File1: Figure S6).
Figure 5 Mean mortality in cone bioassays for pyrethroid resistant An. gambiae s.s. Muleba-Kis exposed to Actellic® 300CS and VECTRON™ T500 at 24 h (A), 48 h (B) and 72 h (C) post exposure on concrete and mud substrates in experimental huts. Error bars represent 95% Wilson’s confidence intervals.
Wild collections in experimental huts were conducted from January to April and July to October 2021 when average densities were more than 1 mosquito/hut/night. This variation in the abundance of wild mosquitoes at the Harusini experimental hut site is reflective of the growing cycles in the surrounding rice fields. During these periods, a total of 5,740 mosquitoes were collected with 2,764 from huts with the concrete substrate and 2,976 from huts with the mud substrate. With this number of mosquitoes collected over 120 days, the power of the study was 80% (95% CI: 77 – 82%). Molecular analysis of all 88 Anopheles species specimens from the first round of wild collections were identified as An. arabiensis and 100% SSe kdr.
Percent mortality was significantly higher in huts sprayed with VECTRON™ T500 on both concrete and mud substrates compared to huts sprayed with Actellic® 300CS on the same substrates (Table 7 and 8). Huts sprayed with Actellic® 300CS on both concrete and mud substrates recorded significantly higher mortality than the control (Tables 7 and 8).
In huts with the concrete substrate, blood feeding was not significantly different between the trial arms (Table 7). In huts with mud substrate, blood feeding was significantly lower in the Actellic® 300CS and VECTRON™ T500 arms compared to the control arm (Table 8).
Exiting from huts with concrete and mud substrates was generally high across trial arms ranging from 95% to 100% (Tables 7 and 8). There was significantly lower exiting in both Actellic® 300CS and VECTRON™ T500 arms compared to control arm (Tables 7 and 8).
Overall, in huts with concrete and mud substrates, Actellic® 300CS and VECTRON ™ T500 significantly increased the likelihood of wild An. arabiensis mortality compared to the control while accounting for random effects due to cows, hut, and month of collections (Additional file 1: Table S3). Further, VECTRON™ T500 significantly increased the likelihood of An. arabiensis mortality compared to Actellic® 300CS on both concrete (Odds ratio, OR: 3.93; 95% CI: 2.93 – 5.27; P < 0.001) and mud (OR: 1.90; (95% CI: 1.37 – 2.62; P < 0.001) substrates.
In huts with the concrete substrate, the likelihood of blood feeding was not significantly different between trial arms (Additional file 1: Table S4). The likelihood of blood feeding in huts with the mud substrate was significantly reduced in the Actellic® 300CS (OR: 0.56; 95% CI: 0.39 – 0.80; P = 0.002) and VECTRON™ T500 (OR: 0.58; 95% CI: 0.42 – 0.79; P = 0.001) arms compared to the control arm.
This study assessed the efficacy of VECTRON™ T500 in comparison to the WHO PQT/VCP listed indoor residual spraying product, Actellic® 300CS, against insectary-reared insecticide susceptible and pyrethroid resistant Anopheles gambiae s.s., and against wild An. arabiensis. Comparing the efficacy of a new vector control product to a standard comparator against malaria vectors is important to demonstrate non-inferiority. The results in this study showed a significant increase in the likelihood of wild An. arabiensis mortality collected in huts treated with VECTRON™ T500 compared to those treated with Actellic® 300CS. This is an indication of the non-inferiority of VECTRON™ T500 to Actellic® 300CS, as the lower 95% confidence limit of the odds ratio was greater than 0.7 (13) in both concrete and mud plaster lined huts. On blocks with concrete substrate in the laboratory, VECTRON™ T500 showed residual efficacy of more than 80% mortality throughout the 12 months post-spraying period against both insecticide susceptible and pyrethroid resistant An. gambiae s.s. strains. Similarly, the residual efficacy of VECTRON™ T500 in concrete plastered huts against both insecticide susceptible and pyrethroid resistant An. gambiae s.s. strains was more than 80% mortality throughout the 12 months post-spraying period. However, the residual efficacy of VECTRON™ T500 on mud substrate in huts was more than 80% mortality for 5 months post-spraying against insecticide susceptible and 3 months post-spraying against pyrethroid resistant An. gambiae s.s. These results are similar to previous findings from experimental hut studies using different formulations of broflanilide 50WP, including VECTRON™ T500, reported by Snetselaar et al. (31). The exception was the result of residual efficacy against pyrethroid resistant An. gambiae s.s. Muleba-Kis on mud substrate. Whereas in the present study, residual efficacy of more than 80% mortality was seen only up to 3 months, the previous study reported more than 80% mortality up to 5 months (8). The difference in residual efficacy between concrete and mud substrates is further highlighted with wild An. arabiensis mortality on blocks in the laboratory. Shorter residual efficacy is seen in both IRS products applied to mud compared to concrete blocks, with VECTRON™ T500 providing above 80% mortality for 2 months whilst Actellic® 300CS did not reach the 80% mortality threshold. Broflanilide susceptibility testing, using the WHO bottle bioassay method, has not detected any cross-resistance to broflanilide in any An. arabiensis strains so far tested (9, 11). Further investigations are required to understand the shorter residual efficacy of VECTRON™ T500 against the wild An. arabiensis population in lower Moshi when applied to the local mud. This should include a study of the properties of broflanilide residues in VECTRON™ T500 on lower Moshi mud post-application; changes in the surface available residues and absorption of residues into the mud so that they are no longer available for pick-up by mosquitoes. VECTRON™ T500 has shown residual efficacy of 18 months when applied to a mud substrate in an experimental hut study conducted in Benin (34). While cement is added to mud substrate in the Benin experimental hut study (34), it is not in the current study. This could have led to the observed differences in the residue efficacy of the two studies.
Control mortality on blocks and in huts was always below 20% after 24 h post exposure indicating that the data collected were within the set acceptability criteria for valid cone bioassays in this study. Furthermore, control mortality at 72 h exceeded 20% in some instances particularly in blocks, an observation that could be seen as a possible source of bias. However, Abbott’s formula was used to adjust for 72 h control mortality thereby correcting for any introduced bias.
A general observation on the cone bioassay data across all mosquito strains tested, treatments and substrate type, is that there were sometimes large fluctuations in mortality from one month to another: this was particularly the case for the Actellic® 300CS. All treated surfaces were held under environmentally controlled conditions post-treatment. Cone bioassays and subsequent holding of mosquitoes for mortality observations post-exposure were also environmentally controlled. Whilst the reason behind month-to-month fluctuations in mortality following exposure of mosquitoes to treated surfaces in cones is not known, this observation justifies our approach of continuing to conduct cone bioassays on all treatments for the entire duration of a study, regardless of whether mortality had declined to below 80% following cone bioassays on a preceding month.
Pyrethroid resistant An. gambiae s.s. Muleba-Kis used in cone bioassays on blocks showed a mixture of genotypes for target site kdr mutation L1014S. Apart from target-site mutation such as kdr, other mechanisms are also responsible for insecticide resistance (4). Metabolic resistance mechanism perpetuated by enzyme systems have a much stronger influence to confer insecticide resistance and can occur together with target site resistance in the same population and within individual mosquitoes (4) Anopheles gambiae s.s. Muleba-Kis is also known to have metabolic mechanisms for pyrethroid resistance (27). Therefore, it served as a suitable test system to evaluate the residual efficacy of VECTRON™ T500 against a pyrethroid resistant strain.
Interestingly, in both VECTRON™ T500 and Actellic® 300CS sprayed huts with mud substrate, a significant reduction in blood feeding was reported. Further investigations are required to establish whether this is an artefact or indeed type of insecticide sprayed substrates can influence blood feeding. More so than in an earlier study by Snetselaar et al. (8), some results showed differences in blood feeding according to type of insecticide sprayed substrate. The results also show that both Actellic® 300CS and VECTRON ™ T500 induced significantly lower exiting of mosquitoes compared to the control. This is likely due to a higher mortality of mosquitoes occurring inside the huts sprayed with Actellic® 300CS and VECTRON ™ T500 than in the control huts.
Comparison of the quality of VECTRON ™ T500 spray applications (using the Potter tower for the laboratory study and Micron CS-14 backpack sprayer for the experimental hut study) as assessed from the weight/volume of spray solution applied and from the amount of AI (broflanilide) extracted from treated filter papers did not show a good correlation. Focussing on the quality of the VECTRON ™ T500 spray applications in the experimental hut study, the treatment of filter papers attached to the hut walls during the treatment applications, and subsequent chemical analysis, is the method recommended in the current WHO guideline (19). However, whilst the assessment of spray quality by measuring the volume of spray solution applied (and the concentration of AI in the spray solution) suggested that the VECTRON ™ T500 spray applications deviated by a maximum of 12% from the target application rate, the data from the filter paper analysis suggested that VECTRON ™ T500 was underapplied by up to 48%. Nevertheless, a consideration of the average application rate and 95% confidence interval of AI on filter paper samples, indicates that the deviation was within ±50% acceptable ranges as stipulated in WHO guidelines on non-inferiority hut trials (13).
In conclusion, this study demonstrated that VECTRON™ T500 has an efficacy of ≥ 80% mortality against pyrethroid susceptible and resistant An. gambiae s.s. strains for a duration of at least 12 months on a concrete substrate. The efficacy can last for a minimum of 3 months against pyrethroid resistant An. gambiae s.s. Muleba-Kis and a minimum of 5 months for the susceptible Kisumu strain on a mud substrate in experimental huts. Finally, the study has shown that VECTRON™ T500 is non-inferior to Actellic® 300CS in inducing mortality of wild An. arabiensis and thus could be an important addition to the arsenal of vector control products to be used in insecticide resistance management via the rotations of products containing AIs with different modes of action.
The raw data supporting the conclusions of this article will be made available by the authors, without undue reservation.
The animal study was reviewed and approved by London School of Hygiene and Tropical Medicine’s Animal Welfare and Ethical Review Board ethical rules for studies with animals under reference 2020-02 and Tanzania’s National Institute for Medical Research reference NIMR/HQ/R.8a/Vol.IX/1656. Written informed consent was obtained from the owners for the participation of their animals in this study.
NM, MK, JS, RK, FM, MR, GS conceived and designed the study. SA, KE, BM an contributed to field work. NJM drafted the first version of the manuscript and analysed the data. All authors contributed to the article and approved the submitted version.
This study was funded by the Bill and Melinda Gates Foundation through the Innovative Vector Control Consortium. The funders did not have any role in study design, data collection and analysis, decision to publish or preparation of the manuscript.
The authors would like thank Ms Agness Msapalla, Mr Filemon Tenu, Mr Amandus Kasumbali, and Mr Remigi Pascal at KCMUCo-PAMVERC Test Facility for their technical support. We also thank Kuni Mori at Mitsui Chemicals Agro Group and Abt Associates Benin for providing the test and reference items respectively.
The authors declare that the research was conducted in the absence of any commercial or financial relationships that could be construed as a potential conflict of interest.
All claims expressed in this article are solely those of the authors and do not necessarily represent those of their affiliated organizations, or those of the publisher, the editors and the reviewers. Any product that may be evaluated in this article, or claim that may be made by its manufacturer, is not guaranteed or endorsed by the publisher.
The Supplementary Material for this article can be found online at: https://www.frontiersin.org/articles/10.3389/fitd.2023.1126869/full#supplementary-material
1. World Health Organization. Global technical strategy for malaria, 2016-2030. Geneva: World Health Organization (2015). 29 p.
2. Bhatt S, Weiss DJ, Cameron E, Bisanzio D, Mappin B, Dalrymple U, et al. The effect of malaria control on plasmodium falciparum in Africa between 2000 and 2015. Nature. (2015) 526(7572):207–11. doi: 10.1038/nature15535
4. World Health Organization. Global plan for insecticide resistance management in malaria vectors (2012). Available at: https://apps.who.int/iris/handle/10665/44846.
5. Kleinschmidt I, Rowland M. Insecticides and malaria. In: Koenraadt CJM, Spitzen J, Takken W, editors. Innovative strategies for vector control- ecology and control of vector-borne diseases. Wageningen: Wageningen Academic Publishers (2021). p. 17–32. doi: 10.3920/978-90-8686-895-7_2
6. World Health Organization. Prequalified vector control products (2022). Available at: https://extranet.who.int/pqweb/vector-control-products/prequalified-product-list?field_product_type_tid=83&field_pqt_vc_ref_number_value=&title=&field_applicant_tid=&field_active_ingredient_synergis_tid (Accessed 2022 Oct 10).
7. Oxborough RM, Seyoum A, Yihdego Y, Dabire R, Gnanguenon V, Wat’senga F, et al. Susceptibility testing of anopheles malaria vectors with the neonicotinoid insecticide clothianidin; results from 16 African countries, in preparation for indoor residual spraying with new insecticide formulations. Malar J (2019) 18(1):264. doi: 10.1186/s12936-019-2888-6
8. Snetselaar J, Rowland MW, Manunda BJ, Kisengwa EM, Small GJ, Malone DJ, et al. Efficacy of indoor residual spraying with broflanilide ( TENEBENAL ), a novel meta- diamide insecticide, against pyrethroid- resistant anopheline vectors in northern Tanzania: An experimental hut trial. PloS One (2021) 16(3):e0248026. doi: 10.1371/journal.pone.0248026
9. Ngufor C, Govoetchan R, Fongnikin A, Vigninou E, Syme T, Akogbeto M, et al. Efficacy of broflanilide (VECTRON T500), a new meta-diamide insecticide, for indoor residual spraying against pyrethroid-resistant malaria vectors. Sci Rep (2021) 11(1):7976. doi: 10.1038/s41598-021-86935-3
10. Nakao T, Banba S. Broflanilide: A meta-diamide insecticide with a novel mode of action. Bioorg Med Chem (2016) 24(3):372–7. doi: 10.1016/j.bmc.2015.08.008
11. Portwood NM, Shayo MF, Tungu PK, Mbewe NJ, Mlay G, Small G, et al. Multi-centre discriminating concentration determination of broflanilide and potential for cross-resistance to other public health insecticides in Anopheles vector populations. Sci Rep (2022) 12(1):22359. doi: 10.1038/s41598-022-26990-6
12. IRAC. In: IRAC mode of action classification scheme. IRAC. Available at: https://irac-online.org/documents/moa-classification/?ext=pdf.
13. WHO. Data requirements and protocol for determining non-inferiority of insecticide-treated net and indoor residual spraying products within an established WHO intervention class. report (2019). Available at: https://apps.who.int/iris/handle/10665/276038.
14. WHO. Determining non-inferiority of insecticide-treated nets and indoor residual spray products within an established product class. Geneva: World Health Organization (2018).
15. Organization for Economic Cooperation and Development. OECD principles of good laboratory practice. Paris: OECD (1998).
16. Begg S, Wright A, Small G, Kirby M, Moore S, Koudou B, et al. Multi-site comparison of factors influencing progress of African insecticide testing facilities towards an international quality management system certification. PloS One (2021) 16(11):e0259849. doi: 10.1371/journal.pone.0259849
17. Begg S, Wright A, Small G, Abdoulaye D, Kisinza W, Koudou B, et al. Ripple effects of research capacity strengthening: A study of the effects of a project to support test facilities in three African countries towards good laboratory practice certification. Gates Open Res (2020) 4:175. doi: 10.12688/gatesopenres.13190.1
18. Azizi S, Snetselaar J, Kaaya R, Matowo J, Onen H, Shayo M, et al. Implementing OECD GLP principles for the evaluation of novel vector control tools: a case study with two novel LLINs, SafeNet® and SafeNet NF®. Malar J (2022) 21(1):183. doi: 10.1186/s12936-022-04208-4
19. WHO. Guidelines for testing mosquito adulticides for indoor residual spraying and treatment of mosquito nets. Geneva, Switzerland: WHO (2006). Available at: http://apps.who.int/iris/bitstream/handle/10665/69296/WHO_CDS_NTD_WHOPES_GCDPP_2006.3_eng.pdf;sequence=1.
20. Mahande A, Mosha F, Mahande J, Kweka E. Feeding and resting behaviour of malaria vector, anopheles arabiensis with reference to zooprophylaxis. Malar J (2007) 6(1):100. doi: 10.1186/1475-2875-6-100
21. Kweka EJ, Mahande AM. Comparative evaluation of four mosquitoes sampling methods in rice irrigation schemes of lower moshi, northern Tanzania. Malar J (2009) 8(1):149. doi: 10.1186/1475-2875-8-149
22. Matowo J, Kulkarni MA, Mosha FW, Oxborough RM, Kitau JA, Tenu F, et al. Biochemical basis of permethrin resistance in Anopheles arabiensis from lower moshi, north-eastern Tanzania. Malar J (2010) 9(1):193. doi: 10.1186/1475-2875-9-193
23. Matowo J, Kitau J, Kabula B, Kavishe RA, Oxborough RM, Kaaya R, et al. Dynamics of insecticide resistance and the frequency of kdr mutation in the primary malaria vector Anopheles arabiensis in rural villages of lower moshi, north Eastern Tanzania. J Parasitol Vector Biol (2014) 6(3):31–41. doi: 10.5897/JPVB2013.0143
24. Oxborough RM, Kitau J, Jones R, Mosha FW, Rowland MW. Experimental hut and bioassay evaluation of the residual activity of a polymer-enhanced suspension concentrate (SC-PE) formulation of deltamethrin for IRS use in the control of anopheles arabiensis. Parasit Vectors. (2014) 7(1):454. doi: 10.1186/s13071-014-0454-1
25. Bayili K, Ki HD, Bayili B, Sow B, Ouattara A, Small G, et al. Laboratory and experimental hut trial evaluation of VECTRONTM T500 for indoor residual spraying (IRS) against insecticide resistant malaria vectors in Burkina Faso. Gates Open Res (2022) 6:57. doi: 10.12688/gatesopenres.13578.2
26. Mutagahywa J, Ijumba JN, Pratap HB, Molteni F, Mugarula FE, Magesa SM, et al. The impact of different sprayable surfaces on the effectiveness of indoor residual spraying using a micro encapsulated formulation of lambda-cyhalothrin against anopheles gambiae s.s. Parasit Vectors. (2015) 8(1):203. doi: 10.1186/s13071-015-0795-4
27. Azizi S, Snetselaar J, Wright A, Matowo J, Shirima B, Kaaya R, et al. Colonization and authentication of the pyrethroid-resistant anopheles gambiae s.s. muleba-kis strain; an important test system for laboratory screening of new insecticides. Insects. (2021) 12(8):710. doi: 10.3390/insects12080710
28. Bass C, Williamson MS, Wilding CS, Donnelly MJ, Field LM. Identification of the main malaria vectors in the Anopheles gambiae species complex using a TaqMan real-time PCR assay. Malar J (2007) 6(1):155. doi: 10.1186/1475-2875-6-155
29. Bass C, Nikou D, Donnelly MJ, Williamson MS, Ranson H, Ball A, et al. Detection of knockdown resistance (kdr) mutations in Anopheles gambiae: a comparison of two new high-throughput assays with existing methods. Malar J (2007) 6(1):111. doi: 10.1186/1475-2875-6-111
30. Msapalla A, Azizi S, Jangu M, Mbewe NJ, Matowo J, Shayo MF, et al. Simplified procedures for managing insecticidal waste: A case report of KCMUCo-PAMVERC vector control product testing facility in north-Eastern Tanzania. Environments. (2022) 9(6):65. doi: 10.3390/environments9060065
31. Mbewe NJ, Rowland MW, Snetselaar J, Azizi S, Small G, Nimmo DD, et al. Efficacy of bednets with dual insecticide-treated netting (Interceptor® G2) on side and roof panels against Anopheles arabiensis in north-eastern Tanzania. Parasit Vectors. (2022) 15(1):326. doi: 10.1186/s13071-022-05454-w
32. R Core Team. R: A language and environment for statistical computing. Vienna, Austria: R Foundation for Statistical Computing (2016).
33. Johnson PCD, Barry SJE, Ferguson HM, Müller P. Power analysis for generalized linear mixed models in ecology and evolution. Methods Ecol Evol (2015) 6:133–42. doi: 10.1111/2041-210X.12306
Keywords: broflanilide, indoor residual spraying, pirimiphos methyl, insecticide resistance, residual efficacy, vector control
Citation: Mbewe NJ, Kirby MJ, Snetselaar J, Kaaya RD, Small G, Azizi S, Ezekia K, Manunda B, Shirima B, Mosha FW and Rowland MW (2023) A non-inferiority and GLP-compliant study of broflanilide IRS (VECTRON™ T500), a novel meta-diamide insecticide against Anopheles arabiensis. Front. Trop. Dis 4:1126869. doi: 10.3389/fitd.2023.1126869
Received: 18 December 2022; Accepted: 13 January 2023;
Published: 26 January 2023.
Edited by:
Paul O. Mireji, Kenya Agricultural and Livestock Research Organization, KenyaReviewed by:
Christophe Antonio Nkondjio, Organisation de Coordination pour la lutte contre les Endémies en Afrique Centrale, CameroonCopyright © 2023 Mbewe, Kirby, Snetselaar, Kaaya, Small, Azizi, Ezekia, Manunda, Shirima, Mosha and Rowland. This is an open-access article distributed under the terms of the Creative Commons Attribution License (CC BY). The use, distribution or reproduction in other forums is permitted, provided the original author(s) and the copyright owner(s) are credited and that the original publication in this journal is cited, in accordance with accepted academic practice. No use, distribution or reproduction is permitted which does not comply with these terms.
*Correspondence: Njelembo J. Mbewe, bmplbGVtYm8ubWJld2VAbHNodG0uYWMudWs=
Disclaimer: All claims expressed in this article are solely those of the authors and do not necessarily represent those of their affiliated organizations, or those of the publisher, the editors and the reviewers. Any product that may be evaluated in this article or claim that may be made by its manufacturer is not guaranteed or endorsed by the publisher.
Research integrity at Frontiers
Learn more about the work of our research integrity team to safeguard the quality of each article we publish.