- 1Clinic of Infectious Diseases, Department of Biomedical Sciences and Human Oncology, University of Bari, Bari, Italy
- 2Clinical Infectious Diseases, Department of System Medicine, Tor Vergata University, Rome, Italy
- 3National Institute for Infectious Diseases, Lazzaro Spallanzani, IRCCS, Rome, Italy
- 4Operational Research Unit, Doctors with Africa CUAMM, Padua, Italy
- 5Institut Supérieur des Techniques Médicales (ISTM) Marie-Reine-de-la-Paix de Kenge, Kenge, Democratic Republic of Congo
According to both definitions of US Centers for Disease Control and Prevention and World Health Organization, Neglected Tropical Diseases (NTDs) are a group of preventable and treatable parasitic, viral, and bacterial diseases that affect more than one billion people globally. They generally afflict the more indigent patients of the world and historically have not received as much attention as other diseases. NTDs tend to thrive in low-income regions, where water quality, sanitation and access to health care are substandard. They are common in several countries of Africa, Asia, and Latin America. In this literature review, we want to focus on Human African Trypanosomiasis (HAT), also known as “sleeping sickness”, one of the most common neglected diseases in Africa. It is caused by infection with the subspecies of the parasitic protozoan Trypanosoma brucei, and it is transmitted by the bite of the tsetse fly. It puts 70 million people at risk throughout sub-Saharan Africa and it is usually fatal if untreated or inadequately treated. This review covers several aspects of the disease. We focused our interests on most recent epidemiological data, novel diagnostic methods with their advantages and limitations, new improved treatment and orphan drugs and eradication programs, including vector control, according to a “One Health” approach, to achieve the new goals recently set by WHO.
Introduction
Human African Trypanosomiasis (HAT), also known as sleeping sickness is a parasitic-borne Neglected Tropical Disease (NTD). It is caused by an extracellular protozoon belonging to the genus Trypanosoma, species, brucei. It is spread to the susceptible host due to the bite of the blood-sucking tsetse fly of the genus Glossina (1, 2). Trypanosoma brucei gambiense and rhodesiense are the two subspecies determining the disease in humans. These subspecies have the same morphological structure and life cycle, but they cause unique pathologic entities with distinctive epidemiological and clinical management patterns (3).
Within the vector’s geographical distribution, HAT was a leading cause of death in 36 Sub-Saharan African countries. Nevertheless, as an outcome of systematic and coordinated efforts over the past 25 years, the number of reported cases has decreased to historically low levels (1, 4, 5).
NTDs are a group of diseases caused by infections with parasites, viruses and or bacteria. They disproportionately affect poor people and mostly occur in tropical and subtropical areas. They harm individuals, communities, and economies worldwide. Every year, many people die and hundreds are severely disabled, disfigured or unable to work due to the delay in access to treatment and care. Nevertheless, according to the World Health Organization’s 2021-2030 roadmap on NTDs and Sustainable Development Goals’ targets for NTDs (6, 7), HAT remains one of the most important and challenging NTDs to eradicate due to the numerous gaps in understanding the clinic, the diagnosis, the potential new therapeutic strategies, and complications. Due to the COVID-19 pandemic, numerous essentials tasks of NTDs programs have been postponed, especially the ones directly relating to access to the healthcare system, detection and diagnosis of active cases, rapid drug administration and control and elimination timeline, particularly in high transmission areas. Another risk related to COVID-19 pandemic is the underreporting of the active case and the lack of information about the data collection regarding the eradication program’s activities carried out in these last two years. The impact of COVID-19 was studied using mathematical models (NTD Modelling Consortium) (8–10). For NTDs, like HAT, for which intensified testing and case finding is the primary strategy, the resurgence rates are more difficult to estimate. For gambiense HAT (gHAT), incidence is likely to continue to decline if the disruption of eradication and control program last only for one year but may increase in the second year of disruption especially if diagnosis and treatment of cases active detecting or presenting at health facilities continue to be interrupted. Regarding vector control, during the COVID-19 pandemic, continuation of existing measures may help. In this background, new strategies are needed to implement the efficacy of WHO’s HAT eradication programs and reach the goals established for 2030 (9, 11).
Methods
We searched PubMed, Scopus, Google Scholar, EMBASE, Cochrane Library, and WHO websites (http://www.who.int) for literature addressing Human African Trypanosomiasis, published from 1995 to June 2022. We searched in the current literature, using the following search strategy: African trypanosomiasis [tiab] OR Trypanosomiases, African [mh] OR African Trypanosomiasis [tiab] OR African Sleeping Sickness [tiab] OR african sleeping sickness [tiab] OR Nagana [tiab]). All studies dealing with epidemiology, physiopathology, clinical characteristics, screening and diagnosis, therapy, management and eradication programs were included.
Structure and biological cycle of Trypanosoma brucei
Trypanosoma brucei is a hemoflagellate protozoa. T. b. gambiense and T. b. rhodesiense are morphologically and microscopically identical (3, 12). One species can be distinguished from the other using molecular techniques (13–15).
T. brucei (both T. b. gambiense and T. b. rhodesiense) is transmitted by tsetse flies (Glossina spp) and has a complex life cycle (Figure 1), with different biological stages in both the insect vector and the mammalian host.
During a blood meal, the tsetse fly swallows the bloodstream forms of the parasite. In the vector’s midgut, the parasites can be eliminated or can keep going on their lifecycle (16, 17). In the first case, the infection in the tsetse is unsustainable. Otherwise, if the trypomastigotes differentiate into the pro-cyclic forms, they can start reproduction by binary fission. Pro-cyclic forms enter the proventriculus, where they undergo morphological and structural adaptations, becoming metacyclic trypomastigotes and then epymastigotes. Only the metacyclic form is infectious to vertebrates. It is distinguished by the presence of the variant surface glycoprotein (VSG), which is one of the protection mechanisms used by the parasite to survive in the host. The VSGs are highly immunogenic, but they also protect the parasite from the antibody response because of its rapidly turnover. Antigenic variability causes a three-digit increase in VSGs that could guide the development of new diagnostic tests. Furthermore, genes encoding VSGs are highly susceptible to mutation (18, 19). An internal mechanism that regulates shifting to new variants is the release of a “stumpy induction factor”, which triggers the production of non-replicating stumpy forms, adapted to reestablish the life cycle when ingested by the tsetse fly (20).
In the salivary gland, the parasite can reproduce sexually, allowing for genetic exchange and transmission of virulence factors or drug resistance (21–23). Sexual reproduction is not mandatory but can occur and it is especially common in T.b. rhodesiense (24). Most widely, in the salivary glands, the metacyclic forms proliferate and become infectious, assuming the trypomastigote form in the host’s site of the inoculation. In this shape, the trypanosomes are carried by the bloodstream to other sites. Bloodstream forms can be detected in various body fluids such as lymph and cerebrospinal fluid and are able to cross the placenta (17, 25). The parasitemia is crucial. Individuals with HAT are distinguished into three categories according to parasites detected in the blood and serological tests: Apparently healthy subjects with negative serological and parasitological tests; individuals with both positive tests, and ones with positive serology but negative parasitology. According to WHO guidelines, the treatment is administered to individuals with positive serological and parasitological tests. Untreated subjects are considered a parasite reservoir liable for the persistence of transmission and the occurrence of outbreaks. It has been related to important variations of the parasites’ count in the blood strictly caused by the immune response in the host and the genetic variability of the parasite (26–28).
Epidemiology, transmission and risk factors
Human African Trypanosomiasis is endemic in numerous African regions. Both rhodesiense HAT (rHAT) and gambiense HAT (gHAT) can cause epidemics (29). HAT distribution is limited to circumscribed areas called “foci”, geographical zones where transmission is possible and where the environment is suitable for the survival of the vector, the parasite, the reservoir, and the host (3). Geographical prevalence of HAT is directly proportional to the distribution of the vector which inhabits plants alongside water sources.
The number of new HAT cases has consistently decreased since vector control measures and systematic screening of the whole population in high-risk areas, along with treatment for the cases found, were performed (Figure 2).
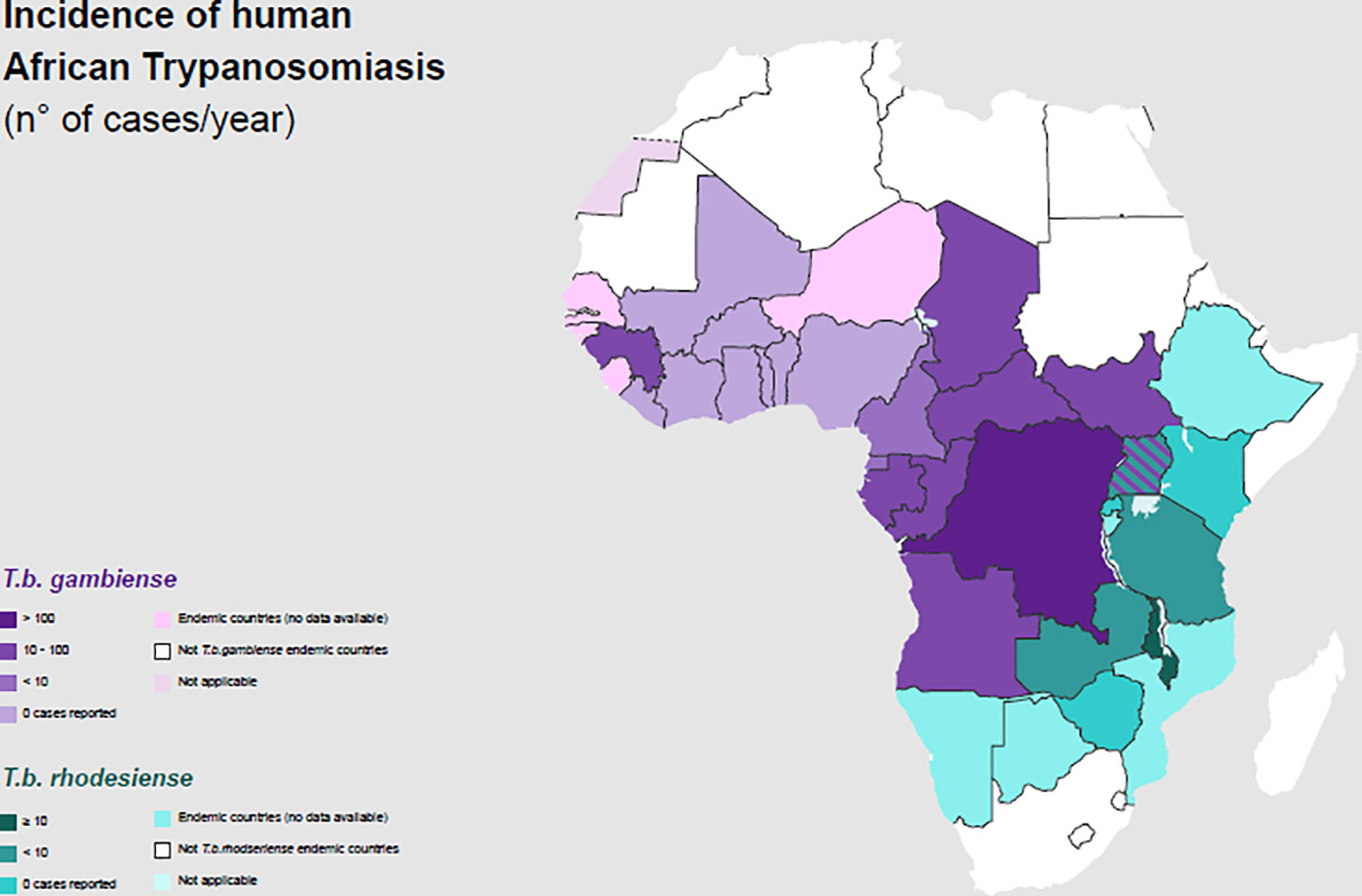
Figure 2 Incidence of Human African Trypanosomiasis: T. b. gambiense and T. b. rhodesiense (number of new reported cases of HAT for year) and geographical distribution in 2020.
Gambiense HAT cases represent 95–97% of the total proportion of HAT, whereas rHAT has accounted for the remaining 3–5% of cases (30, 31). Three hundred gHAT foci are mentioned and, among these, there are some difficult-to-reach areas where transmission intensity is not well known because of environmental conditions and a lack of an effective surveillance system (32).
In 2021 the number of reported cases of gHAT was 747 and more than half of them (425) was reported in Democratic Republic of Congo (33). Gambiense HAT is an anthroponosis: Humans are the main reservoir, while animals are accidental hosts. Several studies have been conducted to understand the hypothetical role of domestic animals as occasional reservoirs of gHAT in the transmission of the disease to humans, but more data are requested (34–38). In the gHAT, human activities are the main determinant risk factors to determine human-fly contact (39–42). In general, gHAT is predominant in young adults and males, involved in productive activities. In some areas, a higher infection rate among adolescent has been documented related to fishing and recreational water activities. Furthermore, equal incidence has been reported between males and females whose primary activity is agriculture or household work near water (Table 1) (43, 44).
Rhodesiense HAT is found in sixty foci spread across thirteen African countries (Figure 2) (30, 45). It is a zoonosis; its transmission cycle mainly involves non-human reservoirs: Wild animals or livestock (46–49). The routes of transmission are strictly related to the geographical distribution of the reservoir. The wild animals are mainly kept in protected areas or national parks, in these areas human cases occur infrequently (3, 50). Exposure is higher for tourists and workers during activities involving wildlife such as trekking and safaris. The livestock is distributed nearby the villages or in the countryside and the human cases occurs regularly (51, 52). The people who carry out activities involving the cattle are at higher risk (Table 1). In some areas, they cohabitate, as is observed in western Tanzania (53). In recent years, only few cases of rHAT have been documented: 55 is the number of reported cases in 2021 and 49 of them were registered in Malawi, however data from several counties are missing (45). The migration of the livestock as a result of human activities and climate changes may be the cause of the expansion of rHAT into new areas, increasing the probability of geographical overlap between both forms. Uganda is the only country in Sub-Saharan Africa where both types of trypanosomiasis are detected (54–56).
The reported cases of HAT in non-endemic countries are strictly related to human population movement, including traveling, business connection and migration. Most non-endemic HAT cases were detected in Europe, USA, and South Africa (57).
Other atypical transmission routes have been described, but they are very rarely:
- vertical transmission (diagnosis of HAT in the days following the birth, also in non-endemic countries from infected mothers) (58–60);
- Accidental mechanical transmission in laboratories (61);
- Blood transfusion and organ transplantation (62, 63);
- Possible sexual contact (only one case has been reported) (64).
Clinical manifestations
Infection occurs in two stages: The first stage is the hemolymphatic stage, characterized by the restriction of trypanosomes to the blood and the lymph systems. The second stage is characterized by the active CNS invasion (Table 2) (65).
T. b. gambiense infection has a chronic progressive course that can last over 3 years, mimicking hematological conditions. In contrast, T. b. rhodesiense disease is more severe and acute and can lead to death in some months; patients usually present acute febrile septic-pyrexia-like illness (Table 2) (66).
Findings from a long-term follow-up in the Ivory Coast carried out on 50 patients with diagnosis revealed that after 15 years patients who refused treatment for gHAT became asymptomatic, with no parasites detectable in the blood and some patients became seronegative. These findings support the existence of the mechanism of trypanosome-tolerance in humans, already known in animals (67). There has been no strong evidence for self-cures or trypanosome-tolerance in rHAT, which is a more acute and severe disease.
Early-stage symptoms
The typical symptoms’ onset is from 1 to 3 weeks after tsetse fly bite, and early-stage phase is non-specific, characterized by headache, malaise, arthralgia, weight loss, fatigue, and intermittent fever. Then, patients may develop various features including lymphadenopathy, splenomegaly and hepatomegaly. In addition, the cardiovascular system can be involved (myocarditis, pericarditis, and cardiac failure). Multiples organ can be target of disease with iritis, keratitis, and conjunctivitis, endocrine impairment (such as dysmenorrhea, sterility, impotence and gynaecomastia), nephropathy; all these clinical presentations are not typically severe, and usually do not lead to death before the initiation of the second phase of the disease. First-stage symptoms may be preceded by the development of a trypanosomal chancre at the site of inoculation within day 2-14 from infected fly bite. It commonly occurs with T. b. rhodesiense, rarely with T. b. gambiense, although chancres are reported in imported cases of T. b. gambiense. Travelers from non-endemic countries have shown atypical manifestations, with mainly gastro-intestinal symptoms and limited lymphatic involvement; this is supposed to be caused by host-genetic factors, but further studies are needed (68).
Posterior cervical lymphadenopathy, known as Winterbottom’s sign, is a typical feature of gHAT.
Late-stage symptoms
T. b. gambiense infection progresses to the second stage after an average of 300–500 days, whereas T. b. rhodesiense infection progresses to the second stage after an estimated 21–60 days.
According to the analysis of Blum et al, almost 74% of patients develops typical symptoms of sleeping disturbance, such as reversal of the normal sleep/wake cycle, with nocturnal insomnia and daytime somnolence, uncontrollable episodes of sleep, and an alteration of the structure of sleep itself (4, 69).
During the late stage of the disease, a wide constellation of symptoms and signs can occur, with almost all regions of the peripheral and central nervous system potentially involved. Motor disturbances include motor weakness, tremor, uncoordinated movements and speech anomalies. Myelitis, myelopathy, muscle fasciculation, and peripheral motor neuropathy might also occur. Psychiatric involvement is frequent with 25% of patients displaying behavioral disturbances (69).
Other features include sensory disturbances such as deep hyperesthesia, pruritus, anesthesia and paresthesia, seizures, and visual problems such as optic neuritis, double vision, optic atrophy, and papilledema (4). In travelers and expatriates with imported HAT from non-endemic countries progression to late-stage disease is rapid (70), while in African immigrants, HAT can be delayed and characterized by a low-grade fever and few neurological and psychiatric symptoms and signs.
Imported HAT in travelers
The HAT clinical presentation in travelers from non-endemic countries is often atypical as they present with mainly gastro-intestinal symptoms such as diarrhea and jaundice, for unknown reasons, which probably are related to host-genetic factors (68).
Due to the variability and non-specificity of the clinical manifestations, the potentially long latent period, even years, for gHAT and the lack of experience of the healthcare staff in non-endemic countries, the initial diagnosis could be missed and this may lead to further clinical progression of the disease: There are anecdotal reports of patients in whom after many years the disease has made its onset with psychiatric symptoms, so they were admitted to psychiatric wards and treated with psychiatric drugs, before reaching the diagnosis (71). In travelers, most patients affected by T. b. rhodesiense or gambiense report an unspecific acute clinical syndrome and only an accurate travel history with the identification of chancre, in the site of the tsetse fly bite raise the clinical hypothesis of a HAT diagnosis. The diagnostic hypothesis is guided by anamnestic data and suggestive clinical presentation, especially in patients from east Africa (72).
Diagnosis
The diagnosis is based on the direct examination and visualization of the parasite in peripheral blood, lymph node aspirate, cerebrospinal fluid (CSF) and in the chancre (fresh or fixed and Giemsa-stained preparation), where they can be detected a few days earlier than in the blood (73).
For T. b. rodesiense, the diagnosis through microscopic observation of blood or other biological fluids is easier due to the high parasitemia that is reached during the infection. In the case of infection with T. brucei gambiense the parasitemia is generally lower and the symptoms often more subtle, therefore serological tests have been introduced to support the diagnosis. The first introduced was CATT (Card Agglutination Test for Trypanosomiasis): This is a fast and simple agglutination assay for detection of T. b. gambiense-specific antibodies in the blood, plasma, or serum directed against purified variable surface antigens of the parasite. However, despite the simplicity of execution, this test has important limitations: in fact, in contexts of low prevalence of the disease, if on the one hand the negative predictive value (NPV) of the test remains high (few false negatives), on the other hand it has a low positive predictive value (PPV), estimated between 5 and 50% due to the high false positive rate. Subsequently different RDTs (Rapid Diagnostic Tests) were introduced: In particular, the lateral flow immunochromatographic assays (LFIAs) are based on the search for antibodies directed against trypanosome antigens and they have high sensibility and specificity, but they have the same limitations of the CATT (low PPV) and are unable to distinguish between previous infection and current infection. This implies that, if the CATT o RDTs are used as screening test for gHAT, a positive result still requires microscopy confirmation of the diagnosis, to avoid overtreatment with potentially toxic drugs (74).
Moreover, very low parasitemia requires concentration techniques to detect infection through microscopic examination. The most sensitive technique is represented by mini anion exchange centrifugation technique (mAECT) which consists in separating trypanosomes present in the blood sample by anion exchange chromatography and then concentrating them by low-speed centrifugation. This allows to examine a large volume of blood and therefore to detect concentrations of parasites lower than 50 trypanosomes/ml (75).
The CSF white blood cell count is the most widely used technique for stage determination; also, protein concentration in the CSF is diagnostic because proteins are elevated in HAT patients and range from 100 to 2,000 mg/liter. High level of IgM in CSF is diagnostic for second-stage disease. Other tests are based on polymerase chain reaction (PCR) technology, and serological tests that can demonstrate the antibodies after 3-4 weeks of infection (73).
The staging of the disease by examining the CSF is essential, and an elevated white blood cell count (>WBC 5 cells/μL) or the presence of trypanosomes in the CSF indicate a second stage disease. The quantitative buffy coat (QBC) has the advantages of concentrating the parasites by centrifugation and is a very sensitive technique (76).
Following the recent introduction of fexinidazole in the therapeutic armamentarium (see below), HAT is classified into 3 subclasses according to the number of WBCs on the CSF (Table 3) (5).

Table 3 Categorization of Human African Trypanosoma based on CSF findings (according to WHO guidelines 2019).
Blood parameters that are suspicious for HAT infection are increased sedimentation rate and low hematocrit, but these are non-specific signs of inflammation. Thrombocytopenia is generally mild or absent, liver and renal function tests are usually within normal limits or slightly elevated. Low serum C3 levels and split C3 products can be found, reflecting complement activation (77).
Treatment and management
All HAT patients should undergo anti-trypanosomal treatment. However, the choice of treatment depends on the infecting subspecies (T. b. gambiense versus T. b. rhodesiense) and the stage of the disease (Table 4).
Drugs overview
● Pentamidine
It is a di-cationic aromatic diamidine and its mechanism of action is due to the binding to the double helix DNA of the trypanosome at the level of the AT-rich sequences, preventing replication and transcription in the kinetoplast and/or in the nucleus. It accumulates in the trypanosome cell and does not leave it even when the drug is removed from the extracellular space (78). Other mechanisms involved in the trypanocide activity of pentamidine appear to be the selective inhibition of the plasma-membrane Ca2+-ATPase of T. brucei (79) and the collapse of the mitochondrial membrane potential (80), but it is not clear if the latter is a consequence of the pentamidine-induced kinetoplast dysfunction or constitutes an independent effect of the drug (81). Due to its chemical structure, pentamidine does not cross cell membranes, but requires transporters. The mutation of the genes encoding these membrane transporters explains the eventual resistance to the drug by T. brucei. The first discovered was P2 (Purine transporter 2), encoded by the TbAT1 gene, and it is responsible for the uptake of both melarsoprol and pentamidine and its mutation determines a high resistance to melarsoprol and low to pentamidine (82).
Therefore, other possible transporters involved in resistance mechanisms have been studied, identifying a high-affinity transporter for pentamidine and melaminophenyl arsenic drugs, whose mutation is the main responsible for resistance to both these drugs (83). Despite the existence of these resistance mechanisms, the cure rate in case of treatment of the first stage of gHAT with pentamidine is between 93 and 98% and it has not decreased over decades (5). Furthermore, pentamidine does not cross the blood-brain barrier and therefore its use is indicated only in the hemolymphatic phase. However, some studies have demonstrated the efficacy of pentamidine also in the early-second stage (84). Currently it is used for the treatment of the first gHAT stage, if fexinidazole is contraindicated (5). Pentamidine is not indicated as a first-line treatment for the first rHAT stage as several therapeutic failures have been recorded in the past. However, it constitutes the second line of treatment in case of unavailability of suramine (3).
● Fexinidazole
It is a new drug approved by EMA in 2018 for the gHAT treatment. It is a nitroimidazole derivative from which the parasite’s nitroreductase generates reactive amines and other metabolites, which have a toxic effect on trypanosomes. The great advantage of this drug over the others is the oral administration, which could avoid hospitalization, and the risks associated with parenteral or intramuscular treatments. Its effectiveness has been demonstrated both in the first and in the second stage of the disease: Specifically, fexinidazole showed an efficacy of over 99% in the first stage and in the early second stage of gHAT, and 91% in the late second stage, lower than the 97% efficacy rate obtained during Nifurtimox/Eflornithine Combination Therapy (NECT). This efficacy rate, however, is fully acceptable considering the great advantage of access to treatment for a greater number of people using the oral formulation and avoiding the need for lumbar puncture to differentiate clinical disease stages (if there is no clinical suspicion of severe second-stage), mostly in areas where access to diagnostic tools and hospital care is limited (85, 86). Fexinidazole could be administered also to non-hospitalized patients. It has few side effects: The most frequent are vomit, nausea and asthenia, prolongation of QT interval and psychiatric disorders (insomnia, hallucinations, ecc) (87). Fexinidazole is currently only indicated for the treatment of gHAT. The safety and efficacy of the drug for the treatment of the first and second stages of rHAT is still being studied with a project launched in 2018 and supported by the Drugs for Neglected Diseases initiative (DNDi) (88, 89).
● NECT (Nifurtimox/Eflornithine Combination Therapy)
Eflornithine is an analogue of the amino acid ornithine and a suicide inhibitor of ornithine decarboxylase (ODC). This is a fundamental enzyme to produce polyamines, essential for cell division. The ODC of T. b. gambiense is very stable and irreversible inhibition by eflornithine ensures that the trypanosome cell is deprived of these polyamines for a long time, since it does not have a transport system for them. The ODC of T. b. rhodesiense has a much shorter half-life than that of T. b. gambiense and this difference seems to explain the ineffectiveness of eflornithine in the treatment of rHAT (78). It is a trypanostatic drug, therefore it is necessary that the immune system is intact in order to achieve the cure. In immunocompromised subjects, Eflornithine alone can cause therapeutic failure (5). The main mechanism of acquisition of trypanosome resistance to eflorinithine seems to be linked to the loss of a membrane transporter for amino acids, caused by the mutation of the TbAAT6 gene that encodes it, rather than to mutations in the gene that encodes for decarboxylase (79, 90).
Nifurtimox is a nitro-heterocyclic trypanocide whose exact mode of action is unknown: It seems that it can generate free radicals such as superoxide (78), through a NADH-dependent type 1 nitroreductase (NTR), whose reduced expression could explain the onset of resistance to nifurtimox (91) and a cross-resistance with fexinidazole (92). Nifurtimox has low trypanocidal activity therefore its use as monotherapy is not indicated, but its use has been approved in combination therapy with eflornithine, NECT (93). This combination regimen replaced the previous 2 weeks eflornithine monotherapy, considering that NECT is non-inferior (94, 95), simpler, shorter, burdened with fewer side effects and less expensive than eflornithine monotherapy (96–100). NECT became the first-choice treatment for severe second stage gHAT with > 100 WBC/μL in CSF and in early second-stage gHAT (> 6-99 WBC/μL in CSF) when fexinidazole is contraindicated (i.e. children aged < 6 years, body weight < 20 kg). Eflornithine alone is only used in second stage gHAT when NECT is not feasible because the companion drug nifurtimox is unavailable or contraindicated and when fexinidazole cannot be given. Hospitalization of the patient is required as these drugs are administered intravenously (5). Moreover, the combination therapy with two drugs with different pharmacological activity seems to protect against the onset of resistance to individual drugs. Nifurtimox causes oxidative stress while eflornithine, by blocking the biosynthesis of polyamines, causes a reduction in the biosynthesis of trypanothione, which constitutes the main oxidative stress protection system. This combined effect may explain the increased parasiticidal effect when used in combination therapy, even if they do not seem to have a synergistic effect (93). NECT is generally well tolerated and the most frequent side effects are gastrointestinal symptoms that occur in more than 50% of cases but are generally mild and do not require discontinuation of therapy (5).
● Suramin
Is the first drug used for the treatment of sleeping sickness. Suramin carries out its antiparasitic activity by inhibiting various enzymatic targets: dihydrofolate reductase (101), thymidine kinases (102), glycolytic enzymes (103) among the others (104). Suramin is a large molecule that has six negative charges at physiological pH, therefore it does not cross cell membranes and needs transporters to enter the cell. This mechanism seems to be represented by at least two different receptor-mediated endocytosis pathways (104). Due to its chemical characteristics, suramine does not cross the blood brain barrier; therefore, it is indicated only for the first rHAT stage. Although it is active in the first gHAT stage, it is preferred to use fexinidazole or pentamidine due to the greater manageability of these drugs and because suramine is also active on onchocerciasis, whose prevalence is higher in endemic regions for T. b. gambiense, and its administration to people co-infected with these two parasites can lead to the development of severe immunological reactions. The main adverse reaction is represented by nephrotoxicity, which however is generally moderate and reversible (3)..
Recent studies have shown that trypanosome strains expressing VSGsur possess heightened resistance to suramine; in fact, VSGsur is capable of binding tightly to suramine unlike the other types, causing phenotypic resistance (105, 106). However, after more than 100 years of using suramine, trypanosome resistance to this drug is still not a problem and, in almost all cases, the treatment of the first rHAT stage is highly effective. Therapeutic failures can occur if the parasite has already crossed the blood brain barrier, where the drug cannot reach it (105).
● Melarsoprol
It is an arsenic-derived drug introduced in 1949 whose mechanism of action seems to be linked to the formation of potentially toxic adducts with trypanothione and to alterations of the parasite’s mitotic processes through its action on multiple kinases (81). Melarsoprol needs a specific uptake to enter the cell and exert its action. This uptake occurs via P2 adenosine transporter (encode by AT1 gene) and aquaglyceporin 2 (AQP2), that are parasite-specific, being therefore responsible for selective toxicity of the drug (94, 107). The mutations of the genes that code for these transporters are partly responsible for the onset of drug resistance, which emerged already in the 1970s and widely spread at the end of the years ‘90. In fact, around the 2000s, high rates of therapeutic failure of melarsoprol therapies were recorded (around 20%), especially in DRC (108), Uganda (109, 110), Angola (111) and southern Sudan (112). Pentamidine uses the same transporters as melarsoprol to enter the cell so there can be cross-resistance between these two drugs (83). However, for now, resistance to melarsoprol remains the only clinically relevant one (107).
Other resistance mechanisms have been studied, such as mutations of the genes encoding the kinases involved in the control of the cell cycle of the parasite (81). The main side effect related to this drug is a severe encephalopathy syndrome, that is likely an immune phenomenon, whose incidence ranges from 2 to 10% of all patients treated with melarsoprol and is fatal in about 50% of those affected. It usually occurs 7–14 days after the first injection of the drug. Three clinical forms of melarsoprol-associated encephalopathy have been proposed: Coma type, convulsion type and psychotic reactions. The first two have a worse prognosis than the last (113, 114). In the event of the onset of this syndrome, discontinuation of administration and the use of corticosteroids are indicated (5). Heart failure is also common in patients treated with melarsoprol, but it is unclear whether this is mainly attributable to drug toxicity or the known pathogenicity of HAT on the cardiovascular system (5). Given the high incidence of serious adverse effects, the availability of other drugs and widespread drug resistance, melarsoprol is now only indicated in rHAT second stage and in the treatment of recurrent relapse after first-line and rescue treatments (including NECT, NECT-long, fexinidazole or eflornithine monotherapy) in gHAT (3, 5). Concomitant administration of oral prednisolone is indicated to prevent encephalopathy. The administration of the drug requires the hospitalization of the patient (5, 115). To solve the problems related to therapy with melarsoprol, the association of melarsoprol with cyclodextrin molecules (melarsoprol-cyclodextrin complex) has been proposed: This association does not seem to affect the trypanocidal effect of the compound, but it seems to improve its ability to cure the second phase of rHAT and reduce the neuroinflammatory reaction; moreover it could be administered orally, avoiding hospitalization and reactions associated with intravenous infusion of the drug (116). This new formulation requires further studies to allow its approval, but in 2012 the WHO added it to the list of orphan drugs (117).
● Acoziborole
Acoziborole is a new oral compound that in preclinical data has shown activity against T. b. gambiense and to reach adequate concentrations for the treatment of all stages of gHAT after a single oral dose of 960 mg in the fasted state.
A recent trial reported a success rate at 18 months of 100% in 41 patients with early/intermediate stage and 95.2% in 167 patients with late stage, with a safety profile. Its approval and introduction would be an important tool in achieving the elimination of T. b. gambiense sleeping sickness, especially in those areas where access to medical care is limited, because it could eliminate the need for lumbar puncture and hospitalization of the patient and would not require adherence to the assumption of the therapy that currently instead fexinidazole requires (118–120).
Treatment of T. b. gambiense HAT
To decide what treatment the patient diagnosed with gHAT will undergo, the presence of signs and symptoms indicative of a severe second-stage disease (e.g. sleep and movement disorders, see above) is evaluated. If these are absent, it is not considered necessary to perform the lumbar puncture and, if the patient is over 6 years old and weighs more than 20 kg, he can be treated with fexinidazole. This therapy will be taken at home if there is probable certainty of the patient’s adherence to the therapy and food intake, otherwise it will be administered in the hospital. If there are criteria for exclusion from therapy with fexinidazole or in case of unavailability of this drug, the patient will be a candidate for NECT or pentamidine therapy, therefore lumbar puncture will be indicated. If there is a clinical suspicion that the disease is in a severe second stage, lumbar puncture is required. If the lumbar puncture cannot be performed, it is indicated to prescribe NECT. If, on the other hand, lumbar puncture is performed and there are less than 100 WBC/μL in CSF and the patient is older than 6 and weighs more than 20 kg, oral therapy with fexinidazole is indicated. If there are less than 100 WBC/μL in CSF and the patient is younger than 6 and weighs less than 20 kg, oral therapy is not possible: So if there are 5 WBC/μL in CSF or less and no trypanosomes are present, the patient will be treated with pentamidine. In case of WBC above 5/μL he will be treated with NECT (5) (Table 5).
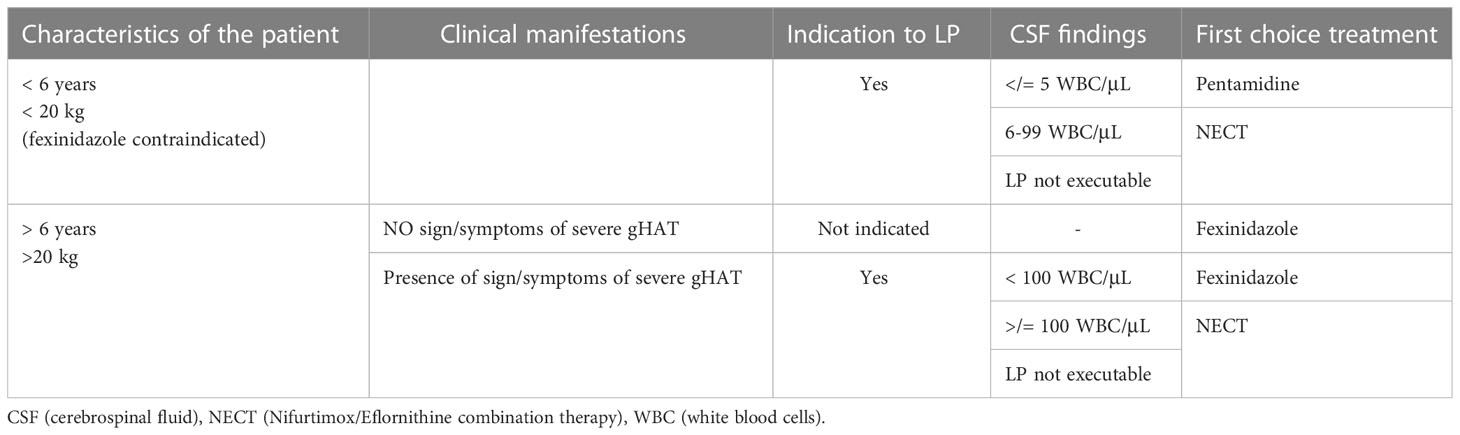
Table 5 Algorithm on the management of persons with Gambience HAT in accordance to WHO recommendation.
Treatment of T. b. rhodesiense HAT
Treatment options for rHAT are more limited: the drug of choice for the first stage is suramine. If this is unavailable or contraindicated, the alternative is represented by pentamidine. The only drug available for the second stage (WBC > 5/μL of CSF) is melarsoprol, which as mentioned above has high toxicity and significant resistance rates (3) (Table 6).
Elimination strategies
gHAT
As mentioned above, humans are the main reservoir of T. b. gambiense, therefore elimination strategies must aim first at the diagnosis and treatment of infected subjects to reduce reservoir and transmission to healthy susceptible patients. Moreover, the symptoms in infected people can be absent or vague for a long time, so often there is an important diagnostic delay. For these reasons, the most effective strategy is the active case detection through mobile teams. The second strategy is vector control, trying to eliminate the tsetse mosquito or prevent its bite. The strategies already used have made it possible to achieve a better target than that set by the WHO for 2020: In fact, the eradication program hoped to reach less than 2000 cases declared per year by 2020, but already in 2019, fewer than 1000 cases were declared. The new goal set by WHO is zero cases of gHAT declared per year by 2030 (interruption of transmission), as indicated in the NTD road map 2021-2030 (6).
● Active case detection
It consists in the mass screening of the population at risk carried out by mobile teams through the use of CATT or RDTs (see above in “Diagnosis” section). The first one requires specialized staff, the maintenance of the cold chain and an agitator (and thus electricity) to be performed and this makes it difficult to use it in screening in rural areas (74, 121).
RDTs are easy to use and interpret, and they have features that allow their use even in rural endemic areas (74). Anyway, both tests are characterized by low PPV (74). In settings where there is a low incidence of the disease, tests with high specificity and high positive predictive value are necessary to identify the reservoirs and treat them (test-and-treat strategy), avoiding false-positives that could generate alarm, the implementation of unnecessary actions and the over-treatment of the population. Therefore, due to their characteristics, both CATT and RDTs are not suitable for screening in the population in which there is a very low incidence of disease or for post-elimination monitoring and other tests are being developed for these settings (122). An algorithm for active screening strategies for the elimination of gHAT in foci where transmission is high or moderate (annual incidence > 1 case per 10,000 people over the last 5 years) has been proposed by WHO (3) (Figure 3).
● Passive case detection
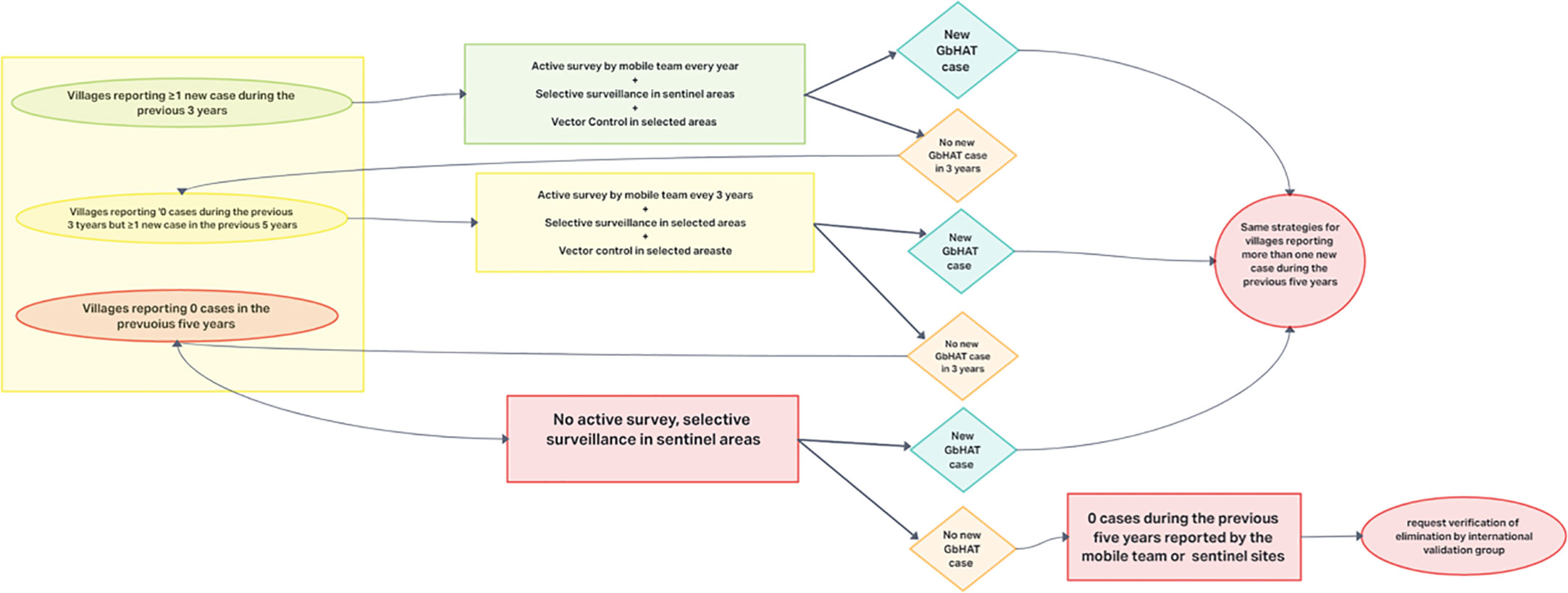
Figure 3 Algorithm proposed by the WHO for active screening strategies for the elimination of gHAT in foci where transmission is high or moderate.
It consists in the diagnosis of trypanosomiasis of patients who seek for care in health centers. The effectiveness of this action in elimination strategies is very limited by several factors: first, in low endemic areas the staff could be unconfident with HAT. Therefore, diagnostic algorithms were developed to suspect the disease based on the three main symptoms: Sleep disturbances, weight loss and neurological symptoms. Health center facilities often do not have rapid tests and blood samples must be sent to referral centers. Third, the patients can be asymptomatic or paucisymptomatic for a long time and can access to a health center several months after symptoms’ onset, representing a potential long-lasting human reservoir (123).
rHAT
Prevention and control measures of rHAT are complex because it is a zoonosis and the main reservoir is represented by animals (livestock and wildlife), which ensure the maintenance of a population of infected tsetse flies that occasionally transmit the disease to humans. At this moment, complete transmission disruption and elimination are not possible. For this reason, in NTD road map 2021-2030 rHAT is targeted for elimination as a public health problem (<1 case/10 000 people/year, in each health district calculated on the average of the previous five years) (6). Control of the parasite in the animal reservoirs and/or reduction of tsetse vector populations play a key part with medical interventions in reducing human cases (3).
● Active case detection
The clinical diagnosis of rHAT is complex because of unspecific symptoms and signs. There are no serological tests to facilitate the diagnosis, although the high level of parasitemia during the infection could improve the detection of an active case. In consideration of the epidemiology and the acute presentation of the disease, active detection of infected individuals is inefficient and cost ineffective.
● Passive case detection
The systemic nature of symptoms in the first stage, the lack of early recognition of the disease by clinicians and the low experience in optical microscopy diagnosis cause important delays in detection and treatment. Such delay increases the chance of the second stage disease, whose fatality rate is 2.5 times higher than that of patients at first-stage disease (124, 125).
The aim of passive case detection is therefore to identify and treat rHAT cases as quickly as possible and to do this it is necessary to develop reliable rapid diagnostic tests and enhance the capacity for treatment of the health care facilities (53).
● Control of animal reservoirs.
Control of transmission from the animal reservoir is required to control rHAT (3). While the treatment of livestock with anti-trypanosome drugs and specific insecticides is relatively cheap and easily feasible and has proven to be effective in reducing reservoir and vectors, on the other hand, treatment of the wildlife is not a viable option as it would be extremely expensive and difficult to implement. For this reason, contact between humans and wildlife should be limited or, if it is not possible, appropriate protective measures should be used to avoid tsetse fly bites (53, 126).
Vector control: Methods and efficacy to reduce the diffusion of Human African Trypanosomiasis
Vector control is used to diminish the tse-tse fly population to a level at which the transmission infection is significantly reduced and is the primary method to control animal trypanosomiasis (3).
Over the years, different methods have been used, depending on economic resources, epidemiology and specific environmental factors. The principal methods are: clearing of vegetation; ground spraying of insecticide at the tse-tse breeding sites (127); persistent insecticides (no longer used due to environmental concerns); live animal or artificial baits like insecticide-treated cattle (128) or traps (129–131) and insecticide impregnated screens (132, 133),
The elaboration of cost-effective vector control strategies needs to consider various factors including the geography of the place, the human and vector population density, the presence of animal reservoirs, the operational costs, the prevalence of the disease, the environmental impact, etc.
With regards to gHAT, although active case detection and treatment and passive surveillance are considered the main strategies for elimination of the disease and have made it possible to greatly reduce the incidence of the disease, several studies have demonstrated the importance and efficacy of vector control in reducing the tsetse fly population to achieve the interruption of the transmission and the elimination of the diseases (134).
Previously vector control strategies for gHAT were considered too expensive for low resource countries (135), however the introduction of Tiny Targets, insecticide-impregnated panels that attract and kill tse tse flies, has proven to be advantageous and economically sustainable in these settings (132, 136–138).
As mentioned, the goal of vector control strategies is not to completely eliminate tse tse flies, but to reduce their density by at least 70%, as it has been seen that this allows to reach the interruption of the transmission of the disease. For example, in a study carried out in Uganda the use of Tiny Target near watercourses has been shown to reduce tsetse population density by more than 90% and achieve the goal of stopping transmission in these areas. However, Tiny Targets require maintenance and a median life of 61 days of these instruments was recorded, with discrete variability based on their location (139).
Moreover the vector control strategies and the use of Tiny Targets cannot be standardized and replicated in the same way for each situation, but it must be elaborated according to the specific characteristics of each setting (140).
Therefore a recent study examined five different health districts of the Democratic Republic of Congo (the country with the highest burden of gHAT) and for each one developed the most appropriate and cost-effective elimination strategy pattern, combining active screening, passive screening and vector control, based on the particular features of the setting and also considering the role of the recently introduced oral drug, fexinidazole (141).
The role of animals as a reservoir of infection for T. b. gambiense and their impact on achieving elimination of the disease is still debated: however, the implementation of vector control strategies can also have an effect in reducing any transmission between animals and humans (142, 143).
A study based on mathematical models found that animal transmission appears to be statistically significant in 24 of 158 geographic areas considered in the study. From the same model that takes animal transmission into account, it emerged that by reducing the population of vectors (responsible for the transmission of the disease from both animals and humans) in addition to the medical activities, in 147/158 zones it would be possible to achieve the goal of elimination of transmission by 2030, compared to 61/158 foreseen by the model without vector control (144).
With regards to rHAT, vector control plays a fundamental role in reducing the animal reservoir and therefore the risk of transmission to humans. Among the methods proposed, one of the most cost-effective seems to be the use of insecticide-treated cattle (ITC), in particular the restricted application of insecticides in the areas of the body of the animals most exposed to vector bites (foot and belly) every two weeks (145). In a study based on mathematical models this vector control strategy was found to be more advantageous than the use of trypanocides in a setting in Uganda where the presence of wild reservoirs is limited (146).
A Progressive Control Pathway has been proposed to achieve elimination of African Trypanosomiasis in animals and, in the context of vector control, suggests a first suppression phase, aimed at reducing the population density of tsetse, followed by an elimination phase. Some techniques are considered more effective in the suppression phase, as ITC (insecticide-treated cattle, see above), ITT (insecticide-treated target) and SAT (aerial spraying), while SIT (Sterile Insect Technique) seems to be more useful for the phase of elimination, considering that it is more effective when the number of vectors is lower (147).
However, according to a One Health approach, not only the economic sustainability of these techniques must be evaluated, but also the impact on the environment, biodiversity and human and animal health. In this regard, the sterile insect technique seems to be one of the most environment friendly (148).
Maybe even more than gHAT, vector control strategies for rHAT are extremely complex and require specific evaluations with teams of experts from various sectors (ecology, veterinary, medicine, epidemiology, etc.) and the awareness and involvement of stakeholders, including farmers, to develop the most appropriate strategies for each setting and the sustainability of these strategies should be assessed periodically.
Moreover, in order to optimize vector control activities for Human and Animal African Trypanosomiasis and not waste important resources in low-income settings, the impact of climate change on the distribution of the tsetse fly population must be considered. In fact, some mathematical models predict that the rising temperatures will cause the disappearance of tsetse flies from some current habitats and their movement to higher and cooler zones, currently inhospitable for them. This could lead to outbreaks of disease in areas that are not currently affected and it could make the strategies already put in place useless (149–151).
Discussion and conclusions
The early achievement of the objectives set by the WHO roadmap makes it possible to reach the goals by 2030. It would be useful to enhance training programs for healthcare workers and implement the development of highly reliable and easy-to-use rapid diagnostic tests taking into account practical needs (e.g. tests that do not require the cold chain and electricity). This would reduce the diagnostic delay which often characterizes both forms of HAT and which implies an increase in mortality and the persistence of human reservoirs.
Furthermore, the availability of new oral drugs for gHAT capable of crossing the blood-brain barrier, such as fexinidazole, would make it possible to overcome the diagnostic and therapeutic difficulties associated with peripheral settings. It would be useful to assess the efficacy of fexinidazole in the treatment of rHAT to expand the therapeutic armamentarium with low toxic, affordable and more manageable drugs, even reducing the direct and indirect costs associated with prolonged hospitalization.
Moreover the availability of an oral drug as acozoribole, which requires once administration and that could be active also in late stage, could make elimination strategies even more effective and simple, especially in peripheral settings (test-and-treat). The integration of efficient surveillance and health systems can play a key role in the elimination strategy of African trypanosomiasis. Furthermore, the effectiveness of these strategies could be increased through the implementation of a One Health approach, coordinating and strengthening the interaction between the various sectors involved (ecology, epidemiology, health system, veterinary services, sociology), as is being experienced in some countries (152, 153) and considering the impact of Climate Change on disease distribution in the coming years.
Author contributions
Conceptualization: RP, RN, and MLM; methodology FDG, DB, and AS; writing - original draft preparation LF, GGP, EP, SC, GG, and EDV; writing -review and editing: AR, VT, MC, GuP, CP, MFM, and FVS; supervision: TAB, GB, GiP, LS, CC, and EN. All authors contributed to the article and approved the submitted version.
Funding
This work has been funded by Ricerca Corrente on Neglected Tropical Diseases (Linea 1, Progetto 4) by Italian Ministry of Health.
Conflict of interest
The authors declare that the research was conducted in the absence of any commercial or financial relationships that could be construed as a potential conflict of interest.
Publisher’s note
All claims expressed in this article are solely those of the authors and do not necessarily represent those of their affiliated organizations, or those of the publisher, the editors and the reviewers. Any product that may be evaluated in this article, or claim that may be made by its manufacturer, is not guaranteed or endorsed by the publisher.
References
1. Kennedy PGE. Human African trypanosomiasis of the CNS: current issues and challenges. J Clin Invest (2004) 113(4):496–504. doi: 10.1172/JCI21052
2. Büscher P, Cecchi G, Jamonneau V, Priotto G. Human African trypanosomiasis. Lancet (London England) (2017) 390(10110):2397–409. doi: 10.1016/S0140-6736(17)31510-6
3. World Health Organization. Control and surveillance of human African trypanosomiasis. World Health Organ Tech Rep Ser (2013)(984), 1–237.
4. Kennedy PG. Clinical features, diagnosis, and treatment of human African trypanosomiasis (sleeping sickness). Lancet Neurol (2013) 12(2):186–94. doi: 10.1016/S1474-4422(12)70296-X
5. WHO. WHO interim guidelines for the treatment of gambiense human African trypanosomiasis. Geneva: World Health Organization (2019).
6. WHO. Ending the neglect to attain the sustainable development goals: a road map for neglected tropical diseases 2021–2030. Geneva: World Health Organization (2020). Available at: https://www.who.int/neglected_diseases/Revised-DraftNTD-Roadmap-23Apr2020.pdf.
7. Casulli A. New global targets for NTDs in the WHO roadmap 2021–2030. PloS Negl Trop Dis (2021) 15(5):e0009373. doi: 10.1371/journal.pntd.0009373
8. Consortium NM, Consortium NM. The potential impact of programmes interruptions due to COVID-19 on 7 neglected tropical diseases: a modelling-based analysis. Gates Open Res (2020) 4(July):115.
9. Toor J, Adams ER, Aliee M, Amoah B, Anderson RM, Ayabina D, et al. Predicted impact of COVID-19 on neglected tropical disease programs and the opportunity for innovation. Clin Infect Dis an Off Publ. Infect Dis Soc Am (2021) 72(8):1463–6. doi: 10.1093/cid/ciaa933
10. Aliee M, Castaño S, Davis CN, Patel S, Miaka EM, Spencer SEF, et al. Predicting the impact of COVID-19 interruptions on transmission of gambiense human African trypanosomiasis in two health zones of the democratic republic of Congo. Trans R Soc Trop Med Hyg (2021) 115(3):245–52. doi: 10.1093/trstmh/trab019
11. Kagabadouno M, Camara O, Camara M, Ilboudo H, Camara M, Rayaisse J-B, et al. Ebola Outbreak brings to light an unforeseen impact of tsetse control on sleeping sickness transmission in Guinea. bioRxiv, 202762. doi: 10.1101/202762
12. WHO Expert Committee on Epidemiology and Control of African Trypanosomiasis & World Health Organization. Epidemiology and control of African trypanosomiasis: report of a WHO expert committee [meeting held in Geneva from 16 to 23 October 1985]. World Health Organization. (1986). Available at: https://apps.who.int/iris/handle/10665/40346
13. Radwanska M, Chamekh M, Vanhamme L, Claes F, Magez S, Magnus E, et al. The serum resistance-associated gene as a diagnostic tool for the detection of trypanosoma brucei rhodesiense. Am J Trop Med Hyg (2002) 67(6):684–90. doi: 10.4269/ajtmh.2002.67.684
14. Berberof M, Pérez-Morga D, Pays E. A receptor-like flagellar pocket glycoprotein specific to trypanosoma brucei gambiense. Mol Biochem Parasitol (2001) 113(1):127–38. doi: 10.1016/s0166-6851(01)00208-0
15. Koffi M, De Meeûs T, Bucheton B, Solano P, Camara M, Kaba D, et al. Population genetics of trypanosoma brucei gambiense, the agent of sleeping sickness in Western Africa. Proc Natl Acad Sci U. S. A. (2009) 106(1):209–14. doi: 10.1073/pnas.0811080106
16. Oberle M, Balmer O, Brun R, Roditi I. Bottlenecks and the maintenance of minor genotypes during the life cycle of trypanosoma brucei. PloS Pathog (2010) 6(7):e1001023. doi: 10.1371/journal.ppat.1001023
17. Dyer NA, Rose C, Ejeh NO, Acosta-Serrano A. Flying tryps: survival and maturation of trypanosomes in tsetse flies. Trends Parasitol (2013) 29(4):188–96. doi: 10.1016/j.pt.2013.02.003
18. McCulloch R. Antigenic variation in African trypanosomes: monitoring progress. Trends Parasitol (2004) 20(3):117–21. doi: 10.1016/j.pt.2003.12.004
19. Berriman M, Ghedin E, Hertz-Fowler C, Blandin G, Renauld H, Bartholomeu DC, et al. The genome of the African trypanosome trypanosoma brucei. Science (2005) 309(5733):416–22. doi: 10.1126/science.1112642
20. Fenn K, Matthews KR. The cell biology of trypanosoma brucei differentiation. Curr Opin Microbiol (2007) 10(6):539–46. doi: 10.1016/j.mib.2007.09.014
21. Peacock L, Bailey M, Carrington M, Gibson W. Meiosis and haploid gametes in the pathogen trypanosoma brucei. Curr Biol (2014) 24(2):181–6. doi: 10.1016/j.cub.2013.11.044
22. Peacock L, Ferris V, Sharma R, Sunter J, Bailey M, Carrington M, et al. Identification of the meiotic life cycle stage of trypanosoma brucei in the tsetse fly. Proc Natl Acad Sci USA (2011) 108(9):3671–6. doi: 10.1073/pnas.1019423108
23. Turner CM, Aslam N, Dye C. Replication, differentiation, growth and the virulence of trypanosoma brucei infections. Parasitology (1995) 111(Pt 3):289–300. doi: 10.1017/s0031182000081841
24. Koffi M, Garcia A, Ravel S, Cuny G, Oury B, Solano P, et al. Genetic characterisation of trypanosoma brucei s.l. using microsatellite typing: new perspectives for the molecular epidemiology of human African trypanosomiasis. Infect Genet Evol J Mol Epidemiol. Evol Genet Infect Dis (2007) 7(6):675–84. doi: 10.1016/j.meegid.2007.07.001
25. Sharma R, Gluenz E, Peacock L, Gibson W, Gull K, Carrington M. The heart of darkness: growth and form of trypanosoma brucei in the tsetse fly. Trends Parasitol (2009) 25(11):517–24. doi: 10.1016/j.pt.2009.08.001
26. Franco JR, Simarro PP, Diarra A, Jannin JG. Epidemiology of human African trypanosomiasis. Clin Epidemiol. (2014) 6:257–75. doi: 10.2147/CLEP.S39728
27. Pépin J, Méda HA. The epidemiology and control of human African trypanosomiasis. Adv Parasitol (2001) 49:71–132. doi: 10.1016/s0065-308x(01)49038-5
28. MacGregor P, Szöőr B, Savill NJ, Matthews KR. Trypanosomal immune evasion, chronicity and transmission: an elegant balancing act. Nat Rev Microbiol (2012) 10(6):431–8. doi: 10.1038/nrmicro2779
29. Louis FJ, Simarro PP, Lucas P. [Sleeping sickness: one hundred years of control strategy evolution]. Bull Soc Pathol Exot. (2002) 95(5):331–6.
30. WHO. Distribution of human African trypanosomiasis (T.B. rhodesiense) (2020). Available at: https://www.who.int/data/gho/map-gallery-search-results?&maptopics=910b5dfc-ce2e-4440-8b43-8d83f4a85485&term=humanAfricantrypanosomiasis.
31. WHO. Distribution of human African trypanosomiasis (T.B. gambiense) (2020). Available at: https://www.who.int/images/default-source/maps/hat_ga_2020.png?sfvrsn=8ea050ef_3.
32. Simarro PP, Cecchi G, Franco JR, Paone M, Diarra A, Ruiz-Postigo JA, et al. Estimating and mapping the population at risk of sleeping sickness. PloS Negl Trop Dis (2012) 6(10):e1859. doi: 10.1371/journal.pntd.0001859
33. WHO. Number of new reported cases of human African trypanosomiasis (2022). Available at: https://www.who.int/data/gho/data/indicators/indicator-details/GHO/hat-tb-gambiense.
34. Njiokou F, Nimpaye H, Simo G, Njitchouang GR, Asonganyi T, Cuny G, et al. Domestic animals as potential reservoir hosts of trypanosoma brucei gambiense in sleeping sickness foci in Cameroon. Parasite (2010) 17(1):61–6. doi: 10.1051/parasite/2010171061
35. Jamonneau V, Ravel S, Koffi M, Kaba D, Zeze DG, Ndri L, et al. Mixed infections of trypanosomes in tsetse and pigs and their epidemiological significance in a sleeping sickness focus of côte d’Ivoire. Parasitology (2004) 129(Pt 6):693–702. doi: 10.1017/s0031182004005876
36. Balyeidhusa ASP, Kironde FAS, Enyaru JCK. Apparent lack of a domestic animal reservoir in gambiense sleeping sickness in northwest Uganda. Vet Parasitol (2012) 187(1–2):157–67. doi: 10.1016/j.vetpar.2011.12.005
37. Njiokou F, Laveissière C, Simo G, Nkinin S, Grébaut P, Cuny G, et al. Wild fauna as a probable animal reservoir for trypanosoma brucei gambiense in Cameroon. Infect Genet Evol J Mol Epidemiol. Evol Genet Infect Dis (2006) 6(2):147–53. doi: 10.1016/j.meegid.2005.04.003
38. Cordon-Obras C, Berzosa P, Ndong-Mabale N, Bobuakasi L, Buatiche JN, Ndongo-Asumu P, et al. Trypanosoma brucei gambiense in domestic livestock of kogo and mbini foci (Equatorial Guinea). Trop Med Int Health (2009) 14(5):535–41. doi: 10.1111/j.1365-3156.2009.02271
39. Grebaut P, Bodo JM, Assona A, Foumane Ngane V, Njiokou F, Ollivier G, et al. [Risk factors for human African trypanosomiasis in the bipindi region of Cameroon]. Med Trop (2001) 61:4–5377–83.
40. Moore A, Richer M, Enrile M, Losio E, Roberts J, Levy D. Resurgence of sleeping sickness in tambura county, Sudan. Am J Trop Med Hyg (1999) 61(2):315–8. doi: 10.4269/ajtmh.1999.61.315
41. Henry MC, Ruppol JF, Bruneel H. “[Distribution of infection by t. brucei gambiense in a population of bandundu in the republic of Zaire]. Ann Soc Belg. Med Trop (1920) 62(4):301–13.
42. Courtin F, Jamonneau V, Camara M, Camara O, Coulibaly B, Diarra A, et al. A geographical approach to identify sleeping sickness risk factors in a mangrove ecosystem. Trop Med Int Health (2010) 15(8):881–9. doi: 10.1111/j.1365-3156.2010.02559
43. Khonde N, Pépin J, Niyonsenga T, De Wals P. Familial aggregation of trypanosoma brucei gambiense trypanosomiasis in a very high incidence community in Zaire. Trans R Soc Trop Med Hyg (1997) 91(5):521–4. doi: 10.1016/s0035-9203(97)90008-0
44. Henry MC. [Importance of familial contamination in trypanosoma brucei gambiense trypanosomiasis]. Bull Soc Pathol Exot. Filiales (1981) 74(1):65–71.
45. WHO. Number of new reported cases of human African trypanosomiasis (T.b. rhodesiense) (2022). Available at: https://www.who.int/data/gho/data/indicators/indicator-details/GHO/number-of-new-reported-cases-of-human-african-trypanosomiasis-(t-b-rhodesiense).
46. Hide G, Tait A, Maudlin I, Welburn SC. The origins, dynamics and generation of trypanosoma brucei rhodesiense epidemics in East Africa. Parasitol Today (1996) 12(2):50–5. doi: 10.1016/0169-4758(96)80654-5
47. Waiswa C, Olaho-Mukani W, Katunguka-Rwakishaya E. Domestic animals as reservoirs for sleeping sickness in three endemic foci in south-eastern Uganda. Ann Trop Med Parasitol (2003) 97(2):149–55. doi: 10.1179/000349803235001688
48. von Wissmann B, Machila N, Picozzi K, Fèvre EM, deC Bronsvoort BM, Handel IG, et al. Factors associated with acquisition of human infective and animal infective trypanosome infections in domestic livestock in Western Kenya. PloS Negl Trop Dis (2011) 5(1):e941. doi: 10.1371/journal.pntd.0000941
49. Njagu Z, Mihok S, Kokwaro E, Verloo D. Isolation of trypanosoma brucei from the monitor lizard (Varanus niloticus) in an endemic focus of Rhodesian sleeping sickness in Kenya. Acta Trop (1999) 72(2):137–48. doi: 10.1016/s0001-706x(98)00092-8
50. Allsopp R. The role of game animals in the maintenance of endemic and enzootic trypanosomiases in the lambwe valley, south nyanza district, Kenya. Bull World Health Organ. (1972) 47(6):735–46.
51. Odiit M, Bessell PR, Fèvre EM, Robinson T, Kinoti J, Coleman PG, et al. Using remote sensing and geographic information systems to identify villages at high risk for rhodesiense sleeping sickness in Uganda. Trans R Soc Trop Med Hyg (2006) 100(4):354–62. doi: 10.1016/j.trstmh.2005.04.022
52. Abaru DE. “Sleeping sickness in busoga, Uganda, 1976-1983.,”. In: Trop. med. parasitol. off. organ dtsch. tropenmedizinische gesellschaft dtsch. gesellschaft fur tech. zusammenarbeit, vol. 36. (1985). p. 72–6.
53. Simarro PP, Franco JR, Diarra A, Ruiz Postigo JA, Jannin J. Diversity of human African trypanosomiasis epidemiological settings requires fine-tuning control strategies to facilitate disease elimination. Res Rep Trop Med (2013) 4:1–6. doi: 10.2147/RRTM.S40157
54. Picozzi K, Fèvre EM, Odiit M, Carrington M, Eisler MC, Maudlin I, et al. Sleeping sickness in Uganda: a thin line between two fatal diseases. BMJ (2005) 331(7527):1238–41. doi: 10.1136/bmj.331.7527.1238
55. Selby R, Bardosh K, Picozzi K, Waiswa C, Welburn SC. Cattle movements and trypanosomes: restocking efforts and the spread of trypanosoma brucei rhodesiense sleeping sickness in post-conflict Uganda. Parasitol Vectors (2013) 6(1):281. doi: 10.1186/1756-3305-6-281
56. Welburn SC, Fèvre EM, Coleman PG, Odiit M, Maudlin I. Sleeping sickness: a tale of two diseases. Trends Parasitol (2001) 17(1):19–24. doi: 10.1016/s1471-4922(00)01839-0
57. Gao J-M, Qian Z-Y, Hide G, Lai D-H, Lun Z-R, Wu Z-D. Human African trypanosomiasis: the current situation in endemic regions and the risks for non-endemic regions from imported cases. Parasitology (2020) 147(9):922–31. doi: 10.1017/S0031182020000645
58. Woodruff AW, Evans DA, Owino NO. A ‘healthy’ carrier of African trypanosomiasis. J Infect (1982) 5(1):89–92. doi: 10.1016/S0163-4453(82)93389-8
59. Sina G, Testa G, Triolo N, Trova P, Cramet B. [Some new cases of congenital human African trypanosomiasis (T. gambiense) (author’s transl)]. Med Trop (1979) 39(1):57–63.
60. Lindner AK, Priotto G. The unknown risk of vertical transmission in sleeping sickness–a literature review. PloS Negl Trop Dis (2010) 4(12):e783. doi: 10.1371/journal.pntd.0000783
61. Herwaldt BL. Laboratory-acquired parasitic infections from accidental exposures. Clin Microbiol Rev (2001) 14(4):659–88. doi: 10.1128/CMR.14.3.659-688.2001
62. Hira PR, Husein SF. Some transfusion-induced parasitic infections in Zambia. J Hyg Epidemiol. Microbiol Immunol (1979) 23(4):436–44.
63. Martín-Dávila P, Fortún J, López-Vélez R, Norman F, Montes de Oca M, Zamarrón P, et al. Transmission of tropical and geographically restricted infections during solid-organ transplantation. Clin Microbiol Rev (2008) 21(1):60–96. doi: 10.1128/CMR.00021-07
64. Rocha G, Martins A, Gama G, Brandão F, Atouguia J. Possible cases of sexual and congenital transmission of sleeping sickness. Lancet (2004) 363(9404):247. doi: 10.1016/S0140-6736(03)15345-7
65. CDC. Parasites - African trypanosomiasis (also known as sleeping sickness) (2020). Available at: https://www.cdc.gov/parasites/sleepingsickness/disease.html (Accessed Apr. 28, 2020).
66. Checchi F, Filipe JAN, Haydon DT, Chandramohan D, Chappuis F. Estimates of the duration of the early and late stage of gambiense sleeping sickness. BMC Infect Dis (2008) 8:16. doi: 10.1186/1471-2334-8-16
67. Jamonneau V, Ilboudo H, Kaboré J, Kaba D, Koffi M, Solano P, et al. Untreated human infections by trypanosoma brucei gambiense are not 100% fatal. PloS Negl Trop Dis (2012) 6(6):e1691. doi: 10.1371/journal.pntd.0001691
68. Kennedy PGE, Rodgers J. Clinical and neuropathogenetic aspects of human African trypanosomiasis. Front Immunol (2019) 10:39. doi: 10.3389/fimmu.2019.00039
69. Blum J, Schmid C, Burri C. Clinical aspects of 2541 patients with second stage human African trypanosomiasis. Acta Trop (2006) 97(1):55–64. doi: 10.1016/j.actatropica.2005.08.001
70. Urech K, Neumayr A, Blum J. Sleeping sickness in travelers - do they really sleep? PloS Negl Trop Dis (2011) 5(11):e1358. doi: 10.1371/journal.pntd.0001358
71. Wengert O, Kopp M, Siebert E, Stenzel W, Hegasy G, Suttorp N, et al. Human African trypanosomiasis with 7-year incubation period: clinical, laboratory and neuroimaging findings. Parasitol Int (2014) 63(3):557–60. doi: 10.1016/j.parint.2014.02.003
72. Paul M, Stefaniak J, Smuszkiewicz P, van Esbroeck M, Geysen D, Clerinx J. Outcome of acute East African trypanosomiasis in a polish traveller treated with pentamidine. BMC Infect Dis (2014) 14(1):111. doi: 10.1186/1471-2334-14-111
73. Jamonneau V, Truc P, Garcia A, Magnus E, Büscher P. Preliminary evaluation of LATEX/T. b. gambiense and alternative versions of CATT/T. b. gambiense for the serodiagnosis of human african trypanosomiasis of a population at risk in côte d’Ivoire: considerations for mass-screening. Acta Trop (2000) 76(2):175–83. doi: 10.1016/s0001-706x(00)00095-4
74. Álvarez-Rodríguez A, Jin B-K, Radwanska M, Magez S. Recent progress in diagnosis and treatment of human African trypanosomiasis has made the elimination of this disease a realistic target by 2030. Front Med (2022) 9:1037094. doi: 10.3389/fmed.2022.1037094
75. Büscher P, Mumba Ngoyi D, Kaboré J, Lejon V, Robays J, Jamonneau V, et al. Improved models of mini anion exchange centrifugation technique (mAECT) and modified single centrifugation (MSC) for sleeping sickness diagnosis and staging. PloS Negl Trop Dis (2009) 3(11):2–4. doi: 10.1371/journal.pntd.0000471
76. Chappuis F, Loutan L, Simarro P, Lejon V, Büscher P. Options for field diagnosis of human african trypanosomiasis. Clin Microbiol Rev (2005) 18(1):133–46. doi: 10.1128/CMR.18.1.133-146.2005
77. Greenwood BM, Whittle HC. Complement activation in patients with Gambian sleeping sickness. Clin Exp Immunol (1976) 24(1):133–8.
78. De Koning HP. The drugs of sleeping sickness: Their mechanisms of action and resistance, and a brief history. Trop Med Infect Dis (2020) 5(1):14. doi: 10.3390/tropicalmed5010014
79. Benaim G, Lopez-Estraño C, Docampo R, Moreno SN. A calmodulin-stimulated Ca2+ pump in plasma-membrane vesicles from trypanosoma brucei; selective inhibition by pentamidine. Biochem J (1993) 296(Pt 3):759–63. doi: 10.1042/bj2960759
80. Lanteri CA, Tidwell RR, Meshnick SR. The mitochondrion is a site of trypanocidal action of the aromatic diamidine DB75 in bloodstream forms of trypanosoma brucei. Antimicrob Agents Chemother (2008) 52(3):875–82. doi: 10.1128/AAC.00642-07
81. Thomas JA, Baker N, Hutchinson S, Dominicus C, Trenaman A, Glover L, et al. Insights into antitrypanosomal drug mode-of-action from cytology-based profiling. PloS Negl Trop Dis (2018) 12(11):e0006980. doi: 10.1371/journal.pntd.0006980
82. Baker N, de Koning HP, Mäser P, Horn D. Drug resistance in African trypanosomiasis: the melarsoprol and pentamidine story. Trends Parasitol (2013) 29(3):110–8. doi: 10.1016/j.pt.2012.12.005
83. Munday JC, Eze AA, Baker N, Glover L, Clucas C, Aguinaga Andrés D, et al. Trypanosoma brucei aquaglyceroporin 2 is a high-affinity transporter for pentamidine and melaminophenyl arsenic drugs and the main genetic determinant of resistance to these drugs. J Antimicrob Chemother (2014) 69(3):651—663. doi: 10.1093/jac/dkt442
84. Doua F, Miezan TW, Sanon Singaro JR, Boa Yapo F, Baltz T. The efficacy of pentamidine in the treatment of early-late stage trypanosoma brucei gambiense trypanosomiasis. Am J Trop Med Hyg (1996) 55(6):586–8. doi: 10.4269/ajtmh.1996.55.586
85. Kande Betu Ku Mesu V, Mutombo Kalonji W, Bardonneau C, Valverde Mordt O, Ngolo Tete D, Blesson S, et al. Oral fexinidazole for stage 1 or early stage 2 African trypanosoma brucei gambiense trypanosomiasis: a prospective, multicentre, open-label, cohort study. Lancet Glob Heal (2021) 9(7):e999–e1008. doi: 10.1016/S2214-109X(21)00208-4
86. Mesu VKBK, Kalonji WM, Bardonneau C, Mordt OV, Blesson S, Simon F, et al. Oral fexinidazole for late-stage African trypanosoma brucei gambiense trypanosomiasis: a pivotal multicentre, randomised, non-inferiority trial. Lancet (2018) 391(10116):144–54. doi: 10.1016/S0140-6736(17)32758-7
87. European Medicines Agency (EMA). Fexinidazole winthrop product information. Available at: https://www.ema.europa.eu/en/documents/outside-eu-product-information/fexinidazole-winthrop-product-information_en.pdf.
88. Drugs for Neglected Diseases initiative. Fexinidazole for t.b. rhodesiense (2021). Available at: https://dndi.org/research-development/portfolio/fexinidazole-tb-rhodesiense/ (Accessed Dec. 13, 2021).
89. Drugs for Neglected Diseases. Efficacy and safety of fexinidazole in patients with human African trypanosomiasis (HAT) due to trypanosoma brucei rhodesiense . Available at: https://clinicaltrials.gov/ct2/show/NCT03974178 (Accessed Aug. 30, 2021).
90. Vincent IM, Creek D, Watson DG, Kamleh MA, Woods DJ, Wong PE, et al. A molecular mechanism for eflornithine resistance in African trypanosomes. PloS Pathog (2010) 6(11):1–9. doi: 10.1371/journal.ppat.1001204
91. Wilkinson SR, Taylor MC, Horn D, Kelly JM, Cheeseman I. A mechanism for cross-resistance to nifurtimox and benznidazole in trypanosomes. Proc Natl Acad Sci (2008) 105(13):5022–7. doi: 10.1073/pnas.0711014105
92. Sokolova AY, Wyllie S, Patterson S, Oza SL, Read KD, Fairlamb AH. “Cross-resistance to nitro drugs and implications for treatment of human African trypanosomiasis.,”. Antimicrob Agents Chemother (2010) 54(7):2893–900. doi: 10.1128/AAC.00332-10
93. Barrett MP, Vincent IM, Burchmore RJS, Kazibwe AJN, Matovu E. “Drug resistance in human African trypanosomiasis.,”. Future Microbiol (2011) 6(9):1037–47. doi: 10.2217/fmb.11.88
94. Priotto G, Fogg C, Balasegaram M, Erphas O, Louga A, Checchi F, et al. Three drug combinations for late-stage trypanosoma brucei gambiense sleeping sickness: a randomized clinical trial in Uganda. PloS Clin Trials (2006) 1(8):e39. doi: 10.1371/journal.pctr.0010039
95. Checchi F, Piola P, Ayikoru H, Thomas F, Legros D, Priotto G. Nifurtimox plus eflornithine for late-stage sleeping sickness in Uganda: a case series. PloS Negl Trop Dis (2007) 1(2):e64. doi: 10.1371/journal.pntd.0000064
96. Kansiime F, Adibaku S, Wamboga C, Idi F, Kato CD, Yamuah L, et al. A multicentre, randomised, non-inferiority clinical trial comparing a nifurtimox-eflornithine combination to standard eflornithine monotherapy for late-stage trypanosoma brucei gambiense human African trypanosomiasis in Uganda. Parasitol Vectors (2018) 11(1):105. doi: 10.1186/s13071-018-2634-x
97. Priotto G, Kasparian S, Ngouama D, Ghorashian S, Arnold U, Ghabri S, et al. Nifurtimox-eflornithine combination therapy for second-stage trypanosoma brucei gambiense sleeping sickness: A randomized clinical trial in Congo. Clin Infect Dis (2007) 45(11):1435–42. doi: 10.1086/522982
98. Priotto G, Kasparian S, Mutombo W, Ngouama D, Ghorashian S, Arnold U, et al. Nifurtimox-eflornithine combination therapy for second-stage African trypanosoma brucei gambiense trypanosomiasis: a multicentre, randomised, phase III, non-inferiority trial. Lancet (2009) 374(9683):56–64. doi: 10.1016/S0140-6736(09)61117-X
99. Alirol E, Schrumpf D, Amici Heradi J, Riedel A, de Patoul C, Quere M, et al. Nifurtimox-eflornithine combination therapy for second-stage gambiense human African trypanosomiasis: Médecins sans frontières experience in the democratic republic of the Congo. Clin Infect Dis (2013) 56(2):195–203. doi: 10.1093/cid/cis886
100. Yun O, Priotto G, Tong J, Flevaud L, Chappuis F. NECT is next: Implementing the new drug combination therapy for trypanosoma brucei gambiense sleeping sickness. PloS Negl Trop Dis (2010) 4(5):e720. doi: 10.1371/journal.pntd.0000720
101. Jaffe JJ, McCormack JJ, Meymarian E. Comparative properties of schistosomal and filarial dihydrofolate reductases. Biochem Pharmacol (1972) 21(5):719–31. doi: 10.1016/0006-2952(72)90064-0
102. Chello PL, Jaffe JJ. Comparative properties of trypanosomal and mammalian thymidine kinases. Comp Biochem Physiol Part B Comp Biochem (1972) 43(3):543–62. doi: 10.1016/0305-0491(72)90138-1
103. Willson M, Callens M, Kuntz DA, Perié J, Opperdoes FR. “Synthesis and activity of inhibitors highly specific for the glycolytic enzymes from trypanosoma brucei.,”. Mol Biochem Parasitol (1993) 59(2):201–10. doi: 10.1016/0166-6851(93)90218-m
104. Wiedemar N, Hauser DA, Mäser P. 100 years of suramin. Antimicrob Agents Chemother (2020) 64(3):e01168-19. doi: 10.1128/AAC.01168-19
105. Wiedemar N, Graf FE, Zwyer M, Ndomba E, Kunz Renggli C, Cal M, Schmidt RS, et al. Beyond immune escape: a variant surface glycoprotein causes suramin resistance in trypanosoma brucei. Mol Microbiol (2018) 107(1):57–67. doi: 10.1111/mmi.13854
106. Zeelen J, van Straaten M, Verdi J, Hempelmann A, Hashemi H, Perez K, et al. Structure of trypanosome coat protein VSGsur and function in suramin resistance. Nat Microbiol (2021) 6(3):392–400. doi: 10.1038/s41564-020-00844-1
107. Fairlamb AH, Horn D. Melarsoprol resistance in African trypanosomiasis. Trends Parasitol (2018) 34(6):481–92. doi: 10.1016/j.pt.2018.04.002
108. Robays J, Nyamowala G, Sese C, Betu Ku Mesu Kande V, Lutumba P, Van der Veken W, et al. High failure rates of melarsoprol for sleeping sickness, democratic republic of Congo. Emerg Infect Dis (2008) 14(6):966–7. doi: 10.3201/eid1406.071266
109. Legros D, Fournier C, Gastellu Etchegorry M, Maiso F, Szumilin E. Therapeutic failure of melarsoprol among patients treated for late stage t.b. gambiense human African trypanosomiasis in Uganda. Bull Soc Pathol Exot. (1999) 92(3):171–2.
110. Legros D, Evans S, Maiso F, Enyaru JC, Mbulamberi D. Risk factors for treatment failure after melarsoprol for trypanosoma brucei gambiense trypanosomiasis in Uganda. Trans R Soc Trop Med Hyg (1999) 93(4):439–42. doi: 10.1016/s0035-9203(99)90151-7
111. Stanghellini A, Josenando T. The situation of sleeping sickness in Angola: a calamity. Trop Med Int Heal (2001) 6(5):330–4. doi: 10.1046/j.1365-3156.2001.00724.x
112. Moore A, Richer M. Re-emergence of epidemic sleeping sickness in southern Sudan. Trop Med Int Heal (2001) 6(5):342–7. doi: 10.1046/j.1365-3156.2001.00714.x
113. Seixas J, Atouguia J, Josenando T, Vatunga G, Bilenge CMM, Lutumba P, et al. Clinical study on the melarsoprol-related encephalopathic syndrome: Risk factors and HLA association. Trop Med Infect Dis (2020) 5(1):5. doi: 10.3390/tropicalmed5010005
114. Blum J, Nkunku S, Burri C. Clinical description of encephalopathic syndromes and risk factors for their occurrence and outcome during melarsoprol treatment of human African trypanosomiasis. Trop Med Int Health (2001) 6(5):390–400. doi: 10.1046/j.1365-3156.2001.00710.x
115. Pepin J, Milord F, Guern C, Mpia B, Ethier L, Mansinsa D. Trial of prednisolone for prevention of melarsoprol-induced encephalopathy in gambiense sleeping sickness. Lancet (1989) 1(8649):1246–50. doi: 10.1016/s0140-6736(89)92340-4
116. Rodgers J, Jones A, Gibaud S, Bradley B, McCabe C, Barrett MP, et al. Melarsoprol cyclodextrin inclusion complexes as promising oral candidates for the treatment of human African trypanosomiasis. PloS Negl Trop Dis (2011) 5(9):e1308. doi: 10.1371/journal.pntd.0001308
117. European Medicines Agency. Committee for orphan medicinal products (COMP) meeting report on the review of applications for orphan designation - 12 January 2015. EMA (2015). Available at: http://www.ema.europa.eu/ema/index.jspcurl=pages/news_and_events/document_listing/document_listing_000201.jsp&mid=WC0b01ac0580028e78.
118. Betu Kumeso VK, Kalonji WM, Rembry S, Valverde Mordt O, Ngolo Tete D, Prêtre A, et al. Efficacy and safety of acoziborole in patients with human African trypanosomiasis caused by trypanosoma brucei gambiense: a multicentre, open-label, single-arm, phase 2/3 trial. Lancet Infect Dis (2023) 23(4):463–470. doi: 10.1016/S1473-3099(22)00660-0
119. Drugs for Neglected Diseases initiative. Acoziborole. Available at: https://dndi.org/research-development/portfolio/acoziborole/ (Accessed Mar. 01, 2022).
120. Drugs for Neglected Diseases initiative. Clinical study protocol: Efficacy and safety study of acoziborole (2020). Available at: https://dndi.org/wp-content/uploads/2022/05/DNDi-Clinical-Study-Protocol-OXA002-V4.pdf (Accessed Aug. 11, 2020).
121. Jamonneau V, Camara O, Ilboudo H, Peylhard M, Koffi M, Sakande H, et al. Accuracy of individual rapid tests for serodiagnosis of gambiense sleeping sickness in West Africa. PloS Negl Trop Dis (2015) 9(2):e0003480. doi: 10.1371/journal.pntd.0003480
122. Geerts M, Van Reet N, Leyten S, Berghmans R, Rock KS, Coetzer THT, et al. Trypanosoma brucei gambiense-iELISA: A promising new test for the post-elimination monitoring of human African trypanosomiasis. Clin Infect Dis an Off Publ. Infect Dis Soc Am (2021) 73(9):e2477–83. doi: 10.1093/cid/ciaa1264
123. Lejon V, Jacobs J, Simarro PP. Elimination of sleeping sickness hindered by difficult diagnosis. Bull World Health Organ (2013) 91(10):718. doi: 10.2471/BLT.13.126474
124. Matemba LE, Fèvre EM, Kibona SN, Picozzi K, Cleaveland S, Shaw AP, et al. Quantifying the burden of rhodesiense sleeping sickness in urambo district, Tanzania. PloS Negl Trop Dis (2010) 4(11):e868. doi: 10.1371/journal.pntd.0000868
125. Odiit M, Kansiime F, Enyaru JC. Duration of symptoms and case fatality of sleeping sickness caused by trypanosoma brucei rhodesiense in tororo, Uganda. East Afr. Med J (1997) 74(12):792–5.
126. Kabasa JD. Public-private partnership works to stamp out sleeping sickness in Uganda. Trends Parasitol (2007) 23(5):191–2. doi: 10.1016/j.pt.2007.03.006
127. Jordan AM. Trypanosomiasis control and African rural development. London; New York: Longman (1986).
128. Magona JW, Walubengo J. Mass-treatment and insecticide-spraying of animal reservoirs for emergency control of rhodesiense sleeping sickness in Uganda. J Vector Borne Dis (2011) 48(2):105–8.
129. Lindh JM, Goswami P, Blackburn RS, Arnold SE, Vale GA, Lehane MJ, et al. Optimizing the colour and fabric of targets for the control of the tsetse fly glossina fuscipes fuscipes. PloS Negl Trop Dis (2012) 6(5):e1661. doi: 10.1371/journal.pntd.0001661
130. Green CH. Effects of colours and synthetic odours on the attraction of glossina pallidipes and g. morsitans morsitans to traps and screens. Physiol Entomol (1986) 11(4):411–21. doi: 10.1111/j.1365-3032.1986.tb00432.x
131. Vale GA, Lovemore DF, Flint S, Cockbill GF. Odour-baited targets to control tsetse flies, glossina spp. (Diptera: Glossinidae), in Zimbabwe. Bull Entomol Res (1988) 78(1):31–49. doi: 10.1017/S0007485300016059
132. Esterhuizen J, Rayaisse JB, Tirados I, Mpiana S, Solano P, Vale GA, et al. Improving the cost-effectiveness of visual devices for the control of riverine tsetse flies, the major vectors of human African trypanosomiasis. PloS Negl Trop Dis (2011) 5(8):e1257. doi: 10.1371/journal.pntd.0001257
133. Tirados I, Esterhuizen J, Rayaisse JB, Diarrassouba A, Kaba D, Mpiana S, et al. How do tsetse recognise their hosts? the role of shape in the responses of tsetse (Glossina fuscipes and g. palpalis) to artificial hosts. PloS Negl Trop Dis (2011) 5(8):e1226. doi: 10.1371/journal.pntd.0001226
134. Solano P, Torr S, & Lehane M. Is vector control needed to eliminate gambiense human African trypanosomiasis? Front Cell Infection Microbiol (2013) 3:33. doi: 10.3389/fcimb.2013.00033
135. Lehane M, Alfaroukh I, Bucheton B, Camara M, Harris A, Kaba D, et al. Tsetse control and the elimination of Gambian sleeping sickness. PloS Negl Trop Dis (2016) 10(4):e0004437. doi: 10.1371/journal.pntd.0004437
136. Rayaisse J-B, Courtin F, Mahamat MH, Chérif M, Yoni W, Gadjibet NMO, et al. Delivering ‘tiny targets’ in a remote region of southern Chad: a cost analysis of tsetse control in the mandoul sleeping sickness focus. Parasites Vectors (2020) 13(1):419. doi: 10.1186/s13071-020-04286-w
137. Tirados I, Hope A, Selby R, Mpembele F, Miaka EM, Boelaert M, et al. Impact of tiny targets on Glossina fuscipes quanzensis, the primary vector of human African trypanosomiasis in the democratic republic of the Congo. PloS Negl Trop Dis (2020) 14(10):e0008270. doi: 10.1371/journal.pntd.0008270
138. Mahamat MH, Peka M, Rayaisse J-B, Rock KS, Toko MA, Darnas J, et al. Adding tsetse control to medical activities contributes to decreasing transmission of sleeping sickness in the mandoul focus (Chad). PloS Negl Trop Dis (2017) 11(7):e0005792. doi: 10.1371/journal.pntd.0005792
139. Hope A, Mugenyi A, Esterhuizen J, Tirados I, Cunningham L, Garrod G, et al. Scaling up of tsetse control to eliminate Gambian sleeping sickness in northern Uganda. PloS Negl Trop Dis (2022) 16(6):e0010222. doi: 10.1371/journal.pntd.0010222
140. Shaw APM, Tirados I, Mangwiro CTN, Esterhuizen J, Lehane MJ, Torr SJ, et al. Costs of using “Tiny targets” to control glossina fuscipes fuscipes, a vector of gambiense sleeping sickness in arua district of Uganda. PloS Negl Trop Dis (2015) 9(3):e0003624. doi: 10.1371/journal.pntd.0003624
141. Antillon M, Huang C-I, Crump RE, Brown PE, Snijders R, Miaka EM, et al. Cost-effectiveness of sleeping sickness elimination campaigns in five settings of the democratic republic of Congo. Nat Commun (2022) 13(1):1051. doi: 10.1038/s41467-022-28598-w
142. Cunningham LJ, Lingley JK, Tirados I, Esterhuizen J, Opiyo M, Mangwiro CTN, et al. Evidence of the absence of human African trypanosomiasis in two northern districts of Uganda: Analyses of cattle, pigs and tsetse flies for the presence of trypanosoma brucei gambiense. PloS Negl Trop Dis (2020) 14(4):e0007737. doi: 10.1371/journal.pntd.0007737
143. Funk S, Nishiura H, Heesterbeek H, Edmunds WJ, Checchi F. Identifying transmission cycles at the human-animal interface: The role of animal reservoirs in maintaining gambiense human African trypanosomiasis. PloS Comput Biol (2013) 9(1):e1002855. doi: 10.1371/journal.pcbi.1002855
144. Crump RE, Huang C-I, Spencer SEF, Brown PE, Shampa C, Mwamba Miaka E, et al. Modelling to infer the role of animals in gambiense human African trypanosomiasis transmission and elimination in the DRC. PloS Negl Trop Dis (2022) 16(7):e0010599. doi: 10.1371/journal.pntd.0010599
145. Torr SJ, Maudlin I, Vale GA. Less is more: restricted application of insecticide to cattle to improve the cost and efficacy of tsetse control. Med Vet Entomol (2007) 21(1):53–64. doi: 10.1111/j.1365-2915.2006.00657.x
146. Hargrove JW, Ouifki R, Kajunguri D, Vale GA, Torr SJ. Modeling the control of trypanosomiasis using trypanocides or insecticide-treated livestock. PloS Negl Trop Dis (2012) 6(5):e1615. doi: 10.1371/journal.pntd.0001615
147. Diall O, Cecchi G, Wanda G, Argilés-Herrero R, Vreysen MJB, Cattoli G, et al. Developing a progressive control pathway for African animal trypanosomosis. Trends Parasitol (2017) 33(7):499–509. doi: 10.1016/j.pt.2017.02.005
148. Bourtzis K, Vreysen MJB. Sterile insect technique (SIT) and its applications. Insects (2021) 12(7):638. doi: 10.3390/insects12070638
149. Longbottom J, Caminade C, Gibson HS, Weiss DJ, Torr S, Lord JS. Modelling the impact of climate change on the distribution and abundance of tsetse in northern Zimbabwe. Parasites Vectors (2020) 13:526. doi: 10.1186/s13071-020-04398-3
150. Nnko HJ, Gwakisa PS, Ngonyoka A, Sindato C, Estes AB. Potential impacts of climate change on geographical distribution of three primary vectors of African trypanosomiasis in tanzania’s maasai steppe. PloS Negl Trop Dis (2021) 15(2):e0009081. doi: 10.1371/journal.pntd.0009081
151. Lord JS, Hargrove JW, Torr SJ, Vale GA. Climate change and African trypanosomiasis vector populations in zimbabwe’s Zambezi valley: A mathematical modelling study. PloS Med (2018) 15(10):e1002675. doi: 10.1371/journal.pmed.1002675
152. Mulenga GM, Namangala B, Chilongo K, Henning L, Gummow B. Policy and linkages in the application of a one health system for reporting and controlling African trypanosomiasis and other zoonotic diseases in Zambia. Pathogens (2022) 11(1):30. doi: 10.3390/pathogens11010030
Keywords: Human African Trypanosomiasis (HAT), sleeping sickness (trypanosomiasis), Neglected Tropical Disease (NTD), acoziborole, fexinidazole, elimination strategies, rhodesiense HAT, gambiense HAT
Citation: Papagni R, Novara R, Minardi ML, Frallonardo L, Panico GG, Pallara E, Cotugno S, Ascoli Bartoli T, Guido G, De Vita E, Ricciardi A, Totaro V, Camporeale M, Segala FV, Bavaro DF, Patti G, Brindicci G, Pellegrino C, Mariani MF, Putoto G, Sarmati L, Castellani C, Saracino A, Di Gennaro F and Nicastri E (2023) Human African Trypanosomiasis (sleeping sickness): Current knowledge and future challenges. Front. Trop. Dis 4:1087003. doi: 10.3389/fitd.2023.1087003
Received: 01 November 2022; Accepted: 27 March 2023;
Published: 09 May 2023.
Edited by:
Angela H. Lopes, Federal University of Rio de Janeiro, BrazilReviewed by:
Adekunle Sanyaolu, Federal Ministry of Health (Nigeria), NigeriaStefan Magez, Vrije University Brussels, Belgium
Copyright © 2023 Papagni, Novara, Minardi, Frallonardo, Panico, Pallara, Cotugno, Ascoli Bartoli, Guido, De Vita, Ricciardi, Totaro, Camporeale, Segala, Bavaro, Patti, Brindicci, Pellegrino, Mariani, Putoto, Sarmati, Castellani, Saracino, Di Gennaro and Nicastri. This is an open-access article distributed under the terms of the Creative Commons Attribution License (CC BY). The use, distribution or reproduction in other forums is permitted, provided the original author(s) and the copyright owner(s) are credited and that the original publication in this journal is cited, in accordance with accepted academic practice. No use, distribution or reproduction is permitted which does not comply with these terms.
*Correspondence: Roberta Papagni, cm9iZXJ0YXBhcGFnbmkwQGdtYWlsLmNvbQ==
†These authors have contributed equally to this work and share first authorship